- 1Julius Kühn Institute – Federal Research Centre for Cultivated Plants, Institute for Ecological Chemistry, Plant Analysis and Stored Product Protection, Berlin, Germany
- 2Institute of Pharmacy, Freie Universität Berlin, Berlin, Germany
- 3Consulting & Project Management for Medicinal and Aromatic Plants, Stahnsdorf, Germany
Based on the current trend towards broad-bandwidth LED light spectra for basil productions in multi-tiered controlled-environment horticulture, a recently developed white broad-bandwidth LED light spectrum (400-780 nm) including far-red wavelengths with elevated red and blue light fractions was employed to cultivate basil. Four Ocimum basilicum L. cultivars (cv. Anise, cv. Cinnamon, cv. Dark Opal and cv. Thai Magic) were exposed to two different rising light intensity conditions (ILow and IHigh). In dependence of the individual cultivar-specific plant height increase over time, basil cultivars were exposed to light intensities increasing from ~ 100 to ~ 200 µmol m-2 s-1 under ILow, and from 200 to 400 µmol m-2 s-1 under IHigh (due to the exponential light intensity increases with decreasing proximity to the LED light fixtures). Within the first experiment, basils’ morphological developments, biomass yields and time to marketability under both light conditions were investigated and the energy consumptions were determined to calculate the basils’ light use efficiencies. In detail, cultivar-dependent differences in plant height, leaf and branch pair developments over time are described. In comparison to the ILow light conditions, IHigh resulted in accelerated developments and greater yields of all basil cultivars and expedited their marketability by 3-5 days. However, exposure to light intensities above ~ 300 µmol m-2 s-1 induced light avoidance responses in the green-leafed basil cultivars cv. Anise, cv. Cinnamon and cv. Thai Magic. In contrast, ILow resulted in consumer-preferred visual qualities and greater biomass efficiencies of the green-leafed basil cultivars and are discussed as a result of their ability to adapt well to low light conditions. Contrarily to the green-leafed cultivars, purple-leafed cv. Dark Opal developed insufficiently under ILow, but remained light-tolerant under IHigh, which is related to its high anthocyanin contents. In a second experiment, cultivars’ volatile organic compound (VOC) contents and compositions over time were investigated. While VOC contents per gram of leaf dry matter gradually decreased in purple-leafed cv. Dark Opal between seedling stage to marketability, their contents gradually increased in the green cultivars. Regardless of the light treatment applied, cultivar-specific VOC compositions changed tremendously in a developmental stage-dependent manner.
1 Introduction
Basil (Ocimum basilicum L.) is by far one of the most popular culinary herbs worldwide and is highly appreciated for its medicinal and aromatic properties. As reviewed by Filip (2017), basil essential oils have antiviral, antioxidant, anti-inflammatory, antidiabetic and antimicrobial properties. Prominent levels of phenolic acids (Jayasinghe et al., 2003; Kwee and Niemeyer, 2011), as well as substantial amounts of anthocyanins found specifically in purple basil varieties (Flanigan and Niemeyer, 2014) further contribute to basils’ potent antioxidant capacities. Besides its health-promoting properties, basil is cherished for its distinct aroma and pleasant taste. Within the species, some of the most important aroma compounds are 1,8-cineole, linalool, ß-caryophyllene, estragole (methyl chavicol), eugenol, methyl eugenol and methyl cinnamate (Makri and Kintzios, 2008; Schulz et al., 2003). Each compound imparts a distinct aroma (Patel et al., 2021), and their varying amounts strongly influence the basils’ flavor and consumer preferences (Walters et al., 2021).
However, the progressing climatic changes and extreme weather conditions continue to result in variable field-grown basil qualities and yields (Barut et al., 2021). To still meet the incessant consumer demand of fresh basil (Market Research Report, 2022), stakeholders are increasingly considering the implementation of environmental-friendly vertical farms and plant factories including light-emitting diodes (e.g., Kozai, 2019; Benke and Tomkins, 2018) for the production of this high-value crop. By conducting a comprehensive economic evaluation, Liaros et al. (2016) showed that basil cultivations in plant factories are principally feasible. However, the fixtures’ light intensity settings were among the most influential factors impacting its profitability. Thus, the scientific community is currently in search of minimal light intensity requirements and optimal light qualities to increase the viability of LED-based indoor basil productions.
Although lighting strategies for commercial indoor plant productions are typically adjusted during different plant stages (Currey et al., 2017) and help in reducing energy costs (Lopez and Runkle, 2008), constant (spectrum-dependent) light intensities between 180 and 545 µmol m-2 s-1 are currently recommended for indoor basil cultivations (Park et al., 2016; Sipos et al., 2021). Pennisi et al. (2020), who employed five different light intensities between 100 and 300 µmol m-2 s-1 emitted by narrow blue (B) and red (R) LEDs with main peaks at 463 and 657 nm, concluded that a constant light intensity of 250 µmol m-2 s-1 optimizes basil yield and as well as water and energy use efficiencies. However, an increase in basil yield of 20 % was recently observed when shifting the narrow B wavelength from 450 to 435 nm (Rihan et al., 2020). While basils’ optimal RB ratios are still under extensive investigation (Rihan et al., 2020; Pennisi et al., 2019; Naznin et al., 2019), inclusions of other wavelengths increasingly show great potentials for improving basil yields and qualities as well. A partial replacement of B and R with green (G) light (500-600 nm) resulted in higher basil productions by inducing stem and leaf elongations (Schenkels et al., 2020). Additions of far-red (FR) light (700-750 nm) have shown to increase basils’ canopy photosynthesis (Zhen and Bugbee, 2020), and explain the increased basil yields observed by Rahman et al. (2021) in comparison to broad white (400-700 nm) and narrow RB spectra under equal light intensity conditions. Increased fresh weights, leaf areas as well as total antioxidant capacities of basil seedlings were found under any RB spectrum that included either yellow (Y, main peak at 600 nm), G (main peak at 520 nm) or FR (main peak at 735 nm) wavelengths when compared to RB alone (Carvalho et al., 2016). However, Lin et al. (2021) demonstrated enhanced photosynthetic activities and growth of green and purple basil varieties when the proportion of R was increased in an RGB spectrum, and Song et al. (2020) showed that the inclusion of B increased the accumulation and antioxidant activity of polyphenols and essential oil content in basil when compared to different combinations of B, R and W LED light. Thus, a growing body of research suggests light spectra that consist of elevated R and B LEDs, supplemented with broad W LEDs including FR wavelengths (Sipos et al., 2021).
Based on this current trend towards broad-bandwidth LED light spectra for basil productions in controlled-environment horticulture, a white broad-bandwidth LED light spectrum (400-780 nm) with elevated R and B light fractions was developed.
It could be assumed that basil plants develop more rapidly under increasing high light conditions. Reaching market maturity earlier allows for higher plant production per cultivation unit and leads to higher profitability due to higher throughput. At the same time, basil developing under rising low light conditions with the same light composition could use light more efficiently, ultimately resulting in greater profitability due to lower energy costs. Since not only morphology but also sensory quality aspects have to be taken into account for the evaluation of profitability, the contents and compositions of essential oil (influencing aroma and flavor), which change considerably over time (i.e., Lewinsohn et al., 2000), also have to be considered.
Therefore, the aim of this study was to explore the photo-morphological development as well as the content and composition of volatile organic compounds (VOS) of four basil cultivars (cv. Anise, cv. Cinnamon, cv. Dark Opal and cv. Thai Magic) over time (from seed to common marketability) under two different rising light intensity conditions (ILow and IHigh). We further aimed at investigating the basils’ biomass efficiencies under both light conditions to determine the basils’ light use efficiencies under these prototype LED light fixtures.
2 Materials and methods
2.1 Experimental design
Using a randomized block design, a three-factorial experiment (including two light intensities (ILow; IHigh), four basil cultivars (‘Anise’, ‘Cinnamon’, ‘Dark Opal’, ‘Thai Magic’) and four non-destructive weekly plant assessments (at 14, 21, 28 and 35 days after sowing (DAS)) with four spatially independent replications (= experimental blocks) per light treatment was conducted (N = 576 plant pots, n = 72 pots per experimental block with n = 18 pots per basil cultivar). To weekly collect plant material for volatile organic compound analyses, the experiment was repeated under identical conditions.
2.2 Plant material and growth conditions
Seeds of four basil cultivars, namely Ocimum basilicum cv. ‘Anise’, O. basilicum cv. ‘Cinnamon’, O. basilicum L. cv. ‘Dark Opal’ and O. basilicum L. var. thyrsiflorum cv. ‘Thai Magic’ were purchased from Rühlemanns’s Kräuter- und Duftpflanzen, Horstedt, Germany. In 32 seed boxes, ~ 150 seeds of each basil cultivar were sown on the surface of moist potting substrate (Fruhstorfer Einheitserde Typ P, Hawita, Vechta, Germany). Four seed boxes (each containing the seeds of one basil cultivar respectively) were placed in the center of each experimental block at equal PFD (photon flux density) of ~ 150 µmol m-2 s-1 and misted daily to allow for optimal light- and moisture-dependent germination. Seven days after sowing, four representative basil seedlings per cultivar were transplanted into pots (Ø = 9 cm, height 6.8 cm, volume 0.28 L) (MXC 9, Kausek, Mittenwalde, Germany). Each of the 576 pots contained a mixture of 0.28 L potting substrate (Fruhstorfer Einheitserde Typ P, Hawita, Vechta, Germany) and 560 mg of a slow-release fertilizer (15-9-11+2MgO+TE (trace elements)) (Osmocote Exact Mini 3-4M, Hermann Meyer KG, Rellingen, Germany) to ensure identical nutrient contents in all plant pots. Eight perforated propagation trays (50 x 32 x 6 cm) (CRP Import – Export GmbH, Hamburg, Germany) equipped with nine equally spaced basil pots were placed evenly under each experimental block within 1.2 m2 under the two different light intensity treatments. To ensure uniform watering, perforated propagation trays were submerged in unperforated propagation trays filled with equal amounts of water for 30 minutes daily. Due to inhomogeneous light intensity distributions underneath each experimental block (Figure 1), propagation trays were rotated and re-positioned daily to minimize positional effects. To prevent sciarid fly (Sciardae) infestations during experiments, nematodes (Steinernema feltiae) (Katz BiotechAG, Baruth, Germany) were preventively applied weekly as recommended by the supplier. At all eight experimental blocks, data loggers (EL-USB-2, Lascar, Conrad, Hirschau, Germany) continuously measured climatic conditions including temperature and relative humidity. With average temperatures (°C ± standard deviation (SD)) of 21.6 ± 2.8 and 21.2 ± 2.6 (with a measuring accuracy of 1°C) and average humidities (%rh ± SD) of 68.9 ± 13.4 and 70.8 ± 13.02 (with a measuring accuracy of 2.25%) under both light treatments, climatic conditions did not differ between light treatments.
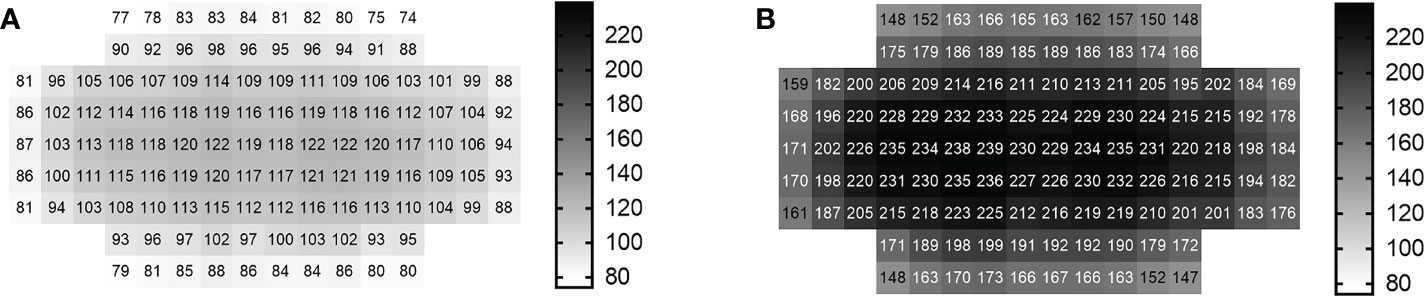
Figure 1 Light intensity distribution underneath each LED light treatment1. (A) Low light intensity treatment (ILow), (B) High light intensity treatment (IHigh) 1Each heat map depicts the photon flux densities (PFDs) between 400 and 780 nm [µmol m-2 s-1] measured every 100 cm2 within one experimental block (1.2 m2) underneath the light treatments at cultivation table level (n = 120 measurements). Gray scales to the right of each light distribution pattern are scaled from minimum to maximum PFDs (74-239 µmol m-2 s-1) measured across both light treatments.
2.3 Lighting systems and illumination conditions
Light experiments were conducted in climate-controlled cultivation rooms. Each of the eight experimental blocks per experiment consisted of two prototype LED lamps (Apollo R1, FUTURELED®, Berlin, Germany) which were mounted 0.67 m apart from another and mounted onto given steel frames resulting in 1.00 m between the cultivation table and the bottom of the LED lights. The illuminated area of 1.2 m2 underneath these two multispectral LED lamps represented one of eight experimental blocks per experiment. Plastic sheeting extending from above the light fixtures to below the cultivation tables eliminated neighboring light pollution from other experimental blocks. Light intensity distributions, broad LED light spectra and spectral compositions during the experiments are depicted in Figures 1, 2 and Table 1, and were measured with an Ocean FX-UV-VIS spectrometer (Ocean Insight, Ostfildern, Germany). All basil seeds and seedlings were subject to a broad LED light spectrum (400-780 nm) with elevated (R) and blue (B) wavelength proportions under a PFD of ~ 150 µmol m-2 s-1 during germination. Starting seven days after sowing, basil plants were then subject to either a PFD of ~ 102 µmol-2 s-1 (= ILow) or a PFD of ~ 200 µmol m-2 s-1 (= IHigh) at cultivation table level for the remainder of the light experiments. Over time, PFDs increased with increasing height of basil cultivars (as the distance of the canopy to the LED light sources decreased) (Figure 3). Plants were subject to artificial lighting from 6.00 am to 10.00 pm for a photoperiod of 16 hours per day during the experiments (duration 35 days).
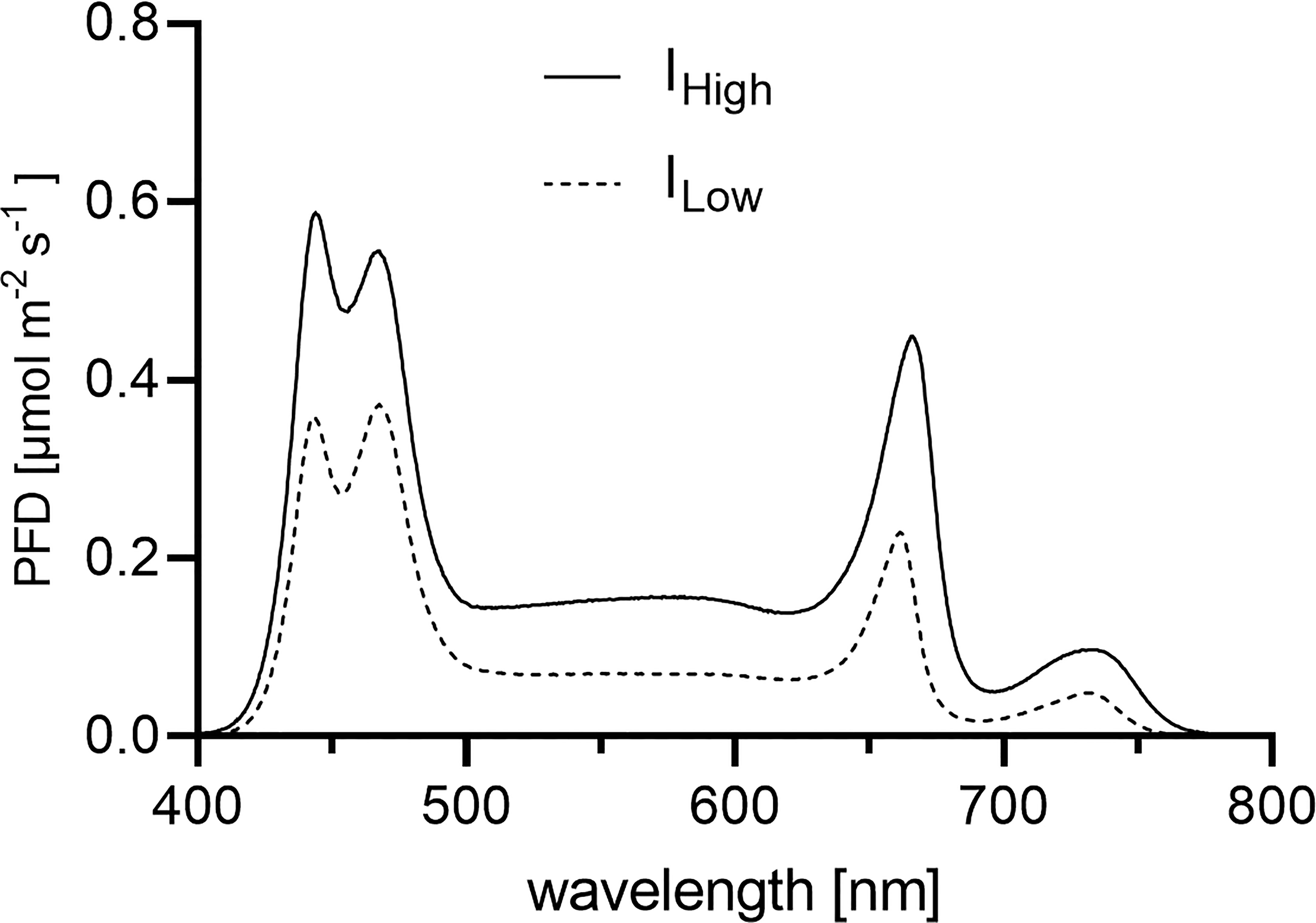
Figure 2 White broad-band LED light spectra applied during the experiments. Depicted are the LED light spectra as PFDs (photon flux densities) [µmol m-2 s-1] per wavelength [nm] between 400 and 780 nm. Each LED light spectrum (ILow light treatment = dashed line; IHigh light treatment = solid line) represents the average PFDs per wavelength obtained from 120 measurements across the entire experimental block (1.2 m2).
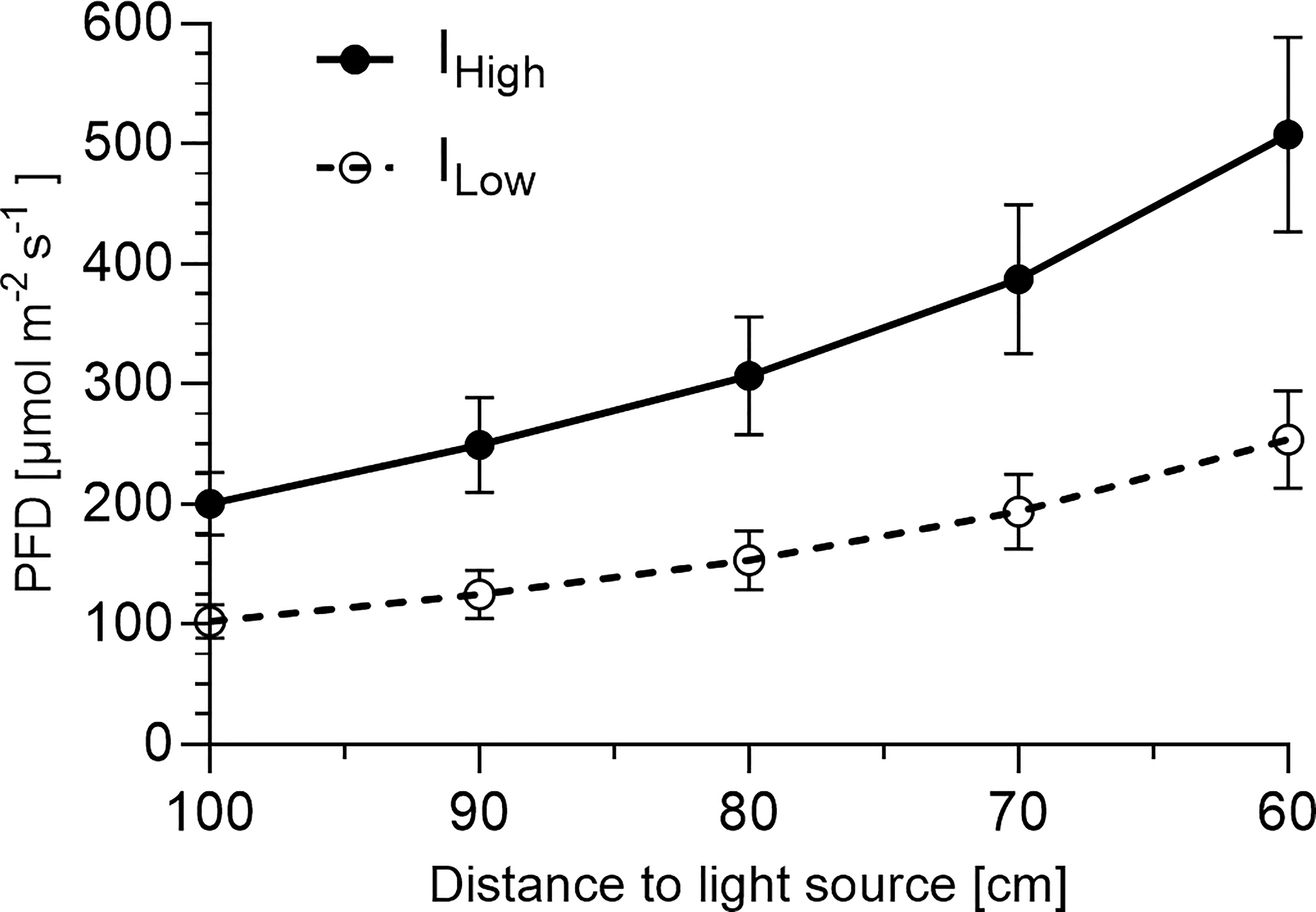
Figure 3 Photon flux density depending on distance to light source1. 1Presented are average photon flux densities (PFD) [µmol m-2 s-1] ± SD between 400 and 780 nm recorded in 10 cm intervals (starting at cultivation table level 100 cm from the light source) from nine representative positions across the cultivation area (1.2 m2) at each distance.
2.4 Energy measurements
The power draw of current (A) and voltage (V) from representative lamps of each light treatment were measured using a power meter (ENERGY MONITOR 3000, Voltcraft ®, Wernberg-Köblitz, Germany) to estimate the yield efficacy of the LED light treatments for each basil cultivar. Electrical consumptions were calculated by multiplying the power consumptions with the running hours of the LED light fixtures (16 hours per day, 35 days in total). Biomass efficacy (total fresh yields per kWh and cultivation area (1.2 m2)) as well as VOC efficacy (volatile organic compound yields per kWh and cultivation area) were calculated by multiplying the cultivars’ average yields per experimental area with 72 (= maximum number of pots per experimental block) and dividing these yields by the total kilowatt hours used per experimental block.
2.5 Extraction of volatiles
After samples were gently air-dried in a drying oven at 30 °C for ≤ 7 days until stable mass was attained and shortly stored under cool, dry and dark conditions, volatile compounds of basil leaves were extracted according to the following procedure: 100 mg (± 2 %) of dried and powdered (3 intervals of 10 seconds at 15,000 rpm via Tube Mill control, IKA®, Staufen, Germany) basil leaves from two basil pots (of the same cultivar, light treatment, harvest date and experimental block) were transferred into 2 mL screw cap micro tubes (Sarstedt AG & Co. KG, Nümbrecht, Germany) including two steal grinding balls (Ø 2 mm) and homogenized in 1.0 mL of high-performance liquid chromatography grade isooctane (Th. Geyer, Renningen, Germany) [containing 1:2000 (v/v) carvacrol as internal standard] for 10 minutes at 30 rps with a ball mill (MM400, Retsch®, Haan, Germany). After 10 minutes of ultra-sonification (Sonorex RK 106, Bandelin electronic GmbH & Co. KG, Berlin, Germany) and 10 minutes of centrifugation at 13,000 rpm (Heraeus™ Labofuge™ 400 R, Thermo Scientific™, Osterode, Germany) at 22 °C respectively, the supernatants were transferred into GC-vials and stored at -70 °C until analysis (N = 256 samples).
2.6 GC-FID and GC-MS analyses
1 µL of the obtained extracts of volatiles were analyzed by GC–FID using an Agilent gas chromatograph 6890N fitted with a HP-5MS column (30 m × 250 µm x 0.5 μm) in split mode (1:20). Detector and injector temperatures were set to 250°C. The following oven temperature program was used: 50°C for 2 min, heating from 50 to 320°C at a rate of 5°C min–1. The final temperature was held for 6 min. Hydrogen was used as carrier gas with a constant flow rate of 1.2 mL min-1. GC-MS was performed using an Agilent 5975 Network mass spectrometer, on a HP-5MS column (see GC), operating at 70 eV ionization energy, using the same temperature program as above. Helium was used as carrier gas with a constant flow rate of 1.2 mL min-1. Retention indices were calculated by using retention times of C6-C24-alkanes (Merck KGaA, Darmstadt, Germany) that were injected under the same chromatographic conditions.
2.7 Identification and quantification of volatile compounds
All main compounds of the volatile extracts were identified by comparing their mass spectra with those of internal reference libraries (Adams, NIST). Additionally, identification of 1,8-cineole, α-pinene, α-terpineol, β-elemene, β-myrcene, β-pinene, borneol, camphor, carvacrol, eugenol, β-farnesene, limonene, linalool, methyl chavicol, methyl cinnamate, methyl eugenol, ocimene and sabinene was affirmed by pure standard substances (purchased as analytical standards with a purity ≥ 95 % for GC reference analysis from Abcam (Cambridge, United Kingdom), Alfa Aesar (Kandel, Germany), Carl Roth (Karlsruhe, Germany), Fluka (Seelze, Germany), Merck KGaA (Darmstadt, Germany) and TCI GmbH (Eschborn, Germany)) and confirmed by comparing their retention indices. Volatile compounds were quantified based on the known concentration of the internal standard carvacrol.
2.8 Statistics and calculations
GraphPad Prism (version 9.3.1.471, San Diego, USA) was used for statistical analyses and graphical representations of all data sets. Plant heights, fresh/dry weights, VOC contents and compositions: Normality of data sets were tested via Shapiro-Wilk or D’Agostino & Pearson tests within and across spatial replications at α < 0.05. When distributions deviated from normality, outliers were identified via ROUT method at Q = 10 % and removed to obtain normality. When standard deviations (SDs) of data sets passed Brown-Forsythe and Bartlett’s tests at α < 0.05, ordinary one-way ANOVAs followed by Tukey’s multiple comparisons test at 95% confidence level (α < 0.05) were applied; when SDs were not equal between light treatments and cultivars, Brown-Forsythe and Welch ANOVAs followed by Dunnett’s T3 multiple comparisons test at 95 % confidence level (α < 0.05) were applied to analyze differences between light treatments and cultivars. (Data set of plant height: N = 566-575, n = 14-18 average plant heights per basil cultivar, light treatment and spatial replication: each n used for statistical analysis represents the average plant height of all four measured basil plants per pot; data sets of fresh/dry weights: N = 570/567, n = 15-18 values per cultivar, light treatment and spatial replication; data sets of volatile organic compound (VOC) content and composition: N = 32-64, n = 4-8 values per cultivar and light treatment). Number of leaf and branch pairs: As no differences within light treatments across spatial replications were observed after applying a Kruskal-Wallis test followed by Dunn’s multiple comparisons test at confidence level 95% (P < 0.05) for basil plants at each time point (p > 0.99), data sets of all four spatial replications per light treatment and cultivar were combined for further statistical analysis. To analyze differences between light treatments and cultivars at each time point, Kruskal-Wallis tests followed by Dunn’s multiple comparisons test at 95 % confidence level (P < 0.05) were conducted, respectively (Data set: N = 576, n = 72 assessed pots per light treatment, cultivar and time point; each n used for statistical analysis represents the average number of leaf pairs/side branches from all four assessed basil plants per pot). Principal component regressions (PCRs) were performed to determine the predictive power of morphological parameters (plant height, number of leaf and branch pairs) on the basils’ VOC compositions by including the average values of all morphological observations (dependent variables) and of all detected VOCs (independent variables) per light treatment and DAS. Thus, data sets of each cultivar consisted of eight average values per each of the three dependent variables (as each value represents the average morphological observation per time (14, 21, 28, 35 DAS) and light treatment (IHigh, ILow) and eight values per each of the 21-25 independent variables (as we detected 21, 22, 23 and 25 VOCs in cv. Anise, Dark Opal, Cinnamon and Thai Magic, respectively). Yield efficacy: The average fresh weights and leaf dry weights from 15-18 harvested basil pots per cultivar and spatial replication were multiplied by factor 72 to approximate the basil cultivars’ fresh weight and VOC productions for the entire illuminated experimental area. Approximated production yields were then divided by the calculated kilowatt hours consumed under the experimental area of 1.2 m2 by the end of the trial period to determine biomass and VOC efficacies. When data sets passed normality via Shapiro-Wilk test at α < 0.05, data sets were analyzed via unpaired t test, otherwise via non-parametric Kolmogorov-Smirnov test at 95 % confidence level (P < 0.05), respectively.
3 Results and discussion
3.1 Cultivar-dependent morphological differences
The species Ocimum basilicum L. exhibits an immense variety of different cultivars and is characterized by a high intra-specific diversity in morphological traits, and the specific appearances of each cultivar observed in our study coincide with their typical phenotypes described by Carović-Stanko et al. (2011) and Lin et al. (2021). For example, basil cultivars cv. Anise, cv. Cinnamon and cv. Thai Magic were characterized by green leaves with purple stems and flowers, while basil cv. Dark Opal was characterized by the purple color of all its aerial parts and its compact growth (Figure 4).
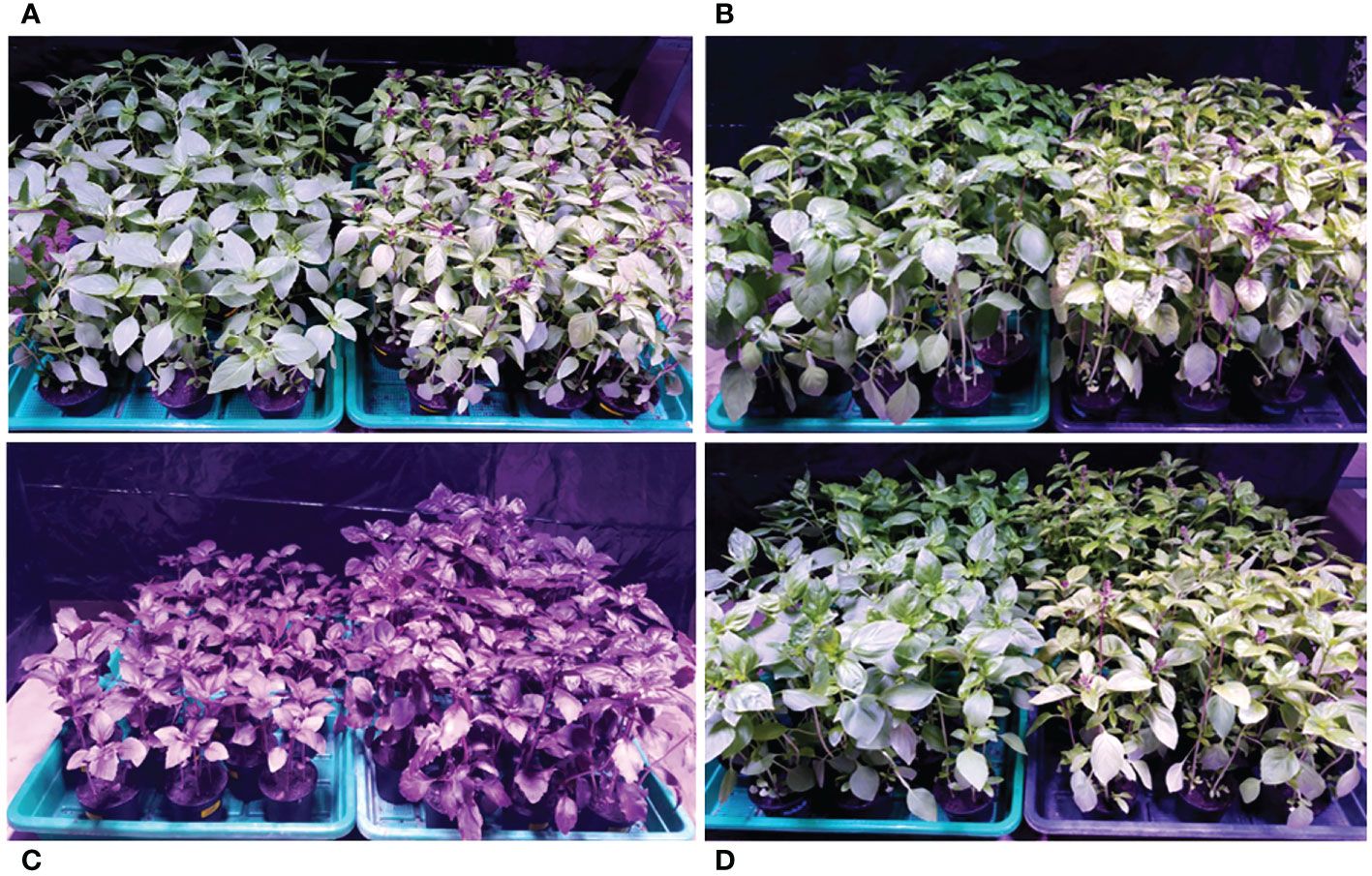
Figure 4 Visual appearance of four Ocimum basilicum L. cultivars at harvest. (A) O. basilicum L. cv. ‘Anise’, (B) O. basilicum L. cv. ‘Cinnamon’, (C) O. basilicum L. cv. ‘Dark Opal’, (D) O. basilicum L. cv. ‘Thai Magic’ under the low (left) and high (right) light intensity, respectively, 35 days after sowing.
With an average plant height of ~ 25 and ~ 27 cm and an average of four and five leaf pairs as well as branch pairs by the end of the trial period (35 days after sowing (DAS)) under ILow and IHigh light conditions, respectively, basil cv. Cinnamon grew the tallest and developed the most leaf and branch pairs from all investigated cultivars (Table 2; Figure 5). In addition, while ~ 43 % of all investigated cv. Cinnamon basil plants had developed flower buds by the end of the experiment under IHigh, no flower buds had formed under ILow (Table 2).
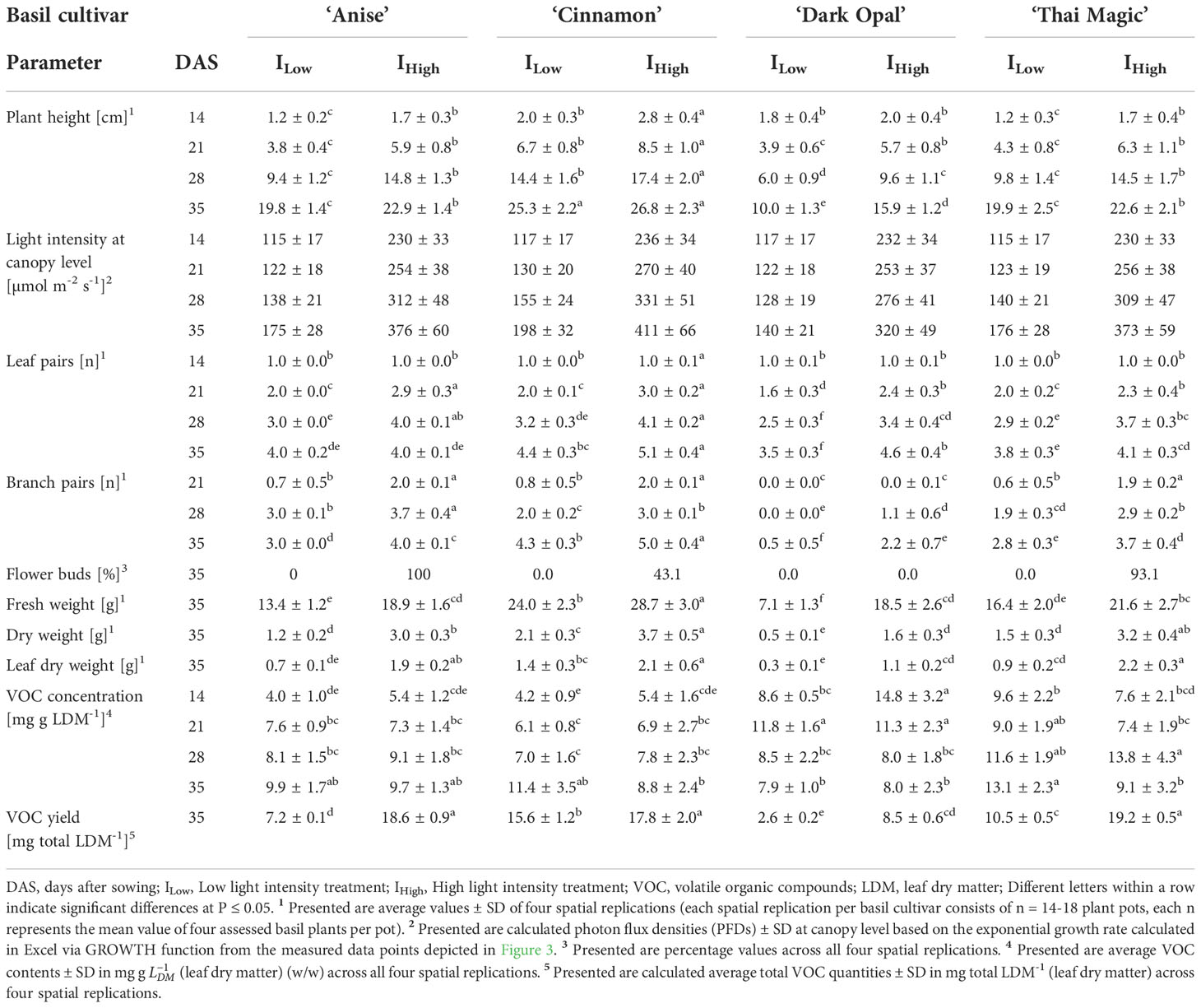
Table 2 Summarized plant characteristics of four basil cultivars grown under two LED light intensities over time (including light intensities at plant canopy levels).
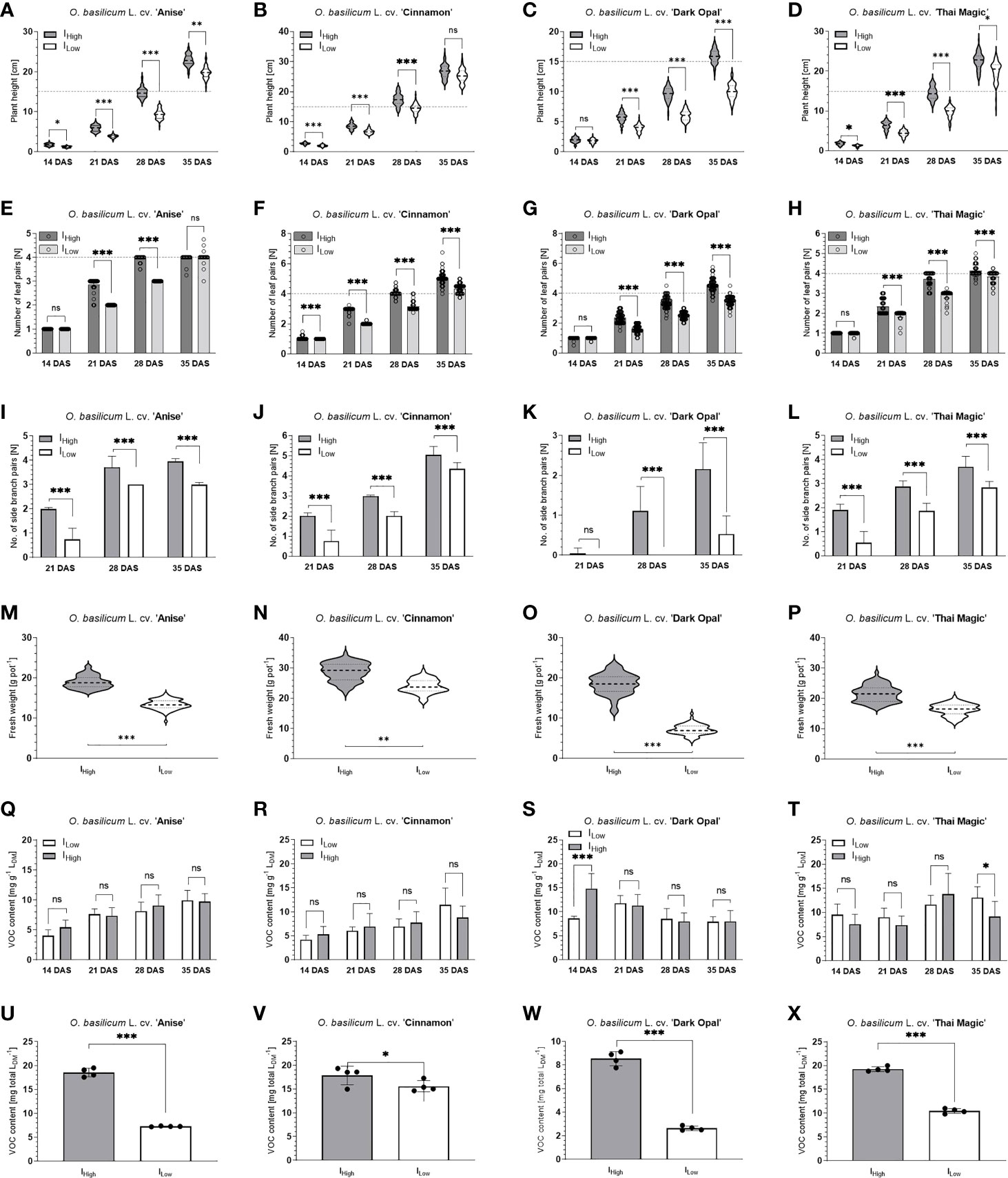
Figure 5 Characteristics of four Ocimum basilicum L. cultivars under two different broad-bandwidth LED light intensities over time. A-D: Plant height development of basil cultivar ‘Anise’ (A), ‘Cinnamon’ (B), ‘ Dark Opal’ (C) and ‘Thai Magic’ (D) over time (dashed and dotted lines within violin plots represent medians and quartiles (25th and 75th percentile) of the data sets, respectively; dashed lines horizontally across the graph represent marketability at height ≥ 15cm); E-H: Leaf pair development of basil cultivar ‘Anise’ (E), ‘Cinnamon’ (F), ‘ Dark Opal’ (G) and ‘Thai Magic’ (H) over time (dashed lines represent marketability at a number of leaf pairs ≥ 4); I-L: Branch pair development of basil cultivar ‘Anise’ (I), ‘Cinnamon’ (J), ‘ Dark Opal’ (K) and ‘Thai Magic’ (L) over time; M-P: Fresh weight per pot of basil cultivar ‘Anise’ (M), ‘Cinnamon’ (N), ‘ Dark Opal’ (O) and ‘Thai Magic’ (P) 35 days after sowing (at harvest; dashed and dotted lines within violin plots represent medians and quartiles (25th and 75th percentile) of the data sets, respectively); Q-T: Volatile organic compound content per gram of leaf dry matter of basil cultivar ‘Anise’ (Q), ‘Cinnamon’ (R), ‘Dark Opal’ (S) and ‘Thai Magic’ (T) over time; U-X: Total volatile organic compound content per total leaf dry matter of basil cultivar ‘Anise’ (U), ‘Cinnamon’ (V), ‘ Dark Opal’ (W) and ‘Thai Magic’ (X) 35 days after sowing (at harvest). IHigh, High light intensity treatment; ILow, Low light intensity treatment; DAS, days after sowing; dashed horizontal lines represent marketability (defined as /plant height ≥ 15cm and/or number of leaf pairs ≥ 4); LDM, leaf dry matter; VOC, volatile organic compound; significance levels: ns = not significant, *≤ 0.05, **≤ 0.02, ***≤ 0.01; please note the differently scaled Y-axes between cultivars.
Though basil cv. Anise showed the most homogeneous development (lowest variance) of all investigated basil cultivars, cv. Anise and cv. Thai Magic developed very similarly under both LED light conditions (Table 2; Figure 5). While both cultivars reached a plant height of ~ 20 cm under ILow, the cultivars grew significantly taller under IHigh and reached heights of ~ 23 cm by the end of the trial period. In addition, both cultivars developed the same number of leaf pairs along the main stem during the experiment and had developed an average of four leaf pairs along the main stem under both light conditions by the end of the experiment. Further, all cv. Anise basil plants and ~ 93 % of the cv. Thai Magic basil plants had formed flower buds under IHigh, whereas no flower bud formation was detected under ILow. Though branching of cv. Anise progressed faster, both cultivars averaged three and four branch pairs at the end of the trial period under ILow and IHigh, respectively.
With heights of ~ 10 and ~ 16 cm, and an average of one and two branch pairs per basil plant under ILow and IHigh at harvest, respectively, the purple-leafed cv. Dark Opal remained the shortest and least branched of the four investigated cultivars (Table 2; Figure 5). Though cv. Dark Opal developed the fewest leaf pairs under the ILow light conditions, its leaf pairs developed rapidly under IHigh by growing an average of ~ 5 leaf pairs per basil plant.
3.2 Enhanced basil development under IHigh results in earlier marketability than under ILow
All four investigated Ocimum basilicum L. cultivars developed more rapidly under IHigh than under ILow light conditions. During all weekly assessments, basil heights as well as the number of leaf and branch pairs were mostly greater under IHigh when compared to the developmental stages reached under ILow during the same assessment week. Further, while basil flower buds developed under IHigh in all three green-leafed basil cultivars during the last week of the trial period, no flower bud formations were observed under ILow. Accordingly, all cultivars had accumulated greater total fresh and dry weights as well as leaf dry weights per basil pot under IHigh at the end of the experiment than under ILow (Table 2; Figures 5A–P).
Thus, marketability based on morphological criteria (defined as basil height ≥ 15 cm and/or number of leaf pairs along the main stem ≥ 4) of cv. Cinnamon, cv. Anise, cv. Thai Magic and cv. Dark Opal was reached 27, 29, 30 and 32 DAS under the IHigh light treatment, respectively (Figures 5A–H). Though the light intensity of ILow represented ~ 50 % of IHigh (Table 1), green-leafed cultivars were marketable only 3-4 days later than under IHigh conditions: While marketability of the cultivars cv. Cinnamon and cv. Thai Magic was reached three days later (30 and 33 DAS, respectively), cv. Anise reached marketability under ILow four days later than under IHigh (33 DAS). Cultivar Dark Opal did not reach the defined marketable stage under ILow during the 35 days of cultivation. However, based on cv. Dark Opals’ growth function, its marketability under ILow can be expected 37 DAS (5 days later than under IHigh) as the leaf pair criterium (≥ 4 leaf pairs along the main stem) should be reached.
3.3 IHigh induced light avoidance responses in green basil cultivars while purple basil remained ‘light-tolerant’
As evident in Figure 4, undesirable morphological and anatomical leaf adaptions to IHigh became clearly visible at the top canopy of the green-leafed cultivars cv. Anise, cv. Cinnamon and cv. Thai Magic during the last week of the trial period when PFDs exceeded an average of 300 µmol m-2 s-1 at canopy level (Table 2). In addition to purple pigment productions observed in some leaves of cv. Anise and cv. Cinnamon, all three green-leafed cultivars displayed leaf curling, reduced and dehydrated leaf blades and a pale green leaf color under the IHigh light treatment, which are associated with reduced consumer preferences (Walters et al., 2021).
These leaf alterations exemplify typical light avoidance responses (Logan et al., 2015). To protect themselves from irreversible damages caused by excessive light (such as loss of oxygen evolution and loss of electron transfer activity of photosystem II (Tyystjärvi, 2013), sessile plants have evolved sophisticated signaling and protective mechanisms such as anthocyanin biosynthesis (Ma et al., 2021), chloroplast avoidance response (Wada, 2013) and thermal energy dissipation (Garcia-Plazaola et al., 2012).
The visible purple pigmentations in cv. Anise and cv. Cinnamon at the end of the trial period result from the biosynthesis of non-photosynthetic anthocyanins. Anthocyanin accumulations were proven to be stimulated by high light intensities to shield photosynthetic apparatuses from exceeding light conditions (Gould et al., 2018; Stetsenko et al., 2020; Ma et al., 2021). In example, anthocyanin contents increased by more than 20 % after LED light exposure to ≥ 300 µmol m-2 s-1 in a green-leafed lemon basil (O. basilicum x O. americanum) (Stetsenko et al., 2020), and their presence has been shown previously for both cultivars (Kwee and Niemeyer, 2011; Carović-Stanko et al., 2011). Thus, the anthocyanin formations observed in some leaves of cv. Anise and cv. Cinnamon under IHigh (but not under ILow) are obviously a light-induced photoprotective mechanism.
In addition, comparative studies investigating high light responses in acyanic (green-leafed) and cyanic (purple-leafed) basil varieties (Landi et al., 2014; Tattini et al., 2014; Logan et al., 2015; Stetsenko et al., 2020) prove and support that the constitutively high anthocyanin concentrations in purple basils are responsible for mitigating light stress responses (while their green basil counterparts start to downregulate their photochemical efficiency under equal light stress conditions). Their findings explain the absence of visible photomorphogenic responses and thus, the ‘light-tolerance’ of purple-leafed ‘Dark Opal’ under IHigh in our study, which represents a cultivar with very high anthocyanin contents (Lin et al., 2021).
The pale green color of leaves observed in all green cultivars under IHigh is a typical sign of a chloroplast avoidance response: While the green color-giving chloroplasts distribute across the upper and lower sides of each palisade cell to achieve maximal light absorption under low to optimal light conditions (explaining the characteristic green leaf colors present under ILow (Figure 4), chloroplasts gather at the side walls of each palisade cell to minimize the damages associated with the absorption of excessive light energy by chlorophyll pigments (Kagawa et al., 2001; Wada, 2013).
Additionally, comparable to our observations, leaves of green basil cultivars tend to reduce in size when exposed to a constant PPFD of 300 µmol m-2 s-1, which was associated with decreased chlorophyll contents, stomatal conductance, stomatal size and density in the green basil cultivar ‘Genovese’ (Pennisi et al., 2020). Reduced leaf sizes were also observed in the green cultivar ‘Tigullio’ under full sunlight when compared to leaves grown under 30 % sunlight (Tattini et al., 2014).
All described light avoidance responses of the green basil cultivars under IHigh during the last week of the trial period are unmistakable signs of non-photochemical quenching (NPQ), a fundamental physiological photoprotective mechanism which involves the conversion and dissipation of excess excitation energy into heat. As NPQ represents a plants’ shift from a photosynthetically efficient state to a state in which a fraction of photosynthetic reaction centers is non-functional, NPQ always occurs at the expense of photosynthetic efficiency and results in limited growth and reduced crop yields (García-Plazaola et al., 2012; Logan et al., 2015).
In addition, IHigh conditions accelerated flower initiation in green basil cultivars. Based on the seed supplier, cv. Anise, cv. Cinnamon and cv. Thai Magic are endogenously programmed to initiate flowering after eight, seven and six nodes have been developed, respectively. However, under IHigh these cultivars initiated flowering after developing only five, six and five nodes (including cotyledons), respectively (Table 2; Figure 5), which is a phenomenon that has recently been categorized as stress-induced flowering in angiosperms (Takeno, 2016). Although stress-induced flowering has received little attention so far, previous studies of Salvia, Calendula, and Pharbitis have shown that intense light stress triggers an earlier flowering pulse (Moccaldi and Runkle, 2007; Hirai et al., 1994).
3.4 ILow resulted in beneficial acclimation responses in green basil cultivars while purple basil performed insufficiently
In contrast to the undesirable light-avoiding leaf adaptations and flower initiations detected under IHigh (section 3.3), the light-orientated leaves of the green cultivars cv. Anise, cv. Cinnamon and cv. Thai Magic were fully expanded, thin and displayed typical green leaf colors under ILow (Figure 4), which represent decisive external quality attributes for consumers (Rouphael et al., 2012). As investigated by Walters et al. (2021), consumers prefer big, fresh basil leaves without detectable discolorations and damages as well as ‘soft’ leaf textures. Consequently, all visual traits detected under ILow supersede the unsatisfactory visual attributes identified under IHigh and translate into highly desirable basil products by meeting the consumers’ basil preferences.
These favored basil leaf qualities under ILow represent acclimation responses often found in green leaves exposed to low light levels to increase light capture and photosynthetic efficiency (Nemali and van Iersel, 2004; Wada, 2013; Stagnari et al., 2018), and are to be ascribed to the great plasticity in morpho-anatomical, physiological and biochemical traits of green basils: As shown by Tattini et al. (2014), the capability of green basil cultivar ‘Tigullio’ to increase its leaf area, photosynthetic pigment concentrations and CO2 assimilation rates under 30% sunlight resulted in greater net photosynthesis and net daily carbon gains than detected in cv. Tigullio under full sunlight. The greater leaf sizes, the evenly distributed chloroplasts across the leaves of cv. Anise, cv. Cinnamon and cv. Thai Magic (Figure 4) as well as the fact that the marketability criteria were reached just 3-4 days later under ILow (as described in section 3.2) are robust signs that very similar (beneficial) low light acclimation responses took place in the green cultivars investigated in our study.
To the contrary, the comparatively small leaf sizes (as evident in Figure 4C) and the slow development of cv. Dark Opal under ILow (as evident by the great differences in plant height, number of developed leaf and branch pairs and thus biomass yields (Table 2, Figures 4C, G, K, O) in comparison to IHigh show clearly, that cv. Dark Opal was not able to adjust as well as its green basil counterparts to the given low light conditions. The reason for cv. Dark Opals poor performance under ILow can be ascribed to its high content of leaf anthocyanins (Lin et al., 2021), which proved to translate into an intrinsically low ability to maximize light interception and transmission in the similar anthocyanin-rich purple leaves of basil cultivar ‘Red Rubin’ under low light conditions (Tattini et al., 2014). The anthocyanins’ role in the observed insufficient ILow performance is further strengthened by Misyura et al. (2013) who showed that anthocyanin-rich Arabidopsis thaliana mutants grew worse compared to the wild type under equal light conditions.
3.5 Cultivar-dependent light intensity requirements under increasing light intensity conditions
Providing photon fluxes that surpass the plants’ ability to absorb the supplied energy decrease photosynthetic rates and consequently result in decreasing photosynthate accumulations and biomass productions (Tyystjärvi, 2013). Hence, plants’ light saturation points (the intensity at which additional increases in light do not increase photosynthesis) should not be exceeded for optimal (and cost-effective) plant development.
The light saturation point of Ocimum basilicum L. was recently determined [under an RB spectrum with narrow peaks at 660 and 445 nm, ratio 7:3, utilized cultivar not provided] to be between 420 and 545 µmol m-2 s-1 (Park et al., 2016). Also Beaman et al. (2009) determined [under cool white fluorescent and incandescent lamps] high light intensity requirements of 500 µmol m-2 s-1 for optimal cultivation of green basil cultivars ‘Italian Large Leaf’, ‘Genovese’ and ‘Nufar’. In contrast, a current review by Sipos et al. (2021) concluded constant spectrum-dependent PPFDs of only 180 to 300 µmol m-2 s-1 to be adequate for basil cultivation. However, the review does not take cultivar-dependent differences into account, even though optimal light intensities are known to vary among cultivars. (Lee et al., 2019; Virŝilė et al., 2019). In our investigation, basil plants developed under rising (not constant) photon fluxes as the basils grew towards the LED light sources in proximity (Figure 3).
If not constantly adjusted manually, increasing light intensity gradients are common under space-limited growing conditions e.g., in vertical farms and plant factories (Tabbert et al., 2022), and could be used strategically since light requirements and the ability to absorb light energy generally increases during plant development until saturation is reached (Currey et al., 2017). For example, providing increasing light intensities resulted in shoot dry weights and leaf numbers alike those generated under constantly high light intensities (Lopez and Runkle, 2008; Oh et al., 2010) but under reduced energy costs (Poulet et al., 2014).
In general, all four of our investigated basil cultivars developed well under rising light intensity conditions which is in accordance with Solis-Toapanta and Gómez (2019), who evaluated growths and photosynthetic capacities of green basil cultivar ‘Genovese Compact’ and purple cultivar ‘Red Rubin’ under increasing and constant light intensities.
However, (as described in section 3.3) the light intensities reached under IHigh during the last week of our experiment (28-35 DAS) led to adverse, quality-reducing effects in the investigated green basil cultivars. Average PFDs above 312, 331 and 309 µmol m-2 s-1 under IHigh became detrimental and should therefore not be exceeded under the applied spectral composition (and growing conditions). In contrast, cv. Anise, cv. Cinnamon and cv. Thai Magic indicated no unfavorable signs under average PFDs of 175, 198 and 176 µmol m-2 s-1 under ILow by the end of the experiment (Table 2). Hence, even under the increasing light intensity conditions applied in our study, the recommended upper limit of 300 µmol m-2 s-1 for commercial basil production under artificial lighting by Sipos et al. (2021) holds true for the investigated cultivars ‘Anise’, ‘Cinnamon’ and ‘Thai Magic’. In contrast, the light-tolerant purple basil cultivar ‘Dark Opal’ could be grown under artificial PFDs greater than 320 µmol m-2 s-1 as no obvious negative effects were detected at this light intensity during harvest, and could generate higher yields than measured in our study.
However, with the high-quality market-ready basils within 30-37 DAS (Figures 5A–H) detected under ILow under increasing light intensities (between ~ 100 and 200 µmol m-2 s-1 (Table 2)) mostly below the lowest recommended constant light intensity of 180 µmol m-2 s-1 as recently reviewed by Sipos et al. (2021), the increasing light conditions applied in this study show that even with lower than previously assumed light requirements, high-quality basil productions under articificial light conditions are possible.
3.6 Cultivar-specific VOC concentrations change over time
The gas chromatographic analyses of basil leaf extracts resulted in the identification of 37 volatile organic compounds (VOCs), representing more than 96 % of all detected compounds. All investigated cultivars showed compositional similarities as many VOC compounds (e.g., 1,8-cineole, ß-elemene and methyl eugenol) were found in all four basil cultivars. Nevertheless, cultivars cv. Anise and cv. Thai Magic were characterized by high concentrations of methyl chavicol (Tables 3, 4), whereas linalool, methyl chavicol, trans-methyl cinnamate and methyl eugenol were the major compounds of cultivar ‘Cinnamon’ (Table 5). Leaf extracts of the cultivar ‘Dark Opal’ were characterized by high concentrations of methyl eugenol, as well as 1,8-cineole, linalool and eugenol (Table 6). Thus, each cultivars’ VOC compositions as affected by the artificial light conditions applied in this study are in good agreement with published VOC compositions identified under greenhouse and field conditions (Elansary and Mahmoud, 2014; Wesolowska and Jadczak, 2016; Maggio et al., 2016; Varga et al., 2017; Dehsheikh et al., 2020).
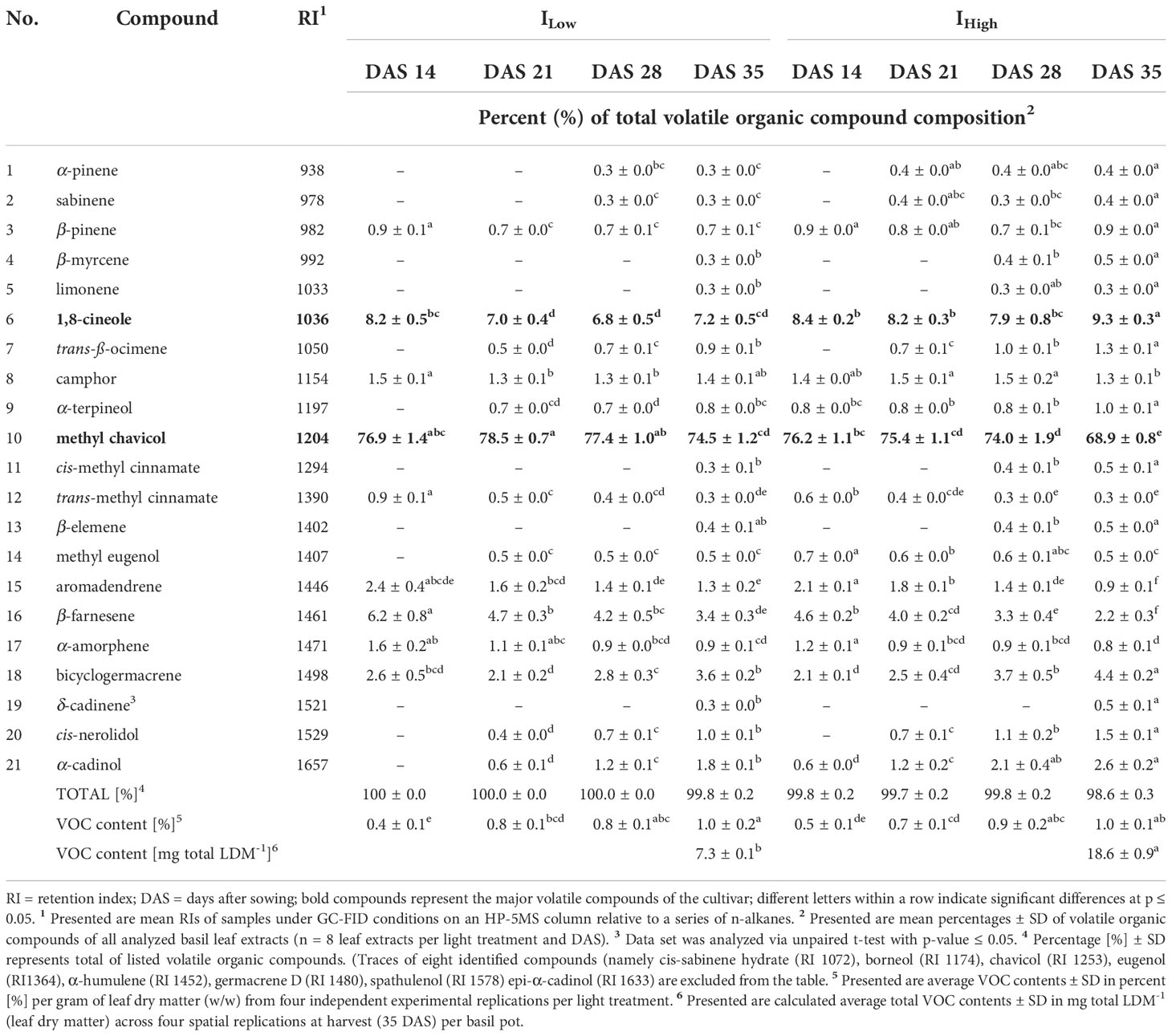
Table 3 Relative abundance of major volatile organic compounds identified in the leaves of O. basilicum L. cv. ‘Anise’ as affected by LED light intensity treatments over time.
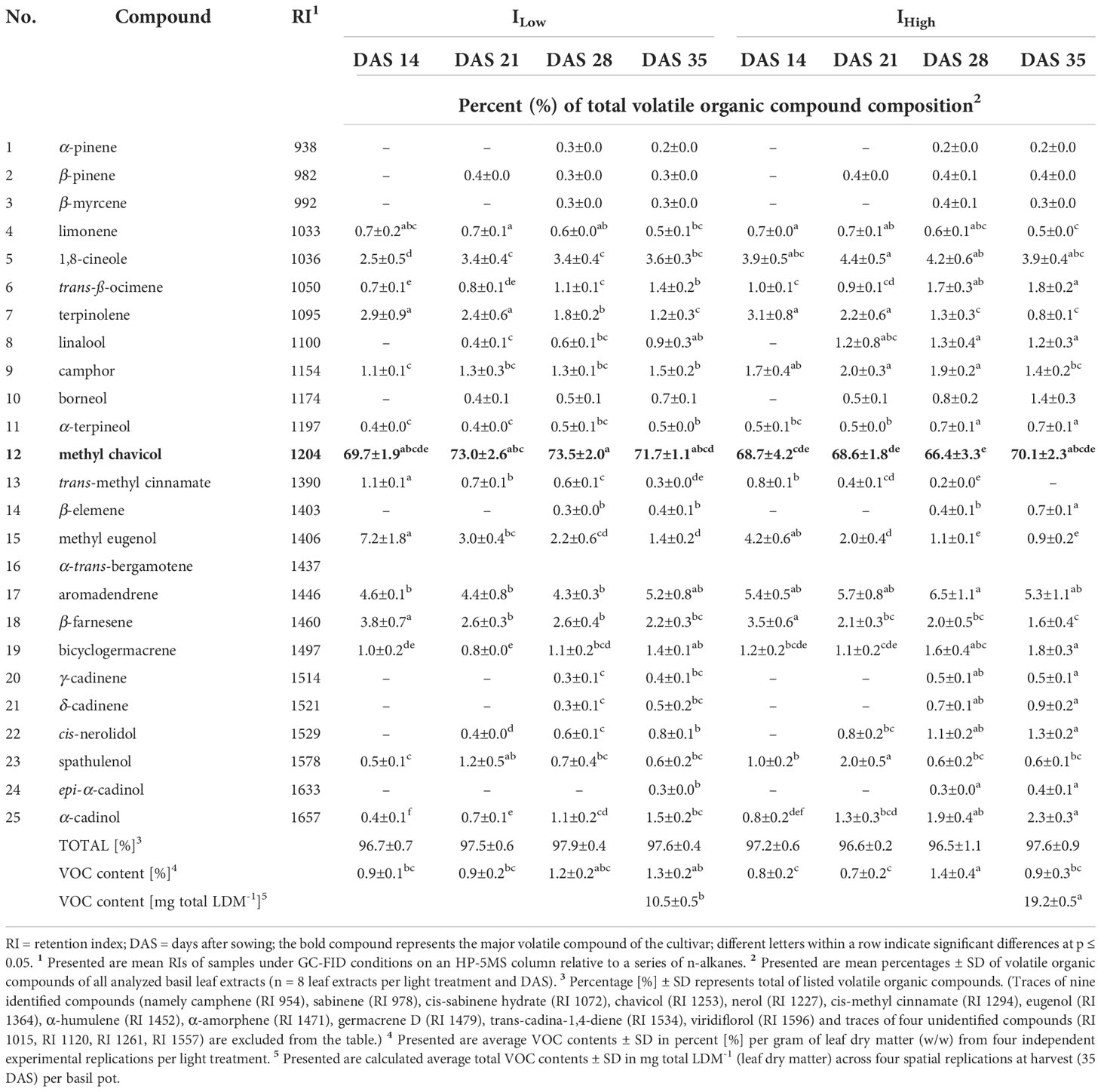
Table 4 Relative abundance of major volatile organic compounds identified in the leaves of O. basilicum L. cv. ‘Thai Magic’ as affected by LED light intensity treatments over time.
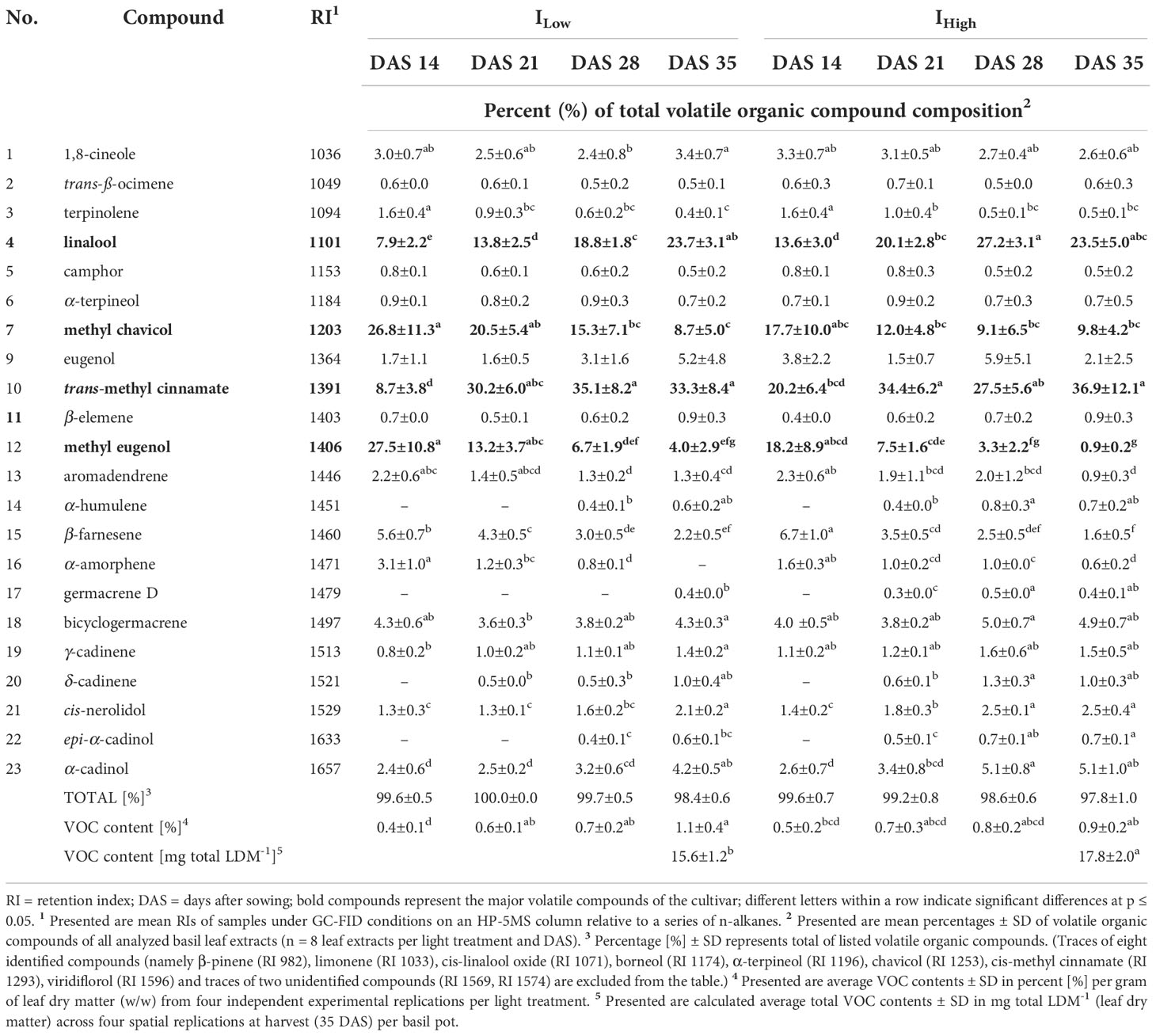
Table 5 Relative abundance of major volatile organic compounds identified in the leaves of O. basilicum L. cv. ‘Cinnamon’ as affected by LED light intensity treatments over time.
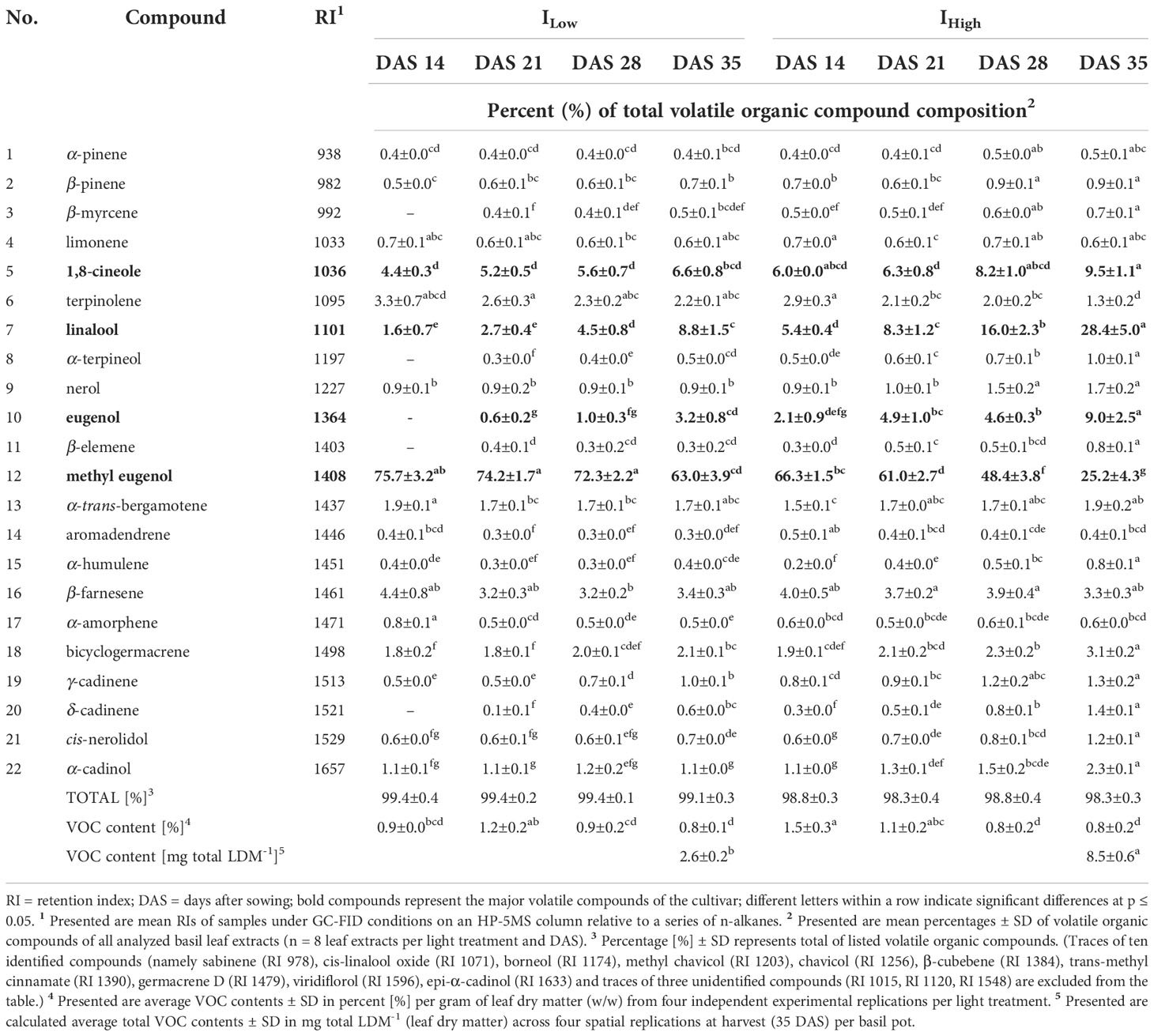
Table 6 Relative abundance of major volatile organic compounds identified in the leaves of O. basilicum L. cv. ‘Dark Opal’ as affected by LED light intensity treatments over time.
The complexity of VOC profiles increased with the basils’ progressing development. In example, while leaf extracts of 14-day-old cv. Anise seedlings were characterized by nine and twelve major volatiles under ILow and IHigh, respectively, the total of detected VOCs increased weekly and reached their highest total in 35-day-old basil plants (Table 3).
Regardless of the light intensities applied, each cultivar underwent analogous compositional changes throughout the trial period. In example, under both light intensity treatments, percentages of linalool and trans-methyl cinnamate increased and percentages of methyl chavicol and methyl eugenol decreased in the developing ‘Cinnamon’ cultivar (Table 5).
However, in comparison to the compositional changes observed in the cultivars under IHigh, the same directional changes occurred time-delayed under ILow. In example, while the percentage of methyl chavicol reduced from ~ 17.7 % at 14 DAS to ~ 12.0 % at 21 DAS and ~ 9.1 % at 28 DAS under IHigh in the basil cultivar ‘Cinnamon’, a comparable drop was observed one week later under ILow as the percentage of methyl chavicol decreased from ~ 20.5 % at 21 DAS to ~ 15.3 % at 28 DAS and finally ~ 8.7 % at 35 DAS (Table 5). In the same manner, the percentage of linalool increased from ~ 5.4 % at 14 DAS to ~ 8.3 % at 21 DAS under IHigh, while linalool increased in the same way (from ~ 4.5 to ~ 8.8 %) two weeks later between 28 DAS and 35 DAS under ILow in the cultivar ‘Dark Opal’, (Table 6).
So far, not many studies have investigated compositional differences in Ocimum basilicum L. under different (LED) light intensities, but their outcomes are highly consistent with our findings. In example, Chang et al. (2008) and Walters et al. (2021) reported increasing linalool concentrations in the green basil cultivars ‘Genovese’ and ‘Nufar’, respectively, with increasing light intensities, which agrees with our findings in the linalool-containing cultivars ‘Cinnamon’, ‘Dark Opal’ and ‘Thai Magic’. Also, the observed relative decrease of methyl eugenol with increasing light intensities in cv. Genovese (Chang et al., 2008) was observed in the three cultivars that were characterized by elevated concentrations of methyl eugenol (cv. Cinnamon, cv. Dark Opal and cv. Thai Magic). The authors also described fairly constant as well as slightly increasing relative concentrations of 1,8-cineole in cv. Genovese and cv. Nufar, respectively. This is also congruent with the indifferent relative concentrations of 1,8-cineole in the cultivars ‘Anise’, ‘Cinnamon’ and ‘Thai Magic, and the slight upwards trend of 1,8-cineole concentrations detected in cv. Dark Opal with increasing light intensity. In addition, the fluctuating relative eugenol concentrations reported by Chang et al. (2008) and Walters et al. (2021) were also detected in our study in the eugenol-containing cultivars ‘Cinnamon’ and ‘Dark Opal’. Alike the findings observed by Walters et al. (2021) in 14-day-old cv. Nufar seedlings, we observed no differences in relative methyl chavicol concentrations between both light treatments throughout the trial period in cv. Cinnamon and cv. Dark Opal and 14 DAS in cv. Anise. In contrast, decreased relative methyl chavicol concentrations under IHigh were observed in older cv. Anise at 21, 28 and 35 DAS.
During the last few years, great progress has been made in the elucidation of most of the genes, transcription factors, enzymes, and substrates involved in the biosynthesis of the individual terpenes and phenylpropenes detected in our study. This led to coherent proposals of their biosynthetic pathways in the peltate glands of basil (Gang et al., 2001; Iijima et al., 2004; Kapteyn et al., 2007; Zvi et al., 2012; Dhar et al., 2020). In vivo however, the individual VOC profiles depend greatly on enzyme abundance and activity, their substrate specificity and availability (Lewinsohn et al., 2000; Muñoz-Bertomeu et al., 2006; Xie et al., 2008; Singh et al., 2015; Yahaa et al., 2019), all of which can be highly affected by abiotic factors (including drought stress (Mandoulakani et al., 2017), carbon dioxide concentration and temperature (Chang et al., 2005; Tursun & Telci, 2020), nutrients and biofertilizers (Hanif et al., 2017, Dehsheikh et al., 2020), light quality (Carvalho et al., 2016; Hosseini et al., 2018; Milenković et al., 2019; Tabbert et al., 2022) and elicitors (Deschamps & Simon, 2006; Loni et al., 2021)). The dynamics are further complicated as basils’ VOC compositions do not only depend on individual leaf maturation (Lewinsohn et al., 2000) but also considerably on the position of the basil leaf along the stem (Fischer et al., 2011). Thus, the underlying processes that regulate these compositional changes are exceptionally complex.
Nevertheless, the weekly assessments of the basils’ VOC compositions clearly show that each basil cultivar undergoes fundamentally well-coordinated compositional changes. As the detected compositional changes were identical under both light intensity treatments and occurred only time-delayed under ILow in comparison to IHigh, it becomes evident that the observed compositional differences in our study (as well as the differences observed by Chang et al., 2008 and Walters et al., 2021) are related to the cultivars’ developmental stage and thus, growth rates. The high correlations between the basils’ complex VOC profiles and their morphological stages as determined by principal component regressions (Table 7) strongly support this developmental stage-dependency.
Different VOC accumulation patterns were observed between the investigated basil cultivars (Figures 5Q–S). While VOC concentrations per gram of leaf dry matter (LDM) gradually increased in the cultivars ‘Anise’, ‘Cinnamon’ and ‘Thai Magic’ under both light intensity treatments during the trial period, a decreasing trend in VOC concentrations was observed in cv. Dark Opal. That VOC accumulation patterns strongly deviate within the species Ocimum basilicum has already been demonstrated by Macchia et al., 2006 and Szabó & Bernáth, 2002.
Within each cultivar, VOC concentrations generally did not differ between ILow and IHigh during each investigated time point, however, exceptions were observed in the cultivars ‘Dark Opal’ and ‘Thai Magic’ (Figures 5Q–S). The reason for the indifferent VOC concentrations observed under both light intensity treatments at each time point are likely to be attributed to the fact that the examined leaf extracts contained all developed leaves per basil plant irrespective of leaf age and maturation: Generally, glandular trichome densities decline while leaves age and expand. Though young leaves generally contain lower VOC quantities than older leaves, younger leaves tend to have higher VOC concentrations due to their lower weight (Fischer et al., 2011). The significantly lower VOC concentration detected under ILow in comparison to IHigh at 14 DAS in ‘Dark Opal’ (Figure 5S) may be ascribed to the cultivars’ limited photosynthetic capabilities under low light conditions as discussed before in section 3.4 and hence, a presumably lower availability of photosynthates for secondary metabolism. The slightly increased VOC concentration observed under ILow in comparison to IHigh at 35 DAS in cv. Thai Magic (Figure 5T) may indicate that this cultivar reaches its maximum VOC accumulation before flower buds arise (Figure 4D) after which VOC concentrations decrease – an accumulation pattern that has previously been described for the Ocimum basilicum cultivars ‘Rit-Sat’ and ‘Lengyel’ (Szabó & Bernáth, 2002) as well as for Ocimum ciliatum (Moghaddam et al., 2015). However, further experiments are needed to prove these hypotheses.
When comparing total VOC yields produced at the end of the experiment (35 DAS), all cultivars had accumulated substantially greater VOC quantities under IHigh than basils of the same age under ILow due to the cultivars’ overall greater leaf biomasses accumulated under IHigh (Table 2, Figures 5U–X). In comparison to VOC yields under ILow, yields were elevated by ~ 12.4, ~ 45.6, ~ 60.8 and ~ 69.2 % under IHigh in cultivars ‘Cinnamon’, ‘Thai Magic’, ‘Anise’ and ‘Dark Opal’, respectively.
It is well known that the flavor of basil is due to the presence and individual concentration of specific terpenes and phenylpropenes (for basil aroma descriptions see Patel et al., 2021). As the leaf extracts of the cultivars ‘Anise’ and ‘Thai Magic’ contained high concentrations of methyl chavicol throughout the investigation, both cultivars should be characterized by strong anise-like/licorice flavors regardless of their developmental stage and light intensity treatment applied (Tables 3, 4). With relative concentrations of 1,8-cineole between 6.8 and 9.3 %, ‘Anise’ should also include a noticeable eucalyptus aroma (Table 3). However, as shown by Lee et al. (2017), a trained panel was able to clearly discriminate between two basil cultivars that contained the same array of flavoring volatiles in different relative ratios. That indicates that the specific flavors of the cultivars ‘Cinnamon’ and ‘Dark Opal’ may tremendously change over time: While relatively high concentrations of methyl chavicol (imparting an anise-like/licorice aroma) and methyl eugenol (imparting a weak clove-like aroma) were detected during ‘Cinnamons’ early development, more mature ‘Cinnamon’ basils were characterized by relatively high concentrations of linalool (imparting a sweet floral aroma) and trans-methyl cinnamate (imparting a cinnamon-like aroma) (Table 5). Similarly, while methyl eugenol (weak clove-like aroma) clearly dominated in young ‘Dark Opal’, the relative abundances of linalool (sweet floral aroma), eugenol (clove-like aroma) and 1,8-cineole (eucalyptus aroma) increased greatly over time (Table 6).
Though concrete health risks for humans have not been confirmed, methyl chavicol (estragole) and methyl eugenol have shown to induce dose-dependent genotoxic and carcinogenic effects in multiple independent animal trails (NTP, 2000; Martins et al., 2012) which raised toxicological concerns of these naturally occurring substances. On grounds of the preventive protection of public health, legislators banned the addition of methyl chavicol and methyl eugenol as food additives and set maximum levels of these substances if naturally present in flavorings and food ingredients (Regulation No 1334, 2008). Consequently, basil producers and food processing industries are obligated to exploit possibilities to largely lower these two critical constituents.
As the main VOC in cv. Anise and cv. Thai Magic, methyl chavicol was found in consistently high percentages regardless of DAS and light intensity treatment (Tab. 3, 4). However, independent of the light intensity treatment, the percentages of methyl chavicol and methyl eugenol continuously decreased during ontogenesis of cv. Cinnamon (Tab. 5). Thus, by thoughtfully managing the time of harvest, levels of both critical constituents can be significantly reduced. Further, with 75.5 % under ILow and 66.3 % under IHigh at DAS 14, the percentages of methyl eugenol are indifferently high under both light treatments in cv. Dark Opal (Tab. 6). While the percent share reduces only to 63 % under ILow, the percent share significantly drops to 25.2 % under IHigh by the end of the experiment. Hence, cultivating cv. Dark Opal under low light intensities is not only unsuitable in terms of low yield and insufficient morphological development, but also with regard to methyl eugenol as a compound of toxicological concern.
In addition, as observed in cv. Dark Opal, via suitable light intensity settings, contents of (critical) compounds can be controlled and significantly reduced.
3.7 In contrast to purple basil, green basil cultivars use energy more efficiently under ILow
Despite the accelerated basil development observed under IHigh, the green-leafed cultivars ‘Anise’, ‘Cinnamon’ and ‘Thai Magic’ converted the available light energy more effectively into biomass under ILow than the basil plants that were cultivated under IHigh (Table 8). If these three cultivars were to be grown until marketability (based on the morphological criteria described in section 3.2), more than 50 % of electrical energy could be saved under ILow in comparison to IHigh, while cultivar-dependent yields would only be decreased by approximately 3-11 % (Table 9). In theory, a little more than one additional crop cycle per year could be generated under IHigh. In contrast, year-round basil cultivations under ILow would save ~ 57 % in energy usage while cultivar-dependent productivity losses would be between 15-19 % (Table 10). Hence, the great low light adaptability of the green basil cultivars (as described in section 3.4) ultimately resulted in improved biomass efficiencies under ILow when compared to the biomass efficiencies reached under IHigh.
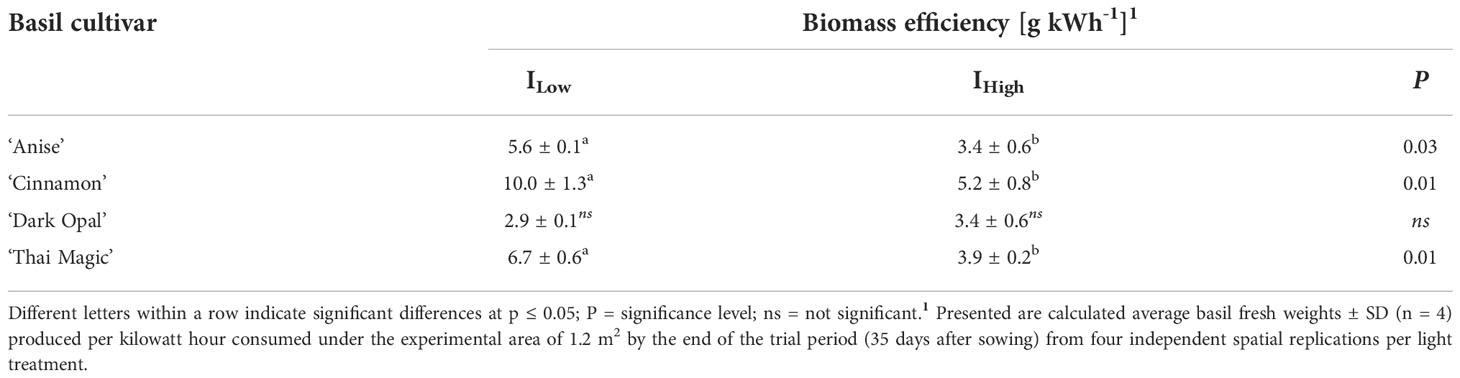
Table 8 Biomass efficacy of four Ocimum basilicum L. cultivars grown under two different light intensity treatments.
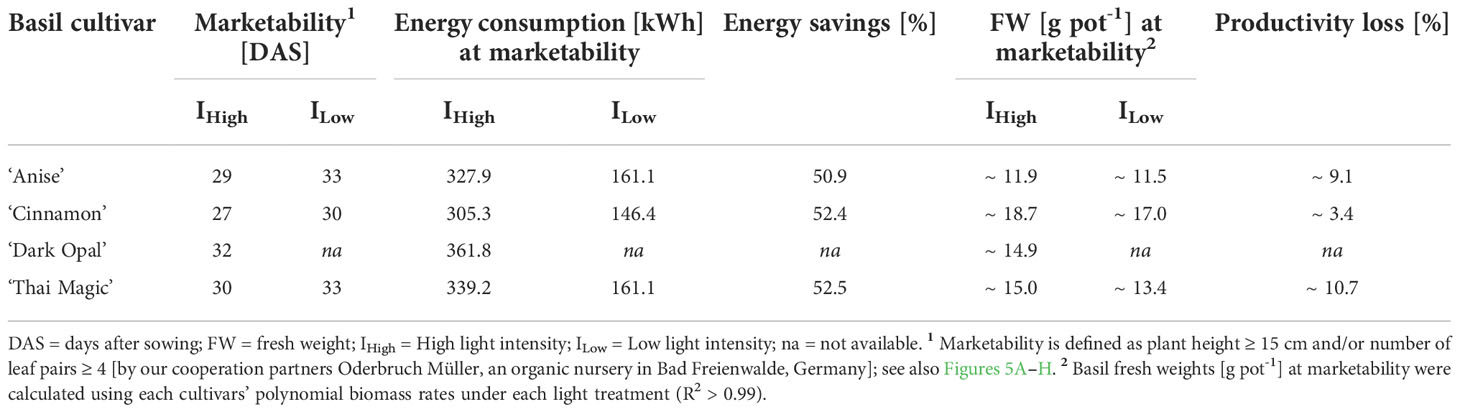
Table 9 Energy consumption and biomass at marketability of four Ocimum basilicum L. cultivars grown under two different light intensities.
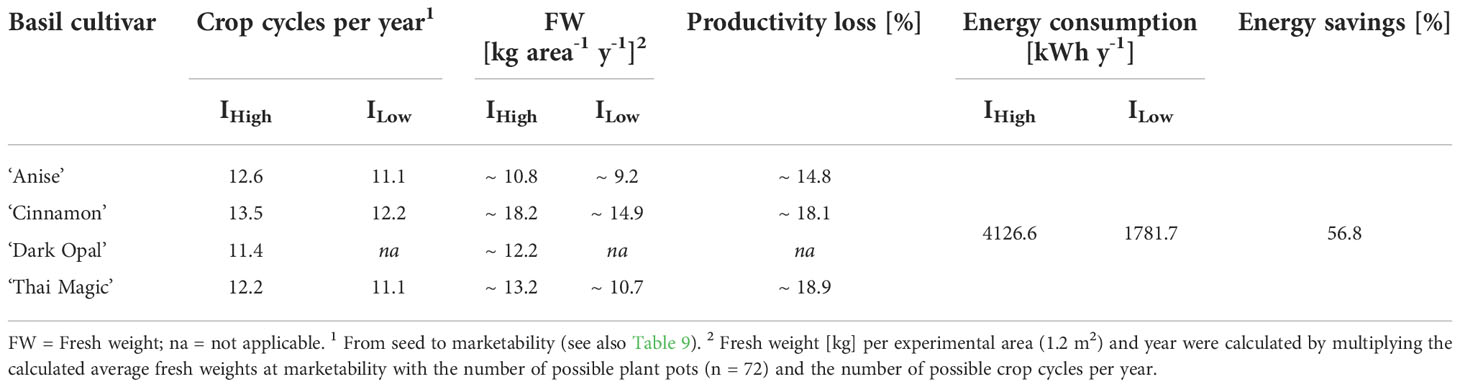
Table 10 Achievable crop cycles, biomass production, and its energy consumption per year when grown until marketability.
In contrast, biomass efficiencies of cv. Dark Opal were statistically indifferent between the two light intensity treatments (Table 8). Because cv. Dark Opal did not reach the marketability threshold under ILow during the trial period, a cultivation under ILow light conditions would result in the lowest number of crop cycles per year and the greatest productivity losses of all investigated cultivars (Tables 9, 10).
3.8 Improvement of basil production via eustress management
In general, beneficial effects induced by small doses and/or durations of stress factors are called eustressor effects (Vázquez-Hernández et al., 2019), and basil producers may be encouraged to provoke slight light and/or shade stresses in basil to stimulate different beneficial effects on their performance and/or human health: Even though the observed accumulations of anthocyanins in the top leaves of cv. Anise, cv. Cinnamon and cv. Thai Magic under IHigh represent direct stress responses triggered by the intense light conditions they grew into to protect their chloroplasts from photoinhibitory and photooxidative effects (as described in section 3.3), other diverse protective roles are ascribed to anthocyanins as members of the flavonoid group (Lila, 2004). These phytochemicals are known to enhance the plants’ resistance to the effects of chilling and freezing and increase their resistance to herbivores and pathogens (Gould, 2004). In addition, numerous protective roles to human health (from antioxidative and free-radical scavenging capabilities, obesity prevention, amelioration of hyperglycemia, neuroprotective effects to positive influences on vascular functions) have been attributed to anthocyanins (well summarized by Lila, 2004). In addition, the light stress under IHigh resulted in accelerated basil growths, accompanied with enhanced VOC maturations and high yields (as described in sections 3.1, 3.2 and 3.6). On the other hand, the shade stress under ILow resulted in consumer-preferred leaf appearances and producer-preferred biomass efficiencies (as described in sections 3.4 and 3.7). Hence, basil producers may be interested in carefully managing light and/or shade stresses to stimulate specific positive effects of interest in basil and/or to improve their production efficiencies (Vázquez-Hernández et al., 2019).
3.9 Conclusion
From seed to marketability, the broad-bandwidth LED light spectrum including FR light with elevated R and B light fractions enabled an adequate growth and development of all four investigated basil cultivars (‘Anise’, ‘Cinnamon’, ‘Dark Opal’ and ‘Thai Magic’) under both rising light intensity conditions applied in this study.
In comparison to the ILow light conditions, IHigh resulted in an accelerated development and thus expedited marketability of all investigated basil cultivars. However, exposure to light intensities above 300 µmol m-2 s-1 caused adverse quality-reducing effects in the green-leafed cultivars ‘Anise’, ‘Cinnamon’ and ‘Thai Magic’ and should therefore not be exceeded.
In contrast, the applied ILow light conditions resulted in consumer-preferred appearances and greater biomass efficiencies and appear to be the result of a great low light adaptability of green-leafed basil cultivars. Despite the time-delayed marketability (based on morphological criteria) under ILow in comparison to IHigh, the superior visual quality of the green-leafed cultivars in combination with the significantly reduced energy consumptions under ILow can ultimately result in greater revenues for basil producers.
Though purple-leafed cultivar ‘Dark Opal’ was able to mitigate high intensity light stress responses under IHigh in comparison to the green-leafed cultivars, its indoor production until the common marketability criteria (plant height ≥ 15 cm and/or leaf pairs ≥ 4) are reached remains the least economical due to the high energy consumptions necessary, the comparatively low yields and number of annual crop cycles possible.
However, basil cultivar-specific VOC contents and profiles proved to change tremendously over time in a developmental stage-correlated manner. Thus, consumer flavor preferences of differently aged basil plants should be further explored, and time of harvest should be individually reconsidered [especially as the demand and popularity of microgreens is on the rise (Kyriacou et al., 2016; Mir et al., 2016)].
Data availability statement
The original contributions presented in the study are included in the article. Further inquiries can be directed to the corresponding authors.
Author contributions
JT: conceptualization, project administration, formal analysis, validation, investigation, data curation, and writing – original draft preparation. DR: methodology, formal analysis, validation. AK: conceptualization, and writing – review and editing. HS: conceptualization, project administration, methodology, funding acquisition, resources, and supervision. All authors contributed to the article and approved the submitted version.
Funding
This work was supported and funded by the European Innovation Partnership for Improvement of Agricultural Productivity and Sustainability (grant number 204016000016/80168353) via the European Agricultural Fund for Rural Development.
Acknowledgments
We sincerely thank Maik Repnack and his team for technical support, Roland Buchhorn, Claudia Könecke and Heike Bäumer for cultivation assistance and Dominique Conrad and Mario Harke for assisting in data curation. We further thank Matthias Melzig from Free University Berlin for his support.
Conflict of interest
HS is the owner of the company Consulting & Project Management for Medicinal and Aromatic Plants.
The remaining authors declare that the research was conducted in the absence of any commercial or financial relationship that could be construed as a potential conflict of interest.
Publisher’s note
All claims expressed in this article are solely those of the authors and do not necessarily represent those of their affiliated organizations, or those of the publisher, the editors and the reviewers. Any product that may be evaluated in this article, or claim that may be made by its manufacturer, is not guaranteed or endorsed by the publisher.
References
Barut, M., Tansi, L. S., Akyuz, A. M., Karaman, S. (2021). Quality and yield of different basil (Ocimum basilicum l.) cultivars with various planting and cutting times under hot Mediterranean climate. Appl. Ecol. Environ. Res. 19 (4), 3115–3136. doi: 10.15666/aeer/1904_31153136
Beaman, A. R., Gladon, R. J., Schrader, J. A. (2009). Sweet basil requires an irradiance of 500 µmol m-2 s-1 for greatest edible biomass production. HortSci. 44 (1), 64–67. doi: 10.21273/HORTSCI.44.1.64
Benke, K., Tomkins, B. (2018) Future food production systems: vertical farming and controlled-environment agriculture. Sustain.: Sci. Pract. Policy. 13(1):13–26. doi: 10.1080/15487733.2017.1394054
Carović-Stanko, K., Šalinović, A., Grdiša, M., Liber, Z., Kolak, I., Satovic, Z. (2011). Efficiency of morphological trait descriptors in discrimination of Ocimum basilicum l. accessions. Plant Biosyst. 145 (2), 298–305. doi: 10.1080/11263504.2011.558677
Carvalho, S. D., Schieterman, M. L., Abrahan, C. E., Colquhoun, T. A., Folta, K. M. (2016). Light quality dependent changes in morphology, antioxidant capacity, and volatile production in sweet basil (Ocimum basilicum). Front. Plant Sci. 7. doi: 10.3389/fpls.2016.01328
Chang, X., Alderson, P., Wright, C. (2005). Effect of temperature integration on the growth and volatile oil content of basil (Ocimum basilicum l.). J. Hortic. Sci. Biotechnol. 80 (5), 593–598. doi: 10.1080/14620316.2005.11511983
Chang, X., Alderson, P. G., Wright, C. J. (2008). Solar irradiance level alters the growth of basil (Ocimum basilicum l.) and its content of volatile oils. Environ. Exp. Bot. 63 (1-3), 216–223. doi: 10.1016/j.envexpbot.2007.10.017
Currey, C. J., Kopsell, D. A., Mattson, N. S., Craver, J. K., Lopez, R. G., Erwin, J. E., et al. (2017). “Supplemental and sole-source lighting of leafy greens, herbs and microgreens,” in Light management in controlled environments. Eds. Lopez, R., Runkle, E. (Willoughby, Ohio, USA: Meister Media Worldwide), 170–175.
Dehsheikh, A. B., Sourestani, M. M., Zolfaghari, M., Enayatizamir, N. (2020). Changes in soil microbial activity, essential oil quantity, and quality of Thai basil as response to biofertilizers and humic acid. J. Clean Prod. 256, 120439. doi: 10.1016/j.jclepro.2020.120439
Deschamps, C., Simon, J. E. (2006). Terpenoid essential oil metabolism in basil (Ocimum basilicum l.) following elicitation. J. Essent. Oil Res. 18 (6), 618–621. doi: 10.1080/10412905.2006.9699183
Dhar, N., Sarangapani, S., Reddy, V. A., Kumar, N., Panicker, D., Jin, J., et al. (2020). Characterization of a sweet basil acyltransferase involved in eugenol biosynthesis. J. Exp. Bot. 71 (12), 3638–3652. doi: 10.1093/jxb/eraa142
Elansary, H. O., Mahmoud, E. A. (2014). Basil cultivar identification using chemotyping still favored over genotyping using core barcodes and possible resources of antioxidants. J. Essent. Oil Res. 27 (1), 82–87. doi: 10.1080/10412905.2014.982874
Flanigan, P. M., Niemeyer, E. D. (2014). Effect of cultivar on phenolic levels, anthocyanin composition, and antioxidant properties in purple basil (Ocimum basilicum l.). Food Chem. 164, 518–526. doi: 10.1016/j.foodchem.2014.05.061
Fischer, R., Nitzan, N., Chaimovitsh, D., Rubin, B., Dudai, N. (2011). Variation in essential oil composition within individual leaves of sweet basil (Ocimum basilicum l.) is more affected by leaf position than by leaf age. J. Agric. Food Chem. 59 (9), 4913–4922. doi: 10.1021/jf200017h
Gang, D. R., Wang, J., Dudareva, N., Simon, J. E., Lewinsohn, E., Pichersky, E. (2001). An investigation of the storage and biosynthesis of phenylpropenes in sweet basil. Plant Physiol. 125 (2), 539–555. doi: 10.1104/pp.125.2.539
Garcia-Plazaola, J. I., Esteban, R., Fernandez-Marin, B., Kranner, I., Porcar-Castell, A. (2012). Thermal energy dissipation and xanthophyll cycles beyond the Arabidopsis model. Photosynth. Res. 113, 89–103. doi: 10.1007/s11120-012-9760-7
Gould, K. S. (2004). Nature’s Swiss army knife: The diverse protective roles of anthocyanins in leaves. J. Biomed. Biotech. 5, 314–320. doi: 10.1155/S1110724304406147
Gould, K. S., Jay-Allemand, C., Logan, B. A., Baissac, Y., Bidel, L. P. R. (2018). When are foliar anthocyanins useful to plants? re-evaluation of the photoprotection hypothesis using Arabidopsis thaliana mutants that differ in anthocyanin accumulation. Environ. Exp. Bot. 154, 11–22. doi: 10.1016/j.envexpbot.2018.02.006
Hanif, M. A., Nawaz, H., Ayub, M. A., Tabassum, N., Kanwal, N., Rashid, N., et al. (2017). Evaluation of the effects of zinc on the chemical composition and biological activity of basil essential oil by using raman spectroscopy. Ind. Crop Prod. 96, 91–101. doi: 10.1016/j.indcrop.2016.10.058
Hirai, N., Yamamuro, M., Koshimizu, K., Shinozaki, M., Takimoto, A. (1994). Accumulation of phenylpropanoids in the cotyledons of morning glory (Pharbitis nil) seedlings during the induction of flowering by low temperature treatment, and the effect of precedent exposure to high-intensity light. Plant Cell Physiol. 35 (4), 691–695. doi: 10.1093/oxfordjournals.pcp.a078644
Hosseini, A., Mehrjerdi, M. Z., Aliniaeifard, S. (2018). Alteration of bioactive compounds in two varieties of basil (Ocimum basilicum) grown under different light spectra. J. Essent. Oil Bear. Plants 21 (4), 913–923. doi: 10.1080/0972060X.2018.1526126
Iijima, Y., Davidovich-Rikanati, R., Fridman, E., Gang, D. R., Bar, E., Lewinsohn, E., et al. (2004). The biochemical and molecular basis for the divergent patterns in the biosynthesis of terpenes and phenylpropenes in the peltate glands of three cultivars of basil. Plant Physiol. 136 (3), 3724–3736. doi: 10.1104/pp.104.051318
Jayasinghe, C., Gotoh, N., Aoki, T., Wada, S. (2003). Phenolics composition and antioxidant activity of sweet basil (Ocimum basilicum l.). J. Agric. Food Chem. 51(15), 4442–4449. doi: 10.1021/jf034269o
Kagawa, T., Sakai, T., Suetsugu, N., Oikawa, K., Ishiguro, S., Kato, T., et al. (2001). Arabidopsis NPL1: a phototropin homolog controlling the chloroplast high-light avoidance response. Science. 291 (5511), 2138–2141. doi: 10.1126/science.291.5511.2138
Kapteyn, J., Qualley, A. V., Xie, Z., Fridman, E., Dudareva, N., Gang, D. R. (2007). Evolution of cinnamate/p-coumarate carboxyl methyltransferase and their role in the biosynthesis of methylcinnamate. Plant Cell. 19 (10), 3212–3229. doi: 10.1105/tpc.107.054155
Kozai, T. (2019). Towards sustainable plant factories with artificial lighting (PFALs) for achieving SDGs. Int. J. Agric. Biol. Eng. 12 (5), 28–37. doi: 10.1080/15487733.2017.1394054
Kwee, E. M., Niemeyer, E. D. (2011). Variations in phenolic composition and antioxidant properties among 15 basil (Ocimum basilicum l.) cultivars. Food Chem. 128 (4), 1044–1050. doi: 10.1016/j.foodchem.2011.04.011
Kyriacou, M. C., Rouphael, Y., Di Gioia, F., Kyratzis, A., Serio, F., Renna, M., et al. (2016). Micro-scale vegetable production and the rise of microgreens. Trends Food Sci. Technol. 57 (A), 103–115. doi: 10.1016/j.tifs.2016.09.005
Landi, M., Guidi, L., Pardossi, A., Tattini, M., Gould, K. S. (2014). Photoprotection by foliar anthocyanins mitigates effects of boron toxicity in sweet basil (Ocimum basilicum). Planta. 240, 941–953. doi: 10.1007/s00425-014-2087-1
Lee, R. J., Bhandari, S. R., Lee, G., Lee, J. G. (2019). Optimization of temperature and light, and cultivar selection for the production of high-quality head lettuce in a closed-type plant factory. Hortic. Environ. Biotechnol. 60, 207–216. doi: 10.1007/s13580-018-0118-8
Lee, R., Simon, J. E., Reichert, W., Juliani, H. R., Tepper, B. J. (2017). A fresh language. Perfum Flavor. 42, 36–50.
Lewinsohn, E., Ziv-Raz, I., Dudai, N., Tadmor, Y., Lastochkin, E., Larkov, O., et al. (2000). Biosynthesis of estragole and methyleugenol in sweet basil (Ocimum basilicum l.). developmental and chemotypic association of allylphenol O-methyltransferase activities. Plant Sci. 160 (1), 27–35. doi: 10.1016/S0168-9452(00)00357-5
Liaros, S., Botsis, K., Xydis, G. (2016). Technoeconomical evaluation of urban plant factories: The case of basil (Ocimum basilicum). Sci. Total Environ. 554-555, 218–227. doi: 10.1016/j.scitotenv.2016.02.174
Lila, M. A. (2004). Anthocyanins and human health: An in vitro investigative approach. J. Biomed. Biotech. 5, 306–313. doi: 10.1155/S111072430440401X
Lin, K.-H., Huan, M.-Y., Hsu, M.-H. (2021). Morphological and physiological response in green and purple basil plants (Ocimum basilicum) under different proportions of red, green, and blue LED lightings. Sci. Hortic. 275, 109677. doi: 10.1016/j.scienta.2020.109677
Logan, B. A., Stafstrom, W. C., Walsh, M. J. L., Reblin, J. S., Gould, K. S. (2015). Examining the photoprotection hypothesis for adaxial foliar anthocyanin accumulation by revisiting comparisons of green- and red-leafed varieties of coleus (Solenostemon scutellarioides). Photosynth. Res. 124, 267–274. doi: 10.1007/s11120-015-0130-0
Loni, A., Saadatmand, S., Yazdi, H. L., Iranbakhsh, A. (2021). Application of nano-ß-cyclodextrin to induce biosynthesis of phenylpropanoids and antioxidant activity in basil. Iran. J. Sci. Technol. Trans. Sci. 45, 1951–1962. doi: 10.1007/s40995-021-01163-8
Lopez, R. G., Runkle, E. S. (2008). Photosynthetic daily light integral during propagation influences rooting and growth of cuttings and subsequent development of new guinea impatiens and petunia. HortScience. 43 (7), 2052–2059. doi: 10.21273/HORTSCI.43.7.2052
Macchia, M., Pagano, A., Ceccarini, L., Benvenuti, S., Cioni, P. L., Flamini, G. (2006). Agronomic and phytochimic characteristics in some genotypes of Ocimum basilicum l. Acta Hortic. 723, 143–150. doi: 10.17660/ActaHortic.2006.723.15
Maggio, A., Roscigno, G., Bruno, M., De Falco, E., Senatore, F. (2016). Essential oil variability in a collection of Ocimum basilicum l. (Basil) cultivars. Chem. Biodivers. 13 (10), 1357–1368. doi: 10.1002/cbdv.201600069
Makri, O., Kintzios, S. (2008). Ocimum sp (Basil): Botany, cultivation, pharmaceutical properties, and biotechnology. J. Herbs Spices Med. Plants. 13 (3), 123–150. doi: 10.1300/J044v13n03_10
Ma, Y., Ma, X., Gao, X., Wu, W., Zhou, B. (2021). Light induced regulation pathway of anthocyanin biosynthesis in plants. Int. J. Mol. Sci. 22, 11116. doi: 10.3390/ijms222011116
Mandoulakani, B. A., Eyvazpour, E., Ghadimzadeh, M. (2017). The effect of drought stress on the expression of key genes involved in the biosynthesis of phenylpropanoids and essential oil components in basil (Ocimum basilicum l.). Phytochem. 139, 1–7. doi: 10.1016/j.phytochem.2017.03.006
Market Research Report (2022) Basil leaves market – global industry analysis, size and forecas-2027. Available at: https://www.futuremarketinsights.com/reports/basil-leaves-market (Accessed August 10th, 2022).
Martins, C., Cacão, R., Cole, K. J., Phillips, D. H., Laires, A., Rueff, J., et al. (2012). Estragole: A weak direct-acting food-borne genotoxin and potential carcinogen. Mutat. Res. 747 (1), 86–92. doi: 10.1016/j.mrgentox.2012.04.009
Milenković, L., Stanojević, J., Cvetković, D., Stanojević, L., Lalević, D., Ŝunić, L., et al. (2019). New technology in basil production with high essential oil yield and quality. Ind. Crops Prod. 140, 111718. doi: 10.1016/j.indcrop.2019.111718
Mir, S. A., Shah, M. A., Mir, M. M. (2016). Microgreens: Production, shelf life, and bioactive components. Crit. Rev. Food Sci. Nutr. 57 (12), 2730–2736. doi: 10.1080/10408398.2016.1144557
Misyura, M., Colasanti, J., Rothstein, S. J. (2013). Physiological and genetic analysis of Arabidopsis thaliana anthocyanin biosynthesis mutants under chronic adverse environmental conditions. J. Exp. Bot. 64 (1), 229–240. doi: 10.1093/jxb/ers328
Moccaldi, L. A., Runkle, E. S. (2007). Modeling the effects of temperature and photosynthetic daily light integral on growth and flowering of Salvia splendens and Tagetes patula. J. Amer. Soc Hortic. Sci. 123 (3), 283–288. doi: 10.21273/JASHS.132.3.283
Moghaddam, M., Pirbalouti, A. G., Mehdizadeh, L., Pirmoradi, M. R. (2015). Changes in composition and essential oil yield of Ocimum ciliatum at different phenological stages. Eur. Food Red. Technol. 240, 199–204. doi: 10.1007/s00217-014-2320-y
Muñoz-Bertomeu, J., Arrillaga, I., Ros, R., Segura, J. (2006). Up-regulation of 1-deoxy-D-xylulose-5-phosphate synthase enhances production of essential oils in transgenic spike lavender. Plant Physiol. 142 (3), 890–900. doi: 10.1104/pp.106.086355
Naznin, M. T., Lefsrud, M., Gravel, V., Azad, M. O. K. (2019). Blue light added with red LEDs enhance growth characteristics, pigments content, and antioxidant capacity in lettuce, spinach, kale, basil, and sweet pepper in a controlled environment. Plants. 8 (4), 93. doi: 10.3390/plants8040093
Nemali, K. S., van Iersel, M. W. (2004). Acclimation of wax begonia to light intensity: changes in photosynthesis, respiration, and chlorophyll concentration. J. Amer. Soc Hortic. Sci. 129 (5), 745–751. doi: 10.21273/jashs.129.5.0745
NTP (2000). NTP toxicology and carcinogenesis studies of methyleugenol (CAS no. 93-15-2) in F344/N rats and B6C3F1 mice (Gavage studies). Natl. Toxicol. Progam Tech. Rep. Ser. 491, 1–412.
Oh, W., Runkle, E. S., Warner, R. M. (2010). Timing and duration of supplemental lighting during the seedling stage influence quality and flowering in petunia and pansy. HortScience 45 (9), 1332–1337. doi: 10.21273/HORTSCI.45.9.1332
Park, K. S., Bekhzod, K., Kwon, J. K., Son, J. E. (2016). Development of a coupled photosynthetic model of sweet basil hydroponically grown in plant factories. Hortic. Environ. Biotechnol. 57 (1), 20–26. doi: 10.1007/s13580-016-0019-7
Patel, M., Lee, R., Merchant, E. V., Juliani, H. R., Simon, J. E., Tepper, B. J. (2021). Descriptive aroma profiles of fresh sweet basil cultivars (Ocimum ssp.): Relationship to volatile chemical composition. J. Food Sci. 86 (7), 3228–3239. doi: 10.1111/1750-3841.15797
Pennisi, G., Blasioli, S., Cellini, A., Maia, L., Crepaldi, A., Braschi, I., et al. (2019). Unraveling the role of red:blue LED lights on resource use efficiency and nutritional properties of indoor grown sweet basil. Front. Plant Sci. 10. doi: 10.3389/fpls.2019.00305
Pennisi, G., Pistillo, A., Orsini, F., Cellini, A., Spinelli, F., Nicola, S., et al. (2020). Optimal light intensity for sustainable water and energy use in indoor cultivation of lettuce and basil under red and blue LEDs. Sci. Hortic. 272, 109508. doi: 10.1016/j.scienta.2020.109508
Poulet, L., Massa, G., Morrow, R. C., Bourget, C. M., Wheeler, R. M., Mitchell, C. A. (2014). Significant reduction in energy for plant-growth lighting in space using targeted LED lighting and spectral manipulation. Life Sci. Space Res. 2, 43–53. doi: 10.1016/j.lssr.2014.06.002
Rahman, M. M., Vasiliev, M., Alameh, K. (2021). LED illumination spectrum manipulation for increasing the yield of sweet basil (Ocimum basilicum l.). Plants 10 (2), 344. doi: 10.3390/plants10020344
Regulation No 1334 (2008) Regulation (EC) no 1334/2008 of the European parliament and of the council of 16 December 2008 on flavourings and certain food ingredients with flavouring properties for use in and on foods and amending council regulation (EEC) no 1601/01, regulations (EC) no 2232/96 and (EC) no 110/2008 and directive 2000/13/EC. Available at: https://www.eumonitor.eu/9353000/1/j9vvik7m1c3gyxp/vi1q025fp0yp-ntr1-L_2008354EN.01004701-E0001 (Accessed September 13th 2022).
Rihan, H. Z., Aldarkazali, M., Mohamed, S. J., McMulkin, N. B., Jbara, M. H., Fuller, M. P. (2020). A novel new light recipe significantly increases the growth and yield of sweet basil (Ocimum basilicum) grown in a plant factory. Agronomy. 10, 934. doi: 10.3390/agronomy10070934
Rouphael, Y., Cardarelli, M., Bassal, A., Leonardi, C., Giuffrida, F., Colla, G. (2012). Vegetable quality as affected by genetic, agronomic and environmental factors. J. Food Agric. Environ. 10 (3), 680–688.
Schenkels, L., Saeys, W., Lauwers, A., De Proft, M. P. (2020). Green light induces shade avoidance to alter plant morphology and increases biomass production in ocimum basilicum l. Sci. Hortic. 261, 109002. doi: 10.1016/j.scienta.2019.109002
Schulz, H., Schrader, B., Quilitzsch, R., Pfeffer, S., Krüger, H. (2003). Rapid classification of basil chemotypes by various vibrational spectroscopy methods. J. Agric. Food Chem. 51 (9), 2475–2481. doi: 10.1021/jf021139r
Singh, P., Kalunke, R. M., Giri, A. P. (2015). Towards comprehension of complex chemical evolution and diversification of terpene and phenylpropanoid pathways in Ocimum species. RSC Adv. 5 (129), 106886–106904. doi: 10.1039/C5RA16637C
Sipos, L., Balázs, L., Székely, G., Jung, A., Sárosi, S., Radácsi, P., et al. (2021). Optimization of basil (Ocimum basilicum l.) production in LED light environments – a review. Sci. Horti. 289, 110486. doi: 10.1016/j.scienta.2021.110486
Solis-Toapanta, E., Gómez, C. (2019). Growth and photosynthetic capacity of basil grown for indoor gardening under constant or increasing daily light integrals. HortTechnology. 29 (6), 880–888. doi: 10.21273/HORTTECH04442-19
Song, T.-E., Moon, J.-K., Lee, C. H. (2020). Polyphenol content and essential oil composition of sweet basil cultured in a plant factory with light-emitting diodes. Hortic. Sci. Technol. 38 (5), 620–630. doi: 10.7235/HORT.20200057
Stagnari, F., Di Mattia, C., Galieni, A., Santarelli, V., D’Egidio, S., Pagnani, G., et al. (2018). Light quantity and quality supplies sharphly affect growth, morphological, physiological and quality traits of basil. Ind. Crop Prod. 122, 277–289. doi: 10.1016/j.indcrop.2018.05.073
Stetsenko, L. A., Pashkovsky, P. P., Voloshin, R. A., Kreslavski, V. D., Kuznetsov, V. V., Allakhverdiev, S. I. (2020). Role of anthocyanin and carotenoids in the adaptation of the photosynthetic apparatus of purple- and green-leaved cultivars of sweet basil (Ocimum basilicum) to high-intensity light. Photosynthetica. 58 (4), 890–901. doi: 10.32615/ps.2020.048
Szabó, K., Bernáth, J. (2002). Investigation of flowering dynamics of the basil (Ocimum basilicum l.) and its production consequences. Acta Hortic. 576, 105–112. doi: 10.17660/ActaHortic.2002.576.18
Tabbert, J. M., Krähmer, A., Schulz, H. (2022). Practical investigation of LED light qualities for peppermint (Mentha x piperita l.) cultivation focusing on plant quality and consumer safety aspects. Front. Food Sci. Technol. 2. doi: 10.3389/frfst.2022.852155
Takeno, K. (2016). Stress-induced flowering: the third category of flowering response. J. Exp. Bot. 67 (17), 4925–4934. doi: 10.1093/jxb/erw272
Tattini, M., Landi, M., Brunetti, C., Giordano, C., Remorini, D., Gould, K. S., et al. (2014). Epidermal coumaroyl anthocyanins protect sweet basil against excess light stress: multiple consequences of light attenuation. Physiol. Plant 152 (3), 585–598. doi: 10.1111/ppl.12201
Tursun, A. O., Telci, I. (2020). The effects of carbon dioxide and temperature on essential oil composition of purple basil (Ocimum basilicum l.). J. Essent. Oil Bear. Plants 23 (2), 255–265. doi: 10.1080/0972060X.2020.1741452
Tyystjärvi, E. (2013). Chapter seven - photoinhibition of photosystem II. Int. Rev. Cell Mol. Biol. 300, 243–303. doi: 10.1016/B978-0-12-405210-9.00007-2
Varga, F., Carović-Stanko, K., Ristić, M., Grdiŝa, M., Liber, Z., Šatović, Z. (2017). Morphological and biochemical intraspecific characterization of Ocimum basilicum l. Ind. Crop Prod. 109, 611–618. doi: 10.1016/j.indcrop.2017.09.018
Vázquez-Hernández, M. C., Parola-Contreras, I., Montoya-Gómez, L. M., Torres-Pacheco, I., Schwarz, D., Guevara-González, R. G. (2019). Eustressors: Chemical and physical stress factors used to enhance vegetables production. Sci. Hortic. 250, 223–229. doi: 10.1016/j.scienta.2019.02.053
Viršilė, A., Brazaitytė, A., Vaštakaitė-Kairienė, V., Miliauskienė, J., Jankauskienė, J., Novičkovas, A., et al. (2019). Lighting intensity and photoperiod serves tailoring nitrate assimilation indices in red and green baby leaf lettuce. J. Sci. Food Agr. 99, 6608–6619. doi: 10.1002/jsfa.9948
Walters, K. J., Lopez, R. G., Bridget, K. B. (2021). Leveraging controlled-environment agriculture to increase key basil terpenoid and phenylpropanoid concentrations: The effects of radiation intensity and CO2 concentrations on consumer preference. Front. Plant Sci. 11. doi: 10.3389/fpls.2020.598519
Wesolowska, A., Jadczak, D. (2016). Composition of the essential oils from inflorescences, leaves and stems of Ocimum basilicum ‘Cinnamon’ cultivated in north-western Poland. J. Essent. Oil Bear. Plants 19 (4), 1037–1042. doi: 10.1080/0972060X.2016.1197801
Xie, Z., Kapteyn, J., Gang, D. R. (2008). A systems biology investigation of the MEP/terpenoid and shikimate/phenylpropanoid pathways points to multiple levels of metabolic control in sweet basil glandular trichomes. Plant J. 54 (3), 349–361. doi: 10.1111/j.1365-313X.2008.03429.x
Yahaa, M., Berim, A., Nawade, B., Ibdah, M., Dudareva, N., Ibdah, M. (2019). Biosynthesis of methyleugenol and methylisoeugenol in Daucus carota leaves: Characterization of eugenol/isoeugenol synthase and O-methyltransferase. Phytochem. 159, 179–189. doi: 10.1016/j.phytochem.2018.12.020
Zhen, S., Bugbee, B. (2020). Far-red photons have equivalent efficiency to traditional photosynthetic photons: Implications for redefining photosynthetically active radiation. Plant Cell Environ. 43 (5), 1259–1272. doi: 10.1111/pce.13730
Keywords: light-emitting diode (LED), morphology, essential oil, volatile organic compound (VOC), energy use efficiency (EUE)
Citation: Tabbert JM, Riewe D, Schulz H and Krähmer A (2022) Facing energy limitations – approaches to increase basil (Ocimum basilicum L.) growth and quality by different increasing light intensities emitted by a broadband LED light spectrum (400-780 nm). Front. Plant Sci. 13:1055352. doi: 10.3389/fpls.2022.1055352
Received: 27 September 2022; Accepted: 31 October 2022;
Published: 24 November 2022.
Edited by:
Claudia Fuentealba, Pontificia Universidad Católica de Valparaíso, ChileReviewed by:
Ramón Gerardo Guevara-Gonzalez, Universidad Autónoma de Querétaro, MexicoRita Maggini, University of Pisa, Italy
Copyright © 2022 Tabbert, Riewe, Schulz and Krähmer. This is an open-access article distributed under the terms of the Creative Commons Attribution License (CC BY). The use, distribution or reproduction in other forums is permitted, provided the original author(s) and the copyright owner(s) are credited and that the original publication in this journal is cited, in accordance with accepted academic practice. No use, distribution or reproduction is permitted which does not comply with these terms.
*Correspondence: Jenny Manuela Tabbert, amVubnkudGFiYmVydEBqdWxpdXMta3VlaG4uZGU=; Andrea Krähmer, YW5kcmVhLmtyYWVobWVyQGp1bGl1cy1rdWVobi5kZQ==
†The author is a member of the MOCS (´more than one constituent substances´) initiative (www.vielstoffgemische.de)