- 1College of Pharmacy, Guizhou University of Traditional Chinese Medicne, Guiyang, China
- 2Guizhou Joint Laboratory for International Cooperation in Ethnic Medicine, Guizhou University of Traditional Chinese Medicne, Guiyang, China
Osthole is a natural coumarin compound which isolated from Cnidium monnieri (L.) Cusson, has extensive pharmacological activities and could be used as a leading compound for drug research and development. In a continuous effort to develop new acetylcholinesterase inhibitors from natural products, eighteen osthole esters were designed, synthesized, and confirmed by 1H NMR, 13C NMR and HRMS. The anti-AChE activity of These derivatives was measured at a concentration of 1.0 mol/mL in vitro by Ellman's method, and the result showed that 4m and 4o had moderate inhibitory activities with 68.8% and 62.6%, respectively. Molecular docking study results further revealed AChE interacted optimally with docking poses 4m and 4o. Network pharmacology also predicted that compound 4m could be involved in Ras signaling pathway, which made it a potential therapeutic target of AD.
Introduction
Alzheimer’s disease (AD) is a neurodegenerative brain disorder characterized by memory loss and cognitive impairments, which has affected 50 million people worldwide, with numbers projected to reach 135.5 million by 2050 (Lane et al., 2018; Bertram and Tanz, 2020). The neuropathological hallmarks of the disease are the presence of numerous senile amyloid β-peptide (Aβ) plaques, neurofibrillary tangles (NFT), synaptic loss and cholinergic neuron degeneration, or atrophy in the basal forebrain (Roberson and Harrell, 1997). With the loss of basal forebrain cholinergic cells, acetylcholine (ACh) decreases sharply, which is thought to contribute to cognitive impairments associated with AD (Bartus et al., 1982; Dunnett and Fobiger, 1993). Currently, one of the most common AD treatments is to suppress acetylcholinesterase activity in the brain in order to improve cognitive function.
Acetylcholinesterase (AChE), which is crucial for nerve conduction, primarily degrades acetylcholine (Nazir et al., 2018; Penumala et al., 2018). Acetylcholine is rapidly hydrolyzed by it at cholinergic synapses to terminate nerve impulse transmission (Silman and Sussman, 2005). X-ray crystallography studies revealed that there were two binding sites, the catalytic active site (CAS) at the bottom and the peripheral anionic site (PAS) near the entrance of the gorge (Bourne et al., 2003; Baharloo et al., 2015). Some studies have revealed that AChE could also play a key role in accelerating Aβ plaque deposition (Inestrosa et al., 1996; Hardy and Selkoe, 2002). AChE was also reported to interact with Aβ and promote amyloid fibril formation via a pool of amino acids located in proximity of the PAS (De Ferrari et al., 2001). Therefore, many pharmaceuticals have been developed for AD symptomatic treatment, such as rivastigmine, galantamine, tacrine, and donepezil (Schneider, 2000; Aranda-Abreu et al., 2011). However, these AChE inhibitors are commonly used in patients with Alzheimer’s to improve their cognitive function. These medications can cause nausea, diarrhea, anorexia, and abdominal pain (Shah and Reichman, 2006; Costantino et al., 2008; Jia et al., 2013). Accordingly, it is being attempted to develop natural AChE inhibitors that can replace the existing AChE inhibitors (Hansen et al., 2008).
Coumarins are a group of plant natural products obtained from the phenylpropanoid pathway, found in a wide range of plant species in nature, and are classified into four main groups (Hawryl et al., 2000; Lin et al., 2013). The biological activities of coumarins have been found to include anticancer, anti-inflammatory, antiviral, antimicrobial, antiasthmatic, antioxidant, antinociceptive, antidiabetic, and antidepressant effects (Nawrot-Modranka et al., 2006; Fylaktakidou et al., 2008; Smyth et al., 2009; Hassan et al., 2016). Some studies also indicated that coumarins exhibited potent AChE inhibitory activity. For instance, decursinol (Figure 1) and scopoletin (Figure 1) were reported to exhibit the most potent AChE inhibition (Kang et al., 2001; Rollinger et al., 2004). Youkwan et al. found that 6′-hydroxy-7′-methoxybergamottin (Figure 1) exhibited anti-AChE activity with IC50 values of 11.2 μM (Youkwan et al., 2010). Thus, scientists increasingly seek to explore the coumarin template for synthesizing novel AChE inhibitors.
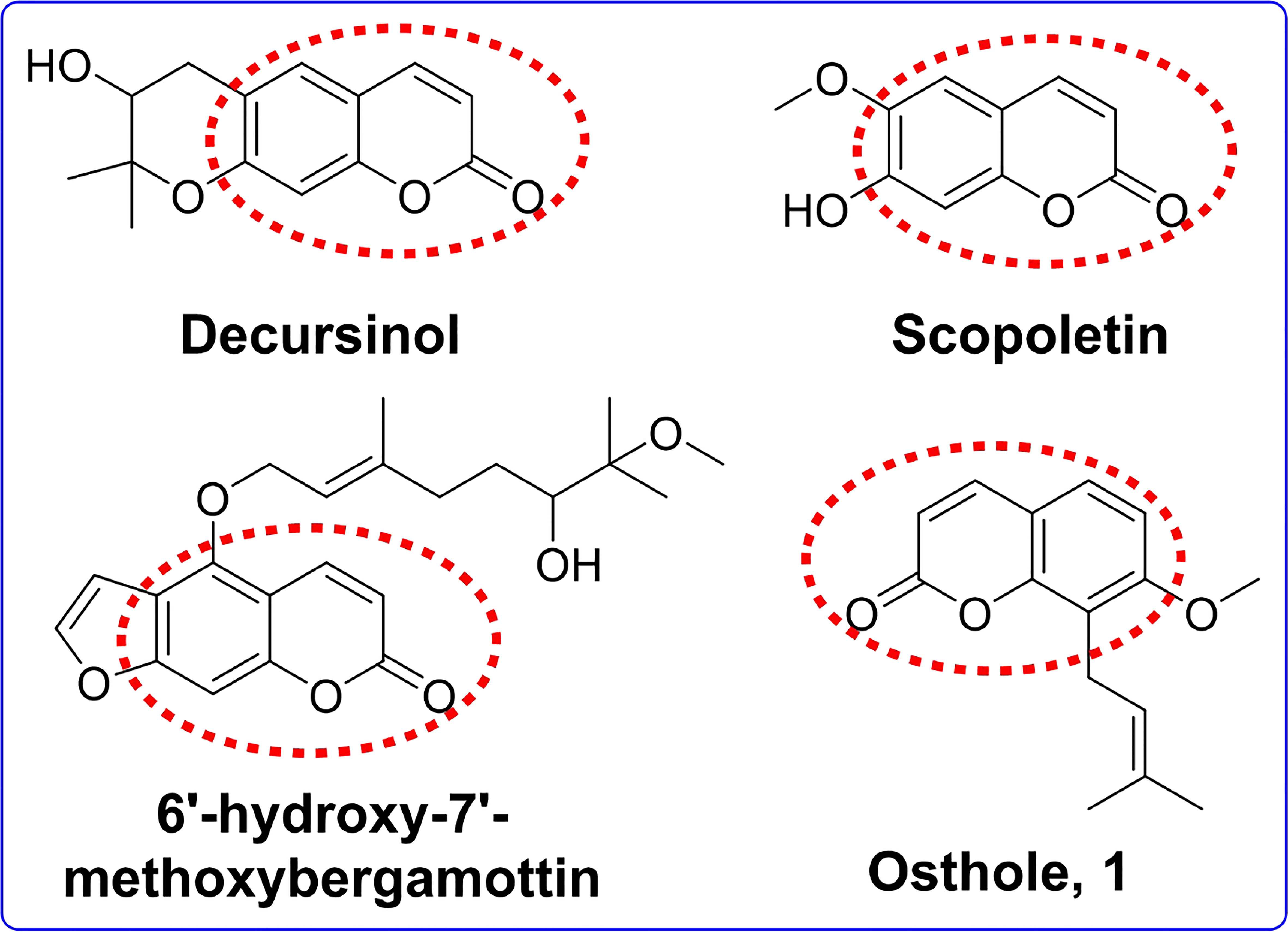
Figure 1 The chemical structures of osthole and several potent acetylcholinesterase inhibitors with coumarin moiety.
Osthole (1, Figure 1), mainly isolated from Cnidium monnieri (L.) Cusson and other forest plant species, is a natural coumarin compound and has extensive pharmacological features, such as anticancer, anti-inflammatory, and neuroprotective activities (Liu et al., 2015; Zhang et al., 2015; Fan et al., 2019; Tang et al., 2020; Bae et al., 2021; Sun et al., 2021). Researchers have found that osthole suppressed inflammation and apoptosis in mouse models of stab wound injuries, thus reducing secondary brain damage, enhancing the memory and learning functions in mechanical brain injury mice, and increasing the number of neurons in the affected brain regions (Xia et al., 2015; Yan et al., 2018). These all show that osthole is a promising skeleton for developing anti-Alzheimer’s drugs. However, few reports are related to the anti-AChE activity of osthole. Following the abovementioned interesting results, and as part of our ongoing search for new potential natural-product-based AChE inhibitors (Yu et al., 2021), in this paper, as part of our study, we prepared a series of osthole-based ester derivatives, measured the anti-AChE activity by Ellman’s method, and explored possible mechanisms of action using molecular modeling. On the other hand, we also used network pharmacology to screen other potential targets of derivatives in AD and molecular mechanisms.
Results and discussion
Chemical synthesis
The synthesis of osthole-based ester derivatives was performed as illustrated in Figure 2 via our previously reported methods. Firstly, oxidation of osthol (1) with SeO2 obtained 3′-formaldehydylosthole (2) in 46% (Yu et al., 2016). After being reduced by NaBH4 at 0° C, compound 2 yielded 4′-hydroxyosthole (3) (Yu et al., 2015). Finally, a series of osthole esters derivatives (4a–4r) were converted in 50%–69% yields by esterification of intermediate 3 with various carboxylic acids using dicyclohexylcarbodiimide (DCC) and 4-dimethylaminopyridine (DMAP) (Yu et al., 2015). They were characterized using 1H NMR, 13C NMR, and HRMS, and exemplary data are listed in Supplementary Data.
Anti-AChE activity in vitro
A preliminary bioassay of these derivatives’ activities inhibiting AChE was performed in vitro using the Ellman method at 0.01, 0.1, and 1 μmol/ml, respectively. As shown in Table 1, most of the target compounds had better inhibitory activities against AChE than raw material 1 at 1 μmol/ml; especially 4e, 4m, and 4o had significant inhibitory effects with inhibitory rates exceeding 50% but did not surpass tacrine. Among them, the most active of these was 4m, which showed an inhibitory rate of 68.8%, followed by 4o, which showed a rate of 62.6%. On the other hand, a structure–activity relationship for these osthole-based esters was also examined. Compounds 4m and 4o, which had superior inhibitory effects on AChE, contained aromatic heterocycles and suggested that the anti-AChE activity might be enhanced by the introduction of aromatic heterocycles compared with compound 1. Our previous research also showed that the introduction of heterocycles in coumarins could improve biological activity (Yu et al., 2021). On the contrary, target compounds with alkyl groups showed lower inhibitory activity at 1 μmol/ml; it showed that alkyl groups did not significantly increase activity when introduced (e.g., 19.4% for 4a, 22.3% for 4b>, 20.2% for 4a).
Meanwhile, compared with the inhibitory rates of 4m and 4o at different concentrations, we found that the inhibitory rates increased in a linear manner with an increase in compound concentration. It indicated that there was a positive correlation between inhibitory activities and concentration.
Molecular docking results
In our previous study, we found that coumarin could conjugate with the amino acid residues of acetylcholinesterase, thus showing a certain inhibitory activity (Yu et al., 2021). To explore the possible inhibition mechanism of the potent compound, molecular modeling studies were also performed on compounds 4m and 4o in the active site of AChE. AChE’s 3D structure was selected for docking studies from the RCSB database (PDB code: 3DHP). In this study, compounds 4m, 4o and AChE binding energies were -11.5 and -10.0 kcal/mol, respectively, which indicated that 4m and 4o had better binding activity with core targets. As illustrated in Figure 3, AChE’s active channel substrate (combinations 4m in Figure 3A and 4o in Figure 3B) contains the coumarin portion, whereas its channel entrance contains the aromatic heterocycles. In parallel, the conjugated aromatic ring of coumarin made a π–π interaction with the Trp86 residue to locate the coumarin core in AChE’s active site. In addition, the aromatic heterocycles were bonded to the Trp286 residue of the channel entrance via the π–π interaction. Acetylcholine could not enter the catalytic center of AChE since 4m and 4o occupied the catalytic site. In combination with the biological assay results, this molecular docking result suggested that compounds 4m and 4o might inhibit AChE.
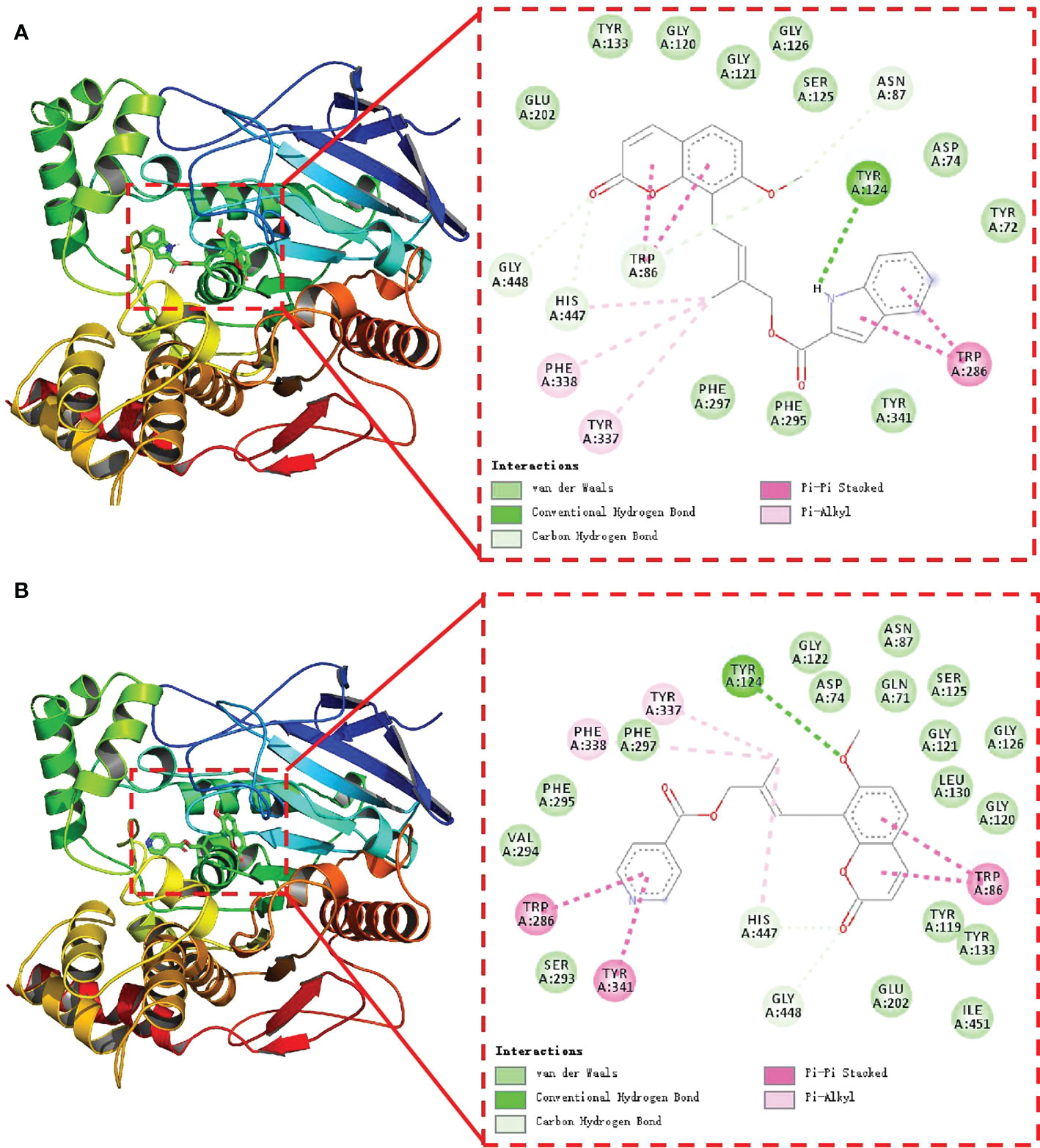
Figure 3 Docking pose of compound 4m (A) and 4o (B) inside AChE (the green dotted lines show the hydrogen bonds, the pink full lines show the π–π interactions.).
Analysis of network pharmacology
By searching the public databases (PubChem, SwissTargetPrediction, PharmMapper, SEA, GEO, GeneCard, OMIM), confining the result to “Homo sapiens,” 241 targets related to compound 4m and 2617 AD targets were collected, respectively. By using R software, the intersection of 4m targets and AD disease targets was calculated, and a Venn diagram was drawn to obtain 115 intersection targets (Figure 4). After that, PPI networks were constructed using target proteins and their corresponding ingredients in the STRING database (http://string-db.org/), and high confidence of protein interaction data with a score >0.7 was selected. By removing free proteins that do not interact, 4m and AD share 98 proteins.
Based on the protein–protein interaction networks, 98 proteins and 330 interactions were identified as potential interactions between compound 4m and AD (Figure 5).
GO enrichment analysis and KEGG pathway enrichment analysis were performed to elucidate the functions and enriched pathways of compound 4m’s potential anti-AD genes. As a result of the GO analysis, 2,151 statistically significant terms were obtained with 1,896 of biological processes (BP), 96 of cellular components (CC), and 159 of molecular functions (MF), according to p < 0.05. As shown in Figure 6A, the bar plot diagram displayed top eight significant enrichment terms of BP, CC, and MF with the highest gene counts, and redder dots indicated a lower q value and greater GO term enrichment. The results showed that compound 4m’s targets in treating AD were mostly enriched by positive regulation of kinase activity, positive regulation of MAPK cascade, rhythmic process, and other biological processes; in membrane raft, membrane microdomain, ficolin-1-rich granule lumen, and other cellular components; and in transmembrane receptor protein kinase activity, transmembrane receptor protein tyrosine kinase activity, protein tyrosine kinase activity, and other molecular functions. In order to explore the functions and signaling pathways of 4m’s identified anti-AD targets, KEGG pathways were applied. As a result, 151 signaling pathways related to 4m-AD were statistically significant, including Ras signaling pathway, MAPK signaling pathway, and Pap1 signaling pathway. An illustrated bubble diagram displayed the top 20 pathways showing significant enrichment potential with the highest number of genes (Figure 6B).
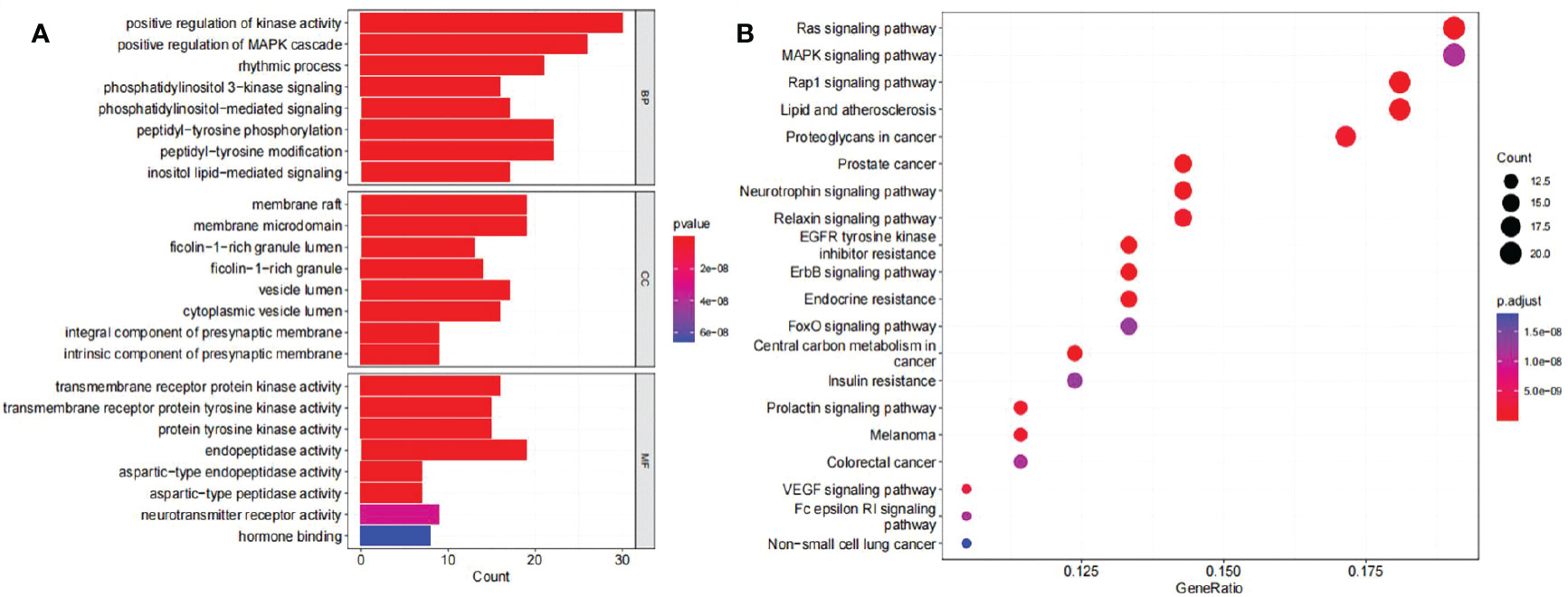
Figure 6 Analysis of potential targets of 4m for AD treatment based on GO and KEGG enrichment. (A) GO enrichment analysis identified genes involved in GO-BP analysis, GO-CC analysis, GO-MF analysis; (B) KEGG pathway analyses from bioinformatics data for the molecular signal pathway).
In addition, a constituent–target–pathway network containing 77 nodes and 348 edges was constructed to examine the interrelationships between ingredients, targets, and the top 20 pathways (Figure 7). Among the top three pathway counts, we found Ras signaling pathway, MAPK signaling pathway, and Pap1 signaling pathway, which may be responsible for the anti-AD effect of 4m. The Ras signaling pathway, in which it was proved that Ras farnesylation was significantly higher than in the elderly with non-cognitive disorders in the brain of AD patients (Dineley et al., 2001), contributed to the most genes and might be the most important 4m-AD pathway (Figure 8).
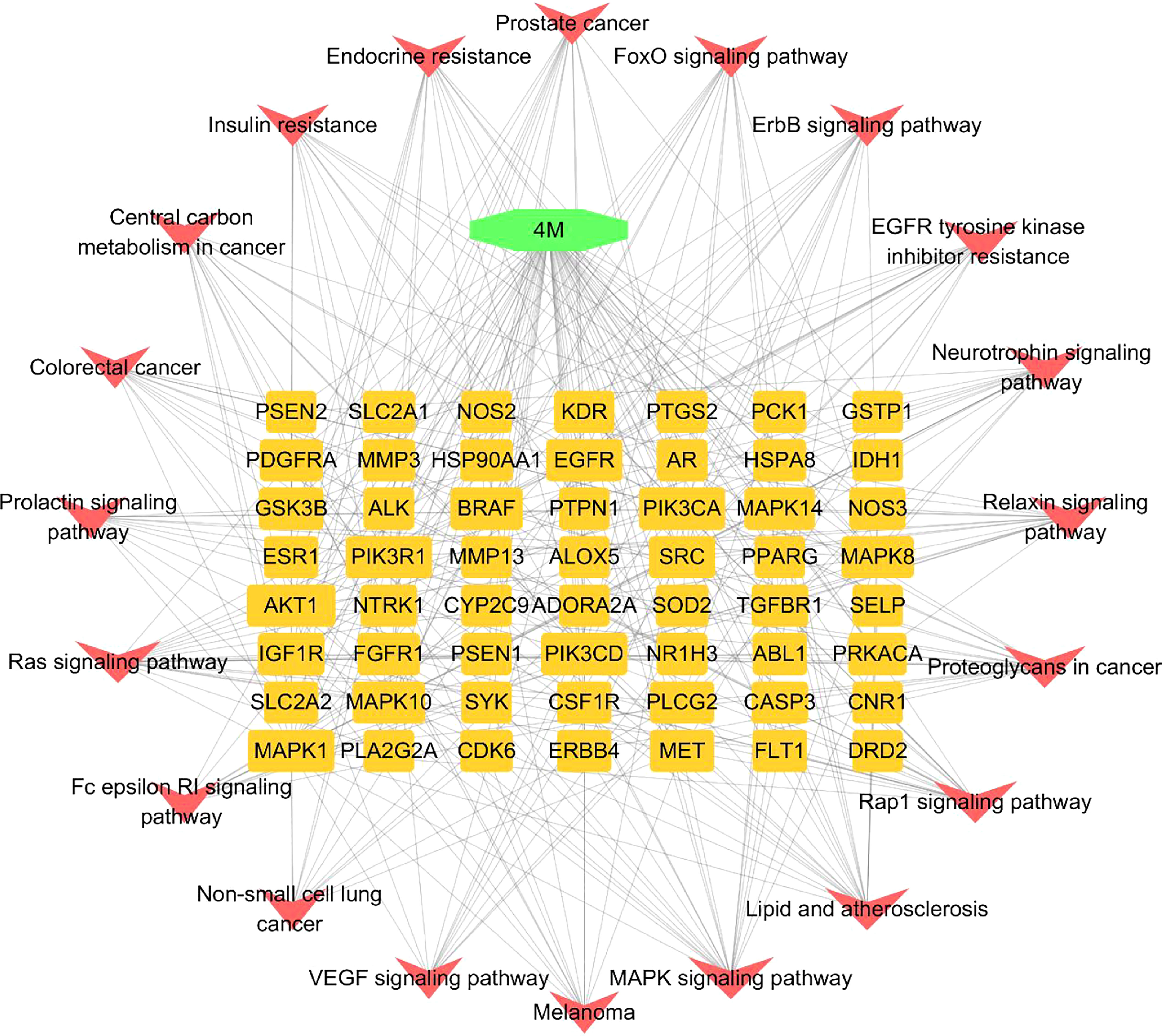
Figure 7 Constituent–target–pathway network of top 20 pathways. (The nodes in green stands for compound 4m. Each yellow oblong on the inner circle stands targets. Each red V node stands for each pathway.).
Finally, molecular docking was conducted between compound 4m and the five key targets (AKT1, PIK3CD, PIK3CA, PIK3R1MAPK1). As shown in Figure 9, it was found that compound 4m bound to target proteins with binding energies lower than -7 kcal/mol, suggesting that 4m inhibited the docking pocket from binding to the target receptor, making it an effective treatment for Alzheimer’s disease.
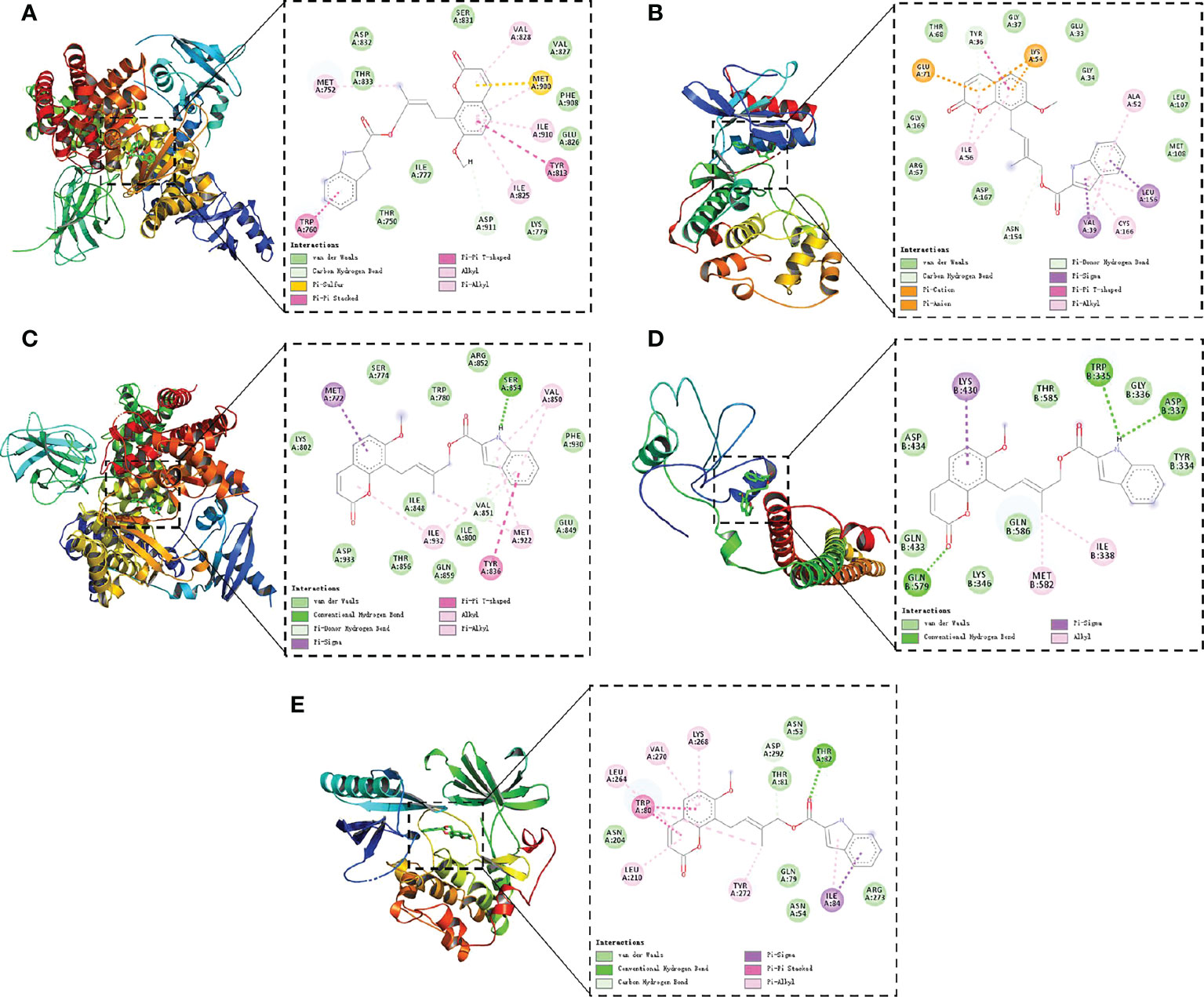
Figure 9 Molecular models of 4m binding to AKT1 (A), PIK3CD (B), PIK3CA (C), PIK3R1 (D), and MAPK1 (E). The green dotted lines show the hydrogen bonds; the pink full lines show the π–π interactions.).
Conclusion
In the present study, we designed, synthesized, and evaluated 18 derivatives of the osthole ester derivative for their in vitro inhibitory activity against AChE. The derivatives 4m and 4o showed moderate inhibitory activities, which were positively correlated with concentrations. Molecular docking results further revealed compounds 4m and 4o could bind to AChE through hydrogen bonds and hydrophobic contact. Network pharmacology also predicted that compound 4m could be involved in the Ras signaling pathway, which made it a potential therapeutic target of AD.
Materials and methods
Materials and Methods see Supplementary Data.
Data availability statement
The original contributions presented in the study are included in the article/Supplementary Material. Further inquiries can be directed to the corresponding authors.
Author contributions
XY, YC, and WY conceived and designed the experiments. XY performed the synthetic experiments, and wrote the manuscript. MZ performed the bioassays. YZ analyzed the data. YZ, YC, and WY revised the manuscript. All authors contributed to the article and approved the submitted version.
Funding
Our work was supported by the Natural Science Foundation of Guizhou Province [QKH-J(2020)1Y070], the Natural Science Foundation of China (No. 82260833), and the Youth Talent Development Project of Education Department of Guizhou Province [2018]209.
Conflict of interest
The authors declare that the research was conducted in the absence of any commercial or financial relationships that could be construed as a potential conflict of interest.
Publisher’s note
All claims expressed in this article are solely those of the authors and do not necessarily represent those of their affiliated organizations, or those of the publisher, the editors and the reviewers. Any product that may be evaluated in this article, or claim that may be made by its manufacturer, is not guaranteed or endorsed by the publisher.
Supplementary material
The Supplementary Material for this article can be found online at: https://www.frontiersin.org/articles/10.3389/fpls.2022.1054650/full#supplementary-material
References
Aranda-Abreu, G. E., Hernández-Aguilar, M. E., Denes, J. M., Hernández, L. I. G., Rivero, M. H. (2011). Rehabilitating a brain with alzheimer’s: A proposal. Clin. Interv. Aging. 6, 53–59. doi: 10.2147/CIA.S14008
Bae, H., Lee, J. Y., Song, J., Song, G., Lim, W. (2021). Osthole interacts with an ER-mitochondria axis and facilitates tumor suppression in ovarian cancer. J. Cell Physiol. 236, 1025–1042. doi: 10.1002/jcp.29913
Baharloo, F., Moslemin, M. H., Nadri, H., Asadipour, A., Mahdavi, M., Firoozpour, L., et al. (2015). Benzofuran-derived benzylpyridinium bromides as potent acetylcholinesterase inhibitors. Eur. J. Med. Chem. 93, 196–201. doi: 10.1016/j.ejmech.2015.02.009
Bartus, R. T., Dean, L. D., Beer, B., Lippa, A. S. (1982). The cholinergic hypothesis of geriatric memory dysfunction. Science 217, 408–414. doi: 10.1126/science.7046051
Bertram, L., Tanz, R. E. (2020). Genomic mechanisms in alzheimer’s disease. Brain Pathol. 30, 966–977. doi: 10.1111/bpa.12882
Bourne, Y., Taylor, P., Radic, Z., Marchot, P. (2003). Structural insights into ligand interactions at the acetylcholinesterase peripheral anionic site. EMBO J. 22, 1–12. doi: 10.1093/emboj/cdg005
Costantino, H. R., Leonard, A. K., Brandt, G., Johnson, P. H., Quay, S. C. (2008). Intranasal administration of acetylcholinesterase inhibitors. BMC Neurosci. 3, S6. doi: 10.1186/1471-2202-9-S3-S6
De Ferrari, G. V., Canales, M. A., Shin, I., Weiner, L. M., Silman, I., Inestrosa, N. C. (2001). A structural motif of acetylcholinesterase that promotes amyloid beta-peptide fibril formation. Biochem. 40, 10447–10457. doi: 10.1021/bi0101392
Dineley, K. T., Westerman, M., Bui, D., Bell, K., Ashe, K. H., Sweatt, J. D. (2001). Beta-amyloid activates the mitogen-activated protein kinase cascade via hippocampal alpha7 nicotinic acetylcholine receptors: In vitro and in vivo mechanisms related to alzheimer’s disease. J. Neurosci. 21, 4125–4133. doi: 10.1523/JNEUROSCI.21-12-04125.2001
Dunnett, S. B., Fobiger, H. C. (1993). Role of forebrain cholinergic systems in learning and memory: relevance to the cognitive deficits of aging and alzheimer’s dementia. Prog. Brain Res. 98, 413–420. doi: 10.1016/s0079-6123(08)62425-5
Fan, H. Y., Gao, Z. F., Ji, K., Li, X., Wu, J. B., Liu, Y., et al. (2019). The in vitro and in vivo antiinflammatory effect of osthole, the major natural coumarin from Cnidium monnieri (L.) cuss, via the blocking of the activation of the NF-κB and MAPK/p38 pathways. Phytomedicine 58, 152864. doi: 10.1016/j.phymed.2019.152864
Fylaktakidou, K. C., Hadjipavlou-Litina, D. J., Litinas, K. E., Varella, E. A., Nicolaides, D. N. (2008). Recent developments in the chemistry and in the biological applications of amidoximes. Curr. Pharm. Des. 14, 1001–1047. doi: 10.2174/138161208784139675
Hansen, R., Gartlehner, G., Webb, A. P., Morgan, L. C., Moore, C. G., Jonas, D. E. (2008). Efficacy and safety of donepezil, galantamine, and rivastigmine for the treatment of alzheimer’s disease: A systematic review and meta-analysis. Clin. Interv. Aging. 3, 211–225.
Hardy, J., Selkoe, D. J. (2002). The amyloid hypothesis of alzheimer’s disease: Progress and problems on the road to therapeutics. Science. 297, 353–356. doi: 10.1126/science.1072994
Hassan, M. Z., Osman, H., Ali, M. A., Ahsan, M. J. (2016). Therapeutic potential of coumarins as antiviral agents. Eur. J. Med. Chem. 123, 236–255. doi: 10.1016/j.ejmech.2016.07.056
Hawryl, M. A., Soczewinski, E., Dzido, T. H. (2000). Separation of coumarins from archangelica officinalis in highperformance liquid chromatography and thin-layer chromatography systems. J. Chromatogr. A. 886, 75–81. doi: 10.1016/S0021-9673(00)00321-6
Inestrosa, N. C., Alvarez, A., Perez, C. A., Moreno, R. D., Vicente, M., Linker, C. (1996). Acetylcholinesterase accelerates assembly of amyloid-beta-peptides into alzheimer’s fibrils: Possible role of the peripheral site of the enzyme. Neuron. 16, 881–891. doi: 10.1016/s0896-6273(00)80108-7
Jia, J. Y., Zhao, Q. H., Liu, Y., Gui, Y. Z., Liu, G. Y., Zhu, D. Y., et al. (2013). Phase I study on the pharmacokinetics and tolerance of ZT-1, a prodrug of huperzine a, for the treatment of alzheimer’s disease. Acta Pharmacol. Sin. 34, 976–982. doi: 10.1038/aps.2013.7
Kang, S. Y., Lee, K. Y., Sung, S. H., Park, M. J., Kim, Y. C. (2001). Coumarins isolated from angelica gigas inhibit acetylcholinesterase: Structure-activity relationships. J. Nat. Prod. 64, 683–685. doi: 10.1021/np000441w
Lane, C. A., Hardy, J., Schott, J. M. (2018). Alzheimer’s disease. Eur. J. Neurol. 25, 59–70. doi: 10.1111/ene.13439
Lin, Y. H., Sun, X. X., Yuan, Q. P., Yan, Y. J. (2013). Combinatorial biosynthesis of plant-specific coumarins in bacteria. Metab. Eng. 18, 69–77. doi: 10.1016/j.ymben.2013.04.004
Liu, M., Liu, Y., Hua, X. W., Wu, C. C., Zhou, S., Wang, B. L., et al. (2015). Synthesis of osthole derivatives with grignard reagents and their larvicidal activities on mosquitoes. Chin. J. Chem. 33, 1353–1358. doi: 10.1002/cjoc.201500620
Nawrot-Modranka, J., Nawrot, E., Graczyk, J. (2006). In vivo antitumor, in vitro antibacterial activity and alkylating properties of phosphorohydrazine derivatives of coumarin and chromone. Eur. J. Med. Chem. 41, 1301–1309. doi: 10.1016/j.ejmech.2006.06.004
Nazir, N., Karim, N., Abdel-Halim, H., Khan, I., Wadood, S. F., Nisar, M. (2018). Phytochemical analysis, molecular docking and antiamnesic effects of methanolic extract of Silybum marianum (L.) gaertn seeds in scopolamine induced memory impairment in mice. Biomed. Pharmacother. 210, 198–208. doi: 10.1016/j.jep.2017.08.026
Penumala, M., Zinka, R. B., Shaik, J. B., Mallepalli, S. K. R., Vadde, R., Amooru, D. G. (2018). Phytochemical profiling and in vitro screening for anticholinesterase, antioxidant, antiglucosidase and neuroprotective effect of three traditional medicinal plants for alzheimer’s disease and diabetes mellitus dual therapy. BMC Complem. Altern. M. 18, 77. doi: 10.1186/s12906-018-2140-x
Roberson, M. R., Harrell, L. E. (1997). Cholinergic activity and amyloid precursor protein metabolism. Brain Res. Rev. 25, 50–69. doi: 10.1016/s0165-0173(97)00016-7
Rollinger, J. M., Hornick, A., Langer, T., Stuppner, H., Prast, H. (2004). Acetylcholinesterase inhibitory activity of scopolin and scopoletin discovered by virtual screening of natural products. J. Med. Chem. 47, 6248–6254. doi: 10.1021/jm049655r
Schneider, L. S. (2000). A critical review of cholinesterase inhibitors as a treatment modality in alzheimer’s disease. Dialogues Clin. Neurosci. 2, 111–128. doi: 10.31887/DCNS.2000.2.2/lschneider
Shah, S., Reichman, W. E. (2006). Treatment of alzheimer’s disease across the spectrum of severity. Clin. Interv. Aging. 1, 131–142. doi: 10.2147/ciia.2006.1.2.131
Silman, I., Sussman, J. L. (2005). Acetylcholinesterase: ‘classical’ and ‘non-classical’ functions and pharmacology. Curr. Opin. Pharmacol. 5, 293–302. doi: 10.1016/j.coph.2005.01.014
Smyth, T., Ramachandran, V. N., Smyth, W. F. (2009). A study of antimicrobial activity of naturally occurring and synthetic coumarins. Int. J. Antimicrob. Ag. 33, 421–426. doi: 10.1016/j.ijantimicag.2008.10.022
Sun, M. N., Sun, M. J., Zhang, J. Y. (2021). Osthole: an overview of its sources, biological activities, and modification development. Med. Chem. Res. 30, 1767–1794. doi: 10.1007/s00044-021-02775-w
Tang, H. Q., Li, K. M., Dou, X. B., Zhao, Y. F., Huang, C. C., Shu, F. Y. (2020). The neuroprotective effect of osthole against chronic sleep deprivation(CSD)-induced memory impairment in rats. Life Sci. 263, 118524. doi: 10.1016/j.lfs.2020.118524
Xia, Y., Kong, L., Yao, Y. J., Jiao, Y. N., Song, J., Tao, Z. Y., et al. (2015). Osthole confers neuroprotection against cortical stab wound injury and attenuates secondary brain injury. J. Neuroinflflammation. 12, 155. doi: 10.1186/s12974-015-0373-x
Yan, Y. H., Kong, L., Xia, Y., Liang, W. B., Wang, L. T., Song, J., et al. (2018). Osthole promotes endogenous neural stem cell proliferation and improved neurological function through notch signaling pathway in mice acute mechanical brain injury. Brain Behav. Immun. 67, 118–129. doi: 10.1016/j.bbi.2017.08.011
Youkwan, J., Sutthivaiyakit, S., Sutthivaiyakit, P. (2010). Citrusosides a-d and furanocoumarins with cholinesterase inhibitory activity from the fruit peels of citrus hystrix. J. Nat. Prod. 73, 1879–1883. doi: 10.1021/np100531x
Yu, X., Ding, G. D., Zhi, X. Y., Xu, H. (2015). Insight into reduction of obacunone, and their ester derivatives as insecticidal agents against mythimna separata walker. Bioorg. Med. Chem. Lett. 25, 25–29. doi: 10.1016/j.bmcl.2014.11.027
Yu, X., Feng, G., Huang, J. L., Xu, H. (2016). Evaluation of some quinoline-based hydrazone derivatives as insecticidal agents. RSC Adv. 6, 30405–30411. doi: 10.1039/C6RA00993J
Yu, X., Zhao, Y. F., Huang, G. J., Chen, Y. F. (2021). Design and synthesis of 7-diethylaminocoumarin-based 1,3,4-oxadiazole derivatives with anti-acetylcholinesterase activities. J. Asian Nat. Prod. Res. 23, 866–876. doi: 10.1080/10286020.2020.1803293
Keywords: osthole, structural modification, acetylcholinesterase inhibitor, molecular docking, network pharmacology
Citation: Yu X, Zhang Y, Zhang M, Chen Y and Yang W (2022) Natural products as sources of acetylcholinesterase inhibitors: Synthesis, biological activities, and molecular docking studies of osthole-based ester derivatives. Front. Plant Sci. 13:1054650. doi: 10.3389/fpls.2022.1054650
Received: 27 September 2022; Accepted: 31 October 2022;
Published: 18 November 2022.
Edited by:
Zhiping Che, Henan University of Science and Technology, ChinaReviewed by:
Chun Yang, Shanxi Agricultural University, ChinaZhenting Du, Northwest A&F University, China
Copyright © 2022 Yu, Zhang, Zhang, Chen and Yang. This is an open-access article distributed under the terms of the Creative Commons Attribution License (CC BY). The use, distribution or reproduction in other forums is permitted, provided the original author(s) and the copyright owner(s) are credited and that the original publication in this journal is cited, in accordance with accepted academic practice. No use, distribution or reproduction is permitted which does not comply with these terms.
*Correspondence: Yafang Chen, chenyafang@gzy.edu.cn; Wude Yang, ywd_680708@sina.com