- 1State Key Laboratory of Biocatalysis and Enzyme Engineering, Hubei Collaborative Innovation Center for Green Transformation of Bio-Resources, Hubei Key Laboratory of Industrial Biotechnology, School of Life Sciences, Hubei University, Wuhan, China
- 2Jiangsu Key Laboratory of Crop Genomics and Molecular Breeding/Key Laboratory of Plant Functional Genomics of the Ministry of Education, College of Agriculture, Yangzhou University, Yangzhou, China
- 3Co-Innovation Center for Modern Production Technology of Grain Crops of Jiangsu Province/Key Laboratory of Crop Genetics and Physiology of Jiangsu Province, Yangzhou University, Yangzhou, China
Ethyl methanesulfonate (EMS)-induced mutagenesis is a powerful tool to generate genetic resource for identifying untapped genes and characterizing the function of genes to understand the molecular basis of important agronomic traits. This review focuses on application of contemporary EMS mutagenesis in the field of plant development and abiotic stress tolerance research, with particular focuses on reviewing the mutation types, mutagenesis site, mutagen concentration, mutagenesis duration, the identification and characterization of mutations responsible for altered stress tolerance responses. The application of EMS mutation breeding combined with genetic engineering in the future plant breeding and fundamental research was also discussed. The collective information in this review will provide good insight on how EMS mutagenesis is efficiently applied to improve abiotic stress tolerance of crops with the utilization of Next-generation sequencing (NGS) for mutation identification.
1. Overview of EMS mutagenesis
Ethyl methanesulfonate (EMS) is one of the most common used alkylating agents that can induce chemical modification of nucleotides through the introducing active alkyl group, which creates base changes and nucleotide mutations (Sabetta et al., 2011; Arisha et al., 2014; Gillmor and Lukowitz, 2020). Modifications caused by alkylating agents include the N7 and O6 of guanine (G), N3 and N7 of adenine (A), N3, O2, and O4 of thymine (T), N3, O2, and N4 of cytosine (C), and the phosphonate backbone (Murray, 1987; Fu et al., 2012). The EMS primarily induces the changes in guanine (Boysen et al., 2009). Compared to cytosine, the O6-exhalation pair is more stable than the thymine pair, so during replication the O6-GC pair is regularly converted into an AT pair. A lot of studies for EMS in the genotoxic activity in vivo and in vitro provide clear evidence, that EMS is a carcinogenic chemical and it is necessary to be very careful when using it (Gocke et al., 2009).
In the vast majority of cases, the mutant phenotype is due to premature stop codons, splice site disruption, deleterious amino acid substitutions, and loss of gene function (Sabetta et al., 2011). Therefore, it is critical to ensure that a large quantity of mutant alleles are present in a small mutant population and that the requirements for efficient homogenization are adequately met.
EMS is a type of non-transgenic chemical mutagen, and EMS mutagenesis is an important way to obtain mutations and the discovery of new genes for plants. Special protocols have been established for many plant species (Unan et al., 2021). The first commercial rice varieties CL112 and CL141, which were M2 from Clearfield rice varieties AS350, promoted the imidazolinone (IMI) resistant commercial progress and has important significance for rice research (Sudianto et al., 2013). Treatment with EMS is inexpensive, easy to implement, and induces point mutations at high rates and with good agreement in most species with different genetic backgrounds (Gillmor and Lukowitz, 2020). Currently, EMS mutagenesis can achieve a large number of mutants in a short period of time, which can facilitate the study of stress tolerance in plants. Moreover, it is necessary to strengthen research of phenomics, transcriptomics, proteomics, metabolomics, and other methods in EMS mutant screening and gene function analysis. It is significant carrying out research on stress tolerance breeding from different directions, and tap the potential of EMS mutagenesis in abiotic stress tolerance research.
2. The application of EMS mutagenesis
When EMS mutates seeds or tissues, several key parameters need to be considered, such as the uniformity of the material to be mutated and the optimization of EMS dose. In a perfect world, mutagenic material should be homologous and isogenic would facilitate evaluation using molecular markers (Sabetta et al., 2011). The use of homogeneous materials reduces the ambiguity between phenotype and genotype. After mutagenizing a plant, the first generation of seeds can be selected and selfed in successive rounds to create a large near-isogenic homozygous seed bank (Till et al., 2003). Homozygote is difficult to achieve in obligate vegetative breeding species, such as bananas (Wang X et al., 2021). However, homogeneous populations with fixed heterozygote and common features in all plants can be produced by clonal reproduction.
The second key parameter and main troubleshooting area in mutagenesis experimentation is optimization of EMS dose. The accumulation of induced mutation densities must be balanced against the viability or fecundity of the material so as to be feasible under existing resource conditions. Unan et al. (2021) believe that rice seed pre-soaking for 12 hours, can effectively promote the mutagenic effect of EMS, and establish the standard of rice EMS mutagenesis. Additionally, the shelf life of EMS is critical. Once the mutagen is turned on, EMS can interact with the outside air, which may reduce its activity.
2.1. EMS is applied to plant research
EMS mutagenesis is suitable for most plants (Kim et al., 2006). For crops, vegetables, and fruits, the purpose of mutagenesis is to obtain varieties with excellent traits. By identifying the agronomic and quality traits of progeny, a population of progeny with the visible mutation type is obtained, followed by further gene function research. As another example, improving ornamental plant ornamental value and stress resistance is a primary purpose of mutagenesis research. For example, common ornamental plants such as Chrysanthemum, moss, and jasmine were applied mutagenesis to obtain new landscape plant varieties (Hossain et al., 2006).
EMS mutagenesis has been used for multi-omits combined analysis, and related genes are obtained by reverse genetics. Subsequently, gene-editing technology is used for research. Editing and recombining genes are the basis for an ideal breeding method, which will bring infinite possibilities and breakthrough developments for human beings in plant genetics. In recent years, these are the primary methods of forwarding genetics among model plants.
2.2. Various plant tissues could be used for mutagenesis
Mutagens can make abundant mutations in different organs of plants, such as seeds, bulbs, callus, pollen, etc. (Mba et al., 2010). Plants make different mutagenesis sites, which can be selected according to the needs of the experiment, and EMS can be used for mutagenesis.
Jankowicz-Cieslak et al. (2012) considered that seeds are the most common mutagenic plant materials, and there is also a wealth of data in other plant species on doses used to achieve high densities of induced mutations. In general, for plants propagated by seeds, their seeds are usually preferentially selected mutagenic material (Gottwald et al., 2009). At present, reports on seed mutations are multifarious, and the materials cover a wide range, including food crops, cash crops, vegetables, fruits, and flowers (Chen et al., 2021). During mutagenesis, seeds are soaked in the mutagenesis solution, and the mutagenesis of plants can be achieved by changing the concentration of EMS and the mutagenesis duration. Mutagenesis of plant seeds is relatively convenient and quick, and not only are the steps simple, the workload is small, and it is suitable for the experimental research that requires the mutagenesis of most plants. Of course, we prefer the method of seed mutagenesis, but some plants may not be able to use the traditional method of seed mutagenesis due to different experimental purposes. It is not easy to obtain homozygous offspring from seeds, and it needs to be screened by techniques such as hybridization. At this time, pollen, callus, spores, plant fragments, etc. can also be selected for mutagenesis, and the mutagenesis method is relatively complicated. Compared with EMS mutagenesis of seeds, EMS mutagenesis of plant pollen has a large workload and complex operation in the process of mutagenesis. A common method is in vivo mutagenesis, that is, mutagenesis is carried out in a pollen tube. Meanwhile, other mutagenesis sites are usually selected for ex vivo mutagenesis methods.
3. The principles, methods and process of EMS mutagenesis
3.1. The concentration of the chemical and the duration of mutation
There is a strict correlation between EMS concentration, mutagenesis duration and lethality. Singh and Sadhukhan (2019) used EMS to mutate three diverged genotypes of grasspea, viz Nirmal, Biol-212 and Berhampur local. The results showed that when the EMS concentration of Nirmal and Biol-212 was higher than 0.5% and that of Berhampur local was higher than 1%, it was found to be fatal (Singh and Sadhukhan, 2019). The most effective concentration of EMS was 0.5%. In addition, with the increase in EMS concentration, the mutagenic efficiency increased and the effectiveness decreased (Singh and Sadhukhan, 2019). Ghosh et al. (2020) treated terminal cuttings with 0.25%, 0.30%, 0.35% and 0.40% of EMS, respectively. They concluded that morphological as well as flowering parameters, namely flower bud length, plant height, leaf area, flower yield and number of primary branches, decreased with increasing mutagenic dose. The study by Roychowdhury and Tah (2011) showed that germination and survival rates were significantly reduced with increasing EMS levels. The tolerance of plants to EMS varies widely, for example, in Arabidopsis, a 0.25-0.5% EMS mutation rate is high, tomato farming populations are constructed by 0.7% and 1% EMS, while in cucumber, 1.5-2% EMS (Greene et al., 2003; Minoia et al., 2010). In common plants, the larger the seed, the higher the EMS concentration required, and the longer the mutagenesis duration necessary, in order to achieve a certain mutation effect.
Mutagenesis duration is also different for different plants. In general, the higher the mutagenesis concentration selected, the shorter the mutagenesis duration. Conversely, the longer the mutagenesis duration, the lower the mutagenesis concentration used. The mutagenesis concentration required for different mutagenesis positions is also different. The mutagenesis concentration of seed mutagenesis and stem node mutagenesis is relatively high, while that of pollen mutagenesis is relatively low. When using plant seeds for mutagenesis, rice was mutagenized with 0.7% EMS, while pepper was mutagenized with 0.02% EMS concentration (Dwinianti et al., 2019; Yan W et al., 2021). The choice of concentration may also be related to the size of the seeds. Mutagenesis may also be affected by the mutagenic environment, which has not been proven due to the uncertain outcome of mutagenesis. When experimenters conduct EMS mutagenesis, they generally refer to published data and make appropriate choices based on the actual situation, instead of blindly conducting experiments according to the published data. In general, 50% of the lethality can be a good mutagenesis effect (Ke et al., 2019). In fact, in order to pursue higher mutation efficiency, the lethality rate we choose generally reaches 80%. Although the specific operation process is different, the basic steps are almost the same, as shown in Figure 1. The EMS mutagenesis concentrations of common plants are shown in Table 1.
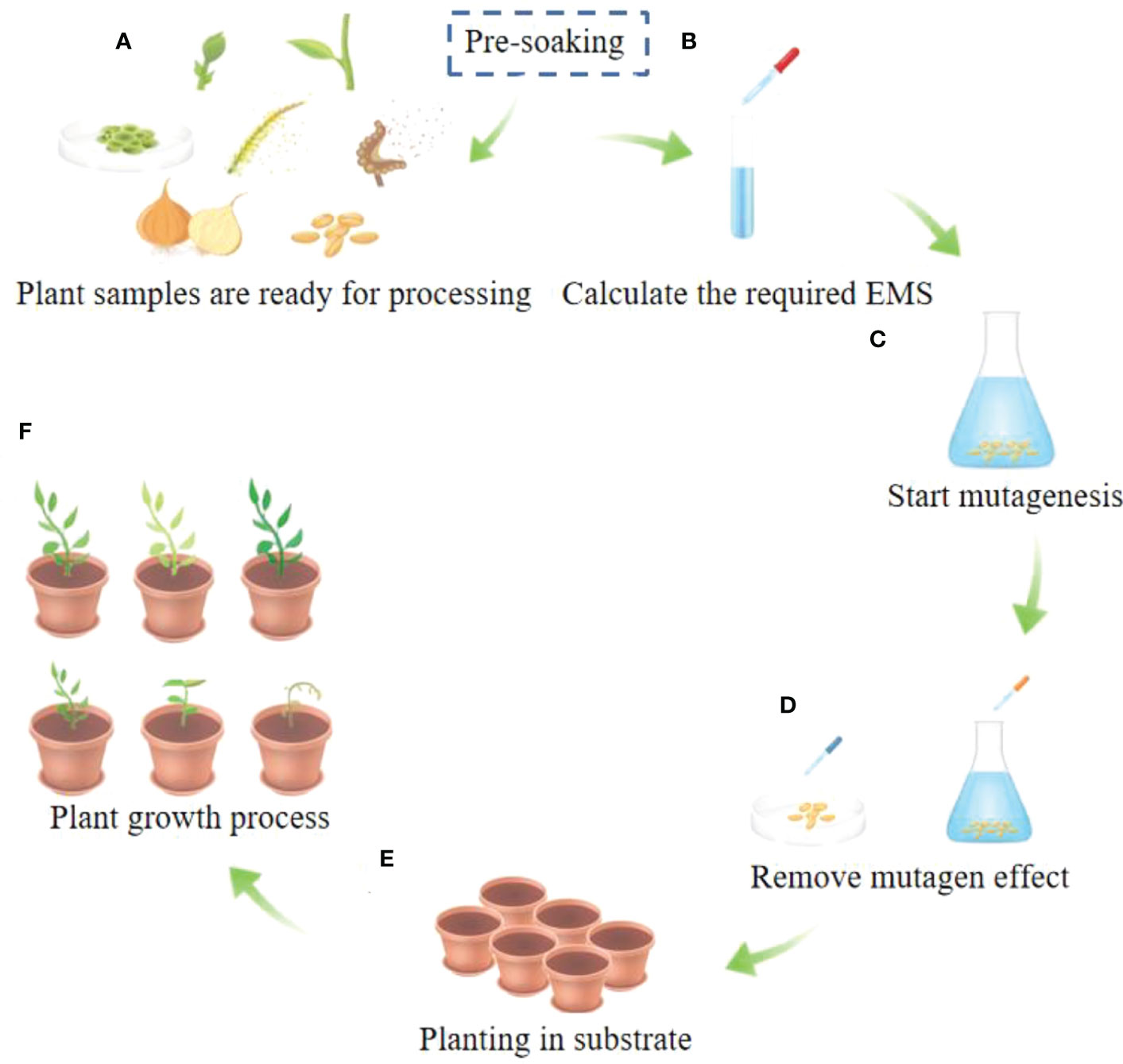
Figure 1 General steps of EMS mutagenesis. (A) Different prats of plants can be used for mutagenesis. The parts of the plant shown in A are buds, branches, callus, pollen, spores, bulbs, seeds and other parts of the plant tissue. (B) Determine the required EMS mutagenesis concentration and mutagenesis duration to profrom mutagenesis, and perform the mutagenesis. The preferred mutagenesis concentration range is 0.01%-4% and the duration range is 0.5h-12h. (C) Start mutagenesis with plant seeds as an example. (D) Rinse after mutagenesis or add mutagenesis terminator. (E) Planting in culture substrate. (F) After the seedlings grow, various phenotype can appear and carry out identification and further experiments.
3.2. Screening and analysis of the mutation lines
Chemical mutagenesis of seeds results in a long period of duration before full display. In experiments, identification of a population of plant mutants suitable for genotypic or phenotypic screening requires at least the production of M2 or later progeny. The duration required to generate a suitable mutagenize population depends on the choice of mutagenic material, and addressing the issue of heterozygote and homozygote is critical.
Plant materials must be non-chimeric before phenotypic or genotype screening, and the duration required varies depending on the species and tissue culture method used. While chimeras may disintegrate in meristem cells within a month of treatment, one or more rounds of post-EMS meristem dissociation and cutting may be required to ensure that all tissues in the resulting plant are genetically homogeneous (Jankowicz-Cieslak et al., 2012). In recent years, the EMS mutagenesis of some plants is shown in Table 1.
Mutagenesis using physical mutagens has been used to generate mutant populations in various plants (Stadler, 1929; Chawade et al., 2010). However, gamma irradiation and high-speed neutrons can lead to greater DNA inversion and deletion, which hinders the recognition of genes under the mutant phenotype (Espina et al., 2018). As an alternative, EMS is a chemical reagent commonly used to induce seed mutations. EMS induces high-frequency random point mutations, some of which can produce new termination codons in genes of interest (Jankowicz-Cieslak and Till, 2015).
EMS has been successfully applied to Solanaceae, resulting in morphological diversity that promotes improvements in fruit quality, yield, and desirable traits such as male sterility and disease resistance. The expected result of EMS treatment of seeds or vegetative tissues is that plant populations contain high-density induced point mutations. Due to the existence of homologous sequences, the density of polyploid plants is higher. As toddlers first observe phenotype, increased ploidy confers greater tolerance to mutations (Stadler, 1929). The mutation density of triploid banana is 1/57 KB, that of tetraploid wheat is 1/40 KB, and that of hexaploid wheat and oats is 1/24 KB (Slade et al., 2005; Chawade et al., 2010; Jankowicz-Cieslak et al., 2012). The evaluation of tillering mutants density usually involves screening a small number of target gene mutations in hundreds of plants. Alternatively, less representative genome sequencing of the full genome or fewer plants can be used to estimate mutation density (Henry et al., 2014). Stable phenotypic variation should be observed in the first non-chimeric generation (M2 for seed mutation) and can be used to determine whether the population is sufficiently mutated (Haughn and Somerville, 1987; Caldwell et al., 2004; Sabetta et al., 2011). The fertility of mutant populations should be reduced in early generations (Gottwald et al., 2009).
The traits mutated by EMS mainly include yield, quality, and resistance (Wang H et al., 2016). EMS has been successfully applied to a large number of plants to produce morphological diversity and promote the improvement of ideal traits (Lethin et al., 2020). Based on the mutation of agronomic and yield traits, new materials with excellent quality can be screened from the mutated offspring after multiple generations of screening and identification.
When a laboratory technician treated saffron with colchicine and EMS together, ALDH, BGL, and CCD2 gene expression increased by 2-fold (Samadi et al., 2022). Mutations of beneficial characteristics for wheat include dwarfing, early maturity, large grain, and more tillers (Ferrie et al., 2020). In the report of Kuraparthy et al. (2007) single tillering mutants were screened from the diploid wheat EMS mutant library by molecular technology, and the exact location of tiller-related genes on the chromosome was determined. Related experimenters identified a new type of rice precocious leaf 85 (psl85) mutant in the rice mutant population, showing an obvious premature senescence leaf phenotype and dwarfism, and the expression of senescence-related genes was up-regulated. Furthermore, genetic analysis showed that the senescent leaf phenotype is controlled by a single recessive nuclear gene, and further studies on senescence genes in rice were conducted (He et al., 2018). Yan W et al. (2021) randomly induced G/C to A/T conversion in rice genome by EMS. Whole-genome resequencing of 52 rice EMS mutants to investigate potential EMS mutational bias and its possible correlation with sequence background and chromatin structure.
After EMS mutagenesis treatment, experimenters observed a significant increase in the average number of shoots and roots of Hyoscyamus, and the EMS treatment also increased the accumulation of scopolamine and scopolamine in the explants compared to control (Shah et al., 2020).
After EMS, sodium azide (NaN3) or gamma-rays mutagenesis, chlorophyll-deficient mutants were most efficiently generated in EMS mutagenesis, three mutants were obtained from soybean seeds, and the progeny isolation of non-nodule mutant plants was completed (Carroll et al., 1986). The experimenter used EMS to mutagenize wheat cv. Gao 8901, and isolated the waxy mutant (Wx-null) by screening the progeny seeds with KI-I, and there was no significant difference in seed morphology and size compared with the wild type, but the mutants contained more B-type starch granules, while the amylose content was reduced (Pang et al., 2010). In the rape study, the Korean rape variety Tamla was used for EMS mutagenesis. The screened mutants were slightly smaller than the control in the early vegetative growth stage, but the oleic acid content in the mutants increased by about 7%. The mutants will be used for developed high oleic rapeseed varieties and conduct related research (Lee et al., 2018). Experimenters performed EMS mutagenesis on 5500 Gossypium herbaceum seeds, and about 5% of the progeny showed visible phenotype, such as leaf shape changes and sterility. The morphological and phenotypic evaluation of more than 4,000 plant materials of progeny was carried out, and the results showed significant differences (Kumar et al., 2022). Munda et al. (2022) treated seeds with different doses of EMS, induced morphological mutants of citronella, analyzed the morphological and physiological characteristics of the obtained mutants, increased herbage yield and citronellal content, and increased economic value. In the EMS mutagenesis of Brassica napus plants, a dwarf mutant bnd2 was successfully isolated. Compared with the wild type, the mutant has a shorter hypocotyl and a reduced plant height, and verified that bnd2 is a single-site recessive mutation that can be used research on the genetic mechanism of Brassica napus (Li et al., 2021). According to official data released by the FAO/IAEA project, 16 pepper varieties have been bred so far through mutation techniques (http://mvd.iaea.org). In pepper mutagenesis, several studies have used high-quality mutant populations obtained from EMS mutagenesis (Arisha et al., 2014). In particular, the EMS mutant population of sweet pepper variety ‘Maoer’ was established to study the genes regulating flower and plant structure (Siddiqe MI et al., 2020).
Nasri et al. (2022) mutated four Chrysanthemum varieties (“Homa”, “Fariba2”, “Arina”, and “Delkash”) with EMS (0-0.5%) as mutagens and leaf discs as explants to obtain new varieties. In addition, ISSR and IRAP molecular markers were used to detect the genetic polymorphism between the mutant and its parents. The ISSR and IRAP primers used can classify Chrysanthemum mutants according to varieties and some extent according to the EMS concentration used, to confirm their effectiveness in distinguishing real mutants, and to allow them to select in advance and reduce the size of the mutant population. In in vitro mutagenesis, EMS-induced mutations may be a useful tool to aid in breeding programs for new generation Chrysanthemum varieties.
4. Recent studies in plants using EMS mutagenesis
Plants are sessile, and they have to withstand various stress tolerance, which largely limit the way plants can survive, so it is crucial to improve their tolerance to stress tolerance. At present, research to screen resistant mutants by mutagenesis has attracted much attention, and stress-tolerant mutants have been successfully screened in many plants (Ge et al., 2018). Improving plant stress tolerance is a powerful measure to improve plant yield. Moreover, there are many studies on improving resistance through EMS mutagenesis (Thangwana et al., 2021; Li et al., 2022).
Salt tolerance has a great impact on plant yield traits, especially the genetic basis of salt tolerance (Costa and Farrant, 2019). However, in Arabidopsis, salt tolerant mutants caused by monocyte mutation have been previously reported, revealing the complexity of the genetic basis of plant salt tolerance. The results show that osmotic pressure, osmotic protectant, free radical detoxification, ion transport system, hormone level changes, and hormone-guided communication are all related to salt stress, which further affects plant yield traits (Quesada et al., 2000; Zhao et al., 2021). EMS induction of 275 mutants has been used in different levels of salinity response study, and research shows N22-L-1013, N22-L-806, and N22-L-1010 were identified as typical Na excluders (Shankar et al., 2021). After four generations of choice, and in the field experiment carried out, the EMS wheat mutants, showed good salt-tolerant phenotypes (Lethin et al., 2020). Hitomebore Salt Tolerant 1 (hst1) of rice mutants from the 6000 EMS by Takagi et al. (2015) in the mutant screening, and researchers verified the OsRR22 role in the process response to salt stress. Due to the complexity of plant salt-tolerant and limited experimental data from previous studies, the NaCl tolerance mechanism of the mutants needs to be further investigated. Salt-tolerant crops play an important role in the use of saline-alkali land for agricultural production (Van Zelm et al., 2020).
EMS-induced yellow seeded Brassica napus was used to analyze the drought tolerance of mutants at the bud stage and seedling stage, and successfully screened for mutants with strong drought resistance (Tang et al., 2020). Screening in mutant nightshade populations restored alleles with improved content comprised of phenolic compounds, namely anthocyanin and chlorogenic acid (CGA) (Xi-Ou et al., 2017). In this study, the author performed High Performance Liquid Chromatography (HPLC) to determine the concentration of the anthocyanin, and the results showed that the CGA content of mutant was significantly higher than that of WT. RT-PCR anlysis showed that levels of expression of anthocyanin biosynthetic genes were increased in S9-1, with the exception of SmPAL (Xi-Ou et al., 2017). After EMS mutagenesis, glyphosate-resistant mutant lines were selected from the M4 generation of soybean seeds with excellent agronomic characteristics. Wheat and Chickpea cicer arietinum L. Plants. beans also won the IMI mutants in this way (Chen et al., 2021; Galili et al., 2021).
In the process of mutant screening, some physiological indexes can be used as stress resistance indexes for resistance screening under different stress conditions (Friesen and Wall, 1986; Sebastian and Chaleff, 1987). Li et al. (2019) induced radish mutation with EMS, which is suitable for obtaining the target characteristics of new radish materials. DY13 radish seeds were treated with 0.5% EMS to produce sterile mutants. Morphological observation showed that the anthers of these male sterile lines were curled, browned and wrinkled, and their disease resistance and drought tolerance were enhanced. The male sterile line was successfully selected from DY13 radish, which provides a new and effective method for the selection of the radish male sterile line.
Munda et al. (2022) used an EMS chemical mutagen to induce the morphological mutant of citronella Indus. The mutant has high puncture strength and an enhanced anti-penetration ability due to a curved stem, which further proves the reliability of practical physiological index analysis of stress-resistant mutants. A large number of studies have shown that it is very reliable to screen the resistance of mutated plants according to physiological indexes, and this will speed up the process of plant stress resistance breeding.
In recent years, various attempts have been made to cultivate crops that are tolerant to herbicide doses, which are usually fatal to weeds (Prakash et al., 2020). Different scientific methods have been used to genetically modify crops to cultivate herbicide-tolerant/herbicide-resistant crops (Halford and Shewry, 2000). Herbicide-resistant mutant plants have been found in many crops, such as corn, wheat, cotton, sorghum, sunflower, lentil, and soybean (Anderson and Georgeson, 1989; Newhouse et al., 1991; Rajasekaran et al., 1996). Rizwan et al. (2017) developed an imidazolinone-resistant lentil mutant (RH44) through EMS mutation. In general, it is not easy to develop crops with high-dose herbicide resistance. Such herbicides can kill all types of weeds at one duration. However, to improve the resistance of crops to higher herbicide doses, new strategies are being adopted, such as large-scale screening of mutagenic populations, re-mutation of independently isolated mutations, screening, and gene polymerization, and selecting hypothetical herbicide-resistant mutants in different crops (Piquerez et al., 2014).
Among various mutagenic methods, chemical reagents that can produce genetic variation in crops have been widely popular for many years. A mutation strategy is considered to be an important step for producing new and valuable genetic variations (Wei et al., 2022. Many important crops, such as wheat, sesame, rice, grapefruit, cotton, and bananas, have produced mutant-derived varieties. In tomato, EMS-based mutations have been used to generate specific alleles such as a purple leaf color, smaller leaves, and early fruit (Silué et al., 2013). Through EMS mutagenesis, researchers have cultivated Targeting Induced Local Lesions IN Genomes (TILLING) populations containing a mutant phenotype of various quality traits in cucumber and pumpkin (Kami et al., 2000; Prakash et al., 2020). Some M1 plants showed chimerism caused by a recessive gene mutation. Some M1 plants showed variation in flower organs, delayed flowering and a pale leaf color at maturity. Fruit color and shape also changed in M1 plants, consistent with EMS-induced changes in tomato fruit (Gauffier et al., 2016). Because EMS can cause morphological variation in fruit, we believe that EMS mutation may have potential value in fruit quality (Bhat et al., 2017).
5. Molecular technology promotes application of EMS mutagenesis technology
In recent years, research on EMS mutagenesis has continued to develop, and the mutagenesis of some plants is shown in Table 1. EMS mutagenesis has been widely studied in mutant screening and mutant library construction. At present, some research progress has been made in Arabidopsis, rice, soybean, and other plants (DeMarini, 2020). Identifying mutants by plant phenotype is the most direct method, but this has significant limitations, and it is easy to miss important mutations. In addition, some researchers have used molecular markers to analyze the EMS mutants of wheat, and to screen mutants with different glutenin subunit deletions from a wheat EMS mutant library (Grover and Sharma, 2016). With the rapid development of molecular sequencing technology, research is also intensifying to identify mutants with TILLING technology (Manzanares et al., 2016; Taheri et al., 2017). Reverse genetics is a potentially important approach to identify new mutations in genes of interest. Reverse genetics can be applied to plant tillering, regardless of the level of plant genome structure (Kurowska et al., 2011). In contrast to other reverse genetics methods (such as RNAi technology) and T-DNA insertional mutagens, this non-transgenic method does not require transformation (Krysan et al., 1999; Travella et al., 2006). TILLING aims to find nucleotide changes caused by chemical mutagenesis in target genes, to enable changes in protein function (Till et al., 2004). The development of molecular technology will be beneficial to the identification of EMS mutagenesis mutants and promote the application of EMS mutagenesis technology.
EMS is the mutagen of choice, which induces single-nucleotide variation through the titillation of a specific nucleoside, resulting in a broad spectrum of mutations (Henikoff et al., 2004). This may be silencing, nonsense, missed, and splicing mutations in the gene coding region (Boualem et al., 2014). TILLING has been applied to different plants, such as Archbishops thali, rapeseed, soybean, rice, wheat, tomato, sunflower, and tobacco, indicating that this method is an important alternative method for plant species function analysis (Till et al., 2007; Reddy et al., 2012; Bovina et al., 2014; Yan Z et al., 2021). The mutant population induced by EMS was used to target induced local damage (TILLING) in the genome. Further screening and identification of EMS mutants were identified by the combination of morphological analysis and biotechnology, which will facilitate the screening of abiotic stress tolerance mutants, and the preliminary screening of mutants in a more efficient and targeted manner.
6. Conclusion
The development of EMS mutagenesis promoted the rapid development of plant functional genomics, resulting in the birth of map-based cloning technology and directed induced local mutation technology (Kim et al., 2006). The latter one effectively combines traditional chemical mutagenesis with mutant screening skills. EMS mutagenesis cause high throughput mutation with low cost, which tremendously speeds up the research for plant genomics and promotes the development of molecular breeding for abiotic stress tolerance plants.
Similar to other breeding methods, EMS mutation also has limitations, including a high level of randomness, low mutation efficiency, and few beneficial mutations. Additionally, it is still difficult to identity, clone and characterize mutations, resulting in limited application of this method in plant species with complicated or polyploid genomes. Although the latest gene editing technology, such as the CRISPR/Cas9 system, which is faster, more accurate, and is widely used in plant abiotic stress tolerance research by altering individual gene loci, it is difficult for genetic improvement of traits controlled by multiple sites. Moreover, the CRISPR/Cas9 system is difficult to apply to the species with little genomic information. On the contrary, EMS mutagenesis results mutation at multi-sites and can obtain abundant traits, which is difficult to be replaced by other mutagenesis breeding. It is also noteworthy that although the CRISPR/Cas9 technology has been widely used in gene editing, EMS mutagenesis is a non-GMO application, which could meet the strict laws for genetic modification of genes.
Author contributions
LC prepared an outline, first draft. LD, MS, ZY, KH, HL, HY, and LL provided literature support, and prepared the figure and table. HY and LL are corresponding to overall editing and finalization of paper drafts. All authors read and approved the published version of the manuscript.
Funding
This work was supported by the National Natural Science Foundation of China (31971410).
Acknowledgments
We would like to express our sincere thanks to everyone who assisted us with this paper.
Conflict of interest
The authors declare that the research was conducted in the absence of any commercial or financial relationships that could be construed as a potential conflict of interest.
Publisher’s note
All claims expressed in this article are solely those of the authors and do not necessarily represent those of their affiliated organizations, or those of the publisher, the editors and the reviewers. Any product that may be evaluated in this article, or claim that may be made by its manufacturer, is not guaranteed or endorsed by the publisher.
References
Anderson, P., Georgeson, M. (2011). Herbicide-tolerant mutants of corn. Genome 31 (2), 994–999. doi: 10.1139/g89-173
Arisha, M. H., Liang, B. K., Muhammad Shah, S. N., Gong, Z. H., Li, D. W. (2014). Kill curve analysis and response of first generation Capsicum annuum l. B12 cultivar to ethyl methane sulfonate. Genet. Mol. Res. 13 (4), 10049–10061. doi: 10.4238/2014.November.28.9
Ashrafi Parchin, R., Nasrollahnezhad Ghomi, A. A., Naghdi Badi, H., Navabpour, S., Mehrafarin, A., Eskandari, A. (2021). Investigation effect of ethyl methane sulfonate (EMS) on some of morphophysiological and phytochemical traits of fenugreek (Trigonella foenum-graecum l.). Ind. Crops 162, 113239. doi: 10.1016/j.indcrop.2021.113239
Awais, A., Nualsri, C., Soonsuwon, W. (2019). Induced mutagenesis for creating variability in thailand's upland rice (cv. Dawk pa-yawm and Dawk kha 50) using ethyl methane sulphonate (EMS). Sarhad J. Agric. 35 (1), 293–301. doi: 10.17582/journal.sja/2019/35.1.293.301
Bhat, S., Sharma, S., Sharma, V. K. (2017). Effect of EMS induced morphological mutation in strawberry. J. Hill Agric. 8 (1), 50–55. doi: 10.5958/2230-7338.2017.00020.9
Boualem, A., Fleurier, S., Troadec, C., Audigier, P., Kumar, A. P., Chatterjee, M., et al. (2014). Development of a Cucumis sativus TILLING platform for forward and reverse genetics. PloS One 9 (5), e97963. doi: 10.1371/journal.pone.0097963
Boysen, G., Pachkowski, B., Nakamura, J., Swenberg, J. (2009). The formation and biological significance of N7-guanine adducts. Mutat. Res. 678, 76–94. doi: 10.1016/j.mrgentox.2009.05.006
Caldwell, D. G., McCallum, N., Shaw, P., Muehlbauer, G. J., Marshall, D. F., Waugh, R., et al. (2004). A structured mutant population for forward and reverse genetics in barley (Hordeum vulgare L.). Plant J. 40 (1), 143–150. doi: 10.1111/j.1365-313X.2004.02190.x
Carroll, B. J., McNeil, D. L., Gresshoff, P. M. (1986). Mutagenesis of soybean (Glycine max (L.) merr.) and the isolation of non-nodulating mutants. Plant Sci. 47, 109–114. doi: 10.1016/0168-9452(86)90057-9
Chawade, A., Sikora, P., Bräutigam, M., Larsson, M., Vivekanand, V., Nakash, M. A, et al. (2010). Development and characterization of an oat TILLING-population and identification of mutations in lignin and β-glucan biosynthesis genes. BMC Plant Biol. 10, 86. doi: 10.1186/1471-2229-10-86
Chen, Z., Wang, Z., Heng, Y., Li, J., Pei, J., Cao, Y., et al. (2021). Generation of a series of mutant lines resistant to imidazolinone by screening an EMS-based mutant library in common wheat. Crop J. 9 (5), 1030–1038. doi: 10.1016/j.cj.2020.11.001
Costa, M.-C. D., Farrant, J. M. (2019). Plant resistance to abiotic stresses. Plants 8, 553. doi: 10.3390/plants8120553
DeMarini, D. M. (2020). The mutagenesis moonshot: The propitious beginnings of the environmental mutagenesis and genomics society. Environ. Mol. Mutag. 61 (1), 8–24. doi: 10.1002/em.22313
Deng, Y., Liu, S., Zhang, Y., Tan, J., Li, X., Chu, X., et al. (2022). A telomere-to-telomere gap-free reference genome of watermelon and its mutation library provide important resources for gene discovery and breeding. Mol. Plant 15 (8), 1268–1284. doi: 10.1016/j.molp.2022.06.010
Desai, S. P., Jadhav, A. G., Ramteke, A. R., Dhole, V. J., Bapat, V. A., and Gaikwad, N. J. A. R. J., et al. (2021). Differential effects of gamma rays, EMS, and SA on biological parameters of ajara ghansal a non-Basmati aromatic rice landrace from kolhapur, maharashtra.
Dong, H., Li, H., Xue, Y., Su, S., Li, S., Shan, X., et al. (2021). E183K mutation in chalcone synthase C2 causes protein aggregation and maize colorless. Front. Plant Sci. 12, 679654. doi: 10.3389/fpls.2021.679654
Dwinianti, E. F., Mastuti, R., Arumingtyas, E. L. J. J. o. T. L. S. (2019). Genetic variation analysis of ems-induced chili pepper (Capsicum frutescens l.) mutants using SSR markers. J. Trop. Life Sci. 9 (3), 223–228.
Espina, M. J., Ahmed, C. M.S., Bernardini, A., Adeleke, E., Yadegari, Z., Arelli, P., et al. (2018). Development and phenotypic screening of an ethyl methane sulfonate mutant population in soybean. Front. Plant Sci. 9, 394. doi: 10.3389/fpls.2018.00394
Ferrie, A. M. R., Bhowmik, P., Rajagopalan, N., Kagale, S., et al. (2020). “CRISPR/Cas9-mediated targeted mutagenesis in wheat doubled haploids,” in Cereal genomics (Springer), 183–198. doi: 10.1007/978-1-4939-9865-4_15
Fora, F., Shimelis, H., Olaolorun, B., Korir, H., Indetie, A., Mashilo, J., et al. (2021). Determining ethyl methane sulfonate-mediated (EMS) mutagenesis protocol for inducing high biomass yield in fodder barley ('Hordeum vulgare' l.). Aust. J. Crop Sci. 15 (7), 983–989. doi: 10.21475/ajcs.21.15.07.p2877
Friesen, G., Wall, D. A. (1986). Tolerance of lentil (Lens culinaris medik.) to herbicides. Can. J. Plant Sci. 66 (1), 131–139. doi: 10.4141/cjps86-017
Fu, D., Calvo, J. A., Samson, L. D. (2012). Balancing repair and tolerance of DNA damage caused by alkylating agents. Nat. Rev. Cancer. 12 (2), 104–120. doi: 10.1038/nrc3185
Galili, S., Hershenhorn, J., Edelman, M., Sobolev, V., Smirnov, E., Amir-Segev, O., et al. (2021). Novel mutation in the acetohydroxyacid synthase (AHAS), gene confers imidazolinone resistance in Chickpea cicer arietinum l. Plants. Plants 10 (12), 2791. doi: 10.3390/plants10122791
Gauffier, C., Lebaron, C., Moretti, A., Constant, C., Moquet, F., Bonnet, G., et al. (2016). A TILLING approach to generate broad-spectrum resistance to potyviruses in tomato is hampered by eIF4E gene redundancy. Plant J. 85 (6), 717–729. doi: 10.1111/tpj.13136
Gautam, V., Swaminathan, M., Akilan, M., Gurusamy, A., Suresh, M., Kaithamalai, B., et al. (2021). Early flowering, good grain quality mutants through gamma rays and EMS for enhancing per day productivity in rice (Oryza sativa L.). Int. J. Radiat. Biol. 97 (12), 1716–1730. doi: 10.1080/09553002.2021.1987563
Ge, F.-y., Zheng, N., Zhang, L.-p., Huang, W.-k., Peng, D.-l., Liu, S.-m, et al. (2018). Chemical mutagenesis and soybean mutants potential for identification of novel genes conferring resistance to soybean cyst nematode. J. Integr. Agric. 17 (12), 2734–2744. doi: 10.1016/S2095-3119(18)62105-7
Ghosh, S., Ganga, M., S K, S., Kumar, A., Kapoor, M., et al. (2020). Induction of mutation in Jasminum grandiflorum with gamma rays and EMS and identification of novel mutants using molecular markers and SEM imaging. Indian J. Hortic. 77 (4), 695–703. doi: 10.5958/0974-0112.2020.00101.2
Gillmor, C. S., Lukowitz, W. (2020). “EMS mutagenesis of arabidopsis seeds,” in Plant embryogenesis (Springer), 15–23. doi: 10.1007/978-1-0716-0342-0_2
Gocke, E., Bürgin, H., Müller, L., Pfister, T. (2009). Literature review on the genotoxicity, reproductive toxicity, and carcinogenicity of ethyl methanesulfonate. Toxicol. Lett. 190 (3), 254–265. doi: 10.1016/j.toxlet.2009.03.016
Gottwald, S., Bauer, P., Komatsuda, T., Lundqvist, U., Stein, N., et al. (2009). TILLING in the two-rowed barley cultivar 'Barke' reveals preferred sites of functional diversity in the gene HvHox1. BMC Res. Notes 2 (1), 1–14. doi: 10.1186/1756-0500-2-258
Greene, E. A., Codomo, C. A., Taylor, N. E., Henikoff, J. G., Till, B. J., Reynolds, S. H., et al. (2003). Spectrum of chemically induced mutations from a large-scale reverse-genetic screen in Arabidopsis. BMC Plant Biol. 164 (2), 731–740. doi: 10.1093/genetics/164.2.731
Grover, A., Sharma, P. c (2016). Development and use of molecular markers: past and present. Crit. Rev. Biotechnol. 36 (2), 290–302. doi: 10.3109/07388551.2014.959891
Habib, S. H., Akanda, M. A.L., Roy, P., Kausar, H., et al. (2021). Effect of different dosage of EMS on germination, survivability and morpho-physiological characteristics of sunflower seedling. Helia 44 (75), 167–180. doi: 10.1515/helia-2021-0008
Hadebe, S., Hadebe, S., Modi, A. T., Hugo, A., Shimelis, H. A, et al. (2019). Seed oil content and fatty acid composition response to ethyl methanesulphonate mutagenesis in vernonia. South Afr. J. Plant Soil 36 (5), 375–380. doi: 10.1080/02571862.2019.1631399
Halford, N. G., Shewry, P. R. (2000). Genetically modified crops: methodology, benefits, regulation and public concerns. Br. Med. Bull. 56 (1), 62–73. doi: 10.1258/0007142001902978
Haughn, G., Somerville, C. R. (1987). Selection for herbicide resistance at the whole-plant level (ACS Publications) American Chemical Society. 334 98–107.
He, Y., Zhang, Z., Li, L., Tang, S., Wu, J. L, et al. (2018). Genetic and physio-biochemical characterization of a novel premature senescence leaf mutant in rice (Oryza sativa l.). Int. J. Mol. Sci. 19 (8), 2339. doi: 10.3390/ijms19082339
Henikoff, S., Till, B. J., Comai, L. (2004). TILLING. traditional mutagenesis meets functional genomics. Plant Physiol. 135 (2), 630–636. doi: 10.1104/pp.104.041061
Henry, I. M., Nagalakshmi, U., Lieberman, M. C., Ngo, K. J., Krasileva, K. V., Vasquez-Gross, H., et al. (2014). Efficient genome-wide detection and cataloging of EMS-induced mutations using exome capture and next-generation sequencing. Plant Cell 26 (4), 1382–1397. doi: 10.1105/tpc.113.121590
Hossain, Z., Mandal, A. K., Datta, S. K., Biswas, A. K., et al. (2006). Development of NaCl-tolerant strain in Chrysanthemum morifolium ramat. through in vitro mutagenesis. Plant Biol. 8 (04), 450–461.
Jankowicz-Cieslak, J., Huynh, O. A., Brozynska, M., Nakitandwe, J., Till, B. J., et al. (2012). “Induction, rapid fixation and retention of mutations in vegetatively propagated banana,” in Advances in plant breeding strategies: breeding, biotechnology, vol. 10. , 1056–1066. doi: 10.1111/j.1467-7652.2012.00733.x
Jankowicz-Cieslak, J., Till, B. J. (2015). “Forward and reverse genetics in crop breeding,” in Al-Khayri, J. M., Jain, S. M., Johnson, D. V., et al (Eds.), Advances in plant breeding strategies: breeding, biotechnology molecular tools, 215–240. Cham: Springer International Publishing.
Jobson, E. M., Martin, J. M., Sharrock, R., Hogg, A. C., Giroux, M. J., et al. (2021). Identification and molecular characterization of novel Rht-1 alleles in hard red spring wheat. Crop Sci. 61 (2), 1030–1037. doi: 10.1002/csc2.20375
Kami, J., Gepts, P. (2000). Development of a BAC library in common bean genotype BAT93. Annu. Rep. Bean Improv. Coop. 43 (2), 208–209. doi: 10.1002/csc2.20375
Karaman, K., Kizil, S., Başak, M., Uzun, B., and Yol, E., et al. (2021). Development of EMS-induced mutagenized groundnut population and discovery of point mutations in the ahFAD2 and Ara h 1 genes by TILLING. J. Oleo Sci. 70 (11), 1631–1640. doi: 10.5650/jos.ess21075
Karunarathna, N. L., Patiranage, D. S.R., Harloff, H. J., Sashidhar, N., Jung, C., et al. (2021). Genomic background selection to reduce the mutation load after random mutagenesis. Sci. Rep. 11 (1), 1–8. doi: 10.1038/s41598-021-98934-5
Ke, C., Guan, W., Bu, S., Li, X., Deng, Y., Wei, Z., et al. (2019). Determination of absorption dose in chemical mutagenesis in plants. PloS One 14, e0210596. doi: 10.1371/journal.pone.0210596
Kim, Y., Schumaker, K. S., Zhu, J.-K. (2006). EMS mutagenesis of arabidopsis. Methods Mol. Biol. 323, 101–103. doi: 10.1385/1-59745-003-0:101
Kong, W., Wang, L., Cao, P., Li, X., Ji, J., Dong, P, et al. (2020). Identification and genetic analysis of EMS-mutagenized wheat mutants conferring lesion-mimic premature aging. BMC Genet. 21 (1), 1–11. doi: 10.1186/s12863-020-00891-x
Krysan, P. J., Young, J. C., Sussman, M. R. (1999). T-DNA As an insertional mutagen in Arabidopsis. The plant cell 11, 12, 2283–2290. doi: 10.1105/tpc.11.12.2283
Kumar, U., Padhan, B., Tripathi, A., Choudhary, J., Kumar, D., Prakash, N., et al. (2022). Breeding for herbicide tolerance in crops: a review. Res. J. Biotech. 15, 154–162.
Kumar, U., Chavan, N. S., Sawant, S. V., Kumar Yadav, H., et al. (2022). Evaluation and characterization of EMS induced mutant population of gossypium herbaceum. Vegetos 35 (4), 1036–1046. doi: 10.1007/s42535-022-00385-4
Kuraparthy, V., Sood, S., Dhaliwal, H. S., Chhuneja, P., Gill, B. S., et al. (2007). Identification and mapping of a tiller inhibition gene (tin3) in wheat. Theor. Appl. Genet. 114 (2), 285–294. doi: 10.1007/s00122-006-0431-y
Kurowska, M., Daszkowska-Golec, A., Gruszka, D., Marzec, M., Szurman, M., Szarejko, I., et al. (2011). TILLING-a shortcut in functional genomics. J. Appl. Genet. 52 (4), 371–390. doi: 10.1007/s13353-011-0061-1
Lee, Y.-H., Park, W., Kim, K.-S., Jang, Y.-S., Lee, J.-E., Cha, Y.-L., et al. (2018). EMS-induced mutation of an endoplasmic reticulum oleate desaturase gene (FAD2-2) results in elevated oleic acid content in rapeseed (Brassica napus L.). Euphytica 214 (2), 1–12. doi: 10.1007/s10681-017-2106-y
Lenawaty, D. Y., Sukma, D., Syukur, M., Suprapta, D., Nurcholis, W., Aisyah, S, et al. (2022). Increasing the diversity of marigold (Tagetes sp.) by acute and chronic chemical induced mutation of EMS (Ethyl methane sulfonate). Biodiversitas J. Biol. Diversity 23 (3). doi: 10.13057/biodiv/d230326
Lethin, J., Shakil, S. S.M., Hassan, S., Sirijovski, N., Töpel, M., Olsson, O., et al. (2020). Development and characterization of an EMS-mutagenized wheat population and identification of salt-tolerant wheat lines. BMC Plant Biol. 20 (1), 1–15. doi: 10.1186/s12870-019-2137-8
Li, S., Zhang, N., Li, J., Xiang, J., et al. (2019). A new male sterile line of “Duanye-13” radish (Raphanus sativus l.) produced by ethyl methanesulfonate mutagenesis. Genet. Resour. Crop Evol. 66 (5), 981–987. doi: 10.1007/s10722-019-00772-y
Li, X., Xiang, F., Zhang, W., Yan, J., Li, X., Zhong, M., et al. (2021). Characterization and fine mapping of a new dwarf mutant in Brassica napus. BMC Plant Biol. 21 (1), 1–12. doi: 10.1186/s12870-021-02885-y
Li, Z., Zhu, L., Zhao, F., Li, J., Zhang, X., Kong, X, et al. (2022). Plant salinity stress response and nano-enabled plant salt tolerance. Front. Plant Sci. 13, 843994. doi: 10.3389/fpls.2022.843994
Lian, X., Liu, Y., Guo, H., Fan, Y., Wu, J., Guo, H., et al. (2020). Ethyl methanesulfonate mutant library construction in Gossypium hirsutum l. for allotetraploid functional genomics and germplasm innovation. Plant J. 103 (2), 858–868. doi: 10.1111/tpj.14755
Liu, C., Song, G., Wang, N., Huang, S., Gao, Y., Fu, W., et al. (2021). A single SNP in Brcer1 results in wax deficiency in Chinese cabbage (Brassica campestris l. ssp. pekinensis). Sci Hortic. 282, 110019. doi: 10.1016/j.scienta.2021.110019
Manzanares, C., Yates, S., Ruckle, M., Nay, M., Studer, B. (2016). TILLING in forage grasses for gene discovery and breeding improvement. New Biotechnol. 33 (5), 594–603. doi: 10.1016/j.nbt.2016.02.009
Mba, C., Afza, R., Bado, S., Mohan Jain, S. (2010). Induced mutagenesis in plants using physical and chemical agents. Plant Cell culture: Essential Methods 20, 111–130. doi: 10.1002/9780470686522.ch7
Minoia, S., Petrozza, A., D'Onofrio, O., Piron, F., Mosca, G., Sozio, G., et al. (2010). A new mutant genetic resource for tomato crop improvement by TILLING technology. BMC Res. Notes 3 (1), 1–8. doi: 10.1186/1756-0500-3-69
Munda, S., Begum, T., Gogoi, A., Pandey, S. K., Sarma, N., Lal, M., et al. (2022). Induced variations by gamma radiation and EMS on the agronomic traits, essential oil yield with its quality and their exploitation in Java citronella (Cymbopogon winterianus jowitt). Int. J. Radiat. Biol. 98 (8), 1376–1387. doi: 10.1080/09553002.2022.2038805
Murray, V. (1987). O6-methylguanine specifically induces AT-GC transition mutations. Mutat. Res. 190 (4), 267–270. doi: 10.1016/0165-7992(87)90007-8
Nasri, F., Zakizadeh, H., Vafaee, Y., Mozafari, A. A., et al. (2022). In vitro mutagenesis of Chrysanthemum morifolium cultivars using ethyl methane sulphonate (EMS) and mutation assessment by ISSR and IRAP markers. Plant Cell Tissue Organ Culture 149 (3), 657–673. doi: 10.1007/s11240-021-02163-7
Newhouse, K., Singh, B., Shaner, D., Stidham, M. (1991). Mutations in corn (Zea mays l.) conferring resistance to imidazolinone herbicides. Theor. Appl. Genet. 83 (1), 65–70.
Pang, H., Li, W. H., Zhang, H. B., Wang, L., Yin, Y. A., Yuan, H. G., et al. (2010). Inheritance analysis of resistant starch content in kernels of wheat. Yi Chuan= Hereditas 32 (2), 170–176. doi: 10.3724/SP.J.1005.2010.00170
Patel, J., Chandnani, R., Khanal, S., Adhikari, J., Brown, N., Chee, P. W., et al. (2022). Pyramiding novel EMS-generated mutant alleles to improve fiber quality components of elite upland cotton germplasm. Ind. Crops Products 178, 114594. doi: 10.1016/j.indcrop.2022.114594
Piquerez, S. J., Harvey, S. E., Beynon, J. L., Ntoukakis, V. (2014). Improving crop disease resistance: lessons from research on Arabidopsis and tomato. Front. Plant Sci. 5, 671. doi: 10.3389/fpls.2014.00671
Prakash, N. R., et al. (2020). Breeding for herbicide tolerance in crops: A review. Res. J. Biotechnol. 15 (4).
Quesada, V., Ponce, M. R., Micol, J. L. (2000). Genetic analysis of salt-tolerant mutants in Arabidopsis thaliana. Genetics 154 (1), 421–436. doi: 10.1093/genetics/154.1.421
Rajasekaran, K., Grula, J. W., Anderson, D. M. (1996). Selection and characterization of mutant cotton (Gossypium hirsutum l.) cell lines resistant to sulfonylurea and imidazolinone herbicides. Plant Science 119 (1), 115–124. doi: 10.1016/0168-9452(96)04444-5
Reddy, T. V., Dwivedi, S., Sharma, N. K. (2012). Development of TILLING by sequencing platform towards enhanced leaf yield in tobacco. Ind. Crops Products 40, 324–335. doi: 10.1016/j.indcrop.2012.03.031
Rizwan, M., Aslam, M., Asghar, M. J., Abbas, G., Shah, T. M., Shimelis, H. (2017). Pre-breeding of lentil (Lens culinaris medik.) for herbicide resistance through seed mutagenesis. PloS One 12 (2), e0171846. doi: 10.1371/journal.pone.0171846
Roychowdhury, R., Tah, J. (2011). Germination behaviors in M2 generation of Dianthus after chemical mutagenesis. Intern. J. Adv. Sci. Tech. Res. 2 (1), 448–454.
Sabetta, W., Alba, V., Blanco, A., Montemurro, C. J. P. M. (2011). sunTILL: a TILLING resource for gene function analysis in sunflower. Plant Methods 7 (1), 1–13. doi: 10.1186/1746-4811-7-20
Saha, A. (2019). EMS induced polygenic mutation in tilottama cultivar of Sesamum indicum. Plant Arch. 19 (1), 630–632.
Samadi, N., Naghavi, M. R., Moratalla-López, N., Alonso, G. L., Shokrpour, M. (2022). Morphological, molecular and phytochemical variations induced by colchicine and EMS chemical mutagens in Crocus sativus l. Food Chem.: Mol. Sci. 4, 100086. doi: 10.1016/j.fochms.2022.100086
Sebastian, S. A., Chaleff, R. S. (1987). Soybean mutants with increased tolerance for sulfonylurea herbicides 1. Crop Sci. 27 (5), 948–952. doi: 10.2135/cropsci1987.0011183X002700050025x
Shah, D., Kamili, A. N., Wani, A. A., Majeed, U., Wani, Z. A., Sajjad, N., et al. (2020). Promoting the accumulation of scopolamine and hyoscyamine in Hyoscyamus niger l. through EMS based mutagenesis. Mutat. Research/Reviews Genet. Toxicol. 15 (5), e0231355.
Shankar, A., Choudhary, O., Singh, K. (2021). Effect of EMS induced mutation in rice cultivar nagina 22 on salinity tolerance. bioRxiv. doi: 10.1101/2021.08.03.455004
Shi, Z., Ren, W., Zhao, Y., Wang, X., Zhang, R., Su, A., et al. (2021). Identification of a locus associated with genic male sterility in maize via EMS mutagenesis and bulked-segregant RNA-seq. Crop J. 9 (6), 1263–1269. doi: 10.1016/j.cj.2020.09.007
Siddique, M. I., Back, S., Lee, J. H., Jo, J., Jang, S., Han, K., et al. (2020). Development and characterization of an ethyl methane sulfonate (EMS) induced mutant population in Capsicum annuum l. Plants 9 (3), 396. doi: 10.3390/plants9030396
Silué, S., Nafan, D., Fofana, I., Muhovski, Y., Toussaint, A., Guy, M., et al. (2013). Description of Phaseolus vulgaris l. aborting embryos from ethyl methanesulfonate (EMS) mutagenized plants. Biotechnol agronomie société environnement, 17(4).
Singh, H., Verma, P., Lal, S., Khar, A. (2021). Optimization of EMS mutagen dose for short day onion. Indian J. Hortic. 78 (1), 35–40. doi: 10.5958/0974-0112.2021.00005.0
Singh, R., Kumar, G. (2022). Analyzing frequency and spectrum of chlorophyll mutation induced through gamma ray and combination treatment (Gamma + EMS) on genetic paradigm of Artemisia annua l.: Effect of gamma and gamma + EMS on the frequency and spectrum of chlorophyll mutation in Artemisia annua l. Caryologia.
Singh, P. K., Sadhukhan, R. (2019). Ems and gamma radiation induced mutation in grasspea (Lathyrus sativus l.). Legume Res. 42, 300–307.
Slade, A. J., Fuerstenberg, S. I., Loeffler, D., Steine, M. N., Facciotti, D. (2005). A reverse genetic, nontransgenic approach to wheat crop improvement by TILLING. Nat. Biotechnol. 23 (1), 75–81. doi: 10.1038/nbt1043
Stadler, L. J. (1929). Chromosome number and the mutation rate in avena and Triticum. Proc. Natl. Acad. Sci. 15 (12), 876–881. doi: 10.1073/pnas.15.12.876
Sudianto, E., Song, B.-K., Neik, T.-X., Saldain, N. E., Scott, R. C., Burgos, N. R. (2013). Clearfield® rice: Its development, success, and key challenges on a global perspective. Crop Protection 49, 40–51. doi: 10.1016/j.cropro.2013.02.013
Sun, X., Li, X., Lu, Y., Wang, S., Zhang, X., Zhang, K., et al. (2022). Construction of a high-density mutant population of Chinese cabbage facilitates the genetic dissection of agronomic traits. Mol. Plant 15 (5), 913–924. doi: 10.1016/j.molp.2022.02.006
Taheri, S., Abdullah, T. L., Jain, S. M., Sahebi, M., Azizi, P. (2017). TILLING, high-resolution melting (HRM), and next-generation sequencing (NGS) techniques in plant mutation breeding. Mol. Breed. 37 (3), 1–23. doi: 10.1007/s11032-017-0643-7
Takagi, H., Tamiru, M., Abe, A., Yoshida, K., Uemura, A., Yaegashi, H., et al. (2015). MutMap accelerates breeding of a salt-tolerant rice cultivar. Nat. Biotechnol. 33 (5), 445–449. doi: 10.1038/nbt.3188
Tang, S., Liu, D. X., Lu, S., Yu, L., Li, Y., Lin, S., et al. (2020). Development and screening of EMS mutants with altered seed oil content or fatty acid composition in Brassica napus. Plant J. 104 (5), 1410–1422. doi: 10.1111/tpj.15003
Thange, V., Bagade, A., Wankhade, M. (2021). Evaluation of mutagenic effects on frequency and spectrum of chlorophyll mutation in sorghum (Sorghum bicolor (L.) Moench).
Thangwana, A., Gwata, E. T., Zhou, M. M. (2021). Impact of chemical mutagenesis using ethyl methane sulphonate on tepary bean seedling vigour and adult plant performance. Heliyon 7 (1), e06103. doi: 10.1016/j.heliyon.2021.e06103
Till, B. J., Reynolds, S. H., Greene, E. A., Codomo, C. A., Enns, L. C., Johnson, J. E., et al. (2003). Large-Scale discovery of induced point mutations with high-throughput TILLING. J. Genome Res. 13 (3), 524–530. doi: 10.1101/gr.977903
Till, B. J., Reynolds, S. H., Weil, C., Springer, N., Burtner, C., Young, K., et al. (2004). Discovery of induced point mutations in maize genes by TILLING. BMC Plant Biol. 4 (1), 12. doi: 10.1186/1471-2229-4-12
Till, B. J., Cooper, J., Tai, T. H., Colowit, P., Greene, E. A., Henikoff, S., et al. (2007). Discovery of chemically induced mutations in rice by TILLING. BMC Plant Biol. 7 (1), 19. doi: 10.1186/1471-2229-7-19
Travella, S., Klimm, T. E., Keller, B. (2006). RNA Interference-based gene silencing as an efficient tool for functional genomics in hexaploid bread wheat. Plant Physiol. 142 (1), 6–20. doi: 10.1104/pp.106.084517
Unan, R., Deligoz, I., Al-Khatib, K., Mennan, H. (2021). Protocol for ethyl methanesulphonate (EMS) mutagenesis application in rice. Open Res. Europe 1, 19. doi: 10.12688/openreseurope.13317.1
Van Zelm, E., Zhang, Y., Testerink, C. (2020). Salt tolerance mechanisms of plants. Annu. Rev. Plant Biol. 71, 403–433. doi: 10.1146/annurev-arplant-050718-100005
Wanga, M. A., Shimelis, H., Horn, L. N., Sarsu, F. (2020). The effect of single and combined use of gamma radiation and ethylmethane sulfonate on early growth parameters in sorghum. Plants 9 (7), 827. doi: 10.3390/plants9070827
Wang, H., Huang, C., Zhao, W., Dai, B., Shen, C., Zhang, B., et al. (2016). Identification of QTL for fiber quality and yield traits using two immortalized backcross populations in upland cotton. PloS One 11 (12), e0166970. doi: 10.1371/journal.pone.0166970
Wang, C., Hao, N., Xia, Y., Du, Y., Huang, K., Wu, T., et al. (2021). CsKDO is a candidate gene regulating seed germination lethality in cucumber. Breed. Sci. 71 (4), 417–425. doi: 10.1270/jsbbs.20149
Wang, X., Wang, A., Li, Y., Xu, Y., Wei, Q., Wang, J., et al. (2021). A novel banana mutant “RF 1”(Musa spp. ABB, pisang awak subgroup) for improved agronomic traits and enhanced cold tolerance and disease resistance. Front. Plant Sci. 12, 730718. doi: 10.3389/fpls.2021.730718
Wei, Y., Liu, Y., Ali, A. M., Xiao, R., Liang, C., Meng, Z., et al. (2022). Rich variant phenotype of Gossypium hirsutum l. saturated mutant library provides resources for cotton functional genomics and breeding. Ind. Crops Products 186, 115232. doi: 10.1016/j.indcrop.2022.115232
Wu, Z., Liu, Z., Chang, S., Zhao, Y. (2020). An EMS mutant library for carrot and genetic analysis of some mutants. Breed. Sci 70 (5), 540–546. doi: 10.1270/jsbbs.20020
Xiong, H., Zhou, C., Guo, H., Xie, Y., Zhao, L., Gu, J., et al. (2020). Transcriptome sequencing reveals hotspot mutation regions and dwarfing mechanisms in wheat mutants induced by γ-ray irradiation and EMS. J. Radiat. Res. 61 (1), 44–57. doi: 10.1093/jrr/rrz075
Xi-Ou, X., Wenqiu, L., Wei, L., Xiaoming, G., Lingling, L., Feiyue, M., et al. (2017). The analysis of physiological variations in M2 generation of Solanum melongena l. mutagenized by ethyl methane sulfonate. Front. Plant Sci. 8, 17. doi: 10.3389/fpls.2017.00017
Yan, W., Deng, X. W., Yang, C., Tang, X. (2021). The genome-wide EMS mutagenesis bias correlates with sequence context and chromatin structure in rice. Front. Plant Sci. 12, 579675. doi: 10.3389/fpls.2021.579675
Yan, Z., Appiano, M., van Tuinen, A., Meijer-Dekens, F., Schipper, D., Gao, D., et al. (2021). Discovery and characterization of a novel tomato mlo mutant from an ems mutagenized micro-tom population. Genes 12 (5), 719. doi: 10.3390/genes12050719
Keywords: ethyl methane sulfonate, molecular breeding, abiotic stress tolerance, plant development, mutation breeding
Citation: Chen L, Duan L, Sun M, Yang Z, Li H, Hu K, Yang H and Liu L (2023) Current trends and insights on EMS mutagenesis application to studies on plant abiotic stress tolerance and development. Front. Plant Sci. 13:1052569. doi: 10.3389/fpls.2022.1052569
Received: 24 September 2022; Accepted: 01 December 2022;
Published: 04 January 2023.
Edited by:
Tianlun Zhao, Zhejiang University, ChinaReviewed by:
Hao Chen, Guangdong Academy of Agricultural Sciences (GDAAS), ChinaDexin Liu, Southwest University, China
Rasim Unan, University of California, Davis
Copyright © 2023 Chen, Duan, Sun, Yang, Li, Hu, Yang and Liu. This is an open-access article distributed under the terms of the Creative Commons Attribution License (CC BY). The use, distribution or reproduction in other forums is permitted, provided the original author(s) and the copyright owner(s) are credited and that the original publication in this journal is cited, in accordance with accepted academic practice. No use, distribution or reproduction is permitted which does not comply with these terms.
*Correspondence: Hong Yang, eWFuZ0BodWJ1LmVkdS5jbg==; Li Liu, bGl1bGkyMDIwQGh1YnUuZWR1LmNu