- Guangdong Key Laboratory of Plant Adaptation and Molecular Design, Guangzhou Key Laboratory of Crop Gene Editing, Innovative Center of Molecular Genetics and Evolution, School of Life Sciences, Guangzhou University, Guangzhou Higher Education Mega Center, Guangzhou, China
Symbiotic nitrogen fixation is an important factor affecting the yield and quality of leguminous crops. Nodulation is regulated by a complex network comprising several transcription factors. Here, we functionally characterized the role of a TOC1 family member, GmTOC1b, in soybean (Glycine max) nodulation. RT-qPCR assays showed that GmTOC1b is constitutively expressed in soybean. However, GmTOC1b was also highly expressed in nodules, and GmTOC1 localized to the cell nucleus, based on transient transformation in Nicotiana benthamiana leaves. Homozygous Gmtoc1b mutant plants exhibited increased root hair curling and produced more infection threads, resulting in more nodules and greater nodule fresh weight. By contrast, GmTOC1b overexpression inhibited nodulation. Furthermore, we also showed that GmTOC1b represses the expression of nodulation-related genes including GmNIN2a and GmENOD40-1 by binding to their promoters. We conclude that GmTOC1b functions as a transcriptional repressor to inhibit nodulation by repressing the expression of key nodulation-related genes including GmNIN2a, GmNIN2b, and GmENOD40-1 in soybean.
Introduction
Soybean (Glycine max L.) is an important oil crop and the main source of protein for animal diets. To meet their high nitrogen demand during growth and reproduction, legumes release flavonoids via root exudates to attract rhizobia with which they then form nodules (Hungria et al., 2005; Ciampitti and Salvagiotti, 2018). Nodules function as a biological nitrogen fixation factory, which can efficiently convert atmospheric N2 into ammonia for their plant hosts (Schipanski et al., 2010; Dong et al., 2021b). In exchange, legumes can provide a stable nitrogen fixation environment that is low in oxygen, as well as carbon and other nutrients for rhizobia. Thus, symbiotic nitrogen fixation not only is a critical factor for improving soybean yield and quality but can also help us reach and maintain sustainable agricultural production practices in an environmentally conscious manner (Gelfand and Philip Robertson, 2015; Santachiara et al., 2019).
The symbiosis between legumes and rhizobia has been well studied. Rhizobia are attracted to plant roots by host-secreted flavonoids and synthesize nodulation (Nod) factors, which are specifically recognized by NOD FACTOR RECEPTOR (NFR) on the root epidermis of legumes (Indrasumunar et al., 2010; Indrasumunar et al., 2011; Liu and Murray, 2016). After initial signal recognition, root hairs curl to surround the rhizobia, followed by the entry of the rhizobia into root endodermis cells through infection lines (Suzaki and Kawaguchi, 2014; Kawaharada et al., 2017). The invaded root cortical cells divide to form a nodule primordium (Heckmann et al., 2011). The rhizobia are finally released into each primordium through cortical cells to develop a mature root nodule (Hayashi et al., 2014).
Many key genes involved in symbiotic nitrogen fixation have been identified. After recognizing Nod factors, NFRs can induce the expression of genes encoding leucine-rich repeat receptor-like kinases (LRR-RLKs) such as Symbiosis receptor kinase (LjSYMRK) from Lotus japonicus (Demchenko et al., 2004), DOES NOT MAKE INFECTION 2 (MtDMI2) from Medicago truncatula (Limpens et al., 2005), and Nodulation receptor kinase (GmNORK) from soybean (Indrasumunar et al., 2015; Wang et al., 2020b), which leads to the activation of downstream gene DMI3, encoding the intracellular calcium and calmodulin-dependent protein kinase (CCaMK) (Rival et al., 2012). CCaMK can phosphorylate the transcription factor CYCLOPS and then activate the expression of its downstream target gene NIN (Nodule induction) (Cerri et al., 2017; Liu et al., 2019). EARLY NODULIN 40 (ENOD40) was the first identified key component of nodulation and encodes a host-derived 12- to 13-amino acid peptide that enhances the stability of sucrose synthase (Crespi et al., 1994; Röhrig et al., 2002). Previous research suggested that ENOD40 expression can be rapidly induced after inoculation with rhizobia or purified Nod factors, with ENOD40 functioning as an intercellular signal molecule or participating in the regulation of carbon metabolism during nodule formation (Campalans et al., 2004; Xu et al., 2021). In addition, the transcription factors Nodulation Signaling Pathway 1 (NSP1) and NSP2 form a heterologous protein complex in the nucleus (Smit et al., 2005) and directly bind to the promoters of early nodulin genes such as ENOD11 (Svistoonoff et al., 2010), NIN and Ethylene response factor required for nodule (ERN) (Cerri et al., 2012), which regulate nodule formation at the early stage. Although many studies have investigated nodule signaling, a full picture of nodulation has yet to emerge due to the underlying complex regulatory pathways.
TOC1 (TIMING OF CAB EXPRESSION 1) is a key component of the circadian clock and controls many biological processes supporting plant growth and development (Sanchez et al., 2011; Scheiermann et al., 2013; Xu et al., 2022). In Arabidopsis (Arabidopsis thaliana), toc1 mutants enhance drought stress tolerance and showed greater sensitivity to the phytohormone abscisic acid (ABA). Moreover, TOC1 was shown to bind to the promoter of the ABA receptor (ABAR) and modulate its expression (Legnaioli et al., 2009). TOC1 also interacts with PHYTOCHROME-INTERACTING FACTOR 4 (PIF4) and represses its ability to suppress thermoresponsive growth in the evening (Zhu et al., 2016). In rice (Oryza sativa), OsTOC1 regulates tiller-bud and panicle development by indirectly repressing the expression of TEOSINTE BRANCHED 1 (TB1), DWARF 14 (D14), and IDEAL PLANT ARCHITECTURE 1 (IPA1) (Wang et al., 2020a). In the model legume M. truncatula, members of other clock components, including LATE ELONGATED HYPOCOTYL (LHY) and LUX ARRHYTHMO (LUX), also regulate nodulation. For instance, MtLHY affected nodulation via the regulation of nodule cysteine-rich peptides (Kong et al., 2020; Achom et al., 2021). MtLUX also plays a role in nodule formation, probably through an indirect regulation with MtLHY (Kong et al., 2022).
In this study, we characterized the role of GmTOC1b in nodule formation. Knockout mutants of GmTOC1b promoted soybean nodulation by affecting hair curling and the number of infection threads. Conversely, overexpression of GmTOC1b significantly inhibited nodulation in transgenic hairy roots. GmTOC1b regulated multiple nodulation-related genes, including GmNIN2a, GmNIN2b, and GmENOD40-1, in the early stage. Further investigation showed that GmTOC1b represses the expression of GmNIN2a and GmENOD40-1 by binding to their promoters. Taken together, our results revealed the vital role of GmTOC1b in regulating nodule formation in soybean and should be useful for genetic improvement in soybean and other legumes.
Materials and methods
Plant materials and growth conditions
Soybean (G. max L.) cultivar Williams 82 (W82) and GUS-tagged Bradyrhizobium japonicum strain USDA110 were used in this study. All soybean plant materials were generated in the W82 background.
For tissue-specific expression analysis, soybean seeds were sown and cultured at 26°C under 16-h-light/8-h-dark conditions. Tissue samples were harvested at the indicated growth periods. All samples were frozen in liquid nitrogen and stored at −80°C until total RNA extraction.
For the nodulation phenotyping assay, uniform healthy seeds were surface sterilized with chlorine gas for 14 h and then germinated in vermiculite under 16-h-light/8-h-dark conditions in a growth room at 26°C. Soybean seedlings were supplied with low-nitrogen nutrient solution containing 150 μM of KNO3, 120 μM of Ca(NO3)2·4H2O, 25 μM of MgCl2, 30 μM of (NH4)2SO4, 40 µM of Fe-Na-EDTA, 500 μM of MgSO4·7H2O, 1.5 μM of MnSO4·H2O, 1.5 μM of ZnSO4·7H2O, 0.5 μM of CuSO4·5 H2O, 0.15 μM of (NH4)6Mo7O24·4H2O, 2.5 μM of NaB4O7·10H2O, 500 μM of KH2PO4, 540 μM of CaCl2, and 345 μM of K2SO4. After unifoliolate leaves were completely opened, the seedlings were inoculated with GUS-tagged B. japonicum USDA110 and resuspended in low-nitrogen nutrient solution (OD600 = 0.1, 5 ml) at Zeitgeber 12. The nodule phenotype was evaluated 14 days after inoculation (DAI).
Phylogenetic analysis
TOC1 homologous protein sequences from monocotyledons and dicotyledons were aligned using the Clustal X. A neighbor-joining tree was constructed with the software MEGA11 (Tamura et al., 2021). Conserved motifs were extracted with the MEME suite (Bailey et al., 2015).
RNA extraction and RT-qPCR analysis
Total RNA was extracted using a FastPure Plant Total RNA Isolation Kit (Vazyme, Nanjing, China). Removal of genomic DNA and first-strand cDNA synthesis was performed using a PrimeScript RT reagent kit with gDNA Eraser (Takara, Dalian, China). qPCR was conducted on a Roche LightCycle480 system (Roche, Mannheim, Germany) with TB Green (Takara). Three biological replicates were used for all experiments. GmActin (Glyma.02g091900) was used as the reference gene. All primers used are listed in Supplementary Table 1.
Plasmid construction
CRISPR/Cas9-mediated gene editing was used to generate Gmtoc1b mutants. Single-guide RNAs (sgRNA) were predicted using the online tool CRISPR-P 2.0 (http://crispr.hzau.edu.cn/cgi-bin/CRISPR2/CRISPR); the two most reliable sgRNAs targeting different regions of GmTOC1b were designed and cloned into the CRISPR/Cas9 vector (Hao et al., 2020). The construct was transformed into Agrobacterium (Agrobacterium tumefaciens) strain EHA105, which was then used to transform soybean cultivar W82 as previously described (Chen et al., 2018). The genotype of Gmtoc1b mutants was verified using Sanger sequencing, and homozygous Gmtoc1b mutants were used for further research. For the GmTOC1b overexpression construct, the GmTOC1b coding sequence without stop codon was amplified and cloned into the proGmUBI:3×Flag vector under the control of the soybean Ubiquitin (UBI) promoter to generate proGmUBI : GmTOC1b-3×Flag. The cauliflower mosaic virus (CaMV) 35S promoter was used to drive the expression of green fluorescent protein (GFP) for screening positive transformation events.
Subcellular localization of GmTOC1b
The GmTOC1b coding sequence without the stop codon was cloned in-frame and upstream of the GFP sequence driven by the GmUBI promoter. The plasmid was transformed into Agrobacterium strain GV3101, which was then transiently infiltrated into Nicotiana benthamiana leaves. The plants were grown at 26°C under 16-h-light/8-h-dark conditions for 48 h. Green fluorescent protein (GFP) fluorescence was observed using a Zeiss LSM 800 confocal laser scanning microscope (Zeiss, Oberkochen, Germany). Transiently infiltrated proGmUBI : GFP N. benthamiana leaves were used as localization controls. Leaves were stained with 4′,6-diamidino-2-phenylindole (DAPI) to label nuclei.
Soybean hairy root transformation
To obtain transgenic composite plants, soybean hairy root transformation was performed according to a previously published method (Kereszt et al., 2007). Positive transgenic hairy roots were confirmed by detecting GFP fluorescence using a hand-held fluorescence lamp (Luyor 3415RG; Luyor, Shanghai, China). The plants were grown under high-humidity conditions for 1 week to adapt to the environment. For the nodulation phenotyping assay, each plant was inoculated with B. japonicum USDA110 (OD600 = 0.1, 5 ml). Nodule numbers per root and shoot dry weights were evaluated at 20 DAI.
Observation of root hair curling and infection threads
To detect infection events, 7-day-old seedlings were inoculated with GUS-tagged B. japonicum USDA110. Lateral root segments (5 cm in length) from W82 and the Gmtoc1b mutants were harvested at 3 and 5 DAI. For the detection of root hair curling, root samples of 3-DAI seedlings were gently washed with sterile water and directly used for observation. For detection of infection threads, 5-DAI root samples were washed and then soaked in X-Gluc solution [100 mM of NaH2PO4, 100 mM of Na2HPO4, 1 mM of K4(Fe(CN)6), 1 mM of K3(Fe(CN)6), 1 mg/ml of X-Gluc, 10 mM of Na2EDTA, 0.1% (v/v) Triton X-100] overnight at 37°C, after which time the stained roots were observed. Digital images were taken with a Zeiss Axio Imager A2 microscope (Zeiss, Oberkochen, Germany).
Transient expression assay
To generate promoter-driven firefly luciferase (LUC) constructs, ~2-kb promoter fragments for NIN2a and ENOD40-1 were individually amplified from W82 genomic DNA; the PCR product was purified and cloned into the pGreenII 0800-LUC vector. The resulting proGmNIN2a:LUC and proGmENOD40-1:LUC constructs were used as reporters, and proGmUBI:3×Flag and proGmUBI : GmTOC1b-3×Flag were used as effectors. The constructs proGmUBI : GmTOC1b-3×Flag and proGmNIN2a:LUC or proGmENOD40-1:LUC were co-infiltrated into N. benthamiana leaves. The constructs proGmUBI:3×Flag and proGmNIN2a-LUC or proGmENOD40-1-LUC were co-infiltrated into N. benthamiana leaves as the blank control. Relative Firefly Luciferase; (LUC)/Renilla Luciferase (REN) was analyzed. Three independent biological replicates were performed in this assay. The firefly and Renilla luciferase signals were assayed using a Dual-Luciferase Reporter Assay System (Promega, Madison, WI, USA) and measured on a Biotek Synergy H1 Microplate Reader (Agilent, Santa Clara, CA, USA). Digital images were taken after spraying 1 mM of D-Luciferin sodium salt onto infiltrated N. benthamiana leaves (Sangon Biotech, Shanghai, China).
Results
Sequence analysis of GmTOC1b
In this study, we characterized the soybean circadian regulator gene GmTOC1b (also known as PSEUDO-RESPONSE REGULATOR 1 [PRR1]), showing high similarity to Arabidopsis TOC1 (Gan et al., 2020). We downloaded the GmTOC1b (Glyma.06G196200) sequence from the Phytozome (https://phytozome-next.jgi.doe.gov) database. The cDNA sequence of GmTOC1b is 2,597 bp with an open reading frame consisting of 1,677 bp that encodes a 559-amino acid protein. GmTOC1b is predicted to contain an N-terminal pseudoreceiver (PR) domain and a C-terminal [CONSTANS, CONSTANS-LIKE, TOC1] (CCT) domain, which are common to all TOC1 orthologs (Figure 1A). Phylogenetic analysis indicated that GmTOC1b groups into the dicots and is closely related to its soybean TOC1 homologs and other TOC1 proteins from Arabidopsis, Brassica rapa, and Solanum lycopersicum (Figure 1C). We also looked for conserved motifs across TOC1 homologs, which identified the PR (motifs 1, 2, and 3) and CCT (motif 9) domains in all TOC1 proteins (Wang et al., 2020a). Notably, motifs 5 and 8 only existed in the TOC1 proteins from monocots (Figure 1B). These results suggest that TOC1 is relatively well conserved with unique characteristics that have arisen since monocotyledons and dicotyledons diverged.
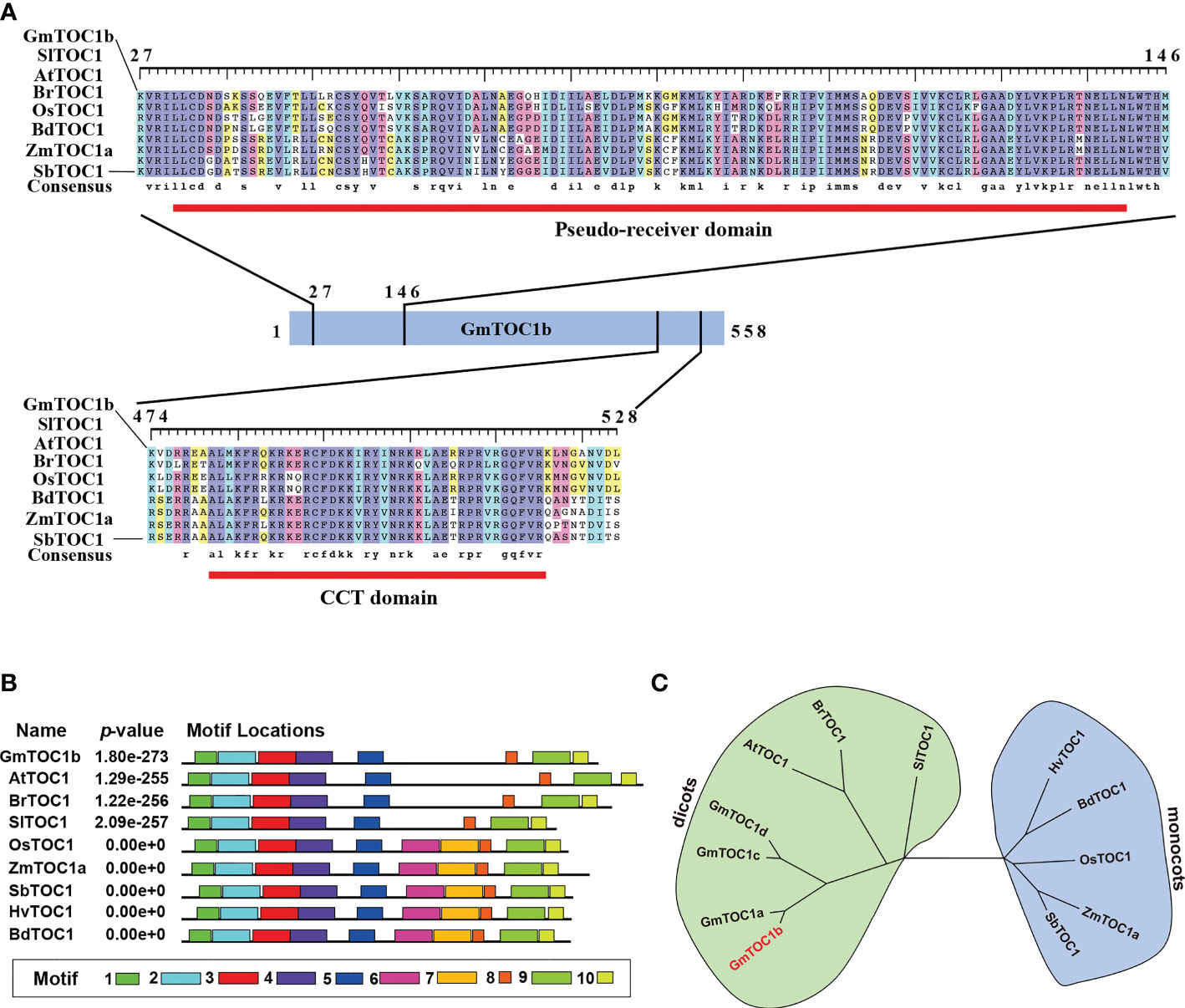
Figure 1 Analysis of TOC1 homologs in soybean and other plants. (A) TOC1 homologs contain conserved an N-terminal pseudo-receiver domain and a C-terminal CCT (CONSTANS, CO-LIKE, and TOC1) domain. Amino acid sequences were aligned using Clustal X. Red lines indicates the highly conserved domains. aa, amino acids; Sl, Solanum lycopersicum; At, Arabidopsis thaliana; Br, Brassica rapa; Os, Oryza sativa; Bd, Brachypodium distachyon; Zm, Zea mays; Sb, Sorghum bicolor. (B) Distribution of conserved motifs in TOC1 homologs. The MEME website tool was used to perform the analysis. (C) Phylogenetic analysis of TOC1 proteins. A neighbor-joining tree was constructed using full-length protein sequences, with bootstrap values set to 1,000 replicates.
GmTOC1b is a nuclear protein
The subcellular distribution of GmTOC1b is essential for the prediction of its function. GmTOC1b-GFP driven by the GmUBI promoter was detectable specifically in the nucleus of N. benthamiana mesophyll cells (Figure 2A), whereas the GFP signal was distributed ubiquitously among the N. benthamiana mesophyll cells in the proGmUBI-GFP control. The results showed that GmTOC1b is a nucleus-localized protein.
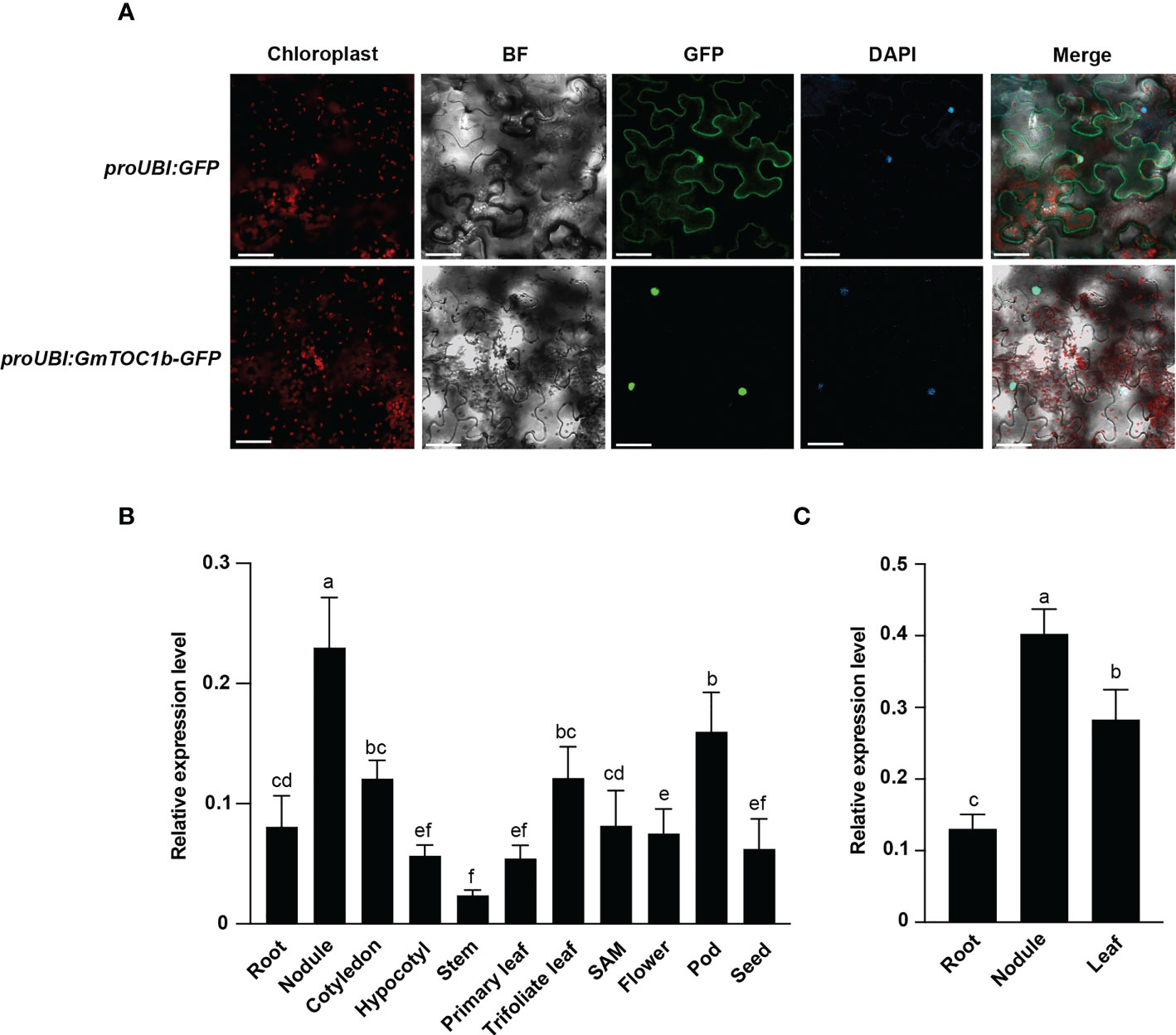
Figure 2 GmTOC1b localization and expression pattern analyses of GmTOC1b. (A) Subcellular localization of GmTOC1b. Nicotiana benthamiana leaves were transiently infiltrated with proUBI : GFP or proUBI : GmTOC1b-GFP; the plants were then grown for 48 h under 16-h-light/8-h-dark condition before GFP fluorescence was observed by microscopy. GFP, green fluorescent protein; BF, brightfield; Merge, GFP and BF images. Scale bars = 20 μm. (B) Relative GmTOC1b transcript levels in various tissues during growth and at different development stages. Relative expression level was calculated as the ratio of the expression value of the target gene to that of reference gene GmActin as an internal standard. (C) RT-qPCR analysis of GmTOC1b in different tissues at 14 days after inoculation (DAI) with rhizobia. Relative expression level was calculated as the ratio of the expression value of the target gene to that of reference gene GmActin as an internal standard. Three biological replicates were performed in all experiments. Different lowercase letters indicate significant differences as determined by Student’s t-test.
Expression patterns of GmTOC1b in soybean
We harvested samples from various tissues such as roots, nodules, cotyledons, hypocotyls, stems, primary leaves, trifoliate leaves, the shoot apical meristem (SAM), flowers, pods, and seeds 12 h after lights on (Zeitgeber 12 [ZT12]) from seedlings or plants grown under a 16-h-light/8-h-dark photoperiod. We detected GmTOC1b transcripts in all tissues tested, but with the highest abundance in nodules (Figure 2B). Furthermore, we also assessed GmTOC1b expression levels in leaves, roots, and nodules of soybean plants 14 days after inoculation (DAI) with B. japonicum strain USDA110. We determined that GmTOC1b is also highly expressed in nodules (Figure 2C), suggesting that GmTOC1b may function in the formation and development of nodules.
Knockout of GmTOC1b promotes soybean nodulation
To investigate a possible role for GmTOC1b in nodulation, we used clustered regularly interspaced short palindromic repeats (CRISPR)/CRISPR-associated nuclease 9 (Cas9)-mediated gene editing to generate Gmtoc1b mutants. We generated three positive transgenic events from 10 T0 generation transgenic plants. After the screening of heterozygous Gmtoc1b mutant lines, we obtained two homozygous T2 generations Gmtoc1b mutants with a 7-bp deletion (Gmtoc1b-1) or a 4-bp deletion (Gmtoc1b-2) (Supplementary Figure 1A). The T3 generation of homozygous Gmtoc1b mutants was used for further study. Reverse transcription–quantitative PCR (RT-qPCR) analysis showed that GmTOC1b expression levels are markedly lower in both Gmtoc1b mutants compared to W82 (Supplementary Figure 1B). These two mutants allowed us to investigate GmTOC1b function by evaluating the number of nodules per plant for the Gmtoc1b mutants and the wild-type W82 after inoculation with β-glucuronidase (GUS)-tagged B. japonicum USDA110. We observed a significant increase in nodule number and nodule fresh weight in the two Gmtoc1b mutants relative to W82 at 14 DAI (Figures 3A–C). As leghemoglobin plays a key role in the biological nitrogen fixation of leguminous plants (Berger et al., 2020; Du et al., 2020; Jiang et al., 2021), we further measured the content of leghemoglobin. The results showed that no significant difference existed between nodules from W82 and Gmtoc1b mutants (Supplementary Figure 3). The results indicated that the increased nodule number may not due to the functional weakening of nodules in Gmtoc1b mutants.
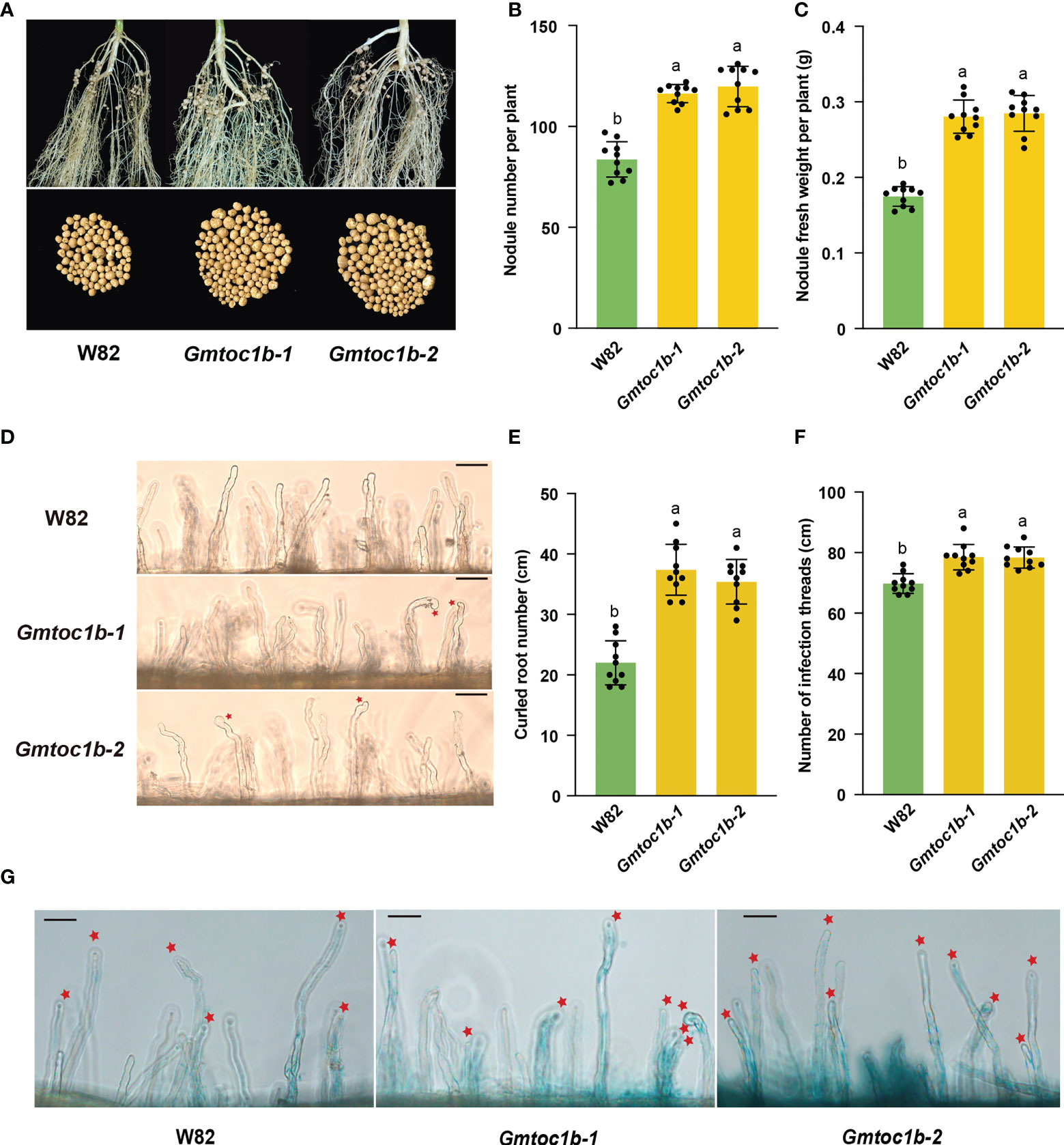
Figure 3 Knockout of GmTOC1b promotes nodulation in soybean. (A) Representative nodule performance of W82 and Gmtoc1b mutants at 14 DAI. (B, C) Nodule number and nodule fresh weight per plant for W82 and Gmtoc1b mutants at 14 DAI. Values are the mean ± SD. (D) Representative image of root hair curling in W82 and the Gmtoc1b mutant at 3 DAI. Scale bar, 100 μm. (E) Number of curled root hairs on W82 and Gmtoc1b mutant plants per centimeter of root length (n = 10). (F) Number of infection threads in W82 and Gmtoc1b mutants per centimeter of root length (n = 10). (G) Representative image of infection numbers observed in the W82 and Gmtoc1b mutants at 5 DAI (n = 10). Scale bars, 100 μm. Different lowercase letters indicate significant differences as determined by Student’s t-test.
Knocking out GmTOC1b enhances rhizobial infection and nodule formation
To explore the roles of GmTOC1b during rhizobial infection and nodule formation, we observed root phenotypes after inoculation. Compared to W82, the two Gmtoc1b mutants both exhibited significantly greater root hair curling at 3 DAI and more infection threads at 5 DAI (Figures 3D–G). Both Gmtoc1b mutants also produced more small nodules at 7 DAI relative to W82 (Supplementary Figures 2A, B).
Overexpression of GmTOC1b inhibits nodulation
In a complementary approach, we also generated transgenic composite plants with hairy roots overexpressing GmTOC1b under the control of the soybean Ubiquitin (GmUBI) promoter, which we then inoculated with rhizobia. Relative GmTOC1b transcript levels were close to 60-fold higher in the overexpression (GmTOC1b-OE) lines; moreover, we detected Flag-tagged GmTOC1b, but not in empty vector control transgenic roots (Figures 4B, C). The transgenic GmTOC1b-OE roots formed fewer nodules compared to empty vector control hair roots at 20 DAI (Figure 4A). The lower number of nodules per gram of root or shoot dry weight also suggested that overexpression of GmTOC1b significantly inhibits nodulation (Figures 4D, E). Together, these data reveal that GmTOC1b plays a negative role in soybean nodulation.
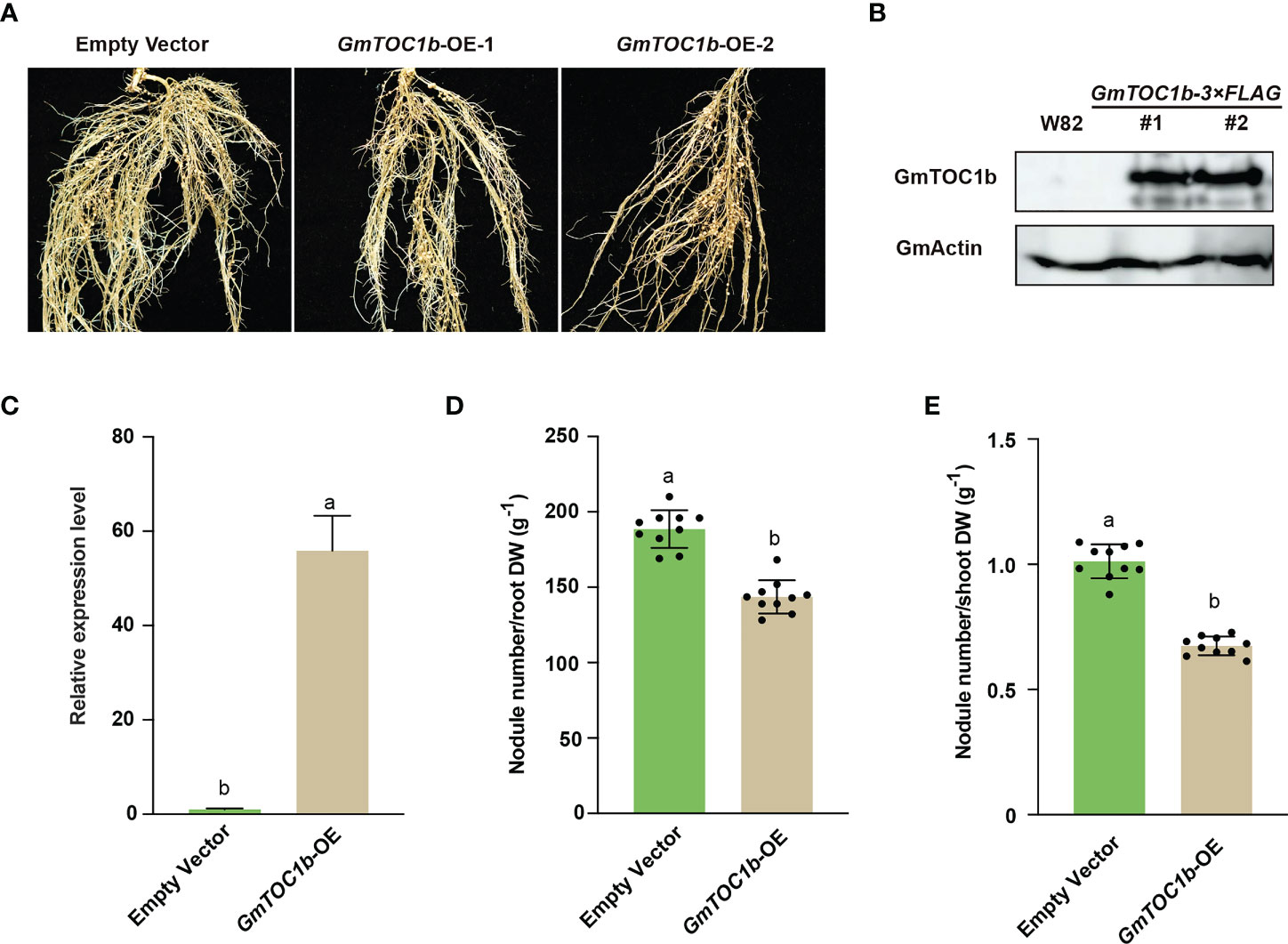
Figure 4 Overexpression of GmTOC1b in transgenic hairy roots inhibits nodulation. (A) Representative nodulation phenotype of GmTOC1b-OE and empty vector control transgenic hairy roots at 14 DAI. (B) Immunoblot analysis of GmTOC1b abundance in W82 and GmTOC1b-3Flag transgenic hairy roots. An anti-Flag antibody was used to recognize GmTOC1b-3Flag; actin was used as loading control. (C) Relative GmTOC1b transcript levels in GmTOC1b-OE and empty vector control transgenic hairy roots. Relative expression level was calculated as the ratio of the expression value of the target gene to that of reference gene GmActin as an internal standard. (D, E) Nodule number per gram of root dry weight or shoot dry weight in GmTOC1b-OE and empty vector control transgenic hairy roots at 14 DAI (n = 10). Different lowercase letters indicate significant differences as determined by Student’s t-test.
GmTOC1b inhibits the transcription of nodulation-related genes
To explore the molecular mechanisms by which GmTOC1b inhibits nodule formation, we analyzed the expression pattern of the nodulation-related marker genes GmNIN2a, GmNIN2b, and GmENOD40-1 in infected soybean roots at 3 DAI. We first assessed their expression levels in the Gmtoc1b mutants, and all three genes were induced in the Gmtoc1b mutants (Figures 5A–C). By contrast, these three genes were expressed at much lower levels in transgenic hairy roots overexpressing GmTOC1b (Figures 5D–F). These results suggest that GmTOC1b functions as a negative regulator of these nodulation-related genes.
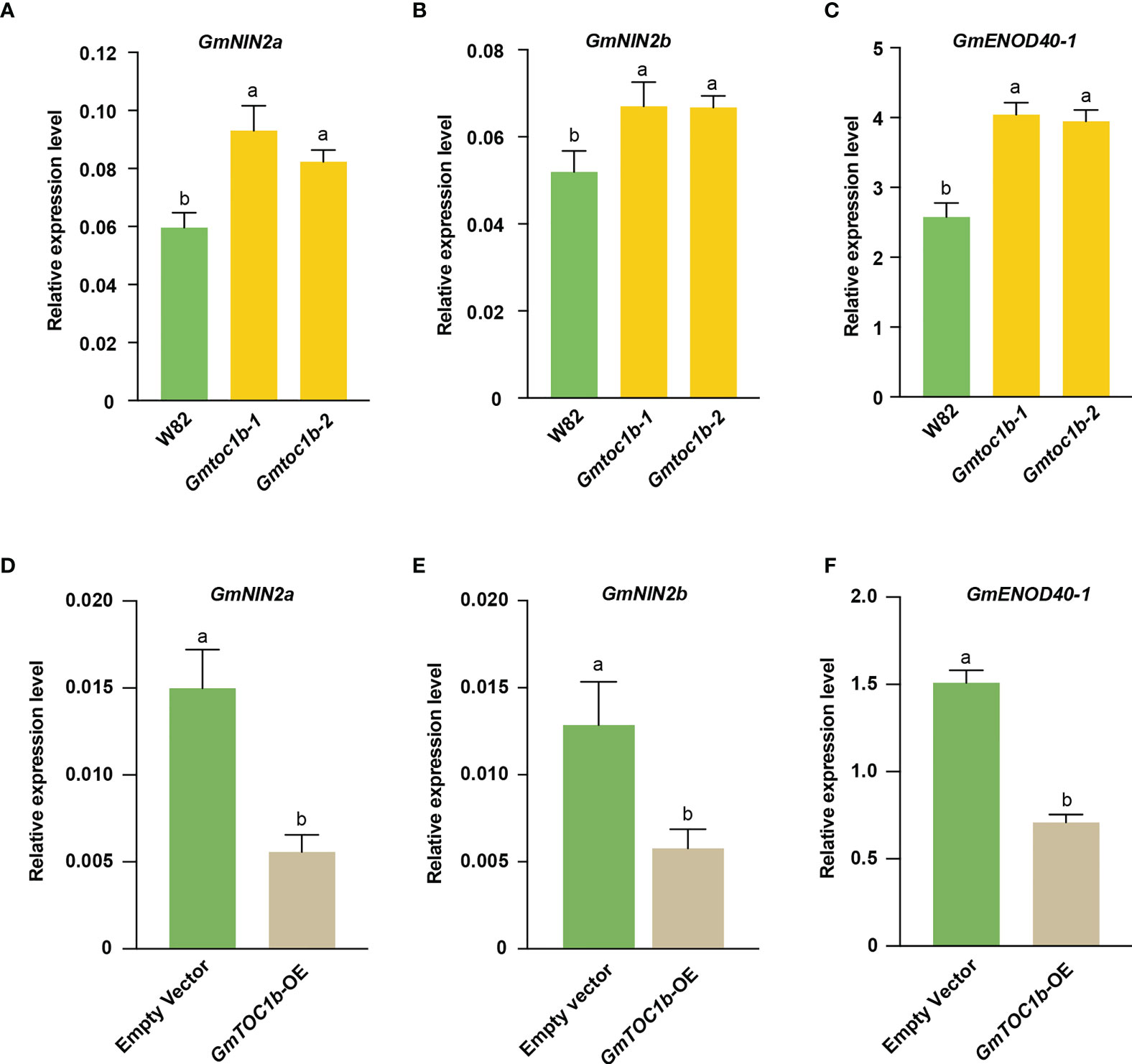
Figure 5 Expression of key nodulation-related genes in the early stage of rhizobia inoculation. Roots were harvested for analysis at 3 DAI. (A–C) Relative transcript levels of GmNIN2a, GmNIN2b, and GmENOD40-1 in the roots of W82 and Gmtoc1b mutants. (D–F) Relative transcript levels of GmNIN2a, GmNIN2b, and GmENOD40-1 in the roots of GmTOC1b-OE and empty vector control transgenic hairy roots. Relative expression level was calculated as the ratio of the expression value of the target gene to that of reference gene GmActin as an internal standard. Three biological replicates were performed in all experiments. Different lowercase letters indicate significant differences as determined by Student’s t-test.
GmTOC1b inhibits GmNIN2a and GmENOD40-1 transcription via binding to their promoters
Previous research showed that Arabidopsis TOC1 functions as a general transcriptional repressor (Gendron et al., 2012). Based on the lower expression of GmNIN2a, GmNIN2b, and GmENOD40-1 in transgenic hairy roots overexpressing GmTOC1b, we speculated that GmTOC1b may bind to the promoters of these genes during nodulation. To test this hypothesis, we first analyzed 2-kb fragments of the GmNIN2a, GmNIN2b, and GmENOD40-1 promoters to look for cis-elements bound by TOC1. We identified two typical HUD motifs in the GmNIN2a promoter (Michael et al., 2008a), one typical morning element motif, and three typical HUD motifs in the GmENOD40-1 promoter (Michael et al., 2008b), suggesting that GmTOC1b may bind to these promoters. We then performed a dual-luciferase transient expression assay using the GmNIN2a and GmENOD40-1 promoters individually driving the firefly luciferase (LUC) reporter gene together with an effector construct harboring GmTOC1b expressed from the soybean Ubiquitin (GmUBI) promoter (proGmUBI : GmTOC1B-3Flag) (Figure 6A). Co-infiltration of N. benthamiana leaves with the effector construct and each LUC reporter construct established that the presence of GmTOC1b significantly inhibits the LUC activity derived from the GmNIN2a and GmENOD40-1 promoters compared to the empty effector vector control (proGmUBI:3Flag). Together, these results indicate that GmTOC1b represses the transcription of GmNIN2a and GmENOD40-1, likely by binding to their promoters (Figures 6B–E).
Discussion
Symbiosis-mediated nitrogen fixation provides a large amount of nitrogen during soybean growth and reproduction (Pagano and Miransari, 2016). Therefore, the effect of nitrogen fixation is particularly important to soybean yield and quality. Importantly, the growth and proliferation of rhizobia require host-derived carbohydrates to support optimal utilization of nutrients, driving the co-evolution of a complex and precise regulation network between legumes and their rhizobial symbionts to modulate nodule number. Over the past 10 years, many key regulators of nodule formation have been reported (Kaló et al., 2005; Smit et al., 2005; Lim et al., 2011; Gamas et al., 2017; Suzaki and Nishida, 2019; Wang et al., 2019; Gao et al., 2021; He et al., 2021; Zhang et al., 2021). In soybean, components of the circadian clock, such as EARLY FLOWERING 3 (GmELF3), LATE ELONGATED HYPOCOTYL (GmLHY), GmPRR3, and LUX ARRHYTHMO (GmLUX), were shown to be involved in the regulation of growth and development or abiotic stress tolerance (Lu et al., 2017; Cheng et al., 2020; Lu et al., 2020; Bu et al., 2021; Dong et al., 2021a; Fang et al., 2021; Li et al., 2021; Wang et al., 2021a). Members of the clock components including LHY and LUX play roles in regulating nodulation in the model legume M. truncatula. However, an effect of the circadian clock on nodulation has not previously been reported in soybean. Our results indicated that GmTOC1b was highly expressed in nodules. Furthermore, GmTOC1b inhibited soybean nodulation by repressing the transcription of the key nodulation-related genes GmNIN2a, GmNIN2b, and GmENOD40-1, demonstrating that GmTOC1 is a transcriptional repressor.
GmTOC1b is predicted to contain the typical N-terminal PR domain and C-terminal CCT domain of pseudo-response regulators. Previous research indicated that TOC1 directly binds to cis-elements located in the promoters of target genes through its CCT domain. Indeed, transient overexpression of TOC1 in Arabidopsis significantly inhibits the transcription of LHY and CIRCADIAN CLOCK-ASSOCIATED 1 (CCA1), demonstrating that TOC1 can repress the transcription of its target genes via binding to their promoters (Gendron et al., 2012). We confirmed that GmTOC1b and AtTOC1 shared the highly conserved CCT domain, which agreed with the notion that GmTOC1b may bind to the promoters of target genes. In this study, we detected two or more TOC1-binding cis-elements in the promoter regions of GmNIN2a and GmENOD40-1. We further confirmed that GmTOC1b can repress their transcription, likely by directly binding to the GmNIN2a and GmENOD40-1 promoters (Figures 6B–E).
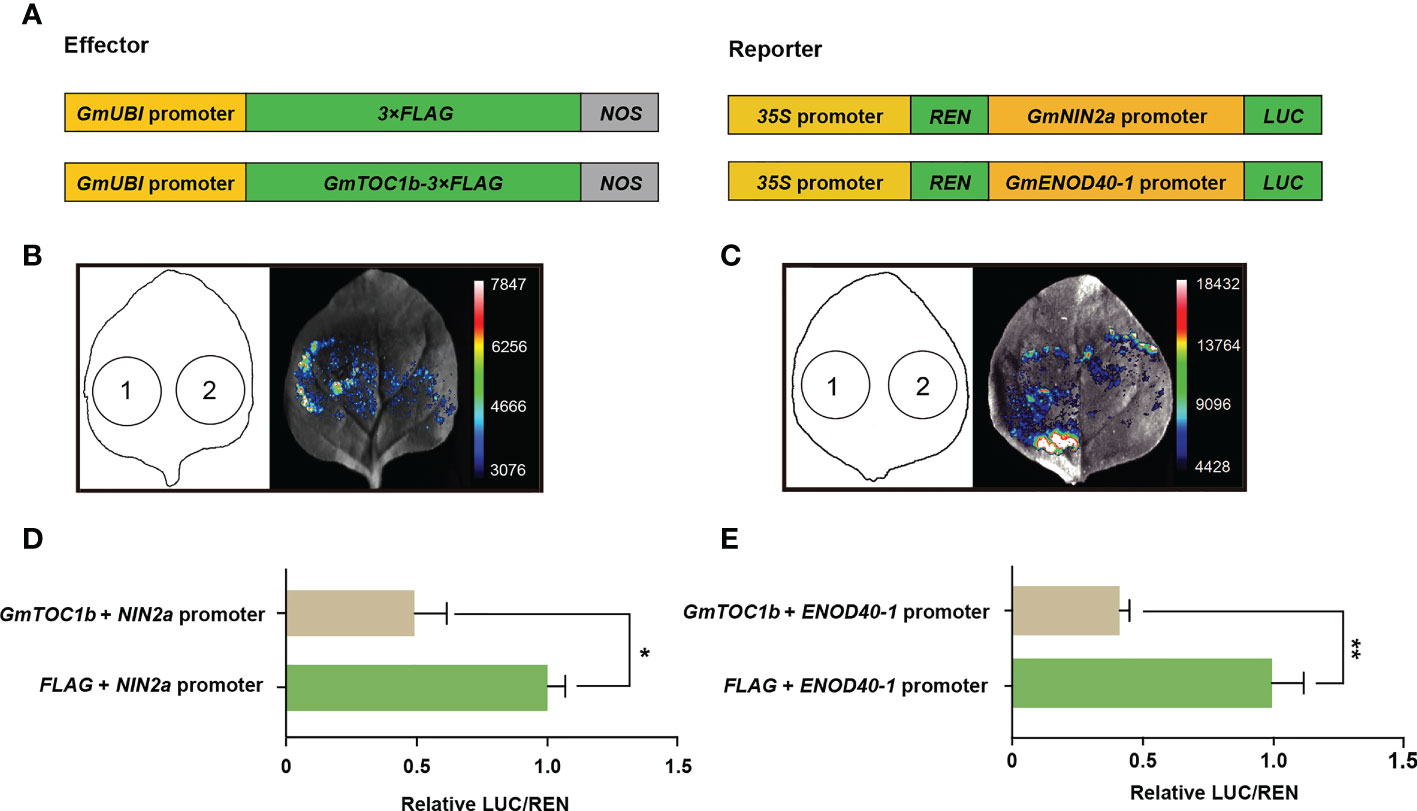
Figure 6 GmTOC1b represses the transcription of GmNIN2a and GmENOD40-1. (A) Schematic diagram of the constructs used for the transient co-transfection assay. (B) Representative image of firefly luciferase (LUC) activity driven by the GmNIN2a promoter. 1, proGmUBI:3×Flag + proGmNIN2a:LUC; 2, proGmUBI : GmTOC1b-3×Flag + proGmNIN2a:LUC. (C) Representative image of luciferase activity driven by the GmENOD40-1 promoter. 1, proGmUBI:3×Flag + proGmENOD40-1:LUC; 2, proGmUBI : GmTOC1b-3×Flag + proGmENOD40-1:LUC. (D, E) GmTOC1b represses GmNIN2a and GmENOD40-1 promoter activities. Relative firefly luciferase activity driven by the GmNIN2a (D) or GmENOD40-1 (E) promoter was normalized to CaMV 35S promoter-driven Renilla luciferase (REN) activity. Data are means ± SD of seven independent samples (*p < 0.05; **p < 0.01).
NINs and ENOD40 play a vital role in rhizobia symbiosis. The expression of their encoding genes is rapidly induced during nodulation, and their corresponding knockout mutants markedly reduced nodule number in both L. japonicus (Kumagai et al., 2006; Yoro et al., 2014) and M. truncatula (Marsh et al., 2007; Wan et al., 2007). A recent study suggested that light-induced GmSTFs and GmFTs move from shoots to roots, and GmFT2a directly interacts with GmCCaMK-phosphorylated GmSTF3 to form a complex, which directly activates the expression of NIN and nuclear factor Y to regulate nodulation (Wang et al., 2021b). Nodule Number Control 1 (GmNNC1) interacts with GmNINa to release the transcriptional repression of GmENON40 imposed by NNC1 to regulate nodulation (Wang et al., 2019). SQUAMOSA PROMOTER BINDING PROTEIN-LIKE 9 (GmSPL9d) was co-expressed with GmNINa and GmENOD40-1, and GmSPL9d positively regulated nodulation by inducing the transcription of GmNINa and GmENOD40-1 (Yun et al., 2022). RT-qPCR analysis of the Gmtoc1b mutants and GmTOC1b-OE transgenic hairy roots showed that the expression levels of GmNIN2a, GmNIN2b, and GmENOD40-1 were negatively regulated by GmTOC1b (Figures 5A–F). However, only the transcription of GmNIN2a and GmENOD40-1 was directly repressed by GmTOC1b, while the change in GmNIN2b expression could not be explained. Because many genes have been reported to regulate NIN expression in soybean, we speculate that GmTOC1b may indirectly regulate GmNIN2b expression by directly regulating other nodulation-related genes upstream of NIN, which needs to be explored in our future studies.
Data availability statement
The original contributions presented in the study are included in the article/Supplementary Material. Further inquiries can be directed to the corresponding authors.
Author contributions
YZ performed phenotypic observation and data analysis. QC performed the gene cloning and generation of Gmtoc1b mutant. CL and LL performed the subcellular localization. CG and ZC performed the soybean hairy root transformation. YW performed the transient expression assay. BL, FK, and LC wrote the manuscript. All authors contributed to the article and approved the submitted version.
Funding
This work was supported by the National Natural Science Foundation of China (32001502, 32090064), the Major Program of Guangdong Basic and Applied Research 2019B030302006, and the China Postdoctoral Science Foundation (2020M682655).
Conflict of interest
The authors declare that the research was conducted in the absence of any commercial or financial relationships that could be construed as a potential conflict of interest.
Publisher’s note
All claims expressed in this article are solely those of the authors and do not necessarily represent those of their affiliated organizations, or those of the publisher, the editors and the reviewers. Any product that may be evaluated in this article, or claim that may be made by its manufacturer, is not guaranteed or endorsed by the publisher.
Supplementary material
The Supplementary Material for this article can be found online at: https://www.frontiersin.org/articles/10.3389/fpls.2022.1052017/full#supplementary-material
Supplementary Figure 1 | Generation and identification of the Gmtoc1b mutants. (A) Sequencing verification of CRISPR/Cas9-edited Gmtoc1b-1 and Gmtoc1b-2 mutants. (B) Relative GmTOC1b transcript levels in W82, Gmtoc1b-1, and Gmtoc1b-2 mutants. Three biological replicates were performed in all experiments. All data were normalized to the transcript level of reference gene GmActin.
Supplementary Figure 2 | Knockout of GmTOC1b increases small nodule numbers at 7 DAI. (A) Representative phenotype of small nodules observed in W82 and the Gmtoc1b mutants at 7 DAI. Scale bars, 100 mm. (B) Number of small nodules in W82 and the Gmtoc1b mutants at 7 DAI (n = 5).
Supplementary Figure 3 | Content of leghemoglobin in nodules from W82 and Gmtoc1b mutants. Data are means ± SD of three independent samples. Lowercase letters indicate significant differences as determined by Student’s t-test.
References
Achom, M., Roy, P., Lagunas, B., Picot, E., Richards, L., Bonyadi-Pour, R., et al. (2021). Plant circadian clock control of Medicago truncatula nodulation via regulation of nodule cysteine-rich peptides. J. Exp. Bot. 73 (7), 2142–2156. doi: 10.1093/jxb/erab526
Bailey, T. L., Johnson, J., Grant, C. E., Noble, W. S. (2015). The MEME suite. Nucleic Acids Res. 43 (W1), W39–W49. doi: 10.1093/nar/gkv416
Berger, A., Guinand, S., Boscari, A., Puppo, A., Brouquisse, R. (2020). Medicago truncatula phytoglobin 1.1 controls symbiotic nodulation and nitrogen fixation via the regulation of nitric oxide concentration. New Phytol. 227, 84–98. doi: 10.1111/nph.16462
Bu, T., Lu, S., Wang, K., Dong, L., Li, S., Xie, Q., et al. (2021). A critical role of the soybean evening complex in the control of photoperiod sensitivity and adaptation. Proc. Natl. Acad. Sci. 118 (8), e2010241118. doi: 10.1073/pnas.2010241118
Campalans, A., Kondorosi, A., Crespi, M. (2004). Enod40, a short open reading frame–containing mRNA, induces cytoplasmic localization of a nuclear RNA binding protein in Medicago truncatula. Plant Cell 16 (4), 1047–1059. doi: 10.1105/tpc.019406
Cerri, M. R., Frances, L., Laloum, T., Auriac, M.-C., Niebel, A., Oldroyd, G. E., et al. (2012). Medicago truncatula ERN transcription factors: regulatory interplay with NSP1/NSP2 GRAS factors and expression dynamics throughout rhizobial infection. Plant Physiol. 160 (4), 2155–2172. doi: 10.1104/pp.112.203190
Cerri, M. R., Wang, Q., Stolz, P., Folgmann, J., Frances, L., Katzer, K., et al. (2017). The ERN1 transcription factor gene is a target of the CCaMK/CYCLOPS complex and controls rhizobial infection in Lotus japonicus. New Phytol. 215 (1), 323–337. doi: 10.1111/nph.14547
Chen, L., Cai, Y., Liu, X., Yao, W., Guo, C., Sun, S., et al. (2018). Improvement of soybean Agrobacterium-mediated transformation efficiency by adding glutamine and asparagine into the culture media. Int. J. Mol. Sci. 19 (10), 3039. doi: 10.3390/ijms19103039
Cheng, Q., Gan, Z., Wang, Y., Lu, S., Hou, Z., Li, H., et al. (2020). The soybean gene J contributes to salt stress tolerance by up-regulating salt-responsive genes. Front. Plant Sci. 11. doi: 10.3389/fpls.2020.00272
Ciampitti, I. A., Salvagiotti, F. (2018). New insights into soybean biological nitrogen fixation. Agron. J. 110 (4), 1185–1196. doi: 10.2134/agronj2017.06.0348
Crespi, M., Jurkevitch, E., Poiret, M., d'Aubenton-Carafa, Y., Petrovics, G., Kondorosi, E., et al. (1994). Enod40, a gene expressed during nodule organogenesis, codes for a non-translatable RNA involved in plant growth. EMBO J. 13 (21), 5099–5112. doi: 10.1002/j.1460-2075.1994.tb06839.x
Demchenko, K., Winzer, T., Stougaard, J., Parniske, M., Pawlowski, K. (2004). Distinct roles of Lotus japonicus SYMRK and SYM15 in root colonization and arbuscule formation. New Phytol. 163 (2), 381–392. doi: 10.1111/j.1469-8137.2004.01123.x
Dong, L., Fang, C., Cheng, Q., Su, T., Kou, K., Kong, L., et al. (2021a). Genetic basis and adaptation trajectory of soybean from its temperate origin to tropics. Nat. Commun. 12 (1), 1–11. doi: 10.1038/s41467-021-25800-3
Dong, W., Zhu, Y., Chang, H., Wang, C., Yang, J., Shi, J., et al. (2021b). An SHR-SCR module specifies legume cortical cell fate to enable nodulation. Nature 589 (7843), 586–590. doi: 10.1038/s41586-020-3016-z
Du, M., Gao, Z., Li, X., Liao, H. (2020). Excess nitrate induces nodule greening and reduces transcript and protein expression levels of soybean leghaemoglobins. Ann. Bot. 126 (1), 61–72. doi: 10.1093/aob/mcaa002
Fang, C., Liu, J., Zhang, T., Su, T., Li, S., Cheng, Q., et al. (2021). A recent retrotransposon insertion of J caused E6 locus facilitating soybean adaptation into low latitude. J. Integr. Plant Biol. 63 (6), 995–1003. doi: 10.1111/jipb.13034
Gamas, P., Brault, M., Jardinaud, M.-F., Frugier, F. (2017). Cytokinins in symbiotic nodulation: when, where, what for? Trends Plant Sci. 22 (9), 792–802. doi: 10.1016/j.tplants.2017.06.012
Gan, Z., Shi, W., Li, Y., Hou, Z., Li, H., Cheng, Q., et al. (2020). Identification of CRISPR/Cas9 knockout targets and tissue expression analysis of circadian clock genes GmLNK1/2, GmRVE4/8 and GmTOC1 in soybean. Acta Agronomica Sin. 46 (8), 1291–1300. doi: 10.3724/sp.J.1006.2020.94169
Gao, Z., Chen, Z., Cui, Y., Ke, M., Xu, H., Xu, Q., et al. (2021). GmPIN-dependent polar auxin transport is involved in soybean nodule development. Plant Cell 33 (9), 2981–3003. doi: 10.1093/plcell/koab183
Gelfand, I., Philip Robertson, G. (2015). A reassessment of the contribution of soybean biological nitrogen fixation to reactive n in the environment. Biogeochemistry 123 (1), 175–184. doi: 10.1007/s10533-014-0061-4
Gendron, J. M., Pruneda-Paz, J. L., Doherty, C. J., Gross, A. M., Kang, S. E., Kay, S. A. (2012). Arabidopsis circadian clock protein, TOC1, is a DNA-binding transcription factor. Proc. Natl. Acad. Sci. 109 (8), 3167–3172. doi: 10.1073/pnas.1200355109
Hao, Y., Zong, W., Zeng, D., Han, J., Chen, S., Tang, J., et al. (2020). Shortened snRNA promoters for efficient CRISPR/Cas-based multiplex genome editing in monocot plants. Sci. China-Life Sci. 63, 933–935. doi: 10.1007/s11427-019-1612-6
Hayashi, T., Shimoda, Y., Sato, S., Tabata, S., Imaizumi-Anraku, H., Hayashi, M. (2014). Rhizobial infection does not require cortical expression of upstream common symbiosis genes responsible for the induction of Ca(2+) spiking. Plant J. 77 (1), 146–159. doi: 10.1111/tpj.12374
Heckmann, A. B., Sandal, N., Bek, A. S., Madsen, L. H., Jurkiewicz, A., Nielsen, M. W., et al. (2011). Cytokinin induction of root nodule primordia in Lotus japonicus is regulated by a mechanism operating in the root cortex. Mol. Plant-Microbe Interact. 24 (11), 1385–1395. doi: 10.1094/MPMI-05-11-0142
He, C., Gao, H., Wang, H., Guo, Y., He, M., Peng, Y., et al. (2021). GSK3-mediated stress signaling inhibits legume–rhizobium symbiosis by phosphorylating GmNSP1 in soybean. Mol. Plant 14 (3), 488–502. doi: 10.1016/j.molp.2020.12.015
Hungria, M., Franchini, J., Campo, R., Graham, P. (2005). “The importance of nitrogen fixation to soybean cropping in south America,” in Nitrogen fixation in agriculture, forestry, ecology, and the environment (Springer), 4, 25–42. doi: 10.1007/1-4020-3544-6_3
Indrasumunar, A., Kereszt, A., Searle, I., Miyagi, M., Li, D., Nguyen, C. D., et al. (2010). Inactivation of duplicated nod factor receptor 5 (NFR5) genes in recessive loss-of-function non-nodulation mutants of allotetraploid soybean (Glycine max l. merr.). Plant Cell Physiol. 51 (2), 201–214. doi: 10.1093/pcp/pcp178
Indrasumunar, A., Searle, I., Lin, M. H., Kereszt, A., Men, A., Carroll, B. J., et al. (2011). Nodulation factor receptor kinase 1α controls nodule organ number in soybean (Glycine max l. merr). Plant J. 65 (1), 39–50. doi: 10.1111/j.1365-313X.2010.04398.x
Indrasumunar, A., Wilde, J., Hayashi, S., Li, D., Gresshoff, P. M. (2015). Functional analysis of duplicated symbiosis receptor kinase (SymRK) genes during nodulation and mycorrhizal infection in soybean (Glycine max). J. Plant Physiol. 176, 157–168. doi: 10.1016/j.jplph.2015.01.002
Jiang, S., Jardinaud, M., GAO, J., Pecrix, Y., Wen, J., Mysore, K., et al. (2021). NIN-like protein transcription factors regulate leghemoglobin genes in legume nodules. Science 374 (6567), 625–628. doi: 10.1126/science.abg5945
Kaló, P., Gleason, C., Edwards, A., Marsh, J., Mitra, R. M., Hirsch, S., et al. (2005). Nodulation signaling in legumes requires NSP2, a member of the GRAS family of transcriptional regulators. Science 308 (5729), 1786–1789. doi: 10.1126/science.1110951
Kawaharada, Y., James, E. K., Kelly, S., Sandal, N., Stougaard, J. (2017). The ethylene responsive factor required for nodulation 1 (ERN1) transcription factor is required for infection-thread formation in Lotus japonicus. Mol. Plant-Microbe Interact. 30 (3), 194–204. doi: 10.1094/MPMI-11-16-0237-R
Kereszt, A., Li, D., Indrasumunar, A., Nguyen, C. D., Nontachaiyapoom, S., Kinkema, M., et al. (2007). Agrobacterium rhizogenes-mediated transformation of soybean to study root biology. Nat. Protoc. 2 (4), 948–952. doi: 10.1038/nprot.2007.141
Kong, Y., Han, L., Liu, X., Wang, H., Wen, L., Yu, X., et al. (2020). The nodulation and nyctinastic leaf movement is orchestrated by clock gene LHY in Medicago truncatula. J. Integr. Plant Biol. 62 (12), 1880–1895. doi: 10.1111/jipb.12999
Kong, Y., Zhang, Y., Liu, X., Meng, Z., Yu, X., Zhou, C., et al. (2022). The conserved and specific roles of the LUX arrhythmo in circadian clock and nodulation. Int. J. Mol. Sci. 23 (7), 3473. doi: 10.3390/ijms23073473
Kumagai, H., Kinoshita, E., Ridge, R. W., Kouchi, H. (2006). RNAi knock-down of ENOD40s leads to significant suppression of nodule formation in Lotus japonicus. Plant Cell Physiol. 47 (8), 1102–1111. doi: 10.1093/pcp/pcj081
Legnaioli, T., Cuevas, J., Mas, P. (2009). TOC1 functions as a molecular switch connecting the circadian clock with plant responses to drought. EMBO J. 28 (23), 3745–3757. doi: 10.1038/emboj.2009.297
Li, Z., Cheng, Q., Gan, Z., Hou, Z., Zhang, Y., Li, Y., et al. (2021). Multiplex CRISPR/Cas9-mediated knockout of soybean LNK2 advances flowering time. Crop J. 9 (4), 767–776. doi: 10.1016/j.cj.2020.09.005
Lim, C. W., Lee, Y. W., Hwang, C. H. (2011). Soybean nodule-enhanced CLE peptides in roots act as signals in GmNARK-mediated nodulation suppression. Plant Cell Physiol. 52 (9), 1613–1627. doi: 10.1093/pcp/pcr091
Limpens, E., Mirabella, R., Fedorova, E., Franken, C., Franssen, H., Bisseling, T., et al. (2005). Formation of organelle-like N2-fixing symbiosomes in legume root nodules is controlled by DMI2. Proc. Natl. Acad. Sci. 102 (29), 10375–10380. doi: 10.1073/pnas.0504284102
Liu, C. W., Murray, J. D. (2016). The role of flavonoids in nodulation host-range specificity: an update. Plants (Basel) 5 (3), 33. doi: 10.3390/plants5030033
Liu, J., Rutten, L., Limpens, E., van der Molen, T., Van Velzen, R., Chen, R., et al. (2019). A remote cis-regulatory region is required for NIN expression in the pericycle to initiate nodule primordium formation in Medicago truncatula. Plant Cell 31 (1), 68–83. doi: 10.1105/tpc.18.00478
Lu, S., Dong, L., Fang, C., Liu, S., Kong, L., Cheng, Q., et al. (2020). Stepwise selection on homeologous PRR genes controlling flowering and maturity during soybean domestication. Nat. Genet. 52 (4), 428–436. doi: 10.1038/s41588-020-0604-7
Lu, S., Zhao, X., Hu, Y., Liu, S., Nan, H., Li, X., et al. (2017). Natural variation at the soybean J locus improves adaptation to the tropics and enhances yield. Nat. Genet. 49 (5), 773. doi: 10.1038/ng.3819
Marsh, J. F., Rakocevic, A., Mitra, R. M., Brocard, L., Sun, J., Eschstruth, A., et al. (2007). Medicago truncatula NIN is essential for rhizobial-independent nodule organogenesis induced by autoactive calcium/calmodulin-dependent protein kinase. Plant Physiol. 144 (1), 324–335. doi: 10.1104/pp.106.093021
Michael, T. P., Breton, G., Hazen, S. P., Priest, H., Mockler, T. C., Kay, S. A., et al. (2008a). A morning-specific phytohormone gene expression program underlying rhythmic plant growth. PloS Biol. 6 (9), e225. doi: 10.1371/journal.pbio.0060225
Michael, T. P., Mockler, T. C., Breton, G., McEntee, C., Byer, A., Trout, J. D., et al. (2008b). Network discovery pipeline elucidates conserved time-of-day-specific cis-regulatory modules. PloS Genet. 4 (2), e14. doi: 10.1371/journal.pgen.0040014
Pagano, M. C., Miransari, M. (2016). “The importance of soybean production worldwide,” in Abiotic and biotic stresses in soybean production (ACADEMIC PRESS), 1–26. doi: 10.1016/B978-0-12-801536-0.00001-3
Rival, P., De Billy, F., Bono, J.-J., Gough, C., Rosenberg, C., Bensmihen, S. (2012). Epidermal and cortical roles of NFP and DMI3 in coordinating early steps of nodulation in Medicago truncatula. Development 139 (18), 3383–3391. doi: 10.1242/dev.081620
Röhrig, H., Schmidt, J., Miklashevichs, E., Schell, J., John, M. (2002). Soybean ENOD40 encodes two peptides that bind to sucrose synthase. Proc. Natl. Acad. Sci. 99 (4), 1915–1920. doi: 10.1073/pnas.022664799
Sanchez, A., Shin, J., Davis, S. J. (2011). Abiotic stress and the plant circadian clock. Plant Signaling Behav. 6 (2), 223–231. doi: 10.4161/psb.6.2.14893
Santachiara, G., Salvagiotti, F., Rotundo, J. L. (2019). Nutritional and environmental effects on biological nitrogen fixation in soybean: A meta-analysis. Field Crops Res. 240, 106–115. doi: 10.1016/j.fcr.2019.05.006
Scheiermann, C., Kunisaki, Y., Frenette, P. S. (2013). Circadian control of the immune system. Nat. Rev. Immunol. 13 (3), 190–198. doi: 10.1038/nri3386
Schipanski, M., Drinkwater, L., Russelle, M. (2010). Understanding the variability in soybean nitrogen fixation across agroecosystems. Plant Soil 329 (1), 379–397. doi: 10.1007/s11104-009-0165-0
Smit, P., Raedts, J., Portyanko, V., Debellé, F., Gough, C., Bisseling, T., et al. (2005). NSP1 of the GRAS protein family is essential for rhizobial nod factor-induced transcription. Science 308 (5729), 1789–1791. doi: 10.1126/science.1111025
Suzaki, T., Kawaguchi, M. (2014). Root nodulation: a developmental program involving cell fate conversion triggered by symbiotic bacterial infection. Curr. Opin. Plant Biol. 21, 16–22. doi: 10.1016/j.pbi.2014.06.002
Suzaki, T., Nishida, H. (2019). Autoregulation of legume nodulation by sophisticated transcriptional regulatory networks. Mol. Plant 12 (9), 1179–1181. doi: 10.1016/j.molp.2019.07.008
Svistoonoff, S., Sy, M.-O., Diagne, N., Barker, D. G., Bogusz, D., Franche, C. (2010). Infection-specific activation of the Medicago truncatula ENOD11 early nodulin gene promoter during actinorhizal root nodulation. Mol. Plant-Microbe Interact. 23 (6), 740–747. doi: 10.1094/MPMI-23-6-0740
Tamura, K., Stecher, G., Kumar, S. (2021). MEGA11: Molecular evolutionary genetics analysis version 11. Mol. Biol. Evol. 38 (7), 3022–3027. doi: 10.1093/molbev/msab120
Wang, K., Bu, T., Cheng, Q., Dong, L., Su, T., Chen, Z., et al. (2021a). Two homologous LHY pairs negatively control soybean drought tolerance by repressing the abscisic acid responses. New Phytol. 229 (5), 2660–2675. doi: 10.1111/nph.17019
Wang, L., Deng, L., Bai, X., Jiao, Y., Cao, Y., Wu, Y. (2020b). Regulation of nodule number by GmNORK is dependent on expression of GmNIC in soybean. Agroforestry Syst. 94 (1), 221–230. doi: 10.1007/s10457-019-00382-8
Wang, T., Guo, J., Peng, Y., Lyu, X., Liu, B., Sun, S., et al. (2021b). Light-induced mobile factors from shoots regulate rhizobium-triggered soybean root nodulation. Science 374 (6563), 65–71. doi: 10.1126/science.abh2890
Wang, F., Han, T., Song, Q., Ye, W., Song, X., Chu, J., et al. (2020a). The rice circadian clock regulates tiller growth and panicle development through strigolactone signaling and sugar sensing. Plant Cell 32 (10), 3124–3138. doi: 10.1105/tpc.20.00289
Wang, L., Sun, Z., Su, C., Wang, Y., Yan, Q., Chen, J., et al. (2019). A GmNINa-miR172c-NNC1 regulatory network coordinates the nodulation and autoregulation of nodulation pathways in soybean. Mol. Plant 12 (9), 1211–1226. doi: 10.1016/j.molp.2019.06.002
Wan, X., Hontelez, J., Lillo, A., Guarnerio, C., van de Peut, D., Fedorova, E., et al. (2007). Medicago truncatula ENOD40-1 and ENOD40-2 are both involved in nodule initiation and bacteroid development. J. Exp. Bot. 58 (8), 2033–2041. doi: 10.1093/jxb/erm072
Xu, H., Li, Y., Zhang, K., Li, M., Fu, S., Tian, Y., et al. (2021). miR169c-NFYA-C-ENOD40 modulates nitrogen inhibitory effects in soybean nodulation. New Phytol. 229 (6), 3377–3392. doi: 10.1111/nph.17115
Xu, X., Yuan, L., Yang, X., Zhang, X., Wang, L., Xie, Q. (2022). Circadian clock in plants: Linking timing to fitness. J. Integr. Plant Biol. 64 (4), 792–811. doi: 10.1111/jipb.13230
Yoro, E., Suzaki, T., Toyokura, K., Miyazawa, H., Fukaki, H., Kawaguchi, M. (2014). A positive regulator of nodule organogenesis, NODULE INCEPTION, acts as a negative regulator of rhizobial infection in Lotus japonicus. Plant Physiol. 165 (2), 747–758. doi: 10.1104/pp.113.233379
Yun, J., Sun, Z., Jiang, Q., Wang, Y., Wang, C., Luo, Y., et al. (2022). The miR156b-GmSPL9d module modulates nodulation by targeting multiple core nodulation genes in soybean. New Phytol. 233 (4), 1881–1899. doi: 10.1111/nph.17899
Zhang, B., Wang, M., Sun, Y., Zhao, P., Liu, C., Qing, K., et al. (2021). Glycine max NNL1 restricts symbiotic compatibility with widely distributed bradyrhizobia via root hair infection. Nat. Plants 7 (1), 73–86. doi: 10.1038/s41477-020-00832-7
Keywords: soybean, nodulation, TOC1, NIN, ENOD40
Citation: Zhang Y, Cheng Q, Liao C, Li L, Gou C, Chen Z, Wang Y, Liu B, Kong F and Chen L (2022) GmTOC1b inhibits nodulation by repressing GmNIN2a and GmENOD40-1 in soybean. Front. Plant Sci. 13:1052017. doi: 10.3389/fpls.2022.1052017
Received: 23 September 2022; Accepted: 25 October 2022;
Published: 11 November 2022.
Edited by:
Zhengjun Xia, Chinese Academy of Sciences (CAS), ChinaReviewed by:
Dawei Xin, Northeast Agricultural University, ChinaPascal Ratet, UMR9213 Institut des Sciences des Plantes de Paris Saclay (IPS2), France
Copyright © 2022 Zhang, Cheng, Liao, Li, Gou, Chen, Wang, Liu, Kong and Chen. This is an open-access article distributed under the terms of the Creative Commons Attribution License (CC BY). The use, distribution or reproduction in other forums is permitted, provided the original author(s) and the copyright owner(s) are credited and that the original publication in this journal is cited, in accordance with accepted academic practice. No use, distribution or reproduction is permitted which does not comply with these terms.
*Correspondence: Liyu Chen, Y2hlbmxpeXUxNzE1QGd6aHUuZWR1LmNu; Fanjiang Kong, a29uZ2ZqQGd6aHUuZWR1LmNu
†These authors have contributed equally to this work