- 1Key Laboratory of Desert and Desertification, Northwest Institute of Eco-Environment and Resources, Chinese Academy of Sciences, Lanzhou, China
- 2College of Resources and Environment, University of Chinese Academy of Sciences, Beijing, China
- 3School of Geography and Ocean Science, Nanjing University, Nanjing, China
- 4Management and Protection Centre of Gansu Qilianshan National Nature Reserve, Zhangye, China
Radial growth is influenced by the local environment, regional climate, and tree species. Assessing the influence of these variables on radial growth can help to reveal the relationships between tree growth and the environment. Here, we used standard dendrochronological approach to explore the response of radial growth to climate factors. We reported ring-width (TRW) residual chronologies from five sites along a longitudinal gradient in the Hexi area, arid northwestern China, based on a total of 249 Qinghai spruce (Picea crassifolia) ring-width records. We found that Qinghai spruce in the west of the Hexi area is more sensitive to climate change than in the east, and that drought condition in the previous growing season and the early growing season (March to June) limits spruce growth. Comparison between the regional standard chronologies of Qinghai spruce and Qilian juniper (Juniperus przewalskii) in the Hexi area during 1813-2001 showed that both chronologies were more consistent in the high-frequency domain than in the low-frequency domain. The findings emphasize the impacts of local environment, regional climate and tree species on radial growth, suggesting that accounting for these variables could improve large-scale and multi-species dendrochronological studies.
Introduction
Radial growth is affected by environmental variables, including climatic factors such as temperature and precipitation (Fritts, 1976). The effects of climate change on radial growth are complex, due to spatial heterogeneity and varied responses between different tree species (Graumlich, 1993; Camarero et al., 2018; Wang and Yang, 2021). Furthermore, radial growth trends of the same tree species can be spatially variable (Danek et al., 2017; Song et al., 2020), reflecting local environmental differences such as slope, elevation, and moisture gradients (Liang et al., 2006; Kharal et al., 2014; Touchan et al., 2016; Klippel et al., 2017; Griesbauer et al., 2021; Du et al., 2022; Guerrero-Hernandez et al., 2022). However, the influences of local environment, regional climate and different tree species on radial growth are often neglected in large-scale and multi-species dendroclimatic or biomass-modeling studies. (Roy and Ravan, 1996; Cook et al., 2010).
The Hexi area is a typical arid to semi-arid region of northwest China. Forests on the mountains to the north and south of the Hexi area have the functions of water conservation, soil conservation and climate regulation (Liu et al., 2016; Deng et al., 2017). Here, Qinghai spruce (Picea crassifolia Kom) and Qilian juniper (Sabina przewalskii Kom) are endemic tree species that are both sensitive to climate change (Chen et al., 2017; Yang et al., 2021). Previous tree-ring studies of Qinghai spruce and Qilian juniper have focused on regional climate reconstruction (Yang et al., 2014; Gou et al., 2015; Yang et al., 2019; Zhang et al., 2021). Several previous studies in the Hexi area have revealed the spatial variability of radial growth along elevation gradients (Zhang and Wilmking, 2010; Gao et al., 2013; Zhang et al., 2017; Wang et al., 2019) and slopes (Liang et al., 2006; Gao, 2011). These studies suggest that climate is the main factor leading to different radial growth patterns at different sites. Most comparative studies on Qinghai spruce and Qilian juniper have focused on the differences in the response of the two species to climatic factors (Liang et al., 2006; Ran et al., 2021). However, it is not clear how climate change affects Qinghai spruce radial growth from east to west along the Hexi area. In addition, the question of consistency between the climatic signals recorded in the standard chronologies of Qilian juniper and Qinghai spruce around the Hexi area on various timescales also remains unresolved. Therefore, it is crucial to explore the effects of local environment, regional climate and tree species on the radial growth in the Hexi area.
In this study, we collected Qinghai spruce samples along an east-west transect along the Hexi area, established and compared five residual chronologies, and produced a regional Qinghai spruce residual chronology to determine the primary factors limiting radial growth in this area. Finally, we compared the regional Qinghai spruce standard chronology with a regional Qilian juniper standard chronology on multiple timescales from 1813-2001. Our study aimed to identify: (i) the main factor limiting radial growth of Qinghai spruce in the Hexi area, (ii) differences in Qinghai spruce radial growth along the east-west transect; and (iii) similarities and differences in the standard chronologies of the two dominant species (Qinghai spruce and Qilian juniper) in the Hexi area from 1813-2001.
Materials and methods
Study area
The study area covers a large area of Hexi area, in arid northwestern China (Figure 1). The Qinghai spruce sampling sites span a distance of about 1000 km from east to west. This area is characterized by a temperate continental climate. The main tree species are Qinghai spruce (Picea crassifolia Kom) which grow in cloudy climates on slopes between 2600 and 3100 m a.s.l (http://www.iplant.cn/frps), and Qilian juniper (Sabina przewalskii Kom) which grow in open stands on south-facing slopes between 3000 and 3500 m a.s.l (Chen et al., 2011; Yang et al., 2011). The forest soil is mainly mountain grey cinnamon soil (Chen et al., 2011).
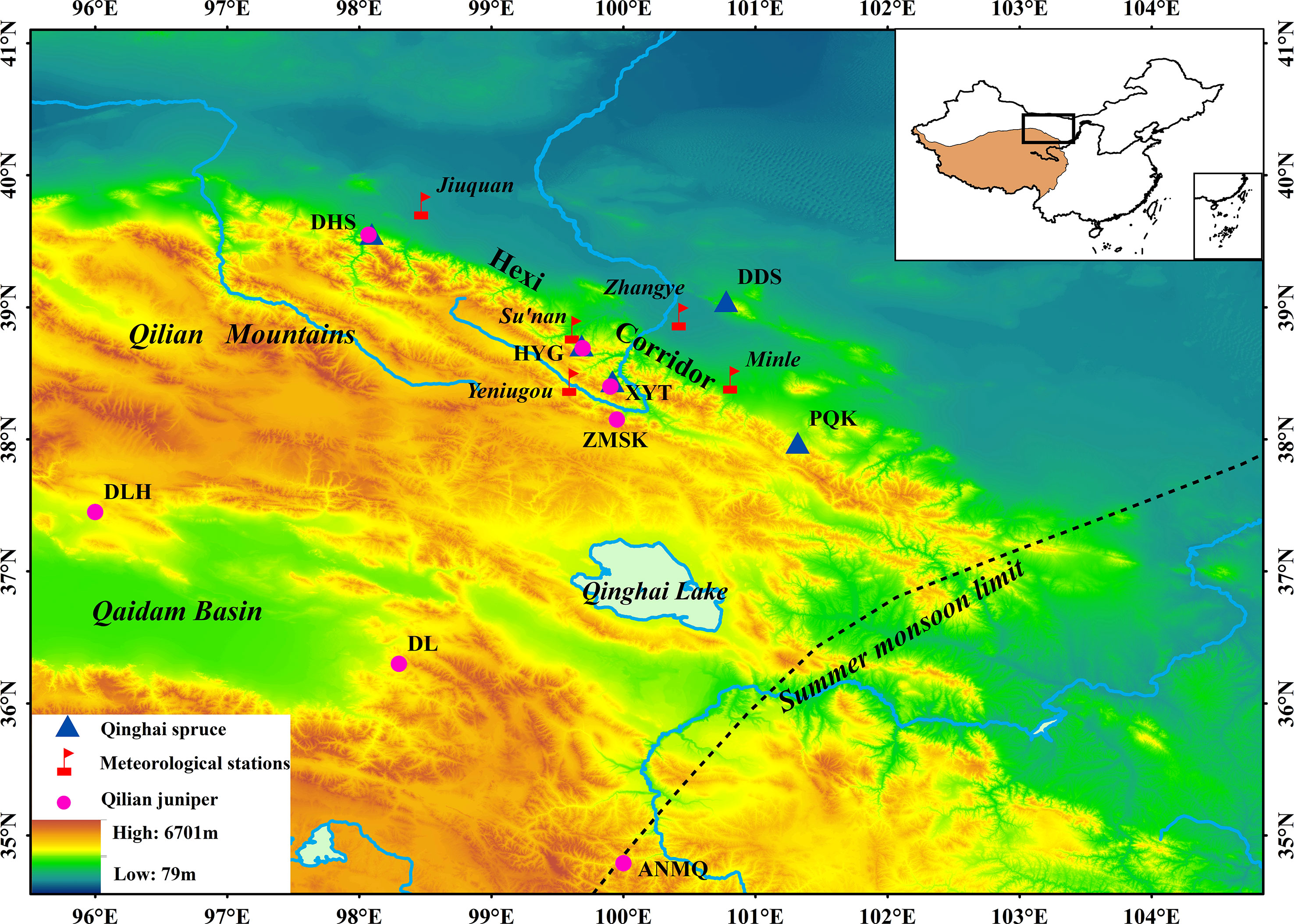
Figure 1 Locations of Qinghai spruce sampling sites (triangles), Qilian juniper sampling sites (circles) and meteorological stations (flags). For Qilian juniper site information, refer to Yang et al. (2010).
Sampling and chronology development
A total of 472 tree cores from 249 living Qinghai spruce were collected at five sampling sites (Figure 1 and Table S1). Tree-ring widths (TRW) were measured using the LINTAB II platform (Germany) with an accuracy of 0.01 mm, and all the measurement series were cross-dated with TSAP software (Rinn, 2003). The quality-check of the cross-dated series was performed using the COFECHA software (Holmes, 1983).
The ARSTAN software was used to develop the ring-width chronologies (Cook, 1985). To remove the inherent biological age trend from the ring-width sequences, we calculated differences between the raw data and an exponential or linear growth curve. The data-adaptive power transformation was used to reduce the potential influence of outliers in the raw data (Cook and Peters, 1997). The de-trended tree-ring indices were used to establish the chronologies by using a robust bi-weighted estimate of the mean (Cook and Kairiukstis, 1990). We used the method described by Osborn et al. (1997) to stabilize the variance before calculating the final chronologies. Finally, both the standard (STD) (Figure S1) and residual (RES) (Figure 2) chronologies were produced. The STD retains low-order persistence, which is routinely used for climate reconstruction to facilitate comparison between various regions. The RES is a pre-whitened chronology in which significant persistence has been removed (Cook, 1985).
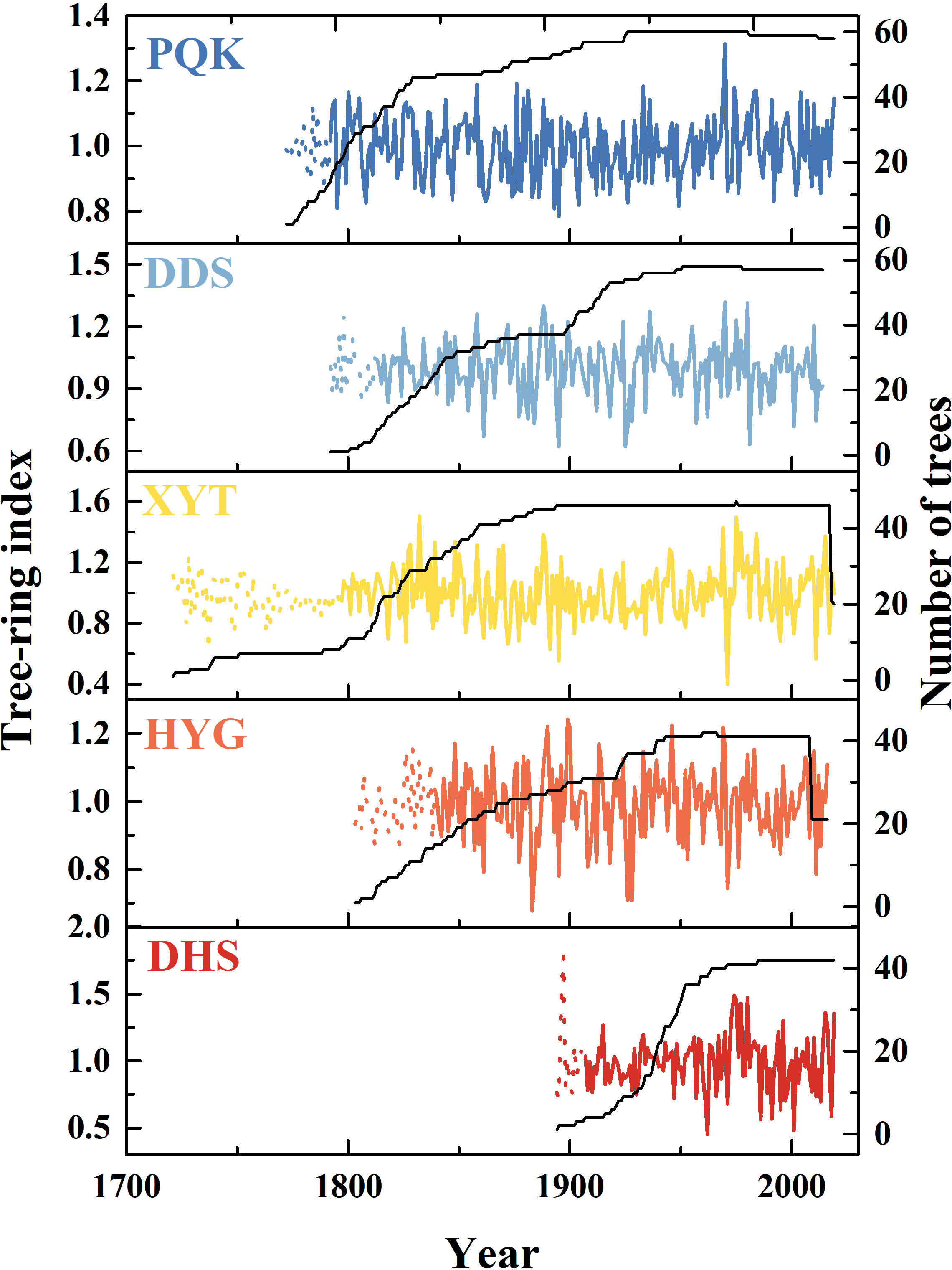
Figure 2 Residual chronologies and sample depths used in the study. Solid lines indicate EPS>0.85 and dotted lines indicate EPS<0.85.
The expressed population signal (EPS) and the mean inter-series correlation (Rbar) were calculated to determine the statistically-reliable periods of the STD and RES chronologies. A 30-year moving window with 15-year overlaps was used. We selected 0.85 as the thresholds of EPS to evaluate the reliable portion of the STD and RES chronologies (Wigley et al., 1984).
Meteorological data and statistical analysis
Monthly temperature, precipitation and relative humidity data from 1960 to 2014 were acquired from the meteorological stations (Minle, Zhangye, Yeniugou, Sunan, Jiuquan) nearest to the respective sampling sites. The self-calibrating Palmer drought severity index (scPDSI; Van der Schrier et al., 2013) is a modified variant of the Palmer drought severity index (PDSI; Palmer, 1965). We used an average series of four half-degree scPDSI grid points close to our sampling site to represent the regional moisture conditions over the period from 1960-2014.
First-order differenced climate series retain the interannual variability of climate factors. Correlation coefficients between the residual chronologies and the first-order differenced monthly values of temperature, precipitation, relative humidity and the scPDSI were calculated from 1961-2014 using the DENDROCLIM2002 software, this uses bootstrapping to assess the significance and stability of the coefficients over a specific time period (Efron, 1979; Biondi and Waikul, 2004).
Five residual chronologies with a common period (1907-2014) were selected for principal component analysis (PCA) in the SPSS (Statistical Product and Service Solutions) software (SPSS 22). Using the PCA, we explored the similarities and differences in the five residual chronologies.
To explore the high- and low- frequency variance of the Qinghai spruce and Qilian juniper chronologies, we compared their standard chronologies in the frequency domain. The Qilian juniper standard chronology was obtained from Yang et al. (2010). The regional standard chronology was produced by applying PCA to seven single-site standard chronologies from Qifeng (DHS), Sidalong (XYT), Zhamashike (ZMSK), Haiyagou (HYG), Dulan (DL), Delingha (DLH) and the Anemaqin Shan (ANMQ) (Figure 1). We used observations from PQK, DDS, XYT, HYG, DHS to develop a regional standard chronology of Qinghai spruce using ASTRAN (Table S2). Fast Fourier transform (FFT) filtering (Cooley and Tukey, 1965) was used to calculate five-year high-pass filtered data and fifty-year low-pass filtered data from the two regional standard chronologies from 1813-2001. Correlations were then calculated between the original unfiltered, first-order differenced, five-year high-pass filtered, and fifty-year low-pass filtered data. We used a Matlab program based on Monte Carlo significance tests (Macias-Fauria et al., 2012) to calculate the correlation and associated significance.
Results
Climate differences and chronology characteristics at the five sampling sites
The temperature, precipitation and relative humidity data during 1960-2014 from all five meteorological stations showed that temperature and precipitation were high in June, July and August, and low in December, January and February (Figure 3). The relative humidity was high in July, August and September and lowest in April. Under the influence of topography, the temperature gradually decreases with increasing altitude. The elevation of Yeniugou meteorological station is 3314 m, which is significantly higher than that of other sites (<2500m) (Table S1), and may explain why its monthly temperature was lowest and precipitation was highest. The higher relative humidity at Yeniugou may be due to the increased rainfall and decreased evaporation at high elevation. There was little change in temperature between areas of similar elevation (Minle and Sunan, Zhangye and Jiuquan), but precipitation and relative humidity gradually decreased from east to west. Overall, local climates at the five meteorological stations present similarities as well as differences, reflecting a common regional climate and the specific local environments.
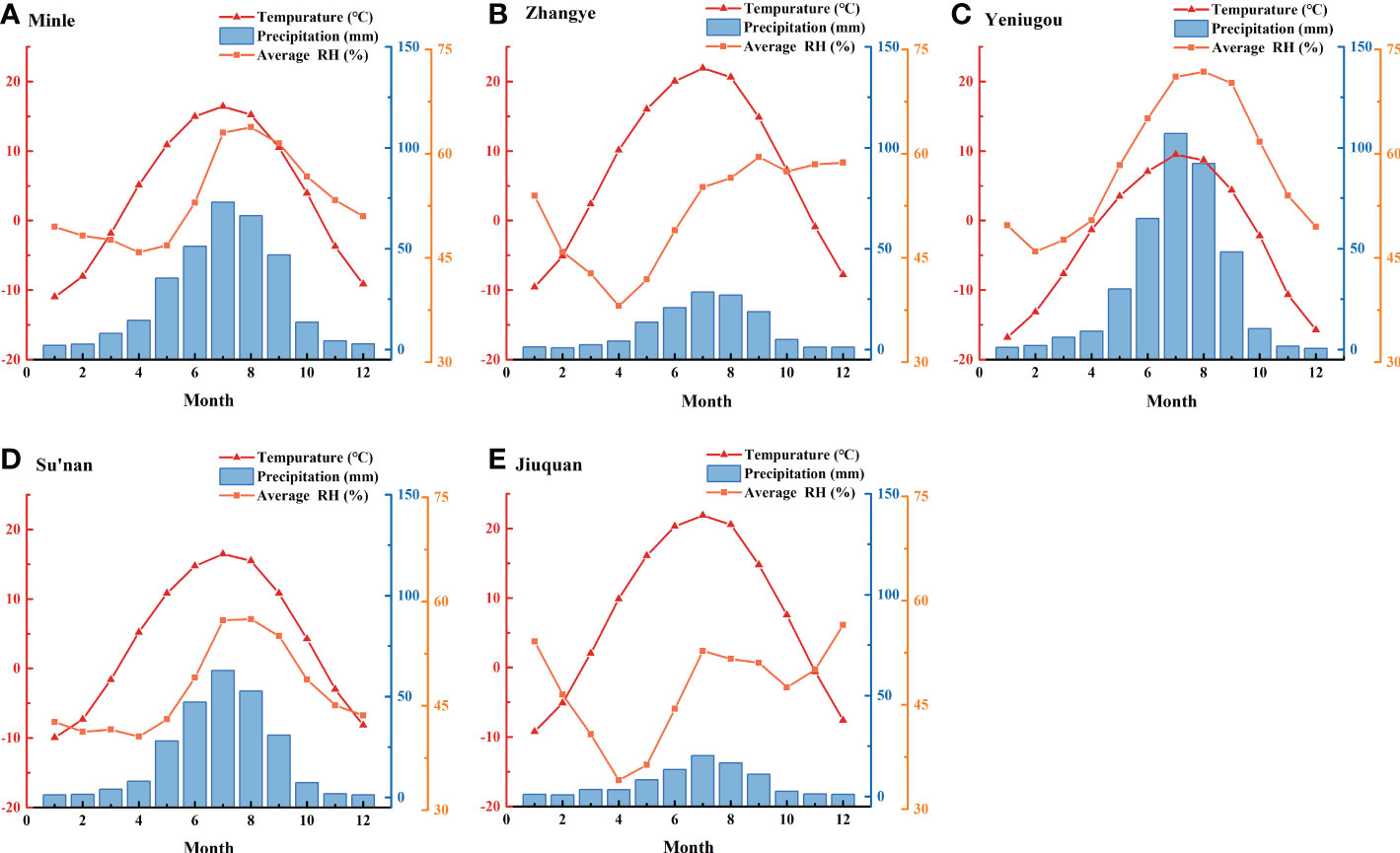
Figure 3 Monthly mean temperature, precipitation and relative humidity (RH) at five meteorological stations along the east-west transect in the Hexi area from 1960-2014.
Five Qinghai spruce residual chronologies were established from east to west in the Hexi area (Figure 2). The time spans of the PQK, DDS, XYT, HYG, DHS chronologies were 227 years (1792-2019), 202 years (1812-2014), 222 years (1797-2019), 177 years (1839-2016) and 112 years (1907-2019), respectively. The mean segment length of all samples was 188 years. All the chronologies showed low growth from 1920 to 1930 and high growth in the 1980s and after 2000.
Principal component analysis of the five residual chronologies
According to the principal component analysis of the five residual chronologies (Figure 4), the first principal components (PC1) of all five chronologies were between 0.5 and 1, and accounted for 60.5% of the variance, indicating that the TRW of most trees in the study area responded in the same way to environmental factors. The second principal components (PC2), accounted for 15.2%, with the easternmost PQK having a negative PC2 (-0.709) and the westernmost DHS having a positive PC2 (0.843), indicating differences between their two residual chronologies. Overall, the TRW growth status of Qinghai spruce at the five sampling sites was affected by both the regional climate and local environments.
Statistical comparison of five local raw chronologies
We compared the statistical characteristics calculated of the five raw ring-width series (Figures 5; S2). The AC1 (first-order autocorrelation) values for the five sampling sites were greater than 0.6, indicating significant low-frequency variance. The means of the SD (standard deviation), Rbar (mean inter-series correlation of all series) and MS (mean sensitivity) of the five raw TRW series were greater than 0.2, indicating considerable high-frequency variance (Fritts, 1976). Ring-width, SD, Rbar and MS were all highest, and AC1 was the lowest at the westernmost site (DHS). The opposite pattern was observed at the easternmost site (PQK). This pattern indicates greater high-frequency variance at the westernmost site (DHS) than at the easternmost site (PQK). From this we infer that tree-ring growth in drier regions is more sensitive to environmental change.
The relationships between TRW and climate
We investigated the correlations between residual chronologies and the first-order differenced climate series from May of the previous year to the current October during 1961-2014. As shown in Figure 6, the correlations between the five residual chronologies and temperature, precipitation, relative humidity and scPDSI were relatively consistent among the five sampling sites. The five residual chronologies showed negative correlation with the temperature in July and August of the previous year (-0.43≤r≤-0.17), while the precipitation and relative humidity were positively correlated (0.04≤r ≤ 0.39). The five residual chronologies also showed positive correlation with March to April relative humidity (0.15≤r ≤ 0.42). Correlation coefficients were much stronger with scPDSI, showing positive correlations (0.2≤r ≤ 0.53) throughout the current March to June, except for at PQK. Although PQK, located in the easternmost area, showed no significant correlation with scPDSI, it nevertheless showed positive correlation with precipitation and relative humidity in the current March to April (0.15≤r ≤ 0.31). Consequently, drought (scPDSI) from March to June is the primary limiting factor for Qinghai spruce growth in our study area. Easternmost site PQK has more precipitation and a better tree-ring growth environment, meaning there is a weaker limiting effect of drought in this area.
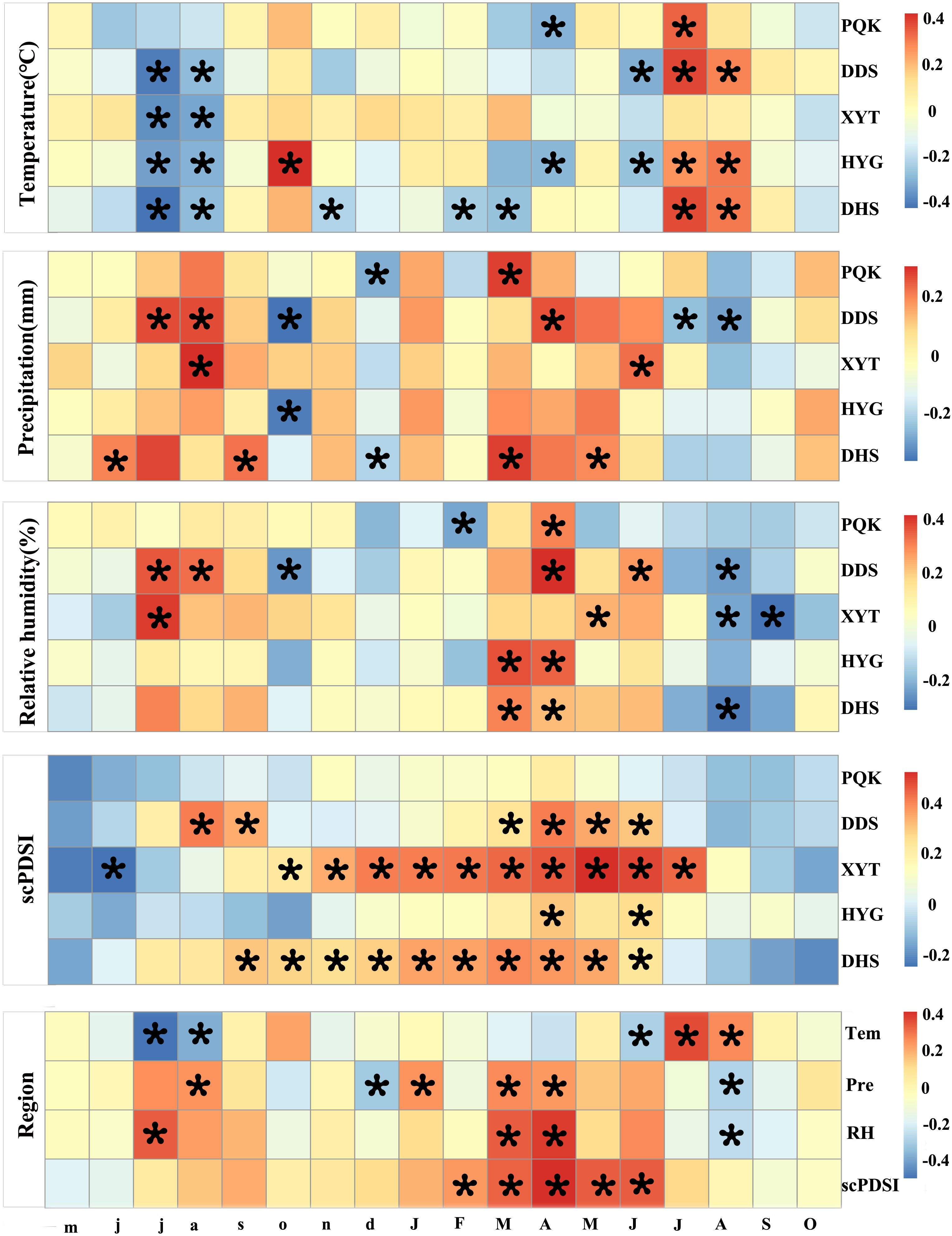
Figure 6 Correlations of the residual chronologies with first-order differences of mean temperature, monthly precipitation, relative humidity and scPDSI from May of the previous year to October of the current year at the five sampling sites and across the whole region (PQK, DDS, XYT, HYG, DHS) during 1961-2014. The previous year’s months are represented in lower case and those of the current year in upper case. Grid boxes with black stars indicate statistically significant results (p<0.05).
The correlation analysis of the five residual chronologies from 1960-2014 yielded significant positive correlations between sites (Table S3). The TRW measurements at PQK, DDS, XYT, HYG, DHS were selected to develop a regional TRW residual chronology that represents the growth status of Qinghai spruce across the whole region. Regional average series of temperature, precipitation (Jones and Hulme, 1996), relative humidity and scPDSI were also established. Correlation between the regional Qinghai spruce residual chronology and climate showed that regional Qinghai spruce TRW was highest when the prior summer was cool and wet. Significant positive correlation was also found between relative humidity and scPDSI in the spring and summer of the current year. These results further suggest that drought (scPDSI) in the previous growing season and early growing season (March-June) is the main limiting factor for Qinghai spruce growth.
Comparisons with the regional Qilian juniper standard chronology
We calculated the correlations between the standard chronologies of Qinghai spruce and Qilian juniper from 1813-2001 (Figure 7), finding a common response of the growth of both species to climate (r=0.51, P<0.001). Annual variability is indicated by the first-order difference of the chronology. The five-year high-pass filtered data preserve the high-frequency variability of the mean of the original series. The fifty-year low-pass filtered data preserve the relatively low-frequency variability of the mean of the original series. Overall, there was a strong correlation between the high-frequency variability of Qinghai spruce and Qilian juniper (r=0.66, r=0.67, P<0.001), while correlation between the fifty-year low-pass filtered domains was not significance (r=0.48, P=0.142).
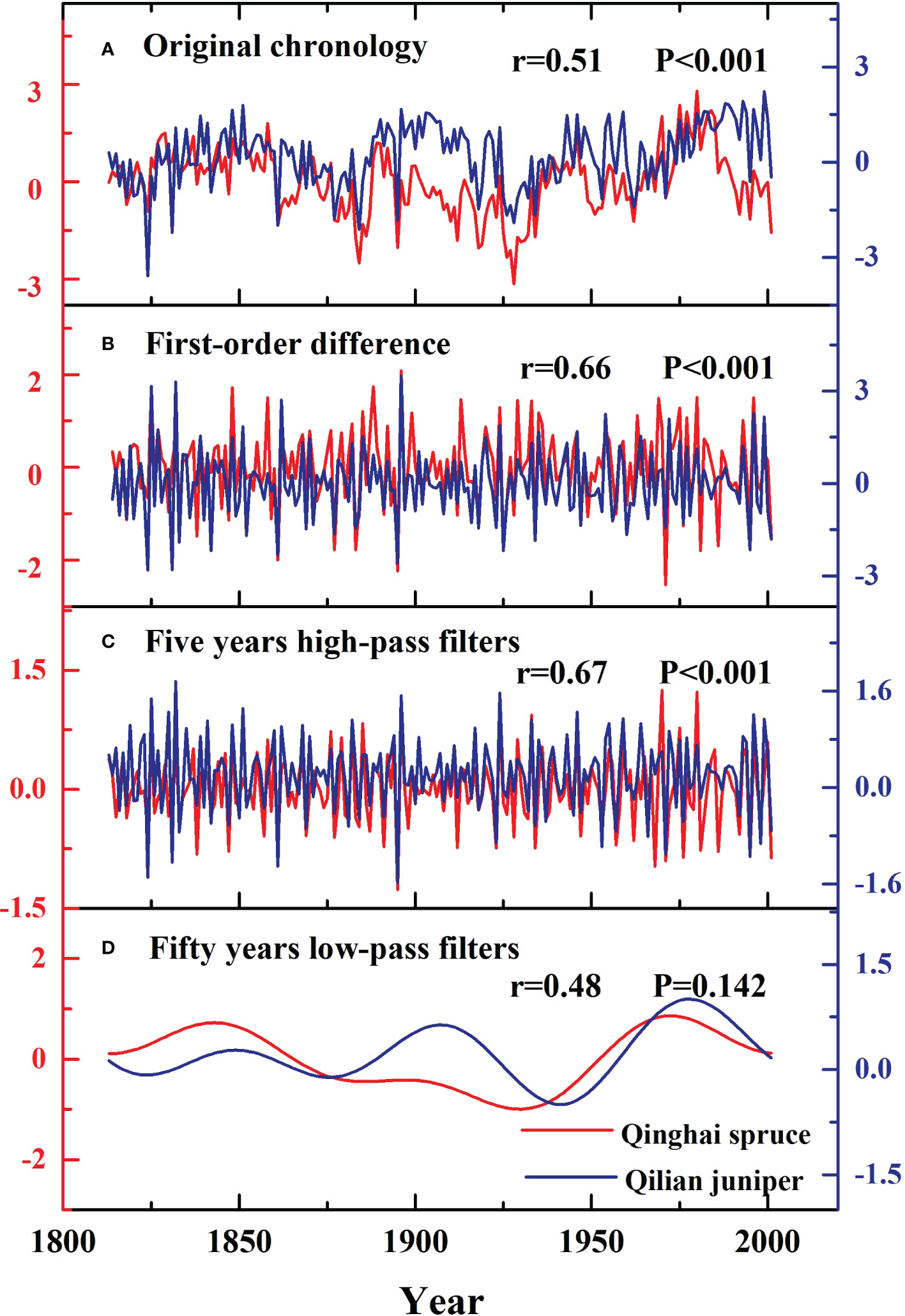
Figure 7 Comparison of the standard chronologies of Qinghai spruce (red lines) and Qilian juniper (blue lines) during the past 189 years.
Discussion
Tree-ring width response of Qinghai spruce to climate variability in the Hexi area
The five residual chronologies all showed low growth from 1920-1930 and high growth in the 1980s and after 2000 (Figure 2). These high and low growth trends are line with previous studies (Liu et al., 2005; Fang et al., 2009; Gao et al., 2018; Cai and Liu, 2021). Despite variations in habitat types and local environment, the PC1 of the five residual chronologies indicated a common influence of the regional climate on tree growth (Figure 4). This suggests that a relatively homogeneous macroclimate, independent of any differences in local ecological conditions, was the underlying cause of the high degree of covariance among the tree-ring chronologies (Peters et al., 1981; Littell et al., 2008; Liang et al., 2010).
The five chronologies showed positive correlation with precipitation and negative correlation with temperature in July and August of the previous year (Figure 6), suggesting that high precipitation in the previous year can promote increased nutrient transformation and storage in the tree, driving rapid growth of the cambium in the following year. At the same time, high temperatures in July and August of the previous year can cause high evaporation, resulting in poor radial growth. (Fritts, 1976). This lag between radial growth and climate suggests that the climate in the previous year may influence TRW in the next year through its effect on nutrient storage in the tree. The same relationship between climate and tree-ring growth has been reported for Qinghai spruce in the Hexi area (Chen et al., 2013) and nearby areas (Chen et al., 2011; Deng et al., 2013; Wang et al., 2016).
In our study, tree-ring growth was positively correlated with scPDSI in all months from the previous August to the current August. In particular, four consecutive months from the current March to June were significant (P<0.05) (Figure 6), indicating that moisture availability during these seasons is the primary limiting factor for the radial growth of Qinghai spruce. Zeng et al. (2020) used the Vaganov–Shashkin (VS) model to assess the response of Qinghai spruce stem radial growth to climate from the perspective of tree physiological processes. Their results showed that soil moisture conditions during the early growing season (May to July) significantly affected tree growth, with the early growing season accounting for > 65% of total tree-ring width index. The availability of moisture in the early growing season enhances the rate of photosynthesis, enabling the assimilation of sufficient carbohydrates to maintain a high rate of cell growth, leading to large ring widths (Zhang et al., 2016).
It is worth noting that the correlation coefficients between precipitation or relative humidity and the chronologies were relatively small. These low values may reflect differences between precipitation and relative humidity at the sampling sites and the meteorological stations. The elevations of Minle, Zhangye, Yeniugou, Sunan, and Jiuquan are 2272 m, 1484 m, 3314 m, 2311 m and 1478 m, respectively, whereas the elevation of the sampling sites ranged from 2600 m to 3100 m (Table S1). This result also highlights that elevation differences between meteorological stations and sampling sites can influence the analysis of relationships between radial growth and climate.
Radial growth differences of Qinghai spruce from east to west along the Hexi area
Although some differences in the radial growth of Qinghai spruce were found from east to west, there was no consistent effect of hydrological gradients on the relationship between climate and radial growth (Figure 6). We found that the chronology of the westernmost region (DHS) was more sensitive to climate than that of the easternmost region (PQK), indicating that the importance of precipitation increased from east to the west along the Hexi area.
Water stress in plants results in a decrease in the total leaf surface area and leaf-level water content, increasing the sensitivity of plant growth to rainfall (Meinzer et al., 2007; Li et al., 2016; Rodgers et al., 2018). According to the principle of ecological amplitude (Fritts, 1976), the water demand of tree growth in arid areas can easily reach the limit of its physiological demand, and water is thus the main limiting factor in this area. In regions with higher precipitation, the limiting effect of precipitation on tree-ring growth is weakened and trees are less sensitive to precipitation. Therefore, the wetter PQK site in the east was less sensitive to climate than the other study sites.
Comparison of the standard chronologies of Qinghai spruce and Qilian juniper at different timescales
We found that the standard chronologies of Qinghai spruce and Qilian juniper were consistent in the high-frequency domain (first-order difference, five-year high-pass filters) but different in the fifty-year low-pass filters (Figure 7). We consider that the relatively arid conditions and associated drought stress probably drive the consistent inter-annual variability of tree growth-climate relationships in the Hexi area. Division and elongation of cambium cells requires enough water to maintain normal growth (Zhang, 2018). Frequent drought conditions can lead to hydraulic failure, deficient carbon recharge, inhibited photosynthesis and thus impaired tree growth in both juniper and spruce (Mitchell et al., 2013; Choat et al., 2018).
The differences in the low-frequency climate domain between the two tree-species may be attributed to their different habitat conditions and lifespans. It is well known that the germination, growth and development of trees can vary significantly between different species due to differences in their habitats (McDowell et al., 2003; Liu et al., 2007; Brandt et al., 2014). For example, Qilian juniper can grow on sunny slopes (Yang et al., 2010). This species has the characteristics of liking sunlight, being drought-tolerant, and having a well-developed root system and strong wind resistance. In contrast, Qinghai spruce is primarily located on shady slopes with thick soil cover, low evaporation (Zheng et al., 2019), and a dense forest canopy. The different habitat conditions have led to different drought tolerance strategies in the two species. Some studies have shown that Qinghai spruce mainly resists drought by delaying dehydration, while Qilian juniper mainly resists drought by enduring dehydration (Dang et al., 2003; Ran et al., 2021). Age differences between the species can also affect the signal strength in the low-frequency domain (Konter et al., 2016). In our study, the average age of Qinghai spruce was about 180 years, and the average age of Qilian juniper was about 550 years. Differences in average age may also cause differences in the recording of climate signals. This result reminds us that when different tree species are used to reflect long-term climate change, these differences should be considered.
Conclusion
Our tree-ring width sampling network covering the main environmental gradient of the Qinghai spruce forest ecosystems in the Hexi area and captured the spatial variability of radial growth. Here, tree growth was predominantly limited by drought (scPDSI) in the previous and early growing seasons (March-June). Increasing sensitivity of radial growth to climate along the east-west aridity gradient was also evident. Despite the physiological differences between Qinghai spruce and Qilian juniper, their standard chronologies were consistent in the high-frequency domain (first-order difference, five-year high-pass filters); however, they were different following fifty years low-pass filtering. These results may be attributed to their different habitat conditions and tree ages. Thus, the tree-ring network in the Hexi area has revealed insights into interactions between tree growth and the environment. Overall, regional climate change, the east–west aridity gradient, and tree species all played important roles in influencing radial growth. These results highlight the need to carefully consider the combined effects of these variables when performing large-scale and multi-species dendrochronological studies.
Data availability statement
The raw data supporting the conclusions of this article will be made available by the authors, without undue reservation.
Author contributions
BY and BF designed the study. GL provided field and data support. BF analyzed the data and wrote the first version of manuscript. BF, BY, and GL revised the manuscript and approved the submitted version. All authors contributed to the article and approved the submitted version.
Funding
This research is supported by the National Natural Science Foundation of China (grant nos. Grant 42130511 and 41602192).
Conflict of interest
The authors declare that the research was conducted in the absence of any commercial or financial relationships that could be construed as a potential conflict of interest.
Publisher’s note
All claims expressed in this article are solely those of the authors and do not necessarily represent those of their affiliated organizations, or those of the publisher, the editors and the reviewers. Any product that may be evaluated in this article, or claim that may be made by its manufacturer, is not guaranteed or endorsed by the publisher.
Supplementary material
The Supplementary Material for this article can be found online at: https://www.frontiersin.org/articles/10.3389/fpls.2022.1046462/full#supplementary-material
Supplementary Figure 1 | Standard chronologies and number of trees used in the study. Solid lines indicate EPS>0.85 and dotted lines indicate EPS<0.85.
Supplementary Figure 2 | Statistical comparison of the raw tree-ring measurements at the five sampling sites (alighed by elevation).
Supplementary Table 1 | Statistical information of sampling sites.
Supplementary Table 2 | Correlation matrix between different pairs of five standard chronologies during the common period 1907-2014.
Supplementary Table 3 | Correlation matrix between different pairs of five residual chronologies during the common period 1960-2014.
References
Biondi, F., Waikul, K. (2004). DENDROCLIM2002: A c++ program for statistical calibration of climate signals in tree-ring chronologies. Comput. Geoen. 30, 303–311. doi: 10.1016/j.cageo.2003.11.004
Brandt, R., Lachmuth, S., Landschulz, C., Hass, F., Hensen, I. (2014). Species-specific responses to environmental stress on germination and juvenile growth of two Bolivian Andean agroforestry species. New For 45, 777–795. doi: 10.1007/s11056-014-9436-6
Cai, Q., Liu, Y. (2021). Temperature variation of the past century in the tongbai mountain, henan province and its relationship with air-sea interaction. Quaternary Sci. 41 (2), 346–355. doi: 10.11928/j.issn.1001-7410.2021.02.04
Camarero, J. J., Sánchez-Salguero, R., Sangüesa-Barreda, G., Matías, L. (2018). Tree species from contrasting hydrological niches show divergent growth and water-use efficiency. Dendrochronologia 52, 87–95. doi: 10.1016/j.dendro.2018.10.003
Chen, F., Wang, H., Yuan, Y. (2017). Divergent growth response of qinghai spruce to recent climate warming in the arid northeastern Tibet Plateau[J]. Asian Geogr. 34 (2), 169–181. doi: 10.1080/10225706.2017.1394886
Chen, F., Yuan, Y., Wei, W. (2011). Climatic response of picea crassifolia tree-ring parameters and precipitation reconstruction in the western qilian mountains, China. J. Arid. Environ. 75 (11), 1121–1128. doi: 10.1016/j.jaridenv.2011.06.010
Chen, F., Yuan, Y., Wei, W., Zhang, R., Yu, S., Shang, H., et al. (2013). Tree-ring-based annual precipitation reconstruction for the hexi corridor, NW China: consequences for climate history on and beyond the mid-latitude Asian continent. Boreas 42 (4), 1008–1021. doi: 10.1111/bor.12017
Choat, B., Brodribb, T. J., Brodersen, C. R., Duursma, R. A., López, R., Medlyn, B. E. (2018). Triggers of tree mortality under drought. Nature 558 (7711), 531–539. doi: 10.1038/s41586-018-0240-x
Cook, E. R. (1985). A time-series analysis approach to tree-ring standardization (Tucson: The University of Arizona Press).
Cook, E. R., Anchukaitis, K. J., Buckley, B. M., D’Arrigo, R. D., Jacoby, G. C., Wright, W. E. (2010). Asian Monsoon failure and megadrought during the last millennium. Science 328 (5977), 486–489. doi: 10.1126/science.118518
Cook, E. R., Kairiukstis, A. (Eds.) (1990). Methods of dendrochronology: Applications in the environmental sciences (Dordrecht: Kluwer Academic Publishers).
Cook, E. R., Peters, K. (1997). Calculating unbiased tree-ring indices for the study of climatic and environmental change. Holocene 7, 361–370. doi: 10.1177/095968369700700314
Cooley, J. W., Tukey, J. W. (1965). An algorithm for the machine calculation of complex Fourier series. Mathematics Comput. 19 (90), 297–301. doi: 10.1090/S0025-5718-1965-0178586-1
Danek, M., Chuchro, M., Walanus, A. (2017). Variability in larch (Larix decidua mill.) tree-ring growth response to climate in the polish Carpathian mountains. Forests 8 (10), 354. doi: 10.3390/f8100354
Dang, H. Z., Zhao, Y. S., Chen, X. W. (2003). Study on drought resistance of tree species on plateau hill in GanSu. Sci. Soil Water Conserv. 1 (4), 21–25.
Deng, Y., Gou, X., Gao, L., Yang, M., Zhang, F. (2017). Tree-ring recorded moisture variations over the past millennium in the hexi corridor, northwest China. Environ. Earth Sci. 76 (7), 1–9. doi: 10.1007/s12665-017-6581-1
Deng, Y., Gou, X., Gao, L., Zhao, Z., Cao, Z., Yang, M. (2013). Aridity changes in the eastern qilian mountains since AD 1856 reconstructed from tree-rings. Quaternary Int. 283, 78–84. doi: 10.1016/j.quaint.2012.04.039
Du, D., Jiao, L., Wu, X., Qi, C., Xue, R., Chen, K., et al. (2022). Responses of radial growth of picea crassifolia to climate change over three periods at different elevations in the qilian mountains, northwest China. Trees, 36, 1721–1734. doi: 10.1007/s00468-022-02323-y
Efron, B. (1979). Bootstrap method: Another look at the jackknife. Ann. Stat 7 (1), 1–26. doi: 10.1214/aos/1176344552
Fang, K., Gou, X., Chen, F., Yang, M., Li, J., He, M., et al. (2009). Drought variations in the eastern part of northwest China over the past two centuries: evidence from tree rings. Clim. Res. 38, 129–135. doi: 10.3354/cr00781
Gao, X. (2011). Ecophysiological adaptation of juniperus przewalskii and picea crassifolia of the different slopes (Lanzhou, China: Lanzhou University).
Gao, L., Gou, X., Deng, Y., Wang, Z., Gu, F., Wang, F. (2018). Increased growth of qinghai spruce in northwestern China during the recent warming hiatus. Agric. For. Meteorol. 260, 9–16. doi: 10.1016/j.agrformet.2018.05.025
Gao, L., Gou, X., Deng, Y., Yang, M., Zhao, Z., Cao, Z. (2013). Dendroclimatic response of picea crassifolia along an altitudinal gradient in the Eastern qilian mountains, Northwest China. Arctic. Antarctic Alpine Res. 45, 491–499. doi: 10.1657/1938-4246-45.4.491
Gou, X., Deng, Y., Gao, L., Chen, F., Cook, E., Yang, M., et al. (2015). Millennium tree-ring reconstruction of drought variability in the eastern qilian mountains, northwest China. Climate Dynam. 45 (7), 1761–1770. doi: 10.1007/s00382-014-2431-y
Graumlich, L. J. (1993). Response of tree growth to climatic variation in the mixed conifer and deciduous forests of the upper great lakes region. Can. J. For. Res. 23 (2), 133–143. doi: 10.1139/x93-020
Griesbauer, H., DeLong, S. C., Rogers, B., Foord, V. (2021). Growth sensitivity to climate varies with soil moisture regime in spruce-fir forests in central British Columbia. Trees 35 (2), 649–669. doi: 10.1007/s00468-020-02066-8
Guerrero-Hernández, R., Muñiz-Castro, M.Á., Villanueva-Díaz, J., Hernández-Vera, G., Vázquez-García, J. A., Ruiz-Corral, J. A. (2022). Tree-ring patterns and growth response of abies jaliscana to climate along elevational gradients in the mountains of Western jalisco, Mexico. Forests 13 (7), 981. doi: 10.3390/f13070981
Holmes, R. L. (1983). Computer-assisted quality control in tree-ring dating and measurement. Tree-Ring Bull. 43, 69–78.
Jones, P. D., Hulme, M. (1996). Calculating regional climatic time series for temperature and precipitation: methods and illustrations. Int. J. Climatol.: A J. R. Meteorol. Soc. 16 (4), 361–377. doi: 10.1002/(SICI)1097-0088(199604)16:4<361::AID-JOC53>3.0.CO;2-F
Kharal, D. K., Meilby, H., Rayamajhi, S., Bhuju, D., Thapa, U. K. (2014). Tree ring variability and climate response of abies spectabilis along an elevation gradient in mustang, Nepal. Banko Janakari 24 (1), 3–13. doi: 10.3126/banko.v24i1.13473
Klippel, L., Krusic, P. J., Brandes, R., Hartl-Meier, C., Trouet, V., Meko, M., et al. (2017). High-elevation inter-site differences in mount smolikas tree-ring width data. Dendrochronologia 44, 164–173. doi: 10.1016/j.dendro.2017.05.006
Konter, O., Büntgen, U., Carrer, M., Timonen, M., Esper, J. (2016). Climate signal age effects in boreal tree-rings: Lessons to be learned for paleoclimatic reconstructions. Quaternary Sci. Rev. 142, 164–172. doi: 10.1016/j.quascirev.2016.04.020
Liang, E., Shao, X., Eckstein, D., Huang, L., Liu, X. (2006). Topography- and species-dependent growth responses of Sabina przewalskii and picea crassifolia to climate on the northeast Tibetan plateau. For. Ecol. Manag. 236, 268–277. doi: 10.1016/j.foreco.2006.09.016
Liang, E., Shao, X., Eckstein, D., Liu, X. (2010). Spatial variability of tree growth along a latitudinal transect in the qilian mountains, northeastern Tibetan plateau. Can. J. For. Res. Rev. Can. Rech. For 40, 200–211. doi: 10.1139/X09-186
Littell, J. S., Peterson, D. L., Tjoelker, M. (2008). Douglas-Fir growth in mountain ecosystems: water limits tree growth from stand to region. Ecol. Monogr. 78 (3), 349–368. doi: 10.1890/07-0712.1
Liu, Y., Cai, Q., Shi, J., Hughes, M. K., Kutzbach, J. E., Liu, Z., et al. (2005). Seasonal precipitation in the south-central helan mountain region, China, reconstructed from tree-ring width for the past 224 years. Can. J. For. Res. Rev. Can. Rech. For 35, 2403–2412. doi: 10.1139/x05-168
Liu, X., Shao, X., Liang, E., Zhao, L., Chen, T., Qin, D., et al. (2007). Species-dependent responses of juniper and spruce to increasing CO2 concentration and to climate in semi-arid and arid areas of northwestern China. Plant Ecol. 193 (2), 195–209. doi: 10.1007/s11258-006-9258-5
Liu, Y., Sun, C., Li, Q., Cai, Q. (2016). A picea crassifolia tree-ring width-based temperature reconstruction for the mt. dongda region, Northwest China, and its relationship to Large-scale climate forcing. PloS One 11 (8), e0160963. doi: 10.1371/journal.pone.0160963
Li, H., Wang, G., Zhang, Y., Zhang, W. (2016). Morphometric traits capture the climatically driven species turnover of 10 spruce taxa across China. Ecol. Evol. 6 (4), 1203–1213. doi: 10.1002/ece3.1971
Macias-Fauria, M., Grinsted, A., Helama, S., Holopainen, J. (2012). Persistence matters: Estimation of the statistical significance of paleoclimatic reconstruction statistics from autocorrelated time series. Dendrochronologia 30 (2), 179–187. doi: 10.1016/j.dendro.2011.08.003
McDowell, N., Brooks, J. R., Fitzgerald, S. A., Bond, B. J. (2003). Carbon isotope discrimination and growth response of old pinus ponderosa trees to stand density reductions. Plant Cell Environ. 26, 631–644. doi: 10.1046/j.1365-3040.2003.00999.x
Meinzer, F. C., Warren, J. M., Brooks, J. R. (2007). Species-specific partitioning of soil water resources in an old-growth Douglas-fir-western hemlock forest. Tree Physiol. 27, 871–880. doi: 10.1093/treephys/27.6.871
Mitchell, P. J., O’Grady, A. P., Tissue, D. T., White, D. A., Ottenschlaeger, M. L., Pinkard, E. A. (2013). Drought response strategies define the relative contributions of hydraulic dysfunction and carbohydrate depletion during tree mortality. New Phytol. 197 (3), 862–872. doi: 10.1111/nph.12064
Osborn, T. J., Biffa, K. R., Jones, P. D. (1997). Adjusting variance for sample-size in tree-ring chronologies and other regional-mean time-series. Dendrochronologia 15, 89–99.
Palmer, W. C. (1965). Meteorological drought (Washington, DC: US Department of Commerce, Weather Bureau Research Paper).
Peters, K., Jacoby, G. C., Cook, E. R. (1981). Principal components analysis of tree-ring sites. Tree-Ring Bull. 41, 1–19.
Ran, Y., Zhang, L., Wang, R., Zhao, S., Liu, X. (2021). Diverse climate sensitivities in picea crassifolia and juniperus przewalskii promote different responses to climate warming in qilian mountains, northwest China. Geografiska Annaler.: Ser. A Phys. Geogr. 103 (1), 33–50. doi: 10.1080/04353676.2020.1796336
Rinn, F. (2003). TSAP-win: Time series analysis and presentation for dendrochronology and related applications (Germany: Heidelberg). Available at: http://www.rimatech.com.
Rodgers, V. L., Smith, N. G., Hoeppner, S. S., Dukes, J. S. (2018). Warming increases the sensitivity of seedling growth capacity to rainfall in six temperate deciduous tree species. Aob. Plants 10 (1), ply003. doi: 10.1093/aobpla/ply003
Roy, P. S., Ravan, S. A. (1996). Biomass estimation using satellite remote sensing data–an investigation on possible approaches for natural forest. J. Biosci. 21 (4), 535–561. doi: 10.1007/BF02703218
Song, W., Mu, C., Zhang, Y., Zhang, X., Li, Z., Zhao, H., et al. (2020). Moisture-driven changes in the sensitivity of the radial growth of picea crassifolia to temperature, northeastern Tibetan plateau. Dendrochronologia 64, 125761. doi: 10.1016/j.dendro.2020.125761
Touchan, R., Shishov, V. V., Tychkov, I. I., Sivrikaya, F., Attieh, J., Ketmen, M., et al. (2016). Elevation-layered dendroclimatic signal in eastern Mediterranean tree rings. Environ. Res. Lett. 11 (4), 044020. doi: 10.1088/1748-9326/11/4/044020
Van der Schrier, G., Barichivich, J., Briffa, K. R., Jones, P. D. (2013). A scPDSI-based global data set of dry and wet spells for 1901-2009. J. Geophys. Res. Atmos. 118 (10), 4025–4048. doi: 10.1002/jgrd.50355
Wang, B., Chen, T., Wu, G., Xu, G., Zhang, Y., Gao, H., et al. (2016). Qinghai spruce (Picea crassifolia) growth-climate response between lower and upper elevation gradient limits: a case study along a consistent slope in the mid-qilian mountains region. Environ. Earth Sci. 75 (3), 1–16. doi: 10.1007/s12665-015-4930-5
Wang, X., Yang, B. (2021). Divergent tree radial growth at alpine coniferous forest ecotone and corresponding responses to climate change in northwestern China. Ecol. Indic. 121, 107052. doi: 10.1016/j.ecolind.2020.107052
Wang, B., Yu, P., Zhang, L., Wang, Y., Yu, Y., Wang, S. (2019). Differential trends of qinghai spruce growth with elevation in northwestern China during the recent warming hiatus. Forests 10 (9), 712. doi: 10.3390/f10090712
Wigley, T. M., Briffa, K. R., Jones, P. D. (1984). On the average value of correlated time series, with applications in dendroclimatology and hydrometeorology. J. Clim. Appl. Meteorol. 23 (2), 201–213. doi: 10.1175/1520-0450(1984)023<0201:OTAVOC>2.0.CO;2
Yang, B., Qin, C., Bräuning, A., Burchardt, I., Liu, J. (2011). Rainfall history for the hexi corridor in the arid northwest China during the past 620 years derived from tree rings. Int. J. Climatol. 31 (8), 1166–1176. doi: 10.1002/joc.2143
Yang, B., Qin, C., Bräuning, A., Osborn, T. J., Trouet, V., Ljungqvist, F. C., et al. (2021). Long-term decrease in Asian monsoon rainfall and abrupt climate change events over the past 6,700 years. PNAS 118 (30), e2102007118. doi: 10.1073/pnas.2102007118
Yang, B., Qin, C., Huang, K., Fan, Z., Liu, J. (2010). Spatial and temporal patterns of variations in tree growth over the northeastern Tibetan plateau during the period AD 1450-2001. Holocene 20 (8), 1235–1245. doi: 10.1177/0959683610371997
Yang, B., Qin, C., Wang, J., He, M., Melvin, T. M., Osborn, T. J., et al. (2014). A 3500-year tree-ring record of annual precipitation on the northeastern Tibetan plateau. PNAS 111, 2903–2908. doi: 10.1073/pnas.1319238111
Yang, B., Wang, J. L., Liu, J. J. (2019). A 1556 year-long early summer moisture reconstruction for the hexi corridor, northwestern China. Sci. China Earth Sci. 62, 953–963. doi: 10.1007/s11430-018-9327-1
Zeng, X., Wei, C., Liu, X., Zhang, L. (2020). Qinghai spruce (Picea crassifolia) and Chinese pine (Pinus tabuliformis) show high vulnerability and similar resilience to early growing-season drought in the helan mountains, China[J]. Ecol. Indic. 2019, 110. doi: 10.1016/j.ecolind.2019.105871
Zhang, J. (2018). Cambial phenology and intra-annual radial growth dynamics of conifers over the qilian mountains (Lan zhou: University of Lan zhou).
Zhang, J., Gou, X., Zhang, Y., Lu, M., Xu, X., Zhang, F., et al. (2016). Forward modeling analyses of qilian juniper (Sabina przewalskii) growth in response to climate factors in different regions of the qilian mountains, northwestern China. Trees 30 (1), 175–188. doi: 10.1007/s00468-015-1286-0
Zhang, Y., Han, L., Shao, X., Yang, Q., Yin, Z. Y. (2021). Moisture variations during the first millennium CE and their linkage with social developments along the silk road in northwestern China. Climatic Change 168 (3), 1–19. doi: 10.1007/s10584-021-03225-7
Zhang, L., Jiang, Y., Zhao, S., Kang, X., Zhang, W., Liu, T. (2017). Lingering response of radial growth of picea crassifolia to climate at different altitudes in the qilian mountains, Northwest China. Trees 31 (2), 455–465. doi: 10.1007/s00468-016-1467-5
Zhang, Y., Wilmking, M. (2010). Divergent growth responses and increasing temperature limitation of qinghai spruce growth along an elevation gradient at the northeast Tibet plateau. For. Ecol. Manag. 260 (6), 1076–1082. doi: 10.1016/j.foreco.2010.06.034
Keywords: Qinghai spruce, tree-ring width, hydrological gradient, tree species, drought, Hexi area
Citation: Fan B, Yang B and Li G (2022) Assessing the influence of local environment, regional climate and tree species on radial growth in the Hexi area of arid northwest China. Front. Plant Sci. 13:1046462. doi: 10.3389/fpls.2022.1046462
Received: 16 September 2022; Accepted: 01 December 2022;
Published: 22 December 2022.
Edited by:
Huanjiong Wang, Key Laboratory of Land Surface Pattern and Simulation, Institute of Geographic Sciences and Natural Resources Research, Chinese Academy of Sciences (CAS), ChinaReviewed by:
Mingqi Li, Key Laboratory of Land Surface Pattern and Simulation, Institute of Geographic Sciences and Natural Resources Research, Chinese Academy of Sciences (CAS), ChinaChangfeng Sun, Xi’an Jiaotong University, China
Eugene Vaganov, Siberian Federal University, Russia
Copyright © 2022 Fan, Yang and Li. This is an open-access article distributed under the terms of the Creative Commons Attribution License (CC BY). The use, distribution or reproduction in other forums is permitted, provided the original author(s) and the copyright owner(s) are credited and that the original publication in this journal is cited, in accordance with accepted academic practice. No use, distribution or reproduction is permitted which does not comply with these terms.
*Correspondence: Bao Yang, eWFuZ2Jhb0BsemIuYWMuY24=; Gang Li, TGlHYW5nMTQyNkAxNjMuY29t