- College of Agriculture, Shanxi Agricultural University, Taigu, China
Photosynthetic carbohydrate metabolism is an important biochemical process related to crop production and quality traits. Potassium (K) critically contributes to the process of photosynthetic carbon assimilation and carbohydrate metabolism. We explored the effects of potassium fertilization on physiological mechanisms including carbohydrate metabolism in foxtail millet and its yield. Field experiments were performed using two foxtail millet (Setaria italica L.) cultivars: 1) Jingu 21 (low-K sensitive); and 2) Zhangza 10 (low-K tolerant). Effect of five different potassium fertilizer (K2O) rates (0, 60, 120, 180, and 240 kg·hm−2) were tested in two consecutive years, 2020 and 2021. We found that potassium application significantly increases the K content, dry matter accumulation and yield. Jingu 21 and Zhangza 10 had maximum yields at 180 kg·hm−2 K application, which were 29.91% and 31.51% larger than without K application, respectively. Excessive K application (K240) did not further improve their yields. The suitable K fertilizer application of Jingu 21 and Zhangza 10 are 195.25–204.27 and 173.95–175.87 kg·K2O·hm−2, respectively. The net photosynthetic rate (Pn), ribulose-1,5-bisphosphate carboxylase-oxygenase (Rubisco), phosphoenolpyruvate carboxylase (PEPC), and fructose-1,6-bisphosphatase (FBPase) were positively correlated with the potassium content. Potassium application improved the availability of carbon sources for carbohydrate synthesis. Compared with the K0 treatment, variations in the activities of sucrose phosphate synthase (SPS) and sucrose synthase (SuSy) in potassium-treated Jingu 21 (K60, K120, K180, and K240) were 17.94%–89.93% and 22.48%–182.10%, respectively, which were greater than those of Zhangza 10 (11.34%–71.12% and 16.18%–109.13%, respectively) and indicate that Jingu 21 is more sensitive to potassium application. The sucrose contents in the Jingu 21 and Zhangza 10 grains were 0.97%–1.15% and 1.04%–1.23%, respectively. The starch contents were 28.99%–37.75% and 24.81%–34.62%, respectively. The sucrose: ratio of Jingu 21 was smaller than that of Zhangza 10, indicating that Jingu 21 utilized nutrients better than Zhangza 10. Stepwise regression and path analysis showed that leaf and grain SuSy activity, by coordinating the source-sink relationship, have the greatest direct effect on Jingu 21 yield, whereas leaf SuSy activity, by promoting the generation of photosynthates at the source leaf, plays a leading role in Zhangza 10 yield increase. In conclusion, optimized K application can increase foxtail millet grain yield by improving photosynthesis and promoting carbohydrate accumulation and distribution.
Introduction
Millet is one of the main multigrain crops grown in the Loess Plateau region of China, and has advantages of drought resistance, barren tolerance, and a wide adaptability. As multiple cropping indices have increased, the available potassium in the soil of some northern regions can no longer meet the requirements for crop growth and development (DU et al., 2019). To achieve high yields and quality during cultivation, the application of exogenous potassium is indispensable. Cereal crops have high K demands, and the amount of K required for 100 kg of grain is 2–3 kg (Xie et al., 2009). Ye et al. (2020) used the linear plus platform model to determine that the suitable potassium application rate for high rice quality and yield was kg·hm−2 of K2O. Sun et al. (2014) suggested that 133.5 kg·hm−2 of K2O was suitable for millet in central Shanxi. Zhang et al. (2014) found that 300 kg·hm−2 of N and 300 kg·hm−2 of K were the best fertilizer application rates for high yields and efficiencies of Zhangza 5.
Potassium acts both as a nutrient and a signaling agent regulating various metabolic processes in crop growth and development; it plays a vital role in optimizing photosynthetic performance, photosynthate transport, and enzyme activation (Mirza et al., 2018; Xu et al., 2022). Yield is determined mainly by the dry matter accumulation in the stem and sheath before the heading stage, and after, it is determined by leaf photosynthesis (Inoue et al., 2004). In cereal crops, such as rice and millet, organs such as grains act as sinks, and leaves and stems act as sources. Carbohydrates, the most important grain-filling assimilate in rice, are produced by photosynthesis and are mainly transported to grains in the form of soluble sugars (Pan et al., 2011). Potassium can regulate the allocation of carbohydrates between the source and sink organs of crops. Appropriate potassium application not only increases the supply of photosynthates at the source end but also improves sink strength and promotes yield. Potassium deficiency is not only detrimental to photosynthesis but also affects the yield and quality by increasing specific leaf weight and accumulation of soluble sugar in leaves, thereby affecting carbohydrates transport to the sink (Asif et al., 2017). In rice and wheat, appropriate potassium fertilization can: a) promote the transport of soluble sugars and free amino acids from leaves and stems to grains, b) provide sufficient substrates for starch synthesis in grains, c) maintain a high grain-filling rate, and d) improve grain weight and yield (Wang et al., 2003; Hou et al., 2019).
Many important enzymes are involved in carbohydrate synthesis, accumulation, and distribution such as: 1) ribulose-1,5-bisphosphate carboxylase-oxygenase (Rubisco) and phosphoenolpyruvate carboxylase (PEPC), which assimilate CO2 into organic carbon (Lian et al., 2021; Siddiqui et al., 2021); 2) fructose-1,6-bisphosphatase (FBPase), which catalyzes the first committed step in the sucrose synthesis pathway and promotes sucrose synthesis by sucrose synthase (SuSy) and sucrose phosphate synthase (SPS) (Ju et al., 2021); 3) acid invertase (AI) and neutral invertase (NI), which control sucrose degradation; and 4) ADPG pyrophosphorylase, which is a rate-limiting enzyme in starch synthesis. Metabolism of carbohydrates (such as sucrose and starch) and the yield are significantly correlated with the activity of these enzymes, which in turn are affected by potassium status. In rice, insufficient absorption of potassium leads to a disorder in glucose metabolism and affects vegetative and reproductive growth (Chen et al., 2018). Potassium deficiency decreases Rubisco activity in cotton leaves (Ali et al., 2018) and PEPC activity in rice (Balkos et al., 2010). However, potassium deficiency does not affect the Rubisco activity and content in ‘Tiefeng 40’, a soybean variety (Wang et al., 2015). In tubers, SuSy activity (decomposition direction) decreased, and AI activity increased with potassium deficiency; the AI activity was positively correlated with the degree of potassium deficiency stress (Liu et al., 2013). In cotton leaves, sufficient potassium supply increases SPS and SuSy activity while decreasing the AI activity (Zahoor et al., 2017). Thus, appropriate potassium application significantly affects carbohydrate accumulation and distribution in crops via modulating the activities of carbon metabolism-related enzymes, ultimately affecting the yield.
Millets require potassium in large amounts. Potassium deficiency decreases yield of millets; the yield decrease is attributable to reduced photosynthetic capacity and dry matter accumulation (Cao et al., 2019). However, there are few reports on potassium mediated regulation of various physiological mechanisms such as accumulation, transport, and distribution of carbohydrate during the critical growth stages of millet. Here, the optimal potassium fertilization rates for foxtail millet and the role of potassium in physiological mechanisms regulating carbohydrate accumulation, transport, and distribution were determined by analyzing its effect on the dry matter accumulation, carbon metabolism-enzymes activities, carbohydrate accumulation, and yield. Thus, we provide a theoretical and practical basis for green and efficient millet cultivation.
Materials and methods
Experimental conditions
Field experiments were conducted from May to October 2020 and from May to October 2021 at the Shanxi Agricultural University experimental station (Shenfeng Village, Taigu County, Jinzhong City, Shanxi Province, China). From May 13 to October 9 for both years, the average temperature and rainfall were calculated every 15 days, as shown in Figure 1. It was sown on May 13, 2020 and harvested on October 6, 2020, and sown on May 18, 2021 and harvested on October 3, 2021. The average temperature during the whole growth period of millet was 21.3°C and 22.2°C, and average rainfall was 419.1 mm and 331.8 mm, in 2020 and 2021, respectively. The rainfall from September 15 to October 9, 2021 (harvest) accounts for 17.4% of the total rainfall received by the crops.
Experimental design
The experiment was performed using a completely randomized design with three replicates. The soil pH 7.63, organic matter content 16.93 g·kg−1, total nitrogen 0.81 g·kg−1, alkali-hydrolyzed nitrogen 51.84 mg·kg−1, available potassium 98.75 mg·kg−1 and available phosphorus 22.37 mg·kg−1 in the 0-20 cm soil layer of the experimental field. The applications consisted of five potassium (K2O) fertilization rates: 0 (K0) as control, 60 (K60), 120 (K120), 180 (K180), and 240 (K240) kg·hm−2 of K2O. Nitrogen (150 kg·hm−2 of N) and phosphorus (90 kg·hm−2 of P2O5) fertilizers were applied, of which the N, P, and K were urea (42% N), superphosphate (12% P2O5), and potassium sulfate (50% K2O). Two foxtail millet cultivars, Jingu 21 (low-K sensitive) and Zhangza 10 (low-K tolerant), were used. Square plots, 25 m2 in size, were used. For Jingu 21, 0.35 m row-separation and 0.08 m plant-separation was adopted, whereas for Zhangza 10, 0.35 m row-separation and 0.12 m plant-separation was adopted. Local production recommendations were used in management of crops in the field.
Sampling and processing
Six representative plants were sampled from each plot at the booting and filling stages of millet. The plant height and stem diameter were also measured. The plant samples were then separated into different parts, including the roots, stems, leaves, and panicles. All samples were oven-dried at 105°C for 30 min and then to a constant weight at 85°C to determine the dry weight.
At the mature stage of millet, six representative plants from each plot were harvested for measurement of millet biomass accumulation and partitioning in stems, leaves, and spikes. Panicles were used to measure yield and yield components: parameters such as panicle number, spikelet number, panicle grain weight, and thousand-grain weight.
Photosynthetic parameters
Photosynthetic parameters were measured at the booting and filling stages in three representative plants from each plot. The functional millet leaves (inverted second leaves) were measured using a photosynthesis system CI-340. After measurement, all leaves were tagged to help sample them again for enzyme extraction and carbohydrate analysis. Photosynthetic parameters were measured from 9:30 to 11:00 A.M. under clear weather at 30 ± 2 °C leaf temperature, 400 ± 50 μmol·mol−1 CO2, and 1000 ± 50 μmol·m−2·s−1 photosynthetic photon flux density.
Enzyme extraction and analysis
The tagged booting stage panicles, leaves, and grains in the mid-filling stage and the corresponding panicle leaves were collected and immediately placed in liquid nitrogen and stored in an ultra-low temperature freezer (–80°C) until enzymatic measurement (each reported index value is the mean value of three repetitions).
The frozen sample (0.2 g) was ground using a chilled mortar containing 2 mL pre-cooled extraction buffer Tris-HCl (pH 8.2) and ground into a slurry in an ice bath. The slurry was centrifuged at 15000 ×g at 4°C for 20 min. The supernatant was a crude enzyme solution, which was used for initial Rubisco and PEPC activity assays.
Rubisco was assayed in a 3-mL reaction mixture (1.4 mL of 100 mmol·L−1 Tris-HCl (pH 7.8), 0.2 mL of 5 mmol·L−1 Adenosine Triphosphate (ATP), 0.2 mL of 0.2 mmol·L−1 NaHCO3, 0.2 mL of 5 mmol·L−1 Nicotinamide adenine dinucleotide (NADH), 0.1 mL of 160 U·L−1 phosphocreatine kinase, 0.1 mL of 160 U·L−1 glyceraldehyde phosphate dehydrogenase, 0.2 mL of 50 mmol·L−1 creatine phosphate, 0.1 mL of 160 U·L−1 phosphoglycerate kinase, 0.3 mL of distilled water, and 0.1 mL of crude enzyme solution). The reaction was activated by adding 0.1 mL 10 mmol·L−1 Ribulose 1,5-bisphosphate (RuBP). Rubisco activity was measured using a UV-visible spectrophotometer (UV-1800), and the absorbance was measured at 340 nm.
PEPC was assayed in a total volume of 3 mL reaction mixture (1.0 mL 100 mmol·L−1 Tris-HCl (pH 8.2), 0.1 mL 5 mmol·L−1 nicotinamide adenine dinucleotide phosphate (NADP), 0.1 mL 10.5U Malate dehydrogenase (MDH), 0.1 mL 100 mmol·L−1 NaHCO3, 1.5 mL distilled water, and 0.1 mL crude enzyme solution). The reaction was activated by adding 0.1 mL 40 mmol·L−1 phosphoenolpyruvate (PEP). PEPC activity was measured at an 340 nm.
FBPase was extracted and assayed according to the modified method of Hu et al. (2015).
The enzymes SPS, SuSy, AI, and NI were extracted and assayed according to the modified method of Yang et al. (2017).
The enzyme ADPG pyrophosphorylase activity was measured using a glucose diphosphate pyrophosphorylase activity detection kit (Beijing Solarbio Company).
Carbohydrate analysis
Dried leaf and grain tissues were ground and passed through a 0.15-mm sieve. The powdered sample (0.2 g) was placed in 6 mL 80% (v/v) ethanol and then incubated in a water bath at 80°C for 30 min. Then, the mixture was centrifuged at 4000× g at 4°C for 5 min, the supernatant was collected, 6 mL of 80% ethanol was added to the residue, and the extraction was repeated twice. The three supernatants were collected and diluted to 25 mL using 80% ethanol. The resulting extract was used to determine the sucrose and soluble sugar content by spectrophotometry at OD480 and OD620, respectively.
The ethanol-insoluble residue was then stored for starch extraction. The starch in the residue was released by boiling with 2 mL of distilled water for 15 min and cooling to 10°C. Then, the starch was hydrolyzed with 9.2 mol·L−1 HClO4 (2 mL) for 15 min. Distilled water (4 mL) was added to the samples, which were centrifuged at 4000 ×g and 4°C for 10 min. The residue was extracted again using 4.6 mol·L−1 HClO4 (2 mL). The supernatants were combined and diluted with distilled water to 25 mL to obtain starch extract. The starch content was measured using a spectrophotometer at OD620.
Plant potassium content
The powdered sample was digested using a mixture of concentrated H2SO4 and H2O2. The potassium content was determined by atomic absorption spectrometry after dilution. Each treatment was repeated thrice.
Statistical analysis
All figures were drawn using Excel 2010, all data were subjected to an analysis of variance with SPSS 25.0, and the difference between mean values was determined using the least significant difference (P = 0.05) test. Stepwise regression, path analyses and linear plus platform model fitting were performed using SPSS 25.0. Stepwise regression and path analyses was performed to clarify the effect of carbohydrate metabolism enzyme activity on yield.
Results
K Content in millet leaf and grains increases with potassium application
The K content of millet leaves and grains was considerably affected by potassium application (Table 1). The K contents of the leaves and grains increased under K application to different degrees than those under K0 treatment. Compared with K0 treatment, the K contents of the leaves and grains of both cultivars under K60 treatment did not increase significantly (except for the K contents of Zhangza 10 leaves in the filling stage in 2021). The K contents reached their maximum levels under the K180 treatment, and no significant difference was observed between the K120 and K180 treatments. Excessive K application (K240) did not increase the K contents of the crops continuously. Compared with the K0 treatment, the K contents of the Jingu 21 leaves in the booting and filling stages increased by 26.15% and 29.61%, respectively, whereas the K content of the grains increased by 9.10% under the K180 treatment in 2020. Similar results were obtained for 2021: leaf K contents increased by 24.35% and 34.51% in the booting and filling stages, respectively, and grain K contents increased by 22.79% in the filling stage. The increases in the K contents of the Zhangza 10 leaves in the booting stage, leaves in the filling stage, and grains in the filling stage were 38.30%, 21.72%, and 23.08% in 2020, and 22.01%, 38.43%, and 34.43% in 2021, respectively.
Net photosynthetic rate increases with potassium application
The net photosynthetic rate (Pn) increased as the millet crops grew leading to leaf expansion and an increase in leaf area. Potassium application increased the Pn at the booting and filling stages (Figure 2). Compared with the control, K180 application significantly increased the Pn in Jingu 21 by 24.90% and 30.23% (2020), and by 28.22% and 52.74% (2021), at the booting and filling stages, respectively. In Zhangza 10, Pn increased by 32.67% and 44.15% (2020), and by 16.89% and 36.11% (2021), at the booting and filling stages, respectively. The Pn variation range at the filling stage was greater than that at the booting stage.
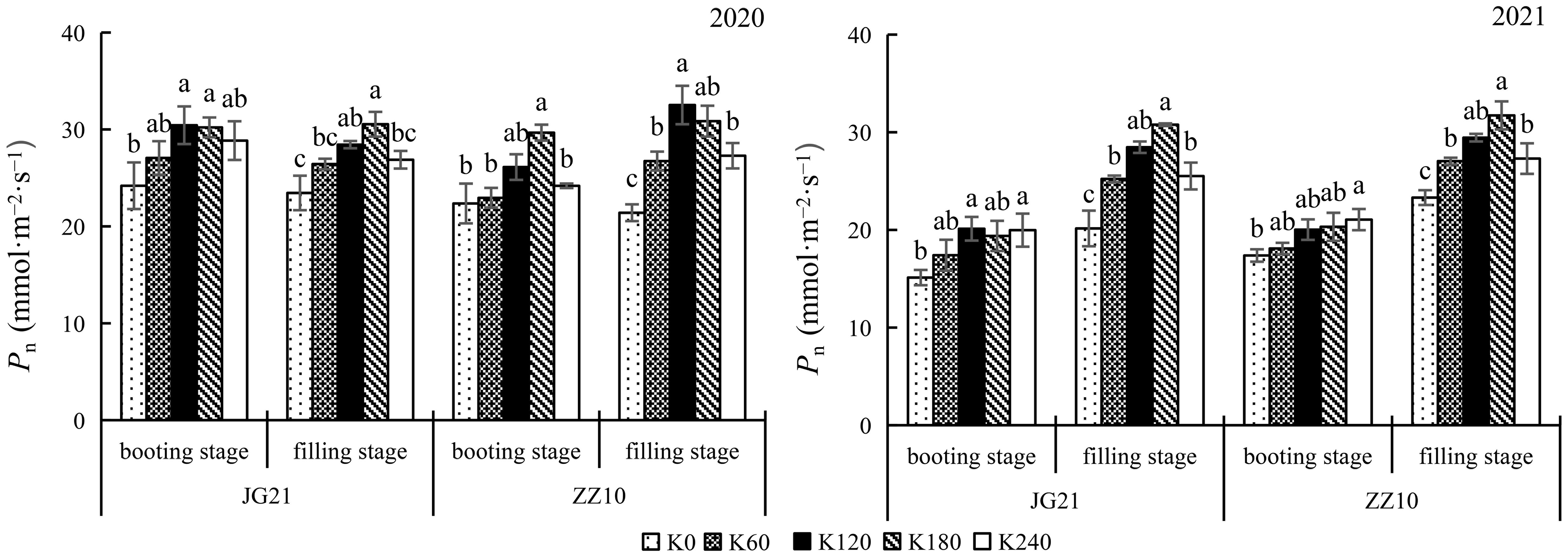
Figure 2 Effects of potassium application rate on net photosynthetic rate of foxtail millet cultivars, Jingu 21 (JG21) and Zhangza 10 (ZZ10). Potassium applications include 5 level 0 (K0) as control, 60 (K60), 120 (K120), 180 (K180), and 240 (K240) kg·hm−2 of K2O. The different normal letters indicate significant differences in different treatments of the same variety at the 0.05 level. Values are means ± SE (n = 3).
Photosynthetic carbon assimilation enzymes activities increased upon potassium application
Activities of carbon assimilation enzymes (PEPC and Rubisco) were enhanced by potassium application at the booting and filling stages (Figure 3). There were no significant differences in the booting stage photosynthetic parameters with K120 and K180 applications for both cultivars; the results were similar for the 2020 and 2021 crops. Compared to the control, K180 application significantly increased the PEPC activity by 69.53% and 81.90%, in Jingu 21 and Zhangza 10, respectively; particularly, the filling stage PEPC activity significantly increased by 56.39% and 51.89% in Jingu 21 and Zhangza 10, respectively (Figure 3A). Compared to the control, K180 application significantly increased the booting stage Rubisco activity by 93.57% and 70.44%, in Jingu 21 and Zhangza 10, respectively; the filling stage Rubisco activity significantly increased by 45.02% and 61.78%, in Jingu 21 and Zhangza 10, respectively (Figure 3B). FBPase activity changes followed a trend similar to that of PEPC and Rubisco activity. The FBPase activity of the two cultivars was not significantly different between the K120 and K180 applications, and not significantly different between the K0 and K60 applications. The average of Jingu 21 FBPase activity increased by 21.98% and 19.62% at the booting and filling stage, respectively; and that of Zhangza 10 was increased by 24.68% and 15.94%, at the booting and filling stages, respectively (Figure 3C).
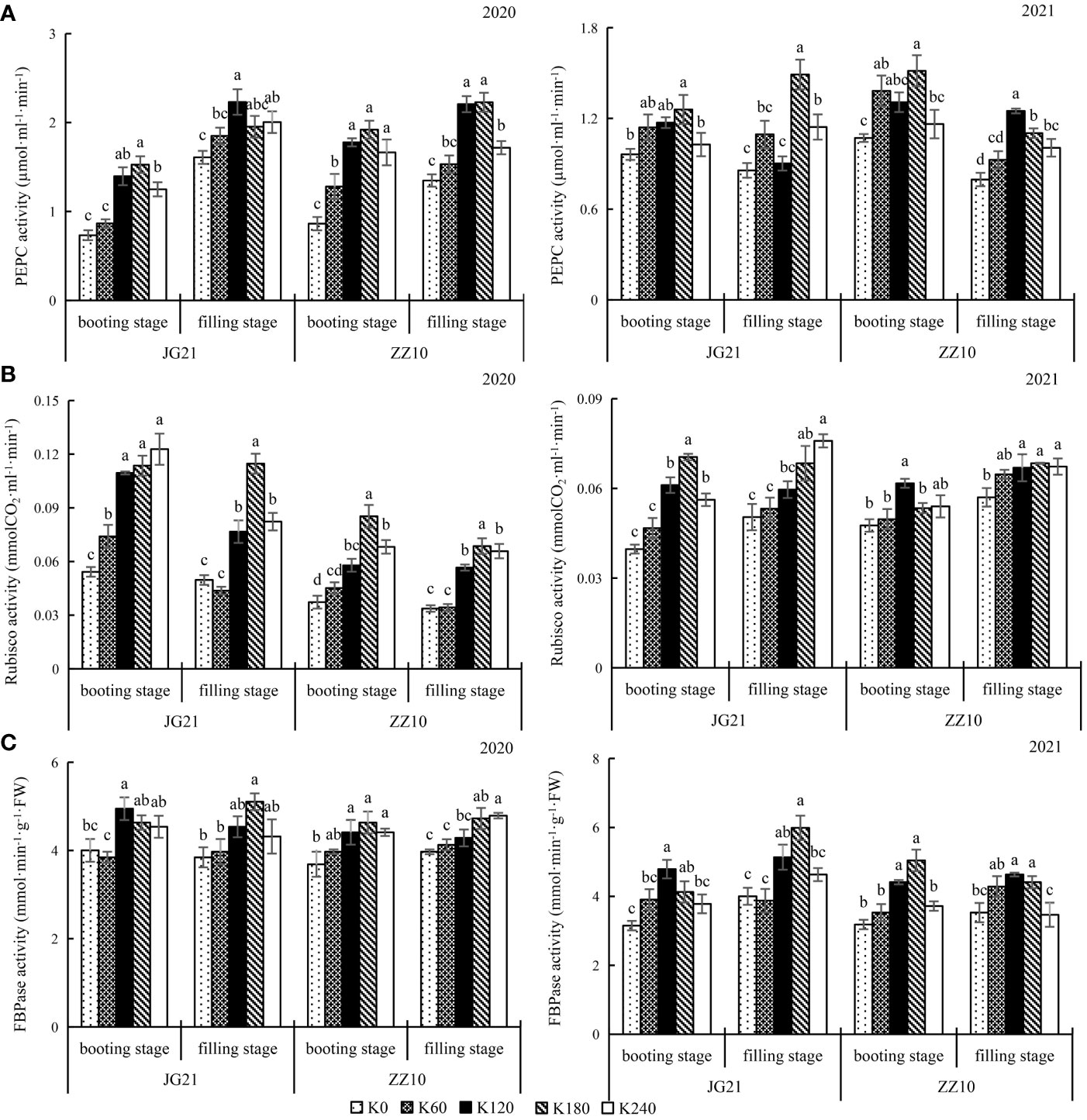
Figure 3 Effects of potassium application rate on key enzymes of carbon assimilation and FBPase of foxtail millet cultivars, Jingu 21 (JG21) and Zhangza 10 (ZZ10). Potassium applications include 5 level 0 (K0) as control, 60 (K60), 120 (K120), 180 (K180), and 240 (K240) kg·hm−2 of K2O. The different normal letters indicate significant differences in different treatments of the same variety at the 0.05 level. Values are means ± SE (n = 3). (A) PEPC, phosphoenolpyruvate carboxylase. (B) Rubisco, ribulose-1,5-bisphosphate carboxylase-oxygenase. (C) FBPase, Fructose-1,6-bisphosphatase.
Potassium application enhances sucrose synthesizing enzymes and diminishes sucrose degrading enzymes
The response of sucrose metabolism-related enzymes to potassium application rates varied with plant parts and the growth stages; however, crops of both the years showed a similar trend (Figure 4). SPS and SuSy are enzymes that catalyze sucrose synthesis. SPS and SuSy activities showed a peaking-response to increases in potassium application rates. For both years, compared with the control, under potassium treated (K60, K120, K180 and K240), the average variation amplitude of SPS activity in leaves at the booting stage, leaves at the filling stage, and grains at the filling stage in Jingu 21 were 47.41%, 47.25%, and 47.70%, respectively, and those in Zhangza 10 were 42.69%, 29.44%, and 44.53%, respectively (Figure 4A). The average changes in SuSy activity in Jingu 21 (at the same stages and organs mentioned above) were 39.92%, 108.46%, and 79.16%, respectively, and those in Zhangza 10 were 29.96%, 86.22%, and 69.55%, respectively (Figure 4B). The enzyme activity variation amplitude was smaller in Zhangza 10.
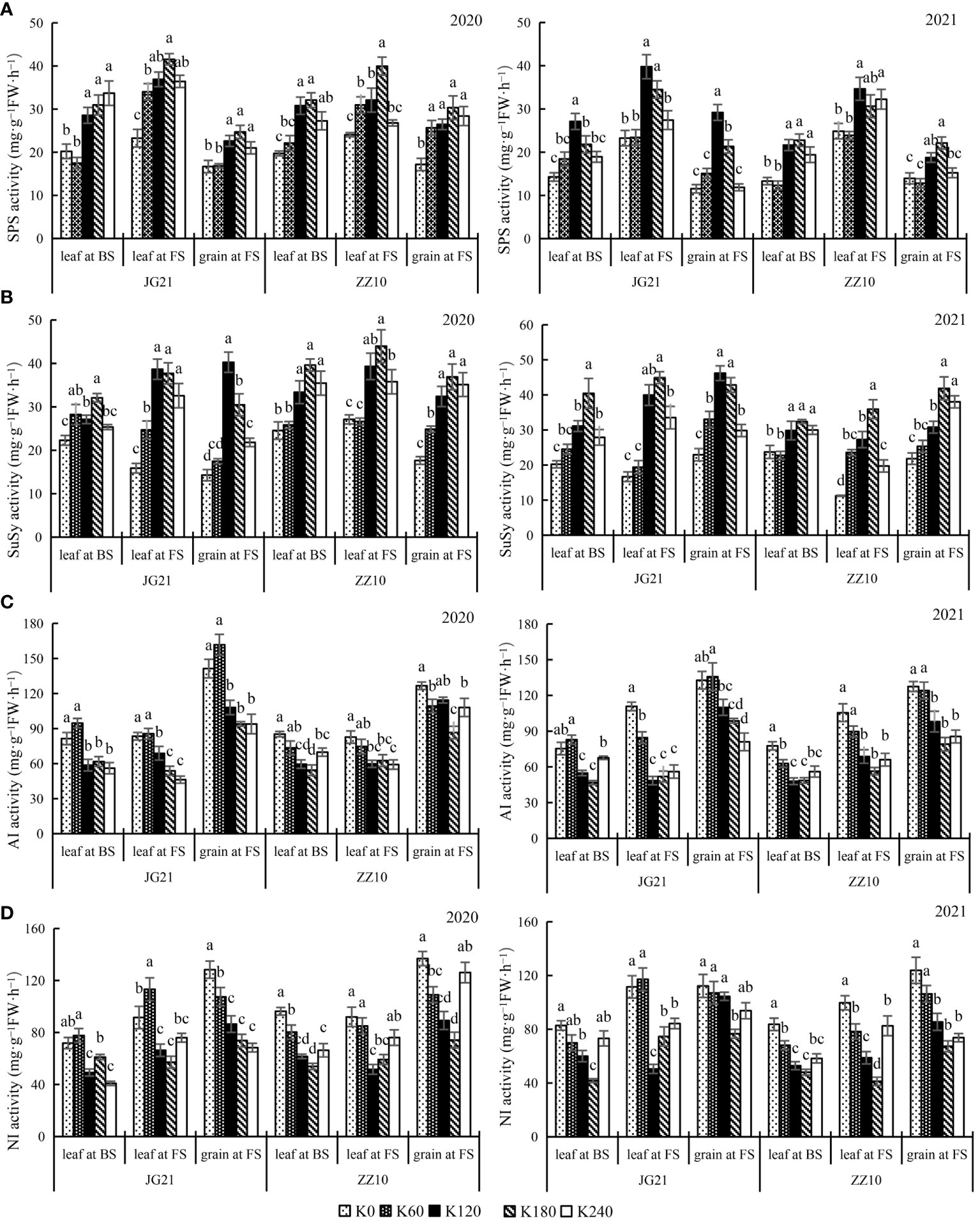
Figure 4 Effects of potassium application rate on sucrose metabolism-related enzyme activities of foxtail millet cultivars, Jingu 21 (JG21) and Zhangza 10 (ZZ10). Potassium applications include 5 level 0 (K0) as control, 60 (K60), 120 (K120), 180 (K180), and 240 (K240) kg·hm−2 of K2O. BS: booting stage, FS: filling stage. The different normal letters indicate significant differences in different treatments of the same variety at the 0.05 level. Values are means ± SE (n = 3). (A) SPS, sucrose phosphate synthase. (B) SuSy, sucrose synthase. (C) AI, Acid invertase. (D) NI, neutral invertase.
AI and NI catalyze the degradation of sucrose (Wang et al., 2019). Their response to potassium application is converse those of SPS and SuSy, indicating that potassium application results in reduced sucrose decomposition. The AI and NI activities of Jingu 21 and Zhangza 10 peaked at K0 or K60 application, whereas those in leaves showed an increasing trend as growth progressed. Moreover, AI and NI activities in grains were greater than those in leaves (Figure 4). Compared with that in the control, the AI activity of Jingu 21 was significantly decreased by 33.55% (2020) and 25.71% (2021) under K180 treatment, and the AI activity of Zhangza 10 was significantly decreased by 31.63% (2020) and 37.95% (2021) under K180 treatment (Figure 4C). Grain NI activity of Jingu 21 was significantly decreased by 42.50% (2020) and 45.83% (2021), and that of Zhangza 10 was significantly decreased by 31.70% (2020) and 45.59% (2021) (Figure 4D).
Potassium application increases soluble carbohydrate contents in grains at filling stage
Soluble sugar content reflects the carbohydrate supply in plants (Sun et al., 2022). Potassium application significantly affects the soluble sugar and sucrose content in leaves and grains. Here, the changes in soluble sugar and sucrose content in leaves and grains in response to potassium application were inconsistent. While potassium application reduced the soluble sugar and sucrose content in leaves, it increased their levels in grains (Figures 5A, B). The soluble sugar content varied as follows: leaves at the filling stage> leaves at the booting stage> grains at the filling stage. The sucrose content varied as follows: grain at the filling stage> leaf at the booting stage> leaf at the filling stage. No significant differences were observed between the soluble sugar and sucrose contents of Jingu 21 leaves in the booting and filling stages under the K120 and K180 treatments, and no significant differences were observed among the K120, K180, and K240 treatments in the booting stage. There were no significant differences in grain soluble sugar contents among the treatments in 2020 of Jingu 21; however, grain soluble sugar content peaked under the K240 treatment in 2021, which was significantly higher (6.85%) than the K0 treatment. The Jingu 21 grain sucrose content peaked under the K120 treatment in 2020, which was significantly higher (12.56%) than the K0 treatment, and peaked under the K180 treatment in 2021, which was significantly higher (14.55%) than the K0 treatment. No significant differences in grain sucrose contents were observed between 2020 and 2021 under the K120 and K180 treatments. The leaf soluble sugar content of Zhangza 10 peaked under the K0 treatment, which differed significantly from those under the K120 and K180 treatments. No significant differences in Zhangza 10 grain sucrose contents were observed among the treatments in 2020, and grain sucrose contents peaked under the K180 treatment in 2021, which was significantly higher (9.70%) than the K0 treatment. Except for the K180 treatment, there were no significant differences between the other treatments.
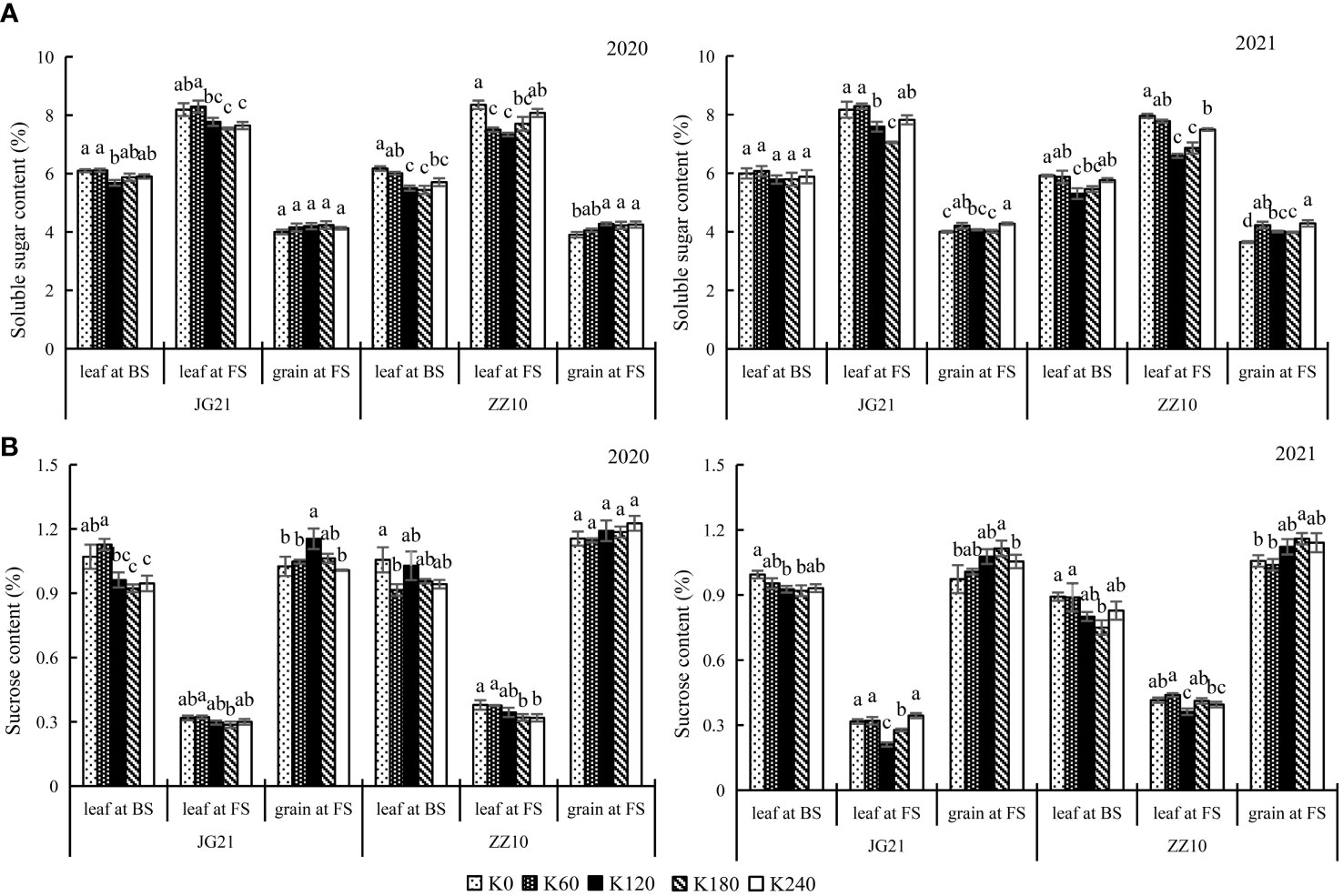
Figure 5 Effects of potassium application rate on soluble sugar and sucrose content of foxtail millet cultivars, Jingu 21 (JG21) and Zhangza 10 (ZZ10). Potassium applications include 5 level 0 (K0) as control, 60 (K60), 120 (K120), 180 (K180), and 240 (K240) kg·hm−2 of K2O. BS, booting stage, FS, filling stage. The different normal letters indicate significant differences in different treatments of the same variety at the 0.05 level. Values are means ± SE (n = 3). (A) soluble sugar content. (B) sucrose content.
Optimal potassium application enhances activity of the starch synthesizing enzyme ADPG pyrophosphorylase
ADPG pyrophosphorylase is a rate-limiting enzyme that catalyzes starch synthesis. With increasing potassium application rates, ADPG pyrophosphorylase activity showed a peaking-response. ADPG pyrophosphorylase activity in Jingu 21 peaked at the K120 and K240 (with a significant increase of 28.57% compared to that in the control). ADPG pyrophosphorylase activity in Zhangza 10 peaked at K180 (with a significant increase of 32.65% compared to that in the control) (Figure 6A). The starch content was significantly affected by potassium application, peaking at K180 application rate, and subsequently decreasing at K240 (Figure 6B). The ADPG pyrophosphorylase activity changes followed the Zhangza 10> Jingu 21 pattern, whereas the changes in starch content followed the Jingu 21> Zhangza 10 pattern. In the middle stage of grain filling, the sucrose content of Jingu 21 ranged from 0.97%–1.15%, and that of Zhangza 10 ranged from 1.04%–1.23% (Figure 5B). The Jingu 21 and Zhangza 10 cultivars had 28.99%–37.75% and 24.81%–34.62% starch contents, respectively. The sucrose/starch ratio of Jingu 21 was less than that of Zhangza 10, and the assimilate utilization ability of Jingu 21 was higher.
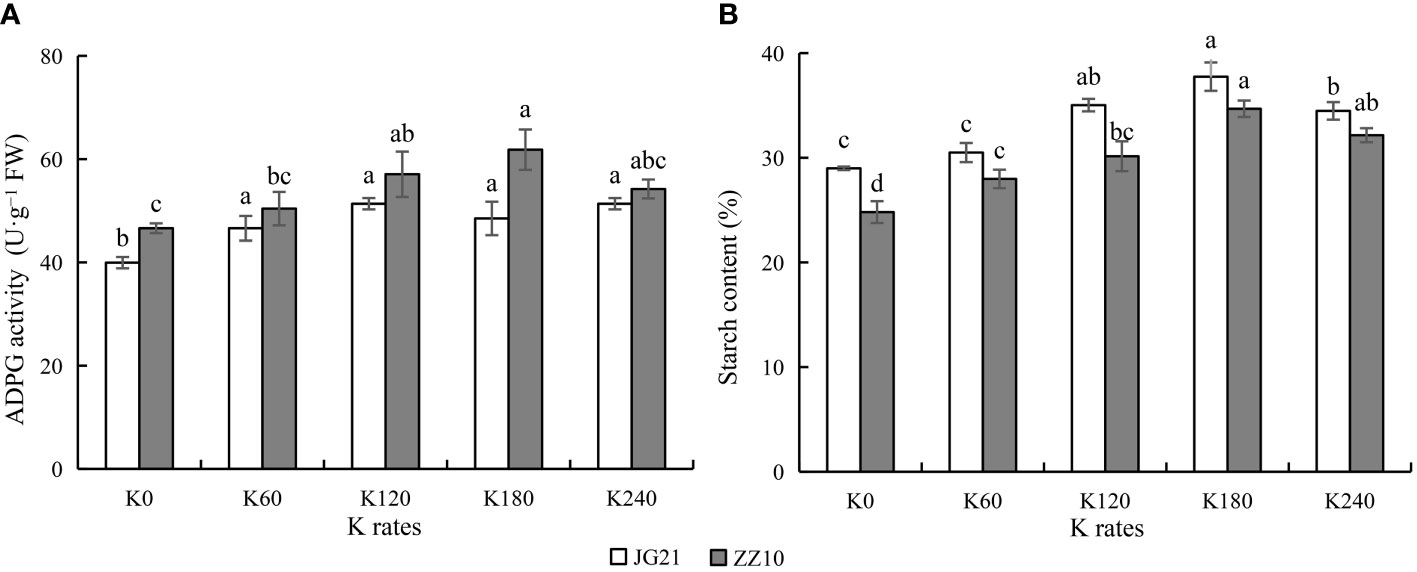
Figure 6 Effects of potassium application rate on ADPG activity and starch content of foxtail millet grains at grain filling stage (2021). Two cultivars were tested, Jingu 21 (JG21) and Zhangza 10 (ZZ10). Potassium applications include 5 level 0 (K0) as control, 60 (K60), 120 (K120), 180 (K180), and 240 (K240) kg·hm−2 of K2O. The different normal letters indicate significant differences in different treatments of the same variety at the 0.05 level. Values are means ± SE (n = 3). (A) ADPG, ADPG pyrophosphorylase. (B) starch content.
Relationship of Pn, carbohydrates, and carbohydrates metabolizing enzymes with K content
A positive correlation between Pn and the activities of PEPC, FBPase, SPS, SuSy, and K content was observed in both cultivars (Table 2). Further, a negative correlation between the activities of AI and NI, soluble sugar and sucrose, and K contents was observed in both cultivars (Table 2). The higher K content in the leaves improves the photosynthetic capacity of millet, by providing more carbon sources for carbohydrate synthesis, better coordination of the source-sink relationship, and preventing accumulation of photosynthates.

Table 2 Correlation analysis of leaf K content with Pn, carbohydrates, and carbohydrate metabolizing enzymes.
Dry matter accumulation and partitioning in the shoots of millet was enhanced by potassium application
Dry matter accumulation in the shoots of millet in different periods was improved by K application (Table 3). During the growth period, dry matter accumulation in the millet shoots increased gradually. With increasing potassium application rates, the stem, leaf, and total weights all increased. In the booting and filling stages, shoot dry matter accumulation peaked under the K180 treatment. When the potassium application reached 240 kg·hm−2 (K240), dry matter accumulation did not increase significantly. Compared with the K0 treatment, the total weights of Jingu 21 in the booting and filling stages increased by 44.40% and 15.74% (2020) and by 49.80% and 31.10% (2021), while those of Zhangza 10 increased by 20.55% and 19.37% (2020) and by 13.74% and 25.70% (2021), respectively. Compared with the K0 treatment, the K180 treatment increased the Jingu 21 grain dry matter by 16.32% (2020) and 56.22% (2021), and that of Zhangza 10 by 19.88% (2020) and 32.87% (2021). Compared with the K0 treatment, the potassium application treatments (K60, K120, K180, and K240) increased the Jingu 21 grain and total weights by 0.46% (2020) and 2.12% (2021), while those of Zhangza 10 increased by 0.49% (2020) and 0.84% (2021) on average. Potassium application increased grain dry matter accumulation; however, the percentage of grain dry matter distribution did not increase significantly.
Potassium application improves yield: Certain yield components are enhanced over others
The millet yields under different K application rates are shown in Figure 7. With increasing potassium application rate, the yield initially increased and then stabilized or decreased slightly (Table 4). The linear plus platform model was used to fit the effect equations between the potassium application rates and millet yields in 2020 and 2021. The optimal potassium application rates for Jingu 21 and Zhangza 10 were 195.25–204.27 and 173.95–175.87 kg·K2O·hm−2, respectively. Compared to the control, the Jingu 21 and Zhangza 10 yields increased by 4.03%–37.84% and 4.65%–31.56% in both years, respectively. The variations in the Jingu 21 yield were larger than those of Zhangza 10, indicating that Jingu 21 was more sensitive to potassium fertilizer application.
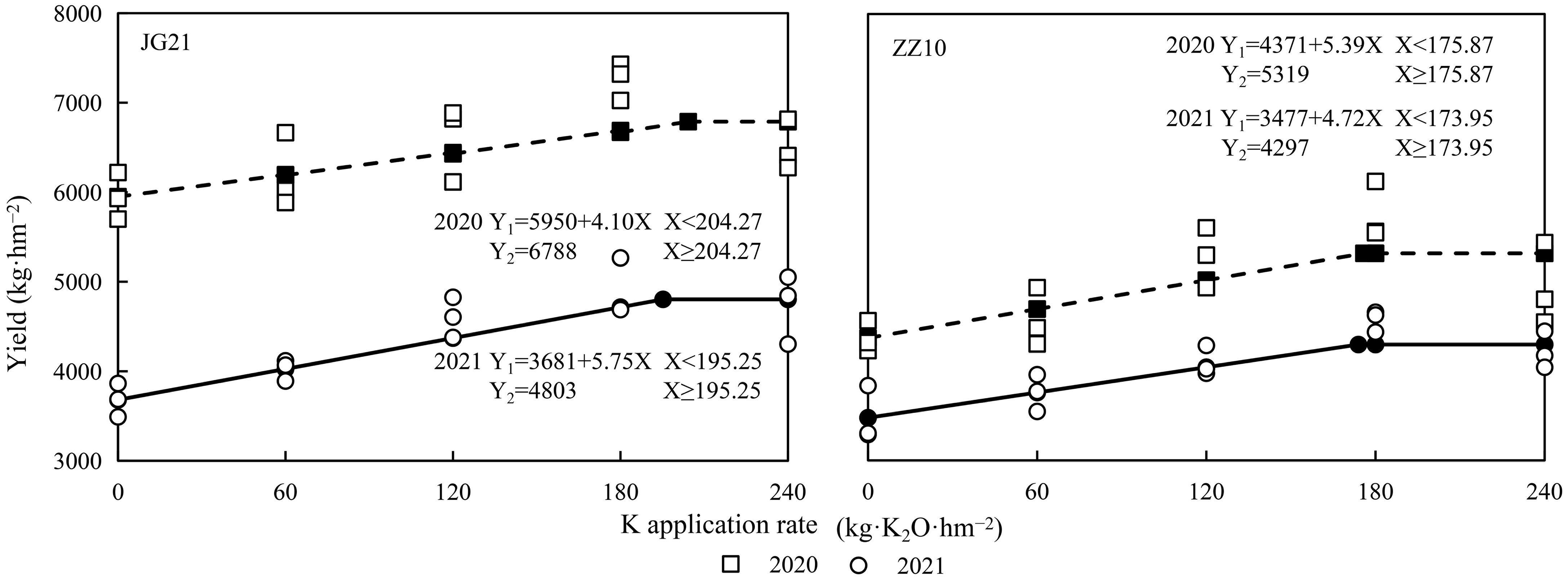
Figure 7 Effect of potassium application rate on yield of millet varieties, Jingu 21 (JG21) and Zhangza 10 (ZZ10).
The results of variance analysis showed that there were significant differences in effective panicle number, grain weight per panicle, 1000-grain weight, and yield between Jingu 21 and Zhangza 10 with different potassium application rates, and the effect on spikelet number was inconsistent for the two years (Table 4). There was no significant difference in 1000-grain weight among the cultivars, but there were significant differences in effective panicle number, spikelet number, panicle grain weight, and yield. Appropriate potassium application resulted in a significant increase in the grain weight and 1000-grain weight of millet. Compared to that in the control, the average grain weight per spike of Jingu 21 was increased by 13.07% (2020) and 18.24% (2021), and the average grain weight per spike of Zhangza 10 was increased by 9.13% (2020) and 7.05% (2021). The average 1000-grain weight of Jingu 21 and Zhangza 10 increased by 7.14% and 5.43%, respectively. Excessive potassium application did not increase the yield composition. The yield in the two years was significantly different. Compared to that in 2020, the yield in 2021 was reduced by 27.18%–38.14% for Jingu 21, and 14.40%–22.41% for Zhangza 10. This can be explained from the perspective of rainfall and temperature during the millet growth period (Figure 1). During the grain filling period (from the end of July to the end of September), the rainfall increased, and the temperature decreased. This greatly impacted the grain-filling in 2021. Zhangza 10 matures early compared to Jingu 21; thus, the impact was smaller. Compared with 2020, the grain weight per panicle of Jingu 21 decreased, the grain-filling degree decreased, and the number of shriveled grains increased. The number of spikelets in Zhangza 10 increased, while the number of effective spikelets decreased.
Stepwise regression analysis and path analysis of yield and carbon metabolism-related enzyme activities
The grain-filling stage is the critical period for millet yield formation, and carbon regulation is the essence of the source-sink relationship balance. To explore the effect of relevant factors (carbon metabolism-related enzyme activities between source and sink, and carbon metabolism-related enzymes in leaves and grains of millet at the grain filling stage) on yield, a stepwise regression analysis and path analysis was done. Leaf SPS (X1), leaf SuSy (X2), grain SPS (X3), grain SuSy (X4), leaf AI (X5), leaf NI (X6), grain AI (X7), and grain NI (X8) were set as independent variables, and millet yield (Y1: yield of Jingu 21; Y2: yield of Zhangza 10) was set as the dependent variable for the stepwise regression analysis. The following multilinear equations and fit values were obtained:
(R = 0.664, F = 15.333, P = 0.000, D = 1.347)
(R = 0.807, F = 41.475, P = 0.000, D = 2.022)
It indicated that the above indexes were the main factors affecting the formation of millet yield. The yields of both cultivars are affected by leaf SuSy and grain AI. Path analysis further clarified the regulatory effects of the indices determined by stepwise regression analysis on the yield (Table 5). The results showed that leaf and grain SuSy had the greatest direct effect on the yield of Jingu 21. The leaf SuSy of Zhangza 10 plays a leading role in yield.
Discussion
Potassium application improves photosynthetic carbon assimilation in millets
Photosynthesis is essential for numerous physiological processes that determine the growth and yield of millet. Rubisco and PEPC are critical enzymes for photosynthesis in C4 plants (such as millets). They determine the rate of carbon assimilation. Potassium deficiency reduces the photosynthetic rate and biomass in cotton; enzyme activity and content of Rubisco play an important role in this effect (Matsumura et al., 2020). Improved stomatal conductance, increased utilization of CO2 in the carbon assimilation process, and enhanced photosynthetic rate occur with an increase in potassium application rates (Lu et al., 2017). Here, with the increase of K content in leaves, Pn, the activities of Rubisco and PEPC were significantly increased at the booting and filling stages, and there was a significant positive correlation between them. Potassium application increased photosynthetic carbon fixation efficiency by increasing the photosynthetic activity. FBPase is a key enzyme in the Calvin cycle and its activity is closely related to photosynthetic efficiency, photosynthate accumulation, and distribution. FBPase has a K+ binding site, and higher potassium fertilizer application rates significantly increase FBPase activity, which subsequently regulates the accumulation and distribution of carbohydrates and promotes yield (Hu et al., 2015).
Effects of potassium application on millet photosynthetic carbohydrate metabolism enzymes activity
The leaf is the main organ for photosynthesis, and the assimilated products are transported to grains in the form of sucrose. The synthesis, transport, and accumulation of photosynthates in plants are regulated by the SuSy, SPS, AI, and NI. In C4 plants, SPS is mainly distributed in the mesophyll cells. SPS is a primary enzyme in sucrose synthesis, and its activity represents the sucrose transformation ability of flag leaf photosynthates (Binod et al., 2021; Hu et al., 2021). SuSy is a reversible enzyme with dual properties of sucrose synthesis and decomposition. The SuSy activity in grains regulates the distribution of carbohydrates in the source and sink organs (Fisher and Wang, 1995). The activities of SPS and SuSy in leaves are low in the early growth stage but increase at the functional transition stage (Wang et al., 2017). Potassium can regulate the activity of glucose metabolism enzymes, increase the reservoir strength, and promote assimilation and transport (Gerardeaux et al., 2010), as shown by several previous studies. Potassium fertilizer increases the activity of sucrose-degrading enzymes and decreases the SPS and SuSy activities at the sink, thereby preventing the resynthesis of decomposed sucrose, improving sink strength, and promoting the transport of photosynthates to the sink (Zhang et al., 2011). The sucrose degradation along with increased SuSy activity caused by potassium fertilizer helps improve sucrose metabolism and sink strength, resulting in the accumulation of soluble sugar at the sink (Wu et al., 2021). Liu et al. (2017) found that potassium application increases SPS activity in soybean seeds, decreases AI and NI activities, and increases sucrose concentration in seeds. Our study showed that sink organs increase the turgor pressure between source-sink organs and increase the SPS and SuSy activity in leaves at the filling stage. This is beneficial for increasing the supply of photosynthates at the source. Sucrose is transported to the sink (grains), where the high AI and NI activities promote sucrose hydrolysis, providing a substrate for the ADPG pyrophosphorylase-catalyzed starch synthesis. The higher activities of SuSy, AI, and NI in grains promote the continuous transport of photosynthetic products from source to sink under the action of turgor pressure differences (Figure 4). Furthermore, SPS and SuSy are primary enzymes mediating potassium sensitivity (Hu et al., 2015). Here, the activities of SPS and SuSy in different stages and parts of the plant showed that the variation range in Jingu 21 was greater than that in Zhangza 10 (Figures 4A, B). This might be one of the reasons for the different sensitivities of the cultivars to potassium. Potassium changes the balance of enzyme activities in sugar and starch metabolic processes; it increases the activities of AI, SuSy, SPS, and starch phosphorylase, and decreases the activity of ADPG pyrophosphorylase, thereby resulting in increased synthesis of hexose sugars, SuSy and ADPG pyrophosphorylase have the largest effect on enzyme activities in fruits (Luo et al., 2021). However, Gao et al. (2021) showed that potassium application significantly increases the activities of starch metabolism enzymes (such as sweet potato SuSy, and ADPG pyrophosphorylase) and starch synthases.
Effects of potassium application on accumulation and distribution of carbohydrates in millet
Sucrose is the main photosynthate in plant transport, and starch is the primary energy storage material (Omondi et al., 2020). Grain-filling involves the translocation of photosynthates from the source (leaves, leaf sheaths, and stems) to the sink (panicle), and the accumulation of photosynthates eventually forms the yield (Wang and Zhang, 2020). As an essential nutrient element affecting the growth and development of crops, K fertilizer application has a significant effect on carbohydrate transport. Potassium deficiency decreases the sucrose conversion rate, which restricts the transfer of sucrose from para-leaf to cotton bolls, and the accumulation of sucrose in leaves leads to increased specific leaf weight or changes in carbohydrate structure (Hu et al., 2016). Hu et al. (2018) found that the soluble sugar and free amino acid contents of cotton leaves decreased with potassium application. The sucrose content of tomato leaves showed a significant increase with the increase in potassium application rates (Wang et al., 2020), while the sucrose content of cotton leaves decreased with an increase in potassium application (Hu et al., 2015). Here, with potassium application, soluble sugar and sucrose content decreased in leaves and increased in grains (Figure 5). High sucrose content in the grains provides sufficient substrate for starch synthesis, which benefits yield formation. The lower sucrose/starch ratio and the higher assimilate utilization capacity may be an important reason for higher grain yield in Jingu 21 compared to that in Zhangza 10 (Figure 6).
Effects of potassium application on dry matter accumulation and yield of millet
Nutrient absorption is the physiological basis for dry matter accumulation; thus, optimizing the dry matter accumulation process is the basis for increasing crop yields (Nabin et al., 2022). Potassium application could effectively increase the K contents of each organ, and higher K applications have yielded higher proportions of stem distribution. However, despite the absorption of stem potassium, no significant changes in grain K contents have been observed (Ye et al., 2020). Potassium uptake by cereal crops mainly occurs before the flowering stage, and the K required during grain development is mainly obtained via transport from the vegetative organs (Zhang et al., 2016). Potassium deficiency restricts rice yield by affecting the potassium content, photosynthetic pigment content, and dry matter in various organs (Ranjan and Singh, 2021). Increasing potassium fertilizer rates could maintain the soil potassium balance, promote dry matter accumulation at the late growth stage, and increase the proportion of dry matter distribution in grains (Islam and Muttaleb, 2016; Hou et al., 2019). An increase in the potassium application rate resulted in improved degree of grain-filling and yield. However, excessive potassium application decreases yield (Qiu et al., 2014). In our study, potassium application significantly promoted K content and dry matter accumulation in the shoots of the two millet species but had no significant effect on the dry matter distribution ratio of the panicle. There was a significant difference in the yields of Jingu 21 and Zhangza 10 between the two years. Less rainfall during the entire growth period followed by higher rainfall during grain-filling period and concomitant drop in temperature affected grain-filling and resulted in a significant decrease in grain yield in 2021 (Figure 1). The optimal potassium application of Jingu 21 and Zhangza 10 were calculated using the linear plus platform model as 195.25–204.27 and 173.95–175.87 kg·K2O·hm−2, respectively (Figure 7).
Assimilates of carbon and nitrogen metabolism are the source materials for plant growth and development. Photosynthate regulation by carbon metabolism-related enzymes and their allocation between source and sink affect crop yield (Hu et al., 2022). Carbon metabolism is positively correlated with panicle number, panicle grain number, and 1000-grain weight (Wang et al., 2021). K+ is an activator for many enzymes and is closely related to enzyme activity. Leaf K content was positively correlated with SPS and SS, and was negatively correlated with AI and NI, which promoted the synthesis of leaf photosynthates and established a foundation for the yield. We further explored the regulatory effects of changes in carbon metabolism-related enzyme activities in the leaves and grains (during the grain-filling stage) of Jingu 21 and Zhangza 10 on yield. The yields of both cultivars were affected by leaf SuSy and grain AI activities. Jingu 21 yield was affected most by SuSy activity in both leaf and grain, which could optimize the sucrose synthesis rate and sink strength, and promote grain photosynthate accumulation under the effect of sink - source organ turgor difference. while leaf SS of Zhangza 10 played a dominant role in yield and promoted the generation of photosynthate at the source end, which laid a foundation for yield formation (Table 5).
Conclusions
Potassium fertilizer application significantly: 1) increases the K content in every organ of the millet plant during the booting and filling stages; 2) enhances the activities of photosynthetic carbon assimilation enzymes as well as sucrose and starch metabolizing enzymes in leaves; 3) promotes carbon metabolism, and carbohydrate accumulation and distribution; 4) increases dry matter accumulation in shoots; 5) promotes carbohydrate transport from source organs to grains, and; 6) improves foxtail millet yield. Potassium promotes Jingu 21 yield mainly by coordinating the source-sink relationship between leaf and grain SuSy. It promotes Zhangza 10 yield by increasing the photosynthates production via leaf SuSy activity increase. The optimal potassium application of Jingu 21 and Zhangza 10 were calculated using the linear plus platform model as 195.25–204.27 and 173.95–175.87 kg·K2O·hm−2, respectively.
Data availability statement
The original contributions presented in the study are included in the article/Supplementary Material. Further inquiries can be directed to the corresponding authors.
Author contributions
YL performed the experiment and wrote the manuscript. MY revised the manuscript. LL modified the details. JZ assisted in the experiment. XY supervised the manuscript. YW provided the ideas and revised the manuscript. All authors contributed to the article and approved the submitted version.
Funding
This work was supported by Research Program Sponsored by the State Key Laboratory of Integrative Sustainable Dryland Agriculture, Shanxi Agricultural University (No.202003-5); China Agriculture Research System of MOF and MARA (CARS-06-14.5-A28); Food System Research and Development Project in Shanxi Province, China (202001); Modern Millet Industry Technology System Project in Shanxi Province, China, and the program for the Scientific Research Foundation for the Returned Overseas Chinese Scholars, Shanxi Province(2020-068).
Conflict of interest
The authors declare that the research was conducted in the absence of any commercial or financial relationships that could be construed as a potential conflict of interest.
Publisher’s note
All claims expressed in this article are solely those of the authors and do not necessarily represent those of their affiliated organizations, or those of the publisher, the editors and the reviewers. Any product that may be evaluated in this article, or claim that may be made by its manufacturer, is not guaranteed or endorsed by the publisher.
References
Ali, S., Hafeez, A., Ma, X., Tung, S. A., Liu, A., Shah, A. N., et al. (2018). Potassium relative ratio to nitrogen considerably favors carbon metabolism in late-planted cotton at high planting density. Field Crops Res. 223, 48–56. doi: 10.1016/j.fcr.2018.04.005
Asif, M., Yilmaz, O., Ozturk, L. (2017). Potassium deficiency impedes elevated carbon dioxide-induced biomass enhancement in well watered or drought-stressed bread wheat. J. Plant Nutr. Soil Sci. 180, 474–481. doi: 10.1002/jpln.201600616
Balkos, K. D., Britto, D. T., Kronzucker, H. J. (2010). Optimization of ammonium acquisition and metabolism by potassium in rice (Oryza sativa l. cv. IR-72). Plant Cell Environ. 33, 23–34. doi: 10.1111/j.1365-3040.2009.02046.x
Binod, B.M.Y, Nasee, A. A. (2021). Comparative expression analysis of microRNAs and their targets in emerging bio-fuel crop sweet sorghum (Sorghum bicolor L.). Plant Gene 26, 100274. doi: 10.1016/J.PLGENE.2021.100274
Cao, X. Y., Hu, L. Q., Chen, X. Y., Zhang, R. Z., Cheng, D. G., Li, H. S., et al. (2019). Genome-wide analysis and identification of the low potassium stress responsive gene SiMYB3 in foxtail millet (Setariaitalica l.). BMC Genomics 20, 136. doi: 10.1186/s12864-019-5519-2
Chen, G., Zhang, Y., Ruan, B. P., Guo, L. B., Zeng, D. L., Gao, Z. Y., et al. (2018). OsHAK1 controls the vegetative growth and panicle fertility of rice by its effect on potassium-mediated sugar metabolism. Plant Sci. 274, 261–270. doi: 10.1016/j.plantsci.2018.05.034
DU, Q., Zhao, X. H., Xia, L., Jiang, C. J., Wang, X. G., Han, Y., et al. (2019). Effects of potassium deficiency on photosynthesis, chloroplast ultrastructure, ROS, and antioxidant activities in maize (Zea mays l.). J. Integr. Agr. 18, 395–406. doi: 10.1016/s2095-3119(18)61953-7
Fisher, D. B., Wang, N. (1995). Sucrose concentration gradients along the post-phloem transport pathway in the maternal tissues of developing wheat grains. Plant Physiol. 109, 587–592. doi: 10.1104/pp.109.2.587
Gao, Y., Tang, Z. H., Xia, H. Q., Sheng, M. F., Liu, M., Pan, S. Y., et al. (2021). Potassium fertilization stimulates sucrose-to-Starch conversion and root formation in sweet potato (Ipomoea batatas (L.) lam.). Int. J. Mol. Sci. 22, 4826–4826. doi: 10.3390/IJMS22094826
Gerardeaux, E., Jordan, M. L., Constantin, J., Pellerin, S., Dingkuhn, M. (2010). Changes in plant morphology and dry matter partitioning caused by potassium deficiency in gossypium hirsutum (L.). Environ. Exp. Bot. 67, 451–459. doi: 10.1016/j.envexpbot.2009.09.008
Hou, W. F., Xue, X. X., Li, X. K., Khan, M. R., Yan, J. Y., Ren, T., et al. (2019). Interactive effects of nitrogen and potassium on: Grain yield, nitrogen uptake and nitrogen use efficiency of rice in low potassium fertility soil in China. Field Crops Res. 236, 14–23. doi: 10.1016/j.fcr.2019.03.006
Hu, W., Loka, D. A., Fitzsimons, T. R., Zhou, Z. G., Oosterhuis, D. K. (2018). Potassium deficiency limits reproductive success by altering carbohydrate and protein balances in cotton (Gossypium hirsutum L.)[J]. Environ. Exp. Bot. 145, 87–94. doi: 10.1016/j.envexpbot.2017.10.024
Hu, W., Yang, J. S., Meng, Y. H., Wang, Y. H., Chen, B. L., Zhao, W. Q., et al. (2015). Potassium application affects carbohydrate metabolism in the leaf subtending the cotton (Gossypium hirsutum l.) boll and its relationship with boll biomass. Field Crops Res. 179, 120–131. doi: 10.1016/j.fcr.2015.04.017
Hu, W., Zhang, J. P., Wu, Z. Q., Loka, D. A., Zhao, W. Q., Chen, B. L., et al. (2022). Effects of single and combined exogenous application of abscisic acid and melatonin on cotton carbohydrate metabolism and yield under drought stress. Ind. Crop Prod. 176, 114302. doi: 10.1016/J.INDCROP.2021.114302
Hu, Y. M., Zhang, P., Zhang, X., Liu, Y. Q., Feng, S. S., Guo, D. W., et al. (2021). Multi-wall carbon nanotubes promote the growth of maize (Zea mays) by regulating carbon and nitrogen metabolism in leaves. J. Agr. Food Chem. 69, 4981–4991. doi: 10.1021/ACS.JAFC.1C00733
Hu, W., Zhao, W. Q., Yang, J. S., Oosterhuis, D. K., Loka, D. A., Zhou, Z. G. (2016). Relationship between potassium fertilization and nitrogen metabolism in the leaf subtending the cotton (Gossypium hirsutum l.) boll during the boll development stage. Plant Physiol. Bioch. 101, 113–123. doi: 10.1016/j.plaphy.2016.01.019
Inoue, T., Inanaga, S., Sugimoto, Y., Siddig, K. E. (2004). Contribution of pre-anthesis assimilates and current photosynthesis to grain yield, and their relationships to drought resistance in wheat cultivars grown under different soil moisture. Photosynthetica. 42, 99–104. doi: 10.1023/B:PHOT.0000040576.52128.ed
Islam, Muttaleb (2016). Effect of potassium fertilization on yield and potassium nutrition of boro rice in a wetland ecosystem of Bangladesh. Arch. Agron. Soil Sci. 62, 1530–1540. doi: 10.1080/03650340.2016.1157259
Ju, F. Y., Pang, J. L., Huo, Y. Y., Zhu, J. J., Yu, K., Sun, L. Y., et al. (2021). Potassium application alleviates the negative effects of salt stress on cotton (Gossypium hirsutum l.) yield by improving the ionic homeostasis, photosynthetic capacity and carbohydrate metabolism of the leaf subtending the cotton boll. Field Crops Res. 272, 108288. doi: 10.1016/J.FCR.2021.108288
Lian, L., Lin, Y. L., Wei, Y. D., Cai, Q. H., Huang, W., Zheng, Y. M., et al. (2021). PEPC of sugarcane regulated glutathione s-transferase and altered carbon-nitrogen metabolism under different n source concentrations in oryza sativa. BMC Plant Biol. 21, 287–287. doi: 10.1186/S12870-021-03071-W
Liu, H. J., Shi, C. Y., Zhang, H. F., Wang, Z. Z., Chai, S. S. (2013). Effects of potassium on yield, photosynthate distribution, enzymes' activity and ABA content in storage roots of sweet potato (Ipomoea batatas lam.). Aust. J. Crop Sci. 7, 735–743.
Liu, C. K., Tu, B. J., Li, Y. S., Tian, B. W., Zhang, Q. Y., Liu, X. B., et al. (2017). Potassium application affects key enzyme activities of sucrose metabolism during seed filling in vegetable soybean. Crop Sci. 57, 2707–2717. doi: 10.2135/cropsci2016.08.0648
Luo, A. R., Zhou, C. N., Chen, J. L. (2021). The associated with carbon conversion rate and source-sink enzyme activity in tomato fruit subjected to water stress and potassium application. Front. Plant Sci. 12. doi: 10.3389/FPLS.2021.681145
Lu, Z. F., Pan, Y. H., Hu, W. S., Cong, R. H., Ren, T., Guo, S. W., et al. (2017). The photosynthetic and structural differences between leaves and siliques of brassica napus exposed to potassium deficiency. BMC Plant Biol. 17, 240. doi: 10.1186/s12870-017-1201-5
Matsumura, H., Shiomi, K., Yamamoto, A., Taketani, Y., Kobayashi, N., Yoshizawa, T., et al. (2020). Hybrid rubisco with complete replacement of rice rubisco small subunits by sorghum counterparts confers C4 plant-like high catalytic activity. Mol. Plant 13, 1570–1581. doi: 10.1016/j.molp.2020.08.012
Mirza, H., Bhuyan, M. H. M. B., Kamrun, N., Hossain, Md. S., Mahmud, J. Al, Hossen, Md. S., et al. (2018). Potassium: A vital regulator of plant responses and tolerance to abiotic stresses. Agron. 8, 31–31. doi: 10.3390/agronomy8030031
Nabin, R., Raj, P. K., Renuka, S., Prasad, V. S. (2022). Accumulation of nitrogen, phosphorus, and potassium in various stages of hybrid maize (Zea mays l.) as affected by different levels of NPK in silty clay loam soil of Nepal. Commun. Soil Sci. Plan. 53, 1176–1195. doi: 10.1080/00103624.2022.2043347
Omondi, J. O., Lazarovitch, N., Rachmilevitch, S., Kukew, T., Yermiyahu, U., Yasuor, H. (2020). Potassium and storage root development: focusing on photosynthesis, metabolites and soluble carbohydrates in cassava. Physiol. Plantarum. 169, 169–178. doi: 10.1111/ppl.13060
Pan, J. F., Cui, K. H., Wei, D., Huang, J. L., Xiang, J., Nie, L. X. (2011). Relationships of nonstructural carbohydrates accumulation and translocation with yield formation in rice recombinant inbred lines under two nitrogen levels. Physiol. Plant 141, 321–331. doi: 10.1111/j.1399-3054.2010.01441.x
Qiu, S. J., Xie, J. G., Zhao, S. C., Xu, X. P., Hou, Y. P., Wang, X. F., et al. (2014). Long-term effects of potassium fertilization on yield, efficiency, and soil fertility status in a rain-fed maize system in northeast China. Field Crops Res. 163, 1–9. doi: 10.1016/j.fcr.2014.04.016
Ranjan, K. R., Singh, Y. V. (2021). Effect of potassium fertilization on growth and yield attributes of rice (Oryza sativa l.) crop in an inceptisol. J. Sci. Res. Rep. 27, 52–57. doi: 10.9734/JSRR/2021/V27I230358
Siddiqui, M. H., Mukherjee, S., Kumar, R., Alansi, S., Shah, A. A., Kalaji, H. M., et al. (2021). Potassium and melatonin regulated–Fructose-1,6-bisphosphatase(FBPase)and sedoheptulose-1,7-bisphosphatase(SBPase)activity improve photosynthetic efficiency, carbon assimilation and modulate glyoxylase system and tolerance to cadmium stress in tomato seedlings. Plant Physiol. Biochem. 171, 49–65. doi: 10.1016/J.PLAPHY.2021.12.018
Sun, C. Q., Guo, Z. L., Qu, F., Wang, X. R., Lu, C. D. (2014). The influence of different fertilization conditions on chlorophyll content of hybrid millet. Crops, 72–76. doi: 10.16035/j.issn.1001-7283.2014.03.019
Sun, L. L., Wang, J. L., Lian, L. Q., Song, J., Du, X. N., Liu., W. K., et al. (2022). Systematic analysis of the sugar accumulation mechanism in sucrose- and hexose- accumulating cherry tomato fruits. BMC Plant Biol. 22, 303. doi: 10.1186/S12870-022-03685-8
Wang, J. W., Huang, K., Dong, Y. X., Li, Z. Q., Ji, Y. N., Wu, Q. Y. (2020). Effects of potassium and nitrogen interaction on carbon and nitrogen metabolism of tomato leaves, fruit yield and quality. China Vegetables., 41–49.
Wang, S. Y., Liu, Q., Shi, Y. X., Li, H. (2017). Interactive effects of nitrogen and potassium on photosynthesis product distribution and accumulation of sweet potato. Sci. Agric. Sin. 50, 2706–2716. doi: 10.3864/j.issn.0578-1752.2017.14.007
Wang, J. F., Wang, Z. Z., Gu, F. X., Mou, H. M., Wang, N., Duan, J. Z., et al. (2021). Effects of nitrogen fertilizer and plant density on carbon metabolism, nitrogen metabolism and grain yield of two winter wheat varieties. Sci. Agric. Sin. 54, 4070–4083. doi: 10.3864/j.issn.0578-1752.2021.19.004
Wang, T., Wright, D., Xu, H., Yang, Y., Zheng, R., Shi, J., et al. (2019). Expression patterns, activities and sugar metabolism regulation of sucrose phosphate synthase, sucrose synthase, neutral invertase and soluble acid invertase in different goji cultivars during fruit development. Russ. J. Plant Physiol. 66, 29–40. doi: 10.1134/S1021443719010199
Wang, X. D., Yu, Z. W., Wang, D. (2003). Effect of potassium on carbohydrate contents in stem and sheath and starch accumulation in kernel of wheat. Plant Nutr. Fert. Sci. 9, 57–62. doi: 10.3321/j.issn:1008-505X.2003.01.010
Wang, G. Q., Zhang, J. H. (2020). Carbohydrate, hormone and enzyme regulations of rice grain filling under post-anthesis soil drying. Environ. Exp. Bot. 178, 104165. doi: 10.1016/j.envexpbot.2020.104165
Wang, X. G., Zhao, X. H., Jiang, C. J., Li, C. H., Cong, S., Wu, D., et al. (2015). Effects of potassium deficiency on photosynthesis and photoprotection mechanisms in soybean (Glycine max (L.) merr.). J. Integr. Agr. 14, 856–863. doi: 10.1016/S2095-3119(14)60848-0
Wu, S. W., Zhang, C. M., Li, M., Tan, Q. L., Sun, X. C., Pan, Z. Y., et al. (2021). Effects of potassium on fruit soluble sugar and citrate accumulations in cara cara navel orange (Citrus sinensis l. osbeck). Sci. Hortic-amsterdam. 283, 110057. doi: 10.1016/J.SCIENTA.2021.110057
Xie, W. Y., Zhou, H. P., Guan, C. L., Jia, W. (2009). Characteristics of n, p and K uptake at different growth stages of spring millet on dry land. Chin. Agric. Sci. Bulletin. 25, 158–163.
Xu, X. X., Wang, F., Xing, Y., Liu, J. Q., Lv, M. X., Meng, H., et al. (2022). Appropriate and constant potassium supply promotes the growth of M9T337 apple rootstocks by regulating endogenous hormones and carbon and nitrogen metabolism. Front. Plant Sci. 13. doi: 10.3389/FPLS.2022.827478
Yang, H. J., Yuan, X. Y., Guo, P. Y., Dong, S. Q., Zhang, L. G., Wen, Y. Y., et al. (2017). Effects of brassinolide on photosynthesis, chlorophyll fluorescence characteristics and carbohydrates metabolism in leaves of foxtail millet (Setaria italica) under sigma broad stress. Sci. Agric. Sin. 50, 2508–2518. doi: 10.3864/j.issn.0578-1752.2017.13.010
Ye, T. H., Xue, X. X., Lu, J. W., Hou, W. F., Ren, T., Cong, R. H., et al. (2020). Yield and potassium uptake of rice as affected by potassium rate in the middle reaches of the Yangtze river, China. Agron. J. 112, 1318–1329. doi: 10.1002/agj2.20092
Zahoor, R., Dong, H. R., Abid, M., Zhao, W. Q., Wang, Y. H., Zhou, Z. G. (2017). Potassium fertilizer improves drought stress alleviation potential in cotton by enhancing photosynthesis and carbohydrate metabolism. Environ. Exp. Bot. 137, 73–83. doi: 10.1016/j.envexpbot.2017.02.002
Zhang, Y. K., Chen, H. Z., Zhang, Y. P., Xiang, J., Zhu, D. F. (2016) Accumulation and distribution of dry matter and potassium in mid-season indica rice cultivars applied at different decades. Acta Agric. Boreali-Sinica. 31, 227–232. doi: 10.7668/hbnxb.2016.06.035
Zhang, X. M., Du, L. Q., Xie, J. H., Dou, M. A., Yao, Y. L., Sun, G. M. (2011). Effects of different potassium fertilization on sugar content and related metabolic enzymes of pineapple during development. Chin. J. Trop. Crop 32, 229–234. doi: 10.3969/j.issn.1000-2561.2011.02.009
Keywords: foxtail millet, potassium fertilization, photosynthetic characteristics, carbohydrate metabolism, yield
Citation: Li Y, Yin M, Li L, Zheng J, Yuan X and Wen Y (2022) Optimized potassium application rate increases foxtail millet grain yield by improving photosynthetic carbohydrate metabolism. Front. Plant Sci. 13:1044065. doi: 10.3389/fpls.2022.1044065
Received: 14 September 2022; Accepted: 15 November 2022;
Published: 30 November 2022.
Edited by:
Ep Heuvelink, Wageningen University and Research, NetherlandsReviewed by:
Renato De Mello Prado, São Paulo State University, BrazilHonghai Luo, Shihezi University, China
Copyright © 2022 Li, Yin, Li, Zheng, Yuan and Wen. This is an open-access article distributed under the terms of the Creative Commons Attribution License (CC BY). The use, distribution or reproduction in other forums is permitted, provided the original author(s) and the copyright owner(s) are credited and that the original publication in this journal is cited, in accordance with accepted academic practice. No use, distribution or reproduction is permitted which does not comply with these terms.
*Correspondence: Yinyuan Wen, wenyinyuan@126.com; Xiangyang Yuan, Yuanxiangyang200@163.com
†These authors share first authorship