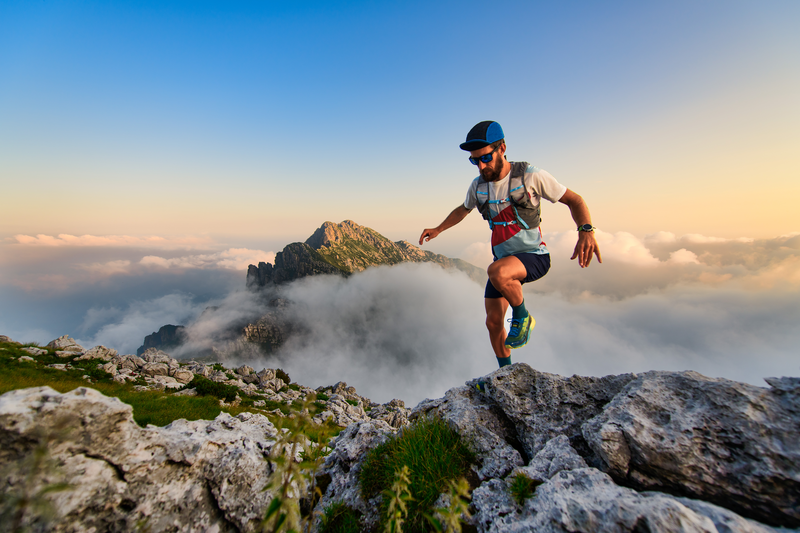
94% of researchers rate our articles as excellent or good
Learn more about the work of our research integrity team to safeguard the quality of each article we publish.
Find out more
ORIGINAL RESEARCH article
Front. Plant Sci. , 21 November 2022
Sec. Plant Bioinformatics
Volume 13 - 2022 | https://doi.org/10.3389/fpls.2022.1042999
This article is part of the Research Topic Creation and utilization of crop germplasm resources View all 18 articles
The rising temperatures are seriously impacting the food crops, including urdbean; hence efforts are needed to identify the sources of heat tolerance in such crops to ensure global food security. In the present study, urdbean genotypes were evaluated for heat tolerance under natural outdoor for two consecutive years (2018, 2019) and subsequently in the controlled environment of the growth chamber to identify high temperature tolerant lines. The genotypes were assessed involving few physiological traits (membrane damage, chlorophyll, photosynthetic efficiency, stomatal conductance, lipid peroxidation), reproductive traits (pollen germination % and pollen viability %) and yield related traits (total number of pods plant-1, total seeds plant-1, single seed weight and seed yield plant-1). Based upon these tested traits, PantU31, Mash114, UTTARA and IPU18-04 genotypes were identified as promising genotypes for both years under heat stress condition. Further confirming heat tolerance, all these four tolerant and four sensitive genotypes were tested under controlled environment under growth chamber condition. All these four genotypes PantU31, Mash114, UTTARA and IPU18-04 showed high chlorophyll content, photosynthetic efficiency, stomatal conductance, leaf area, pods plant-1, total seeds plant-1 and low reduction in pollen germination % and pollen viability under stress heat stress condition. Moreover, yield and yield related traits viz., pods plant-1, seeds plant-1, single seed weight and seed yield plant-1 showed very strong positive correlation with pollen germination and pollen viability except electrolyte leakage and malondialdehyde content. Thus, these genotypes could be potentially used as donors for transferring heat tolerance trait to the elite yet heat-sensitive urdbean cultivars.
Due to global climate change projections, heat waves are predicted to expand in many regions of the world imposing a huge threat to the agricultural security (Riaz et al., 2021). Variability around the optimum temperature is going to surge that will affect the complete life cycle or phenology of the plant (Chaturvedi et al., 2021). Continuously rising temperature (heat stress) has wide range of impacts on the overall morphology, anatomy and physiology of the plants (Chen et al., 2014). At sub-cellular levels, these impacts can be assessed using various biochemical and molecular approaches. Although heat stress has the potential to affect all the stages of plant but some stages are more vulnerable to heat stress; reproductive or seed filling stages are highly affected due to heat stress (Allakhverdiev et al., 2008; Hedhly, 2011). Moreover, the effects of high temperature are plant species- and stage- specific, the severity further depends on the duration and intensity of stress (Li et al., 2018).Various reports have suggested that heat stress disturbs the morphology of the plant by reducing its plant height, leaf area and root architecture (Chaudhary et al., 2020). At the cellular level, heat stress leads to protein denaturation, enzyme inactivation, membrane damage, exaggerate ROS generation, loss in water status and cellular viability. In leaves, photosynthetic machinery is reported as most sensitive to high temperature (Wahid et al., 2007; Bita and Gerats, 2013). Loss of chlorophyll content, denaturation of D1 protein of photosystem II and reduced carbon metabolism are mainly responsible for reducing photosynthetic rate (Allakhverdiev et al., 2008).Of the reproductive organ development stages; male gametophyte development and seed filling processes are reported to be extremely sensitive to even a few degree rise in temperature that can result in substantial yield loss (Hedhly, 2011). Impaired transport of sucrose to the developing reproductive organs under heat stress may restrict the flower development that brings out more flower abortion, pod abortion and shrivelled seeds (Bhandari et al., 2016). Plant responds to such damages by reprogramming and activating various mechanisms related to production of antioxidants, phytohormones, osmolytes, primary and secondary metabolites to ensure their survival (Chebrolu et al., 2016; Sharma et al., 2016; Jha et al., 2022). Therefore, correlation of crop phenology with temperature fluctuations is crucial for the better understanding of the impacts and defence strategies employed by plants for its adaptation.
Urdbean (Vigna mungo L. Hepper) is an important summer season food legume, cultivated mostly in many tropical and sub-tropical countries of Asia, Africa, America, and Australia (Joshi and Rao, 2017).Optimum temperature for its proper growth and development is 25-35°C and being a temperature sensitive crop, its yield is drastically reduced under high temperature exceeding 35°C (Anitha et al., 2016; Sen Gupta et al., 2021).Very little reports are available about the heat stress impacts as well as defence responses, especially at reproductive stage of this crop (Sen Gupta et al., 2021). It is vital to identify and characterise heat tolerant urdbean genotypes as well as to find out some leaf and pollen-based traits and mechanisms underlying heat tolerance. Heat tolerant urdbean genotypes can increase the cultivation of this food legume in summer season as well at warmer locations to extend its cultivation status. Thus, the aim of the present study was to screen selective number of genotypes of urdbean to heat stress in 2 successive years under outdoor environment to identify heat tolerant genotypes, followed by their validation and characterisation under controlled environment of the growth chamber involving some leaf and pollen-based traits.
Urdbean genotypes (26) were procured from Indian Institute of Pulse Research, Kanpur, India and Punjab Agricultural University, Ludhiana, India (Supplementary Table S1). These genotypes were assessed for their heat tolerance under outdoor environment and controlled conditions of the growth chamber at the Department of Botany, Panjab University, Chandigarh, India. Urdbean seeds were raised in pots (8L capacity) containing a mixture of soil, sand, farmyard manure [2:1:1 (v/v)] and Tri-calcium phosphate fertilizer 10 mg kg-1. Seeds were soaked in distilled water overnight (12 h) and subsequently inoculated with suitable strain of Rhizobia before sowing. There were 5 pots genotype-1 and each pot had 5 seeds that were thinned to 3 plants pot-1 after emergence for their proper growth. Plants were fully irrigated daily (twice; morning and evening) to avoid any water paucity. Plants were arranged in a randomized complete block design. Meteorological data (daily temperature and relative humidity) from date of sowing to harvesting was recorded throughout the entire cropping season (Supplementary Figure S1). To evaluate the effects of heat stress against the control temperature, crop was sown twice during cropping season and for two subsequent years (Summers of 2018, 2019) (i) during the normal conditions (control), in the last week of March 2018, when the day/night temperatures (<35/25°C) were optimum for the plant’s growth and ii) in the last week of April, to expose the plants to heat stress (>40/30°C). (Supplementary Figure S1, Supplementary Table S2). The plants after harvest were recorded for number of pods, seeds, seed yield plant-1 and single seed weight.
For validation of the results, a subsequent study was conducted in the growth chamber under the controlled conditions on some selected contrasting genotypes (4 heat-tolerant and 4 heat- sensitive genotypes, 5 pots genotype-1 having 2 plants pot-1). These plants were initially raised in the outdoor natural environment to achieve full vegetative growth (Average temperature<35/25°C; average RH 61/41%; Max/min) and were subsequently transferred to growth chamber at the onset of bud stage for further analysis. To avoid any kind of heat shock situation, temperature was gradually raised (2°C per day) up to 42/32°C. The plants were maintained at this temperature up to maturity. Simultaneously, the control plants were maintained at 35/25°C.
After 10d of heat stress, fully expanded leaves at 2nd and 3rd positions from the topmost youngest leaf were from control and heat-stressed environments were evaluated for various physiological traits viz. SPAD chlorophyll content, chlorophyll fluorescence (Fv/Fm), electrolyte leakage (to assess membrane damage), stomatal conductance, leaf area, relative leaf water content, and malondialdehyde (MDA). The reproductive traits (pollen viability and pollen germination) were tested from flowers after 5d exposure to heat stress. All these traits were further correlated with yield traits like total number of pods plant-1, total number of seeds plant-1, total seed yield plant-1, single seed weight.
To assess the effects of heat stress on the plant growth and yield, various traits were studied; data were taken from three plants in triplicates genotype-1, pooled and averaged. Mean values of replicates are presented through tables and figures.
Chlorophyll content (SPAD value) was measured using Apogee-SPAD meter and its readings were taken between 10.00 and 11.00 h of a fully expanded tagged leaf on alternative days at full vegetative and reproductive stage from 30 DAS (days after sowing) (Devi et al., 2022).
PS II activity/stability or photosynthetic efficiency was measured as chlorophyll fluorescence. Readings were taken between 10.00-11.00 h of a fully expanded leaf using the dark adapted test of modulated chlorophyll fluorometer OS1-FL (Opti-Sciences, Tyngsboro, MA, United States) (Sharma et al., 2016).
Stress injury to leaves was measured as electrolyte leakage. Fresh leaves (1.0 g) were collected and washed three times with deionised water to remove surface adhering electrolytes. Plant tissue was placed in closed vials containing 10 ml of deionised water and incubated it for 25°C on a rotary shaker for 24 h; the electrical conductivity of the solution (L1) was checked using a conductivity meter (ELICO CM 180, Hyderabad, India). Then the final conductivity (L2) was measured after heating samples in a water bath at 120°C for 20 min (Lutts et al., 1996). Electrolyte leakage was calculated as (L1/L2) × 100. The electrolyte leakage was expressed as electrical conductivity in µmhos g–1 DW.
Stomatal conductance was measured from a fully expanded leaf using a portable leaf porometer (model SC1 Decagon Devices, Pullman, WA, United States) at 11.00 h and was expressed as m molm-2s-1 (Awasthi et al., 2014).
Area of fully expanded tagged leaves was determined using a measurement scale and multiplied with a ‘leaf factor’ (method derived from urdbean from the ratio of actual and measured leaf area of many types of leaves from top to bottom of a plant) (Sharma et al., 2016).
RLWC was measured by the method of Barrs and Weatherley (1962). Fresh leaves were collected and were washed three times to remove any kind of debris. After drying with blotters, they were weighed (fresh weight, FW) and then floated in the distilled water in a petri dish. After 2 h, leaves were taken out of petri dish, reweighed and surface dried with blotters. Leaves were then oven-dried at 110°C for 24h and again weighed for dry weight (DW). Final values for relative leaf water content was calculated as (FW-DW)/(TW-DW) × 100.
Lipid peroxidation of the cell membrane was measured as malondialdehyde (MDA) content (Heath and Packer, 1968). One hundred mg fresh leaf tissue was extracted in 10 mL of 0.1% trichloroacetic acid (TCA). The homogenate was centrifuged at 15,000 rpm for 5 min. Supernatant was used as extract. Afterward, 4 mL of 0.5% thiobarbituric acid (in 20% trichloroacetic acid) was added to a 1-ml of the supernatant. This mixture was heated at 95°C for 30 minutes followed by immediate cooling in ice bath. Re-centrifugation of this mixture was performed again at 10,000 rpm for 10 min and the absorbance of the supernatant was taken at 532 nm. Values were expressed as nmol g-1 DW.
For evaluating reproductive function, flowers were collected 5 days after exposure to heat stress and assessed for following traits.
For testing pollen germination, pollen grain samples were taken in three replicates and each replicate consisted of five flowers genotype-1 (Brewbaker and Kwack, 1963). Pollen grains were collected and immersed in few drops of pollen germinating medium (10% sucrose, 990 mM potassium nitrate (pH 6.5), 1.64 mM boric acid, 812 mM magnesium sulphate and 1.3 mM calcium nitrate) (Kaushal et al., 2013).
Around 100 pollen grains were tested for the pollen viability with 0.5% acetocarmine/Alexander stain per genotype in three replicates. Selection of viable pollen grains was made on the basis of size (fully expanded), shape (triangular or spherical) and concentration of stain taken by them. Pollen grains were collected from freshly opened flowers and were pooled and checked for their viability (Kaushal et al., 2013).
For obtaining yield data, three plants genotype-1 in three replications (9 plants genotype-1) were harvested at maturity, wrapped in paper bags and dried in an oven at 65°C for at least three days. After drying, the total number of pods and seeds, total seed weight and single seed weight plant-1 were calculated (Sharma et al., 2016).
Urdbean plants were grown in outdoor environment for 2 consecutive years as well as under controlled environment of the growth chamber using RCBD. The analysis of data for computing standard errors and least significant differences (P<0.05) was performed using 2-factorial (temperature × genotypes) design using OPSTAT statistical software (CCS, HAU, Hisar, India). Genotypic correlation, heritability were analysed by using GenStat 15 software. The Euclidean dissimilarity matrix was constructed involving all the genotypes and traits, and were clustered using Ward’s method (Patterson and Thompson, 1971). The principal component analysis was done using the R package factoextra, and heat map analysis was performed according to Babicki et al. (2016).
Electrolyte leakage (EL%) is one of the important physiological traits measuring membrane damage used for screening heat stress tolerant genotypes in plants. Heat stress significantly (P<0.01) damaged the membranes (Supplementary Figure S2, Supplementary Table S3). EL increased by 49 and 51% in heat-stressed plants, compared to controls, in the first and second years, respectively. Based on this trait, Mash 114 (18.5%, 17.73%), PantU31(21.77%, 20.8%), UTTARA (21.43%, 20.73%), IPU18-04 (18.17%, 19.73%) genotypes revealed low value for EL% under heat stress environment for both years. However, the genotypes IPU 18-6 (25.13%, 26.9%), Mash 218 (26.47%, 26%), SuG1153 (26.23%, 26.9%) exhibited high value for EL% under heat stress environment for both years suggesting their heat stress sensitivity. The high heritability values (82.6% and 86.85, for first and second years, respectively) for this trait were noted under heat stress see Table 1.
Stomatal conductance (gS) varied significantly (P<0.01) across the genotypes in plants exposed to high temperature (Supplementary Figure S3, Supplementary Table S3). As a result of high temperature, gS decreased by 12 and 15% over control in 1st and 2nd year, respectively. Under heat stress environment, Mash 114 (45.6, 40.47) and Pant G 31(43.9, 46.53) genotypes showed high value for stomatal conductance in both years. Regarding heritability for gS, 96.5% (during the first year) and 95.2% (during second year) heritability values were noted (Table 1).
A significant genetic variation (P<0.01) was noticed in chlorophyll content among the genotypes under heat stress (Supplementary Figure S4, Supplementary Table S3). The range of leaf chlorophyll content was noted to be 11.4-19.8 mg g-1 FW during the first year and 12.3- 21.3 mg g-1 FW during second year under heat stress environment. An average reduction of 22 and 30% was observed due to heat stress, relative to controls, in 1st and 2nd year, respectively. High value for chlorophyll content was observed in Mash 114 (19.67, 18.97), PantG31 (19.77, 20.47), UTTARA (17, 20.4) genotypes under heat stress environment for both the years. This trait also showed high heritability (85.5% and 92.7% for 1st and 2nd years, respectively) and could be vital for selecting heat tolerant urdbean lines (Table 1).
Significant genetic variability for chlorophyll fluorescence (ChlF) (Fv/Fm) was noted under heat stress environment (P<0.01) (Supplementary Figure S5, Supplementary Table S3). Mean value for Fv/Fm was noted to be 0.53 during the first year and 0.54 during second year under heat stress environment. Heat stress caused about 28% reduction over control in both the years. The genotypes Mash 114 (0.61, 0.66), PantU31(0.61, 0.65), UTTARA (0.6,0.65) showed high value for ChlF under heat stress for both years. Heritability for this trait was noted to be 90.1% and 97.3% during the first year and second year, respectively (Table 1).
Significant genetic variation (P<0.01) was noted in leaf area (LA) among the tested genotypes under hot environment for both years (Supplementary Figure S6, Supplementary Table S3). LA decreased by 23 and 28% in heat-stressed plants, over controls, in the 1st and 2nd years, respectively. Substantial genetic variability for this trait was noted under heat stress environment ranging from 13.9-21.4 cm2 (during the first year) and 14.7-20.6 cm2 (during second year). High heritability (83.7%) recorded during the first year and 89.8% during second year, suggested that this trait could be used for screening heat tolerance in urdbean (Table 1).
Significant (P<0.01) genetic variability for pods plant-1 (Figure 1) seeds plant-1 (Figure 2), seed yield plant-1 (Figure 3) and single seed weight (Figure 4) were recorded under heat stress environment for both years (Supplementary Table S3).
Figure 1 Pod number plant -1 of Urdbean genotypes under control (normal-sown; Control) and heat stress environment during 2018 (A),2019 (B) and in controlled environment of growth chamber (GC; C). LSD values (P < 0.05); genotype × treatment: 2.6 (2018), 3.1 (2019), 3.46 (GC). Values are means + SE. (n = 3).
Figure 2 Seed number plant -1 of Urdbean genotypes under control (normal-sown; Control) and heat stress environment during 2018 (A),2019 (B) and in controlled environment of growth chamber (C; GC). LSD values (P < 0.05); genotype × treatment:6.9 (2018), 7.5 (2019), 12.1 (GC). Values are means + SE. (n = 3).
Figure 3 Seed yield plant -1 of Urdbean genotypes under control (normal-sown; Control) and heat stress environment during 2018 (A),2019 (B) and in controlled environment of growth chamber (C; GC). LSD values (P < 0.05); genotype × treatment: 1.3 (2018), 1.5 (2019), 1.3 (GC). Values are means + SE. (n = 3).
Figure 4 Single seed weight of Urdbean genotypes under control (normal-sown; Control) and heat stress environment during 2018 (A), 2019 (B) and in controlled environment of growth chamber (C;GC). LSD values (P < 0.05); genotype × treatment: 0.0023 (2018), 0.0021 (2019), 0.0018 (GC). Values are means + SE. (n = 3).
Under high temperature, maximum pods plant-1 decreased by 82.4 and 83.7%, maximum seeds plant-1 by 94.7 and 94.3%, maximum seed yield plant-1 by 91.5 and 95.2% and single seed weight by 26 and 32% over the respective controls in 1st and 2nd year, respectively. The UTTARA genotype retained highest pod number plant-1 (15, 16) followed by PantU31(15,15), IPU18-04 (14,16) under heat stress environment for both years. For seeds plant-1 trait, Mash114 (63, 66), UTTARA (63, 71) and IPU18-04 (62, 63) showed promising results under heat stress environment for both years. Likewise, Mash114 (47.7%, 41.6%), IPU18-04 (43.34%, 48.9%), UTTARA (57.39%, 38%), and PantU31(55.45%, 28.7%) showed lower reduction percentage for seed yield plant-1 for both years, and thus could be highly heat tolerant genotypes. Under heat stress environment, high heritability with 98.5%, 99.4% and 99.2% was noted for pods plant-1, seeds plant-1 and seed yield plant-1, respectively during the first year. Similarly, these traits showed high heritability under hot environment during second year also (Table 1).
Significant genetic variability for the various evaluated traits was recorded in twenty-six selected urdbean genotypes under normal and heat stress condition in both years (Supplementary Table S3). Based on the various physiological and yield and yield related parameters, the following genotypes Mash 114, PantU31, IPU18-04 and UTTARA were identified to be heat tolerant for both years under hot environment. Contrastingly, Mash 118, SuG1153, IPU18-5, IPU5(19-46) were identified to be highly sensitive to heat stress for both years.
To validate the response of selected heat tolerant and sensitive genotypes, a selected set of 4 heat tolerant and 4 heat-sensitive urdbean genotypes among the 26 genotypes were examined under growth chamber subjecting them to normal and heat stress treatments, separately. Among these selected 4 heat tolerant genotypes, Mash114 and IPU18-04 revealed high tolerance to heat stress, evidenced by high number of pods plant-1 (14.5,14.2), high seed number plant-1 (52.5, 46.5) and high efficiency of various physiological traits (chlorophyll content (Supplementary Figure S4), chlorophyll fluorescence (Supplementary Figure S5), stomatal conductance (Supplementary Figure S6), low electrolyte leakage (Supplementary Figure S2), and low malondialdehyde content (Supplementary Figure S7) and reproductive traits [high pollen germination (57.4%, 52.5%) and viability percentage (61.2%, 57.1%)] under heat stress environment (see Supplementary Figure S7).
However, among the heat-sensitive genotypes, IPU5-(19-46) and IPU-18-5 showed high heat stress sensitivity, evidenced by high reduction of yield and yield-related traits as well as physiological and reproductive traits.
Pod number plant-1 showed significant positive correlations with traits-chlorophyll content, chlorophyll fluorescence, leaf area and stomatal conductance-directly contributing to photosynthesis process whereas significant and negative association of electrolyte leakage (EL) percentage was noticed (Table 2). EL also showed negative correlation with traits (chlorophyll content, chlorophyll fluorescence, leaf area and stomatal conductance), yield traits such as pods plant-1, seeds plant-1, single seed weight and seed yield plant-1 under heat stress environment during both the years.
Table 2 Correlation coefficients of various traits with yield traits in plants under heat stress environment.
In urdbean plants grown under growth chamber condition, subjected to heat stress, electrolyte leakage and malondialdehyde (an indicator of oxidative stress) showed highly significant negative correlation with all the physiological traits viz., chlorophyll content, chlorophyll fluorescence, stomatal conductance, relative water content, leaf area, pollen germination % and pollen viability % and yield traits viz. pods plant-1, seed yield plant-1 (Table 2).
High and significant positive correlation of pollen germination % and pollen viability % were noticed with all the traits except electrolyte leakage and malondialdehyde. Likewise, stomatal conductance and RLWC also exhibited high and positive correlation with all the traits except electrolyte leakage and malondialdehyde. The yield and yield related traits viz., pods plant-1, seeds plant-1, single seed weight and seed yield plant-1 showed very strong positive correlation with pollen germination and pollen viability (Table 2) suggesting these traits as vital for screening heat tolerant urdbean genotypes.
During the first year, under heat stress environment, PCA analysis (Figure 5) revealed five principal components correlated to 9 traits accounted for 96.5% of total variability. The individual contribution of each component was 76.8%, 6.84%, 5.18%, 4.23% and 3.38%. Analysis of factor loadings of the traits in the retained PCs suggested that seed yield plant-1 (SPY) (13.76), seeds plant-1(SPP) (13.45) and pods plant-1; PPP (13.12) contributed most positively. In PC2, leaf area (LA) contributed most positively. The trait chlorophyll fluorescence (ChlF) (58.47), chlorophyll (Chl) (76.45) and electrolyte leakage (EL%) (45.1) had highest contribution to PC3, PC4 and PC5, respectively.
Figure 5 Principal component analysis (PCA) of various traits in Urdbean genotypes under heat stress in the year 2018.
Likewise, during second year, PCA analysis (Figure 6) indicated five principal components correlating to 9 traits contributed 97.9% to the total variability. The individual contribution of each component was 81.2%, 9.3%, 3.62%, 2.51% and 1.29%. PPP (12.6) had the highest contribution to PC1. Likewise, LA (50.69) contributed with highest positive value to PC2. EL% (57.38), ChlF (40.18) and Chl (53.1) had highest positive contribution to PC3, PC4 and PC5, respectively.
Figure 6 Principal component analysis (PCA) of various traits in urdbean genotypes under heat stress in the year 2019.
Under growth chamber, PCA analysis (Figure 7) suggested five PCAs attributing to 13 traits contributing 99.8% to the total variability. The individual contribution of each component was PC1 (97.5%), PC2 (1.03%), PC3 (0.64%), PC4 (0.35%) and PC5 (0.30%). Chlorophyll content (7.81) had the highest contribution to PC1, while single seed weight (32.58) had the highest contribution to PC2. Electrolyte leakage (30.42%) had the highest contribution to PC3 and stomatal conductance (42.09) had the highest contribution to PC4. Seeds plant-1 (32.98) had the highest contribution to PC5.
Figure 7 Principal component analysis (PCA) of various traits in urdbean genotypes under heat stress in a growth chamber.
Based on the heat map analysis considering all the physiological and yield-related traits evaluated during the first year in all, the 26 genotypes revealed three major clusters.
Regarding first year, Cluster 1 contained all the highly heat tolerant genotypes, including IPU18-04(43.34%), Mash 114(47.7%), UTTARA (IPU94-1) (57.39%), and PantU31 (55.45%) (Figure 8) relying on low reduction of seed yield plant-1 (SYP) compared under non-stress and heat stress conditions. The heat-sensitive genotypes viz., Mash218 (91.35% SYP reduction), IPU5 (96.79% SYP reduction), IPU18-5(93.40% SYP reduction) remained in second cluster. The 3rd cluster had genotypes such as IPU 11-02 (88.45% SYP reduction), SuG1170 (89.79% SYP reduction), SuG1169 (90.235 SYP reduction), Mash 1008 (92.6% SYP reduction)
Likewise, during second year, the heat map also divided the genotypes into three clusters (Figure 9). The first cluster contained the highly heat tolerant genotypes viz., Mash 114 (41.6% SYP reduction), PantU31 (28.7% SYP reduction), IPU18-04 (48.35% SYP reduction) and UTTARA (38% SYP reduction). In the second cluster, all the heat-sensitive genotypes such as Mash 218 (91.5% SYP reduction), SuG1153 (94.1% SYP reduction), IPU18-5 (94.8% SYP reduction) and IPU5 (95.2% SYP reduction) were placed. Other genotypes, for example, IPU2-43 (62.1% SYP reduction), IPU-11-02 (90.3% SYP reduction), and SuG1169 (90.8% SYP reduction) were found in third cluster.
The cluster analysis of the selected genotypes evaluated for various traits under growth chamber condition resulted in two major clusters (Figure 10). The first cluster contained all the four heat-sensitive genotypes such as IPU18-5, IPU5, SuG1153, and Mash 218, whereas the second cluster contained all the heat tolerant genotypes UTTARA, PantU31, IPU18-04, and Mash114.
Various symptoms of heat stress on urdbean at vegetative and reproductive growth are shown in Figures 11 and 12.
Figure 11 Morphological effects of heat stress on Urdbean plants; plant height under control environment (A), reduced plant height under heat stress (HS) environment (B), healthy leaves under control environment (C), scorching of leaves under HS (D), chlorosis in the HS (E), Leaf senescence and abscissionin the HS (F).
Figure 12 Urdbean plants showing various distinctive impacts on it are the reproductive phase when raised under control and heat stress environment. Plants grown under control temperature have healthy bud (A) healthy flower (C) filled pods (egg), normal seeds (I) compact anther (K, L) receptive stigma (O, P) higher pollen load (S, T) and possess viable pollen grains (W, X). However, plants raised under heat stress conditions have more frequency of aborted buds (B) aborted flowers (D) unfilled and aborted pods (F, H) shrivelled seeds (J) distorted anther (M, N) non-receptive stigma (Q, R) less pollen load (U, V) and non-viable pollen grains (Y, Z) healthy flower bud (A) aborted flower bud (B) healthy flower (C) aborted flower (D) healthy pods (E) aborted pod (F) normal pod length (G) reduced pod length (H) healthy seeds (G) shrivelled seeds (H) healthy anther under stereo-microscope (I) healthy anther under SEM (J) distorted anther.
Increasing frequency of heat stress events poses serious challenges in all the plant growth stages, especially, reproductive stage, resulting in significant yield loss in various crop plants, including urdbean (Jha et al., 2014; Jha et al., 2017; Chaudhary et al., 2020; Chaudhary et al., 2022). Thus, assessing urdbean’s genetic variability for phenological, morpho-physiological, biochemical and yield and yield related traits is one of the prime objectives for developing heat tolerant climate resilient urdbean genotypes.
A selected set of 26 urdbean genotype were examined for heat stress tolerance by growing them under non-stress and heat stress under field condition and under controlled growth chamber conditions. Wide range of genetic variability for various physiological, biochemical and yield and yield related traits was observed. Thus, some genotypes showing promising results based on the physiological traits such as chlorophyll content, chlorophyll fluorescence, and yield traits like high pod setting plant-1 and high seed yield plant-1 under heat stress environment were identified and validated for heat tolerance under controlled high temperature environment. These urdbean genotypes could be a precious resource for heat tolerance. Further, the tolerant urdbean genotypes could be potentially used for investigating the genetic control of heat tolerance in urdbean.
Among the various physiological traits for assessing heat stress response at vegetative stage during photosynthesis, measuring chlorophyll content, electrolyte leakage, stomatal conductance and photosystem II function are essential parameters for selecting heat tolerant genotypes (Srinivasan et al., 1996; Sita et al., 2017; Bhandari et al., 2020; Devi et al., 2022). Chlorophyll is the main photosynthetic pigment, assists in capturing light energy and enables in photosynthesis process (Wang et al., 2018). Heat stress causes reduction in Chl content resulting in leaf senescence (Kim and Nam, 2007). Improved retention of Chl content under heat stress could be an indication of heat stress tolerance. Thus, genetic variability for Chl content could be useful for selecting heat tolerant genotypes. Heat tolerance based on membrane stability measured by electrolyte/ion leakage is an important trait for selecting genotypes for heat tolerance (Bajji et al., 2002). Under high temperature stress, the genotypes showing low electrolyte leakage indicate stable cell membrane stability and thus are considered heat tolerant. Likewise, stomatal conductance and transpiration cooling are important traits for assessing heat tolerance in plants. Leaf cooling is a vital heat stress avoidance mechanism (Deva et al., 2020) thus, enhanced stomatal conductance and transpiration cooling could help plants to conduct photosynthesis process under high temperature stress (Porch and Hall, 2013). Thus, genotypes with high stomatal conductance could be heat tolerant. In the current study, Mash114, UTTARA, PantU31 genotypes showed high chlorophyll content, high stomatal conductance, low electrolyte leakage and high Fv/Fm value under heat stress, both under field and growth chamber condition, indicating their heat stress tolerance. Similar findings were reported in chickpea (Devi et al., 2022), lentil (Srinivasan et al.,1996; Delahunty et al., 2015; Sita et al., 2017; Sehgal et al., 2019; Bhandari et al., 2020) and pea (McDonald and Paulsen, 1997) under heat stress.
Of all the growth stages, reproductive stage is the most sensitive stage affected by negative impact of heat stress (Zinn et al., 2010). High temperature stress causes anomalies and malfunction in reproductive processes ranging from reduction in pollen germination (PGP) percentage, pollen viability percentage (PVP), malformation in ovule to inhibition in fertilization process in various crops, including rice (Xu et al., 2021), wheat (Ullah et al., 2022), chickpea (Bhandari et al., 2020; Devi et al., 2022), common bean (Silva et al., 2019; Soltani et al., 2019) and tomato (Gonzalo et al., 2021). High PGP and PVP values are indicators of efficient reproductive function leading to high pod and seed setting resulting in improved yield under heat stress (Firon et al., 2006; Pham et al., 2020). Sufficient range of genetic variability for PGP (15.4%-57.4%), PVP (24.5%-61.2%) was noted under heat stress in the present study, providing scope for selection and developing heat tolerant urdbean genotypes. Based on these traits, Mash114 and PantU31 genotypes could be promisingly used as donor parents for improving heat tolerance in urdbean. Screening of heat tolerance relying on PGP and PVP has been reported in rice (Zhang et al., 2018), wheat (Bheemanahalli et al., 2019), chickpea (Devi et al., 2022), lentil (Barghi et al., 2013), common bean (Silva et al., 2019; Vargas et al., 2021), tomato (Pham et al., 2020) and sorghum (Djanaguiraman et al., 2018).
Emphasizing on yield and yield-related parameters such as pods plant-1, significant genetic variability ranging from (2.43-15.07 during the first year) and (2.77-15.9 during second year), for seed yield plant-1 (0.11-2.73g during the first year) and (0.16-2.93g during the second year) and for total seeds plant-1 (6.2-62.9 during the first year) and (6.7-71.2 during the second year) under heat stress was recorded. Thus, genotypes with high pod setting, high seed yield plant-1 and high seed number plant-1 under heat stress environment could be promisingly selected as heat tolerant genotypes. Based on these traits, Mash114, PantU31, UTTARA and IPU18-04 were selected as heat tolerant urdbean genotypes. Similarly, in previous studies, based on these yield traits, genotypes “40–10,” “Naparnyk,” and “CDC Meadow” in pea (Jiang et al., 2020), G122, PI 163120, Cornell 503 in common bean (Shonnard and Gepts, 1994; Rainey and Griffiths, 2005a), ICC1205, ICC15614, GNG469, GNG1488, GNG1499, and GNG1969 in chickpea (Devasirvatham et al., 2013), B89-200 and TN88-63 in cowpea (Ehlers and Hall, 1998), 72578, 70548, 71457 and 73838 in lentil (Delahunty et al.,2015) and 55–437 and 796 in groundnut (Ntare et al., 2001) were identified to be heat tolerant.
Correlation studies indicated that electrolyte leakage trait had highly negative association with all the physiological (except MDA) and yield and yield-related traits under heat stress condition, indicating genotypes having high value for electrolyte leakage are highly heat- sensitive genotypes. However, other physiological traits viz., chlorophyll content, chlorophyll florescence, relative water content stomatal conductance showing high and positive association with yield and yield related traits viz., seed yield plant-1, total seeds plant-1 and single seed weight indicated that selection of urdbean genotypes with high chlorophyll content, enhanced stomatal conductance and high relative water content under heat stress could be highly heat tolerant. Positive association of chlorophyll content, stomatal conductance trait related to photosynthesis process with yield and yield-related traits ranging from seed yield plant-1, pod number plant-1 and single seed weight under heat stress has been reported in chickpea (Devi et al., 2022), lentil (Sita et al., 2017), and common bean (Petkova et al., 2007).
Studies conducted in controlled high temperature environment of growth chamber revealed PGP and PVP to be highly correlated with pods plant-1 and could be used as vital indicators of heat tolerance. Earlier studies have also indicated that these traits could be used for selecting heat tolerant genotypes in chickpea (Devi et al., 2022), common bean (Rainey and Griffiths, 2005), lentil (Sehgal et al., 2019) and tomato (Gonzalo et al., 2021).
High heritability of various morpho-physiological, yield and yield related traits could be of great importance for selecting these traits for screening of heat tolerant genotypes in various crops. High heritability for chlorophyll content, stomatal conductance, seed yield plant-1, pods plant-1, single seed weight, as noticed in the present study has also been noted in heat tolerant chickpea (Jha et al., 2019; Devi et al., 2022), rice (Enzi et al., 2022), tomato (Panthee et al., 2018), wheat (Rebetzke et al., 2013) under high temperature environment.
Heat stress related events are becoming serious constraints for crop yield including urdbean thus, causing great concern for global food security. Harnessing the genetic variability for various morpho-physiological and yield and yield related traits existing across the crop gene pool could be one of the important approaches for developing heat tolerant crop cultivars including urdbean. A wide range of genetic variability for various morpho-physiological and yield and yield related traits were captured for a selected 26 urdbean genotypes under both non-stress and heat stress environment for consecutive two years. A selected four heat tolerant and four heat-sensitive genotypes were further validated for their heat stress response under controlled growth chamber condition. Based on the results obtained from both outdoor and controlled growth chamber conditions, yield and yield related traits viz., pods plant-1, seeds plant-1, single seed weight and seed yield plant-1 showed strong positive correlation with chlorophyll, chlorophyll fluorescence, and stomatal conductance. Similarly, these yield traits had very strong correlation with reproductive traits, pollen germination and pollen viability except electrolyte leakage and malondialdehyde content. These results indicated selection for high pollen germination % and high pollen viability % and yield and yield related traits could assist in selecting heat tolerant urdbean genotypes. Thus, the candidate genotypes PantU31, Mash114, UTTARA and IPU18-04 exhibiting high pod setting and high seed yield plant-1 under heat stress imposed under outdoor and growth chamber environment could be potentially used as heat tolerant donor parents for future urdbean breeding programme. Further, these genotypes can be assessed for their heat tolerance across the multiple locations for confirming their heat tolerance based on various locations.
The original contributions presented in the study are included in the article/Supplementary Material. Further inquiries can be directed to the corresponding authors.
SC conducted the experiment. UJ and PJP helped in analysis and writing part of the manuscript. SS and DS contributed in providing the urdbean lines. KS and SK assisted in conducting the biochemical analyses. HN conceived the idea and helped in writing the manuscript. KHMS helped in writing part of the manuscript and edited the manuscript. All authors contributed to the article and approved the submitted version.
SC thanks CSIR for project fellowship and CSIR-UGC for providing a doctoral research fellowship during her course study. The corresponding author HN is thankful to DST (FIST and PURSE), UGC, DBT, CSIR, India, The University of Western Australia (Australia), IIPR (Kanpur, India), PAU (Ludhiana, India), for supporting the research work at various times.
The authors declare that the research was conducted in the absence of any commercial or financial relationships that could be construed as a potential conflict of interest.
All claims expressed in this article are solely those of the authors and do not necessarily represent those of their affiliated organizations, or those of the publisher, the editors and the reviewers. Any product that may be evaluated in this article, or claim that may be made by its manufacturer, is not guaranteed or endorsed by the publisher.
The Supplementary Material for this article can be found online at: https://www.frontiersin.org/articles/10.3389/fpls.2022.1042999/full#supplementary-material
Allakhverdiev, S. I., Kreslavski, V. D., Klimov, V. V., Los, D. A., Carpentier, R., Mohanty, P. (2008). Heat stress: an overview of molecular responses in photosynthesis. Photosynth. Res. 98, 541–550. doi: 10.1007/s11120-008-9331-9330
Anitha, Y., Vanaja, M., Kumar, V. G. (2016). Identification of attributes contributing to high temperature tolerance in blackgram (Vigna mungo l. hepper) genotypes. Int. J. Sci. Res. 5, 1021–1025.
Awasthi, R., Kaushal, N., Vadez, V., Turner, N. C., Berger, J., Siddique, K. H., et al. (2014). Individual and combined effects of transient drought and heat stress on carbon assimilation and seed filling in chickpea. Funct. Plant Biol. 41, 1148–1167. doi: 10.1071/FP13340
Babicki, S., Arndt, D., Marcu, A., Liang, Y., Grant, J. R., Maciejewski, A., et al. (2016). Heatmapper: web-enabled heat mapping for all. Nucleic Acids Res. 44, 147–153. doi: 10.1093/nar/gkw419
Bajji, M., Kinet, J. M., Lutts, S. (2002). The use of the electrolyte leakage method for assessing cell membrane stability as a water stress tolerance test in durum wheat. Plant Growth Regul. 36, 61–70. doi: 10.1023/A:1014732714549
Barghi, S. S., Mostafaii, H., Peighami, F., Zakaria, R. A., Nejhad, R. F. (2013). Response of in vitro pollen germination and cell membrane thermostability of lentil genotypes to high temperature. Intl. J. Agril. 3 (1), 13.
Barrs, H. D., Weatherley, P. E. (1962). A re-examination of the relative turgidity technique for estimating water deficits in leaves. Aust. J. Biol. Sci. 15, 413–428. doi: 10.1071/BI9620413
Bhandari, K., Siddique, K. H., Turner, N. C., Kaur, J., Singh, S., Agrawal, S. K., et al. (2016). Heat stress at reproductive stage disrupts leaf carbohydrate metabolism, impairs reproductive function, and severely reduces seed yield in lentil. J. Crop Improv. 30, 118–151. doi: 10.1080/15427528.2015.1134744
Bhandari, K., Sita, K., Sehgal, A., Bhardwaj, A., Gaur, P., Kumar, S., et al. (2020). Differential heat sensitivity of two cool-season legumes, chickpea and lentil, at the reproductive stage, is associated with responses in pollen function, photosynthetic ability and oxidative damage. J. Agron. Crop Sci. 206, 734–758. doi: 10.1111/jac.12433
Bheemanahalli, R., Sunoj, V. J., Saripalli, G., Prasad, P. V., Balyan, H. S., Gupta, P. K., et al. (2019). Quantifying the impact of heat stress on pollen germination, seed set, and grain filling in spring wheat. Crop Sci. 59, 684–696. doi: 10.2135/cropsci2018.05.0292
Bita, C. E., Gerats, T. (2013). Plant tolerance to high temperature in a changing environment: scientific fundamentals and production of heat stress-tolerant crops. Front. Plant Sci. 4. doi: 10.3389/fpls.2013.00273
Brewbaker, J. L., Kwack, B. H. (1963). The essential role of calcium ion in pollen germination and pollen tube growth. Am. J. Bot. 50, 859–865. doi: 10.1002/j.1537-2197.1963.tb06564.x
Chaturvedi, P., Wiese, A. J., Ghatak, A., ZaveskaDrabkova, L., Weckwerth, W., Honys, D. (2021). Heat stress response mechanisms in pollen development. New Phytol. 231, 571–585. doi: 10.1111/nph.17380
Chaudhary, S., Devi, P., Bhardwaj, A., Jha, U. C., Sharma, K. D., Prasad, P. V., et al. (2020). Identification and characterization of contrasting genotypes/cultivars for developing heat tolerance in agricultural crops: Current status and prospects. Front. Plant Sci. 11. doi: 10.3389/fpls.2020.587264
Chaudhary, S., Devi, P., HanumanthaRao, B., Jha, U. C., Sharma, K. D., Prasad, P. V. V., et al. (2022). Physiological and molecular approaches for developing thermotolerance in vegetable crops: a growth, yield and sustenance perspective. Front. Plant Sci. 13, 878498. doi: 10.3389/fpls.2022.878498
Chebrolu, K. K., Fritschi, F. B., Ye, S., Krishnan, H. B., Smith, J. R., Gillman, J. D. (2016). Impact of heat stress during seed development on soybean seed metabolome. Metabolomics 12, 1–14. doi: 10.1007/s11306-015-0941-941
Chen, W. L., Yang, W. J., Lo, H. F., Yeh, D. M. (2014). Physiology, anatomy, and cell membrane thermostability selection of leafy radish (Raphanus sativus var. oleiformis pers.) with different tolerance under heat stress. Sci. Hortic. 179, 367–375. doi: 10.1016/j.scienta.2014.10.003
Delahunty, A., Nuttall, J., Nicolas, M., Brand, J. (2015). “Genotypic heat tolerance in lentil,” in Proceedings of the 17th ASA Conference. 20–24(Hobart, Australia).
Devasirvatham, V., Gaur, P., Mallikarjuna, N., Raju, T. N., Trethowan, R. M., Tan, D. K. Y. (2013). Reproductive biology of chickpea response to heat stress in the field is associated with the performance in controlled environments. Field Crops Res. 142, 9–19. doi: 10.1016/j.fcr.2012.11.011
Deva, C. R., Urban, M. O., Challinor, A. J., Falloon, P., Svitákova, L. (2020). Enhanced leaf cooling is a pathway to heat tolerance in common bean. Front. Plant Sci. 11, 19. doi: 10.3389/fpls.2020.00019
Devi, P., Jha, U. C., Prakash, V., Kumar, S., Parida, S. K., Paul, P. J., et al. (2022). Response of physiological, reproductive function and yield traits in cultivated chickpea (Cicer arietinum l.) under heat stress. Front. Plant Sci. 13, 880519. doi: 10.3389/fpls.2022.880519
Djanaguiraman, M., Perumal, R., Jagadish, S. V. K., Ciampitti, I. A., Welti, R., Prasad, P. V. V. (2018). Sensitivity of sorghum pollen and pistil to high-temperature stress. Plant Cell Environ. 41 (5), 1065–1082. doi: 10.1111/pce.13089
Ehlers, J. D., Hall, A. E. (1998). Heat tolerance of contrasting cowpea lines in short and long days. Field Crops Res. 55, 11–21. doi: 10.1016/S0378-4290(97)00055-5
Enzi, V., Ahanchede, W. W., Ayenan, M. A. T., Ahanchede, A. (2022). Physiological and agronomical evaluation of elite rice varieties for adaptation to heat stress. BMC Plant Biol. 22 (1), 1–14. doi: https://doi.org/10.1186/s12870-022-03604-x
Firon, N., Shaked, R., Peet, M. M., Pharr, D. M., Zamski, E., Rosenfeld, K., et al. (2006). Pollen grains of heat tolerant tomato cultivars retain higher carbohydrate concentration under heat stress conditions. Sci. Hortic. 109, 212–217. doi: 10.1016/j.scienta.2006.03.007
Gonzalo, M. J., Nájera, I., Baixauli, C., Gil, D., Montoro, T., Soriano, V., et al. (2021). Identification of tomato accessions as source of new genes for improving heat tolerance: From controlled experiments to field. BMC Plant Biol. 21 (1), 1–28. doi: 10.1186/s12870-021-03104-4
Heath, R. L., Packer, L. (1968). Photoperoxidation in isolated chloroplasts: I. kinetics and stoichiometry of fatty acid peroxidation. Arch. Biochem. Biophys. 125, 189–198. doi: 10.1016/0003-9861(68)90654-1
Hedhly, A. (2011). Sensitivity of flowering plant gametophytes to temperature fluctuations. Environ. Exp. Bot. 74, 9–16. doi: 10.1016/j.envexpbot.2011.03.016
Jha, U. C., Bohra, A., Parida, S. K., Jha, R. (2017). Integrated “omics” approaches to sustain global productivity of major grain legumes under heat stress. Plant Breed. 136, 437–459. doi: 10.1111/pbr.12489
Jha, U. C., Bohra, A., Singh, N. P. (2014). Heat stress in crop plants: its nature, impacts and integrated breeding strategies to improve heat tolerance. Plant Breed. 133, 679–701. doi: 10.1111/pbr.12217
Jha, U. C., Kole, P. C., Singh, N. P. (2019). Nature of gene action and combining ability analysis of yield and yield related traits in chickpea (Cicer arietinum l.) under heat stress. Indian J. Agric. Sci. 89, 500–508.
Jha, U. C., Nayyar, H., Jha, R., Singh, P. K., Dixit, G. P., Kumar, Y., et al. (2022). “Improving chickpea genetic gain under rising drought and heat stress using breeding approaches and modern technologies,” in Developing climate resilient grain and forage legumes. Eds. Jha, U. C., Nayyar, H., Agrawal, S. K., Siddique, K. H. M. (Singapore: Springer), 1–25.
Jiang, Y., Lindsay, D. L., Davis, A. R., Wang, Z., MacLean, D. E., Warkentin, T. D., et al. (2020). Impact of heat stress on pod-based yield components in field pea (Pisum sativum l.). J. Agron. Crop Sci. 206, 76–89. doi: 10.1111/jac.12365
Joshi, P. K., Rao, P. P. (2017). Global pulses scenario: status and outlook. Ann. N. Y. Acad. Sci. 1392, 6–17. doi: 10.1111/nyas.13298
Kaushal, N., Awasthi, R., Gupta, K., Gaur, P., Siddique, K. H., Nayyar, H. (2013). Heat-stress-induced reproductive failures in chickpea (Cicer arietinum) are associated with impaired sucrose metabolism in leaves and anthers. Funct. Plant Biol. 40, 1334–1349. doi: 10.1071/FP13082
Kim, H. J., Nam, H. G. (2007). Leaf senescence. Annu. Rev. Plant Biol. 58, 115–136. doi: 10.1146/annurev.arplant.57.032905.105316
Li, B., Gao, K., Ren, H., Tang, W. (2018). Molecular mechanisms governing plant responses to high temperatures. J. Integr. Plant Biol. 60, 757–779. doi: 10.1111/jipb.12701
Lutts, S., Kinet, J. M., Bouharmont, J. (1996). NaCl-Induced senescence in leaves of rice (Oryza sativa l.) cultivars differing in salinity resistance. Ann. Bot. 78, 389–398. doi: 10.1006/anbo.1996.0134
McDonald, G., Paulsen, G. (1997). High temperature effects on photosynthesis and water relations of grain legumes. Plant Soil 196, 47–58. doi: 10.1023/A:1004249200050
Ntare, B. R., Williams, J. H., Dougbedji, F. (2001). Evaluation of groundnut genotypes for heat tolerance under field conditions in a sahelian environment using a simple physiological model for yield. J. Agric. Sci. (Cambridge) 136, 81–88. doi: 10.1017/S0021859600008583
Panthee, D. R., Kressin, J. P., Piotrowski, A. (2018). Heritability of flower number and fruit set under heat stress in tomato. Hortic. Sci. 53 (9), 1294–1299. doi: 10.21273/HORTSCI13317-18
Patterson, H. D., Thompson, R. (1971). Recovery of inter-block information when block sizes are unequal. Biometrika 58, 545–554. doi: 10.2307/2334389
Petkova, V., Denev, I. D., Cholakov, D., Porjazov, I. (2007). Field screening for heat tolerant common bean cultivars (Phaseolus vulgaris l.) by measuring of chlorophyll fluorescence induction parameters. Sci. Hortic. 111, 101–106. doi: 10.1016/j.scienta.2006.10.005
Pham, D., Hoshikawa, K., Fujita, S., Fukumoto, S., Hirai, T., Shinozaki, Y., et al. (2020). A tomato heat-tolerant mutant shows improved pollen fertility and fruit-setting under long-term ambient high temperature. Environ. Expt. Bot. 178, 104150. doi: 10.1016/j.envexpbot.2020.104150
Porch, T. G., Hall, A. E. (2013). “Heat tolerance,” in Genomics and breeding for climate-resilient crops (Berlin, Heidelberg: Springer), 167–202.
Rainey, K. M., Griffiths, P. D. (2005). Differential response of common bean genotypes to high temperature. J. Amer. Hortic. Sci. 130, 18–23. doi: 10.21273/JASHS.130.1.18
Rebetzke, G. J., Rattey, A. R., Farquhar, G. D., Richards, R. A., Condon, A. T. G. (2013). Genomic regions for canopy temperature and their genetic association with stomatal conductance and grain yield in wheat. Funct. Plant Biol. 40, 14–33. doi: 10.1071/FP12184
Riaz, M. W., Yang, L., Yousaf, M. I., Sami, A., Mei, X. D., Shah, L., et al. (2021). Effects of heat stress on growth, physiology of plants, yield and grain quality of different spring wheat (Triticum aestivum l.) genotypes. Sustainability 13, 1–18. doi: 10.3390/su13052972
Sehgal, A., Sita, K., Bhandari, K., Kumar, S., Kumar, J., Vara Prasad, P. V., et al. (2019). Influence of drought and heat stress, applied independently or in combination during seed development, on qualitative and quantitative aspects of seeds of lentil (Lens culinaris medikus) genotypes, differing in drought sensitivity. Plant Cell Environ. 42 (1), 198–211. doi: 10.1111/pce.13328
Sen Gupta, D., Basu, P. S., Souframanien, J., Kumar, J., Dhanasekar, P., Gupta, S., et al. (2021). Morpho-physiological traits and functional markers based molecular dissection of heat-tolerance in urdbean. Front. Plant Sci. 12. doi: 10.3389/fpls.2021.719381
Sharma, L., Priya, M., Bindumadhava, H., Nair, R. M., Nayyar, H. (2016). Influence of high temperature stress on growth, phenology and yield performance of mungbean [Vigna radiata (L.) wilczek] under managed growth conditions. Sci. Hortic. 213, 379–391. doi: 10.1016/j.scienta.2016.10.03
Shonnard, G. C., Gepts, P. (1994). Genetics of heat tolerance during reproductive development in common bean. Crop Sci. 34, 1168–1175. doi: 10.2135/cropsci1994.0011183X003400050005x
Silva, D. A. D., Pinto-Maglio, C. A. F., Oliveira, É.C.D., Reis, R.L.D.M.D., Carbonell, S. A. M., Chiorato, A. F. (2019). Influence of high temperature on the reproductive biology of dry edible bean (Phaseolus vulgaris l.). Scientia Agricola 77, 1–9. doi: https://doi.org/10.1590/1678-992X-2018-0233
Sita, K., Sehgal, A., Kumar, J., Kumar, S., Singh, S., Siddique, K. H., et al. (2017). Identification of high-temperature tolerant lentil (Lens culinaris medik.) genotypes through leaf and pollen traits. Front. Plant Sci. 8. doi: 10.3389/fpls.2017.00744
Soltani, A., Weraduwage, S. M., Sharkey, T. D., Lowry, D. B. (2019). Elevated temperatures cause loss of seed set in common bean (Phaseolus vulgaris l.) potentially through the disruption of source-sink relationships. BMC Genome. 20, 312. doi: 10.1186/s12864-019-5669-2
Srinivasan, A., Takeda, H., Senboku, T. (1996). Heat tolerance in food legumes as evaluated by cell membrane thermostability and chlorophyll fluorescence techniques. Euphytica 88 (1), 35–45. doi: 10.1007/BF00029263
Ullah, A., Nadeem, F., Nawaz, A., Siddique, K. H., Farooq, M. (2022). Heat stress effects on the reproductive physiology and yield of wheat. J. Agron. Crop Sci. 208 (1), 1–17. doi: 10.1111/jac.12572
Vargas, Y., Mayor-Duran, V. M., Buendia, H. F., Ruiz-Guzman, H., Raatz, B. (2021). Physiological and genetic characterization of heat stress effects in a common bean RIL population. PLoS One 16, e0249859. doi: 10.1371/journal.pone.0249859
Wahid, A., Gelani, S., Ashraf, M., Foolad, M. R. (2007). Heat tolerance in plants: an overview. Environ. Exp. Bot. 61, 199–223. doi: 10.1016/j.envexpbot.2007.05.011
Wang, Q. L., Chen, J. H., He, N. Y., Guo, F. Q. (2018). Metabolic reprogramming in chloroplasts under heat stress in plants. Int. J. Mol. Sci. 19, 849. doi: 10.3390/ijms19030849
Xu, Y., Chu, C., Yao, S. (2021). The impact of high-temperature stress on rice: challenges and solutions. Crop J. 9 (5), 963–976. doi: 10.1016/j.cj.2021.02.011
Zhang, C., Li, G., Chen, T., Feng, B., Fu, W., Yan, J., et al. (2018). Heat stress induces spikelet sterility in rice at anthesis through inhibition of pollen tube elongation interfering with auxin homeostasis in pollinated pistils. Rice 11 (1), 1–12. doi: 10.1186/s12284-018-0206-5
Keywords: urdbean, heat stress, genetic variability, physiological traits, biochemical traits
Citation: Chaudhary S, Jha UC, Paul PJ, Prasad PVV, Sharma KD, Kumar S, Gupta DS, Sharma P, Singh S, Siddique KHM and Nayyar H (2022) Assessing the heat sensitivity of Urdbean (Vigna mungo L. Hepper) genotypes involving physiological, reproductive and yield traits under field and controlled environment. Front. Plant Sci. 13:1042999. doi: 10.3389/fpls.2022.1042999
Received: 13 September 2022; Accepted: 07 November 2022;
Published: 21 November 2022.
Edited by:
Xia Xin, Chinese Academy of Agricultural Sciences, ChinaReviewed by:
Lixia Wang, Institute of Crop Sciences, Chinese Academy of Agricultural Sciences, ChinaCopyright © 2022 Chaudhary, Jha, Paul, Prasad, Sharma, Kumar, Gupta, Sharma, Singh, Siddique and Nayyar. This is an open-access article distributed under the terms of the Creative Commons Attribution License (CC BY). The use, distribution or reproduction in other forums is permitted, provided the original author(s) and the copyright owner(s) are credited and that the original publication in this journal is cited, in accordance with accepted academic practice. No use, distribution or reproduction is permitted which does not comply with these terms.
*Correspondence: Harsh Nayyar, aGFyc2huYXl5YXJAaG90bWFpbC5jb20=; Uday Chand Jha, dTk4MTE5ODFAZ21haWwuY29t; Kadambot H. M. Siddique, a2FkYW1ib3Quc2lkZGlxdWVAdXdhLmVkdS5hdQ==
Disclaimer: All claims expressed in this article are solely those of the authors and do not necessarily represent those of their affiliated organizations, or those of the publisher, the editors and the reviewers. Any product that may be evaluated in this article or claim that may be made by its manufacturer is not guaranteed or endorsed by the publisher.
Research integrity at Frontiers
Learn more about the work of our research integrity team to safeguard the quality of each article we publish.