- State Key Laboratory of Crop Biology and College of Agronomy, Shandong Agricultural University, Tai’an, Shandong, China
Extreme rainfall events during the summer maize growth and development periods, which have induced losses in summer maize production. There was a completely randomized block experiment being designed with four treatments: waterlogging for 6 days at the V3 stage (C-W), H2O2-priming + non-waterlogging (H-CK), H2O2-priming + waterlogging for 6 days at the V3 stage (H-W) and control (C-CK). This study investigated the effects of H2O2 priming on yield and photosynthetic parameters of (Zea mays. L) summer maize hybrid DengHai605 (DH605) by measuring the leaf area index (LAI), soil and plant analyzer development (SPAD) value, stomatal morphology, gas exchange parameters, and chlorophyll fluorescence parameters. The results showed that the net photosynthetic rate (Pn) was decreased after waterlogging through the stomatal limitation of CO2 supply and reduction of PSII photochemical efficiency, which led to the decrease in dry matter accumulation and grain yield. H2O2 priming increased the number of opening stomas, the stomatal length, and width, thus increasing Ci by 12.1%, which enhanced the Pn by 37.5%. Additionally, H2O2 priming could improve the energy of dark reaction carbohydrates by increasing the light energy absorption and utilization, alleviating the function of PSII reaction centers, protecting the PSII receptor and donor side, and the electron transport chain. The φEo, φPo, φRo, and Ψo of H-W were increased by 89.9%, 16.2%, 55.4%, and 63.9% respectively, and the φDo was decreased by 23.5%, compared with C-W. Therefore, H2O2 priming significantly enhanced the PSII photochemical efficiency, and increased the CO2 supply in dark reactions to promote carbon assimilation, alleviating the waterlogging-induced damage to maize plant growth and grain yield.
1 Introduction
In recent years, due to anthropogenic contributions including industrialization and urbanization, the environmental conditions have deteriorated, causing waterlogging disasters to occur frequently all over the world (Pan, 2022; Voesenek and Bailey-Serres, 2015). Waterlogging, as one of the main abiotic stresses for the normal growth and development of summer maize, seriously harmed crop production in nearly 16% of the world’s agricultural production areas (Seng et al., 2012; Zhou et al., 2020). In the Huang huai hai region, the seedling stage (especially in the V3 stage) of summer maize coincided with the period of frequent rainfall (Yu, 2015). The Huang huai hai Plain was the main summer maize producing area in China, whose planting area and yield accounted for more than 1/3 of all maize planting areas in China (Gao, 2018), and 70% of annual precipitation was concentrated during the growing period of summer maize. Such a large amount of precipitation would lead to frequent rainfall-induced waterlogging, which could seriously affect the yield and quality of summer maize (Ren et al., 2015). It is thus desirable to explore effective management to mitigate the waterlogging damages to maize production.
When water content exceeded 80% of the maximum field water capacity, plant growth and development of summer maize were significantly inhibited, and the greatest impact occurred at the V3 stage (Ren et al., 2017). From the point of view of phenotypic characteristics: The leaves turn yellow and lose their green colour, which decreased the leaf area index and SPAD values, and reduced the production capacity by optical contraction (Ren et al., 2015). From the physiological point of view: Waterlogging stress resulted in stomatal closure, thus blocking the CO2 uptake, which significantly reduced the photosynthetic rate of summer maize, and inhibited the ability of photosynthetic assimilation ability (Ren et al., 2018). With the prolonged waterlogging duration, the PSII system of summer maize was damaged, photosynthetic electron transport was blocked, and carbon assimilation was inhibited (Hu et al., 2020; Ren et al., 2020; Yu et al., 2021). So, when the plants encountered waterlogging stress, the stomatal performance and the PSII photoinhibition of leaves were limited, which further reduced production capacity and reduced the grain yield finally.
Chemical priming could improve the plant plasticity to abiotic stresses (Kaori et al., 2020). Plant priming was related to stress “memory” which as a potential way of improving cross-tolerance (When the plants were exposed to a mild stress, the subsequence severe stresses were improved) (Munné-Bosch and Alegre, 2013). H2O2, as one of the chemical priming agents, played an important role in the stress signal transduction pathway that was essential for crop growth and adaptation to adversity (Gao et al., 2010; Hossain et al., 2015). H2O2 played a wide role in plants responding to abiotic stresses by regulating a multitude of physiological processes such as acquiring resistance, antioxidant defense, photosynthesis, and stomatal opening (Petrov and Breusegem, 2012; Gdsa et al., 2020). Previous studies have demonstrated that pretreatments with 70 mM H2O2 favored the increase in net physiological rate, PSII efficiency, plant biomass, and antioxidant activity of leaves in soybean (Glycine max [L.] Merr.) (Andrade et al., 2018). It has been well summarized in several reviews that H2O2 priming could enhance plant tolerance to abiotic stress (Perez and Brown, 2014; Ayano et al., 2015; Kaori et al., 2020). Recent publications have reported that exogenous treatment of H2O2 could preserve plants from low temperature (Wahid et al., 2007), drought (Zhang et al., 2022), salt (Gondim et al., 2009), waterlogging (Andrade et al., 2018), and other stresses. Nonetheless, the use of H2O2 as a chemical priming agent has been rarely studied under waterlogging stress, especially in H2O2 alleviating the effects of waterlogging stress on photosynthetic characteristics (Perez & Brown, 2014; Ayano et al., 2015). As one of the most basic physiological activities of plants, photosynthesis which provided material and energy for plant life activities was also one of the important indicators of the ability of plants to withstand adversity and stress. The photosynthesis in the leaves decreased under waterlogging pressure, which would reduce crop yield and quality (Ashraf and Harris, 2013; Tian et al., 2019). This study was to investigate the effects of H2O2 priming on the yield and photosynthetic characteristics of summer maize subjected to waterlogging stress, thus providing theoretical and technical support for the chemical regulation of summer maize under waterlogging stress.
2 Materials and methods
2.1 Experimental materials and design
This experiment was conducted in 2020 and 2021 to explore the effects of H2O2 priming on waterlogged summer maize at the Shandong Agricultural University experimental farm (36.10°N, 117.09°E). Summer maize hybrid Denghai605 (DH605) was used as experimental materials. DH605 was approved by the approval committee of China in 2010 which was suitable for planting with in Shandong province and widely grown in China (Hu et al., 2020). Seed soaking for 12 h with 0.1% H2O2 [relevant literature showed that 0.1% H2O2 significantly promoted the germination of maize seeds (Zhang, 1996)]. Summer maize was sown on June 9 in 2020 and 2021 with the density of 67500 plants ha-1, and waterlogging at the V3 stage for 6 days (Extreme rainfall events were frequent in the Huang huai hai region from June to July which was the V3 stage of summer maize (Supplementary Figure 1). At the same time, the plant growth and development of summer maize were significantly inhibited after waterlogging, and the greatest impact occurred at the V3 stage (Ren et al., 2017)). For details, see (Table 1). N, P, and K fertilizers were applied as base fertilizers: 210 kg ha-1 N (urea, 46% N), 52.5 kg ha-1 P2O5 (calcium superphosphate, 17% P2O5), and 67.5 kg ha-1 K2O (muriate of potash, 60% K2O). Management of other diseases, insects, grasses, and pests by reference to high-yield fields. The details were shown in (Hu et al., 2021). Complete randomized block design. Plot planting test was used in this experiment. A square plot was formed by 4 pieces of PVC board with 4 m × 4 m to form a 16 m2 reservoir. Each piece of PVC plate was a length 4 m and width 2.3 m, which was used to bury 2.0 m below the underground and 0.3m above ground to facilitate the formation of a 2-3 cm water layer. The climatic conditions of the maize growing season in 2020 and 2021 are shown in (Supplementary Figure 1). The total annual precipitations were 805.8 and 939.1mm in 2020 and 2021, and mean temperatures were 25.1 and 25.3°C during the summer maize growth cycles in the two years.
2.2 Leaf area index
After 6 days of continuous waterlogging, six representative plant samples were signed from each plot (H-CK, H-W, C-CK, C-W) to measure and calculate leaf area. LAI was calculated as follows (Ren et al., 2016).
Leaf area (cm2)=L (cm)×W (cm)×0.75
LAI=(leaf area per plant×plant number per plot)/plot area
2.3 Soil and plant analyzer development value
After 6 days of continuous waterlogging, a chlorophyll meter (SPAD-502, Soil-plant Analysis Development Section, MinoltaMinolta-CameraCo., Osaka, Japan) was used to measure 12 times of each plot to obtain the leaf SPAD value.
2.4 Dry matter accumulation
After 6 days of continuous waterlogging, three typical plant samples were selected from each plot. The samples were placed in the oven at 105°C in a force-draft oven for 30 min, and then dried to constant weight at 80°C and weighed separately. Refer to the specific method as follows (Ren et al., 2017).
2.5 Stomatal phenotypic characteristics of leaves
After 6 days of continuous waterlogging, the stomatal samples were collected during 9:00-11:00 am. First of all, we collected stomatal imprints from the middle section of the new fully expanded leaf using colorless transparent nail polish. Three leaves were selected for each plot and two replicates per leaf. After drying, the leaf was cut with scissors and then pasted with colorless transparent tape on the leaf with nail polish to be tested on the slide (Zheng et al., 2013; Yan et al., 2021). The imprints were observed and photographed in the laboratory with a microscope (Biological microscope, Nikon of Japan, Ni-u). A total of 30 photos (3 × 2 × 5) were taken from 5 randomly selected fields of vision at 200 × for stomatal density (SD, number of pores per unit area) and proportion of open stomata (POS), and 30 photos were taken at 400 × for open stomatal length (SL), width (SW), perimeter (SP) and area (SA) measurement and recording (Li et al., 2018).
2.6 Leaf gas exchange parameters
After 6 days of continuous waterlogging, the leaf gas exchange parameters: the net photosynthetic rate (Pn), stomatal conductance (Gs), intercellular CO2 concentration (Ci), and transpiration rate (Tr) of ear leaves were measured between 10:00-12:00 am under saturating irradiance using a portable infrared gas analyzer (CIRAS-3, PP System, Hanstaech, U.K) and then calculated the pore limit value (Ls). Leaves with consistent growth and good light exposure under different treatments were selected. Five leaves were measured randomly for each treatment. Measurement conditions were kept consistent: LED light source and photosynthetically available radiation (PAR) of 1600 μmol m-2. CO2 concentration was maintained at a constant level of 360 μmol mol-1 using a CO2 injector with a high-pressure liquid CO2 cartridge.
Ls=(Ca-Ci)/Ca, in this formula, Ci was intercellular CO2 concentration and Ca was environmental CO2 concentration (Berry, 1982; Seng et al., 2012).
2.7 Chlorophyll fluorescence parameters
After 6 days of continuous waterlogging, photo-induced transients’ photoinduced transients (Supplementary Table 1) of Fo, Fm, Fv/Fm, Wk, Vj, TRo/CSm, ETo/CSm, DIo/CSm, ABS/CSm, ψO, φDo, φEo, φPo, PIABS of the five typical leaves each plot was measured by M-PEA (Multi-Function Plant Efficiency Analyser, Hansatech Instruments Limited, U.K). See (Strasser, 2004) for the specific meanings of the parameters. The M-PEA data version was 1.10, the measurement duration was 10.0s, the number of data points was 145, and the red-light intensity was 3000 μmol m-2 s-1. The determination time was consistent with that of photosynthetic parameters. The leaves should be dark-treated with clamps for 20 min before determination. Representative plants with good growth should be selected, with 5 plants in each treatment.
2.8 Grain yield
At R6, 30 ears harvested continuously selected from three rows were used to determine yield and ear traits. Grain yield was calculated as follows (Ren et al., 2016).
Grain yield (kg ha-1) = Harvest ear (ears ha-1)×Number of kernel per ear×1,000-grain weight (g/1000 grains)/106×(1-moisture content%)/(1-14%)
2.9 Statistical analysis
Microsoft Excel 2016 (Microsoft, Redmond, WA, USA) was used for data processing and analysis. IBM SPSS Statistics 18.0 (IBM Cor-poration, Armonk, NY, USA) was used for data analysis. Analysis of variance (ANOVA) and LSD (Least significant difference) tests was used for comparisons analysis. The coefficient of pearson was used for the analysis of correlation. The significant differences are at P<0.05. The data were plotted using Origin 2021 (OriginLab Corporation, MA, USA) and Sigmaplot 12.5 (Systat Software, Inc., Richmond, CA, USA) software.
3 Results
3.1 Effects of H2O2 priming on summer maize yield under waterlogging stress at the V3 stage
3.1.1 Yield
The summer maize yield was significantly reduced after waterlogging, but H2O2 priming could increase the yield of waterlogged summed maize (Table 2). In 2020, the grain yield of summer maize in C-W and H-W decreased by 24.3% and 12.4% compared with C-CK (Control treatment) respectively, C-W was 15.7% lower than H-W. The two-year results were basically consistent. The increase in grain number per ear was responsible for the significantly greater yields obtained from H2O2 priming waterlogged plants (Table 2). In 2020, the grain number per ear of H-W was 12.4% higher than C-W, C-W and H-W decreased by 16.5% and 6.3% compared with C-CK. The two-year results were basically consistent.
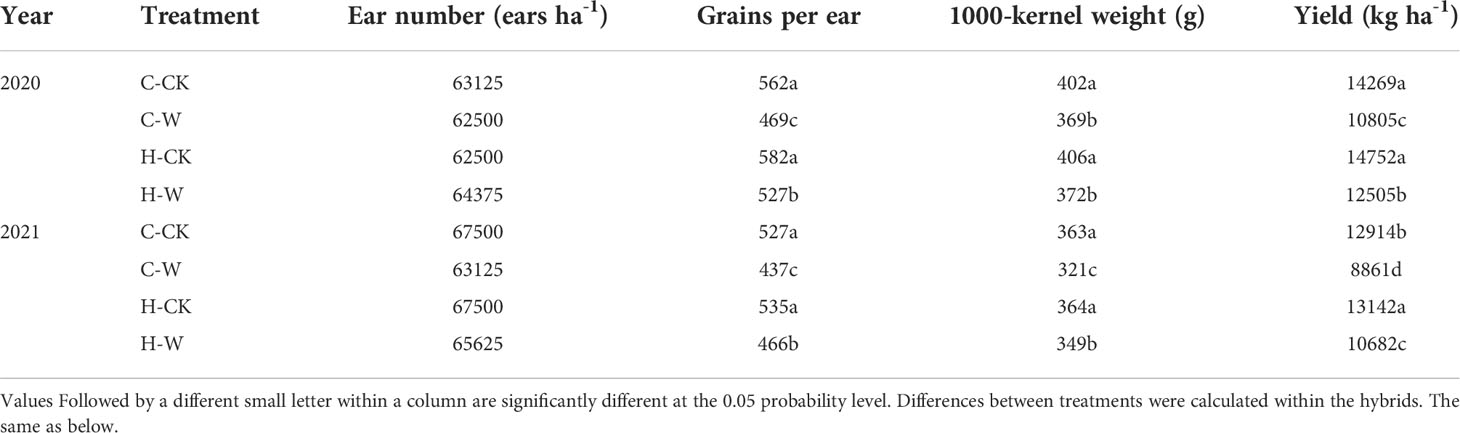
Table 2 Effects of H2O2 seed soaking on yield of summer maize under waterlogging stress at the seedling stage.
3.1.2 Dry matter weight
Waterlogging reduced the dry matter accumulation of summer maize, while H2O2 priming could significantly alleviate waterlogging damages on the weight of dry matter. In 2020, compared with C-CK, waterlogging significantly decreased dry matter weight by respectively 28.7% and 23.9% on C-W and H-W respectively, and H-W was 6.7% higher than C-W. The two-year results were basically consistent (Figure 1).
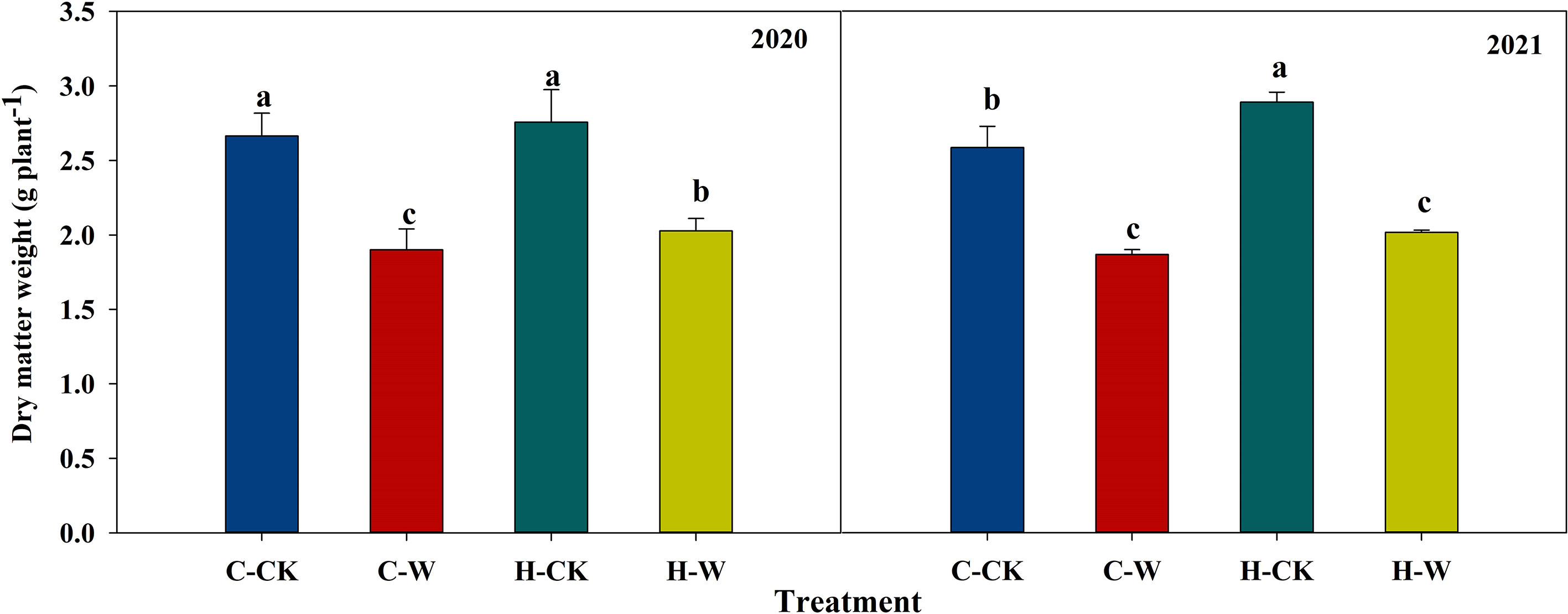
Figure 1 Effects of H2O2 priming on dry matter accumulation of summer maize under waterlogging stress at the seedling stage. Note: C-CK: Control treatment, C-W: Waterlogging for 6 days at the V3 stage; H-CK: H2O2-priming + non-waterlogging; H-W: H2O2-priming + waterlogging for 6 days at the V3 stage. The same as below.
3.2 Stomatal features of maize leaves
Stomatal density, stomatal length-width ratio, and stomatal shape index of summer maize increased significantly after waterlogging, while the proportion of opening stomas, stomatal length, and width was significantly decreased. H2O2 priming alleviated the effect on the stomatal performance of waterlogged summer maize (Table 3). In 2020, the stomatal density (SD) of C-W was increased by 44.3% compared with C-CK, H-W decreased by 11.0% compared with C-CK, and H-W were decreased by 36.4% compared with C-W, but there was no significant difference between H-CK and C-CK. The stomatal length-width ratio (SL/SW) of H-W were decreased by 22.9% compared with C-W. The stomatal opening ratio (POS) of H-W was increased by 137.1% compared with the C-W. The two-year results were basically consistent. Additionally, the stomatal arrangement pattern was irregular, and stomatal density and the number of opening stomas were harmed after waterlogging stress at the V3 stage. H2O2 priming could significantly alleviate waterlogging damages on the stomatal arrangement pattern, density, and size of summer maize. It could be seen that the stomatal size and the degree of the stomatal opening of C-W were significantly smaller, and the open stomas were slender and flat, than those of C-CK. After priming with H2O2, the size and the degree of stomatal opening were significantly increased (Figure 2). The two-year results were basically consistent.
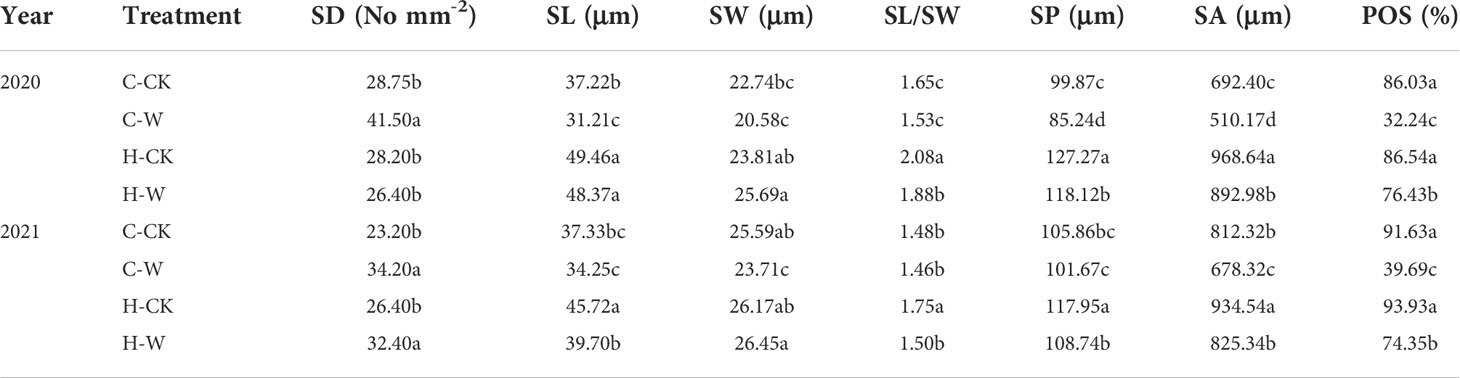
Table 3 Effects of H2O2 priming on leaf stomatal morphology of summer maize under waterlogging stress at the seedling stage.
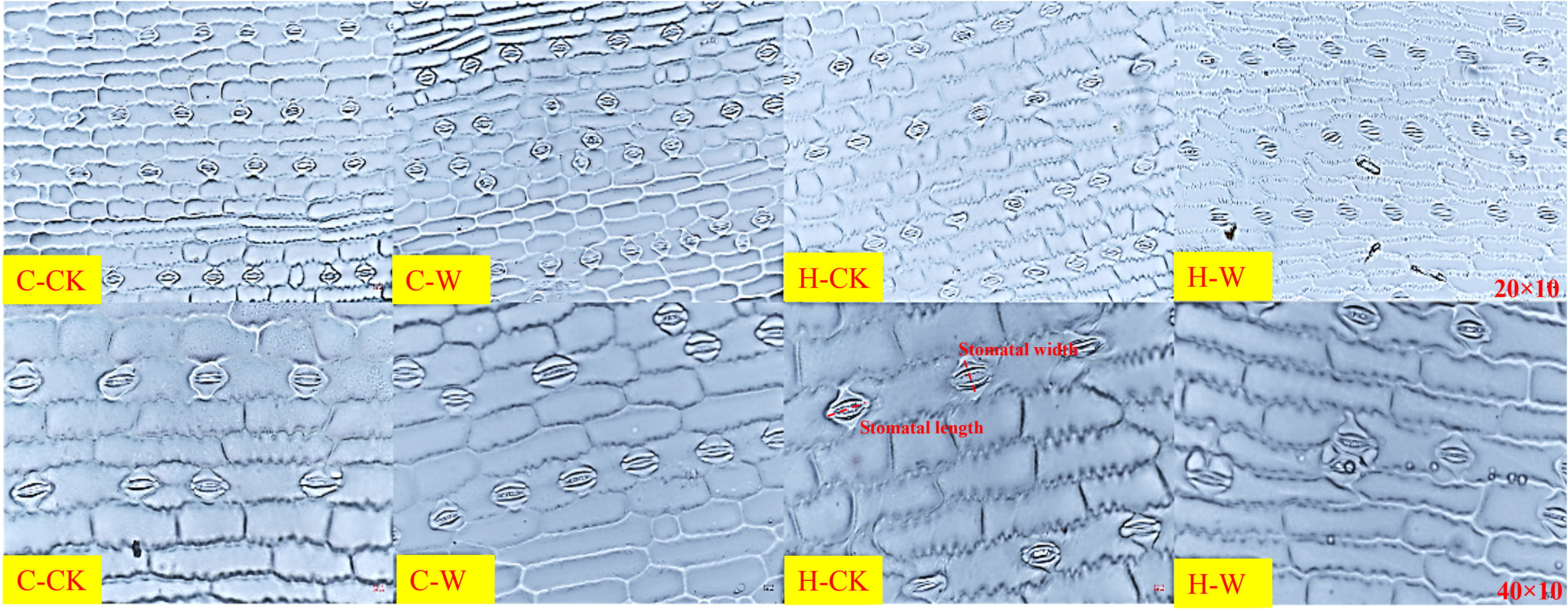
Figure 2 Effects of H2O2 priming on stomatal morphological characteristics of summer maize under waterlogging stress at the seedling stage.
3.3 Photosynthetic characteristics
3.3.1 Leaf area index
The leaf area index (LAI) was decreased significantly after waterlogging, but H2O2 priming could alleviate the negative effects on LAI after waterlogging. In 2020, the LAI of H-W was increased by 9.4% compared with C-W, the C-W and H-W was decreased by 48.6% and 43.7% than C-CK. The two-year results were basically consistent (Figures 3A, B).
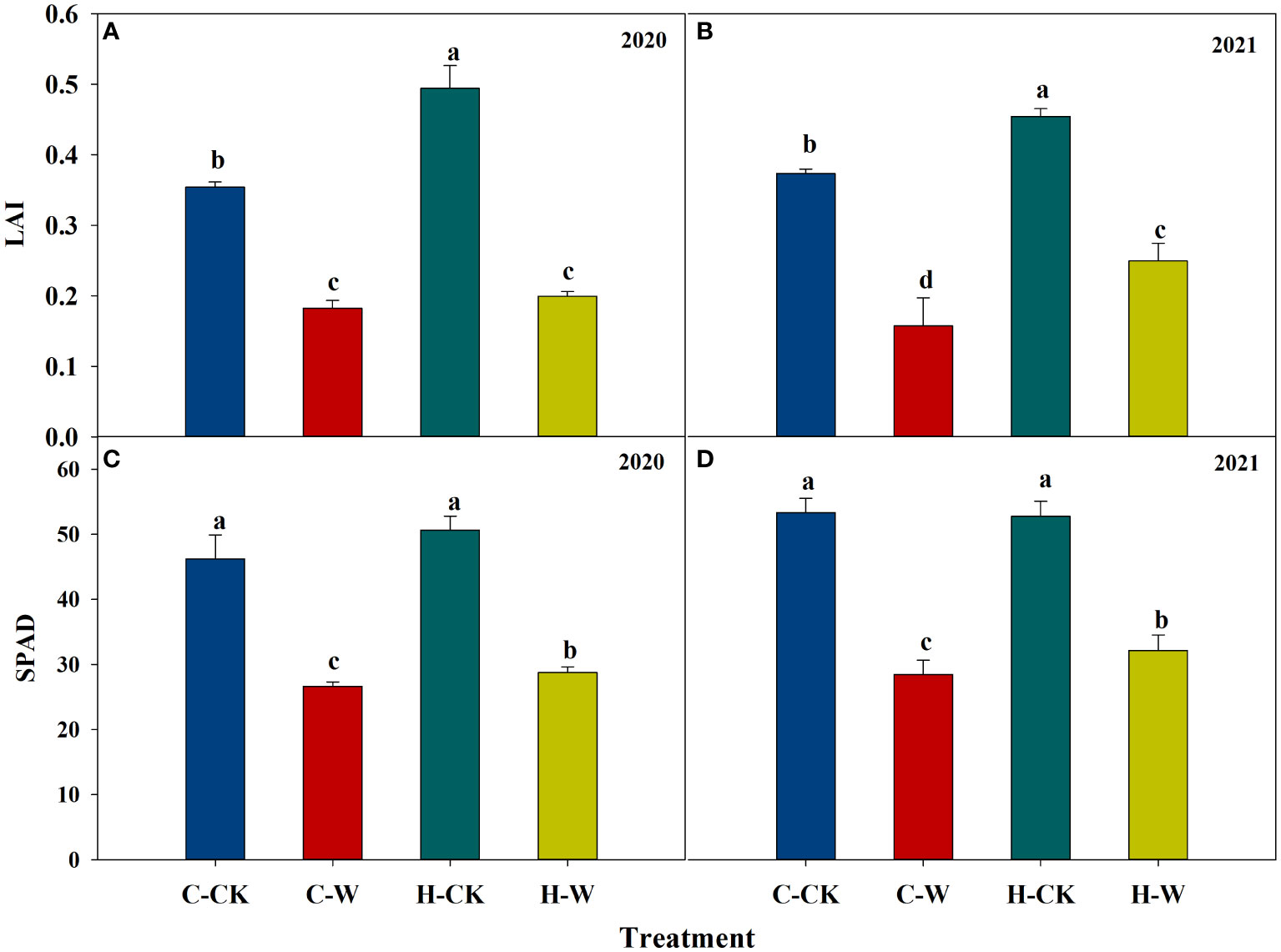
Figure 3 Leaf area index and Soil and plant analyzer development (SPAD) value. (A, B) Leaf area index in 2020 and 2021; (C, D) Soil and plant analyzer development (SPAD) value in 2020 and 2021.
3.3.2 Soil and plant analyzer development value
Soil and Plant Analyzer Development (SPAD) value was decreased significantly after waterlogging, but H2O2 priming could significantly alleviate the effects of waterlogging on SPAD. In 2020, pretreatment with H2O2 significantly increased the SPAD (around 8.0%) compared with C-W. The two-year results were basically consistent (Figures 3C, D).
3.3.3 Leaf gas exchange parameters
Leaf Pn, Gs, Ci, and Tr were significantly decreased after waterlogging. In 2020, a reduction in Pn (around 51.1%), Gs (around 69.5%), Ci (around 14.8%), and Tr (around 46.3%) was observed in C-W treatment compared with C-CK. Pretreatment with H2O2 increased the Pn (around 37.5%), Gs (around 80.4%), Ci (around 12.1%), and Tr (around 46.3%) of summer maize compared with C-W. The two-year results were basically consistent (Figure 4). The stomatal limitation (Ls) of summer maize increased significantly after waterlogging stress, and H2O2 priming could significantly reduce the Ls of waterlogged maize. In 2020, the stomatal limitation of H-W decreased by 15.7% compared with C-W. The two-year results were basically consistent (Figure 4).
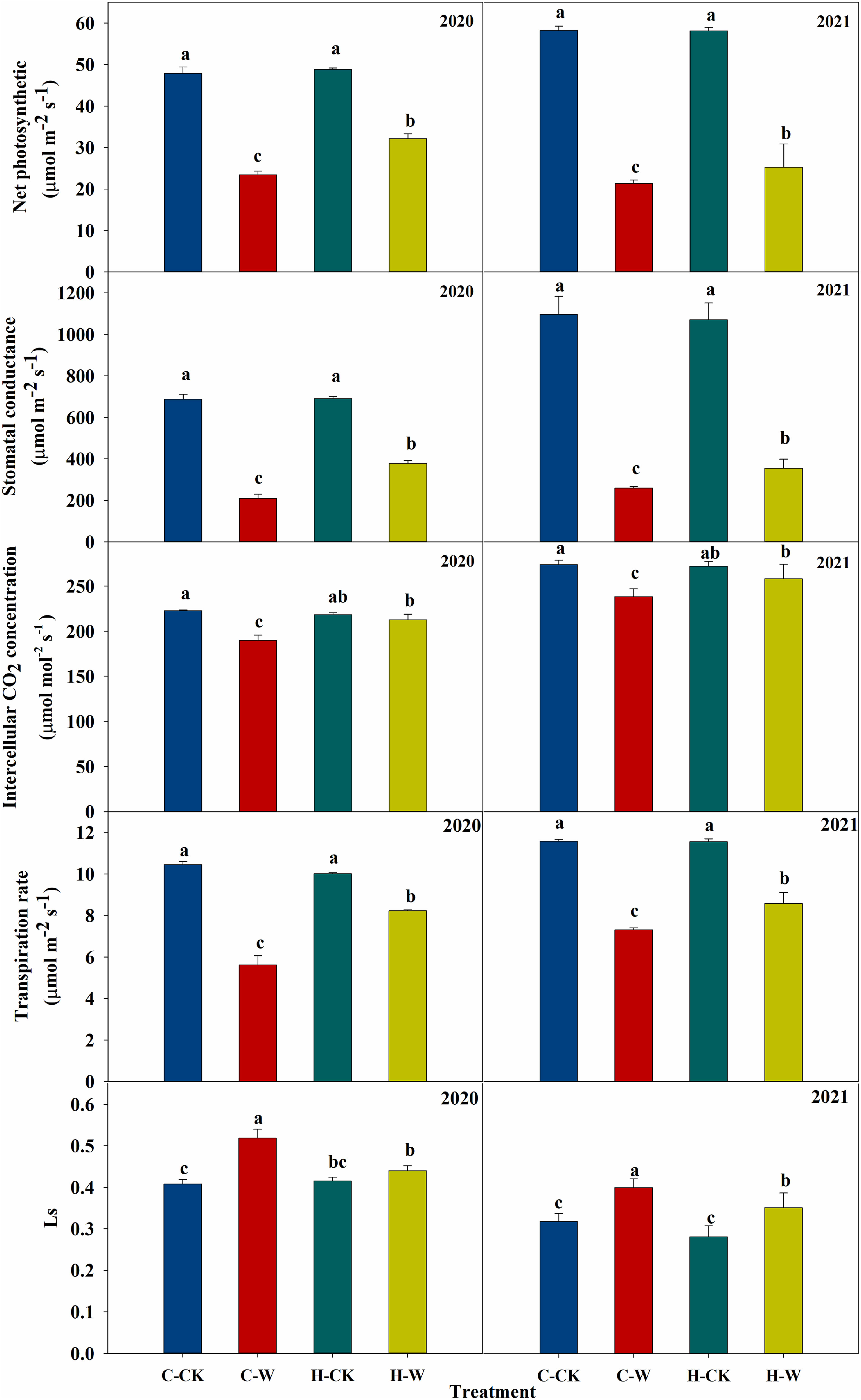
Figure 4 Effects of H2O2 priming on gas exchange parameters of summer maize under waterlogging stress at the seedling stage.
3.4 Chlorophyll fluorescence parameters
3.4.1 Changes in PSII donor/acceptor sides
The shape of the Vt curve was obviously changed after waterlogging stress. As was shown in (Figure 5), the K and J step were significantly increased after waterlogging. The Wk of H-W was decreased by 14.6% compared with that of C-W. The Vj of H-W was 25.7% lower than that of C-W. The two-year results were basically consistent (Figure 5).
3.4.2 PSII biophysical parameters derived by the JIP-test equations
3.4.2.1. Phenomenological energy fluxes (per exciting cross-section - CS)
Under waterlogging stress, electron transport flux per excited CS (ETo/CSo) in maize leaves was significantly decreased, but the heat dissipation per unit area (DIo/CSo) was significantly increased. H2O2 priming significantly increased ETo/CSo and decreased DIo/CSo (Figure 6A). In 2020, ETo/CSo of H-W was increased by 55.3% compared with that of C-W. DIo/CSo of H-W was decreased by 37.6% compared with that of C-W. The two-year results were basically consistent (Figure 6A). There was a decrease in trapping flux per excited CS (TRo/CSm) (blue arrow), electron transport flux per excited CS (ETo/CSm) (purple arrow), absorption flux per excited CS (ABS/CSm) (yellow arrow), but an increase in dissipated energy flux per excited CS (DIo/CSm) (red arrow) in waterlogged maize (Figures 6A, B). In 2020, ETo/CSm of H-W was increased 102.7% compared with that of C-W; DIo/CSm of H-W was decreased 18.7% compared with that of C-W. The two-year results were basically consistent (Figures 6A, B).
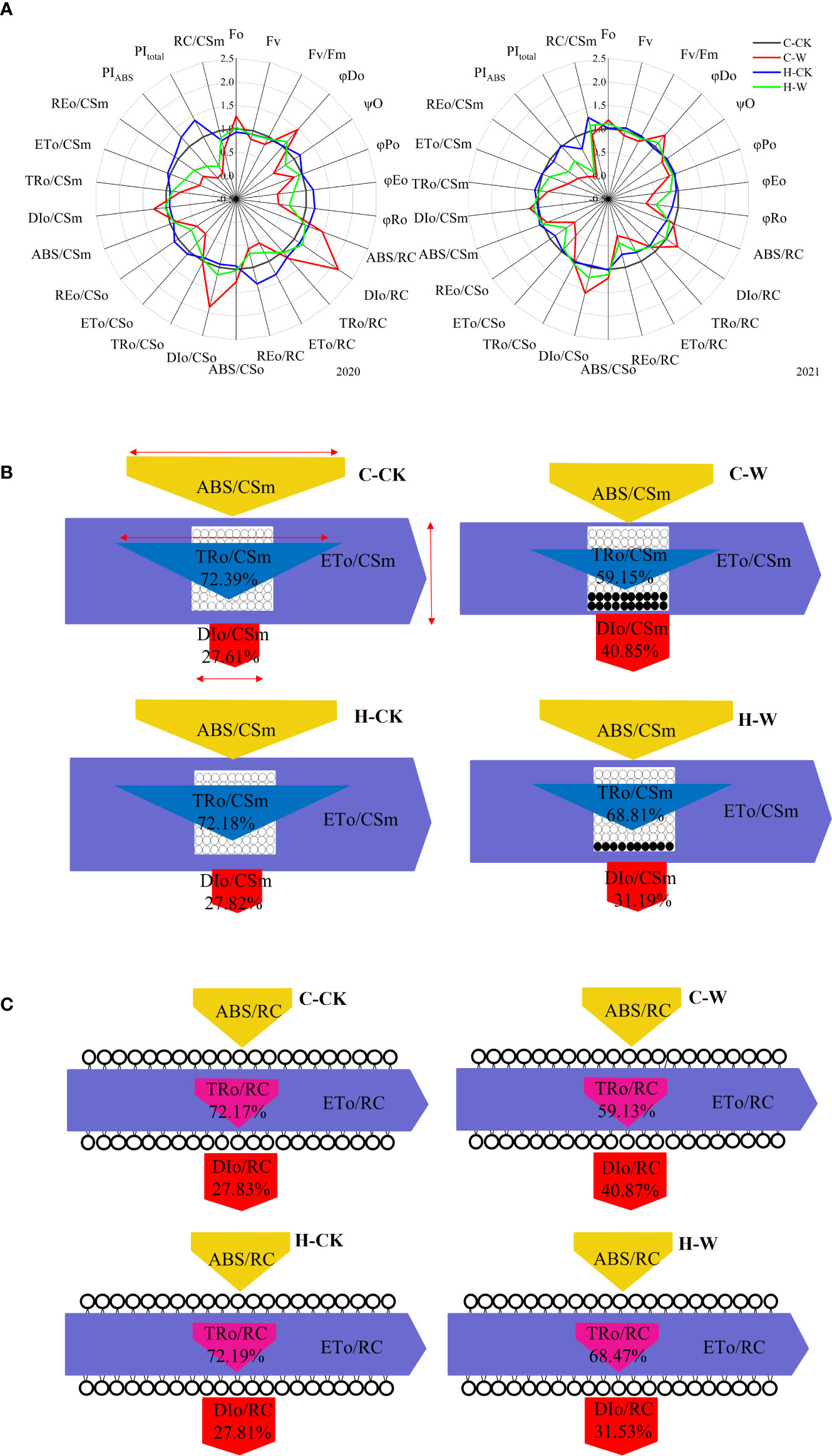
Figure 6 PSII biophysical parameters derived by the JIP-test equations. (A) Changes of JIP parameter radar images in 2020 and 2021. Note: The values were calculated relative to the C-CK, taken as 1, and the fluorescence parameters of other treatments were transformed into the percentage of C-CK fluorescence parameters. (B) Energy pipeline models of phenomenological fluxes (per cross-section, CSm) in 2020. The decrease in absorption (yellow), trapping (cyan), and electron transport (purple), can be seen as changes in the width of each arrow (red arrow = scale). The active reaction center is represented by a white circle, and the inactive reaction center is represented by a black circle. (C) Specific energy flux (membrane model) in 2020.
3.4.2.2 Specific energy fluxes (per QA reducing PSII reaction center – RC)
Waterlogging stress significantly reduced electron transport flux per RC (ETo/RC) and increased dissipated energy flux per RC (DIo/RC) in maize seedling leaves, while H2O2 priming significantly increased ETo/RC and decreased DIo/RC (Figures 6A, C). In 2020, RC/CSm of C-W and H-W were decreased by 42.6% and 15.6% compared with that of C-CK, respectively, and H-W was increased by 47.0% compared with that of C-W; ETo/RC of H-W was increased by 41.0% compared with that of C-W; DIo/RC of H-W was decreased by 43.1% compared with that of C-W (Figures 6A, C). The two-year results were basically consistent (Figures 6A, C).
3.4.2.3 Quantum yields and efficiencies/probabilities
The probability (at t = 0) that a trapped exciton moves an electron into the electron transport chain beyond QA- (Ψo), the quantum yield of electron transport (φEo), and the maximum quantum yield of primary photochemistry (φPo) were decreased significantly and the quantum ratio (φDo) was increased significantly after waterlogging, while H2O2 priming significantly increased Ψo, φEo, φPo and significantly decreased φDo (Figure 6A). In 2020, φEo of H-W was increased by 89.9% compared with that of C-W; φPo of H-W was increased 16.2% compared with that of C-W; φDo of H-W was decreased by 23.5% compared with that of C-W (Figure 6A). In 2020, PIABS of H-W was increased by 352.8% compared with that of C-W (Figure 6A).
3.5 Correlation analysis
Correlation analysis showed that the net photosynthetic rate of summer maize was related to stomatal factors and non-stomatal factors. Pn was positively correlated with POS, Gs, and Ci. From the perspective of chlorophyll fluorescence, Pn was significantly positively correlated with φPo, φEo, φRo, and PIABS, but negatively correlated with φDo (Figure 7). The decrease in grain yield of waterlogged summer maize was significantly correlated with the decreases of 1000-grain weight and grain number per spike. From the perspective of stomata, grain number per ear, 1000-grain weight, and yield were significantly negatively correlated with stomatal density (SD), but positively correlated with the proportion of open stomata (POS). Grain number per ear, 1000-grain weight, and yield was significantly positively correlated with Gs, Ci, Tr and Pn. From the perspective of chlorophyll fluorescence, grain number per ear, 1000-grain weight, and yield were significantly positively correlated with φPo, φEo, φRo, and PIABS, but significantly negatively correlated with φDo (Figure 7).
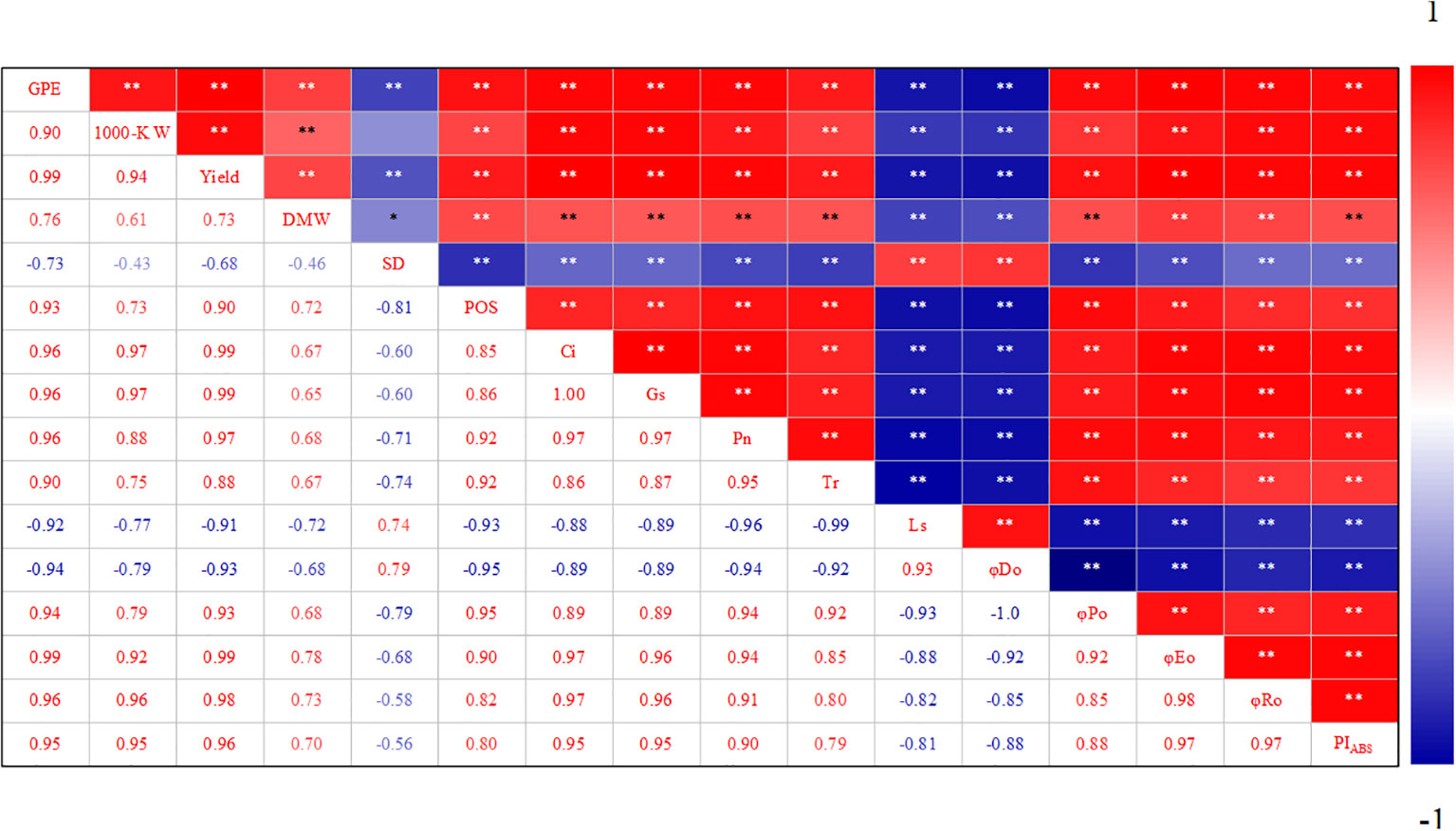
Figure 7 Correlation analysis of photosynthetic characteristics of soaking seeds with H2O2 under waterlogging stress. GPE: Grains per ear; 1000-K W: 1000-kernel weight; DMW: Dry matter weight; SD: Stomatal density; POS: Proportion of open stomata; Ci: Intercellular CO2 concentration; Gs: Stomatal conductance; Pn: The net photosynthetic rate; Tr: Transpiration rate; Ls: Calculate the pore limit value; φDo: Quantum yield of electron transport (at t = 0); φPo: The maximum quantum yield of primary photochemistry (at t = 0); φEo: Quantum yield of electron transport (at t = 0); φRo: Quantum yield for reduction of end electron acceptors at the PSI acceptor side (RE) (at t = 0); PIABS: The performance index for energy conservation from exciton to the reduction of intersystem electron acceptors.
4 Discussion
4.1 The mitigation effect of H2O2 priming on photosynthetic characteristics of waterlogged summer maize at the seedling stage
4.1.1 H2O2 priming improved the stomatal performance improving photosynthetic characteristics
The leaves photosynthetic characteristics are closely related to the leaf structures. The stomatal conductance and photosynthetic rate were affected by the stomata, one of the important leaf structures. The stomatal conductance was positively correlated with stomatal size and negatively correlated with stomatal density (Wu et al., 2014; Haworth et al., 2015). To better adapt to waterlogging stress, stomata would become smaller in size and larger in number which might be related to the decrease of leaf area (Xu, 1997; Yu et al., 2003; Wang et al., 2010; Jurczyk et al., 2016; Ploschuk et al., 2018; Hu et al., 2019). In this study, the stomatal length, width, and the proportion of open stomata of waterlogged summer maize leaves were markedly decreased. Additionally, the stomas of plant leaves altered from regular arrangement to random distribution in waterlogged plants, thereby enhancing the CO2 diffusion distance from stomas to photosynthetic parts. Accordingly, Gs and Ci of C-W were considerably decreased, while Ls were significantly increased, demonstrating that the decrease of Pn was predominantly caused by stomatal limitation factors. These results suggested that waterlogging had a grave effect on stomach traits of plants which blocked the uptake of CO2, and here impeding the photosynthesis, and reducing the dry matter accumulation eventually. It has been demonstrated that H2O2 priming was conducive to regulate stomatal characteristics, thus improving the gas exchange characteristics of leaves under waterlogging conditions (Castro-Duque et al., 2020). This study showed that the stomatal density of leaves reduced considerably and the stomatal size increased significantly in H2O2 priming/waterlogging-stress plants. Additionally, the proportion of opening stomata was significantly increased, and the stomata were arranged in H-W, thus enhancing the uptake of CO2. As a result, the Gs, Ci were increased by H2O2 priming, leading to a higher photosynthetic rate (Figure 8).
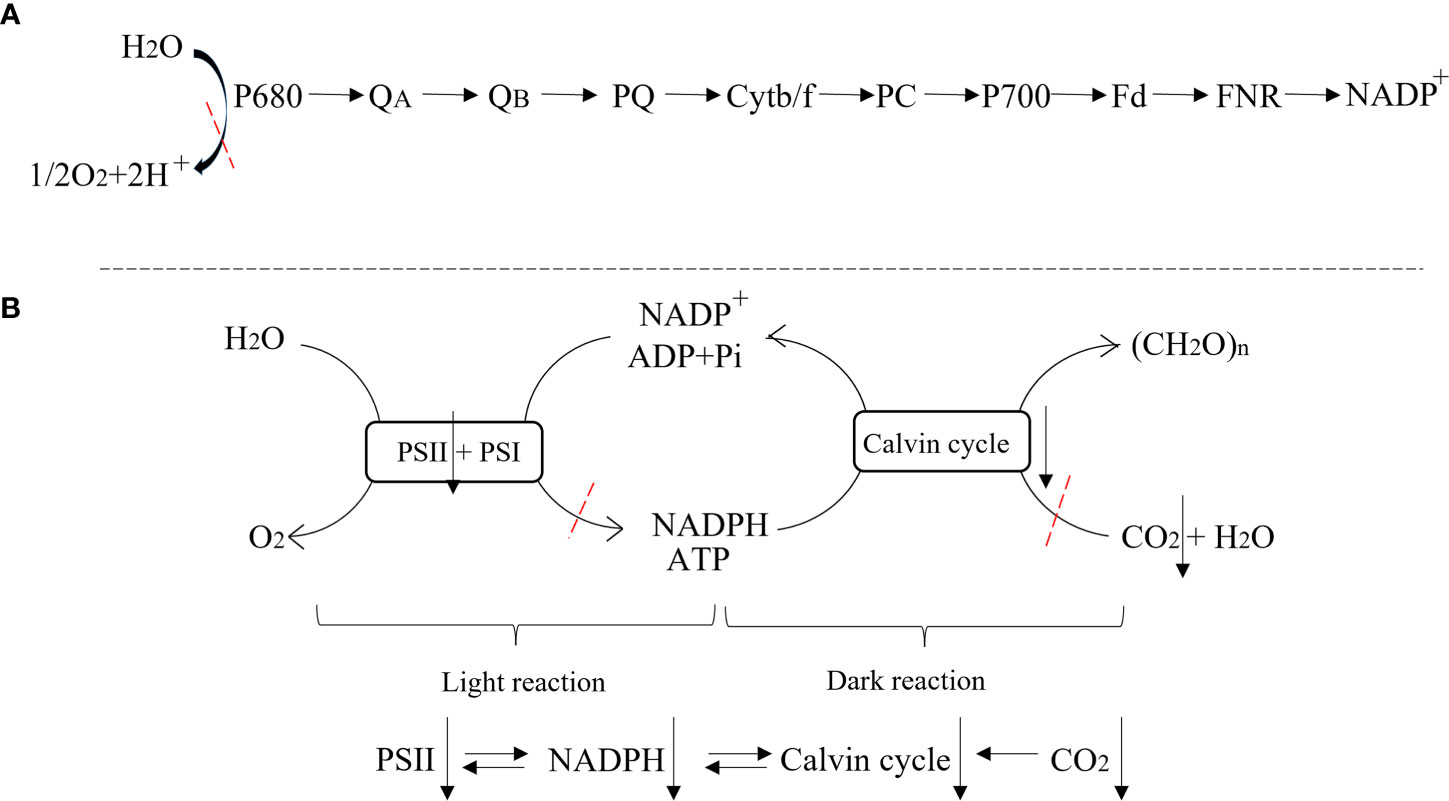
Figure 8 Schematic diagram of photosynthesis process. (A) Photosynthetic electron transport process; (B) Light and dark reaction.
4.1.2 H2O2 priming improved the light capture and conversion improving photosynthetic
Chlorophyll fluorescence induction kinetics is a sensitive probe to study the absorption, transformation, transmission, and distribution of sunlight energy in plants. The changes in chlorophyll fluorescence parameters can effectively reflect the characteristics of light reaction in photosynthesis (Strasserf and Srivastava, 1994; Yang et al., 2004; Kalaji et al., 2014; Li et al., 2020). PSII reaction centers were damaged and the number of active reaction centers was decreased after waterlogging (Li et al., 2005). The PSII reaction center converted the captured light energy into excitation energy. Most of the excitation energy was used to promote carbon assimilation, and the excess excitation energy was lost in the form of heat dissipation (Zuo et al., 2019). The number of RC/CSm was decreased after waterlogging, which damaged the PSII reaction centers and the antenna pigment structure. At the same time, Ro/CSm and ETo/CSm were decreased, but the DIo/CSm was increased, thus, the utilization rate of light energy per cross area, the share of energy devoted to electron transport were reduced, and heat dissipation was increased (Li et al., 2013; Liu et al., 2016). A similar conclusion could be drawn from quantum yields. Thus, waterlogging stress by decreasing the utilization of light energy and increased heat dissipation to limit the PSII photosynthetic electron transport chain. The level of electron transport inhibition at the donor or acceptor was closely related to PSII apparent activation energies (Chen et al., 2013; Sunil et al., 2020; Xing et al., 2021). This research showed that waterlogging stress significantly enhanced the K-step of summer maize, implying that the oxygen release complex (OEC) was damaged, the existence time of strong oxidant P680+ was prolonged, and the D1 protein in the reaction center of PSII was destroyed by oxidation, so as to damage the photosynthetic organs and decrease the photosynthetic activity. Additionally, the J-point was also significantly raised by waterlogging stress, indicating that the electron transfer from QA to QB was also significantly impeded, and reduced QA was accumulated, resulting in the singlet oxygen 1O2 formation and biofilm lipid peroxidation being increased.
Studies have shown that exogenous H2O2 could be used as a signal molecule to alleviate the effects of waterlogging on plants by regulating PSII efficiency and protecting chloroplasts (Castro-Duque et al., 2020; Hasanuzzaman et al., 2020). H2O2 priming significantly increased the RC/CSm, indicating that H2O2 priming protected the PSII reaction center. At the same time, H2O2 priming enhanced the electron transfer at the PSII reaction center and the donor/acceptor side of maize, decreasing the waterlogging induced raise of K and J steps, which effectively promoted the photosynthetic electron transport chain, reduced the oxidant P680+ and 1O2, protected the photosystem from oxidative damages and reduced the damage of membrane lipid peroxidation. Additionally, H2O2 priming significantly reduced DIo/CSm, indicating that the absorption and transfer of PSII light energy were improved. Accordingly, H2O2 priming increased the PIABS, and the photochemical properties were improved, which alleviated the electron transport coupled photosynthetic phosphorylation process, and boosted the Calvin cycle. In summary, H2O2 priming effectively improved the capture, conversion, and transmission of light energy, protecting plants from photoinhibition and enhancing the carbon assimilation processes (Figure 8).
4.2 Yield
The decrease in grain yield was significantly associated with the decrease in 1000-grain weight and grain number ear (Ren et al., 2016). The accumulation of photosynthetic substances depends on the strength of photosynthesis, and the formation of yield was determined by the accumulation and transport of photosynthetic substances (Ren et al., 2015). The results of this study showed that waterlogging stress could significantly reduce the yield of summer maize, and its 1000-grain weight and grain number per ear were significantly affected, with grain number per ear being the most affected. Dry matter accumulation was blocked after waterlogging, resulting in insufficient assimilation for grain development, thus leading to a reduction in grain yield (Ren et al., 2014). In summary, this study showed that waterlogging stress significantly reduced photosynthetic rate through damaging stomatal performance, PSII photoinhibition, and the light capture and conversion, and the LAI and SPAD were decreased, resulting in a blockage in dry matter accumulation, and the yield was decreased finally. However, H2O2 priming could significantly mitigate the waterlogging damages on stomatal characteristic and photosystems, protecting chloroplasts and other photosynthetic organs from oxidative damage to increase the photosynthetic rate, and increase the biomass accumulation rate and grain yield of waterlogged summer maize (Figures 8, 9).
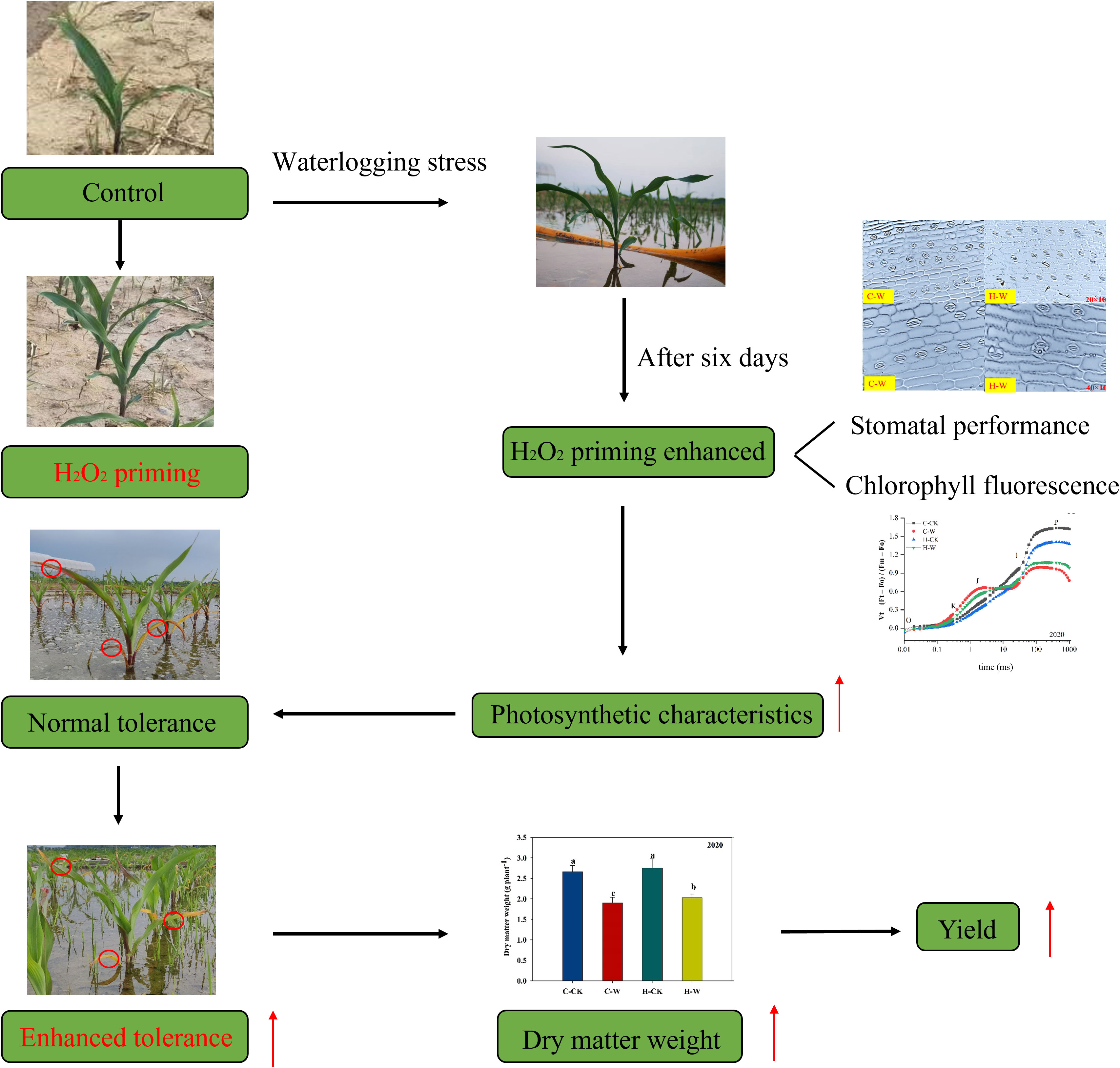
Figure 9 The mechanism of H2O2 priming could enhance the photosynthetic characteristics of summer maize. Note: Red circles indicated H2O2 priming versus flooded maize leaves, and red arrows indicated that H2O2 priming alleviates flooding stress.
5 Conclusions
Waterlogging stress inhibited the light and dark response of the leaves. Waterlogging decreased Pn through stomatal limitation of CO2 supply and reduction of PSII photochemical efficiency by limiting the donor and acceptor side of PSII and the activity of the reaction center, which would lead to the decrease of dry matter weight and grain yield. H2O2 priming could alleviate the negative effects of on waterlogged summer maize by increasing the CO2 supply for carbon fixation, protecting PSII reaction centers, and enhancing electron transport to promote energy supply for carbon fixation. As a result, the photosynthetic capacity of waterlogged summer maize was significantly increased by H2O2 priming, dry matter weight and grain yield were increased eventually. In summary, H2O2 priming could modulate the waterlogging stress tolerance of summer maize: insights from photosynthetic.
Data availability statement
The original contributions presented in the study are included in the article/Supplementary Material. Further inquiries can be directed to the corresponding author.
Author contributions
SW: Conceptualization, Formal analysis, Investigation, Methodology, Writing – original draft.; JH: Conceptualization, Investigation, Methodology, Writing - Review and Editing; BR: Investigation, Resources, Writing – review and editing; BZ: Investigation, Resources, Writing – review and editing; PL: Investigation, Resources, Writing – review and editing; JZ: Conceptualization, Funding acquisition, Data curation, Supervision, Writing – review and editing. All authors contributed to the article and approved the submitted version.
Funding
This work was supported by the Shandong Province key research and development plan (2021LZGC014-2), National Natural Science Foundation of China (32172115), the earmarked fund for CARS (CARS-02-21) and the Shandong Agricultural Application Technology Innovation Project (Grant numbers SD2019ZZ013).
Acknowledgments
We acknowledge the support of the State Key Laboratory of Crop Biology and College of Agronomy, Shandong Agricultural University.
Conflict of interest
The authors declare that the research was conducted in the absence of any commercial or financial relationships that could be construed as a potential conflict of interest.
Publisher’s note
All claims expressed in this article are solely those of the authors and do not necessarily represent those of their affiliated organizations, or those of the publisher, the editors and the reviewers. Any product that may be evaluated in this article, or claim that may be made by its manufacturer, is not guaranteed or endorsed by the publisher.
Supplementary material
The Supplementary Material for this article can be found online at: https://www.frontiersin.org/articles/10.3389/fpls.2022.1042920/full#supplementary-material
Supplementary Figure 1 | The details of meteorological data during maize waterlogging periods.
Supplementary Table 1 | Jip-test specifies the parameter term.
References
Andrade, C. A., Souza, K. R. D., Santos, M.d. O., Silva, D. M., Alves, J. D. (2018). Hydrogen peroxide promotes the tolerance of soybeans to waterlogging. Sci. Hortic. 232, 40–45. doi: 10.1016/j.scienta.2017.12.048
Ashraf, M., Harris, P. J. C. (2013). Photosynthesis under stressful environments: An overview. Photosynthetica 51, 163–190. doi: 10.1007/s11099-013-0021-6
Ayano, M., Kani, T., Kojima, M., Sakakibara, H., Ashikari, M. (2015). Gibberellin biosynthesis and signal transduction is essential for internode elongation in deepwater rice. Plant Cell Environ. 37, 2313–2324. doi: 10.1111/pce.12377
Berry, J. A. (1982). Environmental regulation of photosynthesis. Photosynthesis 263–343. doi: 10.1016/b978-0-12-294302-7.50017-3
Castro-Duque, N. E., Chavez-Arias, C. C., Restrepo-Diaz, H. (2020). Foliar glycine betaine or hydrogen peroxide sprays ameliorate waterlogging stress in cape gooseberry. Plants (Basel) 9, 644. doi: 10.3390/plants9050644
Chen, X. L., Li, G., Liu, P., Gao, H. Y., Dong, S. T., Wang, Z. L., et al. (2013). Effects of exogenous hormone 6-BenzylAdenine (6-BA) on photosystem II performance of maize during process of leaf senescence under different nitrogen fertilization levels. Acta Agron. Sin. 39, 1111–1118. doi: 10.3724/SP.J.1006.2013.01111
Gao, F. (2018). Effect of 4-PBA on the growth and yield of summer maize under flooding stress. PhD dissertation. J. Henan Agric. Univ.
Gao, Y., Guo, Y.-K., Lin, S.-H., Fang, Y.-Y., Bai, J.-G. (2010). Hydrogen peroxide pretreatment alters the activity of antioxidant enzymes and protects chloroplast ultrastructure in heat-stressed cucumber leaves. Sci. Hortic. 126, 20–26. doi: 10.1016/j.scienta.2010.06.006
Gdsa, A., Pm, A., Skdpp, B., Edcm, B., Ldsl, A., Ecm, A., et al. (2020). H2O2 priming promotes salt tolerance in maize by protecting chloroplasts ultrastructure and primary metabolites modulation. Plant Sci 303, 110774. doi: 10.1016/j.plantsci.2020.110774
Gondim, F. A., Gomes-Filho, E., Lacerda, C. F., Prisco, J. T., Marques, E. C. (2009). Pretreatment with H2O2 in maize seeds: Effects on germination and seedling acclimation to salt stress. Braz. J. Plant Physiol. 22, 103–112. doi: 10.1590/S1677-04202010000200004
Hasanuzzaman, M., Bhuyan, M., Zulfiqar, F., Raza, A., Mohsin, S. M., Mahmud, J. A., et al. (2020). Reactive oxygen species and antioxidant defense in plants under abiotic stress: Revisiting the crucial role of a universal defense regulator. Antioxid. (Basel) 9, 681. doi: 10.3390/antiox9080681
Haworth, M., Killi, D., Materassi, A., Raschi, A. (2015). Coordination of stomatal physiological behavior and morphology with carbon dioxide determines stomatal control. Am. J. Bot 102, 677–688. doi: 10.3732/ajb.1400508
Hossain, M. A., Bhattacharjee, S., Armin, S. M., Qian, P., Xin, W., Li, H. Y., et al. (2015). Hydrogen peroxide priming modulates abiotic oxidative stress tolerance: insights from ROS detoxification and scavenging. Front. Plant Sci. 6. doi: 10.3389/fpls.2015.00420
Hu, J., Ren, B. Z., Dong, S. T., Liu, P., Zhao, B., Zhang, J. W. (2020). Comparative proteomic analysis reveals that exogenous 6-benzyladenine (6-BA) improves the defense system activity of waterlogged summer maize. BMC Plant Biol. 20, 44. doi: 10.1186/s12870-020-2261-5
Hu, J., Ren, B. Z., Dong, S. T., Liu, P., Zhao, B., Zhang, J. W. (2021). Poor development of spike differentiation triggered by lower photosynthesis and carbon partitioning reduces summer maize yield after waterlogging. Crop J. 10, 478–489. doi: 10.1016/j.cj.2021.08.001
Hu, X. Y., Yu, H. Y., Cui, Y. F., Fan, S. Q., Bi, Q. X., Li., Y. C., et al. (2019). Response of stomatal distribution characteristics on leaves of xanthocera sorbifolia from different provenances to water stress. For. Res. 32, 169–174. doi: 10.13275/j.cnki.lykxyj.2019.01.023
Jurczyk, B., Pociecha, E., Janowiak, F., Kabala, D., Rapacz, M. (2016). Variation in waterlogging-triggered stomatal behavior contributes to changes in the cold acclimation process in prehardened lolium perenne and festuca pratensis. Plant Physiol. Biochem. 109, 280–292. doi: 10.1016/j.plaphy.2016.10.012
Kalaji, H. M., Oukarroum, A., Alexandrov, V., Kouzmanova, M., Brestic, M., Zivcak, M., et al. (2014). Identification of nutrient deficiency in maize and tomato plants by in vivo chlorophyll a fluorescence measurements. Plant Physiol. Biochem. 81, 16–25. doi: 10.1016/j.plaphy.2014.03.029
Kaori, S., Mai, N. H., Motoaki, S. (2020). Advances in chemical priming to enhance abiotic stress tolerance in plants. Plant Cell Physiol 119. doi: 10.1093/pcp/pcaa119
Li, P. M., Gao, H. Y., Strasser, R. J. (2005). Application of rapid chlorophyll fluorescence induction kinetics analysis in photosynthesis studies. J. Plant Physiol. Mol. Biol. 31, 8. doi: 10.3321/j.issn:1671-3877.2005.06.001
Li, X. X., Liu, B. X., Guo, Z. T., Chang, Y. X., He, L., Chen, F., et al. (2013). Effects of NaCl stress on photosynthesis characteristics and fast chlorophyll fluorescence induction dynamics of pistacia chinensis leaves. Chin. J. Appl. Ecol. 24, 6. doi: doi: CNKI: SUN: YYSB.0.2013-09-013
Li, F., Liu, L., Zhang, H., Wang, Q. T., Guo, L. L., Hao, L. H., et al. (2018). Effects of CO2 concentrations on stomatal traits and gas exchange in leaves of soybean. Acta Agron. Sin. 44, 1212–1220. doi: 10.3724/SP.J.1006.2018.01212
Liu, Q. Q., Ma, S. B., Feng, X. H., Sun, Y., Yi, Y. J., Liu, W. X. (2016). Effects of grafting on the fast chlorophyll fluorescence induction dynamics of pepper seedlings under temperature stress. Acta Hortic. Sin. 43, 885–896. doi: 10.16420/j.issn.0513-353x.2015-0970
Li, Y. T., Xu, W. W., Ren, B. Z., Zhao, B., Zhang, J. W., Liu, P., et al. (2020). High temperature reduces photosynthesis in maize leaves by damaging chloroplast ultrastructure and photosystem II. J. Agron. Crop Sci. 206, 548–564. doi: 10.1111/jac.12401
Munné-Bosch, S., Alegre, L. (2013). Cross-stress tolerance and stress "memory" in plants: an integrated view. Environ. Exp. Bot. 94, 1–2. doi: 10.1016/j.envexpbot.2013.02.002
Perez, I. B., Brown, P. J. (2014). The role of ROS signaling in cross-tolerance: from model to crop. front. Plant Sci. 5. doi: 10.3389/fpls.2014.00754
Petrov, V. D., Breusegem, F. V. (2012). Hydrogen peroxide–a central hub for information flow in plant cells. AoB Plants (0), pls014–pls014. doi: 10.1093/aobpla/pls014
Ploschuk, R. A., Miralles, D. J., Colmer, T. D., Ploschuk, E. L., Striker, G. G. (2018). Waterlogging of winter crops at early and late stages: Impacts on leaf physiology, growth and yield. Front. Plant Sci. 9. doi: 10.3389/fpls.2018.01863
Ren, B. Z., Hu, J., Zhang, J. W., Dong, S. T., Liu, P., Zhao, B. (2020). Effects of urea mixed with nitrapyrin on leaf photosynthetic and senescence characteristics of summer maize (Zea mays L.) waterlogged in the field. J. Integr. Agric. 19, 1586–1595. doi: 10.1016/S2095-3119(19)62725-5
Ren, B. Z., Zhang, J. W., Dong, S. T., Liu, P., Zhao, B. (2016). Effects of waterlogging on leaf mesophyll cell ultrastructure and photosynthetic characteristics of summer maize. PloS One 11 (9), e0161424. doi: 10.1371/journal.pone.0161424
Ren, B. Z., Zhang, J. W., Dong, S. T., Liu, P., Zhao, B. (2018). Exogenous 6-benzyladenine improves antioxidative system and carbon metabolism of summer maize waterlogged in the field. J. Agron. Crop Sci. 204, 175–184. doi: 10.1371/journal.pone.0161424
Ren, B. Z., Zhang, J. W., Dong, S. T., Zhao, B., Liu, P. (2017). Effect of waterlogging at early period on canopy structure andphotosynthetic characteristics of summer maize. Sci. Agric. Sin. 50, 2093–2103. doi: 10.3864/j.issn.0578-1752.2017.11.015
Ren, B. Z., Zhang, J. W., Li, X., Dong, S. T., Liu, P. (2014). Effect of waterlogging on nutrient uptake and transport of summer maize. J. Plant Nutr. Fert. 20, 11. doi: 10.11674/zwyf.2014.0205
Ren, B. Z., Zhu, Y. L., Li, X., Fan, X., Dong, S. T., Zhao, B., et al. (2015). Effects of waterlogging on photosynthetic characteristics of summer maize under field conditions. Acta Agron. Sin. 4 (1), 329–338. doi: 10.3724/SP.J.1006.2015.00329
Seng, S. S., Wang, Q., Zhang, Y. E., Li, C. H., Liu, T. X., Zhao, L. F., et al. (2012). Effects of exogenous spermidine on physiological regulatory of maize after waterlogging stress. Acta Agron. Sin. 38, 1042–1050. doi: 10.3724/SP.J.1006.2012.01042
Strasser, R. J. (2004). Analysis of the chlorophyll a fluorescence transient (Dordrecht: Springer). doi: 10.1007/978-1-4020-3218-9_12
Strasserf, R. J., Srivastava, A. (1994). Polyphasic chlorophyll a fluorescence transient in plants and cyanobacteria. Photochem. Photobiol. 61, 32–42. doi: 10.1111/j.1751-1097.1995.tb09240.x
Sunil, B., Strasser, R. J., Raghavendra, A. S. (2020). Targets of nitric oxide (NO) during modulation of photosystems in pea mesophyll protoplasts: studies using chlorophyll a fluorescence. Photosynthetica 58, 452–459. doi: 10.32615/ps.2019.183
Tian, L. X., Li, J., Bi, W. S., Zuo, S. Y., Li, L. J., Li, W. L., et al. (2019). Effects of waterlogging stress at different growth stages on the photosynthetic characteristics and grain yield of spring maize (Zea mays l.) under field conditions. Agric. Water Manage 218, 250–258. doi: 10.1016/j.agwat.2019.03.054
Voesenek, L., Bailey-Serres, J. (2015). Flood adaptive traits and processes: an overview. New Phytol. 206, 57–73. doi: 10.1111/nph.13209
Wahid, A., Perveen, M., Gelani, S., Basra, S. M. (2007). Pretreatment of seed with H2O2 improves salt tolerance of wheat seedlings by alleviation of oxidative damage and expression of stress proteins. J. Plant Physiol. 164, 283–294. doi: 10.1016/j.jplph.2006.01.005
Wang, Y. J., Fu, Q. S., Zheng, H., Wen, C. L., Cheng., L., Zhao, B., et al. (2010). Effects of drought stress on growth, photosynthetic physiologica features and stomata characters of cucumber seedlings. J. China Agric. Univ. 15, 12–18.
Wu, B. J., Chow, W. S., Liu, Y. J., Shi, L., Jiang, C. D. (2014). Effects of stomatal development on stomatal conductance and on stomatal limitation of photosynthesis in Syringa oblata and Euonymus japonicus thunb. Plant Sci. 229, 23–31. doi: 10.1016/j.plantsci.2014.08.009
Xing, Y. M., Wan, Y. T., Liu, Y. L., Wang, P. F., Fan, Z., Zhao, Y. X., et al. (2021). Effects of extracts from paecilomyces variotii on chlorophyll fluorescence transient in leaves of maize under waterlogging stress. J. Irrig. Drain. 40, 52–57. doi: 10.13522/j.cnki.ggps.2021108
Xu, D. Q. (1997). Some problems in stomatal limitation analysis of photosynthesis. Plant Physiol. Commun. 33, 241–244. doi: 10.13592/j.cnki.ppj.1997.04.001
Yang, X. Q., Zhang, Z. Q., Liang, Z. S., Shan, Y. (2004). Effects of water stress on chlorophyll fluorescence parameters of different drought resistance winter wheat cultivars seedlings. Acta Bot. Boreal. –Occident. Sin. 24, 5. doi: 10.3321/j.issn:1000-4025.2004.05.010
Yan, R. J., Li, F., Zhang, Q. Q., Zhang, Y. X., Wu, H. X., Hao, L. H., et al. (2021). Effects of irrigation and fertilization on the morphological traits and distribution pattern of stomata of maize leaves under straw returning. Water Sav. Irrig. 000, 71–77. Available at: https://kns.cnki.net/kcms/detail/42.1420.TV.20210909.1900.008.html.
Yu, W. D. (2015). Research on the effect of waterlogging on the growth and yield of summer maize and it's temporal-spatial distribution in Huang huai hai region. PhD dissertation. China Agric. Univ. Beijing, China
Yu, H. Q., Wu, Z. H., Shem, X. Y., Xu, K. Z. (2003). Changes of stomatal density, size and microstructure in maize leaves under water stress. J. Jilin Agric. Univ. 25, 239–242. doi: 10.13327/j.jjlau.2003.03.001
Yu, W. Z., Zhang, X. C., Hu, J., Shao, J. Y., Liu, P., Zhao, B., et al. (2021). Combined effects of shade and waterlogging on yield and photosynthetic characteristics of summer maize. Acta Agron. Sin. 54, 3834–3846. doi: 10.3864/j.issn.0578-1752.2021.18.004
Zhang, D. X. (1996). Effect of hydrogen peroxide on germination of rice and maize seeds. Plant Physiol. J. 32, 115–117. doi: 10.13592/j.cnki.ppj.1996.02.008
Zhang, X. H., Zhang, L., Ma, C., Su, M., Wang, J., Zheng, S., et al. (2022). Exogenous strigolactones alleviate the photosynthetic inhibition and oxidative damage of cucumber seedlings under salt stress. Sci. Hortic. 297, 110962. doi: 10.1016/j.scienta.2022.110962
Zheng, Y. P., Xu, M., Hou, R. X., Shen, R. C., Qiu, S., Ouyang, Z. (2013). Effects of experimental warming on stomatal traits in leaves of maize (Zea mays l.). Ecol. Evol. 3, 3095–3111. doi: 10.1002/ece3.674
Zhou, W. G., Chen, F., Meng, Y. J., Chandrasekaran, U., Luo, X. F., Yang, W. Y., et al. (2020). Plant waterlogging/flooding stress responses: From seed germination to maturation. Plant Physiol. Biochem. 148, 228–236. doi: 10.1016/j.plaphy.2020.01.020
Keywords: summer maize, H2O2 priming, photosynthesis characteristics, waterlogging stress, grain yield
Citation: Wang S, Hu J, Ren B, Liu P, Zhao B and Zhang J (2022) Effects of hydrogen peroxide priming on yield, photosynthetic capacity and chlorophyll fluorescence of waterlogged summer maize. Front. Plant Sci. 13:1042920. doi: 10.3389/fpls.2022.1042920
Received: 13 September 2022; Accepted: 07 October 2022;
Published: 21 October 2022.
Edited by:
Mehdi Rahimi, Graduate University of Advanced Technology, IranReviewed by:
Honghai Luo, Shihezi University, ChinaWei Hu, Nanjing Agricultural University, China
Jia Gao, China Agricultural University, China
Copyright © 2022 Wang, Hu, Ren, Liu, Zhao and Zhang. This is an open-access article distributed under the terms of the Creative Commons Attribution License (CC BY). The use, distribution or reproduction in other forums is permitted, provided the original author(s) and the copyright owner(s) are credited and that the original publication in this journal is cited, in accordance with accepted academic practice. No use, distribution or reproduction is permitted which does not comply with these terms.
*Correspondence: Jiwang Zhang, and6aGFuZ0BzZGF1LmVkdS5jbg==
†These authors have contributed equally to this work