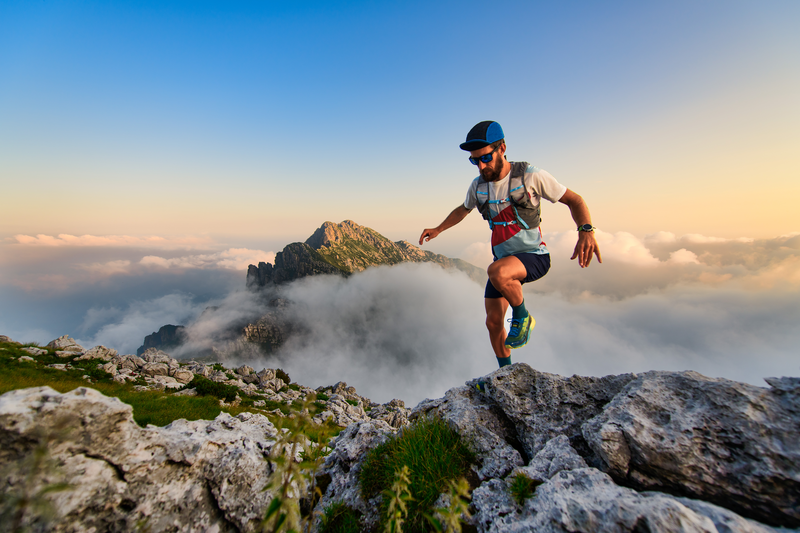
95% of researchers rate our articles as excellent or good
Learn more about the work of our research integrity team to safeguard the quality of each article we publish.
Find out more
ORIGINAL RESEARCH article
Front. Plant Sci. , 08 November 2022
Sec. Plant Symbiotic Interactions
Volume 13 - 2022 | https://doi.org/10.3389/fpls.2022.1040437
This article is part of the Research Topic Organic Amendments: Microbial Communities and their Role in Plant Fitness and Disease Suppression View all 8 articles
As a functional probiotic, Bacillus subtilis can promote crop growth and improve nutrient utilization by various mechanisms, so it has been made into bioorganic fertilizer as a replacement for chemical fertilizer. However, the effects of B. subtilis bioorganic fertilizer application on the yield and quality of commercial crops of Brassica chinensis L., the soil physicochemical properties and the microflora have not been clarified. In this study, pot experiments were conducted using Brassica chinensis L. plants with four fertilization treatments: control without fertilization (CK), chemical fertilizer (CF), organic fertilizer (OF), and bioorganic fertilizer containing B. subtilis (BF). After 30 days of pot experiment, the results showed that BF efficiently improved plant height and biomass (1.20- and 1.93-fold, respectively); as well as significantly increasing soil available potassium and pH value. Using high-throughput sequencing, we examined the bacterial and fungal communities in the soil, and found that their diversity was remarkablely reduced in the BF treatment compared to CK group. A principal coordinate analysis also showed a clear separation of bacterial and fungal communities in the BF and CK groups. After application of B. subtilis bioorganic fertilizer, some beneficial bacteria (such as Bacillus and Ammoniphilus) and fungi (Trichoderma and Mortierella) were enriched. A network analysis indicated that bacteria were the dominant soil microbes and the presence of B. subtilis stimulated the colonization of beneficial microbial communities. In addition, predictive functional profiling demonstrated that the application of bioorganic fertilizer enhanced the function of mineral element metabolism and absorption and increased the relative abundance of saprotrophs. Overall, the application of bioorganic fertilizer effectively changed the soil microflora, improved the soil available potassium and pH value, and boosted the yield of Brassica chinensis L. This work has valuable implications for promoting the safe planting of facility vegetables and the sustainable development of green agriculture.
Brassica chinensis L. (pakchoi) is an annual vegetable in the Cruciferous family (Yang et al., 2010). Due to its short growth cycle, high multiple cropping index and high planting efficiency, Brassica chinensis L. is widely cultivated (Ferreira and Ranal, 1999; Ferreira et al., 2002). However, driven by economic benefits, a high input of chemical fertilizers is employed for its cultivation. Overuse of these synthetic inputs causes adverse effects on the soil ecology and food safety, resulting in a decline of soil quality, nutrient loss and the consequences of an excessive nitrite concentration in crops (Ma et al., 2014; Cai et al., 2016). Therefore, organic, especially the bioorganic fertilizers have recently received much attention as a promising alternative strategy (Yuan et al., 2013; Ling et al., 2014).
Microorganisms, one of the most important and active parts of the soil ecosystem, play a dominant role in promoting soil nutrient cycling and maintaining system stability, and are important for the continuous functioning of soil microecological status (Emery et al., 2019; Nazaries et al., 2021). Additionally, the soil microbial community can provide essential nutrients for crop growth and stimulate crop development by various mechanisms (Yang et al., 2019b). Bioorganic fertilizer is a collection of organic fertilizers and probiotic microorganisms that can activate various microorganisms in the soil. And it is increasingly important in promoting crop production, restoring soil fertility, and inhibiting soil diseases (Huang et al., 2014; Schoebitz et al., 2014; Liu et al., 2016).
Among many bioorganic microbial fertilizers, Bacillus subtilis, which is widely distributed in soil and decaying organic matter, is a representative type. It has been shown in previous studies that B. subtilis has good regulatory effects for promoting crop growth, improving soil quality and the crop microenvironment, controlling soil-borne diseases and remediating farmland pollution (Zhao et al., 2013; Wu et al., 2016). Meanwhile, due to its excellent stress tolerance, short culture period and convenient application, B. subtilis has increasingly become dominant component in bioorganic fertilizer (Sun et al., 2020). Zhao et al. (2019) reported that the application of bioorganic fertilizer including Bacillus could reshape the soil microbial community and promote pepper growth. It was shown that the application of Bacillus bioorganic fertilizer could be a sustainable pathway to improve soil nutrient utilization as well as increase the yield and quality of lettuce (Jin et al., 2022). In addition, Bacillus bioorganic fertilizer has recently been applied in the cultivation of economic crops, and the results show that it could stimulate microbial activity, inhibit banana Fusarium wilt disease and enhance the stress resistance of sorghum (Wu et al., 2019; Wang et al., 2022). However, previous studies only investigated the effects of bioorganic fertilizers on soil physicochemical properties, crop growth and the microbial community composition, without further exploring microbial network relationships and microfloral functions. Moreover, the effects of Bacillus bioorganic fertilizer on the Brassica chinensis L. have not been well characterized.
Base on this, we hypothesized that B. subtilis bioorganic fertilizer could promote the growth of Brassica chinensis L. and improve soil microbial profiles (biodiversity, function and network interactons). Therefore, a novel bioorganic fertilizer was developed by fermenting mature compost with B. subtilis produced by the Shenzhen Batian Ecological Technique Co., Ltd; and pots experiments were performed to investigate the effects of bioorganic fertilizer on plants and soil microbial features. The specific objectives of this study were to (1) evaluate the direct effect of B. subtilis bioorganic fertilizer on Brassica chinensis L. cultivation; (2) explore the potential impact of this fertilizer on the soil characteristics; and (3) determine the alterations of soil microbial community, co-occurrence relationship and functions caused by this type of fertilizer. By revealing the mode of action of bioorganic fertilizers, this study sought to provide the necessary understanding required for the more efficient and informed development of soil microbiome manipulation strategies involving biologically enhanced organic fertilizers.
Greenhouse experiments were carried out in the Shenzhen Batian Ecological Fertilizer Research Center (22° 46′ 6.13′′ N, 113° 48′ 32.70′′ E) in Shenzhen City, Guangdong Province, China from 10 December 2021 to 09 January 2022. The soil was lateritic soil with a pH of 6.30. Soil organic matter (OM), total nitrogen (TN), total soil phosphorus (TP) and potassium (TK) were 34.22, 1.83, 1.97 and 17.80 g/kg soil, respectively. Alkaline hydrolysis nitrogen (AHN), available phosphorus (AP) and available potassium (AK) were 112.22, 164.12 and 200.19 mg/kg soil, respectively. Organic fertilizer and the newly developed bioorganic fertilizer were produced by the Shenzhen Batian Ecological Technique Co., Ltd. (China). The organic fertilizer composted with chicken manure and rice husks with a 1:1 weight ratio. On the 14th day, the composting was completed and granulated to make organic fertilizer containing 42.44% of OM, 1.30% of N, 0.40% of P2O5 and 4.14% of K2O. The bioorganic fertilizer was a secondary fermentation based on organic fertilizer. Bacillus subtilis AMMS-012 was added to the decomposed organic material at a rate of 5×1010 spores per g for further fermentation. On the 5th day, granulation was carried out and air-dried at low temperature to make bioorganic fertilizer, and the number of colonies was detected as 7.5×108 spores g-1. Commerical chemical fertilizers, including urea, monoammonium phosphate and potassium chloride purchased from Yunnan Yuntianhua Co., Ltd., China.
The soil was exposed to the sunlight for 3 days before the experiment and large particles were removed with a 2 mm sieve. The Brassica chinensis L. seeds were placed in the vermiculite matrix to sow and raise seedlings. After 18 days, the seedlings with consistent growth and good health were selected for transplanting. Four fertilization treatments were applied as follows: (1) BF, bioorganic fertilizer; (2) OF, organic fertilizer; (3) CF, chemical fertilizer; and (4) CK, control without fertilization. The nutrient (N, P, and K) supply among the experimental fertilization treatments was equalized by the chemical fertilizers. BF was set as the nutrient standard by applying 12,000 kg·ha-1 of bioorganic fertilizer to the field (Kang et al., 2022). Specifically, BF was applied with 5.0 g bioorganic fertilizer per kilogram of soil in the pots, and the nutrient supply of the other treatments was made equal to it. Bioorganic and organic fertilizers were applied as the base fertilizer once; and for the chemical fertilizer, one-third of the total amount of chemical fertilizer was applied as a basal fertilizer. The remainder of the chemical fertilizer was applied 10 or 20 days after the seedlings were planted. Six pots (replicates) were set up for each treatment, and each pot contained 4 kg soil and three Brassica chinensis L. seedlings. The pots were randomly arranged in the greenhouse with the temperature maintained at 25 ± 1°C under natural light. Conventional operations such as watering, scarifying and disinsection were applied equally when needed. Plants were grown for 30 days and the growth parameters (morphological characteristics) were measured every 5 days, and plant and soil samples were collected on the 30th day for quality and diversity index determination.
The soil pH value was determined with a glass electrode using a soil-to-water ratio of 1:2.5 (w/v). OM was determined by a K2Cr2O7 oxidation-reduction titration method and the Kjeldahl method was used for TN estimation (Guimaraes et al., 2013). TP and TK were digested by HF-HClO4 and determined by molybdenum-blue colorimetry and flame photometry, respectively (Shen et al., 2008). AHN in soil was determined based on the transformation of hydrolyzed nitrogen into ammonia nitrogen with sodium hydroxide (Li et al., 2013). AP in soil was extracted with sodium bicarbonate and determined using the molybdenum blue method (Hedley et al., 1982). AK in soil was extracted with ammonium acetate and determined with inductively coupled plasma spectrophotometry (ICP-9000, Shimadzu, Japan) (Fukuda et al., 2017).
Thirty-six Brassica chinensis L. plants were randomly selected from each treatment plot, and the height, crown width and leaf width of the plants were measured with a tape measure (Liu et al., 2014; Amoah et al., 2017). A handheld, non-destructive SPAD chlorophyll meter (SPAD-502, Konica Minolta, Japan) was used to measure the chlorophyll concentration in the plant leaves (Li et al., 2022c). The leaves of each plant were numbered from the bottom to the top of the stem (Alemayehu et al., 2020). Plant samples (shoot and root) were washed and laid on paper towels to dry, and the fresh weight was recorded. Plant samples were subsequently dried at 70°C for 120 hours before the dry weight was recorded (Cai et al., 2015).
The soluble sugar concentration was measured by anthrone colorimetry (Ibrahim et al., 2013). The soluble protein concentration was determined spectrophotometrically by measuring the absorbance at 595 nm with bovine serum albumin as the standard (Bradford, 1976). The nitrate determination used the salicylic acid colorimetric method (Cataldo et al., 1975).
DNA from all samples was extracted and purified (the details are described in the Additional file). Two specific primers were used to amplify the bacterial 16S rRNA gene and the fungal ITS1 region (Schoch et al., 2012; Mori et al., 2014). Sequencing was performed on an Illumina Miseq platform (Majorbio Bio-Pharm Technology Co., Ltd., Shanghai, China). Sequences were comparedagainst the Silva (SSU123) 16S rRNA Database and the fungal (ITS) Unite Database to obtain the species annotation information (Li et al., 2022c). Sequence data associated with this project have been deposited in the NCBI Short Read Archive database (Accession Numbers: PRJNA862974 and PRJNA863750).
Network analysis was performed based on OTUs to explore the relationships between the soil microbial taxa as described in Additional file. Data set was calculated by Spearman correlation matrix and adjusted for Benjamini-Hochberg’s false discovery rate, and thenetwork visualization as well as topological parameters were analyzed using Gephi 0.9.5 (https://gephi.org/) (Benjamini and Hochberg, 1995; Li et al., 2019).
Statistical analysis of the soil physicochemical properties and plant agronomic traits was carried out by applying a one-way analysis of variance (ANOVA) and the new multiple range method. All experimental parameters were measured at least in triplicate, and the results were expressed as the mean ± standard deviation. Difference analyses were conducted with the SPSS statistical software package, version 22.0 (IBM, New York, USA), with a P value ≤ 0.05 as the standard.
To compare the relative levels of OTU diversity across all samples, a rarefaction curve was formed using the Mothur software (Schloss et al., 2009). Alpha diversity, including the Chao 1 and Shannon indexes, were calculated using QIIME (version 1.9.1) (Zhang et al., 2022). The relative abundance of microbial phylum and genus were defined as the number of reads of that phylum and genus as a percentage of the number of all reads in a sample (Yao et al., 2019). To compare bacterial and fungal community structures among all soil samples, a principal coordinate analysis (PCoA) was set up based on the Bray–Curtis distance metric (Yang et al., 2022). Theigraph and psych packages in R (version 4.2.0) were used to visualize the results (Kang et al., 2022). An ANOVA was used to determine the statistical significance of the differences between species and treatment groups, and the FDR (False Discovery Rate) multiple test was used for correction. A linear discriminant analysis (LDA) effect size (LEfSe) method was performed to find biomarkers (LDA score ≥ 3.5) (Li et al., 2022c).
The function of bacterial communities was predicted using the PICRUSt software program according to the Kyoto Encyclopedia of Gene and Genomes (KEGG) catalog (Langille et al., 2013), and the function of fungal communities was predicted using the FUNGuild software (Nguyen et al., 2016). The results were graphed primarily using Origin 2022 (OriginLab, USA) and Adobe Illustrator CC2018 (Adobe Systems Inc., USA) (Li et al., 2022c). In addition, a structural equation models (SEMs) analysis was conducted by SPSSAU using an online tool (https://spssau.com) (Li et al., 2022a).
The overall trend for plant biomass was that fertilizer treatments were significantly higher than CK, especially BF (Table S1, Figure S1). During the experimental period, the shoot fresh weight, root fresh weight, total fresh weight, and total dry weight were 83.03 ± 10.89, 2.27 ± 0.29, 85.29 ± 11.07, and 4.26 ± 0.43 (g/plant), respectively; which is 1.81–1.93-fold higher than CK (P ≤ 0.05). It was noteworthy that no significant difference was evident between CF and OF, but both were remarkablely lower than the BF group.
For other plant growth factors, compared with CK, all treatments showed greatly increased plant height, crown width and the third leaf width, especially BF (Table S2, Figure S1). Compared with the other three treatments, BF showed dramatically increased plant height, by 5.43%–20.00% on the 30th day. For the leaf number, significant differences were seen among the three test groups and CK in the early stages (i.e., 5 d and 10 d), but the difference in the three test groups was not obvious. From 15 d to 30 d, the leaf number in BF group increased significantly compared with all other groups (CF, OF and CK) (Table S3). Unlike the leaf number, the leaf SPAD value was not statistically different among BF and the other groups in the early stages. A significant difference was observed at 30 d (Table S3).
The soluble sugar concentration of BF and OF was 0.22% and 0.12%, respectively, which was significantly higher than CK (Figure 1A). CK showed the lowest soluble protein concentration, whereas BF was increased by 40.79% and 62.12% compared with CF and OF, respectively (Figure 1B). Unlike the soluble sugar/protein, the nitrate concentration showed a different tendency. BF showed the lowest nitrate with a 30.34% and 23.27% decrease compared with CF and CK, respectively (Figure 1C). These results indicated that application of organic fertilizer effectively improved the quality of Brassica chinensis L.
Figure 1 Effects of different fertilizer treatments on the Brassica chinensis L. soluble sugar concentration (A), soluble protein concentration (B) and nitrate concentration (C). CK is the untreated control; CF is chemical fertilizer; OF is organic fertilizer; BF is bioorganic fertilizer; FW is fresh weight. Means (N = 3) within the same histogram followed by the same letter are not statistically different (P = 0.05) according to Duncan’s new Multiple-Range test. The different small lettles (a, b, c, and d) indicated the significant difference among the different groups at P<0.05 level.
Compared with CK, all treatments except CF showed significantly increased alkaline hydrolysis nitrogen (AHN), available potassium (AK) and organic matter (OM). Especially in, BF group, which effectively increased the AK concentration by 62.21% (Table S4). Additionally, available phosphorous (AP) was dramatically increased (8.69%–14.87%) after fertilization, and the largest increase occurred in BF. At the same time, both BF and OF also had improved soil acid-base status indicated by a higher pH value. The pH value of CK was 6.22 ± 0.01, whereas the value was 6.60 ± 0.08 in BF.
A correlation analysis showed that plant weight, the leaf SPAD value, and the soluble sugar and protein concentration were positively correlated with the environmental factors, whereas the nitrate concentration was negatively correlated with the environmental factors (Table S5). Plant fresh weight was positively correlated with AHN, AP, AK and OM (P ≤ 0.05), and SPAD was positively correlated with AP, AK and pH (P ≤ 0.05). The nitrate level was negatively correlated with AK, OM and pH (P ≤ 0.05). The soluble sugar concentration was positively correlated with all environmental factors values (P ≤ 0.01); and the soluble protein concentration was positively correlated with AHN, AP and AK concentrations (P ≤ 0.05).
After optimizing the original sequence based on a 97% similarity, 37,014 16S rRNA and 58,153 ITS sequences were retained from all samples; a total of 4,673 bacterial OTUs and 1,549 fungal OTUs were obtained. The Shannon and Chao1 indices respectively represent the diversity and richness of microbial communities.
Compared with BF, more OTUs were observed in CF and CK for bacteria and fungi, and CK had the highest value of all treatments (Table S6). In BF, the Chao1 richness of bacteria decreased by 3.21% and 7.01% compared with CF and CK. However, in the fungal biosphere, no significant differences in the Chao1 index occurred among the four treatments. For the Shannon index, CK showed the highest value for both bacteria and fungi. Overall, CK and CF had higher microbial α-diversity compared with BF and OF.
For β-diversity, The differences in community composition of the four treatments were evaluated by using a PCoA based on a Bray-Curtis distance matrix. Figure 2 shows that application of organic fertilizer accounts for the differences in the composition of the soil microbial community. Both bacterial and fungal cluster into two distinct groups representing samples taken from four treatments. The Bray–Curtis distances show that BF was separate from CF and CK along the first component (PCoA1) both for bacteria and fungi (PERMANOVA, F = 6.6612, P = 0.001, bacteria; F = 5.30167, P = 0.001, fungi). The contribution rates of PCoA1 and PCoA2 to the differences in species composition between the treatments were 42.72% and 9.54% (bacteria), and 38.56%, 17.89% (fungi), respectively.
Figure 2 Comparison of the bacterial (A) and fungal (B) communities in soils under the different fertilizer treatments based on a principal coordinates analysis of Bray-Curtis distances. CK is untreated control; CF is chemical fertilizer; OF is organic fertilizer; BF is bioorganic fertilizer.
For the bacteria, Proteobacteria, Actinobacteria, Firmicutes, Chloroflexi and Patescibacteria were the top five abundant phyla (Figure S2A). At the class level, Bacilli, Gammaproteobacteria, Actinobacteria, Alphaproteobacteria, Saccharimonadia, Thermoleophilia and Chloroflexia are the main species (Figure 3A). For the fungi, Ascomycota, followed by Olpidiomycota, Basidiomycota, Mortierellomycota and Chytridiomycota were the abundant phyla (Figure S2B). The class level was dominated by Sordariomycetes, Eurotiomycetes, Olpidiomycetes, Mortierellomycetes and Tremellomycetes (Figure 3B).
Figure 3 Bubble chart of bacterial (A) and fungal (B) classes for different fertilizer treatments. CK is untreated control; CF is chemical fertilizer; OF is organic fertilizer; BF is bioorganic fertilizer. The circular areas represent the average relative abundance (RA) across the six replicate libraries for soil samples collected from each treatment.
At the genus level, Bacillus was the dominant genus in the bacterial community, both OF and BF dramatically (P ≤ 0.001) increased the relative abundance of Bacillus by 129.18% and 278.01% (Figure 4A). Addition of bioorganic fertilizer in BF also significantly increased the relative abundance of Ammoniphilus and norank_f_Chitinophagaceae. Among the declining species, BF significantly decreased (P ≤ 0.01) the relative abundance of Chujaibacter and Streptomyces by 34.53% and 56.65%, respectively.
Figure 4 Differences in the composition of soil bacteria (A) and fungi (B) at the genus level in response to different fertilizer treatments. CK is untreated control; CF is chemical fertilizer; OF is organic fertilizer; BF is bioorganic fertilizer. The number of asterisks indicates significant differences between treatments according to a one-way ANOVA and FDR (False Discovery Rate) adjustment: * 0.01 < P ≤ 0.05; ** 0.001 < P ≤ 0.01; *** P ≤ 0.001.
Trichoderma was the dominant genus in the fungal community. All treatments increased the relative abundance of Trichoderma by 7.13%–31.84% (Figure 4B); while significantly reduced the relative abundance of Aspergillus. The addition of bioorganic fertilizer in BF group greatly enriched the relative abundance of Mortierella, Trichosporon and Neocosmospora, and decreased the relative abundance of Trechispora.
A LefSe analysis showed that at the bacterial genus level, 6, 2, 2 and 4 biomarkers were found in CK, CF, OF and BF, respectively (Figure S3A). At the fungal genus level, 8, 4, 2 and 3 biomarkers were found in CK, CF, OF and BF, respectively (Figure S3B).
The correlation analysis at the genus level showed that bacteria were more closely correlated with the soil parameters and agronomic traits than the fungi were (Figure 5). In the bacterial community, Bacillus and Ammoniphilus were positively correlated with AK, OM, pH, FW, SSC and SPC (P ≤ 0.01); whereas negatively correlated with NC (P ≤ 0.001) (Figure 5A). Chujaibacter, Mizugakiibacter and Streptomyces were negatively correlated with FW and AK (P ≤ 0.01). Paenibacillus, Streptomyces and Nitrospira were negatively correlated with SSC (P ≤ 0.01) and Mizugakiibacter, Tumebacillus and Nitrospira were negatively correlated with OM (P ≤ 0.01).
Figure 5 Heatmap analysis of the correlation between the species composition of soil bacteria (A) and fungi (B) at the genus level and environmental factors and agronomic traits. CK is untreated control; CF is chemical fertilizer; OF is organic fertilizer; BF is bioorganic fertilizer; FW is fresh weight; SPAD is leaf SPAD value; NC is nitrate concentration; SSC is soluble sugar concentration; SPC is soluble protein concentration; AHN is alkaline hydrolysis nitrogen; AP is available phosphorus; AK is available potassium; OM is organic matter. The Spearman method was used for correlation analysis. The legend on the right is the color interval for the different R values. The number of asterisks indicates the degree of correlation: *0.01 < P ≤ 0.05; **0.001 < P ≤ 0.01; *** P ≤ 0.001.
In the fungal community, Trechispora, Aspergillus and Latorua were negatively correlated with AK, OM, pH and SSC; whereas Trichosporon and Saitozyma were positively correlated with these factors (P ≤ 0.001) (Figure 5B). Neocosmospora was positively correlated with AK, FW and SSC (P ≤ 0.01); and negatively correlated with NC (P ≤ 0.001). Talaromyces and Chrysosporium were negatively correlated with AK and OM (P ≤ 0.001).
The co-occurrence networks of microbial communities were constructed for bacteria and fungi to show interactions among genera based on strong and significant correlations (Spearman’s correlation coefficient |r| > 0.6, P < 0.05, FDR-BH tests) (Figure 6). Topological features of the global co-occurrence networks are listed in Table S7. A network analysis showed that the co-occurrence network was more complex in BF compared to the other groups, which consisted of 189 nodes and 1541 edges. The proportion of positive correlation accounts for 50.75%–57.82% in the four networks. The addition of organic amendments (i.e., in OF and BF) increased the network density and the average degree of the microbial networks. Additionally, the average path length decreased from 3.093 to 2.936 following the bioorganic fertilization of the soil.
Figure 6 Network plots of bacterial and fungal communities in CK (A), OF (B), CF (C) and BF (D), CK is untreated control; CF is chemical fertilizer; OF is organic fertilizer; BF is bioorganic fertilizer. Blue nodes indicate bacteria; gray nodes indicate fungi; red lines between nodes indicate positive interaction; and green lines indicate negative interaction.
Regarding microbial function, compared with the other three treatments, microbial activity in sulfur metabolism, phosphonate and phosphinate metabolism were significantly higher in BF (P ≤ 0.01), while other metabolic pathways, such as biofilm formation and plant-pathogen interaction were down-regulated (Figure S4). Significant differences were also seen in carbon fixation, mineral absorption and metabolism among the four treatments. Seven trophic modes of fungi were identified among the different fertilization treatments. They were saprotroph (51.14%–64.45%), pathotroph-saprotroph-symbiotroph (8.97%–15.35%), pathotroph (5.20%–8.21%), saprotroph-symbiotroph (4.00%–8.75%), pathotroph-saprotroph (1.71%–3.29%), symbiotroph (0.02%–0.06%), pathotroph-symbiotroph (0.02%–0.04%) and unassigned (10.88%–20.53%), respectively. (Figure S5A). Saprotroph was the dominant trophic mode and the value for BF was larger than for the other treatments. In addition, the pathotroph mode of BF was significantly lower compared with CK. The dominant groups of functional soil fungi were undefined saprotroph, endophyte-litter saprotroph-soil saprotroph-undefined Saprotroph, animal pathogen-dung saprotroph-endophyte-epiphyte-plant saprotroph-wood saprotroph and wood saprotroph, and the average abundance of all treatments accounted for 49.95%, 7.21%, 5.94% and 4.61%, respectively (Figure S5B). The abundance of unclassified saprotroph in BF increased by 19.54% compared with CK, and the abundance of animal pathogen-dung saprotroph-endophyte-epiphyte-plant saprotroph-wood saprotroph decreased by 35.71%. In addition, BF showed a significantly increased abundance of wood saprotroph over the other treatments.
The effects of microbial diversity, microbial function and environmental factors on plant development were evaluated using the structural equation model (SEM). The findings indicated that the plant growth was positively co-associated with the bacterial diversity and fungal diversity (Figure 7). Both the environmental factors and microbial diversity positively correlated with the microbial function; and the microbial function was promoted plant growth. Moreover, the environmental factors enhanced bacterial diversity, which improved the growth of Brassica chinensis L., indirectly. Taken together, in the analyzed parameters, plant growth was co-regulated bymicrobial composition, microbial metabolic potential and environmental factors. Among them, bacterial function, bacterial diversity, and fungal diversity showed most obvious effects on the growth of Brassica chinensis L. with R2 values ranging from 0.043 to 0.719, and P ≤ 0.05 or 0.01. These results showing that soil microbial profile was an important factor affecting crop growth.
Figure 7 Structural equation modeling (SEM) showing the linkage among environmental factors, species diversity, species function, and plant growth. Dotted arrows indicate non-significant paths (P > 0.05). Red and blue arrows indicate positive and negative relationships, respectively. The path widths are scaled proportionally to the path coefficient. *0.01 < P ≤ 0.05, **P < 0.01.
This study showed that soil physicochemical properties changed significantly after bioorganic fertilizer application. Specifically, both pH value and AK level were significantly improved in BF group compared with the control group (Table S4). Previous studies revealed that increase in soil pH index may be ascribed to the decomposition of organic matter, and the application of bioorganic fertilizer introduced a large amount of organic matter and microorganisms, thus the decomposition of organic matter can be accelerated by microbes to alleviate soil acidification (Hu et al., 2018; Yang et al., 2019a). The efficient soil microorganisms influence the availability of minerals in soil and play a major role in ion cycling and soil fertility. Abou-el-Seoud and Abdel-Megeed (2012) used B. subtilis with B. mucilaginosus to develop efficient microbial consortium, which helps to enhance the potassium availability in agricultural soils. In addition, many researchers have reported that a wide range of rhizosphere microorganisms can act as K solubilizers, including B. circulans, B. edaphicus and B. amyloliquefaciens (Sheng, 2005; El-Hadad et al., 2011). They solubilize the insoluble potassium to soluble forms of potassium mainly by chelation, exchange reactions, complexolysis, and production of organic acids. In this work, the correlation analysis shows that soil AHN, AP, AK, OM and pH were all characteristic factors affecting Brassica chinensis L. growth. Among these parameters, AK has the highest factor loadings and the biggest contribution for plant growth (Tables S1, S2, 3, Figure S1). Hency, our results show that bioorganic fertilizer increased the availability of potassium and provides necessary element for plant growth. Meanwhile, this study also revealed that the addition of B. subtilis bioorganic fertilizer significantly reduced the nitrate concentration in the crop, probably because the soil microbial amendment reduced the nitrate concentration by increasing the nitrate reductase activity, thereby improving the safety and quality of vegetables (Chatterjee et al., 2012; Harindintwali et al., 2021). Previous studies have confirmed that additional bacterial fertilizers can reduce nitrate concentration in vegetables, such as lettuce and tomato (Brunetti et al., 2019; Jiang et al., 2019; Jin et al., 2022). Jin et al. (2022) pointed out the application of B. subtilis bioorganic fertilizer enriched organic matter concentration in the plant-body, which increased the quality of lettuce. Similar results were observed in the present study, where soluble sugar and protein levels were increased to varying degrees in the BF group. This may be related to the effective promotion of nutrient metabolism coordination and the balance of organic fertilizer, so as to ensure a higher yield and better quality of crops (Brunetti et al., 2019).
Base on plant growth or quality is closely related to soil fertility and microecological characteristics (Wu et al., 2021), an analysis of the soil microbial community of Brassica chinensis L. was performed. For the biodiversity, it was found that B. subtilis bioorganic fertilizer could significantly change rhizosphere microbial diversity. Both the diversity and richness of bacteria and fungi were significantly lower for BF compared to CK (Table S6). A possible reason is that the bacterial fertilizer changed the composition of the soil organic matter and enriched the relatively dominant species. Previously, Jiang et al. (2019) pointed out that bioorganic fertilizer is rich in organic components and exogenous strains that can modify the habitat of soil microorganisms. Yang et al. (2022) subsequently showed that the B. subtilis is a typical plant growth-promoting rhizobacteria (PGPR), which can dominantly colonize the soil, decreasing the biodiversity of soil species. At the same time, Wang et al. (2017) pointed out that bioorganic fertilizer is a source of organic matter which promotes the growth of native microbes in soil and decrease the biodiversity. The above-mentioned studies provide evidence for our explanation.
For the microbial communities, the phylum level results showed that the abundance of Firmicutes was higher and Acidobacteriota was lower after the bioorganic fertilizer addition. Previous study reported that Firmicutes are k-strategy species often found in nutrient-rich habitats and can produce antibacterial substances against harmful bacteria and promote host growth (Li et al., 2022c). Unlike the Firmicutes, Acidobacteriota are an r-strategy species and prefer to inhabit an oligotrophic environment, so they show a relatively higher abundance in CK (Liang et al., 2020). In the fungi, compared with the control, bioorganic fertilizer application increased the abundance of Basidiomycota and Mortierellomycota, both of which are key fungal members engaging in nutrient conversion and material decomposition (Detheridge et al., 2016; Kumar et al., 2022). In addition, the PCoA analysis revealed that significant clustering in bacterial and fungal communities in the various treatment groups, which indicated that the bioorganic fertilizer greatly affected the microbial community (Wang et al., 2022).
At the genus level, as expected, it was found that Bacillus was highly enriched in the bioorganic fertilizer treatments. And the Spearman correlation test showed that the relative abundance of Bacillus exhibited a strong positive correlation with soil environmental factors and plant physiological parameters, which might be related to the facility of Bacillus for humification and the mineralization of nutrients (such as C and N), the inhibition of pathogens, and the induction of host resistance (Wu et al., 2019; Lebedev et al., 2021; Li et al., 2022c). Similar to Bacillus, it was also observed that norank_f_Chitinophagaceae was remarkably increased in BF compared with CK. This species degrades chitin and cellulose by secreting β-glucosidase, and inhibits harmful fungi and promotes plant growth (Xu et al., 2021). In this study, the application of bioorganic fertilizer effectively enhanced the yield of Brassica chinensis L., which may be related to the high relatively abundance of norank_f_Chitinophagaceae. Furthermore, Ammoniphilus was significantly enriched in the organic fertilizer treatments and had a strong positive correlation with crop physiological indicators and soil nutrient parameters, probably related to its ability to secrete organic matter hydrolases to accelerate substance degradation and promote nutrient element metabolism (Otto et al., 2013).
In the fungal biosphere, the Trichoderma, Mortierella, Trichosporon and Saitozyma were greatly increased in BF. Trichoderma is a typical activity member, which colonizes the rhizosphere of crops and increases the surface area of the root system to improve the nutrient absorption capacity of crops (Cai et al., 2017). Additionally, it has been found that Trichoderma inhibits the growth of other pathogenic microorganisms by secreting antibiotics and hydrolases, and releases slow-effect nutrients in the soil (Zhang et al., 2016). In the present study, the organic fertilizer treatment groups BF and OF had a high soil nutrient concentration and excellent crop growth parameters, which may be related to the significant enrichment of the abundance of Trichoderma. Similarly, Mortierella was significantly enriched in the organic fertilizer treatment and positively correlated with crop fresh weight, probably related to its ability to improve nutrient availability and protect crops from pathogens (Ozimek and Hanaka, 2021).
As for Trichosporon and Saitozyma, the relative abundance of both was increased in the organic/bioorganic fertilizer groups, and was significantly positively correlated with the soil organic matter, available potassium, and appropriate pH, which may be related to their ability to accelerate cellulose degradation (Stursova et al., 2012; Aliyu et al., 2021). It is also noteworthy that the abundance of Aspergillus decreased significantly after the application of bioorganic fertilizers, and the correlation test results showed that Aspergillus was negatively correlated with crop quality indicators. This indicates the plants are less likely to be damaged by toxins after applying bioorganic fertilizers, because Aspergillus is a common toxin producer fungus often infecting cash crops such as peanuts and corn (Senghor et al., 2020). The above-mentioned results imply that the promotion of beneficial fungi and the inhibition of harmful fungi by organic fertilizers promote plant growth.
The co-occurence analysis indicated the bacteria nodes occupy three-quarters of the network, with a higher proportion of positive than negative correlations, implying bacterial dominance in the microflora and species niches dominated by similarity (Zhang et al., 2022). In this study, the network topological parameters, including number of nodes and edges, the average degree, the network density and the average clustering coefficient of BF were much greater than the other groups, indicating that BF evidenced a higher degree of cooperation and communication (Li et al., 2022a). In addition, the high connectivity implies a great network complexity, which is important for the ecological buffer capability and microbial homeostasis (Kang et al., 2022). It was observed that the complexity of the co-occurrence networks of bacteria and fungi in BF was increased, consistent with previous studies (Gu et al., 2019; Price et al., 2021). The results of our study showed that BF showed a shorter average network path length, suggesting that the application of bioorganic fertilizer improved the efficiency of the co-occurrence network to quickly respond to environmental changes (Kang et al., 2021; Zhu et al., 2022).
For the functional profiles of the microflora, the soil bacterial and fungal ecological functions of the different treatment groups of Brassica chinensis L. were predicted by the PICRUSt2 and FUNGuild methods, respectively. The KEGG results showed that exposure of B. subtilis bioorganic fertilizer significantly enhanced the metabolism and absorption pathways of mineral elements and sulfur/phosphonate/phosphinate metabolism, which was mainly because the addition of B. subtilis stimulated the proliferation of other beneficial microorganisms (including bacteria and fungi). Previous studies also found that the addition of organic fertilizers significantly improved the material metabolism pathway and played an important role in nutrient cycling or decomposition, which was consistent with the predictions of this study (Cai et al., 2017; Liao et al., 2019; Li et al., 2022b). Su et al. (2022) further pointed out that organic fertilizer application improved the soil structure and stimulates beneficial microorganisms, reducing the relative abundance of pathogenic fungi. However, it should be noted that the present results are based on prediction tools, and the actual molecular functions have yet to be confirmed by subsequent genomics or transcriptomics in the future. At the same time, genetic engineering and genome editing for improving nutrients provide efficiency in microbes are needs to be strengthened in the future.
The present study revealed that B. subtilis bioorganic fertilizer can maintain the acid base balance of soil and effectively improve the plant nutritional quality by regulate the pH value and soluble matters content (sugar, protein and nitrate). Meanwhile, the soil microorganisms profiles were improved under the bioorganic fertilizer. The biofertilizer decreased the α diversity and increased soil beneficial microorganisms proliferation. Network analyses revealed closely correlations between bacteria/fungi and soil environmental parameters and crop agronomic traits. Functional profiling revealed that the application of biofertilizer promoted an increase of microbial functional pathways, such as mineral element metabolism and saprotrophy. The SEM results further supplied the evidence that crop growth was co-controlled by the microbial communities and related functions. As a whole, bioorganic fertilizers can effectively improve soil available potassium and pH value as well as microbial activities, and promote B. chinensis L. growth. This study provides a scientific basis for establishing an environmental friendly fertilization technology for green agriculture.
The datasets presented in this study can be found in online repositories. The names of the repository/repositories and accession number(s) can be found in the article/Supplementary Material.
TW: writing-original draft preparation, software, visualization, writing-reviewing and editing; KC: conceptualization, writing-review and process suggestion; XH: project administration, investigation and supervision; PM: supervision and material assistance; ZC: formal analysis and methodology; ZW: experimental design, guidance and funding acquisition; JZ: writing-reviewing and editing, project administration, and funding acquisition. In addition, all authors contributed to the article and approved the submitted version.
This work was supported by the NSFC (41976126), the S&T Projects of Shenzhen Science and Technology Innovation Committee (KCXFZ20201221173211033, RCJC20200714114433069, JCYJ20200109142818589 and WDZC20200819173345002), as well as the Project of Shenzhen Municipal Bureau of Planning and Natural Resources (Grant No. [2021]735-927).
We are grateful to Shenzhen Batian Ecological Fertilizer Research Center for providing the experimental assistance.
Authors XH, PM and ZW are employed by Shenzhen Batian Ecological Engineering Co., Ltd.
The remaining authors declare that the research was conducted in the absence of any commercial or financial relationships that could be construed as a potential conflict of interest.
All claims expressed in this article are solely those of the authors and do not necessarily represent those of their affiliated organizations, or those of the publisher, the editors and the reviewers. Any product that may be evaluated in this article, or claim that may be made by its manufacturer, is not guaranteed or endorsed by the publisher.
The Supplementary Material for this article can be found online at: https://www.frontiersin.org/articles/10.3389/fpls.2022.1040437/full#supplementary-material
Abou-el-Seoud, I. I., Abdel-Megeed, A. (2012). Impact of rock materials and biofertilizations on p and K availability for maize (Zea maize) under calcareous soil conditions. Saudi J. Biol. Sci. 19 (1), 55–63. doi: 10.1016/j.sjbs.2011.09.001
Alemayehu, Y. A., Demoz, A. A., Degefu, M. A., Gebreeyessus, G. D., Demessie, S. F. (2020). Effect of human urine application on cabbage production and soil characteristics. J. Water Sanit. Hyg. Dev. 10 (2), 262–275. doi: 10.2166/washdev.2020.136
Aliyu, H., Gorte, O., Neumann, A., Ochsenreither, K. (2021). Global transcriptome profile of the oleaginous yeast Saitozyma podzolica DSM 27192 cultivated in glucose and xylose. J. Fungi 7 (9), 758–779. doi: 10.3390/jof7090758
Amoah, P., Adamtey, N., Cofie, O. (2017). Effect of urine, poultry manure, and dewatered faecal sludge on agronomic characteristics of cabbage in Accra, Ghana. Resources-Basel 6 (2), 19–33. doi: 10.3390/resources6020019
Benjamini, Y., Hochberg, Y. (1995). Controlling the false discovery rate - a practical and powerful approach to multiple testing. J. R. Stat. Soc. Ser. B-Stat. Method. 57 (1), 289–300. doi: 10.1111/j.2517-6161.1995.tb02031.x
Bradford, M. M. (1976). Rapid and sensitive method for quantitation of microgram quantities of protein utilizing principle of protein-dye binding. Anal. Biochem. 72 (1-2), 248–254. doi: 10.1006/abio.1976.9999
Brunetti, G., Traversa, A., De Mastro, F., Cocozza, C. (2019). Short term effects of synergistic inorganic and organic fertilization on soil properties and yield and quality of plum tomato. Sci. Hortic. 252, 342–347. doi: 10.1016/j.scienta.2019.04.002
Cai, F., Chen, W., Wei, Z., Pang, G., Li, R., Ran, W., et al. (2015). Colonization of Trichoderma harzianum strain SQR-T037 on tomato roots and its relationship to plant growth, nutrient availability and soil microflora. Plant Soil 388 (1-2), 337–350. doi: 10.1007/s11104-014-2326-z
Cai, F., Pang, G., Li, R.-X., Li, R., Gu, X.-L., Shen, Q.-R., et al. (2017). Bioorganic fertilizer maintains a more stable soil microbiome than chemical fertilizer for monocropping. Biol. Fertil. Soils 53 (8), 861–872. doi: 10.1007/s00374-017-1216-y
Cai, S., Zhu, H., Wang, J., Yu, T., Qian, X., Shan, Y., et al. (2016). Fertilization impacts on green leafy vegetables supplied with slow release nitrogen fertilizers. J. Plant Nutr. 39 (10), 1421–1430. doi: 10.1080/01904167.2015.1050508
Cataldo, D. A., Haroon, M., Schrader, L. E., Youngs, V. L. (1975). Rapid colorimetric determination of nitrate in plant-tissue by nitration of salicylic-acid. Commun. Soil Sci. Plant Anal. 6 (1), 71–80. doi: 10.1080/00103627509366547
Chatterjee, R., Jana, J. C., Paul, P. K. (2012). Enhancement of head yield and quality of cabbage (Brassica oleracea) by combining different sources of nutrients. Indian J. Agric. Sci. 82 (4), 323–327.
Detheridge, A. P., Brand, G., Fychan, R., Crotty, F. V., Sanderson, R., Griffith, G. W., et al. (2016). The legacy effect of cover crops on soil fungal populations in a cereal rotation. Agric. Ecosyst. Environ. 228, 49–61. doi: 10.1016/j.agee.2016.04.022
El-Hadad, M. E., Mustafa, M. I., Selim, S. M., El-Tayeb, T. S., Mahgoob, A. E. A., Aziz, N. H. A. (2011). The nematicidal effect of some bacterial biofertilizers on meloidogyne incognita in sandy soil. Braz. J. Microbiol. 42 (1), 105–113. doi: 10.1590/s1517-83822011000100014
Emery, H. E., Angell, J. H., Fulweiler, R. W. (2019). Salt marsh greenhouse gas fluxes and microbial communities are not sensitive to the first year of precipitation change. J. Geophys. Res.-Biogeosci. 124 (5), 1071–1087. doi: 10.1029/2018jg004788
Ferreira, W. R., Ranal, M. A. (1999). Seed germination and seedlng growth of Brassica chinensis l. var. parachinensis (Bailey) sinskaja (flowering white cabbage). Pesquisa Agropecu. Bras. 34 (3), 353–361. doi: 10.1590/s0100-204x1999000300005
Ferreira, W. R., Ranal, M. A., Filgueira, F. A. R. (2002). Fertilizantes e espaçamento entre plantas na produtividade da couve-da-Malásia. Hortic. Bras. 20 (4), 635–640. doi: 10.1590/s0102-05362002000400025
Fukuda, M., Nakamura, S., Lopes Fonseca, A., Nasukawa, H., Ibraimo, M. M., Naruo, K., et al. (2017). Evaluation of the mehlich 3 reagent as an extractant for cations and available phosphorus for soils in Mozambique. Commun. Soil Sci. Plant Anal. 48 (12), 1462–1472. doi: 10.1080/00103624.2017.1373789
Gu, S., Hu, Q., Cheng, Y., Bai, L., Liu, Z., Xiao, W., et al. (2019). Application of organic fertilizer improves microbial community diversity and alters microbial network structure in tea (Camellia sinensis) plantation soils. Soil Tillage Res. 195, 104356–104366. doi: 10.1016/j.still.2019.104356
Guimaraes, D. V., Silva Gonzaga, M. I., da Silva, T. O., da Silva, T. L., Dias, N., Silva Matias, M. I. (2013). Soil organic matter pools and carbon fractions in soil under different land uses. Soil Tillage Res. 126, 177–182. doi: 10.1016/j.still.2012.07.010
Harindintwali, J. D., Zhou, J., Muhoza, B., Wang, F., Herzberger, A., Yu, X. (2021). Integrated eco-strategies towards sustainable carbon and nitrogen cycling in agriculture. J. Environ. Manage. 293, 112856–112868. doi: 10.1016/j.jenvman.2021.112856
Hedley, M. J., Stewart, J. W. B., Chauhan, B. S. (1982). Changes in inorganic and organic soil-phosphorus fractions induced by cultivation practices and by laboratory incubations. Soil Sci. Soc. America J. 46 (5), 970–976. doi: 10.2136/sssaj1982.03615995004600050017x
Huang, Y., Li, R., Liu, H., Wang, B., Zhang, C., Shen, Q. (2014). Novel resource utilization of refloated algal sludge to improve the quality of organic fertilizer. Environ. Technol. 35 (13), 1658–1667. doi: 10.1080/09593330.2013.878397
Hu, X., Liu, J., Wei, D., Zhu, P., Cui, X.A., Zhou, B., et al. (2018). Soil bacterial communities under different long-term fertilization regimes in three locations across the black soil region of northeast China. Pedosphere 28 (5), 751–763. doi: 10.1016/s1002-0160(18)60040-2
Ibrahim, M. H., Jaafar, H. Z. E., Karimi, E., Ghasemzadeh, A. (2013). Impact of organic and inorganic fertilizers application on the phytochemical and antioxidant activity of kacip fatimah (Labisia pumila benth). Molecules 18 (9), 10973–10988. doi: 10.3390/molecules180910973
Jiang, S. Q., Yu, Y. N., Gao, R. W., Wang, H., Zhang, J., Li, R., et al. (2019). High-throughput absolute quantification sequencing reveals the effect of different fertilizer applications on bacterial community in a tomato cultivated coastal saline soil. Sci. Total Environ. 687, 601–609. doi: 10.1016/j.scitotenv.2019.06.105
Jin, N., Jin, L., Wang, S., Li, J., Liu, F., Liu, Z., et al. (2022). Reduced chemical fertilizer combined with bio-organic fertilizer affects the soil microbial community and yield and quality of lettuce. Front. Microbiol. 13. doi: 10.3389/fmicb.2022.863325
Kang, Y., An, X., Ma, Y., Zeng, S., Jiang, S., Wu, W., et al. (2021). Organic amendments alleviate early defoliation and increase fruit yield by altering assembly patterns and of microbial communities and enzymatic activities in sandy pear (Pyrus pyrifolia). Amb Express 11 (1), 1–15. doi: 10.1186/s13568-021-01322-5
Kang, Y., Ma, Y., Wu, W., Zeng, S., Jiang, S., Yang, H., et al. (2022). Bioorganic and silicon amendments alleviate early defoliation of pear trees by improving the soil nutrient bioavailability, microbial activity, and reshaping the soil microbiome network. Appl. Soil Ecol. 173, 104383–104394. doi: 10.1016/j.apsoil.2021.104383
Kumar, U., Saqib, H. S. A., Islam, W., Prashant, P., Patel, N., Chen, W., et al. (2022). Landscape composition and soil physical-chemical properties drive the assemblages of bacteria and fungi in conventional vegetable fields. Microorganisms 10 (6), 1202–1220. doi: 10.3390/microorganisms10061202
Langille, M. G. I., Zaneveld, J., Caporaso, J. G., McDonald, D., Knights, D., Reyes, J. A., et al. (2013). Predictive functional profiling of microbial communities using 16S rRNA marker gene sequences. Nat. Biotechnol. 31 (9), 814–821. doi: 10.1038/nbt.2676
Lebedev, V. G., Popova, A. A., Shestibratov, K. A. (2021). Genetic engineering and genome editing for improving nitrogen use efficiency in plants. Cells 10 (12), 3303–3343. doi: 10.3390/cells10123303
Liang, R., Hou, R., Li, J., Lyu, Y., Hang, S., Gong, H., et al. (2020). Effects of different fertilizers on rhizosphere bacterial communities of winter wheat in the north China plain. Agronomy-Basel 10 (1), 93–105. doi: 10.3390/agronomy10010093
Liao, J., Ye, J., Liang, Y., Khalid, M., Huang, D. (2019). Pakchoi antioxidant improvement and differential rhizobacterial community composition under organic fertilization. Sustainability 11 (8), 2424–2440. doi: 10.3390/su11082424
Li, K., Guo, X. W., Xie, H. G., Guo, Y., Li, C. (2013). Influence of root exudates and residues on soil microecological environment. Pakistan J. Bot. 45 (5), 1773–1779.
Ling, N., Deng, K., Song, Y., Wu, Y., Zhao, J., Raza, W., et al. (2014). Variation of rhizosphere bacterial community in watermelon continuous mono-cropping soil by long-term application of a novel bioorganic fertilizer. Microbiol. Res. 169 (7-8), 570–578. doi: 10.1016/j.micres.2013.10.004
Liu, H., Chen, D., Zhang, R., Hang, X., Li, R., Shen, Q. (2016). Amino acids hydrolyzed from animal carcasses are a good additive for the production of bio-organic fertilizer. Front. Microbiol. 7. doi: 10.3389/fmicb.2016.01290
Liu, C. W., Sung, Y., Chen, B. C., Lai, H.-Y. (2014). Effects of nitrogen fertilizers on the growth and nitrate content of lettuce ( Lactuca sativa l.). Int. J. Environ. Res. Public Health 11 (4), 4427–4440. doi: 10.3390/ijerph110404427
Li, Q. C., Wang, B., Zeng, Y. H., Cai, Z. H., Zhou, J. (2022a). The microbial mechanisms of a novel photosensitive material (treated rape pollen) in anti-biofilm process under marine environment. Int. J. Mol. Sci. 23 (7), 3837–3859. doi: 10.3390/ijms23073837
Li, Y., Wu, H., Shen, Y., Wang, C., Wang, P., Zhang, W., et al. (2019). Statistical determination of crucial taxa indicative of pollution gradients in sediments of lake taihu, China. Environ. pollut. 246, 753–762. doi: 10.1016/j.envpol.2018.12.087
Li, Q., Xing, Y., Huang, B., Chen, X., Ji, L., Fu, X., et al. (2022b). Rhizospheric mechanisms of Bacillus subtilis bioaugmentation-assisted phytostabilization of cadmium-contaminated soil. Sci. Total Environ. 825, 154136–154149. doi: 10.1016/j.scitotenv.2022.154136
Li, Q., Zhang, D., Song, Z., Ren, L., Jin, X., Fang, W., et al. (2022c). Organic fertilizer activates soil beneficial microorganisms to promote strawberry growth and soil health after fumigation. Environ. pollut. 295, 118653–118664. doi: 10.1016/j.envpol.2021.118653
Ma, J. J., Ren, Y. J., Yan, L. Y. (2014). Effects of spray application of lanthanum and cerium on yield and quality of Chinese cabbage (Brassica chinensis l) based on different seasons. Biol. Trace Elemen. Res. 160 (3), 427–432. doi: 10.1007/s12011-014-0062-0
Mori, H., Maruyama, F., Kato, H., Toyoda, A., Dozono, A., Ohtsubo, Y., et al. (2014). Design and experimental application of a novel non-degenerate universal primer set that amplifies prokaryotic 16S rRNA genes with a low possibility to amplify eukaryotic rRNA genes. DNA Res. 21 (2), 217–227. doi: 10.1093/dnares/dst052
Nazaries, L., Singh, B. P., Sarker, J. R., Fang, Y., Klein, M., Singh, B. K. (2021). The response of soil multi-functionality to agricultural management practices can be predicted by key soil abiotic and biotic properties. Agric. Ecosyst. Environ. 307:107206–107219. doi: 10.1016/j.agee.2020.107206
Nguyen, N. H., Song, Z., Bates, S. T., Branco, S., Tedersoo, L., Menke, J., et al. (2016). FUNGuild: An open annotation tool for parsing fungal community datasets by ecological guild. Fungal Ecol. 20, 241–248. doi: 10.1016/j.funeco.2015.06.006
Otto, T. C., Scott, J. R., Kauffman, M. A., Hodgins, S. M., diTargiani, R. C., Hughes, J. H., et al. (2013). Identification and characterization of novel catalytic bioscavengers of organophosphorus nerve agents. Chemico-Biol. Interact. 203 (1), 186–190. doi: 10.1016/j.cbi.2012.09.009
Ozimek, E., Hanaka, A. (2021). Mortierella species as the plant growth-promoting fungi present in the agricultural soils. Agriculture-Basel 11 (1), 7–25. doi: 10.3390/agriculture11010007
Price, G. W., Langille, M. G. I., Yurgel, S. N. (2021). Microbial co-occurrence network analysis of soils receiving short- and long-term applications of alkaline treated biosolids. Sci. Total Environ. 751, 141687–141698. doi: 10.1016/j.scitotenv.2020.141687
Schloss, P. D., Westcott, S. L., Ryabin, T., Hall, J. R., Hartmann, M., Hollister, E. B., et al. (2009). Introducing mothur: open-source, platform-independent, community-supported software for describing and comparing microbial communities. Appl. Environ. Microbiol. 75 (23), 7537–7541. doi: 10.1128/aem.01541-09
Schoch, C. L., Seifert, K. A., Huhndorf, S., Robert, V., Spouge, J. L., Levesque, C. A., et al. (2012). Nuclear ribosomal internal transcribed spacer (ITS) region as a universal DNA barcode marker for fungi. Proc. Natl. Acad. Sci. U.S.A. 109 (16), 6241–6246. doi: 10.1073/pnas.1117018109
Schoebitz, M., Mengual, C., Roldan, A. (2014). Combined effects of clay immobilized azospirillum brasilense and Pantoea dispersa and organic olive residue on plant performance and soil properties in the revegetation of a semiarid area. Sci. Total Environ. 466, 67–73. doi: 10.1016/j.scitotenv.2013.07.012
Senghor, L. A., Ortega-Beltran, A., Atehnkeng, J., Callicott, K. A., Cotty, P. J., Bandyopadhyay, R. (2020). The atoxigenic biocontrol product aflasafe SN01 is a valuable tool to mitigate aflatoxin contamination of both maize and groundnut cultivated in Senegal. Plant Dis. 104 (2), 510–520. doi: 10.1094/pdis-03-19-0575-re
Sheng, X. F. (2005). Growth promotion and increased potassium uptake of cotton and rape by a potassium releasing strain of Bacillus edaphicus. Soil Biol. Biochem. 37 (10), 1918–1922. doi: 10.1016/j.soilbio.2005.02.026
Shen, W., Lin, X., Gao, N., Zhang, H., Yin, R., Shi, W., et al. (2008). Land use intensification affects soil microbial populations, functional diversity and related suppressiveness of cucumber fusarium wilt in China's Yangtze River delta. Plant Soil 306 (1-2), 117–127. doi: 10.1007/s11104-007-9472-5
Stursova, M., Zifcakova, L., Leigh, M. B., Burgess, R., Baldrian, P. (2012). Cellulose utilization in forest litter and soil: Identification of bacterial and fungal decomposers. FEMS Microbiol. Ecol. 80 (3), 735–746. doi: 10.1111/j.1574-6941.2012.01343.x
Sun, B., Gu, L., Bao, L., Zhang, S., Wei, Y., Bai, Z., et al. (2020). Application of biofertilizer containing Bacillus subtilis reduced the nitrogen loss in agricultural soil. Soil Biol. Biochem. 148:107911–107921. doi: 10.1016/j.soilbio.2020.107911
Su, Y., Zi, H., Wei, X., Hu, B., Deng, X., Chen, Y., et al. (2022). Application of manure rather than plant-origin organic fertilizers alters the fungal community in continuous cropping tobacco soil. Front. Microbiol. 13. doi: 10.3389/fmicb.2022.818956
Wang, L., Li, J., Yang, F., Yaoyao, E., Raza, W., Huang, Q., et al. (2017). Application of bioorganic fertilizer significantly increased apple yields and shaped bacterial community structure in orchard soil. Microbial. Ecol. 73 (2), 404–416. doi: 10.1007/s00248-016-0849-y
Wang, B., Sun, M., Yang, J., Shen, Z., Ou, Y., Fu, L., et al. (2022). Inducing banana fusarium wilt disease suppression through soil microbiome reshaping by pineapple-banana rotation combined with biofertilizer application. Soil 8 (1), 17–29. doi: 10.5194/soil-8-17-2022
Wu, K., Fang, Z., Wang, L., Yuan, S., Guo, R., Shen, B., et al. (2016). Biological potential of bioorganic fertilizer fortified with bacterial antagonist for the control of tomato bacterial wilt and the promotion of crop yields. J. Microbiol. Biotechnol. 26 (10), 1755–1764. doi: 10.4014/jmb.1604.04021
Wu, A. L., Jiao, X. Y., Fan, F. F., Wang, J. S., Guo, J., Dong, E. W., et al. (2019). Effect of continuous sorghum cropping on the rhizosphere microbial community and the role of Bacillus amyloliquefaciens in altering the microbial composition. Plant Growth Regul. 89 (3), 299–308. doi: 10.1007/s10725-019-00533-y
Wu, X., Zhang, T., Zhao, J., Wang, L., Yang, D., Li, G., et al. (2021). Variation of soil bacterial and fungal communities from fluvo-aquic soil under chemical fertilizer reduction combined with organic materials in north China plain. J. Soil Sci. Plant Nutr. 21 (1), 349–363. doi: 10.1007/s42729-020-00365-0
Xu, Y., Zheng, C., Liang, L., Yi, Z., Xue, S. (2021). Quantitative assessment of the potential for soil improvement by planting Miscanthus on saline-alkaline soil and the underlying microbial mechanism. Global Change Biol. Bioenergy 13 (7), 1191–1205. doi: 10.1111/gcbb.12845
Yang, Y., Li, G., Min, K., Liu, T., Li, C., Xu, J., et al. (2022). The potential role of fertilizer-derived exogenous bacteria on soil bacterial community assemblage and network formation. Chemosphere 287, 132338–132348. doi: 10.1016/j.chemosphere.2021.132338
Yang, H., Ma, J., Rong, Z., Zeng, D., Wang, Y., Hu, S., et al. (2019a). Wheat straw return influences nitrogen-cycling and pathogen associated soil microbiota in a wheat-soybean rotation system. Front. Microbiol. 10. doi: 10.3389/fmicb.2019.01811
Yang, Y., Wang, P., Zeng, Z. (2019b). Dynamics of bacterial communities in a 30-year fertilized paddy field under different organic-inorganic fertilization strategies. Agronomy-Basel 9 (1), 14–27. doi: 10.3390/agronomy9010014
Yang, J., Zhu, Z., Wang, Z., Zhu, B. (2010). Effects of storage temperature on the contents of carotenoids and glucosinolates in pakchoi (Brassica rapa l. ssp. Chinensis var. communis). J. Food Biochem. 34 (6), 1186–1204. doi: 10.1111/j.1745-4514.2010.00358.x
Yao, H., Sun, X., He, C., Maitra, P., Li, X.-C., Guo, L.-D. (2019). Phyllosphere epiphytic and endophytic fungal community and network structures differ in a tropical mangrove ecosystem. Microbiome 7, 57–72. doi: 10.1186/s40168-019-0671-0
Yuan, J., Ruan, Y., Wang, B., Zhang, J., Waseem, R., Huang, Q., et al. (2013). Plant growth-promoting rhizobacteria strain Bacillus amyloliquefaciens NJN-6-enriched bio-organic fertilizer suppressed fusarium wilt and promoted the growth of banana plants. J. Agric. Food Chem. 61 (16), 3774–3780. doi: 10.1021/jf400038z
Zhang, J., Akcapinar, G. B., Atanasova, L., Rahimi, M. J., Przylucka, A., Yang, D., et al. (2016). The neutral metallopeptidase NMP1 of Trichoderma guizhouense is required for mycotrophy and self-defence. Environ. Microbiol. 18 (2), 580–597. doi: 10.1111/1462-2920.12966
Zhang, S. J., Zeng, Y. H., Zhu, J. M., Cai, Z. H., Zhou, J. (2022). The structure and assembly mechanisms of plastisphere microbial community in natural marine environment. J. Hazard. Mater. 421, 126780–126793. doi: 10.1016/j.jhazmat.2021.126780
Zhao, Q., Ran, W., Wang, H., Li, X., Shen, Q., Shen, S., et al. (2013). Biocontrol of fusarium wilt disease in muskmelon with Bacillus subtilis y-IVI. Biocontrol 58 (2), 283–292. doi: 10.1007/s10526-012-9496-5
Zhao, Y., Zhang, M., Yang, W., Di, H. J., Ma, L., Liu, W., et al. (2019). Effects of microbial inoculants on phosphorus and potassium availability, bacterial community composition, and chili pepper growth in a calcareous soil: A greenhouse study. J. Soils Sediments 19 (10), 3597–3607. doi: 10.1007/s11368-019-02319-1
Keywords: bioorganic fertilizer, soil microbial profiles, Brassica chinensis L., promote plant growth, green agriculture
Citation: Wang T, Cheng K, Huo X, Meng P, Cai Z, Wang Z and Zhou J (2022) Bioorganic fertilizer promotes pakchoi growth and shapes the soil microbial structure. Front. Plant Sci. 13:1040437. doi: 10.3389/fpls.2022.1040437
Received: 09 September 2022; Accepted: 24 October 2022;
Published: 08 November 2022.
Edited by:
Patrizia Cesaro, University of Eastern Piedmont, ItalyReviewed by:
Qi Shen, Zhejiang Academy of Agricultural Sciences, ChinaCopyright © 2022 Wang, Cheng, Huo, Meng, Cai, Wang and Zhou. This is an open-access article distributed under the terms of the Creative Commons Attribution License (CC BY). The use, distribution or reproduction in other forums is permitted, provided the original author(s) and the copyright owner(s) are credited and that the original publication in this journal is cited, in accordance with accepted academic practice. No use, distribution or reproduction is permitted which does not comply with these terms.
*Correspondence: Jin Zhou, zhou.jin@sz.tsinghua.edu.cn; Zongkang Wang, zongkang@vip.qq.com
†These authors have contributed equally to this work and share last authorship
Disclaimer: All claims expressed in this article are solely those of the authors and do not necessarily represent those of their affiliated organizations, or those of the publisher, the editors and the reviewers. Any product that may be evaluated in this article or claim that may be made by its manufacturer is not guaranteed or endorsed by the publisher.
Research integrity at Frontiers
Learn more about the work of our research integrity team to safeguard the quality of each article we publish.