- 1State Key Laboratory of Ecological Pest Control for Fujian and Taiwan Crops, College of Plant Protection, Fujian Agriculture and Forestry University, Fuzhou, China
- 2Institute of Subtropical Fruits, Fujian Agriculture and Forestry University, Fuzhou, China
- 3Institute of Horticultural Sciences, University of Agriculture, Faisalabad, Pakistan
- 4Department of Horticulture, Faculty of Food and Crop Science, Pir Mehr Ali Shah (PMAS)-Arid Agriculture University, Rawalpindi, Pakistan
- 5Department of Horticulture, Faculty of Agricultural Sciences and Technology, Bahauddin Zakariya University, Multan, Pakistan
- 6Department of Horticulture, College of Agriculture, University of Al-Azhar (Branch Assiut), Assiut, Egypt
- 7Department of Horticulture, Agricultural Faculty, Ataturk University, Erzurum, Turkey
Soluble sugars and organic acids are the most abundant components in ripe fruits, and they play critical roles in the development of fruit flavor and taste. Some loquat cultivars have high acid content which seriously affect the quality of fruit and reduce the value of commodity. Consequently, studying the physiological mechanism of sugar-acid metabolism in loquat can clarify the mechanism of their formation, accumulation and degradation in the fruit. Minerals application has been reported as a promising way to improve sugar-acid balance of the fruits. In this study, loquat trees were foliar sprayed with 0.1, 0.2 and 0.3% borax, and changes in soluble sugars and organic acids were recorded. The contents of soluble sugars and organic acids were determined using HPLC-RID and UPLC-MS, respectively. The activities of enzymes responsible for the metabolism of sugars and acids were quantified and expressions of related genes were determined using quantitative real-time PCR. The results revealed that 0.2% borax was a promising treatment among other B applications for the increased levels of soluble sugars and decreased acid contents in loquats. Correlation analysis showed that the enzymes i.e., SPS, SS, FK, and HK were may be involved in the regulation of fructose and glucose metabolism in the fruit pulp of loquat. While the activity of NADP-ME showed negative and NAD-MDH showed a positive correlation with malic acid content. Meanwhile, EjSPS1, EjSPS3, EjSS3, EjHK1, EjHK3, EjFK1, EjFK2, EjFK5, and EjFK6 may play an important role in soluble sugars metabolism in fruit pulp of loquat. Similarly, EjPEPC2, EjPEPC3, EjNAD-ME1, EjNAD-MDH1, EjNAD-MDH5-8, EjNAD-MDH10, and EjNAD-MDH13 may have a vital contribution to malic acid biosynthesis in loquat fruits. This study provides new insights for future elucidation of key mechanisms regulating soluble sugars and malic acid biosynthesis in loquats.
Introduction
Fruits have their own biochemical and nutritional features over the course of their growth, which eventually results in their distinct fruit quality (Bermejo and Cano, 2012; Ren et al., 2015; Zhang et al., 2021). This process promotes the development of sugar and organic acid metabolites, which are important for the development of fruit flavor since the growth of fruit is usually accompanied by the accumulation and degradation of sugars and organic acids (; Zhang et al., 2014: 2021). The balance between the sugar-acid production, breakdown, and vacuole storage determines their ultimate content in ripe fruits (Ruffner et al., 1984; Pan et al., 2021). Fruits are categorised into three major categories based on the amount of organic acid they contain: malic acid, citric acid, and tartaric acid (Batista-Silva et al., 2018). Malic acid is the primary organic acid type in loquat (Li et al., 2015b; Ali et al., 2021a).
Loquat (Eriobotrya japonica Lindl.) is an evergreen fruit tree native to China (Ali et al., 2021b). It is a member of family Rosaceae, subfamily Maloideae (Ali et al., 2021c). Vitamin A, vitamin B6, potassium, magnesium, and dietary fibre are all abundant in this fruit (Badenes et al., 2003). It is an orange-colored fruit with a mildly sweet flavor (Zhi et al., 2021). Loquat fruit cannot be preserved for lengthy periods of time due to its soft and juicy flesh and thin peel (Tian et al., 2011). Besides its utilization as fruit, it is a good source of honey. Its flowers are much attractive to honey bees, especially white-colored flowers (Karadeniz et al., 2012). Japan, Korea, India, Pakistan, and China’s south-central area are the main producers of loquat (Ali et al., 2021d). In California, it’s also cultivated as an ornamental shrub (LaRue, 2020). Loquat is grown on more than 130 thousand hectares in China, making it the world’s biggest producer and exporter. China produces 650 thousand tonnes of loquats each year (Zheng et al., 2019). High fruit acidity and low sugars have been major factors lowering fruit quality and commodity value in commercial loquat production (Chen et al., 2009).
The mineral elements are absorbed to variable degrees and play important roles in fruit quality, since many of them are required for photosynthesis, respiration, energy metabolism, and cell structure (Broadley et al., 2012; Engels et al., 2012; Wiesler, 2012; Ali et al., 2021d). In comparison to soil application, foliar application of nutrients has a 10-20 percent greater influence (Zaman and Schumann, 2006; Ali et al., 2021b). Boron (B) is involved in a variety of metabolic functions, such as sugar transport and respiration (Ali et al., 2019), cell wall formation (Brown et al., 2002), cell division and elongation (Goldbach et al., 2001; de Oliveira et al., 2006), membrane stability, carbohydrate metabolism and Ca2+ uptake, hormone activation, root development, water translocation (Zhao and Oosterhuis, 2002; Sheng et al., 2009), and the activation of dehydrogenase enzymes (Marschner, 1995; El-Sheikh et al., 2007). However, the physiological and molecular functions of boron in regulating sugar-acid metabolism are not fully known at this time, and more research is required to clarify this.
Here, in this study, loquat trees were foliar sprayed with 0.1, 0.2 and 0.3% borax, and changes in soluble sugars and organic acids were recorded. Soluble sugars (fructose, glucose and sucrose) and organic acids (fumaric acid, ascorbic acid, malic acid, cis-aconitic acid and acetic acid) were quantified using HPLC and UPLC, respectively. The HPLC method can directly determine oligosaccharide with a simple sample preparation. It is one of the most promising methods for sugar analysis, due to its universality, time efficiency, accuracy, and selectivity for the quantification of carbohydrates (Kakita et al., 2002). Similarly, UPLC method is used to determine organic acid content of fruits, because of its simplicity, speed and stability (Fedorova et al., 2020). We not only investigated the effect of different concentrations of B on yet unexplored aspects of loquat sugar-acid metabolism but also segregated concentration-dependent variations in activities of related enzymes and relative expression of their biosynthesis-related genes.
Materials and methods
Plant material, experimental design and treatments
The young loquat trees (Cv. Jiefangzhong), growing in an orchard located in the subtropical area of Fujian province (Fuqing) (25°47’26.0”N 119°20’31.0”E), were selected for this study. The loquat trees ranged in height from 4 to 5.5 m and had a canopy diameter of 4 to 5 m. The spacing between each tree in the planting was roughly 6 × 6 m. Throughout the last three growing seasons, loquat trees have been subjected to methodical pruning and thinning, as well as fertilization with nitrogen (N), phosphorus (P) and potassium (K) (15:15:15) at a rate of 5 kg per plant every season. A randomized block design was used to allocate the distribution of plants for various treatments (RCBD). Each treatment had a total of four replications, or blocks, allocated to it, and each individual tree was counted as a single replicate for each treatment. Standard agricultural procedures were used throughout the production of loquats. These activities included drip irrigation, mineral supplementation, weed management, and the management of insects/pests and diseases. The experiment consisted of four separate treatments, the control (water spray), 0.1% borax, 0.2% borax, and 0.3% borax respectively. These foliar concentrations were chosen after an earlier study about phyto-nutritional composition of loquat (Ali et al., 2021b). The foliar treatment was done twice during the full bloom stage (the first week of January, 2020), with a three-week break in between each application. Early in the morning, a foliar spray of B was applied to loquat trees using an electronic sprayer with a capacity of 5 L that was set at a constant pace. Fully ripened fruits were sampled from the sun-exposed tree canopy (Ali et al., 2021c; Ali et al., 2021b), at about 1.5–2.5 m height, 90 days after first foliar spray, and brought back immediately to the laboratory (Institute of Tropical and Subtropical Fruits, FAFU).
Fruit weight and size
The average fresh weight, length (from the tallest point), and width (from the widest point) of the fruit were determined by averaging five batches of fruit, each of which consisted of 10 loquats from the same treatment. The fruit’s weight was measured using a digital weighing balance (MJ-W176P, Panasonic, Japan), and its length and diameter were gauged with digital Vernier callipers (DR-MV0100NG, Ningbo Dongrun Imp. & Exp. Co., Ltd., China). The length-to-width ratio, hereafter referred to as the fruit shape index, was determined by dividing each fruit’s length by its diameter.
Soluble solids, titratable acids, sugar-acid ratio and fruit juice pH
Using a titrimetric approach based on NaOH (Hortwitz, 1960), the total titratable acids were calculated and shown as a percentage of citric acid. A portable digital refractometer was used to calculate the total soluble solids (Atago, Hybrid PAL-BXIACID F5, Japan). Total soluble solids in the sample were divided by total titratable acids to get the sugar-acid ratio. The acidity level of fruit juice was measured using a digital pH meter (Hanna, HI-98107, Mauritius).
Soluble sugars determination through HPLC-RID
The contents of soluble sugars were determined through high-performance liquid chromatography – refractive index detection (HPLC-RID) as earlier described by Yu et al. (2021). Fruit samples (pulp stored at -80°C) were ground up in liquid nitrogen, and the resulting 2 g of fine powder was mixed with a modest quantity of polyvinylpyrrolidone in 10 mL of 95% methanol. The supernatant fluid was collected after ultra-sonification at 40°C for 30 min and centrifugation at 1000 rpm for 10 min. With 8 mL of ultrapure water, the procedure was repeated using the leftover residue. A 0.22 m syringe filter was then used to filter the clear liquid (ANPEL, China). A Waters 2695 autosampler system was used for HPLC-RID analysis. Ellistat Supersil NH2 column (4.6 mm × 250 mm, 5 µm particle size) (Waters Inc, Zellik, Belgium) was used to separate soluble sugars, operated at 40°C. The mobile phases consisted of 82% acetonitrile and 18% ultrapure water solution mixture. The amount of the injection was 20 µL, and the flow rate was 1.2 mL per min. In the end, the concentration of each and every solitary soluble sugar was determined by using the calibration curve of the standard that corresponded to it. The standards of fructose (99%), glucose (99.5%)) and sucrose (99.5%) were obtained from Sigma-Aldrich, USA. Every single one of the assays for soluble sugars was carried out using three separate samples. The output was given in milligrams per milliliter, which was denoted with the notation mg·ml-1.
Organic acids determination through UPLC-MS
The extraction of organic acids was carried out using the method outlined by Nour et al. (2010), although with minor adjustments. In order to extract juice from the loquat fruits, they were first halved and then pressed. After going through three layers of gauze material, the pulp was removed. Following centrifugation of the juice at 4000 rpm for 15 min, the supernatant was diluted 25 times and passed through an MF-Millipore™ Membrane Filter with a pore size of 0.22 μm in diameter. The ultra-performance liquid chromatography – mass spectrometry (UPLC-MS) technique was used in order to investigate organic acids. A sample of 10 µL of eluate was injected into an Acquity UPLC HSS T3 column (1.8 µm particle size, 2.1 mm × 100 mm). When employing a solution containing 0.025% H3PO4 as the solvent, the flow rate was 0.2 mL per min. Organic acids were detected at 210 nm, while column temperature was 30°C. A Waters 2996 diode array detector (Waters Corporation, USA) was used to detected the eluted peaks. Using the calibration curve of the relevant standard, the contents of the various organic acids were able to be determined and computed. The standards of fumaric acid (99%), ascorbic acid (99%), malic acid (99%), cis-aconitic acid (98%) and acetic acid (99.7%) were obtained from Sigma-Aldrich, USA. Every one of the assays for organic acids was carried out using three separate samples. The output was reported in milligrams per milliliter of fresh juice, which is abbreviated as mg·ml-1 juice.
Limits of detection and quantification were included as parts of the HPLC-RID and UPLC-MS procedures’ validation parameters (Ribani et al., 2004). The peaks were identified by their retention times, comparing the UV–Visible spectra and spiking with standards. Quantification has been done using an external standard curve with five points (Table 1; Figures S1, S2).
Enzymes extraction and activity assay
The enzymes responsible for sugar [sucrose-phosphate synthase (SPS), sucrose synthase (SS), hexokinase (HK) and fructokinase (FK)] and acid metabolism [phosphoenolpyruvate carboxylase (PEPC), NADP – dependent malic enzyme (NADP-ME) and NAD – malate dehydrogenase (NAD-MDH)] were extracted and measured using the Solarbio enzyme activity kits (Solarbio Life Sciences, Beijing, China) according to the manufacturer’s instructions (Zhang et al., 2021). The extraction kits were based on the earlier determined methods for SPS (Schrader and Sauter, 2002), SS (Schrader and Sauter, 2002), HK (Pancera et al., 2006), FK (Papagianni and Avramidis, 2011), PEPC (Zhang et al., 2008), NADP-ME (Spampinato et al., 1994), and NAD-MDH (Yao et al., 2011).
RNA extraction and real-time quantitative PCR
Total RNA was extracted from loquat fruit pulp using a Total RNA kit (TianGen Biotech, Beijing, P.R. China). NanoDrop N-1000 spectrophotometer (NanoDrop technologies, Wilmington, DE, USA) was used to analyze RNA concentration and purity. First-strand cDNA was synthesized from 1 µg of total RNA using the Prime Script RT Reagent Kit with a gDNA Eraser (TaKaRa, Dalian, China). High-performance real-time PCR (LightCycler® 96, Roche Applied Science, Penzberg, Germany) was used for the qPCR analysis. Primers used in quantitative real-time polymerase chain reaction (qRT-PCR) are included in Table S1, which were designed using Primer-blast.
The reaction mixture contained 10 μL 2×RealStar Green Fast Mixture (GenStar, Bejing, China), 1 µL cDNA, 0.25 µM of each primer and water was added to make a final volume of 20 µL. The qRT-PCR protocol started with a 5 min “preincubation” at 95°C, then 40 cycles at 95°C for 10 s and 60°C for 30 s, a “melting” step at 95°C for 10 s, 65°C for 1 min, and 97°C for 1 s, and a “cooling” phase at 37°C for 30 s. The 2-ΔΔct approach (Munhoz et al., 2015) was used to determine relative gene expression, with the actin protein (EVM0004523.1) serving as the internal control (Gan et al., 2020). The validation of 2−ΔΔCt method was carried out by ΔCt variation analysis at different template concentrations (Livak and Schmittgen, 2001; Xu et al., 2017; De Rossi et al., 2021). Each sample was analysed using three biological replicates.
Statistical analysis
The collected data was analyzed using an ANOVA with the help of the statistical programme “Statistix 8.1” (https://www.statistix.com/). Means of replicated data from each treatment were compared using Fisher’s least significant difference (LSD) method, when p ≤ 0.05. The Pearson (n) approach was used in ‘Statistix 8.1’ to calculate the correlation coefficient values, and “TBtools ver. 0.6655” (https://github.com/CJ-Chen/TBtools) was used to depict the data as a heat map. Principle component analysis (PCA) of treatments and tested variable was done through Pearson (n) method using “XLSTAT ver. 2019” (https://www.xlstat.com/en/).
Results
Fruit weight and size
Loquat plants treated with foliar supplied B exhibited a significant increase (p ≤ 0.05) in fruit weight and size (fruit length, width, and fruit shape index) as compared to untreated plants. The plants receiving 0.2% borax exhibited maximum fruit weight (57.95 g), which was 18.19% higher than that of untreated plants (Figure 1A). Regardless of concentration, B improved the loquat fruit length by 8-18% as compared to control (Figure 1B). The maximum fruit width (43.52 mm) was recorded in the plants treated with 0.2% borax followed by the plants receiving 0.1% (40.88 mm) and 0.3% borax (39.11 mm) (Figure 1C). Boron application reduced fruit shape index regardless of concentration applied, indicating its possible role in improving fruit size in terms of diameter. The minimum fruit shaped index (1.25) was recorded in the plants treated with 0.1-0.2% borax, which was 8% less as compared to that of untreated plants (Figure 1D).
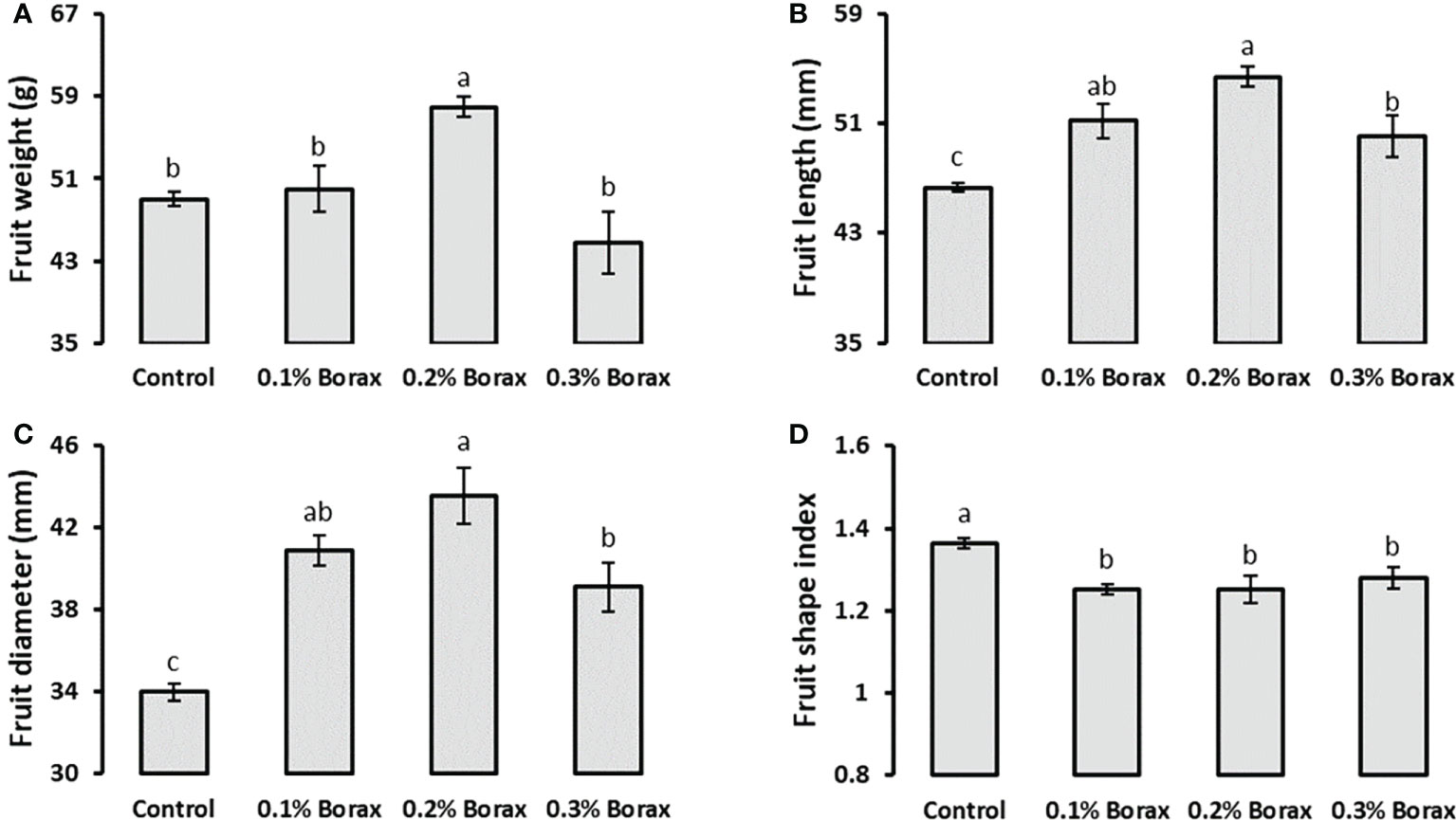
Figure 1 Effect of foliar application of B on weight (A), length (B), diameter (C), and shape index (D) of loquat fruits. Loquat plants were foliar sprayed with B twice at blooming stage. Same letters indicate non-significant difference among treatments according to Fisher’s least significant difference (LSD) test, when p ≤ 0.05. Vertical bars indicate mean ± standard error (n=4, 4-block RCBD arrangement).
Soluble solids, titratable acids, sugar-acid ratio and fruit juice pH
Total soluble solids (TSS) and titratable acidity (TTA) of loquat fruits showed reciprocal responses to each other. Foliar application of 0.2% borax enhanced TSS by 36.86%, while reduced the TTA by 61.90% comparing with control (Figures 2A, B). The plants receiving foliar application of 0.2% B exhibited 3.60-fold increase in sugar-acid ratio, as compared to control. The increased sugar-acid ratio and fruit juice pH indicates the positive influence of applied treatments on sugars accumulation in loquat fruits (Figures 2C, D).
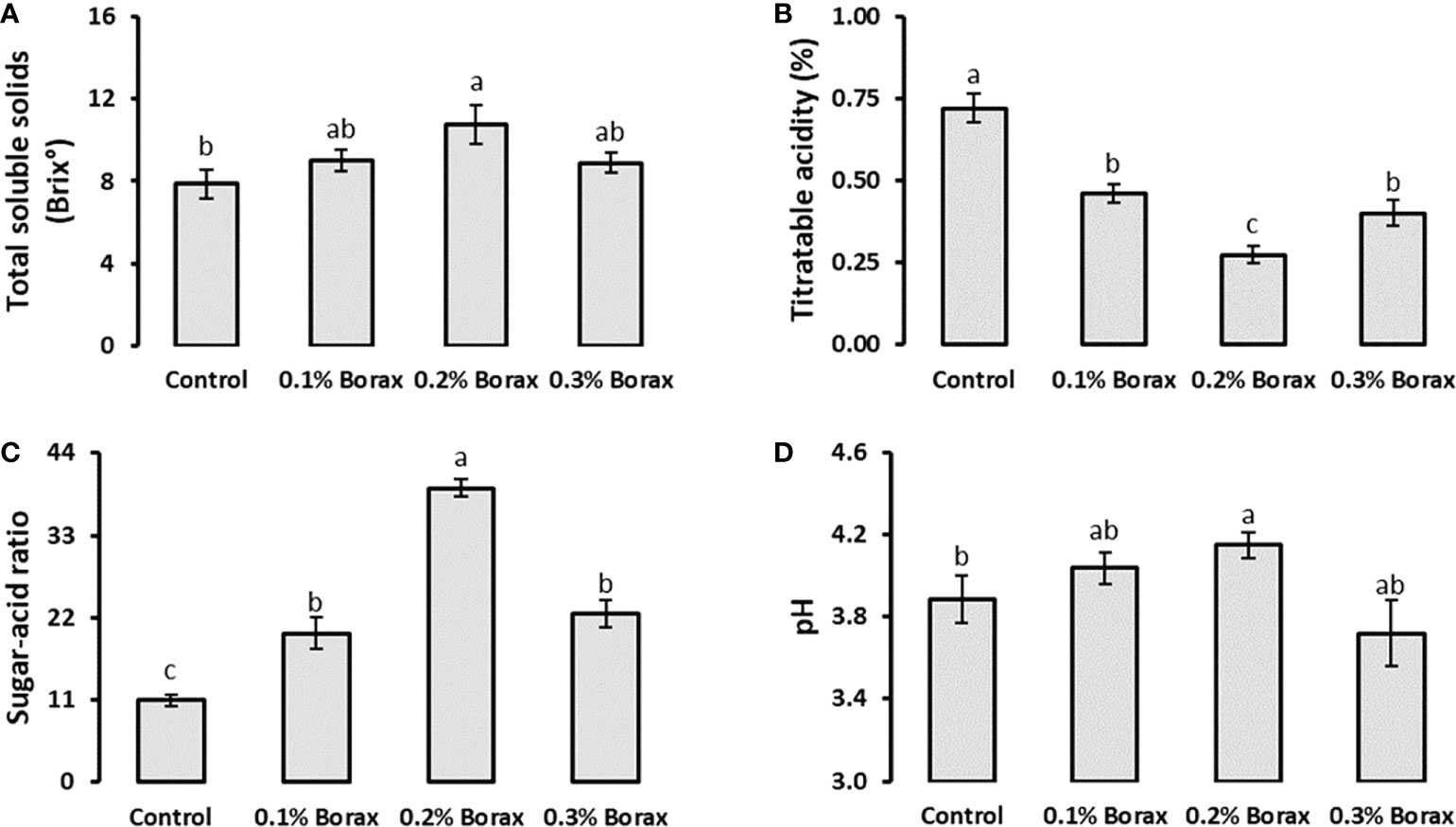
Figure 2 Effect of foliar application of B on total soluble solids (A), titratable acidity (B), sugar-acid ratio (C), and pH (D) of loquat fruits. Same letters indicate non-significant difference among treatments according to Fisher’s least significant difference (LSD) test, when p ≤ 0.05. Vertical bars indicate mean ± standard error (n=4, 4-block RCBD arrangement).
Soluble sugars
Three soluble sugars i.e., fructose, glucose and sucrose were quantified in the fruit pulp of B-treated loquat fruits through HPLC (Figure 3). The results revealed that fructose and glucose were the abundant soluble sugars in loquat pulp as compared to sucrose contributing 45.31%, 41.95% and 12.74%, respectively. The fructose and glucose accumulation in loquat fruits showed same pattern with respect to applied treatments. The plants receiving exogenous application of 0.2% borax exhibited maximum fruit fructose level (8.91 mg·ml-1) among all other treatments, which was 1.34-times higher (33.89%) than that of untreated plants (Figure 3A). Among B treatments, 0.2% borax improved fruit glucose level by 58.21%, as compared to the fruit glucose level of untreated plants (Figure 3B). In case of fruit sucrose, plants receiving 0.3% borax showed a significant (p ≤ 0.05) improvement in sucrose level, which was recorded as 3.31-fold higher than control (Figure 3C).
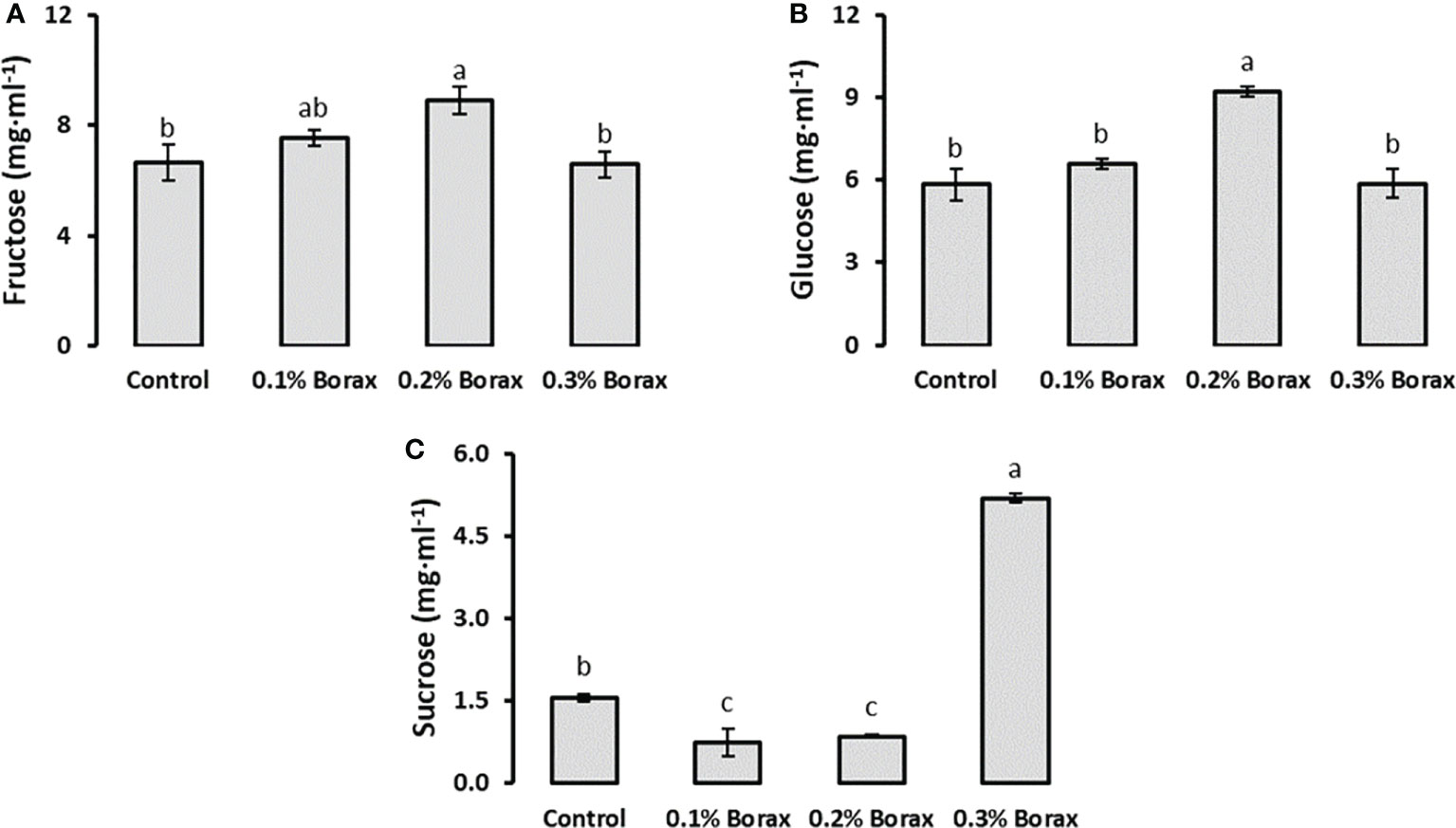
Figure 3 Effect of foliar application of B on soluble sugars i.e., fructose (A), glucose (B), and sucrose (C) content of loquat fruits. Same letters indicate non-significant difference among treatments according to Fisher’s least significant difference (LSD) test, when p ≤ 0.05. Vertical bars indicate mean ± standard error (n=4, 4-block RCBD arrangement).
Organic acids
Five organic acids i.e., fumaric acid, ascorbic acid, malic acid, cis-aconitic acid and acetic acid were quantified in the fruit pulp of B-treated loquat fruits through UPLC. The results revealed that malic acid was the most abundant organic acid in loquat pulp followed by acetic acid contributing 81.12% and 18.21%, respectively. The proportion of fumaric acid, ascorbic acid and cis-aconitic acid was less than 1% among tested organic acids (Figures 4A, B, E). The exogenous application of B significantly reduced the malic acid concentration as compared to control, ultimately reduced overall acidity of the fruits. The plants receiving 0.2% borax exhibited minimum fruit malic acid level (1.31 mg·ml-1) among all other treatments, which was 1.56-times (36.07%) lower than that of untreated plants (Figure 4C). In case of acetic acid content, it was observed that B treatments reduced the acetic acid content in dose-dependent manner (Figure 4D).
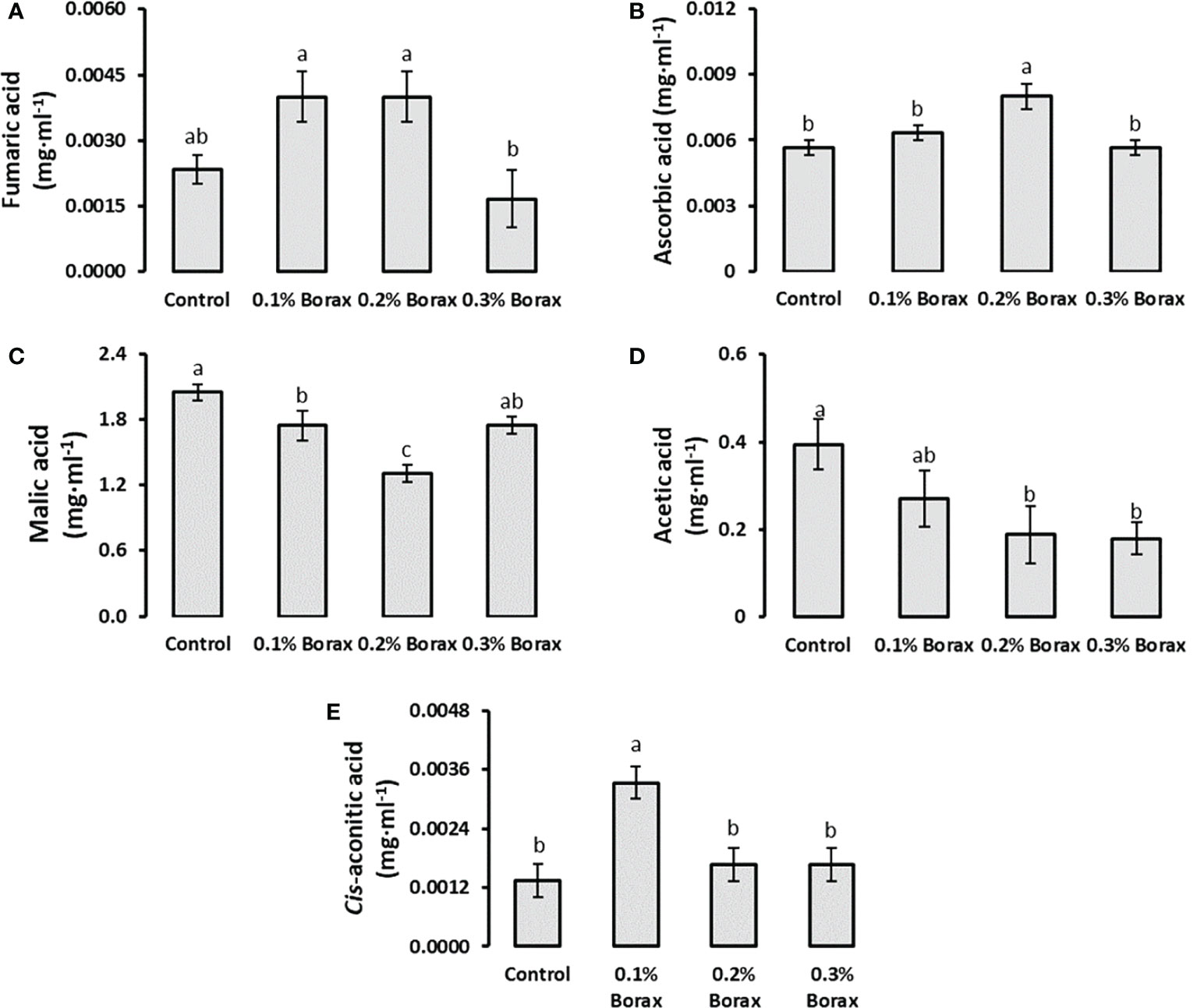
Figure 4 Effect of foliar application of B on the level of organic acids i.e., fumaric acid (A), ascorbic acid (B), malic acid Ali et al.,, acetic acid (D), and cis-aconitic acid (E) in loquat fruits. Same letters indicate non-significant difference among treatments according to Fisher’s least significant difference (LSD) test, when p ≤ 0.05. Vertical bars indicate mean ± standard error (n=4, 4-block RCBD arrangement).
Key enzymes involved in soluble sugars metabolism
The SPS activity in the fruit pulp of loquat significantly (p ≤ 0.05) increased with B application. The maximum SPS activity was detected in the fruit pulp of the plants treated with 0.2% borax (3237.07 U·g-1 protein) (Figure 5A). Interestingly, the SS activity was found decreased with the foliar application of 0.2% borax (401.97 U·g-1 protein) as compared to control (669.69 U·g-1 protein), which was 39.98% reduced as compared to control (Figure 5B). Unlike SS, HK and FK activities were recorded improved in fruit pulp of loquat with all preharvest treatments of B. The maximum HK activity (61.22 U·g-1 protein) was measured in the fruit pulp of the plants treated with 0.2% borax (Figure 5C). Similarly, maximum FK activity was recorded in fruit pulp of the loquat plants receiving foliar application of 0.2% borax (391.50 U·g-1 protein), which was 5.61-fold higher than that of untreated plants (Figure 5D).
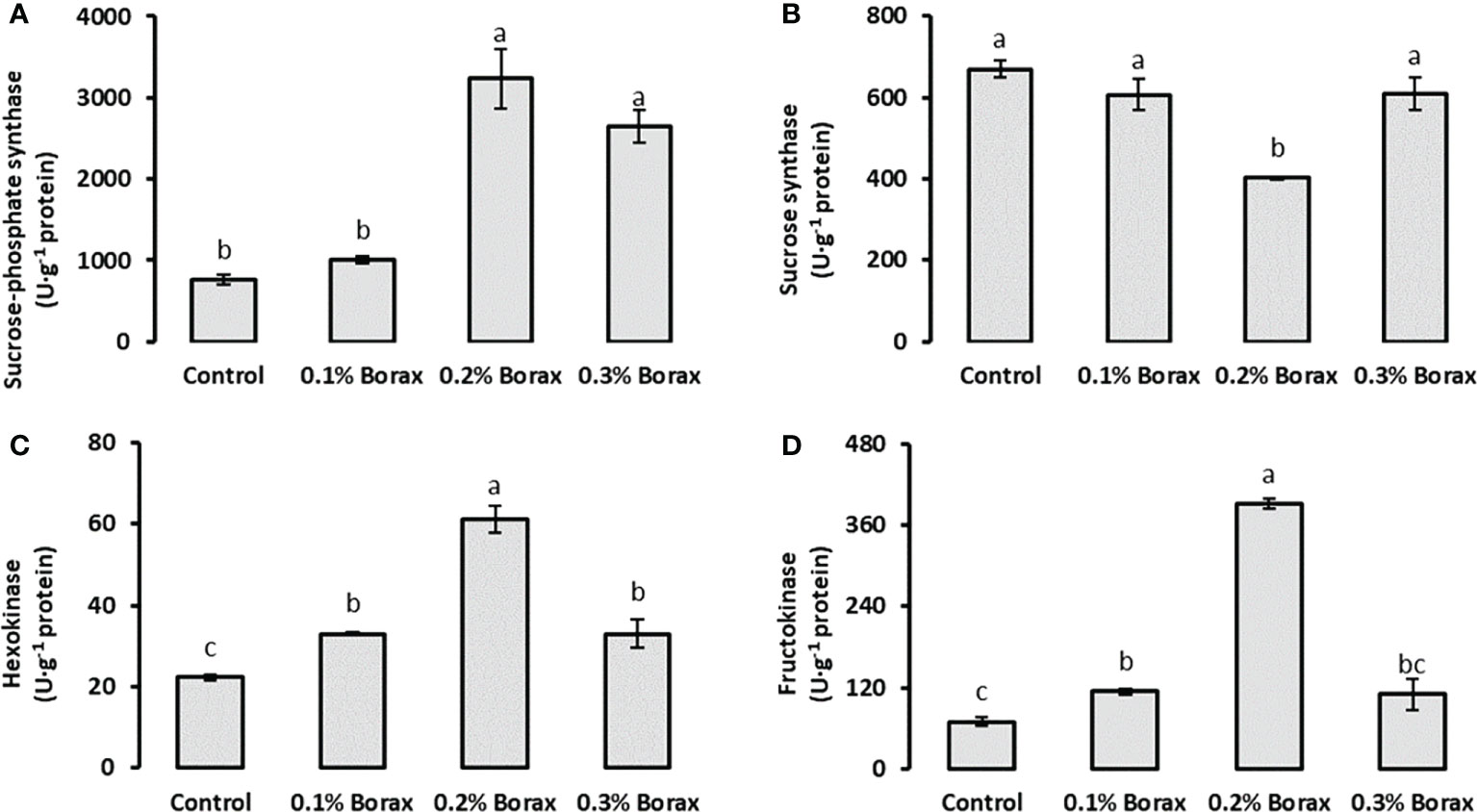
Figure 5 Effect of foliar application of B on the activities of key enzymes involved in soluble sugars metabolism i.e., sucrose-phosphate synthase (A), sucrose synthase (B), hexokinase (C) and fructokinase (D) of loquat fruits. Same letters indicate non-significant difference among treatments according to Fisher’s least significant difference (LSD) test, when p ≤ 0.05. Vertical bars indicate mean ± standard error (n=4, 4-block RCBD arrangement).
Key enzymes involved in malic acid metabolism
The PEPC activity in the fruit pulp of loquat significantly (p ≤ 0.05) reduced with B application. The minimum PEPC activity was detected in the fruit pulp of the plants treated with 0.2% borax (150.87 U·g-1 protein) (Figure 6A). Interestingly, the NADP-ME activity was found increased with the foliar application of B at the concentration of 0.2%. The maximum NADP-ME activity was recorded in the fruit pulp of loquats receiving 0.2% borax (1578.76 U·g-1 protein) in comparison with control (771.68 U·g-1 protein), which were 2.04-fold higher that of untreated plants, respectively (Figure 6B). Conversely, the exogenous application of B significantly reduced the activity of NAD-MDH as compared to control. Among B treatments, the minimum NAD-MDH activity level was recorded in the loquats treated with 0.20-0.30% borax (2668.87-2733.18 U·g-1 protein) (Figure 6C).
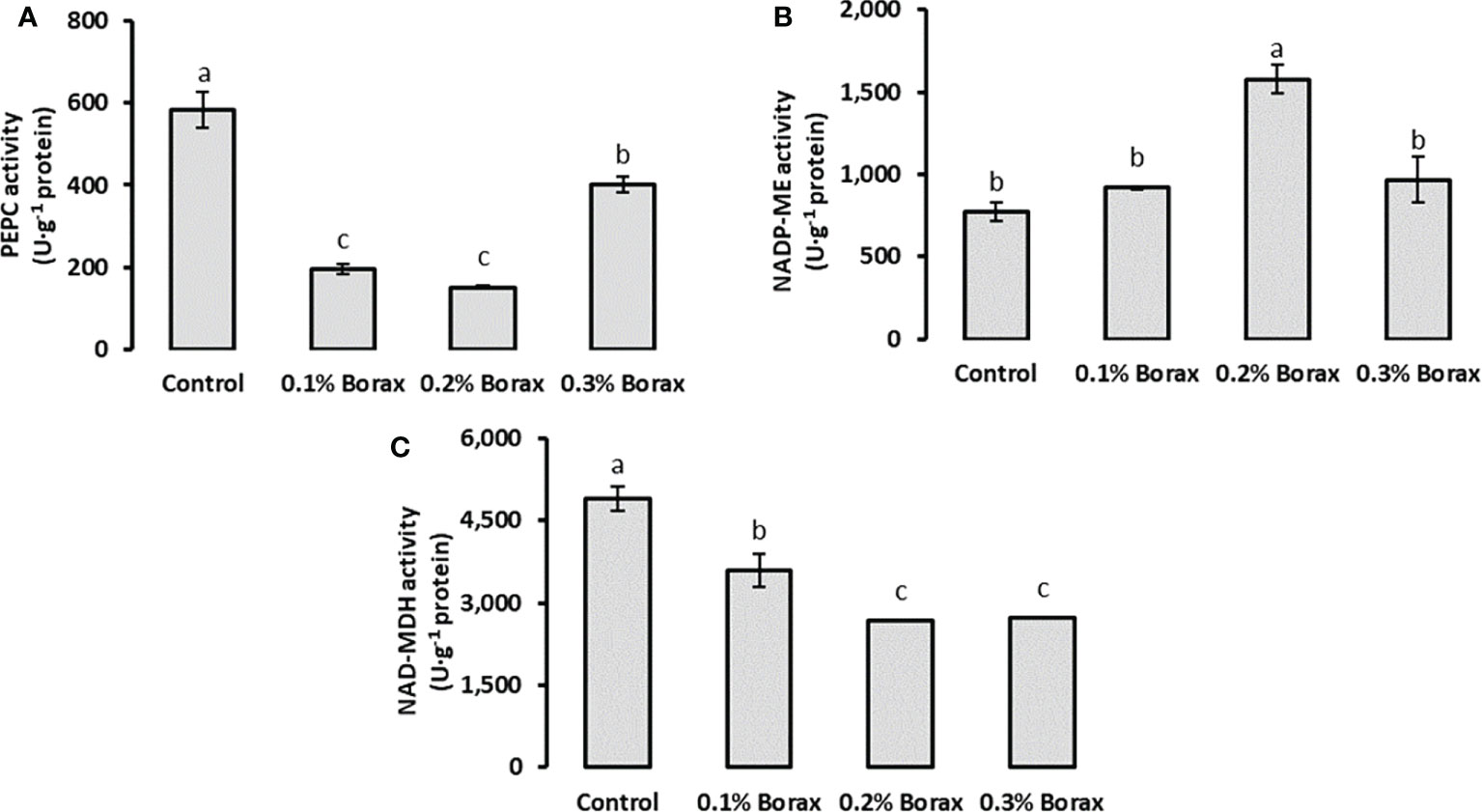
Figure 6 Effect of foliar application of B on the activities of key enzymes involved in malic acid metabolism i.e., PEPC (A), NADP-ME (B), and NAD-MDH (C) of loquat fruits. Same letters indicate non-significant difference among treatments according to Fisher’s least significant difference (LSD) test, when p ≤ 0.05. Vertical bars indicate mean ± standard error (n=4, 4-block RCBD arrangement).
Expression profiling of soluble sugars metabolism-related genes
The expression patterns of core genes i.e., EjSPS1-4, EjSS1-5, EjHK1-3, and EjHK1-6 encoding key enzymes i.e., SPS, SS, HK and FK responsible for the metabolism of soluble sugars in fruit pulp of loquat were studied (Figure 7). The expression patterns of EjSPS1-4 genes increased with the foliar application of B. Briefly, the relative expression of EjSPS1 was recorded maximum in the fruit pulp of loquat when treated with 0.2% borax. Similarly, EjSPS2 was maximally expressed under the influence of 0.2% borax, which was 2.14-fold higher than that of control. The EjSPS3 and EjSPS4 exhibited maximum upregulation under the influence of 0.1-0.2% and 0.2-0.3% borax, respectively.
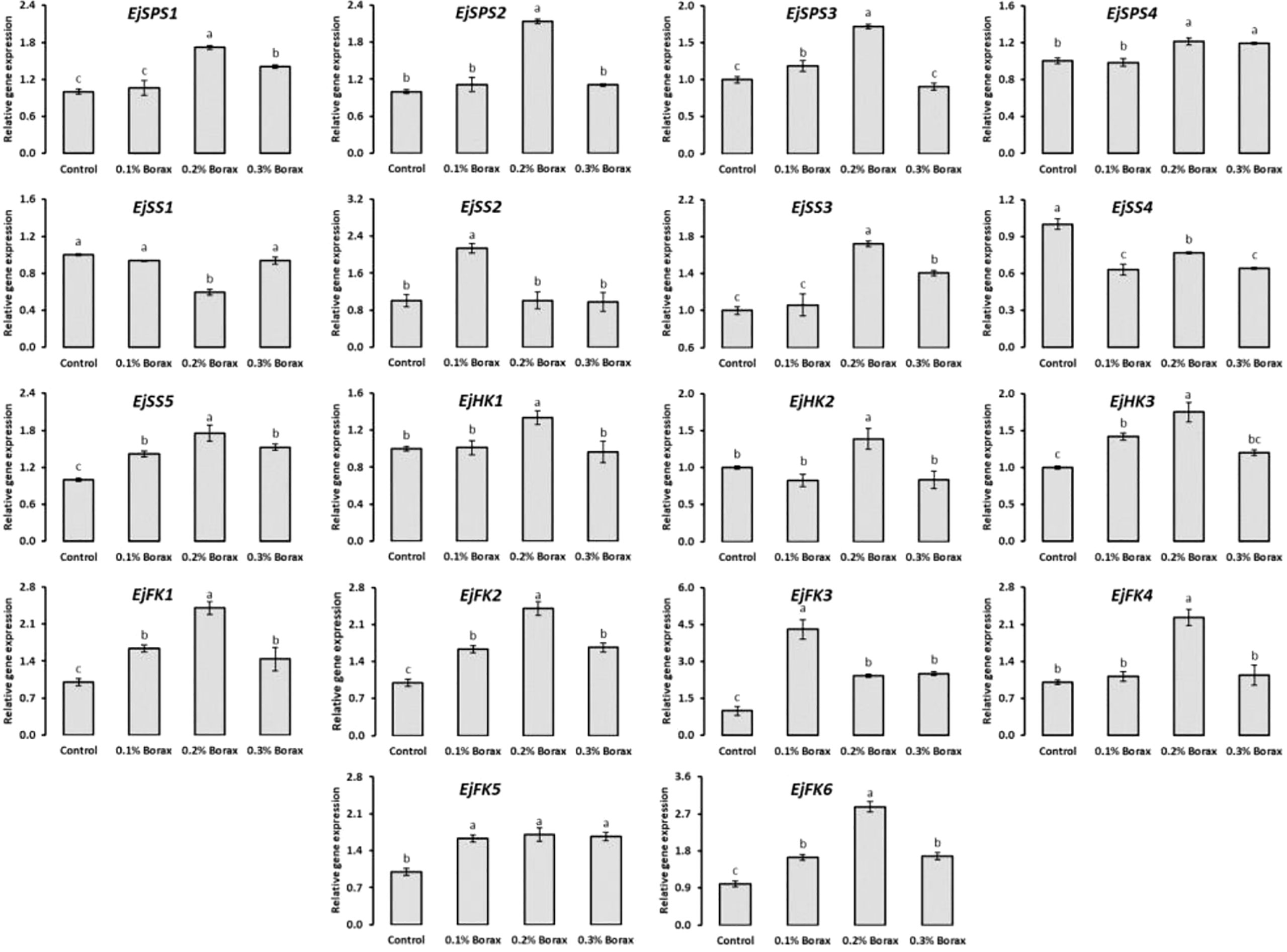
Figure 7 The expression profiling of core genes involved in soluble sugars metabolism of loquat as influenced by the foliar application of B Same letters indicate non-significant difference among treatments according to Fisher’s least significant difference (LSD) test, when p ≤ 0.05. Vertical bars indicate mean ± standard error (4 biological and 3 technical replicates).
The EjSS3 and EjSS5 were maximally expressed under the influence of 0.2% borax. Specifically, EjSS1 was significantly (p ≤ 0.05) down-regulated by aforementioned treatment, while showed non-significant (p ≤ 0.05) variation under the influence of 0.1 and 0.3% borax. The EjSS2 significantly (p ≤ 0.05) upregulated under the influence of 0.1% borax by 2.14-fold. The maximum expression of EjSS3 was recorded in the loquats treated with 0.2% borax. Foliar application of B significantly (p ≤ 0.05) reduced the expression of EjSS4 as compared to control. Conversely, EjSS5 was found upregulated under the influence of B. The maximum relative expression of EjSS5 was recorded in the loquat treated with 0.2% borax.
Among B treatments, 0.2% borax significantly (p ≤ 0.05) upregulated the expressions of EjHK1-3. The expressions of EjHK1 and EjHK2 remained unchanged with B treatments except when loquats received 0.20% borax. While, in the case of EjHK3, 0.1% borax also upregulated the expression by 41.9%.
The relative expression level of EjFK1 was significantly (p ≤ 0.05) increased with the foliar application of B. The EjFK1 was maximally upregulated under the influence of 0.2% borax (by 2.41-fold). Similarly, in the case of EjFK2, the maximum transcript level was observed in the loquats treated with 0.2% borax (3.43). The borax application significantly (p ≤ 0.05) upregulated EjFK3 when applied at the concentration of 0.10% and reduced with increase in its concentration. Among B treatments, 0.2% borax maximally upregulated the EjFK4 by 2.23-fold. Although the maximum expressions of EjFK5 and EjFK6 were recorded in the loquats treated with 0.2% borax, their levels remained upregulated with all B treatments (Figure 7).
Expression profiling of malic acid metabolism-related genes
The expression patterns of core genes i.e., EjPEPC, EjNAD(P)ME and EjNAD-MDH encoding key enzymes i.e., PEPC, NADP-ME and NAD-MDP responsible for the malic acid metabolism in fruit pulp of loquat were studied (Figure 8). The expression of EjPEPC1 found significantly decreased with the foliar application of 0.2-0.3% borax. The loquats treated with 0.1-0.2% borax exhibited downregulated expression of EjPEPC2. EjPEPC2 was minimally expressed under the influence of 0.1 and 0.2% borax, which was ≥40% lower than that of control. The EjPEPC3 also exhibited maximum downregulation under the influence of 0.2% borax.
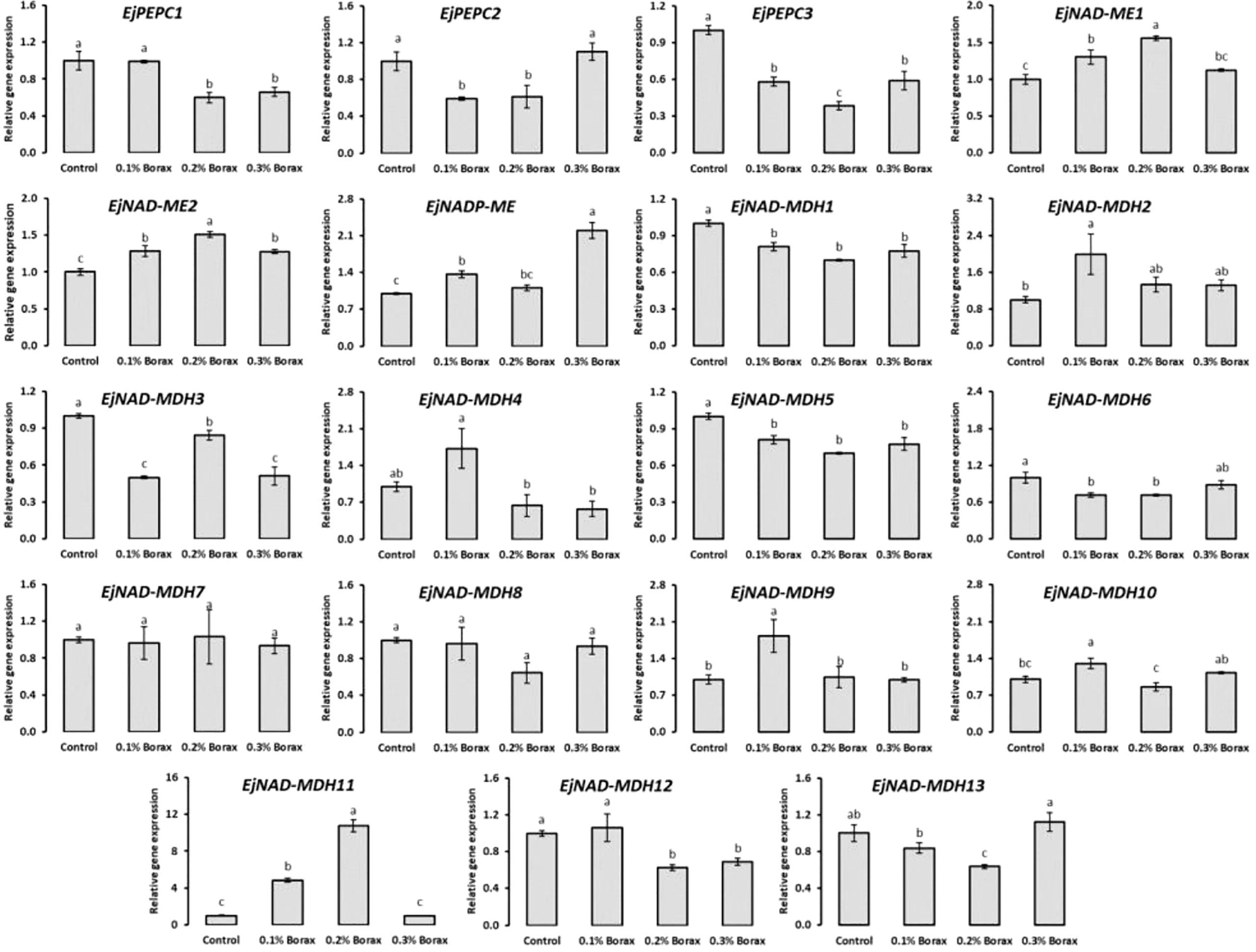
Figure 8 The expression profiling of core genes involved in malic acid metabolism of loquat as influenced by the foliar application of B. Same letters indicate non-significant difference among treatments according to Fisher’s least significant difference (LSD) test, when p ≤ 0.05. Vertical bars indicate mean ± standard error (4 biological and 3 technical replicates).
The relative expression patterns of EjNAD(P)-ME genes increased with the foliar application of B. Among B treatments, 0.1-0.2% borax significantly (p ≤ 0.05) upregulated the expressions of EjNAD-ME1. Specifically, the maximum expression of EjNAD-ME1 was recorded in the loquats treated with 0.2% borax, which were 1.56-fold higher than that of untreated loquats. Similarly, 0.1-0.3% borax significantly (p ≤ 0.05) improved the expression of EjNAD-ME2 in fruit pulp. The expression of EjNADP-ME remained unchanged with B treatments except when loquats received 0.1 and 0.3% borax. Its maximum expression was recorded under the influence of 0.3% borax which was 2.20-fold higher than that of control loquats.
The expression of EjNAD-MDH1 decreased with the application of borax, regardless of its concentration. The lowest EjNAD-MDH1 transcript was recorded in the loquats treated with 0.2% borax. EjNAD-MDH2 was maximally expressed under the influence of 0.1% borax, which was 2-fold higher than that of control. The EjNAD-MDH3 exhibited downregulation under the influence of 0.1-0.3% borax. The relative expression pattern of EjNAD-MDH4 comparatively increased with the foliar application of 0.1% borax, while remained unchanged under the influence of other treatments. The expression of EjNAD-MDH5 significantly reduced with the foliar application of B, regardless of its concentration applied. The expression of EjNAD-MDH6 reduced with the application of 0.1-0.2% borax. The minimum expression of EjNAD-MDH6 was observed in the loquats treated with 0.2% borax, which was 29% less than that of control. The relative expression pattern of EjNAD-MDH7 and EjNAD-MDH8 remained unchanged under the influence of B treatments. The EjNAD-MDH9 and EjNAD-MDH10 exhibited its upregulated expression only under the influence of 0.1% borax. The 0.1-0.2% borax significantly (p ≤ 0.05) improved the expressions of EjNAD-MDH11. Its maximum expression level was recorded in the loquats treated with 0.2% borax, which was 10.75-fold higher than control. The expression of EjNAD-MDH12 significantly (p ≤ 0.05) reduced under the influence of 0.2-0.3% borax. The EjNAD-MDH13 was significantly downregulated in fruit pulp of loquat with the foliar application of 0.2% borax (Figure 8).
Correlation analysis
Principal component analysis (PCA) was conducted to delineate concentration-dependent effects of B on basic fruit quality variables (i.e., fruit weight, size, total soluble solids, total titratable acidity and fruit juice pH), soluble sugars (i.e., fructose, glucose and sucrose), organic acids (i.e., fumaric acid, ascorbic acid, malic acid, cis-aconitic acid and acetic acid), key enzymes related to soluble sugars (i.e., SPS, SS, HK and FK) and malic acid metabolism (i.e., PEPC, NADP-ME and NAD-MDH), and relative expression levels of sugar-acid metabolic pathway genes (Figure 9A). Based on the highest squared cosine values corresponding to factors F1 or F2, measured attributes were clustered around B treatments. Factor F1, covering 64.18% variability in data (eigenvalue 38.51), showed clustering of fruit weight, fruit length, fruit diameter, total soluble solids, sugar-acid ratio, fruit fructose, fruit glucose, ascorbic acid, activity of SPS, HK, FK, NADP-ME, expression of EjSPS1-4, EjSS3, EjHK1-3, EjFK1, EjFK2, EjFK4, EjFK6, EjNAD-MDH11, and EjNAD-MDH13 with 0.2% borax suggesting its positive influence on these parameters. While, the clustering in opposite quadrant exhibited negative association of 0.2% borax with aforementioned variables. Second factor, covering 19.28% variability in data (eigenvalue 11.566), showed clustering of cis-aconitic acid, EjNAD-MDH2, EjNAD-MDH4, EjNAD-MDH9, EjNAD-MDH10, EjSS2, and EjFK3 with 0.1% borax. The presence of EjSS4 and EjNAD-MDH3 in opposite quadrant indicated the negative association of 0.1% borax with these parameters. Third factor of PCA (not shown), covering 16.55% variability in data (eigenvalue 9.927), showed clustering of pH, fruit sucrose, fumaric acid, EjNADP-ME, and EjNAD-MDH7 with 0.3% borax. Thus, principal component analysis helped to delineate individual roles of B concentrations in regulating sugar-acid metabolism of loquat (Figure 9A).
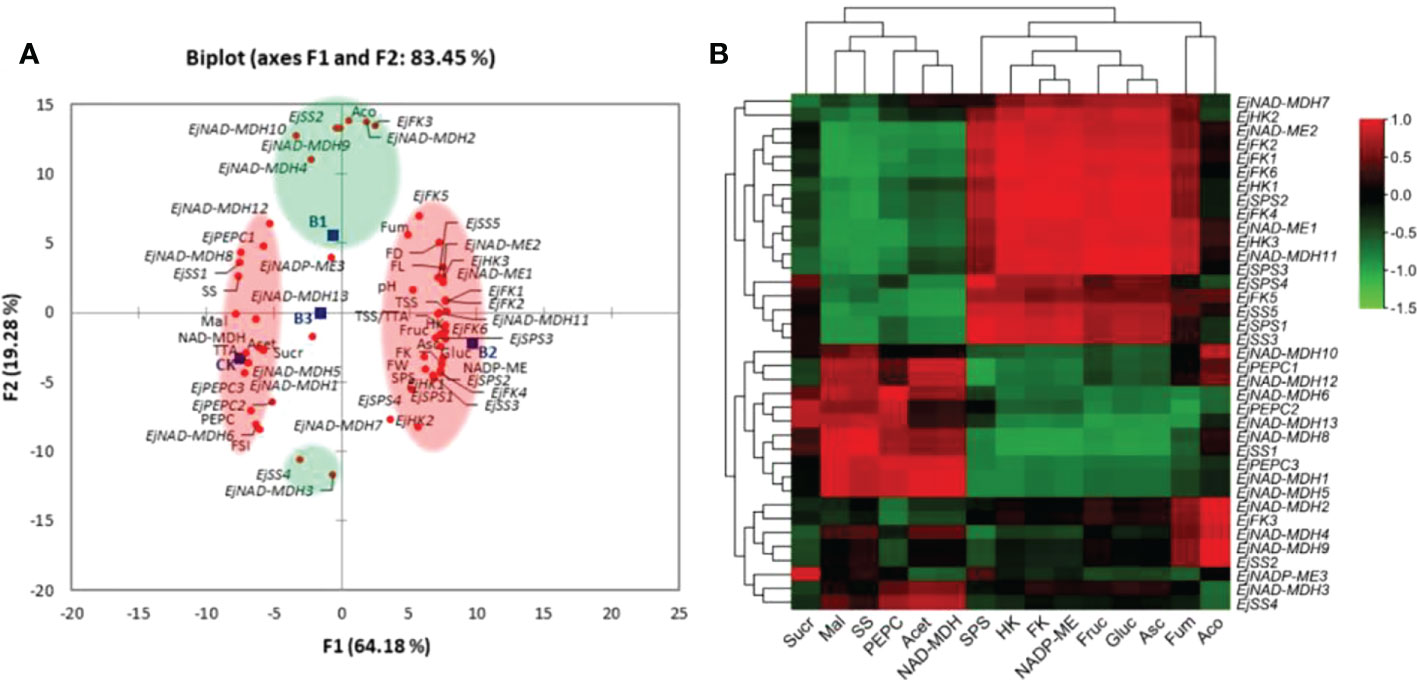
Figure 9 (A) Principal component analysis (PCA)among B treatments and sugar-acid attributes of loquat. Clustering of B treatments and measured attributes into groups (coloured circles) is based on their highest squared cosine values corresponding to the factor, F1 (red) or F2 (green). (B) Pearson (n) correlation analysis between “sugar-acid profile and key enzymes involved in their metabolism” and “relative expressions of related genes” in fruit pulp of loquat. CK, control; B1, 0.1% borax; B2, 0.2% borax; B3, 0.3% borax; FW, fruit weight; FL, fruit length; FD, fruit diameter; FSI, fruit shape index; TSS, soluble solid contents; TTA, total titratable acidity; TSS/TTA, sugar-acid ratio; pH, fruit juice pH; Fruc, fruit fructose; Gluc, fruit glucose; Sucr, fruit sucrose; SPS, sucrose-phosphate synthase; SS, sucrose synthase; HK, Hexokinase; FK, fructokinase.
The correlation between “relative expression levels of sugar-acid metabolism-related genes” and “sugar-acid profile and key enzymes related to their metabolism” was analysed (Figure 9B). The fructose and glucose were positively correlated with EjSPS1-4, EjSS5, EjHK1-3, EjFK1,2,4-6, EjNAD-ME1,2, and NAD-MDH7,11. The fruit sucrose content was negatively correlated with most of the studied genes except EjSPS4, EjPEPC2, EjNAD-ME3, and EjNAD-MDH6,12. The ascorbic acid was significantly (p ≤ 0.05) positively correlated with EjSPS1-3, EjSS5, EjHK1-3, EjFK1,2,4,6, EjNAD-ME1,2, and NAD-MDH7,11. The fruit malic acid content was significantly (p ≤ 0.05) positively correlated with EjSS1, EjPEPC3, EjNAD-MDH1, EjNAD-MDH5, EjNAD-MDH8 and EjNAD-MDH13, while significantly (p ≤ 0.05) negatively correlated with EjSPS1-3, EjSS5, EjHK1,3, EjFK1,2,4, EjNAD-ME1,2, and NAD-MDH11. The negative association of cis-aconitic acid was observed with the expression of EjSS4 and EjNAD-MDH3. A positive correlation was also found between acetic acid and EjPEPC3, EjNAD-MDH1 and EjNAD-MDH5. The enzymatic activity of SPS was positively correlated with EjSPS1-4, EjSS5, EjHK1-3, EjFK1,2,4-6, EjNAD-ME1,2, and NAD-MDH7,11, while SS only found significantly (p ≤ 0.01) and positively associated with EjSS1 and EjNAD-MDH8. The HK and FK activity significantly (p ≤ 0.05) and positively correlated with EjSPS1-4, EjSS5, EjHK1-3, EjFK1,2,4-6, EjNAD-ME1,2, and NAD-MDH7,11. The enzymatic activity of PEPC was positively correlated with EjSS1, EjPEPC2,3 and EjNAD-MDH1,5,6,8,13. The NADP-ME activity was found significantly (p ≤ 0.05) positively associated with EjSPS1-3, EjHK1, EjFK1,2,4,6 and NAD-MDH11, while negatively correlated with EjSS1 and EjNAD-MDH8. The NAD-MDH activity positively correlated with EjPEPC3, EjNAD-MDH1, EjNAD-MDH5 and EjNAD-MDH6 (Figure 9B).
Discussion
Soluble sugars
The quality of fruit is heavily influenced by soluble sugars and organic acids, the two main components of fruit flavor (Borsani et al., 2009). At the ripe fruit stage, the soluble sugar content of ‘Jiefangzhong’ loquat was increased to a maximum of 19 mg·ml-1 in the current investigation. Previous research has shown that the primary sugars that may be found in loquat fruits are sucrose, glucose, and fructose (Toker et al., 2013; Wei et al., 2017). When it comes to the amount of sugar that they contain, various loquat cultivars have varying degrees of variation. The majority of cultivars have a high concentration of sucrose, followed by fructose and glucose, although wild species do not have any sucrose (Liu et al., 2016). According to the findings of our study, the fructose level was found to be the highest among the soluble sugars that were examined.
In the present study, maximum fructose and glucose were recorded in the loquats treated with 0.2% borax. In a previously reported study, foliar spraying of B alone or in combination with Zn led to a large rise in the concentration of non-reducing sugar in fruit juice. On the other hand, these sprays led to a significant reduction in the concentration of reducing sugar and total sugar in pomegranate (Maity et al., 2021). After being sprayed with B, it was found that the content of sugar in papaya, mandarin orange, mango, and pomegranate fruits increased (Babu and Yadav, 2005; Anees et al., 2011; Davarpanah et al., 2016; Subedi et al., 2019). The effect of B on sugar concentration could be attributed to its role in photosynthesis, starch and nucleic acid metabolism, the transport of sugars, and carbohydrate metabolism (Maity et al., 2021).
During soluble sugars metabolism in plant cell, sucrose is cleaved into UDG-glucose and fructose by sucrose synthase (SS), or can be cleaved into glucose and fructose by invertases (Ghosh et al., 2013; Irfan et al., 2014; Chen et al., 2017). Fructokinases (FKs) and hexokinases (HKs) can phosphorylate free fructose with high substrate specificity and affinity (Renz and Stitt, 1993; Irfan et al., 2016; 2021; 2022). The cleavage of sucrose and the metabolism of sugar are crucial processes in the formation of healthy vascular tissue, and hence it is thought that fructose phosphorylation by FKs and HKs is required for these processes to take place German et al., 2003; Damari-Weissler et al., 2009; Kumar et al., 2016; 2019; Kumari et al., 2022). Both sucrose phosphate synthase (SPS) and sucrose synthase (SS) are important prerequisites in the biochemical process that results in the formation of sucrose. The synthesis of 6-phosphate sucrose from UDP-glucose and 6-phosphate fructose is facilitated by the presence of SPS (Stein and Granot, 2019), whereas SS converts sucrose into UDP-glucose and fructose (Ruan, 2014) (Figure 10A). The majority of the SS proteins may be found in either the cytosol or the plasma membrane, but some can be localized in the vacuole, the cell wall, or the mitochondria (Stein and Granot, 2019). In Arabidopsis thaliana and Malus domestica, there are 4 and 6 SPS genes, respectively (Langenkämper et al., 2002; Li et al., 2012). The number of SS genes varies greatly among plant species. There are 6, 8, 12, and 14 SS genes in Arabidopsis, carrot (Daucus carota), soybean (Glycine max) and tobacco (Nicotiana tabacum), respectively (Wang et al., 2015; Xu et al., 2019). In Chinese pear, it is reported that there were thirty SS genes (Abdullah et al., 2018).
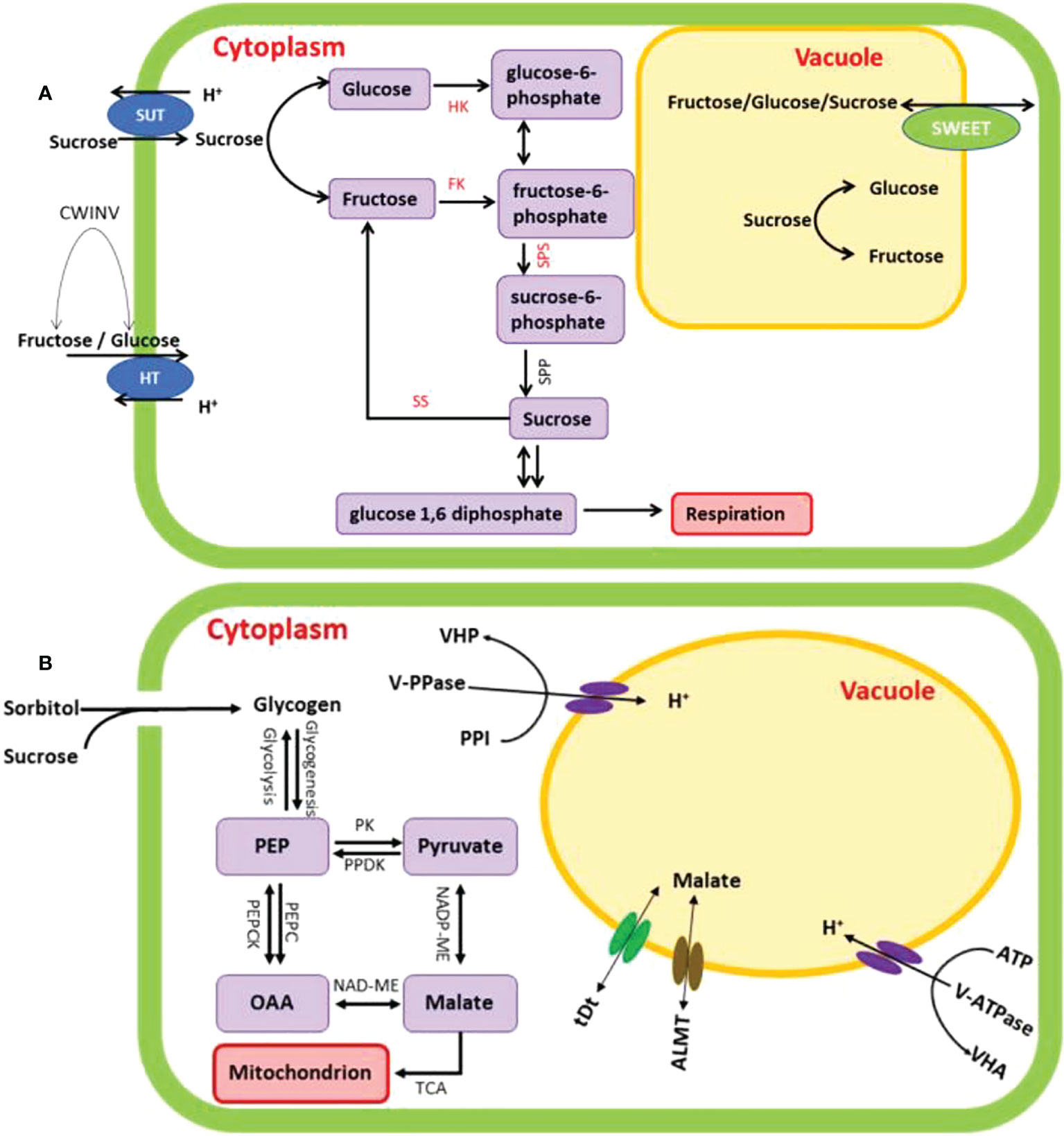
Figure 10 Proposed schematic representation of soluble sugars (A) and malic acid (B) metabolic pathway in fruit cell. SUT, sucrose transporters; CWINT, cell wall invertase; HT - hexose transporters; HK, hexokinase; FK, fructokinase; SPS, sucrose phosphate synthase; SS, sucrose synthase; SPP, sucrose-phosphate phosphatase; SWEET, sugars will eventually be exported transporter; PK, phosphokinase; PPDK, pyruvate phosphate dikinase; PEPC, phosphoenolpyruvate carboxylase; NADP-ME, NADP-dependent malic enzyme; NAD-MDH, NAD-malate dehydrogenase; ALMT, aluminium activated malate transporter; tDt, terminal deoxynucleotidyl transferase.
The expressions of most important genes involved in sugar metabolism and accumulation were studied under the influence of B treatments. The SPS genes showed relative high expression level, while sucrose content was very low. The high transcript level, indicated a negative correlation between sucrose accumulation and SPS activity. A possible reason for this discrepancy is that sucrose is the major soluble sugar in tomato (Dali et al., 1992) and watermelon (Liu et al., 2013; Zhu et al., 2017) but the ‘Jiefangzhong’ loquat mainly showed the accumulation of fructose (Li et al., 2015a).
The enzyme known as sucrose synthase (SS) is also capable of catalyzing the reversible process of the production of sucrose. In peaches and pears, the SS activity has a positive correlation with the amount of sucrose present, but in strawberries and papaya, the correlation is negative (Moriguchi et al., 1992; Lo Bianco et al., 2000; Zhou and Paull, 2001; Basson et al., 2010). It has been hypothesised that when there is a high quantity of glucose and fructose, the SS may be able to be down-regulated in order to lower the enzyme activity (Stein and Granot, 2019). In this study, the EjSS1-4 showed negative correlation with sucrose content. The EjSS3,5 showed positive correlation with glucose and fructose. Similar to sucrose, EjSS2,4 showed negative correlation with glucose and fructose. These results indicated that EjSS3 or EjSS5 might were the candidate genes related to fructose and glucose synthesis, while EjSS2,4 for sucrose degradation at fruit ripening stage.
The glucose and fructose content, activities of their metabolism related enzymes and expressions EjHK and EjFK genes increased in loquats with the application of B. All EjHK and EjFK genes exhibited significantly positive correlation with fructose and glucose contents as well as SPS, HK and FK activity of loquat fruits. However, the expression level of EjFK3 showed negative correlation with glucose and fructose contents, suggesting that EjFK3 may play a crucial role in regulating the accumulation of glucose through post-transcriptional level (Jiang et al., 2019).
Organic acids
Aliphatic carboxylic acids, sugar-derived organic acids, and phenolic acids are the three categories that may be used to classify fruit organic acids (Ren et al., 2017). The majority of the organic acids found in loquat fruits are aliphatic carboxylic acids. Some examples of these types of acids are fumaric acid, ascorbic acid, and malic acid (Kumar et al., 2017; Batista-Silva et al., 2018; Sáenz-Galindo et al., 2018). In the present study, the optimized UPLC-MS method was used to detect fumaric acid, ascorbic acid, malic acid, cis-aconitic acid and acetic acid from the fruit pulp of loquat under the influence of foliar applied B. Among them, the content of malic acid accounted for about 70%-80% of the total acid. These results are in line with the previous findings about organic acid profile of loquat (2009; Chen et al., 2007; Yang et al., 2021). The composition and content of organic acids in loquat fruits have genetic variation (Famiani et al., 2015), and the differences are also manifested between different varieties (Chen et al., 2009). In present study, the cultivar “Jiefangzhong” was used as plant material which is already reported as high-acid cultivar (Chen et al., 2007; 2009; Ali et al., 2021a; Yang et al., 2021).
Fruits with intermediate acidity tend to be more palatable, but increasing acid content can often lower the quality of the fruit (Zhang et al., 2021). Organic acids build throughout fruit development and are utilised as respiratory substrates as the fruit ripens (Raza et al., 2022). The balance of organic acid production, membrane transit, and breakdown or use determines the ultimate organic acid content in ripened fruits (Sadka et al., 2000; Cercós et al., 2006; Sharma et al., 2022). In this process, malic acid metabolism-related enzymes including phosphoenolpyruvate carboxylase (PEPC), NADP – dependent malic enzyme (NADP-ME), and NAD-malate dehydrogenase (NAD-MDH) may potentially play a role in fruit malic acid biosynthesis and degradation (Chen et al., 2009; Wu and Chen, 2016). The first step in the production of malic acid begins in the cytosol with phosphoenolpyruvate (PEP), which is then actively transported into the mitochondria and transformed to oxaloacetate (OAA) by phosphoenolpyruvate carboxylase (PEPC) (Tayal et al., 2022). Then, NAD-malate dehydrogenase (NAD-MDH) catalyses the condensation of OAA to produce malic acid. The cytosolic enzyme NADP - dependent malic enzyme (NADP-ME) catalyses the conversion of pyruvate to malic acid (Chen et al., 2009; Ma et al., 2019). According to these metabolic routes, malic acid is mostly synthesised by the catalytic activities of PEPC, NADP-ME and NAD-MDH (Wu and Chen, 2016; Zhou et al., 2019) (Figure 10B).
Because of the relevance and high quantity of malic acid in fruits, great progress has been made in determining the metabolism of malic acid in fruits. NAD-MDH activity is inversely linked to NADP-ME activity (Chen et al., 2009), indicating that NAD-MDH and NADP-ME may both play essential roles in malate production and degradation, respectively. Yang et al. (Yang et al., 2011) also cloned the genes encoding EjPEPC, EjNADP-ME, and EjcyNAD-MDH, and they discovered that the transcript level of EjNADP-ME in the high-acid cultivar was considerably greater than that in the low-acid cultivar, but EjNADP-ME and EjmNAD-MDH expression patterns were comparable in both cultivars, however EjPEPC and EjcyNAD-MAD expression patterns were different, suggesting that the expression of these genes may be crucial in controlling malic acid production in loquat fruit.
Due to coenzyme specificity, subcellular localization, and biochemical function, NAD-malic enzyme (NAD-ME) contributes 70-80% to malic acid accumulation (Rao and Dixon, 2016). In a recent study, it has been proved that NAD-cytMDH is a key gene that regulates the acidity of peach fruits (Etienne et al., 2002). The overexpression of MdNAD-ME genes significantly increased the content of malic acid in apple callus (Drincovich et al., 2001). It has been reported earlier that NADP-ME catalyzes the carboxylation of pyruvate and fixes CO2 to produce malic acid in grapes (Sweetman et al., 2009). NADP-ME plays a major role in the degradation of malic acid in the cytoplasm, such as the content of organic acids in apple were negatively correlated with NADP-ME activity (Yao et al., 2009). The change in the activity of NAD-ME is consistent with the biosynthesis of malic acid, the increase in fruit respiration, and the gradual decrease of malic acid during fruit ripening (Khan et al., 2018). During storage of climacteric fruits e.g., apple, pear, banana, etc. the respiration increases, accompanied by accelerated degradation of malic acid (Farrar et al., 2000; Ruan, 2014). In the current study, the fruit malic acid content was significantly and positively correlated with EjSS1, EjPEPC3, EjNAD-MDH1, EjNAD-MDH5, EjNAD-MDH8 and EjNAD-MDH13, while significantly (p ≤ 0.05) negatively correlated with EjSPS1-3, EjSS5, EjHK1,3, EjFK1,2,4, EjNAD-ME1,2, and NAD-MDH11.
Conclusions
The results of the current study suggest that fructose and malic acid are the predominant sugar and acid in fruit pulp of loquat, respectively. Among B treatments, 0.2% borax was the promising treatment to enhance soluble sugars and reduce malic acid concentration in fruit pulp of loquat. Boron treatments remarkably improved the soluble sugars content by regulating the activities of SPS, SS, HK and FK enzymes, and altering the expressions of related genes. The combined activity of many enzymes, including PEPC, NADP-ME, and NAD-MDH, was responsible for controlling the dynamics of the malic acid concentrations evaluated in the present investigation. Correlation analysis suggested that NAD-MDH played a vital role in the decrease of malic acid. These findings not only elucidated previously unknown aspects of the metabolism of soluble sugars and organic acids, but they also provide a significant resource for prospective studies on the application of molecular breeding techniques to loquat fruit.
Data availability statement
The original contributions presented in the study are included in the article/Supplementary Material. Further inquiries can be directed to the corresponding authors.
Author contributions
Conceptualization, MA, YH, and FC; Data curation, MA and RA; Funding acquisition, YH; Methodology, MA, AY, and FC; Supervision, YH and FC; Writing – original draft, MA; Writing – review & editing, RA, RR, SEj, SA, SEr, YH, and FC. All authors contributed to the article and approved the submitted version.
Funding
This research was funded by Ministerial and Provincial Joint Innovation Centre for Safety Production of Cross-Strait Crops, Fujian Agriculture and Forestry University, Fuzhou 350002, China.
Conflict of interest
The authors declare that the research was conducted in the absence of any commercial or financial relationships that could be construed as a potential conflict of interest.
Publisher’s note
All claims expressed in this article are solely those of the authors and do not necessarily represent those of their affiliated organizations, or those of the publisher, the editors and the reviewers. Any product that may be evaluated in this article, or claim that may be made by its manufacturer, is not guaranteed or endorsed by the publisher.
Supplementary material
The Supplementary Material for this article can be found online at: https://www.frontiersin.org/articles/10.3389/fpls.2022.1039360/full#supplementary-material
References
Abdullah, M., Cao, Y., Cheng, X., Meng, D., Chen, Y., Shakoor, A., et al. (2018). The sucrose synthase gene family in chinese pear (Pyrus bretschneideri rehd.): structure, expression, and evolution. Molecules 23, 1144. doi: 10.3390/molecules23051144
Ali, M. M., Alam, S. M., Anwar, R., Ali, S., Shi, M., Liang, D., et al. (2021a). Genome-wide identification, characterization and expression profiling of aluminum-activated malate transporters in Eriobotrya japonica lindl. Horticulturae 7, 441. doi: 10.3390/horticulturae7110441
Ali, M. M., Anwar, R., Shafique, M. W., Yousef, A. F., Chen, F. (2021b). Exogenous application of mg, zn and b influences phyto-nutritional composition of leaves and fruits of loquat (Eriobotrya japonica lindl.). Agronomy 11, 224. doi: 10.3390/agronomy11020224
Ali, M., Ezz, T., Abd El-Megeed, N., Flefil, M. (2019). Effect of foliar application with some micronutrients on ‘Florida prince’ cultivar peach tree. J. Adv. Agric. Res. 24, 410–425. doi: 10.21608/jalexu.2019.163473
Ali, M. M., Li, B., Zhi, C., Yousef, A. F., Chen, F. (2021c). Foliar-supplied molybdenum improves phyto-nutritional composition of leaves and fruits of loquat (Eriobotrya japonica lindl.). Agronomy 11, 892. doi: 10.3390/agronomy11050892
Ali, M. M., Rizwan, H. M., Yousef, A. F., Zhi, C., Chen, F. (2021d). Analysis of toxic elements in leaves and fruits of loquat by inductively coupled plasma-mass spectrometry (ICP-MS). Acta Sci. Pol. Hortorum Cultus 20, 33–42. doi: 10.24326/asphc.2021.5.4
Anees, M., Tahir, F. M., Shahzad, J., Mahmood, N., Genetics, M. (2011). Effect of foliar application of micronutrients on the quality of mango (Mangifera indica l .) cv . dusehri fruit. Mycopath 9, 25–28.
Babu, K. D., Yadav, D. S. (2005). Foliar spray of micronutrients for yield and quality improvement in khasi mandarin (Citrus reticulata blanco.). Indian J. Hortic. 62, 280–281.
Badenes, M. L., Canyamas, T., Romero, C., Soriano, J. M., Martínez, J., Llácer, G. (2003). Genetic diversity in european collection of loquat (Eriobotrya japonica lindl.). Acta Hortic. 620, 169–174. doi: 10.17660/ActaHortic.2003.620.17
Basson, C. E., Groenewald, J.-H., Kossmann, J., Cronjé, C., Bauer, R. (2010). Sugar and acid-related quality attributes and enzyme activities in strawberry fruits: Invertase is the main sucrose hydrolysing enzyme. Food Chem. 121, 1156–1162. doi: 10.1016/j.foodchem.2010.01.064
Batista-Silva, W., Nascimento, V. L., Medeiros, D. B., Nunes-Nesi, A., Ribeiro, D. M., Zsögön, A., et al. (2018). Modifications in organic acid profiles during fruit development and ripening: correlation or causation? Front. Plant Sci. 9. doi: 10.3389/fpls.2018.01689
Bermejo, A., Cano, A. (2012). Analysis of nutritional constituents in twenty citrus cultivars from the mediterranean area at different stages of ripening. Food Nutr. Sci. 03, 639–650. doi: 10.4236/fns.2012.35088
Borsani, J., Budde, C. O., Porrini, L., Lauxmann, M. A., Lombardo, V. A., Murray, R., et al. (2009). Carbon metabolism of peach fruit after harvest: changes in enzymes involved in organic acid and sugar level modifications. J. Exp. Bot. 60, 1823–1837. doi: 10.1093/jxb/erp055
Broadley, M., Brown, P., Cakmak, I., Rengel, Z., Zhao, F. (2012). “Function of nutrients,” in Marschner’s mineral nutrition of higher plants (Amsterdam, Netherlands:Elsevier), 191–248. doi: 10.1016/B978-0-12-384905-2.00007-8
Brown, P. H., Bellaloui, N., Wimmer, M. A., Bassil, E. S., Ruiz, J., Hu, H., et al. (2002). Boron in plant biology. Plant Biol. 4, 205–223. doi: 10.1055/s-2002-25740
Cercós, M., Soler, G., Iglesias, D. J., Gadea, J., Forment, J., Talón, M. (2006). Global analysis of gene expression during development and ripening of citrus fruit flesh. a proposed mechanism for citric acid utilization. Plant Mol. Biol. 62, 513–527. doi: 10.1007/s11103-006-9037-7
Chen, F. X., Lin, Q. L., Liu, X. H., She, W. Q., Chen, L. S., Wu, D. Y. (2007). Effects of magnetic field and fertilizations on organic acids in loquat fruit. Acta Hortic. 750, 355–360. doi: 10.17660/ActaHortic.2007.750.56
Chen, F., Liu, X., Chen, L. (2009). Developmental changes in pulp organic acid concentration and activities of acid-metabolising enzymes during the fruit development of two loquat (Eriobotrya japonica lindl.) cultivars differing in fruit acidity. Food Chem. 114, 657–664. doi: 10.1016/j.foodchem.2008.10.003
Chen, Y., Zhang, Q., Hu, W., Zhang, X., Wang, L., Hua, X., et al. (2017). Evolution and expression of the fructokinase gene family in saccharum. BMC Genomics 18, 197. doi: 10.1186/s12864-017-3535-7
Dali, N., Michaud, D., Yelle, S. (1992). Evidence for the involvement of sucrose phosphate synthase in the pathway of sugar accumulation in sucrose-accumulating tomato fruits. Plant Physiol. 99, 434–438. doi: 10.1104/pp.99.2.434
Damari-Weissler, H., Rachamilevitch, S., Aloni, R., German, M. A., Cohen, S., Zwieniecki, M. A., et al. (2009). LeFRK2 is required for phloem and xylem differentiation and the transport of both sugar and water. Planta 230, 795–805. doi: 10.1007/s00425-009-0985-4
Davarpanah, S., Tehranifar, A., Davarynejad, G., Abadía, J., Khorasani, R. (2016). Effects of foliar applications of zinc and boron nano-fertilizers on pomegranate (Punica granatum cv. ardestani) fruit yield and quality. Sci. Hortic. (Amsterdam) 210, 57–64. doi: 10.1016/j.scienta.2016.07.003
de Oliveira, R. H., Dias Milanez, C. R., Moraes-Dallaqua, M. A., Rosolem, C. A. (2006). Boron deficiency inhibits petiole and peduncle cell development and reduces growth of cotton. J. Plant Nutr. 29, 2035–2048. doi: 10.1080/01904160600932617
De Rossi, S., Di Marco, G., Bruno, L., Gismondi, A., Canini, A. (2021). Investigating the drought and salinity effect on the redox components of Sulla coronaria (L.) medik. Antioxidants 10, 1048. doi: 10.3390/antiox10071048
Drincovich, M. F., Casati, P., Andreo, C. S. (2001). NADP-malic enzyme from plants: a ubiquitous enzyme involved in different metabolic pathways. FEBS Lett. 490, 1–6. doi: 10.1016/S0014-5793(00)02331-0
El-Sheikh, M. H., Khafgy, S. A. A., Zaied, S. S. (2007). Effect of foliar application with some micronutrients on leaf mineral content, yield and fruit quality of Florida prince desert red peach trees. J. Agric. Biol. Sci. 3, 309–315.
Engels, C., Kirkby, E., White, P. (2012). “Mineral nutrition, yield and source–sink relationships,” in Marschner’s mineral nutrition of higher plants (Amsterdam, Netherlands:Elsevier), 85–133. doi: 10.1016/B978-0-12-384905-2.00005-4
Etienne, C., Moing, A., Dirlewanger, E., Raymond, P., Monet, R., Rothan, C. (2002). Isolation and characterization of six peach cDNAs encoding key proteins in organic acid metabolism and solute accumulation: involvement in regulating peach fruit acidity. Physiol. Plant 114, 259–270. doi: 10.1034/j.1399-3054.2002.1140212.x
Famiani, F., Battistelli, A., Moscatello, S., Cruz-Castillo, J. G., Walker, R. P. (2015). The organic acids that are accumulated in the flesh of fruits: occurrence, metabolism and factors affecting their contents – a review. Rev. Chapingo Ser. Hortic. XXI, 97–128. doi: 10.5154/r.rchsh.2015.01.004
Farrar, J., Pollock, C., Gallagher, J. (2000). Sucrose and the integration of metabolism in vascular plants. Plant Sci. 154, 1–11. doi: 10.1016/S0168-9452(99)00260-5
Fedorova, E. N., Varlamova, D. O., Kivero, A. D., Guk, K. D., Ptitsyn, L. R. (2020). Ultra-performance liquid chromatography (UPLC) for the determination of organic acids – the intermediates of branched-chain amino acid biosynthesis in Escherichia coli strains. J. Liq. Chromatogr. Relat. Technol. 43, 844–851. doi: 10.1080/10826076.2020.1832894
Gan, X., Jing, Y., Shahid, M. Q., He, Y., Baloch, F. S., Lin, S., et al. (2020). Identification, phylogenetic analysis, and expression patterns of the SAUR gene family in loquat (Eriobotrya japonica). Turkish J. Agric. For. 44, 15–23. doi: 10.3906/tar-1810-98
German, M. A., Dai, N., Matsevitz, T., Hanael, R., Petreikov, M., Bernstein, N., et al. (2003). Suppression of fructokinase encoded by LeFRK2 in tomato stem inhibits growth and causes wilting of young leaves. Plant J. 34, 837–846. doi: 10.1046/j.1365-313X.2003.01765.x
Ghosh, S., Singh, U. K., Meli, V. S., Kumar, V., Kumar, A., Irfan, M., et al. (2013). Induction of senescence and identification of differentially expressed genes in tomato in response to monoterpene. PloS One 8, e76029. doi: 10.1371/journal.pone.0076029
Goldbach, H. E., Yu, Q., Wingender, R., Schulz, M., Wimmer, M., Findeklee, P., et al. (2001). Rapid response reactions of roots to boron deprivation. J. Plant Nutr. Soil Sci. 164, 173–181. doi: 10.1002/1522-2624(200104)164:2<173::AID-JPLN173>3.0.CO;2-F
Hortwitz, W. (1960). Official and tentative methods of analysis. Assoc. Off. Anal. Chem. Washington U.S.A.
Irfan, M., Ghosh, S., Kumar, V., Chakraborty, N., Chakraborty, S., Datta, A. (2014). Insights into transcriptional regulation of -D-N-acetylhexosaminidase, an n-glycan-processing enzyme involved in ripening-associated fruit softening. J. Exp. Bot. 65, 5835–5848. doi: 10.1093/jxb/eru324
Irfan, M., Ghosh, S., Meli, V. S., Kumar, A., Kumar, V., Chakraborty, N., et al. (2016). Fruit ripening regulation of α-mannosidase expression by the MADS box transcription factor RIPENING INHIBITOR and ethylene. Front. Plant Sci. 7. doi: 10.3389/fpls.2016.00010
Irfan, M., Kumar, P., Ahmad, I., Datta, A. (2021). Unraveling the role of tomato bcl-2-associated athanogene (BAG) proteins during abiotic stress response and fruit ripening. Sci. Rep. 11, 21734. doi: 10.1038/s41598-021-01185-7
Irfan, M., Kumar, P., Kumar, V., Datta, A. (2022). Fruit ripening specific expression of β-D-N-acetylhexosaminidase (β-hex) gene in tomato is transcriptionally regulated by ethylene response factor SlERF.E4. Plant Sci. 323, 111380. doi: 10.1016/j.plantsci.2022.111380
Jiang, C.-C., Fang, Z.-Z., Zhou, D.-R., Pan, S.-L., Ye, X.-F. (2019). Changes in secondary metabolites, organic acids and soluble sugars during the development of plum fruit cv. ‘Furongli’ (Prunus salicina lindl). J. Sci. Food Agric. 99, 1010–1019. doi: 10.1002/jsfa.9265
Kakita, H., Kamishima, H., Komiya, K., Kato, Y. (2002). Simultaneous analysis of monosaccharides and oligosaccharides by high-performance liquid chromatography with postcolumn fluorescence derivatization. J. Chromatogr. A 961, 77–82. doi: 10.1016/S0021-9673(02)00655-6
Karadeniz, T., Șenyurt, M., Bak, T. (2012). Loquat as a source of nectar and pollen in the winter for beekeeping. Sci. Pap. - Ser. B Hortic. 56, 319–322. doi: 10.13140/RG.2.1.1796.6567
Khan, A. S., Singh, Z., Ali, S. (2018). “Postharvest biology and technology of plum,” in Postharvest biology and technology of temperate fruits (Cham: Springer International Publishing), 101–145. doi: 10.1007/978-3-319-76843-4_5
Kumar, V., Irfan, M., Datta, A. (2019). Manipulation of oxalate metabolism in plants for improving food quality and productivity. Phytochemistry 158, 103–109. doi: 10.1016/j.phytochem.2018.10.029
Kumar, V., Irfan, M., Ghosh, S., Chakraborty, N., Chakraborty, S., Datta, A. (2016). Fruit ripening mutants reveal cell metabolism and redox state during ripening. Protoplasma 253, 581–594. doi: 10.1007/s00709-015-0836-z
Kumari, C., Sharma, M., Kumar, V., Sharma, R., Kumar, V., Sharma, P., et al. (2022). Genome editing technology for genetic amelioration of fruits and vegetables for alleviating post-harvest loss. Bioengineering 9, 176. doi: 10.3390/bioengineering9040176
Kumar, V., Sharma, A., Bhardwaj, R., Thukral, A. K. (2017). Analysis of organic acids of tricarboxylic acid cycle in plants using GC-MS, and system modeling. J. Anal. Sci. Technol. 8, 20. doi: 10.1186/s40543-017-0129-6
Langenkämper, G., Fung, R. W. M., Newcomb, R. D., Atkinson, R. G., Gardner, R. C., MacRae, E. A. (2002). Sucrose phosphate synthase genes in plants belong to three different families. J. Mol. Evol. 54, 322–332. doi: 10.1007/s00239-001-0047-4
LaRue, R. G. (2020) Loquat fact sheet. fruit nut res. inf. center, univ. calif. Available at: http://fruitsandnuts.ucdavis.edu/dsadditions/Loquat_Fact_Sheet/ (Accessed January 30, 2020).
Li, M., Feng, F., Cheng, L. (2012). Expression patterns of genes involved in sugar metabolism and accumulation during apple fruit development. PloS One 7, e33055. doi: 10.1371/journal.pone.0033055
Li, S., Liu, X., Xie, X., Sun, C., Grierson, D., Yin, X., et al. (2015b). CrMYB73 , a PH -like gene, contributes to citric acid accumulation in citrus fruit. Sci. Hortic. (Amsterdam) 197, 212–217. doi: 10.1016/j.scienta.2015.09.037
Liu, J., Guo, S., He, H., Zhang, H., Gong, G., Ren, Y., et al. (2013). Dynamic characteristics of sugar accumulation and related enzyme activities in sweet and non-sweet watermelon fruits. Acta Physiol. Plant 35, 3213–3222. doi: 10.1007/s11738-013-1356-0
Liu, S., Liu, Y., Liu, N., Zhang, Y., Zhang, Q., Xu, M., et al. (2016). Sugar and organic acid components in fruits of plum cultivar resources of genus prunus. Sci. Agric. Sin. 49, 3188–3198. doi: 10.3864/j.issn.0578-1752.2016.16.012
Livak, K. J., Schmittgen, T. D. (2001). Analysis of relative gene expression data using real-time quantitative pcr and the 2–ΔΔCT method. Methods 25, 402–408. doi: 10.1006/meth.2001.1262
Li, P., Wu, W., Chen, F., Liu, X., Lin, Y., Chen, J. (2015a). Prunus salicina ‘Crown’, a yellow-fruited chinese plum. HortScience 50, 1822–1824. doi: 10.21273/HORTSCI.50.12.1822
Lo Bianco, R., Rieger, M., Sung, S.-J. S. (2000). Effect of drought on sorbitol and sucrose metabolism in sinks and sources of peach. Physiol. Plant 108, 71–78. doi: 10.1034/j.1399-3054.2000.108001071.x
Maity, A., Gaikwad, N., Babu, K. D., Sarkar, A., Patil, P. (2021). Impact of zinc and boron foliar application on fruit yield, nutritional quality and oil content of three pomegranate (Punica granatum l.) cultivars. J. Plant Nutr. 44, 1841–1852. doi: 10.1080/01904167.2021.1884711
Ma, B., Liao, L., Fang, T., Peng, Q., Ogutu, C., Zhou, H., et al. (2019). A Ma10 gene encoding p-type ATPase is involved in fruit organic acid accumulation in apple. Plant Biotechnol. J. 17, 674–686. doi: 10.1111/pbi.13007
Moriguchi, T., Abe, K., Sanada, T., Yamaki, S. (1992). Levels and role of sucrose synthase, sucrose-phosphate synthase, and acid invertase in sucrose accumulation in fruit of asian pear. J. Am. Soc Hortic. Sci. 117, 274–278. doi: 10.21273/JASHS.117.2.274
Munhoz, C. F., Santos, A. A., Arenhart, R. A., Santini, L., Monteiro-Vitorello, C. B., Vieira, M. L. C. (2015). Analysis of plant gene expression during passion fruit- Xanthomonas axonopodis interaction implicates lipoxygenase 2 in host defence. Ann. Appl. Biol. 167, 135–155. doi: 10.1111/aab.12215
Nour, V., Trandafir, I., Ionica, M. E. (2010). Organic acid analysis in different citrus juices under reversed phase conditions. Not. Bot. Horti. Agrobot. Cluj-Napoca 38, 44–48. doi: 10.15835/nbha3814569
Pan, T., Ali, M. M., Gong, J., She, W., Pan, D., Guo, Z., et al. (2021). Fruit physiology and sugar-acid profile of 24 pomelo (Citrus grandis (L.) osbeck) cultivars grown in subtropical region of china. Agronomy 11, 2393. doi: 10.3390/agronomy11122393
Pancera, S. M., Gliemann, H., Schimmel, T., Petri, D. F. S. (2006). Adsorption behavior and activity of hexokinase. J. Colloid Interface Sci. 302, 417–423. doi: 10.1016/j.jcis.2006.06.066
Papagianni, M., Avramidis, N. (2011). Lactococcus lactis as a cell factory: A twofold increase in phosphofructokinase activity results in a proportional increase in specific rates of glucose uptake and lactate formation. Enzyme Microb. Technol. 49, 197–202. doi: 10.1016/j.enzmictec.2011.05.002
Rao, X., Dixon, R. A. (2016). The differences between NAD-ME and NADP-ME subtypes of C4 photosynthesis: more than decarboxylating enzymes. Front. Plant Sci. 7. doi: 10.3389/fpls.2016.01525
Raza, A., Salehi, H., Rahman, M. A., Zahid, Z., Madadkar, H. M., Najafi-Kakavand, S., et al. (2022). Plant hormones and neurotransmitter interactions mediate antioxidant defenses under induced oxidative stress in plants. Front. Plant Sci. 13. doi: 10.3389/fpls.2022.961872
Ren, J.-N., Tai, Y.-N., Dong, M., Shao, J.-H., Yang, S.-Z., Pan, S.-Y., et al. (2015). Characterisation of free and bound volatile compounds from six different varieties of citrus fruits. Food Chem. 185, 25–32. doi: 10.1016/j.foodchem.2015.03.142
Ren, M., Wang, X., Tian, C., Li, X., Zhang, B., Song, X., et al. (2017). Characterization of organic acids and phenolic compounds of cereal vinegars and fruit vinegars in China. J. Food Process. Preserv. 41, e12937. doi: 10.1111/jfpp.12937
Renz, A., Stitt, M. (1993). Substrate specificity and product inhibition of different forms of fructokinases and hexokinases in developing potato tubers. Planta 190, 166–175. doi: 10.1007/BF00196608
Ribani, M., Bottoli, C. B. G., Collins, C. H., Jardim, I. C. S. F., Melo, L. F. C. (2004). Validation for chromatographic and electrophoretic methods. Quim. Nova 27, 771–780. doi: 10.1590/S0100-40422004000500017
Ruan, Y.-L. (2014). Sucrose metabolism: gateway to diverse carbon use and sugar signaling. Annu. Rev. Plant Biol. 65, 33–67. doi: 10.1146/annurev-arplant-050213-040251
Ruffner, H. P., Possner, D., Brem, S., Rast, D. M. (1984). The physiological role of malic enzyme in grape ripening. Planta 160, 444–448. doi: 10.1007/BF00429761
Sadka, A., Dahan, E., Cohen, L., Marsh, K. B. (2000). Aconitase activity and expression during the development of lemon fruit. Physiol. Plant 108, 255–262. doi: 10.1034/j.1399-3054.2000.108003255.x
Sáenz-Galindo, A., López-López, L. I., Cruz-Duran, F. N., de la, Castañeda-Facio, A. O., Rámirez-Mendoza, L. A., Córdova-Cisneros, K. C., et al. (2018). “Applications of carboxylic acids in organic synthesis, nanotechnology and polymers,” in Carboxylic acid - key role in life sciences (London, United Kingdom:InTech). doi: 10.5772/intechopen.74654
Schrader, S., Sauter, J. J. (2002). Seasonal changes of sucrose-phosphate synthase and sucrose synthase activities in poplar wood (Populus × canadensis moench ‘ robusta ‘) and their possible role in carbohydrate metabolism. J. Plant Physiol. 159, 833–843. doi: 10.1078/0176-1617-00730
Sharma, S., Shree, B., Aditika, Sharma, A., Irfan, M., Kumar, P. (2022). Nanoparticle-based toxicity in perishable vegetable crops: Molecular insights, impact on human health and mitigation strategies for sustainable cultivation. Environ. Res. 212, 113168. doi: 10.1016/j.envres.2022.113168
Sheng, O., Song, S. W., Chen, Y. J., Peng, S. A., Deng, X. X. (2009). Effects of exogenous b supply on growth, b accumulation and distribution of two navel orange cultivars. Trees 23, 59. doi: 10.1007/s00468-008-0254-3
Spampinato, C. P., Colombo, S. L., Andreo, C. S. (1994). Interaction of analogues of substrate with NADP-malic enzyme from maize leaves. Photosynth. Res. 39, 67–73. doi: 10.1007/BF00027144
Stein, O., Granot, D. (2019). An overview of sucrose synthases in plants. Front. Plant Sci. 10. doi: 10.3389/fpls.2019.00095
Subedi, A., Shrestha, A. K., Tripathi, K. M., Shrestha, B. (2019). Effect of foliar spray of boron and zinc on fruit quality of papaya (Carica papaya l.) cv. red lady in chitwan, Nepal. Int. J. Hortic 9, 10–14. doi: 10.5376/ijh.2019.09.0002
Sweetman, C., Deluc, L. G., Cramer, G. R., Ford, C. M., Soole, K. L. (2009). Regulation of malate metabolism in grape berry and other developing fruits. Phytochemistry 70, 1329–1344. doi: 10.1016/j.phytochem.2009.08.006
Tayal, R., Kumar, V., Irfan, M. (2022). Harnessing the power of hydrogen sulphide (H 2 s) for improving fruit quality traits. Plant Biol. 24, 594–601. doi: 10.1111/plb.13372
Tian, S., Qin, G., Li, B. (2011). “Loquat,” in Postharvest biology and technology of tropical and subtropical fruits (Sawston, Cambridge:Woodhead Publishing), vol. 444. doi: 10.1016/B978-1-84569-735-8.50017-6
Toker, R., Gölükcü, M., Tokgöz, H., Tepe, S. (2013). Organic acids and sugar compositions of some loquat cultivars (Eriobotrya japonica l.) grown in Turkey. Tarım Bilim. Derg. 19, 121–128. doi: 10.1501/Tarimbil_0000001236
Wang, Z., Wei, P., Wu, M., Xu, Y., Li, F., Luo, Z., et al. (2015). Analysis of the sucrose synthase gene family in tobacco: structure, phylogeny, and expression patterns. Planta 242, 153–166. doi: 10.1007/s00425-015-2297-1
Wei, Y., Xu, F., Shao, X. (2017). Changes in soluble sugar metabolism in loquat fruit during different cold storage. J. Food Sci. Technol. 54, 1043–1051. doi: 10.1007/s13197-017-2536-5
Wiesler, F. (2012). “Nutrition and quality,” in Marschner’s mineral nutrition of higher plants (Amsterdam, NetherlandsElsevier), 271–282. doi: 10.1016/B978-0-12-384905-2.00009-1
Wu, W., Chen, F. (2016). Malate transportation and accumulation in fruit cell. Endocytobiosis Cell Res. 27, 107–112.
Xu, L., Xu, H., Cao, Y., Yang, P., Feng, Y., Tang, Y., et al. (2017). Validation of reference genes for quantitative real-time pcr during bicolor tepal development in asiatic hybrid lilies (Lilium spp.). Front. Plant Sci. 8. doi: 10.3389/fpls.2017.00669
Xu, X., Yang, Y., Liu, C., Sun, Y., Zhang, T., Hou, M., et al. (2019). The evolutionary history of the sucrose synthase gene family in higher plants. BMC Plant Biol. 19, 566. doi: 10.1186/s12870-019-2181-4
Yang, L. T., Xie, C. Y., Jiang, H. X., Chen, L. S. (2011). Expression of six malate-related genes in pulp during the fruit development of two loquat (Eriobotrya japonica) cultivars differing in fruit acidity. Afr. J. Biotechnol. 10, 2414–2422. doi: 10.4314/ajb.v10i13
Yang, J., Zhang, J., Niu, X.-Q., Zheng, X.-L., Chen, X., Zheng, G.-H., et al. (2021). Comparative transcriptome analysis reveals key genes potentially related to organic acid and sugar accumulation in loquat. PloS One 16, e0238873. doi: 10.1371/journal.pone.0238873
Yao, Y.-X., Li, M., Liu, Z., You, C.-X., Wang, D.-M., Zhai, H., et al. (2009). Molecular cloning of three malic acid related genes MdPEPC, MdVHA-a, MdcyME and their expression analysis in apple fruits. Sci. Hortic. (Amsterdam) 122, 404–408. doi: 10.1016/j.scienta.2009.05.033
Yao, Y.-X., Li, M., Zhai, H., You, C.-X., Hao, Y.-J. (2011). Isolation and characterization of an apple cytosolic malate dehydrogenase gene reveal its function in malate synthesis. J. Plant Physiol. 168, 474–480. doi: 10.1016/j.jplph.2010.08.008
Yu, X., Ali, M. M., Li, B., Fang, T., Chen, F. (2021). Transcriptome data-based identification of candidate genes involved in metabolism and accumulation of soluble sugars during fruit development in ‘Huangguan’ plum. J. Food Biochem. 45, e13878. doi: 10.1111/jfbc.13878
Zaman, Q. U., Schumann, A. W. (2006). Nutrient management zones for citrus based on variation in soil properties and tree performance. Precis. Agric. 7, 45–63. doi: 10.1007/s11119-005-6789-z
Zhang, Y., Hu, C.-X., Tan, Q.-L., Zheng, C.-S., Gui, H.-P., Zeng, W.-N., et al. (2014). Plant nutrition status, yield and quality of satsuma mandarin (Citrus unshiu marc.) under soil application of fe-EDDHA and combination with zinc and manganese in calcareous soil. Sci. Hortic. (Amsterdam) 174, 46–53. doi: 10.1016/j.scienta.2014.05.005
Zhang, Y. H., Wang, Z. M., Huang, Q., Shu, W. (2008). Phosphoenolpyruvate carboxylase activity in ear organs is related to protein concentration in grains of winter wheat. J. Cereal Sci. 47, 386–391. doi: 10.1016/j.jcs.2007.04.011
Zhang, X., Wei, X., Ali, M. M., Rizwan, H. M., Li, B., Li, H., et al. (2021). Changes in the content of organic acids and expression analysis of citric acid accumulation-related genes during fruit development of yellow (Passiflora edulis f. flavicarpa) and purple (Passiflora edulis f. edulis) passion fruits. Int. J. Mol. Sci. 22, 5765. doi: 10.3390/ijms22115765
Zhao, D., Oosterhuis, D. M. (2002). Cotton carbon exchange, nonstructural carbohydrates, and boron distribution in tissues during development of boron deficiency. F. Crop Res. 78, 75–87. doi: 10.1016/S0378-4290(02)00095-3
Zheng, S., Johnson, A. J., Li, Y., Chu, C., Hulcr, J. (2019). Cryphalus eriobotryae sp. nov. (Coleoptera: Curculionidae: Scolytinae), a new insect pest of loquat Eriobotrya japonica in China. Insects 10, 180. doi: 10.3390/insects10060180
Zhi, C., Ali, M. M., Zhang, J., Shi, M., Ma, S., Chen, F. (2021). Effect of paper and aluminum bagging on fruit quality of loquat (Eriobotrya japonica lindl.). Plants 10, 2704. doi: 10.3390/plants10122704
Zhou, D., Chen, S., Xu, R., Tu, S., Tu, K. (2019). Interactions among chilling tolerance, sucrose degradation and organic acid metabolism in UV-c-irradiated peach fruit during postharvest cold storage. Acta Physiol. Plant 41, 79. doi: 10.1007/s11738-019-2871-4
Zhou, L., Paull, R. E. (2001). Sucrose metabolism during papaya (Carica papaya) fruit growth and ripening. J. Am. Soc Hortic. Sci. 126, 351–357. doi: 10.21273/JASHS.126.3.351
Keywords: malic acid, PEPC, fruit quality, malate dehydrogenase, borax, fructokinase, sucrose, liquid chromatography
Citation: Ali MM, Anwar R, Rehman RNU, Ejaz S, Ali S, Yousef AF, Ercisli S, Hu X, Hou Y and Chen F (2022) Sugar and acid profile of loquat (Eriobotrya japonica Lindl.), enzymes assay and expression profiling of their metabolism-related genes as influenced by exogenously applied boron. Front. Plant Sci. 13:1039360. doi: 10.3389/fpls.2022.1039360
Received: 08 September 2022; Accepted: 03 October 2022;
Published: 20 October 2022.
Edited by:
Pankaj Kumar, Dr. Yashwant Singh Parmar University of Horticulture and Forestry, IndiaReviewed by:
Ilian Badjakov, Agrobioinstitute (CAB), BulgariaAhmad Latif Virk, China Agricultural University, China
Behiye Banu Bilgen, Namik Kemal University, Turkey
Copyright © 2022 Ali, Anwar, Rehman, Ejaz, Ali, Yousef, Ercisli, Hu, Hou and Chen. This is an open-access article distributed under the terms of the Creative Commons Attribution License (CC BY). The use, distribution or reproduction in other forums is permitted, provided the original author(s) and the copyright owner(s) are credited and that the original publication in this journal is cited, in accordance with accepted academic practice. No use, distribution or reproduction is permitted which does not comply with these terms.
*Correspondence: Youming Hou, eW1ob3VAZmFmdS5lZHUuY24=; Faxing Chen, ZnhjaGVuQGZhZnUuZWR1LmNu