- 1Department of Biology, Faculty of Sciences and Techniques, Hassan II University, Casablanca, Morocco
- 2Department of Agricultural Sciences, University of Naples Federico II, Naples, Italy
Currently, the use of phosphate (P) biofertilizers among many bioformulations has attracted a large amount of interest for sustainable agriculture. By acting as growth promoters, members of the Streptomyces genus can positively interact with plants. Several studies have shown the great potential of this bacterial group in supplementing P in a soluble, plant-available form by several mechanisms. Furthermore, some P-solubilizing Streptomyces (PSS) species are known as plant growth-promoting rhizobacteria that are able to promote plant growth through other means, such as increasing the availability of soil nutrients and producing a wide range of antibiotics, phytohormones, bioactive compounds, and secondary metabolites other than antimicrobial compounds. Therefore, the use of PSS with multiple plant growth-promoting activities as an alternative strategy appears to limit the negative impacts of chemical fertilizers in agricultural practices on environmental and human health, and the potential effects of these PSS on enhancing plant fitness and crop yields have been explored. However, compared with studies on the use of other gram-positive bacteria, studies on the use of Streptomyces as P solubilizers are still lacking, and their results are unclear. Although PSS have been reported as potential bioinoculants in both greenhouse and field experiments, no PSS-based biofertilizers have been commercialized to date. In this regard, this review provides an overview mainly of the P solubilization activity of Streptomyces species, including their use as P biofertilizers in competitive agronomic practices and the mechanisms through which they release P by solubilization/mineralization, for both increasing P use efficiency in the soil and plant growth. This review further highlights and discusses the beneficial association of PSS with plants in detail with the latest developments and research to expand the knowledge concerning the use of PSS as P biofertilizers for field applications by exploiting their numerous advantages in improving crop production to meet global food demands.
Introduction
Phosphorus (P) is a macronutrient required in abundance by plants and is essential in many metabolic reactions, as P acts as a backbone in molecules such as deoxyribonucleic acid (DNA), ribonucleic acid (RNA) and phospholipids of plant cells, as well as it is essential in photosynthesis and improves flower formation, seed production, crop maturity and, resistance to plant diseases, stimulates root development and supports the plant development throughout entire life cycle (Soumare et al., 2020). Additionally, P is the second limiting nutrient after nitrogen in agricultural production. For this reason, most farmers regularly apply P chemical fertilizers to avoid P deficiency, which leads to stunted growth and dark-green coloration of plants, alters the plant metabolism by accumulating carbohydrates especially sucrose in leaves and secretion of organic acid (Meng et al., 2021). Typically, the challenge of soil phosphorus deficiency is addressed by the use of P-based fertilizers, which usually precipitate immediately after their application and tend to be unavailable to plants, thus wasting P (Urrutia et al., 2014). Often, the excess use of chemical P can lead to severe risks to environmental and human health, such as groundwater contamination and waterway eutrophication (Alori and Babalola, 2018). Beside their negative environmental impact, chemical P-based fertilizers are expensive due to their extraction costs from the raw materials (P rock) and the substantial amounts of energy required to transform them into forms that farmers can use (Abebe et al., 2022). This high cost could be balanced by investing money in an alternative procedure with a greater potential return. P-biofertilizers provides benefits from an economic, social, and environmental point of view since they can help to reduce the cost of P input because of their possibility to use the accumulated P in soils and enhance plant growth (Khan et al., 2007), as well as is need a smaller amount of energy for their production in respect to chemical fertilizers (Carvajal-Muñoz and Carmona García, 2012) and they do require only a specific set of equipment to ferment correctly microbial strains and store them until use (Malusá et al., 2012). Therefore, the development and use of bioformulations with beneficial microbes able to improve the growth, fitness and health of plants growing in soils lacking nutrients and/or experiencing stress is an attractive low-cost alternative to agrochemical management (Romano et al., 2020a). In particular, the use of phosphate-solubilizing microorganism (PSM)-based inocula is a promising ecofriendly strategy to increase soil P availability as well as to enhance P absorption by plants, thereby limiting the use of chemical fertilizers (Walpola and Yoon, 2012; Kishore et al., 2015). Among soil microbes, Streptomyces and other Actinomycetes are among the dominant prokaryotic taxa living in these environments. Streptomyces contains many species with different plant growth-promoting activities (Olanrewaju and Babalola, 2019; Di Mola et al., 2021); these activities affect a wide range of biological processes by increasing soil nutrient availability to plants and phytohormone production and/or by suppressing plant disease by inhibiting the growth of soil-borne plant pathogens (Bhatti et al., 2017). Specifically, several species with different morphological and functional characteristics are considered PSMs for their potential ability to release insoluble P and make it available for plants (Hamdali et al., 2010; Chouyia et al., 2020). Despite their several interesting biotechnological activities, Streptomyces species have been scarcely used as commercial microbe-based biofertilizer components.
Although the various implications and interactions in plant growth promotion by member of the genus Streptomyces have been largely reviewed (Amaresan et al., 2018; Olanrewaju and Babalola, 2019) and the use of Streptomyces species in agriculture has been recently reported (Rey and Dumas, 2017), very little is known about their ability to solubilize P. Thus, based on these considerations, this review gives insights into the current knowledge on the ability of Streptomyces species to solubilize P, with a focus on the mechanisms through which the solubilize and mineralize P. Moreover, this review also describes plant growth-promoting traits coupled to P solubilization and examines the potential of applying Streptomyces an innovative biofertilizer in crop production.
Taxonomic characteristics, nature, and habitat of Streptomyces
Streptomyces, belonging to the order Streptomycetales, family Streptomycetaceae, are aerobic spore-forming filamentous gram-positive bacteria, which comprises 1131 species and 72 subspecies that are validly described (https://lpsn.dsmz.de/genus/streptomyces). They are distinguished from most other bacteria by their mode of growth and their properties of both fungal and bacterial cell morphologies (Das et al., 2008). Streptomyces colonies are slow growing and produce aerial mycelia responsible for the characteristic soil odor because of the production of the geosmin as volatile metabolite (Ikeda et al., 2003; Jüttner and Watson, 2007). Preferring a neutral or slightly alkaline pH, Streptomyces species are generally mesophilic, while others are thermophilic and tolerate temperatures of approximately 50°C and even up to 60°C (Shivlata and Satyanarayana, 2015). The genome of Streptomyces is characterized by large linear chromosomes with a high guanine–cytosine content, while the most marked characteristic of the Streptomyces genome is the high degree of chromosomal instability, which leads to frequent spontaneous deletions and rearrangements, especially at the ends of the chromosome (Lee et al., 2020). They are well known as dominant prokaryotic taxa that live in the soil and have been found in various habitats, including rivers, lakes, and marine ecosystems; this ubiquitousness is due to their ability to produce a large number of specific enzymes and secondary metabolites as well as their filamentous forms which cause the strength of soil texture and allow them to compete in various microbial communities and survive in various habitats, even in desert soils (Jensen et al., 2005; Radhakrishnan and Balagurunathan, 2011; Ventorino et al., 2016; Montella et al., 2017; Sheridan et al., 2017; Pennacchio et al., 2018; Ventorino et al., 2019). The size and the activity of the Streptomyces population increase in soil comparing to other bacteria because of their ability to decompose plant and animal residues, degrade cotton textiles, plastics, rubber, and paper as well as cellulose, lignocellulose, chitin, and different organic compounds to survive as they can recovered even from horizon C of the soil (Hasani et al., 2014). Based on those functions, Streptomyces influence their environment, e.g., soil biogeochemical cycles, soil community structure and/or other microorganisms (Bontemps et al., 2013; Donald et al., 2022). In a recent study, denaturing gradient gel electrophoresis (DGGE) analysis indicated that S. roseocinereus MS1B15 inoculation affected microbiota in the rhizosphere of barley plants by increasing prokaryotic and eukaryotic diversity comparing to un-inoculated soil (Chouyia et al., 2020). In addition, Wang et al. (2020) pointed that the application of the strain S. lydicus M01, which exhibited growth promoting characteristics such as P-solubilization, IAA secretion, siderophore and ACC deaminase production as well as increased cucumber plant growth, in addition to determining an increase in the abundance of Streptomyces genus altered the microbiota composition by promoting prokaryotic and eukaryotic populations known as potentially beneficial microorganisms, such as Pseudarthrobacter, Sphingomonas, Rhodanobacter, Pseudomonas, Fusicolla, Humicola, Solicoccozyma, and Paraphaeosphaeria.
Phosphate-solubilizing Streptomyces
Phosphorus is considered an essential nonrenewable macronutrient required for the growth and development of plants. P nutrition is associated with several key functions of plants, including the development of roots, the strengthening of stems, the formation of flowers and seeds, plant maturity and quality of production (Walpola and Yoon, 2012). Although a substantial reserve of P is present in the soil, a large portion is unavailable to plants, and a considerable portion of phosphate applied to soils as fertilizer is rapidly immobilized, leading to low P-use efficiency and potentially excess P (Kochian, 2012). The solubilization and microbial mineralization of P is an ecofriendly and low-cost strategy to exploit native soil P, limiting the application of chemical fertilizers and providing both environmental and economic benefits (Zaidi et al., 2009). Microorganisms capable of solubilizing phosphate are called PSMs, which mainly include Actinomycetes. Hamdali et al. (2008a) reported that among 300 actinobacterial isolates, approximately 20% were able to efficiently solubilize P and belong to the Streptomyces genus. Numerous Streptomyces species isolated from rhizosphere soils have been reported as potential P solubilizers (Hamdali et al., 2010), and they are able to solubilize different sources of P; several works have reported the ability of Streptomyces to solubilize the tricalcium P form. In particular, different Streptomyces species isolated from wheat and tomato rhizospheres were shown to be able to produce high amounts of P, ranging from 70.36 µg/ml to 1916.12 ± 10.35 µg/ml (Jog et al., 2012; Sadeghi et al., 2012; Jog et al., 2014; Anwar et al., 2016; Table 1). By the use of a semiquantitative method, the strain Streptomyces sp. PSA-7, isolated from forest soil in the Mahabubnagar district, was shown to solubilize tricalcium P, producing a halo of 27 mm (Balakrishna et al., 2012). Similarly, the strains Streptomyces violascens TNC-1 and Streptomyces asenjonii MNC-1, isolated from extreme environments in Morocco, as well as Streptomyces sp. VITMS22, isolated from an agricultural field, were able to produce P starting from its tricalcium form, albeit at low amounts ranging from 8.56 µg/ml to 20 µg/ml (Kizhakedathil and Devi, 2018; Nafis et al., 2019). In contrast, Jog et al. (2014) reported that the strain Streptomyces cellulose mhcr 0816, isolated from a wheat rhizosphere, exhibited high P solubilization (up to 1916 mg/L), a value that exceeded the level of other well-known P solubilizing bacterial genera, such as Bacillus (up to 957 mg/L; Mehta et al., 2010) and Pseudomonas (up to 66.2 ± 13.4 mg/L; Blanco-Vargas et al., 2020), which were previously reported as potential PSMs (Sharma et al., 2013; Kalayu, 2019).
In addition to their ability to solubilize tricalcium P, Streptomyces spp. are also able to solubilize other inorganic P forms, such as rock P (Nandimath et al., 2017; Boubekri et al., 2021), aluminum P (Chaiharn et al., 2018), and dicalcium P (Chouyia et al., 2020). However, Streptomyces species are also able to mineralize organic P (Han et al., 2018), as reported also Ghorbani-Nasrabadi et al. (2012); specifically, Streptomyces alboniger, Streptomyces venezuelae 43, Streptomyces ambofaciens and Streptomyces lienomycini 63, isolated from different soil ecosystems, were shown to solubilize both tricalcium and organic P by producing phytase enzymes (Table 1). Nevertheless, the strain Streptomyces rishiriensis 3AS4, isolated from a wheat rhizosphere cultivated in the Cerrado biome, was found to use several P forms. In detail, this strain was able to mineralize and solubilize phytate as well as tricalcium and rock P, reaching final concentrations of 400 μg/ml, 300 μg/ml and 200 μg/ml, respectively (Vargas Hoyos et al., 2021; Table 1).
Mechanism of P solubilization by Streptomyces
The chemical P added to the soil by farmers to avoid P-limiting conditions in cropping systems usually precipitates after the application by the formation of unavailable P complexes, whether in acidic or alkaline soils (Urrutia et al., 2014). This mechanism generally results in a slow release of P, causing great challenges for remediation of these soils, with a high level of P unavailable to plants (Roy, 2017); plants prefer P in a water-soluble form, i.e., PO43−, H2PO42−, HPO42−. Concentrations of these water-soluble P forms are very low in soils and vary from 0.001 mg/L (poor soils) to 1 mg/L (highly fertile soils). PSMs are known to transform inorganic and organic P sources to soluble forms and make them available to plants by solubilization (Toro, 2007; Walpola and Yoon, 2012) and/or by mineralization of complex P compounds (Glick, 2012). There are several hypotheses for describing the mechanisms of P solubilization by microbes (Khan et al., 2007; Buch et al., 2008). Figure 1 shows a schematic representation of the solubilization/mineralization of P forms by PSS. First, the excretion of organic acids has been widely accepted as the main mechanism of inorganic P solubilization, and various studies have pointed out the role of organic acid production in the solubilization process (Antoun, 2012; Marra et al., 2012; Alori and Babalola, 2018). The organic acids produced by PSMs in nature or under in vitro conditions act through i) reducing the pH, ii) enhancing the chelation of cations bound to P, iii) forming complexes with P-associated metal ions and iv) competing with P for adsorption (Pradhan and Sukla, 2006; Ingle and Padole, 2017). Consequently, the excretion of these organic acids is accompanied by acidification of the microbial cells and their surroundings; therefore, P ions are released by H+ being substituted for Ca2+ (Jones and Oburger, 2011). Hence, Sharan and Darmwal (2008) proposed that assimilation of NH4+ together with H+ elicits about P solubilization. Indeed, Park et al. (2009) confirmed that for some microorganisms, NH4+-driven H+ release seems to be the sole mechanism to promote P solubilization.
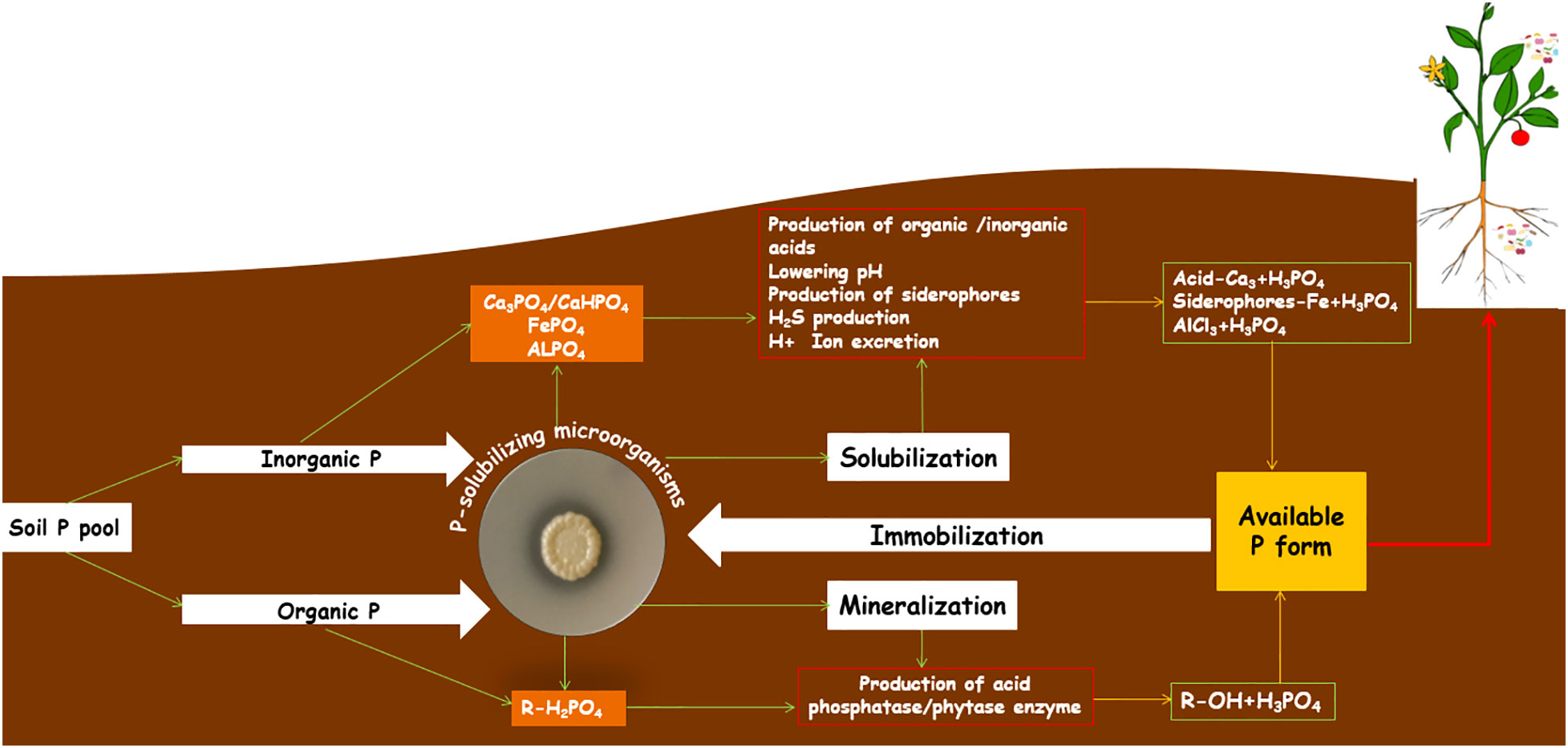
Figure 1 Schematic representation of solubilization/mineralization mechanisms of different P forms by P-solubilizing Streptomyces. Soil P is present in inorganic and organic forms. Phosphate-solubilizing Streptomyces induce P reactions by several mechanisms (production of organic and inorganic acids, siderophores, etc.) to release unavailable and immobilized inorganic P forms for plants (Ca-P, calcium phosphate; Fe-P, iron phosphate; Al-P, aluminum phosphate) by solubilization; while they secret extracellular enzymes (phosphatase and phytase) to release the organic P forms (orthophosphate, phosphonates, etc.) by mineralization process in order to provide the water-soluble form H3PO4 to the plant.
The excretion of inorganic acids, e.g., HCl, H2SO4 and HNO3, has also been reported to lead to the solubilization of P (Reyes et al., 2001; Richardson, 2001). The release of bound inorganic P by the production of H2S is another mechanism through which ferric phosphate is involved, resulting in the formation of ferrous sulfate and P release. This could be because of microbial S oxidation, nitrate production and CO2 formation, which lead to the formation of inorganic acids such as H2SO4. Jones and Oburger (2011) suggested that P microbial solubilization might also occur in response to the excretion of chelating substances such as siderophores that form stable complexes with P adsorbents. Interestingly, mineralization and immobilization of soil organic P play a key role in the phosphorus cycling of agricultural lands. PSMs also play an important role in mineralizing organic P forms and making organic P available to plants principally through the production of acid phosphatases (Beech et al., 2001; Kishore et al., 2015). Richardson and Simpson (2011) documented that half of the soil microorganisms and plant roots have P mineralization potential through the action of phosphatases. In the case of P solubilization by Streptomyces, several researchers have reported that this genus involves acidification of the growth medium and production of organic acids by the release of P (Arunachalam Palaniyandi et al., 2013; Farhat et al., 2015; Biglari et al., 2016). Recently, Chaiharn et al. (2018) indicated that numerous Streptomyces species were able to solubilize P by resulting in a significant pH decrease ranging from culture growth or the production of organic acids but with the secretion of chelating siderophore-like molecules.
Moreover, several Streptomyces species demonstrated the ability to mineralize organic P by the production of phosphoric acid. Walpola and Yoon (2012) documented that members of the genera Bacillus and Streptomyces can mineralize complex organic phosphates through the production of extracellular enzymes such as phosphoesterases, phosphodiesterases, phytases, and phospholipases. Additionally, Khan et al. (2009) reported that the ability of Bacillus and Streptomyces species to mineralize organic P was higher than that exhibited by Aspergillus, Penicillium, Proteus, Serratia, Pseudomonas and Micrococcus species, although the mixed PSM cultures (Bacillus, Streptomyces, Pseudomonas, etc.) were the most effective at mineralizing organic phosphate.
Plant growth-promoting traits by phosphate-solubilizing Streptomyces
Beneficial soil bacteria such as PSS, which have been shown to make mainly soluble P accessible to plants in different production systems, are usually referred to as plant growth-promoting Streptomyces (PGPS) (Amaresan et al., 2018). In addition, by providing soluble P to plants, Streptomyces species exhibit a range of traits that promote plant growth, such as N2 fixation and the production of both growth-promoting hormones and many secondary metabolites. These beneficial attributes can be divided into those associated with biofertilization, biostimulation, and bioprotection.
Phosphate-solubilizing Streptomyces as biofertilizers
Biofertilizers are organic in nature and include secondary metabolites of microbial origin or microorganisms themselves (Mishra and Dash, 2014). Thus, living microorganisms that directly affect processes that improve macro- and micronutrient acquisition, such as nitrogen fixation, P solubilization or nutrient mobilization, to increase soil or plant productivity can be used as biofertilizers. In addition to their ability to solubilize P, Streptomyces members can also facilitate the growth and development of plants by producing essential nutrients. Sellstedt and Richau (2013) pointed out that gram-positive actinobacteria, including species of Streptomyces, had nitrogen-fixing ability. Dahal et al. (2017) considered Streptomyces species that have the nitrogen fixation-related gene nifH to be a nitrogen-fixing bacteria, and as N-fixers essential for the functioning of soil ecosystem (Ventorino et al., 2007). On the other hand, Streptomyces thermoautotrophicus demonstrated the ability to fix atmospheric nitrogen into biological form by molybdenum denitrogenase and manganese-superoxide oxidoreductase (Ribbe et al., 1997).
Controversially, Mackellar et al. (2016) reported that S. thermoautotrophicus is a highly effective nitrogen scavenger but not a nitrogen fixer. Chouyia et al. (2020) established that, like PSS, the P-solubilizing strain Streptomyces roseocinereus MS1B15 isolated from the Moroccan oat rhizosphere did not contain nifH genes and therefore was considered a P-solubilizer with negative nitrogen fixation activity. By evaluating their growth on N-free media, Fatmawati et al. (2019) and Wahyudi et al. (2019) demonstrated that the PSS species isolated from a soybean rhizosphere were able to fix nitrogen (Table 2). To date, there is no convincing evidence for free-living and endophytic Streptomyces that can fix nitrogen, and their mechanism remains unclear.
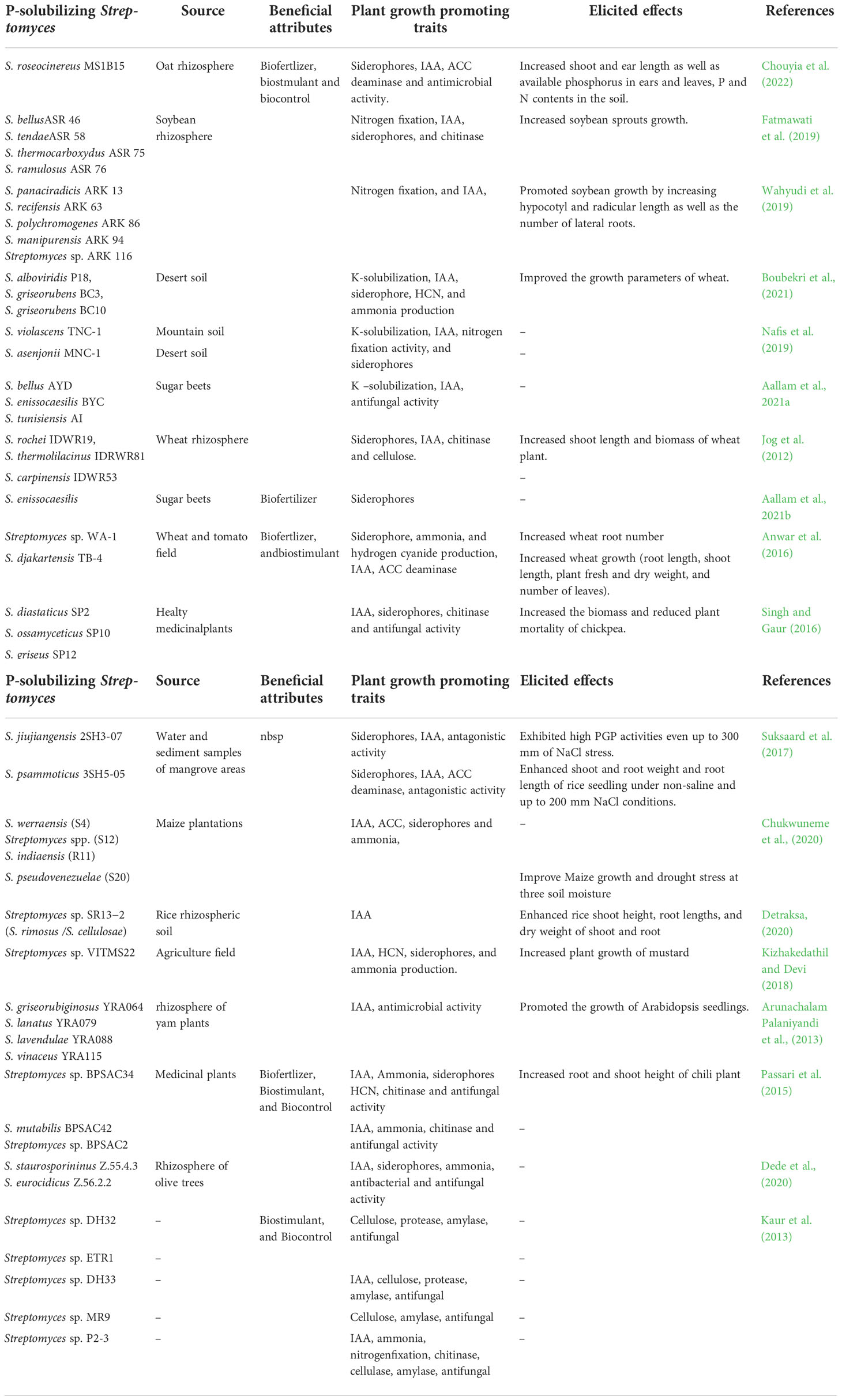
Table 2 Studies on the plant growth promoting traits released by P-solubilizing Streptomyces species within past 10 years.
Indeed, potassium (K), together with nitrogen and phosphorus, is among the essential elements needed for plant growth (Amtmann and Armengaud, 2007; Chen et al., 2008). It is known that potassium-solubilizing bacteria (KSB) can solubilize K-bearing minerals and convert insoluble K to soluble forms available for plant uptake (Etesami et al., 2017). Several studies have reported the potential effect of KSB as biofertilizers for agricultural improvement by reducing the use of agrochemicals and supporting ecofriendly crop production (Archana et al., 2013; Prajapati et al., 2013). Streptomyces strains have been found to release potassium-bearing rock powder by solid-state fermentation (Liu et al., 2016). Recently, Boubekri et al. (2021) reported that 30% of Streptomyces isolated from a Moroccan desert soil were able to solubilize both K and P and could be promising candidates for the implementation of efficient biofertilization strategies to improve soil fertility and plant yield under rock P and rock K fertilization. Indeed, S. violascens TNC-1 and S. asenjonii MNC-1 isolated from montane and desert soils, respectively, were reported to solubilize inorganic K, as they produced a clear halo zone on Aleksandrov agar (Nafis et al., 2019), and several PSS strains isolated from sugar beet plants were found to be able to release soluble K from insoluble orthoclase in large amounts ranging from 3.8 mg/L to 216.6 mg/L (Aallam et al., 2021a; Table 2).
In addition, iron (Fe) is an essential trace element required by all organisms. However, the oxidation state of iron can reversibly change, causing the ion to become inaccessible by microorganisms. The production of siderophores is one strategy employed by microorganisms to survive in iron-stressed environments and overcome low iron availability (Haas, 2003; Ma, 2005). Imbert et al. (1995) reported that different Streptomyces species were able to produce different types of siderophores. In particular, Streptomyces viridosporus produced both linear desferrioxamine B and cyclic desferrioxamine E, while S. ambofaciens synthesized only cyclic desferrioxamine E as the main siderophore; finally, linear desferrioxamine G was the major form produced by Streptomyces coelicolor and Streptomyces lividans. Streptomyces sp. GMKU 3100 isolated from the roots of a Thai jasmine rice (Oryza sativa L. cv. KDML105) plant and selected as the highest siderophore producer on CAS agar media, significantly promoted rice and mungbean plant growth in a greenhouse experiment and therefore could be applied as a potentially safe and environmentally friendly biofertilizer in agriculture (Rungin et al., 2012). The strains Streptomyces rochei, Streptomyces carpinensis and Streptomyces thermolilacinus isolated from a wheat rhizosphere as well as Streptomyces enissocaesilis isolated from sugar beet selected as the best P solubilizers were also capable of producing siderophores on CAS agar media (Jog et al., 2012; Aallam et al., 2021b; Table 2). Similarly, several works have demonstrated the ability of numerous PSS strains isolated from different sources to produce siderophores in addition to their main P activity (Anwar et al., 2016; Suksaard et al., 2017; Singh et al., 2019; Table 2).
Phosphate-solubilizing Streptomyces as biostimulants
PGPS can regulate plant growth by the production and/or degradation of plant hormones composing major groups (Duncan et al., 2015). Phytohormones, such as auxins, cytokinins (CKs), gibberellin (GA), and ethylene (ET), are important growth regulators synthesized in defined organs of plants and have a prominent impact on plant metabolism (Fahad et al., 2015; Shi et al., 2017). Numerous Streptomyces strains, such as Streptomyces CMU-PA101, Streptomyces CMU-SK126 (Khamna et al., 2009), Streptomyces atrovirens ASU14 (Abd-Alla et al., 2013) and Streptomyces fradiae NKZ-259 (Myo et al., 2019), have been extensively reported to produce the auxin indole-3-acetic acid (IAA). Similarly, Rashad et al. (2015) reported the ability of Streptomyces strains isolated from a marine environment to produce a wide range of phytohormones, including GA, IAA, abscisic acid, kinetin and benzyladenine, and were able to positively influence the growth parameters of eggplant (Solanum melongena), including root length and fresh/dry root weight, in pot experiments. Furthermore, numerous Streptomyces strains, in addition to their main P-solubilizing activity, were able to secrete IAA in broth media, reaching values from 7.9 ± 0.1 µg/ml to 122.3 ± 0.1 µg/ml (Aallam et al., 2021a; Table 2). The P-solubilizing, Streptomyces griseorubiginosus YRA064, Streptomyces lanatus YRA079, Streptomyces lavendulae YRA088, Streptomyces vinaceus YRA115, isolated from the rhizosphere of yam plants, Streptomyces sp. SR13−2, isolated from a rice rhizosphere, Streptomyces pseudovenezuelae (S20), isolated from a maize plantation, and Streptomyces sp. VITMS22, isolated from an agricultural field, were reported to secrete IAA and enhance biometric parameters of Arabidopsis seedlings, rice plants, maize plants, and mustard plants (Arunachalam Palaniyandi et al., 2013; Kizhakedathil and Devi, 2018; Chukwuneme et al., 2020; Detraksa, 2020; Table 2).
Streptomyces strains also degrade 1-aminocyclopropane-1-carboxylate (ACC). ACC deaminase-producing Streptomyces filipinensis No. 15 and S. atrovirens No. 26 were shown to increase plant growth significantly through the reduction of levels of endogenous ACC and the consequent lowering of endogenous ET levels in plant tissues (El-Tarabily, 2008). P-solubilizing Streptomyces nobilis WA-3 was selected as the most active IAA producer by Anwar et al. (2016); this strain showed a significant ability to produce ACC deaminase. Due to its ability, this strain significantly increased several growth parameters of wheat plants in pot experiments under standard conditions (Table 2).
Phosphate-solubilizing Streptomyces as biocontrol agents
Streptomyces has also been investigated for the production of bioactive molecules with an antagonistic function against plant pathogens (Barakate et al., 2002; Toumatia et al., 2015). The main biocontrol ability of Streptomyces species is attributed to their high production of antibiotics and hydrolytic enzymes such as cellulase, amylase, protease, and xylanase (Dhanasekaran et al., 2008; Al-Askar et al., 2015). Streptomyces griseoviridis, which was isolated from a tomato rhizosphere soil, was shown not only to exhibit antagonistic activity against Fusarium spp., Botrytis spp., and Alternaria spp. but also to exert potential antagonistic activity against Rhizoctonia solani, Pythium spp., Phytophthora spp., Thielaviopsis basicola, and Verticillium dahlia, demonstrating the wide-ranging potential of the members of this genus (Sabaratnam and Traquair, 2015). In addition, several Streptomyces strains, such as Streptomyces hygroscopicus, Streptomyces malaysiensis, Streptomyces violaceusniger YH27A, S. coelicolor, S. violaceusniger YCED-9, and Streptomyces sp. isolate KY-33, isolated from rhizosphere soil, have been reported to be potential antifungal agents against different soil-borne plant pathogens (Cheng et al., 2010; Gherbawy et al., 2012; Wu et al., 2012; Ghadge and Patil, 2016; Som et al., 2017; Shrivastava and Kumar, 2018).
The P-solubilizing Streptomyces griseus BH7 strain, isolated from Moroccan phosphate mines, was shown to inhibit the growth of potentially phytopathogenic fungi, bacteria (gram+/-) and yeasts. Therefore, this strain could be used for the development of novel biofertilizer and biocontrol products composed of spores and/or mycelia of the ad hoc Actinobacteria in association with pulverized rock P (Hamdali et al., 2008b).
Furthermore, the strains Streptomyces sp. BPSAC34, Streptomyces mutabilis BPSAC42, and Streptomyces sp. BPSAC2, isolated from medicinal plants, demonstrated potential to solubilize inorganic P for sustainable agriculture, as these strains positively affected multiple plant growth traits as well as exerted antifungal activity against fungal phytopathogens (Passari et al., 2015). The P-solubilizing strain S. griseus SP12 demonstrated a strong inhibitory effect—more than 60%—against Sclerotium rolfsii, R. solani, and F. oxysporum, reducing chickpea mortality (Singh and Gaur, 2016; Table 2), while Suksaard et al. (2017) reported that, in addition to their strong ability to solubilize P under various saline conditions, the P-solubilizing strains Streptomyces jiujiangensis 2SH3-07 and Streptomyces psammoticus 3SH5-05 have potential antagonistic activity against the rice pathogenic bacterial strains Xanthomonas oryzae pv. oryzae and X. oryzae pv. oryzicola. Other P-solubilizing actinobacterial strains also exert antimicrobial activity against bacteria. In particular, Streptomyces eurocidicus Z.56.2.2 and Streptomyces staurosporininus Z.55.4.3 showed very high inhibitory activity against several pathogenic bacteria and fungi, including Pseudomonas pv. glycinea, Staphylococcus aureus, Enterococcus faecalis, Aspergillus parasiticus, and Aspergillus niger and against S. aureus Phaseolus vulgaris, respectively (Dede et al., 2020). In some cases, the antimicrobial activity of several PSS species was associated with the hydrolytic activity of specific enzymes, such as cellulose, protease, and amylase, as reported by Kaur et al. (2013) (Table 2).
Development and applications of phosphate-solubilizing Streptomyces based biofertlizers
The use of PSMs as microbial inoculants in the soil appears to be an attractive, ecofriendly, and low-cost alternative for meeting agricultural challenges. In fact, the PSM strategy provides an excellent opportunity to satisfy global food demands, maintain a high quality of agricultural production, and limit both yield losses and the use of chemical fertilizers (Figueiredo et al., 2010; Fiorentino et al., 2018; Romano et al., 2020b). Many works have reported the potential biotechnological use of Streptomyces in agriculture. Streptomyces griseoflavus was tested in combination with Bradyrhizobium strains as a biofertlizer in pot experiments, and these strains demonstrated a significant increase in plant growth; nodulation; nitrogen fixation; seed yield; and nitrogen, phosphorus and potassium (NPK) uptake and in mung bean and soybean (Htwe et al., 2019). Doolotkeldieva et al. (2015) reported a strong stimulatory effect of Streptomyces fumanus-based biofertilizer on the growth of seeds and seedlings of wheat and soybeans; this biofertilizer improved the composition of the rhizosphere microflora, attracted saprophytic microorganisms as ammonifiers and oligotrophs and promoted the growth and development of useful groups of nitrogen-fixing bacteria. Additionally, thermotolerant P-solubilizing Streptomyces fulvissimus AN-12, Streptomyces olivoverticillatum AN-20, Streptomyces nogalater AN-24, Streptomyces longisporoflavus AN-27 and Streptomyces cellulosae AN-31 have also been reported to be potential biofertilizers; these strains can be added as raw materials to agricultural waste to make the biofertilizer multifunctional and provide the ability to solubilize P and degrade all types of wastes, such as cellulose, carbohydrate, lipids, chitin and pectin, all of which represent the main macromolecular components of agricultural waste (Nandimath et al., 2017). Although several studies have reported the potential use of Streptomyces species as microbial inoculants in soils, only a few Streptomyces strains, such as S. griseoviridis and Streptomyces lydicus, have been developed commercially as biofertilizers to promote plant growth (Hamedi and Mohammadipanah, 2015). In fact, the development and production of a successful biofertilizer based on beneficial microorganisms such as phosphate-solubilizing Streptomyces needs a long process to supply reliable and contaminant-free bioproducts to the farmers. The full process usually includes i) preparation of inoculum by evaluating its properties on a proper medium to optimize growth parameters (Zhang et al., 2019), ii) selection of specific method of propagation, pilot-scale study, large-scale production, and quality testing at each level to ensure the success of fermentation process and to produce enough amounts of microbial cells (Trujillo-Roldán et al., 2013), iii) selection of the best carrier which can help to keep the microbes in good physiological conditions and provide better shelf life to biofertilizer formulation during the storage and in the application site, iv) evaluation of bioformulation efficiency by greenhouse and field experiments as thoroughly established by Ahmad et al. (2018), testing different environmental conditions and different plant species (Bashan et al., 2014), v) and suitable packaging reporting recommendation for application and the expiration date (Bhattacharjee and Dey, 2014). In this context, PSS have been applied as potential inoculants for many crop plant species under pot and field conditions, some of which are previously presented in Table 2. Table 3 lists the beneficial effects exhibited by PSS species on different crop plants under greenhouse and natural conditions. Chaiharn et al. (2018) and Suárez-Moreno et al. (2019) reported that PSS species enhanced biometric parameters of rice plants under greenhouse and gnotobiotic conditions through P-solubilization activity as well as through promoting other plant growth-promoting traits (Table 3). In a study of wheat plants in a greenhouse experiment conducted by Sadeghi et al. (2012), Streptomyces sp. C significantly increased the germination rate, percentage and uniformity; shoot length; and dry weight, as well as the concentrations of N, P, Fe and Mn in the shoots. A similar study was conducted on wheat plants using Streptomyces tricolor mhce 0811, which was able to induce strong P-solubilizing activity that significantly increased wheat plant growth and mineral nutrient (Fe, Mn, and P) contents in the plants (Jog et al., 2014). The P-solubilizing strains S. rochei WZS1-1, Streptomyces sundarbansensis WZS2-1 and S. rochei UU07 significantly increased several biometric parameters of wheat plants (Han et al., 2018; Singh et al., 2019; Table 3). A greenhouse experiment was conducted on chickpea plants involving the use of S. griseus SP12, which caused a significant increase in several plant biometric parameters (Singh and Gaur, 2016). Similarly, maize (Zea mays L.) plants inoculated with Streptomyces sp. KP109810 and cultivated in a greenhouse presented significant increases in growth and P nutrition (Mohammed, 2020), while soybean plant height as well as shoot and root volumes significantly increased in response to S. rishiriensis 3AS4 inoculation under glasshouse conditions (Vargas Hoyos et al., 2021). In addition, owing to their P-solubilizing activity and production of auxin, numerous Streptomyces species were shown to act as plant promoters of tomato (Solanum lycopersicum) plants (Djebaili et al., 2020). A more recent study by Chouyia et al. (2022) reported that, compared with a popular commercial biofertilizer containing arbuscular mycorrhizal fungi, the strain S. roseocinereus MS1B15, which has multiple plant growth-promoting activities but was selected mainly for its strong P-solubilizing ability, significantly enhanced tomato growth as well as yield and quality under different crop rotation schemes, indicating that this strain could represent a sustainable and feasible model system for tomato production and thus could be a potential biofertilizer candidate to increase plant growth as well as P uptake.
The most reported PSS have been used as bioinoculants for sustainable agriculture not only for their P-solubilizing activity but also for their multiple functionalities to benefit plants by improving nutrient contents and inhibiting the growth of plant-borne pathogens. However, extensive research and more field trials are needed to study the mechanisms of P solubilization by Streptomyces and to create and develop a new commercial PSS-based biofertilizer.
Challenges for developing phosphate-solubilizing Streptomyces-based biofertilizer
Numerous bio-inoculants do not show the same efficient potential after having been commercialized, presenting extremely bad quality and totally unreliable under field conditions. The main challenges in the industrial development of biofertilizers are often related to the performance of microbial inoculum and the quality control of the products at each stage of the process. In fact, during the process should be necessary to evaluate physiological parameters such as the medium composition, temperature, pH, moisture content, aeration, and agitation as well as identify produced cells by gram reaction and microscope observation and perform viable counting to ensure the purity and high quantity of cells (Herrmann and Lesueur, 2013).
The inadequate formulation is another bottleneck for the commercialization, which requires a particular suitable carrier to keep the microbial cells alive during the storage and transport. A good carrier should be: (i) nontoxic to organisms (ii) easily pulverized and sterilized (iii) able to carry exceptionally high number of microbial cells (iv) easily available and cost effective, and (v) should have a good absorption capacity (Mishra and Arora, 2016). Finally, bioformulates should have a long shelf life and stability.
The abiotic (e.g., soil type, soil characteristics, water content, environmental conditions and agriculture practices) and biotic factors (e.g., plant varieties and genetics and soil microbiome) and the mode of application, can be a critical issue to the biofertilizer efficiency (Newitt et al., 2019). However, the development of PSS Streptomyces-based biofertilizers is related to the performance of production of this genus which is limited by the unique, long, and complex life cycle of Streptomyces species, which produce as one of two distinct filamentous cell forms. They first form vegetative hyphae firmly attached to the growth substrate and subsequently develop aerial hyphae, which differentiate into long-chain prespore compartments that subsequently mature into individual spores (Bush et al., 2015). Khushboo et al. (2022) indicated that the growth of Streptomyces in liquid media by large scale production may reaches up to pellet formation starting by development of hyphae instead of spore formation, while formation of spores and aerial mycelium become blocked in various Streptomyces strains. Physiological conditions including pH, medium composition and the stirring speed of the reactor should be considered to regulate mycelia morphology.
Another difficulty in handling Streptomyces is the contamination which could occur specially during the early growth phase (Shepherd et al., 2010). Moreover, there are also lack available data on Streptomyces-based biofertilizer interaction with soil microbial communities, probably due to the high number of experiment under controlled conditions rather than under field (Pacios-Michelena et al., 2021).
Thus, to highlight the advantage of Streptomyces at the commercial level, more studies on growth parameters are needed such as formulating novel media, defining the optimal conditions of each species, designing a simplified fermentation process, ensuring their purity to achieve higher production in less time and reduce production cost. Moreover, extensive field experiments are necessary to better understand their complex relations with plants varieties, soil microbial communities and environmental conditions to ensure their persistence in soil and produce an efficient and competitive PSS-based biofertilizer.
Conclusions and perspectives
Streptomyces species have been reported to be effective in various ways that naturally improve plant growth and development. The present review highlighted the potential of Streptomyces as a P solubilizer, as it demonstrated the mechanisms these bacteria employ to solubilize different P sources, as well as their involvement in other activities; their various plant growth-promoting traits; and their ability to act as biofertilizers, bioregulators and biocontrol agents. All these qualities appear to characterize the members of this genus and play the most important role in increasing agricultural productivity. Moreover, this review showed that the numerous studies on selection and characterization and numerous greenhouse experiments that have been performed until now are still insufficient. What is clear, though, is that PSS play an important role in the mobilization of soil P, while no studies have reported PSS to be commercial biofertilizers or demonstrated their potential effects under field conditions. For these reasons, we suggest that i) vigorous research at the field level is needed to focus on the use of Streptomyces in crop production, ii) that studies on their association effects under different crop species and in different environmental conditions are need, and that iii) there is also a need for further research to better understand how it could be possible to develop potential multifunctional bioformulations with PSS as biofertilizers.
Author contributions
FC drafted the manuscript. VV reviewed and editing the manuscript, conceived the concepts of the study and participated in its design. OP reviewed the manuscript and contributed to its conceiving. All authors contributed to the article and approved the submitted version.
Conflict of interest
The authors declare that the research was conducted in the absence of any commercial or financial relationships that could be construed as a potential conflict of interest.
Publisher’s note
All claims expressed in this article are solely those of the authors and do not necessarily represent those of their affiliated organizations, or those of the publisher, the editors and the reviewers. Any product that may be evaluated in this article, or claim that may be made by its manufacturer, is not guaranteed or endorsed by the publisher.
References
Aallam, Y., Dhiba, D., Lemriss, S., Souiri, A., Karray, F., Rasafi, T. E., et al. (2021b). Isolation and characterization of phosphate solubilizing streptomyces sp. endemic from sugar beet fields of the beni-mellal region in Morocco. Microorganisms. 9, 914. doi: 10.3390/microorganisms9050914
Aallam, Y., Maliki, B. E., Dhiba, D., Lemriss, S., Souiri, A., Haddioui, A., et al. (2021a). Multiple potential plant growth promotion activities of endemic streptomyces spp. from Moroccan sugar beet fields with their inhibitory activities against fusarium spp. Microorganisms. 9, 1429. doi: 10.3390/microorganisms9071429
Abd-Alla, M. H., El-Sayed, E.-S. A., Rasmey, A.-H. M. (2013). Indole-3-acetic acid (IAA) production by streptomyces atrovirens isolated from rhizospheric soil in Egypt. J. Biol. Earth Sci. 3, 182–193.
Abebe, T. G., Tamtam, M. R., Abebe, A. A., Abtemariam, K. A., Shigut, T. G., Dejen, Y. A., et al. (2022). Growing use and impacts of chemical fertilizers and assessing alternative organic fertilizer sources in ethiopia. Appl. Environ. Soil Sci. 2022, 4738416. doi: 10.1155/2022/4738416
Ahmad, M., Pataczek, L., Hilger, T., Zahir, Z., Hussain, A., Rasche, F., et al. (2018). Perspectives of microbial inoculation for sustainable development and environmental management. Front. Microbiol. 9. doi: 10.3389/fmicb.2018.02992
Al-Askar, A. A., Baka, Z. A., Rashad, Y. M., Ghoneem, K. M., Abdulkhair, W. M., Hafez, E. E., et al. (2015). Evaluation of streptomyces griseorubens E44G for the biocontrol of fusarium oxysporum f. sp. lycopersici: Ultrastructural and cytochemical investigations. Ann. Microbiol. 65, 1815–1824. doi: 10.1007/s13213-014-1019-4
Alori, E. T., Babalola, O. O. (2018). Microbial inoculants for improving crop quality and human health in Africa. Front. Microbiol. 9. doi: 10.3389/fmicb.2018.02213
Amaresan, N., Kumar, K., Naik, J. H., Bapatla, K. G., Mishra, R. K. (2018). “Streptomyces in plant growth promotion: mechanisms and role, chapter 8,” in New and future developments in microbial biotechnology and bioengineering, actinobacteria: Diversity and biotechnological applications. Eds. Singh, B. P., Gupta, V. K., Passari, A. K. (Amsterdam: Elsevier), 125–135. doi: 10.1016/B978-0-444-63994-3.00008-4
Amtmann, A., Armengaud, P. (2007). The role of calcium sensor-interacting protein kinases in plant adaptation to potassium-deficiency: new answers to old questions. Cell Res. 17, 483–485. doi: 10.1038/cr.2007.49
Antoun, H. (2012). Beneficial microorganisms for the sustainable use of phosphates in agriculture. Proc. Eng. 46, 62–67. doi: 10.1016/j.proeng.2012.09.446
Anwar, S., Ali, B., Sajid, I. (2016). Screening of rhizospheric actinomycetes for various in-vitro and in-vivo plant growth promoting (PGP) traits and for agroactive compounds. Front. Microbiol. 7. doi: 10.3389/fmicb.2016.01334
Archana, D. S., Nandish, M. S., Savalagi, V. P., Alagawadi, A. R. (2013). Characterization of potassium solubilizing bacteria (KSB) from rhizosphere soil. Bioinfolet Q. J. Life Sci. 10, 248–257.
Arunachalam Palaniyandi, S., Yang, S. H., Damodharan, K., Suh, J. W. (2013). Genetic and functional characterization of culturable plant-beneficial actinobacteria associated with yam rhizosphere. J. Basic Microbiol. 53, 985–995. doi: 10.1002/jobm.201200531
Balakrishna, G., Shiva Shanker, A., Pindi, P. K. (2012). Isolation of phosphate solubilizing actinomycetes from forest soils of mahabubnagar district. IOSR J. Pharm. 2, 271–275. doi: 10.9790/3013-0220271275
Barakate, M., Ouhdouch, Y., Oufdou, K., Beaulieu, C. (2002). Characterization of rhizospheric soil streptomycetes from Moroccan habitats and their antimicrobial activities. World J. Microbiol. Biotechnol. 18, 49–54. doi: 10.1023/A:1013966407890
Bashan, Y., De-Bashan, L. E., Prabhu, S., Hernandez, J.-P. (2014). Advances in plant growth-promoting bacterial inoculant technology: Formulations and practical perspectives, (1998–2013). Plant Soil. 378, 1–33. doi: 10.1007/s11104-013-1956-x
Beech, I., Paiva, M., Caus, M., Coutinho, C. (2001). “Enzymatic activities within biofilms of sulphate-reducing bacteria, chapter,” in Biofilm community interactions: Chance or necessity, BioLine. Eds. Gilbert, P. G., Allison, D., Brading, M., Verran, J., Walker, J. (Cardiff, UK: BioLine), 231–239.
Bhattacharjee, R., Dey, U. (2014). Biofertilizer, a way towards organic agriculture: A review. Afr. J. Microbiol. Res. 8, 2332–2343. doi: 10.5897/AJMR2013.6374
Bhatti, A. A., Haq, S., Bhat, R. A. (2017). Actinomycetes benefaction role in soil and plant health. Microb. Pathog. 111, 458–467. doi: 10.1016/j.micpath.2017.09.036
Biglari, N., Hassan, H. M., Amini, J. (2016). The ability of streptomyces spp. isolated from Iranian soil to solubilize rock phosphate. ABCmed. 4, 15–25.
Blanco-Vargas, A., Rodríguez-Gacha, L. M., Sánchez-Castro, N., Garzón-Jaramillo, R., Pedroza-Camacho, L. D., Poutou-Piñales, R. A., et al. (2020). Phosphate-solubilizing pseudomonas sp., and serratia sp., co-culture for allium cepa l. growth promotion. Heliyon. 6, e05218. doi: 10.1016/j.heliyon.2020.e05218
Bontemps, C., Toussaint, M., Revol, P.-V., Hotel, L., Jeanbille, M., Uroz, S., et al. (2013). Taxonomic and functional diversity of streptomyces in a forest soil. FEMS Microbiol. Lett. 342, 157–167. doi: 10.1111/1574-6968.12126
Boubekri, K., Soumare, A., Mardad, I., lyamlouli, K., Hafidi, M., Ouhdouch, Y., et al. (2021). The screening of potassium- and phosphate-solubilizing actinobacteria and the assessment of their ability to promote wheat growth parameters. Microorganisms. 9, 470. doi: 10.3390/microorganisms9030470
Buch, A., Archana, G., Gattupalli, N. K. (2008). Metabolic channeling of glucose towards gluconate in phosphate-solubilizing pseudomonas aeruginosa P4 under phosphorus deficiency. Res. Microbiol. 159, 635–642. doi: 10.1016/j.resmic.2008.09.012
Bush, M. J., Tschowri, N., Schlimpert, S., Flärdh, K., Buttner, M. J. (2015). C-di-GMP signalling and the regulation of developmental transitions in streptomycetes. Nat. Rev. Microbiol. 13, 749–760.
Carvajal-Muñoz, J., Carmona García, C. (2012). Benefits and limitations of biofertilization in agricultural practices. Livest. Res. Rural Dev. 24, 1–8.
Chaiharn, M., Pathom-aree, W., Sujada, N., Lumyong, S. (2018). Characterization of phosphate solubilizing streptomyces as a biofertilizer. Chiang Mai J. Sci. 45, 701–716.
Cheng, J., Yang, S. H., Palaniyandi, S. A., Han, J. S., Yoon, T.-M., Kim, T.-J., et al. (2010). Azalomycin f complex is an antifungal substance produced by streptomyces malaysiensis MJM1968 isolated from agricultural soil. J. Korean Soc Appl. Biol. Chem. 53, 545–552. doi: 10.3839/jksabc.2010.084
Chen, Y.-F., Wang, Y., Wu, W.-H. (2008). Membrane transporters for nitrogen, phosphate and potassium uptake in plants. J. Integr. Plant Biol. 50, 835–848. doi: 10.1111/j.1744-7909.2008.00707.x
Chouyia, F. E., Fiorentino, N., Rouphael, Y., Ventorino, V., Fechtali, T., Visconti, D., et al. (2022). Assessing the effect of p-solubilizing bacteria and mycorrhizal fungi on tomato yield and quality under different crop rotations. Sci. Hortic. 293, 110740. doi: 10.1016/j.scienta.2021.110740
Chouyia, F. E., Romano, I., Fechtali, T., Fagnano, M., Fiorentino, N., Visconti, D., et al. (2020). P-solubilizing streptomyces roseocinereus MS1B15 with multiple plant growth-promoting traits enhance barley development and regulate rhizosphere microbial population. Front. Plant Sci. 11. doi: 10.3389/fpls.2020.01137
Chukwuneme, C. F., Babalola, O. O., Kutu, F. R., Ojuederie, O. B. (2020). Characterization of actinomycetes isolates for plant growth promoting traits and their effects on drought tolerance in maize. J. Plant Interact. 15, 93–105. doi: 10.1080/17429145.2020.1752833
Dahal, B., NandaKafle, G., Perkins, L., Brözel, V. S. (2017). Diversity of free-living nitrogen fixing streptomyces in soils of the badlands of south Dakota. Microbiol. Res. 195, 31–39. doi: 10.1016/j.micres.2016.11.004
Das, S., Lyla, P. S., Ajmal Khan, S. (2008). Distribution and generic composition of culturable marine actinomycetes from the sediments of Indian continental slope of bay of Bengal. Chin. J. Ocean. Limnol. 26, 166–177. doi: 10.1007/s00343-008-0166-5
Dede, A., Güven, K., Şahi̇n, N. (2020). Isolation, plant growth-promoting traits, antagonistic effects on clinical and plant pathogenic organisms and identification of actinomycetes from olive rhizosphere. Microb. Pathog. 143, 104134. doi: 10.1016/j.micpath.2020.104134
Detraksa, J. (2020). Evaluation of plant growth–promoting streptomyces sp.SR13–2 immobilized with sugarcane bagasse and filter cake for promoting rice growth. Food Appl. Biosci. J. 8, 1–13.
Dhanasekaran, D., Thajuddin, N., Panneerselvam, A. (2008). An antifungal compound: 4′ phenyl-1-napthyl–phenyl acetamide from streptomyces sp. DPTB16. Med. Biol. 15, 7–12.
Di Mola, I., Ventorino, V., Cozzolino, E., Ottaiano, L., Romano, I., Duri, L. G., et al. (2021). Biodegradable mulching vs traditional polyethylene film for sustainable solarization: Chemical properties and microbial community response to soil management. Appl. Soil Ecol. 163, 103921. doi: 10.1016/j.apsoil.2021.103921
Djebaili, R., Pellegrini, M., Smati, M., Del Gallo, M., Kitouni, M. (2020). Actinomycete strains isolated from saline soils: Plant-growth-promoting traits and inoculation effects on solanum lycopersicum. Sustainability. 12, 4617. doi: 10.3390/su12114617
Donald, L., Pipite, A., Subramani, R., Owen, J., Keyzers, R. A., Taufa, T. (2022). Streptomyces: Still the biggest producer of new natural secondary metabolites, a current perspective. Microbiol. Res. 13, 418–465. doi: 10.3390/microbiolres13030031
Doolotkeldieva, T., Bobusheva, S., Konurbaeva, M. (2015). Effects of streptomyces biofertilizer to soil fertility and rhizosphere’s functional biodiversity of agricultural plants. Adv. Microbiol. 5, 555–571. doi: 10.4236/aim.2015.57058
Duncan, K. R., Haltli, B., Gill, K. A., Correa, H., Berrué, F., Kerr, R. G. (2015). Exploring the diversity and metabolic potential of actinomycetes from temperate marine sediments from Newfoundland, Canada. J. Ind. Microbiol. Biotechnol. 42, 57–72. doi: 10.1007/s10295-014-1529-x
El-Tarabily, K. A. (2008). Promotion of tomato (Lycopersicon esculentum mill.) plant growth by rhizosphere competent 1-aminocyclopropane-1-carboxylic acid deaminase-producing streptomycete actinomycetes. Plant Soil. 308, 161–174. doi: 10.1007/s11104-008-9616-2
Etesami, H., Emami, S., Alikhani, H. A. (2017). Potassium solubilizing bacteria (KSB): mechanisms, promotion of plant growth, and future prospects a review. J. Soil Sci. Plant Nutr. 17, 897–911. doi: 10.4067/S0718-95162017000400005
Fahad, S., Hussain, S., Bano, A., Saud, S., Hassan, S., Shan, D., et al. (2015). Potential role of phytohormones and plant growth-promoting rhizobacteria in abiotic stresses: Consequences for changing environment. Environ. Sci. pollut. Res. 22, 4907–4921. doi: 10.1007/s11356-014-3754-2
Farhat, M. B., Boukhris, I., Chouayekh, H. (2015). Mineral phosphate solubilization by streptomyces sp. CTM396 involves the excretion of gluconic acid and is stimulated by humic acids. FEMS Microbiol. Lett. 362, fnv008. doi: 10.1093/femsle/fnv008
Fatmawati, U., Meryandini, A., Nawangsih, A. A., Wahyudi, A. T. (2019). Screening and characterization of actinomycetes isolated from soybean rhizosphere for promoting plant growth. Biodivers. J. Biol. Divers. 20, 2970–2977. doi: 10.13057/biodiv/d201027
Figueiredo, M. D. B., Seldin, L., de Araujo, F. F., Mariano, R. D. R. (2010). “Plant growth promoting rhizobacteria: Fundamentals and applications,” in Plant growth and health promoting bacteria. microbiology monographs. Ed. Maheshwari, D. K. (Berlin: Springer-Verlag), 21–43. doi: 10.1007/978-3-642-13612-2_2
Fiorentino, N., Ventorino, V., Woo, S. L., Pepe, O., De Rosa, A., Gioia, L., et al. (2018). Trichoderma-based biostimulants modulate rhizosphere microbial populations and improve N uptake efficiency, yield, and nutritional quality of leafy vegetables. Front. Plant Sci. 9. doi: 10.3389/fpls.2018.00743
Ghadge, S., Patil, R. (2016). Antagonistic activity of actinomycetes isolated from soil samples of satara district, maharastra, India against selected human pathogens. Int. J. Adv. Biol. Res. 6, 188–194.
Gherbawy, Y., Elhariry, H., Altalhi, A., El-Deeb, B., Khiralla, G. (2012). Molecular screening of streptomyces isolates for antifungal activity and family 19 chitinase enzymes. J. Microbiol. 50, 459–468. doi: 10.1007/s12275-012-2095-4
Ghorbani-Nasrabadi, R., Greiner, R., Alikhani, H. A., Hamedi, J. (2012). Identification and determination of extracellular phytate-degrading activity in actinomycetes. World J. Microbiol. Biotechnol. 28, 2601–2608. doi: 10.1007/s11274-012-1069-3
Glick, B. R. (2012). Plant growth-promoting bacteria: Mechanisms and applications. Scientifica 2012, 963401. doi: 10.6064/2012/963401
Haas, H. (2003). Molecular genetics of fungal siderophore biosynthesis and uptake: the role of siderophores in iron uptake and storage. Appl. Microbiol. Biotechnol. 62, 316–330. doi: 10.1007/s00253-003-1335-2
Hamdali, H., Bouizgarne, B., Hafidi, M., Lebrihi, A., Virolle, M. J., Ouhdouch, Y. (2008a). Screening for rock phosphate solubilizing actinomycetes from Moroccan phosphate mines. Appl. Soil Ecol. 38, 12–19. doi: 10.1016/j.apsoil.2007.08.007
Hamdali, H., Hafidi, M., Virolle, M.-J., Ouhdouch, Y. (2008b). Rock phosphate-solubilizing actinomycetes: Screening for plant growth-promoting activities. World J. Microbiol. Biotechnol. 24, 2565–2575. doi: 10.1007/s11274-008-9817-0
Hamdali, H., Smirnov, A., Esnault, C., Ouhdouch, Y., Virolle, M. J. (2010). Physiological studies and comparative analysis of rock phosphate solubilization abilities of actinomycetales originating from Moroccan phosphate mines and of streptomyces lividans. Appl.Soil Ecol. 44, 24–31. doi: 10.1016/j.apsoil.2009.09.001
Hamedi, J., Mohammadipanah, F. (2015). Biotechnological application and taxonomical distribution of plant growth promoting actinobacteria. J. Ind. Microbiol. Biotechnol. 42, 157–171. doi: 10.1007/s10295-014-1537-x
Han, D., Wang, L., Luo, Y. (2018). Isolation, identification, and the growth promoting effects of two antagonistic actinomycete strains from the rhizosphere of mikania micrantha kunth. Microbiol. Res. 208, 1–11. doi: 10.1016/j.micres.2018.01.003
Hasani, A., Kariminik, A., Issazadeh, K. (2014). Streptomycetes: characteristics and their antimicrobial activities. Int. J. Adv. Biol. Biomed. Res. 2, 63–75.
Herrmann, L., Lesueur, D. (2013). Challenges of formulation and quality of biofertilizers for successful inoculation. Appl. Microbiol. Biotechnol. 97, 8859–8873. doi: 10.1007/s00253-013-5228-8
Htwe, A. Z., Moh, S. M., Soe, K. M., Moe, K., Yamakawa, T. (2019). Effects of biofertilizer produced from bradyrhizobium and streptomyces griseoflavus on plant growth, nodulation, nitrogen fixation, nutrient uptake, and seed yield of mung bean, cowpea, and soybean. Agronomy. 9, 77. doi: 10.3390/agronomy9020077
Ikeda, H., Ishikawa, J., Hanamoto, A., Shinose, M., Kikuchi, H., Shiba, T., et al. (2003). Complete genome sequence and comparative analysis of the industrial microorganism streptomyces avermitilis. Nat. Biotechnol. 21, 526–531. doi: 10.1038/nbt820
Imbert, M., Béchet, M., Blondeau, R. (1995). Comparison of the main siderophores produced by some species of streptomyces. Curr. Microbiol. 31, 129–133. doi: 10.1007/BF00294289
Ingle, K. P., Padole, D. A. (2017). Phosphate solubilizing microbes: An overview. Int. J. Curr. Microbiol. App. Sci. 6, 844–852. doi: 10.20546/ijcmas.2017.601.099
Jensen, P. R., Mincer, T. J., Williams, P. G., Fenical, W. (2005). Marine actinomycete diversity and natural product discovery. Antonie Van Leeuwenhoek. 87, 43–48. doi: 10.1007/s10482-004-6540-1
Jog, R., Nareshkumar, G., Rajkumar, S. (2012). Plant growth promoting potential and soil enzyme production of the most abundant streptomyces spp. from wheat rhizosphere. J. App. Microbiol. 113, 1154–1164. doi: 10.1111/j.1365-2672.2012.05417.x
Jog, R., Pandya, M., Nareshkumar, G., Rajkumar, S. (2014). Mechanism of phosphate solubilization and antifungal activity of streptomyces spp. isolated from wheat roots and rhizosphere and their application in improving plant growth. Microbiology. 160, 778–788. doi: 10.1099/mic.0.074146-0
Jones, D. L., Oburger, E. (2011). “Solubilization of phosphorus by soil microorganisms,” in Phosphorus in action. biological processes in soil phosphorus cycling. Eds. Bunemann, E. K., Oberson, A., Frossard, E. (Berlin; Heidelberg: Springer-Verlag), 169–198.
Jüttner, F., Watson, S. B. (2007). Biochemical and ecological control of geosmin and 2-methylisoborneol in source waters. Appl. Environ. Microbiol. 73, 4395–4406. doi: 10.1128/AEM.02250-06
Kalayu, G. (2019). Phosphate solubilizing microorganisms: promising approach as biofertilizers. Int. J. Agron. 2019, 4917256. doi: 10.1155/2019/4917256
Kaur, T., Sharma, D., Kaur, A., Manhas, R. K. (2013). Antagonistic and plant growth promoting activities of endophytic and soil actinomycetes. Arch. Phytopathol. Plant Prot. 46, 1756–1768. doi: 10.1080/03235408.2013.777169
Khamna, S., Yokota, A., Lumyong, S. (2009). Actinomycetes isolated from medicinal plant rhizospheric soils: Diversity and screening of antifungal compounds, indole-3-acetic acid and siderophore production. World J. Microbiol. Biotechnol. 25, 649–655. doi: 10.1007/s11274-008-9933-x
Khan, A. A., Jilani, G., Akhtar, M. S., Naqvi, S. S., Rasheed, M. (2009). Phosphorus solubilizing bacteria: Occurrence, mechanisms and their role in crop production. J. Agric. Biol. Sci. 1, 48–58.
Khan, M. S., Zaidi, A., Wani, P. A. (2007). Role of phosphate-solubilizing microorganisms in sustainable agriculture- A review. Agron. Sustain. Dev. 27, 29–43. doi: 10.1051/agro:2006011
Khushboo, Kumar, P., Dubey, K. K., Usmani, Z., Sharma, M., Gupta, V. K. (2022). Biotechnological and industrial applications of streptomyces metabolites. Biofuels Bioprod. Biorefining. 16, 244–264. doi: 10.1002/bbb.2294
Kishore, N., Pindi, P. K., Ram Reddy, S. (2015). “Phosphate-solubilizing microorganisms: a critical review,” in Plant biology biotechnology. Eds. Bahadur, B., Venkat Rajam, M., Sahijram, L., Krishnamurthy, K. (New Delhi: Springer). doi: 10.1007/978-81-322-2286-6_12
Kizhakedathil, M. P. J., Devi, C. S. (2018). Rhizoshpheric bacteria isolated from the agricultural fields of kolathur, tamilnadu promotes plant growth in mustard plants. Biocatal. Agric. Biotechnol. 16, 293–302. doi: 10.1016/j.bcab.2018.08.019
Kochian, L. V. (2012). Rooting for more phosphorus. Nature. 488, 466–467. doi: 10.1016/j.csbj.2020.06.024
Lee, N., Hwang, S., Kim, J., Cho, S., Palsson, B., Cho, B.-K., et al (2020). Mini review: genome mining approaches for the identification of secondary metabolite biosynthetic gene clusters in Streptomyces. Comput. Struct. Biotechnol. J. 18, 1548–1556. doi: 10.1016/j.csbj.2020.06.024
Liu, D., Lian, B., Wang, B. (2016). Solubilization of potassium containing minerals by high temperature resistant streptomyces sp. isolated from earthworm’s gut. Acta Geochim. 35, 262–270. doi: 10.1007/s11631-016-0106-6
Ma, J. F. (2005). Plant root responses to three abundant soil minerals: silicon, alu1minum and iron. Crit. Rev. Plant Sci. 24, 267–281. doi: 10.1080/07352680500196017
Mackellar, D., Lieber, L., Norman, J. S., Bolger, A., Tobin, C., Murray, J. W., et al. (2016). Streptomyces thermoautotrophicus does not fix nitrogen. Sci. Rep. 6, 20086. doi: 10.1038/srep20086
Malusá, E., Sas-Paszt, L., Ciesielska, J. (2012). Technologies for beneficial microorganisms inocula used as biofertilizers. Sci. World J. 2012, e491206. doi: 10.1100/2012/491206
Marra, L. M., Fonsêca Sousa Soares, C. R., de Oliveira, S. M., Avelar Ferreira, P. A., Lima Soares, B., de Fráguas Carvalho, R., et al. (2012). Biological nitrogen fixation and phosphate solubilization by bacteria isolated from tropical soils. Plant Soil 357, 289–307. doi: 10.1007/s11104-012-1157-z
Mehta, P., Chauhan, A., Mahajan, R., Mahajan, P. K., Shirkot, C. K. (2010). Strain of bacillus circulans isolated from apple rhizosphere showing plant growth promoting potential. Curr. Sci. 98, 538–542.
Meng, X., Chen, W.-W., Wang, Y.-Y., Huang, Z.-R., Ye, X., Chen, L.-S., et al. (2021). Effects of phosphorus deficiency on the absorption of mineral nutrients, photosynthetic system performance and antioxidant metabolism in citrus grandis. PloS One 16, e0246944. doi: 10.1371/journal.pone.0246944
Mishra, J., Arora, N. K. (2016). “Bioformulations for plant growth promotion and combating phytopathogens: A sustainable approach,” in Bioformulations: For sustainable agriculture. Eds. Arora, N. K., Mehnaz, S., Balestrini, R. (New Delhi: Springer India), 3–33. doi: 10.1007/978-81-322-2779-3_1
Mishra, P., Dash, D. (2014). Rejuvenation of biofertilizer for sustainable agriculture and economic development. Consilience. 11, 41–61.
Mohammed, A. (2020). Influence of streptomyces sp. Kp109810 on solubilization of inorganic phosphate and growth of maize (Zea mays L.). J. App. Plant Prot. 9, 17–24. doi: 10.21608/japp.2020.130644
Montella, S., Ventorino, V., Lombard, V., Henrissat, B., Pepe, O., Faraco, V. (2017). Discovery of genes coding for carbohydrate-active enzyme by metagenomic analysis of lignocellulosic biomasses. Sci. Rep. 7, 42623. doi: 10.1038/srep42623
Myo, E. M., Ge, B., Ma, J., Cui, H., Liu, B., Shi, L., et al. (2019). Indole-3-acetic acid production by streptomyces fradiae NKZ-259 and its formulation to enhance plant growth. BMC Microbiol. 19, 155. doi: 10.1186/s12866-019-1528-1
Nafis, A., Raklami, A., Bechtaoui, N., Khalloufi, F. E., Alaoui, A. E., Glick, B. R., et al. (2019). Actinobacteria from extreme niches in morocco and their plant growth-promoting potentials. Diversity 11, 139. doi: 10.3390/d11080139
Nandimath, A. P., Karad, D. D., Gupta, S. G., Kharat, A. S. (2017). Consortium inoculum of five thermo-tolerant phosphate solubilizing actinomycetes for multipurpose biofertilizer preparation. Iran. J. Microbiol. 9, 295–304.
Newitt, J. T., Prudence, S. M. M., Hutchings, M. I., Worsley, S. F. (2019). Biocontrol of cereal crop diseases using streptomycetes. Pathogens. 8, 78. doi: 10.3390/pathogens8020078
Olanrewaju, O. S., Babalola, O. O. (2019). Streptomyces: implications and interactions in plant growth promotion. Appl. Microbiol. Biotechnol. 103, 1179–1188. doi: 10.1007/s00253-018-09577-y
Pacios-Michelena, S., Aguilar González, C. N., Alvarez-Perez, O. B., Rodriguez-Herrera, R., Chávez-González, M., Arredondo Valdés, R., et al. (2021). Application of streptomyces antimicrobial compounds for the control of phytopathogens. Front. Sustain. Food Syst. 5. doi: 10.3389/fsufs.2021.696518
Park, K. H., Lee, C. Y., Son, H.-J. (2009). Mechanisms of insoluble phosphate solubilization by pseudomonas fluorescens RAF15 isolated from ginseng rhizosphere and its plant growth-promoting activities. Lett. Appl. Microbiol. 49, 222–228. doi: 10.1111/j.1472-765X.2009.02642.x
Passari, A. K., Mishra, V. K., Gupta, V. K., Yadav, M. K., Saikia, R., Singh, B. P. (2015). In vitro and in vivo plant growth promoting activities and DNA fingerprinting of antagonistic endophytic actinomycetes associates with medicinal plants. PloS One 10, e0139468. doi: 10.1371/journal.pone.0139468
Pennacchio, A., Ventorino, V., Cimini, D., Pepe, O., Schiraldi, C., Inverso, M., et al. (2018). Isolation of new cellulase and xylanase producing strains and application to lignocellulosic biomasses hydrolysis and succinic acid production. Bioresour. Technol. 259, 325–333. doi: 10.1016/j.biortech.2018.03.027
Pradhan, N., Sukla, L. B. (2006). Solubilization of inorganic phosphates by fungi isolated from agriculture soil. Afr. J. Biotechnol. 5, 850–854. doi: 10.4314/ajb.v5i10.42884
Prajapati, K., Sharma, M., Modi, H. (2013). Growth promoting effect of potassium solubilizing microorganisms on abelmoscus esculantus. Int. J. Agric. Sci. 3, 181–188.
Radhakrishnan, M., Balagurunathan, R. (2011). Antioxidant activity of melanin pigment from streptomyces species D5 isolated from desert soil. Drug Invent Today 3, 12–13.
Rashad, F. M., Fathy, H. M., El-Zayat, A. S., Elghonaimy, A. M. (2015). Isolation and characterization of multifunctional streptomyces species with antimicrobial, nematicidal and phytohormone activities from marine environments in Egypt. Microbiol. Res. 175, 34–47. doi: 10.1016/j.micres.2015.03.002
Rey, T., Dumas, B. (2017). Plenty is no plague: Streptomyces symbiosis with crops. Trends Plant Sci. 22, 30–37. doi: 10.1016/j.tplants.2016.10.008
Reyes, I., Baziramakenga, R., Bernier, L., Antoun, H. (2001). Solubilization of phosphate rocks and minerals by a wild-type strain and two UV-induced mutants of penicillium rugulosum. Soil Biol. Biochem. 33, 1741–1747. doi: 10.1016/S0038-0717(01)00099-2
Ribbe, M., Gadkari, D., Meyer, O. (1997). N2 fixation by streptomyces thermoautotrophicus involves a molybdenum-dinitrogenase and a manganese-superoxide oxidoreductase that couple N2-reduction to the oxidation of superoxide produced from O2 by a molybdenum-CO dehydrogenase. J. Biol. Chem. 272, 26627–26633. doi: 10.1074/jbc.272.42.26627
Richardson, A. E. (2001). Prospects for using soil microorganisms to improve the acquisition of phosphorus by plants. Aust. J. Plant Physiol. 28, 897–906. doi: 10.1071/pp01093
Richardson, A. E., Simpson, R. J. (2011). Soil microorganisms mediating phosphorus availability update on microbial phosphorus. Plant Physiol. 156, 989–996. doi: 10.1104/pp.111.175448
Romano, I., Ventorino, V., Ambrosino, P., Testa, A., Chouyia, F. E., Pepe, O. (2020b). Development and application of low-cost and eco-sustainable bio-stimulant containing a new plant growth-promoting strain kosakonia pseudosacchari TL13. Front. Microbiol. 11. doi: 10.3389/fmicb.2020.02044
Romano, I., Ventorino, V., Pepe, O. (2020a). Effectiveness of plant beneficial microbes: overview of the methodological approaches for the assessment of root colonization and persistence. Front. Plant Sci. 11. doi: 10.3389/fpls.2020.00006
Roy, E. D. (2017). Phosphorus recovery and recycling with ecological engineering: A review. Ecol. Eng. 98, 213–227. doi: 10.1016/j.ecoleng.2016.10.076
Rungin, S., Indananda, C., Suttiviriya, P., Kruasuwan, W., Jaemsaeng, R., Thamchaipenet, A. (2012). Plant growth enhancing effects by a siderophore-producing endophytic streptomycete isolated from a Thai jasmine rice plant (Oryza sativa l. cv. KDML105). Antonie Van Leeuwenhoek 102, 463–472.
Sabaratnam, S., Traquair, J. A. (2015). Mechanism of antagonism by streptomyces griseocarneus (strain Di944) against fungal pathogens of greenhouse-grown tomato transplants. Can. J. Plant Pathol. 37, 197–211. doi: 10.1080/07060661.2015.1039062
Sadeghi, A., Karimi, E., Dahaji, P. A., Javid, M. G., Dalvand, Y., Askari, H. (2012). Plant growth promoting activity of an auxin and siderophore producing isolate of streptomyces under saline soil conditions. World J. Microbiol. Biotechnol. 28, 1503–1509. doi: 10.1007/s11274-011-0952-7
Sellstedt, A., Richau, K. H. (2013). Aspects of nitrogen-fixing actinobacteria, in particular free-living and symbiotic frankia. FEMS Microbiol. Lett. 342, 179–186. doi: 10.1111/1574-6968.12116
Sharan, A., Darmwal, N. S. (2008). Efficient phosphorus solubilization by mutant strain of xanthomonas campestris using different carbon, nitrogen and phosphorus sources. World J. Microbiol. Biotechnol. 24, 3087–3090. doi: 10.1007/s11274-008-9807-2
Sharma, S. B., Sayyed, R. Z., Trivedi, M. H., Gobi, T. A. (2013). Phosphate solubilizing microbes: sustainable approach for managing phosphorus deficiency in agricultural soils. SpringerPlus 2, 587. doi: 10.1186/2193-1801-2-587
Shepherd, M. D., Kharel, M. K., Bosserman, M. A., Rohr, J. (2010). Laboratory maintenance of streptomyces species. Curr. Protoc. Microbiol 18, 10E–1. doi: 10.1002/9780471729259.mc10e01s18
Sheridan, C., Depuydt, P., De Ro, M., Petit, C., Van Gysegem, E., Delaere, P., et al. (2017). Microbial community dynamics and response to plant growth-promoting microorganisms in the rhizosphere of four common food crops cultivated in hydroponics. Microb. Ecol. 73, 378–393. doi: 10.1007/s00248-016-0855-0
Shi, T.-Q., Peng, H., Zeng, S.-Y., Ji, R.-Y., Shi, K., Huang, H., et al. (2017). Microbial production of plant hormones: Opportunities and challenges. Bioengineered. 8, 124–128. doi: 10.1080/21655979.2016.1212138
Shivlata, L., Satyanarayana, T. (2015). Thermophilic and alkaliphilic actinobacteria: biology and potential applications. Front. Microbiol. 6. doi: 10.3389/fmicb.2015.01014
Shrivastava, P., Kumar, R. (2018). “Actinobacteria: Eco-friendly candidates for control of plant diseases in a sustainable manner, chapter 5,” in New and future developments in microbial biotechnology and bioengineering. Eds. Singh, B. P., Gupta, V. K., Passari, A. K. (Elsevier), 79–91. doi: 10.1016/B978-0-444-63994-3.00005-9
Singh, S. P., Gaur, R. (2016). Evaluation of antagonistic and plant growth promoting activities of chitinolytic endophytic actinomycetes associated with medicinal plants against sclerotium rolfsii in chickpea. J. Appl. Microbiol. 121, 506–518. doi: 10.1111/jam.13176
Singh, R. P., Manchanda, G., Maurya, I. K., Maheshwari, N. K., Tiwari, P. K., Rai, A. R. (2019). Streptomyces from rotten wheat straw endowed the high plant growth potential traits and agro-active compounds. Biocatal. Agric. Biotechnol. 17, 507–513. doi: 10.1016/j.bcab.2019.01.014
Som, N. F., Heine, D., Holmes, N., Knowles, F., Chandra, G., Seipke, R. F., et al. (2017). The MtrAB two-component system controls antibiotic production in streptomyces coelicolor A3(2). Microbiology 163, 1415–1419. doi: 10.1099/mic.0.000524
Soumare, A., Boubekri, K., Lyamlouli, K., Hafidi, M., Ouhdouch, Y., Kouisni, L. (2020). From isolation of phosphate solubilizing microbes to their formulation and use as biofertilizers: status and needs. Front. Bioeng. Biotechnol. 7. doi: 10.3389/fbioe.2019.00425
Suárez-Moreno, Z. R., Vinchira-Villarraga, D. M., Vergara-Morales, D. I., Leonardo, C., Ramos, F. A., Corrado, G., et al. (2019). Plant-growth promotion and biocontrol properties of three streptomyces spp. isolates to control bacterial rice pathogens. Front. Microbiol. 10. doi: 10.3389/fmicb.2019.00290
Suksaard, P., Pathom-aree, W., Duangmal, K. (2017). Diversity and plant growth promoting activities of actinomycetes from mangroves. Chiang Mai J. Sci. 44, 1210–1223.
Toro, M. (2007). “Phosphate solubilizing microorganisms in the rhizosphere of native plants from tropical savannas: An adaptive strategy to acid soils?,” in Developments in plant and soil sciences. Eds. Velázquez, E., Rodríguez-Barrueco, C. (Dordrecht: Springer Netherlands), 249–252. doi: 10.1007/978-1-4020-5765-6_37
Toumatia, O., Yekkour, A., Goudjal, Y., Riba, A., Coppel, Y., Mathieu, F., et al. (2015). Antifungal properties of an actinomycin d-producing strain, streptomyces sp. IA1, isolated from a Saharan soil. J. Basic Microbiol. 55, 221–228. doi: 10.1002/jobm.201400202
Trujillo-Roldán, M. A., Valdez-Cruz, N. A., Gonzalez-Monterrubio, C. F., Acevedo-Sánchez, E. V., Martínez-Salinas, C., García-Cabrera, R. I., et al. (2013). Scale-up from shake flasks to pilot-scale production of the plant growth-promoting bacterium azospirillum brasilense for preparing a liquid inoculant formulation. Appl. Microbiol. Biotechnol. 97, 9665–9674. doi: 10.1007/s00253-013-5199-9
Urrutia, O., Erro, J., Guardado, I., Francisco, S. S., Mandado, M., Baigorri, R., et al. (2014). Physico-chemical characterization of humic-metal-phosphate complexes and their potential application to the manufacture of new types of phosphate-based fertilizers. J. Soil Sci. Plant Nutr. 177, 128–136. doi: 10.1002/jpln.201200651
Vargas Hoyos, H. A., Chiaramonte, J. B., Barbosa-Casteliani, A. G., Fernandez Morais, J., Perez-Jaramillo, J. E., Nobre Santos, S., et al. (2021). An actinobacterium strain from soil of cerrado promotes phosphorus solubilizatio and plant growth in soybean plants. Front. Bioeng. Biotechnol. 9, 579906.
Ventorino, V., Chiurazzi, M., Aponte, M., Pepe, O., Moschetti, G. (2007). Genetic diversity of a natural population of rhizobium leguminosarum bv. viciae nodulating plants of vicia faba in the vesuvian area. Curr. Microbiol. 55, 512–517. doi: 10.1007/s00284-007-9024-5
Ventorino, V., Ionata, E., Birolo, E., Montella, S., Marcolongo, L., De Chiaro, A., et al. (2016). Lignocellulose-adapted endo-cellulase producing streptomyces strains for bioconversion of cellulose-based materials. Front. Microbiol. 7. doi: 10.3389/fmicb.2016.02061
Ventorino, V., Pascale, A., Fagnano, M., Adamo, P., Faraco, V., Rocco, C., et al. (2019). Soil tillage and compost amendment promote bioremediation and biofertility of polluted area. J. Clean. Prod. 239, 118087. doi: 10.1016/j.jclepro.2019.118087
Wahyudi, A., Priyanto, J., Afrista, R., Kurniati, D., Astuti, R., Akhdiya, A. (2019). Plant growth promoting activity of actinomycetes isolated from soybean rhizosphere. Online J. Biol. Sci. 19, 1–8. doi: 10.3844/ojbsci.2019.1.8
Walpola, B. C., Yoon, M. H. (2012). Prospectus of phosphate solubilizing microorganisms and phosphorus availability in agricultural soils: a review. Afr. J. Microbiol. Res. 6, 6600–6605. doi: 10.5897/AJMR12.889
Wang, M, Xue, J, Ma, J, Feng, X, Ying, H., Xu, H., et al (2020). Streptomyces lydicus M01 regulates soil microbial community and alleviates foliar disease caused by alternaria alternata on cucumbers. Front. Microbiol. 11, 942. doi: 10.3389/fmicb.2020.00942
Wu, H., Qu, S., Lu, C., Zheng, H., Zhou, X., Bai, L., et al. (2012). Genomic and transcriptomic insights into the thermo-regulated biosynthesis of validamycin in streptomyces hygroscopicus 5008. BMC Genomics 13, 337. doi: 10.1186/1471-2164-13-337
Zaidi, A., Khan, M., Ahemad, M., Oves, M. (2009). Plant growth promotion by phosphate solubilizing bacteria. Acta Microbiol. Immunol. Hung. 56, 263–284. doi: 10.1556/AMicr.56.2009.3.6
Keywords: phosphate-solubilizing Streptomyces, plant growth-promoting rhizobacteria, bioinoculant, biofertilizer, biostimulant, biocontrol
Citation: Chouyia FE, Ventorino V and Pepe O (2022) Diversity, mechanisms and beneficial features of phosphate-solubilizing Streptomyces in sustainable agriculture: A review. Front. Plant Sci. 13:1035358. doi: 10.3389/fpls.2022.1035358
Received: 02 September 2022; Accepted: 21 November 2022;
Published: 06 December 2022.
Edited by:
Amri Ismail, National Center for Nuclear Science and Technology, TunisiaReviewed by:
Vishal Tripathi, GLA University, IndiaCamila Gazolla Volpiano, Albert Einstein College of Medicine, United States
Copyright © 2022 Chouyia, Ventorino and Pepe. This is an open-access article distributed under the terms of the Creative Commons Attribution License (CC BY). The use, distribution or reproduction in other forums is permitted, provided the original author(s) and the copyright owner(s) are credited and that the original publication in this journal is cited, in accordance with accepted academic practice. No use, distribution or reproduction is permitted which does not comply with these terms.
*Correspondence: Valeria Ventorino, valeria.ventorino@unina.it