- 1State Key Laboratory of Desert and Oasis Ecology, Xinjiang Institute of Ecology and Geography, Chinese Academy of Sciences, Urumqi, China
- 2University of Chinese Academy of Sciences, Beijing, China
- 3Research Center for Ecology and Environment of Central Asia, Xinjiang Institute of Ecology and Geography, Chinese Academy of Sciences, Urumqi, China
The chemical profile of Ajania tibetica essential oil (EO) and its phytotoxic, insecticidal, and antimicrobial activities were assessed. Monoterpenes (79.05%) and sesquiterpenes (10.33%) were dominant in the EO, with camphor, (+/-)-lavandulol and eucalyptol being the major constituents, representing 55.06% of the total EO. The EO possessed potent phytotoxicity against Poa annua and Medicago sativa starting from 0.5 mg/mL, and when the concentration rose to 5 mg/mL, seed germination of both tested species was 100% suppressed. Ajania tibetica EO displayed significant pesticidal activity against Aphis gossypii with an LC50 value of 17.41 μg/mL; meanwhile, the EO also showed antimicrobial activity against Escherichia coli, Bacillus subtilis, Verticillium dahlia and Aspergillus niger using broth microdilution and disc diffusion methods. For the tested bacterial and fungal strains, the EO exhibited a repressing effect, with minimum inhibitory concentrations (MICs) ranging from 0.3125 to 1.25 mg/mL for bacteria and from 1.25 to 2.5 mg/mL for fungi, whereas the minimum microbicidal concentrations (MMCs) were 5 mg/mL for bacteria and 2.5 mg/mL for fungi. Our study is the first report on the chemical profile as well as the phytotoxicity, insecticidal and antimicrobic activity of A. tibetica EO, indicating its potential value as an alternative synthetic pesticide.
Introduction
Synthetic chemicals are extensively used in productive activities in agriculture worldwide, but their extensive application has resulted in many challenges, such as the evolution of weed or pest resistance, soil or groundwater pollution, and especially harm to human health. Compared with synthesized compounds, natural products can be alternatives due to their rapid biological degradation ability, low-risk evolution of pest or weed resistance, and weak toxicity to living organisms (Isman, 2015; Pavela and Benelli, 2016).
As natural products, essential oils (EOs), which are secondary metabolites synthesized in plants, have been widely used in safeguarding medical and food applications for many hundreds of years (Suteu et al., 2020; Giunti et al., 2021). As complex volatile liquids, EOs are usually obtained by cold pressing, steam distillation, or mechanical processes (Ferhat et al., 2007) and contain a high diversity of terpenoids and derivatives. The yield and constituents of EOs depend on climatic, ecological, and harvesting period effects, species gene, and extraction technology (Burt, 2004; Wissal et al., 2016). Most aromatic plants produce a large quantity of EOs, which can kill pests and sterilize or suppress the growth of weeds (Insawang et al., 2019; Saleh et al., 2020; Aungtikun et al., 2021; Sousa et al., 2021). Previous reports have demonstrated that certain EOs can produce phytotoxic activity against plants, affecting their seed germination as well as root and shoot growth of seedlings (Dutra et al., 2020; Vasconcelos et al., 2022), causing changes of their protective enzymes’ activity and chlorophyll content (Kong et al., 2021; Han et al., 2021); in addition, the cytotoxicity and aneugenic potential of EOs were evidenced by the reduction of the mitotic index and the presence of chromosomal and nuclear alterations (Singh et al., 2020; Valente et al., 2022). Owing to these properties, some EOs, which are extracted from aromatic plants, have the potential value to be further used as environmentally friendly alternatives to synthesized insecticides, weedicides, or bactericides. As a successful commercial example, clove oil was a main active ingredient in the Burnout II herbicide (Bonide Products Inc., Oriskany, NY, USA). Another commercial product, “Rice Weevil Eradication” (producer: Hub Club, Siheung, Korea), contains an active ingredient of cinnamon (Cinnamomum cassia Bark) oil (Yang et al., 2020).
Ajania, a genus of the Compositae family, comprises approximately 30 species that are perennial herbs or small semishrubs. Most Ajania species are aromatic and can be used as folk medicine; moreover, they have been used in dispelling wind and sedation, clearing heat, relieving cough, reducing swelling and bleeding, diminishing inflammation and itching, and repelling mosquitoes and killing insects (Wu et al., 2015; Wangchuk et al., 2016; Shepherd et al., 2018; Wangchuk et al., 2018). The EOs of Ajania species, including A. nubigena (Wallich ex Candolle) C. Shih, A. fruticulosa (Ledeb.) Poljak., A. przewalskii Poljak., and A. semnanensis Sonboli, have been confirmed to possess antimicrobial activities (Wangchuk et al., 2013; Salehi et al., 2015; Sampietro et al., 2017); moreover, it have been reported that the EOs of Ajania species also display other activities, including insecticidal and antagonistic activity (from A. nitida Shih, A. nematoloba (Hand.-Mazz.) Ling et Shih, A. potaninii (Krasch.) Poljak. and A. fruticulosa) (Li et al., 2018; Liang et al., 2018; Shao et al., 2021).
Ajania tibetica (Hook.f. & Thomson) Tzvelev is distributed in the Tibet, Sichuan and Xinjiang provinces of China, growing at an elevation of approximately 3000-5000 m. It has also been discovered in India and some countries of Central Asia, such as Pakistan, Tajikistan, Kazakhstan, Afghanistan, Kyrgyzstan, and the Syrian Arab Republic (https://www.cvh.ac.cn; https://www.gbif.org). It was reported that A. tibetica was a controversial taxonomic status and should be merged into Phaeostigma resulting from the pollen morphology of representative species of Phaeostigma and Ajania genera (Huang et al., 2017). Some reports have shown that A. tibetica and Ceratoides compacta (Losinsk.) Tsien et C. G. Ma are the main species forming the alpine desert vegetation in the Qaidam Basin, which lives at 2600~3200 m in altitude (Wu et al., 2011) and is also frequently found in sandy gravelly deserts (Hou, 1983). Although there have been some reports regarding the research of A. tibetica species as above, very little is known about the physiological and biochemical characteristics of A. tibetica.
To date, the chemical compositions and bioactivity characteristics of A. tibetica EO are unknown. The purposes of our study were as follows: (i) to evaluate the chemical profile of A. tibetica EO and (ii) to determine the phytotoxicity and insecticidal and antimicrobial activity of A. tibetica EO. It is expected to lay a foundation for utilizing A. tibetica EO as a botanical herbicide, insecticide or fungicide.
Materials and methods
Experimental material
The aerial parts of A. tibetica at the flowering stage were sampled from Taxkorgan County in southeastern Pamir Plateau, Xinjiang Province, China, in August 2021. Plant samples were air-dried in the laboratory before they were used. It was identified by Dr. Cao Qiumei from Xinjiang Institute of Ecology and Geography, Chinese Academy Sciences, and a voucher specimen was deposited at the herbarium of Xinjiang Institute of Ecology and Geography, Chinese Academy of Sciences.
Seeds of Medicago sativa L. and Poa annua L. were collected in suburban Urumqi city in 2020 and were surface sterilized using 75% alcohol for 3 min, followed by rinsing with distilled water 3 times before the phytotoxic activity assay (Han et al., 2021)
Aphis gossypii Glover was collected from Solanum nigrum L. plants and used as a tested pest prepared for the detection of insecticidal activity of A. tibetica EO.
The antimicrobial activity of A. tibetica EO against bacteria (Bacillus subtilis (Ehrenberg) Cohn CICC 21897, Escherichia coli (Migula) Castellani et Chalmers CICC 10305) and fungi (Aspergillus niger van Tiegh CICC 41255, Verticillium dahlia Kleb. V991) was measured. The V. dahlia strain was isolated from the rhizosphere soil of cotton plants growing in Urumqi, Xinjiang, and identified by Dr. Yang Honglan according to its morphological characteristics combined with molecular identification, and kept at Xinjiang Institute of Ecology and Geography, Chinese Academy Sciences, China. Other strains were purchased from the China Center of Industrial Culture Collection, CICC (http://m.China-cicc.org).
EO extraction
Ajania tibetica EO was obtained using the steam distillation method with a Clevenger apparatus for 4 h. The extracted EO was stored in a brown vial at 4°C for further study. The A. tibetica EO production was determined by the following formula:
The constituents of the EO extracted from A. tibetica plants were detected by a 7890A/5975C gas chromatography−mass spectrometry (GC/MS) system (Agilent Technologies, Palo Alto, CA, USA) equipped with an HP-5MS (5%-phenyl)-methylpolysiloxane phase column (30 m×0.25 mm; film thickness 0.25 μm) with helium, a carrier gas, with a flow rate of 1 mL/min. The oven temperature was initiated at 50°C for 10 min then programmed from 50°C to 120°C at a rate of 1.5°C/min; from 120°C to 240°C at a rate of 20°C/min and then maintained at this temperature for 5 min. Mass spectra were taken at 70 eV. Mass range was m/z 35–600 Da. The temperature of both detector and injector were held at 280°C. The compounds were determined by comparing their mass spectra and retention indices (RIs) with the data stored in the NIST database (National Institute of Standards and Technology). The retention index was calculated using linear interpolation relative to retention times of a standard mixture of C7–C40 n-alkanes, as following formula: RI=100[n + (N - n) x (Log RT (unknown) – log RT (n))/log RT(N) – log RT (n)], was used; where n=no. of carbon atoms in the smaller alkane, N=no. of carbon atoms of the larger alkane, RT=retention time of the individual compound (Han et al., 2021; Zhou et al., 2021).
Phytotoxic activity
Poa annua and M. sativa were used to examine the phytotoxic effect of the EO. Ajania tibetica EO was dissolved in Tween 20 (final concentration 0.1%) to obtain solutions at 0.25, 0.5, 1, 2, and 5 mg/mL for the bioassay. Three milliliters of solution were spread onto each Petri dish (9 cm in diameter), and distilled water containing 0.1% Tween-20 was used as the control, followed by sowing 20 seeds of the test weeds. Petri dishes were placed in a growth incubator at 25°C with a 16 h/8 h light/dark photoperiod for 7 days. The seedlings of M. sativa and P. annua were measured after 7 days. There were 3 repetitions for the assay, and a total of 60 seedlings were measured for each treatment.
Insecticidal Activity
The insecticidal activity of A. tibetica EO was assessed according to Zhou et al. (2021)’s method with minor modifications. Ajania tibetica EO was dissolved in 0.1% Tween-20 solution to achieve concentrations of 5, 10, 20, 40, 80, and 100 μg/mL suspension, which were then impregnated into the paper discs (Whatman #2, USA, 1 × 1 cm). The paper discs were then tapped into the inner side of each Petri dish lid (9 cm in diameter) to separate the EO from A. gossypii. Thirty adults of A. gossypii were placed on a fresh healthy leaf of S. nigrum plants on a layer of wet filter paper. Petri dishes were sealed using Parafilm® film and placed in a growth incubator set at 25°C temperature and a photoperiod of 16 h/8 h light/dark for 2 days. The lethal rate of A. gossypii adults was tested at 24 h intervals after treatment. Each treatment was performed in triplicate.
Antimicrobial activity
Diffusion method
The inhibitory effect of A. tibetica EO was evaluated using the disc diffusion method according to Lu et al. (2018) with minor modifications. All bacteria were cultured in Luria-Bertani (LB) agar medium at 37°C for 24 h, while fungi were cultured in potato dextrose agar (PDA) at 28°C for 7 days to obtain the fungal spore solution. The active bacteria were prepared in LB broth to obtain 1× 108 colony forming units/mL; the active fungal spores were also cultivated on potato dextrose broth (PDB) to obtain 1× 108 colony forming units/mL using the cell counting in the blood ball counting board; then, one hundred microliters of bacterial/fungal broth were spread on the surface of the agar plates prepared previously. One milliliter of A. tibetica EO solutions with concentrations of 5, 10, 20 and 40 µg/mL prepared in 0.1% Tween-20 was impregnated on serialized 5 mm diameter paper discs (Whatman#2, USA), which were then placed in agar plates (the controls received 0.1% Tween-20 solution) and incubated at 37°C for 24 h for bacteria and 28°C for 48 h for fungi. The diameter of the zone inhibition was measured. Each treatment was conducted in triplicate.
Determination of MIC and MMC
The minimum inhibitory concentration (MIC) and the minimal microbicidal concentration (MMC) were confirmed using Teh et al. (2017)’s method with some modifications. The EO was prepared in 0.1% Tween-20 to yield the following concentrations: 0.3125, 0.625, 1.25, 2.5, 5, and 10 mg/mL. The reaction was achieved by mixing 100 µL of different concentration solutions with 100 µL of microbial suspension on a 96-well microtiter plate; the controls received 200 µL of 0.1% Tween-20 solutions. The fungal and bacterial plates were then incubated at 28°C for 48 h and 37°C for 24 h, respectively. The optical density (OD) value of the mixed solution was measured at an absorbance of 600 nm by a multimode microplate reader (Varioskan® Flash, Thermo Fisher Scientific Technology Co., Ltd, China). The MIC was detected by considering the OD values of the mixed solutions compared with those of the controls. Meanwhile, MMC was also confirmed by a mixed solution from the well with relatively low OD values and spreading it on LB or PDA plates to incubate at 37°C for 24 h for bacteria and 28°C for 48 h for fungi, thereby determined by assessing the plates without microbial colonies. Three replicates were prepared for each treatment.
Statistical analysis
One-way ANOVA (P< 0.05) was applied to measure the phytotoxic, insecticidal and antimicrobial activities of the EO at different concentrations using SPSS statistics software (IBM SPSS Statistics for Windows, Version 23.0). PROBIT analysis was used to calculate moderate inhibition/lethal (IC50) values for the inhibitory/lethal concentration (SAS/STAT User’s Guide; SAS Institute Inc., Cary, NC, USA).
Results
Yield and Composition of A. tibetica EO
Ajania tibetica EO was extracted from the dry aboveground plant materials by the traditional steam distillation method. The yield was 0.01% (v/w, volume/dry weight). Eventually, forty-nine constituents were confirmed, which shared 95.93% of the total oil, whereas 4.07% of the oil remained unclassified (Table 1). The major compounds were camphor (29.76%), (+/-)-lavandulol (13.23%), and eucalyptol (12.07%), which represented 55.06% of the total oil. In general, the EO was composed of 79.05% monoterpenes (including 11.41% monoterpene hydrocarbons and 67.64% oxygenated monoterpenes) and 10.33% sesquiterpenes (including 0.18% sesquiterpene hydrocarbons and 10.15% oxygenated sesquiterpenes) (Table 1).
Phytotoxicity Bioassay
The phytotoxicity of the EO (concentrations ranged from 0 to 5 mg/mL) was estimated by comparing their plant growth regulating effect on M. sativa and P. annua. In general, the EO impacted the growth of the tested plants in a dose-dependent manner. Root development of M. sativa and P. annua was promoted at a concentration of 0.25 mg/mL and then reduced with increasing EO concentration, although the EO started to inhibit shoot growth of M. sativa and P. annua at 0.25 mg/mL. Under the treatment with 0.5 mg/mL, significant inhibition of root and shoot development was observed in both test species. Meanwhile, the seed germination of both tested plants was completely suppressed at the highest concentration of 5 mg/mL tested (Figure 1). In detail, M. sativa and P. annua root length were promoted by 37.89% and 101.40% at the concentration of 0.25 mg/mL EO, respectively. When the treatment with 0.5, 1, 2, and 5 mg/mL EO was compared to the control, the root length of M. sativa significantly declined by 7.94%, 60.71%, 85.12%, and 100%, respectively, and the root length of P. annua decreased by 47.88%, 93.17%, 100%, and 100%, respectively (Figure 1). Similarly, M. sativa shoot length was significantly inhibited by 9.14%, 33.88%, 62.09%, 89.33% and 100%, and P. annua shoot length was suppressed by 29.48%, 52.44%, 93.72%, 100%, and 100% when treated with 0.25, 0.5, 1, 2, and 5 mg/mL EO in comparison to the control. The EO showed an inhibitory effect with IC50 values of 0.865, 0.729, 0.53, and 0.402 mg/mL on the root and shoot growth of M. sativa and P. annua, respectively (Figures 1, 2). The dose–response curve of the phytotoxic activity is shown in Figure 2.
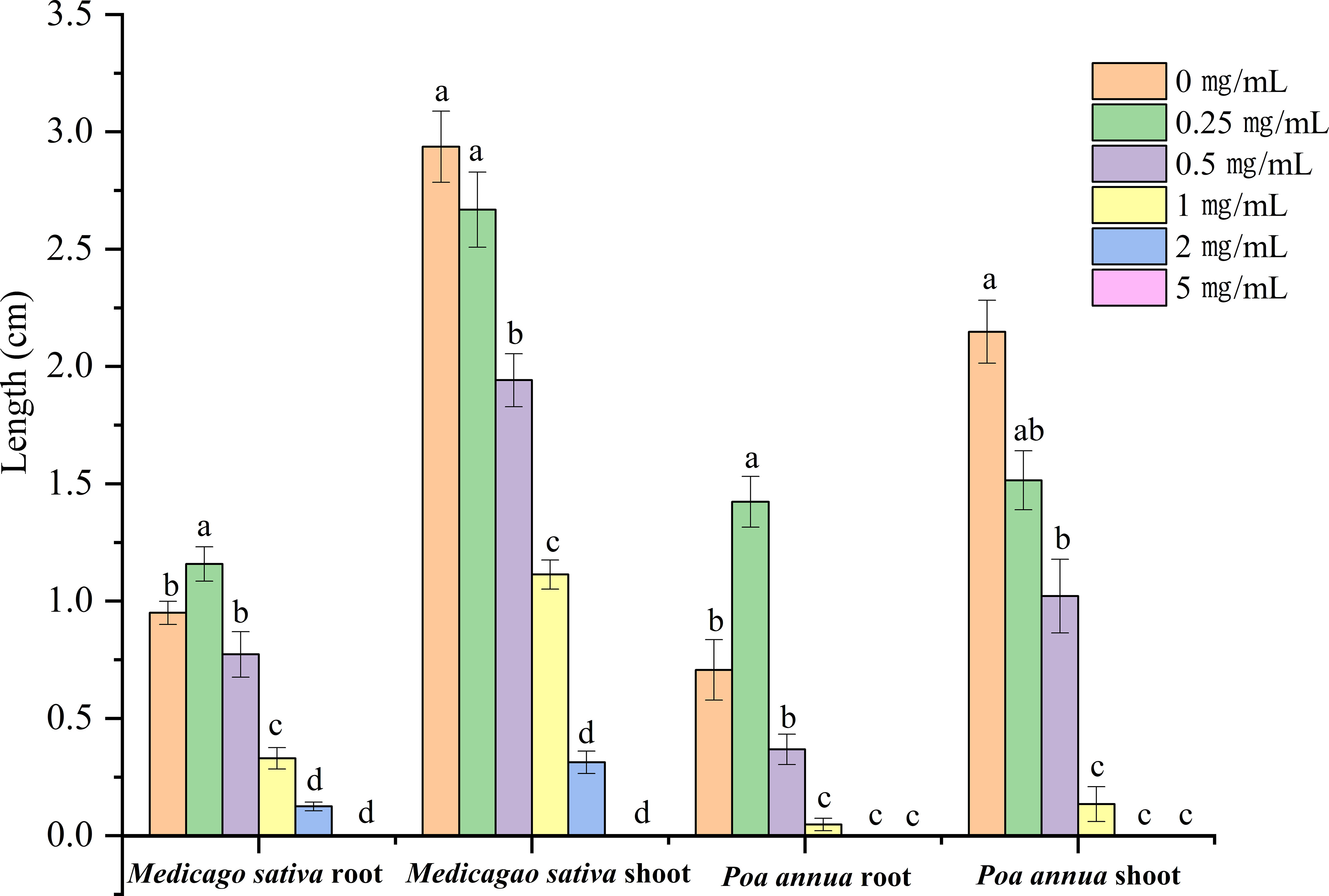
Figure 1 Phytotoxic effects of A. tibetica EO on seedling growth of P. annua and M. sativa (n = 60). Different letters represent a significant difference at P< 0.05 level according to Fisher’s LSD test.
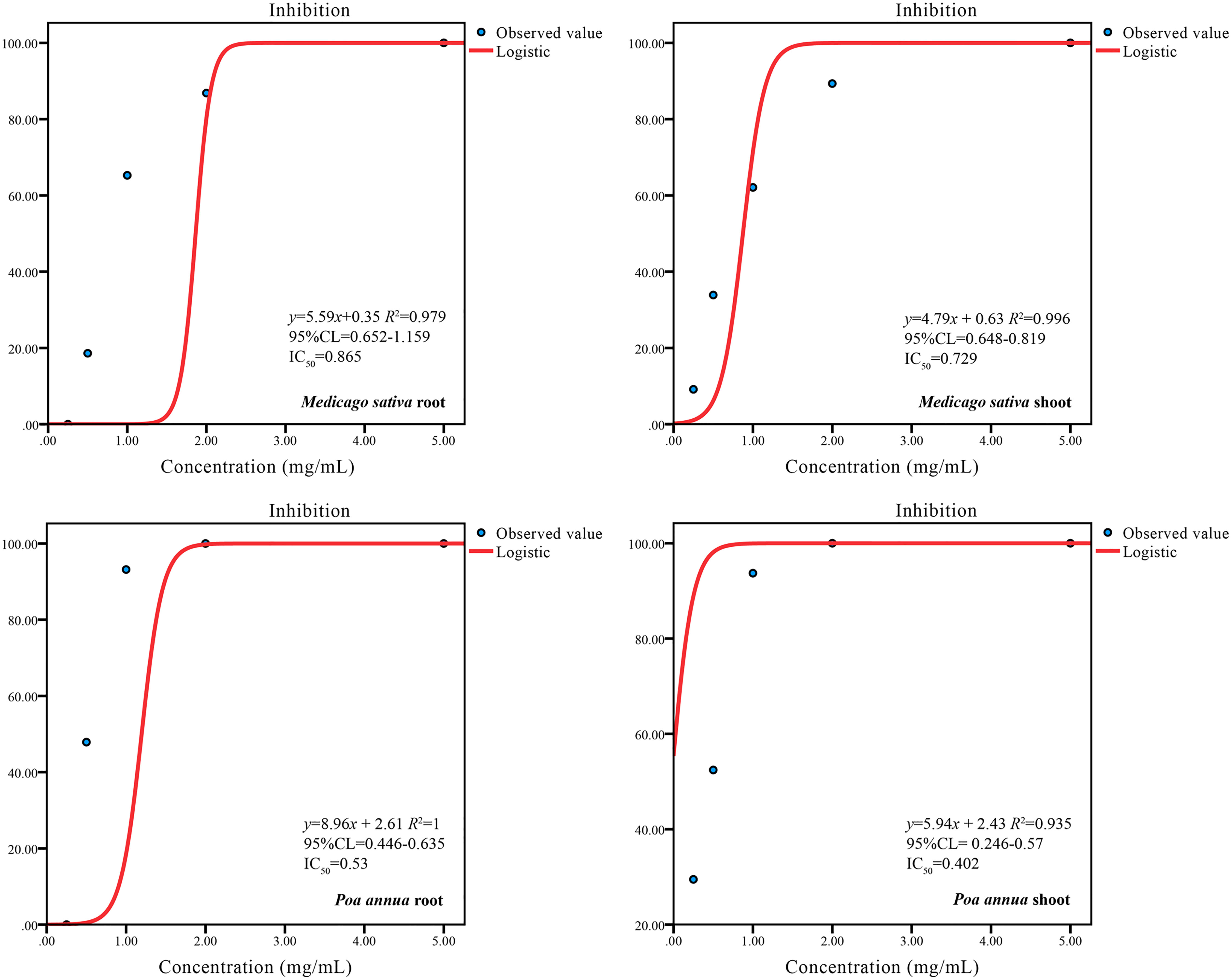
Figure 2 Dose–response curves of A. tibetica EO on seedling growth of M. sativa and P. annua. R2adj: adjusted coefficient of determination. IC50: 50% inhibit concentration of bested plants. 95% CL: 95% confidence limits.
Pesticidal Activity
The pesticidal activity of A. tibetica EO was assessed on adjusted mortality rates of A. gossypii at concentrations ranging from 0 to 100 μg/mL. The results showed that the EO exerted lethal effects and induced obvious behavioral avoidance in A. gossypii. The EO completely killed all the tested insects at a dose of 100 μg/mL after 24 h of exposure. The mortality rates of A. tibetica EO reached 21.11%, 32.22%, 48.89%, 76.67%, and 90.00%, respectively, under 5, 10, 20, 40, and 80 μg/mL EO treatments for 24 h of application. The EO exhibited significant pesticidal activity against A. gossypii with an LC50 value of 17.41 μg/mL (Figure 3). The dose–response curve of the insecticidal activity is shown in Figure 3.
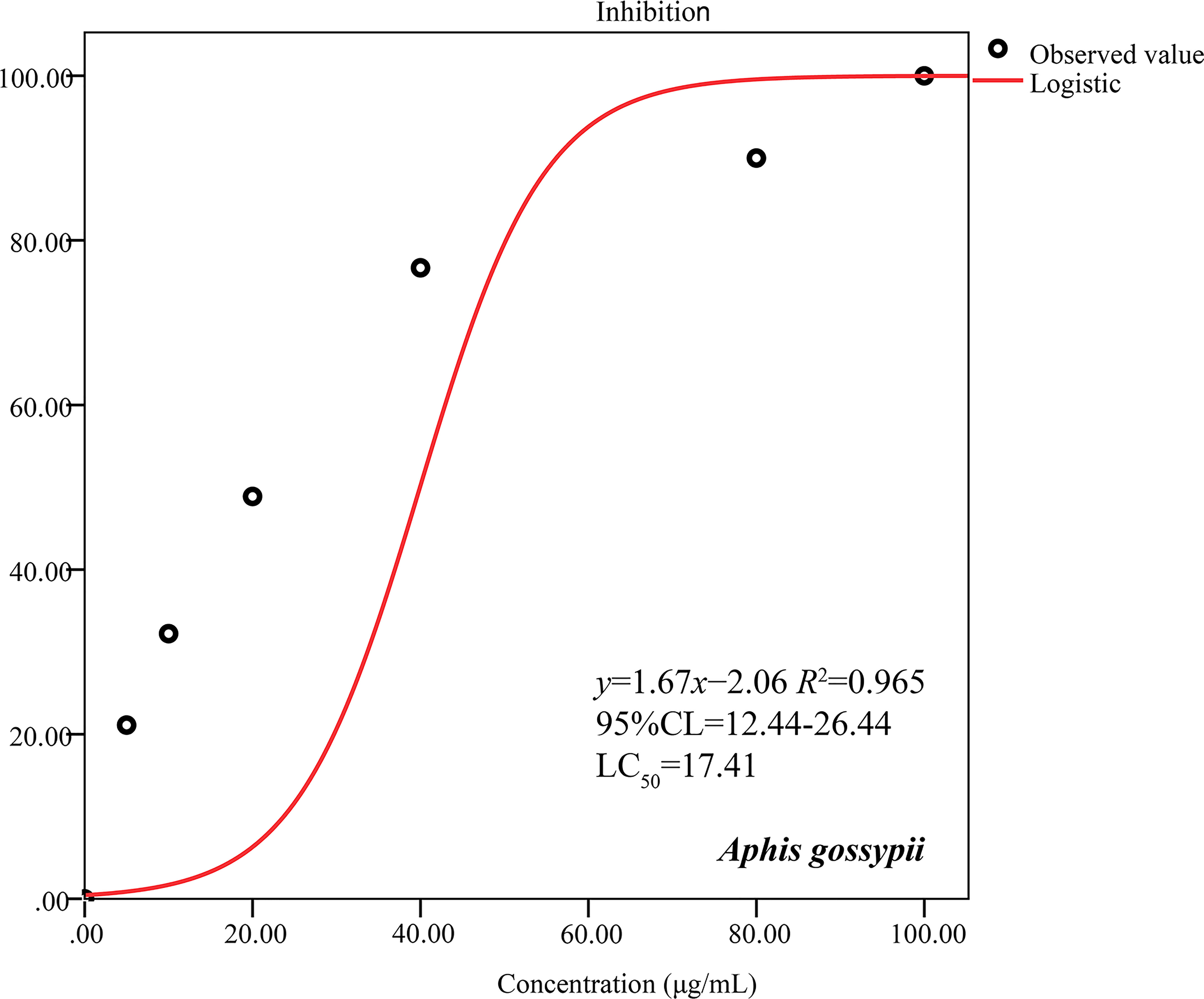
Figure 3 Dose–response curves of A. tibetica EO against A. gossypii adults. R2adj: adjusted coefficient of determination. LC50: 50% lethal concentration of A. gossypii. 95% CL: 95% confidence limits.
Antimicrobial activity
The antimicrobial activity of A. tibetica EO against 4 microorganisms was estimated using both the disc diffusion and broth microdilution methods. All the tested microorganisms were suppressed by the EO, as the diameter of the zone of inhibition significantly increased with increasing EO concentration. The results from the disc diffusion method indicated that B. subtilis of the bacterial strains was the most sensitive to the high concentration of 40 µg/mL of A. tibetica EO with a zone diameter of 1.15 mm compared to those obtained from E. coli with 1.03 mm; in addition, V. dahliae with a zone diameter of 1.21 mm in the fungal strains was more sensitive than A. niger with 0.93 mm at the high concentration of 40 µg/mL of A. tibetica EO (Figure 4). In addition, the optical density (OD) values of antimicrobial activity of A. tibetica EO against all the test microorganisms declined with increasing concentration, which also showed an inhibitory effect of the EO on the tested microorganisms. The optical density (OD) values of all the tested microorganisms were significantly reduced at a concentration of 2.5 mg/mL EO (Figure 5). The IC50 values of the EO inhibited B. subtilis, E. coli, A. niger, and V. dahliae were 1.004, 3.705, 2.533, and 1.536 mg/mL, respectively (Figure 6). The dose–response curve of the antimicrobial activity is shown in Figure 6. The MICs for B. subtilis, E. coli, A. niger, and V. dahliae were 0.3125, 1.25, 2.5, and 1.25 mg/mL, respectively; the MIC of the bacteria ranged from 0.3125 to 1.25 mg/mL, whereas the MIC for fungi ranged from 1.25 to 2.5 mg/mL. The results of the MMC test indicated that A. tibetica EO had MMC values of 2.5 and 5 mg/mL for all tested bacteria and fungi, respectively.
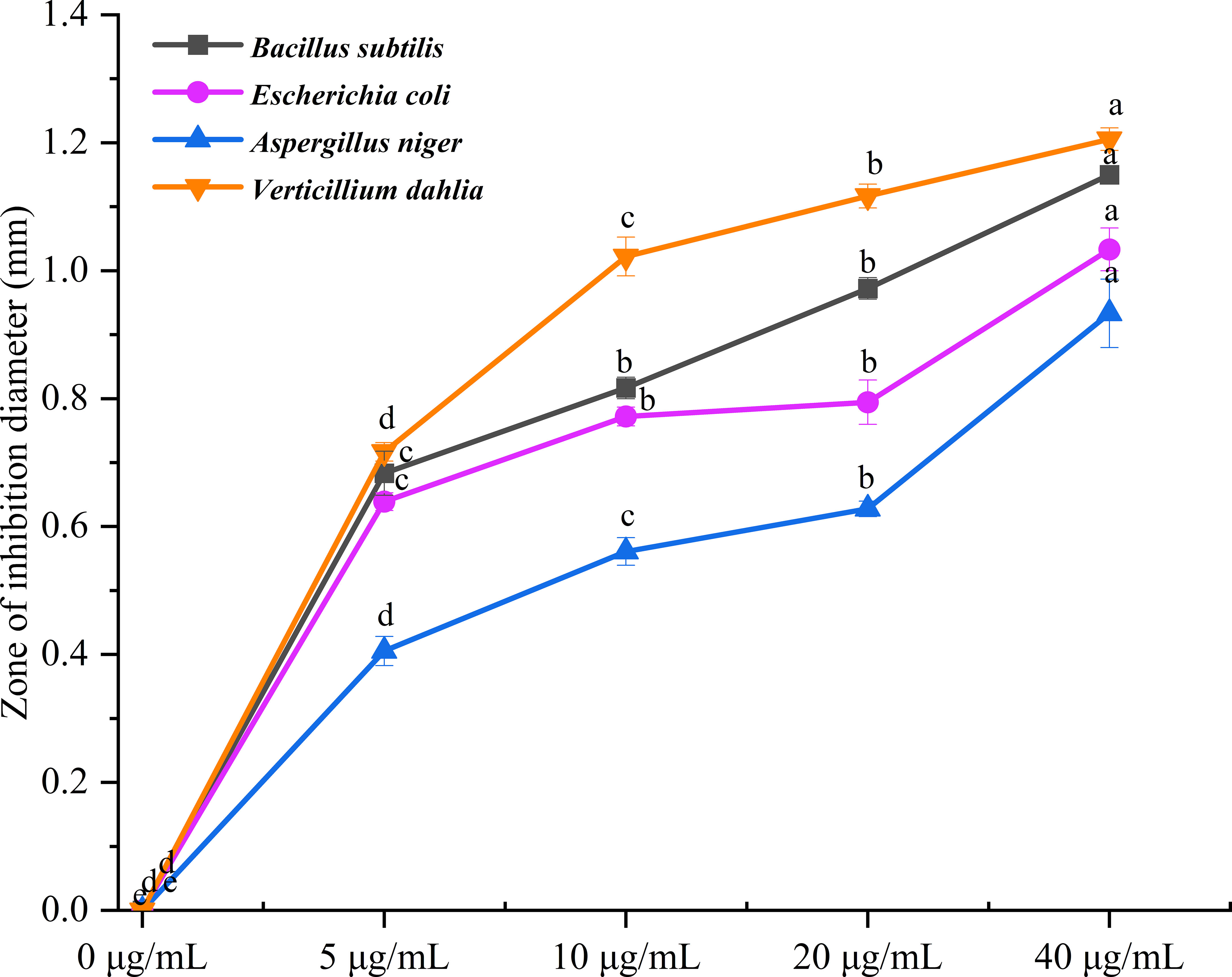
Figure 4 Antimicrobial activity of A. tibetica EO. Different letters indicated significant differences (P< 0.05) level according to Fisher’s LSD test.
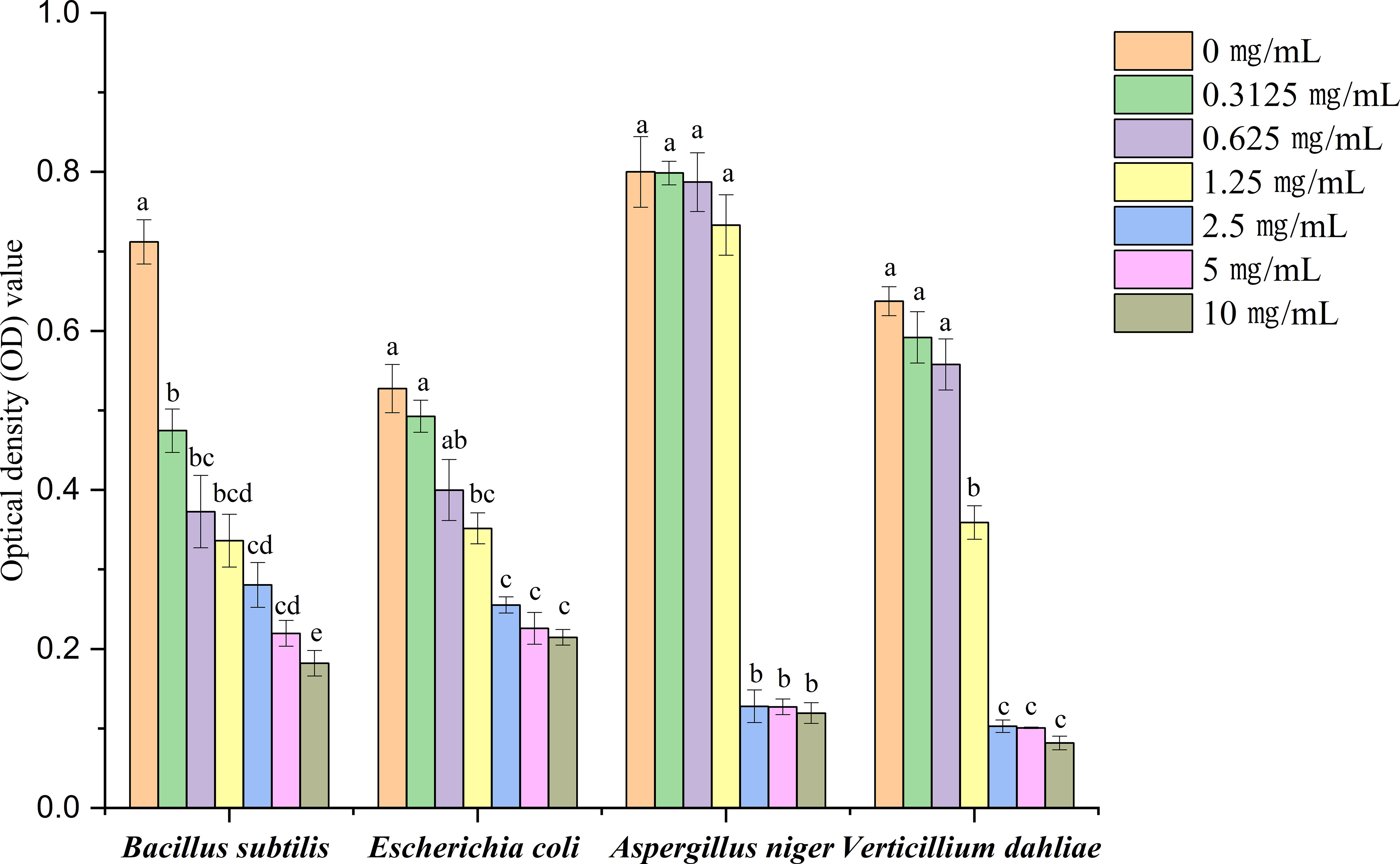
Figure 5 The optical density (OD) value on antimicrobial activity of A. tibetica EO. Different letters indicated significant differences (P< 0.05) level according to Fisher’s LSD test.
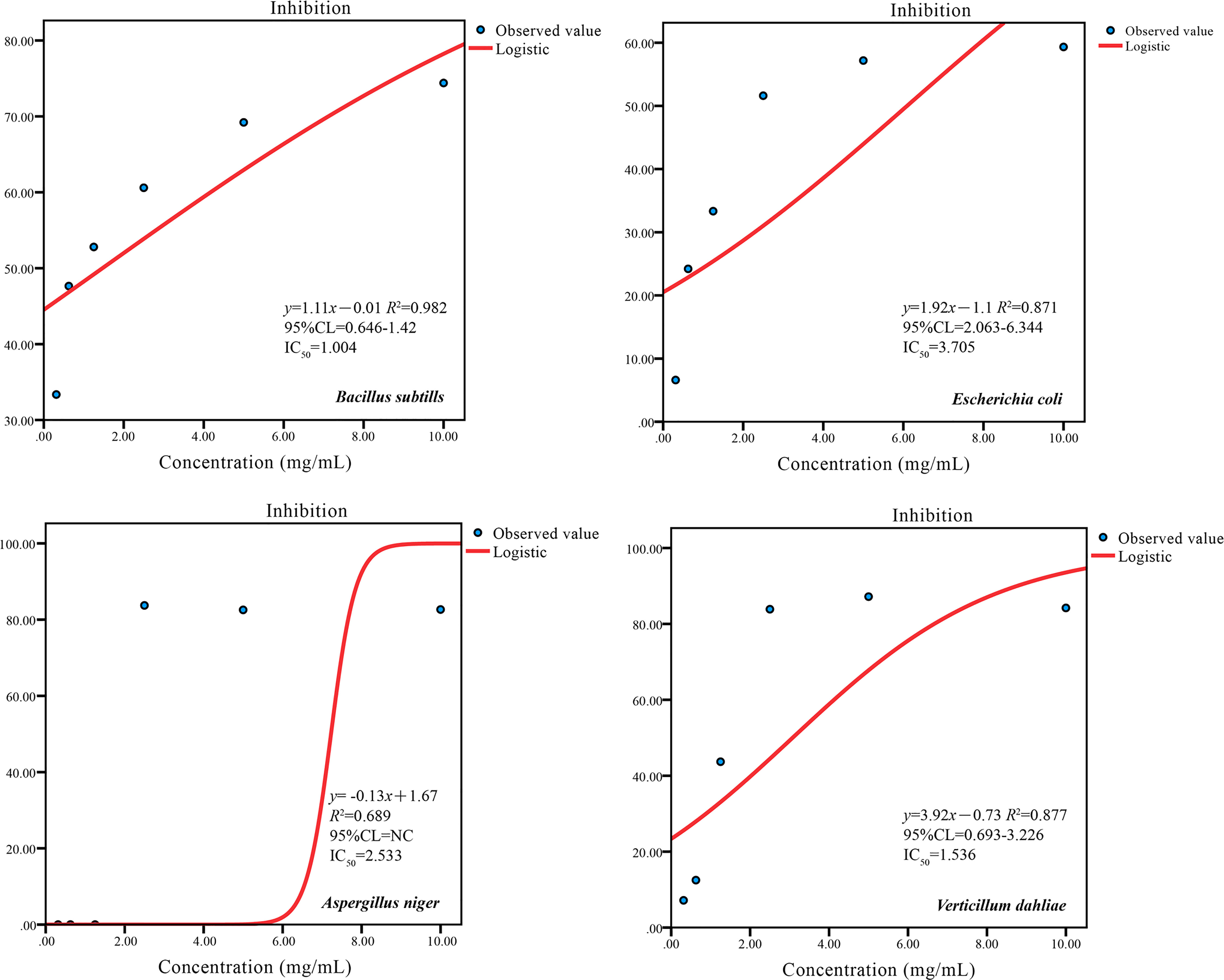
Figure 6 Dose–response curves of A. tibetica EO against tested microorganisms. R2adj: adjusted coefficient of determination. IC50: 50% inhibit concentration of microorganisms. 95% CL: 95% confidence limits; NC, not calculable.
Discussion
The results on the chemical profile of EO obtained from A. tibetica were distinct from other species of the Ajania genus in previous reports. Previously, 1,8-cineole and camphor were determined to be the main chemical constituents in no less than 5 species of Ajania plants (Shatar et al., 2010; Salehi et al., 2015; Shao et al., 2021). The EO of A. nematoloba revealed beta-pinene (34.72%), eucalyptol (24.97%), and verbenol (20.39%) as the major compounds, whereas the main components of A. nitida EO were camphor (20.76%), thujone (18.64%), eucalyptol (13.42%) and borneol (8.32%); there were differences in terms of type and amount of main component in the EOs of A. nematoloba and A. nitida (Li et al., 2018). In addition, myrcene (19.1%), 1,8-cineole (34.2%), and –pinene (9.4%) were found to be the main compounds in A. fruticulosa EO reported by Sampietro et al. (2017), while Liang et al. (2016) previously revealed that the main constituents of A. fruticulosa EO were myrtenol (8.15%), (+)-camphor (32.10%), and 1,8-cineole (41.40%). Hence, it was demonstrated that there were also differences in the principal components of essential oils extracted from the same A. fruticulosa species.
Similarly, in the present work, the chemical composition of A. tibetica EO was different from other Ajania species. For instance, the main constituents of A. tibetica EO were camphor, (+/-)-lavandulol, and eucalyptol, compared with A. nitida EO whose major constituents were camphor, thujone, eucalyptol and borneol; the relative percentage of eucalyptol in A. tibetica EO (12.07%) was less than that in A. nitida EO (13.42%) and A. nematoloba EO (24.97%), respectively, whereas camphor was the most abundant component in A. tibetica EO (29.76%), compared with 20.76% camphor in A. nitida EO reported by Li et al. (2018). Moreover, previous studies have demonstrated the diversity of essential oil profiles of Ajania plants; for example, Liang et al. (2016) described that the content of 1,8-cineole was 41.4% in A. fruticulosa EO growing in China, while it was 34.2% in those cultivated in Kazakhstan by Sampietro et al. (2017). On the other hand, Salehi et al. (2015) found that the chemical composition of A. semnanensis EO varied with the different growth stages. These results revealed that species belonging to the same genus usually have specific volatile components. Meanwhile, a number of biotic and abiotic factors including growing stages, geography, light, temperature, water, nutrient conditions, climatic conditions, etc. might also affect the EOs’ chemical profiles, thereby leading to differences in the biosynthetic pathways of the plant, chemotypes, compounds, and contents (Zheng et al., 1999; Han et al., 2021).
It has been reported that plant-derived EOs and their constituents possess phytotoxic activity against seed germination and seedling growth of tested species (Langenheim, 1994; Vokou et al., 2003; Salamci et al., 2007; Abd-Elgawad et al., 2021). However, to the best of our knowledge, the phytotoxic activity of EOs obtained from Ajania plants have not yet been evaluated. In the present work, we found A. tibetica EO exhibited inhibitory effects on the tested species in a dose-dependent manner. Under 0.5 and 1 mg/mL A. tibetica EO treatment, the root length of P. annua decreased by 47.88% and 93.17%, respectively. In comparison, glyphosate as a commercial herbicide presented stronger phytotoxic effect, inhibiting P. annua’s root elongation by 79.08% at 0.25 mg/mL and 93.44% at 0.5 mg/mL (Wei et al., 2020). For the dicot plant M. sativa, A. tibetica EO suppressed its root length by 7.94%, 60.71%, 85.12%, and 100% under 0.5, 1, 2, and 5 mg/mL treatment respectively, which was similar to the inhibitory effect of Artemisia absinthium EO and Ambrosia artemisiifolia EO on root growth of M. sativa in previous reports by Jiang et al. (2021) and Han et al. (2021); however, at the dose of 0.25mg/mL, A. tibetica EO promoted M. sativa root length by 37.89%, which were completely different from the suppressing effect of A. absinthium EO and A. artemisiifolia EO. These results revealed that A. tibetica EO exhibited different biological activity compared with other species. Additionally, the phytotoxic activity of A. tibetica EO could be ascribed to the diversity of chemical constituents in A. tibetica EO, especially the monoterpene compounds (79.05%) compared with sesquiterpenes (10.33%), which were the main class of terpenoids in A. tibetica EO. Some earlier studies reported that monoterpenes, including monoterpene hydrocarbons and oxygenated monoterpenes, suppressed the growth of many crops and weeds (Lopez et al., 2008; Li et al., 2011; Ali et al., 2015); it was also reported that the phytotoxic activity of oxygenated monoterpenes could be much stronger than that of monoterpene hydrocarbons (Kordali et al., 2007; Amri et al., 2017). Moreover, it was confirmed that the monoterpenes camphor (29.76%) and eucalyptol (12.07%), the main compounds of A. tibetica EO, exhibited phytotoxic activity against the tested plants in previous reports (Shao et al., 2018). It has also been reported that sesquiterpene compounds possessed strong phytotoxic potential; for example, roots treated with farnesene was negatively affected with obvious tissue and cellular alterations and morphological modifications (Araniti et al., 2016). Therefore, it needs to be further confirmed whether the observed phytotoxicity of A. tibetica EO is attributed to the monoterpene compounds in the EO.
Phytochemicals play pivotal roles in pest management action for agricultural sustainability (Duke et al., 2003; Boulogne et al., 2012; Gahukar, 2014). Previously, EOs obtained from Ajania species were confirmed to possess pesticidal activity, such as, A. nematoloba and A. nitida EO showed contact toxicity with LD50 values of 102.29 and 30.10 µg/adult, respectively, and fumigant toxicity with LC50 values of 69.45 and 20.07 mg/L, respectively, against the red flour beetle Tribolium castaneum Herbst after 24 h of exposure (Li et al., 2018). Ajania potaninii EO was also evaluated for pesticidal activity against Plodia interpunctella Hubner, which is a major pest of many economically storage crops (Shao et al., 2021). Our study also found that A. tibetica EO exhibited significant pesticidal activity against A. gossypii with an LC50 value of 17.41 μg/mL for 24 h of application. In addition, A. fruticulosa EO also exposed contact effects with LD50 values of 89.85 g/cm2 and 105.67 g/adult and fumigant effects with LC50 values of 0.65 and 11.52 mg/L on Liposcelis bostrychophila Badonnel and T. castaneum adults for 24 h exposure; moreover, (+)-camphor of its most common compounds exhibited a strong fumigant effect with an LC50 of 0.43 mg/L on L. bostrychophila (Liang et al., 2016). Similarly, eucalyptol as a monoterpene compound with insecticidal activity, was detected to have a significant contact effect with an LD50 of 76.97 μL/mL on the larvae of Plutella xylostella L. after 24 h of exposure and strong fumigant activity with an LC50 of 3.25 μL/mL against P. xylostella adults (Gharib et al., 2020; Huang et al., 2021). Hence, future works is necessary to evaluate whether camphor and eucalyptol are the major components playing a critical role in the insecticidal activity of the EO. Meanwhile, previous studies found that A. gossypii was susceptible to diverse EOs. For instance, Santalum austrocaledonicum Vieill. EO showed insecticidal activity of 94.0% mortality against A. gossypii infesting hot peppers (Roh et al., 2015). It has also been found that EOs of Pistacia lentiscus L. and Mentha pulegium L. exhibited insecticidal activities against A. gossypii, resulting in 70% and 94% mortality rates and LC50 values of 759 and 478 ppm, respectively; there was no difference from the toxic effect of the chemical insecticide imidacloprid used as the positive control against A. gossypii (Behi et al., 2019), By comparison, A. tibetica EO exerted stronger insecticidal activity than imidacloprid. However, it has been found that Melaleuca styphelioides Smith EO showed strong fumigant toxicity of 100% mortality against A. gossypii adults and nymphs at a concentration of 263.18 μL/L air EO (Albouchi et al., 2018); these results illustrated that A. tibetica EO showed much weaker activity than M. styphelioides EO. Therefore, future work should focus on comparing the strength of the insecticidal activity of A. tibetica EO with commercial pesticides.
The antimicrobial activity of EOs of Ajania plants against bacteria and fungi has been previously examined. Ajania semnanensis EO exhibited inhibitory effects on bacteria (E. coli, B. subtilis, B. cereus, Staphylococcus aureus) and fungi (Candida albicans), showing better inhibitory effects of EOs on fungi than bacteria (Salehi et al., 2015). Unlike reported by Salehi et al. (2015), A. tibetica EO exhibited antimicrobial activity with the order of E. coli>A. niger>V. dahliae>B. subtilis according to IC50 values of 3.705, 2.533, 1.536 and 1.004 mg/mL, respectively, which didn’t show an effect pattern on activity on fungi and bacteria. Additionally, A. nubigena EO exhibited moderate antifungal activity against C. albicans and strong antibacterial activity against B. subtilis (compared with the standard, amoxicillin) with minimum inhibition zones of 11 mm and 13 mm, respectively (Wangchuk et al., 2013). Relatively, A. tibetica EO exposed much weaker antimicrobial activity against B. subtilis with a zone diameter of 1.15 mm than A. nubigena EO and amoxicillin. Moreover, A. fruticulosa EO also showed an inhibitory effect on strains of Fusarium verticillioides, F. graminearum, A. niger and A. carbonarius, while showing no antifungal effects on both Aspergillus strains and weak antimicrobial activity against both Fusarium strains (Sampietro et al., 2017). These reports demonstrate that EOs from Ajania species have different antibacterial activities. Ajania tibetica EO showed an inhibitory effect on B. subtilis, E. coli, A. niger, and V. dahlia according to the definition of antimicrobic activity of the natural product by Holetz et al. (2002). The antimicrobial activity of A. tibetica EO might also be attributed to the synergism of the EO constituents. Therefore, additional studies will be needed to unravel how the components of A. tibetica EO play a functional role in antimicrobial activity against the microorganisms.
Conclusion
The present study demonstrated the phytotoxic, insecticidal and antimicrobial potential of A. tibetica EO; in particular, the EO displayed potent suppressive effect on the test weeds and insect, implying that it has the potential to be further explored as eco-friendly agrochemicals for the management of weeds and insects. Future studies should focus on the bioactivity of single/combined constituents of the EO to determine the strength of each compound, and whether synergistic effect occur when different constituents work together; on the other hand, the EO’s phytotoxic effect on other weed species as well as the crops should also be evaluated under field conditions.
Data availability statement
The original contributions presented in this study are included in the article/supplementary material. Further inquiries can be directed to the corresponding author.
Author contributions
CH developed the idea for research, with extensive discussion with SZ and YM. CH performed the bioactivity experiments and analyzed its results. SZ, YM and QC collected all experimental material and identified the specimen of A. tibetica species plant. KS conducted the analyses relating to antimicrobial activity of A. tibetica essential oil. HS edited the manuscript. All authors contributed to the article and approved the submitted version.
Funding
This research was funded by the National Natural Science Foundation of China (U2003214), the Second Tibetan Plateau Scientific Expedition and Research (STEP) Program(2019QZKK0502), and the Third Xinjiang Scientific Expedition Program (2022xjkk1505).
Conflict of interest
The authors declare that the research was conducted in the absence of any commercial or financial relationships that could be construed as a potential conflict of interest.
Publisher’s note
All claims expressed in this article are solely those of the authors and do not necessarily represent those of their affiliated organizations, or those of the publisher, the editors and the reviewers. Any product that may be evaluated in this article, or claim that may be made by its manufacturer, is not guaranteed or endorsed by the publisher.
References
Abd-Elgawad, A. M., El Gendy, A. E. G., Assaeed, A. M., Al-Rowaily, S. L., Alharthi, A. S., Mohamed, T. A., et al. (2021). Phytotoxic effects of plant essential oils: a systematic review and structure-activity relationship based on chemometric analyses. Plants-Basel 10, 36. doi: 10.3390/plants10010036
Albouchi, F., Ghazouani, N., Souissi, R., Abderrabba, M., Boukhris-Bouhachem, S. (2018). Aphidicidal activities of Melaleuca styphelioides sm. essential oils on three citrus aphids: Aphis gossypii glover; Aphis spiraecola patch and Myzus persicae (Sulzer). South Afr. J. Bot. 117, 149–154. doi: 10.1016/j.sajb.2018.05.005
Ali, I. B., Chaouachi, M., Bahri, R., Chaieb, I., Boussaid, M., Harzallah-Skhiri, F. (2015). Chemical composition and antioxidant, antibacterial, allelopathic and insecticidal activities of essential oil of Thymus algeriensis boiss. et reut. Ind. Crops Products 77, 631–639. doi: 10.1016/j.indcrop.2015.09.046
Amri, I., Hanana, M., Jamoussi, B., Hamrouni, L. (2017). Essential oils of Pinus nigra J.F. Arnold subsp. laricio maire: Chemical composition and study of their herbicidal potential. Arabian J. Chem. 10, S3877–S3882. doi: 10.1016/j.arabjc.2014.05.026
Araniti, F., Grana, E., Krasuska, U., Bogatek, R., Reigosa, M. J., Abenavoli, M. R., et al. (2016). Loss of gravitropism in farnesene-treated arabidopsis is due to microtubule malformations related to hormonal and ROS unbalance. PloS One 11, e0160202. doi: 10.1371/journal.pone.0160202
Aungtikun, J., Soonwera, M., Sittichok, S. (2021). Insecticidal synergy of essential oils from Cymbopogon citratus (Stapf.), Myristica fragrans (Houtt.), and Illicium verum hook. f. and their major active constituents. Ind. Crops Products 164, 113386. doi: 10.1016/j.indcrop.2021.113386
Behi, F., Bachrouch, O., Boukhris-Bouhachem, S. (2019). Insecticidal activities of Mentha pulegium l., and Pistacia lentiscus l., essential oils against two citrus aphids Aphis spiraecola patch and Aphis gossypii glover. J. Essential Oil Bearing Plants 22, 516–525. doi: 10.1080/0972060X.2019.1611483
Boulogne, I., Petit, P., Ozier-Lafontaine, H., Desfontaines, L., Loranger-Merciris, G. (2012). Insecticidal and antifungal chemicals produced by plants: a review. Environ. Chem. Lett. 10, 325–347. doi: 10.1007/s10311-012-0359-1
Burt, S. (2004). Essential oils: their antibacterial properties and potential applications in foods - a review. Int. J. Food Microbiol 94, 223–253. doi: 10.1016/j.ijfoodmicro.2004.03.022
Duke, S. O., Baerson, S. R., Dayan, F. E., Rimando, A. M., Scheffler, B. E., Tellez, M. R., et al. (2003). United states department of agriculture - agricultural research service research on natural products for pest management. Pest Manage. Sci. 59, 708–717. doi: 10.1080/03601234.2013.795839
Dutra, Q. P., Christ, J. A., Carrijo, T. T., Alves, T. D., Alves, T. D., Mendes, L. A., et al. (2020). Phytocytotoxicity of volatile constituents of essential oils from sparattanthelium mart. species (Hernandiaceae). Sci. Rep. 10, 12213. doi: 10.1038/s41598-020-69205-6
Ferhat, M. A., Meklati, B. Y., Chemat, F. (2007). Comparison of different isolation methods of essential oil from citrus fruits: cold pressing, hydrodistillation and microwave ‘dry’ distillation. Flavour Fragrance J. 22, 494–504. doi: 10.1038/s41598-020-69205-6
Gahukar, R. T. (2014). Factors affecting content and bioefficacy of neem (Azadirachta indica a. juss.) phytochemicals used in agricultural pest control: A review. Crop Prot. 62, 93–99. doi: 10.1016/j.cropro.2014.04.014
Gharib, R., Ben Jemaa, J. M., Charcosset, C., Fourmentin, S., Greige-Gerges, H. (2020). Retention of eucalyptol, a natural volatile insecticide, in delivery systems based on hydroxypropyl-beta-cyclodextrin and liposomes. Eur. J. Lipid Sci. Technol. 122, 1900402. doi: 10.1002/ejlt.201900402
Giunti, G., Campolo, O., Laudani, F., Zappala, L., Palmeri, V. (2021). Bioactivity of essential oil-based nano-biopesticides toward Rhyzopertha dominica (Coleoptera: Bostrichidae). Ind. Crops Products 162, 113257. doi: 10.1016/j.indcrop.2021.113257
Han, C. X., Shao, H., Zhou, S. X., Mei, Y., Cheng, Z. R., Huang, L., et al. (2021). Chemical composition and phytotoxicity of essential oil from invasive plant, Ambrosia artemisiifolia l. Ecotoxicol0 Environ. Saf. 211, 111879. doi: 10.1016/j.ecoenv.2020.111879
Holetz, F. B., Pessini, G. L., Sanches, N. R., Cortez, D. A. G., Nakamura, C. V., Dias, B. P. (2002). Screening of someplants used in the Brazilian folk medicine for the treat-ment of infectious diseases. Memórias do Instituto Oswaldo Cruz 97, 1027–1031. doi: 10.5539/ijb.v3n4p13
Hou, X. Y. (1983). Vegetation of China with reference to its geographical distribution. Ann. Missouri Botanical Garden 70, 509–549. doi: 10.2307/2992085
Huang, Y., An, Y. M., Meng, S. Y., Guo, Y. P., Rao, G. Y. (2017). Taxonomic status and phylogenetic position of Phaeostigma in the subtribe artemisiinae (Asteraceae). J. Systematics Evol. 55, 426–436. doi: 10.1111/jse.12257
Huang, X., Huang, Y. L., Yang, C. Y., Liu, T. T., Liu, X., Yuan, H. B. (2021). Isolation and insecticidal activity of essential oil from Artemisia lavandulaefolia DC. against Plutella xylostella. Toxins 13, 842. doi: 10.3390/toxins13120842
Insawang, S., Pripdeevech, P., Tanapichatsakul, C., Khruengsai, S., Monggoot, S., Nakham, T., et al. (2019). Essential oil compositions and antibacterial and antioxidant activities of five Lavandula stoechas cultivars grown in Thailand. Chem. Biodiver. 16, e1900371. doi: 10.1002/cbdv.201900371
Isman, M. B. (2015). A renaissance for botanical insecticides? Pest Manage. Sci. 71, 1587–1590. doi: 10.1002/cbdv.201900371
Jiang, C. Y., Zhou, S. X., Liu, L., Zokir, T., Huang, L., Shi, K., et al. (2021). Evaluation of the phytotoxic effect of the essential oil from artemisia absinthium. Ecotoxicol0 Environ. Saf. 226, 112856. doi: 10.1016/j.ecoenv.2021.112856
Kong, Q. B., Zhou, L. J., Wang, X. J., Luo, S. Y., Li, J. J., Xiao, H. Y., et al. (2021). Chemical composition and allelopathic effect of essential oil of litsea pungens. Agronomy-Basel 11 (6), 1115. doi: 10.3390/agronomy11061115
Kordali, S., Cakir, A., Sutay, S. (2007). Inhibitory effects of monoterpenes on seed germination and seedling growth. Z. Fur Naturforschung Section C-a J. Biosci. 62, 207–214. doi: 10.1515/znc-2007-3-409
Langenheim, J. H. (1994). Higher-plant terpenoids - a phytocentric overview of their ecological roles. J. Chem. Ecol. 20, 1223–1280. doi: 10.1007/BF02059809
Liang, J. Y., Guo, S. S., You, C. X., Zhang, W. J., Wang, C. F., Geng, Z. F., et al. (2016). Chemical constituents and insecticidal activities of Ajania fruticulosa essential oil. Chem. Biodiver. 13, 1053–1057. doi: 10.1002/cbdv.201500377
Liang, J. Y., Liu, Y., Zhang, X. X., Zhang, L. J., Chen, Y., Li, Y., et al. (2018). Antagonistic activity of essential oils and their main constituents extracted from Ajania fruticulosa and A. potaninii against Ditylenchus destructor. Nematology 20, 911–916. doi: 10.1163/15685411-00003185
Li, J., Liu, X. G., Dong, F. S., Xu, J., Li, Y. B., Shan, W. L., et al. (2011). Potential allelopathic effects of volatile oils from Descurainia sophia (L.) Webb ex prantl on wheat. Biochem. Systematics Ecol. 39, 56–63. doi: 10.1016/j.bse.2010.12.022
Li, Y., Yan, S. S., Wang, J. J., Li, L. Y., Zhang, J., Wang, K., et al. (2018). Insecticidal activities and chemical composition of the essential oils of Ajania nitida and Ajania nematoloba from China. J. Oleo Sci. 67, 1571–1577. doi: 10.5650/jos.ess18102
Lopez, M. L., Bonzani, N. E., Zygadlo, J. A. (2008). Allelopathic potential of Tagetes minuta terpenes by a chemical, anatomical and phytotoxic approach. Biochem. Systematics Ecol. 36, 882–890. doi: 10.1016/j.bse.2008.11.003
Lu, W. C., Huang, D. W., Wang, C. C. R., Yeh, C. H., Tsai, J. C., Huang, Y. T., et al. (2018). Preparation, characterization, and antimicrobial activity of nanoemulsions incorporating citral essential oil. J. Food Drug Anal. 26, 82–89. doi: 10.1016/j.jfda.2016.12.018
Pavela, R., Benelli, G. (2016). Essential oils as ecofriendly biopesticides? challenges and constraints. Trends Plant Sci. 21, 1000–1007. doi: 10.1016/j.tplants.2016.10.005
Roh, H. S., Kim, J., Shin, E. S., Lee, D. W., Choo, H. Y., Park, C. G. (2015). Bioactivity of sandalwood oil (Santalum austrocaledonicum) and its main components against the cotton aphid, Aphis gossypii. J. Pest Sci. 88, 621–627. doi: 10.1007/s10340-014-0631-1
Salamci, E., Kordali, S., Kotan, R., Cakir, A., Kaya, Y. (2007). Chemical compositions, antimicrobial and herbicidal effects of essential oils isolated from Turkish Tanacetum aucheranum and Tanacetum chiliophyllum var. chiliophyllum. Biochem. Systematics Ecol. 35, 569–581. doi: 10.1016/j.bse.2007.03.012
Saleh, I., Abd-Elgawad, A., El Gendy, A. N., Aty, A. A., Mohamed, T., Kassem, H., et al. (2020). Phytotoxic and antimicrobial activities of teucrium polium and thymus decussatus essential oils extracted using hydrodistillation and microwave-assisted techniques. Plants-Basel 9, 716. doi: 10.3390/plants9060716
Salehi, P., Abedini, N., Sonboli, A., Aliahmadi, A., Ayyari, M. (2015). Chemical composition and antimicrobial activity of Ajania semnanensis essential oil in two growing stages. J. Essential Oil Res. 27, 96–100. doi: 10.1080/10412905.2014.987928
Sampietro, D. A., Gomez, A. D., Jimenez, C. M., Lizarraga, E. F., Ibatayev, Z. A., Suleimen, Y. M., et al. (2017). Chemical composition and antifungal activity of essential oils from medicinal plants of Kazakhstan. Natural Product Res. 31, 1464–1467. doi: 10.1080/14786419.2016.1258560
Shao, H., Hu, Y. X., Han, C. X., Wei, C. X., Zhou, S. X., Zhang, C. P., et al. (2018). Chemical composition and phytotoxic activity of Seriphidium terrae-albae (Krasch.) poljakov (Compositae) essential oil. Chem. Biodiver. 15, e2000042. doi: 10.1002/cbdv.201800348
Shao, Y. Z., Ning, A. Q., An, Y., Hou, Z. B., Zhou, F., He, C. Y., et al. (2021). Evaluation of the composition and fumigant toxicity against plodia interpunctella of essential oils from Ajania potaninii and Ajania fruticulosa. J. Asia-Pacific Entomol. 24, 1235–1238. doi: 10.1016/j.aspen.2021.08.012
Shatar, S., Staneva, J., Altantsetseg, S., Todorova, M. (2010). Essential oil composition of three Ajania species from Mongolia. J. Essential Oil Bearing Plants 13, 460–464. doi: 10.1080/0972060X.2010.10643850
Shepherd, C., Giacomin, P., Navarro, S., Miller, C., Loukas, A., Wangchuk, P. (2018). A medicinal plant compound, capnoidine, prevents the onset of inflammation in a mouse model of colitis. J. Ethnopharmacol. 211, 17–28. doi: 10.1016/j.jep.2017.09.024
Singh, N., Singh, H. P., Batish, D. R., Kohli, R. K., Yadav, S. S. (2020). Chemical characterization, phytotoxic, and cytotoxic activities of essential oil of mentha longifolia. Environ. Sci. pollut. Res. 27 (12), 13512–13523. doi: 10.1007/s11356-020-07823-3
Sousa, R. M. O. F., Cunha, A. C., Fernandes-Ferreira, M. (2021). The potential of apiaceae species as sources of singular phytochemicals and plant-based pesticides. Phytochemistry 187, 112714. doi: 10.1016/j.phytochem.2021.112714
Suteu, D., Rusu, L., Zaharia, C., Badeanu, M., Daraban, G. M. (2020). Challenge of utilization vegetal extracts as natural plant protection products. Appl. Sciences-Basel 10, 8913.
Teh, C. H., Nazni, W. A., Norazah, A., Lee, H. L. (2017). Determination of antibacterial activity and minimum inhibitory concentration of larval extract of fly via resazurin-based turbidometric assay. BMC Microbiol. 17, 3. doi: 10.1186/s12866-017-0936-3
Valente, P. M., Valente, V. M. M., Silva, M. C., dos Reis, L. B., Silva, F. D., Praca-Fontes, M. M. (2022). Phytotoxicity and cytogenotoxicity of dionaea muscipula Ellis extracts and its major compound against lactuca sativa and allium cepa. Biologia. 77, 2975–2988 doi: 10.1007/s11756-022-01153-0
Vasconcelos, L. C., Carrijo, T. T., Venancio, A. N., Alves, T. A., Tuler, A. C., Hollunder, R. K., et al. (2022). Phytochemical screening and phytocytotoxic effects of the tropical myrcia vittoriana (Myrtaceae). Anais Da Academia Bras. Cienc. 94, e20210820. doi: 10.1590/0001-3765202220210820
Vokou, D., Douvli, P., Blionis, G. J., Halley, J. M. (2003). Effects of monoterpenoids, acting alone or in pairs, on seed germination and subsequent seedling growth. J. Chem. Ecol. 29, 2281–2301. doi: 10.1023/A:1026274430898
Wangchuk, P., Apte, S. H., Smout, M. J., Groves, P. L., Loukas, A., Doolan, D. L. (2018). Defined small molecules produced by Himalayan medicinal plants display immunomodulatory properties. Int. J. Mol. Sci. 19, 3490. doi: 10.3390/ijms19113490
Wangchuk, P., Keller, P. A., Pyne, S. G., Korth, J., Samten, D., Taweechotipatr, M., et al. (2013). Antimicrobial, antimalarial and cytoxicity activities of constituents of a bhutanese variety of Ajania nubigena. Natural Product Commun. 8, 733–736. doi: 10.1177/1934578X1300800613
Wangchuk, P., Pearson, M. S., Giacomin, P. R., Becker, L., Sotillo, J., Pickering, D., et al. (2016). Compounds derived from the bhutanese daisy, Ajania nubigena, demonstrate dual anthelmintic activity against Schistosoma mansoni and Trichuris muris. PloS Negl. Trop. Dis. 10, e0004908. doi: 10.1371/journal.pntd.0004908
Wei, C. X., Zhou, S. X., Shi, K., Zhang, C., Shao, H., et al. (2020). Chemical profile and phytotoxic action of Onopordum acanthium essential oil. Scientific Reports 10, 13568. doi: 10.1038/s41598-020-70463-7
Wissal, D., Sana, B., Sabrine, J., Nada, B., Wissem, M. J. M. (2016). Essential oils’ chemical characterization and investigation of some biological activities: a critical review. Medicines (Basel Switzerland) 3, 4. doi: 10.3390/medicines3040025
Wu, F. L., Fang, X. M., Herrmann, M., Mosbrugger, V., Miao, Y. F. (2011). Extended drought in the interior of central Asia since the pliocene reconstructed from sporopollen records. Global Planetary Change 76, 16–21. doi: 10.1016/j.gloplacha.2010.11.007
Wu, H. R., Zhang, W., Pang, X. Y., Gong, Y., Obulqasim, X. M. U., Li, H. F., et al. (2015). Quinones and coumarins from Ajania salicifolia and their radical scavenging and cytotoxic activity. J. Asian Natural Products Res. 17, 1196–1203. doi: 10.1080/10286020.2015.1117456
Yang, Y., Isman, M. B., Tak, J. H. J. I. (2020). Insecticidal activity of 28 essential oils and a commercial product containing Cinnamomum cassia bark essential oil against Sitophilus zeamais motschulsky. Insects 11, 474. doi: 10.3390/insects11080474
Zheng, S. Z., Kang, S. H., Shen, Y. W., Sun, L. P. (1999). Three new c-methylated flavones from elsholtzia stauntonii. Planta Med. 65, 173–175. doi: 10.1055/s-2006-960459
Keywords: Ajania tibetica, essential oil, phytotoxicity, insecticidal activity, antimicrobial activity
Citation: Han C, Zhou S, Mei Y, Cao Q, Shi K and Shao H (2022) Phytotoxic, insecticidal, and antimicrobial activities of Ajania tibetica essential oil. Front. Plant Sci. 13:1028252. doi: 10.3389/fpls.2022.1028252
Received: 25 August 2022; Accepted: 04 November 2022;
Published: 18 November 2022.
Edited by:
Paolo Giordani, University of Genoa, ItalyReviewed by:
Dušica Ćalić, University of Belgrade, SerbiaHarminder Pal Singh, Panjab University, India
Copyright © 2022 Han, Zhou, Mei, Cao, Shi and Shao. This is an open-access article distributed under the terms of the Creative Commons Attribution License (CC BY). The use, distribution or reproduction in other forums is permitted, provided the original author(s) and the copyright owner(s) are credited and that the original publication in this journal is cited, in accordance with accepted academic practice. No use, distribution or reproduction is permitted which does not comply with these terms.
*Correspondence: Hua Shao, c2hhb2h1YUBtcy54amIuYWMuY24=