Introduction
Plant specialized metabolites, such as alkaloids, flavonoids, terpenoids, and lignans, have health-promoting benefits for the world’s increasingly aging population. Lignans are phenylpropanoid dimers of various chemical structures with diverse health benefits (Suzuki and Umezawa, 2007; Rodríguez-García et al., 2019; Hano et al., 2021). Dietary lignans are obtained from oil seeds, cereal grains, legumes, vegetables, fruits, and beverage, and have attracted attention as food nutrients with potential health promoting activities (Durazzo et al., 2018; Rodríguez-García et al., 2019). Lignan-rich plants have been used in Chinese medicines (Teodor et al., 2020; Xu et al., 2022). (+)-Sesamin is a furofuran lignan, highly accumulated in Sesamum indicum (sesame) seeds, and commercially available as a health-promoting supplement. In mammals, (+)-sesamin metabolites attenuate oxidation and inflammation, thereby protecting the liver (Nakai et al., 2003; Kabe et al., 2020). (+)-Sesamin also exhibits several anti-tumor and anti-bacterial effects (Majdalawieh et al., 2017; Oikawa et al., 2022). (-)-Podophyllotoxin is an aryltetralin lignan found in the rhizomes of Podophyllum plants, and is utilized as a leading compound in anti-cancer drugs (Ardalani et al., 2017; Changxing et al., 2020).
Though (+)-sesamin and (-)-podophyllotoxin are important to human health, their plant sources are limited. Specific plant species accumulate large quantities of beneficial lignans, but model plants, such as Arabidopsis thaliana, produce them in small amounts (Umezawa, 2003a; Nakatsubo et al., 2008; Okazawa et al., 2011). Natural growth and agricultural cultivation of lignan-producing plants is frequently threatened owing to climate change. S. indicum plants, the strongest known synthesizers of (+)-sesamin, are constrained by environmental stress (Islam et al., 2016; Dossa et al., 2017; Dossa et al., 2019). Podophyllum species, natural sources of (-)-podophyllotoxin, grow naturally in very limited regions; hence they are an endangered species (Chaurasia and Tayade, 2012; Chaudhari et al., 2014; Singh et al., 2021). The regeneration of Podophyllum species is considerably slower than their harvest rate (Singh et al., 2021).
Cultured cells and hairy root lines from natural plant sources have been established in vitro, and developed to produce beneficial lignans (Fuss, 2003; Bayindir et al., 2008; Ragamustari et al., 2014; Lalaleo et al., 2018; Renouard et al., 2018; Bazaldúa et al., 2019; Mikac et al., 2021). Growth conditions to induce the accumulation of beneficial lignans have also been optimized practically (Satake et al., 2015; Renouard et al., 2018; Changxing et al., 2020). However, cost-effective scalable production of beneficial lignans using these culture systems is yet to be developed (Mikac et al., 2021).
Therefore, new plant sources are required to address these issues. The novelty of this article opinion is to highlight the prospects for the development of heterologous production of beneficial lignans such as (+)-sesamin and (-)-podophyllotoxin-related lignans, as transgenic cells and plants are excellent hosts for heterologous production of other beneficial chemicals (Lu et al., 2016; Arya et al., 2020; Wu et al., 2021). This is underpinned by the identification of the lignan-biosynthetic enzymes, and generation of transgenic plants expressing the lignan-metabolic enzyme genes as heterologous hosts.
The heterologous hosts
Heterologous production of (+)-sesamin and related lignans in Forsythia plants
The knowledge of lignan biosynthetic enzymes, from different plants, is necessary for the selection of hosts for heterologous lignan production. The biosynthetic pathways for the sesamin-related lignans have a common beginning in the conversion of phenylalanine to coniferyl alcohol (CA), followed by the dimerization of CA, by dirigent proteins (DIR), to form the lignan precursor (+)-pinoresinol (Figure 1A) (Davin et al., 1997; Davin and Lewis, 2000; Umezawa, 2003b).
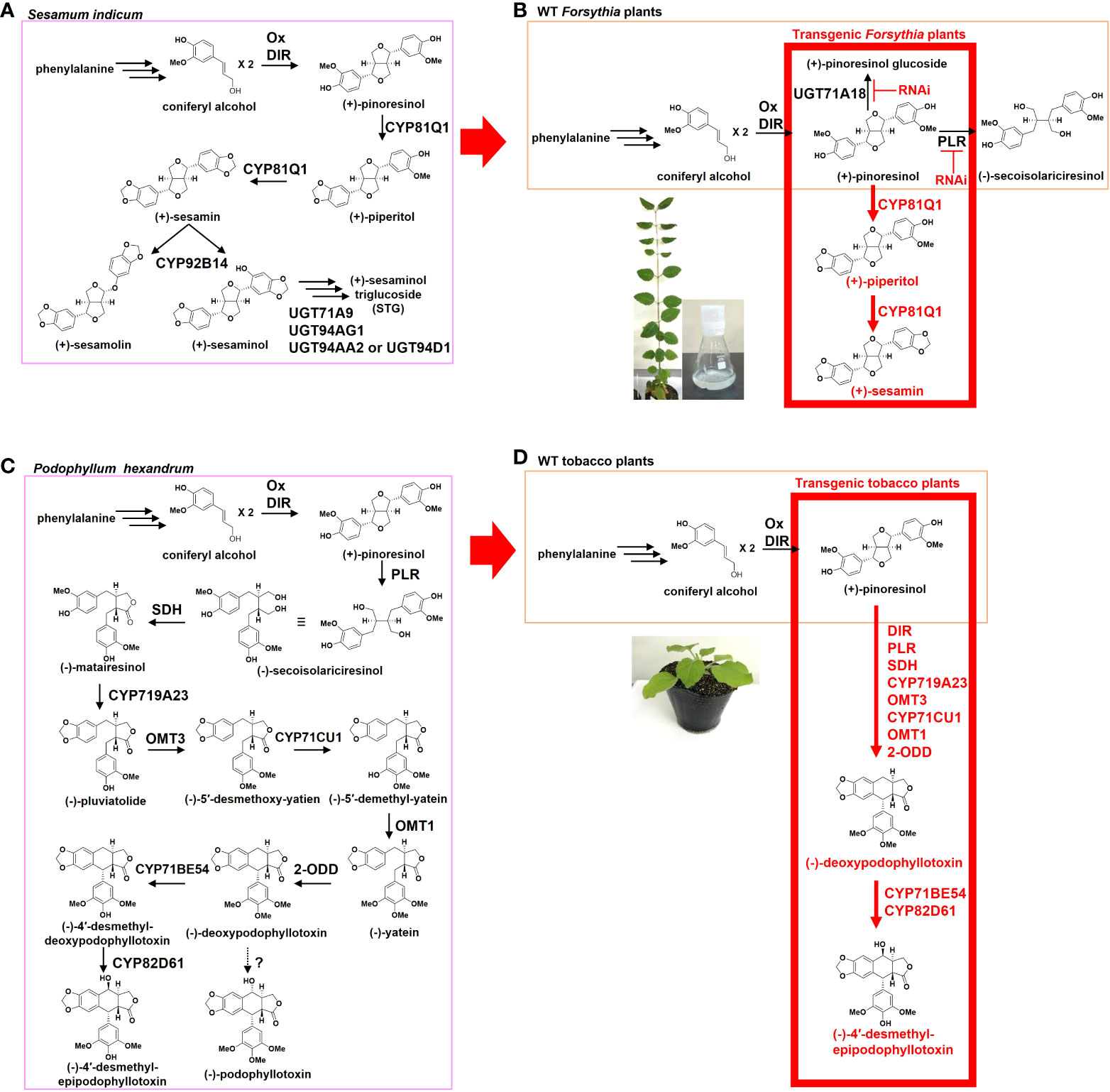
Figure 1 The heterologous production of beneficial lignans in transgenic plants. (A) Sesamum indicum lignan-metabolic pathway (pink). (B) Heterologous production of sesame lignans in Forsythia plants. Wild-type (WT) Forsythia plants biosynthesize (+)-pinoresinol (orange). Ectopic expression of CYP81Q1 gene produces sesame lignans, (+)-piperitol, and (+)-sesamin in transgenic Forsythia plants (red). RNAi of PLR and UGT71A18 increase the accumulation of (+)-sesamin in transgenic Forsythia cells. (C) Podopophyllum hexandrum lignan-metabolic pathway (pink) (D) Heterologous production of Podopophyllum lignans in tobacco plants. WT tobacco plants biosynthesize (+)-pinoresinol (orange). Transient co-expression of DIR, PLR, SDH, CYP719A23, OMT3, CYP71CU1, OMT1, and 2-ODD genes produces (-)-deoxypodophyllotoxin in the leaves of tobacco (red). Co-expression of additional cytochrome P450 enzymes (CYP71BE54 and CYP82D61) produces (-)-4′-desmethyl-epipodophyllotoxin (etoposide precursor). DIR, Dirigent proteins; PLR, Pinoresinol-lariciresinol reductase; SDH, Secoisolariciresinol dehydrogenase; OM,: O-methyltransferase; 2-ODD, 2-oxoglutarate/Fe(II)-dependent dioxygenase.
S. indicum seeds accumulate (+)-sesamin, (+)-sesamolin, and (+)-sesaminol triglucoside (Beroza and Kinman, 1955; Bedigian, 2010). (+)-Pinoresinol is oxygenated and converted to (+)-sesamin by the piperitol/sesamin synthase enzyme, also known as CYP81Q1 (Ono et al., 2006) (Figure 1A). (+)-Sesamin is further oxygenated to either (+)-sesamolin or (+)-sesaminol by CYP92B14, depending on the position of the oxygenated carbon in the aromatic ring system of (+)-sesamin (Murata et al., 2017). (+)-Sesaminol is further glucosylated by uridine diphosphate (UDP)-glucosyltransferases and is accumulated as (+)-sesaminol triglucoside (Noguchi et al., 2008; Ono et al., 2020).
Heterologous production of sesame lignans has been achieved in Forsythia-based transgenic systems. Forsythia plants have characteristics suitable for the heterologous production of lignans. They are perennial shrubs, propagated vegetatively, and distributed widely (Rosati et al., 2007). They accumulate high levels of the lignan precursor (+)-pinoresinol, and other beneficial lignans (Umezawa, 2003b; Guo et al., 2007; Suzuki and Umezawa, 2007; Chang et al., 2008; Morimoto and Satake, 2013; Wang et al., 2018). Extracts of Forsythia plants have been used empirically in traditional medicines (Dong et al., 2017; Wang et al., 2018). The regulation of lignan biosynthesis has been extensively analyzed (Shiraishi et al., 2016; Sun et al., 2018).
Forsythia plants synthesize (+)-pinoresinol but not (+)-sesamin. Therefore, (+)-sesamin was expressed heterologously in transgenic Forsythia cells and plants (Figure 1B). Kim et al. (Kim et al., 2009) demonstrated the production of (+)-sesamin in double-transgenic Forsythia koreana (Fk) cells, CPi-Fk, which had been stably transformed with CYP81Q1 and pinoresinol lariciresinol reductase-RNA interference (PLR-RNAi) (Figure 1B). Since CYP81Q1 converts only (+)-pinoresinol aglycone into (+)-sesamin, and 90% of the (+)-pinoresinol is glucosylated in Forsythia wild type cells or leaves (Ono et al., 2006; Morimoto and Satake, 2013, Satake et al., 2015; Satake et al., 2016), the triple-transgenic Fk cells, U18i-CPi-Fk, were generated by stable transformation of CPi-Fk with an RNAi sequence against the (+)-pinoresinol-glucosylating enzyme, UGT71A18 (Ono et al., 2010). These U18i-CPi-Fk cells, in comparison with the CPi-Fk cells, showed approximately 5- and 1.4-fold increase in the synthesis of (+)-pinoresinol aglycone and (+)-sesamin, respectively (Murata et al., 2015). (+)-Sesamin production in U18i-CPi-Fk was upregulated approximately 3-fold, specifically under red light, for two weeks (Murata et al., 2015; Satake et al., 2016). These findings confirmed the potential of the Fk-transgenic cells for metabolic engineering-based lignan production, and paved the way for generating (+)-sesamin-producing Forsythia plants.
An illustration of the heterologous production of lignans in Forsythia plants: the generation of the transgenic Forsythia plants expressing sesame CYP81Q1 gene results in the production of (+)-sesamin and its intermediate (+)-piperitol (Figure 1B) (Koyama et al., 2022). These transgenic Forsythia plants produced (+)-sesamin and (+)-piperitol sustainably, even after repeated vegetative propagation via explants (Koyama et al., 2022). Therefore, the transgenic Forsythia plants serve as prototype heterologous host plants, with the potential for mass propagation as alternative sources of (+)-sesamin and (+)-piperitol.
Heterologous production of (-)-podophyllotoxin-related lignans in tobacco plants
In (-)-podophyllotoxin biosynthesis in Podophyllum plants, (-)-matairesinol, a downstream lignan of (+)-pinoresinol, is initially converted to (-)-pluviatolide by CYP719A23 (Figure 1C) (Marques et al., 2013). Methylation of (-)-pluviatolide is catalyzed by (-)-pluviatolide-O-methyltransferase (OMT3), which yields (-)-5′-desmethoxy-yatien. (-)-5′-Desmethoxy-yatien is hydroxylated to (-)-5′-demethyl-yatein by CYP71CU1 (Law and Sattely, 2015), which is further methylated by (-)-5′-demethyl-yatein-O-methyltransferase (OMT1) and converted to (-)-yatein. Thereafter, (-)-yatein is catalyzed by (-)-2-oxoglutarate/Fe(II)-dependent dioxgenase (2-ODD) to (-)-deoxypodophyllotoxin, the proposed precursor of (-)-podophyllotoxin. To date, the final step from (-)-deoxypodophyllotoxin to (-)-podophyllotoxin has not been identified (Figure 1C) (Law and Sattely, 2015). Instead, (-)-deoxypodophyllotoxin has been shown to be converted into (-)-4′-desmethyl-deoxypodophyllotoxin via demethylation by CYP71BE54, and is further metabolized by CYP82D61 to (-)-4′-desmethyl-epipodophyllotoxin, the direct precursor of etoposide (Law and Sattely, 2015).
(-)-Podophyllotoxin-related lignans were produced heterologously in Nicotiana benthamiana (Figure 1D). The cultivation of N. benthamiana has been established well by many research institutions and biotechnology companies, worldwide (Bally et al., 2018). Leaves of N. benthamiana are excellent hosts for the Agrobacterium-mediated transient gene expression assay, which has been developed well for high production of proteins and other compounds (Bally et al., 2018; Molina-Hidalgo et al., 2021). Thus, N. benthamiana is a promising heterologous host for the production of beneficial lignans.
The transient co-expression of Podophyllum (-)-podophyllotoxin-biosynthetic enzyme genes for CYP719A23, OMT3, CYP71CU1, OMT1, and 2-ODD, as well as previously known lignan-biosynthetic enzyme genes, metabolize exogenously applied (+)-pinoresinol to (-)-deoxypodophyllotoxin in the leaves of N. benthamiana (Figure 1D) (Law and Sattely, 2015). Furthermore, the transient co-expression of two additional cytochrome P450 enzymes, CYP71BE54 and CYP82D61, with the above-mentioned (-)-podophyllotoxin-biosynthetic enzymes, produced (-)-4′-desmethylepipodophyllotoxin (Figure 1D) (Law and Sattely, 2015). This study demonstrates the heterologous production of (-)-podophyllotoxin-related lignans in N. benthamiana.
The lignan precursor (+)-pinoresinol is biosynthesized via CA, and CA is produced from phenylalanine (CA pathway, Figure 1D), so to increase the supply of CA in the leaves of N. benthamiana transient co-expression of CA pathway enzymes was carried out (Schultz et al., 2019). Co-expression of 16 genes of the CA pathway, along with the (-)-podophyllotoxin-biosynthetic enzymes, greatly increased the content of (-)-deoxypodophyllotoxin in the leaves of N. benthamiana (Schultz et al., 2019). This co-expression system did not require exogenous application of (+)-pinoresinol, and thus, enabled de novo production of (-)-podophyllotoxin-related lignans in N. benthamiana leaves (Schultz et al., 2019). This study demonstrates the applicability of engineering to supply endogenous precursors to increase the yield of (-)-podophyllotoxin-related lignans in the leaves of N. benthamiana.
Challenges for the heterologous production of beneficial lignans in microbes
Microbes are also important hosts for the heterologous production of plant specialized metabolites (Sato and Kumagai, 2013; Kotopka et al., 2018; Pyne et al., 2019; Birchfield and McIntosh, 2020). Previous studies have suggested the potential capability of bacteria to produce lignans. Two sequential Escherichia coli lines, harboring individual metabolic modules of (-)-matairesinol to (-)-5′desmetyl-yatein, and (−)-5′desmetyl-yatein to (−)-deoxypodophyllotoxin, reportedly metabolized exogenously applied (-)-matairesinol to (-)-deoxypodophyllotoxin (Decembrino et al., 2021). The human gut microbiome also metabolizes exogenously applied (+)-pinoresinol to various lignans (Xie et al., 2003; Espín et al., 2017; Bess et al., 2020). However, these bacteria require the exogenous application of lignan precursors to produce the beneficial lignans. The lignan precursor (+)-pinoresinol is, apparently, not produced by many bacteria. Therefore, it would be impossible to produce beneficial lignans without exogenous application of precursor lignans. Therein, de novo production of lignans would require synthetic biotechnology approaches that include tremendous bioinformatics and large-scale screening processes (Choi et al., 2019; Birchfield and McIntosh, 2020). In addition, ethical issues may arise in using the gut bacterium for the scalable production of lignans (Choi et al., 2019). Thus, the microbial production of beneficial lignans is more challenging than the plant-based production process.
Discussion
The novelty of this article is to underscore the importance of lignan production in heterologous host plants. Transgenic expression of the lignan-biosynthetic enzyme genes in heterologous hosts, Forsythia and tobacco, is particularly promising for the production of (+)-sesamin and (-)-podophyllotoxin-related lignans, respectively. These heterologous lignan production strategies may circumvent the need for sesame and Podophyllum plants as natural sources of (+)-sesamin and (-)-podophyllotoxin-related lignans in future.
To develop more efficient transgenic plant-based lignan production systems, we emphasize the importance of increasing the lignan content in future studies. Introducing additional genes to activate the enzymes or stimulate the accumulation of the precursor (+)-pinoresinol in transgenic plants will pave the way for increasing the content of beneficial lignans. To activate cytochrome P450 enzymes that function in complex with native reductases (Murata et al., 2017), co-expression of cytochrome P450 enzymes with their native reductases may lead to an increase in the content of (+)-sesamin and (-)-podophyllotoxin-related lignans in transgenic Forsythia and tobacco plants, respectively. Introducing RNAi into the lignan-metabolic pathway stimulates the accumulation of (+)-pinoresinol in transgenic Forsythia cell lines (Figure 1B) (Kim et al., 2009b; Murata et al., 2015), therefore, co-expression of (-)-podophyllotoxin-metabolic enzymes with an RNAi construct to stimulate the accumulation of (+)-pinoresinol may increase the content of (-)-podophyllotoxin-related lignans in N. benthamiana. Similarly, activation of the CA pathway stimulates the accumulation of (+)-pinoresinol in N. benthamiana (Figure 1D) (Schultz et al., 2019); thus, co-expression of CYP81Q1 with the CA pathway enzymes may increase the content of (+)-sesamin in transgenic Forsythia plants. Furthermore, since the elicitor treatments and light changes induce the production of beneficial lignans in cultured cells and hairy root lines (Satake et al., 2015; Changxing et al., 2020; Markulin et al., 2021; Mikac et al., 2021), it is important to optimize the growth conditions also of the heterologous host plants in future studies. Finally, other lignan-rich plants, such as flax species (Markulin et al., 2021), are expected to be useful heterologous hosts for the transgenic expression of lignan biosynthetic enzyme genes.
Author contributions
TK, JM, and HS wrote the manuscript, and TK and MH prepared the figure. All the authors approved the submitted version.
Conflict of interest
The authors declare that the research was conducted in the absence of any commercial or financial relationships that could be construed as a potential conflict of interest.
Publisher’s note
All claims expressed in this article are solely those of the authors and do not necessarily represent those of their affiliated organizations, or those of the publisher, the editors and the reviewers. Any product that may be evaluated in this article, or claim that may be made by its manufacturer, is not guaranteed or endorsed by the publisher.
References
Ardalani, H., Avan, A., Ghayour-Mobarhan, M. (2017). Podophyllotoxin: a novel potential natural anticancer agent avicenna. J. Phytomed 7, 285–294.
Arya, S. S., Rookes, J. E., Cahill, D. M., Lenka, S. K. (2020). Next-generation metabolic engineering approaches towards development of plant cell suspension cultures as specialized metabolite producing biofactories. Biotechnol. Adv. 45, 107635. doi: 10.1016/j.biotechadv.2020.107635
Bally, J., Jung, H., Mortimer, C., Naim, F., Philips, J. G., Hellens, R., et al. (2018). The rise and rise of nicotiana benthamiana: A plant for all reasons. Ann. Rev. Phytopathol. 56, 405–426. doi: 10.1146/annurev-phyto-080417
Bayindir, Ü., Alfermann, A. W., Fuss, E. (2008). Hinokinin biosynthesis in linum corymbulosum reichenb. Plant J. 55, 810–820. doi: 10.1111/j.1365-313X.2008.03558.x
Bazaldúa, C., Cardoso-Taketa, A., Trejo-Tapia, G., Camacho-Diaz, B., Arellano, J., Ventura-Zapata, E., et al. (2019). Improving the production of podophyllotoxin in hairy roots of hyptis suaveolens induced from regenerated plantlets. PloS One 14 (9), e0222464. doi: 10.1371/journal.pone.0222464
Bedigian, D. (2010). “Introduction: history of the cultivation and use of sesame,” in Sesame: The genus sesamum. Ed. Bedigian, D. (Boca Raton: CRC Press), 1–31.
Beroza, M., Kinman, M. L. (1955). Sesamin, sesamolin, and sesamol content of the oil of sesame seed as affected by strain, location grown, ageing, and frost damage. J. Am. Oil Chem. Soc. 32, 348–350. doi: 10.1007/BF02640380
Bess, E. N., Bisanz, J. E., Yarza, F., Bustion, A., Rich, B. E., Li, X., et al. (2020). Genetic basis for the cooperative bioactivation of plant lignans by eggerthella lenta and other human gut bacteria. Nat. Microbiol. 5, 56–66. doi: 10.1038/s41564-019-0596-1
Birchfield, A. S., McIntosh, C. A. (2020). Metabolic engineering and synthetic biology of plant natural products – a mini review. Curr. Plant Biol. 24, 100163. doi: 10.1016/j.cpb.2020.100163
Chang, M. J., Hung, T. M., Min, B. S., Kim, J. C., Woo, M. H., Choi, J. S., et al. (2008). Lignans from the fruits of forsythia suspensa (Thunb.) vahl protect high-density lipoprotein during oxidative stress. Biosci. Biotechnol. Biochem. 72, 2750–2755. doi: 10.1271/bbb.80392
Changxing, L., Galani, S., Hassan, F., Rashid, ,. Z., Naveed, M., Fang, D., et al. (2020). Biotechnological approaches to the production of plant-derived promising anticancer agents: An update and overview. Biomed. Pharmacother. 132, 110918. doi: 10.1016/j.biopha.2020.110918
Chaudhari, S. K., Bibi, Y., Arshad, M. (2014). Podophyllum hexandrum: An endangered medicinal plant from Pakistan. Pure Appl. Bio 3, 19–24. doi: 10.19045/bspab.2014.31003
Chaurasia, O. P., Tayade, A. (2012). Podophyllum l.: An endangered and anticancerous medicinal plant. an overview. Indian J. Traditional Knowledge 11, 234–241.
Choi, K. R., Jang, W. D., Yang, D., Cho, J. S., Park, D., Lee, S. Y. (2019). Systems metabolic engineering strategies: integrating systems and synthetic biology with metabolic engineering. Trends Biotechnol. 37, 817–837. doi: 10.1016/j.tibtech.2019.01.003
Davin, L. B., Lewis, N. G. (2000). Update on lignins and lignans. dirigent proteins and dirigent sites explain the mystery of specificity of radical precursor coupling in lignan and lignin biosynthesis. Plant Physiol. 123, 453–446. doi: 10.1104/pp.123.2.453
Davin, L. B., Wang, H. B., Crowell, A. L., Bedgar, D. L., Martin, D. M., Sarkanen, S., et al. (1997). Stereoselective bimolecular phenoxy radical coupling by an auxiliary (dirigent) protein without an active center. Science 275, 362–366. doi: 10.1126/science.275.5298.362
Decembrino, D., Raffaele, A., Knöfel, R., Girhard, M., Urlacher, V. B. (2021). Synthesis of (−)−deoxypodophyllotoxin and (−)−epipodophyllotoxin via a multi-enzyme cascade in e. coli. Microb. Cell Fact 20, 183. doi: 10.1186/s12934-021-01673-5
Dong, Z., Lu, X., Tong, X., Dong, Y., Tang, L., Liu, M. (2017). Forsythiae fructus: A review on its phytochemistry, quality control, pharmacology and pharmacokinetics. Molecules 22, 1466. doi: 10.3390/molecules22091466
Dossa, K., Diouf, D., Wang, L., Wei, X., Zhang, Y., Niang, M., et al. (2017). The emerging oilseed crop sesamum indicum enters the “Omics” era. Front. Plant Sci. 8. doi: 10.3389/fpls.2017.01154
Dossa, K., Li, D., Zhou, R., Yu, J., Wang, L., Zhang, Y., et al. (2019). The genetic basis of drought tolerance in the high oil crop sesamum indicum. Plant Biotechnol. J. 17, 1788–1803. doi: 10.1111/pbi.13100
Durazzo, A., Lucarini, M., Camilli, E., Marconi, S., Gabrielli, P., Lisciani, S., et al. (2018). Dietary lignans: Definition, description and research trends in databases development. Molecules 23, 3251. doi: 10.3390/molecules23123251
Espín, J. C., González-Sarrías, A., Tomás-Barberán, F. A. (2017). The gut microbiota: A key factor in the therapeutic effects of (poly)phenols. Biochem. Pharmacol. 139, 82–93. doi: 10.1016/j.bcp.2017.04.033
Fuss, E. (2003). Lignans in plant cell and organ cultures: An overview. Phytochem. Rev. 2, 307–320. doi: 10.1023/B:PHYT.0000045500.56476.f5
Guo, H., Liu, A. H., Ye, M., Yang, M., Guo, D. A. (2007). Characterization of phenolic compounds in the fruits of forsythia suspensa by high-performance liquid chromatography coupled with electrospray ionization tandem mass spectrometry. Rapid Commun. Mass Spectrom 21, 715–729. doi: 10.1002/rcm.2875
Hano, C. F., Dinkova-Kostova, A. T., Davin, L. B., Cort, J. R., Lewis, N. G. (2021). Lignans: Insights into their biosynthesis, metabolic engineering, analytical methods and health benefits. Front. Plant Sci. 11. doi: 10.3389/fpls.2020.630327
Islam, F., Gill, R. A., Ali, B., Farooq, M. A., Xu, L., Najeeb, U., et al. (2016). “Sesame,” in Breeding oilseed crops for sustainable production: Opportunities and constraints (Amsterdam, Netherlands: Elsevier Inc), 135–147.
Kabe, Y., Takemoto, D., Kanai, A., Hirai, M., Ono, Y., Akazawa, S., et al. (2020). Annexin A1 accounts for an anti-inflammatory binding target of sesamin metabolites. NPJ Sci. Food 4. doi: 10.1038/s41538-020-0064-6
Kim, H. J., Ono, E., Morimoto, K., Yamagaki, T., Okazawa, A., Kobayashi, A., et al. (2009). Metabolic engineering of lignan biosynthesis in forsythia cell culture. Plant Cell Physiol. 50, 2200–2209. doi: 10.1093/pcp/pcp156
Kotopka, B. J., Li, Y., Smolke, C. D. (2018). Synthetic biology strategies toward heterologous phytochemical production. Nat. Prod. Rep. 35, 902–920. doi: 10.1039/c8np00028j
Koyama, T., Matsumoto, E., Okuda, T., Murata, J., Horikawa, M., Hata, N., et al. (2022). Transgenic forsythia plants expressing sesame cytochrome P450 produce beneficial lignans. Sci. Rep. 12, 10152. doi: 10.1038/s41598-022-14401-9
Lalaleo, L., Testillano, P., Risueño, M. C., Cusidó, R. M., Palazon, J., Alcazar, R., et al. (2018). Effect of in vitro morphogenesis on the production of podophyllotoxin derivatives in callus cultures of linum album. J. Plant Physiol. 228, 47–58. doi: 10.1016/j.jplph.2018.05.007
Law, W., Sattely, E. S. (2015). Six enzymes from mayapple that complete the biosynthetic pathway to the etoposide aglycone. Science 349, 1224–1227. doi: 10.1126/science.aac7202
Lu, X., Tang, K., Li, P. (2016). Plant metabolic engineering strategies for the production of pharmaceutical terpenoids. Front. Plant Sci. 7. doi: 10.3389/fpls.2016.01647
Majdalawieh, A. F., Massri, M., Nasrallah, G. K. (2017). A comprehensive review on the anti-cancer properties and mechanisms of action of sesamin, a lignan in sesame seeds (Sesamum indicum). Eur. J. Pharmacol. 815, 512–521. doi: 10.1016/j.ejphar.2017.10.020
Markulin, L., Drouet, S., Garros, L., Aujum, ,. S., Tungmunnithum, D., Abbasi, B. H., et al. (2021). “Production of antidiabetic lignans in flax cell cultures,” in Biotechnology of anti-diabetic medicinal plants. Ed. Gantait, S., 383–408. doi: 10.1007/978-981-16-3529-8_13
Marques, J. V., Kim, K. W., Lee, C., Costa, M. A., May, G. D., Crow, J. A., et al. (2013). Next generation sequencing in predicting gene function in podophyllotoxin biosynthesis. J. Biol. Chem. 288, 466–479. doi: 10.1074/jbc.M112.400689
Mikac, S., Markulin, L., Drouet, S., Corbin, C., Tungmunnithum, D., Kiani, R., et al. (2021). Bioproduction of anticancer podophyllotoxin and related aryltretralin-lignans in hairy root cultures of linum flavum l. Reference Ser. phytochemistry Plant Cell Tissue differentiation secondary metabolites, 503–540. doi: 10.1007/978-3-030-30185-9_20
Molina-Hidalgo, F. J., Vazquez-Vilar, M., D’Andrea, L., Demurtas, O. C., Fraser, P., Giuliano, G., et al. (2021). Engineering metabolism in nicotiana species: A promising future. Trends Biotechnol. 39, 901–913. doi: 10.1016/j.tibtech.2020.11.012
Morimoto, K., Satake, H. (2013). Seasonal alteration in amounts of lignans and their glucosides and gene expression of the relevant biosynthetic enzymes in the forsythia suspensa leaf. Biol. Pharm. Bull. 36, 1519–1523. doi: 10.1248/bpb.b13-00437
Murata, J., Matsumoto, E., Morimoto, K., Koyama, T., Satake, H. (2015). Generation of triple-transgenic forsythia cell cultures as a platform for the efficient, stable, and sustainable production of lignans. PloS One 10 (12), e0144519. doi: 10.1371/journal.pone.0144519
Murata, J., Ono, E., Yoroizuka, S., Toyonaga, H., Shiraishi, A., Mori, S., et al. (2017). Oxidative rearrangement of (+)-sesamin by CYP92B14 co-generates twin dietary lignans in sesame. Nat.Commun 8, 2155. doi: 10.1038/s41467-017-02053-7
Nakai, M., Harada, M., Nakahara, K., Akimoto, K., Shibata, H., Miki, W., et al. (2003). Novel antioxidative metabolites in rat liver with ingested sesamin. J. Agric. Food Chem. 51, 1666–1670. doi: 10.1021/jf0258961
Nakatsubo, T., Mizutani, M., Suzuki, S., Hattori, T., Umezawa, T. (2008). Characterization of arabidopsis thaliana pinoresinol reductase, a new type of enzyme involved in lignan biosynthesis. J. Biol. Chem. 283, 15550–15557. doi: 10.1074/jbc.M801131200
Noguchi, A., Fukui, Y., Iuchi-Okada, A., Kakutani, S., Satake, H., Iwashita, T., et al. (2008). Sequential glucosylation of a furofuran lignan, (+)-sesaminol, by sesamum indicum UGT71A9 and UGT94D1 glucosyltransferases. Plant J. 54, 415–427. doi: 10.1111/j.1365-313X.2008.03428.x
Oikawa, D., Yamashita, S., Takahashi, S., Waki, T., Kikuchi, K., Abe, T., et al. (2022). (+)-sesamin, a sesame lignan, is a potent inhibitor of gut bacterial tryptophan indole-lyase that is a key enzyme in chronic kidney disease pathogenesis. Biochem. Biophys. Res.Commun 590, 158–162. doi: 10.1016/j.bbrc.2021.12.088
Okazawa, A., Hori, K., Okumura, Y., Izumi, Y., Hata, N., Bamba, T., et al. (2011). Simultaneous quantification of lignans in arabidopsis thaliana by highly sensitive capillary liquid chromatography-electrospray ionization-ion trap mass spectrometry. Plant Biotechnol. 28, 287–293. doi: 10.5511/plantbiotechnology.11.0221a
Ono, E., Kim, H. J., Murata, J., Morimoto, K., Okazawa, A., Kobayashi, A., et al. (2010). Molecular and functional characterization of novel furofuran-class lignan glucosyltransferases from forsythia. Plant Biotechnol. 27, 317–324. doi: 10.5511/plantbiotechnology.27.317
Ono, E., Nakai, M., Fukui, Y., Tomimori, N., Fukuchi-Mizutani, M., Saito, M., et al. (2006). Formation of two methylenedioxy bridges by a sesamum CYP81Q protein yielding a furofuran lignan, (+)-sesamin. Proc. Natl. Acad. Sci. U.S.A. 103, 10116–10121. doi: 10.1073/pnas.0603865103
Ono, E., Waki, T., Oikawa, D., Murata, J., Shiraishi, A., Toyonaga, H., et al. (2020). Glycoside-specific glycosyltransferases catalyze regio-selective sequential glucosylations for a sesame lignan, sesaminol triglucoside. Plant J. 101, 1221–1233. doi: 10.1111/tpj.14586
Pyne, M. E., Narcross, L., Martin, V. J. J. (2019). Engineering plant secondary metabolism in microbial systems. Plant Physiol. 179, 844–861. doi: 10.1104/pp.18.01291
Ragamustari, S. K., Yamamura, M., Ono, E., Hattori, T., Suzuki, S., Suzuki, H., et al. (2014). Substrate-enantiomer selectivity of matairesinol O-methyltransferases. Plant Biotechnol. 31, 257–267. doi: 10.5511/plantbiotechnology.14.0722a
Renouard, S., Corbin, C., Drouet, S., Medvedec, B., Doussot, J., Colas, C., et al. (2018). Investigation of linum flavum (L.) hairy root cultures for the production of anticancer aryltetralin lignans. Int. J. Mol. Sci. 19, 990. doi: 10.3390/ijms19040990
Rodríguez-García, C., Sánchez-Quesada, C., Toledo, E., Delgado-Rodríguez, M., Gaforio, J. J. (2019). Naturally lignan-rich foods: A dietary tool for health promotion? Molecules 24, 917. doi: 10.3390/molecules24050917
Rosati, C., Cadic, A., Duron, M., Simoneau, P. (2007). “Forsythia,” in Biotechnology in agriculture and forestry, transgenic crops VI. Eds. Pua, E. C., Davey, M. R., 299–318.
Satake, H., Koyama, T., Bahabadi, S. E., Matsumoto, E., Ono, E., Murata, J. (2015). Essences in metabolic engineering of lignan biosynthesis. Metabolites 5, 270–290. doi: 10.3390/metabo5020270
Satake, H., Koyama, T., Matsumoto, E., Morimoto, K., Ono, E., Murata, J. (2016). “Metabolic engineering of lignan biosynthesis pathways for the production of transgenic plant-based foods,” in Transgenesis and secondary metabolism (Cham, Switzerland: Springer International Publishing), 1–26.
Sato, F., Kumagai, H. (2013). Microbial production of isoquinoline alkaloids as plant secondary metabolites based on metabolic engineering research. Proc. Jpn. Acad. B. 89, 165–182. doi: 10.2183/pjab.89.165
Schultz, B. J., Kim, S. Y., Lau, W., Sattely, E. S. (2019). Total biosynthesis for milligram-scale production of etoposide intermediates in a plant chassis. J. Am. Chem. Soc 141, 19231–19235. doi: 10.1021/jacs.9b10717
Shiraishi, A., Murata, J., Matsumoto, E., Matsubara, S., Ono, E., Satake, H. (2016). De novo transcriptomes of forsythia koreana using a novel assembly method: Insight into tissue- and species-specific expression of lignan biosynthesis-related gene. PloS One 11, e0164805. doi: 10.1371/journal.pone.0164805
Singh, J., Singh, J., Lata, S. (2021). “Podophyllum hexandrum,” in Himalayan Medicinal plants: Advances in botany, production & research (Amsterdam, Netherlands: Elsevier), 85–110.
Sun, L., Rai, A., Rai, M., Nakamura, M., Kawano, N., Yoshimatsu, K., et al. (2018). Comparative transcriptome analyses of three medicinal forsythia species and prediction of candidate genes involved in secondary metabolisms. J. Nat. Med. 72, 867–881. doi: 10.1007/s11418-018-1218-6
Suzuki, S., Umezawa, T. (2007). Biosynthesis of lignans and norlignans. J. Wood Sci. 53, 273–284. doi: 10.1007/s10086-007-0892-x
Teodor, E. D., Moroeanu, V., Radu, G. L. (2020). Lignans from medicinal plants and their anticancer effect. Mini-Rev. Med. Chem. 20, 1083–1090. doi: 10.2174/1389557520666200212110513
Umezawa, T. (2003a). “Phylogenetic distribution of lignan producing plants,” in Wood research: bulletin of the wood research institute Kyoto university, vol. 90. , 27–110.
Umezawa, T. (2003b). Diversity in lignan biosynthesis. Phytochem. Rev. 2, 371–390. doi: 10.1023/B:PHYT.0000045487.02836.32
Wang, Z., Xia, Q., Liu, X., Liu, W., Huang, W., Mei, X., et al. (2018). Phytochemistry, pharmacology, quality control and future research of forsythia suspensa (Thunb.) vahl: A review. J. Ethnopharmacol 210, 318–339. doi: 10.1016/j.jep.2017.08.040
Wu, T., Kerbler, S. M., Fernie, A. R., Zhang, Y. (2021). Plant cell cultures as heterologous bio-factories for secondary metabolite production. Plant Commun. 2, 100235. doi: 10.1016/j.xplc.2021.100235
Xie, L. H., Akao, T., Hamasaki, K., Deyama, T., Hattori, M. (2003). Biotransformation of pinoresinol diglucoside to mammalian lignans by human intestinal microflora, and isolation of enterococcus faecalis strain pdg-1 responsible for the transformation of (+)-pinoresinol to (+)-lariciresinol. Chem. Pharm. Bull. 51, 508–515. doi: 10.1248/cpb.51.508.
Keywords: Forsythia species, heterologous production, lignans, podophyllotoxin, tobacco, sesamin
Citation: Koyama T, Murata J, Horikawa M and Satake H (2022) Production of beneficial lignans in heterologous host plants. Front. Plant Sci. 13:1026664. doi: 10.3389/fpls.2022.1026664
Received: 24 August 2022; Accepted: 26 September 2022;
Published: 11 October 2022.
Edited by:
Suprasanna Penna, Bhabha Atomic Research Centre (BARC), IndiaReviewed by:
Neelakantan Arumugam, Pondicherry University, IndiaAlessandra Durazzo, Council for Agricultural Research and Economics, Italy
Katarína Ražná, Slovak University of Agriculture, Slovakia
Copyright © 2022 Koyama, Murata, Horikawa and Satake. This is an open-access article distributed under the terms of the Creative Commons Attribution License (CC BY). The use, distribution or reproduction in other forums is permitted, provided the original author(s) and the copyright owner(s) are credited and that the original publication in this journal is cited, in accordance with accepted academic practice. No use, distribution or reproduction is permitted which does not comply with these terms.
*Correspondence: Tomotsugu Koyama, koyama@sunbor.or.jp