- 1Key Laboratory of Sugarcane Biotechnology and Genetic Improvement (Guangxi), Ministry of Agriculture and Rural Affairs/Guangxi Key Laboratory of Sugarcane Genetic Improvement/Sugarcane Research Institute, Guangxi Academy of Agricultural Sciences, Nanning, Guangxi, China
- 2International Co-operation Division, Guangxi Academy of Agricultural Sciences, Nanning, Guangxi, China
- 3Department of Botany, University of Lucknow, Lucknow, India
- 4Academy of Biology and Biotechnology, Southern Federal University, Rostov-on-Don, Russia
Abiotic stresses cause extensive yield loss in various crops globally. Over the past few decades, the application of silicon nanoparticles (nSi) has emerged as an abiotic stress mitigator. The initial responses of plants are exemplified by the biogenesis of reactive oxygen species (ROS) to sustain cellular/organellar integrity, ensuring in vivo operation of metabolic functions by regulating physiological and biochemical pathways during stress conditions. Plants have evolved various antioxidative systems to balance/maintain the process of homeostasis via enzymatic and non-enzymatic activities that repair any losses. In an adverse environment, supplementation of Si mitigates the stress condition and improves the growth and development of plants. Its ameliorative effects are correlated with enhanced antioxidant enzymes activities, maintaining the equilibrium between ROS generation and reduction. However, a limited number of studies cover the role of nSi in abiotic stress conditions. This review addresses the accumulation and/or uptake of nSi in several crops, as well as its mode of action, which are linked with improved plant growth and tolerance capabilities, contributing to sustainable agriculture.
Introduction
Sustainable agriculture is a major economic sector associated with a wide range of food crops. Finding sustainable solutions for crop adaptation strategies to adverse environmental conditions and enhancing crop production are key to guaranteeing food security and safety worldwide. Abiotic stresses damage plant productivity of food crops by approximately 51–82% annually. Farmers regularly use pesticides and synthetic fertilizers to enhance crop production, which pose threats to the agricultural ecosystem. However, this approach may assist plant performance/fitness for crop improvement and increased fruit and grain quality (Verma et al., 2022a; Wang et al., 2022) during adverse environmental conditions. The enhancement of crop production is an emerging interdisciplinary area that can potentially promote plant growth and stress tolerance.
Nanoparticles (NPs) are 1–100 nm in size and have unique physiological features such as a large surface area, enhanced solubility, and translocation/uptake in an entire plant system. Several NPs, for example, Fe3O4, MgO, SiO2, and CeO2 are beneficial for plant development, playing an essential role in enhancing the seed germination rate and plant tolerance, reducing pesticide residues, and improving soil fertility (Salajegheh et al., 2020; Verma et al., 2022b; Wang et al., 2022). Silicon (Si) strongly bonds with oxygen in the earth, and its uptake was found to be approximately 0.1–10% (dry weight basis) in terrestrial plants (Epstein, 2009; Mathur and Roy, 2020). Si is available as silicates, oxides, aluminum silicates, and silica (SiO2), and these forms are easily accessible to plants since they are naturally available in the rhizosphere. Silicon is not considered an essential element for plant growth and development. It is classified as a ‘multi-talented,’ quasi-essential element due to its important role in physiological/metabolic pathways, cell structure, and plant survival during adverse environmental conditions. Among the various types of NPs, nSi has exhibited a significant ability to enhance plant performance in stressful conditions (Liang et al., 2015; Hussain et al., 2019; Verma et al., 2022d).
Soil fortification using nano-materials (NMs) is a trending development. Scientific groups have demonstrated various novel nano-stabilizers for soil improvement technologies. Among the various well-established additives (Kannan and Sujatha, 2022), nSi shows remarkable performance in Hordeum vulgare, Phaseolus vulgaris, Cucumis sativus, and Saccharum officinarum crops (Yassen et al., 2017; Elsheery et al., 2020; Ghorbanpour et al., 2020; Koleva et al., 2022). Studies have also suggested that nSi, as a sole-additive, boosts soil fertility. Furthermore, nSi helps in reducing the hydraulic conductivity and compression index of the soil (Kannan and Sujatha, 2022).
Recent investigations have indicated that the fertilization of soil with nSi stimulates the photosynthetic CO2 assimilation rate as well as biochemical and molecular responses in plants that resist unfavorable environmental conditions (Verma et al., 2022a). Abiotic stresses induce the generation of reactive oxygen species (ROS), i.e., singlet oxygen, superoxide, hydrogen peroxide, and hydroxyl radicals in cells (Das and Roychoudhury, 2014; Kim et al., 2017). ROS can cause severe oxidative injury to the protein, DNA, and lipids of the cell components. This review explores developments in crop improvement based on the existing literature and the current understanding of the action mechanisms of nSi in response to abiotic stresses, which improve physiological fitness/performance associated with sustainable agriculture.
Advantages and disadvantages of silicon nanoparticles
There is growing pressure on the agricultural sector to fulfill the requirements of the increasing human population. Synthetic fertilizers are indispensable in enhancing plant production and they are extensively applied through different approaches (Feregrino-Perez et al., 2018). However, plants utilize less than half of fertilizers applied and the remaining minerals may leach down, so that they become fixed in rhizospheric soil, contributing to water pollution (Liu and Lal, 2015; Zulfiqar et al., 2019). The uneven use of fertilization, without control of nutrient release, may affect crop quality. Thus, it is important to design slow/controlled-release fertilizers to sustain agricultural productivity (Rajput et al., 2021; Verma et al., 2022a). Consequently, the unique properties of NPs have attracted considerable attention in sustainable agriculture and environmental protection. Differences in the physical and chemical properties of nSi relative to their bulk counterparts occur due to their small size, higher surface area/weight ratio, and structure (Mathur and Roy, 2020). Recent findings showed better performance in plant development and alleviating environmental stresses when using nSi (Bhat et al., 2021; Verma et al., 2022b) (Figure 1 and Table 1).
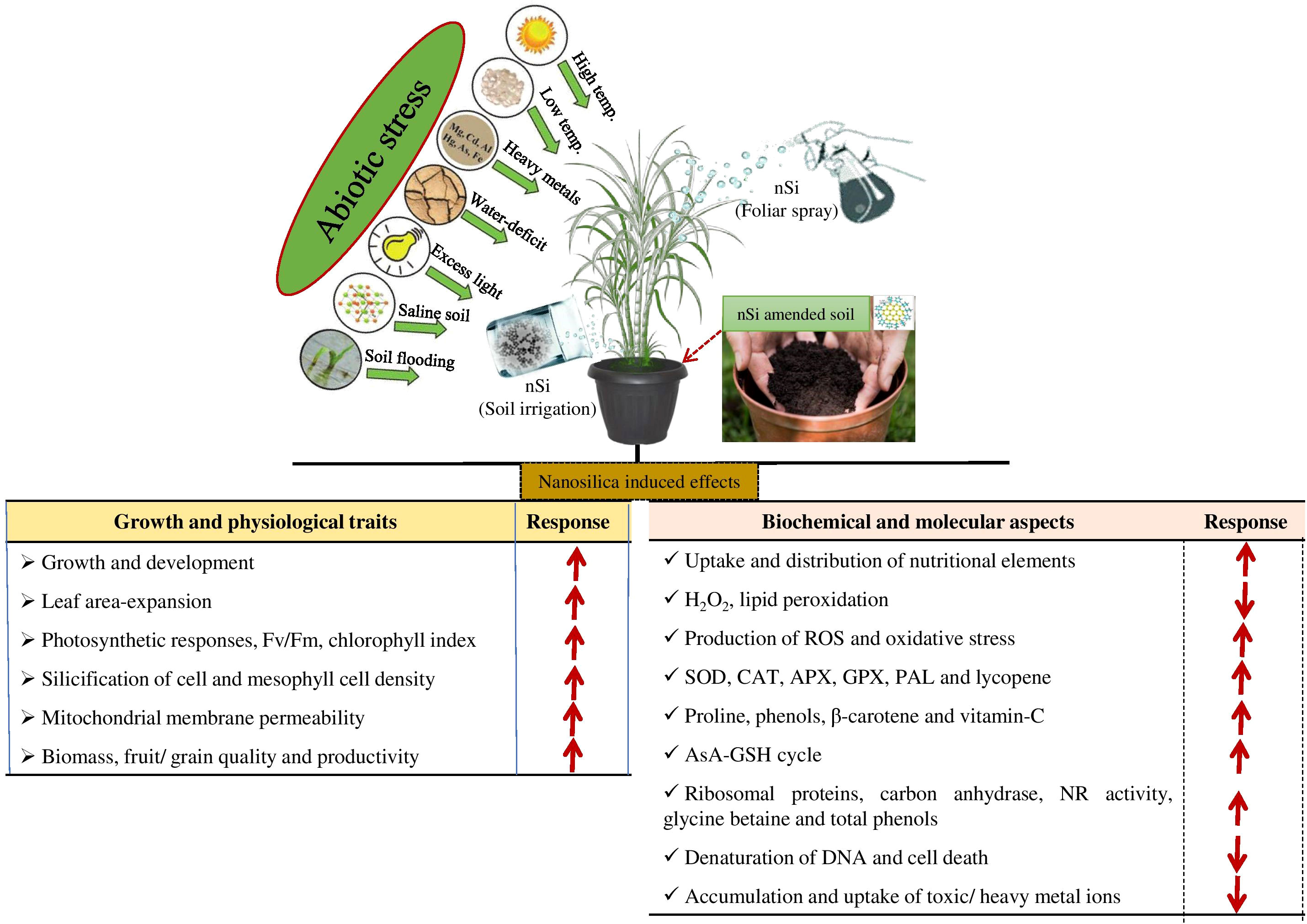
Figure 1 The potential mechanisms of nanosilicon (nSi) on plant physiological, biochemical, and molecular responses during unfavorable environmental conditions.
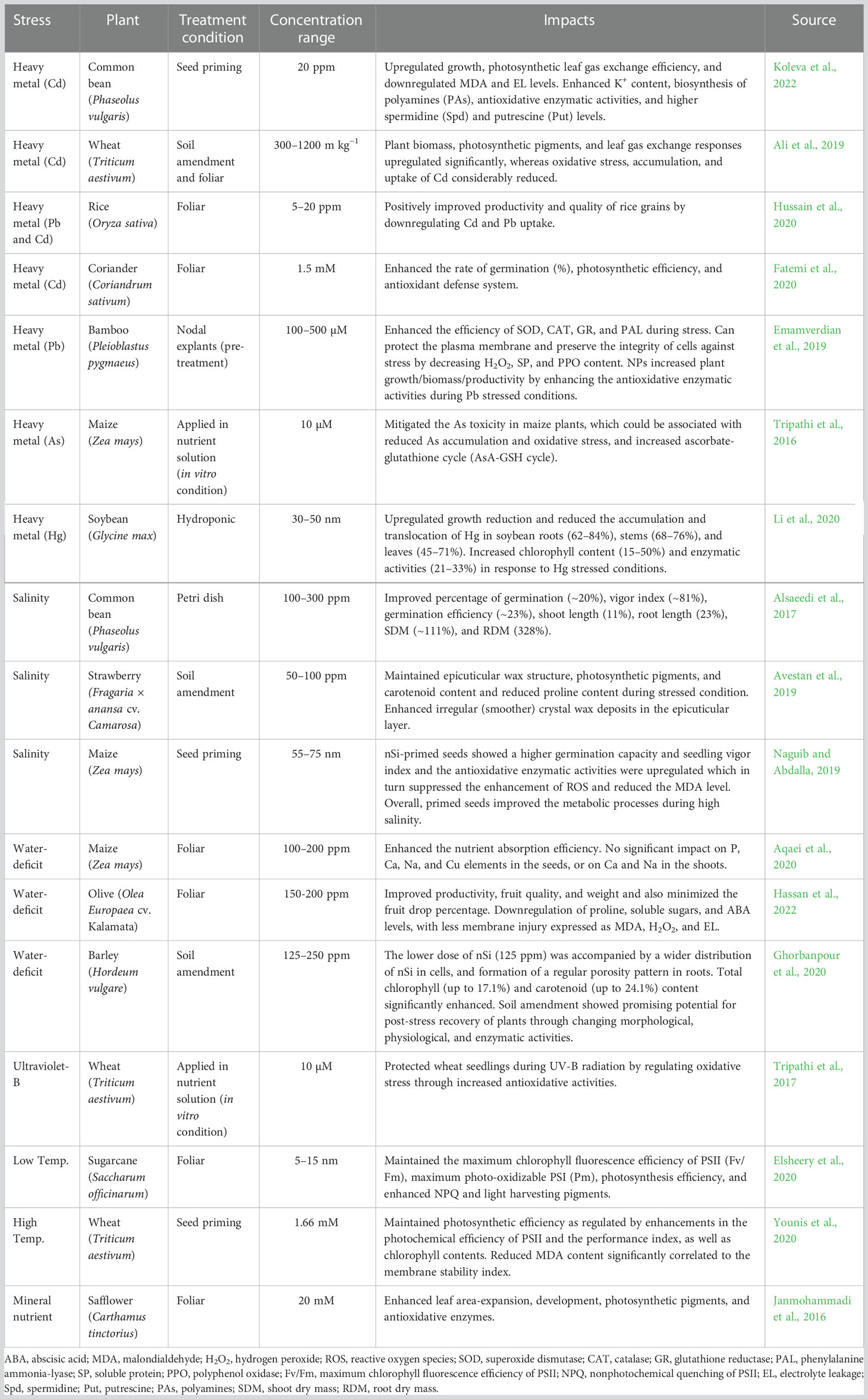
Table 1 Summary of the effects of nanosilicon (nSi) for the management of unfavorable environmental conditions in a variety of crops.
NPs may also have toxic effects on sustainable crop production, depending on various factors such as size, concentration, stability, application, and synthesis method (Rajput et al., 2021). A higher concentration of nSi reduced Triticum aestivum plant growth by affecting enzymatic and non-enzymatic activities, i.e., reduced photosynthetic pigments, lipid peroxidation (MDA), and enhanced antioxidative enzymatic responses (Karimi and Mohsenzadeh, 2016). Similar results were also noted in Bt-transgenic cotton (Le et al., 2014). With increasing concentration of nSi, the germination efficiency (%), root development, chromosomal aberration, and decrease in the mitotic index in Allium cepa were all affected (Silva and Monteiro, 2017). The application of nSi improved the root length, root volume, and dry mass of shoots and roots of O. sativa plants (Adhikari et al., 2013), while no phytotoxic effects were found in potato tubers (Mushinskiy et al., 2018). nSi was found to be toxic toward a number of bacterial species, viz., Bacillus subtilis, Escherichia coli, and Pseudomonas fluorescens (Jiang et al., 2009). Nanofertilizers can solve some limitations of biofertilizers, but this technology still requires further research and development.
Synthesis, characteristics, and absorption of nanosilicon
Nanosilicons are one of the most advanced innovations of nanoscience, and, in many regards, are more efficient than their bulk silica counterparts (Jeelani et al., 2019). Naturally, nSi exists in numerous crystalline forms, such as sand and quartz, while SiO4 units are arranged in a tetrahedral geometry, with various architectural compositions. Common types of nSi may be synthesized and separated in various forms such as monodisperse spherical, hollow, porous, etched, and colloidal molecules (Jeelani et al., 2019; Mathur and Roy, 2020). The synthesis of nSi from agricultural waste is cost-effective and sustainable for NM production. Mesoporous nSi (5–30 nm) with pore sizes of 3–9 nm has been synthesized by the precipitation of rice husk (Liou and Yang, 2011; Gu et al., 2015). Bentonite clay was found to be a good source of nSi (Zulfiqar et al., 2016), which may also be synthesized from tetraethyl orthosilicate (Pham et al., 2017).
Nanoparticles can be absorbed by plant roots or leaves. The uptake and accumulation of NPs may differ from plant to plant, depending on their morphology, various uptake mechanisms, transport, and allocation in certain plant organs which may activate defense-responsive mechanisms against the NPs (Verma et al., 2022c). Si can be applied as a foliar spray input on the plant leaves, or directly to the root system as a basal dressing. Foliar spray may enter into the leaves and be transported to different plant organs through the cuticular or stomata (Figure 1). The transport of solutes via the cuticle may occur through lipophilic pathways for non-polar solutes via diffusion and penetration, and hydrophilic pathways for polar solutes via water pores (Wang et al., 2022).
Application of nanosilicon for abiotic stress tolerance
Environmental stresses are major factors for plant productivity, have detrimental effects on plant development, and are a big problem for food security and safety worldwide (Verma et al., 2022a). This has urged botanists/agriculturists to enhance plant production by ~70% in the next three decades to overcome the present yield-limiting factors and to improve resource use efficiency. Recently, various studies have indicated that the application of nSi can positively reduce adverse responses to abiotic stresses such as soil texture, structure, clay minerals, pH, cation exchange capacity, soil organic matter, and soil microbial community, which affect the dispersion, aggregation, stability, solubility, bioavailability, and uptake or distribution of nSi (Rajput et al., 2021; Verma et al., 2022b). The application of nSi also mitigates the negative responses of abiotic stresses by upgrading the plants’ photosynthetic, antioxidative, and cellular processes. These comprehensive findings on the efficiency of nSi in mitigating adverse environmental conditions are shown in Figure 1 and Table 1.
Salt stress
The supplementation of nSi improved the photosynthetic leaf gas exchange, water-use efficiency (WUE), chlorophyll fluorescence yield of PSII, and photosynthetic pigments during saline stress conditions, thereby conferring increased stress resistance efficiency (Rajput et al., 2021; Verma et al., 2022a). During saline stress, nSi was shown to promote the germination (%) efficiency/rate, vigor index, plant biomass, root development, plant length, and leaf area expansion in cucumber plants (Alsaeedi et al., 2018). nSi enhanced the root and plant length of Glycine max (Farhangi-Abriz and Torabian, 2018), and the photosynthetic pigments also increased in Cynodon dactylon with the increasing level of salinity (Sharifiasl et al., 2019). In response to saline-sodic soils, foliar spray of nSi also enhanced the photosynthetic pigments, productivity, and grain quality in Oryza sativa plants (Kheir et al., 2019). It also maintains the deposition of epicuticular wax on the plant leaf surfaces and their stress tolerance capacity (Rajput et al., 2021).
The potential of nSi in seed priming subjected to saline conditions was demonstrated by the promotion of the germination rate and seedling vigor index, through upregulating the antioxidant enzyme activities, which, in turn, suppress the ROS increase and decrease the MDA content (Naguib and Abdalla, 2019). In natural conditions, plants produce ROS in cell organelles during photosynthetic and respiration processes. Plants may balance homeostasis via enzymatic and non-enzymatic detoxification mechanisms. nSi enhanced leaf proline and free amino acids to resist the penetration of NaCl in O. sativa and Ocimum basilicum plants (Abdel-Haliem et al., 2017; Kalteh et al., 2018). nSi reduced the influence of salt ions in plants by reducing Na− absorption (Abdel-Haliem et al., 2017; Kalteh et al., 2018). However, nSi activated defense-related enzymes in plants under saline conditions to alleviate injury caused by ROS accumulation (Naguib and Abdalla, 2019). The expression of stress-related genes (RBOH1, MAPK2, APX2, ERF5, MAPK3, and DDF2) was found to be reduced with increasing stress resistance capacity in Solanum lycopersicum (Almutairi, 2016; Wang et al., 2022) (Figure 1 and Table 1).
Water-stress
Using nSi in soil amendment, soil irrigation, and foliar spray effectively increased plant productivity and fruit/grain quality during stress conditions and maintained root development and photosynthetic CO2 assimilation (Rajput et al., 2021; Verma et al., 2022a). Water stress in general causes a reduction in the uptake of minerals such as nitrogen, sodium, calcium, iron, zinc, copper, manganese, silicon, etc. However, nSi upregulates nitrogen, potassium, and other nutrient uptake in T. aestivum plants during water stress (Aqaei et al., 2020; Mathur and Roy, 2020), with aggregation of nSi in plant leaves, which initiated stomatal closure to prevent water loss in Hordeum vulgare (Ghorbanpour et al., 2020). Water stress enhances ROS production, leading to the overproduction of MDA and causing plants to suffer oxidative damage. nSi enhanced antioxidative enzyme activities and reduced MDA concentration during stress (Rajput et al., 2021; Verma et al., 2022b) (Table 1).
Heavy metal stress
Excess uptake and accumulation of heavy metals (HMs) such as Pb, Cu, Cd, Cr, Hg, etc., in plant tissues via plant roots severely affect plant growth and development (Verma et al., 2022b). HMs persist in the soil for a long duration due to their adherent qualities; therefore, the soil is considered the major sink for HMs (Borah and Deka, 2023). Once HMs enter into the soil, they may percolate deep beneath the ground, leading to groundwater contamination. Monitoring and assessment of HM-associated risk factors in contaminated lands are essential for adopting a risk-based remediation approach (Borah et al., 2021). HMs adversely affect the soil’s biological properties and hinder ecosystem processes. Soil enzyme activities are considered one of the most reliable indicators of the biological state of soil and can also be used to measure the collective metabolism of the terrestrial ecosystem (Chakravarty and Deka, 2021; Kalita et al., 2022). However, soil enzyme activities such as phosphatase, urease, cellulase, dehydrogenase, and others are sensitive to the presence of HMs (Chakravarty and Deka, 2021; Borah and Deka, 2023).
nSi minimizes Cd accumulation in rice grains (by up to 30–60%), with enhanced translocation of potassium, magnesium, and iron (Chen et al., 2018). The interactive impact of Cd, Pb, Cu, and Zn in O. sativa cultivars with the use of nSi has revealed the potential of nSi to reduce the uptake of toxic ions in the grains (Wang et al., 2016). Soil amendment and foliar spray employing nSi were found to enhance agronomic characteristics and antioxidative enzymatic activities. The upregulated activities of antioxidant enzymes led to a reduction of H2O2, membrane MDA, and electrolyte leakage of primed seeds with increasing concentrations of nSi (Wang et al., 2022). The uptake of Cd in the roots and shoots of O. sativa plants was reduced by the interactive application of nSi and TiO2 NPs (Rizwan et al., 2019).
nSi increases the phytoremediation of Cd and Pb in Secale montanum plants (Moameri and Khalaki, 2019). The addition of nSi to the soils reduced the levels of extractable, exchangeable, and carbonate-bound Cd in the soil, thereby decreasing the metal toxicity (Mathur and Roy, 2020). The application of nSi increased the aluminum resistance in Zea mays by enhancing the uptake of nSi (de Sousa et al., 2019), significantly mitigated arsenic toxicity in Zea mays (Tripathi et al., 2016), and downregulated the expression of some HM transport genes, i.e., OsLCT1 and OsNramp5, decreasing the uptake and translocation of toxic metals in O. sativa (Cui et al., 2017).
Heat stress
Heat stress is the most crucial factor that affects plant growth and yield globally. Applying nSi effectively restored the heat stress-provoked ultracellular distortions in cellular organelles. nSi increased the photosynthetic efficiency, as revealed by the enhancement in the photochemical efficiency of PSII performance with chlorophyll content. Downregulation of MDA accumulation in nSi-applied plants was found to be correlated to their membrane stability index (Sun et al., 2014; Younis et al., 2020). However, prior treatment of T. aestivum with nSi reduced heat stress-induced negative ultrastructural variations, as revealed by the improved integrity of the nuclear envelope and the normal dispersion of chromatin (Younis et al., 2020). A high reactivity characterized the nSi binding affinity with PS II, which stabilized the photosynthetic activity during stress (Noji et al., 2010) and regulated the integrity of cell walls and membranes due to deposition of nSi at the interface of the plasma membrane-cell wall and/or in intercellular spaces (Bauer et al., 2011; Sun et al., 2014; Asgari et al., 2018) (Figure 1 and Table 1).
Cold stress
Cold stress causes significant loss to plant production in arid and semi-arid areas (Selvarajan et al., 2018). During cold stress, foliar application of nSi upregulated the chlorophyll fluorescence yield of PSII (Fv/Fm) and the maximum photo oxidizable P700 (Pm) activity. The improved Fv/Fm and Pm activity indicated the beneficial effects of NPs in S. officinarum plants during cold (Elsheery et al., 2020). nSi enhanced the photosynthetic pigments in S. officinarum plant leaves, which reveals that the NPs protected the plants from synthesizing different light-harvesting complexes, allowing the capture of large amounts of light energy, leading to enhanced photosynthetic responses (Ghafariyan et al., 2013; Verma et al., 2022a) (Figure 1 and Table 1). nSi also enhanced the growth and development of Solanum lycopersicum plants during short-term cold stress (Elsheery et al., 2020).
Technological improvements can increase the production of agro-industrially, physiologically, and agronomically essential NPs that can in turn be utilized to produce fertilizers with decreased nutritional losses and improved nutrient use efficiency (NUE), employing smart delivery systems. NPs can be applied as nanofertilizers on the plants or in the rhizospheric soil to boost fertilizer uptake and utilization. They can also be used to upgrade plant development through advanced nanobiotechnology, supporting nutrient delivery systems with targeted approaches and multifunctional features for improved sustainable agriculture in years to come (Wang et al., 2022; Verma et al., 2022a). In addition, many of the aforementioned results have been obtained from field experiments, and thus, further exploration in hydroponics/soilless cultures is required. The study of how to adjust the levels and initial adaptive responses of physiological, biochemical, and molecular levels using nSi can be an interesting research field in the near future.
Conclusions and future perspectives
Recent advanced sustainable agriculture strategies may be explored and applied to alleviate adverse environmental variables. Nowadays, the main objective of agricultural approaches is to promote plant production and quality. Hence, cost-effective technologies may be helpful for agri-farmers. Owing to their easy synthesis, maximum uptake, and large surface-to-volume ratio, nSi-based biofertilizers will be an excellent alternative to conventional synthetic fertilizers. Using nSi is an efficient approach for farmers to promote growth and production by increasing plant stress tolerance capacity. The drawback of using NPs on plants is DNA damage, which can occur through direct or indirect pathways. DNA damage occurs due to the degeneration of mitochondrial cristae, peroxisome proliferation, NO generation, and vacuolization. DNA repair processes are responsible for circumventing DNA damage; thus it also appears to be very important to evaluate the effects of NP exposure on these processes. The results presented above suggest that changes in stoichiometry are a potential morpho-functional adaptive response to NP exposure, caused by variations in the bioenergetic redox balance, which reduces the photosynthesis or cellular respiration rates.
Variations in nSi chemistry, size, shape, and electromagnetic properties lead to different findings on the effects and mechanisms of nSi in mitigating plant stresses. Earlier studies mainly discussed increasing physical barriers, growth promotion, inducing plant tolerance, and activating antioxidative enzymatic mechanisms, but rarely elucidated the impact of nSi on plant metabolites and the soil microbial community during stress conditions. Thus, the whole system should be considered, and in-depth omics research is needed on the nSi mechanisms that enhance a plant’s tolerance capacity to adversity, from the standpoint of physio-biochemical, transcriptomic, and proteomics levels. nSi-based fertilizers have opened up a whole new area of research opportunities for agro-scientists to synthesize advanced products that could help enhance agricultural productivity and reduce sustainable development challenges, without affecting the environment. The responsible application of nanotechnologies can hopefully play an important role in reaching this goal.
Author contributions
KV and Y-RL conceptualized the article. All authors contributed to the article and approved the submitted version.
Funding
This research was financially supported by the Guangxi Innovation Teams of Modern Agriculture Technology (nycytxgxcxtd-2021-03), the Youth Program of National Natural Science Foundation of China (31901594), The National Natural Science Foundation of China (31760415), Guangxi Natural Science Foundation (2021GXNSFAA220022), the Fund of Guangxi Academy of Agricultural Sciences (2021YT011), and the Guangxi Key Laboratory of Sugarcane Genetic Improvement Project (21-238-16-K-04-02).
Acknowledgments
The authors would like to thank the Guangxi Academy of Agricultural Sciences, Nanning, Guangxi, China, for providing the necessary facilities for this study.
Conflict of interest
The authors declare that the research was conducted in the absence of any commercial or financial relationships that could be construed as a potential conflict of interest.
The reviewer AK declared a shared affiliation with the author VR to the handling editor at the time of review.
Publisher’s note
All claims expressed in this article are solely those of the authors and do not necessarily represent those of their affiliated organizations, or those of the publisher, the editors and the reviewers. Any product that may be evaluated in this article, or claim that may be made by its manufacturer, is not guaranteed or endorsed by the publisher.
References
Abdel-Haliem, M. E. F., Hegazy, H. S., Hassan, N. S., Naguib, D. M. (2017). Effect of silica ions and nano silica on rice plants under salinity stress. Ecol. Eng. 99, 282–289. doi: 10.1016/j.ecoleng.2016.11.060
Adhikari, T., Kundu, S., Rao, A. S. (2013). Impact of SiO2 and Mo nano particles on seed germination of rice (Oryza sativa L.). Int. J. Agric. Food Sci. Technol. 4, 2249–3050.
Ali, S., Rizwan, M., Hussain, A., ur Rehman, M. Z., Ali, B., Yousaf, B., et al. (2019). Silicon nanoparticles enhanced the growth and reduced the cadmium accumulation in grains of wheat (Triticum aestivum L.). Plant Physiol. Biochem. 140, 1–8. doi: 10.1016/j.plaphy.2019.04.041
Almutairi, Z. M. (2016). Effect of nano-silicon application on the expression of salt tolerance genes in germinating tomato (Solanum lycopersicum L.) seedlings under salt stress. Plant Omics 9, 106–114.
Alsaeedi, A. H., El-Ramady, H., Alshaal, T., El-Garawani, M., Elhawat, N., Almohsen, M. (2017). Engineered silica nanoparticles alleviate the detrimental effects of Na+ stress on germination and growth of common bean (Phaseolus vulgaris). Environ. Sci. pollut. Res. 24, 21917–21928. doi: 10.1007/s11356-017-9847-y
Alsaeedi, A., El-Ramady, H., Alshaal, T., El-Garawani, M., Elhawat, N., Al-Otaibi, A. (2018). Exogenous nanosilica improves germination and growth of cucumber by maintaining K+/Na+ ratio under elevated Na+ stress. Plant Physiol. Biochem. 125, 164–171. doi: 10.1016/j.plaphy.2018.02.006
Aqaei, P., Weisany, W., Diyanat, M., Razmi, J., Struik, P. C. (2020). Response of maize (Zea mays L.) to potassium nano-silica application under drought stress. J. Plant Nutr. 43, 1205–1216. doi: 10.1080/01904167.2020.1727508
Asgari, F., Majd, A., Jonoubi, P., Najafi, F. (2018). Effects of silicon nanoparticles on molecular, chemical, structural and ultrastructural characteristics of oat (Avena sativa L.). Plant Physiol. Biochem. 127, 152–160. doi: 10.1016/j.plaphy.2018.03.021
Avestan, S., Ghasemnezhad, M., Esfahani, M., Byrt, C. S. (2019). Application of nano-silicon dioxide improves salt stress tolerance in strawberry plants. Agronomy 9 (5), 246. doi: 10.3390/agronomy9050246
Bauer, P., Elbaum, R., Weiss, I. M. (2011). Calcium and silicon mineralization in land plants: transport, structure and function. Plant Sci. 180, 746–756. doi: 10.1016/j.plantsci.2011.01.019
Bhat, J. A., Rajora, N., Raturi, G., Sharma, S., Dhiman, P., Sanand, S., et al. (2021). Silicon nanoparticles (SiNPs) in sustainable agriculture: major emphasis on the practicality, efficacy and concerns. Nanoscale Adv. 3, 4019–4028. doi: 10.1039/d1na00233c
Borah, G., Deka, H. (2023). Crude oil associated heavy metals (HMs) contamination in agricultural land: Understanding risk factors and changes in soil biological properties. Chemosphere 310, 136890. doi: 10.1016/j.chemosphere.2022.136890
Borah, G., Nath, N., Deka, H. (2021). Effects on anatomy of some abundantly growing herbs in the effluents contaminated soil of oil refinery. Environ. Sci. pollut. Res. 28, 11549–11557. doi: 10.1007/s11356-020-11407-6
Chakravarty, P., Deka, H. (2021). Enzymatic defense of cyperus brevifolius in hydrocarbons stress environment and changes in soil properties. Sci. Rep. 11, 718. doi: 10.1038/s41598-020-80854-5
Chen, R., Zhang, C., Zhao, Y., Huang, Y., Liu, Z. (2018). Foliar application with nano-silicon reduced cadmium accumulation in grains by inhibiting cadmium translocation in rice plants. Environ. Sci. pollut. Res. 25, 2361–2368. doi: 10.1007/s11356-017-0681-z
Cui, J., Liu, T., Li, F., Yi, J., Liu, C., Yu, H. (2017). Silica nanoparticles alleviate cadmium toxicity in rice cells: Mechanisms and size effects. Environ. pollut. 228, 363–369. doi: 10.1016/j.envpol.2017.05.014
Das, K., Roychoudhury, A. (2014). Reactive oxygen species (ROS) and response of antioxidants as ROS-scavengers during environmental stress in plants. Front. Environ. Sci. 2. doi: 10.3389/fenvs.2014.00053
de Sousa, A., Saleh, A. M., Habeeb, T. H., Hassan, Y. M., Zrieq, R., Wadaan, M. A. M., et al. (2019). Silicon dioxide nanoparticles ameliorate the phytotoxic hazards of aluminum in maize grown on acidic soil. Sci. Total Environ. 693, 133636. doi: 10.1016/j.scitotenv.2019.133636
Elsheery, N. I., Sunoj, V. S. J., Wen, Y., Zhu, J. J., Muralidharan, G., Cao, K. F. (2020). Foliar application of nanoparticles mitigates the chilling effect on photosynthesis and photoprotection in sugarcane. Plant Physiol. Biochem. 149, 50–60. doi: 10.1016/j.plaphy.2020.01.035
Emamverdian, A., Ding, Y., Mokhberdoran, F., Xie, Y., Zheng, X., Wang, Y. (2019). Silicon dioxide nanoparticles improve plant growth by enhancing antioxidant enzyme capacity in bamboo (Pleioblastus pygmaeus) under lead toxicity. Trees - Struct. Funct. 34, 469–481. doi: 10.1007/s00468-019-01929-z
Epstein, E. (2009). Silicon: its manifold roles in plants. Ann. Appl. Biol. 155, 155–160. doi: 10.1111/j.1744-7348.2009.00343.x
Farhangi-Abriz, S., Torabian, S. (2018). Nano-silicon alters antioxidant activities of soybean seedlings under salt toxicity. Protoplasma 255, 953–962. doi: 10.1007/s00709-017-1202-0
Fatemi, H., Pour, B. E., Rizwan, M. (2020). Isolation and characterization of lead (Pb) resistant microbes and their combined use with silicon nanoparticles improved the growth, photosynthesis and antioxidant capacity of coriander (Coriandrum sativum L.) under Pb stress. Environ. pollut. 266, 114982. doi: 10.1016/j.envpol.2020.114982
Feregrino-Perez, A. A., Magana-Lopez, E., Guzman, C., Esquivel, K. (2018). A general overview of the benefits and possible negative effects of the nanotechnology in horticulture. Sci. Hortic. 238, 126–137. doi: 10.1016/j.scienta.2018.03.060
Ghafariyan, M. H., Malakouti, M. J., Dadpour, M. R., Stroeve, P., Mahmoudi, M. (2013). Effects of magnetite nanoparticles on soybean chlorophyll. Environ. Sci. Technol. 47 (18), 10645–10652. doi: 10.1021/es402249b
Ghorbanpour, M., Mohammadi, H., Kariman, K. (2020). Nanosilicon-based recovery of barley (Hordeum vulgare) plants subjected to drought stress. Environ. Sci. Nano 7, 443–461. doi: 10.1039/C9EN00973F
Gu, S., Zhou, J., Yu, C., Luo, Z., Wang, Q., Shi, Z. (2015). A novel two-staged thermal synthesis method of generating nanosilica from rice husk via pre-pyrolysis combined with calcination. Ind. Crops Prod. 65, 1–6. doi: 10.1016/j.indcrop.2014.11.045
Hassan, I. F., Ajaj, R., Gaballah, M. S., Ogbaga, C. C., Kalaji, H. M., Hatterman-Valenti, H. M., et al. (2022). Foliar application of nano-silicon improves the physiological and biochemical characteristics of 'Kalamata' olive subjected to deficit irrigation in a semi-arid climate. Plants 11 (12), 1561. doi: 10.3390/plants11121561
Hussain, B., Lin, Q., Hamid, Y., Sanaullah, M., Di, L., Khan, M. B., et al. (2020). Foliage application of selenium and silicon nanoparticles alleviates Cd and Pb toxicity in rice (Oryza sativa L.). Sci. Total Environ. 712, 136497. doi: 10.1016/j.scitotenv.2020.136497
Hussain, A., Rizwan, M., Ali, Q., Ali, S. (2019). Seed priming with silicon nanoparticles increased biomass and yield while reduced the oxidative stress and cadmium concentration in wheat grains. Environ. Sci. pollut. Res. 26, 7579–7588. doi: 10.1007/s11356-019-04210-5
Janmohammadi, M., Amanzadeh, T., Sabaghnia, N., Ion, V. (2016). Effect of nano-silicon foliar application on safflower growth under organic and inorganic fertilizer regimes. Bot. Lith. 22, 53–64. doi: 10.1515/botlit-2016-0005
Jeelani, P. G., Mulay, P., Venkat, R., Ramalingam, C. (2019). Multifaceted application of silica nanoparticles. A review. Silicon. Silicon 12, 1337–1354. doi: 10.1007/s12633-019-00229-y
Jiang, W., Mashayekhi, H., Xing, B. (2009). Bacterial toxicity comparison between nano- and micro-scaled oxide particles. Environ. pollut. 157, 1619–1625. doi: 10.1016/j.envpol.2008.12.025
Kalita, M., Chakravarty, P., Deka, H. (2022). Understanding biochemical defense and phytoremediation potential of Leucas aspera in crude oil polluted soil. Environ. Sci. pollut. Res. 29, 57579–57590. doi: 10.1007/s11356-022-19922-4
Kalteh, M., Alipour, Z. T., Ashraf, S., Aliabadi, M. M., Nosratabadi, A. F. (2018). Effect of silica nanoparticles on basil (Ocimumbasilicum) under salinity stress. J. Chem. Health Risks 4, 49–55.
Kannan, G., Sujatha, E. R. (2022). A review on the choice of nano-silica as soil stabilizer. Silicon 14, 6477–6492. doi: 10.1007/s12633-021-01455-z
Karimi, J., Mohsenzadeh, S. (2016). Effects of silicon oxide nanoparticles on growth and physiology of wheat seedlings. Russ. J. Plant Physiol. 63, 119–123. doi: 10.1134/S1021443716010106
Kheir, A. M. S., Abouelsoud, H. M., Hafez, E. M., Ali, O. A. M. (2019). Integrated effect of nano-zn, nano-Si, and drainage using crop straw–filled ditches on saline sodic soil properties and rice productivity. Arab. J. Geosci. 12, 471. doi: 10.1007/s12517-019-4653-0
Kim, Y.-H., Khan, A. L., Waqas, M., Lee, I.-J. (2017). Silicon regulates antioxidant activities of crop plants under abiotic-induced oxidative stress: A review. Front. Plant Sci. 8. doi: 10.3389/fpls.2017.00510
Koleva, L., Umar, A., Yasin, N. A., Shah, A. A., Siddiqui, M. H., Alamri, S., et al. (2022). Iron oxide and silicon nanoparticles modulate mineral nutrient homeostasis and metabolism in cadmium-stressed phaseolus vulgaris. Front. Plant Sci. 13. doi: 10.3389/fpls.2022.806781
Le, V. N., Rui, Y., Gui, X., Li, X., Liu, S., Han, Y. (2014). Uptake, transport, distribution and bio-effects of SiO2 nanoparticles in bt-transgenic cotton. J. Nanobiotechnol. 12, 1–15. doi: 10.1186/s12951-014-0050-8
Li, Y., Zhu, N., Liang, X., Bai, X., Zheng, L., Zhao, J., et al. (2020). Silica nanoparticles alleviate mercury toxicity via immobilization and inactivation of Hg(II) in soybean (Glycine max). Environ. Sci.: Nano 7, 1807–1817. doi: 10.1039/D0EN00091D
Liang, Y., Nikolic, M., Belanger, R., Gong, H., Song, A. (2015). Silicon in agriculture: From theory to practice (Dordrecht, The Netherlands: Springer Science+Business Media). doi: 10.1007/978-94-017-9978-2
Liou, T. H., Yang, C. C. (2011). Synthesis and surface characteristics of nanosilica produced from alkali-extracted rice husk ash. Mater. Sci. Eng. B Solid-State Mater. Adv. Technol. 176, 521–529. doi: 10.1016/j.mseb.2011.01.007
Liu, R., Lal, R. (2015). Potentials of engineered nanoparticles as fertilizers for increasing agronomic productions. Sci. Total Environ. 514, 131–139. doi: 10.1016/j.scitotenv.2015.01.104
Mathur, P., Roy, S. (2020). Nanosilica facilitates silica uptake, growth and stress tolerance in plants. Plant Physiol. Biochem. 157, 114–127. doi: 10.1016/j.plaphy.2020.10.011
Moameri, M., Abbasi Khalaki, M. (2019). Capability of secale montanum trusted for phytoremediation of lead and cadmium in soils amended with nano-silica and municipal solid waste compost. Environ. Sci. pollut. Control Ser. 26, 24315–24322. doi: 10.1007/s11356-017-0544-7
Mushinskiy, A. A., Aminovа, E. V., Korotkova, A. M. (2018). Evaluation of tolerance of tubers Solanum tuberosum to silicа nanoparticles. Environ. Sci. pollut. Res. 25, 34559–34569. doi: 10.1007/s11356-018-3268-4
Naguib, D. M., Abdalla, H. (2019). Metabolic status during germination of nano silica primed Zea mays seeds under salinity stress. J. Crop Sci.Biotechnol. 22, 415–423. doi: 10.1007/s12892-019-0168-0
Noji, T., Kamidaki, C., Kawakami, K., Shen, J. R., Kajino, T., Fukushima, Y., et al. (2010). Photosynthetic oxygen evolution in mesoporous silica material: adsorption of photosystem II reaction center complex into 23 nm nanopores in SBA. Langmuir 27, 705–713. doi: 10.1021/la1032916
Pham, Q. T., Yao, Z. H., Wang, Y. T., Wu, Y. T., Chern, C. S. (2017). Preparation and characterization of monodisperse silica nanoparticles via miniemulsion sol–gel reaction of tetraethyl orthosilicate. J. Mater. Sci. 52, 12706–12716. doi: 10.1007/s10853-017-1402-1
Rajput, V. D., Minkina, T., Feizi, M., Kumari, A., Khan, M., Mandzhieva, S., et al. (2021). Effects of silicon and silicon-based nanoparticles on rhizosphere microbiome, plant stress and growth. Biology 10, 791. doi: 10.3390/biology10080791
Rizwan, M., Noureen, S., Ali, S., Anwar, S., Qayyum, M. F., Hussain, A. (2019). Influence of biochar amendment and foliar application of iron oxide nanoparticles on growth, photosynthesis, and cadmium accumulation in rice biomass. J. Soils Sediments 19 (11), 3749–3759. doi: 10.1007/s11368-019-02327-1
Salajegheh, M., Yavarzadeh, M., Payandeh, A., Payandeh, A., Akbarian, M. M. (2020). Effects of titanium and silicon nanoparticles on antioxidant enzymes activity and some biochemical properties of Cuminum cyminum L. under drought stress. Banat’s J. Biotechnol. 11, 19–25. doi: 10.7904/2068-4738-XI(21)-19
Selvarajan, D., Mohan, C., Dhandapani, V., Nerkar, G., Jayanarayanan, A., Mohanan, V., et al. (2018). Differential gene expression profiling through transcriptome approach of Saccharum spontaneum L. under low temperature stress reveals genes potentially involved in cold acclimation. 3 Biotech. 8, 195.
Sharifiasl, R., Kafi, M., Saidi, M., Kalatejari, S. (2019). Influence of nano-silica and humic acid on physiological characteristics of Bermuda grass (Cynodondactylon L.) under salinity stress. Acta Sci. Pol. Hortorum Cultus 18, 203–212. doi: 10.24326/asphc.2019.4.19
Silva, G. H., Monteiro, R. T. R. (2017). Toxicity assessment of silica nanoparticles on allium cepa. Ecotoxicol. Environ. Contam. 12, 25–31. doi: 10.5132/eec.2017.01.04
Sun, D., Hussain, H. I., Yi, Z., Siegele, R., Cresswell, T., Kong, L., et al. (2014). Uptake and cellular distribution, in four plant species, of fluorescently labeled mesoporous silica nanoparticles. Plant Cell Rep. 33, 1389–1402. doi: 10.1007/s00299-014-1624-5
Tripathi, D. K., Singh, S., Singh, V. P., Prasad, S. M., Chauhan, D. K., Dubey, N. K. (2016). Silicon nanoparticles more efficiently alleviate arsenate toxicity than silicon in maize cultiver and hybrid differing in arsenate tolerance. Front. Environ. Sci. 4. doi: 10.3389/fenvs.2016.00046
Tripathi, D. K., Singh, S., Singh, V. P., Prasad, S. M., Dubey, N. K., Chauhan, D. K. (2017). Silicon nanoparticles more effectively alleviated UV-b stress than silicon in wheat (Triticum aestivum) seedlings. Plant Physiol.Biochem. 110, 70–81. doi: 10.1016/j.plaphy.2016.06.026
Verma, K. K., Song, X. P., Chen, Z. L., Tian, D. D., Rajput, V. D., Singh, M., et al. (2022c). “Silicon and nanosilicon mitigate nutrient deficiency under stress for sustainable crop improvement,” in Silicon and nano-silicon in environmental stress management and crop quality improvement: progress and prospects. Ed. Etesami, et al (UK: Academic Press), 207–218.
Verma, K. K., Song, X.-P., Joshi, A., Rajput, V. D., Singh, M., Sharma, A., et al. (2022a). Nanofertilizer possibilities for healthy soil, water, and food in future: An overview. Front. Plant Sci. 13. doi: 10.3389/fpls.2022.865048
Verma, K. K., Song, X.-P., Joshi, A., Tian, D.-D., Rajput, V., Singh, M., et al. (2022b). Recent trends in nano-fertilizers for sustainable agriculture under climate change for global food security. Nanomaterials 12, 173. doi: 10.3390/nano12010173
Verma, K. K., Song, X.-P., Singh, M., Huang, H.-R., Bhatt, R., Xu, L., et al. (2022d). Influence of nanosilicon on drought tolerance in plants: An overview. Front. Plant Sci. 13. doi: 10.3389/fpls.2022.1014816
Wang, L., Ning, C., Pan, T., Cai, K. (2022). Role of silica nanoparticles in abiotic and biotic stress tolerance in plants: A review. Int. J. Mol. Sci. 23, 1947. doi: 10.3390/ijms23041947
Wang, S., Wang, F., Gao, S., Wang, X. (2016). Heavy metal accumulation in different rice cultivars as influenced by foliar application of nano-silicon. Water. Air. Soil pollut. 227, 228. doi: 10.1007/s11270-016-2928-6
Yassen, A., Abdallah, E., Gaballah, M., Zaghloul, S. (2017). Role of silicon dioxide nano fertilizer in mitigating salt stress on growth, yield and chemical composition of cucumber (Cucumis sativus L.). Int. J. Agric. Res. 12, 130–135. doi: 10.3923/ijar.2017.130.135
Younis, A. A., Khattab, H., Emam, M. M. (2020). Impacts of silicon and silicon nanoparticles on leaf ultrastructure and TaPIP1 and TaNIP2 gene expressions in heat stressed wheat seedlings. Biol. Plantarum 64, 343–352. doi: 10.32615/bp.2020.030
Zulfiqar, F., Navarro, M., Ashraf, M., Akram, N. A., Munne-Bosch, S. (2019). Nanofertilizer use for sustainable agriculture: advantages and limitations. Plant Sci. 289, 110270. doi: 10.1016/j.plantsci.2019.110270
Keywords: leaf gas exchange, enzymatic and non-enzymatic activities, abiotic stress, nano-silica, stress relief, environmental health
Citation: Verma KK, Zeng Y, Song X-P, Singh M, Wu K-C, Rajput VD and Li Y-R (2022) Nanosilicon: An approach for abiotic stress mitigation and sustainable agriculture. Front. Plant Sci. 13:1025974. doi: 10.3389/fpls.2022.1025974
Received: 23 August 2022; Accepted: 12 December 2022;
Published: 20 December 2022.
Edited by:
Prabhakaran Soundararajan, National Institute of Plant Genome Research (NIPGR), IndiaCopyright © 2022 Verma, Zeng, Song, Singh, Wu, Rajput and Li. This is an open-access article distributed under the terms of the Creative Commons Attribution License (CC BY). The use, distribution or reproduction in other forums is permitted, provided the original author(s) and the copyright owner(s) are credited and that the original publication in this journal is cited, in accordance with accepted academic practice. No use, distribution or reproduction is permitted which does not comply with these terms.
*Correspondence: Xiu-Peng Song, eGl1cGVuZ3NvbmdAZ3hhYXMubmV0; Yang-Rui Li, bGl5ckBneGFhcy5uZXQ=
†These authors have contributed equally to this work
‡ORCID: Yang-Rui Li, orcid.org/0000-0002-7559-9244