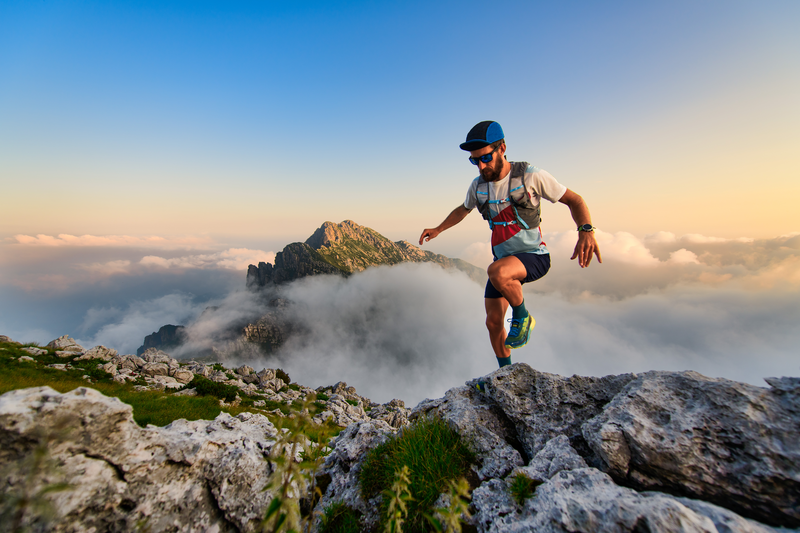
94% of researchers rate our articles as excellent or good
Learn more about the work of our research integrity team to safeguard the quality of each article we publish.
Find out more
REVIEW article
Front. Plant Sci. , 11 October 2022
Sec. Plant Abiotic Stress
Volume 13 - 2022 | https://doi.org/10.3389/fpls.2022.1023636
This article is part of the Research Topic Nanofertilizers and Abiotic Stress Tolerance in Plants View all 9 articles
The efficient use of natural resources without negative repercussions to the environment has encouraged the incursion of nanotechnology to provide viable alternatives in diverse areas, including crop management. Agriculture faces challenges due to the combination of different abiotic stresses where nanotechnology can contribute with promising applications. In this context, several studies report that the application of nanoparticles and nanomaterials positively affects crop productivity through different strategies such as green synthesis of nanoparticles, plant targeted protection through the application of nanoherbicides and nanofungicides, precise and constant supply of nutrients through nanofertilizers, and tolerance to abiotic stress (e.g., low or high temperatures, drought, salinity, low or high light intensities, UV-B, metals in soil) by several mechanisms such as activation of the antioxidant enzyme system that alleviates oxidative stress. Thus, the present review focuses on the benefits of NPs against these type of stress and their possible action mechanisms derived from the interaction between nanoparticles and plants, and their potential application for improving agricultural practices.
Climate change, water deficit, low nutrient use efficiency, crop pests, and decreasing availability of land for crop development, combined with the increasing demand for food due to a growing world population, require the sustainable development of agriculture (Seleiman et al., 2021; Ayub et al., 2022; Gil-Díaz et al., 2022). The efficient use of natural resources without negative repercussions to the environment has encouraged the incursion of new technologies, such as nanotechnology to provide viable alternatives in diverse areas, including agriculture. According to the definition, first coined in 1974 at the Tokyo University of Science, nanotechnology is simply the manipulation of matter at the nanometer scale, and nanoparticles (NPs) are defined as molecules manufactured or synthesized at this scale (Khan and Rizvi, 2014; Seleiman et al., 2020; Badawy et al., 2021; Yu et al., 2021). Nanotechnology involves the utilization of NPs within a size range of 1 to 100 nm with exceptional characteristics such as a high surface/volume ratio and various physicochemical and biochemical properties, which depends on their high surface energy (Dahoumane et al., 2017; Sukhanova et al., 2018) (Ijaz et al., 2020). provides detailed information on the classification of NPs and NMs, where a general classification is into organic (e.g., ferritin, micelles, dendrimers, and liposomes) and inorganic. However, according to their size, shape, structure, and synthesis, they can be more specifically classified into metals (e.g., aluminum, gold, iron, lead, silver, cobalt, zinc, cadmium, and copper), metal oxides (e.g., zinc oxide, silicon dioxide, iron oxide, aluminum oxide, cerium oxide, titanium oxide, and magnetite), ceramics, bio-NPs (e.g., magnetosomes, exosomes, lipoproteins, and viruses), and carbon-based NMs (fullerenes, carbon nanotubes (CNT), graphene, carbon black and carbon nanofibers) (Ijaz et al., 2020; Khan and Hossain, 2022). Novel agricultural applications of nanotechnology in agriculture has focused on the research for the activation of the secondary metabolism in plants, a mechanism of adaptation and evolution as a defense to harsh environmental conditions (biotic and abiotic) (Aguirre-Becerra et al., 2021). The effect of NPs in plant protection against abiotic stress has been documented in several studies. Several applications of NPs and NMs have been proposed and studied to achieve this goal. For example, producing nanocomposites containing fertilizers, pesticides, and microorganisms is one of the most common applications (Usman et al., 2020). Nanoencapsulation of macronutrients, micronutrients, microorganisms, and organic compounds for nano-biofertilization has demonstrated an interesting synergy between crop and substrate microorganisms (Sambangi et al., 2022). Additionally, green methodologies for synthesizing NPs through extracts of plant, fungi, and algae that minimize environmental impact has demonstrated lower phytotoxicity and less environmental impact than the traditional physicochemical approach (Kaur and Sidhu, 2021). Those features impact the production of plant growth regulators and the activation of the secondary metabolism to produce bioactive compounds that protect plants from abiotic stress. Thus, the present article reviews the recent developments on applications of nanotechnology in agriculture, focusing on the benefits of NPs and NMs against drought and salinity, temperature, soil contamination and light stress (Figure 1) and their possible action mechanisms without ignoring the potentially toxic effects derived from the interaction between NPs-Plant-Substrate.
The characteristics of NPs and NMs are adequate and promising for improving plant traits and their implementation in agricultural practices. Dimensions smaller than 100 nm, high surface-volume ratio, slow and sustained release potential, diverse nature (i.e., organic, inorganic, and hybrid), shapes, textures, and surface charge diversity, specific interaction, and stability, are useful characteristics of NPs that generate a high potential for their implementation in agricultural practices (Farokhzad and Langer, 2009; Paramo et al., 2020) (Ali et al., 2021; Ali et al., 2021). indicate that the aforementioned characteristics of NPs increase their permeability, promoting cell membrane penetration, and therefore, facilitating their entrance through the leaves and roots of plants (Liscano et al., 2000). Additionally, hollow formations can protect against degradation processes and increase flexibility and elasticity in non-rigid structures, although they can also provide rigidity and compactness to solid structures. The diverse behavior of NPs also makes them viable for developing nanopesticides, nanoherbicides, and nanosensors for plant, soil, and pest monitoring in an intelligently controlled environment, a trend in agriculture 4.0 (Kah et al., 2019; Rastogi et al., 2019; Shawon et al., 2020). However, some of the mentioned physical and chemical characteristics are also related to phytotoxicity, potential environmental toxicity, and human health hazards (Paramo et al., 2020; Ayub et al., 2022), which involves mandatory experimentation to find the appropriate doses, exposure times, and types of NP types for every crop.
The incursion of nanotechnology in agriculture is relatively recent, where the production of nanocomposites containing fertilizers, pesticides, and microorganisms is one of the most important applications (Usman et al., 2020; Elshayb et al., 2022). For instance, nano-biosensors are playing an important role in revolutionizing farming through the development of diagnostic tools and techniques for identifying heavy metal (HM) ions, pollutants, microbial load, and pathogens, along with fast environmental monitoring. Some are placed on the leaves to measure the hydrogen peroxide, a molecule for plant “communication,” producing physical signals (e.g., electric and light) that can be observed and analyzed (Johnson et al., 2021). Nanotechnology also contributes to remediation strategies for contaminated soils that are important regarding the mobility, fate, and toxicity of soil pollutants that considerably decrease crop yield due to plant stress (Usman et al., 2020). In this regard, NPs and NMs can also provide plant protection from abiotic stress conditions (e.g., drought, salinity, low temperatures, UV rays, and toxic trace metals) by several mechanisms such as activating the antioxidant enzyme system that alleviates oxidative stress.
Nanoencapsulation of nutrients or microorganisms for nanofertilization is becoming popular in agriculture because the material that constitutes the NPs can generate synergy with the crop and the microorganisms present in the substrate, providing plants with compounds that are important for their development (Tan et al., 2017). For example, nanoencapsulated B was foliar applied to sweet potato plants, increasing B, Fe, and Mn concentration in leaves, and NIP aquaporins and Boron transporters expression (Nicolas-Espinosa et al., 2022). Nano-chelated Fe fertilizer on rice increased biological yield by 27%, decreased hollow grain number by 254% and raised protein content and N, P, K, Fe, and Zn concentrations (Fakharzadeh et al., 2020). In the same way, the combination of FeNPs, ZnNPs, and CuNPs with a commercial fertilizer in a tomato crop increased the plant’s height, stem diameter, root length, photosynthetic pigments, leaf minerals, and protein, fiber, Fe, Zn, and K, and antioxidant properties of tomatoes (Rahman et al., 2021). Additionally, the fertilization with NPK with the spraying of VNPs and SeNps in rice increased plant height, chlorophyll index, biological yield, grain yield, harvest index, and agronomic efficiency (Al-Karaawi and Al-Juthery, 2022). Nanoencapsulation of microorganisms or organic compounds is a trend in nano-biofertilizing. For example, growth-promoting rhizobacteria and their metabolites were nanoencapsulated using SiNPs and carbon nanotubes to improve pistachio micropropagation, where the use of Pseudomonas fluorescens VUPF5 and Bacillus subtilis VRU1 nanocapsules significantly enhanced the root length, shoot length, fresh weight, and proliferation (Moradipour et al., 2019). Moreover, a foliar application of nano-chitosan under different phosphorus fertilizer levels on hot pepper increased plant height, the number of branches per plant, total plant dry weight, fruit set, fruit length, fruit diameter, yield, total chlorophyll, total soluble solids, vitamin C, total nitrogen, total phosphorus, total potassium, and capsaicin content (Helaly and EL-Bauome, 2020).
In addition to mitigating environmental stress on crops, one of the trends in this area is the search for green methodologies for the synthesis of NPs that minimize environmental impact. Different techniques to synthesize NPs result in compounds with different shapes, sizes, and biological activities and applications in different areas (Ali et al., 2021; Ali et al., 2021). In recent times, the synthesis of metal and metal oxide NPs using plant, fungi and algae extracts has recently emerged in the field of nanoscience and nanobiotechnology, with numerous advantages over conventional physicochemical approaches (Karthik et al., 2022; Srivastava and Bhargava, 2022a; Srivastava and Bhargava, 2022b). Chemical reduction is the most common procedure to synthetize NPs. Other chemical techniques include electrochemical techniques, solvo-thermal reduction, and photochemical reaction in reverse micelles (Gudikandula and Charya Maringanti, 2016). However, the implementation of chemical reducing agents requires considerable amounts of energy and generates byproducts that are dangerous for the environment and human health (Guan et al., 2022). Some bacteria, fungi and plant extracts can be used to synthesize NPs (Table 1), simplifying the production process and decreasing the use of solvents and energy, making this green technology increasingly attractive to both scientists and industry (Panpatte et al., 2016; Park et al., 2016; Dahoumane et al., 2017). These environmentally friendly methodologies are cost-effective, rapid, less arduous and more proficient than the conventional chemical procedures and cost-effective, rapid, less arduous and more proficient than the conventional chemical procedures (Willner et al., 2007; Katiyar et al., 2020). Green synthesis of nanoparticles has provided an eco-friendly alternative in agricultural applications (Kaur and Sidhu, 2021). Innovative pest-resistive, eco-friendly and intelligent nano-pesticides have emerged due to the negative impact of traditional pesticides (Dangi and Verma, 2021). Implementing NPs for nanoformulation and nanoencapsulation methods has proven adequate efficiencies in plant pathogenic organisms (e.g., Streptomyces sannanesis, Bacillus subtilis, Pseudomonas aeruginosa, Salmonella enterica, Candida albicans, and Aspergillus niger) with attenuated phytotoxicity (Keerthana et al., 2021). Nanopesticides offer sustained release kinetics, solubility efficiency, enhanced permeability, and stability (Dangi and Verma, 2021). Additionally, this technology has also been implemented as a nanoscale growth regulator in agriculture, improving the yield of many crops (e.g., tomato, rice, cucumber, lettuce, corn, and wheat) (Pelegrino et al., 2020; Tovar et al., 2020; Wahid et al., 2020; Rahman et al., 2021; Al-Karaawi and Al-Juthery, 2022; Ghani et al., 2022).
Table 1 Recent examples of the implementation of bacteria, fungi and plant extracts for the synthesis of NPs and NMs.
Crop breeding has focused on two main strategies: natural selection and genetic modification, where the latter consists of the insertion of the desired DNA into the plant genome (Godbey, 2022); however, although this technique is considered a reasonably successful technique, it presents several challenges such as cell toxicity and penetration power of foreign DNA, both at the cell wall level and in the genome of the target cell (Krishna et al., 2018). In this regard, nanotechnology provides new tools to enhance gene modification (Singh et al., 2021). In their review (Sheikh Mohamed and Sakthi Kumar, 2019), indicate that a wide range of NPs can be used for the transport and carriage of DNA, RNA, and proteins into the host genome, ensuring successful and correct transgene incorporation. Additionally (Jat et al., 2020), observed that NPs exerted direct and specific penetration into plant sites and organelles such as protoplasts, chloroplasts, pollens, and leaves under transgenic and non-transgenic techniques (Kwak et al., 2019). reported an effective chloroplast translocation using chitosan-functionalized carbon nanotubes, improving plasmid DNA transfer efficiency in chloroplasts. Although their effectiveness and efficacy have been demonstrated, the use of NPs as carriers of DNA, RNA, and proteins is still under discussion and study since the design, toxicity, and effectiveness depend on the type, size, dosage, exposure time, and crop.
Plant growth and development, germination, and production of primary and secondary metabolites are regulated by compounds of chemical nature that are present endogenously in the plant or are obtained from the metabolism of microorganisms or by laboratory synthesis (Bisht et al., 2018). Phytohormones and growth regulators can act punctually in germination, photosynthesis, fruit filling, antioxidant enzyme system, and biotic and abiotic stress alleviation. Studies have demonstrated that NPs and NMs can induce the production of these compounds, enhancing the biological and biochemical processes of plants. For example (Siegel et al., 2018), observed that AuNPs up-regulated the gene expression related to oxidative and metallic stress response in Arabidopsis thaliana but down-regulated the gene expression related to pathogen response and exerted various hormonal stimuli such as ethylene signaling and auxin response for tissue growth (Manickavasagam et al., 2019). showed the hormetic influence of biosynthesized Ag NPs on the expression of plant growth regulator genes of regenerating rice calli, finding that he transcript levels of ethylene, ABA, auxin, cytokinin and gibberellic acid responsive genes decreased by a maximum of 0.3, 0.35, 0.2, 0.3 and 0.4 fold, respectively, after culture in medium with 10 mg L−1, but above this concentration the expression of all the genes markedly increased, demonstrating that the dose-effect curve is not always linear. Additionally (Pociecha et al., 2021), indicated that the application of two different AgNPs suspensions differentially affected the phytohormone balance in wheat, where after three weeks, the proportions of cytokinins (29%, 42%) and gibberellins (63%, 55%), respectively, were reversed compared to the control.
Table 2 shows more examples of the application of NPs and NMs that impacted the production of plant growth regulators. Finally, as phytohormones regulates different plant processes, novel research has proposed the implementation of these compounds to increase the efficiency in the process of NPs biosynthesis. For example (Mohamed et al., 2022), found that the addition of indole acetic acid and kinetin to the cyanobacterium Cyanothece sp provoked a maximum conversion (87.29% and 55.16%, respectively) for the production of Ag NPs, and that methyl jasmonate increased the NPs conversion rate to 90.29%, although nearly all the cyanobacterial cultures died at the end. Although the effect of various nanoparticles on plant growth and development has been extensively investigated, there are still many questions about their action. For example, in seed priming (Chandrasekaran et al., 2020) establishes that it needs to be first cleared whether IAA have a role in nanoparticle internalization and transport across tissues in primed seeds, that the regulation of carriers involved in transporting nanoparticles from seed coat to endosperm and then to embryonic tissues has not been characterized, whether in vivo seed mineral content have a role during internalization of various mineral based nanoparticles, how seeds recognize nanoparticles as external agents, and a detailed description of crosstalk between reactive oxygen species (ROS) and growth regulators due to NPs addition.
Plants are sessile organisms exposed to constant environmental changes, nutrient competition, pests, diseases, water changes, and variations in solar radiation (Vermeulen et al., 2012; Aguirre-Becerra et al., 2021). To counteract these phenomena, plants have developed defense systems consisting of diverse chemical and physical reactions to synthesize compounds that contribute to the response to these stimuli (Alvarado et al., 2019; Aguirre-Becerra et al., 2021). The medicinal value of these compounds, called secondary metabolites, has called for the need for their enhanced production in plants, mainly through plant cell and organ cultures and by providing precursors and elicitors to alter the environmental conditions causing eustress (Khan et al., 2019; Khan et al., 2019). In this sense, according to the hormesis curve of each plant model, the stress can be divided into distress (bad stress that leads to damage and ultimately plant death) or eustress (good stress that leads to activation of secondary metabolism); therefore, the objective of elicitation will be to cause eustress by searching the adequate doses, exposure times, type of application (foliar or irrigation), and type of elicitor (Aguirre-Becerra et al., 2021).
Agriculture faces challenges due to biotic and abiotic stresses where nanotechnology can contribute with promising applications (Usman et al., 2020). In this context, several studies report that the application of NPs positively affects crop productivity through different strategies such as favorable DNA carriers or carriers that improve genetic traits and seeds, plant targeted protection through the application of nanoherbicides and nanofungicides, precise and constant supply of nutrients through nanofertilizers, and tolerance to abiotic stress (e.g., low or high temperatures, drought, salinity, low or high light intensities, UV-B) (Usman et al., 2020; Predoi et al., 2020; Ali et al., 2021). NPs can also act as gene expression modulators in response to abiotic stress conditions (e.g., such as drought, salinity, low temperatures, UV rays, and HMs), providing plant protection by several mechanisms such as activation of the antioxidant enzyme system that alleviates oxidative stress, intervening in biosynthesis and cellular organization, as well as in electron and energy transport (Vishwakarma et al., 2018; Faraji and Sepehri, 2020; Mittal et al., 2020; Sarraf et al., 2022). Examples of the interaction of NPs with different types of abiotic stresses are presented in Table 3 and the description of the most revised types of abiotic stress are next described.
The incidence and extent of drought are predicted to increase, creating considerable pressure on global agricultural yields (Seleiman et al., 2021). This type of abiotic stress has the strongest effect on soil biota and plants, generating osmotic stress and reducing nutrient access to plant roots (Zia et al., 2021). Furthermore, soil salinity is the most common abiotic stress with a significant impact on crop production and development, causing massive economic damage in 1,125 million hectares, with 1.5 million hectares of arable land lost each year due to salinization and sodification (Abou-Zeid and Ismail, 2018; Taha R et al., 2020; Alabdallah and Hasan, 2021; Alharbi et al., 2021). Salt stress disrupts the plasma membrane integrity, reduces photosynthetic efficiency, decreases stomata aperture and accessibility of antioxidant enzymes, generating excessive amount of ROS, resulting in cellular oxidative-burst and affecting proteins, DNA, and lipids (Muchate et al., 2016; Tanveer and Ahmed, 2020; Seleiman et al., 2020). Ionic stress is caused by a high accumulation of sodium (Na+) and chloride (Cl−) ions in plants, leading to disturbance in distribution, uptake, and availability of macro and microelements, and integrity and selectivity of cellular membranes (Thor, 2019). To handle this stress, plants have adaptation mechanisms such as salt ion exclusion, changes in membrane-permeability, synthesis and accumulation of compatible metabolites or osmolytes such as proline, promoting ionic homeostasis, and hormonal regulation such as ABA (Banik and Bhattacharjee, 2020; Van Zelm et al., 2020) that contributes in conserving cellular water by initiating a cascade signaling pathway that leads to efflux of calcium ions (Ca2+), NO3− ions, and potassium ions (K+) which results in stomatal closure (Medeiros et al., 2019). Positive effects of NPs on plants under drought and salinity stress have been demonstrated by changing the phytohormone concentrations (An et al., 2020), gene expression (Linh et al., 2020), secondary metabolites production (Etesami et al., 2021), increasing nutrient concentration and availability (Mahmoud et al., 2020), decreasing plasma membrane damage and chlorophyll degradation (Karimi et al., 2020), enhancing K+ uptake, K+/Na+ ratio (Abdoli et al., 2020), and activating the antioxidant enzyme activity (Faizan et al., 2018; Moradbeygi et al., 2020; Alabdallah and Alzahrani, 2020). In this way, NPs mitigate drought-induced ROS through the aggregation of osmolytes, which results in enhanced osmotic adaptation and crop water balance (Alabdallah et al., 2021). Furthermore, NPs increase the photosynthetic activity, upregulate aquaporins, modify the intracellular water metabolism, accumulates compatible solutes, maintain intracellular ion homeostasis, increase stomatal density, and reduce water loss from leaves through stomatal closure due to fostered ABA accumulation (Attaran Dowom et al., 2022; Kandhol et al., 2022).
Environmental temperature is rising due to greenhouse-gas emissions in the atmosphere, where the average global temperature has continuously increased and is predicted to rise by 2°C until 2100 (Raftery et al., 2017; Malhi et al., 2021), causing substantial agricultural production losses worldwide. The major proportion of greenhouse gases has several effects on crops (Mukhtar et al., 2020). A major concentration of CO2 is related to increased photosynthesis (i.e., higher growth and plant productivity), however, an increased temperature counteracts this effect by increasing evapotranspiration and crop respiration rate, promoting pest infestation and weed flora, reducing crop duration, and damaging the microbial population and enzymatic activities of the soil (Malhi et al., 2021; Battaglia et al., 2022). Plants induce morphological and biochemical changes to survive to heat stress that leads to oxidative stress caused by an overproduction of ROS, severely affecting growth, development, and yield (Hasanuzzaman et al., 2013). During this type of abiotic stress, plants induce signaling processes to produce osmolytes that sustain cell turgidity by osmotic adjustment and other secondary metabolites to modify the antioxidant system (Arif et al., 2020). High temperatures have detrimental effects on the photosynthetic functions by damaging the oxygen-evolving complex, PSII cofactors, carbon assimilation, and the ATP generation (Allakhverdiev et al., 2008). Additionally, in low-temperature stress, freezing injury and fluidity reduction occurs in cell membranes (Los and Murata, 2004). Positive effects of NPs on plants under temperature stress have been demonstrated by enhancing the photophosphorylation, oxygen evolution, and splitting of water CP43 protein (Pradhan et al., 2013), nitrogen metabolism (Pradhan et al., 2014), photosynthetic capacity (Younis et al., 2020), antioxidant enzymes activity, decreasing lipid peroxidation (Hassan et al., 2018), and restoration of ultrastructural distortions of chloroplasts and the nucleus (Younis et al., 2020).
The accumulation of toxic HMs and metalloids (e.g., As, Pb, Cd, Cu, Cr, Mn, Hg, Ni, Se, Sb, and Zn) in soil is a severe environmental issue that causes detrimental effects on every living organism (Seleiman and Kheir, 2018; Seleiman et al., 2020). Human activities (e.g., industrial and municipal discharge, mining, smelting, hazardous solid waste disposal, extensive use of agrochemicals) mainly contribute to the accumulation of these compounds (Seleiman et al., 2012; Ali et al., 2019; Shah and Daverey, 2020; Seleiman et al., 2021). Persisting HMs in the soil can absorbed by crops that reaches human consumption, causing cancer (due to DNA damage), vascular disease, dermal disease, gastrointestinal problems, respiratory damage, brain damage, renal disorder, degenerative bone disease, liver damage, depression, mental retardation, cardiovascular and respiratory system disorders, etc. (Ye et al., 2017; Ali et al., 2019; Shah and Daverey, 2020). HMs persist in the soil for many years due to its non-biodegradable nature, and their mobility and availability is controlled by biogeochemical process (i.e., mineralization, precipitation, adsorption, and protonation), the type of soil, and rhizosphere which contains a diverse root microbiome that contributes in soil fertility and is highly affected by these compounds (He et al., 2015; Shah and Daverey, 2020). Several mechanisms have been proposed to counteract the negative effect of this abiotic stress. The immobilization of metal ions by absorption, oxidation, or chemical reduction processes is another aspect to highlight in NPs used for soil remediation in several countries (Shen et al., 2019; Qian et al., 2020; Usman et al., 2020). In plants, the formation of apoplastic barriers, which control the flow of water, ions and oxygen, can be influenced by NPs, decreasing the amount of HMs in the roots (Yang et al., 2013); metal transport genes can be regulated by specific NPs, enhancing the plant’s extracellular barrier to intercept HM (Zhou et al., 2020); organic acids accumulated in the cell walls of roots and leaves can be chelated with HM to reduce the damage of HM stress to plants; and finally the activation of the oxidation defense system influenced by NPs can also alleviate HM stress (Marslin et al., 2017).
Light from sunlight, and recently from artificial sources, is not only essential for plant growth and development through photosynthesis but also in gene expression, production of natural bioactive compounds, and synchronization of the circadian clock (Aguirre-Becerra et al., 2020; Aguirre-Becerra et al., 2021; Xu et al., 2022). Light intensity, direction, quality (wavelength), and photoperiod are sensed by specialized designed proteins that sense light named photoreceptors, triggering chain reactions that have been studied in terms of photomorphogenesis and primary and secondary metabolites production (Alvarado et al., 2019). Five types of photoreceptors have been identified: phytochromes perceiving red (660–700 nm) and far-red (700–750 nm), cryptochromes, phototropins, and members of the Zeitlupe family perceiving blue (495–400 nm) and UV-A (400–315 nm), and UV Resistance Locus 8 (UVR8) sensing (315–280 nm) (Bantis et al., 2018; Alvarado et al., 2019; Aguirre-Becerra et al., 2021). Insufficient or excess light levels can result in plant stress, producing adverse effects that trigger the defense mechanisms for secondary metabolite production (Akula and Ravishankar, 2011; Müller-Xing et al., 2014). Additionally, the impact of UV radiation on organisms has focused the scientific community’s interest due to the depletion of the stratospheric ozone layer and more UV-B, very harmful for most organism, could reach the Earth biosphere (Bernhard et al., 2020).
Photoactive NPs (e.g., cadmium sulfide, gold nanoclusters, and indium phosphide nanoparticles) have been used to construct functional biological−inorganic hybrid systems for improving light absorption, typically transferring light into photogenerated electrons for regulating CO2 fixation and NADPH generation (Li et al., 2020). Moreover, luminescent NPs (e.g., carbon dots, silicon nanoparticles, and nanophosphors) have been involved in plant research to improve photosynthesis efficiency due to their tunable photoluminescence, biocompatibility, low cytotoxicity, and chemical/physical stability (Dong et al., 2019). However, higher concentrations of NPs can show adverse effects, damage the photosynthetic apparatus, inhibit photosynthetic electron transport or CO2 reduction by suppressing Rubisco activity and production of harmful ROS (Jampílek and Kráľová, 2019); therefore, correct doses for achieving enhanced photosynthesis are still under research. Additionally, most experiments evaluate the effect of NPs on photosynthesis due to stress (e.g., temperature, drought, trace metals) conditions or to enhance this biological process under normal light conditions. This measurement is related to the efficiency of light energy conversion; however, just a few experiments were found where light is the stress condition (i.e., low or high light intensities, harmful wavelengths, or not adequate photoperiods). For example, hydroponic wheat (Triticum aestivum) seedlings were exposed to UV-B and treated with SiNPs. These compounds alleviated the toxic effects as fresh mass, leaf area, leaf area, leaf fresh and dry mass increased, and lipid peroxidation (as MDA) decreased. UV-B significantly reduced the total chlorophyll, and SiNPs restricted such reductions. Additionally, the intensity and numbers of formazan spots were reduced in the leaves, suggesting a protective role against UV-B-induced generation of H2O2 (i.e., oxidative stress). Furthermore, internal injuries of leaves related to the distorted palisade and mesophyll layers were reduced; therefore, the reduction in plant leaf thickness was alleviated (Tripathi et al., 2017). Phytotoxicity considerations
The type, size, morphology, crystallinity, concentration, electric-magnetic-optical properties, type of application (i.e., foliar or substrate), and vegetal species determine the phytotoxicity of NPSs. The accumulation of these compounds can lead to an imbalance in the interaction of soil, plant, and microbial communities resulting in phytotoxicity and plant death. NPs mainly accumulate in the soil due to atmospheric deposition, rain erosion, agricultural application, and surface runoff, facilitating their transfer into the plant and moving to different organs (Agrawal et al., 2022). Due to the characteristics of NPs (i.e., size, morphology, and surface charge), they can easily penetrate the vegetal tissues and the human body with possible adverse effects (Ndaba et al., 2022). Risks associated with NPs should be assessed along with their benefits. Most of the mentioned studies evaluated the advantages of using these compounds, but only a few consider the adverse effects and negative downstream implications.
Once NPs move into the plant, mainly by absorption, different chemical elements, and heavy metals could be dissolved, released, and even bio-transformed, generating potentially toxic compounds for the environment (Li and Yan, 2020). As previously discussed, nanotechnology applications in agriculture are potentially beneficial; nevertheless, the diverse nature and characteristics of NPs generate a challenging and not completely understood interaction with living organisms, which can potentially damage the food chain. Additionally, NPs can harm plants by clogging pores and blocking the apoplastic movement causing decreased nutrient absorption, photosynthesis, and hydraulic transfer, DNA damage, and ROS production (Ganie et al., 2021). Plants exposed to NPs have also presented altered physiology (e.g., root and shoot elongation), germination percentage, leaf number, malfunction of cell organelles, tissue damage, structural modification of enzymes, protein denaturation, destruction of cell membranes, and non-beneficial responses to biotic and abiotic stresses (Agrawal et al., 2022). These effects are schematically presented in Figure 2.
Knowing how NPs affect plants, humans, and the environment helps generate safe protocols to reduce their adverse effects and create “safer” NPs through the green or biosynthesis approach. Further studies on genetic alterations are needed to clarify toxic effects and the plant-triggered adaptation mechanisms to propose specific doses according to the particular plant and NP to potentiate the beneficial responses (eustress) in the plant physiology and defense mechanisms (secondary metabolism) (Aguirre-Becerra et al., 2021; Ranjan et al., 2021).
In this manuscript, a general overview was performed on the effect of nanomaterials on the plant resistance to abiotic stress. The implementation of NMs and NPs is one of the main proposed strategies for substituting conventional fertilizers, herbicides, and pesticides in agriculture and enhancing the effect of growth regulators and the defense system of plants. This relatively new technology generates diverse effects depending on the crop, NM, concentration, and type and frequency of application. The particular effect of each NP depends on the size, surface area, thermal stability, crystallinity, concentration, electric-magnetic-optical properties, and origin, where the biosynthesis through the implementation of bacteria, fungi, and plant extracts has resulted in greater effectiveness in plant resistance to abiotic stress and lower phytotoxicity. Additionally, the nutrient diversity, controlled release, and rapid absorption make NPs a promising tool for the agri-food sector, generating enhanced growth and crop yield and accelerating the germination processes.
Different studies indicate that the interaction NP-plant induces reactions that help the plant cope with adverse environmental factors. Among these reactions, higher plant plasticity, generation of secondary metabolites, enhancement of the immune system of plants, and acceleration of plant growth and development, make plants more resilient. Most reactions are favorable for the adaptability of crops to stress situations; however, some reactions are not desirable, and there is little information about them. How NPs and NMs could affect not only the crops but also the environment: substrates, water resources, substrate microorganisms, fauna, flora, and the short, medium, and long-term effect of the consumption of these crops on humans.
Another aspect that has not been completely understood or about which there is little information is the overuse that can lead to the accumulation of NPs in the natural environment. Most of the available information focuses on NPs applied under laboratory and controlled and supervised conditions, with particular and correct disposal of experimental waste material. Therefore, the interaction between NPs and open-air plants and the effect of their accumulation in natural environments is still unknown. As mentioned in this chapter, many variables must be considered when applying NPs to enhance plant resistance to abiotic stress. Many studies and research areas are still to be more involved (e.g., genetics, omics sciences) to achieve secure and effective protocols for using NPs and NMs in agriculture.
HA-B, KE, CP-G, and AF-P: data acquisition, drafting of the manuscript, and conceptualization; MV-H and AM-A: bibliographic review; AF-P, KE, and CP-G: obtained funds and responsibility for managing and coordinating the planning and execution of the research activity and revision of the manuscript. All authors assisted in the critical review of this manuscript approved the version presented for publication and agreed to be responsible for all aspects of the article.
The authors appreciate the support provided by FONDEC-UAQ 2021 FIN202115 and Autonomous University of Queretaro for the realization of this project.
The authors declare that the research was conducted in the absence of any commercial or financial relationships that could be construed as a potential conflict of interest.
All claims expressed in this article are solely those of the authors and do not necessarily represent those of their affiliated organizations, or those of the publisher, the editors and the reviewers. Any product that may be evaluated in this article, or claim that may be made by its manufacturer, is not guaranteed or endorsed by the publisher.
Abdoli, S., Ghassemi-Golezani, K., Alizadeh-Salteh, S. (2020). Responses of ajowan (Trachyspermum ammi l.) to exogenous salicylic acid and iron oxide nanoparticles under salt stress. Environ. Sci. pollut. Res. 27 (29), 36939–36953. doi: 10.1007/s11356-020-09453-1
Abou-Zeid, H., Ismail, G. (2018). The role of priming with biosynthesized silver nanoparticles in the response of triticum aestivum l to salt stress. Egypt J. Bot. 58 (1), 73–85. doi: 10.21608/EJBO.2017.1873.1128
Agrawal, S., Kumar, V., Kumar, S., Shahi, S. K. (2022). Plant development and crop protection using phytonanotechnology: A new window for sustainable agriculture. Chemosphere 134465. doi: 10.1016/j.chemosphere.2022.134465
Aguirre-Becerra, H., García-Trejo, J. F., Vázquez-Hernández, C., Alvarado, A. M., Feregrino-Pérez, A. A., Contreras-Medina, L. M., et al. (2020). Effect of extended photoperiod with a fixed mixture of light wavelengths on tomato seedlings. HortScience 1 (aop), 1–8. doi: 10.21273/HORTSCI15342-20
Aguirre-Becerra, H., Vazquez-Hernandez, M. C., Alvarado-Mariana, A., Guevara-Gonzalez, R. G., Garcia-Trejo, J. F., Feregrino-Perez, A. A. (2021). “Role of stress and defense in plant secondary metabolites production,” in Bioactive natural products for pharmaceutical applications (Switzerland: Springer Nature), 151–195.
Ahmed, M., Qadeer, U., Ahmed, Z. I., Hassan, F. (2016). Improvement of wheat (Triticum aestivum) drought tolerance by seed priming with silicon. Arch. Agron. Soil Sci. 62 (3), 299–315. doi: 10.1080/03650340.2015.1048235
Akula, R., Ravishankar, G. A. (2011). Influence of abiotic stress signals on secondary metabolites in plants. Plant Signal Behav. 6 (11), 1720–1731. doi: 10.4161/psb.6.11.17613
Alabdallah, N. M., Alzahrani, H. S. (2020). The potential mitigation effect of ZnO nanoparticles on [Abelmoschus esculentus l Moench] metabolism under salt stress conditions. Saudi J. Biol. Sci. 27 (11), 3132–3137. doi: 10.1016/j.sjbs.2020.08.005
Alabdallah, N. M., Hasan, M. M. (2021). Plant-based green synthesis of silver nanoparticles and its effective role in abiotic stress tolerance in crop plants. Saudi J. Biol. Sci. 28 (10), 5631–5639. doi: 10.1016/j.sjbs.2021.05.081
Alabdallah, N. M., Hasan, M. M., Hammami, I., Alghamdi, A. I., Alshehri, D., Alatawi, H. A. (2021). Green synthesized metal oxide nanoparticles mediate growth regulation and physiology of crop plants under drought stress. Plants 10 (8), 1730. doi: 10.3390/plants10081730
Alharbi, B. M., Elhakem, A. H., Alnusairi, G. S. H., Soliman, M. H., Hakeem, K. R., Hasan, M. M., et al. (2021). Exogenous application of melatonin alleviates salt stress-induced decline in growth and photosynthesis in glycine max (L.) seedlings by improving mineral uptake, antioxidant and glyoxalase system. Plant Soil Environ. 67 (4), 208–220. doi: 10.17221/659/2020-PSE
Ali, S. S., Al-Tohamy, R., Koutra, E., Moawad, M. S., Kornaros, M., Mustafa, A. M., et al. (2021). Nanobiotechnological advancements in agriculture and food industry: Applications, nanotoxicity, and future perspectives. Sci. Total Environ. 792, 148359. doi: 10.1016/j.scitotenv.2021.148359
Ali, S. S., Darwesh, O. M., Kornaros, M., Al-Tohamy, R., Manni, A., El-Shanshoury, AE-RR, et al. (2021). “Nano-biofertilizers: Synthesis, advantages, and applications,” in Biofertilizers (ELSEVIER – Science Direct), 359–370.
Ali, H., Khan, E., Ilahi, I. (2019). Environmental chemistry and ecotoxicology of hazardous heavy metals: environmental persistence, toxicity, and bioaccumulation. J. Chem. 2019. doi: 10.1155/2019/6730305
Ali, S. S., Kornaros, M., Manni, A., Al-Tohamy, R., El-Shanshoury, AE-RR, Matter, I. M., et al. (2021). “Advances in microorganisms-based biofertilizers: Major mechanisms and applications,” in Biofertilizers (Elsevier), 371–385.
Al-Karaawi, M. D. K., Al-Juthery, H. W. A. (2022). “Effect of NPK, NPK organic fertilizers and spraying nano-vanadium and nano-selenium on the growth and yield of rice,” in IOP Conference Series: Earth and Environmental Science, Vol. p. 12035 (Bristol, England: IOP Publishing).
Allakhverdiev, S. I., Kreslavski, V. D., Klimov, V. V., Los, D. A., Carpentier, R., Mohanty, P. (2008). Heat stress: an overview of molecular responses in photosynthesis. Photosynth. Res. 98 (1), 541–550. doi: 10.1007/s11120-008-9331-0
Alvarado, A. M., Aguirre-Becerra, H., Vázquez-Hernández, M. C., Magaña-Lopez, E., Parola-Contreras, I., Caicedo-Lopez, L. H., et al. (2019). “Influence of elicitors and eustressors on the production of plant secondary metabolites,” in Natural bio-active compounds (Switzerland: Springer Nature), 333–388.
An, J., Hu, P., Li, F., Wu, H., Shen, Y., White, J. C., et al. (2020). Emerging investigator series: molecular mechanisms of plant salinity stress tolerance improvement by seed priming with cerium oxide nanoparticles. Environ. Sci. Nano. 7 (8), 2214–2228. doi: 10.1039/D0EN00387E
Arif, Y., Singh, P., Siddiqui, H., Bajguz, A., Hayat, S. (2020). Salinity induced physiological and biochemical changes in plants: An omic approach towards salt stress tolerance. Plant Physiol. Biochem. 156, 64–77. doi: 10.1016/j.plaphy.2020.08.042
Attaran Dowom, S., Karimian, Z., Mostafaei Dehnavi, M., Samiei, L. (2022). Chitosan nanoparticles improve physiological and biochemical responses of salvia abrotanoides (Kar.) under drought stress. BMC Plant Biol. 22 (1), 1–17. doi: 10.1186/s12870-022-03689-4
Ayub, M. A., Naeem, A., Rehman, M. Z., Farooqi, Z. U. R., Umar, W., Fatima, H., et al. (2022). Role of nanotechnology in enhancing crop production and produce quality. In Sustainable Nanotechnology for Environmental Remediation - Micro and Nano Technologies, 703–764. doi: 10.1016/B978-0-12-824547-7.00014-X
Badawy, S. A., Zayed, B. A., Bassiouni, S. M. A., Mahdi, A. H. A., Majrashi, A., Ali, E. F., et al. (2021). Influence of nano silicon and nano selenium on root characters, growth, ion selectivity, yield, and yield components of rice (Oryza sativa l.) under salinity conditions. Plants 10 (8), 1657. doi: 10.3390/plants10081657
Banik, N., Bhattacharjee, S. (2020). Complementation of ROS scavenging secondary metabolites with enzymatic antioxidant defense system augments redox-regulation property under salinity stress in rice. Physiol. Mol. Biol. Plants. 26 (8), 1623–1633. doi: 10.1007/s12298-020-00844-9
Bantis, F., Smirnakou, S., Ouzounis, T., Koukounaras, A., Ntagkas, N., Radoglou, K. (2018). Current status and recent achievements in the field of horticulture with the use of light-emitting diodes (LEDs). Sci. Hortic. (Amsterdam). 235, 437–451. doi: 10.1016/j.scienta.2018.02.058
Battaglia, M. L., Thomason, W. E., Fike, J. H., Evanylo, G. K., Stewart, R. D., Gross, C. D., et al. (2022). Corn and wheat residue management effects on greenhouse gas emissions in the mid-Atlantic USA. Land 11 (6), 846. doi: 10.3390/land11060846
Bernhard, G. H., Neale, R. E., Barnes, P. W., Neale, P. J., Zepp, R. G., Wilson, S. R., et al. (2020). Environmental effects of stratospheric ozone depletion, UV radiation and interactions with climate change: UNEP environmental effects assessment panel, update 2019. Photochem. Photobiol. Sci. 19 (5), 542–584. doi: 10.1039/D0PP90011G
Bisht, T. S., Rawat, L., Chakraborty, B., Yadav, V. (2018). A recent advances in use of plant growth regulators (PGRs) in fruit crops-a review. doi: 10.20546/ijcmas.2018.705.159
Chandrasekaran, U., Luo, X., Wang, Q., Shu, K. (2020). Are there unidentified factors involved in the germination of nanoprimed seeds? Front. Plant Sci. 11, 832. doi: 10.3389/fpls.2020.00832
Chau, T. P., Veeraragavan, G. R., Narayanan, M., Chinnathambi, A., Alharbi, S. A., Subramani, B., et al. (2022). Green synthesis of zirconium nanoparticles using punica granatum (pomegranate) peel extract and their antimicrobial and antioxidant potency. Environ. Res. 209, 112771. doi: 10.1016/j.envres.2022.112771
Dahoumane, S. A., Jeffryes, C., Mechouet, M., Agathos, S. N. (2017). Biosynthesis of inorganic nanoparticles: A fresh look at the control of shape, size and composition. Bioengineering 4 (1), 14. doi: 10.3390/bioengineering4010014
Dangi, K., Verma, A. K. (2021). Efficient & eco-friendly smart nano-pesticides: Emerging prospects for agriculture. Mater. Today Proc. 45, 3819–3824. doi: 10.1016/j.matpr.2021.03.211
Dong, R., Li, Y., Li, W., Zhang, H., Liu, Y., Ma, L., et al. (2019). Recent developments in luminescent nanoparticles for plant imaging and photosynthesis. J. Rare Earths. 37 (9), 903–915. doi: 10.1016/j.jre.2019.04.001
El-Naggar, M. E., Hasanin, M., Hashem, A. H. (2022). Eco-friendly synthesis of superhydrophobic antimicrobial film based on cellulose acetate/polycaprolactone loaded with the green biosynthesized copper nanoparticles for food packaging application. J. Polym Environ. 30 (5), 1820–1832. doi: 10.1007/s10924-021-02318-9
Elshayb, O. M., Nada, A. M., Farroh, K. Y., AL-Huqail, A. A., Aljabri, M., Binothman, N., et al. (2022). Utilizing urea–chitosan nanohybrid for minimizing synthetic urea application and maximizing oryza sativa l. productivity and n uptake. Agriculture 12 (7), 944. doi: 10.3390/agriculture12070944
Eltaweil, A. S., Fawzy, M., Hosny, M., Abd El-Monaem, E. M., Tamer, T. M., Omer, A. M. (2022). Green synthesis of platinum nanoparticles using atriplex halimus leaves for potential antimicrobial, antioxidant, and catalytic applications. Arab J. Chem. 15 (1), 103517. doi: 10.1016/j.arabjc.2021.103517
Etesami, H., Fatemi, H., Rizwan, M. (2021). Interactions of nanoparticles and salinity stress at physiological, biochemical and molecular levels in plants: A review. Ecotoxicol. Environ. Saf. 225, 112769. doi: 10.1016/j.ecoenv.2021.112769
Faizan, M., Faraz, A., Yusuf, M., Khan, S. T., Hayat, S. (2018). Zinc oxide nanoparticle-mediated changes in photosynthetic efficiency and antioxidant system of tomato plants. Photosynthetica 56 (2), 678–686. doi: 10.1007/s11099-017-0717-0
Fakharzadeh, S., Hafizi, M., Baghaei, M. A., Etesami, M., Khayamzadeh, M., Kalanaky, S., et al. (2020). Using nanochelating technology for biofortification and yield increase in rice. Sci. Rep. 10 (1), 1–9. doi: 10.1038/s41598-020-60189-x
Faraji, J., Sepehri, A. (2020). Exogenous nitric oxide improves the protective effects of TiO2 nanoparticles on growth, antioxidant system, and photosynthetic performance of wheat seedlings under drought stress. J. Soil Sci. Plant Nutr. 20 (2), 703–714. doi: 10.1007/s42729-019-00158-0
Farokhzad, O. C., Langer, R. (2009). Impact of nanotechnology on drug delivery. ACS Nano. 3 (1), 16–20. doi: 10.1021/nn900002m
Ganie, A. S., Bano, S., Khan, N., Sultana, S., Rehman, Z., Rahman, M. M., et al. (2021). Nanoremediation technologies for sustainable remediation of contaminated environments: Recent advances and challenges. Chemosphere 275, 130065. doi: 10.1016/j.chemosphere.2021.130065
Ghani, M. I., Saleem, S., Rather, S. A., Rehmani, M. S., Alamri, S., Rajput, V. D., et al. (2022). Foliar application of zinc oxide nanoparticles: An effective strategy to mitigate drought stress in cucumber seedling by modulating antioxidant defense system and osmolytes accumulation. Chemosphere 289, 133202. doi: 10.1016/j.chemosphere.2021.133202
Gil-Díaz, M., García-Gonzalo, P., Mancho, C., Hernández, L. E., Alonso, J., Lobo, M. C. (2022). Response of spinach plants to different doses of two commercial nanofertilizers. Sci. Hortic. (Amsterdam). 301, 111143. doi: 10.1016/j.scienta.2022.111143
Godbey, W. T. (2022). “Chapter 18 - transgenics and genetically modified organisms in agriculture,” in Biotechnology and its Applications (Second Edition). (Elsevier), 411–428.
Guan, Z., Ying, S., Ofoegbu, P. C., Clubb, P., Rico, C., He, F., et al. (2022). Green synthesis of nanoparticles: Current developments and limitations. Environ. Technol. Innov. 26, 102336. doi: 10.1016/j.eti.2022.102336
Gudikandula, K., Charya Maringanti, S. (2016). Synthesis of silver nanoparticles by chemical and biological methods and their antimicrobial properties. J. Exp. Nanosci. 11 (9), 714–721. doi: 10.1080/17458080.2016.1139196
Guo, K.-R., Adeel, M., Hu, F., Xiao, Z.-Z., Wang, K.-X., Hao, Y., et al. (2021). Absorption of carbon-13 labelled fullerene (C60) on rice seedlings and effect of phytohormones on growth. J. Nanosci. Nanotechnol. 21 (6), 3197–3202. doi: 10.1166/jnn.2021.19307
Gur, T., Meydan, I., Seckin, H., Bekmezci, M., Sen, F. (2022). Green synthesis, characterization and bioactivity of biogenic zinc oxide nanoparticles. Environ. Res. 204, 111897. doi: 10.1016/j.envres.2021.111897
Hasanuzzaman, M., Nahar, K., Fujita, M. (2013). Extreme temperature responses, oxidative stress and antioxidant defense in plants. Abiotic Stress responses Appl. Agric. 13, 169–205. doi: 10.5772/54833
Hassan, N. S., Salah El Din, T. A., Hendawey, M. H., Borai, I. H., Mahdi, A. A. (2018). Magnetite and zinc oxide nanoparticles alleviated heat stress in wheat plants. Curr. Nanomater. 3 (1), 32–43. doi: 10.2174/2405461503666180619160923
Hatami, M., Hadian, J., Ghorbanpour, M. (2017). Mechanisms underlying toxicity and stimulatory role of single-walled carbon nanotubes in hyoscyamus niger during drought stress simulated by polyethylene glycol. J. Hazard Mater. 324, 306–320. doi: 10.1016/j.jhazmat.2016.10.064
He, S., He, Z., Yang, X., Stoffella, P. J., Baligar, V. C. (2015). Soil biogeochemistry, plant physiology, and phytoremediation of cadmium-contaminated soils. Adv. Agron. 134, 135–225. doi: 10.1016/bs.agron.2015.06.005
Helaly, A. A. E., EL-Bauome, H. A. A. (2020). Phosphorus fertilizer level related with nano-chitosan concentration and their influence on growth, yield and chemical constituents of hot pepper. J. Plant Prod. 11 (12), 1311–1317. doi: 10.21608/jpp.2020.149802
Hussain, A., Ali, S., Rizwan, M., ur Rehman, M. Z., Qayyum, M. F., Wang, H., et al. (2019). Responses of wheat (Triticum aestivum) plants grown in a cd contaminated soil to the application of iron oxide nanoparticles. Ecotoxicol. Environ. Saf. 173, 156–164. doi: 10.1016/j.ecoenv.2019.01.118
Ijaz, I., Gilani, E., Nazir, A., Bukhari, A. (2020). Detail review on chemical, physical and green synthesis, classification, characterizations and applications of nanoparticles. Green Chem. Lett. Rev. 13 (3), 223–245. doi: 10.1080/17518253.2020.1802517
Iqbal, M., Raja, N. I., Mashwani, Z., Wattoo, F. H., Hussain, M., Ejaz, M., et al. (2019). Assessment of AgNPs exposure on physiological and biochemical changes and antioxidative defence system in wheat (Triticum aestivum l) under heat stress. IET nanobiotechnol. 13 (2), 230–236. doi: 10.1049/iet-nbt.2018.5041
Jampílek, J., Kráľová, K. (2019). Impact of nanoparticles on photosynthesizing organisms and their use in hybrid structures with some components of photosynthetic apparatus. In: Plant Nanobionics. Springer;, 255–332. doi: 10.1007/978-3-030-12496-0_11
Jat, S. K., Bhattacharya, J., Sharma, M. K. (2020). Nanomaterial based gene delivery: a promising method for plant genome engineering. J. Mater. Chem. B. 8 (19), 4165–4175. doi: 10.1039/D0TB00217H
Jiang, F., Shen, Y., Ma, C., Zhang, X., Cao, W., Rui, Y. (2017). Effects of TiO2 nanoparticles on wheat (Triticum aestivum l.) seedlings cultivated under super-elevated and normal CO2 conditions. PloS One 12 (5), e0178088. doi: 10.1371/journal.pone.0178088
Johnson, M. S., Sajeev, S., Nair, R. S. (2021). “Role of nanosensors in agriculture,” in 2021 International Conference on Computational Intelligence and Knowledge Economy (ICCIKE). 58–63 (IEEE). doi: 10.1109/ICCIKE51210.2021
Kah, M., Tufenkji, N., White, J. C. (2019). Nano-enabled strategies to enhance crop nutrition and protection. Nat. Nanotechnol. 14 (6), 532–540. doi: 10.1038/s41565-019-0439-5
Kandhol, N., Jain, M., Tripathi, D. K. (2022). Nanoparticles as potential hallmarks of drought stress tolerance in plants. Physiol. Plant 174 (2), e13665. doi: 10.1111/ppl.13665
Karimi, S., Tavallali, V., Ferguson, L., Mirzaei, S. (2020). Developing a nano-fe complex to supply iron and improve salinity tolerance of pistachio under calcium bicarbonate stress. Commun. Soil Sci. Plant Anal. 51 (14), 1835–1851. doi: 10.1080/00103624.2020.1798985
Karthik, K. V., Raghu, A. V., Reddy, K. R., Ravishankar, R., Sangeeta, M., Shetti, N. P., et al. (2022). Green synthesis of Cu-doped ZnO nanoparticles and its application for the photocatalytic degradation of hazardous organic pollutants. Chemosphere 287, 132081. doi: 10.1016/j.chemosphere.2021.132081
Katiyar, P., Yadu, B., Korram, J., Satnami, M. L., Kumar, M., Keshavkant, S. (2020). Titanium nanoparticles attenuates arsenic toxicity by up-regulating expressions of defensive genes in vigna radiata l. J. Environ. Sci. 92, 18–27. doi: 10.1016/j.jes.2020.02.013
Kaur, K., Sidhu, A. K. (2021). Green synthesis: An eco-friendly route for the synthesis of iron oxide nanoparticles. Front. Nanotechnol. 3, 655062. doi: 10.3389/fnano.2021.655062
Keerthana, P., Vijayakumar, S., Vidhya, E., Punitha, V. N., Nilavukkarasi, M., Praseetha, P. K. (2021). Biogenesis of ZnO nanoparticles for revolutionizing agriculture: A step towards anti-infection and growth promotion in plants. Ind. Crops Prod. 170, 113762. doi: 10.1016/j.indcrop.2021.113762
Khan, N., Bano, A. (2016). Role of plant growth promoting rhizobacteria and Ag-nano particle in the bioremediation of heavy metals and maize growth under municipal wastewater irrigation. Int. J. Phytoremediation. 18 (3), 211–221. doi: 10.1080/15226514.2015.1064352
Khan, K. D., Hanif, U., Liaqat, I., Shaheen, S., Awan, U. F., Ishtiaq, S., et al. (2022). Application of green silver nanoparticles synthesized from the red seaweeds halymenia porphyriformis and solieria robusta against oral pathogenic bacteria by using microscopic technique. Front. Biosci. 14 (2), 13. doi: 10.31083/j.fbe1402013
Khan, S., Hossain, M. K. (2022). “Classification and properties of nanoparticles,” in Nanoparticle-based polymer composites (Elsevier), 15–54.
Khan, T., Khan, T., Hano, C., Abbasi, B. H. (2019). Effects of chitosan and salicylic acid on the production of pharmacologically attractive secondary metabolites in callus cultures of fagonia indica. Ind. Crops Prod. 129, 525–535. doi: 10.1016/j.indcrop.2018.12.048
Khan, M. A., Khan, T., Riaz, M. S., Ullah, N., Ali, H., Nadhman, A. (2019). “Plant cell nanomaterials interaction: Growth, physiology and secondary metabolism,” in Comprehensive analytical chemistry (Elsevier), 23–54.
Khan, M. R., Rizvi, T. F. (2014). Nanotechnology: scope and application in plant disease management. Plant Pathol. J. 13 (3), 214–231. doi: 10.3923/ppj.2014.214.231
Krishna, V. D., Wu, K., Su, D., Cheeran, M. C. J., Wang, J.-P., Perez, A. (2018). Nanotechnology: Review of concepts and potential application of sensing platforms in food safety. Food Microbiol. 75, 47–54. doi: 10.1016/j.fm.2018.01.025
Kumar, S. A., Jarvin, M., Inbanathan, S. S. R., Umar, A., Lalla, N. P., Dzade, N. Y., et al. (2022). Facile green synthesis of magnesium oxide nanoparticles using tea (Camellia sinensis) extract for efficient photocatalytic degradation of methylene blue dye. Environ. Technol. Innov. 28, 102746. doi: 10.1016/j.eti.2022.102746
Kumar, R. V., Vinoth, S., Baskar, V., Arun, M., Gurusaravanan, P. (2022). Synthesis of zinc oxide nanoparticles mediated by dictyota dichotoma endophytic fungi and its photocatalytic degradation of fast green dye and antibacterial applications. South Afr. J. Bot. doi: 10.1016/j.sajb.2022.03.016
Kwak, S.-Y., Lew, T. T. S., Sweeney, C. J., Koman, V. B., Wong, M. H., Bohmert-Tatarev, K., et al. (2019). Chloroplast-selective gene delivery and expression in planta using chitosan-complexed single-walled carbon nanotube carriers. Nat. Nanotechnol. 14 (5), 447–455. doi: 10.1038/s41565-019-0375-4
Linh, T. M., Mai, N. C., Hoe, P. T., Lien, L. Q., Ban, N. K., Hien, L. T. T., et al. (2020). Metal-based nanoparticles enhance drought tolerance in soybean. J. Nanomater. 2020, 13. doi: 10.1155/2020/4056563
Liscano, J. F., Wilson, C. E., Norman-Jr, R. J., Slaton, N. A. (2000). Zinc availability to rice from seven granular fertilizers Vol. 963 (CA, USA: Arkansas Agricultural Experiment Station Fayetteville).
Li, X., Sun, H., Mao, X., Lao, Y., Chen, F. (2020). Enhanced photosynthesis of carotenoids in microalgae driven by light-harvesting gold nanoparticles. ACS Sustain Chem. Eng. 8 (20), 7600–7608. doi: 10.1021/acssuschemeng.0c00315
Liu, J., Li, G., Chen, L., Gu, J., Wu, H., Li, Z. (2021). Cerium oxide nanoparticles improve cotton salt tolerance by enabling better ability to maintain cytosolic K+/Na+ ratio. J. Nanobiotechnol. 19 (1), 1–16. doi: 10.1186/s12951-021-00892-7
Li, C., Yan, B. (2020). Opportunities and challenges of phyto-nanotechnology. Environ. Sci. Nano. 7 (10), 2863–2874. doi: 10.1039/D0EN00729C
Los, D. A., Murata, N. (2004). Membrane fluidity and its roles in the perception of environmental signals. Biochim. Biophys. Acta - Biomembr. 1666 (1), 142–157. doi: 10.1016/j.bbamem.2004.08.002
Mahmoud, L. M., Dutt, M., Shalan, A. M., El-Kady, M. E., El-Boray, M. S., Shabana, Y. M., et al. (2020). Silicon nanoparticles mitigate oxidative stress of in vitro-derived banana (Musa acuminata ‘Grand nain’) under simulated water deficit or salinity stress. South Afr. J. Bot. 132, 155–163. doi: 10.1016/j.sajb.2020.04.027
Malhi, G. S., Kaur, M., Kaushik, P. (2021). Impact of climate change on agriculture and its mitigation strategies: A review. Sustainability 13 (3), 1318. doi: 10.3390/su13031318
Manickavasagam, M., Pavan, G., Vasudevan, V. (2019). A comprehensive study of the hormetic influence of biosynthesized AgNPs on regenerating rice calli of indica cv. IR64. Sci. Rep. 9 (1), 1–11. doi: 10.1038/s41598-019-45214-y
Marslin, G., Sheeba, C. J., Franklin, G. (2017). Nanoparticles alter secondary metabolism in plants via ROS burst. Front. Plant Sci. 8, 832. doi: 10.3389/fpls.2017.00832
Medeiros, D. B., da Luz, L. M., de Oliveira, H. O., Araújo, W. L., Daloso, D. M., Fernie, A. R. (2019). Metabolomics for understanding stomatal movements. Theor. Exp. Plant Physiol. 31 (1), 91–102. doi: 10.1007/s40626-019-00139-9
Mishra, B. K., Srivastava, J. P., Lal, J. P., Sheshshayee, M. S. (2016). Physiological and biochemical adaptations in lentil genotypes under drought stress. Russ J. Plant Physiol. 63 (5), 695–708. doi: 10.1134/S1021443716040117
Mittal, D., Kaur, G., Singh, P., Yadav, K., Ali, S. A. (2020). Nanoparticle-based sustainable agriculture and food science: Recent advances and future outlook. Front. Nanotechnol. 2, 10. doi: 10.3389/fnano.2020.579954
Mohamed, M. E., El Semary, N. A., Younis, N. S. (2022). Silver nanoparticle production by the cyanobacterium cyanothece sp.: De Novo manipulation of nano-biosynthesis by phytohormones. Life 12 (2), 139. doi: 10.3390/life12020139
Moradbeygi, H., Jamei, R., Heidari, R., Darvishzadeh, R. (2020). Investigating the enzymatic and non-enzymatic antioxidant defense by applying iron oxide nanoparticles in dracocephalum moldavica l Plant under salinity stress. Sci. Hortic. (Amsterdam). 272, 109537. doi: 10.1016/j.scienta.2020.109537
Moradipour, M., Saberi-Riseh, R., Mohammadinejad, R., Hosseini, A. (2019). Nano-encapsulation of plant growth-promoting rhizobacteria and their metabolites using alginate-silica nanoparticles and carbon nanotube improves ucb1 pistachio micropropagation. J. Microbiol. Biotechnol. 29 (7), 1096–1103. doi: 10.4014/jmb.1903.03022
Muchate, N. S., Nikalje, G. C., Rajurkar, N. S., Suprasanna, P., Nikam, T. D. (2016). Plant salt stress: adaptive responses, tolerance mechanism and bioengineering for salt tolerance. Bot. Rev. 82 (4), 371–406. doi: 10.1007/s12229-016-9173-y
Mukhtar, T., ur Rehman, S., Smith, D., Sultan, T., Seleiman, M. F., Alsadon, A. A., et al. (2020). Mitigation of heat stress in solanum lycopersicum l. by ACC-deaminase and exopolysaccharide producing bacillus cereus: Effects on biochemical profiling. Sustainability 12 (6), 2159.
Müller-Xing, R., Xing, Q., Goodrich, J. (2014). Footprints of the sun: memory of UV and light stress in plants. Front. Plant Sci. 5, 474. doi: 10.3389/fpls.2014.00474
Ndaba, B., Roopnarain, A., Haripriya, R., Maaza, M. (2022). Biosynthesized metallic nanoparticles as fertilizers: An emerging precision agriculture strategy. J. Integr. Agric. 21 (5), 1225–1242. doi: 10.1016/S2095-3119(21)63751-6
Nejatzadeh, F. (2021). Effect of silver nanoparticles on salt tolerance of satureja hortensis l. during in vitro and in vivo germination tests. Heliyon 7 (2), e05981. doi: 10.1016/j.heliyon.2021.e05981
Nguyen, T. H. A., Nguyen, V.-C., Phan, T. N. H., Le, V. T., Vasseghian, Y., Trubitsyn, M. A., et al. (2022). Novel biogenic silver and gold nanoparticles for multifunctional applications: Green synthesis, catalytic and antibacterial activity, and colorimetric detection of Fe(III) ions. Chemosphere 287, 132271. doi: 10.1016/j.chemosphere.2021.132271
Nicolas-Espinosa, J., Garcia-Gomez, P., Rios, J. J., Piqueras, A., Bárzana, G., Carvajal, M. (2022). Nanoencapsulated boron foliar supply increased expression of NIPs aquaporins and BOR transporters of In vitro ipomoea batatas plants. Appl. Sci. 12 (4), 1788. doi: 10.3390/app12041788
Oves, M., Ahmar Rauf, M., Aslam, M., Qari, H. A., Sonbol, H., Ahmad, I., et al. (2022). Green synthesis of silver nanoparticles by conocarpus lancifolius plant extract and their antimicrobial and anticancer activities. Saudi J. Biol. Sci. 29 (1), 460–471. doi: 10.1016/j.sjbs.2021.09.007
Panpatte, D. G., Jhala, Y. K., Shelat, H. N., Vyas, R. V. (2016). “Nanoparticles: the next generation technology for sustainable agriculture,” in Microbial inoculants in sustainable agricultural productivity (Switzerland: Springer), 289–300.
Paramo, L. A., Feregrino-Pérez, A. A., Guevara, R., Mendoza, S., Esquivel, K. (2020). Nanoparticles in agroindustry: Applications, toxicity, challenges, and trends. Nanomaterials 10 (9), 1654. doi: 10.3390/nano10091654
Park, T. J., Lee, K. G., Lee, S. Y. (2016). Advances in microbial biosynthesis of metal nanoparticles. Appl. Microbiol. Biotechnol. 100 (2), 521–534. doi: 10.1007/s00253-015-6904-7
Pelegrino, M. T., Kohatsu, M. Y., Seabra, A. B., Monteiro, L. R., Gomes, D. G., Oliveira, H. C., et al. (2020). Effects of copper oxide nanoparticles on growth of lettuce (Lactuca sativa l.) seedlings and possible implications of nitric oxide in their antioxidative defense. Environ. Monit Assess. 192 (4), 1–14. doi: 10.1007/s10661-020-8188-3
Pociecha, E., Gorczyca, A., Dziurka, M., Matras, E., Oćwieja, M. (2021). Silver nanoparticles and silver ions differentially affect the phytohormone balance and yield in wheat. Agriculture 11 (8), 729. doi: 10.3390/agriculture11080729
Pradhan, S., Patra, P., Das, S., Chandra, S., Mitra, S., Dey, K. K., et al. (2013). Photochemical modulation of biosafe manganese nanoparticles on vigna radiata: a detailed molecular, biochemical, and biophysical study. Environ. Sci. Technol. 47 (22), 13122–13131. doi: 10.1021/es402659t
Pradhan, S., Patra, P., Mitra, S., Dey, K. K., Jain, S., Sarkar, S., et al. (2014). Manganese nanoparticles: impact on non-nodulated plant as a potent enhancer in nitrogen metabolism and toxicity study both in vivo and in vitro. J. Agric. Food Chem. 62 (35), 8777–8785. doi: 10.1021/jf502716c
Predoi, D., Ghita, R. V., Iconaru, S. L., Cimpeanu, C. L., Raita, S. M. (2020). Application of nanotechnology solutions in plants fertilization. Urban Hortic. Futur. 9, 12–40. doi: 10.5772/intechopen.91240
Qian, Y., Qin, C., Chen, M., Lin, S. (2020). Nanotechnology in soil remediation– applications vs. implications. Ecotoxicol. Environ. Saf. 201, 110815. doi: 10.1016/j.ecoenv.2020.110815
Raftery, A. E., Zimmer, A., Frierson, D. M. W., Startz, R., Liu, P. (2017). Less than 2 c warming by 2100 unlikely. Nat. Clim. Change 7 (9), 637–641. doi: 10.1038/nclimate3352
Rahman, M. H., Hasan, M. N., Nigar, S., Ma, F., Aly Saad Aly, M., Khan, M. Z. H. (2021). Synthesis and characterization of a mixed nanofertilizer influencing the nutrient use efficiency, productivity, and nutritive value of tomato fruits. ACS omega. 6 (41), 27112–27120. doi: 10.1021/acsomega.1c03727
Ranjan, A., Rajput, V. D., Minkina, T., Bauer, T., Chauhan, A., Jindal, T. (2021). Nanoparticles induced stress and toxicity in plants. Environ. Nanotechnol. Monit Manage. 15, 100457. doi: 10.1016/j.enmm.2021.100457
Rashtbari, Y., Sher, F., Afshin, S., Hamzezadeh, A., Ahmadi, S., Azhar, O., et al. (2022). Green synthesis of zero-valent iron nanoparticles and loading effect on activated carbon for furfural adsorption. Chemosphere 287, 132114. doi: 10.1016/j.chemosphere.2021.132114
Rastogi, A., Tripathi, D. K., Yadav, S., Chauhan, D. K., Živčák, M., Ghorbanpour, M., et al. (2019). Application of silicon nanoparticles in agriculture. 3 Biotech. 9 (3), 1–11. doi: 10.1007/s13205-019-1626-7
Rizwana, H., Alwhibi, M. S., Al-Judaie, R. A., Aldehaish, H. A., Alsaggabi, N. S. (2022). Sunlight-mediated green synthesis of silver nanoparticles using the berries of ribes rubrum (red currants): Characterisation and evaluation of their antifungal and antibacterial activities. Molecules 27 (7), 2186. doi: 10.3390/molecules27072186
Salam, A., Khan, A. R., Liu, L., Yang, S., Azhar, W., Ulhassan, Z., et al. (2022). Seed priming with zinc oxide nanoparticles downplayed ultrastructural damage and improved photosynthetic apparatus in maize under cobalt stress. J. Hazard Mater. 423, 127021. doi: 10.1016/j.jhazmat.2021.127021
Sambangi, P., Gopalakrishnan, S., Pebam, M., Rengan, A. K. (2022). Nano-biofertilizers on soil health, chemistry, and microbial community: benefits and risks. Proc. Indian Natl. Sci. Acad., 1–12. doi: 10.1007/s43538-022-00094-1
Sarraf, M., Vishwakarma, K., Kumar, V., Arif, N., Das, S., Johnson, R., et al. (2022). Metal/metalloid-based nanomaterials for plant abiotic stress tolerance: An overview of the mechanisms. Plants 11 (3), 316. doi: 10.3390/plants11030316
Seleiman, M. F., Almutairi, K. F., Alotaibi, M., Shami, A., Alhammad, B. A., Battaglia, M. L. (2020). Nano-fertilization as an emerging fertilization technique: why can modern agriculture benefit from its use? Plants 10 (1), 2. doi: 10.3390/plants10010002
Seleiman, M. F., Al-Suhaibani, N., Ali, N., Akmal, M., Alotaibi, M., Refay, Y., et al. (2021). Drought stress impacts on plants and different approaches to alleviate its adverse effects. Plants 10 (2), 259. doi: 10.3390/plants10020259
Seleiman, M. F., Al-Suhaibani, N., El-Hendawy, S., Abdella, K., Alotaibi, M., Alderfasi, A. (2021). Impacts of long- and short-term of irrigation with treated wastewater and synthetic fertilizers on the growth, biomass, heavy metal content, and energy traits of three potential bioenergy crops in arid regions. Energies 14 (11), 3037. doi: 10.3390/en14113037
Seleiman, M. F., Kheir, A. M. S. (2018). Maize productivity, heavy metals uptake and their availability in contaminated clay and sandy alkaline soils as affected by inorganic and organic amendments. Chemosphere 204, 514–522. doi: 10.1016/j.chemosphere.2018.04.073
Seleiman, M. F., Santanen, A., Mäkelä, P. S. A. (2020). Recycling sludge on cropland as fertilizer – advantages and risks. Resour. Conserv. Recycl. 155, 104647. doi: 10.1016/j.resconrec.2019.104647
Seleiman, M. F., Santanen, A., Stoddard, F. L., Mäkelä, P. (2012). Feedstock quality and growth of bioenergy crops fertilized with sewage sludge. Chemosphere 89 (10), 1211–1217. doi: 10.1016/j.chemosphere.2012.07.031
Seleiman, M. F., Semida, W. M., Rady, M. M., Mohamed, G. F., Hemida, K. A., Alhammad, B. A., et al. (2020). Sequential application of antioxidants rectifies ion imbalance and strengthens antioxidant systems in salt-stressed cucumber. Plants 9 (12). doi: 10.3390/plants9121783
Shah, V., Daverey, A. (2020). Phytoremediation: A multidisciplinary approach to clean up heavy metal contaminated soil. Environ. Technol. Innov. 18, 100774. doi: 10.1016/j.eti.2020.100774
Sharma, A., Sagar, A., Rana, J., Rani, R. (2022). Green synthesis of silver nanoparticles and its antibacterial activity using fungus talaromyces purpureogenus isolated from taxus baccata Linn. Micro. Nano Syst. Lett. 10 (1), 1–12. doi: 10.1186/s40486-022-00144-9
Shawon, Z. B. Z., Hoque, M. E., Chowdhury, S. R. (2020). “Nanosensors and nanobiosensors: Agricultural and food technology aspects,” in Nanofabrication for smart nanosensor applications (Elsevier), 135–161.
Sheikh Mohamed, M., Sakthi Kumar, D. (2019). “Application of nanotechnology in genetic improvement in crops,” in Nanoscience for sustainable agriculture Springer, 3–24.
Shen, Z., Jin, F., O’Connor, D., Hou, D. (2019). Solidification/stabilization for soil remediation: an old technology with new vitality (Washington, DC. United States: ACS Publications).
Siegel, J., Záruba, K., Švorčík, V., Kroumanová, K., Burketová, L., Martinec, J. (2018). Round-shape gold nanoparticles: effect of particle size and concentration on arabidopsis thaliana root growth. Nanoscale Res. Lett. 13 (1), 1–7. doi: 10.1186/s11671-018-2510-9
Singh, R. P., Handa, R., Manchanda, G. (2021). Nanoparticles in sustainable agriculture: An emerging opportunity. J. Control Release. 329, 1234–1248. doi: 10.1016/j.jconrel.2020.10.051
Srivastava, S., Bhargava, A. (2022a). “Biological synthesis of nanoparticles: Algae,” in Green nanoparticles: The future of nanobiotechnology (Switzerland: Springer Nature), 139–171.
Srivastava, S., Bhargava, A. (2022b). “Biological synthesis of nanoparticles: Fungi,” in Green nanoparticles: The future of nanobiotechnology (Switzerland: Springer Nature), 101–137.
Sukhanova, A., Bozrova, S., Sokolov, P., Berestovoy, M., Karaulov, A., Nabiev, I. (2018). Dependence of nanoparticle toxicity on their physical and chemical properties. Nanoscale Res. Lett. 13 (1), 1–21. doi: 10.1186/s11671-018-2457-x
Taha R, S., Seleiman, M. F., Alotaibi, M., Alhammad, B. A., Rady, M. M., Mahdi, A. H. A. (2020). Exogenous potassium treatments elevate salt tolerance and performances of glycine max l. by boosting antioxidant defense system under actual saline field conditions. Agronomy 10 (11), 1741. doi: 10.3390/agronomy10111741
Tan, W., Du, W., Barrios, A. C., Armendariz, R., Zuverza-Mena, N., Ji, Z., et al. (2017). Surface coating changes the physiological and biochemical impacts of nano-TiO2 in basil (Ocimum basilicum) plants. Environ. pollut. 222, 64–72. doi: 10.1016/j.envpol.2017.01.002
Tanveer, M., Ahmed, H. A. I. (2020). “ROS signalling in modulating salinity stress tolerance in plants,” in Salt and drought stress tolerance in plants (Switzerland: Springer Nature), 299–314.
Thor, K. (2019). Calcium–nutrient and messenger. Front. Plant Sci. 10, 440. doi: 10.3389/fpls.2019.00440
Torres-Limiñana, J., Feregrino-Pérez, A. A., Vega-González, M., Escobar-Alarcón, L., Cervantes-Chávez, J. A., Esquivel, K. (2022). Green synthesis via eucalyptus globulus l. extract of Ag-TiO2 catalyst: Antimicrobial activity evaluation toward water disinfection process. Nanomaterials 12 (11), 1944. doi: 10.3390/nano12111944
Tovar, G. I., Briceño, S., Suarez, J., Flores, S., González, G. (2020). Biogenic synthesis of iron oxide nanoparticles using moringa oleifera and chitosan and its evaluation on corn germination. Environ. Nanotechnol. Monit Manage. 14, 100350. doi: 10.1016/j.enmm.2020.100350
Tripathi, D. K., Singh, S., Singh, V. P., Prasad, S. M., Dubey, N. K., Chauhan, D. K. (2017). Silicon nanoparticles more effectively alleviated UV-b stress than silicon in wheat (Triticum aestivum) seedlings. Plant Physiol. Biochem. 110, 70–81. doi: 10.1016/j.plaphy.2016.06.026
Usman, M., Farooq, M., Wakeel, A., Nawaz, A., Cheema, S. A., ur Rehman, H., et al. (2020). Nanotechnology in agriculture: Current status, challenges and future opportunities. Sci. Total Environ. 721, 137778. doi: 10.1016/j.scitotenv.2020.137778
Van Zelm, E., Zhang, Y., Testerink, C. (2020). Salt tolerance mechanisms of plants. Annu. Rev. Plant Biol. 71, 403–433. doi: 10.1146/annurev-arplant-050718-100005
Vermeulen, S. J., Aggarwal, P. K., Ainslie, A., Angelone, C., Campbell, B. M., Challinor, A. J., et al. (2012). Options for support to agriculture and food security under climate change. Environ. Sci. Policy. 15 (1), 136–144. doi: 10.1016/j.envsci.2011.09.003
Vinodhini, S., Vithiya, B. S. M., Prasad, T. A. A. (2022). Green synthesis of silver nanoparticles by employing the allium fistulosum, tabernaemontana divaricate and basella alba leaf extracts for antimicrobial applications. J. King Saud Univ - Sci. 34 (4), 101939. doi: 10.1016/j.jksus.2022.101939
Vishwakarma, K., Upadhyay, N., Kumar, N., Tripathi, D. K., Chauhan, D. K., Sharma, S., et al. (2018). “Potential applications and avenues of nanotechnology in sustainable agriculture,” in Nanomaterials in plants, algae, and microorganisms (Elsevier), 473–500.
Viswanathan, S., Palaniyandi, T., Kannaki, P., Shanmugam, R., Baskar, G., Rahaman, A. M., et al. (2022). Biogenic synthesis of gold nanoparticles using red seaweed champia parvula and its anti-oxidant and anticarcinogenic activity on lung cancer. Part Sci. Technol., 1–9. doi: 10.1080/02726351.2022.2074926
Wahid, I., Kumari, S., Ahmad, R., Hussain, S. J., Alamri, S., Siddiqui, M. H., et al. (2020). Silver nanoparticle regulates salt tolerance in wheat through changes in ABA concentration, ion homeostasis, and defense systems. Biomolecules 10 (11), 1506. doi: 10.3390/biom10111506
Wang, Y., Zhang, P., Li, M., Guo, Z., Ullah, S., Rui, Y., et al. (2020). Alleviation of nitrogen stress in rice (Oryza sativa) by ceria nanoparticles. Environ. Sci. Nano. 7 (10), 2930–2940. doi: 10.1039/D0EN00757A
Willner, I., Basnar, B., Willner, B. (2007). Nanoparticle–enzyme hybrid systems for nanobiotechnology. FEBS J. 274 (2), 302–309. doi: 10.1111/j.1742-4658.2006.05602.x
Wu, H., Tito, N., Giraldo, J. P. (2017). Anionic cerium oxide nanoparticles protect plant photosynthesis from abiotic stress by scavenging reactive oxygen species. ACS Nano. 11 (11), 11283–11297. doi: 10.1021/acsnano.7b05723
Xu, X., Yuan, L., Yang, X., Zhang, X., Wang, L., Xie, Q. (2022). Circadian clock in plants: Linking timing to fitness. J. Integr. Plant Biol. 64 (4), 792–811. doi: 10.1111/jipb.13230
Yang, C., Zhang, X., Liu, G., Zhang, J., Bao, M., Zhou, Z. (2013). Progress on the structure and physiological functions of apoplastic barriers in root. Bull. Bot. Res. 33 (1), 114–119.
Yassin, M. T., Mostafa, A. A.-F., Al-Askar, A. A., Al-Otibi, F. O. (2022). Facile green synthesis of silver nanoparticles using aqueous leaf extract of origanum majorana with potential bioactivity against multidrug resistant bacterial strains. Crystals 12 (5), 603. doi: 10.3390/cryst12050603
Ye, S., Zeng, G., Wu, H., Zhang, C., Dai, J., Liang, J., et al. (2017). Biological technologies for the remediation of co-contaminated soil. Crit. Rev. Biotechnol. 37 (8), 1062–1076. doi: 10.1080/07388551.2017.1304357
Younis, A. A., Khattab, H., Emam, M. M. (2020). Impacts of silicon and silicon nanoparticles on leaf ultrastructure and TaPIP1 and TaNIP2 gene expressions in heat stressed wheat seedlings. Biol. Plant 64, 343–352. doi: 10.32615/bp.2020.030
Yuan, H., Liu, Q., Fu, J., Wang, Y., Zhang, Y., Sun, Y., et al. (2023). Co-Exposure of sulfur nanoparticles and Cu alleviate Cu stress and toxicity to oilseed rape brassica napus l. J. Environ. Sci. 124, 319–329. doi: 10.1016/j.jes.2021.09.040
Yu, S., Sun, J., Shi, Y., Wang, Q., Wu, J., Liu, J. (2021). Nanocellulose from various biomass wastes: Its preparation and potential usages towards the high value-added products. Environ. Sci. Ecotechnol. 5, 100077. doi: 10.1016/j.ese.2020.100077
Zhang, H., Yue, M., Zheng, X., Xie, C., Zhou, H., Li, L. (2017). Physiological effects of single-and multi-walled carbon nanotubes on rice seedlings. IEEE Trans. Nanobiosci. 16 (7), 563–570. doi: 10.1109/TNB.2017.2715359
Zhou, P., Adeel, M., Shakoor, N., Guo, M., Hao, Y., Azeem, I., et al. (2020). Application of nanoparticles alleviates heavy metals stress and promotes plant growth: An overview. Nanomaterials 11 (1), 26. doi: 10.3390/nano11010026
Keywords: nanoparticles, nanomaterials, abiotic stress, plant eustress, secondary metabolites, elicitor
Citation: Aguirre-Becerra H, Feregrino-Pérez AA, Esquivel K, Perez-Garcia CE, Vazquez-Hernandez MC and Mariana-Alvarado A (2022) Nanomaterials as an alternative to increase plant resistance to abiotic stresses. Front. Plant Sci. 13:1023636. doi: 10.3389/fpls.2022.1023636
Received: 20 August 2022; Accepted: 20 September 2022;
Published: 11 October 2022.
Edited by:
Mahmoud F. Seleiman, Department of Plant Production, King Saud University, Saudi ArabiaReviewed by:
Omnia Elshayb, Agricultural Research Center, EgyptCopyright © 2022 Aguirre-Becerra, Feregrino-Pérez, Esquivel, Perez-Garcia, Vazquez-Hernandez and Mariana-Alvarado. This is an open-access article distributed under the terms of the Creative Commons Attribution License (CC BY). The use, distribution or reproduction in other forums is permitted, provided the original author(s) and the copyright owner(s) are credited and that the original publication in this journal is cited, in accordance with accepted academic practice. No use, distribution or reproduction is permitted which does not comply with these terms.
*Correspondence: Ana Angélica Feregrino-Pérez, ZmVyZWdyaW5vLmFuZ2dlQGhvdG1haWwuY29t
Disclaimer: All claims expressed in this article are solely those of the authors and do not necessarily represent those of their affiliated organizations, or those of the publisher, the editors and the reviewers. Any product that may be evaluated in this article or claim that may be made by its manufacturer is not guaranteed or endorsed by the publisher.
Research integrity at Frontiers
Learn more about the work of our research integrity team to safeguard the quality of each article we publish.