- 1College of Life Sciences, Xinyang Normal University, Xinyang, China
- 2Wuhan Botanical Garden, Chinese Academy of Sciences, Wuhan, China
Variations in latitudinal gradients could lead to changes in the performance and ecological effects of invasive plants and thus may affect the species composition, distribution and interspecific substitution of native plant communities. However, variations in structure, similarity and beta (β) diversity within invaded communities across latitudinal gradients in heterogeneous habitats remain unclear. In this study, we conducted a two-year field survey along 21°N to 37°N in China, to examine the differential effects of the amphibious invasive plant Alternanthera philoxeroides on native plant communities in terrestrial and aquatic habitats. We compared the differences in the invasion importance value (IV), species distribution, community similarity (Jaccard index and Sorenson index) and β diversity (Bray−Curtis index and βsim index) between terrestrial and aquatic communities invaded by A. philoxeroides, as well as analyzed their latitudinal trends. We found that the IV of A. philoxeroides and β diversity in aquatic habitats were all significantly higher than that of terrestrial, while the terrestrial habitat had a higher community similarity values. The aquatic A. philoxeroides IV increased with increasing latitude, while the terrestrial IV had no significant latitudinal trend. With increasing latitude, the component proportion of cold- and drought-tolerant species in the terrestrial communities increased, and the dominant accompanying species in the aquatic communities gradually changed from hygrophytes and floating plants to emerged and submerged plants. In addition, the aquatic communities had lower community similarity values and higher β diversity in higher latitudinal regions, while terrestrial communities had the opposite parameters in these regions. Our study indicates that the bioresistance capacities of the native communities to invasive A. philoxeroides in heterogeneous habitats are different; A. philoxeroides invasion leads to higher community homogenization in terrestrial habitats than in aquatic habitats, and terrestrial communities experience more severe homogenization in higher latitudinal regions. These findings are crucial for predicting the dynamics of invasive plant communities under rapid global change.
Introduction
Under rapid global change, alien plant invasions increasingly cause serious threats to biodiversity, community stability and ecosystem functioning (Reaser et al., 2020; Peng et al., 2022). Many invasive plants significantly change the structure of native communities through interspecific competition and accelerate community homogenization (Vítková et al., 2020; Wang et al., 2021a). Lots of studies have showed that plant invasions greatly reduce the biomass, coverage, evenness, richness and diversity of native plant species (Flory and Clay, 2010; Yuguda et al., 2022), and plant invasions also could cause a significant increase in the litter biomass of invaded communities, which further benefits for excluding native plants (Gooden and French, 2014). Additionally, plant communities containing only native species have a higher invasibility than those that included alien species, and alien plants that have similar functional traits with natives are more easily to enter and dominate the native plant communities (Gooden and French, 2015; Li et al., 2015; Rijal et al., 2017). Moreover, environmental changes may affect the invasion process, e.g., seedling survival rate and drought tolerance of the invasive species Pennisetum ciliare are all increased by combinations of drought and warming, while this synergistic effect decreases leaf respiration of the native accompanying species Heteropogon contortus and causes substantial carbon loss, which accelerates native plant death (Ravi et al., 2022).
Latitudinal variations cause hydrothermal fluctuations, which could significantly affect the performance of invasive plants (Liu et al., 2022). The invaders usually have more active responses to latitudinal variations than their native congeners. E.g., the flowering period, biomass, individual height, seed size, leaf length-width ratio, water-use efficiency, seed abscisic acid and aliphatic acid contents of many invasive plants all increase with increasing latitude (Li et al., 2016; Zhou et al., 2021; Liu et al., 2022), and they also increase the reproductive allocation at higher latitudes by advancing the flowering onset (Helsen et al., 2020), which all benefits the expansion of invasion scopes. The territory of China spans approximately 50 degrees of latitude, and the variable climatic conditions along the latitudinal gradient provide conditions for plant invasions. Although the richness of invasive plants is high in the low latitudes of southern China, most have a lower noxious risk, while the invaders in the high latitudes of northern China have low richness but pose a higher risk (Feng and Zhu, 2010). In addition, global warming will accelerate the spread of these invasive plants originating from South America and Mexico to the high latitudinal regions of China (Feng et al., 2011). Thus, exploring the dynamics of invasive plant communities along latitudinal gradients is critical for maintaining native community stability and predicting plant invasion situations under global change.
Beta (β) diversity represents the differentiation of species composition and turnover between communities across environmental gradients. Compared with alpha (α) diversity, it is more conducive to understand community construction process and optimize biodiversity protection measurements at the larger biogeography scale (Cao et al., 2021; Yu et al., 2021). Community similarity is usually negatively related to β diversity; higher similarity indicates that communities tend to be stable, while higher β diversity shows faster species turnover (Chao and Chiu, 2016; Qian et al., 2022). Neutral theory proposes that community similarity decreases with increasing spatial distances, while β diversity is formed by the combined effect of species dispersal and ecological drift, which are also affected by climatic factors and soil types; in fact, these various environmental factors are comprehensively reflected by variations in latitude (Martin and Wilsey, 2015; Ricotta et al., 2017; Godsoe et al., 2022; Peng et al., 2022). Qian (2009) found that the β diversity of seed plants and fern plants in North America shows negative correlations with latitude, while Martin and Wilsey (2015) found that the community similarity of grasslands in Texas decreases with increasing latitude. However, latitudinal trends in the similarity and β diversity of plant community with single dominant invader are not well understood.
The alligator weed Alternanthera philoxeroides is a noxious invasive weed that is native to South America and has spread to a latitudinal gradient of 42°S to 37°N around the world (Wu et al., 2016). Because of its strong phenotypic plasticity, it has extensively invaded the aquatic and terrestrial habitats of nearly 20 provinces in China, causing a serious loss of native biodiversity (Wu et al., 2017a; Wu and Ding, 2019). Recently studies have shown that α diversity within the A. philoxeroides community decreases with increasing latitude in China (Wu et al., 2016). The chemical defence and growth rate of A. philoxeroides show opposite variations along a latitudinal gradient (Yang et al., 2021). Lu et al. (2013) found that climate warming facilitated faster A. philoxeroides expansion to higher latitudes than that of the biocontrol beetle Agasicles hygrophila and thus increased enemy release in those regions. Lu et al. (2016, 2018) also found nonparallel latitudinal patterns of rhizospheric pathogenic fungi and root-knot nematodes associated with A. philoxeroides and the native A. sessilis, which may enhance bioresistance to A. philoxeroides invasion at higher latitudes under climate change. However, differences among plant communities invaded by A. philoxeroides along a latitudinal gradient are unclear. In this study, we hypothesize that the structure, similarity and β diversity of the A. philoxeroides community may be significantly affected by latitude. Specifically, we addressed the following questions: (1) Are invasive dominance, species distribution, community similarity and β diversity in terrestrial and aquatic A. philoxeroides communities different? (2) Do these four parameters vary across a latitudinal gradient?
Materials and methods
Field survey and data collection
We conducted field surveys of A. philoxeroides-invaded communities during July and August of 2019-2020. Our study areas covered 18 cities of 10 provinces in China, which nearly contain the latitudinal bounds of present wild A. philoxeroides distributions in mainland China. We chose locations where A. philoxeroides covered an area of more than 100 m2 to establish plots. We selected the plots along eight latitudinal clusters (approximately 2° apart) from 21°N to 37°N in China (Clusters 1 to 8). In each latitudinal cluster, we selected five terrestrial plots (10×10 m for each) scattered in two or three sampling cities and selected five aquatic plots in the same latitudinal cluster close to terrestrial plots. We established 40 terrestrial and 40 aquatic plots, and plots with the same habitat type were at least 10 kilometers apart (as shown in Figure 1). The detailed geographic information of the plots is shown in the Supplementary Table 1.
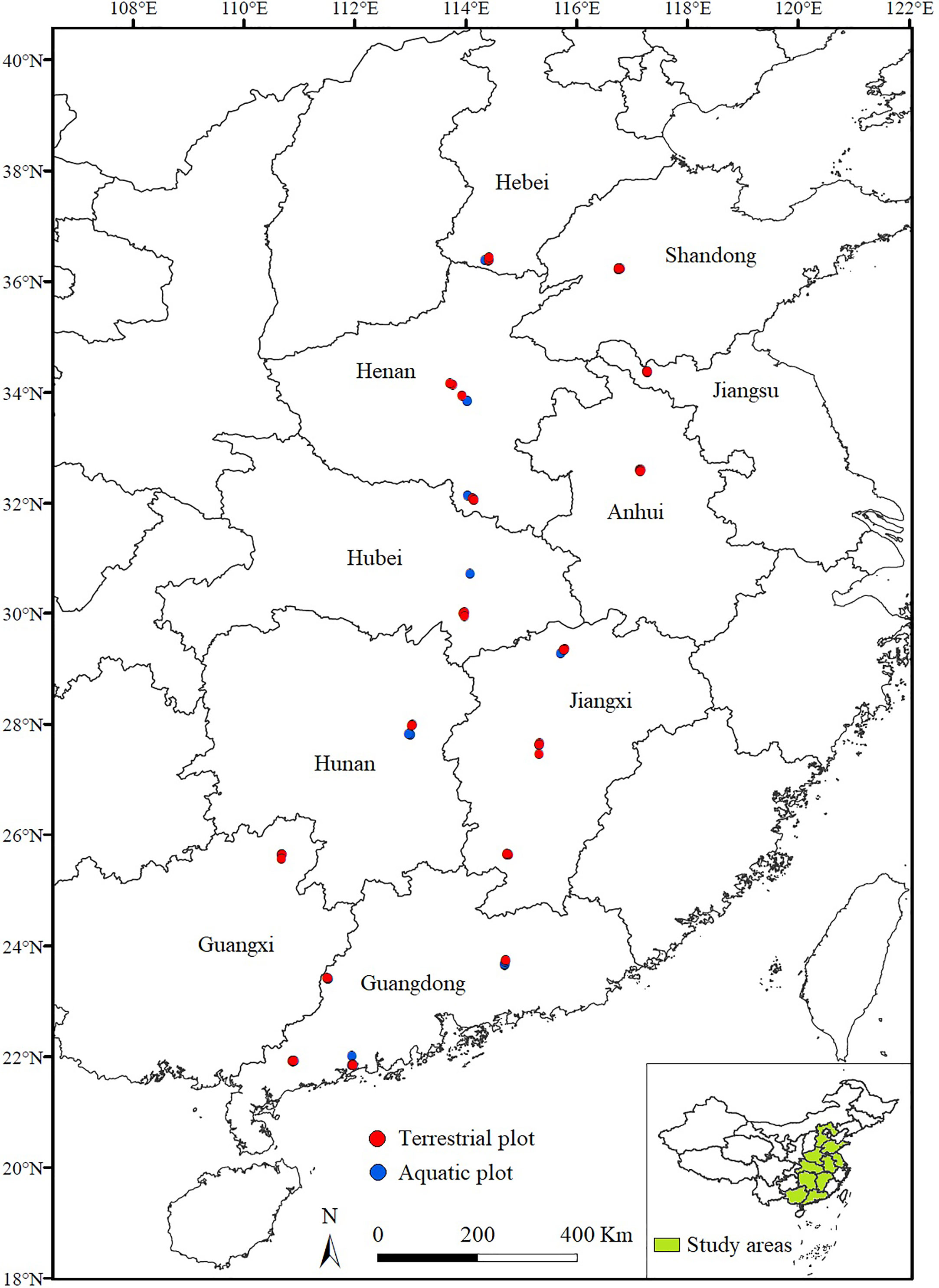
Figure 1 Sampling plot distributions of A. philoxeroides communities across amphibious habitats along a latitudinal gradient in China.
We evenly set up three 10 m transects in each plot, and five quadrats (0.5×0.5 m) were uniformly spaced 2 m apart along each transect. According to the survey methods of Wu et al. (2016), we recorded the species name, abundance, individual height and species coverage of plants in each quadrat. We used the ‘Flora of China’ website (http://www.iplant.cn/frps) and the ‘Chinese Virtual Herbarium’ website (http://www.cvh.ac.cn) to identify unknown plant species. We also measured the longitude, latitude and elevation data of each plot using a handheld GPS receiver (Garmin GPSMAP 639csx, USA).
Data analysis
We used the importance value (IV) to evaluate the dominance of plant species in each plot, and the IV was also used as matrix data in the numerical ordination of plant species distributions in this study. The calculation formula of IV in each plot was as follows (Wu et al., 2016; Wu et al., 2017a; Wu et al., 2017b):
Where relative abundance, relative height and relative coverage refer to the percentages of each species abundances, height and coverage over the sum of all species total abundances, height and coverage within a plot, respectively.
The total IV was the sum of a plant species’ IV in all sampling plots.
We calculated the Jaccard index and Sorenson index using the following formulas, to evaluate the community similarity (Marzialetti et al., 2021):
where a and b are the total number of plant species in communities A and B, respectively, and j is the number of plant species shared between these two communities.
β diversity represents the variation among communities in both species composition and abundance values, and we use the Bray−Curtis index and the βsim index to evaluate β diversity with the following formulas (Chao and Chiu, 2016):
where Na and Nb are the total abundance values of all species in communities A and B, respectively; jN= ∑ min (jNa, jNb). a is the number of plant species shared between communities A and B, while b and c are the number of unique plant species in each community.
We conducted one-way analysis of variance (ANOVA) and multiple comparisons of Fisher’s protected least significant difference (LSD) (Subset for α = 0.05) by using SPSS 16.0 software (SPSS Inc., Chicago, USA) to examine the differences in A. philoxeroides IVs along the eight latitudinal clusters, and normality and homogeneity of our data were verified by using the homogeneity of variance test before ANOVA. We also examined the difference in A. philoxeroides IV, accompanying species richness, community similarity and β diversity between terrestrial and aquatic habitats by using the independent sample t test (Subset for α = 0.05) in SPSS 16.0.
We established the IV matrix (40×30) based on the main plants (top 30 plant species in total IV) in the terrestrial and aquatic habitats, respectively and established the latitude matrix (40×1 for terrestrial and 40×1 for aquatic). We then conducted detrended canonical correspondence analysis (DCCA) using Canoco 4.5 software (Microcomputer Power, Ithaca New York, USA) to explore the effect of latitudinal gradients on the plant species distributions in A. philoxeroides-invaded communities in heterogeneous habitats (Feurdean et al., 2012).
Results
Species dominance and composition along latitudinal gradients
The mean A. philoxeroides IV of the terrestrial and aquatic plots was 0.434 and 0.594, respectively, and the A. philoxeroides IV in the aquatic habitats was extremely significantly higher than that in the terrestrial habitats (t=5.820, P<0.001). There was no significant difference in the A. philoxeroides IV in the terrestrial habitats among the eight latitudinal clusters, while there was an extremely significant difference in the aquatic habitats (F7, 39 = 3.481, P=0.007) (Figure 2). Specifically, the aquatic A. philoxeroides IVs at higher latitudes (Clusters 7 and 8) were significantly higher than those at lower latitudes (Clusters 1 to 3) (Figure 2).
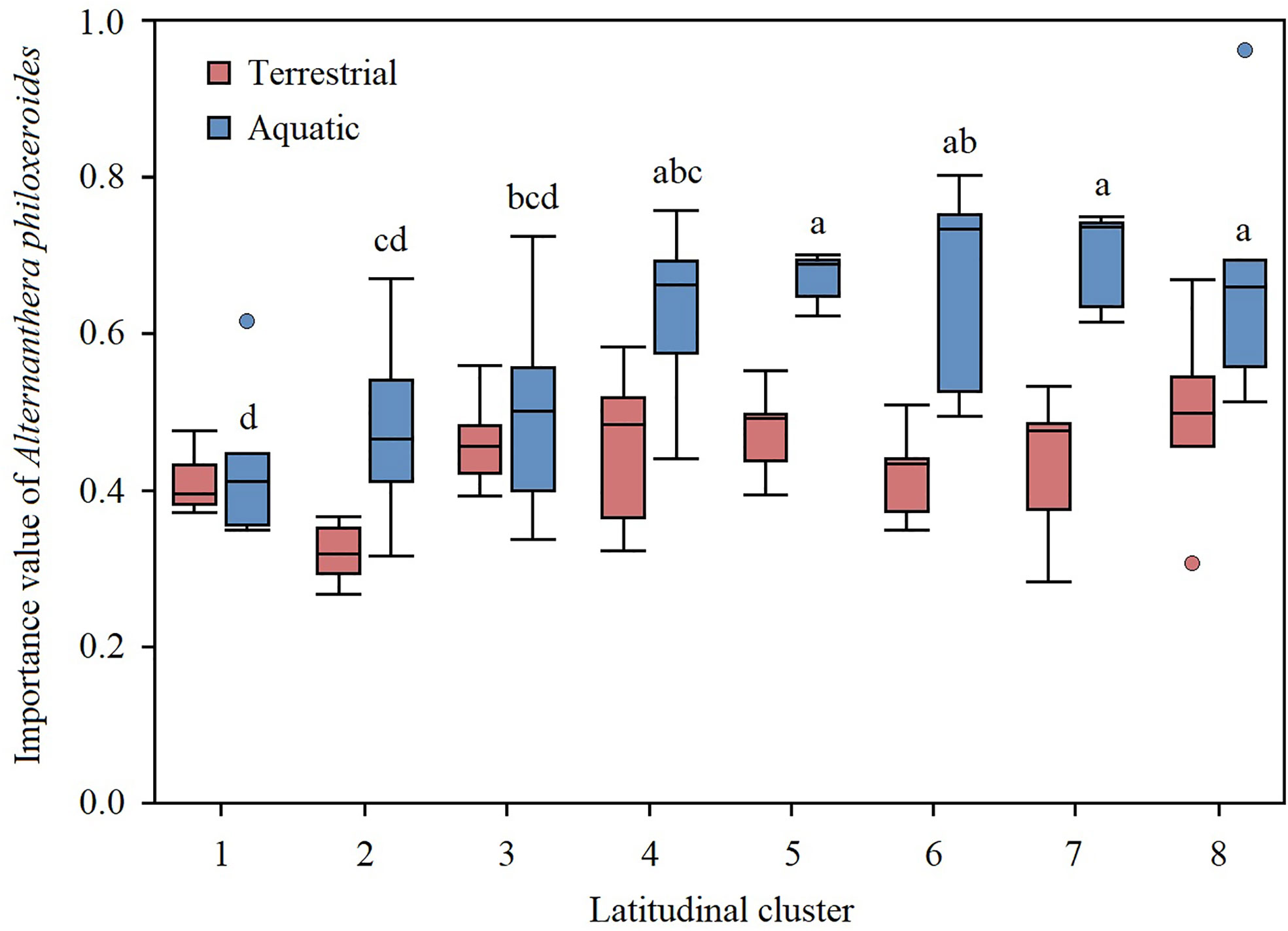
Figure 2 Variations in A. philoxeroides dominance in terrestrial and aquatic habitats among different latitudinal transects. The upper and lower bounds of the box represent the 75% and 25% percentiles, respectively; the line in the middle represents the mean and the whiskers extended to the 5% and 95% percentiles, respectively. Solid dots represent the outliers. The different letters indicate that the importance value has significant differences at the 0.05 level.
We recorded 178 plant species belonging to 51 families and 149 genera in 40 terrestrial plots. Asteraceae had the most species (31 spp.), followed by Poaceae (21 spp.). We recorded 104 plant species belonging to 43 families and 90 genera in 40 aquatic plots, and Poaceae (16 spp.) and Asteraceae (11 spp.) had higher species richness. The mean accompanying species richness of eight latitudinal clusters in the terrestrial habitats was extremely significantly higher than that in the aquatic habitats (t=5.354, P<0.001), and the highest difference was in latitudinal Cluster 3 (21°N-37°N) (Figures 3, 4).
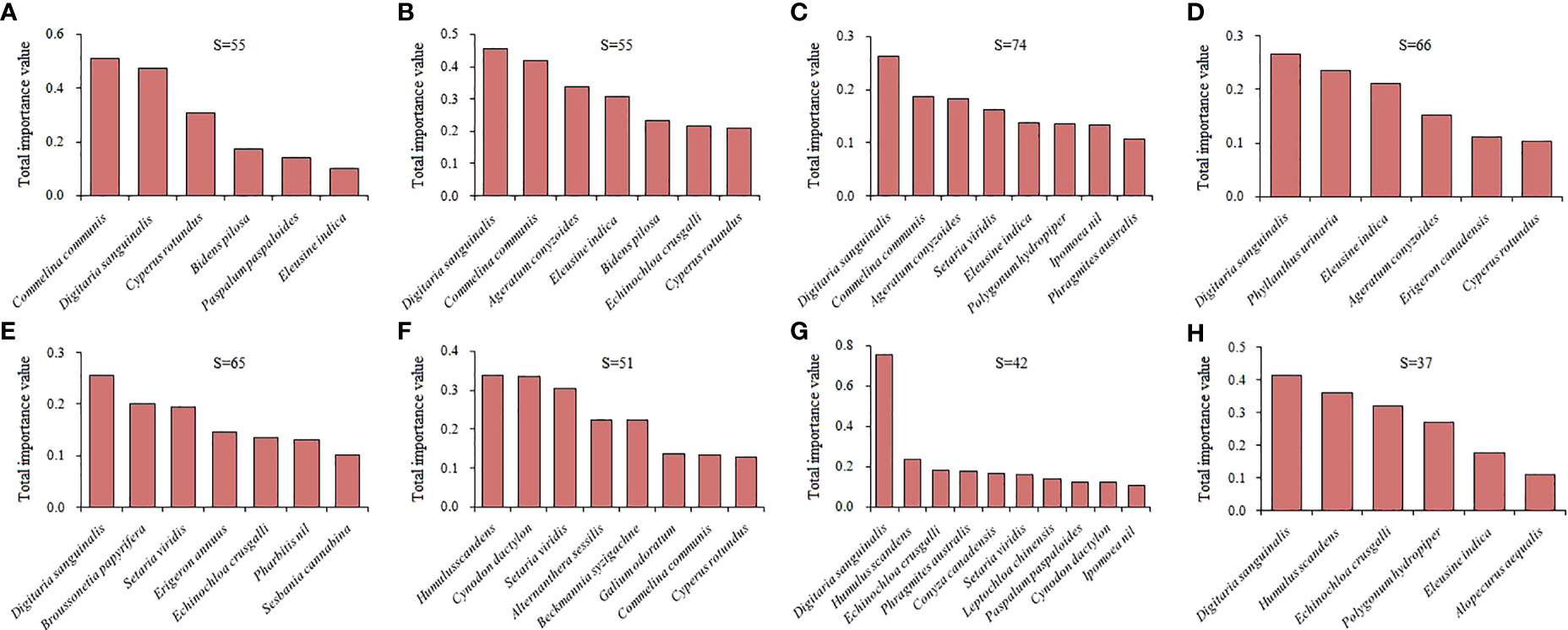
Figure 3 Variations in the dominant accompanying plant species of the A. philoxeroides communities among different latitudinal clusters in terrestrial habitats. S values represent the total number of accompanying plant species in the A. philoxeroides-invaded communities along each latitudinal cluster. (A–H) represent the 1 to 8 latitudinal clusters, respectively. The same is as follows.
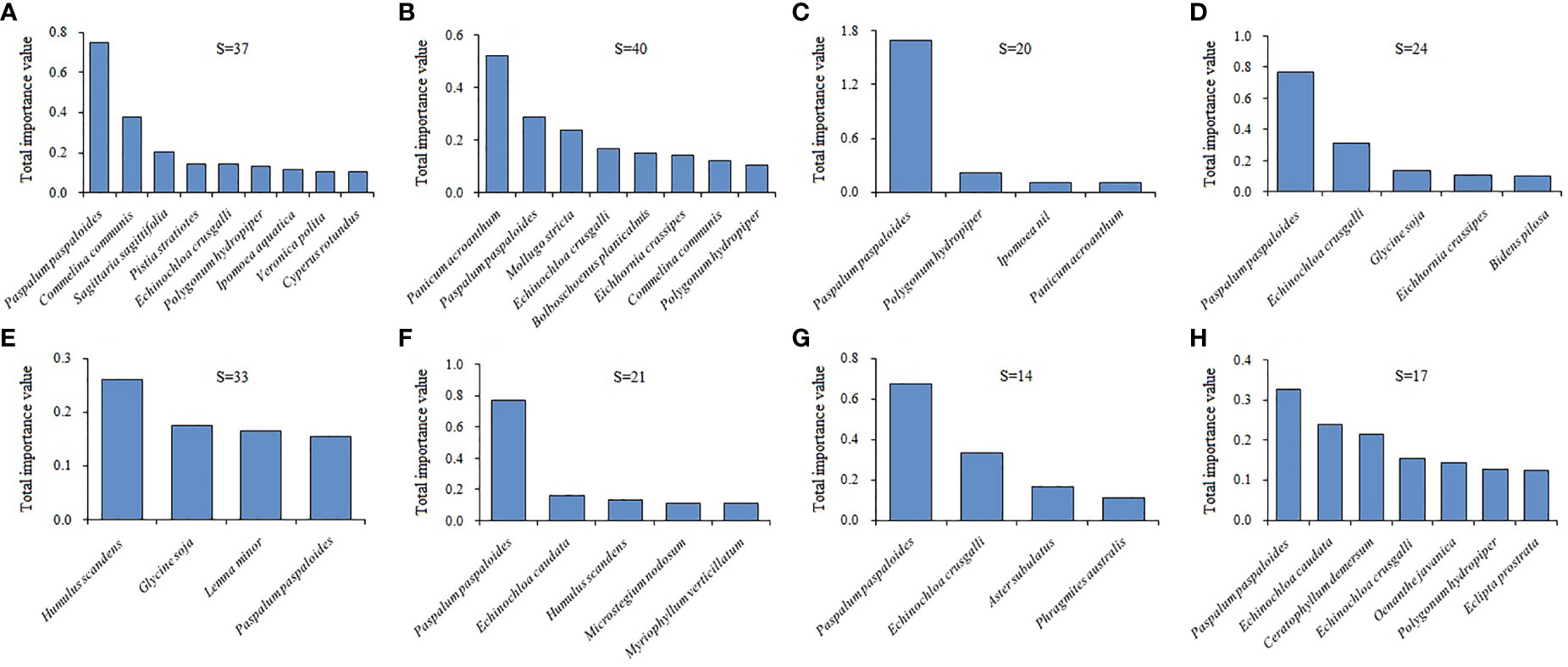
Figure 4 Variations in the dominant accompanying plant species of the A. philoxeroides communities among different latitudinal clusters in aquatic habitats. (A–H) represent the 1 to 8 latitudinal clusters, respectively.
In the terrestrial A. philoxeroides communities, the dominant accompanying species were Digitaria sanguinalis, Commelina communis, Humulus scandens, Echinochloa crusgalli and Eleusine indica (Figure 3). D. sanguinalis had the highest dominance in the most latitudinal clusters. The dominance of C. communis, Cyperus rotundus and Bidens pilosa decreased with increasing latitude. The dominance of H. scandens and E. crusgalli rapidly increased north of 31°N (latitudinal Cluster 6 to 8). In the aquatic communities, Paspalum paspaloides, Polygonum hydropiper, Panicum acroanthum and C. communis were the dominant accompanying species (Figure 4); among them, P. paspaloides had the highest dominance. The dominance of tall hygrophytes (e.g., Oenanthe javanica, Phragmites australis), as well as submerged plants (e.g., Myriophyllum verticillatum, Ceratophyllum demersum), increased at the higher latitudes.
Species distribution along latitudinal gradients
Top 30 plant species in total IV in the amphibious habitats are shown in Table 1. The terrestrial communities had an obvious latitudinal trend of species distributions in the DCCA ordination diagram (Figure 5A). C. communis (3), Ageratum conyzoides (11), C. rotundus (8) and B. pilosa (9) are all distributed in the far left of the ordination diagram, showing that these plant species were intensively distributed in the lower latitudinal regions. Conversely, H. scanden (4) tended to be distributed in higher latitudinal regions. A. philoxeroides (1) had wide latitudinal adaptability, and it drastically competed with Ludwigia prostrate (29) as these two species located very closely. In addition, the distributions of Oxalis corniculata (22) and Beckmannia syzigachne (25) were weakly affected by latitude.
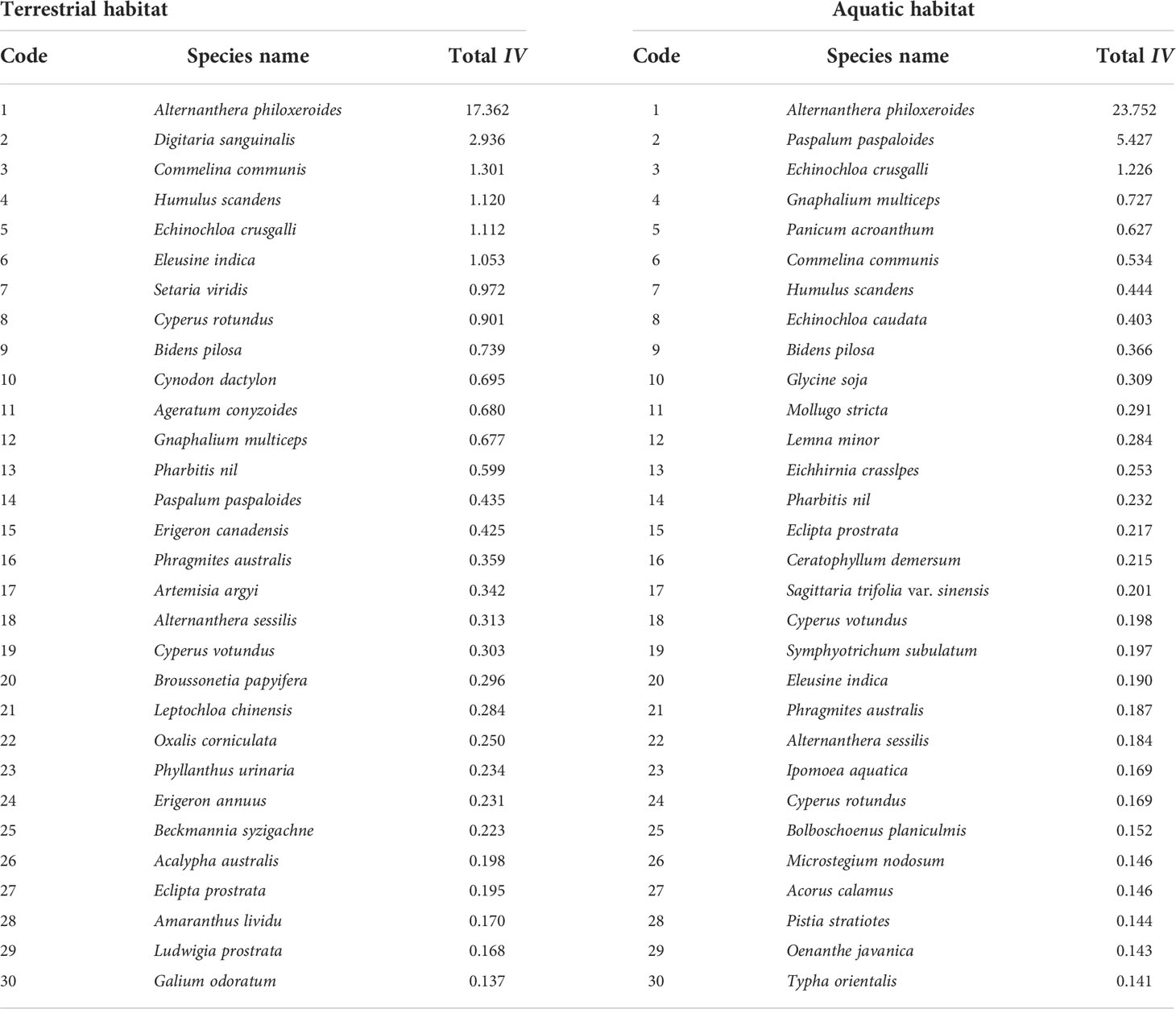
Table 1 The total importance values of dominant plant species in terrestrial and aquatic Alternanthera philoxeroides communities.
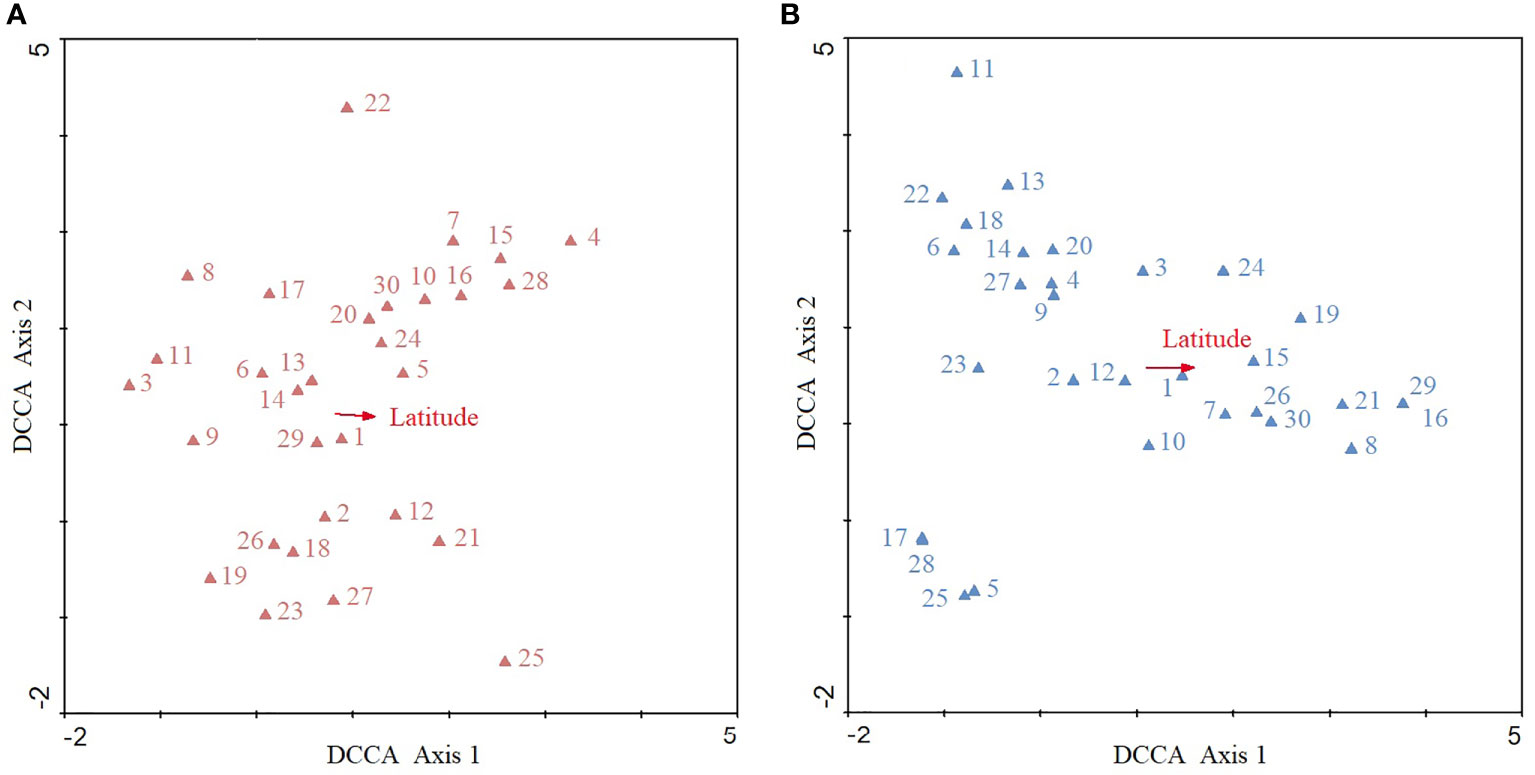
Figure 5 DCCA ordination diagrams of 30 dominant plant species of the A. philoxeroides communities in terrestrial (A) and aquatic (B) habitats along a latitudinal gradient. The triangle is the optimal distribution position of each plant species along the latitudinal gradient. The arrow indicates the direction of increase in latitude from the ordination centre. The vertical distance between the triangle and vector represents the correlation between the species distribution and latitude. Arabic numbers are codes of the dominant plant species (as Table 1 shows for terrestrial and aquatic habitats).
The latitudinal trend of species distributions in aquatic habitats was more obvious (Figure 5B). C. communis (6), A. sessilis (22), C. rotundus (18) and Ipomoea aquatica (23) were distributed in lower latitudes, while C. demersum (16), O. javanica (29), P. australis (21) and Echinochloa caudata (8) tended to be distributed at higher latitudes. A. philoxeroides (1) have intense interspecific competition with Lemna minor (12). However, latitude weakly affected the distributions of Mollugo stricta (11), Sagittaria trifolia var. sinensis (17), Pistia stratiotes (28), Bolboschoenus planicalmis (25) and P. acroanthum (5).
Community similarity and β diversity along latitudinal gradients
For community similarity, the Jaccard index (t=3.078, P=0.010) and Sorenson index (t=3.035, P=0.010) in the terrestrial communities were significantly higher than those in the aquatic communities. For β diversity, the Bray−Curtis index (t=4.002, P=0.006) and βSim index (t=2.302, P=0.040) in the aquatic communities were significantly higher than those in the terrestrial communities.
The latitudinal variation in community similarity is shown as Figures 6A, B. With the increasing latitudinal gradient, terrestrial and aquatic community similarity showed similar repeated fluctuations. The terrestrial communities had the highest similarity at the higher latitudinal gradient (VII) and the lowest similarity at the middle latitudinal gradient (V). The aquatic communities had the highest similarity at the middle latitudinal gradient (IV) and the lowest similarity at the higher latitudinal gradient (VI).
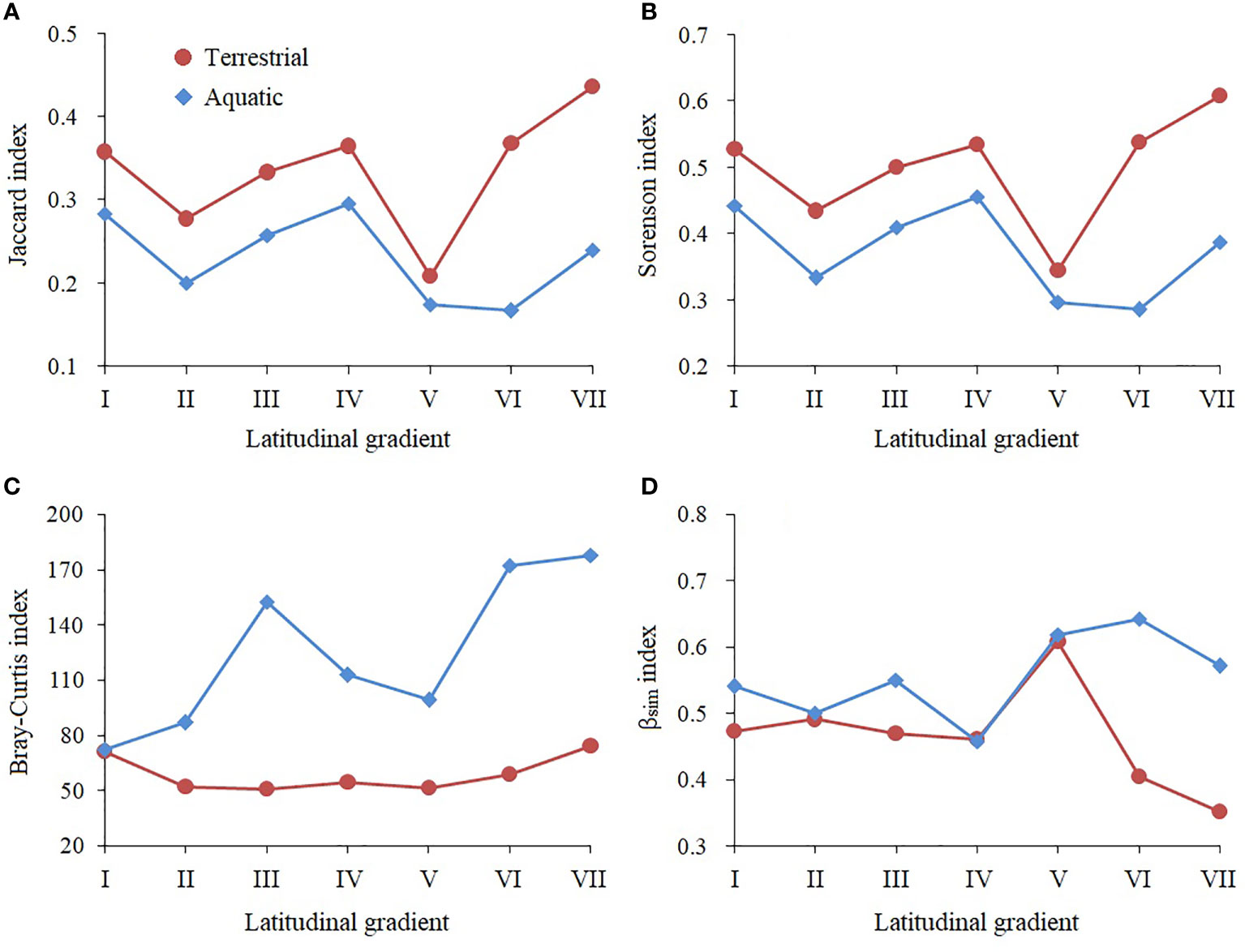
Figure 6 Variations in the β diversity index and community similarity index of the A. philoxeroides communities along the latitudinal gradient. Latitudinal gradients I to VII represent the pairwise comparison between the A. philoxeroides community across latitudinal Clusters 1 to 8. (A, B) represent the community similarity, (C, D) represent the β diversity.
The latitudinal variation in the β diversity between the terrestrial and aquatic communities showed great differences (Figures 6C, D). The terrestrial Bray−Curtis index was relatively stable with latitude, while the aquatic Bray−Curtis index was fluctuated, with a maximum value at a higher latitudinal gradient (VII) and a minimum value at a lower latitudinal gradient (I). The terrestrial βsim index showed a rising first and then declining trend with latitude; it had a maximum value at the middle latitudinal gradient (V) and a minimum value at the higher latitudinal gradient (VII). However, the aquatic βSim index fluctuated greatly, with a maximum value at the higher latitudinal gradient (VI) and a minimum value at the middle latitudinal gradient (IV).
Discussion
Habitat heterogeneity promotes niche differentiation, which provides more opportunities for plant species coexistence and affects the structure of an invaded community (Czarniecka-Wiera et al., 2020). In our study, the aquatic A. philoxeroides IV was significantly higher than the terrestrial A. philoxeroides IV along the whole latitudinal gradient, possibly because although A. philoxeroides invades both terrestrial and aquatic habitats in China, it prefers to invade aquatic ecosystems around the word (e.g., in the United States, New Zealand, and Australia), showing its original aquatic preference (You et al., 2018). In addition, the richness of terrestrial plants in the world is much higher than that of aquatic plants; therefore, the terrestrial bioresistance of a native community in terms of inhibiting an A. philoxeroides invasion may be higher than that of aquatic plants (You et al., 2016; Wu et al., 2017b), which further facilitates aquatic A. philoxeroides dominance and its significant latitudinal trend. In particular, the resource pulses caused by climate change in different latitudinal regions will also significantly change invaded community structures (Lu et al., 2018; Jentsch and White, 2019).
One of the important factors driving plant species coexistence in invaded communities is interspecific competition (Wang et al., 2021b). D. sanguinalis, E. crusgalli and Setaria vulgaris in terrestrial A. philoxeroides communities have rapid tillering ability and/or relatively higher individual height and can effectively resist A. philoxeroides invasion, and these plants could be considered biosubstitute species for invasion control (Wu et al., 2017a; Wu et al., 2017b; Yu et al., 2020). In addition, the B. pilosa in terrestrial communities possesses a larger seed yield and faster growth rate, combined with the capacity of uniparental reproduction, making it able to coexist with A. philoxeroides (Hao et al., 2018). The DCCA indicated that the proportion of cold-and drought-tolerant plant species rose with increasing latitude in the terrestrial communities. For example, native H. scandens at higher latitudes has superior stress resistance and vigorous twining stems allowing it to occupy spatial resources, and its strong allelopathy could also inhibit A. philoxeroides growth (Wang et al., 2021c). The dominant accompanying species in the aquatic habitats gradually changed from hygrophytes and floating plants to emerged and submerged plants with increasing latitude. For instance, P. paspaloides has strong photosynthetic carbon absorption and assimilation rates, allowing it to widely co-occur in aquatic invasive communities. O. javanica, P. australis, M. verticillatum and C. demersum have higher adaptability to water level fluctuations, which allows them to fully use heterogeneous light sources in aquatic ecosystems and coexist with invaders (Crisman et al., 2014; Saluja and Garg, 2017).
Plant invasions usually facilitate species turnover and the homogenization of flora, thus reducing β diversity (Yu et al., 2021; Petsch et al., 2022). In our study, the aquatic A. philoxeroides communities had lower similarity and higher β diversity than those of the terrestrial communities, which may have been related to the simpler community structure and the narrower species niche in the aquatic habitats that made them more vulnerable to external environmental fluctuations at large latitudinal scales; thus, they had higher rates of species turnover (Pennington et al., 2006; Wu et al., 2017b). In addition, comparing with the terrestrial habitats, aquatic habitats usually have the higher thermal stability, which makes aquatic plants have higher energy utilization rate than terrestrial plants, and thus benefits improving the specialization and species turnover rates of aquatic plants (Landeiro et al., 2014; Wu et al., 2017b).
Previous studies found that the α diversity of terrestrial A. philoxeroides communities was higher than that of aquatic communities, which create a higher bioresistance to terrestrial A. philoxeroides invasion and may reduce species turnover between invaders and native plants (Wu et al., 2017b). In fact, as a plant species, the dispersal of A. philoxeroides into terrestrial communities across a large latitudinal scale also directly increases community similarity by increasing the number of shared species (Ricotta et al., 2017). Our study emphasized that only the plant species with traits for resisting A. philoxeroides invasion could continuously occur in the invaded communities. Similar to Poaceae and Asteraceae, these highly invasion-tolerant functional groups occupy a large proportion of terrestrial accompanying species compositions in all latitudinal clusters. This may be because with an ongoing invasion, the phylogenetic relationships in the terrestrial A. philoxeroides community experience rapid convergent evolution, which increases community similarity, and a similar phenomenon has also been found in terrestrial invaded communities in India and North America (Dar and Reshi, 2015; Stotz et al., 2016; Petsch et al., 2022). Compared to aquatic communities, terrestrial A. philoxeroides communities experience stronger disturbances from human activities, climate change and herbivores, which may interrupt ongoing species turnover and thereby reduce β diversity (Lu et al., 2016; Ricotta et al., 2017; Xiao et al., 2020).
The latitudinal trend of community similarity and β diversity in terrestrial habitats may be associated with the growth-defence tradeoffs of invasive plants (Ulrich et al., 2014; Martin and Wilsey, 2015). As the variations of microclimate and herbivore pressure along latitudes, terrestrial A. philoxeroides synthesizes more secondary metabolites at mid-latitudes, for increasing the chemical defence, but relatively reduces its growth and dominance (Liu et al., 2021). This may provide more opportunities for native species establishment and turnover, thus decreasing community similarity in middle latitudes. However, A. philoxeroides spreads faster than its natural enemy A. hygrophila along latitudes with recent global warming, and this invader has shifted its defence strategy to a growth strategy at higher latitudes due to ‘enemy release’ (Lu et al., 2013). This scenario would generally increase the component proportion of A. philoxeroides in the invaded terrestrial community and increase community similarity at higher latitudes. These findings also indirectly support the latitudinal herbivory defence hypothesis (LHDH), which predicts that the herbivory damage and plant defence all increase toward lower latitudes (Johnson and Rasmann, 2011). In this study, the terrestrial Bray−Curtis index was not sensitive to latitudinal gradients, perhaps because this parameter was calculated based on abundance, and our survey was conducted in summer when terrestrial plants had higher species richness values; thus, the response of richness to latitude masks that of abundance. Field surveys in different seasons should be conducted in the future to more accurately evaluate the β diversity of invaded terrestrial communities.
In our study, aquatic A. philoxeroides had lower similarity but higher β diversity at higher latitudinal gradients, which was associated with the dispersal limitation of native aquatic plant species (Qian, 2009; Shi et al., 2021). Compared with the developed hydrographic networks of central and southern China, the number of wild aquatic habitats in the higher latitudinal regions of North China have sharply decreased (Liu et al., 2017), which has reduced the connectivity of the aquatic plant community at higher latitudes with that at middle and lower latitudes. This may cause greater geographical isolation and benefit aquatic species specialization at higher latitudes and decrease the community similarity in those regions (Wu and Ding, 2019). For instance, in comparison to other species, the emerging dominant accompanying species C. demersum in aquatic habitats in higher latitudinal clusters has superior asexual reproduction and high assimilation efficiency of nitrogen (Xiong et al., 2010), and these advantages in competition may lead to higher species turnover.
In conclusion, we found significant differences in the structure, similarity and β diversity of A. philoxeroides communities in heterogeneous habitats, as well as their latitudinal trends, which were quite different from the latitudinal α-diversity gradient (LDG) rule of the A. philoxeroides community in our previous studies (Wu et al., 2016; Wu et al., 2017b). These results indicate that the terrestrial and aquatic A. philoxeroides communities had higher species turnover rates at middle and high latitudes, respectively, and native plants in these regions have potential substitutions for A. philoxeroides invasions. However, a rainfall belt moving northwards in China would further improve the connectivity of aquatic A. philoxeroides invasions, which would increase community similarity at higher latitudes (Wu and Ding, 2020), while climate warming aggravates the nontarget effects of terrestrial A. philoxeroides invasion control at middle latitudes and may thus decrease terrestrial β diversity (Lu et al., 2015; Liu et al., 2021). We should intensify the invasion assessment of A. philoxeroides at middle and high latitudes to prevent community homogenization in those regions. Our study explored the differences among A. philoxeroides-invaded communities among all latitudinal bounds of its present distributions on mainland China, and these findings could provide important implications for better understanding the construction process in invaded communities and predicting plant invasions under global change.
Data availability statement
The original contributions presented in the study are included in the article/Supplementary Material. Further inquiries can be directed to the corresponding authors.
Author contributions
HW and BR designed the experiments. HW and SD performed the experiments and analyzed the data. HW, SD and BR wrote the manuscript. All authors contributed to the article and approved the submitted version.
Funding
This study was funded by the National Natural Science Foundation of China (31800460) and the Nanhu Scholars Program for Young Scholars of Xinyang Normal University (XYNU).
Acknowledgments
We would like to thank Yanyan Wang, Li Wang, Junhui Jiang, Tiantian Zhang, Qiubo Ji, Nannan Xiao, Wenhao Wang, Shaoqi Jia, Junda Wang for their help in field surveys and laboratory analysis. We are grateful for comments by the editor and reviewers that improved this manuscript. We are also grateful to Springer Nature Author Services for language editing.
Conflict of interest
The authors declare that the research was conducted in the absence of any commercial or financial relationships that could be construed as a potential conflict of interest.
Publisher’s note
All claims expressed in this article are solely those of the authors and do not necessarily represent those of their affiliated organizations, or those of the publisher, the editors and the reviewers. Any product that may be evaluated in this article, or claim that may be made by its manufacturer, is not guaranteed or endorsed by the publisher.
Supplementary material
The Supplementary Material for this article can be found online at: https://www.frontiersin.org/articles/10.3389/fpls.2022.1021337/full#supplementary-material
References
Cao, K., Condit, R., Mi, X. C., Chen, L., Ren, H. B., Xu, W. B., et al. (2021). Species packing and the latitudinal gradient in beta-diversity. P. R. Soc B-Biol. Sci. 288, 20203045. doi: 10.1098/rspb.2020.3045
Chao, A., Chiu, C. H. (2016). Bridging the variance and diversity decomposition approaches to beta diversity via similarity and differentiation measures. Methods Ecol. Evol. 7, 919–928. doi: 10.1111/2041-210X.12551
Crisman, T. L., Alexandridis, T. K., Zalidis, G. C., Takavakoglou, V. (2014). Phragmites distribution relative to progressive water level decline in lake koronia, Greece. Ecohydrology 7, 1403–1411. doi: 10.1002/eco.1466
Czarniecka-Wiera, M., Szymura, T. H., Kcki, Z. (2020). Understanding the importance of spatial scale in the patterns of grassland invasions. Sci. Total. Environ. 727, 138669. doi: 10.1016/j.scitotenv.2020.138669
Dar, ,. P. A., Reshi, Z. A. (2015). Do alien plant invasions cause biotic homogenization of terrestrial ecosystems in the Kashmir valley, India? Trop. Ecol. 56, 111–123.
Feng, J. M., Zhang, Z., Nan, R. Y. (2011). The roles of climatic factors in spatial patterns of alien invasive plants from America into China. Biodivers. Conserv. 20, 3385–3391. doi: 10.1007/s10531-011-0122-0
Feng, J. M., Zhu, Y. Y. (2010). Alien invasive plants in China: risk assessment and spatial patterns. Biodivers. Conserv. 19, 3489–3497. doi: 10.1007/s10531-010-9909-7
Feurdean, ,. A., Tamas, T., Tantau, I., Farcas, S. (2012). Elevational variation in regional vegetation responses to late-glacial climate changes in the carpathians. J. Biogeogr. 39, 258–271. doi: 10.1111/j.1365-2699.2011.02605.x
Flory, S. L., Clay, K. (2010). Non-native grass invasion alters native plant composition in experimental communities. Biol. Invasions 12, 1285–1294. doi: 10.1007/s10530-009-9546-9
Godsoe, W., Bellingham, P. J., Moltchanova, E. (2022). Disentangling niche theory and beta diversity change. Am. Nat. 199, 510–522. doi: 10.1086/718592
Gooden, B., French, K. (2014). Non-interactive effects of plant invasion and landscape modification on native communities. Divers. Distrib. 20, 626–639. doi: 10.1111/ddi.12178
Gooden, B., French, K. (2015). Impacts of alien plant invasion on native plant communities are mediated by functional identity of resident species, not resource availability. Oikos 124, 298–306. doi: 10.1111/oik.01724
Hao, J. H., Bhattacharya, S., Ma, L., Wang, L. X. (2018). Breeding systems and seed production for six weedy taxa of Bidens. weed. Biol. Manage. 18, 41–49. doi: 10.1111/wbm.12142
Helsen, K., Acharya, K. P., Graae, B. J., De Kort, H., Brunet, J., Chabrerie, O., et al. (2020). Earlier onset of flowering and increased reproductive allocation of an annual invasive plant in the north of its novel range. Ann. Bot. 126, 1005–1016. doi: 10.1093/aob/mcaa110
Jentsch, A., White, P. (2019). A theory of pulse dynamics and disturbance in ecology. Ecology 100, e02734. doi: 10.1002/ecy.2734
Johnson, M. T. J., Rasmann, S. (2011). The latitudinal herbivory defence hypothesis takes a detour on the map. New. Phytol. 191, 589–592. doi: 10.1111/j.1469-8137.2011.03816.x
Landeiro, V. L., Waldez, F., Menin, M. (2014). Spatial and environmental patterns of Amazonian anurans: differences between assemblages with aquatic and terrestrial reproduction, and implications for conservation management. Nat. Conservacao 12, 42–46. doi: 10.4322/natcon.2014.008
Li, S. P., Cadotte, M. W., Shu, W. S. (2015). The effects of phylogenetic relatedness on invasion success and impact: deconstructing darwin’s naturalisation conundrum. Ecol. Lett. 18, 1285–1292. doi: 10.1111/ele.12522
Li, J. M., Du, L. S., Guan, W. B., YU, F. H., van Kleunen, M. (2016). Latitudinal and longitudinal clines of phenotypic plasticity in the invasive herb Solidago canadensis in China. Oecologia 182, 755–764. doi: 10.1007/s00442-016-3699-x
Liu, W., Chen, X., Wang, J., Zhang, Y. H. (2022). Does the effect of flowering time on biomass allocation across latitudes differ between invasive and native salt marsh grass Spartina alterniflora? Ecol. Evol. 12, e8681. doi: 10.1002/ece3.8681
Liu, M., Pan, Y. F., Pan, X. Y., Sosa, A., Blumenthal, D. M., van Kleunen, M., et al. (2021). Plant invasion alters latitudinal pattern of plant-defense syndromes. Ecology 102, e03511. doi: 10.1002/ecy.3511
Liu, D. S., Wang, R., Gordon, D. R., Sun, X. H., Chen, L., Wang, Y. W. (2017). Predicting plant invasions following china’s water diversion project. Environ. Sci. Technol. 5, 1450–1457. doi: 10.1021/acs.est.6b05577
Lu, X. M., He, M. Y., Ding, J. Q., Siemann, E. (2018). Latitudinal variation in soil biota: testing the biotic interaction hypothesis with an invasive plant and a native congener. ISME. J. 12, 2811–2822. doi: 10.1038/s41396-018-0219-5
Lu, X. M., Siemann, E., He, M. Y., Wei, H., Shao, X., Ding, J. Q. (2015). Climate warming increases biological control agent impact on a non-target species. Ecol. Lett. 18, 48–56. doi: 10.1111/ele.12391
Lu, X. M., Siemann, E., He, M. Y., Wei, H., Shao, X., Ding, J. Q. (2016). Warming benefits a native species competing with an invasive congener in the presence of a biocontrol beetle. New. Phytol. 211, 1371–1381. doi: 10.1111/nph.13976
Lu, X. M., Siemann, E., Shao, X., Wei, H., Ding, J. Q. (2013). Climate warming affects biological invasions by shifting interactions of plants and herbivores. Global. Change. Biol. 19, 2339–2347. doi: 10.1111/gcb.12244
Martin, L. M., Wilsey, B. J. (2015). Differences in beta diversity between exotic and native grasslands vary with scale along a latitudinal gradient. Ecology 96, 1042–1051. doi: 10.1890/14-0772.1
Marzialetti, F., Cascone, S., Frate, L., Di Febbraro, M., Acosta, A.T.R., Carranza, M.L. (2021). Measuring alpha and beta diversity by field and remote-sensing data: A challenge for coastal dunes biodiversity monitoring. Remote Sens. 13 (10), 1928. doi: 10.3390/rs13101928
Peng, Y., Feng, J., Sang, W., Axmacher, J. C. (2022). Geographical divergence of species richness and local homogenization of plant assemblages due to climate change in grasslands. Biodivers. Conserv. 31, 797–810. doi: 10.1007/s10531-022-02364-2
Pennington, R. T., Richardson, J. E., Lavin, M. (2006). Insights into the historical construction of species-rich biomes from dated plant phylogenies, neutral ecological theory and phylogenetic community structure. New. Phytol. 172, 605–616. doi: 10.1111/j.1469-8137.2006.01902.x
Petsch, D. K., Bertoncin, A. P. D., Ortega, J. C. G., Thomaz, S. M. (2022). Non-native species drive biotic homogenization, but it depends on the realm, beta diversity facet and study design: a meta-analytic systematic review. Oikos. 2022, e08768. doi: 10.1111/oik.08768
Qian, H. (2009). Beta diversity in relation to dispersal ability for vascular plants in north America. Global. Ecol. Biogeogr 18, 327–332. doi: 10.1111/j.1466-8238.2009.00450.x
Qian, H., Leprieur, F., Jin, Y., Wang, X. L., Deng, T. (2022). Influence of phylogenetic scale on the relationships of taxonomic and phylogenetic turnovers with environment for angiosperms in China. Ecol. Evol. 12, e8544. doi: 10.1002/ece3.8544
Ravi, S., Law, D. J., Caplan, J. S., Barron-Gafford, G. A., Dontsova, K. M., Espeleta, J. F., et al. (2022). Biological invasions and climate change amplify each other’s effects on dryland degradation. Global. Change. Biol. 28, 285–295. doi: 10.1111/gcb.15919
Reaser, J. K., Burgiel, S. W., Kirkey, J., Brantley, K. A., Veatch, S. D., Burgos-Rodriguez, J. (2020). The early detection of and rapid response (EDRR) to invasive species: a conceptual framework and federal capacities assessment. Biol. Invasions 22, 1–19. doi: 10.1007/s10530-019-02156-w
Ricotta, C., Rapson, G. L., Asmus, U., Pysek, P., Kuehn, I., La Sorte, F. A., et al. (2017). British Plants as aliens in new Zealand cities: residence time moderates their impact on the beta diversity of urban floras. Biol. Invasions. 19, 3589–3599. doi: 10.1007/s10530-017-1590-2
Rijal, D. P., Alm, T., Nilsen, L., Alsos, I. G. (2017). Giant invasive Heracleum persicum: Friend or foe of plant diversity? Ecol. Evol. 7, 4936–4950. doi: 10.1002/ece3.3055
Saluja, ,. R., Garg, J. K. (2017). Macrophyte species composition and structure along littoral region in relation to limnological variables of a tropical wetland ecosystem. Chem. Ecol. 33, 499–515. doi: 10.1080/02757540.2017.1328502
Shi, W., Wang, Y. Q., Xiang, W. S., Li, X. K., Cao, K. F. (2021). Environmental filtering and dispersal limitation jointly shaped the taxonomic and phylogenetic beta diversity of natural forests in southern China. Ecol. Evol. 11, 8783–8794. doi: 10.1002/ece3.7711
Stotz, G. C., Gianoli, E., Cahill, J. F. (2016). Spatial pattern of invasion and the evolutionary responses of native plant species. Evol. .Appl 9, 939–951. doi: 10.1111/eva.12398
Ulrich, W., Soliveres, S., Maestre, F. T., Gotelli, N. J., Quero, J. L., Delgado-Baquerizo, M., et al. (2014). Climate and soil attributes determine plant species turnover in global drylands. J. Biogeogr 41, 2307–2319. doi: 10.1111/jbi.12377
Vítková, ,. M., Sádlo, J., Roleček, J., Petrik, P., Sitzia, T., Mullerova, J., et al. (2020). Robinia pseudoacacia-dominated vegetation types of southern Europe: Species composition, history, distribution and management. Sci. Total. Environ. 707, 134857. doi: 10.1016/j.scitotenv.2019.134857
Wang, ,. H., Fu, H., Wen, Z. H., Yuan, C. B., Zhang, X. L., Ni, L. Y., et al. (2021b). Seasonal patterns of taxonomic and functional beta diversity in submerged macrophytes at a fine scale. Ecol. Evol. 11, 9827–9836. doi: 10.1002/ece3.7811
Wang, L. C., Liu, Y., Zhu, X. M., Zhang, Z., Huang, X. Q. (2021c). Identify potential allelochemicals from Humulus scandens (Lour.) merr. root extracts that induce allelopathy on Alternanthera philoxeroides (Mart.) griseb. Sci. Rep. 11, 7068. doi: 10.1038/s41598-021-86656-7
Wang, ,. Y., Xiong, Y. T., Wang, Y., Li, Q. J. (2021a). Long period exposure to serious cadmium pollution benefits an invasive plant (Alternanthera philoxeroides) competing with its native congener (Alternanthera sessilis). Sci. Total. Environ. 786, 147456. doi: 10.1016/j.scitotenv.2021.147456
Wu, H., Carrillo, J., Ding, J. Q. (2016). Invasion by alligator weed, Alternanthera philoxeroides, is associated with decreased species diversity across the latitudinal gradient in china. J. Plant Ecol. 93, 311–319. doi: 10.1093/jpe/rtv060
Wu, H., Carrillo, J., Ding, J. Q. (2017b). Species diversity and environmental determinants of aquatic and terrestrial communities invaded by Alternanthera philoxeroides. sci. Total. Environ. 581, 666–675. doi: 10.1016/j.scitotenv.2016.12.177
Wu, H., Ding, J. Q. (2019). Global change sharpens the double-edged sword effect of aquatic alien plants in china and beyond. Front. Plant Sci. 10, 787. doi: 10.3389/fpls.2019.00787
Wu, H., Ding, J. Q. (2020). Abiotic and biotic determinants of plant diversity in aquatic communities invaded by water hyacinth (Eichhornia crassipes (Mart.) solms). Front. Plant Sci. 11, 306. doi: 10.3389/fpls.2020.01306
Wu, H., Ismail, M., Ding, J. Q. (2017a). Global warming increases the interspecific competitiveness of the invasive plant alligator weed, Alternanthera philoxeroides. Sci. Total. Environ. 575, 1415–1422. doi: 10.1016/j.scitotenv.2016.09.226
Xiao, L., Ding, J. Q., Zhang, J. L., Huang, W., Siemann, E. (2020). Chemical responses of an invasive plant to herbivory and abiotic environments reveal a novel invasion mechanism. Sci. Total. Environ. 74, 140452. doi: 10.5897/AJB10.421
Xiong, ,. H. F., Tan, Q. L., Hu, C. X. (2010). Structural and metabolic responses of Ceratophyllum demersum to eutrophic conditions. Afr. J. Biotechnol. 9, 5722–5729.
Yang, Y., Liu, M., Pan, Y. F., Huang, H. Y., Pan, X. Y., Sosa, A., et al. (2021). Rapid evolution of latitudinal clines in growth and defence of an invasive weed. New. Phytol. 230, 845–856. doi: 10.1111/nph.17193
You, ,. W. H., Fang, L. X., Xi, D. G., Du, D. L., Xie, D. (2018). Difference in capacity of clonal integration between terrestrial and aquatic Alternanthera philoxeroides in response to defoliation: implications for biological control. Hydrobiologia 817, 319–328. doi: 10.1007/s10750-017-3418-6
You, W. H., Han, C. M., Fang, L. X., Du, D. L. (2016). Propagule pressure, habitat conditions and clonal integration influence the establishment and growth of an invasive clonal plant, Alternanthera philoxeroides. Front. Plant Sci. 7, 568. doi: 10.3389/fpls.2016.00568
Yu, C. T., Fan, C. Y., Zhang, C. Y., Zhao, X. H., von Gadow, K. (2021). Decomposing spatial β-diversity in the temperate forests of northeastern China. Ecol. Evol. 11, 11362–11372. doi: 10.1002/ece3.7926
Yuguda, T. K., Wu, Y., Leng, Z., Gao, G. F., Li, G. L., Dai, Z. C., et al. (2022). Impact of Spartina alterniflora invasion on evapotranspiration water loss in Phragmites australis dominated coastal wetlands of east China. Ecol. Eng. 179, 106605. doi: 10.1016/j.ecoleng.2022.106605
Yu, H. X., Yue, M. F., Wang, C., Le Roux, J., Peng, C. L., Li, W. H. (2020). Priority effects and competition by a native species inhibit an invasive species and may assist restoration. Ecol. Evol. 10, 13355–13369. doi: 10.1002/ece3.6938
Keywords: plant invasions, beta diversity, community similarity, latitude, Alternanthera philoxeroides
Citation: Wu H, Dong S and Rao B (2022) Latitudinal trends in the structure, similarity and beta diversity of plant communities invaded by Alternanthera philoxeroides in heterogeneous habitats. Front. Plant Sci. 13:1021337. doi: 10.3389/fpls.2022.1021337
Received: 17 August 2022; Accepted: 22 September 2022;
Published: 06 October 2022.
Edited by:
Lei Deng, Northwest A&F University, ChinaReviewed by:
Xiaoqiong Li, Guangxi University, ChinaYi Wang, Yunnan University, China
Wenyong Guo, East China Normal University, China
Copyright © 2022 Wu, Dong and Rao. This is an open-access article distributed under the terms of the Creative Commons Attribution License (CC BY). The use, distribution or reproduction in other forums is permitted, provided the original author(s) and the copyright owner(s) are credited and that the original publication in this journal is cited, in accordance with accepted academic practice. No use, distribution or reproduction is permitted which does not comply with these terms.
*Correspondence: Hao Wu, wuhao86868686@163.com; Benqiang Rao, rbqxy@163.com