- 1Laboratory of Plant Molecular Pathology and Functional Genomics, Department of Plant Biosciences, School of Applied Biosciences, College of Agriculture & Life Science, Kyungpook National University, Daegu, South Korea
- 2Laboratory of Horticultural Crop Protection, Department of Horticultural Science, Gyeongsang National University, Jinju, South Korea
- 3Department of Entomology, Abdul Wali Khan University, Mardan, Pakistan
- 4Institute of Molecular Plant Sciences, The University of Edinburgh, Edinburgh, United Kingdom
Environmental pollutants like heavy metals are toxic, persistent, and bioaccumulative in nature. Contamination of agricultural fields with heavy metals not only hampers the quality and yield of crops but also poses a serious threat to human health by entering the food chain. Plants generally cope with heavy metal stress by regulating their redox machinery. In this context, nitric oxide (NO) plays a potent role in combating heavy metal toxicity in plants. Studies have shown that the exogenous application of NO donors protects plants against the deleterious effects of heavy metals by enhancing their antioxidative defense system. Most of the studies have used sodium nitroprusside (SNP) as a NO donor for combating heavy metal stress despite the associated concerns related to cyanide release. Recently, NO-releasing nanoparticles have been tested for their efficacy in a few plants and other biomedical research applications suggesting their use as an alternative to chemical NO donors with the advantage of safe, slow and prolonged release of NO. This suggests that they may also serve as potential candidates in mitigating heavy metal stress in plants. Therefore, this review presents the role of NO, the application of chemical NO donors, potential advantages of NO-releasing nanoparticles, and other NO-release strategies in biomedical research that may be useful in mitigating heavy metal stress in plants.
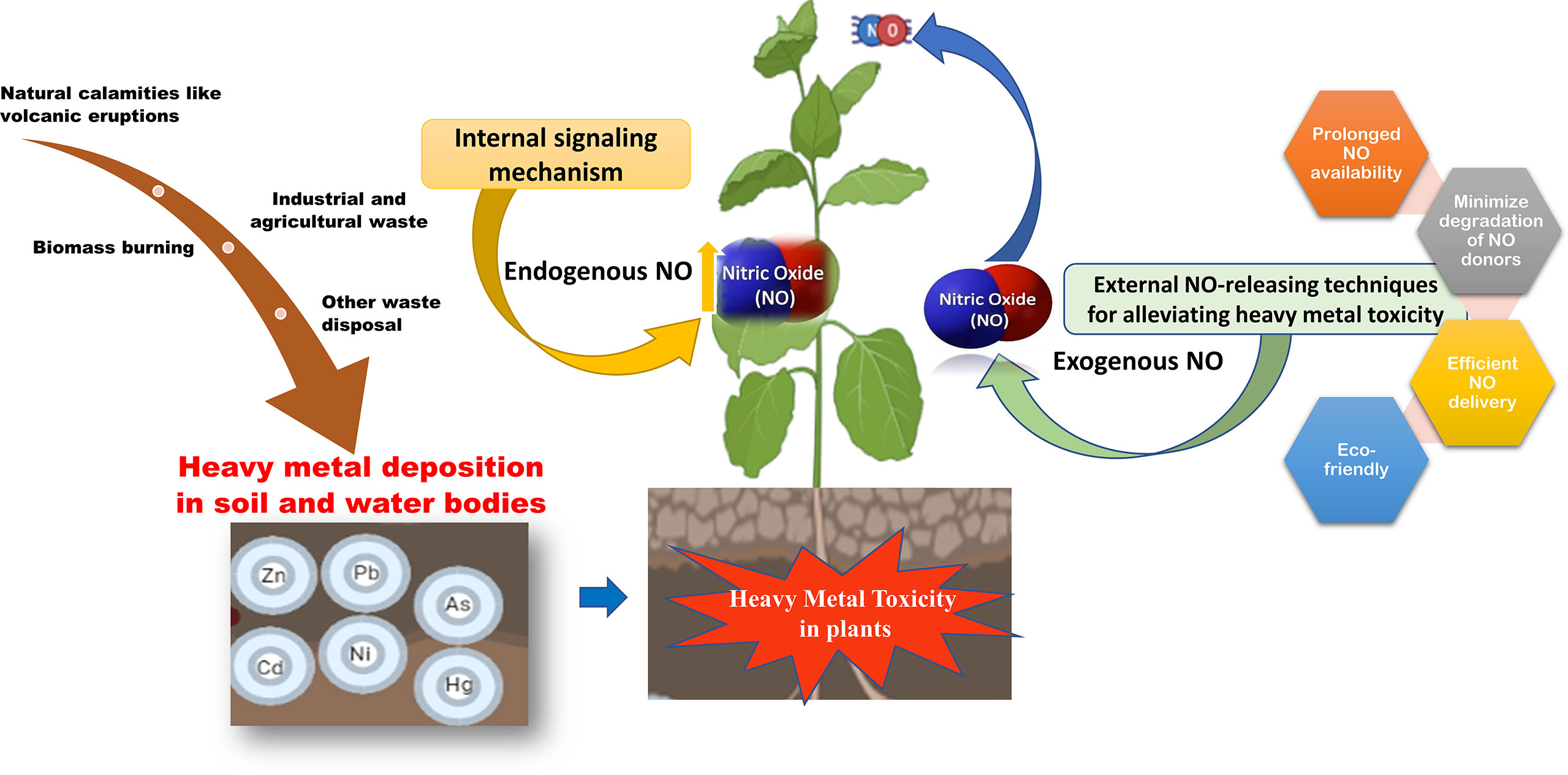
Graphical Abstract Heavy metal toxicity reduces plant growth and productivity. Since traditionally used NO donors do not provide a sustained NO-release, therefore heavy metal toxicity can be mitigated by novel NO-delivery techniques.
Introduction
Industrialization and the increasing human population have led to the exploitation of natural resources for anthropogenic activities leading to ecological imbalance. Heavy metal contamination in soil and water is one of the major examples of such human-centric activities that pose a serious threat to the environment (Chen et al., 2022b). However, natural phenomenon like volcanic eruptions and weathering of rocks also contributes to the contamination of soil and water bodies with heavy metals. Contamination of heavy metals in agricultural soil leads to decreased growth and productivity of crops and their bioaccumulation in crops poses serious health hazards as they enter the food chain (Emurotu and Onianwa, 2017). Due to their persistent, bioaccumulative, and toxic nature, these are known as major environmental pollutants (Tchounwou et al., 2012).
Recently, agricultural lands contaminated with heavy metals have gained much attention because of their detrimental effect on the agro-ecosystem. Any adverse effect on the agro-ecosystem directly affects various active and dynamic physical, chemical, and biological activities involved in plant growth and productivity. A principal consequence of heavy metal toxicity in plants is the overproduction of reactive oxygen species (ROS) leading to oxidative stress (Romero-Puertas et al., 2019). However, certain heavy metals like Cadmium (Cd) may not induce ROS production in plants but act as pro-oxidants and suppress the availability of antioxidants (Singh and Shah, 2015; Loix et al., 2017). Thus, heavy metals disturb the equilibrium between the production and scavenging of ROS resulting in oxidative stress (Demecsová and Tamás, 2019). While heavy metals induce the production of ROS, nitric oxide plays an important role in stimulating the antioxidant signaling response in plants, thus alleviating the toxic effects of ROS (Terrón-Camero et al., 2019; Sharma et al., 2020).
Nitric oxide (NO) is a versatile and key molecule known for its role in enhancing plant tolerance to abiotic stresses like drought, salinity, heavy metals, and extreme temperatures (Simontacchi et al., 2015; Begara-Morales et al., 2019; Nabi et al., 2019). Additionally, it plays an important role in various growth and developmental processes in plants such as germination, root development, photomorphogenesis (Corpas and Palma, 2020). Nitric oxide not only activates the antioxidative machinery but also activates the synthesis of phytochelatins which helps plants to cope with the deleterious effects of ROS (Groß et al., 2013). It is also documented that both endogenous and exogenous NO contribute to stress tolerance in plants (Wei et al., 2020). Moreover, the exogenous application of NO (in the form of NO donor) is highly dose-dependent and varies from plant to plant. A detailed account on the dose-dependent application of different NO donors in various plants has been reviewed by Terrón-Camero et al. (2019).
In the last decade, several studies described the use of different NO donors to understand the effects and the mechanism of NO under heavy metal stress in plants (Gill et al., 2013; Singh and Shah, 2014; Imran et al., 2016; Hashem et al., 2018; Li et al., 2019; Piacentini et al., 2020b; Ahmad et al., 2021b). These studies support the notion that the exogenous application of NO donors counterbalances the toxic and detrimental effects of heavy metals on overall plant physiology. Despite the vast application of exogenous chemical NO donors in plants, these are generally unstable and prone to decomposition by high light or temperatures leading to rapid and uncontrolled release of NO which reduces their efficacy (Seabra and Durán, 2010; Seabra et al., 2015). Furthermore, all the NO donors have different molecular weights, different rates of absorption by the plants, and different rates of NO release in planta. In addition, some of these NO donors are known to release toxic byproducts along with NO. For example, Sodium Nitroprusside (SNP) is known to release cyanide once absorbed by the plants (Norris and Hume, 1987). Therefore, efforts have been made in developing biomaterials that can release NO in a controlled, efficient, and bio-safe manner. In this context, nanotechnology offers major advantages like easy and efficient encapsulation for better storage and controlled release of such chemicals to the targeted sites.
Recently, the application of nano-NO donors as potential alternatives to chemical NO donors has gained much attention. Studies have reported that the application of nanomaterials enhances the level of endogenous NO, promotes growth, and mitigates environmental stress in plants (Oliveira et al., 2016). Evaluation of the potential of nanoparticles as NO donors have recently begun for agricultural and biomedical purposes. This review aims at discussing the potential advantages of NO-releasing nanomaterials in plants and their usefulness in mitigating heavy metal stress in plants. The review also offers thoughtful insights on the prospects of applying other NO delivery platforms that are so far used in biomedical research but may be useful in plant science as well.
Heavy metal toxicity and the role of nitric oxide in mitigating heavy metal stress in plants
Heavy metals are serious environmental pollutants due to their acute and chronic toxic effects and widespread occurrence. The most hazardous heavy metals and metalloids in the environment include chromium (Cr), Nickel (Ni), Copper (Cu), Zinc (Zn), cadmium (Cd), Lead (Pb), Mercury (Hg), and Arsenic (As). The toxicity caused by these heavy metals on living organisms depends on the dose and duration of exposure (Chen et al., 2022a). However, certain heavy metals like cd, Pb, and Hg may be toxic even at very low concentrations. Heavy metal toxicity in plants leads to several physiological and morphological changes, responsible for the decline in growth (Chen et al., 2022a). For instance, plants exposed to cadmium showed reduced water and nutrient uptake and a decline in the rate of photosynthesis along with other morphological symptoms like chlorosis, inhibition of growth and browning of root tips that ultimately lead to cell death (Wojcik and Tukiendorf, 2004; Mohanpuria et al., 2007). It has been reported that heavy metals can lead to oxidative deterioration of biological molecules causing DNA fragmentation, lipid peroxidation, and protein oxidation. They can alter the content of antioxidants and may change the antioxidative enzyme activity (Sharma and Dietz, 2009).
The role of nitric oxide in mitigating the toxicity induced by heavy metals is well known and thoroughly studied. Nitric oxide (NO) is a key molecule involved in several physiological and biochemical processes in plants. NO is involved in root hair development (Lombardo et al., 2006), enabling plant-microbe interaction during nitrogen fixation (Pande et al., 2021), regulating a balance between auxin and reactive oxygen intermediates (Yu et al., 2014), and for maintaining iron homeostasis (Graziano and Lamattina, 2007). NO also plays a vital role in enhancing the immune response (Tada et al., 2008; Wang et al., 2009) and hypersensitive cell death response (Romero-Puertas et al., 2007; Yun et al., 2011). As a signaling molecule, NO plays a protective role in alleviating abiotic stress conditions (Zhang et al., 2007; Cantrel et al., 2011) including mitigation of heavy metal toxicity in plants (Singh et al., 2016; Nabi et al., 2019; Wei et al., 2020) as shown in Figure 1. Most of the studies on heavy metal toxicity in plants indicate that NO reduces the ROS levels by enhancing the levels of antioxidative enzymes (Wang et al., 2010b; Nabi et al., 2019; Terrón-Camero et al., 2019). During heavy metal stress, NO regulates the excessive production of ROS by forming less stable peroxynitrite from the superoxide radical (O2.-) (Groß et al., 2013). Moreover, NO also regulates the antioxidant enzyme activity in the cell to control the ROS levels during heavy metal stress (Begara-Morales et al., 2019; Khator et al., 2021). Accumulation of NO also leads to the reduction of heavy metal uptake by metal transporters in the roots (Zhao et al., 2013; Singh et al., 2016).
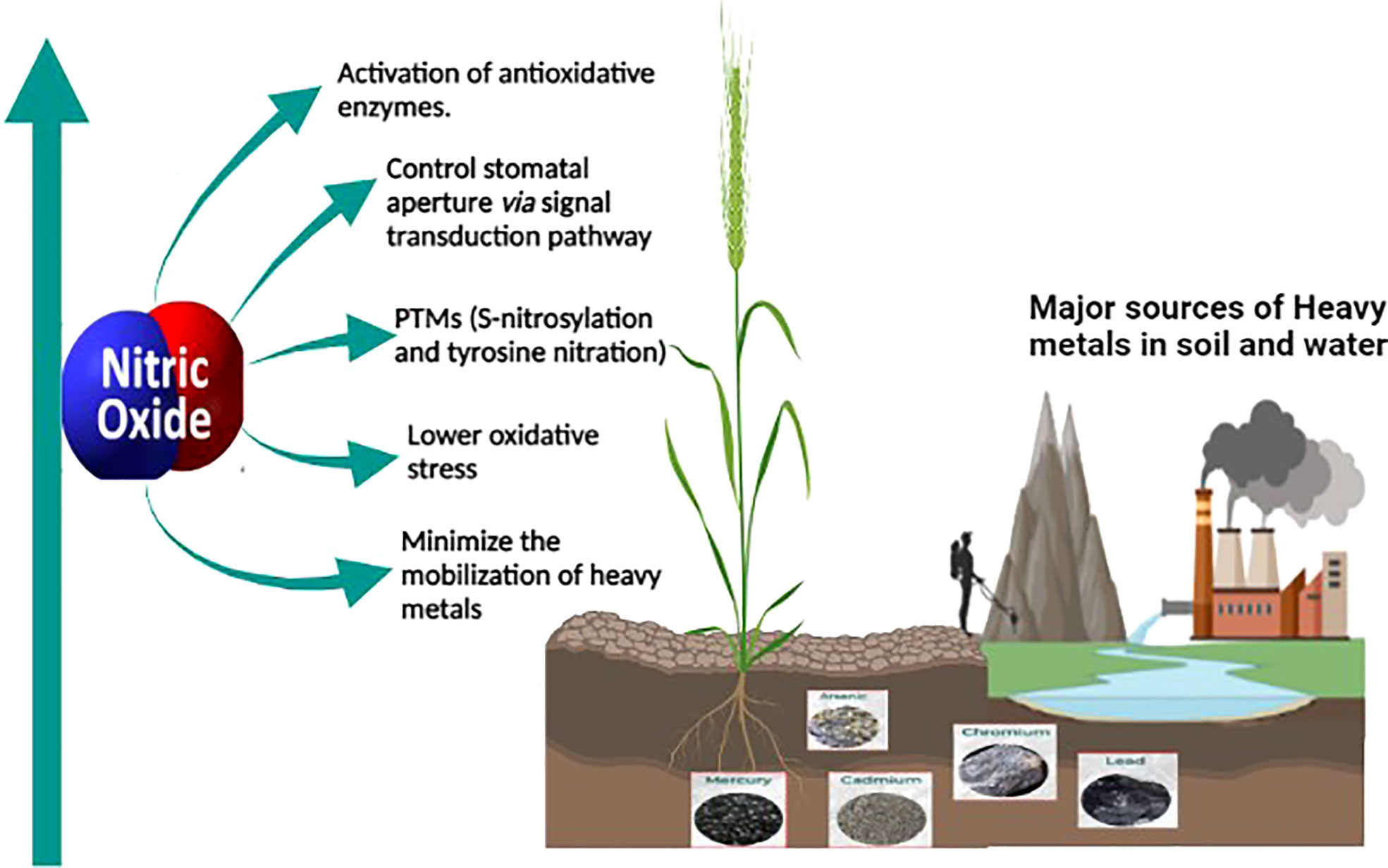
Figure 1 Sources of heavy metal contamination in agricultural land and the role of NO in mitigating heavy metal toxicity in plants. Anthropogenic activities such as industrialization and mining leads to heavy metal contamination in agricultural soil. The oxidative stress caused by the heavy metal toxicity is alleviated by endogenous or exogenously supplied nitric oxide which alleviates it. Nitric oxide is a versatile signaling molecule activating the antioxidative enzymes, controlling stomatal aperture or modifying important proteins through post-translational modification, minimizing mobilization of heavy metals through enhancing the phytochelatins, and thus reducing the toxicity caused by heavy metals.
Other studies on heavy metal toxicity suggested the role of nitric oxide in controlling the stomatal aperture (Nabi et al., 2019), in modifying proteins through S-nitrosylation or tyrosine nitration (Saxena and Shekhawat, 2013), and also in minimizing the mobility of heavy metals by enhancing the expression of phytochelatins in plants and thus reducing the heavy metal toxicity in plants (Groß et al., 2013).
Nitric oxide interacts with different biomolecules like phytohormones in response to heavy metal stress in plants. Nitric oxide regulates phytohormonal levels under heavy metal stress conditions. NO is suggested to reduce AsIII toxicity by regulating Jasmonic acid biosynthesis (Singh et al., 2017). It also increases the levels of indole acetic acid (IAA), cytokinins and gibberellic acid while decreasing the levels of ABA in order to lower lead (Pb) uptake and transport (Sadeghipour, 2017).
The interaction between NO and phytohormones is mainly influenced by NO-mediated post-translational modifications (PTMs) under basal as well as induced conditions (Terrile et al., 2012). Protein S-nitrosylation is the most prominent and widely studied PTM among others. It is the selective but reversible redox-based covalent addition of a NO moiety to the sulfhydryl group of cysteine (Cys) molecule(s) on a target protein to form S-nitrosothiols. Our group has recently reviewed a detailed account of phytohormonal regulation through S-nitrosylation under stress (Pande et al., 2022). However, in case of heavy metal stress this is still a potential and important line of inquiry in future.
NO donors used for alleviating heavy metal toxicity in plants
Exogenous application of NO is most commonly done by supplementing NO donors. Direct application of exogenous nitric oxide to plants is difficult due to its gaseous nature and requires specific equipment (Rodríguez-Ruiz et al., 2017). Moreover, a short half-life (<6 s) of NO makes it difficult to be supplied constantly at the tissue level (Seabra et al., 2015). Therefore, NO is mainly delivered through donor molecules (Wang et al., 2005; Barraud et al., 2009). The commonly used NO donors include SNP, diethylenetriamine NONOate (DETA NONOate), S-nitroso N-acetyl-DL-penicillamine (SNAP), diethylenetriamine/nitric oxide (DETANO), S-nitrosothiols (RSNO), S-nitrosocysteine (CysNO) and S-nitrosoglutathione (GSNO). Recently, a study reported the synthesis and application of N-nitrosomelatonin (NOMela) as a more efficient NO donor than GSNO in Arabidopsis seedlings (Singh et al., 2021a). However, specifically for heavy metal stress tolerance the most commonly used NO donor is SNP (Bothof et al., 2020), treated alone or in combination with other stress ameliorating agents as shown in Table 1.
However, due to the relatively unstable nature and susceptibility to decomposition by heat or light, the release of NO is uncontrolled, resulting in unpredictable and random signaling and other physiological effects (Seabra et al., 2015). This problem may be overcome by encapsulating the NO donor molecules of slow and consistent release. Therefore, NO-releasing nanoparticles may be considered as potential alternatives to unstable chemical NO donors.
Nanoparticles used for alleviating heavy metal toxicity in plants
Nanotechnological interventions in the field of agriculture have paved a way for attaining the long-term goal of sustainable agriculture by improving plant health and productivity under varying environmental conditions (Pande and Arora, 2019). The application and use of nanomaterials not only enhance plant growth and productivity but also help in mitigating biotic and abiotic stress in plants (Arora et al., 2012; Nayan et al., 2016; Bhatt et al., 2020; Zhou et al., 2021). Nanoparticles offer various advantages as compared to their macro counterparts, these include higher surface activity (more surface area available for reaction), enhanced catalytic efficiency, and unique optical and magnetic properties (Wang et al., 2019). Such unique properties add specialized functions to the nanoparticles making them effective in repairing the damage by soil remediation (Liu et al., 2021). The metal adsorption property of magnetite nanoparticles has been found to lower the accumulation of Cd and Na in rice plants (Sebastian et al., 2019). Nanoparticles also influence the formation of apoplastic barriers which suppresses the accumulation of heavy metals in the soil (Rossi et al., 2017). Nanoparticles are useful in mitigating heavy metals in various ways. For instance, they prevent the translocation of heavy metals by forming complexes with them which leads to their immobilization at inactive sites like vacuoles (Wang et al., 2021). These complexes also get adsorbed on the cell surfaces, restricting their movement and biological activity (Cui et al., 2017; Wang et al., 2021). Activation of enzymatic (superoxide dismutase (SOD), catalase (Cantrel et al., 2011), ascorbate peroxidase (APX), glutathione reductase (GR), glutathione peroxidase (GPX), and peroxidase (POD), and non-enzymatic (such as vitamin C, vitamin E, and polyphenols) anti-oxidative defense system is another strategy to cope with the toxicity caused by heavy metals (Zhou et al., 2021). However, a more effective strategy would be to combine the properties of nanoparticles with NO donors as this will have a more profound effect in combating heavy metal stress. In this context, the characteristics properties of nanoparticles like high permeability, film-forming ability, prolonged contact with the active ingredient, and high diffusion would add to the characteristic properties of NO donors for double protection against heavy metal stress.
Nitric-oxide releasing nanoparticles as potential alternatives to chemical NO donors in alleviating heavy metal toxicity in plants
The limitations associated with NO donors have led to the development of new biomaterials for the controlled and prolonged release of NO into biological systems including plants (Kim et al., 2014; Seabra et al., 2014). Evaluation of NO-releasing nanoparticles has recently begun as an alternative to chemical NO donors for various biomedical and agricultural purposes (Lopes-Oliveira et al., 2019; Ma et al., 2019; Liang et al., 2020; Pieretti and Seabra, 2020; Pelegrino et al., 2020; Pieroni, 2020; Ahmad et al., 2021a). Some of the NO-releasing nanoparticles used in biomedical research have been listed in Table 2 with their various applications in different plants.
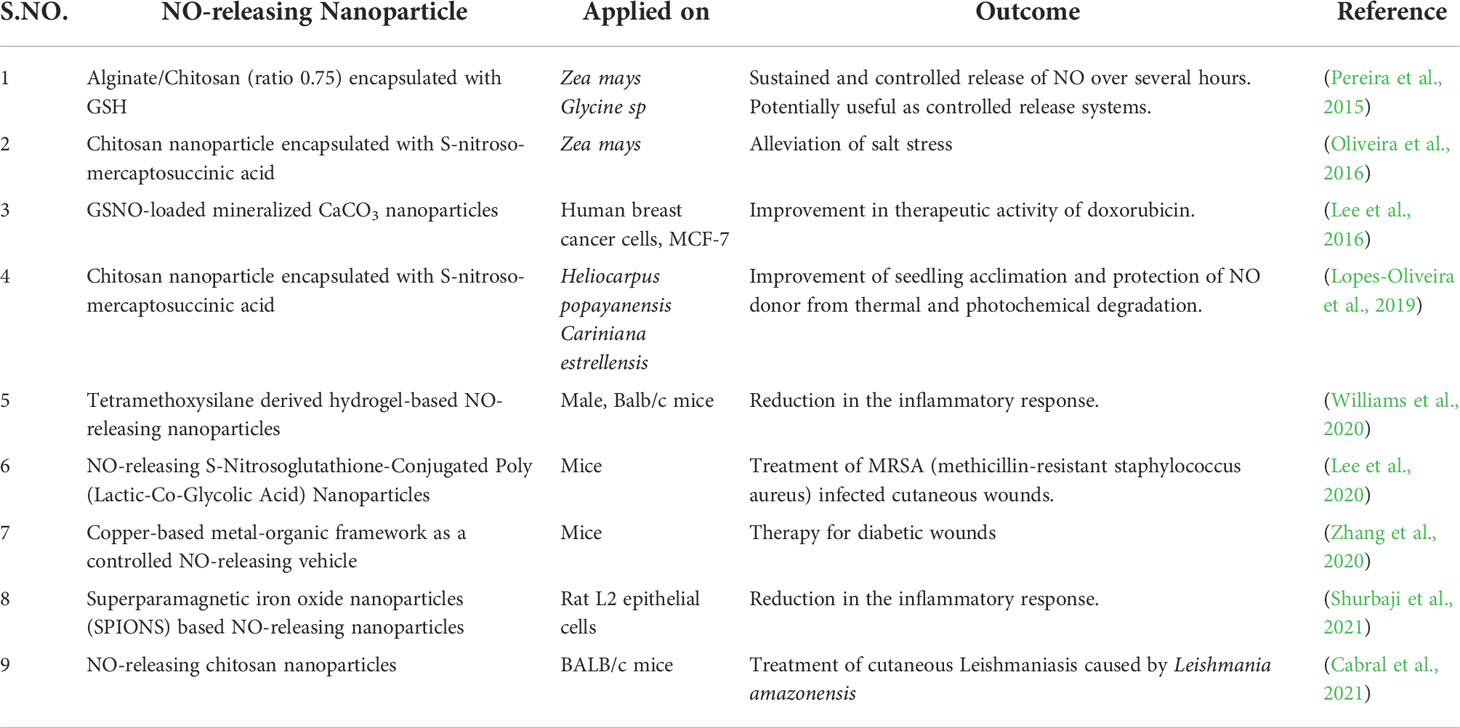
Table 2 Recent examples of the advances in the applications of NO-releasing nanoparticles in agriculture and biomedical research.
A recent study reported the effect of free and nanoencapsulated nitric oxide donor, S-nitroso-mercaptosuccinic acid on neotropical tree seedlings, under field conditions. In this study, the donor molecule was coated with chitosan nanoparticles which protected the molecule from thermal and photochemical degradation. Their study suggested that depending on the tree species, seedling acclimation in the nursery was improved using these nanoencapsulated NO donors (Lopes-Oliveira et al., 2019). Besides, delivering NO is considered a promising approach in biomedical research and applications. The biomedical applications of NO-releasing nanoparticles suggest their importance and also provides insights into their adequacy in plant system as well. Therefore, based on the scientific knowledge we report that the application of NO-releasing nanoparticles is a useful approach to alleviate the detrimental effects of heavy metal stress on plants. The combined effects of nanoparticles and the stress mitigating properties of NO may provide an advantageous approach for combating heavy metal stress in plants. Figure 2, demonstrates the advantages of NO-releasing nanoparticles over chemical NO donors in alleviating heavy metal toxicity in plants.
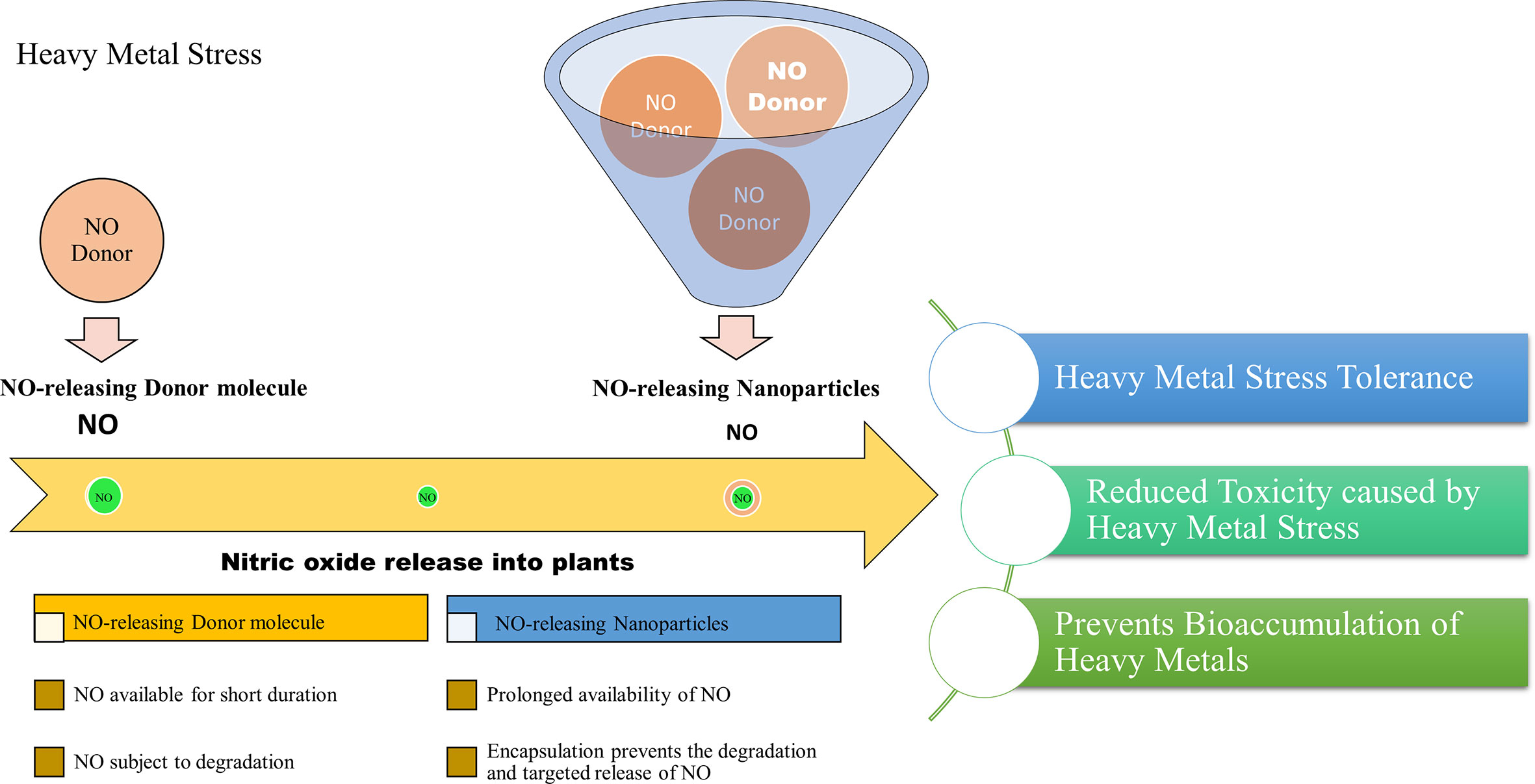
Figure 2 Projected advantages of NO-releasing nanoparticles in alleviating heavy metal toxicity in plants.
Recent studies have synthesized NO-releasing nanoparticles for studying their effect in plants. NO-releasing nanoparticles are formulated by the addition of NO donor molecules in chitosan nanoparticles which encapsulates the NO donor for slow and prolonged release (Oliveira et al., 2016; Pelegrino et al., 2017a; Pelegrino et al., 2017b; Cabral et al., 2019). These have also been tested for their efficacy in mitigating environmental stress in different plants (Oliveira et al., 2016; Lopes-Oliveira et al., 2019).
Other NO-releasing techniques used in biomedical research as alternate potential strategies for efficient NO-release in plants
NO is an important signaling molecule that has a significant role in biomedical research. In mammalian tissues, the kinetics and exposure time of NO are key determinants in its biological applications. However, its therapeutic applications are limited due to its extremely short half-life, aimless diffusion into the vasculature, and limited accumulation in the target tissues (Wang et al., 2020).
Traditionally, NO donors were used for NO-release in biomedical sciences which included various types of nitrates, N-diazeniumdiolates, Nitrosothiols, Furoxans, Metal nitrosyl compounds, and Nitrobenzenes (Yang et al., 2021). Nanotechnology has been recently employed for the delivery of NO. In such cases, the nanomaterial is usually degraded once absorbed into the system thereby releasing NO gas through NO donor. Though several different nanotech-based NO delivery platforms have been developed, some interesting studies carry significant potential for application in plant sciences. For example, Lee et al. (2016) described the pH-sensitive release of NO by CaCO3 mineralized nanoparticles. This is specifically important in plant sciences as plant growth under basal conditions and soil-related stress conditions such as heavy metal stress, and salinity is significantly related to the pH of the soil. Therefore, such nanoparticle carriers can be engineered to release NO or NO donors at a specific soil pH or under a range of pH conditions. Jia et al. (2018) developed a redox-active nanosilicon-NO donor system that released NO only in response to over-accumulated GSH in tumor tissues. The same concept can be employed in plant sciences by developing nanomaterial-NO donor systems that release NO only in response to over-accumulated chemicals such as salts, heavy metal ions, phytohormones, secondary metabolites or other chemicals/ions in plant tissues. In addition, biomedical researchers developed nanomaterial-NO donors triggered by external cues such as light, heat, X-rays and ultrasound (Fan et al., 2015; Guo et al., 2017; Jin et al., 2017; Hotta et al., 2020; Zhou et al., 2020). Although their clinical applications are limited in mammals, we believe that these may prove to be highly useful in plant sciences. Sunlight is mandatory for photosynthesis. However, intense light for a longer duration and/or certain wavelengths of light are harmful to plant growth. Similarly, intense heat also limits plant growth and development. Nanomaterial-NO delivery systems that release NO under specific light and temperature conditions can be designed for target NO delivery. An alternate biomedical study also suggests the use of nanostructured CuO/SiO2 catalysts for releasing NO by the catalytic decomposition of NO-releasing metabolites like GSNO (Kulyk et al., 2020). However, these nanoparticles are suggested to be useful in medical applications and their possibilities and applications need to be explored for agriculture purposes.
Besides, NO donor-conjugated chemical drugs were also designed with more sophisticated NO linkage, release position, selectivity, and amount of NO release. Chen et al. (2008) designed and synthesized multiple NO-releasing derivatives of oleanolic acid (NO-OA) with anti-hepatocellular carcinoma activity. To our knowledge, such types of conjugated NO-donors have not been tested in plants. Moreover, NO release strategies are mostly limited to a few NO donors only (as mentioned in the previous sections) therefore these can be utilized and tested in crop research as well.
The development of targeted prodrugs for gases like NO has been a special challenge in biomedical sciences that carries great prospects. Prodrugs are medications that, after administration, are metabolized and converted into a pharmacologically active drug within the body. Specific enzymes can activate NO prodrugs and release NO gas at specific sites, greatly reducing the side effects. Such types of targeted NO prodrugs developed so far are activated by glycosidases (Wu et al., 2001; Cai et al., 2004), cytochrome enzymes (Saavedra et al., 1997), oxidoreductases (Sharma et al., 2013a), esterases (Saavedra et al., 2000) and reductase enzymes (Sharma et al., 2013b) that trigger the release of NO. Interestingly, plants express a plethora of all these different enzymes in various types of tissues offering the possibility of using NO prodrugs in plant sciences.
Similarly, drug delivery systems based on monoclonal antibodies also offer high target specificity in mammals. Antibody/peptides conjugated NO donors have been widely used in cancer treatment (Sievers and Senter, 2013; Chari et al., 2014; Sun et al., 2019) offering significantly higher specificity and release of NO following the detection of target cells only (such as cancer cells) by the monoclonal antibodies. NO donors conjugated to monoclonal antibodies can be engineered for the targeted, specific, and safe release of NO in plant systems under various circumstances. Such monoclonal antibodies can be tailored to recognize specific fungal, bacterial, and viral peptides (during infection), receptor proteins for various phytohormones (for regulating plant development and responses to various abiotic stresses), and several other peptides with spatial and temporal expression profiles; for the targeted delivery of NO in plant systems. Figure 3 summarize the suggested NO-releasing techniques for potential application in plants.
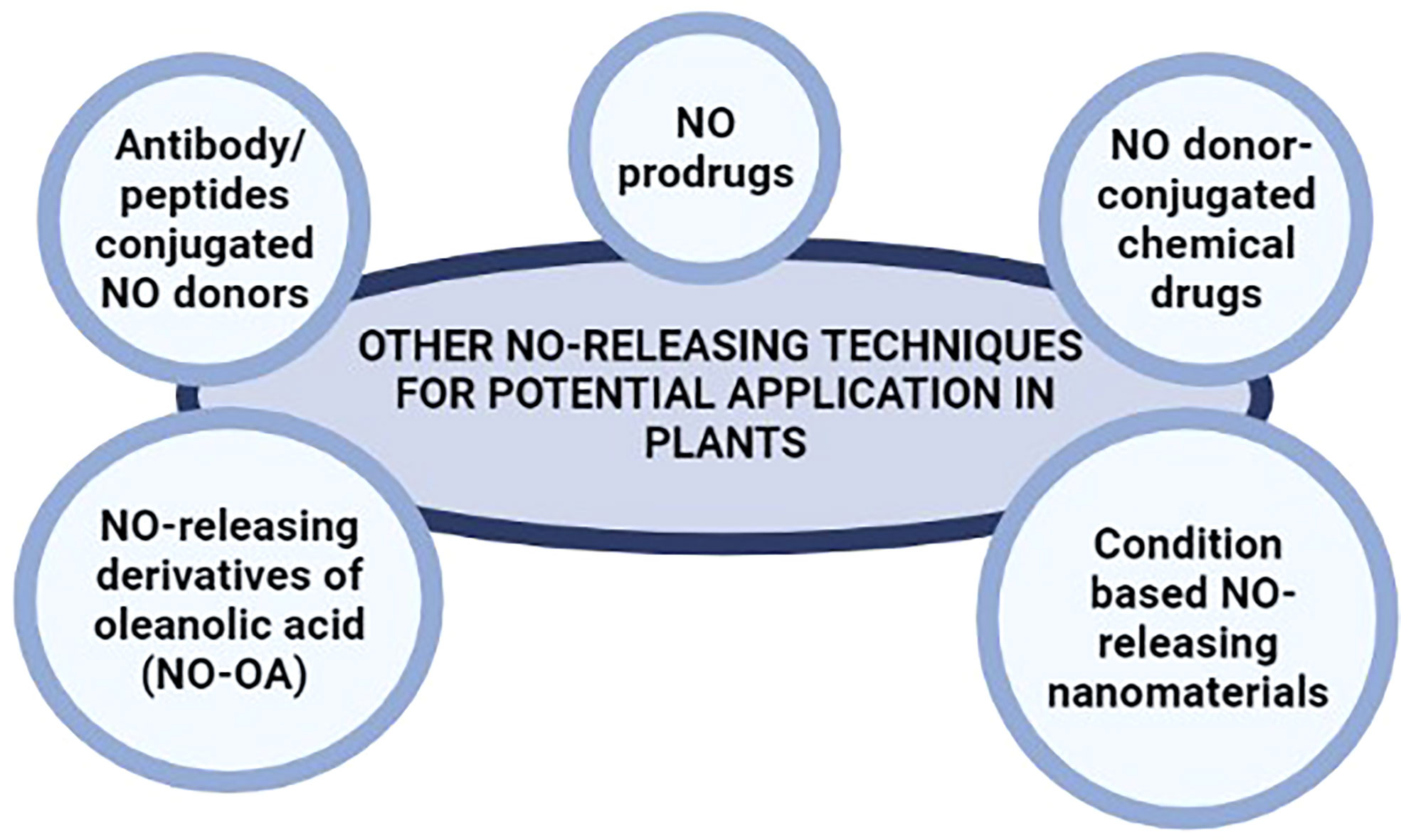
Figure 3 NO-releasing techniques used in biomedical research that may be potential alternatives to NO donors used in plant.
Conclusions and future prospects
Heavy metals occur naturally in the earth’s crust. They are often needed in very small amounts to carry out essential role in the metabolic systems of living organisms. However, natural calamities like weathering of rocks and volcanic eruptions, and other anthropogenic activities like mining and industrialization have largely overwhelmed their natural geochemical cycles (Nriagu and Pacyna, 1988). As a result, their concentration has increased in agricultural lands which negatively affects the growth and productivity of crops. To make things worse the application of chemical fertilizers containing heavy metals has further deteriorated the soil profile of agriculturally useful lands (Curtis and Smith, 2002).
Exposure of plant roots to heavy metals like cadmium (Cd), arsenic (As), lead (Pb), and copper (Cu), enhances endogenous levels of NO. NO is involved in various physiological and biochemical processes in plants that ensure optimal growth and development of plants exposed to various environmental stress conditions. Exogenous application of NO in the form of NO donors has been reported to lower oxidative stress by enhancing the activity of antioxidative enzymes under various environmental stress conditions. However, the use of NO donors is disadvantageous due to the short half-life of NO and its degradation by heat and light. A potential strategy is to use nanoparticles as encapsulating agents to effectively release NO in the plants for their optimal growth in heavy metal contaminated soils. Furthermore, to alleviate the toxicity caused by heavy metals in plants, NO needs to be delivered efficiently and for a prolonged duration. Therefore, conjugating them with the right nanoparticle is an important consideration. In this context, chitosan nanoparticles are suggested to be the most suitable candidates for this purpose owing to their unique properties such as biodegradable and biocompatible nature. Therefore, these nanoparticles need to be tested for their role in mitigating heavy metal stress in plants to sustain agricultural productivity. In conclusion, any NO-releasing technique that promises prolonged and efficient delivery of NO in an eco-friendly manner has the potential of alleviating heavy metal toxicity in plants.
Author contributions
AP: conceptualization, visualization, writing - original draft. B-GM: project administration. NM: resources. WR: investigation. D-SL: visualization. GML resources. JH: writing - review and editing. AH: writing - review and editing. GL: final review and editing. B-WY: supervision, funding acquisition. All authors contributed to the article and approved the submitted version.
Funding
This research was supported by Basic Science Research Program through the National Research Foundation of Korea (NRF) funded by the Ministry of Education (Grant number 2020R1I1A3073247), Republic of Korea and Korea Basic Science Institute (National Research Facilities and Equipment Center) grant funded by the Ministry of Education (NRF-2021R1A6C101A416).
Acknowledgments
The graphical abstract and Figures 1 and 3 were made in BioRender (BioRender.com).
Conflict of interest
The authors declare that the research was conducted in the absence of any commercial or financial relationships that could be construed as a potential conflict of interest.
Publisher’s note
All claims expressed in this article are solely those of the authors and do not necessarily represent those of their affiliated organizations, or those of the publisher, the editors and the reviewers. Any product that may be evaluated in this article, or claim that may be made by its manufacturer, is not guaranteed or endorsed by the publisher.
References
Abdel-Kader, D. Z. E.-A. (2007). Role of nitric oxide, glutathione and sulfhydryl groups in zinc homeostasis in plants. Am. J. Plant Physiol. 2, 59–75. doi: 10.3923/ajpp.2007.59.75
Ahmad, P., Ahanger, M. A., Alyemeni, M. N., Wijaya, L., Alam, P. (2018). Exogenous application of nitric oxide modulates osmolyte metabolism, antioxidants, enzymes of ascorbate-glutathione cycle and promotes growth under cadmium stress in tomato. Protoplasma. 255, 79–93. doi: 10.1007/s00709-017-1132-x
Ahmad, P., Alam, P., Balawi, T. H., Altalayan, F. H., Ahanger, M. A., Ashraf, M. (2020). Sodium nitroprusside (SNP) improves tolerance to arsenic (As) toxicity in vicia faba through the modifications of biochemical attributes, antioxidants, ascorbate-glutathione cycle and glyoxalase cycle. Chemosphere. 244, 125480. doi: 10.1016/j.chemosphere.2019.125480
Ahmad, P., Alyemeni, M. N., Wijaya, L., Ahanger, M. A., Ashraf, M., Alam, P., et al. (2021b). Nitric oxide donor, sodium nitroprusside, mitigates mercury toxicity in different cultivars of soybean. J. Hazardous Materials. 408, 124852. doi: 10.1016/j.jhazmat.2020.124852
Ahmad, A., Khan, W. U., Ali Shah, A., Yasin, N. A., Naz, S., Ali, A., et al. (2021a). Synergistic effects of nitric oxide and silicon on promoting plant growth, oxidative stress tolerance and reduction of arsenic uptake in brassica juncea. Chemosphere. 262, 128384. doi: 10.1016/j.chemosphere.2020.128384
Amnan, M. A. M., Pua, T.-L., Lau, S.-E., Tan, B. C., Yamaguchi, H., Hitachi, K., et al. (2021). Osmotic stress in banana is relieved by exogenous nitric oxide. PeerJ. 9, e10879. doi: 10.7717/peerj.10879
Arora, S., Sharma, P., Kumar, S., Nayan, R., Khanna, P., Zaidi, M. (2012). Gold-nanoparticle induced enhancement in growth and seed yield of brassica juncea. Plant Growth Regul. 66, 303–310. doi: 10.1007/s10725-011-9649-z
Bai, X. Y., Dong, Y. J., Wang, Q. H., Xu, L. L., Kong, J., Liu, S. (2015). Effects of lead and nitric oxide on photosynthesis, antioxidative ability, and mineral element content of perennial ryegrass. Biol. Plantarum. 59, 163–170. doi: 10.1007/s10535-014-0476-8
Barraud, N., Storey, M. V., Moore, Z. P., Webb, J. S., Rice, S. A., Kjelleberg, S. (2009). Nitric oxide-mediated dispersal in single-and multi-species biofilms of clinically and industrially relevant microorganisms. Microbial Biotechnol. 2, 370–378. doi: 10.1111/j.1751-7915.2009.00098.x
Begara-Morales, J. C., Chaki, M., Valderrama, R., Mata-Pérez, C., Padilla, M. N., Barroso, J. B. (2019). The function of s-nitrosothiols during abiotic stress in plants. J. Exp. Bot. 70, 4429–4439. doi: 10.1093/jxb/erz197
Bhatt, D., Bhatt, M. D., Nath, M., Dudhat, R., Sharma, M., Bisht, D. S. (2020). Application of nanoparticles in overcoming different environmental stresses. Protective Chem. Agents Amelioration Plant Abiotic Stress: Biochem. Mol. Perspect., 635–654. doi: 10.1002/9781119552154.ch32
Bothof, G., Van Rhee, K. P., Koomen, E., Veldhoen, E. S. (2020). Change in national dosing advice of nitroprusside after potentially fatal cyanide intoxication. SN Compr. Clin. Med. 2, 522–525. doi: 10.1007/s42399-020-00261-9
Cabral, F. V., Pelegrino, M. T., Sauter, I. P., Seabra, A. B., Cortez, M., Ribeiro, M. S. (2019). Nitric oxide-loaded chitosan nanoparticles as an innovative antileishmanial platform. Nitric. Oxide. 93, 25–33. doi: 10.1016/j.niox.2019.09.007
Cabral, F. V., Pelegrino, M. T., Seabra, A. B., Ribeiro, M. S. (2021). Nitric-oxide releasing chitosan nanoparticles towards effective treatment of cutaneous leishmaniasis. Nitric. Oxide. 113, 31–38. doi: 10.1016/j.niox.2021.04.008
Cai, T. B., Lu, D., Landerholm, M., Wang, P. G. (2004). Sialated diazeniumdiolate: A new sialidase-activated nitric oxide donor. Organic Lett. 6, 4203–4205. doi: 10.1021/ol048397p
Cantrel, C., Vazquez, T., Puyaubert, J., Rezé, N., Lesch, M., Kaiser, W. M., et al. (2011). Nitric oxide participates in cold-responsive phosphosphingolipid formation and gene expression in arabidopsis thaliana. N. Phytol. 189, 415–427. doi: 10.1111/j.1469-8137.2010.03500.x
Chari, R. V. J., Miller, M. L., Widdison, W. C. (2014). Antibody–drug conjugates: An emerging concept in cancer therapy. Angewandte Chemie In. Ed. 53, 3796–3827. doi: 10.1002/anie.201307628
Chen, L., Beiyuan, J., Hu, W., Zhang, Z., Duan, C., Cui, Q., et al. (2022a). Phytoremediation of potentially toxic elements (PTEs) contaminated soils using alfalfa (Medicago sativa l.): A comprehensive review. Chemosphere. 293, 133577. doi: 10.1016/j.chemosphere.2022.133577
Chen, W., Dong, Y., Hu, G., Bai, X. (2018). Effects of exogenous nitric oxide on cadmium toxicity and antioxidative system in perennial ryegrass. J. Soil Sci. Plant Nutr. 18, 129–143. doi: 10.4067/S0718-95162018005000601
Chen, L., Zhang, Y., Kong, X., Lan, E., Huang, Z., Peng, S., et al. (2008). Design, synthesis, and antihepatocellular carcinoma activity of nitric oxide releasing derivatives of oleanolic acid. J. Medicinal Chem. 51, 4834–4838. doi: 10.1021/jm800167u
Chen, L., Zhou, M., Wang, J., Zhiqin, Z., Chengjiao, D., Xiangxiang, W., et al. (2022b). A global meta-analysis of heavy metal (loid) s pollution in soils near copper mines: Evaluation of pollution level and probabilistic health risks. Sci. Total Environ. 835, 155441. doi: 10.1016/j.scitotenv.2022.155441
Corpas, F. J., Palma, J. M. (2020). Assessing nitric oxide (NO) in higher plants: an outline. Nitrogen. 1, 12–20. doi: 10.3390/nitrogen1010003
Cui, J., Liu, T., Li, F., Yi, J., Liu, C., Yu, H. (2017). Silica nanoparticles alleviate cadmium toxicity in rice cells: Mechanisms and size effects. Environ. Pollut. 228, 363–369. doi: 10.1016/j.envpol.2017.05.014
Cui, X., Zhang, Y., Wu, X., Liu, C. (2010). The investigation of the alleviated effect of copper toxicity by exogenous nitric oxide in tomato plants. Plant Soil Environ. 56, 274–281. doi: 10.17221/98/2009-PSE
Curtis, L. R., Smith, B. W. (2002). Heavy metal in fertilizers: Considerations for setting regulations in Oregon. Oregon: Department of Environmental and Molecular Toxicology Oregon State University Corvallis.
Da-Silva, C. J., Canatto, R. A., Cardoso, A. A., Ribeiro, C., De Oliveira, J. A. (2018). Oxidative stress triggered by arsenic in a tropical macrophyte is alleviated by endogenous and exogenous nitric oxide. Braz. J. Bot. 41, 21–28. doi: 10.1007/s40415-017-0431-y
Demecsová, L., Tamás, L. (2019). Reactive oxygen species, auxin and nitric oxide in metal-stressed roots: Toxicity or defence. BioMetals. 32, 717–744. doi: 10.1007/s10534-019-00214-3
Emurotu, J., Onianwa, P. (2017). Bioaccumulation of heavy metals in soil and selected food crops cultivated in Kogi State, North Central Nigeria. Environ. Syst. Res. 6, 1–9. doi: 10.1186/s40068-017-0098-1
Fan, W., Bu, W., Zhang, Z., Shen, B., Zhang, H., He, Q., et al. (2015). X-Ray radiation-controlled NO-release for on-demand depth-independent hypoxic radiosensitization. Angewandte Chemie In. Ed. 54, 14026–14030. doi: 10.1002/anie.201504536
Faraji, J., Sepehri, A., Salcedo-Reyes, J. C. (2018). Titanium dioxide nanoparticles and sodium nitroprusside alleviate the adverse effects of cadmium stress on germination and seedling growth of wheat (Triticum aestivum l.). Universitas Scientiarum. 23, 61–87. doi: 10.11144/Javeriana.SC23-1.tdna
Gill, S. S., Hasanuzzaman, M., Nahar, K., Macovei, A., Tuteja, N. (2013). Importance of nitric oxide in cadmium stress tolerance in crop plants. Plant Physiol. Biochem. 63, 254–261. doi: 10.1016/j.plaphy.2012.12.001
Graziano, M., Lamattina, L. (2007). Nitric oxide accumulation is required for molecular and physiological responses to iron deficiency in tomato roots. Plant J. 52, 949–960. doi: 10.1111/j.1365-313X.2007.03283.x
Groß, F., Durner, J., Gaupels, F. (2013). Nitric oxide, antioxidants and prooxidants in plant defence responses. Front. Plant Sci. 4, 419. doi: 10.3389/fpls.2013.00419
Guo, R., Tian, Y., Wang, Y., Yang, W. (2017). Near-infrared laser-triggered nitric oxide nanogenerators for the reversal of multidrug resistance in cance. Advanced Functional Mat. 27, 1606398. doi: 10.1002/adfm.201606398
Hamidi, H., Masoudian, N., Ebadi, M., Roudi, B. (2020). Relationship of sodium nitroprusside with growth and antioxidant enzymes of canola under lead stress. J. Chem. Health Risks. 10, 57–65. doi: 10.22034/jchr.2020.1863574.1020
Hasanuzzaman, M., Fujita, M. (2013). Exogenous sodium nitroprusside alleviates arsenic-induced oxidative stress in wheat (Triticum aestivum l.) Seedlings by enhancing antioxidant defense and glyoxalase system. Ecotoxicology. 22, 584–596. doi: 10.1007/s10646-013-1050-4
Hashem, H. A., Hassanein, A. A., Esmail, N. Y. (2018). Nitric oxide enhances the adaptive responses of lupine plants against heavy-metal stress. Aust. J. Crop Sci. 12, 1962. doi: 10.21475/ajcs.18.12.12.p1379
Hassanein, A., Esmail, N., Hashem, H. (2020). Sodium nitroprusside mitigates the inhibitory effect of salt and heavy metal stress on lupine yield and downregulates antioxidant enzyme activities. Acta Agrobotanica. 73, 7336–7351. doi: 10.5586/aa.7336
He, H., Oo, T. L., Huang, W., He, L.-F., Gu, M. (2019). Nitric oxide acts as an antioxidant and inhibits programmed cell death induced by aluminum in the root tips of peanut (Arachis hypogaea l.). Sci. Rep. 9, 9516. doi: 10.1038/s41598-019-46036-8
Hotta, Y., Kataoka, T., Mori, T., Kimura, K. (2020). Review of a potential novel approach for erectile dysfunction: Light-controllable nitric oxide donors and nanoformulations. Sex Med. Rev. 8, 297–302. doi: 10.1016/j.sxmr.2019.05.006
Hsu, Y. T., Kao, C. H. (2004). Cadmium toxicity is reduced by nitric oxide in rice leaves. Plant Growth Regul. 42, 227–238. doi: 10.1023/B:GROW.0000026514.98385.5c
Imran, Q. M., Falak, N., Hussain, A., Mun, B.-G., Sharma, A., Lee, S.-U., et al. (2016). Nitric oxide responsive heavy metal-associated gene AtHMAD1 contributes to development and disease resistance in arabidopsis thaliana. Front. Plant Sci. 7, 1712>. doi: 10.3389/fpls.2016.01712
Jia, X., Zhang, Y., Zou, Y., Wang, Y., Niu, D., He, Q., et al. (2018). Dual intratumoral Redox/Enzyme-responsive NO-releasing nanomedicine for the specific, high-efficacy, and low-toxic cancer therapy. Advanced Mats. 30, 1704490. doi: 10.1002/adma.201704490
Jin, Z., Wen, Y., Hu, Y., Chen, W., Zheng, X., Guo, W., et al. (2017). MRI-Guided and ultrasound-triggered release of NO by advanced nanomedicine. Nanoscale. 9, 3637–3645. doi: 10.1039/C7NR00231A
Kaur, G., Singh, H. P., Batish, D. R., Mahajan, P., Kohli, R. K., Rishi, V. (2015). Exogenous nitric oxide (NO) interferes with lead (Pb)-induced toxicity by detoxifying reactive oxygen species in hydroponically grown wheat (Triticum aestivum) roots. PloS One. 10, e0138713. doi: 10.1371/journal.pone.0138713
Kaya, C., Akram, N. A., Sürücü, A., Ashraf, M. (2019). Alleviating effect of nitric oxide on oxidative stress and antioxidant defence system in pepper (Capsicum annuum l.) plants exposed to cadmium and lead toxicity applied separately or in combination. Scientia Hortic. 255, 52–60. doi: 10.1016/j.scienta.2019.05.029
Khator, K., Saxena, I., Shekhawat, G. S. (2021). Nitric oxide induced cd tolerance and phytoremediation potential of b. juncea by the modulation of antioxidant defense system and ROS detoxification. Biometals. 34, 15–32. doi: 10.1007/s10534-020-00259-9
Kim, J., Saravanakumar, G., Choi, H. W., Park, D., Kim, W. J. (2014). A platform for nitric oxide delivery. J. Materials Chem. B. 2, 341–356. doi: 10.1039/C3TB21259A
Kopyra, M., Gwóźdź, E. A. (2003). Nitric oxide stimulates seed germination and counteracts the inhibitory effect of heavy metals and salinity on root growth of lupinus luteus. Plant Physiol. Biochem. 41, 1011–1017. doi: 10.1016/j.plaphy.2003.09.003
Kotapati, K. V., Palaka, B. K., Ampasala, D. R. (2017). Alleviation of nickel toxicity in finger millet (Eleusine coracana l.) germinating seedlings by exogenous application of salicylic acid and nitric oxide. Crop J. 5, 240–250. doi: 10.1016/j.cj.2016.09.002
Kulyk, K., Azizova, L., Cunningham, J. M., Mikhalovska, L., Borysenko, M., Mikhalovsky, S. (2020). Nanosized copper(ii) oxide/silica for catalytic generation of nitric oxide from s-. nitrosothiols. J. Materials Chem. B 8, 4267–4277. doi: 10.1039/D0TB00137F
Kumari, A., Sheokand, S., Swaraj, K. (2010). Nitric oxide induced alleviation of toxic effects of short term and long term cd stress on growth, oxidative metabolism and cd accumulation in chickpea. Braz. J. Plant Physiol. 22, 271–284. doi: 10.1590/S1677-04202010000400007
Laspina, N., Groppa, M., Tomaro, M., Benavides, M. (2005). Nitric oxide protects sunflower leaves against cd-induced oxidative stress. Plant Sci. 169, 323–330. doi: 10.1016/j.plantsci.2005.02.007
Lee, H. J., Kim Da, E., Park, D. J., Choi, G. H., Yang, D. N., Heo, J. S., et al. (2016). pH-responsive mineralized nanoparticles as stable nanocarriers for intracellular nitric oxide delivery. Colloids Surf B Biointerfaces. 146, 1–8. doi: 10.1016/j.colsurfb.2016.05.039
Lee, J., Kwak, D., Kim, H., Kim, J., Hlaing, S. P., Hasan, N., et al. (2020). Nitric oxide-releasing s-Nitrosoglutathione-Conjugated Poly(Lactic-Co-Glycolic acid) nanoparticles for the treatment of MRSA-infected cutaneous wounds. Pharmaceutics. 12, 618–631. doi: 10.3390/pharmaceutics12070618
Liang, H., Li, Z., Ren, Z., Jia, Q., Guo, L., Li, S., et al. (2020). Light-triggered NO-releasing nanoparticles for treating mice with liver fibrosis. Nano Res. 13, 2197–2202. doi: 10.1007/s12274-020-2833-6
Li, S.-W., Li, Y., Leng, Y., Zeng, X.-Y., Ma, Y.-H. (2019). Nitric oxide donor improves adventitious rooting in mung bean hypocotyl cuttings exposed to cadmium and osmotic stresses. Environ. Exp. Bot. 164, 114–123. doi: 10.1016/j.envexpbot.2019.05.004
Liu, Y., Wu, T., White, J. C., Lin, D. (2021). A new strategy using nanoscale zero-valent iron to simultaneously promote remediation and safe crop production in contaminated soil. Nat. Nanotechnology. 16, 197–205. doi: 10.1038/s41565-020-00803-1
Loix, C., Huybrechts, M., Vangronsveld, J., Gielen, M., Keunen, E., Cuypers, A. (2017). Reciprocal interactions between cadmium-induced cell wall responses and oxidative stress in plants. Front. Plant Sci. 8, 1867. doi: 10.3389/fpls.2017.01867
Lombardo, M. C., Graziano, M., Polacco, J. C., Lamattina, L. (2006). Nitric oxide functions as a positive regulator of root hair development. Plant Signaling Behav. 1, 28–33. doi: 10.4161/psb.1.1.2398
Lopes-Oliveira, P. J., Gomes, D. G., Pelegrino, M. T., Bianchini, E., Pimenta, J. A., Stolf-Moreira, R., et al. (2019). Effects of nitric oxide-releasing nanoparticles on neotropical tree seedlings submitted to acclimation under full sun in the nursery. Sci. Rep. 9, 17371. doi: 10.1038/s41598-019-54030-3
Ma, Y., Fu, L., Hussain, Z., Huang, D., Zhu, S. (2019). Enhancement of storability and antioxidant systems of sweet cherry fruit by nitric oxide-releasing chitosan nanoparticles (GSNO-CS NPs). Food Chem. 285, 10–21. doi: 10.1016/j.foodchem.2019.01.156
Mohanpuria, P., Rana, N. K., Yadav, S. K. (2007). Cadmium induced oxidative stress influence on glutathione metabolic genes of camellia sinensis (L.) o. kuntze. Environ. Toxicology: Int. J. 22, 368–374. doi: 10.1002/tox.20273
Mostofa, M. G., Seraj, Z. I., Fujita, M. (2014). Exogenous sodium nitroprusside and glutathione alleviate copper toxicity by reducing copper uptake and oxidative damage in rice (Oryza sativa l.) seedlings. Protoplasma. 251, 1373–1386. doi: 10.1007/s00709-014-0639-7
Mostofa, M. G., Seraj, Z. I., Fujita, M. (2015). Interactive effects of nitric oxide and glutathione in mitigating copper toxicity of rice (Oryza sativa l.) seedlings. Plant Signaling Behav. 10, e991570. doi: 10.4161/15592324.2014.991570
Nabaei, M., Amooaghaie, R. (2019). Interactive effect of melatonin and sodium nitroprusside on seed germination and seedling growth of catharanthus roseus under cadmium stress. Russian J. Plant Physiol. 66, 128–139. doi: 10.1134/S1021443719010126
Nabi, R. B. S., Tayade, R., Hussain, A., Kulkarni, K. P., Imran, Q. M., Mun, B.-G., et al. (2019). Nitric oxide regulates plant responses to drought, salinity, and heavy metal stress. Environ. Exp. Bot. 161, 120–133. doi: 10.1016/j.envexpbot.2019.02.003
Namdjoyan, S., Kermanian, H. (2013). Exogenous nitric oxide (as sodium nitroprusside) ameliorates arsenic-induced oxidative stress in watercress (Nasturtium officinale r. br.) plants. Scientia Hortic. 161, 350–356. doi: 10.1016/j.scienta.2013.07.035
Namdjoyan, S., Kermanian, H., Soorki, A. A., Tabatabaei, S. M., Elyasi, N. (2018). Effects of exogenous salicylic acid and sodium nitroprusside on α-tocopherol and phytochelatin biosynthesis in zinc-stressed safflower plants. Turkish J. Bot. 42, 271–279. doi: 10.3906/bot-1707-48
Naseem, M., Anwar-Ul-Haq, M., Akhtar, J., Jaskani, M. J. (2020). Effect of exogenous application of salicylic acid and sodium nitroprusside on maize under selenium stress. Pakistan J. Agric. Sci. 57, 150–158. doi: 10.21162/PAKJAS/20.8562
Nayan, R., Rawat, M., Negi, B., Pande, A., Arora, S. (2016). Zinc sulfide nanoparticle mediated alterations in growth and anti-oxidant status of brassica juncea. Biologia. 71, 896–902. doi: 10.1515/biolog-2016-0107
Norris, J., Hume, A. (1987). In vivo release of cyanide from sodium nitroprusside. Br. J. anaesthesia. 59, 236–239. doi: 10.1093/bja/59.2.236
Nriagu, J. O., Pacyna, J. M. (1988). Quantitative assessment of worldwide contamination of air, water and soils by trace metals. nature. 333, 134–139. doi: 10.1038/333134a0
Oliveira, H. C., Gomes, B. C. R., Pelegrino, M. T., Seabra, A. B. (2016). Nitric oxide-releasing chitosan nanoparticles alleviate the effects of salt stress in maize plants. Nitric. Oxide. 61, 10–19. doi: 10.1016/j.niox.2016.09.010
Pande, A., Arora, S. (2019). “Nanotechnological interventions for improving plant health and productivity,” in Plant biotechnology: Progress in genomic era (Singapore: Springer).
Pande, A., Mun, B.-G., Lee, D.-S., Khan, M., Lee, G.-M., Hussain, A., et al. (2021). NO network for plant–microbe communication underground: A review. Front. Plant Sci. 12, 431. doi: 10.3389/fpls.2021.658679
Pande, A., Mun, B. G., Rahim, W., Khan, M., Lee, D. S., Lee, G. M., et al. (2022). Phytohormonal regulation through protein s-nitrosylation under stress. Front. Plant Sci. 13, 65542–865542. doi: 10.3389/fpls.2022.865542
Pelegrino, M. T., Kohatsu, M. Y., Seabra, A. B., Monteiro, L. R., Gomes, D. G., Oliveira, H. C., et al. (2020). Effects of copper oxide nanoparticles on growth of lettuce (Lactuca sativa l.) seedlings and possible implications of nitric oxide in their antioxidative defense. Environ. Monit. Assess. 192, 1–14. doi: 10.1007/s10661-020-8188-3
Pelegrino, M. T., Silva, L. C., Watashi, C. M., Haddad, P. S., Rodrigues, T., Seabra, A. B. (2017b). Nitric oxide-releasing nanoparticles: Synthesis, characterization, and cytotoxicity to tumorigenic cells. J. Nanoparticle Res. 19, 57. doi: 10.1007/s11051-017-3747-4
Pelegrino, M., Weller, R., Chen, X., Bernardes, J., Seabra, A. B. (2017a). Chitosan nanoparticles for nitric oxide delivery in human skin. MedChemComm. 8, 713–719. doi: 10.1039/C6MD00502K
Pereira, A. E. S., Narciso, A. M., Seabra, A. B., Fraceto, L. F. (2015). Evaluation of the effects of nitric oxide-releasing nanoparticles on plants. J. Physics: Conf. Ser. 617, 012025. doi: 10.1088/1742-6596/617/1/012025
Phang, C., Leung, D. W., Taylor, H. H., Burritt, D. J. (2011). The protective effect of sodium nitroprusside (SNP) treatment on arabidopsis thaliana seedlings exposed to toxic level of Pb is not linked to avoidance of Pb uptake. Ecotoxicology Environ. Saf. 74, 1310–1315. doi: 10.1016/j.ecoenv.2011.02.006
Piacentini, D., Della Rovere, F., Sofo, A., Fattorini, L., Falasca, G., Altamura, M. M. (2020a). Nitric oxide cooperates with auxin to mitigate the alterations in the root system caused by cadmium and arsenic. Front. Plant Sci. 11, 1182. doi: 10.3389/fpls.2020.01182
Piacentini, D., Ronzan, M., Fattorini, L., Della Rovere, F., Massimi, L., Altamura, M., et al. (2020b). Nitric oxide alleviates cadmium-but not arsenic-induced damages in rice roots. Plant Physiol. Biochem. 151, 729–742. doi: 10.1016/j.plaphy.2020.04.004
Pieretti, J. C., Seabra, A. B. (2020). “Nitric oxide-releasing nanomaterials and skin infections,” in Nanotechnology in skin, soft tissue, and bone infections (Cham: Springer).
Pieroni, M. (2020). Nitric oxide-releasing cyclodextrins as biodegradable antibacterial scaffolds: A patent evaluation of US2019343869 (A1). Expert Opin. Ther. Patents. 30, 1–5. doi: 10.1080/13543776.2020.1822328
Praveen, A., Gupta, M. (2018). Nitric oxide confronts arsenic stimulated oxidative stress and root architecture through distinct gene expression of auxin transporters, nutrient related genes and modulates biochemical responses in oryza sativa l. Environ. Pollut. 240, 950–962. doi: 10.1016/j.envpol.2018.04.096
Rizwan, M., Mostofa, M. G., Ahmad, M. Z., Imtiaz, M., Mehmood, S., Adeel, M., et al. (2018). Nitric oxide induces rice tolerance to excessive nickel by regulating nickel uptake, reactive oxygen species detoxification and defense-related gene expression. Chemosphere. 191, 23–35. doi: 10.1016/j.chemosphere.2017.09.068
Rodríguez-Ruiz, M., Mateos, R. M., Codesido, V., Corpas, F. J., Palma, J. M. (2017). Characterization of the galactono-1,4-lactone dehydrogenase from pepper fruits and its modulation in the ascorbate biosynthesis. Role of nitric oxide. Redox Biol. 12, 171–181. doi: 10.1016/j.redox.2017.02.009
Romero-Puertas, M. C., Laxa, M., Matte, A., Zaninotto, F., Finkemeier, I., Jones, A. M., et al. (2007). S-nitrosylation of peroxiredoxin II e promotes peroxynitrite-mediated tyrosine nitration. Plant Cell. 19, 4120–4130. doi: 10.1105/tpc.107.055061
Romero-Puertas, M. C., Terrón-Camero, L. C., Peláez-Vico, M. Á., Olmedilla, A., Sandalio, L. M. (2019). Reactive oxygen and nitrogen species as key indicators of plant responses to cd stress. Environ. Exp. Bot. 161, 107–119. doi: 10.1016/j.envexpbot.2018.10.012
Rossi, L., Zhang, W., Schwab, A. P., Ma, X. (2017). Uptake, accumulation, and in planta distribution of coexisting cerium oxide nanoparticles and cadmium in glycine max (L.) merr . Environ. Sci. Technol. 51, 12815–12824. doi: 10.1021/acs.est.7b03363
Saavedra, J. E., Billiar, T. R., Williams, D. L., Kim, Y.-M., Watkins, S. C., Keefer, L. K. (1997). Targeting nitric oxide (NO) delivery in vivo. Design of a liver-selective NO donor prodrug that blocks tumor necrosis factor-α-Induced apoptosis and toxicity in the liver. J. Medicinal Chem. 40, 1947–1954. doi: 10.1021/jm9701031
Saavedra, J. E., Shami, P. J., Wang, L. Y., Davies, K. M., Booth, M. N., Citro, M. L., et al. (2000). Esterase-sensitive nitric oxide donors of the diazeniumdiolate family: In vitro antileukemic activity. J. Medicinal Chem. 43, 261–269. doi: 10.1021/jm9903850
Sadeghipour, O. (2017). Nitric oxide increases Pb tolerance by lowering Pb uptake and translocation as well as phytohormonal changes in cowpea (Vigna unguiculata (L.) walp.). Sains Malaysiana. 46, 189–195. doi: 10.17576/jsm-2017-4602-02
Samet, H. (2020). Alleviation of cobalt stress by exogenous sodium nitroprusside in iceberg lettuce. Chilean J. Agric. Res. 80, 161–170. doi: 10.4067/S0718-58392020000200161
Saxena, I., Shekhawat, G. (2013). Nitric oxide (NO) in alleviation of heavy metal induced phytotoxicity and its role in protein nitration. Nitric. Oxide. 32, 13–20. doi: 10.1016/j.niox.2013.03.004
Seabra, A. B., Durán, N. (2010). Nitric oxide-releasing vehicles for biomedical applications. J. Of Materials Chem. 20, 1624–1637. doi: 10.1039/B912493B
Seabra, A. B., Justo, G. Z., Haddad, P. S. (2015). State of the art, challenges and perspectives in the design of nitric oxide-releasing polymeric nanomaterials for biomedical applications. Biotechnol. Adv. 33, 1370–1379. doi: 10.1016/j.biotechadv.2015.01.005
Seabra, A. B., Rai, M., Durán, N. (2014). Nano carriers for nitric oxide delivery and its potential applications in plant physiological process: A mini review. J. Plant Biochem. Biotechnol. 23, 1–10. doi: 10.1007/s13562-013-0204-z
Sebastian, A., Nangia, A., Prasad, M. N. V. (2019). Cadmium and sodium adsorption properties of magnetite nanoparticles synthesized from hevea brasiliensis muell. arg. bark: Relevance in amelioration of metal stress in rice. J. Hazardous Materials. 371, 261–272. doi: 10.1016/j.jhazmat.2019.03.021
Sharma, S. S., Dietz, K.-J. (2009). The relationship between metal toxicity and cellular redox imbalance. Trends Plant Sci. 14, 43–50. doi: 10.1016/j.tplants.2008.10.007
Sharma, K., Iyer, A., Sengupta, K., Chakrapani, H. (2013a). INDQ/NO, a bioreductively activated nitric oxide prodrug. Organic Lett. 15, 2636–2639. doi: 10.1021/ol400884v
Sharma, K., Sengupta, K., Chakrapani, H. (2013b). Nitroreductase-activated nitric oxide (NO) prodrugs. Bioorganic Medicinal Chem. Lett. 23, 5964–5967. doi: 10.1016/j.bmcl.2013.08.066
Sharma, A., Soares, C., Sousa, B., Martins, M., Kumar, V., Shahzad, B., et al. (2020). Nitric oxide-mediated regulation of oxidative stress in plants under metal stress: a review on molecular and biochemical aspects. Physiologia plantarum 168, 318–344. doi: 10.1111/ppl.13004
Shurbaji, S., El-Sherbiny, I. M., Alser, M., Ali, I. H., Kordi, H., Al-Sadi, A., et al. (2021). Nitric oxide releasing hydrogel nanoparticles decreases epithelial cell injuries associated with airway reopening. Front. bioengineering Biotechnol. 8, 579788–579788. doi: 10.3389/fbioe.2020.579788
Sievers, E. L., Senter, P. D. (2013). Antibody-drug conjugates in cancer therapy. Annual Rev. Med. 64, 15–29. doi: 10.1146/annurev-med-050311-201823
Simontacchi, M., Galatro, A., Ramos-Artuso, F., Santa-María, G. E. (2015). Plant survival in a changing environment: the role of nitric oxide in plant responses to abiotic stress. Front. Plant Sci. 6, 977. doi: 10.3389/fpls.2015.00977
Singh, H. P., Batish, D. R., Kaur, G., Arora, K., Kohli, R. K. (2008). Nitric oxide (as sodium nitroprusside) supplementation ameliorates cd toxicity in hydroponically grown wheat roots. Environ. Exp. Bot. 63, 158–167. doi: 10.1016/j.envexpbot.2007.12.005
Singh, A. P., Dixit, G., Kumar, A., Mishra, S., Singh, P. K., Dwivedi, S., et al. (2016). Nitric oxide alleviated arsenic toxicity by modulation of antioxidants and thiol metabolism in rice (Oryza sativa l.). Front. Plant Sci. 6, 1272. doi: 10.3389/fpls.2015.01272
Singh, S., Husain, T., Kushwaha, B. K., Suhel, M., Fatima, A., Mishra, V., et al. (2021b). Regulation of ascorbate-glutathione cycle by exogenous nitric oxide and hydrogen peroxide in soybean roots under arsenate stress. J. Hazardous Materials. 409, 123686. doi: 10.1016/j.jhazmat.2020.123686
Singh, P. K., Indoliya, Y., Chauhan, A. S., Singh, S. P., Singh, A. P., Dwivedi, S., et al. (2017). Nitric oxide mediated transcriptional modulation enhances plant adaptive responses to arsenic stress. Sci. Rep. 7, 1–13. doi: 10.1038/s41598-017-03923-2
Singh, N., Jain, P., Gupta, S., Khurana, J. M., Bhatla, S. C. (2021a). N-nitrosomelatonin, an efficient nitric oxide donor and transporter in arabidopsis seedlings. Nitric. Oxide. 113-114, 50–56. doi: 10.1016/j.niox.2021.05.001
Singh, P., Shah, K. (2014). Evidences for reduced metal-uptake and membrane injury upon application of nitric oxide donor in cadmium stressed rice seedlings. Plant Physiol. Biochem. 83, 180–184. doi: 10.1016/j.plaphy.2014.07.018
Singh, I., Shah, K. (2015). Evidences for suppression of cadmium induced oxidative stress in presence of sulphosalicylic acid in rice seedlings. Plant Growth Regul. 76, 99–110. doi: 10.1007/s10725-015-0023-4
Souri, Z., Karimi, N. (2021). The effect of arsenic and sodium nitroprusside on the physiological responses and antioxidant enzymes activity of isatis cappadocica. jispp 10, 187–208. doi: 20.1001.1.23222727.1400.10.41.3.3
Sun, F., Wang, Y., Luo, X., Ma, Z., Xu, Y., Zhang, X., et al. (2019). Anti-CD24 antibody–nitric oxide conjugate selectively and potently suppresses hepatic carcinoma. Cancer Res. 79, 3395–3405. doi: 10.1158/0008-5472.CAN-18-2839
Tada, Y., Spoel, S. H., Pajerowska-Mukhtar, K., Mou, Z., Song, J., Wang, C., et al. (2008). Plant immunity requires conformational charges of NPR1 via s-nitrosylation and thioredoxins. Science. 321, 952–956. doi: 10.1126/science.1156970
Tchounwou, P. B., Yedjou, C. G., Patlolla, A. K., Sutton, D. J. (2012). Heavy metal toxicity and the environment. Molecular Clin. Environ. Toxicol. 101, 133–164. doi: 10.1007/978-3-7643-8340-4_6
Terrile, M. C., París, R., Calderón-Villalobos, L. I., Iglesias, M. J., Lamattina, L., Estelle, M., et al. (2012). Nitric oxide influences auxin signaling through s-nitrosylation of the arabidopsis TRANSPORT INHIBITOR RESPONSE 1 auxin receptor. Plant J. 70, 492–500. doi: 10.1111/j.1365-313X.2011.04885.x
Terrón-Camero, L. C., Peláez-Vico, M. Á., Del-Val, C., Sandalio, L. M., Romero-Puertas, M. C. (2019). Role of nitric oxide in plant responses to heavy metal stress: Exogenous application versus endogenous production. J. Exp. Bot. 70, 4477–4488. doi: 10.1093/jxb/erz184
Tian, Q. Y., Sun, D. H., Zhao, M. G., Zhang, W. H. (2007). Inhibition of nitric oxide synthase (NOS) underlies aluminum-induced inhibition of root elongation in hibiscus moscheutos. N. Phytol. 174, 322–331. doi: 10.1111/j.1469-8137.2007.02005.x
Wang, P. G., Cai, T. B., Taniguchi, N. (2005). Nitric oxide donors: For pharmaceutical and biological applications (John Wiley & Sons).
Wang, Y.-Q., Feechan, A., Yun, B.-W., Shafiei, R., Hofmann, A., Taylor, P., et al. (2009). S-nitrosylation of AtSABP3 antagonizes the expression of plant immunity. J. Biol. Chem. 284, 2131–2137. doi: 10.1074/jbc.M806782200
Wang, H.-H., Huang, J.-J., Bi, Y.-R. (2010a). Nitrate reductase-dependent nitric oxide production is involved in aluminum tolerance in red kidney bean roots. Plant Sci. 179, 281–288. doi: 10.1016/j.plantsci.2010.05.014
Wang, Y., Jiang, F., Ma, C., Rui, Y., Tsang, D. C. W., Xing, B. (2019). Effect of metal oxide nanoparticles on amino acids in wheat grains (Triticum aestivum) in a life cycle study. J. Environ. Manage. 241, 319–327. doi: 10.1016/j.jenvman.2019.04.041
Wang, K., Wang, Y., Wan, Y., Mi, Z., Wang, Q., Wang, Q., et al. (2021). The fate of arsenic in rice plants (Oryza sativa l.): Influence of different forms of selenium. Chemosphere. 264, 128417. doi: 10.1016/j.chemosphere.2020.128417
Wang, Y.-S., Yang, Z.-M. (2005). Nitric oxide reduces aluminum toxicity by preventing oxidative stress in the roots of cassia tora l. Plant Cell Physiol. 46, 1915–1923. doi: 10.1093/pcp/pci202
Wang, Y., Yang, T., He, Q. (2020). Strategies for engineering advanced nanomedicines for gas therapy of cancer. Natl. Sci. Rev. 7, 1485–1512. doi: 10.1093/nsr/nwaa034
Wang, S. H., Zhang, H., Jiang, S. J., Zhang, L., He, Q. Y., He, H. Q. (2010b). Effects of the nitric oxide donor sodium nitroprusside on antioxidant enzymes in wheat seedling roots under nickel stress. Russian J. Plant Physiol. 57, 833–839. doi: 10.1134/S1021443710060129
Wei, L., Zhang, M., Wei, S., Zhang, J., Wang, C., Liao, W. (2020). Roles of nitric oxide in heavy metal stress in plants: Cross-talk with phytohormones and protein s-nitrosylation. Environ. Pollut. 259, 113943. doi: 10.1016/j.envpol.2020.113943
Williams, A. T., Muller, C. R., Govender, K., Navati, M. S., Friedman, A. J., Friedman, J. M., et al. (2020). Control of systemic inflammation through early nitric oxide supplementation with nitric oxide releasing nanoparticles. Free Radical Biol. Med. 161, 15–22. doi: 10.1016/j.freeradbiomed.2020.09.025
Wojcik, M., Tukiendorf, A. (2004). Phytochelatin synthesis and cadmium localization in wild type of arabidopsis thaliana. Plant Growth Regul. 44, 71–80. doi: 10.1007/s10725-004-1592-9
Wu, X., Tang, X., Xian, M., Wang, P. G. (2001). Glycosylated diazeniumdiolates: A novel class of enzyme-activated nitric oxide donors. Tetrahedron Lett. 42, 3779–3782. doi: 10.1016/S0040-4039(01)00614-1
Xiong, J., An, L., Lu, H., Zhu, C. (2009). Exogenous nitric oxide enhances cadmium tolerance of rice by increasing pectin and hemicellulose contents in root cell wall. Planta. 230, 755–765. doi: 10.1007/s00425-009-0984-5
Yang, Y., Huang, Z., Li, L.-L. (2021). Advanced nitric oxide donors: Chemical structure of NO drugs, NO nanomedicines and biomedical applications. Nanoscale. 13, 444–459. doi: 10.1039/D0NR07484E
Yu, L., Gao, R., Shi, Q., Wang, X., Wei, M., Yang, F. (2013). Exogenous application of sodium nitroprusside alleviated cadmium induced chlorosis, photosynthesis inhibition and oxidative stress in cucumber. Pak J. Bot. 45, 813–819.
Yu, C. C., Hung, K. T., Kao, C. H. (2005). Nitric oxide reduces Cu toxicity and Cu-induced NH4+ accumulation in rice leaves. J. Plant Physiol. 162, 1319–1330. doi: 10.1016/j.jplph.2005.02.003
Yu, M., Lamattina, L., Spoel, S. H., Loake, G. J. (2014). Nitric oxide function in plant biology: A redox cue in deconvolution. N. Phytol. 202, 1142–1156. doi: 10.1111/nph.12739
Yun, B.-W., Feechan, A., Yin, M., Saidi, N. B., Le Bihan, T., Yu, M., et al. (2011). S-nitrosylation of NADPH oxidase regulates cell death in plant immunity. Nature. 478, 264–268. doi: 10.1038/nature10427
Yu, Q., Sun, L., Jin, H., Chen, Q., Chen, Z., Xu, M. (2012). Lead-induced nitric oxide generation plays a critical role in lead uptake by pogonatherum crinitum root cells. Plant Cell Physiol. 53, 1728–1736. doi: 10.1093/pcp/pcs116
Zhang, A., Jiang, M., Zhang, J., Ding, H., Xu, S., Hu, X., et al. (2007). Nitric oxide induced by hydrogen peroxide mediates abscisic acid-induced activation of the mitogen-activated protein kinase cascade involved in antioxidant defense in maize leaves. N. Phytol. 175, 36–50. doi: 10.1111/j.1469-8137.2007.02071.x
Zhang, P., Li, Y., Tang, Y., Shen, H., Li, J., Yi, Z., et al. (2020). Copper-based metal–organic framework as a controllable nitric oxide-releasing vehicle for enhanced diabetic wound healing. ACS Appl. Materials Interfaces. 12, 18319–18331. doi: 10.1021/acsami.0c01792
Zhao, H., Jin, Q., Wang, Y., Chu, L., Li, X., Xu, Y. (2016). Effects of nitric oxide on alleviating cadmium stress in typha angustifolia. Plant Growth Regul. 78, 243–251. doi: 10.1007/s10725-015-0089-z
Zhao, X.-F., Lin, C., Rehmani, M. I., Wang, Q.-S., Wang, S.-H., Hou, P.-F., et al. (2013). Effect of nitric oxide on alleviating cadmium toxicity in rice (Oryza sativa l.). J. Integr. Agric. 12, 1540–1550. doi: 10.1016/S2095-3119(13)60417-7
Zhou, P., Adeel, M., Shakoor, N., Guo, M., Hao, Y., Azeem, I., et al. (2021). Application of nanoparticles alleviates heavy metals stress and promotes plant growth: An overview. Nanomaterials. 11, 26. doi: 10.3390/nano11010026
Keywords: heavy metal toxicity, nitric oxide, NO donors, NO-release, nanoparticles, encapsulation, agriculture
Citation: Pande A, Mun B-G, Methela NJ, Rahim W, Lee D-S, Lee G-M, Hong JK, Hussain A, Loake G and Yun B-W (2022) Heavy metal toxicity in plants and the potential NO-releasing novel techniques as the impending mitigation alternatives. Front. Plant Sci. 13:1019647. doi: 10.3389/fpls.2022.1019647
Received: 15 August 2022; Accepted: 07 September 2022;
Published: 23 September 2022.
Edited by:
Liu Haitao, Henan Agricultural University, ChinaReviewed by:
Li Chen, Lanzhou University, ChinaZhixin Guo, Henan Agricultural University, China
Chengming Zhang, Hokkaido University, Japan
Copyright © 2022 Pande, Mun, Methela, Rahim, Lee, Lee, Hong, Hussain, Loake and Yun. This is an open-access article distributed under the terms of the Creative Commons Attribution License (CC BY). The use, distribution or reproduction in other forums is permitted, provided the original author(s) and the copyright owner(s) are credited and that the original publication in this journal is cited, in accordance with accepted academic practice. No use, distribution or reproduction is permitted which does not comply with these terms.
*Correspondence: Gary Loake, gloake@staffmail.ed.ac.uk; Byung-Wook Yun, bwyun@knu.ac.kr; Anjali Pande, anjali.pande23@gmail.com