- 1Key Laboratory of Bio-Resource and Eco-Environment of Ministry of Education, College of Life Sciences, Sichuan University, Chengdu, China
- 2The Engineering Research Institute of Agriculture and Forestry, Ludong University, Yantai, China
Drought, one of the most severe and complex abiotic stresses, is increasingly occurring due to global climate change and adversely affects plant growth and yield. Grafting is a proven and effective tool to enhance plant drought resistance ability by regulating their physiological and molecular processes. In this review, we have summarized the current understanding, mechanisms, and perspectives of the drought stress resistance of grafted plants. Plants resist drought through adaptive changes in their root, stem, and leaf morphology and structure, stomatal closure modulation to reduce transpiration, activating osmoregulation, enhancing antioxidant systems, and regulating phytohormones and gene expression changes. Additionally, the mRNAs, miRNAs and peptides crossing the grafted healing sites also confer drought resistance. However, the interaction between phytohormones, establishment of the scion-rootstock communication through genetic materials to enhance drought resistance is becoming a hot research topic. Therefore, our review provides not only physiological evidences for selecting drought-resistant rootstocks or scions, but also a clear understanding of the potential molecular effects to enhance drought resistance using grafted plants.
Introduction
Water is essential for the growth and development of plants, and it accounts for 70%-90% of the plant’s fresh weight. With global climate change, arid and semi-arid areas are accounting for ~40% of global land area and will cross over 50% by the end of the 21st century (Huang et al., 2015). This will cause a severe water shortage for plants in this area, resulting in a series of morphological, physiological, and molecular changes in them (Ahmed et al., 2009; Liu et al., 2014; Kumar et al., 2017). For example, drought reduces the plant cell’s water potential, transpiration rate, turgor pressure, and photosynthetic rate, while increasing their reactive oxygen species (ROS) accumulation, which causes poor plant growth, early flowering, and ultimately plant yield losses (Alan et al., 2007; Kumar et al., 2017; Chaimala et al., 2020; Gupta et al., 2020; Zia et al., 2021). According to the past decade’s data, drought caused global crop yield losses of ~$30 billion (Gupta et al., 2020). With the rapid growth of global population and moderate increase in global arable land, water demand for crop growth could double by 2050, whereas the available freshwater is predicted to drop by 50% due to climate change (Gupta et al., 2020). Under such conditions, as plants are stationary, it is crucial to cultivate plants that can adapt to the arid environment and maintain their normal yield and growth. Additionally, using artificial methods (e.g., drought hardening, directive breeding, and exogenous application of plant growth regulators) can also improve plant drought resistance (Farooq et al., 2009; Tesfahun and Yildiz, 2018). Interestingly, grafting has shown promise in improving plant drought resistance through the use of drought-resistant plant materials.
Grafting is a type of asexual propagation method that connects the budding stem segment of one plant (scion) to that of another plant containing roots (rootstock), to allow them to grow together. It is widely used in commercial fruit and vegetable cultivation, landscaping, and for verifying molecular movement in plants (Fullana-Pericàs et al., 2018; Li et al., 2020). Moreover, it can promote the growth and development of the grafted plants, maintain the excellent properties of the parents, change their branch structure, increase plant yield, positively influence fruit flavor, improve nutritional value, and enhance abiotic stress resistance (Sánchez-Rodríguez et al., 2014; Melnyk and Meyerowitz, 2015; Thomas and Frank, 2019; Ellenberger et al., 2021). Unfortunately, grafting cannot be applied to all plants, as it is determined by grafting compatibility (Habibi et al., 2022). Grafting compatibility means the successful connection between the vascular and non-vascular systems at the grafted junction. Generally, the survival rate of intra-generic grafting is high, while that of inter-genera is low. Recently, studies on the physiological and molecular mechanisms of grafting junction have gradually become a new hotspot, like the key responsive genes in grafted healing sites (Melnyk et al., 2018; Notaguchi et al., 2020; Kurotani and Notaguchi, 2021; Thomas et al., 2021; Chambaud et al., 2022) and genetic information exchange between rootstocks and scions (Thomas and Frank, 2019; Yang et al., 2019; Cerruti et al., 2021; Okamoto et al., 2022).
Since drought greatly influences plant growth and development, it is essential to learn how to mitigate the negative effects through grafting (Zia et al., 2021). Grafting improves plant drought resistance (Ellialtioglu et al., 2019; Lopez-Serrano et al., 2019; Chen et al., 2020), which is primarily determined by the rootstock, despite the scion also affecting the grafted plant (Han et al., 2013; Han et al., 2019; Chen et al., 2020; Lopez-Hinojosa et al., 2021). Despite there being multiple studies and reviews on grafting and drought resistance, few have summarized how the molecular response of grafted plants is linked to their physiology and phenotype under drought stress. Therefore, this paper mainly summarizes the research to date on molecular mechanisms and physiological and morphological changes in grafted plants, while also listing the drought responsive genes and mobile molecules that generate drought resistance in grafted plants (Tables 1, 2).
Rootstock changes
The root is an important organ for water and nutrient uptake from the soil to support plant growth and development. Drought usually reduces root biomass, damages root system architecture (RSA) and decreases root hydraulic conductivity (Yang et al., 2021; Zia et al., 2021). As shown in Figure 1, drought-resistant grafted plants usually mitigate these effects by appropriately adjusting their roots.
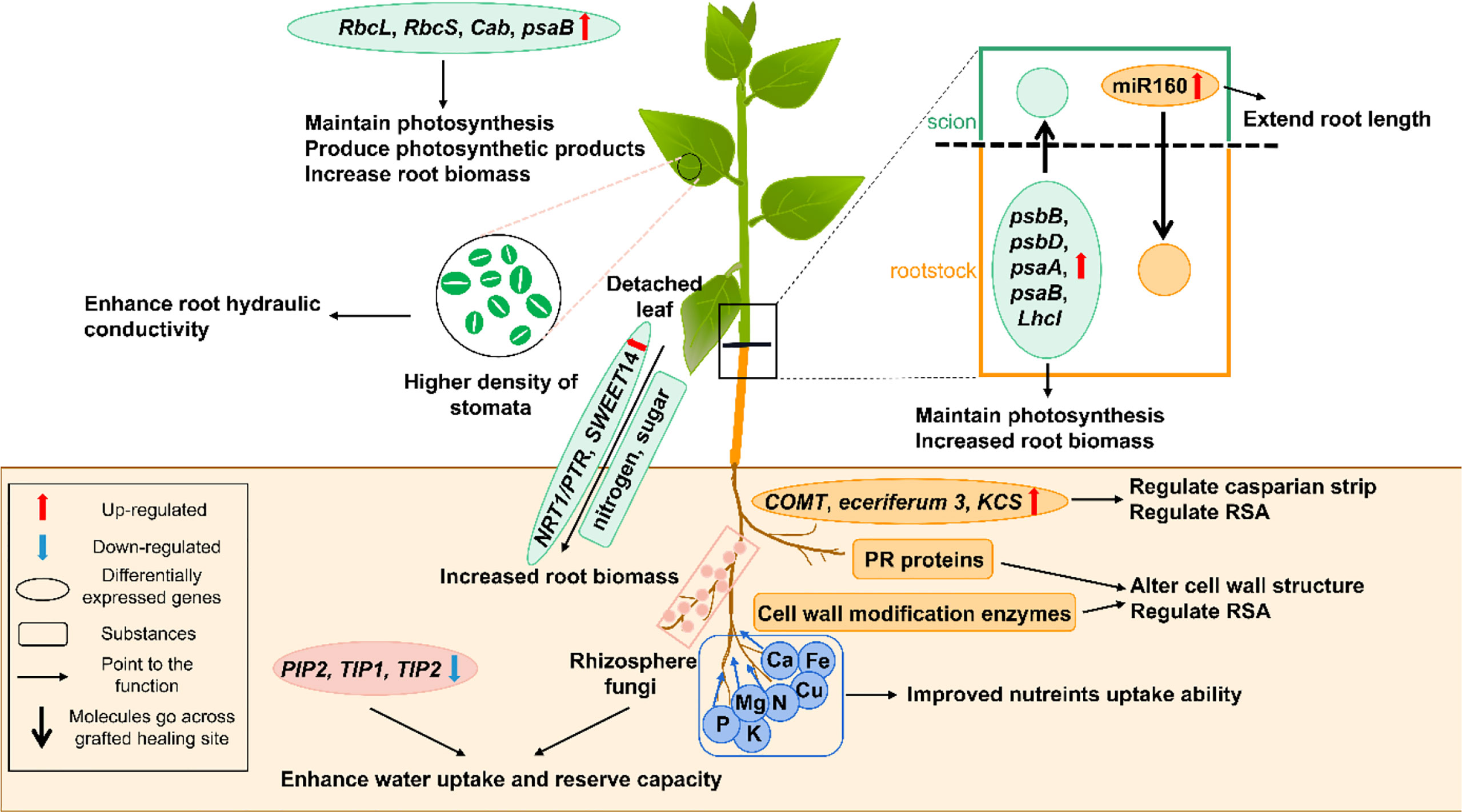
Figure 1 A schematic model shows the physiological and molecular mechanisms that enhancing drought resistance by root and shoot regulation in grafted plants. The green part represents for the scion, and the brown part represents for the rootstock. The black box shows the figure notes. Cab, chlorophyll a/b binding protein; COMT, caffeic acid 3-O-methyltransferase; KCS, 3-ketoacyl-CoA synthase; LhcI, photosystem I light-harvesting chlorophyll a/b-binding protein; NRT1/PTR, nitrate transporter 1/peptide transporter; PIP, plasma membrane intrinsic proteins; psaA, photosystem I P700 chlorophyll a apoprotein A1; psaB, photosystem I P700 chlorophyll a apoprotein A2; psbB, photosystem II CP47 reaction center protein; psbD, photosystem II protein D2; RbcS, ribulose-1,5-bisphosphate carboxylase/oxygenase small subunit; RbcL, Ribulose-1,5-diphosphate carboxylase/oxygenase large subunit; SWEET14, bidirectional sugar transporter; TIP1 and 2, tonoplast intrinsic proteins 1 and 2.
Morphological changes of rootstock
Increased root biomass and root-shoot ratio confer great advantages to grafted plants under drought stress (Li et al., 2020). The grafted grapevine and pepper plants display enhanced drought resistance by maintaining high root biomass and water absorption and storage ability (Lopez-Serrano et al., 2019; Prinsi et al., 2021). The accumulation of carbohydrates and nitrogen which need transporter proteins to move to drought-resistant roots, on the one hand, is from detached leaves, and on the other hand, is from photosynthesis (Yildirim et al., 2018; Han et al., 2019). The bidirectional sugar transporter (SWEET14) and nitrate transporter 1/peptide transporter (NRT1/PTR), were two significantly accumulating proteins in drought-resistant grapevine rootstock 110R, which promoted the carbohydrates and nitrogen accumulation in roots (Chen et al., 2012; Yildirim et al., 2018; Ji et al., 2022). Although we believe that during drought stress, transporter proteins will accumulate in the grafted plants, the underlying regulated pathways need further investigation.
Besides accumulating greater root biomass, drought-resistant rootstocks display higher root plasticity and root vigor than drought-sensitive ones (Han et al., 2019; Silva et al., 2021). Maintaining the rapid growth and large roots under water stress is important for drought-resistant improvement (Li et al., 2020). By using the accumulated assimilate, the root system architecture (RSA) (e.g., root length, root diameter, root area, and root volume) of drought-resistant grapevine rootstocks changes to better absorb the water and nutrients in deep soil, with the root hydraulic conductivity being greater than drought-sensitive ones (Alsina et al., 2011; Sucu et al., 2017; Yildirim et al., 2018). Similar results were obtained in tomatoes (Suchoff et al., 2018). These root architecture changes depend on signal molecules and multiple genes. Glutamate is a common signaling molecule in RSA under drought stress (Qiu et al., 2019). Decreased glutamate in drought-resistant citrus rootstocks during severe drought stress inhibited the growth of lateral roots, which allocated more resources towards the primary root elongation to expand their reach of the available water supply (Sousa et al., 2022). Additionally, the up-regulation of genes encoding cell wall modification enzymes and pathogenesis-related (PR) proteins in the rootstock can also alter cell wall structure to easily modify the RSA to enhance the water uptake capacity (Sels et al., 2008; Yildirim et al., 2018). The miR160 moving from scion to rootstock in the grafted apple and tobacco plants improved root development by extending the root length (Shen et al., 2022).
Root water preservation and nutrient uptake
Root biomass accumulation and RSA regulation are the two primary water storage methods in roots. Besides these, genes related to suberization and wax biosynthesis, like caffeic acid 3-O-methyltransferase (COMT), eceriferum 3 and 3-ketoacyl-CoA synthase (KCS), were up-regulated to build and thicken the Casparian strip (Vincent et al., 2005; Lee et al., 2009; Li et al., 2015; Yildirim et al., 2018). The Casparian strip helped prevent the water backflow from the root to the soil (Yildirim et al., 2018). Aquaporins, are water channels encoded by plasma membrane intrinsic proteins (PIPs) and tonoplast intrinsic proteins (TIPs) genes, and their expression pattern varied with the extent of drought stress and plant species (Gautam and Pandey, 2021; Shivaraj et al., 2021). The PIP genes were differentially expressed in the grafted plants of two plum rootstocks (R20 and R40), but it was difficult to explain their drought-induced expression patterns and their effects (Opazo et al., 2020). However, during severe drought-stressed conditions, TIP1, TIP2, and PIP2 were down-regulated in the grapevine rootstocks, which reduced aquaporins and prevented water loss from roots (Afzal et al., 2016; Yildirim et al., 2018). The PIP1;2 was considered a candidate gene for improving the plant water conductivity in grafted hickory plants (Kumar et al., 2018). Additionally, aquaporins were reported to be transported from pumpkin rootstocks to cucumber scions during drought (Davoudi et al., 2022). Therefore, aquaporins and their related genes need further exploration in grafted plants under drought stress. Furthermore, the root’s water and nutrient uptake ability was improved in the grafted poplars, which may be related to the high relative abundance of rhizosphere fungi (Liu et al., 2019).
The plant nutrient uptake and utilization depend on the root’s nutrient absorption capacity or the strength of signals arising from the scions (Savvas et al., 2010). Obviously, grafting with vigorous drought-resistant rootstocks can enhance the nutrient uptake ability to improve plant yield when compared to self-rooted plants (Savvas et al., 2010; Zhang et al., 2020). For example, compared with the scions grafted with drought-sensitive tomato rootstocks ‘S’, the concentrations of vital macronutrients like N, P, K, Ca, and Mg were higher in the leaves of scions grafted with drought-resistant ‘T’ under drought-stressed conditions, thereby indicating that drought-resistant rootstocks enhanced the uptake and translocation of nutrients toward the shoots (Zhang et al., 2020). Similarly, plants grafted with drought-tolerant tomato rootstocks (Zarina) showed higher macronutrient (N, P and K) and micronutrient (Fe and Cu) concentrations under water stressed conditions (Sánchez-Rodríguez et al., 2013; Sánchez-Rodríguez et al., 2014). Water stress may also inhibit the nitrogen metabolism enzymes, thereby limiting the plant’s nitrogen assimilation ability (Sánchez-Rodríguez et al., 2013). When drought-tolerant tomato variety was used as rootstocks, the grafted plants showed an improved N uptake and NO3- assimilation, which promoted their growth (Sánchez-Rodríguez et al., 2013).
Scion changes
Scions maintain their own growth and development through photosynthetic products. The drought stress-induced adverse effects on scions included leaf wilting, reduction of leaf area and numbers, reduced leaf and stem biomass, and weakened photosynthesis, all of which decreased the photosynthetic products and water contents, and water use efficiency of scions (Shao et al., 2008). Grafting onto drought-resistant rootstocks can be a good strategy to alleviate these problems (Shehata et al., 2022).
Morphological changes of the scion
Morphological observations post water deficit conditions showed a lower proportion of yellow and dry leaves in the scions grafted onto drought-resistant grapevine rootstocks (Sucu et al., 2017). The leaf area size directly affects plant photosynthetic intensity (Yang et al., 2021). Under the drought treatment, the drought-resistant apple rootstocks had a greater leaf area, which improved photosynthesis, thereby positively affecting the whole plant productivity (Valverdi and Kalcsits, 2021). Shoot growth is sensitive to water stress and may stop even with minor water reduction (Sabir and Kucukbasmaci, 2019). Naturally, the drought-resistant grapevine rootstocks were found to maintain the shoot growth of scions under water deficit conditions. (Sabir and Kucukbasmaci, 2019). Fresh and dry weights of the leaf and shoot are considered important indexes to screen and identify drought-tolerant genotypes (Bikdeloo et al., 2021). When scions are grafted onto drought-tolerant grapevine rootstocks, their leaf dry weight were higher than the grafted plants containing sensitive rootstocks (Gullo et al., 2018). Plants grafted onto high vigor watermelon rootstocks exhibited a relatively lower reduction in growth and shoot biomass (Ali et al., 2019; Bikdeloo et al., 2021).
The micro-morphological changes of leaves and stems also promoted the water absorption of scions. Drought-resistant rootstocks increased the diameter and density of the xylem vessels and decreased the numbers of emboli at the grafted site to increase the hydraulic conductance capacity and ultimately altered the drought resistance of the scion (Bauerle et al., 2011). When two plum scions (An and Np) were grafted onto the same rootstocks, the grafted combination with An scion had higher root hydraulic conductivity, probably due to the higher stomatal density of scions, thereby causing great internal pressure in the transpiration stream (Opazo et al., 2020). Using scions and rootstocks of xeric origin can not only improve the drought tolerance of grafted plants, but also shape their phenology, including delaying bud-break and reducing stem secondary growth of trees (Camisón et al., 2021).
Photosynthesis
Scions that are grafted with drought-resistant tomato rootstocks demonstrated a lower photosynthetic rate reduction (Alves et al., 2021). The maintenance of a high CO2 assimilation rate in drought-resistant grafted plants provides the basis for good plant growth and productivity under long-term drought situations. This causes drought-resistant grafted plants to accumulate greater biomass accumulation than their drought-sensitive counterparts, despite this accumulation being lesser than during normal conditions. Photosynthesis is adversely affected by stomatal and non-stomatal factors. The stomatal movement and development will be discussed later. The non-stomatal factors are the main photosynthesis inhibitory factors and they include chloroplast rupturing, inhibition of chlorophyll synthesis, a decrease of photosystem II reaction center activity, and inhibition of ribulose-1,5-diphosphate carboxylase/oxygenase (Rubisco) activity (Flexas and Medrano, 2002; Flexas et al., 2004; Twalla et al., 2021). As shown in Figure 1, these problems are alleviated by grafting onto high vigor rootstocks. The chlorophyll content is a useful index for evaluating plant drought resistance. The chlorophyll content of leaves was the highest in the scions grafted with the strongest drought-resistant rootstocks among three different rootstocks during drought stress treatment, which also indicated higher photosystem II activity (Dong et al., 2021). The Rubisco large subunit (RbcL), Rubisco small subunit (RbcS), chlorophyll a/b binding protein (Cab) and photosystem I p700 chlorophyll a apoprotein A2 (psaB) genes were up-regulated in chrysanthemum scions of the drought-resistant grafted plants and it helped maintain the photosynthetic performance (Chen et al., 2018; Wilson and Hayer-Hartl, 2018). The movement of a series of mobile mRNAs from the pumpkin roots to the scions, also called scion-rootstock communication, can also improve the photosynthetic performance during drought conditions (Davoudi et al., 2022). Specifically, multiple genes, including photosystem II CP47 reaction center protein (psbB), photosystem I p700 chlorophyll a apoprotein A2 (psaB), photosystem II protein D2 (psbD), photosystem I p700 chlorophyll a apoprotein A1 (psaA) and photosystem I light-harvesting chlorophyll a/b-binding protein (LhcI), functional in different photosynthesis-related processes, were induced to maintain the photosynthetic activity of the grafted plants (Davoudi et al., 2022). Among them, psaB is the common gene that was not only detected in chrysanthemum scions, but also identified in the molecule movement from pumpkin rootstocks to cucumber scions. Therefore, we believe that some photosynthesis-related genes are being activated in the roots and their transcripts are being moved to scions.
Crop yield and quality
For trees, improving drought resistance through grafting is the most important aspect, as it allows better growth under drought stressed conditions. However, for grafted vegetables and fruits, it is not only important to improve their drought resistance, but also to retain adequate yield and high vegetable/fruit quality in the process (Fullana-Pericàs et al., 2020). Many studies have suggested that the drought-induced negative effects on the yield and quality of fruits and vegetables can be mitigated by grafting with drought-resistant rootstocks (Supplementary Table 1) (Ellenberger et al., 2021).
To improve plant yield during drought stress, there are two pivotal indices we must consider: water use efficiency and photosynthesis. Drought always negatively affects the plants due to the lack of both water and photosynthetic products, which directly reduces plant biomass production (Proietti et al., 2008; Rouphael et al., 2008). Therefore, it is imperative to select rootstocks capable of raising the water and nutritional status of scions. (López-Marín et al., 2017). Previous studies found that some drought-resistant pepper, watermelon, and tomato rootstocks could alleviate the negative effects on roots and improve their water use efficiency to increase yields under limited irrigation conditions (Poudyal et al., 2017; Al-Harbi et al., 2018; Yavuz et al., 2020). Thus, the less the reduction of photosynthetic ability, the higher the yield in grafted vegetables (López-Marín et al., 2017; Gisbert-Mullor et al., 2020).
The fruit quality includes nutritional value, taste, shape, uniformity, and odor (Khadivi-Khub and Anjam, 2016; Ellenberger et al., 2021). Drought causes yield reduction, but its effects on fruit quality are still undetermined. Nevertheless, grafting has been widely recommended as a useful tool to help plants acclimatize to drought conditions. Many studies have found that grafting altered the composition of secondary metabolites to improve the fruit’s nutrients and flavor (Čolić et al., 2021; Ellenberger et al., 2021; Seymen et al., 2021; El-Mogy et al., 2022). By using drought-tolerant ‘Zarina’ as the rootstock, the grafted tomato plants showed a moderate accumulation of antioxidant compounds, sugars, organic acids, and minerals, which ultimately improved the quality of fruits under moderate water stressed conditions (Sánchez-Rodríguez et al., 2012). Similarly, under water stress, ‘Durinta’ cultivars that were grafted with ‘Beaufort’ rootstocks had accumulated vitamin C and total soluble solids, which could improve tomato quality (Abdulaziz et al., 2017).
Due to the different genotypes of scions and rootstocks, connecting the two may cause genetic limitations. For example, in some grafted combinations, when drought-resistant plants and drought-sensitive plants were used as rootstocks and scions, respectively, the grafted plants had better drought resistance than the contrary combinations where drought-resistant plants and drought-sensitive plants were respectively used as scions and rootstocks. However, sometimes despite the drought-resistant soybean being used as the scion or the rootstock, the grafted plants could maintain photosynthesis (Li et al., 2019; Spiral et al., 2022). This genetic limitation is more obvious when we consider fruit quality. The scion variety theoretically determines the fruit composition in grafted plants. Due to the genetic limitation in grafted plants, varied rootstocks can drastically influence and alter the agronomic traits as well, whereas the role of the scion will be weakened (Zhang et al., 2016). Sometimes when drought-resistant rootstock was grafted with high-yielding scion, this combination did not always have high yield and fruit quality, thus indicating that the effect of grafting partly depended on the grafted combination (Sánchez-Rodríguez et al., 2012). Ignoring the scion-rootstock interaction or randomly choosing only vigorous rootstock and high-yielding scion is not a good strategy to improve yield and fruit quality (Karunakaran and Ilango, 2019). Therefore, besides the drought-resistant rootstock, considering the scion-rootstock interaction, and choosing the best combination for better drought acclimatization and the consequent increase in yield and fruit quality is a commonly used strategy (Khadivi-Khub and Anjam, 2016; Al-Harbi et al., 2018). Various grafted combinations must be thoroughly evaluated through studies in the field for the final identification of the most suitable combination (Karunakaran and Ilango, 2019).
Phytohormones
Abscisic acid (ABA) is the most important phytohormone for intensifying plant drought resistance via various morpho-physiological and molecular processes. Other phytohormones, like jasmonic acid (JA), salicylic acid (SA), ethylene (ET), auxins (IAA), gibberellins (GAs), cytokinins (CKs), and brassinosteroids (BRs), are also important for water deficit conditions (Ullah et al., 2018). These phytohormones usually cross-talk with each other to promote the plant’s survival during drought stress. In grafted plants, as shown in Figure 2, roots can primarily sense soil water deficit and activate the appropriate signaling molecules to resist drought stress (Acharya and Assmann, 2009; Gaion et al., 2018; Zhang et al., 2019a).
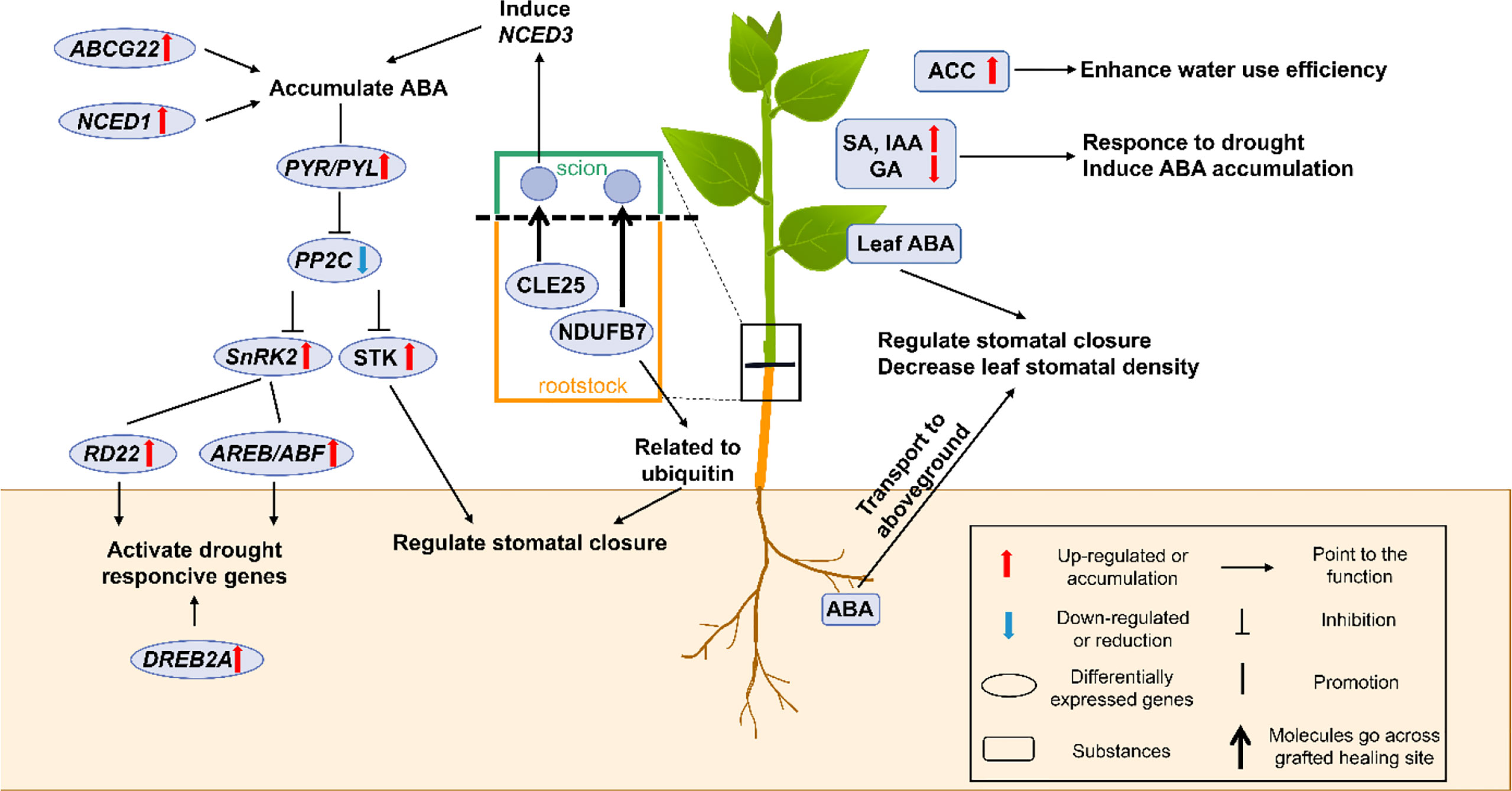
Figure 2 A schematic model shows the physiological and molecular mechanisms that enhancing drought resistance by the accumulation of phytohormones (especially ABA) in grafted plants. In grafted plants, the green part represents for the scion, and the brown part represents for the rootstock. The box shows the figure notes. ABA, abscisic acid; ABCG22, ATP-binding cassette subfamily G transporter 22; ACC, ethylene 1-amynocyclopropane-1-carboxylic; AREB/ABF, ABA-responsive element binding protein/ABA-responsive element binding factor; CLE25, clavata3/embryo-surrounding region-related 25; DREB2A, dehydration-responsive element-binding protein 2 A; GA, gibberellin; IAA, Indole-3-acetic acid; NCED1 and 3, 9-cis-epoxycarotenoid dioxygenase 1 and 3; NDUFB7, NADH-ubiquinone oxidoreductase B18 subunit; PP2C, protein phosphatase 2C; PYR/PYL, pyrabactin resistance1/pyr1-like; RD22, responsive to dehydration 22; SA, salicylic acid; SnRK2, sucrose non-fermenting-1-related protein kinase 2; STK, serine/threonine-protein kinase-transforming protein.
ABA
Stomata are vital organs that exchange gas and water with the external environment to ensure maximum CO2 absorption for photosynthesis and efficient control of optimal transpiration during plant life. To cope with drought stress, plants mainly use stomatal movement to control transpiration and the stomatal density changes for a long-term drought period (Marguerit et al., 2012). ABA is considered a key regulator in stomatal closure and is important in drought stress resistance. Extensive studies on the synergetic role of drought and ABA have already been conducted (Muhammad Aslam et al., 2022). They have shown that ABA triggered diverse physiological and molecular responses, like stomatal closure, cuticular wax deposition, root system modulation, activation of transcriptional and post-transcriptional gene expression, and metabolic changes in regular plants (Muhammad Aslam et al., 2022). In grafted plants, the mechanisms of ABA-mediated stomatal movement under drought stress have also been reported in detail. Under short-term drought, ABA was synthesized in roots and then transported into the guard cells to trigger stomatal closure and reduced transpiration via the branch signal cascade (Wilkinson and Davies, 2002; Allario et al., 2013; Sarwat and Tuteja, 2017). Under long-term drought, decreased stomatal density was found in the newly developed leaves of the grafted plants, thereby demonstrating that the high ABA content in new leaves decreased the leaf stomatal density and enabled the grafted plants to maintain long-term drought resistance (Tanaka et al., 2013; Liu et al., 2016). Despite their usual function, studies have also shown that the accumulated ABA in the grafted cucumber/luffa combination might also improve the activity of antioxidative enzymes (Liu et al., 2016). Since the ABA-dependent signaling pathway is one of the stress signal transduction-related pathways, ABA can be the signal that triggers the expression of drought-resistant genes in grafted plants. This will be discussed in the following parts (Gong et al., 2015; Du et al., 2018; Silva et al., 2018). Therefore, ABA has multiple functions in grafted plants and its significant accumulation of ABA is a helpful feature for selecting drought-resistant grafted combinations.
Other phytohormones
SA is a common defensive hormone that also participates in drought resistance (Santana-Vieira et al., 2016). Studies revealed that foliar SA accumulation was detected in grafted citrus plants, which could positively regulate stomatal closure (Santana-Vieira et al., 2016). Furthermore, exogenous SA application before drought treatment triggered the ABA synthesis during water deficiency (Bandurska and Stroinski, 2005; Santana-Vieira et al., 2016). IAA is synthesized in the root tip or shoot apex and has been thoroughly studied to date (Ullah et al., 2018). It was reported that IAA could regulate drought resistance by adjusting root architecture and promoting the ABA-responsive genes’ expression in citrus rootstocks (Shi et al., 2014). The transgenic gretchen hagen 3 (GH3) RNAi plants (GH3 silencing) used as rootstock caused IAA accumulation, which further induced RSA enlargement to absorb water and maintain water use efficiency (Jiang et al., 2022). GA plays a vital role throughout the plant life cycle (Ullah et al., 2018). Studies have revealed that a low GA level is useful for improving plant drought resistance (Ullah et al., 2018). Similarly, the high ABA content triggered by the low GA content in the grafted tomato plants could finally enhance their drought resistance (Gaion et al., 2018). However, the role of other phytohormones, like JA, CKs, and BRs during drought treatment, is poorly studied in grafted plants. In summary, the changes in the SA, IAA, and GA contents are commonly accompanied by ABA synthesis and they interact synergistically and antagonistically to regulate each other in the grafted plants (Santana-Vieira et al., 2016; Gaion et al., 2018; Sousa et al., 2022).
Additionally, in the grafted tomato plants, the root-derived precursor of ethylene 1-aminocyclopropane-1-carboxylic (ACC) could increase the fruit yield and agronomic water use efficiency (Cantero-Navarro et al., 2016). Applying exogenous melatonin onto the rootstocks improved their drought resistance by regulating the key metabolic pathways, like the phenylpropanoid pathway, chlorophyll and carotenoid biosynthesis, carbon fixation, and sugar metabolism (Lunn et al., 2014; Sharma et al., 2020). However, how the accumulation of melatonin and ACC can enhance drought resistance in grafted plants still needs further investigation.
Signaling pathway
ABA-dependent pathway
As shown in Figure 2, the ABA-dependent signaling pathway is crucial in regulating stomatal movement and activating drought-responsive gene expression in plants (Ullah et al., 2018). This includes the accumulation and signal transduction pathways. Roots are the main sites of ABA biosynthesis, followed by leaves. 9-cis-epoxycarotenoid dioxygenase (NCED) is the key rate-limiting enzyme in ABA biosynthesis (Seo and Koshiba, 2002; Ksouri et al., 2016; Ali et al., 2020). In autotetraploid clones of citrus rootstock (Rangpur lime), NCED1 was found highly up-regulated, and it improved ABA accumulation that ultimately enhanced drought resistance (Allario et al., 2013). Additionally, an ABA transporter gene, ATP-binding cassette subfamily G transporter 22 (ABCG22) was also indirectly up-regulated in the cucumber/luffa grafted combination, thereby suggesting an ABA accumulation (Liu et al., 2016). There are molecules crossing grafted healing sites to build the scion-rootstock communication. Grafting experiments have demonstrated that clavata3/embryo-surrounding region-related 25 (CLE25) was transported from the roots to leaves, where it induced NCED3 expression and ABA accumulation to promote stomatal closure under drought stress (Takahashi et al., 2018).
Besides the ABA accumulation, the leaf transcriptome showed that drought resistance in scions induced by rootstocks was related to transcriptional activation of ABA-dependent signaling pathway genes (Goncalves et al., 2019). ABA sensing and signaling are mediated by three classes of proteins: PYR/PYL, PP2C, and SnRK2. Being a hormonal signal, ABA first binds to the pyrabactin resistance1/pyr1-like (PYR/PYL) receptor, followed by constant binding with protein phosphatase 2C (PP2C) to form a ternary complex, which triggers the release of the transcription factor sucrose non-fermenting-1-related protein kinase 2 (SnRK2) (Zhang et al., 2019b). This is the main ABA signal transduction pathway. The up-regulated PYR/PYL, and SnRK2 along with down-regulated PP2C were detected in the grafted tomato plants during drought stress, thereby indicating that the ABA signal transduction pathway was activated in the grafted tomato (Zhang et al., 2019b). SnRK2 plays important role in ABA-responsive stomatal closure and ABA-dependent gene expression. ABA-responsive element binding protein/ABA-responsive element binding factor (AREB/ABF) is activated through multi-site phosphorylation of the conserved domains by SnRK2 in the grafted tobacco plants, and it directly acts on numerous drought-responsive genes (Liu et al., 2014; Ullah et al., 2018; Soma et al., 2021). Furthermore, the activated SnRK2 induces stomatal closure through downstream regulation of ion channels and transcription factors (Soma et al., 2021). For example, the serine/threonine-protein kinase (STK) transported from the pumpkin rootstocks to cucumber scions participated in ABA-dependent stomatal movement by phosphorylating the ion channels (Staples, 2003; Ali et al., 2020). Moreover, molecules like the NADH-ubiquinone oxidoreductase B18 subunit (NDUFB7), transported from Arabidopsis rootstock to the tobacco scion, were related to ubiquitin, which was an important drought stress response occurring through regulating ABA signals and stomatal aperture (Notaguchi et al., 2015; Yang et al., 2017; Pan et al., 2020; Yu et al., 2020). Thus, we can speculate that ubiquitination and phosphorylation exist in the ABA-dependent signaling pathway and play important roles in grafted plants (Soma et al., 2021).
Gene overexpression in scions can also affect the ABA-dependent signaling pathway and enhance their drought resistance. YTH domain-containing RNA binding proteins (YTPs) are important in conferring drought resistance to plants (Li et al., 2014; Wang et al., 2017). The overexpression of YTP1 in transgenic apple scions promotes the expression of the ABF3 and stress response monitor gene responsive to dehydration 22 (RD22) genes, which were involved in the ABA-dependent pathway to stimulate stomatal aperture reduction and improve water use efficiency under long-term drought-stressed conditions (Guo et al., 2019). Researchers also pointed out that grafted plants with YTP1 transgenic apple being used as the scions exhibited better drought resistance (Guo et al., 2019).
ABA-independent pathway
Besides the ABA-dependent signaling pathway, there are ABA-independent pathways that activate the plant drought defense system (Figure 2). According to microarray analysis in Arabidopsis, there were several pathways that independently were abiotic stress-responsive and one such important pathway is the dehydration-responsive element-binding protein (DREB) regulon (Agarwal et al., 2006). The transcription factor DREB2 is involved in the ABA-independent pathway and uses the DRE cis-acting element to activate the drought responsive genes (Agarwal et al., 2006). In the grafted citrus and tomato plants, the upregulated DREB2A, a key drought response regulator, was detected, thus indicating that the ABA-independent pathway was activated to resist drought stress (Gaion et al., 2018; Goncalves et al., 2019).
Osmotic adjustment
Drought causes water deficiency and osmotic stress in plant cells, leading to ion imbalance and adversely affecting cellular functions (Zia et al., 2021). Under low water potential, the osmoregulation of grafted plants depends on the accumulation of different osmotically active compounds, which can improve their water retention ability and water use efficiency (Figure 3) (Ozturk et al., 2021). Due to the higher osmoregulatory capacity, grafted plants with drought-resistant grapevine rootstocks showed greater drought tolerance than those with drought-sensitive grapevine rootstocks (Lucini et al., 2020). Thus, choosing the right rootstocks is pivotal for grafted plants.
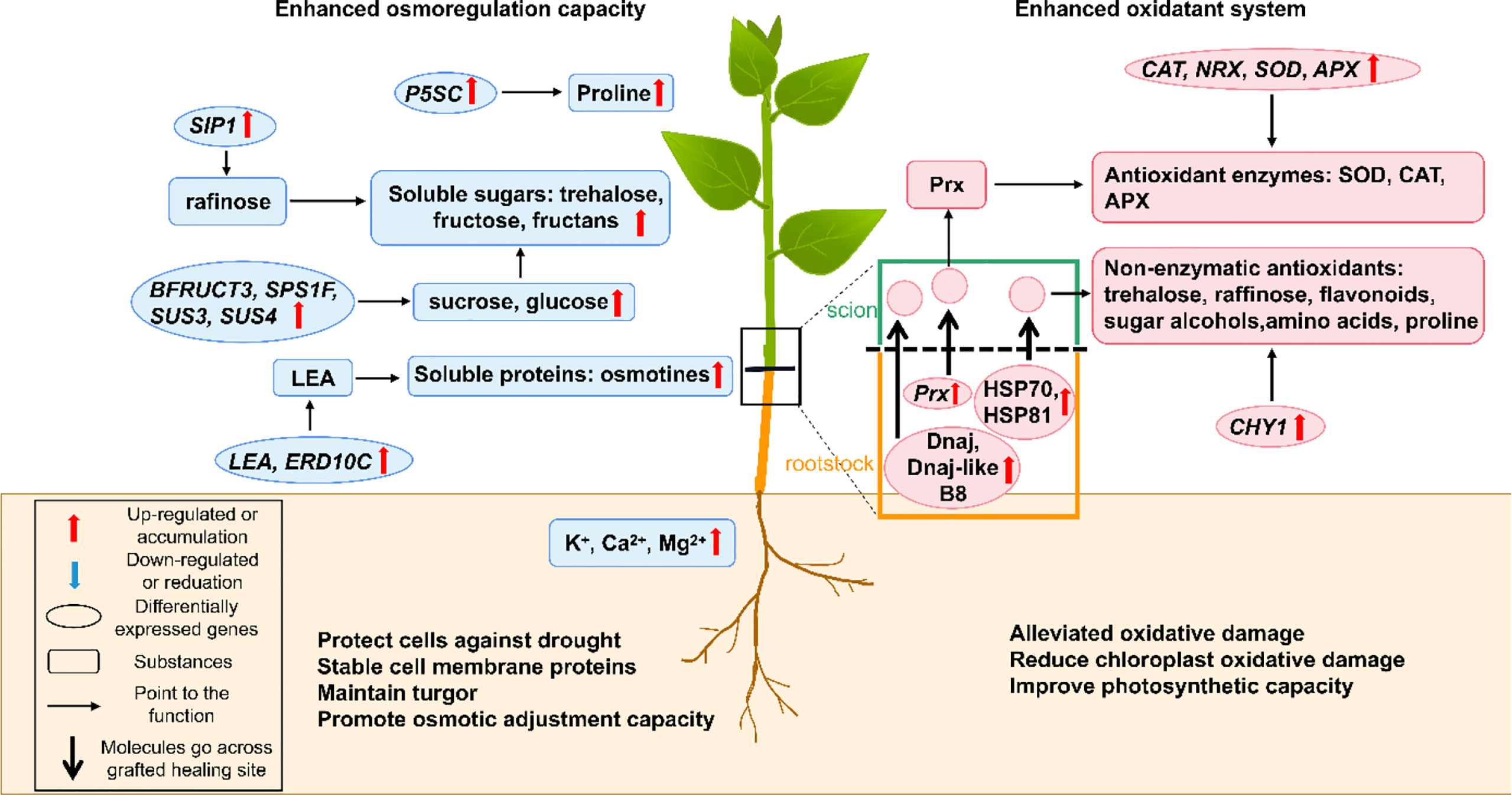
Figure 3 A schematic model shows the physiological and molecular mechanisms that enhancing drought resistance by osmoregulation and anti-oxidant system in grafted plants. The green part represents for the scion while the brown part represents for the rootstock. The box shows the figure notes. APX, ascorbate peroxidase; BFRUCT3, β-fructosidase 3; CAT, catalase; CHY1, chloroplast β-carotene hydroxylase; Dnaj, Chaperone protein DnaJ; Dnaj-like B8, DnaJ-like subfamily B member 8; ERD10C, early responsive to dehydration 10C; GA, gibberellin; HSP70, 70-kDa heat shock protein; HSP81, heat shock protein 81.4; LEA, late embryogenesis abundant protein; NRX, nucleoredoxin; P5SC, Δ-1-pyrroline-carboxylate synthase; Prx, peroxiredoxin; SIP1, raffinose synthase; SOD, superoxide dismutase; SPS1F, sucrose phosphate synthase 1F; SUS3 and 4, sucrose synthase 3 and 4.
Proline is one of the most important osmoprotectants that can stabilize membrane and protein conformation by forming protective films with water molecules on their surface (Ozturk et al., 2021). In many grafted plants, proline was found accumulating in plant tissues to improve drought resistance (Liang et al., 2013; Penella et al., 2014; Ozturk et al., 2021). The proline accumulation in plant tissues under drought conditions can be obtained from the activation of proline biosynthesis, inactivation of proline degradation, protein hydrolysis, or oxidative inhibition of protein synthesis (Szabados and Savoure, 2010; Ozturk et al., 2021). In higher plants, the glutamate and the ornithine pathways of proline biosynthesis are known, with the former being the primary pathway in response to osmotic stress (Ozturk et al., 2021). Δ-1-pyrroline-carboxylate synthase (P5SC) encoded a key enzyme involved in proline anabolism that converts L-glutamate to glutamate γ-semialdehyde and was found to be up-regulated in the grafted plum plants. Thus, the glutamate pathway of proline biosynthesis was proven to be activated in grafted plants under water-deficit conditions (Jimenez et al., 2013). Additionally, when suffering from repeated-drought stress, the grafted plants continuously accumulated osmoregulatory substances by changing epigenetic modifications. For example, during the repeated-drought conditions, turnip rootstock altered the epigenetic modification of the Δ1-pyrroline-5-carboxylate synthetase 1-2 (P5CS1-2) gene and improved its expression, which led to proline accumulation, when compared with the none-grafted rapeseed (Luo et al., 2020). However, under recurring drought stressed conditions, how the rootstock signal mediates the histone H3K4me3 modification of the P5CS1-2 locus in the scion to regulate proline biosynthesis, is still not understood clearly.
The higher contents of soluble sugars and soluble proteins were found in the grafted poplar, tomato, and citrus plants under drought stress, which generated a stronger osmotic adjustment effect (Han et al., 2018; Zhang et al., 2020; Dong et al., 2021). Soluble proteins, e.g., late embryogenesis abundant (LEA) proteins and osmotins, are common osmoprotectants that stabilize cell membrane proteins and promote osmoregulation capacity (Ozturk et al., 2021). Many genes involved in soluble protein biosynthesis were found up-regulated in drought-resistant grafted plants. The LEA5 and early responsive to dehydration 10C (ERD10C) genes encoding dehydrins in the grafted tobacco plants and genes encoding osmotins in drought-resistant rootstocks were up-regulated during drought treatment (Kovacs et al., 2008; Witte et al., 2010; Liu et al., 2014; Yildirim et al., 2018; Yang et al., 2021). Soluble sugars, e.g., sucrose, trehalose, fructose, and fructan, can significantly reduce the cell osmotic potential, stabilize cellular membrane and protein conformation, or promote the osmotic adjustment and turgor maintenance as osmotic agents (Goncalves et al., 2019; Ozturk et al., 2021). Soluble sugars biosynthesis genes were also up-regulated in drought-resistant grafted plants under drought conditions. The raffinose synthase (SIP1) gene was found up-regulated in the grafted peach plants (Jimenez et al., 2013). Furthermore, the genes encoding starch branching enzymes increased the starch content in the cucumber/pumpkin grafted combination (Davoudi et al., 2022). The carbohydrate metabolism-related genes β-fructosidase 3 (BFRUCT3), sucrose phosphate synthase 1F (SPS1F), sucrose synthase 3 and 4 (SUS3 and SUS4) were upregulated in grafted drought-resistant rootstocks (Goncalves et al., 2019).
The concentrations of inorganic ions also affect osmotic regulation, as they are closely related to ion pumps (Yang et al., 2021). For example, the K+ pump can change the cell osmotic potential by regulating the inorganic ion concentration both inside and outside the cells (Yang et al., 2021). Thus, the accumulated inorganic ions (K+, Ca2+, and Mg2+) in grafted plants protect cell membrane integrity and enhance osmoregulatory capacity (Zhang et al., 2020). Additionally, the osmotic substances, like glycine betaine, sugar alcohols, and polyamines, are also drought-inducible. However, this phenomenon is poorly reported in grafted plants (Ozturk et al., 2021).
Antioxidative regulation
Drought leads to excessive ROS accumulation in plant chloroplast, mitochondria, and peroxisomes, thereby resulting in membrane peroxidation, enzyme inactivation, protein degradation, and even cell death (Dubey et al., 2021; Qamer et al., 2021). The grafted plants could improve drought resistance by activating the antioxidative defense system to scavenge the excess stress-induced ROS being generated (Figure 3) (Sanchez-Rodriguez et al., 2012).
Antioxidative enzymes
Oxidative damage is first alleviated by antioxidant enzymes including superoxide dismutase (SOD), catalase (CAT), ascorbate peroxidase (APX), and peroxidase (POD). It is a passive mechanism primarily mediated by ROS overproduction (Sucu et al., 2017; Dubey et al., 2021). Besides ROS, ABA and free polyamines could also activate the antioxidant enzymes in grafted plants during drought stress (Liu et al., 2016; Sanchez-Rodriguez et al., 2016). SOD is a metalloenzyme that catalyzes superoxide anion (O2-) molecules into oxygen (O2) and hydrogen peroxide (H2O2) (Laxa et al., 2019). CAT, APX, and POD can convert H2O2 into water (H2O) and O2 (Laxa et al., 2019). High activity and content of these enzymes alleviate oxidative stress by reducing ROS production in the scions of grafted plants with drought-resistant poplar, citrus, apple, cucumber, and tobacco rootstocks (Liu et al., 2012; Liu et al., 2014; Melnikova et al., 2017; Chen et al., 2020; Balfagon et al., 2021; Shehata et al., 2022). Furthermore, the high antioxidant enzymatic activity of these grafted plants can reduce chlorophyll decomposition, alleviate chloroplast membrane damage, and improve photosynthesis (Sanchez-Rodriguez et al., 2012; Zhang et al., 2019a). Several up-regulated genes related to antioxidative enzyme biosynthesis were detected in grafted plants having drought-resistant rootstocks. The SOD and APX genes were up-regulated in citrus grafted plants (Goncalves et al., 2019). The nucleoredoxin (NRX) encodes a protein that protects the CAT enzyme from abiotic stress-induced damage (Kneeshaw et al., 2017). The up-regulated NRX and CAT genes could strengthen the antioxidant system in the grafted cucumber/pumpkin combination (Davoudi et al., 2022). Additionally, the mobile mRNA, encoding peroxiredoxin (Prx) (an antioxidant cysteine-dependent peroxidase) was also identified in the grafted plants (Davoudi et al., 2022). We believe that drought stress-induced antioxidant system activation is a trait transmissible from the rootstock to the scion, which depends on scion-rootstock communication (Balfagon et al., 2021). Additionally, previous studies showed that drought-resistant plants induced greater activation of the ascorbate-dependent scavenging system. Contrastingly, the drought-sensitive plants only activated the glutathione-dependent scavenging system, with only moderate induction or even down-regulation of the ascorbate-dependent system (Laxa et al., 2019). When they used as rootstocks grafted onto the same scions, this different antioxidative activated mechanisms existed between two grafted combinations or not can be explored further in depth.
Non-enzymatic antioxidants
Drought resistance can also be improved by non-enzymatic antioxidants. Flavonoids, the common non-enzymatic antioxidants, could enhance antioxidative capacity (Lopez-Hinojosa et al., 2021). Studies have shown that most flavonoid biosynthesis-related genes were up-regulated in grafted plants (Lopez-Hinojosa et al., 2021). Furthermore, it has been reported that trehalose and raffinose in the citrus rootstock, and sugar alcohols (e.g., sorbitol) and amino acids (e.g., tyrosine, aspartic acid, methionine, ornithine, and tryptophan) in drought-resistant rootstocks could support the antioxidant system and scavenge ROS to protect the membrane integrity (Jimenez et al., 2013; Keunen et al., 2013; Lunn et al., 2014; Santana-Vieira et al., 2016; Sousa et al., 2022). It also indicated that the proline accumulation in the plants grafted with drought-resistant rootstocks could stabilize the membrane and proteins under water-deficient conditions (Jimenez et al., 2013; Schneider et al., 2018; Ozturk et al., 2021). Some photosynthesis-related molecules may play antioxidant roles in grafted plants. Decreased tetrapyrrole, the chlorophyll intermediates in grafted grapevine plants, could scavenge the excess ROS (Lucini et al., 2020). Carotenoid is also another well-known non-enzymatic antioxidant (Laxa et al., 2019). Upregulation of the chloroplast β-carotene hydroxylase (CHY1), encoding a carotenoid biosynthesis enzyme, indicated that the accumulated carotenoid could enhance osmoregulation in grafted citrus plants (Goncalves et al., 2019). DnaJ-like subfamily B member 8 (DnaJ-like B8) and Chaperone protein DnaJ (DnaJ), which was transported from the pumpkin rootstock to cucumber scion, helped prevent protein misfolding and aggregation, thus contributing to stress tolerance, redox maintenance and photosynthetic balance (Wang et al., 2014; Amiya and Shapira, 2021; Davoudi et al., 2022). The 70-kDa heat shock protein (HSP70) and heat shock protein 81.4 (HSP81) were transported from the pumpkin to the cucumber and they accumulated in the cucumber scions during drought stress to enhance membrane stability and detoxify the accumulating ROS (Ul Haq et al., 2019; Davoudi et al., 2022). Additionally, ascorbate (ASA), glutathione (GSH) and α-tocopherol are important plant non-enzymatic antioxidants, which need further exploration in grafted plants (Schneider et al., 2018; Hasanuzzaman et al., 2019).
Summary and outlook
The selection of suitable rootstocks or scions is crucial in generating the drought resistance of grafted plants. Besides enabling the grafted plants to regulate general morphological, physiological, and molecular processes and allow adaptive changes to improve drought resistance, the graft-responsive genes, especially through the exchange of genetic information between rootstocks and scions, are important in improving plant performance under drought stress. It also is vital in promoting water use efficiency, osmoregulation, anti-oxidant-mediated stress tolerance, etc. Furthermore, future research can focus more on the improvement of drought resistance of grafted trees, which is a potential research avenue that can be applied in arid and semi-arid areas. Moreover, with the increasing in-depth research, we believe that the scion-rootstock communication is a complex bidirectional feedback loop that is crucial for enhancing drought resistance via transport molecules. Therefore, studying the scion-rootstock communication holds the key to future understanding and improving plant drought resistance.
Author contributions
LY collected and analyzed the data, and written the manuscript. LX and YZ collected some data. QH and SZ designed the framework, given some advises and revised the manuscript. All authors read and approved the final manuscript.
Funding
This work was supported by the National Natural Science Foundation of China (32101478), the Second Tibetan Plateau Scientific Expedition and Research Program (2019QZKK0404) and China Postdoctoral Science Foundation (2021M702360).
Acknowledgments
The authors would like to express their gratitude to EditSprings (https://www.editsprings.cn) for the expert linguistic services provided.
Conflict of interest
The authors declare that the research was conducted in the absence of any commercial or financial relationships that could be construed as a potential conflict of interest.
Publisher’s note
All claims expressed in this article are solely those of the authors and do not necessarily represent those of their affiliated organizations, or those of the publisher, the editors and the reviewers. Any product that may be evaluated in this article, or claim that may be made by its manufacturer, is not guaranteed or endorsed by the publisher.
Supplementary material
The Supplementary Material for this article can be found online at: https://www.frontiersin.org/articles/10.3389/fpls.2022.1015317/full#supplementary-material
References
Abdulaziz, A. H., Abdulrasoul, A. O., Thabet, A., Hesham, A. R., Khadejah, A., Saad, M., et al. (2017). Tomato grafting impacts on yield and fruit quality under water stress conditions. J. Exp. Bio. Agric. Sci. 5, 136–147. doi: 10.18006/2017.5(Spl-1-SAFSAW).S136.S147
Acharya, B. R., Assmann, S. M. (2009). Hormone interactions in stomatal function. Plant Mol. Biol. 69, 451–462. doi: 10.1007/s11103-008-9427-0
Afzal, Z., Howton, T. C., Sun, Y., Mukhtar, M. S. (2016). The roles of aquaporins in plant stress responses. J. Dev. Biol. 4, 9. doi: 10.3390/jdb4010009
Agarwal, P. K., Agarwal, P., Reddy, M. K., Sopory, S. K. (2006). Role of DREB transcription factors in abiotic and biotic stress tolerance in plants. Plant Cell Rep. 25, 1263–1274. doi: 10.1007/s00299-006-0204-8
Ahmed, C. B., Rouina, B. B., Sensoy, S., Boukhris, M., Abdallah, F. B. (2009). Changes in gas exchange, proline accumulation and antioxidative enzyme activities in three olive cultivars under contrasting water availability regimes. Environ. Exp. Bot. 67, 345–352. doi: 10.1016/j.envexpbot.2009.07.006
Alan, O., Ozdemir, N., Gunen, Y. (2007). Effect of grafting on watermelon plant growth, yield and quality. J. Agron. 6, 362–365. doi: 10.3923/ja.2007.362.365
Al-Harbi, A. R., Al-Omran, A. M., Alqardaeai, T. A., Abdel-Rassak, H. S., Alharbi, K. R., Obadi, A., et al. (2018). Grafting affects tomato growth, productivity, and water use efficiency under different water regimes. J. Agr. Sci. Tech. 20, 1227–1241. Available at: http://jast.modares.ac.ir/article-23-20094-en.html
Ali, S., Hayat, K., Iqbal, A., Xie, L. (2020). Implications of abscisic acid in the drought stress tolerance of plants. Agronomy 10. doi: 10.3390/agronomy10091323
Ali, P., Jabe, P., Davoud, Z. (2019). Assessment of iranian rainfed and seedy watermelon landraces as potential rootstocks for enhancing drought tolerance. Hortic. Sci. Technol. 37, 354–364. doi: 10.7235/HORT.20190036
Allario, T., Brumos, J., Colmenero-Flores, J. M., Iglesias, D. J., Pina, J. A., Navarro, L., et al. (2013). Tetraploid rangpur lime rootstock increases drought tolerance via enhanced constitutive root abscisic acid production. Plant Cell Environ. 36, 856–868. doi: 10.1111/pce.12021
Alsina, M. M., Smart, D. R., Bauerle, T., de Herralde, F., Biel, C., Stockert, C., et al. (2011). Seasonal changes of whole root system conductance by a drought-tolerant grape root system. J. Exp. Bot. 62, 99–109. doi: 10.1093/jxb/erq247
Alves, F. M., Joshi, M., Djidonou, D., Joshi, V., Gomes, C. N., Leskovar, D. I. (2021). Physiological and biochemical responses of tomato plants grafted onto Solanum pennellii and Solanum peruvianum under water-deficit conditions. Plants (Basel) 10, 2236. doi: 10.3390/plants10112236
Amiya, R., Shapira, M. (2021). ZnJ6 is a thylakoid membrane DnaJ-like chaperone with oxidizing activity in Chlamydomonas reinhardtii. Int. J. Mol. Sci. 22, 1136. doi: 10.3390/ijms22031136
Balfagon, D., Teran, F., de Oliveira, T. D. R., Santa-Catarina, C., Gomez-Cadenas, A. (2021). Citrus rootstocks modify scion antioxidant system under drought and heat stress combination. Plant Cell Rep. 41, 593–602. doi: 10.1007/s00299-021-02744-y
Bandurska, H., Stroinski, A. (2005). The effect of salicylic acid on barley response to water deficit. Acta Physiol. Plant 27, 379–386. doi: 10.1007/s11738-005-0015-5
Bauerle, T. L., Centinari, M., Bauerle, W. L. (2011). Shifts in xylem vessel diameter and embolisms in grafted apple trees of differing rootstock growth potential in response to drought. Planta 234, 1045–1054. doi: 10.1007/s00425-011-1460-6
Bikdeloo, M., Colla, G., Rouphael, Y., Hassandokht, M. R., Soltani, F., Salehi, R., et al. (2021). Morphological and physio-biochemical responses of watermelon grafted onto rootstocks of wild watermelon [Citrullus colocynthis (L.) schrad] and commercial interspecific cucurbita hybrid to drought stress. Horticulturae 7, 359. doi: 10.3390/horticulturae7100359
Camisón, Á., Martín, M.Á., Flors, V., Sánchez-Bel, P., Pinto, G., Vivas, M., et al. (2021). Exploring the use of scions and rootstocks from xeric areas to improve drought tolerance in Castanea sativa miller. Environ. Exp. Bot. 187, 104467. doi: 10.1016/j.envexpbot.2021.104467
Cantero-Navarro, E., Romero-Aranda, R., Fernandez-Munoz, R., Martinez-Andujar, C., Perez-Alfocea, F., Albacete, A. (2016). Improving agronomic water use efficiency in tomato by rootstock-mediated hormonal regulation of leaf biomass. Plant Sci. 251, 90–100. doi: 10.1016/j.plantsci.2016.03.001
Cerruti, E., Gisbert, C., Drost, H. G., Valentino, D., Portis, E., Barchi, L., et al. (2021). Grafting vigour is associated with DNA de-methylation in eggplant. Hortic. Res. 8, 241. doi: 10.1038/s41438-021-00660-6
Chaimala, A., Jogloy, S., Vorasoot, N., Toomsan, B., Jongrungklang, N., Kesmala, T., et al. (2020). Responses of total biomass, shoot dry weight, yield and yield components of Jerusalem artichoke (Helianthus tuberosus l.) varieties under different terminal drought duration. Agriculture 10. doi: 10.3390/agriculture10060198
Chambaud, C., Cookson, S. J., Ollat, N., Bayer, E., Brocard, L. (2022). A correlative light electron microscopy approach reveals plasmodesmata ultrastructure at the graft interface. Plant Physiol. 188, 44–55. doi: 10.1093/plphys/kiab485
Chen, C. Z., Lv, X. F., Li, J. Y., Yi, H. Y., Gong, J. M. (2012). Arabidopsis NRT1.5 is another essential component in the regulation of nitrate reallocation and stress tolerance. Plant Physiol. 159, 1582–1590. doi: 10.1104/pp.112.199257
Chen, Y., Sun, X., Zheng, C., Zhang, S., Yang, J. (2018). Grafting onto Artemisia annua improves drought tolerance in Chrysanthemum by enhancing photosynthetic capacity. Hortic. Plant J. 4, 117–125. doi: 10.1016/j.hpj.2018.03.008
Chen, S., Yi, L., Korpelainen, H., Yu, F., Liu, M. (2020). Roots play a key role in drought-tolerance of poplars as suggested by reciprocal grafting between male and female clones. Plant Physiol. Bioch. 153, 81–91. doi: 10.1016/j.plaphy.2020.05.014
Čolić, S. D., Bakić, I. V., Dabić Zagorac, D.Č., Natić, M. M., Smailagić, A. T., Pergal, M. V., et al. (2021). Chemical fingerprint and kernel quality assessment in different grafting combinations of almond under stress condition. Sci. Hortic-Amsterdam 275, 10975. doi: 10.1016/j.scienta.2020.109705
Davoudi, M., Song, M., Zhang, M., Chen, J., Lou, Q. (2022). Long-distance control of pumpkin rootstock over cucumber scion under drought stress as revealed by transcriptome sequencing and mobile mRNAs identifications. Hortic. Res. 9, uhab033. doi: 10.1093/hr/uhab033
Dong, T., Xi, L., Xiong, B., Qiu, X., Huang, S., Xu, W., et al. (2021). Drought resistance in harumi tangor seedlings grafted onto different rootstocks. Funct. Plant Biol. 48, 529–541. doi: 10.1071/FP20242
Dubey, A., Kumar, A., Malla, M. A., Chowdhary, K., Singh, G., Ravikanth, G., et al. (2021). Approaches for the amelioration of adverse effects of drought stress on crop plants. Front. Biosci. (Landmark Ed) 26, 928–947. doi: 10.52586/4998
Du, H., Huang, F., Wu, N., Li, X., Hu, H., Xiong, L. (2018). Integrative regulation of drought escape through ABA-dependent and -independent pathways in rice. Mol. Plant 11, 584–597. doi: 10.1016/j.molp.2018.01.004
Ellenberger, J., Bulut, A., Blömeke, P., Röhlen-Schmittgen, S. (2021). Novel S. pennellii × S. lycopersicum hybrid rootstocks for tomato production with reduced water and nutrient supply. Horticulturae 7, 355. doi: 10.3390/horticulturae7100355
Ellialtioglu, S. S., Talhouni, M., Kiran, S., Ates, C., Kusvuran, S. (2019). Antioxidative response of grafted and non-grafted eggplant seedlings under drought and salt stresses. Agrochimica 63, 123–137. doi: 10.12871/00021857201922
El-Mogy, M. M., Atia, M. A. M., Dhawi, F., Fouad, A. S., Bendary, E. S. A., Khojah, E., et al. (2022). Towards better grafting: SCoT and CDDP analyses for prediction of the tomato rootstocks performance under drought stress. Agronomy 12, 153. doi: 10.3390/agronomy12010153
Farooq, M., Wahid, A., Lee, D. J., Ito, O., Siddique, K. H. M. (2009). Advances in drought resistance of rice. Crit. Rev. Plant Sci. 28, 199–217. doi: 10.1080/07352680902952173
Flexas, J., Bota, J., Loreto, F., Cornic, G., Sharkey, T. D. (2004). Diffusive and metabolic limitations to photosynthesis under drought and salinity in C(3) plants. Plant Biol. (Stuttg) 6, 269–279. doi: 10.1055/s-2004-820867
Flexas, J., Medrano, H. (2002). Drought-inhibition of photosynthesis in C3 plants: stomatal and non-stomatal limitations revisited. Ann. Bot. 89, 183–189. doi: 10.1093/aob/mcf027
Fullana-Pericàs, M., Conesa, M.À., Ribas-Carbó, M., Galmés, J. (2020). The use of a tomato landrace as rootstock improves the response of commercial tomato under water deficit conditions. Agronomy 10, 748. doi: 10.3390/agronomy10050748
Fullana-Pericàs, M., Ponce, J., Conesa, M.À., Juan, A., Ribas-Carbó, M., Galmés, J. (2018). Changes in yield, growth and photosynthesis in a drought-adapted Mediterranean tomato landrace (Solanum lycopersicum ‘Ramellet’) when grafted onto commercial rootstocks and Solanum pimpinellifolium. Sci. Hort-Amsterdam 233, 70–77. doi: 10.1016/j.scienta.2018.01.045
Gaion, L. A., Monteiro, C. C., Cruz, F. J. R., Rossatto, D. R., Lopez-Diaz, I., Carrera, E., et al. (2018). Constitutive gibberellin response in grafted tomato modulates root-to-shoot signaling under drought stress. J. Plant Physiol. 221, 11–21. doi: 10.1016/j.jplph.2017.12.003
Gautam, A., Pandey, A. K. (2021). Aquaporins responses under challenging environmental conditions and abiotic stress tolerance in plants. Botanical Rev. 87, 467–495. doi: 10.1007/s12229-021-09249-z
Gisbert-Mullor, R., Pascual-Seva, N., Martínez-Gimeno, M. A., López-Serrano, L., Badal Marín, E., Pérez-Pérez, J. G., et al. (2020). Grafting onto an appropriate rootstock reduces the impact on yield and quality of controlled deficit irrigated pepper crops. Agronomy 10, 1529. doi: 10.3390/agronomy10101529
Goncalves, L. P., Boscariol Camargo, R. L., Takita, M. A., Machado, M. A., Dos Soares Filho, W. S., Costa, M. G. C. (2019). Rootstock-induced molecular responses associated with drought tolerance in sweet orange as revealed by RNA-seq. BMC Genomics 20, 110. doi: 10.1186/s12864-019-5481-z
Gong, X., Liu, M., Zhang, L., Ruan, Y., Ding, R., Ji, Y., et al. (2015). Arabidopsis AtSUC2 and AtSUC4, encoding sucrose transporters, are required for abiotic stress tolerance in an ABA-dependent pathway. Physiol. Plant 153, 119–136. doi: 10.1111/ppl.12225
Gullo, G., Dattola, A., Vonella, V., Zappia, R. (2018). Evaluation of water relation parameters in vitis rootstocks with different drought tolerance and their effects on growth of a grafted cultivar. J. Plant Physiol. 226, 172–178. doi: 10.1016/j.jplph.2018.04.013
Guo, T., Wang, N., Xue, Y., Guan, Q., van Nocker, S., Liu, C., et al. (2019). Overexpression of the RNA binding protein MhYTP1 in transgenic apple enhances drought tolerance and WUE by improving ABA level under drought condition. Plant Sci. 280, 397–407. doi: 10.1016/j.plantsci.2018.11.018
Gupta, A., Rico-Medina, A., Caño-Delgado, A. I. (2020). The physiology of plant responses to drought. Science 368, 266–269. doi: 10.1126/science.aaz7614
Habibi, F., Liu, T., Folta, K., Sarkhosh, A. (2022). Physiological, biochemical, and molecular aspects of grafting in fruit trees. Hortic. Res. 9, uhac032. doi: 10.1093/hr/uhac032
Han, Q., Guo, Q., Korpelainen, H., Niinemets, U., Li, C. (2019). Rootstock determines the drought resistance of poplar grafting combinations. Tree Physiol. 39, 1855–1866. doi: 10.1093/treephys/tpz102
Han, Q., Luo, J., Li, Z., Korpelainen, H., Li, C. (2018). Improved drought resistance by intergeneric graftingin salicaceae plants under water deficits. Environ. Exp. Bot. 155, 217–225. doi: 10.1016/j.envexpbot.2018.07.003
Han, Y., Wang, Y., Jiang, H., Wang, M., Korpelainen, H., Li, C. (2013). Reciprocal grafting separates the roles of the root and shoot in sex-related drought responses in populus cathayana males and females. Plant Cell Environ. 36, 356–364. doi: 10.1111/j.1365-3040.2012.02578.x
Hao, L., Zhang, Y., Wang, S., Zhang, W., Wang, S., Xu, C., et al. (2020). A constitutive and drought-responsive mRNA undergoes long-distance transport in pear (Pyrus betulaefolia) phloem. Plant Sci. 293, 110419. doi: 10.1016/j.plantsci.2020.110419
Hasanuzzaman, M., Bhuyan, M., Anee, T. I., Parvin, K., Nahar, K., Mahmud, J. A., et al. (2019). Regulation of ascorbate-glutathione pathway in mitigating oxidative damage in plants under abiotic stress. Antioxid. (Basel) 8, 384. doi: 10.3390/antiox8090384
Huang, J., Yu, H., Guan, X., Wang, G., Guo, R. (2015). Accelerated dryland expansion under climate change. Nat. Clim. Change 6, 166–171. doi: 10.1038/nclimate2837
Jiang, L., Shen, W., Liu, C., Tahir, M. M., Li, X., Zhou, S., et al. (2022). Engineering drought-tolerant apple by knocking down six GH3 genes and potential application of transgenic apple as a rootstock. Hortic. Res. 9, uhac122. doi: 10.1093/hr/uhac122
Jimenez, S., Dridi, J., Gutierrez, D., Moret, D., Irigoyen, J. J., Moreno, M. A., et al. (2013). Physiological, biochemical and molecular responses in four prunus rootstocks submitted to drought stress. Tree Physiol. 33, 1061–1075. doi: 10.1093/treephys/tpt074
Ji, J., Yang, L., Fang, Z., Zhang, Y., Zhuang, M., Lv, H., et al. (2022). Plant SWEET family of sugar transporters: structure, evolution and biological functions. Biomolecules 12, 205. doi: 10.3390/biom12020205
Karunakaran, R., Ilango, R. V. J. (2019). Grafting influence on productivity and drought tolerance of tea clones. J. Agr. Sci-cambridag. 157, 217–225. doi: 10.1017/s0021859619000480
Keunen, E., Peshev, D., Vangronsveld, J., Van Den Ende, W., Cuypers, A. (2013). Plant sugars are crucial players in the oxidative challenge during abiotic stress: extending the traditional concept. Plant Cell Environ. 36, 1242–1255. doi: 10.1111/pce.12061
Khadivi-Khub, A., Anjam, K. (2016). Prunus scoparia, a suitable rootstock for almond (Prunus dulcis) under drought condition based on vegetative and fruit characteristics. Sci. Hort-Amsterdam 210, 220–226. doi: 10.1016/j.scienta.2016.07.028
Kneeshaw, S., Keyani, R., Delorme-Hinoux, V., Imrie, L., Loake, G. J., Le Bihan, T., et al. (2017). Nucleoredoxin guards against oxidative stress by protecting antioxidant enzymes. Proc. Natl. Acad. Sci. U. S. A. 114, 8414–8419. doi: 10.1073/pnas.1703344114
Kovacs, D., Kalmar, E., Torok, Z., Tompa, P. (2008). Chaperone activity of ERD10 and ERD14, two disordered stress-related plant proteins. Plant Physiol. 147, 381–390. doi: 10.1104/pp.108.118208
Ksouri, N., Jimenez, S., Wells, C. E., Contreras-Moreira, B., Gogorcena, Y. (2016). Transcriptional responses in root and leaf of prunus persica under drought stress using RNA sequencing. Front. Plant Sci. 7, 1715. doi: 10.3389/fpls.2016.01715
Kumar, R. M. S., Ji, G., Guo, H., Zhao, L., Zheng, B. (2018). Over-expression of a grafting-responsive gene from hickory increases abiotic stress tolerance in arabidopsis. Plant Cell Rep. 37, 541–552. doi: 10.1007/s00299-018-2250-4
Kumar, P., Rouphael, Y., Cardarelli, M., Colla, G. (2017). Vegetable grafting as a tool to improve drought resistance and water use efficiency. Front. Plant Sci. 8, 1130. doi: 10.3389/fpls.2017.01130
Kurotani, K. I., Notaguchi, M. (2021). Cell-to-cell connection in plant grafting-molecular insights into symplasmic reconstruction. Plant Cell Physiol. 62, 1362–1371. doi: 10.1093/pcp/pcab109
Laxa, M., Liebthal, M., Telman, W., Chibani, K., Dietz, K. J. (2019). The role of the plant antioxidant system in drought tolerance. Antioxid. (Basel) 8, 94. doi: 10.3390/antiox8040094
Lee, S. B., Jung, S. J., Go, Y. S., Kim, H. U., Kim, J. K., Cho, H. J., et al. (2009). Two arabidopsis 3-ketoacyl CoA synthase genes, KCS20 and KCS2/DAISY, are functionally redundant in cuticular wax and root suberin biosynthesis, but differentially controlled by osmotic stress. Plant J. 60, 462–475. doi: 10.1111/j.1365-313X.2009.03973.x
Liang, X., Zhang, L., Natarajan, S. K., Becker, D. F. (2013). Proline mechanisms of stress survival. Antioxid. Redox Sign. 19, 998–1011. doi: 10.1089/ars.2012.5074
Li, S., Cao, Y., Wang, C., Sun, X., Wang, W., Song, S. (2020). Contribution of different genotypic roots to drought resistance in soybean by a grafting experiment. Plant Prod. Sci. 24, 317–325. doi: 10.1080/1343943x.2020.1839350
Li, C., Liu, C., Ma, X., Wang, A., Duan, R., Nawrath, C., et al. (2015). Characterization and genetic mapping of eceriferum-ym (cer-ym), a cutin deficient barley mutant with impaired leaf water retention capacity. Breed Sci. 65, 327–332. doi: 10.1270/jsbbs.65.327
Liu, B. H., Cheng, L., Ma, F. W., Liang, D., Zou, Y. J. (2012). Influence of rootstock on drought response in young ‘Gale gala’ apple (Malus domestica borkh.) trees. J. Sci. Food Agric. 92, 2421–2427. doi: 10.1002/jsfa.5647
Liu, S., Li, H., Lv, X., Ahammed, G. J., Xia, X., Zhou, J., et al. (2016). Grafting cucumber onto luffa improves drought tolerance by increasing ABA biosynthesis and sensitivity. Sci. Rep. 6, 20212. doi: 10.1038/srep20212
Liu, J., Li, J., Su, X., Xia, Z. (2014). Grafting improves drought tolerance by regulating antioxidant enzyme activities and stress-responsive gene expression in tobacco. Environ. Exp. Bot. 107, 173–179. doi: 10.1016/j.envexpbot.2014.06.012
Liu, W., Wang, Y., Gong, X., Li, S., Smoak, J. M., Duan, B. (2019). Changes in growth and soil microbial communities in reciprocal grafting clones between populus deltoides males and females exposed to water deficit conditions. Ann. For. Sci. 76, 118. doi: 10.1007/s13595-019-0897-9
Li, S. Y., Wang, W. B., Yao, X. D., Wang, C. L., Cao, Y. Q., Zhang, L. J., et al. (2019). Photosynthesis in reciprocal grafts of drought-tolerant and drought-sensitive cultivars of soybean under water stress. Photosynthetica 57, 942–949. doi: 10.32615/ps.2019.109
Li, D., Zhang, H., Hong, Y., Huang, L., Li, X., Zhang, Y., et al. (2014). Genome-wide identification, biochemical characterization, and expression analyses of the YTH domain-containing RNA-binding protein family in arabidopsis and rice. Plant Mol. Bio. Rep. 32, 1169–1186. doi: 10.1007/s11105-014-0724-2
Lopez-Hinojosa, M., de Maria, N., Guevara, M. A., Velez, M. D., Cabezas, J. A., Diaz, L. M., et al. (2021). Rootstock effects on scion gene expression in maritime pine. Sci. Rep. 11, 11582. doi: 10.1038/s41598-021-90672-y
López-Marín, J., Gálvez, A., del Amor, F. M., Albacete, A., Fernández, J. A., Egea-Gilabert, C., et al. (2017). Selecting vegetative/generative/dwarfing rootstocks for improving fruit yield and quality in water stressed sweet peppers. Sci. Hortic-Amsterdam 214, 9–17. doi: 10.1016/j.scienta.2016.11.012
Lopez-Serrano, L., Canet-Sanchis, G., Vuletin Selak, G., Penella, C., San Bautista, A., Lopez-Galarza, S., et al. (2019). Pepper rootstock and scion physiological responses under drought stress. Front. Plant Sci. 10, 38. doi: 10.3389/fpls.2019.00038
Lucini, L., Miras-Moreno, B., Busconi, M., Marocco, A., Gatti, M., Poni, S. (2020). Molecular basis of rootstock-related tolerance to water deficit in Vitis vinifera L. cv. sangiovese: A physiological and metabolomic combined approach. Plant Sci. 299, 110600. doi: 10.1016/j.plantsci.2020.110600
Lunn, J. E., Delorge, I., Figueroa, C. M., Van Dijck, P., Stitt, M. (2014). Trehalose metabolism in plants. Plant J. 79, 544–567. doi: 10.1111/tpj.12509
Luo, L., Zheng, Y., Gao, Z., Chen, Q., Kong, X., Yang, Y. (2020). Grafting improves drought stress memory by increasing the P5CS1 gene expression in brassica rapa. Plant Soil 452, 61–72. doi: 10.1007/s11104-020-04547-8
Marguerit, E., Brendel, O., Lebon, E., Van Leeuwen, C., Ollat, N. (2012). Rootstock control of scion transpiration and its acclimation to water deficit are controlled by different genes. New Phytol. 194, 416–429. doi: 10.1111/j.1469-8137.2012.04059.x
Melnikova, N. V., Borkhert, E. V., Snezhkina, A. V., Kudryavtseva, A. V., Dmitriev, A. A. (2017). Sex-specific response to stress in populus. Front. Plant Sci. 81827. doi: 10.3389/fpls.2017.01827
Melnyk, C. W., Gabel, A., Hardcastle, T. J., Robinson, S., Miyashima, S., Grosse, I., et al. (2018). Transcriptome dynamics at arabidopsis graft junctions reveal an intertissue recognition mechanism that activates vascular regeneration. Proc. Natl. Acad. Sci. U. S. A. 115, E2447–E2456. doi: 10.1073/pnas.1718263115
Melnyk, C. W., Meyerowitz, E. M. (2015). Plant grafting. Curr. Biol. 25, R183–R188. doi: 10.1016/j.cub.2015.01.029
Muhammad Aslam, M., Waseem, M., Jakada, B. H., Okal, E. J., Lei, Z., Saqib, H. S. A., et al. (2022). Mechanisms of abscisic acid-mediated drought stress responses in plants. Int. J. Mol. Sci. 23, 1084. doi: 10.3390/ijms23031084
Notaguchi, M., Higashiyama, T., Suzuki, T. (2015). Identification of mRNAs that move over long distances using an RNA-seq analysis of Arabidopsis/Nicotiana benthamiana heterografts. Plant Cell Physiol. 56, 311–321. doi: 10.1093/pcp/pcu210
Notaguchi, M., Kurotani, K. I., Sato, Y., Tabata, R., Kawakatsu, Y., Okayasu, K., et al. (2020). Cell-cell adhesion in plant grafting is facilitated by β-1,4-glucanases. Science 369, 698–702. doi: 10.1126/science.abc3710
Okamoto, S., Kawasaki, A., Makino, Y., Ishida, T., Sawa, S. (2022). Long-distance translocation of CLAVATA3/ESR-related 2 peptide and its positive effect on root sucrose status. Plant Physiol. 189, 2357–2367. doi: 10.1093/plphys/kiac227
Opazo, I., Toro, G., Salvatierra, A., Pastenes, C., Pimentel, P. (2020). Rootstocks modulate the physiology and growth responses to water deficit and long-term recovery in grafted stone fruit trees. Agr. Water Manage 228, 105897. doi: 10.1016/j.agwat.2019.105897
Ozturk, M., Turkyilmaz Unal, B., Garcia-Caparros, P., Khursheed, A., Gul, A., Hasanuzzaman, M. (2021). Osmoregulation and its actions during the drought stress in plants. Physiol. Plant 172, 1321–1335. doi: 10.1111/ppl.13297
Pan, W., Lin, B., Yang, X., Liu, L., Xia, R., Li, J., et al. (2020). The UBC27-AIRP3 ubiquitination complex modulates ABA signaling by promoting the degradation of ABI1 in arabidopsis. Proc. Natl. Acad. Sci. U. S. A. 117, 27694–27702. doi: 10.1073/pnas.2007366117
Penella, C., Nebauer, S. G., Bautista, A. S., Lopez-Galarza, S., Calatayud, A. (2014). Rootstock alleviates PEG-induced water stress in grafted pepper seedlings: physiological responses. J. Plant Physiol. 171, 842–851. doi: 10.1016/j.jplph.2014.01.013
Poudyal, D., Akash, M., Khatri, L., Shrestha, D. S., Uptmoor, R. (2017). Solanum habrochaites introgression line grafted as rootstock in cultivated tomato maintains growth and improves yield under cold and drought stresses. J. Crop Improvement 31, 589–607. doi: 10.1080/15427528.2017.1319887
Prinsi, B., Simeoni, F., Galbiati, M., Meggio, F., Tonelli, C., Scienza, A., et al. (2021). Grapevine rootstocks differently affect physiological and molecular responses of the scion under water deficit condition. Agronomy 11, 289. doi: 10.3390/agronomy11020289
Proietti, S., Rouphael, Y., Colla, G., Cardarelli, M., De Agazio, M., Zacchini, M., et al. (2008). Fruit quality of mini-watermelon as affected by grafting and irrigation regimes. J. Sci. Food Agric. 88, 1107–1114. doi: 10.1002/jsfa.3207
Qamer, Z., Chaudhary, M. T., Du, X., Hinze, L., Azhar, M. T. (2021). Review of oxidative stress and antioxidative defense mechanisms in Gossypium hirsutum L. in response to extreme abiotic conditions. J. Cotton Res. 4, 9. doi: 10.1186/s42397-021-00086-4
Qiu, X. M., Sun, Y. Y., Ye, X. Y., Li, Z. G. (2019). Signaling role of glutamate in plants. Front. Plant Sci. 101743. doi: 10.3389/fpls.2019.01743
Rouphael, Y., Cardarelli, M., Colla, G., Rea, E. (2008). Yield, mineral composition, water relations, and water use efficiency of grafted mini-watermelon plants under deficit irrigation. Hortscience 43, 730–736. doi: 10.21273/HORTSCI.43.3.730
Sabir, A., Kucukbasmaci, A. (2019). Long-term impact of deficit irrigation on the physiology and growth of grapevine cv. ‘Prima’ grafted on various rootstocks. Acta Sci. Pol-Hortoru. 18, 57–70. doi: 10.24326/asphc.2019.4.6
Sánchez-Rodríguez, E., Leyva, R., Constán-Aguilar, C., Romero, L., Ruiz, J. M. (2012). Grafting under water stress in tomato cherry: improving the fruit yield and quality. Ann. Appl. Biol. 161, 302–312. doi: 10.1111/j.1744-7348.2012.00574.x
Sánchez-Rodríguez, E., Leyva, R., Constán-Aguilar, C., Romero, L., Ruiz, J. M. (2014). How does grafting affect the ionome of cherry tomato plants under water stress? Soil Sci. Plant Nutr. 60, 145–155. doi: 10.1080/00380768.2013.870873
Sánchez-Rodríguez, E., Romero, L., Ruiz, J. M. (2013). Role of grafting in resistance to water stress in tomato plants: Ammonia production and assimilation. J. Plant Growth Regul. 32, 831–842. doi: 10.1007/s00344-013-9348-2
Sanchez-Rodriguez, E., Romero, L., Ruiz, J. M. (2016). Accumulation of free polyamines enhances the antioxidant response in fruits of grafted tomato plants under water stress. J. Plant Physiol. 190, 72–78. doi: 10.1016/j.jplph.2015.10.010
Sanchez-Rodriguez, E., Rubio-Wilhelmi Mdel, M., Blasco, B., Leyva, R., Romero, L., Ruiz, J. M. (2012). Antioxidant response resides in the shoot in reciprocal grafts of drought-tolerant and drought-sensitive cultivars in tomato under water stress. Plant Sci. 188, 89–96. doi: 10.1016/j.plantsci.2011.12.019
Santana-Vieira, D. D., Freschi, L., Almeida, L. A., Moraes, D. H., Neves, D. M., Santos, L. M., et al. (2016). Survival strategies of citrus rootstocks subjected to drought. Sci. Rep. 6, 38775. doi: 10.1038/srep38775
Sarwat, M., Tuteja, N. (2017). Hormonal signaling to control stomatal movement during drought stress. Plant Gene 11, 143–153. doi: 10.1016/j.plgene.2017.07.007
Savvas, D., Colla, G., Rouphael, Y., Schwarz, D. (2010). Amelioration of heavy metal and nutrient stress in fruit vegetables by grafting. Sci. Hortic-Amsterdam 127, 156–161. doi: 10.1016/j.scienta.2010.09.011
Schneider, J. R., Caverzan, A., Chavarria, G. (2018). Water deficit stress, ROS involvement, and plant performance. Arch. Agron. Soil Sci. 65, 1160–1181. doi: 10.1080/03650340.2018.1556789
Sels, J., Mathys, J., De Coninck, B. M., Cammue, B. P., De Bolle, M. F. (2008). Plant pathogenesis-related (PR) proteins: a focus on PR peptides. Plant Physiol. Biochem. 46, 941–950. doi: 10.1016/j.plaphy.2008.06.011
Seo, M., Koshiba, T. (2002). Complex regulation of ABA biosynthesis in plants. Trends Plant Sci. 7, 41–48. doi: 10.1016/S1360-1385(01)02187-2
Seymen, M., Yavuz, D., Ercan, M., Akbulut, M., Çoklar, H., Kurtar, E. S., et al. (2021). Effect of wild watermelon rootstocks and water stress on chemical properties of watermelon fruit. Hortic. Environ. Biote. 62, 411–422. doi: 10.1007/s13580-020-00329-4
Shao, H. B., Chu, L. Y., Jaleel, C. A., Zhao, C. X. (2008). Water-deficit stress-induced anatomical changes in higher plants. C. R. Biol. 331, 215–225. doi: 10.1016/j.crvi.2008.01.002
Sharma, A., Wang, J., Xu, D., Tao, S., Chong, S., Yan, D., et al. (2020). Melatonin regulates the functional components of photosynthesis, antioxidant system, gene expression, and metabolic pathways to induce drought resistance in grafted carya cathayensis plants. Sci. Total Environ. 713, 136675. doi: 10.1016/j.scitotenv.2020.136675
Shehata, S. A., Omar, H. S., Elfaidy, A. G. S., El-Sayed, S. S. F., Abuarab, M. E., Abdeldaym, E. A. (2022). Grafting enhances drought tolerance by regulating stress-responsive gene expression and antioxidant enzyme activities in cucumbers. BMC Plant Biol. 22, 408. doi: 10.1186/s12870-022-03791-7
Shen, X., He, J., Ping, Y., Guo, J., Hou, N., Cao, F., et al. (2022). The positive feedback regulatory loop of miR160-auxin response factor 17-HYPONASTIC LEAVES 1 mediates drought tolerance in apple trees. Plant Physiol. 188, 1686–1708. doi: 10.1093/plphys/kiab565
Shi, H., Chen, L., Ye, T., Liu, X., Ding, K., Chan, Z. (2014). Modulation of auxin content in arabidopsis confers improved drought stress resistance. Plant Physiol. Biochem. 82, 209–217. doi: 10.1016/j.plaphy.2014.06.008
Shivaraj, S. M., Sharma, Y., Chaudhary, J., Rajora, N., Sharma, S., Thakral, V., et al. (2021). Dynamic role of aquaporin transport system under drought stress in plants. Environ. Exp. Bot. 184, 104367. doi: 10.1016/j.envexpbot.2020.104367
Silva, S. F., Miranda, M. T., Costa, V. E., Machado, E. C., Ribeiro, R. V. (2021). Sink strength of citrus rootstocks under water deficit. Tree Physiol. 41, 1372–1383. doi: 10.1093/treephys/tpab008
Silva, V. A., Prado, F. M., Antunes, W. C., Paiva, R. M. C., Ferrão, M. A. G., Andrade, A. C., et al. (2018). Reciprocal grafting between clones with contrasting drought tolerance suggests a key role of abscisic acid in coffee acclimation to drought stress. Plant Growth Regul. 85, 221–229. doi: 10.1007/s10725-018-0385-5
Soma, F., Takahashi, F., Yamaguchi-Shinozaki, K., Shinozaki, K. (2021). Cellular phosphorylation signaling and gene expression in drought stress responses: ABA-dependent and ABA-independent regulatory systems. Plants (Basel) 10, 10756. doi: 10.3390/plants10040756
Sousa, A. R., d., O., d. A., E. M., Filho, M. A. C., Costa, M. G. C., Filho, W., et al. (2022). Metabolic responses to drought stress and rehydration in leaves and roots of three citrus scion/rootstock combinations. Sci. Hortic-Amsterdam 292. doi: 10.1016/j.scienta.2021.110490
Spiral, J., Ouazzani, S., Vial, N. H., Michaux, S., Barro, L., Darracq, O., et al. (2022). Reciprocal grafting reveals differential metabolic responses between robusta clones with contrasting tolerances to drought. Agric. Res. doi: 10.1007/s40003-022-00622-x
Staples, R. C. (2003). A novel gene for rust resistance. Trends Plant Sci. 8, 149–151. doi: 10.1016/s1360-1385(03)00030-x
Suchoff, D. H., Gunter, C. C., Schultheis, J. R., Kleinhenz, M. D., Louws, F. J. (2018). Rootstock effect on grafted tomato transplant shoot and root responses to drying soils. HortScience 53, 1586–1592. doi: 10.21273/hortsci13215-18
Sucu, S., Yağcı, A., Yıldırım, K. (2017). Changes in morphological, physiological traits and enzyme activity of grafted and ungrafted grapevine rootstocks under drought stress. Erwerbs-Obstbau 60, 127–136. doi: 10.1007/s10341-017-0345-7
Szabados, L., Savoure, A. (2010). Proline: a multifunctional amino acid. Trends Plant Sci. 15, 89–97. doi: 10.1016/j.tplants.2009.11.009
Takahashi, F., Suzuki, T., Osakabe, Y., Betsuyaku, S., Kondo, Y., Dohmae, N., et al. (2018). A small peptide modulates stomatal control via abscisic acid in long-distance signalling. Nature 556, 235–238. doi: 10.1038/s41586-018-0009-2
Tanaka, Y., Nose, T., Jikumaru, Y., Kamiya, Y. (2013). ABA inhibits entry into stomatal-lineage development in arabidopsis leaves. Plant J. 74, 448–457. doi: 10.1111/tpj.12136
Tesfahun, W., Yildiz, F. (2018). A review on: Response of crops to paclobutrazol application. Cogent Food Agric. 4, 1525169. doi: 10.1080/23311932.2018.1525169
Thomas, H. R., Frank, M. H. (2019). Connecting the pieces: uncovering the molecular basis for long-distance communication through plant grafting. New Phytol. 223, 582–589. doi: 10.1111/nph.15772
Thomas, H., Van den Broeck, L., Spurney, R., Sozzani, R., Frank, M. (2021). Gene regulatory networks for compatible versus incompatible grafts identify a role for SlWOX4 during junction formation. Plant Cell 34, 535–556. doi: 10.1093/plcell/koab246
Twalla, J. T., Ding, B., Cao, G., Bao, S., Li, M., Chen, X., et al. (2021). Roles of stomata in gramineous crops growth and biomass production. Cereal Res. Commun. doi: 10.1007/s42976-021-00216-3
Ul Haq, S., Khan, A., Ali, M., Khattak, A. M., Gai, W. X., Zhang, H. X., et al. (2019). Heat shock proteins: Dynamic biomolecules to counter plant biotic and abiotic stresses. Int. J. Mol. Sci. 20, 5321. doi: 10.3390/ijms20215321
Ullah, A., Manghwar, H., Shaban, M., Khan, A. H., Akbar, A., Ali, U., et al. (2018). Phytohormones enhanced drought tolerance in plants: a coping strategy. Environ. Sci. pollut. Res. Int. 25, 33103–33118. doi: 10.1007/s11356-018-3364-5
Valverdi, N. A., Kalcsits, L. (2021). Apple rootstock genotype affects scion responses to water limitations under field conditions. Acta Physiol. Plant 43, 97. doi: 10.1007/s11738-021-03266-6
Vincent, D., Lapierre, C., Pollet, B., Cornic, G., Negroni, L., Zivy, M. (2005). Water deficits affect caffeate O-methyltransferase, lignification, and related enzymes in maize leaves. a proteomic investigation. Plant Physiol. 137, 949–960. doi: 10.1104/pp.104.050815
Wang, G., Cai, G., Kong, F., Deng, Y., Ma, N., Meng, Q. (2014). Overexpression of tomato chloroplast-targeted DnaJ protein enhances tolerance to drought stress and resistance to pseudomonas solanacearum in transgenic tobacco. Plant Physiol. Biochem. 82, 95–104. doi: 10.1016/j.plaphy.2014.05.011
Wang, N., Guo, T., Wang, P., Sun, X., Shao, Y., Jia, X., et al. (2017). MhYTP1 and MhYTP2 from apple confer tolerance to multiple abiotic stresses in arabidopsis thaliana. Front. Plant Sci. 81367. doi: 10.3389/fpls.2017.01367
Wilkinson, S., Davies, W. J. (2002). ABA-based chemical signalling: the co-ordination of responses to stress in plants. Plant Cell Environ. 25, 195–210. doi: 10.1046/j.0016-8025.2001.00824.x
Wilson, R. H., Hayer-Hartl, M. (2018). Complex chaperone dependence of rubisco biogenesis. Biochemistry 57, 3210–3216. doi: 10.1021/acs.biochem.8b00132
Witte, C. P., Keinath, N., Dubiella, U., Demouliere, R., Seal, A., Romeis, T. (2010). Tobacco calcium-dependent protein kinases are differentially phosphorylated in vivo as part of a kinase cascade that regulates stress response. J. Biol. Chem. 285, 9740–9748. doi: 10.1074/jbc.M109.052126
Yang, X., Lu, M., Wang, Y., Wang, Y., Liu, Z., Chen, S. (2021). Response mechanism of plants to drought stress. Horticulturae 7, 50. doi: 10.3390/horticulturae7030050
Yang, L., Perrera, V., Saplaoura, E., Apelt, F., Bahin, M., Kramdi, A., et al. (2019). m(5)C methylation guides systemic transport of messenger RNA over graft junctions in plants. Curr. Biol. 29, 2465–2476.e5. doi: 10.1016/j.cub.2019.06.042
Yang, W., Zhang, W., Wang, X. (2017). Post-translational control of ABA signalling: the roles of protein phosphorylation and ubiquitination. Plant Biotechnol. J. 15, 4–14. doi: 10.1111/pbi.12652
Yavuz, D., Seymen, M., Süheri, S., Yavuz, N., Türkmen, Ö., Kurtar, E. S. (2020). How do rootstocks of citron watermelon (Citrullus lanatus var. citroides) affect the yield and quality of watermelon under deficit irrigation? Agr. Water Manage 241, 106351. doi: 10.1016/j.agwat.2020.106351
Yildirim, K., Yagci, A., Sucu, S., Tunc, S. (2018). Responses of grapevine rootstocks to drought through altered root system architecture and root transcriptomic regulations. Plant Physiol. Biochem. 127, 256–268. doi: 10.1016/j.plaphy.2018.03.034
Yu, S. G., Kim, J. H., Cho, N. H., Oh, T. R., Kim, W. T. (2020). Arabidopsis RING E3 ubiquitin ligase JUL1 participates in ABA-mediated microtubule depolymerization, stomatal closure, and tolerance response to drought stress. Plant J. 103, 824–842. doi: 10.1111/tpj.14775
Zhang, Z., Cao, B., Gao, S., Xu, K. (2019a). Grafting improves tomato drought tolerance through enhancing photosynthetic capacity and reducing ROS accumulation. Protoplasma 256, 1013–1024. doi: 10.1007/s00709-019-01357-3
Zhang, Z., Cao, B., Li, N., Chen, Z., Xu, K. (2019b). Comparative transcriptome analysis of the regulation of ABA signaling genes in different rootstock grafted tomato seedlings under drought stress. Environ. Exp. Bot. 166, 103814. doi: 10.1016/j.envexpbot.2019.103814
Zhang, Z., Liu, Y., Cao, B., Chen, Z., Xu, K. (2020). The effectiveness of grafting to improve drought tolerance in tomato. Plant Growth Regul. 91, 157–167. doi: 10.1007/s10725-020-00596-2
Zhang, L., Marguerit, E., Rossdeutsch, L., Ollat, N., Gambetta, G. A. (2016). The influence of grapevine rootstocks on scion growth and drought resistance. Theor. Exp. Plant Phys. 28, 143–157. doi: 10.1007/s40626-016-0070-x
Zia, R., Nawaz, M. S., Siddique, M. J., Hakim, S., Imran, A. (2021). Plant survival under drought stress: Implications, adaptive responses, and integrated rhizosphere management strategy for stress mitigation. Microbiol. Res. 242, 126626. doi: 10.1016/j.micres.2020.126626
Glossary
Keywords: scion, rootstock, drought tolerance, phytohormones, miRNA
Citation: Yang L, Xia L, Zeng Y, Han Q and Zhang S (2022) Grafting enhances plants drought resistance: Current understanding, mechanisms, and future perspectives. Front. Plant Sci. 13:1015317. doi: 10.3389/fpls.2022.1015317
Received: 09 August 2022; Accepted: 20 September 2022;
Published: 06 October 2022.
Edited by:
Muhammad Waseem, Hainan University, ChinaReviewed by:
Shah Fahad, The University of Haripur, PakistanMd. Rafiqul Islam, Bangabandhu Sheikh Mujibur Rahman Agricultural University, Bangladesh
Copyright © 2022 Yang, Xia, Zeng, Han and Zhang. This is an open-access article distributed under the terms of the Creative Commons Attribution License (CC BY). The use, distribution or reproduction in other forums is permitted, provided the original author(s) and the copyright owner(s) are credited and that the original publication in this journal is cited, in accordance with accepted academic practice. No use, distribution or reproduction is permitted which does not comply with these terms.
*Correspondence: Qingquan Han, aGFuX3FpbmdfcXVhbkAxMjYuY29t; Sheng Zhang, c2hlbmd6aGFuZ0BzY3UuZWR1LmNu