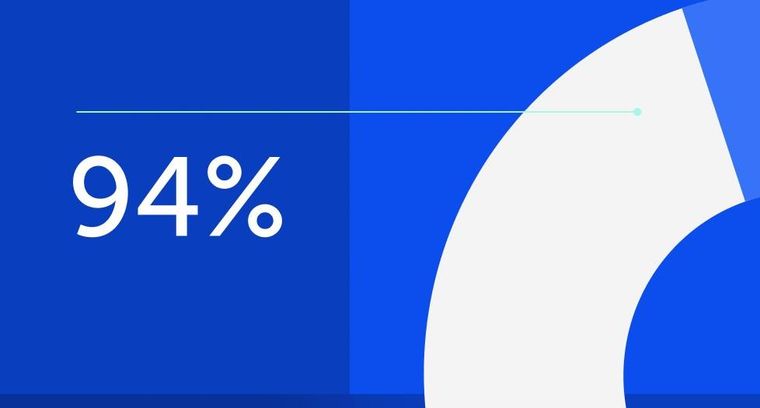
94% of researchers rate our articles as excellent or good
Learn more about the work of our research integrity team to safeguard the quality of each article we publish.
Find out more
ORIGINAL RESEARCH article
Front. Plant Sci., 12 October 2022
Sec. Plant Metabolism and Chemodiversity
Volume 13 - 2022 | https://doi.org/10.3389/fpls.2022.1015095
This article is part of the Research TopicNatural Products from Plants and Applications for Pest ManagementView all 10 articles
The oriental armyworm Mythimna separata (Walker) (Lepidoptera: Noctuidae) can feed on the leaves of many crops, resulting in vast areas of damage and severe losses. Therefore, this insect has become a significant agricultural pest in north Asia. In this study, we fed 3rd instar larvae with artificial diets containing different concentrations of chlorogenic acid and found a significant lethal effect and the mortality increased with increasing chlorogenic acid concentration. Next, we measured the sublethal effect of chlorogenic acid at LC20 on the growth and development of M. separata larvae. The durations of the 4th and 5th instar were longer than those of the control group (prolonged by 0.8 and 0.6 days, respectively), and the 6th instar was shorter (by 1.1 days). The total survival rate, pupation rate, eclosion rate, sex ratio, and oviposition amount in the LC20 chlorogenic acid-treated group were significantly lower than those in the control group. Furthermore, transcriptome analysis of 3rd instar larvae fed various concentrations of chlorogenic acid revealed that several MsCYP450 genes were significantly up-regulated, and this finding was further validated by qRT-PCR. In addition, various concentrations of chlorogenic acid and different treatment times significantly affected the enzyme activity of CYP450 in 3rd instar larvae. Importantly, dietary ingestion of dsMsCYP450 significantly reduced the mRNA level of MsCYP450 genes and increased mortality in the presence of chlorogenic acid. Our results revealed that MsCYP6B6, MsCYP321A7, and MsCYP6B7-like play an essential role in the detoxification of chlorogenic acid by M. separata. This study provides evidence of control effect by botanical insecticide chlorogenic acid on M. separata, and potential detoxification mechanism mediated by P450 of botanical insecticide in arthropods.
Through long-term co-evolution, insects and plants have formed a relatively stable ecological relationship. Plants not only provide nutrients for phytophagous insects, but also initiate a series of physical and chemical defenses to resist insect feeding. Generally, these defense mechanisms can be divided into two categories: constitutive defense and induced defense (Fornoff and Gross, 2014; Chen, 2015; Fyllas et al., 2022; Sobhy et al., 2022). Both defense mechanisms mainly include physical and chemical defenses. Physical defenses specifically include plant morphology, leaf thickness, and fluff, which negatively impact insect feeding behavior (Gary et al., 2006; Whitehill et al., 2016). Chemical defense refers to physiological and biochemical changes, such as induction of plant phytohormone signaling, a decrease in nutritional components, and production of defense proteins and plant secondary metabolites (Ahn et al., 2007; Body et al., 2019; Shen et al., 2021; Divekar et al., 2022). However, insects have also evolved several behaviors to overcome plant defenses, such as changing feeding strategies and regulating growth rhythm and development. In addition to these behavioral changes, biochemical and molecular characteristics also contribute greatly to adaptation to plant defense systems. For example, phytophagous insects regulate the composition, quantity, and quality of digestive enzymes to overcome the protease inhibitors in plants (Cloutier et al., 2000). Other strategies, such as the inhibition of plant defense injury signals and the detoxification to plant secondary substances, also enable insects to escape from the plant defense system.
Insect cytochrome P450 (CYP450) is a terminal oxidase in the multifunctional oxidase system, and it has a catalytic activity on various substrates. Metabolic resistance is an important mechanism underlying insect resistance to traditional insecticides, and CYP450 plays a crucial role in the detoxification of endogenous and exogenous toxic compounds because of its broad-spectrum substrate specificity (Schuler, 2012; Bao et al., 2016; Xu et al., 2020). It has been reported that plant secondary metabolites and insecticides can induce insect CYP450 gene expression; for example, coumarin can induce overexpression of CYP6B2, CYP6B6, and CYP6B7 in Helicoverpa armigera and reduce the sensitivity of the insect to methomyl (Chen et al., 2018). Gossypol can induce high expression of CYP6AB14 and CYP9A98 in Spodoptera exigua, and after RNA interference (RNAi)-mediated silencing of these genes, larvae are more sensitive to deltamethrin (Hafeez et al., 2019).
The oriental armyworm, Mythimna separata (Walker), is a serious polyphagous and migratory insect pest with strong adaptability, and it shows a preference for high-temperature and -humidity environments (Jiang et al., 2011; Kong et al., 2019). Local outbreaks have caused significant damage to crops, such as corn, rice, and sugarcane (Mishra et al., 2021; Yang, 2021). In addition, some economically important crops such as cotton, beans, and vegetables have also suffered damage (Krempl et al., 2021). The long-term continuous cropping of gramineous crops will increase the probability of crop pests and diseases of M. separata (Pang et al., 2021). Chemical treatments are the most common and effective method to control M. separata (Wang et al., 2018). However, frequent use of pesticides often leads to serious environmental problems and insecticide resistance (Song et al., 2017). Therefore, there is an urgent need to develop new methods and materials with low toxicity to beneficial organisms and high specificity for target insects.
Plant secondary metabolites, including phenolics and flavonoids, play essential roles in insect resistance (Chen et al., 2015; Chen et al., 2018; Xu et al., 2019). The results from various studies have demonstrated that plant phenolic metabolites such as chlorogenic acid (CGA), methyl jasmonate, and tannic acid negatively affect insect feeding behavior, growth, development, and reproduction, and they may have lethal effects on specific insects (Kundu and Vadassery, 2019; Li et al., 2019; Lin et al., 2021). CGA (C16H18O9) is a dihydroxy phenolic compound that is a common secondary metabolite in plants, including higher dicotyledons and ferns (Xi et al., 2014). CGA has been shown to be involved in plant chemical defenses against insect herbivores (Kundu and Vadassery, 2019); for example, it can be used as a resistance factor for thrips in chrysanthemum (Leiss et al., 2009), and induced biosynthesis of CGA in sweet potato confers resistance against sweet potato weevil (Liao et al., 2020). In addition, CGA is the main component in the anti-insect defenses of Vernonia anthelmintica Willd (Liu et al., 2020). Besides protecting plants from herbivores, CGA is also involved in plant growth and development processes, such as shoot organogenesis and fruit ripening (A. Neşe Çokuğraş, and Ebru, 2003; Liu, 2016). In our previous research, we found that the attack of M. separata on sugarcane induced significant accumulation of CGA and that CGA has lethal effect on larvae (Wang et al., 2021). Therefore, CGA is a promising environmentally friendly insecticide that is safer for biological use compared with traditional synthetic pesticides.
Although several studies have focused on CGA-mediated plant chemical defenses against insects and the lethal effect of CGA on target insects (Kundu and Vadassery, 2019; Pan et al., 2020), there is no solid evidence of the role of CGA in inhibiting herbivore attack, and the sublethal effects of CGA on insect development and reproduction remain to be determined. In addition, the effect of the CGA regulatory mechanism on an insect is poorly understood. This study aimed to elucidate the lethal and sublethal effects of CGA on M. separata larval growth and development. The effect of CGA on detoxification enzyme activity in M. separata and the potential key detoxifying genes were investigated by RNA sequencing (RNA-seq). The findings of this study provide the basis for further understanding the detoxification mechanism of CGA in arthropods and a new method in the management of pests with P450-mediated resistance.
M. separata larvae were raised in the lab of National Engineering Research Center for Sugarcane, Fujian A&F University, in a controlled temperature (26 ± 1°C) and fixed photoperiod (L16:D8). Preparation of artificial feed and feeding were performed using the feeding method of Lepidoptera insects described by Cao et al. (Cao et al., 2014).
In this study, the concentrations of CGA (purchased from Beijing Solarbio Science & Technology Co., Ltd., purity ≥98%) in the artificial diet were 5mg/mL, 10mg/mL, 20mg/mL, 40mg/mL, and 80mg/mL. During the preparation of the artificial diet, all the main materials were mixed under liquid conditions. To prepare the 80mg/mL CGA artificial diet, 0.4 g of CGA was dissolved in 5 mL of 25% absolute ethanol at room temperature, and 15 g of artificial diet was added. The other artificial diets with different concentrations of CGA were prepared in the same way with the appropriate amounts of CGA, and the artificial diet supplemented with 5 mL of 25% absolute ethanol was used as the control. A piece of the artificial diet was placed in each well of a 24-well plate, and one pre-starvation (12 h) 2nd instar larva was placed on the surface of the diet. Three replicates of 24 larvae were tested for every concentration. The same treatment was also for 3rd and 4th instar larvae. Feeding conditions were the same as those in section 2.1. Each day the artificial diet was checked for freshness, and stale food was replaced, and the death of larvae was recorded. The experiment was terminated after five days of treatment, and the statistical data were collected. Larvae were considered dead when they did not respond when stimulated with an ink brush. The LC50 for each treatment was determined by Probit analysis in SPSS 18.0.
The 3rd instar larvae were collected to determine the sublethal effects of CGA on larval growth and development parameters as described by Wang et al. (Wang et al., 2014). LC20 was chosen as the concentration for CGA treatment because it resulted in a specific amount of mortality. Healthy 3rd instar larvae were starved for 12 h, then a single 3rd instar larva was placed on the surface of the artificial diet containing the LC20 dose of CGA in an individual 25 ml plastic cup and sealed with a lid. Each treatment was performed with 30 larvae, and the treatment was replicated three times. Similarly, twenty 3rd instar larvae were placed on the artificial diet without CGA as a control group, and the treatment was repeated three times. Larvae were examined every day till pupation, and the developmental stage, including molting, pupation, and death, of the larvae was recorded every day. Then we determined the sex of each M. separata by pupa and calculated the sex ratio (Chen et al., 2019). After eclosion, the male and female adults were paired and transferred to a cage for mating. After eggs were laid, the egg masses were counted. The date was analysis by t-test in SPSS 18.0.
The artificial diets with CGA at LC20, LC50, and LC80 were fed to healthy 3rd instar larvae for 5 days, then four to six surviving larvae were randomly selected for further transcriptome analysis with three biological replicates. RNA isolation, cDNA synthesis, library construction, and Illumina sequencing were all performed at Berry Genomics Co., Ltd. (Beijing, China) (Hansen et al., 2010). The RNeasy Micro Kit (Qiagen, Hilden, Germany) was used to isolate total RNA from each sample. RNA purity and concentration were then examined using the NanoDrop 2000, and RNA integrity and quantity were measured using the Agilent 2100 system. Next, an NEB library was established for each sample using mRNA as a template. All libraries were pooled together and subjected to Illumina sequencing with paired-end sequencing. Trinity was used to assemble clean reads, Benchmarking Universal Single-Copy Orthologs (BUSCO) was used to evaluate the integrity of transcript assembly, and Corset program transcripts were used for hierarchical clustering. All unigenes were obtained after assembly, and unigene functional annotation was based on the non-redundant protein sequence (Nr), nucleotide sequence (Nt), protein families (PFAM), KOG and Swiss-Prot databases. Open reading frames (ORFs) were predicted by TransDecoder software with the default setting. Then, paired-end reads were aligned to the unigene sequence using bowtie, and RSEM was used to count the number of reads mapped to each gene and estimate gene expression levels. Differential expression was analyzed using EdgeR. P-values of the results were adjusted to control for the false discovery rate. Genes with |log2 (Fold Change)| > 1 and q value < 0.05 were designated as differentially expressed. Finally, Gene ontology (GO) and Kyoto Encyclopedia of Genes and Genomes (KEGG) enrichment analyses of differentially expressed gene (DEG) sets were performed using GOseq R and KOBAS 3.0, respectively. GO terms with an adjusted p-value below 0.05 were considered significantly enriched in DEGs.
The assembled unigenes were used as queries in searches against the Nr database with a cut-off E-value < 1.0 E−5. The unigenes found in the same BLAST search or that shared high homology with other unigenes were regarded as allelic variants or as different parts of the same gene. The gene with hit result of CYP450 was screened from the Nr results after BLAST. All CYP450 genes of M. separata were identified by sequence alignment, and the amino acid sequences were aligned using the default settings in ClustalW 2.0. Then, the CYP450s genes of M. separata were compared with those of H. armigera and Spodoptera litura (obtained from InsectBase 2.0) by performing phylogenetic analysis in MEGA-X.
Healthy 3rd instar larvae were starved for 12 h, then transferred to an artificial diet with different concentrations (LC20, LC50, and LC80) of CGA. Feeding conditions were the same as those in section 2.1. Healthy 3rd instar larvae were fed an artificial diet with 25% absolute ethanol as a control. After feeding for 1, 3, 5, and 7 days, ten surviving larvae were randomly selected with three replicates per group to analyze the effects of different concentrations of CGA in the artificial diet and different treatment times on P450 enzyme activities using the CYP450 enzyme assay kit (CK-E93532, Shanghai Enzymatic Biotechnology Co., Ltd.) according to the kit instructions.
RNA-seq analysis and assays of detoxification-related CYP450 protein activities in M. separata treated for different times with different concentrations of CGA revealed that MsCYP450 genes and CYP450 detoxification proteins were significantly up-regulated. Therefore, we selected seven MsCYP450 genes that were up-regulated considerably in response to different concentrations of CGA for validation by qRT-PCR using the same RNA that was used in RNA-seq. Primer 5 was used to design specific primer pairs (Table S1), and primers were synthesized by Tsingke Biotechnology Co., Ltd. in China. The cDNA synthesis reaction was performed using the HiScript® II Q RT SuperMix kit with gDNA wiper (Vazyme, China) according to the manufacturer’s protocol using 1 μg of total RNA as a template per reaction. QRT-PCR was performed with Hieff® qPCR Green Master Mix (Yeasen, China). Finally, data were analyzed using the 2-ΔΔCT method (Sun et al., 2017), and EF-1α was used as a control to correct for sample-to-sample variation. Three technical replicates were performed for each replicate, and the data were expressed as mean ± standard error (SE).
Fragments of MsCYP321A7, MsCYP6k1-like, MsCYP6B6, MsCYP324A1, MsCYP4V2-like, MsCYP6B7-like, MsCYP6AE88, and green fluorescent protein gene (GFP) were amplified by PCR using specific primers (Table S2) conjugated with the T7 RNA polymerase promoter (TAATACGACTCACTATAGGG). The T7 Ribomax ™ Express RNAi System (Promega, Madison, WI, USA) was used to synthesize double-stranded RNAs (dsRNAs) as described in the manual. Thirty M. separata larvae at the late 2nd instar stage were fed an artificial diet containing 100 μg dsRNA in a 24-well plate for 3 days, and fresh dsRNA was added every day. The control group was treated with the same amount of ddH2O. After 3 days, five living larvae in each group (control, MsCYP321A7-dsRNA, MsCYP6k1-like-dsRNA, MsCYP6B6-dsRNA, MsCYP324A1-dsRNA, MsCYP4V2-like-dsRNA, MsCYP6B7-like-dsRNA, MsCYP6AE88-dsRNA, and GFP-dsRNA) were collected for total RNA extraction for determination of gene expression. The approximately 25 larvae remaining in each treatment were used for bioassays with CGA at LC50 as described above (section 2.3). The number of dead larvae was recorded after CGA application for 6 days. The experiment was replicated three times. After dsRNA treatment with the same method, the growth and development period were determined by 2.3 method that using LC20 CGA mixed artificial diet.
The toxicity of CGA against M. separata larvae was determined using the feeding method. The mortality was calculated after feeding the 2st, 3rd and 4th instar larvae with an artificial diet containing various concentrations of CGA for 5 days. The results (Table S3) showed that the mortalities of 3rd larvae fed diets with different concentrations of CGA were significantly different after 5 days (P<0.05). The higher the concentration of CGA, the higher the mortality. The mortality rate of the 5mg/mL CGA treatment group was 13.33% ± 6.67%, while the mortality rate of the 80mg/mL CGA treatment group reached 80.00% ± 13.33%. Based on the bioassay results, 74.48 mg/mL CGA (LC80 dose), 26.29 mg/mL CGA (LC50 dose) and 7.15 mg/mL CGA (LC20 dose) for 3rd larvae were used for further treatment of larvae.
The LC20 concentration 7.15 mg/mL was used to assess the sublethal effects of CGA on M. separata development and reproduction. The duration of development for each instar is shown in Table 1. The results showed that there was no significant difference in the duration of the 3rd instar stage of larvae treated with LC20, but there were significant differences in the durations of the 4th and 5th instars, which were prolonged by 36.87% (0.8 days) and 38.22% (0.6 days), respectively (P < 0.0001). However, the duration of the 6th instar larval stage was significantly shortened by 18.15% (1.1 days), and the overall developmental duration of the 3rd to 6th instars was extended by about 0.5 days. Measurement of other growth and development indices of larvae showed that the total survival rate of larvae (90%), eclosion rate (34.09%), sex ratio (0.86), and the number of eggs laid per female (427.8 ± 48.88) of the CGA treatment group were significantly lower than those of the control group. It can be seen that CGA harms the growth, development, and reproduction of larvae.
To assess the potential mechanism underlying the lethal effects of CGA on M. separata and potential detoxification pathways, RNA-seq was carried out to identify genes encoding target proteins and potential insecticide detoxification enzymes.
Twelve M. separata libraries were sequenced on the Illumina platform and pooled together for assembly. All reads were cleaned, and Trinity was used to conduct quality checks. A total of 310,336,443 reads were assembled into 257,014 transcripts with an N50 length of 1,892. The contigs were assembled into 134,240 unigenes with an average length of 1,176 bp (Table 2) using paired-end joining and gap-filling methods. The length distribution was mainly between 300 and 500 bp (35.66% of sequences); there were no sequences < 300 bp, and 14.91% of sequences were longer than 2 kb (Figure S1).
To annotate unigenes, a BLASTX search of the Nr protein database of the National Center for Biotechnology Information (NCBI), was performed with a cut-off E-value of 10−5. A BLAST hit was obtained for 27,039 distinct sequences (20.1% of the total). Sequences were also used as queries in searches against several other databases, including the Nt, Swiss-Prot, PFAM protein, GO, and KOG databases (Table 2). Based on the best hit in the Nr database, 7300 (27.0%) annotated unigenes had the highest homology to sequences in H. armigera. In comparison, fewer matched sequences in S. litura (19.7%) and Heliothis virescens (16.5%). The fewest sequences matched hits in the more distantly related species Trichoplusia ni (6.3%) and Chilo suppressalis (5.5%) (Figure S2).
Expression levels of genes in M. separata treated with artificial diets containing one of three different concentrations of CGA (LC20, LC50, and LC80) were compared with those of genes in the control group (CK). The comparison LC20vsCK had the most significant DEGs, 1764 (1015 up-regulated and 749 down-regulated). LC80vsCK had the least number of DEGs, of which 967 were up-regulated and 319 were down-regulated. There were 671 up-regulated DEGs and 696 down-regulated DEGs identified in the LC50vsCK comparison (Figure 1A). Analysis of the intersection of DEGs revealed that 229 genes were up-regulated and 173 genes were down-regulated in response to all three CGA treatments (Figures 1B, C).
Figure 1 The number of DEGs in M. separata larvae treated with different concentrations of CGA. (A) The number of DEGs in different treatment. The number in the column indicates the number of DEGs. (B) The number of DEGs up-regulated by all treatments. (C) The number of DEGs down-regulated by all treatments.).
GO and KEGG enrichment analyses were performed for all DEGs to understand the possible mechanisms underlying gene expression differences between control and CGA-treated M. separata larvae. DEGs from the LC20vsCK, LC50vsCK, and LC80vsCK comparisons were all mainly enriched in the GO biological process (BP) term transmembrane transport, the cellular component (CC) term extracellular region, and the molecular function (MF) term oxidoreductase activity (Figure S3). In KEGG enrichment analysis, DEGs from LC20vsCK, LC50vsCK, and LC80vsCK were all enriched primarily in the metabolism of xenobiotics by cytochrome P450, drug metabolism - cytochrome P450, and chemical carcinogenesis (Figure 2).
Figure 2 KEGG enrichment analyses of DEGs from different comparisons. (A) LC20vsCK comparison. (B) LC50vsCK comparison. (C) LC80vsCK comparison.
P450 enzyme activity assays showed that P450 activity was induced by different concentrations of CGA (Figure 3A). P450 activity significantly increased with increasing CGA concentration and reached the maximum at LC50. In addition, we also assessed the effect of duration of LC20 CGA treatment on M. separata P450 enzyme activity (Figure 3B). The P450 enzyme activity increased significantly post-treatment and reached the maximum at 7 days.
Figure 3 The activity of CYP450 proteins in the 3rd instar larvae of M. separata fed artificial diets containing CGA. (A) Artificial diet containing different concentrations of CGA; (B) Artificial diet containing CGA at LC20 with different treatment times. Different letters above the bars show significant differences between groups according to Tukey’s multiple comparisons tests at P < 0.05. Error bars represent the standard deviation (SD) of the means.).
From our transcriptome data, 139 sequences encoding CYP450s were identified, and these sequences corresponded to 61 non-redundant unigenes. Of these, 44 CYP450 genes encoding proteins with more than 200 amino acids identical to annotated CYP450 proteins were used for further analysis (Table S4). The lengths of these CYP450 genes ranged from 662 to 4858 bp. Then, from phylogenetic tree analysis, the CYP450 genes were categorized into four CYP450 (CYP) clans: CYP2, CYP3, CYP4, and the mitochondrial clan (Figure 4). Twenty-eight genes were assigned to the CYP3 clan, which was the most prominent clan; the CYP4 clan was the second largest with 8 genes; 2 genes were assigned to the CYP2 clan; and 6 genes were assigned to the mitochondrial clan, which is only found in animals (Figure 4).
Figure 4 Phylogenetic analysis of the CYP450 genes from M. separata, H. armigera, and S. litura. The tree was constructed from multiple sequence alignments using MEGA-X software.
To verify the accuracy of the expression profiles obtained by RNA-seq, we used the same RNA sample as template in qRT-PCR analysis to determine the expression levels of seven MsCYP450 DEGs. As shown in Figure 5, the RNA-seq expression patterns of the DEGs were similar to those determined by qRT-PCR. After treatment with CGA at LC20, LC50 and LC80, gene expression was up-regulated. Of the MsCYP450 genes, MsCYP321A7 was the most significantly up-regulated. MsCYP6k1-like, MsCYP324A1, and MsCYP6AE88 were all expressed at high levels after treatment with different concentrations of CGA. MsCYP4V2-like was the most highly expressed after treatment with CGA at LC20, and its expression then decreased with increasing CGA concentration. We also found that the seven MsCYP450 genes had high expression levels after being treated with CGA at LC50. This is consistent with the observation of the highest activity of CYP450 detoxification enzymes in M. separata treated with CGA at LC50.
Figure 5 Comparison of relative fold change determined from RNA-seq and qRT-PCR analysis of the same samples. (From A–G the genes are MsCYP321A7, MsCYP6k1-like, MsCYP6B6, MsCYP324A1, MsCYP4V2-like, MsCYP6B7-like, and MsCYP6AE88, respectively).
After continuous ingestion of dsGFP and dsMsCYP450 genes for three days, the late 2nd instar molted to the 3rd instar. Few larvae died after being fed dsRNA, but the level of MsCYP450 gene expression decreased significantly (43.89%–69.39%) compared with the control group (Figure 6). The lowest expression level was found in larvae fed dsMsCYP6B6, with a 56.11% reduction in expression. Next, approximately 25 surviving larvae exposed to CK (ddH2O), dsGFP, or dsMsCYP450 genes were used for further bioassay experiments. After 2 days, larval mortality among the dsMsCYP6B6, dsMsCYP321A7, and dsMsCYP6B7-like treatment groups (60.71%, 57.94%, and 47.22%, respectively) was much higher than that in the CK and dsGFP treatment groups (36.45% and 36.90%, respectively, Figure 7). The larval mortality in the dsMsCYP6k1-like, dsMsCYP324A1, dsMsCYP4V2-like, and dsMsCYP6AE88 groups was not significantly different compared with that in the CK and dsGFP treatment groups. After 5 days, the largest larval mortality was dsMsCYP6B6 treatment groups reached 78.97% (Figure S4).
Figure 6 The relative gene expression levels of MsCYP450 genes after dsMsCYP450 gene treatment for 3 days. (From A–G, the genes are MsCYP321A7, MsCYP6k1-like, MsCYP6B6, MsCYP324A1, MsCYP4V2-like, MsCYP6B7-like, and MsCYP6AE88, respectively. Different lowercase letters above the bars indicate a significant difference (p < 0.05) based on one-way ANOVA followed by Tukey’s HSD test for multiple comparisons. Means ± SE from three replicates are shown).
Figure 7 The larval mortality and survival rates for M. separata larvae after dsRNA treatment. (A) Larvae were continuously fed dsRNA for 3 days, and mortality was evaluated for 2 days; (B) The survival rates of larvae scored for 2 days after dsMsCYP450 gene treatment for 3 days. Different lowercase letters (a, b and c) above the bars indicate significant differences (p < 0.05) based on one-way ANOVA followed by Tukey’s HSD test for multiple comparisons. Means ± SE from three replicates are shown).
In addition, we also tested larvae growth and development parameter of M. separata larvae after different dsRNA treatment showed that (Table S5). Compared with the control group, the 3rd instar duration of the treatment groups was not significantly different, while the 4th and 5th instars began to be significantly prolonged. The longest of 4th instar duration was dsMsCYP321A-7 treatment (3.19 days) and the 5th instar duration was dsMsCYP6B7-like treatment (2.53 days). Compared with the control group, the 6th instar duration of the treatment groups were all shorten and the shortest was dsMsCYP6B6 treatment (5.32 days).
In this study, the effects of different concentrations of CGA on M. separata larvae were investigated by adding different concentrations of CGA to an artificial diet. The larvae in the CGA treatment group started to die after 3 days, and peak death was observed between days 3 and 5. This indicates that CGA has no acute insecticidal effect on M. separata larvae within the experimental concentration range of this study, but with prolonged feeding time, CGA may accumulate in larvae and have an insecticidal effect. Similar results have been obtained when treating 3rd instar larvae of Plutella americana with 0.500% CGA. The mortality of 5th instar larvae of P. americana in the treatment group increased with increasing CGA concentration in the artificial diet and was significantly higher than that in the control group (Pan et al., 2020). Similar results were also obtained when 2nd instar larvae of Plutella viridis were treated with 0.3% CGA. The mortality rate increased rapidly from 20% to 50% after 20 days of treatment and reached 100% after 34 days of treatment (Wang et al., 2014).
Generally speaking, botanical insecticides are thought to be eco-friendly and relatively safe. These insecticides have the following main properties: pest selectivity, low risk to non-target organisms, biodegradability, and low risk of inducing insect resistance (Benelli et al., 2019; Zainab and Manfred, 2020). In recent years, many studies have concentrated on the use of plant extracts, particularly biologically active compounds of plant-derived and essential oils, as potential alternatives to commercial insecticides (Isman and Grieneisen, 2014). Therefore, it is necessary to pay attention to the excavation and use of botanical pesticides. Other phenolic substances have shown insectidal activity; for example, 50 μg/mL kaempferol treatment for 72 h, caused 82% mortality of 4th instar larvae of Culex quinquefasciatus (Huang et al., 2014); 10 mg/mL periplocosides treatment for 24 h resulted in 76.2% mortality of Schizaphis graminum (Rondani) and 37.5% mortality of M. separata (Li et al., 2019). The essential oils of Schinus areira and Thymus hyemalis (in the family Lamiaceae) had insecticidal activity against Rhipibruchus picturatus (F.) (Coleoptera: Bruchinae) and Eceratoniae ceratoniae Zeller (Lepidoptera: Pyralidae), respectively (Mattar et al., 2022; Adouane et al., 2022).
Studies to date have shown that CGA has adverse effects on the growth and development of insects and can even be oxidized to more toxic quinones in insects, which have a direct poisoning effect. (Hu et al., 2009; Kundu and Vadassery, 2019) In this study, feeding the 3rd instar larvae with a LC20 CGA diet prolonged the duration of M. separata larval development (instars 4–5), probably due to a fitness penalty from resisting CGA. Part of the energy intake is used for growth and development, while the other part is used for detoxification metabolism of CGA. But the developmental duration of the mature larvae (6th instar) was shortened, which may be related to the tendency of Lepidoptera to survive poor environments as pupae and the premature pupation of the mature larvae caused by CGA treatment (Deng, 2018). This result was similar to that of previous studies in Lepidoptera, which showed that the duration of Helicoverpa zea development was significantly prolonged after ingesting artificial diets containing CGA and caffeic acid (Summers and Felton, 1994), and that CGA reduces the growth, development, and fecundity of Hyphantria cunea larvae (Pan et al., 2020). All the results mentioned above show that CGA can prolong the growth period of insects, thereby reducing rate of insect reproduction and the occurrence of disease. In addition, CGA reduced the pupation rate, eclosion rate, sex ratio, and the number of eggs laid per female, indicating that dietary CGA can negatively affect larval development and insect reproduction. These results were consistent with the conclusions reached for other lepidopteran insects. For example, egg production by the gypsy moth is inversely proportional to the phenolic acid content of its food (Tod et al., 2000). Lymantria dispar larvae could not complete normal growth and development after feeding on an artificial diet containing tannic acid or CGA. The body weights of the larvae were about 67.2%–75.0% lower than that of the control, and the duration of the larval stage was prolonged by 2–4 times. (Wang et al., 2014). This provides new evidence supporting the hypothesis proposed by Caroline and Simon (Caroline and Simon, 2002) that insects can adapt to different foods by adjusting their reproductive capacity during long-term evolution. After being treated with sulfoxaflor at sublethal concentrations, fatty acids, amino acids, and the composition and content of carbohydrates all changed to different degrees, indicating these energy substances mentioned above participate in detoxification metabolism of M. persicae to some extent (Zhang et al., 2021). We speculate that the effect of CGA on the growth and development of M. separata might be related to some energy substances involved in detoxification metabolism, which needs further research.
Plant secondary metabolites can induce changes in detoxification-related protein activities in phytophagous insects, which may improve the adaptability of these insects (Chen et al., 2015; Wang et al., 2022). CYP450 enzymes are the primary detoxifying enzymes in many organisms. Multiple signaling pathways and critical effector molecules are involved in regulating insect P450s. CYP450 genes play a significant role in detoxification in insects, and insecticide resistance largely depends on the metabolism of exogenous toxic substances by CYP450s (Nauen et al., 2021; Lu et al., 2020). Increased P450 activity is a key mechanism inducing insect resistance (Ye et al., 2022). Wang et al. demonstrated that AmCYP9q1 plays an important role in the metabolic detoxification of imidacloprid by Apis mellifera larvae (Wang et al., 2022). The expression levels of CYP4L13 and CYP4M14 genes in the midgut and fat bodies of Spodoptera frugiperda increased significantly after larvae were fed exogenous insecticides such as nicotine and flavonoids (Wang et al., 2022). Here, we performed assays of P450 enzyme activity in M. separata larvae treated with CGA to investigate the role of MsCYP450 genes. We found that CGA could induce P450 enzyme activity in M. separata larvae fed with different concentrations of CGA for different amounts of time (Figure 3). Studies have shown that P450 can add various chemical groups, including hydroxyl, carboxyl, and amino groups, to toxic secondary metabolites in the insect digestive tract. They can improve the water solubility and reactivity of toxic secondary metabolites and degrade them into less harmful forms (Chung et al., 2007; Cui et al., 2016). In this study, we found evidence that CYP450 enzymes are essential for the detoxification metabolism of CGA in M. separata.
In transcriptome analysis, we found 139 McCYP450 genes, which is a much higher number than those in the lepidopteran insects H. armigera (112) and S. litura (67) (Zhang, 2018 and Zhang, 2019). Fewer MsCYP450 genes were identified in this study than in our previous transcriptome sequencing study (Wang et al., 2018), possibly because of insufficient sequencing depth or redundant sequencing. According to phylogenetic tree analysis, the 139 MsCYP450 genes were divided into four clans, of which the CYP3 clan was most closely related to drug resistance metabolism (Wan et al., 2013). By comparing the transcriptomes of M. separata treated with different concentrations of CGA with that of the control group, 179 commonly up-regulated genes were identified. Among these up-regulated genes, seven were MsCYP450 genes and five were from the CYP3 clan. Therefore, we speculated that the seven MsCYP450 genes might be involved in metabolism. To further confirm the function of these genes, we used the RNAi to knock down their expression. Insect RNAi has been widely used to identify or validate insecticide target genes (Young et al., 2015; Dulbecco et al., 2021) and the use of this technology in M. separeata has been reported (Wang et al., 2019). Also this method is widely used in identification of insecticide target. In our study, M. separata larvae were continuously fed dsRNA for 3 days, and the mRNA level of MsCYP450 genes were significantly lower after treatment. The surviving larvae were then exposed to the CGA at LC50 for 6 days, and treatment with dsMsCYP6B6, dsMsCYP321A7, and dsMsCYP6B7-like caused a significant reduction in survival compared with the CK and the dsGFP treatment groups. These three MsCYP450 genes all belonged to the CYP3 clan, which indicates they might play an important role in the detoxification of CGA. The same results have also been observed for similar genes in other insects. Bagchi et al. also demonstrated that CGA significantly induces the CYP450 genes of Amyelois transitella and increases the tolerance to CGA (Bagchi et al., 2016), and silencing of the cytochrome P450 gene CYP321A1 was found to affect tannin detoxification in S. litura (Zhao et al., 2021). CYP6B6 was shown to be involved in esfenvalerate detoxification in the polyphagous insect H. armigera (Tian et al., 2017), and CYP6B7 was shown to play an important role in the resistance of H. armigera to fenvalerate (Tang et al., 2007). In this study, we confirmed that MsCYP6B6, MsCYP321A7, and MsCYP6B7-like play an essential role in the detoxification of CGA in M. separata.
In this study, we found that CGA had a lethal effect on M. separata and that a sublethal concentration harmed larval growth and development. Seven MsCYP450 genes that may be involved in the detoxification process were identified by performing P450 enzyme assays and transcriptome analysis. By treating larvae with dsMsCYP450 genes, we determined that MsCYP6B6, MsCYP321A7, and MsCYP6B7-like play a vital role in the detoxification of CGA by M. separata. The findings of this functional study of the CGA detoxification genes of this major phytophagous insect provides new insight into this biological process and new targets for agricultural pest control. This study also provides a new method for managing P450-mediated resistance in insect pests.
The data presented in the study are deposited in the Genebank repository, accession number MsCYP321A7 (OP254196),MsCYP6k1-like(OP254197), MsCYP6B6(OP254198),MsCYP324A1 (OP254199),MsCYP4V2-like(OP254200),MsCYP6B7-like (OP254201),MsCYP6AE88 (OP254202).
D-jL, L-yL, and L-zZ conducted the experiment, D-jL manuscript writing, YF and S-jG data analysis, RW and J-dWdesigned the experiment and funding support. All authors haveread and agreed to the published version of the manuscript.
This work was supported by the Scientific and Technological Innovation Capacity Construction Special Funds of the Beijing Academy of Agriculture and Forestry Sciences, China (KJCX20210437), the key research and development program of Hunan Province (China) (2020NK2034), Talent Programs of Fujian Agriculture and Forestry University (xjq202119), the Sugar Crop Research System, CARS (CARS-17) and Special Technology Innovation Funding of Fujian Agriculture and Forestry University (CXZX2020084A).
The authors declare that the research was conducted in the absence of any commercial or financial relationships that could be construed as a potential conflict of interest.
The reviewer ZG declared a past collaboration RW with the author to the handling editor.
All claims expressed in this article are solely those of the authors and do not necessarily represent those of their affiliated organizations, or those of the publisher, the editors and the reviewers. Any product that may be evaluated in this article, or claim that may be made by its manufacturer, is not guaranteed or endorsed by the publisher.
The Supplementary Material for this article can be found online at: https://www.frontiersin.org/articles/10.3389/fpls.2022.1015095/full#supplementary-material
Adouane, S., Mehaoua, M. S., Bouatrous, Y., Tudela, J., Flamini, G., Mechaala, S. (2022) Natural insecticides from native plants of the Mediterranean basin and their activity for the control of the date moth ectomyelois ceratoniae (Zeller) (Lepidoptera: Pyralidae). J. Plant Dis. Prot. 129 (4). doi: 10.1007/S41348-022-00593-9
Ahn, J., Guarino, L. A., Zhu-Salzman, K. (2007). Seven-up facilitates insect counter-defense by suppressing cathepsin b expression. FEBS J. 274, 2800–2814. doi: 10.1111/j.1472-4658.2007. 05816.x.
Bagchi, V. A., Siegel, J. P., Demkovich, M. R., Zehr, L. N., Berenbaum, M. R. (2016). Impact of pesticide resistance on toxicity and tolerance of hostplant phytochemicals in amyelois transitella (lepidoptera: pyralidae). J. Insect Sci. (Online) 16 (1), 063. doi: 10.1093/jisesa/iew063
Bao, H., Gao, H., Zhang, Y., Fan, D. Z., Fang, J. C., Liu, Z. W. (2016). The roles of CYP6AYI and CYP6ERI in imidacloprid resistance in the brown planthopper: Expression levels and detoxification efficiency. Pesticide Biochem. Physiol. 129, 70–74. doi: 10.1016/j.pestbp.2015.10.020
Benelli, G., Pavela, R., Zorzettoc, C., Sánchez-M, C. C., Santini, G., Canale, A., et al. (2019). Insecticidal activity of the essential oil from schizogyne sericea (Asteraceae) on four insect pests and two non-target species. Entomol. Gen. 39 (1), 9–18. doi: 10.1127/entomologia/2019/0662
Body, M. J. A., Zinkgraf, M. S., Whitham, T. G., Lin, C., Richardson, R. A., Ryan, A., et al. (2019). Heritable phytohormone profiles of poplar genotypes vary in resistance to a galling aphid. Mol. Plant-Microbe Interactions: Mpmi 32 (6), 654–672. doi: 10.1094/MPMI-11-18-0301-R
Cao, L. J., Yang, F., Tang, S. Y., Chen, M. (2014) Development of an artificial diet forthree lepidopteran insects. Chinese Journal of Applied Entomology 51 (05),1376–1386. doi: 10.7679/j.issn.2095?1353.2014.165
Caroline, S. A., Simon, R. L. (2002). Host plant quality and fecundity in herbivorous insects. Annu. Rev. Entomology 47, 817–844. doi: 10.1146/annurev.ento.47.091201.145300
Chen, Z. (2015). Effects of plant constitutive defense on phytophagous insects. Beijing Agric. 9, 137. doi: 10.16380/j.kcxb.2015.10.011
Chen, S., Elzaki, M. E. A., Ding, C., Li, Z. F., Wang, J., Zeng, R. S., et al. (2018). Plant allelochemicals affect tolerance of polyphagous lepidopteran pest helicoverpa armigera (Hibner) against insecticides. Pesticide Biochem. Physiol. 154, 32–38. doi: 10.1016/j.pestbp.2018.12.009
Chen, Q., Hou, Y. H., Duan, Y., Fan, Z. Y., Shen, H. L., Chen, L., et al. (2019). Rapid sex identification of mythimna separata pupae. Plant Protection. 45, 157–159. doi: 10.16688/j.zwbh.2018127
Chen, C. Y., Kang, Z. J., Shi, X. Y., Gao, X. W. (2015). Metabolic adaptation mechanisms of insects to plant secondary metabolites and their implications for insecticide resistance of insects. Acta Entomologica Sinica. 58, 1126–1139. doi: 10.16380/j.kcxb.2015.10.011
Chung, H., Bogwitz, M. R., McCart, C., Andrianopoulos, A., Ffrench-Constant, R. H., Batterham, P., et al. (2007). Cis-regulatory elements in the accord retrotransposon result in tissue-specific expression of the drosophila melanogaster insecticide resistance gene cyp6g1. Genetics 175, 1071–1077. doi: 10.1534/genetics.106.066597
Cloutier, C., Jean, C., Fournier, M., Yelle, S., Michaud, D. (2000). Adult colorado potato beetles, leptinotarsa decemlineata compensate for nutritional stress on oryzacystatin i-transgenic potato plants by hypertrophic behavior and over-production of insensitive proteases. Arch. Insect Biochem. Physiol. 44 (2), 69–81. doi: 10.1002/1520-6327(200006)44:2<69::AID-ARCH2>3.0.CO;2-6
Cui, S. F., Wang, L., Ma, L., Geng, X. Q. (2016). P450-mediated detoxification of botanicals in insects. Phytoparasitica 44, 585–599. doi: 10.1007/s12600-016-0550-1
Deng, Y. (2018). Transcriptome sequencing for ldentification of diapause-associatedGenes and genetic differentiation of hyphantria cunea (Drury) (Liaoning, China: Shenyang Agricultural University).
Divekar, P. A., Narayana, S., Divekar, B. A., Kumar, R., Gadratagi, B. G., Ray, A., et al. (2022). Plant secondary metabolites as defense tools against herbivores for sustainable crop protection. Int. J. Mol. Sci. 23 (5), 2690. doi: 10.3390/ijms23052690
Dulbecco, A. B., Moriconi, D. E., Pedrini, N. (2021). Knockdown of cyp4pr1, a cytochrome p450 gene highly expressed in the integument tissue of triatoma infestans, increases susceptibility to deltamethrin in pyrethroid-resistant insects. Pesticide Biochem. Physiol. 173, 104781. doi: 10.1016/j.pestbp.2021.104781
Fornoff, F., Gross, E. M. (2014). Induced defense mechanisms in an aquatic angiosperm to insect herbivory. Oecologia 175, 173–185. doi: 10.1007/s00442-013-2880-8
Fyllas, N. M., Chrysafi, D., Avtzis, D. N., Moreira, X. (2022). Photosynthetic and defensive responses of two mediterranean oaks to insect leaf herbivory. Tree Physiol. doi: 10.1093/treephys/tpac067
Gary, C. C., Jeffrey, N., Sanford, D. E. (2006). Leaf surface wax and plant morphology of peas influence insect density. Entomologia Experimentalis Et Applicata 119 (3), 197–205. doi: 10.1111/j.1570-7458.2006.00410.x
Hafeez, M., Liu, S., Yousaf, K., Jan, S., Wang, R. L., Fernández-Grandon, G. M., et al. (2019). RNA Interference -mediated knockdown of a cytochrome P450 gene enhanced the toxicity of a-cypermethrin in xanthotoxin-fed larvae of spodoptera exigua (Hibner). Pesticide Biochem. Physiol. 162, 6–14. doi: 10.1016/j.pestbp.2019.07.003
Hansen, K. D., Brenner, S. E., Dudoit, S. (2010). Biases in illumina transcriptome sequencing caused by random hexamer priming. Nucleic Acids Res. 38 (12), e131. doi: 10.1093/nar/gkq224
Huang, J. G., Yang, W. J., Sang, X. Q., Zhao, H. H. (2014). Insecticidal activities of nine compounds extracted from cacalia tangutica. J. South China Agric. University. 35, 64–68. doi: 10.7671/j
Hu, Z. H., Yang, D., Shen, Y. B. (2009). Difference of phenolic contents in leaves of populus simoniixP. pyramidalis ‘Opera 8277’ cuttings induced by various damages. Acta Botanica Boreali-Occidentalia Sin. 29, 332–337. doi: 1000-4025(2009)02-0332-06
Isman, M. B., Grieneisen, M. L. (2014). Botanical insecticide research: many publications, limited useful data. Trends Plant Sci. 19 (3), 140–145. doi: 10.1016/j.tplants.2013.11.005
Jiang, X. F., Luo, L. Z., Zhang, L., Thomas, W. S., Hu, y. (2011). Regulation of migration in mythimna separata (walker) in china: a review integrating environmental, physiological, hormonal, genetic, and molecular factors. Environ. Entomology 4 0 (3), 516–533. doi: 10.1603/EN10199
Kong, H. L., Dong, C. L., Jing, W. H., Zheng, M. Y., Tian, Z., Hou, Q. L., et al. (2019). Transcriptomic insight into antimicrobial peptide factors involved in the prophylactic immunity of crowded mythimna separata larvae. Dev. Comp. Immunol. 98, 34–41. doi: 10.1016/j.dci.2019.02.009
Krempl, C., Joußen, N., Reichelt, M., Kai, M., Vogel, H., David, G. (2021). Consumption of gossypol increases fatty acid-amino acid conjugates in the cotton pests helicoverpa armigera and heliothis virescens. Arch. Insect Biochem. Physiol. 108 (3), e21834. doi: 10.1002/arch.21843
Kundu, A., Vadassery, J. (2019). Chlorogenic acid-mediated chemical defence of plants against insect herbivores. Plant Biol. (Stuttg) 21, 185–189. doi: 10.1111/plb.12947
Leiss, K. A., Maltese, F., Choi, Y. H., Verpoorte, R., Klinkhamer, P. G. L. (2009). Identification of chlorogenic acid as a resistance factor for thrips in chrysanthemum. Plant Physiol. 150 (3), 1567–1575. doi: 10.1104/pp.109.138131
Liao, Y., Zeng, L., Rao, S., Gu, D., Liu, X., Wang, Y. R., et al. (2020). Induced biosynthesis of chlorogenic acid in sweetpotato leaves confers the resistance against sweetpotato weevil attack. J. Adv. Res. 24, 513–522. doi: 10.1016/j.jare.2020.06.011
Li, S., Huang, X., McNeill, M. R., Liu, W., Tu, X., Ma, Z. C., et al. (2019). Dietary stress from plant secondary metabolites contributes to grasshopper (oedaleus asiaticus) migration or plague by regulating insect insulin-like signaling pathway. Front. Physiol. 10. doi: 10.3389/fphys.2019.00531
Li, Y. K., Lv, B., Hu, Z. N., Wu, W. J. (2019). Research advances in the insecticidal plant periploca sepium. Chin. J. Pesticide Science. 21 (5-6), 709–717. doi: 10.16801/j.issn.1008-7303.2019.0088
Lin, D. J., Lin, G. H., Wang, Y. R., Gao, S. J., Wang, J. D. (2021). Effects of methyl jasmonate induced corn on the growth and development of mythimna separata. J. South. Agriculture. 52, 2465–2472. doi: 10.3969/j.issn.2095-1191.2021.09.016
Liu, H. J., Duan, L. Q., Li, H. P., Feng, S. J., Zhang, B. B. (2016). Chlorogenic acid enhances the virulence of Lymantria dispar necleopolyhydrovirus(LdNPV). Acta Entomologica Sinica. 59 (05), 568–572. doi: 10.16380/j.kcxb.2016.05.012
Liu, J., Chen, Z. S., Wu, H. J., Wu, C., Wu, H. J., Zhang, C.Z., et al (2016). Effect of exogenous chlorogenic acid onAdventitious rooting of soybean hypocotyl. Natural Product Res. Dev. 28, 262–265. doi: 10.16333/j.1001-6880.2016.2.017
Liu, Z. Y., Jiu, K., Wu, X., Guo, L. N., Ma, S. C., et al. (2020). HPLC specific chromatogram of vernonia anthelmintica and determination of six components. China J. Chin. Materia Med. 45, 910–915. doi: 10.19540/j.cnki.cjcmm
Lu, K., Song, Y. Y., Zeng, R. S. (2020). The role of cytochrome P450-mediated detoxification in insect adaptation to xenobiotics. Curr. Opin. Insect Sci. 43, 103–107. doi: 10.3389/fpls.2018.01651
Mattar Valeria, T., Borioni, José L., Hollmann, A., Rodriguez, S. A. (2022). Insecticidal activity of the essential oil of schinus areira against rhipibruchus picturatus (F.) (Coleoptera: Bruchinae), and its inhibitory effects on acetylcholinesterase. Pesticide Biochem. Physiol. 185. doi: 10.1016/J.PESTBP.2022.105134
Mishra, V. K., Tomar, A., Upadhyay, S., Singh, S. S., Vishnu, K., et al. (2021). Survey of the farmer's fields for studying the infestation caused by fall army worm and corn earworm in bundelkhand region. J. Entomological Res. 45, 482–485. doi: 10.5958/0974-4576.2021.00075.x
Nauen, R., Bass, C., Feyereisen, R., Vontas, J. (2021). The role of cytochrome p450s in insect toxicology and resistance. Annu. Rev. Entomology 67, 105–124. doi: 10.1146/annurev-ento-070621-061328
Neşe Çokuğraş, A., Ebru, B. (2003). Comparative effects of two plant growth regulators; indole-3-acetic acid and chlorogenic acid on human and horse serum butyrylcholinesterase. Pesticide Biochem. Physiol. 77, 24–33. doi: 10.1016/S0048-3575(03)00071-3
Pang, Z., Dong, F., Liu, Q., Lin, W. X., Hu, C. H., Yuan, Z. N. (2021). Soil metagenomics reveals effects of continuous sugarcane cropping on the structure and functional pathway of rhizospheric microbial community. Front. Microbiol. 12. doi: 10.3389/FMICB.2021.627569
Pan, Z. Y., Mo, X. N., Meng, X., Chen, M. (2020). Effects of chlorogenic acid on the growth and development and detoxification-related (Lepidoptera: Arctiidae) larvae. Acta Entomologica Sinica. 63, 1081–1090. doi: 10.16380/j.kcxb.2020.09.005
Schuler, M. A. (2012). Insect P450s: mounted for battle in their war against toxins. Mol. Ecol. 21 (17), 4157–4159. doi: 10.1111/j.1365-294X.2012.05657.x
Shen, J. C., Liao, W. C., Peng and Wang, G. L. (2021). A correlation analysis of host selection of apriona germari (Coleoptera:Cerambycidae)with content of plant nutrition component and secondary metabolites. Acta Agriculturae Universitatis Jiangxiensis. 43, 783–791. doi: 10.13836/j.jjau.2021086
Sobhy, I. S., Lou, Y., Bruce, T. J. A. (2022). Editorial: inducing plant resistance against insects using exogenous bioactive chemicals: key advances and future perspectives. Front. Plant Sci. 13. doi: 10.3389/fpls.2022.890884
Song, Y. Q., Wang, H. T., Chen, Y. G., Wang, S. Y., Sun, H. Z. (2017). Cross-resistance and biochemical resistance mechanisms of emamectin benzoate resistant population of mythimna separate. Chin. J. Pesticide Science. 19, 18–24. doi: 10.16801/j.issn.1008-7303.2017.0001
Summers, C. B., Felton, G. W. (1994). Prooxidant effects of phenolic acids on the generalist herbivore helicoverpa zea (lepidoptera: noctuidae): potential mode of action for phenolic compounds in plant anti-herbivore chemistry. Insect Biochem. Mol. Biol. 24, 943–953. doi: 10.1016/0965-1748(94)90023-X
Sun, Z., Lv, M., Yu, X., Xu, H. (2017). Application of sustainable natural bioesources in crop protection: insight into a podophyllotoxin-derived botanical pesticide for regulating insect vestigial wing of mythimna separata walker. ACS Sustain. Chem. Eng. 5, 3945–3954. doi: 10.1021/acssuschemeng.6b03145
Tang, T., Cheng, Y. H., Wang, C. J., Zhang, W. J., Qiu, L. H. (2007). Cloning and characterization of cytochrome P450 CYP6B7 from fenvalerate resistant cotton bollworm, helicoverpa armigera. Chin. J. Pesticide Science. 04), 370–375. doi: 1008-7303(2007)04-0370-06
Tian, K., Liu, D., Yuan, Y. Y., Li, M., Qiu, X. H. (2017). CYP6B6 is involved in esfenvalerate detoxification in the polyphagous lepidopteran pest, helicoverpa armigera. Pestic Biochem. Physioli. 138, 51–56. doi: 10.1016/j.pestbp.2017.02.006
Tod, L. O., Shaw, Y. H., Richard, L. L. (2000). Effects of phytochemical variation in quaking aspen populus tremuloides clones on gypsy moth lymantria dispar performance in the field and laboratory. Ecol. Entomology 25 (2), 197–207. doi: 10.1046/j.1365-2311.2000.00245.x
Wan, P. J., Shi, X. Q., Kong, Y., Zhou, L. T., Gou, W. C., Ahmat, T., et al (2022). Identification of cytochrome P450 monooxygenase genes and their expression profiles in cyhalothrin-treated Colorado potato beetle, Leptinotarsadecemlineata. Pesticide Biochem. Physiol. 107, 360–368. doi: 10.1016/j.pestbp.2013.10.004
Wang, B. Q., Cheng, L. H., Fang, Y., Li, W. W., Liu, J. N. (2022). Effects of plant secondary metabolites on detoxification enzyme activity and related gene expression of spodoptera frugiperda. Jiangsu Agric. Sci. 50, 11–15. doi: 10.15889/j.issn.1002-1302.2022.08.003
Wang, J. D., Chen, L. F., Lin, D. J., Zhang, J. S., Zhao, J. H., Xiao, D., et al. (2019). Molecular cloning, characterization and functional analysis of glucl from the oriental armyworm, mythimna separata walker. Pesticide Biochem. Physiol. 156, 56–62. doi: 10.1016/j.pestbp.2019.02.004
Wang, Y. D., Chen, L. C., Qin, Q. Q., Li, Z. (2022). Expression analysis of cytochrome P450 monooxidase AmCYP9q1 in honeybee under lmidacloprid stress. J. Chongqing Normal Univ. (Natural Science) 39, 67–77. doi: 10.11721/cqnuj20220305
Wang, Y. R., Zhang, J. S., Wang, R., Hou, Y. M., Fu, H. A., Xie, Y., et al. (2021). Unveiling sugarcanedefense response to mythimna separata herbivory by a combination of transcriptome and metabolicanalyses. Pest Manage Sci 77, 4799–809. doi: 10.1002/ps.6526
Wang, J. D., Chen, L. F., Wang, Y. R., Fu, H. Y., Ahmad, A., Xiao, D., et al. (2018). Silence of ryanodine receptor gene decreases susceptibility to chlorantraniliprole in the oriental armyworm, mythimna separata walker. Pesticide Biochem. Physiol. 148, 34–41. doi: 10.1016/j.pestbp.2018.03.012
Wang, X. L., Wang, Y. T., Duan, L. Q., Li, H. P., Feng, S. J. (2014). Effects of four plant phenolics on the growth and development and fecundity of the gypsy moth, lymantria dispar (Lepidoptera: Lymantriidae). Acta Entomologica Sinica. 57, 831–836. doi: 10.16380/j.kcxb.2014.07.013
Wang, J. D., Wang, W. Z., Wang, Y. R., Gao, S. J., Elzaki, M., Wang, N., et al. (2018). Response of detoxification and immune genes and of transcriptome expression in mythimna separata following chlorantraniliprole exposure. Comp. Biochem. Physiol. - Part D. 28, 90–98. doi: 10.1016/j.cbd.2018.07.001
Whitehill, J. G. A., Henderson, H., Strong, W., Jaquish, B., Bohlmann, J. (2016). Function of sitka spruce stone cells as a physical defence against white pine weevil. Plant Cell Environ. 39 (11), 2545–2556. doi: 10.1111/pce.12810
Xi, L. S., Mu, T. H., Sun, H. N. (2014). Progresses in the research of chlorogenic acids. J. Nucl. Agric. Sci. 282014 1000–8551, 02–0292-10. doi: 1000-8551(2014)02-0292-10
Xu, H. P., Xie, H. C., Wu, s. y., Wang, Z. Y., He, K. L. (2019). Effects of elevated co2 and increased n fertilization on plant secondary metabolites and chewing insect fitness. Front. Plant Sci. 10. doi: 10.3389/fpls.2019.00739
Xu, D. D., Zhang, Y., Zhang, Y. J., Wu, Q. G., Guo, Z. J., Xie, W., et al. (2020). Transcriptome profiling and functional analysis suggest that the constitutive overexpression of four cytochrome P450s confers resistance to abamectin in tetranychus urticae from China. Pest Manage. Sci. 77 (3), 1204–1213. doi: 10.1002/ps.6130
Yang, H. L. (2021). Control techniques for main pests and diseases of rice in heqing county, yunnan province. Agric. Eng. Technol. 41, 34–35. doi: 10.16815/j.cnki.11-5436/s.2021.29.019
Ye, M., Nayak, B., Xiong, L., Xie, C., Dong, Y., You, M. S., et al. (2022). The role of insect cytochrome p450s in mediating insecticide resistance. Agriculture 12 (1), 53. doi: 10.3390/agriculture12010053
Young, H. K., Moustapha, S. I., Anastasia, M. W. C., Kun, Y. Z. (2015). Rna interference: applications and advances in insect toxicology and insect pest management. Pesticide Biochem. Physiol. 120, 109–117. doi: 10.1016/j.pestbp.2015.01.002
Zainab, A. S. A., Manfred, H. (2020). Plant oil mixtures as a novel botanical pesticide to control gregarious locusts. Pest Sci. 93, 341–353. doi: 10.1007/s10340-019-01169-7
Zhang, X. (2018). Preliminary studies on the genomic bases of polyphagy in helicoverpa armigera (Beijing, China: Agricultural Entomology and Pest Management).
Zhang, G. (2019). Expression analysis of P450 and GST in the transcriptome sequencing and lambda-cyhalothrin stress of spodoptera litura (Anhui, China: Anhui Agricultural University). doi: 10.26919/d.cnki.gannu.2019.000025
Zhang, L. Y., Ruan, C. C., Hou, Z. G., Lu, Z. B., Wang, X. M. (2021). Effects on the energy substances of myzus persicae by the sublethal concentration of sulfoxaflor. Agrochemicals 60 (01), 28–31+34. doi: 10.16820/j.cnki.1006-0413.2021.01.007
Keywords: chlorogenic acid, sublethal effect, P450, RNAi, mythinma seperata
Citation: Lin D-j, Fang Y, Li L-y, Zhang L-z, Gao S-j, Wang R and Wang J-d (2022) The insecticidal effect of the botanical insecticide chlorogenic acid on Mythimna separata (Walker) is related to changes in MsCYP450 gene expression. Front. Plant Sci. 13:1015095. doi: 10.3389/fpls.2022.1015095
Received: 09 August 2022; Accepted: 23 September 2022;
Published: 12 October 2022.
Edited by:
Minmin Li, Institute of Food Science and Technology (CAAS), ChinaReviewed by:
Lin Jin, Nanjing Agricultural University, ChinaCopyright © 2022 Lin, Fang, Li, Zhang, Gao, Wang and Wang. This is an open-access article distributed under the terms of the Creative Commons Attribution License (CC BY). The use, distribution or reproduction in other forums is permitted, provided the original author(s) and the copyright owner(s) are credited and that the original publication in this journal is cited, in accordance with accepted academic practice. No use, distribution or reproduction is permitted which does not comply with these terms.
*Correspondence: Jin-da Wang, amR3YW5nQGZhZnUuZWR1LmNu; Ran Wang, cndhbmcxMTA1QDEyNi5jb20=
Disclaimer: All claims expressed in this article are solely those of the authors and do not necessarily represent those of their affiliated organizations, or those of the publisher, the editors and the reviewers. Any product that may be evaluated in this article or claim that may be made by its manufacturer is not guaranteed or endorsed by the publisher.
Research integrity at Frontiers
Learn more about the work of our research integrity team to safeguard the quality of each article we publish.