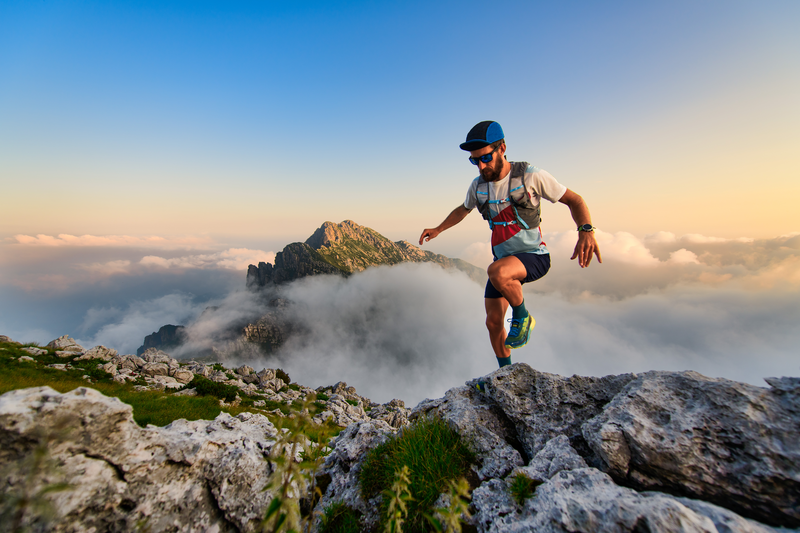
94% of researchers rate our articles as excellent or good
Learn more about the work of our research integrity team to safeguard the quality of each article we publish.
Find out more
MINI REVIEW article
Front. Plant Sci. , 01 December 2022
Sec. Plant Abiotic Stress
Volume 13 - 2022 | https://doi.org/10.3389/fpls.2022.1014816
This article is part of the Research Topic Nanofertilizers and Abiotic Stress Tolerance in Plants View all 9 articles
Insufficient availability of water is a major global challenge that plants face and that can cause substantial losses in plant productivity and quality, followed by complete crop failure. Thus, it becomes imperative to improve crop cultivation/production in unsuitable agricultural fields and integrate modern agri-techniques and nanoparticles (NPs)-based approaches to extend appropriate aid to plants to handle adverse environmental variables. Nowadays, NPs are commonly used with biological systems because of their specific physicochemical characteristics, viz., size/dimension, density, and surface properties. The foliar/soil application of nanosilicon (nSi) has been shown to have a positive impact on plants through the regulation of physiological and biochemical responses and the synthesis of specific metabolites. Reactive oxygen species (ROS) are produced in plants in response to drought/water scarcity, which may enhance the ability for adaptation in plants/crops to withstand adverse surroundings. The functions of ROS influenced by nSi and water stress have been assessed widely. However, detailed information about their association with plants and stress is yet to be explored. Our review presents an update on recent developments regarding nSi and water stress in combination with ROS accumulation for sustainable agriculture and an eco-friendly environment.
Insufficient water availability is a big problem that causes substantial global losses in plant performance and productivity both quantitatively and qualitatively. The plants are the main producers of bio-ecosystems, and they often have to respond to different agroclimatic conditions such as water deficits, submergence/soil flooding, heavy metal toxicity, pesticides/herbicides, salt stress, high and low light intensities, pests/insects, and pathogens (Ghorbanpour et al., 2020; Verma et al., 2022a; Verma et al., 2022b). It is well documented that water deficits may impair photosynthetic and metabolic processes associated with the regulation of proper plant growth and development (Verma et al., 2021).
Enhancing plant yield in marginal agricultural areas is an integral part of the second agro-technological revolution, and NP-based approaches have already demonstrated the significance of their added advantages in plants: the ability to adapt to harsh atmospheric conditions (Adisa et al., 2019). Consequently, research into nanomaterials (NMs) has received significant attention due to their unique physical-chemical characteristics. Furthermore, in terms of diffusivity, electrical resistivity, and electrical conducting characteristics, nanoparticles are considered completely different from bulk materials (Khashan et al., 2016; Mary et al., 2019). Upon comparison with their respective bulk forms, nanoparticles are found to be almost “identical” with molecular sizes of around 1–100 nm in diameter with specific characteristics (Verma et al., 2022a). The rapid use and accumulation of engineered nanoparticles (ENPs) in the environment, and their unknown interactions with various species, revealed SNPs that are more toxic, which has raised concerns regarding environmental health (Nel et al., 2006; Thwala et al., 2016). Apart from this, the use of several ENPs has had a negative impact on the natural environment, including the quality of the water, air, and soil (Hashimoto et al., 2017). Plants are crucial components of the biosphere and actively interact with ENPs, and ENPs could thus easily be absorbed by plant roots, enter the food chain through dietary intake, and eventually have an adverse impact on human health (Pittol et al., 2017).
Silicon (Si) is a potential element that may help plants when responding to a water deficit by providing structural cellular stability, including for cell organelles. Silicon constitutes a major part of the soil in the form of silicate and aluminum silicates. It exists as monomeric or monosilicic acid in the soil solution, where it may be taken by plant roots and supplied to above-ground plant parts (Verma et al., 2020; Song et al., 2021). Silicon nanoparticles (nSi) demonstrated a potential role in enhancing proper plant development, especially crop productivity during biotic or abiotic stresses (Adisa et al., 2019; Hussain et al., 2019; Rajput et al., 2021). The studies made so far observed the potential influence of nSi through foliar/soil application or seed priming to acquire abilities to combat metal toxicity, UV-B radiation, alkalinity, salinity, water deficit or water surplus, low or high light intensity, and oxidative stress (Siddiqui et al., 2014; Cui et al., 2017; Hussain et al., 2019; Elsheery et al., 2020; Rajput et al., 2021).
Insufficient water may promote the generation of ROS: hydrogen peroxide (H2O2), hydroxyl radical (•OH), and superoxide anion . Plants have developed a number of adaptative and defensive mechanisms, including the activation of efficient enzymatic and non-enzymatic antioxidative defense systems, to ameliorate the damaging effects of ROS (Adisa et al., 2019; Verma et al., 2022a) (Figure 1); limited findings have been made regarding the effects of nSi on the mechanisms of ROS during the water-deficit condition. Therefore, various possibilities are reported in our review on the importance of nSi in regulation of ROS mechanisms linked with stress tolerance in plants and crop production for sustainable agriculture.
Figure 1 Schematic presentation indicating the possible causes for overproduction of ROS that could damage the normal functions of the cells. , Superoxide; OH·, hydroxyl; , hydroperoxyl; , peroxyle; , carbonate; RO·, alkoxyl; SQ·, semiquinone; O3, ozone; H2O2, hydrogen peroxide; 1O2, singlet oxygen; HOBr, hypobromous acid; ROOH, hydroperoxides; HOI, hypoiodous acid; and HOCl, hypochlorous acid.
The generation of ROS is found to be triggered from available molecular oxygen in vivo. The adaptive mechanism of ROS detoxification in plant cells is also supported by a variety of adaptive metabolic strategies that balance the level of free transient metals (Fe2+) and downregulate the production of ROS to prevent the formation of an excessive amount of hydroxyl radicals via the Fenton reaction (Choudhury et al., 2017). All cellular compartments (the apoplast, chloroplast, peroxisome, mitochondria, vacuole, cytosol, and nuclei) continuously produce ROS, and the ROS gene network regulates the process (Choudhury et al., 2017). The plants respond to environmental challenges in controlling the formation of ROS (Foyer and Noctor, 2016) in the form of the singlet oxygen (1O2), superoxide anion , hydroxyl radical (•OH), and hydrogen peroxide (H2O2), all of which contain oxygen and are extremely reactive due to their electron receptivity. ROS production also results from aerobic metabolic activities like photosynthesis and enzymatic and non-enzymatic processes (Apel and Hirt, 2004; Jiang et al., 2021).
The stresses may enhance ROS formation by raising ROS levels in plants (Ahmad et al., 2010). Besides these events, genetically programmed enzymatic mechanisms such generation by NADPH oxidases or the production of photoactivation phytoalexins may actively produce ROS in response to stress (Flors and Nonell, 2006). By reprogramming gene expression, altering cell walls, and rarely inducing programmed cell death (i.e., the hypersensitive response) to protect against viruses and other hazards, ROS may help cells adapt to stress (Waszczak et al., 2018). However, if the plant’s antioxidant system is unable to regulate the timing and amount of ROS generation, ROS may disrupt the plants’ own membrane lipids, proteins, and DNA (Demidchik, 2015; Czarnocka and Karpinski, 2018). Apart from this, a few studies also indicated the time and intensity of ROS generation and their types, produced in various cellular compartments to mitigate the consequences of oxidative stress (Gadjev et al., 2006; Shapiguzov et al., 2012).
ROS has been confirmed to play an important role in connecting various morpho-physiological processes in living organisms (Jiang et al., 2021). The chloroplast, mitochondria, peroxisome, and apoplast are the four important ROS-producing organelles (Jiang et al., 2021) subjected to environmental stresses. The production of ROS may also maintain balance in the energy transfer between PSII and PSI under stressed conditions (Kleine and Leister, 2016). The alleviation of chloroplastic ROS was found to be influenced by an array of ROS-scavenging enzymes and Fe- and CuZn-SODs, Asada-Foyer-Halliwell pathways, and excess levels of antioxidatives (Choudhury et al., 2017). ROS may cause proteins to undergo reversible and/or irreversible modifications and may also alter plant metabolism through transcriptional regulatory systems along with sulfonylation, carbonylation, glutathionylation, and S-nitrosylation found to be regulated by ROS-induced post-translational modifications (Choudhury et al., 2017).
Various reports demonstrated the efficiency of ENPs in agriculture (Manjunatha et al., 2016; Adisa et al., 2019; Verma et al., 2022a; Verma et al., 2022b). However, the majority of earlier investigations on the interactions between plants and ENPs concentrated on the possible toxicity of nanoparticles to higher plants. ENPs have been found to have both a significant and insignificant impact on plants (Hatami and Ghorbanpour, 2014). Generally, the formation of ROS in plant cells creates an interface for the phytotoxicity of ENPs (Melegari et al., 2013). According to Rico et al. (2015) and Ma et al. (2015), plants typically produce ROS as a byproduct of metabolic processes in chloroplasts and other organelles. However, excessive ROS production may harm the photosynthetic apparatus and other physiological and biochemical systems, eventually leading to the activation of defense mechanisms in plants, like increased antioxidant activity (Du et al., 2017). Additionally, the formation of ENPs may activate defense systems by activating antioxidant enzymes to eliminate the toxicity of ROS (Rai et al., 2018). The variations in nSi types, exposure situations, and variety of crops may influence its generation/accumulation levels and antioxidant responses (Ghorbanpour et al., 2020).
Reactive oxygen species are generally formed as a by-product of plant metabolic processes. Numerous ecological conditions may cause overproduction of ROS in plants with progressive oxidative damage (Chahardoli et al., 2020; Elsheery et al., 2020). The activities of antioxidant enzymes in plants increase in response to atmospheric environmental variables (Adisa et al., 2019). Plant tolerance to oxidative stress may be improved by increased antioxidant enzyme activities (Mittler, 2002) with the activation to catalase (CAT), a crucial enzyme that scavenges ROS in plant cells. CAT participates in the main defensive mechanism against the enhancement of H2O2 and may control H2O2 levels in cells (Song et al., 2016). The enhanced CAT may be attributed to more elevated superoxide dismutase (SOD) and higher production of H2O2 (Chahardoli et al., 2020).
Peroxidase (POD) influences the production of lignin, ethylene, and the breakdown of indole-3-acetic acid (IAA) in addition to lowering H2O2 generation under oxidative stress; it is also associated with plant tolerance to pathogens and an aid for wound healing (Song et al., 2016). By accelerating the dismutation of free hydroxyl radicals to H2O2 and O2, SOD was found to be essential (Chahardoli et al., 2020), enhancing the development of plant incase associated with nano-based approaches. Thus, a variety of nano-sized particles have been manufactured recently to enhance the productivity of crops in marginal agricultural areas subjected to adverse environmental variables (Elsheery et al., 2020; Rajput et al., 2021; Verma et al., 2022a).
Plants need to balance/maintain their ROS levels inside the cell to deal with oxidative damage during environmental stress, and this is accomplished through complex enzymatic activities, such as SOD, CAT, POD, GR, and APX, and also non-enzymatic activities, i.e., carotenoids, non-protein amino acids and phenolic compounds (Yang et al., 2010; Adisa et al., 2019). The minimal oxidative damage indices that followed rehydrating plants with nSi indicated its major role in scavenging excessive ROS and activating antioxidant defense mechanisms in plants during drought. The excess level of ROS could be due to decreased CAT activity during unfavorable environmental variables (Chahardoli et al., 2020; Verma et al., 2022a; Verma et al., 2022b). The enhanced CAT activity in the plants associated with nSi reveals an enhancement in the ROS-scavenging capacity of stressed plants, which is accompanied by plant protection/production from oxidative damage. POD activity was substantially enhanced during water-stressed conditions; the production of H2O2 found apparently beyond capacity of plant cells creates ROS formation/oxidative stress in plants. POD facilitates the conversion of H2O2 into OH˙ (Chen and Schopfer, 1999). Plant cells are protected against oxidative stress and lipid peroxidation, APX—a component of the ascorbate-glutathione (AsA-GSH) cycle, and the major ROS scavenging process (Candan and Tarhan, 2003; Elsheery et al., 2020). Hence, activation of SOD, if accompanied by the other ROS-scavenging enzymes, enables defense strategies to alleviate oxidative burst during times of drought in plants (Ghorbanpour et al., 2013) (Figure 1).
To alleviate the various adverse environmental variables, recent agricultural approaches need to fine-tune the intrinsic capabilities of cellular systems/plants. Agricultural applications and approaches may also aim to enhance plant production during climate change, which may cause major losses to overall plant performance and productivity. Hence, we require the implementation of newer approaches to ensure food security in developing countries, adapting to cropping systems growing under changing environmental conditions associated with application of suitable irrigation methods and fertilizers. To solve this concern, effective low-cost agro-technologies will be useful for agrofarmers. ROS appear to be important to plants faced with environmental challenges, as ROS enable them to change their metabolic activities and develop a suitable acclimation response: as long as the cells are balanced, there are adequate energy stores to detoxify ROS. nSi has been found to have a significant role in agro-ecosystems and to increase stress resistance capacities for future sustainable agriculture.
KV and Y-RL conceived the article. All authors contributed to the discussion and approved the final manuscript for publication.
This research was financially supported by the Guangxi Innovation Teams of Modern Agriculture Technology (nycytxgxcxtd-2021-03), Youth Program of National Natural Science Foundation of China (31901594), The National Natural Science Foundation of China (31760415), Guangxi Natural Science Foundation (2021GXNSFAA220022), Fund of Guangxi Academy of Agricultural Sciences (2021YT011) and Guangxi Key Laboratory of Sugarcane Genetic Improvement Project (21-238-16-K-04-02).
The authors would like to thank the Guangxi Academy of Agricultural Sciences, Nanning, Guangxi, China, for providing the necessary facilities for this study.
The authors declare that the research was conducted in the absence of any commercial or financial relationships that could be construed as a potential conflict of interest.
All claims expressed in this article are solely those of the authors and do not necessarily represent those of their affiliated organizations, or those of the publisher, the editors and the reviewers. Any product that may be evaluated in this article, or claim that may be made by its manufacturer, is not guaranteed or endorsed by the publisher.
Adisa, I. O., Pullagurala, V. L. R., Peralta-Videa, J. R., Dimkpa, C. O., Elmer, W. H., Gardea-Torresdey, J. L., et al. (2019). Recent advances in nano-enabled fertilizers and pesticides: a critical review of mechanisms of action. Environ. Sci.: Nano 6, 2002–2030. doi: 10.1039/C9EN00265K
Ahmad, P., Jaleel, C. A., Salem, M. A., Nabi, G., Sharma, S. (2010). Roles of enzymatic and nonenzymatic antioxidants in plants during abiotic stress. Crit. Rev. Biotech. 30, 161–175. doi: 10.3109/07388550903524243
Apel, K., Hirt, H. (2004). Reactive oxygen species: metabolism, oxidative stress, and signal transduction. Annu. Rev. Plant Biol. 55, 373–399. doi: 10.1146/annurev.arplant.55.031903.141701
Candan, N., Tarhan, L. (2003). The correlation between antioxidant enzyme activities and lipid peroxidation levels in mentha pulegium organs grown in Ca2+, Mg2+, Cu2+, Zn2+ and Mn2+ stress conditions. Plant Sci. 163, 769–779. doi: 10.1016/S0168-9452(03)00269-3
Chahardoli, A., Karimi, N., Ma, X., Qalekhani, F. (2020). Effects of engineered aluminum and nickel oxide nanoparticles on the growth and antioxidant defense systems of Nigella arvensis l. Sci. Rep. 10, 3847. doi: 10.1038/s41598-020-60841-6
Chen, S., Schopfer, P. (1999). Hydroxyl-radical production in physiological reactions. a novel function of peroxidase. Eur. J. Biochem. 260, 726–735. doi: 10.1046/j.1432-1327.1999.00199.x
Choudhury, F., Rivero, R. M., Blumwald, E., Mittler, R. (2017). Reactive oxygen species, abiotic stress and stress combination. Plant J. 90, 856–867. doi: 10.1111/tpj.13299
Cui, J., Liu, T., Li, F., Yi, J., Liu, C., Yu, H. (2017). Silica nanoparticles alleviate cadmium toxicity in rice cells: mechanisms and size effects, environ. Pollut. 228, 363–369. doi: 10.1016/j.envpol.2017.05.014
Czarnocka, W., Karpinski, S. (2018). Friend or foe? reactive oxygen species production, scavenging and signaling in plant response to environmental stresses. Free Radic. Biol. Med. 122, 4–20. doi: 10.1016/j.freeradbiomed.2018.01.011
Demidchik, V. (2015). Mechanisms of oxidative stress in plants: from classical chemistry to cell biology. Environ. Exp. Bot. 109, 212–228. doi: 10.1016/j.envexpbot.2014.06.021
Du, W., Tan, W., Peralta-Videa, J. R., Gardea-Torresdey, J. L., Ji, R., Yin, Y., et al. (2017). Interaction of metal oxide nanoparticles with higher terrestrial plants: Physiological and biochemical aspects. Plant Physiol. Biochem. 110, 210–225. doi: 10.1016/j.plaphy.2016.04.024
Elsheery, N. I., Sunoj, V. S. J., Wen, Y., Zhu, J. J., Muralidharan, G., Cao, K. F. (2020). Foliar application of nanoparticles mitigates the chilling effect on photosynthesis and photoprotection in sugarcane. Plant Physiol. Biochem. 149, 50–60. doi: 10.1016/j.plaphy.2020.01.035
Flors, C., Nonell, S. (2006). Light and singlet oxygen in plant defense against pathogens: phototoxic phenalenone phytoalexins. Acc. Chem. Res. 39, 293–300. doi: 10.1021/ar0402863
Foyer, C. H., Noctor, G. (2016). Stress-triggered redox signalling: what’s in pROSpect? Plant Cell Environ. 39, 951–964. doi: 10.1111/pce.12621
Gadjev, I., Vanderauwera, S., Gechev, T. S., Laloi, C., Minkov, I. N., Shulaev, V., et al. (2006). Transcriptomic footprints disclose specificity of reactive oxygen species signaling in arabidopsis. Plant Physiol. 141, 436–445. doi: 10.1104/pp.106.078717
Ghorbanpour, M., Hatami, M., Khavazi, K. (2013). Role of plant growth promoting rhizobacteria on antioxidant enzyme activities and tropane alkaloids production of Hyoscyamus niger under water deficit stress. Turk. J. Biol. 37, 350–360. doi: 10.3906/biy-1209-12
Ghorbanpour, M., Mohammadi, H., Kariman, K. (2020). Nanosilicon-based recovery of barley (Hordeum vulgare) plants subjected to drought stress. Environ. Sci.: Nano 7, 443–461. doi: 10.1039/C9EN00973F
Hashimoto, Y., Takeuchi, S., Mitsunobu, S., Ok, Y.-S. (2017). Chemical speciation of silver (Ag) in soils under aerobic and anaerobic conditions: Ag nanoparticles vs. ionic Ag. J. Hazard Mater. 322, 318–324. doi: 10.1016/j.jhazmat.2015.09.001
Hatami, M., Ghorbanpour, M. (2014). Defense enzyme activities and biochemical variations of pelargonium zonale in response to nanosilver application and dark storage. Turk. J. Biol. 38, 130–139. doi: 10.3906/biy-1304-64
Hussain, A., Rizwan, M., Ali, Q., Ali, S. (2019). Seed priming with silicon nanoparticles increased biomass and yield while reduced the oxidative stress and cadmium concentration in wheat grains. Environ. Sci. Pollut. Res. 26, 7579–7588. doi: 10.1007/s11356-019-04210-5
Jiang, H., Lin, Q., Yu, Z., Wang, C., Zhang, R. (2021). Nanotechnologies for reactive oxygen species “turn-on” detection. Front. Bioeng. Biotechnol. 9, 780032. doi: 10.3389/fbioe.2021.780032
Khashan, K. S., Sulaiman, G. M., Abdulameer, F. A. (2016). Synthesis and antibacterial activity of CuO nanoparticles suspension induced by laser ablation in liquid. Arab. J. Sci. Eng. 41, 301–310. doi: 10.1007/s13369-015-1733-7
Kleine, T., Leister, D. (2016). Retrograde signaling: Organelles go networking. Biochim. Biophys. Acta (BBA) – Bioenergetics 1857(8), 1313–1325. doi: 10.1016/j.bbabio.2016.03.017
Manjunatha, S., Biradar, D., Aladakatti, Y. (2016). Nanotechnology and its applications in agriculture: A review. J. Farm. Sci. 29, 1–13.
Mary, A. P. A., Ansari, A. T., Subramanian, R. (2019). Sugarcane juice mediated synthesis of copper oxide nanoparticles, characterization and their antibacterial activity. J. King Saud Univ. Sci. 31, 1103–1114. doi: 10.1016/j.jksus.2019.03.003
Ma, C., White, J. C., Dhankher, O. P., Xing, B. (2015). Metal-based nanotoxicity and detoxification pathways in higher plants. Environ. Sci. Technol. 49, 7109–7122. doi: 10.1021/acs.est.5b00685
Melegari, S. P., Perreault, F., Costa, R. H. R., Popovic, R., Matias, W. G. (2013). Evaluation of toxicity and oxidative stress induced by copper oxide nanoparticles in the green alga chlamydomonas reinhardtii. Aquat. Toxicol. 142, 431–440. doi: 10.1016/j.aquatox.2013.09.015
Mittler, R. (2002). Oxidative stress, antioxidants and stress tolerance. Trends Plant Sci. 7, 405–410. doi: 10.1016/S1360-1385(02)02312-9
Nel, A., Xia, T., Mädler, L., Li, N. (2006). Toxic potential of materials at the nanolevel. Sci. 311, 622–627. doi: 10.1126/science.1114397
Pittol, M., Tomacheski, D., Simões, D. N., Ribeiro, V. F., Santana, R. M. C. (2017). Macroscopic effects of silver nanoparticles and titanium dioxide on edible plant growth. Environ. Nanotechnol. Monit. Manage. 8, 127–133. doi: 10.1016/j.enmm.2017.07.003
Rai, P. K., Kumar, V., Lee, S. S., Raza, N., Kim, K. H., Ok, Y. S., et al. (2018). Nanoparticle-plant interaction: Implications in energy, environment, and agriculture. Environ. Int. 119, 1–19. doi: 10.1016/j.envint.2018.06.012
Rajput, V. D., Minkina, T., Feizi, M., Kumari, A., Khan, M., Mandzhieva, S., et al. (2021). ). effects of silicon and silicon-based nanoparticles on rhizosphere microbiome, plant stress and growth. Biology 10, 791. doi: 10.3390/biology10080791
Rico, C. M., Peralta-Videa, J. R., Gardea-Torresdey, J. L. (2015). Chemistry, biochemistry of nanoparticles, and their role in antioxidant defense system in plants"". In: Nanotechnology and plant sciences (Cham: Springer), 1–17.
Shapiguzov, A., V ainonen, J., Wrzaczek, M., Kangasjärvi, J. (2012). ROS-talk –how the apoplast, the chloroplast, and the nucleus get the message through. Front. Plant Sci. 3. doi: 10.3389/fpls.2012.00292
Siddiqui, M. H., Al-Whaibi, M. H., Faisal, M., Al-Sahli, A. A. (2014). Nano-silicon dioxide mitigates the adverse effects of salt stress on Cucurbita pepo l, environ. Toxicol. Chem. 33, 2429–2437. doi: 10.1002/etc.2697
Song, G., Hou, W., Gao, Y., Wang, Y., Lin, L., Zhang, Z., et al. (2016). Effects of CuO nanoparticles on Lemna minor. bot Stud. 57, 1–8. doi: 10.1186/s40529-016-0118-x
Song, X. P., Verma, K. K., Tian, D. D., Zhang, X. Q., Liang, Y. J., Huang, X., et al. (2021). Exploration of silicon functions to integrate with biotic stress tolerance and crop improvement. Biol. Res. 54, 19. doi: 10.1186/s40659-021-00344-4
Thwala, M., Klaine, S. J., Musee, N. (2016). Interactions of metal-based engineered nanoparticles with aquatic higher plants: A review of the state of current knowledge. Environ. Toxicol. Chem. 35, 1677–1694. doi: 10.1002/etc.3364
Verma, K. K., Li, D. M., Singh, M., Rajput, V. D., Malviya, M. K., Minkina, T., et al. (2020). Interactive role of silicon and plant-rhizobacteria mitigating abiotic stresses: A new approach for sustainable agriculture and climate change. Plants 9, 1055. doi: 10.3390/plants9091055
Verma, K. K., Song, X. P., Joshi, A., Rajput, V. D., Singh, M., Sharma, A., et al. (2022b). Nanofertilizer possibilities for healthy soil, water and food in future: An overview. Front. Plant Sci. 13. doi: 10.3389/fpls.2022.865048
Verma, K. K., Song, X. P., Joshi, A., Tian, D. D., Rajput, V. D., Singh, M., et al. (2022a). Recent trends in nano-fertilizer for advancing sustainable agriculture under the era of climate change to ensure food security for future generations. Nanomaterials 12, 173. doi: 10.3390/nano12010173
Verma, K. K., Song, X. P., Zeng, Y., Guo, D. J., Singh, M., Rajputm, V. D., et al. (2021). Foliar application of silicon boosts growth, photosynthetic leaf gas exchange, antioxidative response and resistance to limited water irrigation in sugarcane (Saccharum officinarum l.). Plant Physiol. Biochem. 166, 582–592. doi: 10.1016/j.plaphy.2021.06.032
Waszczak, C., Carmody, M., Kangasjärvi, J. (2018). Reactive oxygen species in plant signaling. Annu. Rev. Plant Biol. 69, 209–236. doi: 10.1146/annurev-arplant-042817-040322
Keywords: antioxidants, reactive oxygen species, water scarcity, nanosilicon, stress resistance efficiency, plants
Citation: Verma KK, Song X-P, Singh M, Huang H-R, Bhatt R, Xu L, Kumar V and Li Y-R (2022) Influence of nanosilicon on drought tolerance in plants: An overview. Front. Plant Sci. 13:1014816. doi: 10.3389/fpls.2022.1014816
Received: 09 August 2022; Accepted: 28 October 2022;
Published: 01 December 2022.
Edited by:
Heba Mahmoud Mohammad Abdel-Aziz, Mansoura University, EgyptReviewed by:
Marzieh Babashpour-Asl, Islamic Azad University, Maragheh, IranCopyright © 2022 Verma, Song, Singh, Huang, Bhatt, Xu, Kumar and Li. This is an open-access article distributed under the terms of the Creative Commons Attribution License (CC BY). The use, distribution or reproduction in other forums is permitted, provided the original author(s) and the copyright owner(s) are credited and that the original publication in this journal is cited, in accordance with accepted academic practice. No use, distribution or reproduction is permitted which does not comply with these terms.
*Correspondence: Yang-Rui Li, bGl5ckBneGFhcy5uZXQ=
†ORCID: Yang-Rui Li, orcid.org/0000-0002-7559-9244
Disclaimer: All claims expressed in this article are solely those of the authors and do not necessarily represent those of their affiliated organizations, or those of the publisher, the editors and the reviewers. Any product that may be evaluated in this article or claim that may be made by its manufacturer is not guaranteed or endorsed by the publisher.
Research integrity at Frontiers
Learn more about the work of our research integrity team to safeguard the quality of each article we publish.