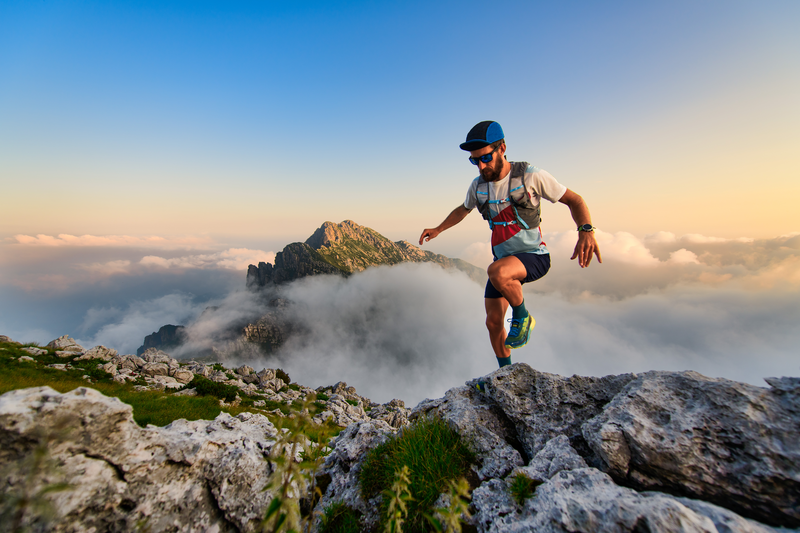
94% of researchers rate our articles as excellent or good
Learn more about the work of our research integrity team to safeguard the quality of each article we publish.
Find out more
ORIGINAL RESEARCH article
Front. Plant Sci. , 14 December 2022
Sec. Plant Physiology
Volume 13 - 2022 | https://doi.org/10.3389/fpls.2022.1014396
Uniconazole, a triazole plant growth regulator, is widely used to regulate plant height and prevent the overgrowth of seedlings. However, the underlying molecular mechanism of uniconazole in inhibiting the hypocotyl elongation of seedlings is still largely unclear, and there has been little research on the integration of transcriptomic and metabolomic data to investigate the mechanisms of hypocotyl elonga-tion. Herein we observed that the hypocotyl elongation of flowering Chinese cabbage seedings was significantly inhibited by uniconazole. Interestingly, based on combined transcriptome and metabolome analyses, we found that the “phenylpropanoid biosynthesis” pathway was significantly affected by uniconazole. In this pathway, only one member of the portal enzyme gene family, named BrPAL4, was remarkably downregulated, which was related to lignin biosynthesis. Furthermore, the yeast one-hybrid and dual-luciferase assays showed that BrbZIP39 could directly bind to the promoter region of BrPAL4 and activate its transcript. The virus-induced gene silencing system further demonstrated that BrbZIP39 could positively regulate hypocotyl elongation and the lignin biosynthesis of hypocotyl. Our findings provide a novel insight into the molecular regulatory mechanism of uniconazole inhibiting hypocotyl elongation in flowering Chinese cabbage and confirm, for the first time, that uniconazole decreases lignin content through repressing the BrbZIP39–BrPAL4 module-mediated phenylpropanoid biosynthesis, which leads to the hypocotyl dwarfing of flowering Chinese cabbage seedlings.
The elongation of hypocotyl is a complex and orderly process, which is affected by external and internal factors (Li et al., 2020; Khalaki et al., 2021). Understanding the molecular mechanism of hypocotyl elongation can establish the foundation for strong seedling cultivation (Song et al., 2022). The intensive production of vegetable seedlings with porous connected potholes as containers and artificial mixing substrates instead of soil often results in leggy seedlings. The hypocotyl length or hypocotyl diameter of leggy seedlings significantly increases or decreases the ratio of plant height to hypocotyl diameter increase. In addition, leggy seedlings display lower chlorophyll contents and elongated and weak hypocotyls which greatly influence their subsequent field performance (Zhou et al., 2019). Therefore, the control of hypocotyl overgrowth is a crucial part of the seedling stage.
Plant hormones significantly regulate plant height. Reducing bioactive gibberellins (GAs) has been widely employed for controlling stem elongation in various plants (Yan et al., 2021)—for example, AtGA2ox7 or AtGA2ox8 overexpression leads to a dwarf phenotype in both tobacco (Nicotiana tabacum) and Arabidopsis (Schomburg et al., 2003). Overexpressing PcGA2ox1 of transgenic Solanum nigrum and Solanum melanocerasum from a runner bean resulted in a dwarf phenotype (Dijkstra et al., 2008). The overexpressing OsGA2ox5 of rice leads to a dwarf plant with a shorter stem (Shan et al., 2014). BnGA2ox2 and BnGA2ox6 overexpression in Arabidopsis inhibits hypocotyl and stem growth (Yan et al., 2017; Yan et al., 2021). Moreover, biosynthesis inhibitor brassinazole of brassinosteroid (BR) restrains tomato hypocotyl growth through inhibiting the canonical cell cycle in the longitudinal direction, which is related to downregulating the expression of cell cycle genes (Song et al., 2022). Melatonin is drop by knocking out the pivotal enzyme gene such as the serotonin N-acetyltransferase 2 and tryptophan decarboxylase that is involved in the melatonin biosynthesis, which result in the DWARF4 expression downregulating and endogenous BR levels decreasing as well as a semi-dwarf phenotype of rice (Lee and Back, 2018). It is reported that mutants with a deficient auxin synthesis exhibit shorter hypocotyl length, whereas mutants with an excessive auxin synthesis in the light display a longer hypocotyl length (Cheng et al., 2006). These previous investigations reveal that the growth and development of hypocotyls essentially need an appropriate concentration of auxin, and auxin can successfully affect hypocotyl length by promoting IAA3 degradation, which in turn results in the transcription factor of ARF being released (Vernoux et al., 2011; Oh et al., 2014). It can be seen that previous investigations have mainly conducted to explore the molecular mechanism of endogenous growth hormones on seedling dwarfing, but few studies have been focused on probing the molecular regulatory mechanism of exogenous regulators on hypocotyl dwarfing.
The plant growth regulators of triazole, such as uniconazole and paclobutrazol, can shorten internodes and dwarf plants (Tesfahun and Menzir, 2018). Uniconazole is a triazole compound that has similar structural and biological effects to paclobutrazol, and it is four to 10 times more active than paclobutrazol in controlling plant height (Blanchard and Runkle, 2007; Carver et al., 2014). Moreover, it has a shorter degradation cycle and less application dosage, and it is more environmentally friendly (Tao et al., 1997). Previous reports showed that uniconazole is widely applied to control plant height and enhance plant lodging resistance (Ahmad et al., 2021a; Lv et al., 2022). To date, uniconazole treatment in the process of intensive vegetable seedling cultivation mostly focuses on the screening of the application concentration (Zuo et al., 2020), and there is little information about its molecular regulatory mechanism. The key pathway of uniconazole on plant dwarfing remains largely unknown.
Basic leucine zipper (bZIP), which is widely existing in plants, is a family of transcription factors. It is involved in the growth and development of many plants and in response processes to biotic and abiotic stresses (Hossain et al., 2010; Tak and Mhatre, 2013). CAbZIP1 is involved in plant development and function as a possible regulator in increased disease resistance and environment stress tolerance (Lee et al., 2006). Fukazawa et al. (2000) indicated that bZIP regulates the morphology of plants by controlling the endogenous contents of GAs. Van Leene et al. (2016) showed that bZIP29 is involved in the leaf and root development of Arabidopsis. ZmbZIP4 can enhance root development in maize (Ma et al., 2018). Moreover, Weiste et al. (2017) demonstrated that AtbZIP11 can efficiently inhibit the expression of auxin-transport-promoting gene family PIN-FORMED, resulting in root growth retardation. bZIP39, a member of the A subgroup of the bZIP family, is extensively involved in many development processes in plants (Dröge-Laser et al., 2018)—for example, bZIP39 is extensively characterized concerning its function of ABA-dependent maturation and germination of seed (Skubacz et al., 2016) and takes part in floral transition regulation of plant by directly influencing FLC transcription, which suppresses the florigen gene FT (Shu et al., 2016; Shu et al., 2018). Furthermore, bZIP39 can also directly control genes linked with abiotic stress responses (Finkelstein and Lynch, 2000). Nevertheless, the available investigations on the molecular regulation mechanism of hypocotyl dwarfing in crops under the impact of bZIP are limited.
Flowering Chinese cabbage (Brassica campestris L. ssp. chinensis var. utilis Tsen et Lee) belongs to the Brassica, which is a famous, annually produced cruciferous vegetable widely distributed in China (Wang et al., 2022; Yue et al., 2022). In recent years, the production scale of flowering Chinese cabbage has been expanding, and its cultivation mode has changed from conventional direct sowing to intensive seedling transplantation; however, during the process of intensive seedling transplantation, leggy seedlings easily occur due to the overgrowth of hypocotyl, which leads to the decline of seedling quality. Thus, how to control the excessive growth of hypocotyl is an urgent problem to be solved in intensive seedling transplantation of flowering Chinese cabbage. So far, there has been little research on the integration of transcriptomic and metabolomic data to investigate the mechanisms of hypocotyl elongation. The molecular regulatory mechanism of uniconazole involved in regulating the overgrowth of hypocotyl in flowering Chinese cabbage has not been explored. We aimed to identify which genes and molecular pathways are responsive to hypocotyl dwarfing of seedlings mediated by uniconazole. By transcriptomic and metabolomic analyses, yeast one-hybrid, dual-luciferase, and virus-induced gene silencing (VIGS) assay, we revealed that uniconazole could induce hypocotyl dwarfing through inhibiting lignin biosynthesis in flowering Chinese cabbage seedlings. Our findings provide a novel insight into the molecular regulatory mechanism of uniconazole involved in inhibiting hypocotyl elongation through repressing the BrbZIP39–BrPAL4 module-mediated phenylpropanoid biosynthesis. These results might have important practical implications for improving commercial seedling quality and greatly assist in guaranteeing the yield and quality of vegetables.
The cultivar ‘Sijiu-19’ of flowering Chinese cabbage was used in the present study. The seeds were sowed using substrate plugs. After sowing, the seedling plugs were placed in artificial climatic chambers (RXZ-500D, Ningbo Jiangnan Instrument Factory, China) with a light intensity of 3,000 lx, relative humidity of 85%, and day (16 h)/night (8 h) temperature of 28°C/20°C. Five uniconazole concentrations of 0 mg·L-1 (pure water, CK), 25 mg·L-1 (T1), 50 mg·L-1 (T2), 100 mg·L-1 (T3), and 200 mg·L-1 (T4) were applied in the experiment. The different concentrations of uniconazole were sprayed into each seedling plug at 48 h after sowing, respectively, and the application dosage of each concentration was 100 ml per replicate. All treatments were carried out with three biological replicates. The length and diameter of the hypocotyls were measured by a vernier caliper at 9 days after treatment.
The hypocotyls were collected and fixed in formaldehyde–acetic acid–ethanol fixative. The tissue section method was as described in Zhang et al. (2020). Observation was conducted using a light microscope after making the sections (10 μm).
The lignin content of hypocotyls was determined using a plant lignin ultraviolet spectrophotometer kit (Solarbio, Beijing, China). Briefly, the hypocotyl samples were dried at 80°C and then ground to powder. Moreover, 10 mg of the samples was placed into test tubes, and then 1 ml acetyl bromide solution (25% v/v acetyl bromide in glacial acetic acid) and 40 µl perchloric acid were added, fully mixed, and heated for 40 min at 80°C with vortexing every 10 min. After cooling (room temperature), 1 ml of a solution of glacial acetic acid and sodium hydroxide was added to end the reaction. Finally, after centrifugation at 8,000 g for 10 min, 20 µl of supernatant was mixed with 1.98 ml glacial acetic acid, and 1 ml liquid was added to a quartz cuvette to determine the absorbance using an ultraviolet spectrophotometer (Shimadzu Corp., Kyoto, Japan) at 280 nm. The results were expressed on dry weight basis as mg cm-1.
The transcriptome and metabolome analyses of the hypocotyls in flowering Chinese cabbage treated with uniconazole were performed according to our previous report (Yue et al., 2022) based on the MetWare database (Metware Biotechnology Co., Ltd., Wuhan, China). The value of variable importance in the project (VIP) was calculated by orthogonal partial least square discriminant analysis. Differentially accumulated metabolites (DAMs) between the two groups were determined by VIP ≥1 and absolute log2(fold change, FC) ≥1. The differential expression analysis was consistent with our previous report (Yue et al., 2022). Differentially expressed genes (DEGs) between groups were determined by P ≤0.05 and absolute log2(FC) ≥1. The joint analysis was carried out to analyze the biological changes of the hypocotyls in flowering Chinese cabbage treated with uniconazole. DEGs and DAMs were mapped to the Kyoto Encyclopedia of Genes and Genomes (KEGG, https://www.kegg.jp/) pathway, and enrichment analysis was performed.
The BrbZIP39 sequence was ligated into the pGADT7 vector using the BamHι and EcoRI restriction sites. The BrPAL4 promoter (1,899 bp upstream of the predicted translation start site) fragment was cloned into the pAbAi vector using the SacI and XhoI restriction sites. They were then transferred into the cell of yeast strain Y1H Gold (TransGen, Beijing, China) through Yeastmaker™ Yeast Transformation System 2. Strains introduced with plasmids of pGADT7 and/or p53 served as negative and positive controls, respectively. The transformants were grown on SD/-Leu media and incubated at 30°C for 5 days.
Full-length ORFs of BrbZIP39 were inserted into pGreenll 62-SK to generate the effector. In addition, the promoter fragment of BrPAL4 was fused into pGreenll 0800-luciferase reporter (LUC) to obtain the reporter. The effector and reporter vectors were co-transformed into tobacco (Nicotiana benthamiana) leaves.
The vectors of pTRV1 and pTRV2 based on the tobacco rattle virus (TRV) were employed to silence the BrbZIP39. A 300-bp fragment of BrbZIP39 was amplified and cloned into pTRV2 to generate pTRV2–BrbZIP39. Then, pTRV1, pTRV2, and pTRV2–BrbZIP39 were transformed into GV3101, respectively. The GV3101 strains with pTRV2–BrbZIP39 and pTRV1 were syringe-infiltrated into flowering Chinese cabbage which was designated as pTRV : BrbZIP39. A mixture of pTRV1 and pTRV2 empty was used as control (pTRV:00).
Total RNA was extracted from the samples using a plant RNA extraction kit (Magen, Guangzhou, China) according to the manufacturer’s instructions. qRT-PCR was performed using the Q711-ChamQTM Universal SYBR® qPCR Master Mix (Vazyme, Nanjing, China) with Bio-Rad CFX96 Real-Time PCR System. The qRT-PCR reaction conditions were described as follows: 30 s at 95°C, 40 cycles of 10 s at 95°C, and 30 s at 60°C. The transcript abundance of 12 randomly selected DEGs was calculated according to the 2−△△Ct method. BrGAPDH was used as the internal control. All primers used herein are listed in Supplementary Table S3.
All data were derived from three biological replicates and expressed as mean ± SE. R v4.1.1 and IBM SPSS Statistics 24 (SPSS Inc., Chicago, IL, USA) were used for statistical analysis. Tukey’s test (P < 0.05) was carried out to evaluate the treatment effects. All figures were generated using R v4.1.1 and Origin 2021 (OriginLab Corp., Northampton, MA, USA).
Uniconazole is widely applied to control plant height and enhance plant lodging resistance (Ahmad et al., 2021a; Lv et al., 2022). In this study, uniconazole treatment remarkably inhibited hypocotyl elongation and increased the hypocotyl diameter of flowering Chinese cabbage seedlings (Figure 1A). Compared with CK, the hypocotyl length of T1, T2, T3, and T4 treatments significantly decreased by 12.65%, 30.18%, 43.08%, and 46.67%, and the hypocotyl diameter of T1, T2, T3, and T4 treatments significantly increased by 4.36%, 10.07%, 13.76%, and 16.11%, respectively. Additionally, there was no significant difference in hypocotyl length and diameter between the T3 and T4 treatments (Figures 1B, C). These results showed that, with the increase of uniconazole concentration in the range of 0–100 mg·L−1, the hypocotyl length became shorter obviously, but there was no significant difference at the level of 100–200 mg·L−1. Based on the above-mentioned results, the CK and T3 (100 mg·L−1 uniconazole) treatments were selected for histological observation, metabolome detection, and transcriptome sequencing.
Figure 1 The phenotype and hypocotyl growth of flowering Chinese cabbage seedlings under uniconazole treatment. The phenotype (A), length (B), and diameter (C) of hypocotyl at 9 days after uniconazole treatment in flowering Chinese cabbage seedlings. CK, 0 mg·L-1 uniconazole (pure water); T1, 25 mg·L-1 uniconazole; T2, 50 mg·L-1 uniconazole; T3, 100 mg·L-1 uniconazole; T4, 200 mg·L-1 uniconazole. The vertical bars show the standard error of the mean (n = 10). Red bar, 2 cm. Letters above columns mean significant difference at P <0.05.
To further explore the cause of hypocotyl dwarfing induced by uniconazole, the hypocotyl was dissected at 9 days after treatment. We observed that the shape of the parenchyma cells changed significantly after uniconazole treatment (Figures 2A, B). The average cell width of hypocotyls was 17.93 and 21.63 μm, respectively, in uniconazole treatment and CK, which was about 1.2-folds wider than in uniconazole treatment (Figure 2C). The average cell length of hypocotyls was 60.65 μm in CK, while that in uniconazole treatment was only 17.29 μm (Figure 2D). That was to say, the cell morphology of the hypocotyl has changed dramatically after uniconazole treatment, which implied that the hypocotyl dwarfing of flowering Chinese cabbage seedlings may be related to the cell size.
Figure 2 Longitudinal section and cell size of hypocotyl under uniconazole in flowering Chinese cabbage seedlings. (A) Parenchyma cells of CK. (B) Parenchyma cells of DWA. (C) Cell length. (D) Cell width. CK, 0 mg L-1 uniconazole (pure water); DWA, 100 mg L-1 uniconazole. The vertical bars show the standard error of the mean (n = 10). Letters above columns mean significant difference at P <0.01.
To profile the metabolic changes of hypocotyl dwarfing induced by uniconazole, we performed non-targeted metabolome detection using a UPLC-ESI-MS/MS system. We identified and annotated 467 and 479 metabolites in the tested samples. The quality of the metabolome data was good, showing high intra-group correlations (Figure 3A) and a clear separation trend in the principal component analysis (Figure 3B).
Figure 3 Metabolome analysis of flowering Chinese cabbage under uniconazole treatment. (A) Correlation profile between the samples. (B) Principal component analysis of metabolome samples. (C) Volcano map of DAMs. (D) Kyoto Encyclopedia of Genes and Genomes pathway enrichment of DAMs. DAMs, differentially accumulated metabolites; CK, 0 mg·L-1 uniconazole (pure water); DWA, 100 mg·L-1 uniconazole.
The results revealed that the metabolites in hypocotyls under uniconazole treatment were remarkably changed. The 201 DAMs including 94 downregulated and 107 upregulated metabolites were filtered out through setting a threshold value of absolute log2(FC) ≥1 and VIP ≥1 (Figure 3C). The KEGG enrichment analysis indicated that three flavonoid-related pathways including “flavonoid biosynthesis”, “flavone and flavonol biosynthesis”, and “isoflavonoid biosynthesis” were significantly overrepresented in DAMs (Figure 3D). Among the enriched DAMs, eight were associated with “flavonoid biosynthesis” pathway, seven were associated with “flavone and flavonol biosynthesis” pathway, four were associated with the “isoflavone biosynthesis” pathway, and six were associated with “phenylpropanoid biosynthesis” pathway (Supplementary Figure S1). Amid the top 10 DAMs, the quantities of flavonoids were all downregulated except qercetin-3-O-xyloside, and nine of the top 10 downregulated DAMs were flavonoids (Supplementary Figure S2). Considering that flavonoids are secondary metabolites derived from the pathway of phenylpropanoid, we speculated that the phenylpropanoid pathway may play a critical role in the hypocotyl dwarfing of flowering Chinese cabbage seedlings under uniconazole treatment.
The transcriptomes of the seedling hypocotyls from the CK and uniconazole treatment groups were applied by Illumina sequencing. A total of 41.48 Gb clean data was generated from the six samples with Q30 values being all higher than 93% (shown in Supplementary Table S1). The clean reads data were compared with the flowering Chinese cabbage genome (unpublished) by hisat2 v2.1.0; a total of 42,491 unigenes were obtained. Based on transcriptome annotation and functional classification, 97.53% (41,441) unigenes were assigned putative functional annotation (Supplementary Figure S3). Gene Ontology analysis showed that “transcription, DNA-templated” and “regulation of transcription, DNA-templated” containing 3,232 (11.48%) and 1,881 (6.68%) unigenes, respectively, were the two largest sub-categories in the biological process. The “nucleus” and “integral component of membrane”, which accounted for 6,771 (24.06%) and 6,611 (23.49%) unigenes, respectively, were the two largest sub-categories in the cellular component. “ATP binding” and “metal ion binding” contained 3,698 (13.14%) and 3,073 (10.92%) unigenes, respectively, and were the two sub-categories in the molecular function cluster (Supplementary Figure S4).
The unigene expression levels were described as fragments per kilobase of transcript per million fragments mapped values. The calculation of the correlations between every two samples demonstrated high intra-group correlations (Figure 4A), and the principal component analysis indicated a clear separation trend (Figure 4B). The 3,449 DEGs including 2,246 downregulated and 1,203 upregulated unigenes were filtered out by setting a threshold of P-adj <0.05 and absolute log2 (FC) ≥1 (Figure 4C). The KEGG enrichment analysis revealed that two pathways—”plant hormone signal transduction” and “phenylpropanoid biosynthesis”—were significantly overrepresented in DEGs (Figure 4D).
Figure 4 Transcriptome analysis of flowering Chinese cabbage under uniconazole treatment. (A) Correlation heat map between the samples (based on fragments per kilobase of transcript per million fragments mapped). (B) Principal component analysis of transcriptome samples. (C) Volcano map of DEGs. (D) Kyoto Encyclopedia of Genes and Genomes pathway enrichment of DEGs. DEGs, differentially expressed genes; CK, 0 mg·L-1 uniconazole (pure water); DWA, 100 mg·L-1 uniconazole.
To confirm the relationship between metabolites and genes, we conducted a joint analysis of metabolome and transcriptome. The KEGG pathway enrichment analysis showed 33 co-enriched KEGG pathways of DEGs and DAMs (Figure 5A). Among them, “phenylpropanoid biosynthesis” pathway is the most significant enrichment pathway; “carbon fixation in photosynthetic organisms” pathway, “flavonoid biosynthesis” pathway, “pentose and glucuronate interconversions” pathway, “tryptophan metabolism” pathway, and “starch and sucrose metabolism” pathway are also significantly enriched. The clustered heat map (Figure 5B) revealed that the DAMs associated with DEGs were classified into several categories, with flavonoids as the largest category, which indicated that “phenylpropanoid biosynthesis” pathway plays a crucial role in hypocotyl dwarfing.
Figure 5 Conjoint analysis transcriptome and metabolome. (A) Kyoto Encyclopedia of Genes and Genomes pathway enrichment of DEGs and DAMs. (B) Clustered heat map of DEGs and DAMs. DAMs, differentially accumulated metabolites; DEGs, differentially expressed genes.
Based on the omics analysis, we speculated that hypocotyl dwarfing of flowering Chinese cabbage seedlings caused by uniconazole was associated with lignin biosynthesis. To confirm the hypothesis, we determined the lignin content of hypocotyl, and the results demonstrated that the lignin content of the hypocotyl in uniconazole treatment was remarkably decreased by 50.15% compared with CK (Figure 6A). Surprisingly, we found only one member (Br00009249) in BrPALs among DEGs, which was significantly downregulated after uniconazole treatment (Figures 6B, C). We applied the protein sequence of Br00009249 as the query to BLASTP against the Arabidopsis genome database; it was named BrPAL4. Moreover, uniconazole treatment also downregulated most of the BrbZIPs family genes, including BrbZIP39 (Figures 6D, E). These findings showed that uniconazole inhibiting hypocotyl elongation was associated with lignin content, BrPAL4, and BrbZIP39 expression.
Figure 6 Effects of uniconazole on lignin content, BrPALs and BrbZIPs expression of hypocotyls in flowering Chinese cabbage. Lignin content (A), fragments per kilobase of transcript per million fragments mapped (FPKM) of BrPALs in DEGs (B), relative expression of BrPAL4 (C), FPKM of BrbZIPs in DEGs (D), and relative expression of BrbZIP39 (E) in hypocotyl of flowering Chinese cabbage seedlings under CK and DWA. The vertical bars show the standard error of the mean (n = 3). CK, 0 mg·L-1 uniconazole (pure water); DWA, 100 mg·L-1 uniconazole. Letters above columns mean significant difference at P <0.05.
To identify the regulatory relationship between BrbZIP39 and BrPAL4, we further analyzed the cis-acting elements of BrPAL4. The promoter region of BrPAL4 (upstream 2,000 bp) contained nine ABRE and four G-box that could be bound by bZIP39 (Supplementary Table S2). Therefore, we inferred that uniconazole probably regulated BrPAL4 through mediating BrbZIP39 expression, which might lead to hypocotyl dwarfing of flowering Chinese cabbage seedlings.
To verify whether BrbZIP39 has a regulatory effect on BrPAL4, we performed Y1H and LUC experiments. The results displayed that the Y1H Gold yeast transformed with pGADT7-BrbZIP39 in deficient media SD/-Leu grew normally, the negative control yeast transformed with pGADT7 did not grow, and the positive control yeast transformed with p53 grew normally (Figure 7A). Additionally, compared with the control that was cotransfected with the empty pSK vector, the relative luciferase activity of pSK-BrbZIP39 increased significantly (Figure 7B). The above-mentioned findings demonstrated that BrbZIP39 could directly bind to the promoter region of BrPAL4 and activate its transcript, indicating that BrbZIP39 is the transcriptional activator of BrPAL4.
Figure 7 BrbZIP39 could activate BrPAL4 expression. (A) Yeast one-hybrid analysis showed that BrbZIP39 could bind to the promoter of BrPAL4. (B) Dual-luciferase assay indicated that BrbZIP39 was the activator of BrPAL4.
To unravel whether BrbZIP39 is required for hypocotyl dwarfing, the VIGS system was employed to silence BrbZIP39 in flowering Chinese cabbage. The results displayed that BrbZIP39 expression in the hypocotyl was significantly decreased after silencing BrbZIP39, and the BrbZIP39 transcript abundance in TRV : BrbZIP39 plants was remarkably reduced by 62.33% compared with that of TRV:00 (Figure 8A). Meanwhile, compared with TRV:00, the hypocotyl length of TRV : BrbZIP39 was significantly decreased by 44.78% (Figures 8B, C), and the hypocotyl diameter of TRV : BrbZIP39 was significantly increased by 42.42% (Figures 8B, D). Furthermore, the lignin content and the expression of BrPAL4 in the hypocotyl were significantly decreased in TRV : BrbZIP39 plants (Figures 8E, F). These results further confirmed that uniconazole decreased the lignin content through repressing BrbZIP39–BrPAL4 module-mediated phenylpropanoid biosynthesis, which resulted in the hypocotyl dwarfing of flowering Chinese cabbage seedlings.
Figure 8 Silencing of BrbZIP39 in flowering Chinese cabbage by virus-induced gene silencing. The phenotype (A), relative expression of BrbZIP39 (B), hypocotyl length (C), hypocotyl diameter (D), relative expression of BrPAL4 (E), and lignin content (F) of TRV:00 and TRV : BrbZIP39 plants in flowering Chinese cabbage. The vertical bars show the standard error of the mean (n = 6). Red bar, 2 cm. Letters above columns mean significant difference at P < 0.05.
To verify the results obtained in the RNA-seq experiments, the expressions of 12 DEGs were analyzed using qRT-PCR. The transcriptome sequencing and bioinformatics analyses showed that Br00009249, Br00020065, Br00036384, Br00043806, Br00055757, Br00058057, and Br00062465 were downregulated, while Br00030167, Br00052005, Br00066177, Br00066395, and Br00067601 were upregulated under uniconazole treatment. The qRT-PCR results revealed that the expression patterns of these representative genes were in line with the RNA-seq data (Supplementary Figure S5A). To evaluate RNA-seq data, linear regression analysis was performed, and he results showed that the data from qRT-PCR and RNA-seq of the 12 DEGs were obviously correlated (R2 = 0.66008, P < 0.001, Supplementary Figure S5B), implying the reliability of the RNA-Seq data.
The hypocotyl length, closely associated with the biomass and the yield of plants, is an important component of plant architecture (Zhang et al., 2019). Factory-raised seedlings, at present, are widely applied in vegetable production; however, the conditions of growth in factories—including low light, high humidity, and high temperature—result in the excessive growth of hypocotyls during seedling cultivation and obviously affect the survival rate of vegetable seedlings (Gomez and Mitchell, 2014; Song et al., 2022). Uniconazole, a plant growth regulator, can effectively decrease plant height, increase root–shoot ratio and stem diameter, and enhance lodging resistance (Currey et al., 2016; Ahmad et al., 2020; Zuo et al., 2020). In our present study, uniconazole treatment significantly decreased plant height and hypocotyl length but enhanced hypocotyl diameter, and the level of increase or decrease was correlated with the applied concentration in a certain range (0–100 mg·L-1) in flowering Chinese cabbage (Figures 1A–C). These results suggested that uniconazole could effectively control hypocotyl overgrowth and be used in the cultivation of strong seedlings of flowering Chinese cabbage. In addition, previous studies indicated that BR-deficient or BR-insensitive mutants displayed a dwarf phenotype of the plant due to shortened cell length (Gupta et al., 2021). In this study, the histological observation showed that cell length was obviously shortened under uniconazole treatment (Figure 2C), which was similar to previous studies (Kato et al., 2011; Lv et al., 2022). This finding indicated that shortened cells might be a response to the decrease of hypocotyl length, and the enlargement of parenchyma cells might be a response to the increase of hypocotyl diameter (Supplementary Figure S6).
Although uniconazole has a wide application in crop production, the molecular mechanism about inhibiting hypocotyl elongation mediated by uniconazole remains largely unknown. To investigate the molecular regulatory mechanism of uniconazole involved in inhibiting hypocotyl elongation, we conducted transcriptomic and metabolomic analyses. The dataset provided a useful resource for the clarification of the key dwarfing pathways not yet deciphered in seedling hypocotyl under uniconazole treatment in flowering Chinese cabbage. Our investigation indicated that a total of 3,499 DEGs and 201 DAMs were affected by uniconazole (Figures 4C, 6C), and the KEGG pathway analysis demonstrated that most DEGs were remarkably enriched in the pathway of “plant hormone signal transduction” (Figure 6D). These findings also explain why the previous research on dwarf phenotype mainly focused on endogenous hormone changes in plants (Liu et al., 2015; Ahmad et al., 2021b; Zhou et al., 2021). Interestingly, in this study, “phenylpropanoid biosynthesis” pathway, which is involved in hypocotyl dwarfing of flowering Chinese cabbage, was proposed for the first time by combined metabolome and transcriptome analyses. The joint metabolome and transcriptome analyses revealed that the DEGs were significantly enriched in “phenylpropanoid biosynthesis” pathway, while the DAMs were significantly enriched in “flavonoid biosynthesis” pathway. Considering that flavonoids are secondary metabolites derived from the pathway “phenylpropanoid biosynthesis”, we thus speculated that “phenylpropanoid biosynthesis” pathway plays a vital role in hypocotyl dwarfing induced by uniconazole in flowering Chinese cabbage seedlings.
Previous studies have also pointed out that there is a close relationship between plant dwarfing and lignin, which is an important phenylpropanoid. Li et al. (2010) have indicated that the dwarf phenotype results instead from disruptions in lignin biosynthesis. The dwarf mutant with cinnamoyl-CoA reductase deficiency has significantly less lignin, while the cellulose and hemicellulose levels are unchanged, implying that plant dwarfing is related to lignin (Goujon et al., 2003). The mutation of UGT72B1 results in ectopic lignification and lignin biosynthesis, which is responsible for the glycosylation of monolignols that leads to growth suppression (Lin et al., 2016). The lac4, lac11, and lac17 triple mutation in Arabidopsis lacks lignification in the vascular tissues, which represses plant growth (Zhao et al., 2013). The irregular xylem4 mutants accounted for about 50% less lignin content than the wild type, have collapsed xylem, and are seriously dwarfed (Jones et al., 2001). The overexpression of R2R3-MYB transcription factor ZmMYB31 also shows a dwarf phenotype, while the lignin content is significantly decreased in Arabidopsis thaliana (Fornalé et al., 2010). Our results displayed that the lignin level in the hypocotyl of the dwarf phenotype was significantly decreased in flowering Chinese cabbage seedlings (Figures 3A, B). This finding further confirmed that the inhibition of hypocotyl elongation caused by uniconazole was related to the phenylpropanoid biosynthesis pathway. However, how does uniconazole cause hypocotyl dwarfing by inhibiting the formation of lignin? The knowledge in this area is still limited.
Phenylalanine ammonia-lyase (PAL) is a portal enzyme in the pathway of phenylpropanoid biosynthesis (Barros et al., 2016). PALs family contains multiple family members; however, it has not identified which members are the pivotal regulators in inducing hypocotyl dwarfing of flowering Chinese cabbage seedlings mediated by uniconazole. Different PAL gene members have different functions in plants—for example, PAL1 and PAL2 have functionally participated in flavonoid synthesis; PAL4 may be a good candidate for playing an important role in lignin synthesis (Olsen et al., 2008). Previous reports found that pal1 pal2 mutant reduces lignin content, with deficiency in anthocyanin and tannin biosynthesis (Rohde et al., 2004; Huang et al., 2010). Furthermore, Rohde et al. (2004) also noted that mutations in each of PAL1 and PAL2 alone do not exhibit a noticeable dwarfing phenotype. In this study, we found that BrPAL4 was downregulated in the hypocotyl of the dwarf phenotype caused by uniconazole, accompanied by a decrease of lignin content (Figure 6). Our results further demonstrated that “phenylpropanoid biosynthesis” pathway played an important role in the hypocotyl dwarfing of flowering Chinese cabbage seedlings, while the reduction of lignin content was a reason for the hypocotyl dwarfing induced by uniconazole.
bZIP transcription factors are widely existing in eukaryotes and involve in the growth and development of many plants (Fukazawa et al., 2000). HY5, a variety of the bZIP transcription factor family, plays a vital role in hypocotyl elongation in plants (Oyama et al., 1997). Li et al. (2011) showed that HY5 can mediate AtERF11 to inhibit ethylene biosynthesis in Arabidopsis, thereby affecting seedling growth and development. It has been reported that bZIP30 overexpression induces a dwarf phenotype and affects reproductive development in Arabidopsis, while loss-of-function mutants for bZIP30 and its closest homolog have more floral buds and longer fruits (Lozano-Sotomayor et al., 2016). Overexpression of bZIP53 transgenic Arabidopsis also shows a dwarf phenotype associated with reduced seed production and an elevated gene expression of seed maturation genes (Alonso et al., 2009; Garg et al., 2019). Taken together, the bZIP transcription factors play a vital role in the growth and development of plants. Our results also demonstrated that uniconazole could downregulate the expression of BrbZIP39, indicating that BrbZIP39 is involved in the regulation of hypocotyl dwarfing in flowering Chinese cabbage. However, to date, the previous investigation focused mainly on bZIP transcription factors and the effects of photomorphogenesis and reproductive development in plants; there were few reports on the effects of “phenylpropanoid biosynthesis” pathway which is a key pathway between the primary and secondary metabolic pathways. Moreover, it is still unclear whether bZIP transcription factors can regulate hypocotyl dwarfing by mediating PAL expression and lignin biosynthesis. To determine whether BrbZIP39 could transcriptionally activate BrPAL4, we performed the yeast one-hybrid and dual-luciferase assays. Our results exhibited that BrbZIP39 could directly bind to the promoter region of BrPAL4 and activate its transcription (Figure 7). To further verify whether BrbZIP39 could dwarf the hypocotyl of flowering Chinese cabbage seedlings by reducing the content of lignin in the hypocotyl, we silenced BrbZIP39 using the VIGS system. We found that positive plants had shorter hypocotyls and that there was less lignin content in the hypocotyl of flowering Chinese cabbage seedlings (Figure 8). These results confirmed that BrbZIP39 was related to the dwarfing of hypocotyl. Hence, we further proved that the inhibition of BrbZIP39–BrPAL4 decreased the content of lignin in the hypocotyl, which was a reason for hypocotyl dwarfing mediated by uniconazole. Taken together, our results propose that uniconazole can decrease lignin content via repressing BrbZIP39–BrPAL4 module-mediated phenylpropanoid biosynthesis, which leads to hypocotyl dwarfing of flowering Chinese cabbage seedlings. Our present findings provide a new insight into the molecular regulatory mechanism and enrich our understanding of inhibiting hypocotyl elongation in flowering Chinese cabbage.
The datasets presented in this study can be found in online repositories. The names of the repository/repositories and accession number(s) can be found below: https://www.ncbi.nlm.nih.gov/, PRJNA855288.
XY designed the research. LZ, MZ, and LY performed the experiments and wrote the paper. XC and PZ analyzed the data. YK and MZ reviewed the manuscript. All authors contributed to the article and approved the submitted version.
This work was financially supported by Guangdong Provincial Special Fund for Modern Agriculture Industry Technology Innovation Teams (numbers 2022KJ122 and 2023KJ122), Natural Science Foundation of Guangdong Province (number 2018A0303130316), and Key-Area Research and Development Program of Guangdong Province (number 2018B020202010).
The authors declare that the research was conducted in the absence of any commercial or financial relationships that could be construed as a potential conflict of interest.
All claims expressed in this article are solely those of the authors and do not necessarily represent those of their affiliated organizations, or those of the publisher, the editors and the reviewers. Any product that may be evaluated in this article, or claim that may be made by its manufacturer, is not guaranteed or endorsed by the publisher.
The Supplementary Material for this article can be found online at: https://www.frontiersin.org/articles/10.3389/fpls.2022.1014396/full#supplementary-material
Ahmad, I., Ahmad, S., Yang, X. N., Meng, X. P., Yang, B. P., Liu, T., et al. (2021a). Effect of uniconazole and nitrogen level on lodging resistance and yield potential of maize under medium and high plant density. Plant Biol. 23, 485–496. doi: 10.1111/plb.13235
Ahmad, I., Kamran, M., Meng, X. P., Ali, S., Ahmad, S., Gao, Z. Q., et al. (2021b). Hormonal changes with uniconazole trigger canopy apparent photosynthesis and grain filling in wheat crop in a semi-arid climate. Protoplasma 258, 139–150. doi: 10.1007/s00709-020-01559-0
Ahmad, I., Meng, X. P., Kamran, M., Ali, S., Ahmad, S., Liu, T. N., et al. (2020). Effects of uniconazole with or without micronutrient on the lignin biosynthesis, lodging resistance, and winter wheat production in semiarid regions. J. Integr. Agricult. 19, 62–77. doi: 10.1016/S2095-3119(19)62632-8
Alonso, R., Onate-Sánchez, L., Weltmeier, F., Ehlert, A., Diaz, I., Dietrich, K., et al. (2009). A pivotal role of the basic leucine zipper transcription factor bZIP53 in the regulation of arabidopsis seed maturation gene expression based on heterodimerization and protein complex formation. Plant Cell. 21, 1747–1761. doi: 10.1105/tpc.108.062968
Barros, J., Serrani-Yarce, J. C., Chen, F., Baxter, D., Venables, B. J., Dixon, R. A. (2016). Role of bifunctional ammonia-lyase in grass cell wall biosynthesis. Nat. Plants 2, 16050. doi: 10.1038/nplants.2016.50
Blanchard, M. G., Runkle, E. S. (2007). Dipping bedding plant liners in paclobutrazol or uniconazole inhibits subsequent stem extension. Hort. Technol. Hort. 17, 178–182. doi: 10.21273/HORTTECH.17.2.178
Carver, S. T., Arnold, M. A., Byrne, D. H., Armitage, A. R., Daniel Lineberger, R., King, A. R. (2014). Growth and flowering responses of sea marigold to daminozide, paclobutrazol, or uniconazole applied as drenches or sprays. J. Plant Growth Regulation 33, 626–631. doi: 10.1007/s00344-014-9411-7
Cheng, Y. F., Dai, X. H., Zhao, Y. D. (2006). Auxin biosynthesis by the YUCCA flavin monooxygenases controls the formation of floral organs and vascular tissues in arabidopsis. Genes Dev. 20, 1790–1799. doi: 10.1101/gad.1415106
Currey, C. J., Flax, N. J., Walters, K. J. (2016). Foliar sprays of flurprimidol, paclobutrazol, and uniconazole suppress height of seed-propagated new guinea impatiens. Hort. Technol. Hort. 26, 20–25. doi: 10.21273/HORTTECH.26.1.20
Dijkstra, C., Adams, E., Bhattacharya, A., Page, A. F., Anthony, P., Kourmpetli, S., et al. (2008). Over-expression of a gibberellin 2-oxidase gene from Phaseolus coccineus l. enhances gibberellin inactivation and induces dwarfism in Solanum species. Plant Cell Rep. 27, 463–470. doi: 10.1007/s00299-007-0471-z
Dröge-Laser, W., Snoek, B. L., Snel, B., Weiste, C. (2018). The arabidopsis bZIP transcription factor family — an update. Curr. Opin. Plant Biol. 45, 36–49. doi: 10.1016/j.pbi.2018.05.001
Finkelstein, R. R., Lynch, T. J. (2000). The arabidopsis abscisic acid response gene ABI5 encodes a basic leucine zipper transcription factor. Plant Cell. 12, 599–609. doi: 10.1105/tpc.12.4.599
Fornalé, S., Shi, X. H., Chai, C. L., Encina, A., Irar, S., Capellades, M., et al. (2010). ZmMYB31 directly represses maize lignin genes and redirects the phenylpropanoid metabolic flux. Plant J. 64, 633–644. doi: 10.1111/j.1365-313X.2010.04363.x
Fukazawa, J., Sakai, T., Ishida, S., Yamaguchi, I., Kamiya, Y., Takahashi, Y. (2000). REPRESSION OF SHOOT GROWTH, a bZIP transcriptional activator, regulates cell elongation by controlling the level of gibberellins. Plant Cell. 12, 901–915. doi: 10.1105/tpc.12.6.901
Garg, A., Kirchler, T., Fillinger, S., Wanke, F., Stadelhofer, B., Stahl, M., et al. (2019). Targeted manipulation of bZIP53 DNA-binding properties influences arabidopsis metabolism and growth. J. Exp. Bot. 70, 5659–5671. doi: 10.1093/jxb/erz309
Gomez, C., Mitchell, C. A. (2014). Growth responses of greenhouse tomato seedlings to different spectra of supplemental lighting are season-specific in a northern climate. HortScience 49, S273–S273.
Goujon, T., Ferret, V., Mila, I., Pollet, B., Ruel, K., Burlat, V., et al. (2003). Down-regulation of the AtCCR1 gene in Arabidopsis thaliana: Effects on phenotype, lignins and cell wall degradability. Planta 217, 218–228. doi: 10.1007/s00425-003-0987-6
Gupta, A., Hua, L., Lin, G. F., Molnár, I., Doležel, J., Liu, S. Z., et al. (2021). Multiple origins of Indian dwarf wheat by mutations targeting the TREE domain of a GSK3-like kinase for drought tolerance, phosphate uptake, and grain quality. Theor. Appl. Genet. 134, 633–645. doi: 10.1007/s00122-020-03719-5
Hossain, M. A., Cho, J. I., Han, M., Ahn, C. H., Jeon, J. S., An, G., et al. (2010). The ABRE-binding bZIP transcription factor OsABF2 is a positive regulator of abiotic stress and ABA signaling in rice. J. Plant Physiol. 167, 1512–1520. doi: 10.1016/j.jplph.2010.05.008
Huang, J. L., Gu, M., Lai, Z. B., Fan, B. F., Shi, K., Zhou, Y. H., et al. (2010). Functional analysis of the arabidopsis PAL gene family in plant growth, development, and response to environmental stress. Plant Physiol. 153, 1526–1538. doi: 10.1104/pp.110.157370
Jones, L., Ennos, A. R., Turner, S. R. (2001). Cloning and characterization of irregular xylem4 (irx4): a severely lignin-deficient mutant of Arabidopsis. Plant J. 26, 205–216. doi: 10.1046/j.1365-313x.2001.01021.x
Kato, F., Araki, M., Miyazawa, Y., Fujii, N., Takeda, K., Suge, H., et al. (2011). Factors responsible for deep-sowing tolerance in wheat seedlings: Varietal differences in cell proliferation and the coordinated synchronization of epidermal cell expansion and cortical cell division for the gibberellin-mediated elongation of first internodes. Ann. Bot. 108, 439–447. doi: 10.1093/aob/mcr173
Khalaki, M. A., Moameri, M., Lajayer, B. A., Astatkie, T. (2021). Influence of nano-priming on seed germination and plant growth of forage and medicinal plants. Plant Growth Regulation 93, 13–28. doi: 10.1007/s10725-020-00670-9
Lee, K., Back, K. (2018). Melatonin-deficient rice plants show a common semidwarf phenotype either dependent or independent of brassinosteroid biosynthesis. J. Pineal Res. 66, e12537. doi: 10.1111/jpi.12537
Lee, S. C., Choi, H. W., Hwang, I. S., Choi, D. S., Hwang, B. K. (2006). Functional roles of the pepper pathogen-induced bZIP transcription factor, CAbZIP1, in enhanced resistance to pathogen infection and environmental stresses. Planta 224, 1209–1225. doi: 10.1007/s00425-006-0302-4
Li, X., Bonawitz, N. D., Weng, J. K., Chapple, C. (2010). The growth reduction associated with repressed lignin biosynthesis in Arabidopsis thaliana is independent of flavonoids. Plant Cell. 22, 1620–1632. doi: 10.1105/tpc.110.074161
Lin, J. S., Huang, X. X., Li, Q., Cao, Y. P., Bao, Y., Meng, X. F., et al. (2016). UDP-Glycosyltransferase 72B1 catalyzes the glucose conjugation of monolignols and is essential for the normal cell wall lignification in Arabidopsis thaliana. Plant J. 88, 26–42. doi: 10.1111/tpj.13229
Li, J., Terzaghi, W., Gong, Y. Y., Li, C. R., Ling, J. J., Fan, Y. Y., et al. (2020). Modulation of BIN2 kinase activity by HY5 controls hypocotyl elongation in the light. Nat. Commun. 11, 1592. doi: 10.1038/s41467-020-15394-7
Liu, Y., Fang, Y., Huang, M. J., Jin, Y. L., Sun, J. L., Tao, X., et al. (2015). Uniconazole-induced starch accumulation in the bioenergy crop duckweed (Landoltia punctata) I: transcriptome analysis of the effects of uniconazole on chlorophyll and endogenous hormone biosynthesis. Biotechnol. Biofuels 8, 57. doi: 10.1186/s13068-015-0246-7
Li, Z. F., Zhang, L. X., Yu, Y. W., Quan, R. D., Zhang, Z. J., Zhang, H. W., et al. (2011). The ethylene response factor AtERF11 that is transcriptionally modulated by the bZIP transcription factor HY5 is a crucial repressor for ethylene biosynthesis in arabidopsis. Plant J. 68, 88–99. doi: 10.1111/j.1365-313X.2011.04670.x
Lozano-Sotomayor, P., Chávez Montes, R. A., Silvestre-Vañó, M., Herrera-Ubaldo, H., Greco, R., Pablo-Villa, J., et al. (2016). Altered expression of the bZIP transcription factor DRINK ME affects growth and reproductive development in Arabidopsis thaliana. Plant J. 88, 437–451. doi: 10.1111/tpj.13264
Lv, R. J., Zhang, W. J., Xie, X. B., Wang, Q. J., Gao, K. G., Zeng, Y. H., et al. (2022). Foliar application uniconazole enhanced lodging resistance of high-quality indica rice (Oryza sativa l.) by altering anatomical traits, cell structure and endogenous hormones. Field Crops Res. 277, 108425. doi: 10.1016/j.fcr.2021.108425
Ma, H. Z., Liu, C., Li, Z. X., Ran, Q. J., Xie, G. N., Wang, B. M., et al. (2018). ZmbZIP4 contributes to stress resistance in maize by regulating ABA synthesis and root development. Plant Physiol. 178, 753–770. doi: 10.1104/pp.18.00436
Oh, E., Zhu, J. Y., Bai, M. Y., Arenhart, R. A., Sun, Y., Wang, Z. Y. (2014). Cell elongation is regulated through a central circuit of interacting transcription factors in the arabidopsis hypocotyl. ELife 3, e03031. doi: 10.7554/eLife.03031
Olsen, K. M., Lea, U. S., Slimestad, R., Verheul M. and Lillo, C. (2008). Differential expression of four Arabidopsis PAL genes; PAL1 and PAL2 have functional specialization in abiotic environmental-triggered flavonoid synthesis. J. Plant Physiol. 165, 1491–1499. doi: 10.1016/j.jplph.2007.11.005
Oyama, T., Shimura, Y., Okada, K. (1997). The arabidopsis HY5 gene encodes a bZIP protein that regulates stimulus-induced development of root and hypocotyl. Genes Dev. 11, 2983–2995. doi: 10.1101/gad.11.22.2983
Rohde, A., Morreel, K., Ralph, J., Goeminne, G., Hostyn, V., De Rycke, R., et al. (2004). Molecular phenotyping of the pal1 and pal2 mutants of Arabidopsis thaliana reveals far-reaching consequences on phenylpropanoid, amino acid, and carbohydrate metabolism. Plant Cell. 16, 2749–2771. doi: 10.1105/tpc.104.023705
Schomburg, F. M., Bizzell, C. M., Lee, D. J., Zeevaart, J. A. D., Amasino, R. M. (2003). Overexpression of a novel class of gibberellin 2-oxidases decreases gibberellin levels and creates dwarf plants. Plant Cell. 15, 151–163. doi: 10.1105/tpc.005975
Shan, C., Mei, Z. L., Duan, J. L., Chen, H. Y., Feng, H. F., Cai, W. M. (2014). OsGA2ox5, a gibberellin metabolism enzyme, is involved in plant growth, the root gravity response and salt stress. PloS One 9, e87110. doi: 10.1371/journal.pone.0087110
Shu, K., Chen, Q., Wu, Y. R., Liu, R. J., Zhang, H. W., Wang, S. F., et al. (2016). ABSCISIC ACID-INSENSITIVE 4 negatively regulates flowering through directly promoting arabidopsis FLOWERING LOCUS c transcription. J. Exp. Bot. 67, 195–205. doi: 10.1093/jxb/erv459
Shu, K., Luo, X. F., Meng, Y. J., Yang, W. Y. (2018). Toward a molecular understanding of abscisic acid actions in floral transition. Plant Cell Physiol. 59, 215–221. doi: 10.1093/pcp/pcy007
Skubacz, A., Daszkowska-Golec, A., Szarejko, I. (2016). The role and regulation of ABI5 (ABA-insensitive 5) in plant development, abiotic stress responses and phytohormone crosstalk. Front. Plant Sci. 7, 1884. doi: 10.3389/fpls.2016.01884
Song, S. Y., Liu, G. Z., Ma, F. F., Bao, Z. L. (2022). Brassinazole represses tomato hypocotyl elongation via inhibition of cell division. Plant Growth Regulation 96, 463–472. doi: 10.1007/s10725-022-00798-w
Tak, H., Mhatre, M. (2013). Cloning and molecular characterization of a putative bZIP transcription factor VvbZIP23 from Vitis vinifera. Protoplasma 250, 333–345. doi: 10.1007/s00709-012-0417-3
Tao, L. X., Wang, X., Yu, M. Y., Huang, X. L., Xu, R. S. (1997). Effects of environmental conditions on degradation of s-07 (uniconazole, pentefezole) and PP333 (MET, paclobutrazol) in soil. Acta Agricult. Zhejiangensis 9, 246–250.
Tesfahun, W., Menzir, A. (2018). Effect of rates and time of paclobutrazol application on growth, lodging, yield and yield components of tef [Eragrostis tef (Zucc.) trotter] in ada woreda, East shewa, Ethiopia. J. Biol. Agric. Healthc. 8, 104–117.
Van Leene, J., Blomme, J., Kulkarni, S. R., Cannoot, B., De Winne, N., Eeckhout, D., et al. (2016). Functional characterization of the arabidopsis transcription factor bZIP29 reveals its role in leaf and root development. J. Exp. Bot. 67, 5825–5840. doi: 10.1093/jxb/erw347
Vernoux, T., Brunoud, G., Farcot, E., Morin, V., Van den Daele, H., Legrand, J., et al. (2011). The auxin signalling network translates dynamic input into robust patterning at the shoot apex. Mol. Syst. Biol. 7, 508. doi: 10.1038/msb.2011.39
Wang, Y. P., Kang, Y. Y., Zhong, M., Zhang, L., Chai, X. R., Jiang, X. X., et al. (2022). Effects of iron deficiency stress on plant growth and quality in flowering Chinese cabbage and its adaptive response. Agronomy 12, 875. doi: 10.3390/agronomy12040875
Weiste, C., Pedrotti, L., Selvanayagam, J., Muralidhara, P., Fröschel, C., Novák, O., et al. (2017). The arabidopsis bZIP11 transcription factor links low-energy signalling to auxin-mediated control of primary root growth. PloS Genet. 132, e1006607. doi: 10.1371/journal.pgen.1006607
Yan, J. D., Liao, X. Y., He, R. Q., Zhong, M., Feng, P. P., Li, X. M., et al. (2017). Ectopic expression of GA 2-oxidase 6 from rapeseed (Brassica napus l.) causes dwarfism, late flowering and enhanced chlorophyll accumulation in Arabidopsis thaliana. Plant Physiol. Biochem. 111, 10–19. doi: 10.1016/j.plaphy.2016.11.008
Yan, J. D., Xiang, F. J., Yang, P., Li, X., Zhong, M., He, R., et al. (2021). Overexpression of BnGA2ox2, a rapeseed gibberellin 2-oxidase, causes dwarfism and increased chlorophyll and anthocyanin accumulation in arabidopsis and rapeseed. Plant Growth Regulation 93, 65–77. doi: 10.1007/s10725-020-00665-6
Yue, L. Q., Li, Y. S., Zhong, M., Chai, X. R., Zhao, P. Y., Huang, R. M., et al. (2022). Benzoic acid, chlorine dioxide, and 1-methylcyclopropene induce flavonoid metabolic shifts in postharvest flowering Chinese cabbage revealed by high-dimensional analytical data. Int. J. Mol. Sci. 23, 6011. doi: 10.3390/ijms23116011
Zhang, L. L., Li, Z. P., Garraway, J., Cai, Q. Z., Zhou, Y. F., Li, X., et al. (2020). The casein kinase 2 β subunit CK2B1 is required for swollen stem formation via cell cycle control in vegetable Brassica juncea. Plant J. 104, 706–717. doi: 10.1111/tpj.14958
Zhang, W. F., Tan, L. B., Sun, H. Y., Zhao, X. H., Liu, F. X., Cai, H. W., et al. (2019). Natural variations at TIG1 encoding a TCP transcription factor contribute to plant architecture domestication in rice. Mol. Plant 12, 1075–1089. doi: 10.1016/j.molp.2019.04.005
Zhao, Q., Nakashima, J., Chen, F., Yin, Y. B., Fu, C. X., Yun, J. F., et al. (2013). LACCASE is necessary and nonredundant with PEROXIDASE for lignin polymerization during vascular development in arabidopsis. Plant Cell. 25, 3976–3987. doi: 10.1105/tpc.113.117770
Zhou, H., Liang, X. Y., Feng, N. J., Zheng, D. F., Qi, D. Q. (2021). Effect of uniconazole to soybean seed priming treatment under drought stress at VC stage. Ecotoxicol. Environ. Safety 224, 112619. doi: 10.1016/j.ecoenv.2021.112619
Zhou, T. M., Wu, Z., Wang, Y. C., Su, X. J., Qin, C. X., Huo, H. Q., et al. (2019). Modelling seedling development using thermal effectiveness and photosynthetically active radiation. J. Integr. Agricult. 18, 2521–2533. doi: 10.1016/S2095-3119(19)62671-7
Keywords: Brassica campestris L. ssp. chinensis var. utilis Tsen et Lee, uniconazole, hypocotyl elongation, phenylpropanoid biosynthesis pathway, basic leucine zipper
Citation: Zhang L, Zhong M, Yue L, Chai X, Zhao P, Kang Y and Yang X (2022) Transcriptomic and metabolomic analyses reveal the mechanism of uniconazole inducing hypocotyl dwarfing by suppressing BrbZIP39–BrPAL4 module mediating lignin biosynthesis in flowering Chinese cabbage. Front. Plant Sci. 13:1014396. doi: 10.3389/fpls.2022.1014396
Received: 08 August 2022; Accepted: 24 November 2022;
Published: 14 December 2022.
Edited by:
Huanzhong Wang, University of Connecticut, United StatesReviewed by:
Muhammad Aamir Manzoor, Anhui Agricultural University, ChinaCopyright © 2022 Zhang, Zhong, Yue, Chai, Zhao, Kang and Yang. This is an open-access article distributed under the terms of the Creative Commons Attribution License (CC BY). The use, distribution or reproduction in other forums is permitted, provided the original author(s) and the copyright owner(s) are credited and that the original publication in this journal is cited, in accordance with accepted academic practice. No use, distribution or reproduction is permitted which does not comply with these terms.
*Correspondence: Yunyan Kang, a2FuZ3l1bnlhbkBzY2F1LmVkdS5jbg==; Xian Yang, eWFuZ3hpYW5Ac2NhdS5lZHUuY24=
†These authors have contributed equally to this work
Disclaimer: All claims expressed in this article are solely those of the authors and do not necessarily represent those of their affiliated organizations, or those of the publisher, the editors and the reviewers. Any product that may be evaluated in this article or claim that may be made by its manufacturer is not guaranteed or endorsed by the publisher.
Research integrity at Frontiers
Learn more about the work of our research integrity team to safeguard the quality of each article we publish.