- 1National Research Center of Intercropping, The Islamia University of Bahawalpur, Bahawalpur, Pakistan
- 2College of Agronomy, Sichuan Agricultural University, Chengdu, China
- 3Gansu Academy of Agricultural Sciences, Lanzhou, Gansu, China
- 4National Center for Industrial Biotechnology, Pir Mehar Ali Shah-Arid Agricultural University, Rawalpindi, Pakistan
- 5Hermiston Agricultural Research and Extension Center, Oregon State University, Hermiston, OR, United States
- 6College of Agriculture, Nanjing Agricultural University, Nanjing, China
- 7Department of Agricultural Science and Technology, School of Agriculture and Enterprise Development, Kenyatta University, Nairobi, Kenya
- 8Shaanxi Key Laboratory of Earth Surface System and Environmental Carrying Capacity, College of Urban and Environmental Science, Northwest University, Xian, China
- 9Department of Plant Production and Genetics, Faculty of Agriculture, Urmia University, Urmia, Iran
- 10Department of Field Crops, Faculty of Agriculture, Siirt University, Siirt, Turkey
- 11Institute of Plant Breeding and Biotechnology, Muhammad Nawaz Sharif-University of Agriculture, Multan, Pakistan
- 12Department of Agronomy, Bahauddin Zakariya University, Multan, Multan, Pakistan
- 13Department of Botany and Plant Physiology, Faculty of Agrobiology, Food and Natural Resources, Czech University of Life Sciences Prague, Prague, Czechia
Sustainable increases in crop production require efficient use of resources, and intercropping can improve water use efficiency and land productivity at reduced inputs. Thus, in a three-year field experiment, the performance of maize/soybean strip intercropping system differing with maize plant density (6 maize plants m-2, low, D1; 8 maize plants m-2, medium, D2; and 10 maize plants m-2, high, D3) was evaluated in comparison with sole maize or soybean cropping system. Results revealed that among all intercropping treatments, D2 had a significantly higher total leaf area index (maize LAI + soybean LAI; 8.2), total dry matter production (maize dry matter + soybean dry matter; 361.5 g plant-1), and total grain yield (maize grain yield + soybean grain yield; 10122.5 kg ha-1) than D1 and D3, and also higher than sole maize (4.8, 338.7 g plant-1, and 9553.7 kg ha-1) and sole soybean (4.6, 64.8 g plant-1, and 1559.5 kg ha-1). The intercropped maize was more efficient in utilizing the radiation and water, with a radiation use efficiency of 3.5, 5.2, and 4.3 g MJ-1 and water use efficiency of 14.3, 16.2, and 13.3 kg ha-1 mm-1, while that of intercropped soybean was 2.5, 2.1, and 1.8 g MJ-1 and 2.1, 1.9, and 1.5 kg ha-1 mm-1 in D1, D2, and D3, respectively. In intercropping, the land and water equivalent ratios ranged from 1.22 to 1.55, demonstrating that it is a sustainable strategy to improve land and water use efficiencies; this maximization is likely associated with the species complementarities for radiation, water, and land in time and space, which resulted in part from competition avoidance responses that maximize the economic profit (e. g., 1300 US $ ha-1 in D2) over sole maize (798 US $ ha-1) or sole soybean (703 US $ ha-1). Overall, these results indicate that optimizing strip intercropping systems can save 20–50% of water and land, especially under the present scenario of limited resources and climate change. However, further research is required to fully understand the resource capture mechanisms of intercrops in intercropping.
Introduction
Food security is a prerequisite for ensuring national security and human survival. The global human population is projected to cross nine billion in 2050 (Thornton et al., 2014). Thus, to fulfill the enhanced demands of an increasing population for food and feed, it is estimated that the current crop yield needs to be increased by 50% in 2030 and 100% in 2050 (Li et al., 2020). The continuous decline in cultivable lands due to urbanization and industrialization has limited the further expansion in cultivation area of cereals (e. g., maize; Zea mays L.) and legumes (e. g., soybean; Glycine max L.). This situation is more serious in the developing countries (e. g., China, Pakistan, and India) that have more population and less cultivable land (Du et al., 2017; Iqbal et al., 2018). Furthermore, researchers have reported that the expansion in the cultivation area for food crops is the leading cause of deforestation in many regions that adversely affect the environment (Barona et al., 2010). Therefore, in the present scenario of limited resources (i. e., land and water) and climate change, it is important to develop new cropping systems (i. e., intercropping or agroforestry), which can increase crop yields by effectively using the limited resources without affecting the environment.
Intercropping, the cultivation of two or more crop species on the same land, provides opportunities for sustainable crop production and agricultural intensification (Feng et al., 2019). Intercropping results in higher crop yield at the system level (grain yield of species one + grain yield of species two) and less yield variation than mono-cropping systems (Martin-Guay et al., 2018). This higher and stable yield, particularly with reduced inputs, are mainly ascribed to resources (i. e., water, sunlight, and nutrients) complementarity (Liu et al., 2017; Gitari et al., 2018; Raza et al., 2019), in which intercrop species utilize available resources more adequately due to different spatial (Raza et al., 2021a), temporal (Yang et al., 2017), and phenological characteristics (Li et al., 2013). The intra- and interspecific competition (Yang et al., 2015), availability of environmental resources (Liu et al., 2017), and planting density of the intercrop species influenced the degree of resource complementarity (Ren et al., 2016) and the yield of intercropping (Hauggaard-Nielsen and Jensen, 2005). For instance, maize and soybean produced larger relative grain yields in strip intercropping than in mono-cropping (Chen et al., 2017; Du et al., 2017); and intercropping of maize with soybean achieved high land productivity (estimated as a land equivalent ratio; LER) with high maize planting density compared to low maize planting density under strip intercropping (Muoneke et al., 2007). These findings conclude that strip intercropping produces higher yields at the system level than mono-cropping due to complementarity and facilitation interactions.
Determining the optimum planting density of intercrop species is a paramount for higher crop yields in intercropping. Compared with mono-cropping, crops in intercropping use planting space more efficiently and effectively (Raza et al., 2020). The optimum planting density in intercropping outweighs the optimum planting density in mono-cropping (Willey and Osiru, 1972). Nevertheless, the optimum planting density of one intercrop species at one location, i. e., maize in maize/soybean intercropping at Sichuan under high-rainfall conditions (Feng et al., 2020), maize in maize/wheat intercropping at Wageningen under medium-rainfall conditions (Gou et al., 2016), maize in maize/pea intercropping at Gansu under low-rainfall conditions (Mao et al., 2012), and maize in maize/pigeon pea intercropping at Trinidad under irrigated conditions (Dalal, 1974), may not be applicable to other sites because of the regional variations in soil properties (water holding capacity, total available nitrogen, phosphorus, and potassium, and organic matter) and weather (precipitation, temperature, and solar radiation). However, lack of appropriate study and relevant literatures on determining the optimum planting density of maize in cereal/legume intercropping systems under irrigated conditions, especially in semi-arid areas (high-temperature regions, where farmers are using extra water for the production of cereals and legumes).
Researchers have previously reported that a higher planting density of intercropped maize resulted in greater intercropping advantages (Willey and Osiru, 1972; Muoneke et al., 2007). Whereas it significantly affects the competitive interactions between intercrops; for instance, the dominance of maize over soybean was enhanced with increased maize density, which ultimately decreased the grain yield of soybean in maize/soybean intercropping (Muoneke et al., 2007). In addition, the planting density of intercrop species, especially of tall crops, adversely affects the root growth and distribution (Hauggaard-Nielsen et al., 2001), sunlight transmittance (Li et al., 2001), leaf area development (Prasad and Brook, 2005), dry matter production (Ren et al., 2016), and resource capturing (Gao et al., 2009) of understory crops in cereal/legume intercropping systems. However, most past studies on the plant density response of intercrops have mainly been conducted by changing the row ratio or sowing proportions (Ofori and Stern, 1987; Ijoyah and Fanen, 2012; Mao et al., 2012). Thus, the response of intercrops to equal row-ratio and sowing proportion under strip intercropping systems remains unclear. The interaction (below and above ground) of intercrops species has been reported to enhance the water and light utilization efficiency. Furthermore, it has been rarely investigated how changing maize planting density affects the interspecific interactions, competition for the acquisition of available resources (i. e., water and radiation), and land productivity of maize/soybean strip intercropping (maize/soybean intercropping) under irrigated conditions. Therefore, the main aims of this study were to determine the effects of changing maize planting density on (i) growth and crop yields of maize and soybean in maize/soybean intercropping, (ii) resource (water or sunlight) utilization dynamics of intercrops under maize/soybean intercropping, and (iii) land productivity and economic viability of maize/soybean intercropping compared to sole cropping of maize and soybean using data from a three-year field experiment.
Materials and methods
Field experiments
The field study was conducted in 2018, 2019, and 2020 at Khairpur Tamewali (29.57°N, 72.25°E; altitude 130 m), Bahawalpur, Punjab Province, Pakistan, a research site of Sichuan Agricultural University, P. R. China. The research site has a continental monsoon climate, with a mean annual precipitation of 143 mm and a temperature of 25.7°C. The soil was a sandy clay loam, with 7.7 pH, 7.3 g kg-1 organic matter, 0.5 g kg-1 total nitrogen (N), 5.0 mg kg-1 available phosphorus (P), 341.5 mg kg-1 available potassium (K), and 1.47 Mg m-3 bulk density. Daily incident solar radiation, air temperature, and rainfall of 2018, 2019, and 2020 are shown in Figure 1. During the planting period (from sowing to harvest), total rainfall was 77, 105, and 280 mm in 2018, 2019, and 2020, respectively.
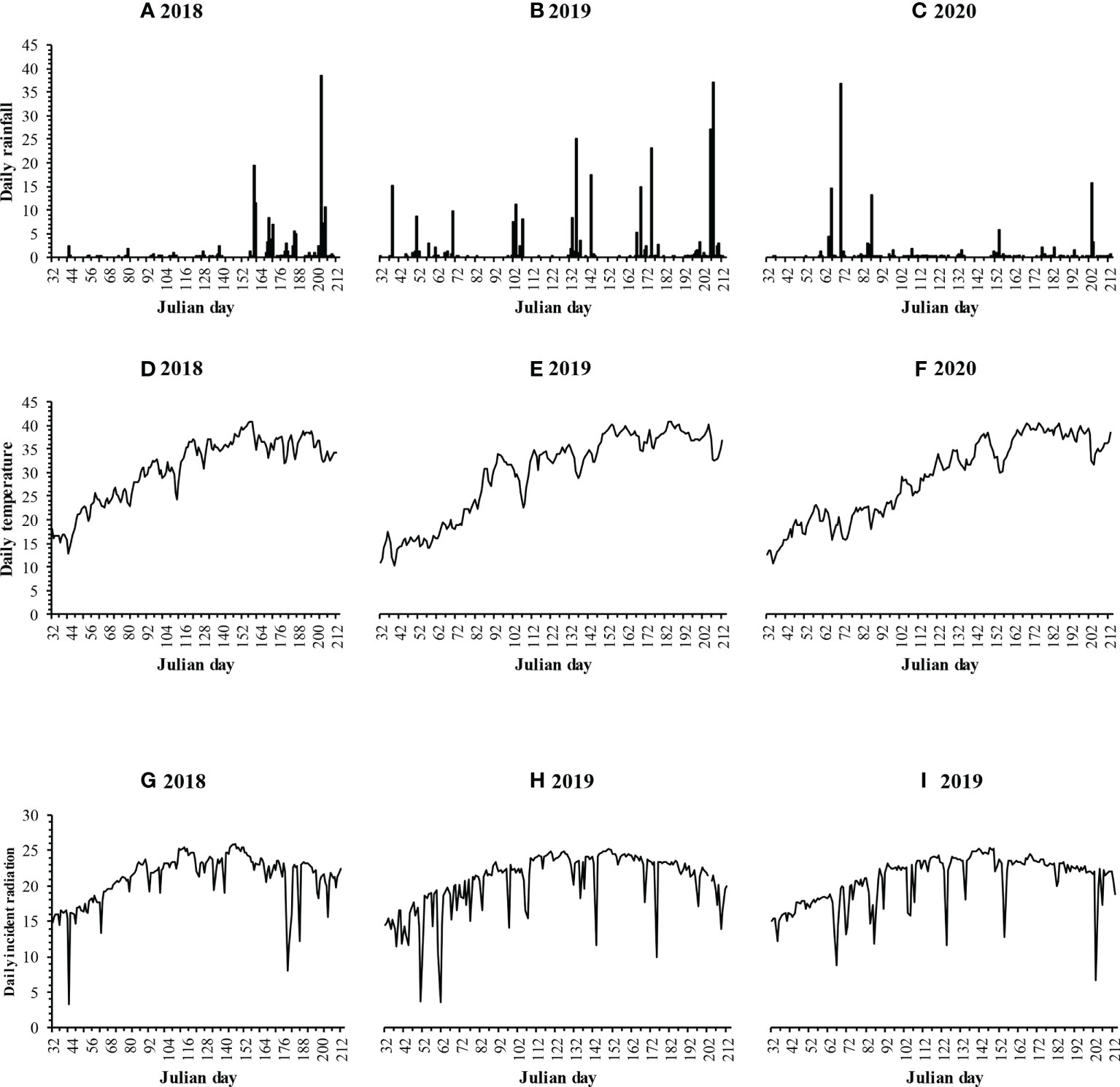
Figure 1 Daily rainfall (mm), temperature (°C), and incident radiation (MJ m-2 day-1) during the summer season of maize and soybean in 2018, 2019, and 2020.
The experiment was laid out in a randomized complete block design with three replications. The study consisted of three maize/soybean intercropping treatments differing with maize plant density (6 maize plants m-2, low, D1; 8 maize plants m-2, medium, D2; and 10 maize plants m-2, high, D3) and two sole cropping treatments of maize (M) and soybean (S). The intercropping treatments comprised of two rows of maize with two rows of soybean in each intercropping strip (Figure 2); six intercropping strips were arranged in each intercropping plot. The size of each plot was 144 m2 (12 m in width and 12 m in length). The plant configuration (i. e., row spacings, plant distances, and planting densities) in D1, D2, D3, M, and S are presented in Table 1. According to the local recommended planting densities, both sole crops were planted: 80000 plants ha-1 for maize and 140000 plants ha-1 for soybean. In addition, all agronomic practices, i. e., sowing, weeding, and harvesting, were done manually.
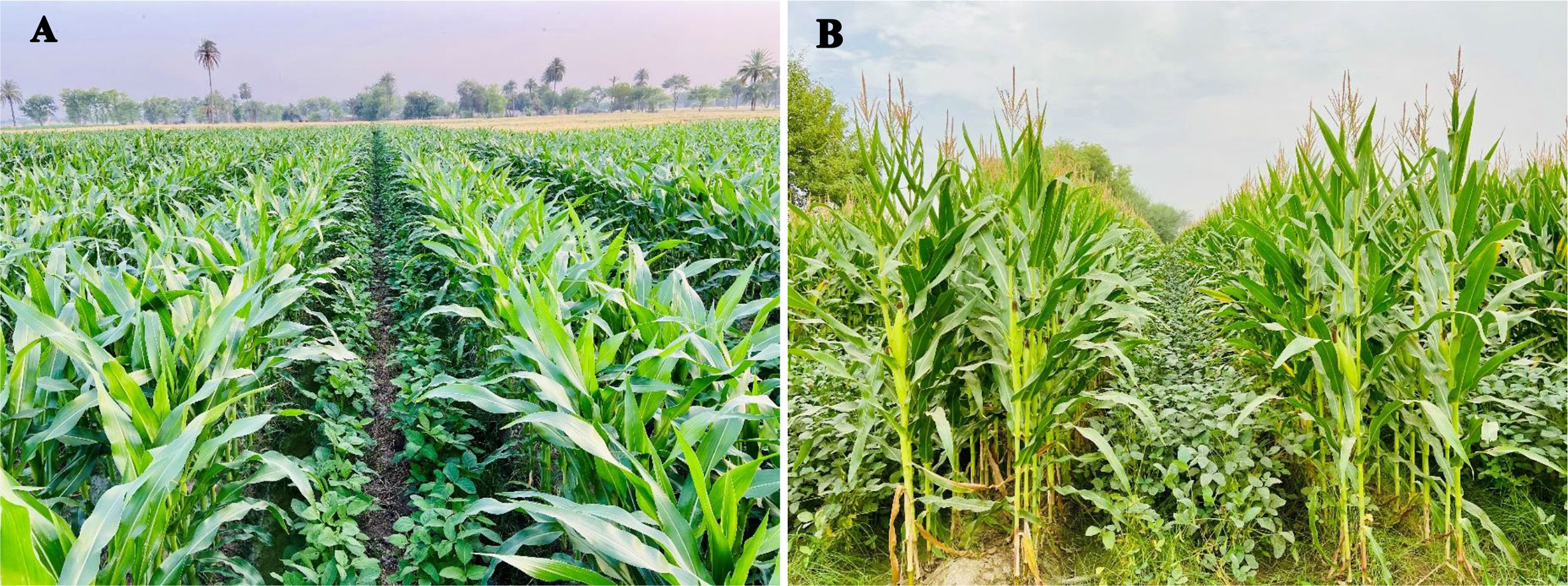
Figure 2 Field demonstration of maize/soybean strip intercropping system. (A) Intercrops were at the vegetative growth stage, and (B) Intercrops were at the reproductive growth stage (Photos: Muhammad Ali Raza). Location: Punjab Province, Pakistan.
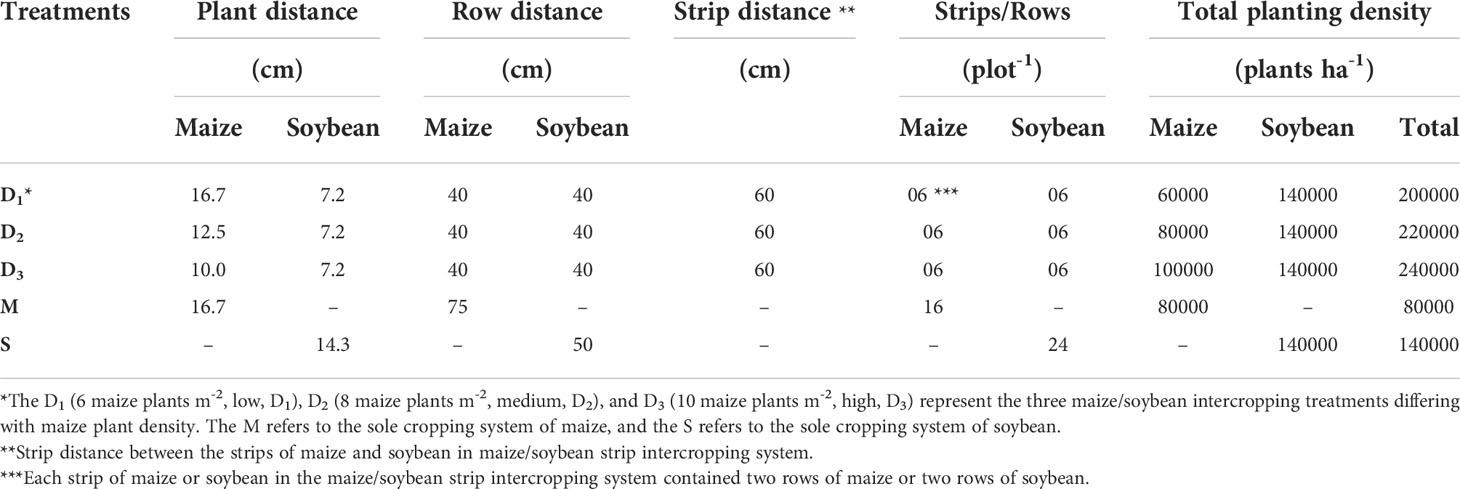
Table 1 The plant to plant, row to row, strip to strip distances for maize and soybean, and total planting densities of maize and soybean in intercropping and sole cropping systems.
The soybean (determinate) variety ‘NARC-16’ and maize (semi-compact) variety ‘DK-6317’ were used in the study. Both crops were planted and harvested on the same date, on February 03rd in 2018, February 05th in 2019, and February 7th in 2020; and harvested on June 30th in 2018, July 7th in 2019, and July 5th in 2020. Before sowing, for maize, basal N at 120 kg ha−1 as urea, P at 205 kg ha−1 as diammonium phosphate (DAP), and K at 150 kg ha−1 as potassium sulfate (SOP) were applied between maize rows in D1, D2, D3, and M. For soybean, basal N at 75 kg ha−1 as urea, P at 150 kg ha−1 as DAP, and K at 100 kg ha−1 as SOP were used between soybean rows in D1, D2, D3, and S. At the V6 and tasseling stages of maize, the second and third doses of N were applied at 60 and 100 kg ha−1, respectively, as urea between maize rows under D1, D2, D3, and M. Besides, all treatments were irrigated with the same amount of water across the whole experiment, and the detailed information is shown in Table 2. According to the local water application advisory for maize and soybean production, irrigation water was applied, which is equal to 550 ± 100 mm water for both crops depending on the crop or weather conditions. Groundwater was pumped out using a tube well and applied via the furrow irrigation method.
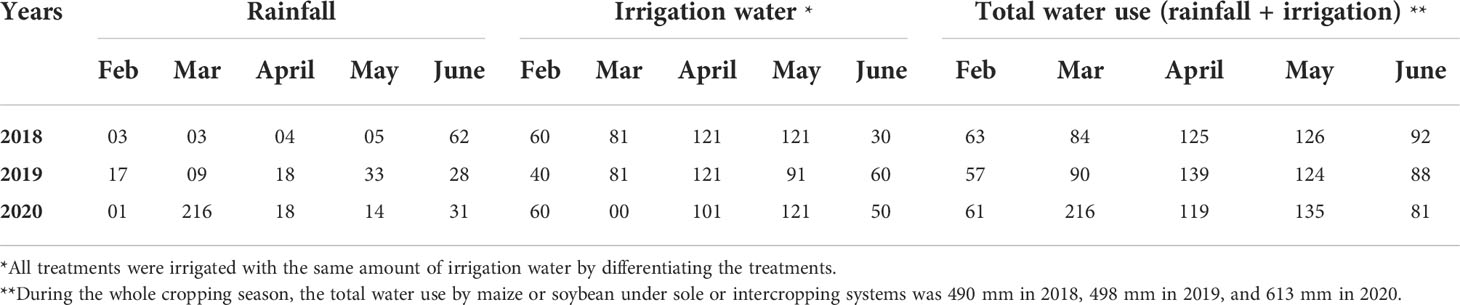
Table 2 Rainfall (mm), irrigation water (mm), and total water use (mm) of maize and soybean under sole and intercropping systems at the experimental site of Sichuan Agricultural University, Bahawalpur, South Punjab, Pakistan.
Measurements
Leaf area of maize and soybean was measured five times at 45, 65, 85, 105, and 125 days after sowing (DAS) in all years of this study. For this purpose, three maize and five soybean plants were destructively sampled from each plot at each sampling time. The leaf area of all leaves was determined by multiplying the greatest leaf width and length with the crop-specific co-efficient factor of 0.70 for maize and 0.75 for soybean (Gao et al., 2009). Then, the leaf area index (LAI) was calculated using the following equation (Montgomery, 1911).
Three maize and five soybean plants from each plot were collected at 45, 65, 85, 105, and 125 DAS for total dry matter production and partitioning analysis. Then, all samples were divided into various plant parts (root, straw (leaves + stem + non-grain parts), and grain) and sun-dried for the next seven to ten days to achieve a constant weight and presented as g plant-1. The total dry matter (TDM; g plant-1) of maize and soybean was determined from the summation of the dry matter of root, straw, and grain. Additionally, the total dry matter (g plant-1) of intercropping treatments was calculated from the summation of the total dry matter of maize and soybean in D1, D2, and D3.
To determine the grain yield of maize and soybean, 24 maize-ears and 40 soybean plants were collected from each plot of D1, D2, D3, M, and S at the maturity of both crops. These samples were used to quantify the yield response of maize and soybean to changing planting density in intercropping. All the harvested samples were sun-dried for the next seven to ten days. Then, the dried samples were manually threshed and weighed to determine the maize and soybean grain yield and converted into kg ha-1. Additionally, the total grain yield of intercropping treatments was calculated from the summation of the grain yield of maize and soybean in D1, D2, and D3.
To calculate the radiation use efficiency of both crops under different treatments, we first determine the daily total incident solar radiation (MJ m-2 day-1) using the following equation (Angstrom, 1924).
Where, SR0 was the extraterrestrial radiation. The a and b were the constants and used for those areas where the data for SR is not available (Allen et al., 1998). The n was the measured sunshine hours and the data for n was obtained from near the weather observatory, and N was the maximum possible sunshine hours.
The fraction of intercepted radiation (Fi) of maize and soybean in sole and intercropping systems was calculated using the exponential equation from their respective LAI values (Monteith and Elston, 1983).
Where, k was the extinction coefficient for total solar radiation (Monteith, 1977; Muurinen and Peltonen-Sainio, 2006), and the values of k for maize and soybean were 0.70 (Lindquist et al., 2005) and 0.45 (Zhang et al., 2014), respectively.
The total amount of incident photosynthetically active radiation (Si) was determined by multiplying the total incident radiation by 0.50 because researchers have concluded that the incident photosynthetically active radiation is equal to half (50%) of the daily total incident radiation (Szeicz, 1974; Sinclair and Muchow, 1999; Tesfaye et al., 2006). Then, the amount of intercepted radiation (Sa) for maize and soybean under sole and intercropping systems was calculated using the following equation (Szeicz, 1974).
Finally, the radiation use efficiency (RUE) of maize and soybean under sole and intercropping systems were calculated individually using the following equation (Monteith, 1977).
Where, TDM was the total dry matter of maize or soybean, ∑Sa was the cumulative intercepted photosynthetically active radiation of maize or soybean.
For calculating water use efficiency (WUE), we first measured the total water use (TWU) of maize and soybean in different treatments using the simplified water balance equation (Raza et al., 2021b).
Where P was the total precipitation (mm) received during the whole growing period (from February to July), IW was the total amount of applied irrigation water (mm), SWs and SWh were the soil water content (mm) at sowing and harvesting of the experiment, respectively. Then, the water use efficiency of both crops was calculated using the following equation (Zhang et al., 1998):
Where, GY was the grain yield of maize or soybean in intercropping or sole cropping systems, and TWU was the total water use calculated using the simplified water balance equation.
Furthermore, we calculated the water equivalent ratio (WER) to estimate the water-use advantage of intercropping over sole cropping system, and the partial WER of maize (WERMaize) and soybean (WERSoybean), and total WER was calculated using the following equations (Mao et al., 2012):
Where, WUEIM and WUEIS were the water use efficiency of intercropped maize and soybean, respectively. The WUEM and WUES were the grain yield of sole cropped maize and soybean, respectively.
We measured the land equivalent ratio (LER) to determine the land use advantage of intercropping over the sole cropping system (Raza et al., 2021b). The partial LER of maize (LERMaize) and soybean (LERSoybean), and total LER was calculated using the following equations:
Where, GYIM and GYIS were the grain yield of intercropped maize and soybean, respectively. The GYM and GYS were the grain yield of sole cropped maize and soybean, respectively.
Economic analysis
An economic analysis was performed to assess the economic viability of the maize/soybean intercropping system. Total expenditure for maize and soybean production under intercropping and sole cropping system was included; the cost of land rent, maize and soybean grains, land preparation, fertilizer (i.e., Urea, DAP, and SOP), weeding, thinning, irrigation, harvesting, and threshing of crops. Each treatment’s total income (gross income) was estimated according to the yearly local market prices for maize and soybean grains in Pakistan. The net profit was calculated by subtracting the total expenditure from the total income (Raza et al., 2018).
Statistical analysis
All data analyses were performed using Statistix 8.1. Significant differences were determined using ANOVA, and the LSD (Least Significance Difference) test was used to compare the means at a 5% probability level. Mean values are presented mean ± SE (standard error), based on the three independent replicates per treatment.
Results
Growth parameters
The LAI of maize and soybean under different planting systems is shown in Figure 3. At all sampling times, the LAI of maize and soybean were significantly lower under intercropping than sole maize and soybean. In intercropping treatments, at the final sampling time (125 DAS), the average highest soybean (4.2) and maize (4.6) LAI was measured under D1 and D2, whereas the average lowest soybean (3.1) and maize (3.5) LAI was recorded in D3 and D1, respectively. However, at all sampling times, the total LAI of maize and soybean in intercropping treatments was significantly higher than M and S (Table 3). For instance, at 125 DAS, the total LAI in D1, D2, and D3,
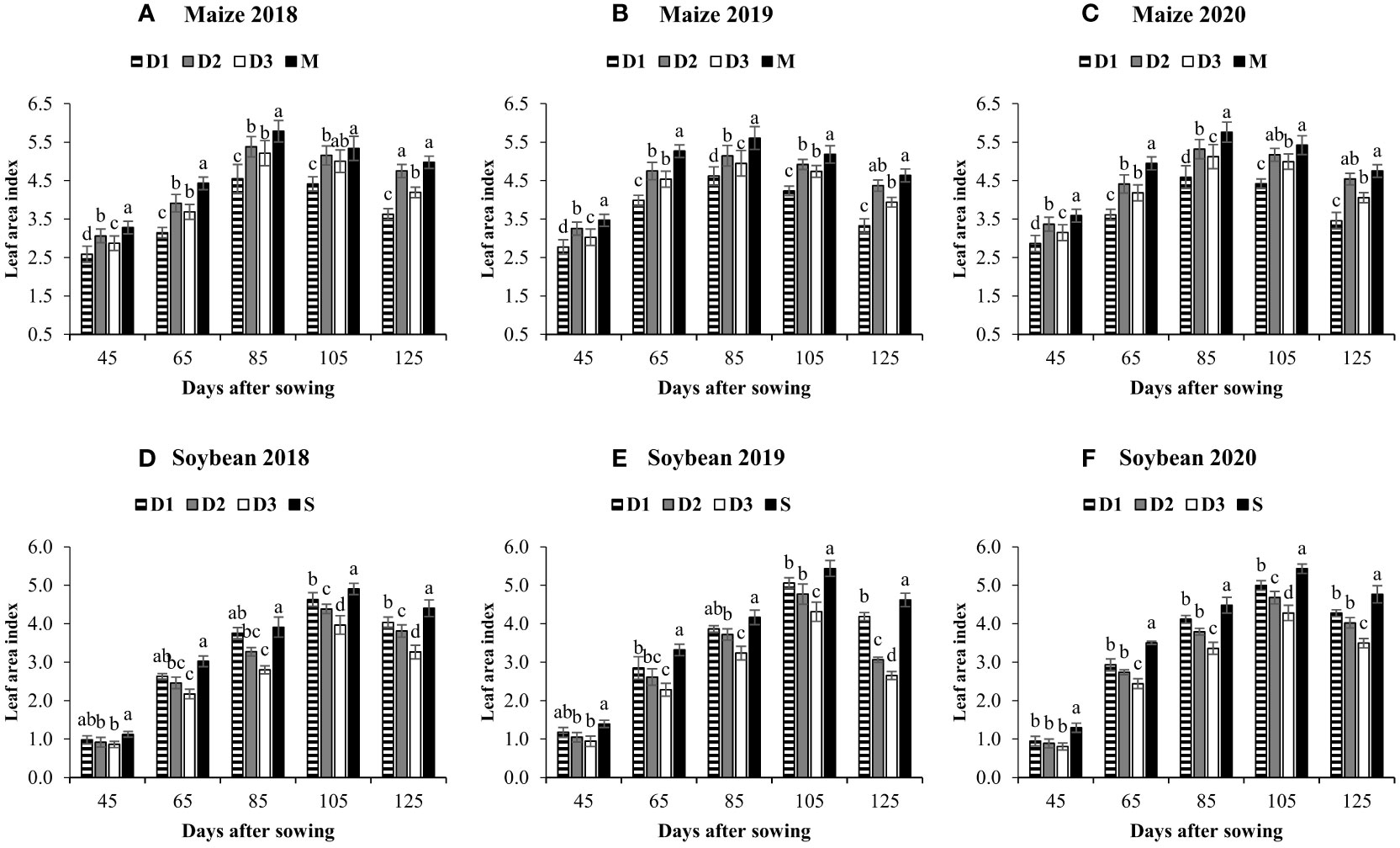
Figure 3 Leaf area index of maize (A–C) and soybean (D–F) in response to different maize planting densities (6 maize plants m-2, low, D1; 8 maize plants m-2, medium, D2; and 10 maize plants m-2, high, D3) under maize/soybean strip intercropping. Bars show ± standard errors (n = 3). The different lowercase letters within a bar show a significant difference (p < 0.05) among treatments. The M and S represent the sole maize and soybean, respectively.
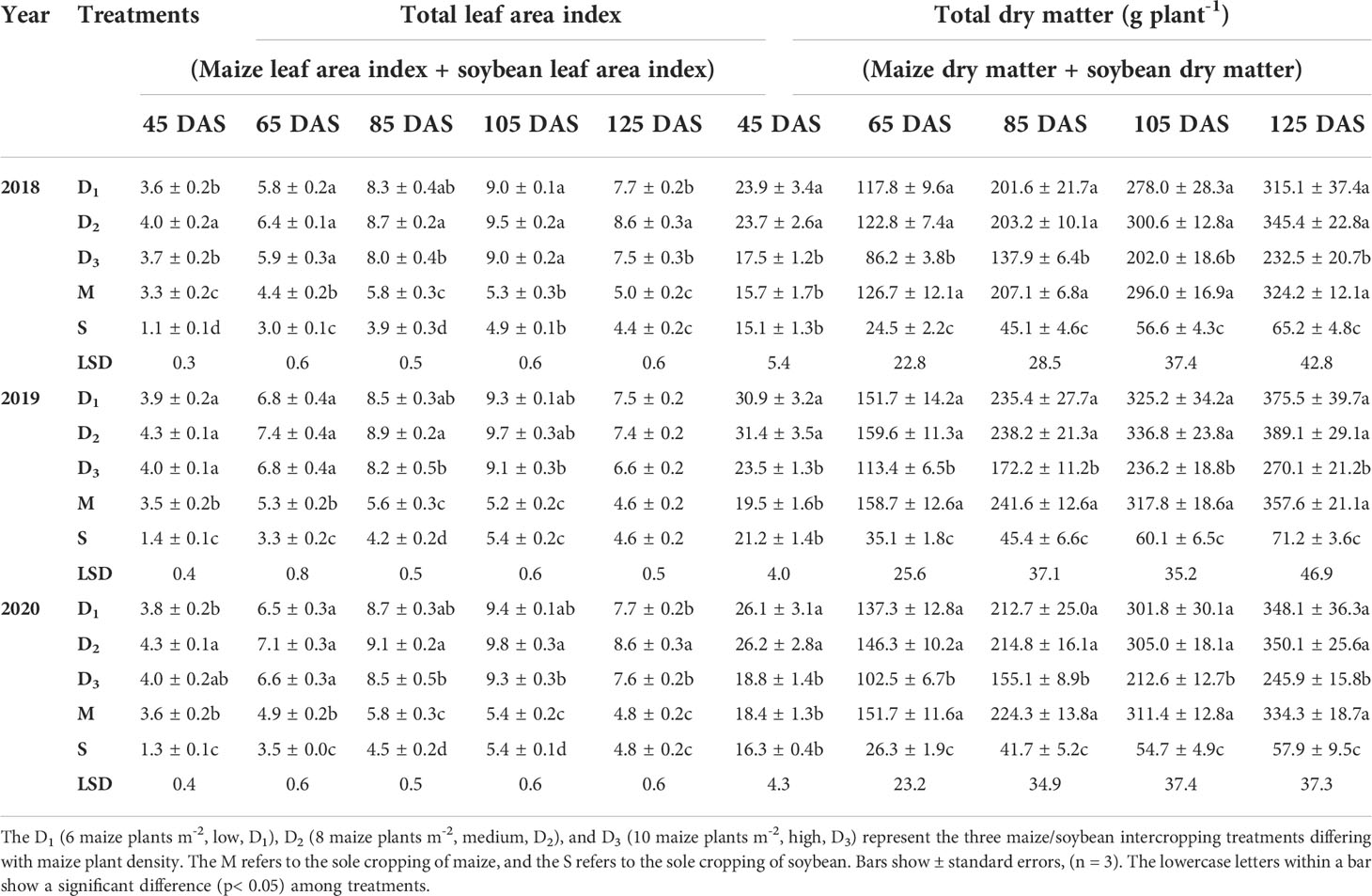
Table 3 Total leaf area index and total dry matter of maize and soybean at 45, 65, 85, 105, and 125 days after sowing (DAS) under different maize/soybean strip intercropping treatments and sole cropping of maize and soybean.
Different treatments significantly affected the total dry matter production of maize and soybean. Across different sampling stages and treatments, maize and soybean plants accumulated higher dry matter in M and S, respectively, than intercropping treatments. In contrast, at the final sampling stage (125 DAS), the average total dry matter (maize dry matter + soybean dry matter; Table 3) of D2 (361.2 g plant-1) was higher than the corresponding values of dry matter in M (338.7 g plant-1) and S (64.8 g plant-1). In intercropping treatments, maize accumulated the highest (319.9 g plant-1) and lowest (218.6 g plant-1) dry matter under D2 and D3, while soybean accumulated the maximum (52.4 g plant-1) and minimum (30.9 g plant-1) dry matter in D1 and D3, respectively (Figure 4). In addition, different maize planting density treatments in intercropping not only affected dry matter production of intercrops but also changed dry matter partitioning in various plant parts of maize (Table 4) and soybean (Table 5). For example, across the years, at 125 DAS, treatment D2 significantly increased dry matter of maize grains by 13% and 46% compared to D1 and D3, while treatment D1 enhanced dry matter of soybean grains by 21% and 47% compared to D2 and D3, respectively. Whereas, relative to D2, the treatment D3 significantly decreased dry matter of maize and soybean roots (by 29% and 19%), straw (by 32% and 29%), and grains (by 31% and 18%), respectively, indicating that the high maize planting density in intercropping caused a significant reduction in dry matter accumulation and partitioning to economic parts (i. e., grains).
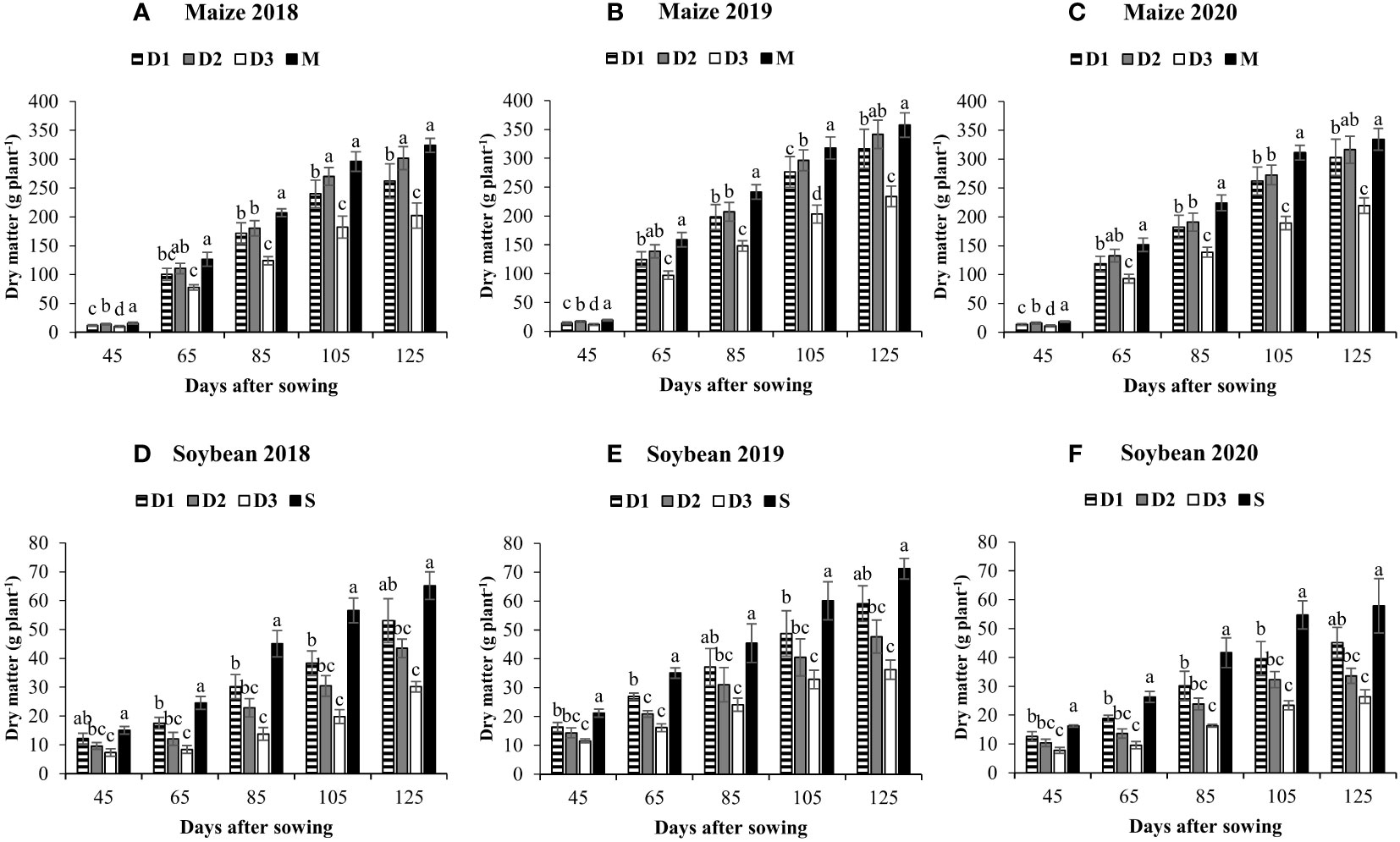
Figure 4 Dry matter of maize (A–C) and soybean (D–F) in response to different maize planting densities (6 maize plants m-2, low, D1; 8 maize plants m-2, medium, D2; and 10 maize plants m-2, high, D3) under maize/soybean strip intercropping. Bars show ± standard errors (n = 3). The different lowercase letters within a bar show a significant difference (p < 0.05) among treatments. The M and S represent the sole maize and soybean, respectively.
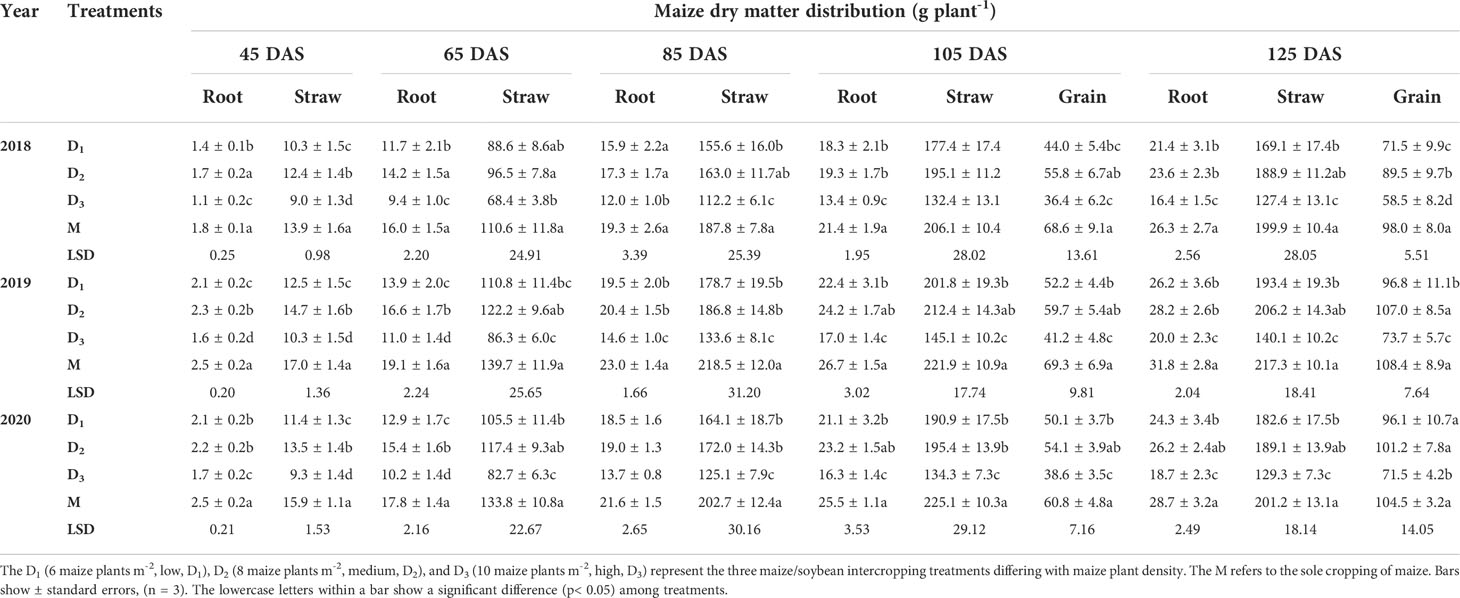
Table 4 Dry matter partitioning in different plant parts of maize at 45, 65, 85, 105, and 125 days after sowing (DAS) under different maize/soybean strip intercropping treatments and sole cropping of maize.
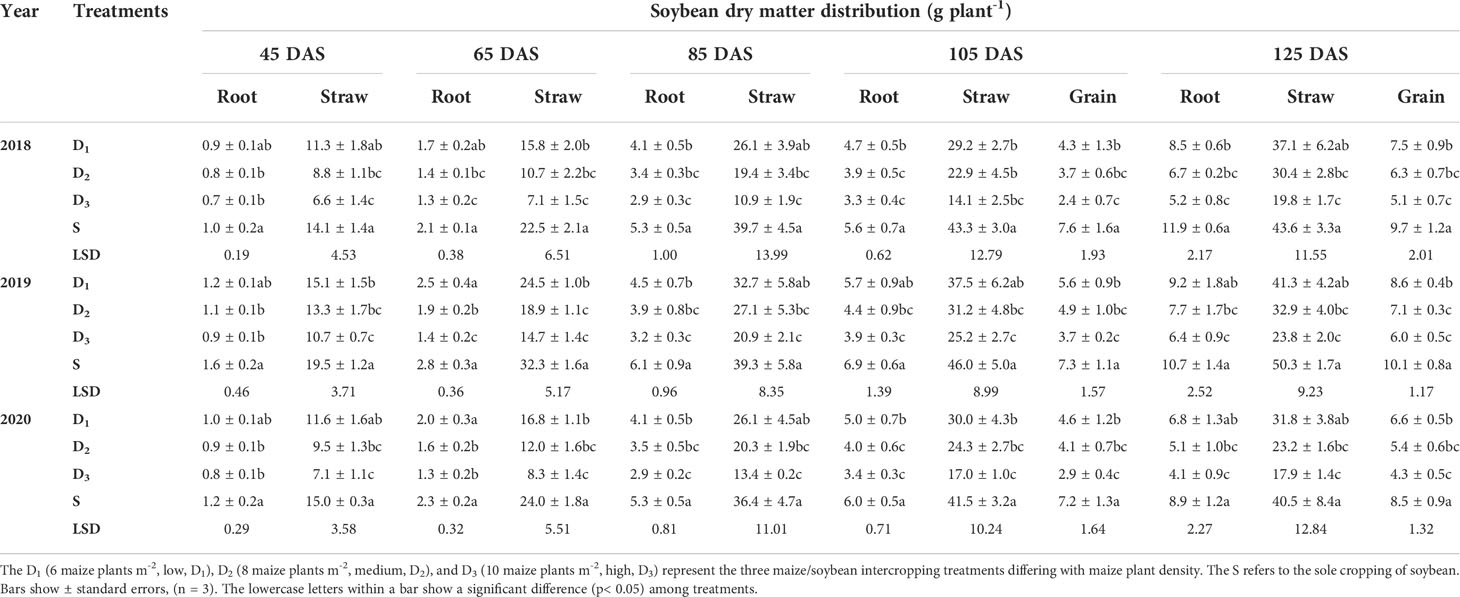
Table 5 Dry matter partitioning in different plant parts of soybean at 45, 65, 85, 105, and 125 days after sowing (DAS) under different maize/soybean strip intercropping treatments and sole cropping of soybean.
Crop level yields and system-level yield
Grain yield by the intercropped maize and soybean in D1, D2, and D3, compared to sole cropping treatments, is presented in Figure 5. The grain yield of maize and soybean in intercropping treatments ranged from 7376.9 to 9047.5 kg ha-1 and 830.9 to 1193.5 kg ha-1, respectively, which were significantly lower than the three-years average grain yield of M (9553.7 kg ha-1) and S (1826.2 kg ha-1). However, across the years, the total grain yield of maize and soybean was significantly higher in D2 (10122.5 kg ha-1) compared to D1 (9160.7 kg ha-1) and D3 (8207.9 kg ha-1), and it was also higher than the grain yield of M and S (Figure 5C). Furthermore, among the intercropping treatments, the grain yield of maize significantly increased with increasing maize density from 6 maize plants m-2 (D1) to 8 maize plants m-2 (D2), while it decreased under 10 maize plants m-2 (D3). Contrarily, soybean grain yield significantly reduced with increasing maize density, and the maximum (1193.5 kg ha-1) and minimum (830.9 kg ha-1) soybean grain yield were obtained in D1 and D3, respectively. Overall, in D1, D2, and D3, maize produced 83%, 95%, and 77% of M yield, and soybean produced 65%, 59%, and 45% of S yield, respectively.
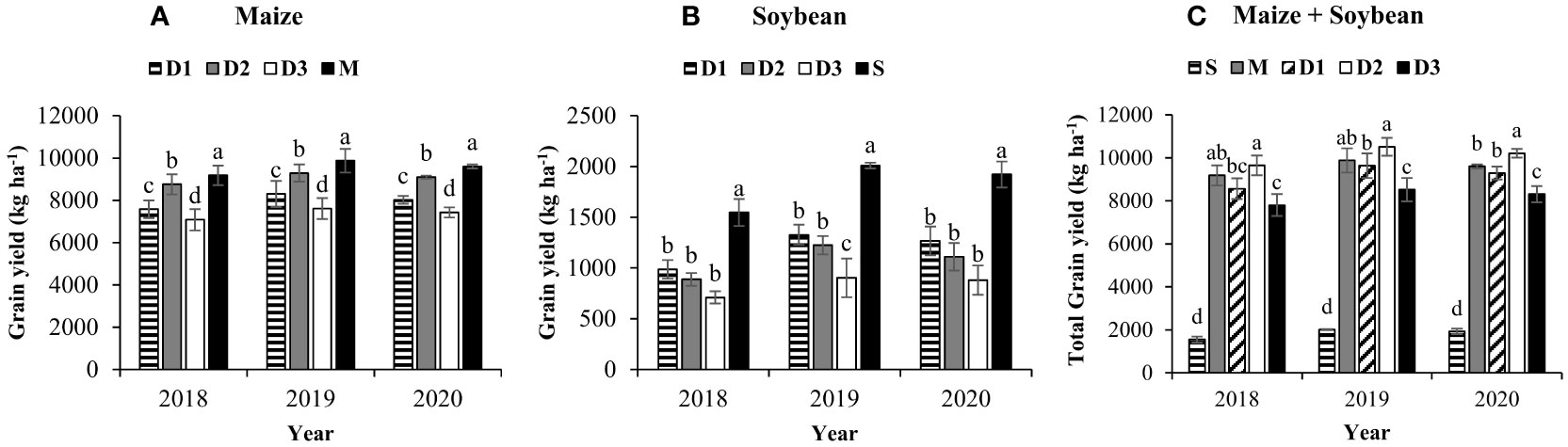
Figure 5 Three years average grain yield of maize (A), soybean (B), total grain yield (C) in response to different maize planting densities (6 maize plants m-2, low, D1; 8 maize plants m-2, medium, D2; and 10 maize plants m-2, high, D3) under maize/soybean strip intercropping. Bars show ± standard errors (n = 3). The different lowercase letters within a bar show a significant difference (p < 0.05) among treatments. The M and S represent the sole maize and soybean, respectively.
Resource (water and radiation) utilization dynamics
The RUE of maize and soybean differed significantly in all treatments, and data are presented in Table 6. Across the years, the partial RUE of intercropped maize (3.5 g MJ-1 in D1, 5.2 g MJ-1 in D2, and 4.3 g MJ-1 in D3) and soybean (2.5 g MJ-1 in D1, 2.1 g MJ-1 in D2, and 1.8 g MJ-1 in D3) were significantly lower than the corresponding values of M (5.9 g MJ-1) and S (3.2 g MJ-1). However, the total RUE of maize and soybean in intercropping was considerably higher than that of the M and S, indicating the advantage of intercropping in utilizing the sunlight than sole systems. Additionally, in intercropping, the RUE of maize was higher than that of soybean, demonstrating the dominance of maize over soybean. On average, D2 enhanced the total RUE by 20% and 18% compared to D1 and D3, respectively.
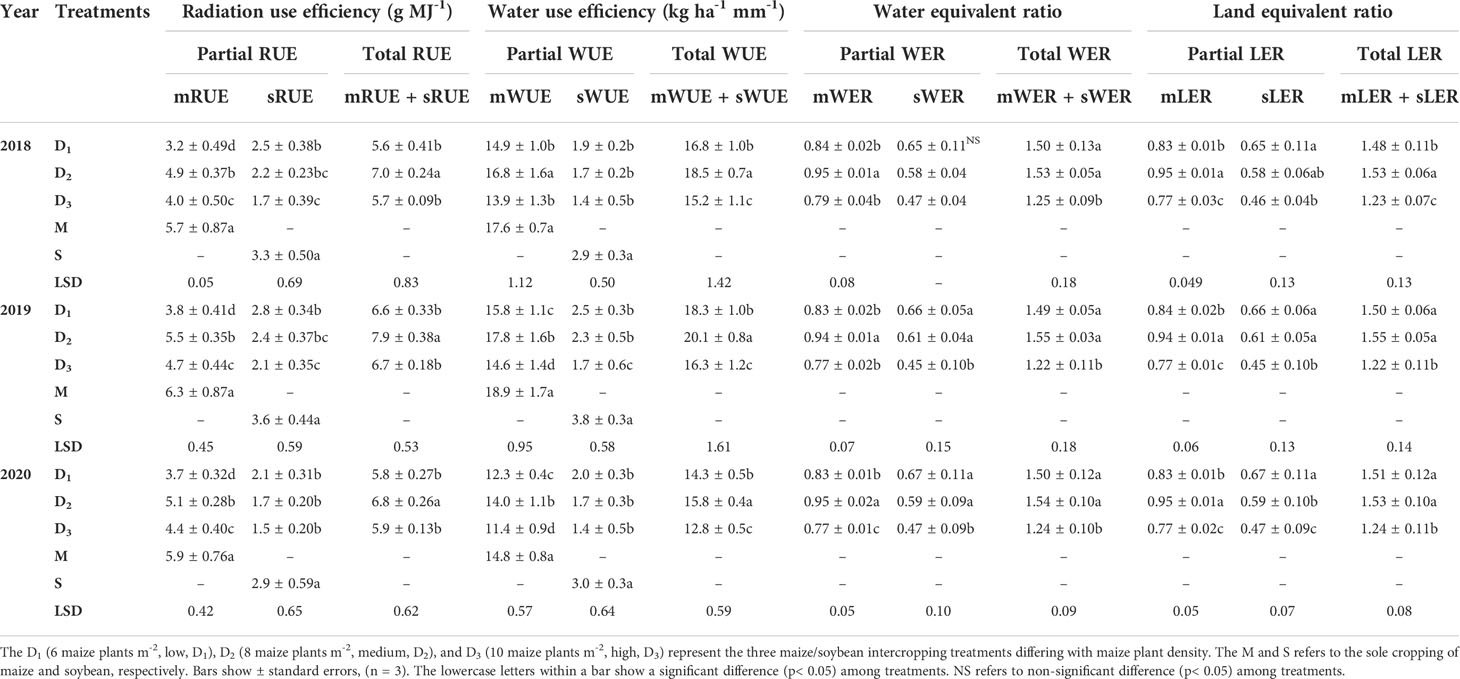
Table 6 Radiation-use-efficiency (RUE), water-use-efficiency (WUE), water equivalent ratio (WER), and land equivalent ratio (LER) of maize and soybean under different maize/soybean strip intercropping treatments and sole cropping of maize and soybean.
There were significant differences in WUE of maize and soybean in intercropping and sole cropping treatments, and data are shown in Table 6. Based on average WUE values in three years, the WUE of maize (14.3 kg ha-1 mm-1 in D1, 16.2 kg ha-1 mm-1 in D2, and 13.3 kg ha-1 mm-1 in D3) and soybean (2.1 kg ha-1 mm-1 in D1, 1.9 kg ha-1 mm-1 in D2, and 1.5 kg ha-1 mm-1 in D3) in intercropping treatments was found significantly lower than that of M (17.1 kg ha-1 mm-1) and S (3.2 kg ha-1 mm-1), respectively. However, the effect of intercropping on WUE was determined using the values of WER because it characterizes whether the total yield of maize and soybean in D1, D2, and D3 will be produced with more water (WER > 1) or less water (WER< 1) in sole maize and soybean treatments, and data are shown in Table 6. In this study, the mean total WER (WERMaize + WERSoybean) values of D1 (1.50), D2 (1.54), and D3 (1.24) were consistently higher than unity, demonstrating the water use advantage of intercropping over sole cropping. Moreover, in intercropping treatments, the partial WER values of maize were consistently higher than the partial WER values of soybean, showing that the maize had a competitive advantage over soybean in using the available water. The maximum WERMaize and WERSoybean were in D2 and D1, while the minimum WERMaize and WERSoybean were in D1 and D3, respectively.
Land productivity and economic viability
The total LER (LERMaize + LERSoybean) of intercropping treatments ranged from 1.22 to 1.55 in the three years of this experiment, and data are given in Table 6. Thus, there was a substantial land-use advantage under intercropping over sole cropping treatments. On average, in intercropping, the total LER was consistently higher in D2 (1.54) than D1 (1.50) and D3 (1.23). Across years and intercropped species, the partial LER values of maize and soybean in intercropping treatments ranged from 0.77 to 0.95 and 0.45 to 0.67, respectively. In intercropping treatments, soybean had the lowest partial LER values, and it decreased with increasing maize planting density. In contrast, maize had the high partial LER values, and it increased from low to medium maize planting density, and then decreased with high maize planting density. Despite the low soybean partial LER values, all the intercropping treatments achieved the high total LER values because the considerable yield of soybean compensated the slight yield loss of maize in D1, D2, and D3 compared to M. Overall, the medium (D2) maize planting density treatment increased the total LER by 3% and 25% relative to low (D1) and high (D3) maize planting density treatments, respectively.
Variations in grain yield directly affected the gross income and net income of D1, D2, D3, M, and S, and data are presented in Table 7. Across the years, the highest gross (2624 US $ ha-1) and net (1300 US $ ha-1) income were obtained under treatment D2, whereas the lowest gross (1539 US $ ha-1) and net (703 US $ ha-1) income were noticed in S treatment. Overall, the intercropping treatment D2, enhanced the net income by 63% compared to M and by 85% compared to S, respectively, indicating that the intercropping had an advantage over M and S in utilizing the available resources, i. e., radiation, water, and land.
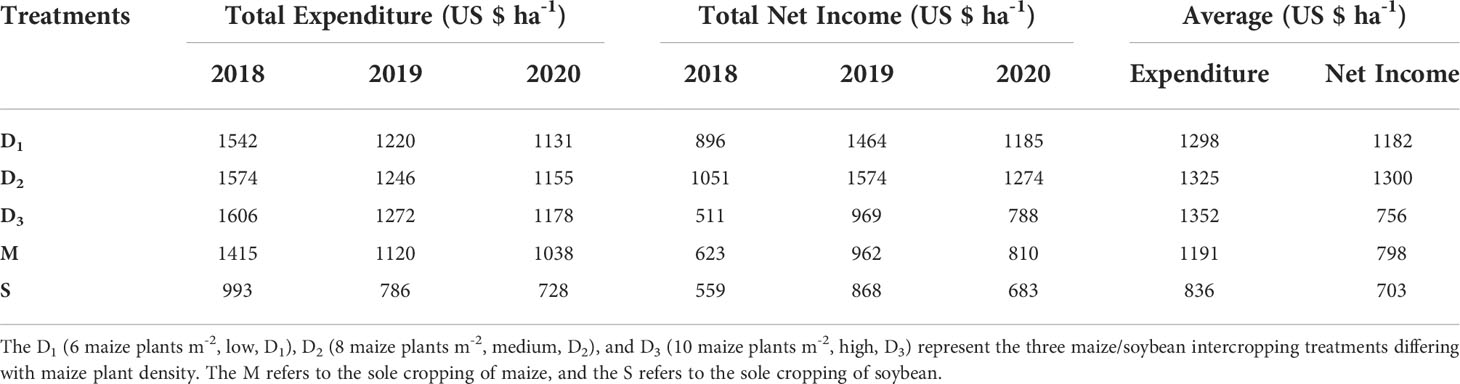
Table 7 Total expenditure and total net income of maize and soybean under different maize/soybean strip intercropping treatments and sole cropping of maize and soybean.
Discussion
The combination of maize and soybean as intercropping is a better option for irrigated areas under semi-arid conditions. Our three-year field study proved this, where we recorded high land- and water-equivalent ratios, showing a substantial increase in land and water use in intercropping treatments over sole cropping systems. Notably, just 50% of the total land was available for maize or soybean in intercropping treatments, while maize or soybean yield in intercropping treatments was higher than half of the sole maize or soybean yield. These results are aligned with the previously observed growth and yield pattern of cereals and legumes under intercropping systems (Li et al., 2020; Raza et al., 2021a). Overall, this shows that the extra yield produced by soybean in intercropping had minor consequences for maize production, and the interaction between maize and soybean was not highly competitive in intercropping treatments. Therefore, the system as a whole (maize + soybean) enhanced the total resource capturing and utilization beyond that of the sole cropping systems due to the complementary resource use of both species in intercropping (Yang et al., 2017; Iqbal et al., 2018; Liu et al., 2018; Ren et al., 2019; Li et al., 2020).
In intercropping, the better growth (measured as leaf area index and total dry matter production) of maize was likely associated with greater light use efficiency (Liu et al., 2018), water use efficiency (Rahman et al., 2017), nutrient accumulation (Ahmed et al., 2018), and plasticity of edge-row plants (Zhu et al., 2016). In contrast, the intercropped soybean growth was significantly lower in intercropping treatments than in sole soybean and this difference was increased with increasing maize density where soybean suffered from heavy maize shading (Yang et al., 2017) and water stress than sole soybean (Raza et al., 2021a). Thus, optimum maize planting density in intercropping (8 maize plants m-2) can increase maize yield with maintained soybean yield by improving the light transmittance at the soybean canopy and reducing the intra-specific competition for available resources, especially for light and water (Zhang 2007; Yang et al., 2015; Feng et al., 2020). Additionally, under semi-arid conditions, maize and soybean growth and yield are easily subjected to water stress (Cui et al., 2020). Therefore, the intercropping of maize with soybean could play a vital role in saving water, especially under semi-arid conditions, because intercropping systems reduce water evaporation due to greater canopy closure, which means that intercrops can produce more grains per mm of water than sole crops (Cooper et al., 1987; Wallace, 2000; Raza et al., 2021b).
Compared to past studies (Gao et al., 2010), the enhanced radiation use efficiency in different maize planting density treatments under maize/soybean intercropping was mainly associated with density and planting arrangement advantage. In this study, we planted both crops using the narrow-wide-row planting arrangement (narrow inter-row distance between maize or soybean rows and wide intra-row distance between maize and soybean strips), which gives the edge row advantage and spatial light distribution advantage. Besides, the total planting density (maize planting density + soybean planting density; Table 1) in intercropping treatments was considerably higher than sole crops (Feng et al., 2019), which resulted in increased radiation use efficiency as it was followed by a high leaf area index (Raza et al., 2021a). Although the individual leaf area index values of intercrop species were lower in intercropping, but the total leaf area index of maize and soybean was relatively higher than sole crops. This might have resulted in an increased light interception in intercropping, which consequently increased the total radiation use efficiency of maize/soybean intercropping than sole maize or sole soybean. Our results are in line with the previous report (Feng et al., 2019), in which they reported greater light interception and radiation use efficiency in maize/soybean intercropping and linked it with an improved leaf area index, light interception, and dry matter production (Liu et al., 2018). However, the partial RUE of intercropped maize or soybean in intercropping was significantly lower than that of sole maize or soybean, indicating the competition for solar radiations between intercrops in intercropping, as reported in many previous studies (Gao et al., 2010; Feng et al., 2020; Raza et al., 2020). Therefore, the radiation use efficiency of intercropping systems can be increased by selecting the optimum planting density of intercrop species, especially of tall crops (i. e., maize, millet, sorghum, etc.) because it directly influences the light environment of short stature crops (i. e., soybean, peanut, pea, etc.) in cereal legume intercropping systems.
The data of water equivalent ratio indicated that maize/soybean intercropping considerably increased the water use efficiency. Considering that the intercropping had a 175% planting density in D1, 200% planting density in D2, and 225% planting density in D3, indicating that the intercropped soybean and maize produced more seeds mm-1 of water than sole maize or soybean because under intercropping treatments the total available water was halved for soybean and maize. In addition, the different maize planting density treatments significantly affected the water use efficiency of intercropped species. The increasing maize density from 8 to 10 maize plants m-2 decreased the water use efficiency and partial water equivalent ratio of maize and soybean, suggesting the competition for water first among maize plants and second between maize and soybean plants, which means that appropriate planting density of intercrop species is critical in achieving high water productivity through resource complementarity (Mao et al., 2012), especially under the scenario of limited water resources (Ren et al., 2016). Interestingly, in all treatments, maize produced more grains mm-1 of water than soybean because maize had a competitive advantage over soybean in root growth and development, which ultimately increased the water uptake and used in maize than soybean (Raza et al., 2021b). However, despite this asymmetry in water uptake and use between soybean and maize, all intercropping treatments were still advantageous in translating water into grains, as indicated by total grain yields. This improvement in water use efficiency in D1, D2, and D3 might be caused by: (i) the water use efficiency of maize and soybean in intercropping depends on the selection of appropriate planting density, especially of maize (Ren et al., 2016); (ii) medium planting density of maize (8 maize plants m-2; D2) increased the water use efficiency of maize and maintained the water use efficiency of soybean under maize/soybean intercropping, which in return increased the total water equivalent ratio (Raza et al., 2021b); and (iii) all intercropping treatments were irrigated with the same amount of water as sole soybean or maize but produced more grains mm-1 of water, which might be associated with reduced evapotranspiration from the soil and plant surface due to greater canopy closure in intercropping (Cooper et al., 1987; Wallace, 2000). Another possible reason for high WER is related to complementarity in water uptake lower and upper soil depths by maize and soybean, respectively (Bai et al., 2016). However, more research is needed to understand complementarity in water acquisition from different soil depths by intercrops.
Total economic return (net profit) is the main factor for adopting any new planting method or practice (Piepho, 1998; Raza et al., 2019). Agreeing with previous results (Du et al., 2017; Li et al., 2020), the findings of this study demonstrate high resource (radiation, water, and land) use advantages, crop yield stability, and total net profit of all intercropping treatments over the sole maize and sole soybean under semi-arid conditions with irrigation. Additionally, the higher net profit of intercropping over sole cropping suggested that farmers could plant soybean and maize together in intercropping with a minimal overall yield penalty. The improvement in greater economic returns mainly attributed to an extra yield of soybean with maintained maize yield, especially under D2, which ultimately increased the total profit by 63% and 85% over sole maize and soybean because, in the local market, the price of soybean is three times expensive than maize price. Therefore, we can conclude that intercropping of soybean with maize, especially at eight maize plants m-2, is the better planting practice to obtain high economic returns with limited resources. Moreover, with appropriate planting configuration and density in maize/soybean strip intercropping, farmers can increase soybean production without decreasing the maize production and area, ultimately improving soil fertility and productivity through nitrogen fixation and release of root exudates (Chen et al., 2017). However, future studies are needed to quantify the resource use mechanism of intercropped maize and soybean in intercropping, especially under the changing climate scenarios. For instance, crops under intercropping may perform differently under low light regions (i. e., Sichuan in China), and farmers need to reduce the overall planting density to avoid the mutual shading effect on intercrops.
Conclusion
The system yield (maize yield + soybean yield), resource utilization (radiation and water), and net income advantages of intercropping over sole cropping were high and consistent over three years, indicating that intercropping is a more effective and profitable planting system than sole systems. Overall, these results indicate that optimizing strip intercropping systems can save 20–50% of water and land, especially under the present scenario of limited resources and climate change. Therefore, we can conclude that intercropping could be a productive and sustainable system to alleviate poverty and drought risk, especially for small landholder farmers in developing countries. However, future studies are required to quantify the resource use mechanism of intercrops in intercropping, particularly in the present climate change scenario. Moreover, intercropping-specific small farm machinery is needed (sowing and haversting specific equipments) to obtain the maximum advantages of intercropping; without resolving this issue, we cannot attain the full benefits of intercropping systems.
Data availability statement
The original contributions presented in the study are included in the article/supplementary material. Further inquiries can be directed to the corresponding authors.
Author contributions
MAR, HSY and HG: design and conceived research and writing original draft; RQ, AMD, MHBK, and SH: writing, reviewing and editing; JW, HGT, AS; reviewing, editing and analysis; AM, ERC, AF, and SA: data curation; FY, MS and WY: project administration and supervision; WY: reviewing and supervision. All authors contributed to the article and approved the submitted version.
Funding
This research was financially supported by the International Cooperation Project of Sichuan Province (2020YFH0126) and the Program on Industrial Technology System of National Soybean (CARS-04-PS19). This publication has been supported by the project OP VVV CZ.02.2.69/0.0/0.0/18_054/0014642 and the Operational Program Integrated Infrastructure within the project: Demand-driven Research for the Sustainable and Innovative Food, Drive4SIFood 313011V336, co-financed by the European Regional Development Fund.
Acknowledgments
MAR would sincerely express his gratitude to Prof. Athar Mahboob, who made possible whatever he deemed, especially for strip intercropping’s promotion and demonstrations in Pakistan. Even though he found it difficult to substitute his feelings and respect in words, he would say that every aspect regarding your support, including preliminary discussions and funds, encouragement and compliments, belief and love, finally made him to confidently set goal-oriented priorities; you are an honorable and great teacher who brilliantly tracked the points regarding the strengths and weaknesses of my work and personality.
Conflict of interest
The authors declare that the research was conducted in the absence of any commercial or financial relationships that could be construed as a potential conflict of interest.
Publisher’s note
All claims expressed in this article are solely those of the authors and do not necessarily represent those of their affiliated organizations, or those of the publisher, the editors and the reviewers. Any product that may be evaluated in this article, or claim that may be made by its manufacturer, is not guaranteed or endorsed by the publisher.
References
Ahmed, S., Raza, M., Zhou, T., Hussain, S., Khalid, M., Feng, L., et al. (2018). Responses of Soybean Dry Matter Production, Phosphorus Accumulation, and Seed Yield to Sowing Time under Relay Intercropping with Maize. Agronomy 8, 282.
Allen, R. G., Pereira, L. S., Raes, D., Smith, M. J. F. (1998). Crop evapotranspiration-guidelines for computing crop water requirements-FAO irrigation and drainage paper 56 Vol. 300 (9), (Rome: FAO), D05109.
Angstrom, A. (1924). Report to the international commission for solar research on actinometric investigations of solar and atmospheric radiation. Q. J. R. Meteorol. Soc. 50 (210), 121–126.
Bai, W., Sun, Z., Zheng, J., Du, G., Feng, L., Cai, Q., et al (2016). Mixing trees and crops increases land and water use efficiencies in a semi-arid area. Agric. Water Manag. 178, 281–290.
Barona, E., Ramankutty, N., Hyman, G., Coomes, O. T. (2010). The role of pasture and soybean in deforestation of the Brazilian Amazon. Environ. Res. Lett. 5 (2), 024002. doi: 10.1088/1748-9326/5/2/024002
Chen, P., Du, Q., Liu, X., Zhou, L., Hussain, S., Lei, L., et al. (2017). Effects of reduced nitrogen inputs on crop yield and nitrogen use efficiency in a long-term maize-soybean relay strip intercropping system. PLoS One 12 (9), e0184503. doi: 10.1371/journal.pone.0184503
Cooper, P., Gregory, P., Keatinge, J., Brown, S. (1987). Effects of fertilizer, variety and location on barley production under rainfed conditions in Northern Syria 2. Soil water dynamics and crop water use. Field Crop. Res. 16 (1), 67–84.
Cui, Y., Ning, S., Jin, J., Jiang, S., Zhou, Y., Wu, C., et al (2020). Quantitative lasting effects of drought stress at a growth stage on soybean evapotranspiration and aboveground BIOMASS. Water 13 (1), 18.
Dalal, R. (1974). Effects of intercropping maize with pigeon peas on grain yield and nutrient uptake. Agriculture 10 (3), 219–224. doi: 10.1017/S0014479700000454
Du, J.-b., Han, T.-f., Gai, J.-y., Yong, T.-w., Sun, X., Wang, X.-c., et al. (2017). Maize-soybean strip intercropping: Achieved a balance between high productivity and sustainability. J. Integr. Agric. 16, 60345–60347. doi: 10.1016/S2095-3119(17)61789-1
Feng, L. Y., Raza, M. A., Chen, Y., Khalid, M. H. B., Meraj, T. A., Ahsan, F., et al. (2019). Narrow-wide row planting pattern improves the light environment and seed yields of intercrop species in relay intercropping system. PLoS One 14 (2), e0212885. doi: 10.1371/journal.pone.0212885
Feng, L. Y., Raza, M. A., Shi, J., Ansar, M., Titriku, J. K., Meraj, T. A., et al. (2020). Delayed maize leaf senescence increases the land equivalent ratio of maize soybean relay intercropping system. Eur. J. Agronomy. 118, 126092. doi: 10.1016/j.eja.2020.126092
Gao, Y., Duan, A., Sun, J., Li, F., Liu, Z., Liu, H., et al. (2009). Crop coefficient and water-use efficiency of winter wheat/spring maize strip intercropping. Field Crops Res. 111 (1-2), 65–73. doi: 10.1016/j.fcr.2008.10.007
Gao, Y., Duan, A., Qiu, X., Sun, J., Zhang, J., Liu, H., et al (2010). Distribution and use efficiency of photosynthetically active radiation in strip intercropping of maize and soybean. Agron. J. 102 (4), 1149–1157.
Gitari, H. I., Gachene, C. K., Karanja, N. N., Kamau, S., Nyawade, S., Sharma, K., et al. (2018). Optimizing yield and economic returns of rain-fed potato (Solanum tuberosum l.) through water conservation under potato-legume intercropping systems. Agric. Water Manag. 208, 59–66. doi: 10.1016/j.agwat.2018.06.005
Gou, F., van Ittersum, M. K., Wang, G., van der Putten, P. E., van der Werf, W. (2016). Yield and yield components of wheat and maize in wheat–maize intercropping in the Netherlands. Eur. J. Agronomy. 76, 17–27. doi: 10.1016/j.eja.2016.01.005
Hauggaard-Nielsen, H., Ambus, P., Jensen, E. S. (2001). Interspecific competition, n use and interference with weeds in pea–barley intercropping. Field Crops Res. 70 (2), 101–109. doi: 10.1016/S0378-4290(01)00126-5
Hauggaard-Nielsen, H., Jensen, E. S. (2005). Facilitative root interactions in intercrops, in: Root physiology: from gene to function. Springer 4, 237–250. doi: 10.1007/1-4020-4099-7_13
Ijoyah, M., Fanen, F. (2012). Effects of different cropping pattern on performance of maize-soybean mixture in makurdi, Nigeria. Sci. J. Crop Sci. 1 (2), 39–47.
Iqbal, N., Hussain, S., Ahmed, Z., Yang, F., Wang, X., Liu, W., et al. (2018). Comparative analysis of maize-soybean strip intercropping systems. a review. Plant Product. Sci 22 (2), 131–142. doi: 10.1080/1343943X.2018.1541137
Li, C., Hoffland, E., Kuyper, T. W., Yu, Y., Zhang, C., Li, H., et al. (2020). Syndromes of production in intercropping impact yield gains. Nat. Plants 6, 1–8. doi: 10.1038/s41477-020-0680-9
Lindquist, J. L., Arkebauer, T. J., Walters, D. T., Cassman, K. G., Dobermann, A. J. A. J. (2005). Maize radiation use efficiency under optimal growth conditions. Weed Res. 97 (1), 72–78. doi: 10.2134/agronj2005.0072
Li, L., Sun, J., Zhang, F., Li, X., Rengel, Z., Yang, S. (2001). Wheat/maize or wheat/soybean strip intercropping: II. Recovery Compensat. maize Soybean after wheat Harvest. Field Crops Res. 71 (3), 173–181. doi: 10.1016/S0378-4290(01)00157-5
Liu, X., Rahman, T., Song, C., Su, B., Yang, F., Yong, T., et al. (2017). Changes in light environment, morphology, growth and yield of soybean in maize-soybean intercropping systems. Field Crops Res. 200, 38–46. doi: 10.1016/j.fcr.2016.10.003
Li, H., Zhao, C., Huang, W., Yang, G. (2013). Non-uniform vertical nitrogen distribution within plant canopy and its estimation by remote sensing: A review. Field Crops Res. 142, 75–84. doi: 10.1016/j.fcr.2012.11.017
Liu, X., Rahman, T., Song, C., Yang, F., Su, B., Cui, L., et al. (2018). Relationships among light distribution, radiation use efficiency and land equivalent ratio in maize-soybean strip intercropping. Field Crop. Res. 224, 91–101. doi: 10.1016/j.fcr.2012.09.019
Mao, L., Zhang, L., Li, W., van der Werf, W., Sun, J., Spiertz, H., et al. (2012). Yield advantage and water saving in maize/pea intercrop. Field Crop. Res. 138, 11–20. doi: 10.1016/j.fcr.2012.09.019
Martin-Guay, M.-O., Paquette, A., Dupras, J., Rivest, D. (2018). The new green revolution: Sustainable intensification of agriculture by intercropping. Sci. Tot. Env. 615, 767–772. doi: 10.1016/j.scitotenv.2017.10.024
Monteith, J. L. (1977). Climate and the efficiency of crop production in Britain. Biol. Sci. 281 (980), 277–294.
Monteith, J., Elston, J. (1983). “Performance and productivity of foliage in the field,” in Growth and functioning of leaves: Proceedings of a symposium held prior to the 13th international botanical congress at the university of Sydney, 18-20 august 1981. Eds. Dale, J. E., Milthorpe, F. L. (Cambridge: Cambridge University Press), 1983.
Muoneke, C., Ogwuche, M., Kalu, B. A. (2007). Effect of maize planting density on the performance of maize/soybean intercropping system in a guinea savannah agroecosystem. Afr. J. Agric. Res. 2 (12), 667–677.
Muurinen, S., Peltonen-Sainio, P. (2006). Radiation-use efficiency of modern and old spring cereal cultivars and its response to nitrogen in northern growing conditions. Field Crop Res. 96 (2-3), 363–373. doi: 10.1016/j.fcr.2005.08.009
Ofori, F., Stern, W. (1987). Cereal–legume intercropping systems. Advanced Agronomy 41, 41–90. doi: 10.1016/S0065-2113(08)60802-0
Prasad, R., Brook, R. (2005). Effect of varying maize densities on intercropped maize and soybean in Nepal. Experimental Agriculture 41 (3), 365–382. doi: 10.1017/S0014479705002693
Piepho, H. P. (1998). Methods for comparing the yield stability of cropping systems. J. Agron. Crop Sci. 180 (4), 193–213.
Raza, M. A., Cui, L., Khan, I., Din, A. M. U., Chen, G., Ansar, M., et al. (2021a). Compact maize canopy improves radiation use efficiency and grain yield of maize/soybean relay intercropping system. Environmental Science and Pollution Research 28 (30), 41135–41148. doi: 10.1007/s11356-021-13541-1
Raza, M. A., Cui, L., Qin, R., Yang, F., Yang, W. J. S. R. (2020). Strip-width determines competitive strengths and grain yields of intercrop species in relay intercropping system. Sci. Rep. 10 (1), 1–12. doi: 10.1038/s41598-020-78719-y
Raza, M., Feng, L., Iqbal, N., Manaf, A., Khalid, M., Wasaya, A., et al. (2018). Effect of sulphur application on photosynthesis and biomass accumulation of sesame varieties under rainfed conditions. Agronomy 8 (8), 149. doi: 10.3390/agronomy8080149
Raza, M. A., Gul, H., Wang, J., Yasin, H. S., Qin, R., Khalid, M. H. B., et al. (2021b). Land productivity and water use efficiency of maize-soybean strip intercropping systems in semi-arid areas: A case study in punjab province, Pakistan. Journal of Cleaner Production 308, 127282. doi: 10.1016/j.jclepro.2021.127282
Raza, M. A., Khalid, M. H. B., Zhang, X., Feng, L. Y., Khan, I., Hassan, M. J., et al. (2019). Effect of planting patterns on yield, nutrient accumulation and distribution in maize and soybean under relay intercropping systems. Sci. Rep. 9 (1), 4947. doi: 10.1038/s41598-019-41364-1
Ren, Y., Liu, J., Wang, Z., Zhang, S. (2016). Planting density and sowing proportions of maize–soybean intercrops affected competitive interactions and water-use efficiencies on the loess plateau, China. Eur. J. Agron. 72, 70–79. doi: 10.1016/j.eja.2015.10.001
Ren, J., Zhang, L., Duan, Y., Zhang, J., Evers, J.B., Zhang, Y., et al. (2019). Intercropping potato (Solanum tuberosum L.) with hairy vetch (Vicia villosa) increases water use efficiency in dry conditions. Field Crops Res. 240, 168–176.
Rahman, T., Liu, X., Hussain, S., Ahmed, S., Chen, G., Yang, F., et al. (2017). Water use efficiency and evapotranspiration in maize-soybean relay strip intercrop systems as affected by planting geometries. PloS One 12 (6), e0178332.
Sinclair, T. R., Muchow, R. C. (1999). Radiation use efficiency. Radiat. Use efficiency 65, 215–265. doi: 10.1016/S0065-2113(08)60914-1
Szeicz, G. J. (1974). Solar radiation in crop canopies. J. Appl. Ecol. 1, 1117–1156. doi: 10.2307/2401769
Tesfaye, K., Walker, S., Tsubo, M. (2006). Radiation interception and radiation use efficiency of three grain legumes under water deficit conditions in a semi-arid environment. Eur. J. Agron. 25 (1), 60–70. doi: 10.1016/j.eja.2006.04.014
Thornton, P. K., Ericksen, P. J., Herrero, M., Challinor, A. J. (2014). Climate variability and vulnerability to climate change: A review. Global Change Biol. 20 (11), 3313–3328. doi: 10.1111/gcb.12581
Wallace, J. S. (2000). Increasing agricultural water use efficiency to meet future food production. Agric. Ecosyst. Environ. 82 (1–3), 105–119.
Willey, R., Osiru, D. (1972). Studies on mixtures of maize and beans (Phaseolus vulgaris) with particular reference to plant population. J. Agric. Sci 79 (3), 517–529. doi: 10.1017/S0021859600025909
Yang, F., Liao, D., Wu, X., Gao, R., Fan, Y., Raza, M. A., et al. (2017). Effect of aboveground and belowground interactions on the intercrop yields in maize-soybean relay intercropping systems. Field Crops Res. 203, 16–23. doi: 10.1016/j.fcr.2016.12.007
Yang, F., Lou, Y., Liao, D., Gao, R., Yong, T., Wang, X., et al. (2015). Effects of row spacing on crop biomass, root morphology and yield in maize-soybean relay strip intercropping system. Acta Agron. Sin. 41, 642–650. doi: 10.3724/SP.J.1006.2015.00642
Zhang, L., Hu, Z., Fan, J., Zhou, D., Tang, F. (2014). A meta-analysis of the canopy light extinction coefficient in terrestrial ecosystems. Frontiers of Earth Sciences 8 (4), 599–609. doi: 10.1007/s11707-014-0446-7
Zhang, H., Oweis, T. Y., Garabet, S., Pala, M. (1998). Water-use efficiency and transpiration efficiency of wheat under rain-fed conditions and supplemental irrigation in a Mediterranean-type environment. Plant Soil. 201 (2), 295–305. doi: 10.1023/A:1004328004860
Zhang, J. (2007). Barriers to water markets in the Heihe River basin in northwest China. Agricultural Water Management 87, 32–40.
Keywords: land productivity, water use efficiency, competition, sustainability, economic profit
Citation: Raza MA, Yasin HS, Gul H, Qin R, Mohi Ud Din A, Khalid MHB, Hussain S, Gitari H, Saeed A, Wang J, Rezaei-Chiyaneh E, Sabagh AE, Manzoor A, Fatima A, Ahmad S, Yang F, Skalicky M and Yang W (2022) Maize/soybean strip intercropping produces higher crop yields and saves water under semi-arid conditions. Front. Plant Sci. 13:1006720. doi: 10.3389/fpls.2022.1006720
Received: 29 July 2022; Accepted: 03 October 2022;
Published: 01 November 2022.
Edited by:
Hans-Peter Kaul, University of Natural Resources and Life Sciences Vienna, AustriaReviewed by:
Nasir Iqbal, University of Adelaide, AustraliaSabin Saurav Pokharel, Nanjing Agricultural University, China
Copyright © 2022 Raza, Yasin, Gul, Qin, Mohi Ud Din, Khalid, Hussain, Gitari, Saeed, Wang, Rezaei-Chiyaneh, Sabagh, Manzoor, Fatima, Ahmad, Yang, Skalicky and Yang. This is an open-access article distributed under the terms of the Creative Commons Attribution License (CC BY). The use, distribution or reproduction in other forums is permitted, provided the original author(s) and the copyright owner(s) are credited and that the original publication in this journal is cited, in accordance with accepted academic practice. No use, distribution or reproduction is permitted which does not comply with these terms.
*Correspondence: Milan Skalicky, skalicky@af.czu.cz; Wenyu Yang, mssiyangwy@sicau.edu.cn
†These authors share first authorship