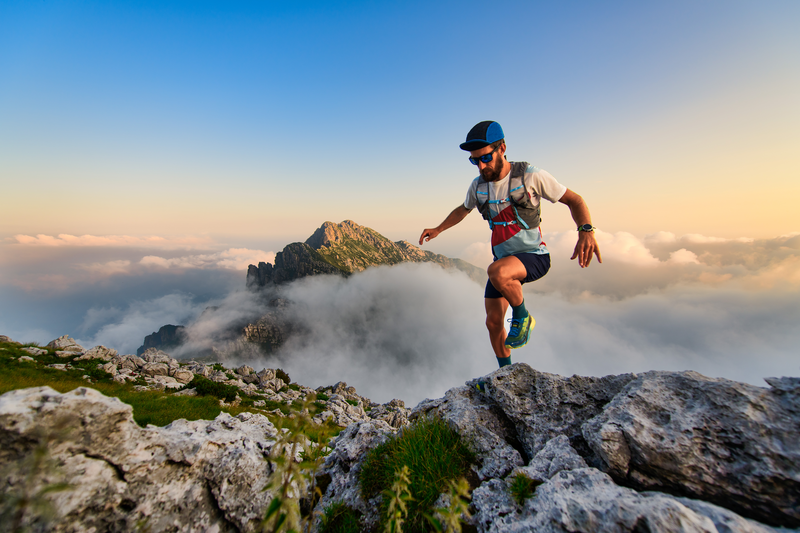
94% of researchers rate our articles as excellent or good
Learn more about the work of our research integrity team to safeguard the quality of each article we publish.
Find out more
ORIGINAL RESEARCH article
Front. Plant Sci. , 11 November 2022
Sec. Plant Metabolism and Chemodiversity
Volume 13 - 2022 | https://doi.org/10.3389/fpls.2022.1006303
This article is part of the Research Topic Biosynthesis, Purification, Characterization and Uses of Natural Compounds in Plants View all 6 articles
Sabina chinensis is a woody plant with important ecological functions in different regions of China, but its essential oils (EO) against plant pathogenic fungi remain largely undetermined. The purpose of our study was to assess the chemical composition and antifungal activity of S. chinensis EO based on optimization of the extraction process. In this study, an actionable and effective model with the experimental results and identified optimum conditions (crushing degree of 20 mesh, liquid–solid ratio of 10.1:1, immersion time of 9.1 h) was established successfully to achieve an extraction yield of 0.54%, which was basically consistent with the theoretical value. A total of 26 compounds were identified using headspace gas chromatography–mass spectrometry (GC–MS) and showed that the major constituent was β-phellandrene (26.64-39.26%), followed by terpinen-4-ol (6.53-11.89%), bornyl acetate (6.13-10.53%), etc. For Petri plate assays, our experiments found for the first time that S. chinensis EO revealed high and long-term antifungal activity against the tested strains, including Fusarium oxysporum and Fusarium incarnatum, at EC50 values of 1.42 and 1.15 µL/mL, which especially reached approximately 76% and 90% growth inhibition at a dose of 0.2 µL/mL, respectively. Furthermore, the antifungal activity of EO from different harvest periods showed remarkable variation. The orthogonal partial least-squares discriminant analysis (OPLS-DA) method revealed 11 metabolites with chemical marker components, and 5 of its potential antifungal activities, terpinen-4-ol, α-terpineol, α-elemol, γ-eudesmol, and bornyl acetate, were strongly correlated with the mycelial inhibition rate. In total, this study explored the antifungal activity of EO against root rot fungus as a potential fungicide and provided valuable information into developing potential products from natural agents.
Soil-borne plant diseases are increasingly causing a considerable destructive impact on agricultural production systems worldwide and increasing the incidence of soil disease with a direct huge economic loss for farmers (Wei et al., 2015; Delgado-Baquerizo et al., 2020; Yuan et al., 2020). Among these, root rot caused by fungal pathogens such as Fusarium oxysporum and Fusarium incarnatum has continued to be a primary production and quality limitation in global crop production and even the culture of herb materials in China (Chand et al., 2016; Deng et al., 2022). At present, the main method for controlling medicinal herbs and crop diseases remains highly dependent on chemical fungicides (Liu et al., 2017). However, these extensive applications of chemical fungicides cause serious pollution problems and threaten human health owing to increased inevitable exposure to fungicides from field, food, and water sources. Resistance to insecticides could result in several billion dollars in the agricultural sector annually worldwide and even limited effects due to evolving resistance (Gould et al., 2018). Moreover, biocide poisoning not only increases the risk of numerous health disorders, such as endocrine disruption, Alzheimer’s disease and cancers, in humans but also directly causes nearly 300,000 deaths worldwide (Freire et al., 2013; Yan et al., 2016; Sabarwal et al., 2018). Thus, it is urgent to find a more efficient and environmentally compatible alternative to control pathogenic microorganisms in agriculture.
In recent years, essential oils (EO) from plants against pathogenic fungi have received increased attention owing to their natural active ingredients, which have the advantages of easy degradation, low environmental pollution, high efficiency, and easy development (Dorman and Deans, 2000; Pateiro et al., 2021). Importantly, EO contain complicated components and show multiple target synergistic effects to exert antipathogen effects, so it is not easy to develop drug resistance (Hou et al., 2022). Furthermore, some constituents occurring in the EO, including carvacrol and thymol (Zhao et al., 2021), viridiflorol, pinocarveol, bornyl acetate, ledol (El Karkouri et al., 2021), citral and geraniol (López-Meneses et al., 2017), have been found to have antifungal activity. It has been previously reported that many species are important EO sources and have potent antimicrobial effects, such as Allium sativum, Foeniculum vulgare (Li et al., 2022a), Eucalyptus camaldulensis (Gakuubi et al., 2017), and Artemisia annua (Ma et al., 2019), but Sabina chinensis is relatively unknown. Of traditional Chinese plants, the S. chinensis tree is widely distributed throughout the northern region of China for air quality improvement on roadsides and parks, and its pollen also has strong allergenicity to the pollen allergic population (Cui et al., 2022). Unfortunately, the leaves of S. chinensis have not been fully utilized and developed, which wastes large biomass resources. Other previous studies have only focused on EO extracted from Cupressus sempervirens L., Juniperus chinensis, and Juniperus seravschanica, which exhibit high antibacterial activities, whereas research on inhibiting numerous root rot diseases has not been previously reported (Rguez et al., 2019; Khamis and Chai, 2021). As such, S. chinensis leaves may be a chance to search for effective alternatives in disease control and reduce future fungicide costs.
Consider the case of classical methods for hydrodistillation with simplicity in installation and ease of implementation, which are widely used in terms of experimental research and industrial scale (Wu et al., 2019). In the Chinese pharmacopoeia, the hydrodistillation process is also a recommended evaluation method to determine the EO content of drugs (e.g., Angelicae sinensis Radix., Forsythia suspensa, and Schizonepeta tenuifolia) (Commission, 2020). However, this simple heating process makes it difficult to adequately extract EO due to the complexity of plant tissues. Response surface methodology (RSM) based on combining experimental design with mathematical modelling has been widely used to process optimization methods (Bas and Boyacı, 2007). It has also been made to improve the extraction efficiency of EO, for instance, in Origanum vulgare L. and Citrus latifolia (Tan et al., 2012; Wu et al., 2019). For this purpose, this study will establish optimal extraction conditions using hydrodistillation combined with regression equations to evaluate the extraction efficiency of S. chinensis EO.
Hence, the objective of this study was to explore the optimal extraction process using the RSM method and to identify whether EO from S. chinensis leaves possesses antifungal properties. The in vitro antifungal activity of EO from different harvest periods was compared to further analyze the possible active ingredient and antimicrobial properties using gas chromatography–mass spectrometry (GC–MS). The results identify compounds in the S. chinensis EO that have the main antifungal activity, which could potentially be used against the root rot disease of plants. This study provides new insight into the rational development of plant fungicides simultaneously.
Plants of Sabina chinensis (Linn.) Ant. var. chinensis (S. chinensis) were collected from trees grown in the herbal garden of Hebei University of Chinese Medicine in Hebei Province, China (115°19′58.05E, 38°24′27.64N). To ensure the standardization of all sample-collection conditions, fresh leaf samples were collected from mature, healthy plants, approximately 20 cm in diameter, from the bottom and middle with scissors. The leaves of S. chinensis are scaly when mature and are manually discarded in the process of growth (Kang et al., 2019). The botanical identification of the plant was performed by a botanist, Professor Yun-Sheng Zhao. A voucher specimen of the plant was deposited at our herbarium of Hebei University of Chinese Medicine under the number ZYF022364. All fresh leaf samples of S. chinensis were harvested in March, April, and May 2022 to investigate the influence of different harvest periods on the chemical composition and antifungal activity. The fresh leaves were chopped into 0.5-1 cm segments and dried within the shade under natural conditions to a water content of approximately 5-10%. Then, the dried leaf samples were packaged, labeled, and stored in the herbarium during the experiment. These fungal strains of Fusarium incarnatum and Fusarium oxysporum were obtained from Dr. Bo Zhu, Zhejiang Chinese Medicine University, Zhejiang Province, China (Wu et al., 2021). Fungal pathogens were maintained on potato dextrose agar (PDA) slants at 4°C.
EO was extracted from S. chinensis leaves by hydrodistillation, for which leaf samples were placed in a flask with a capacity of 1000 mL and extracted with reverse-osmosis (RO) water. The EO was then collected, dried with anhydrous sodium sulfate and stored at 4°C. On the basis of the preexperiment, taking the yield of EO from S. chinensis leaves as the evaluation index, a single-factor experiment was adopted to investigate the crushing degree (16, 20, 24, 30, 40), liquid–solid ratio (8:1, 10:1, 12:1, 14:1, 16:1) and immersion time (3, 6, 9, 12, 15). A Box–Behnken scheme was established by Box–Behnken Design Expert V8.0.6 software (State-Ease Inc., USA), in which the parameters under consideration varied among three levels: low, middle, or high based on single-factor experimental data (Supplementary Table 1). All experiments were performed three times. The statistical significance and regression coefficients were evaluated to test the validity of the model and estimated parameters (Tan et al., 2012).
The extraction yield of EO was calculated based on the dried weight of the leaves using the following formula (1):
where YEO is the yield of essential oils (%), WEO is the weight of the essential oils (g), and WDL is the weight of dry leaves (g).
The EO was analyzed by GC–MS using an Agilent 7890B-5977B system equipped with a headspace automatic sampler and FID detector (Agilent Technologies Inc., USA) (Yuan et al., 2021). GC separations were carried out on an Agilent HP-5 MS capillary column (30 m × 0.25 mm, 0.25 μm) with high purity hydrogen as the carrier gas (1.0 mL/min) at a splitting ratio of 1:20. The oven temperature program was as follows: 52°C held for 1 min, followed by temperature increases from 52°C to 100°C at 4°C/min and then held for 1 min. Then, it was increased from 100°C to 140°C by 10°C/min and kept for 2 min, increased from 140°C to 150°C by 2°C/min and kept for 1 min. Finally, it was increased to 200°C by 25°C/min and kept for 1 min. The detailed mass spectrometry conditions were as follows: EI source operated at 70 eV; acquisition mass range from 50 to 500 m/z; ion source temperature at 230°C; quadrupole temperature at 150°C; solvent delay of 5 min.
Each EO sample was prepared by diluting 10 times with pure N-hexane chromatography (99.99%, Aladdin, China). After drying anhydrous sodium sulfate (≥99.0%, Tianjian Yongda Chemical Co., Ltd), a precision suction of 50 μL EO was placed in 20 mL Agilent headspace glass vials. Compared to the total peak area and GC retention times, the peak area percentage of the EO constituents was detected using MassHunter Quantitative Analysis software (version B.09, Agilent). The main chemical compounds of EO were tentatively identified based on the comparison of their GC Kovats retention indices (RIs) with reference to a homologous series of C8-C20 n-alkanes (Cazella et al., 2019). The mass spectra of each compound were matched with authentic standards from the NIST17 library data and with those reported in the literature (Wu et al., 2019).
In this study, the antifungal activity of EO at different concentrations was assessed using an airtight fumigation assay with modifications (Li et al., 2020). Sterilized Petri dishes (90 mm diameter) were filled with 10 mL of PDA (potato dextrose agar) medium for preparation. Then, a 5 mm diameter pathogenic fungi cake was obtained with a hole punch at the edge of the pathogen colony and inoculated in the center. Sterile filter paper (20 mm diameter) with tweezers was placed in the center of the dish cover, and the doses of 2.5, 5, 10, 15, and 20 μL EO were removed with a pipette gun. The residual volume of each Petri dish was calculated as approximately 100 mL, and the final concentration in each dish reached 0.025, 0.05, 0.10, 0.15, and 0.20 µL/mL to evaluate the antifungal profiles. Petri dishes were inverted, sealed with parafilm, and incubated in an incubator (Yiheng Instrument Co., Ltd., Shanghai, China) at a constant temperature of 28°C for 120 h. The inhibitory antifungal activity in each treatment was recorded at 24 h intervals and measured using the mycelial growth rate method (Chutia et al., 2009) until the end of the experiment. For each treatment, three repeats were carried out. The antifungal activity of S. chinensis EO from different harvest periods was compared according to the above method. The doses of 5 μL EO from S. chinensis in March, April, and May were added to the filter paper, and the assay plates were incubated at 28°C for 120 h. The rest was the same as above.
All data are presented as the mean values and standard deviation (SD). Data were checked for normality using the Shapiro–Wilk method and by visualizing the histogram. Data on the inhibitory effect of EO on mycelial growth were subjected to analysis by one-way analysis of variance (ANOVA) using SPSS 26.0 (SPSS Inc., USA) (Moghaddam et al., 2015). Mean comparisons were made using Duncan’s multiple range tests at P< 0.05. The EC50 values with their confidence intervals were determined with GraphPad Prism 8.0.2 software. Data preprocessing is imperative to ensure that the relative content of compounds in the GC–MS data is standardized and entirely comparable using Agilent MassHunter Quantitative Analysis B.09.00 software (Li et al., 2022b). An orthogonal partial least square discriminant analysis (OPLS-DA) model was established with chemical components as variables using SIMCA-P software (version 14, Umetrics). The explanatory power of the models was evaluated by calculating the cumulative modeled variation in the marginal R2 (cum) and the predictive ability parameter Q2 (cum) (Su et al., 2021). For correlation analysis, Spearman’s correlation coefficient was calculated and analyzed with Origin 2019 software (Origin Lab, Northampton, MA, USA).
EO plays an important role in the pharmacological, food processing, biopesticide, and industrial fields because of its complicated and varied chemical composition and extensive pharmacological activities. Previous research showed that the extraction method not only affects the content and composition of EO but is also critical in enhancing process yields, understanding biological activities, and further applications, especially for the components accounted for less (Taticchi et al., 2021). Therefore, the selection and optimization of extraction parameters were first performed.
The response surface method (RSM) is a statistical comprehensive testing technique that uses deterministic experiments and multivariate quadratic regression equations to fit, which can reduce the number of tests and solve the multivariable factors (Ferreira et al., 2007). Therefore, the Box–Behnken model was used to design the experiment based on the results of the single-factor experiment. Finally, crushing degree (16, 20, 24), liquid–solid ratio (8:1, 10:11, 12:1), and immersion time (6, 9, 12) are selected as the best horizontal range. Using the Box–Behnken experimental design, 17 experimental groups of three factors and a three-level optimization model were obtained. The Box–Behnken design of each factor and level is shown in Supplementary Table 1. Our mathematical model can be expressed as the following model (2):
The statistical significance and regression coefficients were evaluated based on ANOVA with the F test and P value (Table 1). The regression equation model showed that the model was significant (P = 0.0061< 0.01). The lack-of-fit statistic was not significant (P = 0.4455 > 0.05), indicating that the model can be matched with the experimental data. The coefficient of determination (R2 = 0.9110) revealed good fitness and reliability for matching the model equations (Y) to actual measurements (yield). The p value of each model term indicated that factor (A) and interactive coefficients (A2, B2, C2) were significant and constituted the model between the interactive term and independent variables. Contour plots and response surfaces of the effect of three factors and three levels on the extraction yield of EO are shown in Figure 1. We can conclude that the crush degree had the largest effect on the yield of EO, followed by the liquid–solid ratio and immersion time (Figure 1 and Table 1). According to the mathematical model, the optimal extraction conditions of RSM were predicted as follows: crushing degree 18.95 mesh, liquid–solid ratio of 10.1:1, immersion time 9.10 h, theoretical yield 0.55%. To validate the model accuracy and feasibility, triplicate extraction experiments of process conditions were performed as follows: crushing degree 20 mesh, liquid–solid ratio of 10.1:1, immersion time 9.1 h. The actual yield of EO was 0.54%, which was basically consistent with the theoretical value, and the error was small. Overall, the design model could be effectively used to optimize the extraction efficiency for the extraction yield of S. chinensis EO.
Figure 1 Response surface plots and contour map for the interaction analyzes. (A) Crush degree and liquid–solid ratio; (B) Crush degree and immersion time; (C) Liquid–solid ratio and immersion time.
Our results showed that crushing degree was a major factor in the optimal extraction parameters of S. chinensis EO, consistent with a previous study (Gong et al., 2009). Dissolution, diffusion, and infiltration are believed to be essential for improving the extraction yield related to EO from broken plant tissue structures in hydrodistillation extraction processes (Díaz-Suárez et al., 2021). The EO yield extracted from S. chinensis leaves by optimizing the extraction parameters reached 0.54%, which was higher than that obtained by Gong et al. (2009) and slightly lower than Zhao obtained by the microwave-assisted extraction method (Zhao, 2018). This could be due to the difference in the investigated factors, such as enzymolysis temperature and time, and ultrasound-assisted extraction also affects the EO yield owing to mechanical and thermal effects that cause the rupture of plant cell walls (Vila Verde et al., 2018).
The properties against the root rot fungus strains, including F. oxysporum and F. incarnatum, of EO after inoculation with different doses and treatments are shown in Figure 2. The experimental results revealed that S. chinensis EO has a high antifungal potential against pathogenic fungi using only a low concentration to the effective concentration of inhibiting 50% of mycelial growth (EC50 values). The EO showed high efficiency against F. oxysporum with EC50 values of 1.42 µL/mL. Furthermore, the EO also showed higher antifungal activity against F. incarnatum, with an EC50 value of 1.15 µL/mL. Our study reported is consistent with previous findings, which tested the EO of Thymus vulgaris grown in Iran for in vitro antifungal activity against F. oxysporum and Drechslera spicifera (Moghaddam and Mehdizadeh, 2020). Additionally, EO inhibited mycelial growth in all tested strains selected and correlated with the effective time and dose-dependent manner. In Petri plate assays, at a higher concentration with EO at 0.2 µL/mL within 24 h, the inhibition of mycelial growth against F. incarnatum reached approximately 90%, and F. oxysporum reached 76%. The antifungal properties are more effective against F. incarnatum than F. oxysporum. It is worth noting that the EO concentration (from 0.1 to 0.2 µL/mL) within 120 h inhibited the mycelial growth of F. incarnatum by approximately 48.76% to 60.29%, but F. oxysporum reached 14.61% to 32.03%. Although the trend of antifungal activity declined with increasing treatment time, it still showed inhibitory effects. The results in this study are also similar to those of the study obtained by Parikh et al. (2021), who determined that the seven essential oils inhibited mycelial growth of all tested pathogens, including Fusarium avenaceum, by 50 to 100%. López-Anchondo et al. (2021) also reported that the EO from Prosopis glandulosa controls the growth rate reduction of Colletotrichum gloeosporoides (74.92%) and F. oxysporum (64.82%) but was less efficient against in vivo conditions. Another recent study revealed that Cumin EO induced the expression of most genes against F. oxysporum from Panax notoginseng pathogens (Huo et al., 2021), which provided a theoretical basis for controlling root diseases at the transcriptional level.
Figure 2 (A) Antifungal activity of the EO of Sabina chinensis leaves tested by the airtight fumigation method against Fusarium incarnatum (a) and Fusarium oxysporum (b); (c, d) are controls for F. incarnatum and F. oxysporum, respectively. (B) Inhibitory rate after an airtight fumigation assay test with different doses of S. chinensis EO. The inhibitory effect of EO (means ± SDs) against F. incarnatum (pink curve) and F. oxysporum (blue curve). (C, D) The percentage of inhibition with different treatment times and doses of EO. Vertical bars represent the standard errors. Different lowercase letters indicate that there are significant differences between different treatments at the same time (P< 0.05).
In the present study, 26 compounds were identified in the EO from S. chinensis leaves by GC–MS analysis (Table 2 and Figure 3). The major constituent and the relative content of the EO were β-phellandrene (26.64-39.26%), followed by terpinen-4-ol (6.53-11.89%), bornyl acetate (6.13-10.53%), α-terpinene (3.35-4.53%), and β-pinene (4.20-5.69%). Compared with previous studies, the chemical composition of EO was close, but the percentages of those constituents were different (Gong et al., 2009). Nevertheless, β-phellandrene, terpinen-4-ol and bornyl acetate were the dominant compounds in this study, and they are not major component constituents in previous reports on the EO constituent of closely related species such as Sabina chinensis cv. Kaizuca (Gu et al., 2018). Our study also found that all leaf samples from different harvesting times contained 26 volatile components, whereas their contents were different. Among these chemical components, the contents of β-phellandrene, terpinen-4-ol, α-elemene, and bornyl acetate in the EO from different harvest periods were quite different, of which the highest levels of β-phellandrene were found in May, terpinen-4-ol and α-elemol in March and bornyl acetate in April. The variability in chemical composition and content in aromatic plants may be attributed to differences in harvest periods, geographical factors, drying methods, and storage conditions (Zhao et al., 2021).
Figure 3 Total ion current chromatogram of essential oils from Sabina chinensis leaves analyzed using a gas chromatograph-mass spectrometer. Zoomed areas view insets show the chemical composition of TIC identified from March (A), April (B), and May (C) samples. Peak numbers (No.) correspond to the main compounds mentioned in Table 2.
To further determine the difference in antifungal characteristics in different harvest periods, EO in March, April and May was tested by Petri plate assays, and the antifungal effect is shown in Figure 4. As a result, the inhibition rate against F. incarnatum by March EO treatment was higher than that by May EO treatment but was not significant by April EO treatment (P > 0.05). March EO treatment significantly inhibited F. oxysporum compared with April and May EO treatment (P< 0.05). Similarly, the treatment with the April EO sample resulted in a significantly higher inhibitory effect than the May EO sample (P< 0.05). Collectively, the antifungal effect of EO was different in different harvest periods, revealing that March EO had better inhibitory mycelium growth than April and May EO. This observation is in agreement with a previous study showing that Psidium myrtoides O. Berg EO from different harvest periods has significantly different chemical constituents and antimicrobial activities (de Macêdo et al., 2020). This dynamic variation may be related to the plant development stages and response to seasonality influenced by the accumulation and transformation of secondary metabolites (Pan et al., 2021; Dias et al., 2022).
Figure 4 Antifungal activity of essential oils from different harvest periods (March, April, and May). The percent inhibition (means ± SDs) of Fusarium oxysporum (A) and Fusarium incarnatum (B). The significance symbol “*” indicates a significant difference (*, P< 0.05; **, P< 0.01; ***, P< 0.001). The symbol "ns" indicates there was no significant difference (P> 0.05).
The major and minor compounds of EO may be related to their potential individual or synergetic antifungal effects (Aimad et al., 2022). Orthogonal partial least-squares discriminant analysis (OPLS-DA) is a supervised discriminant analysis statistical method that was employed to find potential biomarkers and reflect the metabolic variations between groups. Specifically, the OPLS-DA model was established based on 26 metabolites identified as S. chinensis EO, which had a reliable explanatory and predictive ability (R2Y=0.973, Q2 = 0.937). Moreover, variable importance in projection greater than 1 (VIP>1) was calculated and considered a potential chemical marker compound (Supplementary Table S2). A total of 11 differential compounds were selected, including camphene (VIP=1.58), β-phellandrene (VIP=1.58), cyclofenchene (VIP=1.58), α-terpineol (VIP=1.51), terpinen-4-ol (VIP=1.51), β-pinene (VIP=1.46), α-thujene (VIP=1.45), rosifoliol (VIP=1.44), bornyl acetate (VIP=1.24), α-elemol (VIP=1.20), and γ-eudesmol (VIP=1.15). This identification of chemical markers exhibited a wide range of antimicrobial properties and applications in various commercial preparations, such as plant pathogens, food spoilage bacteria, and foodborne pathogens, which have been examined by many researchers (Haznedaroglu et al., 2001; Benali et al., 2020; Medina-Romero et al., 2022).
Furthermore, a correlation analysis was performed between the mycelial growth inhibition rate and compound content by screening 11 main components in S. chinensis EO (Figure 5). As shown in Figure 5A, the contents of rosifoliol, terpinen-4-ol, and α-terpineol were significantly positively correlated with the antifungal level (P< 0.05). Among these markers, previous studies found that the α-terpineol and terpinen-4-ol of major components from Artemisia species effectively inhibited Alternaria solani, reaching over 60% (Huang et al., 2019). Our findings also revealed that α-elemol and γ-eudesmol were significantly positively correlated with the antifungal level of F. oxysporum, and bornyl acetate was significantly positively correlated with the antifungal level of F. incarnatum (P< 0.05). Previous research has also demonstrated some similar findings, with α-pinene, γ-eudesmol and bornyl acetate demonstrating antifungal activity and potential bioactivity in the control of plant diseases (Sarpeleh et al., 2009; Waikedre et al., 2012; Morales-Rabanales et al., 2021). Overall, this study speculated possible key chemical components and content and antifungal activity on enhancing antimicrobial activities and suggests that EO from S. chinensis contributed to a main source of natural plant sources (Figure 5B). The important next steps will be to expand the range of tested fungal species, and the formulation of a plant-derived fungicide based on S. chinensis leaf EO will be developed by screening solvents and emulsifiers and conducting toxicity tests. Then, pot experiments and field experiments will be carried out to develop future bio fungicides from plants for soil-borne pathogenic fungi control.
Figure 5 (A) Spearman’s correlation between variable importance in projection (VIP >1) contents and antifungal activity. Blue circles represent negative correlations, and red circles represent positive correlations. Circles with red and blue represent negative and positive correlations, respectively, and sizes represent the compound contents. (B) Structures of the related components found in the essential oils of Sabina chinensis leaves: (a) terpinen-4-ol; (b) α-terpineol; (c) α-elemol; (d) γ-eudesmol; (e) bornyl acetate. The significance symbol “*” indicates a significant difference (*, P< 0.05; **, P< 0.01; ***, P< 0.001).
Developing future biofungicides from the EO of plants will most likely be a critical issue for future work. In the present study, our work is the first to extend the findings of the strong antifungal activity of S. chinensis EO against F. oxysporum and F. incarnatum by Petri plate assays. Moreover, the chemical composition, contents and potential antifungal activity of three different harvest periods of EO were evaluated. It was of interest to note that the main components of EO, such as terpinen-4-ol and α-terpineol, exhibited significant antifungal activity. In summary, S. chinensis EO, which has been shown to have significant antifungal potential, is worth further exploration as a source of promising natural agents in soil-borne disease control and environmentally friendly agriculture.
The original contributions presented in the study are included in the article/Supplementary Material. Further inquiries can be directed to the corresponding authors.
HF, XG, JZ, and ZZ conceived the project and designed the experiments. JZ and ZZ prepared the original draft preparation. WL and JB performed the data analyzes. YZ reviewed and edited the manuscript. HF and XG contributed to supervision, data validation, project administration, and funding acquisition. All authors contributed to the article and approved the submitted version.
This research was supported financially by the scientific research project of the Hebei Administration of Traditional Chinese Medicine, China (Grant No. 2022098, 2022365), the Doctoral Research Foundation of Hebei University of Chinese Medicine, China (BSZ2020007, BSZ2021019), the Modern Agricultural Technology Innovation Team Project in Hebei Province, China (HBCT2018060205), the Basic Scientific Research Foundation of Hebei University of Chinese Medicine (JCYJ202206), the Traditional Chinese Medicine Resources Survey Project of China (Z135080000022), and the scientific research project of the Natural Science Foundation of Hebei Province, China (H2022423004).
We are grateful to the teachers, administrators, and students at the Traditional Chinese Medicine Processing Technology Innovation Center of Hebei Province for their support and cooperation in this project.
The authors declare that the research was conducted in the absence of any commercial or financial relationships that could be construed as a potential conflict of interest.
All claims expressed in this article are solely those of the authors and do not necessarily represent those of their affiliated organizations, or those of the publisher, the editors and the reviewers. Any product that may be evaluated in this article, or claim that may be made by its manufacturer, is not guaranteed or endorsed by the publisher.
The Supplementary Material for this article can be found online at: https://www.frontiersin.org/articles/10.3389/fpls.2022.1006303/full#supplementary-material
Aimad, A., Youness, E. A., Sanae, R., Moussaoui, E. A., Bourhia, M., Salamatullah, A. M., et al. (2022). Chemical composition and antifungal, insecticidal and repellent activity of essential oils from Origanum compactum benth. used in the mediterranean diet. Front. Plant Sci. 13. doi: 10.3389/fpls.2022.798259
Bas, D., Boyacı, I. H. (2007). Modeling and optimization I: Usability of response surface methodology. J. Food Eng 78, 836–845. doi: 10.1016/j.jfoodeng.2005.11.024
Benali, T., Habbadi, K., Khabbach, A., Marmouzi, I., Zengin, G., Bouyahya, A., et al. (2020). GC-MS analysis, antioxidant and antimicrobial activities of Achillea odorata subsp. Pectinata and Ruta montana assential oils and their potential use as food preservatives. Foods 9, 668. doi: 10.3390/foods9050668
Cazella, L. N., Glamoclija, J., Soković, M., Gonçalves, J. E., Linde, G. A., Colauto, N. B., et al. (2019). Antimicrobial activity of essential oil of Baccharis dracunculifolia DC (Asteraceae) aerial parts at flowering period. Front. Plant Sci. 10. doi: 10.3389/fpls.2019.00027
Chand, S. K., Nanda, S., Joshi, R. K. (2016). Regulation of miR394 in response to Fusarium oxysporum f. sp. cepae (FOC) infection in garlic (Allium sativum l). Front. Plant Sci. 7. doi: 10.3389/fpls.2016.00258
Chutia, M., Bhuyan, P. D., Pathak, M. G., Sarma, T. C., Boruah, P. (2009). Antifungal activity and chemical composition of Citrus reticulata blanco essential oil against phytopathogens from north East India. Lwt-Food Sci. Technol. 42, 777–780. doi: 10.1016/j.lwt.2008.09.015
Commission, C. P. (2020). Pharmacopoeia of the people’s republic of China (Beijing: People’s Medical Publishing House).
Cui, N., Qu, L. Y., Wu, G. (2022). Heavy metal accumulation characteristics and physiological response of Sabina chinensis and Platycladus orientalis to atmospheric pollution. J. Environ. Sci. (China) 112, 192–201. doi: 10.1016/j.jes.2021.05.013
Delgado-Baquerizo, M., Guerra, C. A., Cano-Díaz, C., Egidi, E., Wang, J. T., Eisenhauer, N., et al. (2020). The proportion of soil-borne pathogens increases with warming at the global scale. Nat. Clim. Change 10, 550–554. doi: 10.1038/s41558-020-0759-3
de Macêdo, D. G., de Almeida Souza, M. M., Morais-Braga, M. F. B., Coutinho, H. D. M., dos Santos, A. T. L., Machado, A. J. T., et al. (2020). Seasonality influence on the chemical composition and antifungal activity of Psidium myrtoides O. Berg S Afr J. Bot. 128, 9–17. doi: 10.1016/j.sajb.2019.10.009
Deng, X. H., Zhang, N., Li, Y. C., Zhu, C. Z., Qu, B. Y., Liu, H. J., et al. (2022). Bio-organic soil amendment promotes the suppression of Ralstonia solanacearum by inducing changes in the functionality and composition of rhizosphere bacterial communities. New Phytol. 235, 1558–1574. doi: 10.1111/nph.18221
Dias, A., Batista, H., Sousa, W. C., Bailão, E., Rocha, J. D., Sperandio, E. M., et al. (2022). Psidium myrtoides o. berg fruit and leaves: physicochemical characteristics, antifungal activity and chemical composition of their essential oils in different seasons. Nat. Prod. Res. 36, 1043–1047. doi: 10.1080/14786419.2020.1844689
Díaz-Suárez, P., Rosales-Quintero, A., Fernandez-Lafuente, R., Pola-Sánchez, E., Hernández-Cruz, M. C., Ovando-Chacón, S. L., et al. (2021). Aqueous enzymatic extraction of Ricinus communis seeds oil using viscozyme l. Ind. Crops Prod. 170, 113811. doi: 10.1016/j.indcrop.2021.113811
Dorman, H. J. D., Deans, S. G. (2000). Antimicrobial agents from plants: antibacterial activity of plant volatile oils. J. Appl. Microbiol. 88, 308–316. doi: 10.1046/j.1365-2672.2000.00969.x
El Karkouri, J., Bouhrim, M., Al Kamaly, O. M., Mechchate, H., Kchibale, A., Adadi, I., et al. (2021). Chemical composition, antibacterial and antifungal activity of the essential oil from Cistus ladanifer l. Plants 10, 2068. doi: 10.3390/plants10102068
Ferreira, S. L. C., Bruns, R. E., Ferreira, H. S., Matos, G. D., David, J. M., Brandão, G. C., et al. (2007). Box-behnken design: an alternative for the optimization of analytical methods. Anal. Chim. Acta 597, 179–186. doi: 10.1016/j.aca.2007.07.011
Freire, C., Koifman, R. J., Sarcinelli, P. N., Simões Rosa, A. C., Clapauch, R., Koifman, S. (2013). Long-term exposure to organochlorine pesticides and thyroid status in adults in a heavily contaminated area in Brazil. Environ. Res. 127, 7–15. doi: 10.1016/j.envres.2013.09.001
Gakuubi, M. M., Maina, A. W., Wagacha, J. M. (2017). Antifungal activity of essential oil of Eucalyptus camaldulensis dehnh. against selected Fusarium spp. Int. J. Microbiol. 2017, 8761610. doi: 10.1155/2017/8761610
Gong, Y. L., Jin, H., Wang, H. B. (2009). Study on extraction technique and antibacterial activity for essential oil from Platycladus orientalis. Chem. Bioengineering (China) 26, 36–38. doi: 10.3969/j.issn.1672-5425.2009.02.010
Gould, F., Brown, Z. S., Kuzma, J. (2018). Wicked evolution: Can we address the sociobiological dilemma of pesticide resistance? Science 360, 728–732. doi: 10.1126/science.aar3780
Gu, D. Y., Fang, C., Yang, J., Li, M. J., Liu, H. M., Yang, Y. (2018). Chemical composition and α-amylase inhibitory activity of the essential oil from Sabina chinensis cv. kaizuca leaves. Nat. Prod Res. 32, 711–713. doi: 10.1080/14786419.2017.1332612
Haznedaroglu, M. Z., Karabay, N. U., Zeybek, U. (2001). Antibacterial activity of Salvia tomentosa essential oil. Fitoterapia 72, 829–831. doi: 10.1016/s0367-326x(01)00335-5
Hou, T. Y., Sana, S. S., Li, H. Z., Xing, Y., Nanda, A., Netala, V. R., et al. (2022). Essential oils and its antibacterial, antifungal and anti-oxidant activity applications: A review. Food Bioscience 47, 101716. doi: 10.1016/j.fbio.2022.101716
Huang, X., Chen, S. Y., Zhang, Y., Wang, Y. H., Zhang, X., Bi, Z. Y., et al. (2019). Chemical composition and antifungal activity of essential oils from three Artemisia species against Alternaria solani. J. Essent. Oil Bear Plants 22, 1581–1592. doi: 10.1080/0972060X.2019.1708812
Huo, Y. Y., Li, T. T., Yang, J., Huang, H. Y., Chen, C. J., Xu, F. R., et al. (2021). Chemical constituents of the essential oil from Cuminum cyminum l. and its antifungal activity against Panax notoginseng pathogens. Chem. Biodivers. 18, e2100638. doi: 10.1002/cbdv.202100638
Kang, Y., Li, S. Y., Sun, X. Y., Gong, X. Q., Yu, K. F., Cai, L. L., et al. (2019). Study on screening, identification and capability of lignin-degrading fungi for landscaping waste. For. Res. 32, 80–87. doi: 10.13275/j.cnki.lykxyj.2019.03.011
Khamis, A. S., Chai, L. C. (2021). Chemical and antimicrobial analyses of Juniperus chinensis and Juniperus seravschanica essential oils and comparison with their methanolic crude extracts. Int. J. Anal. Chem. 2021, 9937522. doi: 10.1155/2021/9937522
Li, W., Li, J., Qin, Z., Wang, Y., Zhao, P., Gao, H. (2022b). Insights into the composition and antibacterial activity of Amomum tsao-ko essential oils from different regions based on GC-MS and GC-IMS. Foods 11, 1402. doi: 10.3390/foods11101402
Li, Y. B., Liu, Y. X., Zhang, Z. P., Cao, Y. S., Li, J. Q., Luo, L. X. (2020). Allyl isothiocyanate (AITC) triggered toxicity and FsYvc1 (a STRPC family member) responded sense in Fusarium solani. Front. Microbiol. 11. doi: 10.3389/fmicb.2020.00870
Liu, X., Xu, G. C., Wang, Q. S., Hang, Y. H. (2017). Effects of insect-proof net cultivation, rice-duck farming, and organic matter return on rice dry matter accumulation and nitrogen utilization. Front. Plant Sci. 8. doi: 10.3389/fpls.2017.00047
Li, T. T., Yang, J., Huo, Y. Y., Zeng, Z. Y., Huang, H. Y., Xu, F. R., et al. (2022a). Control of pathogenic fungi on panax notoginseng by volatile oils from the food ingredients Allium sativum and Foeniculum vulgare. Lett. Appl. Microbiol. 75, 89–102. doi: 10.1111/lam.13706
López-Anchondo, A. N., Lopez-de la Cruz, D., Gutiérrez-Reyes, E., Castañeda-Ramírez, J. C., De la Fuente-Salcedo, N. M. (2021). Antifungal activity in vitro and in vivo of mesquite extract (Prosopis glandulosa) against phytopathogenic fungi. Indian J. Microbiol. 61, 85–90. doi: 10.1007/s12088-020-00906-2
López-Meneses, A. K., Sánchez-Mariñez, R. I., Quintana-Obregón, E. A., Parra-Vergara, N. V., González-Aguilar, G. A., López-Saiz, C. M., et al. (2017). In vitro antifungal activity of essential oils and major components against fungi plant pathogens. J. Phytopathol. 165, 232–237. doi: 10.1111/jph.12554
Ma, Y. N., Chen, C. J., Li, Q. Q., Xu, F. R., Cheng, Y. X., Dong, X. (2019). Monitoring antifungal agents of Artemisia annua against Fusarium oxysporum and Fusarium solani, associated with Panax notoginseng root-rot disease. Molecules 24, 213. doi: 10.3390/molecules24010213
Medina-Romero, Y. M., Rodriguez-Canales, M., Rodriguez-Monroy, M. A., Hernandez-Hernandez, A. B., Delgado-Buenrostro, N. L., Chirino, Y. I., et al. (2022). Effect of the essential oils of Bursera morelensis and Lippia graveolens and five pure compounds on the mycelium, spore production, and germination of species of Fusarium. J. Fungi 8, 617. doi: 10.3390/jof8060617
Moghaddam, M., Mehdizadeh, L. (2020). Chemical composition and antifungal activity of essential oil of Thymus vulgaris grown in Iran against some plant pathogenic fungi. J. Essent. Oil Bear Plants 23, 1072–1083. doi: 10.1080/0972060X.2020.1843547
Moghaddam, M., Taheri, P., Pirbalouti, A. G., Mehdizadeh, L. (2015). Chemical composition and antifungal activity of essential oil from the seed of Echinophora platyloba DC. against phytopathogens fungi by two different screening methods. LWT Food Sci. Technol. 61, 536–542. doi: 10.1016/j.lwt.2014.12.008
Morales-Rabanales, Q. N., Coyotl-Pérez, W. A., Rubio-Rosas, E., Cortes-Ramírez, G. S., Ramírez, J. F. S., Villa-Ruano, N. (2021). Antifungal properties of hybrid films containing the essential oil of Schinus molle: Protective effect against postharvest rot of tomato. Food Control 134, 108766. doi: 10.1016/j.foodcont.2021.108766
Pan, X., Li, H. Y., Chen, D. F., Zheng, J. J., Yin, L. H., Zou, J., et al. (2021). Comparison of essential oils of Houttuynia cordata thunb. from different processing methods and harvest seasons based on GC-MS and chemometric analysis. Int. J. Anal. Chem. 2021, 8324169. doi: 10.1155/2021/8324169
Parikh, L., Agindotan, B. O., Burrows, M. E. (2021). Antifungal activity of plant-derived essential oils on pathogens of pulse crops. Plant Dis. 105, 1692–1701. doi: 10.1094/PDIS-06-20-1401-RE
Pateiro, M., Munekata, P. E. S., Sant'Ana, A. S., Domínguez, R., Rodríguez-Lázaro, D., Lorenzo, J. M. (2021). Application of essential oils as antimicrobial agents against spoilage and pathogenic microorganisms in meat products. Int. J. Food Microbiol. 337, 108966. doi: 10.1016/j.ijfoodmicro.2020.108966
Rguez, S., Essid, R., Adele, P., Msaada, K., Hammami, M., Mkadmini, K., et al. (2019). Towards the use of Cupressus sempervirens l. organic extracts as a source of antioxidant, antibacterial and antileishmanial biomolecules. Ind. Crops Prod. 131, 194–202. doi: 10.1016/j.indcrop.2019.01.056
Sabarwal, A., Kumar, K., Singh, R. P. (2018). Hazardous effects of chemical pesticides on human health-cancer and other associated disorders. Environ. Toxicol. Pharmacol. 63, 103–114. doi: 10.1016/j.etap.2018.08.018
Sarpeleh, A., Sharifi, K., Sonbolkar, A. (2009). Evidence of antifungal activity of wild rue (Peganum harmala l.) on phytopathogenic fungi. J. Plant Dis. Prot. 116, 208–213. doi: 10.1007/BF03356312
Su, Y., Renz, M., Cui, B., Sun, X., Ouyang, Z., Wang, X. (2021). Leaf morphological and nutrient traits of common woody plants change along the urban-rural gradient in Beijing, China. Front. Plant Sci. 12. doi: 10.3389/fpls.2021.682274
Tan, Q., Thi Kieu, X., Thi Kim, N., Thi Hong., X. (2012). Application of response surface methodology (rsm) in condition optimization for essential oil production from citrus latifolia. Emir J. Food Agr 24, 25–30. doi: 10.9755/ejfa.v24i1.10595
Taticchi, A., Esposto, S., Veneziani, G., Minnocci, A., Urbani, S., Selvaggini, R., et al. (2021). High vacuum-assisted extraction affects virgin olive oil quality: Impact on phenolic and volatile compounds. Food Chem. 342, 128369. doi: 10.1016/j.foodchem.2020.128369
Vila Verde, G. M., Barros, D. A., Oliveira, M. S., Aquino, G. L. B., Santos, D. M., De Paula, J. R., et al. (2018). A green protocol for microwave-assisted extraction of volatile oil terpenes from Pterodon emarginatus vogel. (Fabaceae). Molecules 23, 651. doi: 10.3390/molecules23030651
Waikedre, J., Vitturo, C. I., Molina, A., Theodoro, P. N. E. T., Silva, M. D. R. R., Espindola, L. S., et al. (2012). Antifungal activity of the essential oils of Callitris neocaledonica and C. sulcata heartwood (Cupressaceae). Chem. Biodivers. 9, 644–653. doi: 10.1002/cbdv.201100229
Wei, Z., Yang, T. J., Friman, V. P., Xu, Y. C., Shen, Q. R., Jousset, A. (2015). Trophic network architecture of root-associated bacterial communities determines pathogen invasion and plant health. Nat. Commun. 6, 8413. doi: 10.1038/ncomms9413
Wu, W., Liu, S. Y., Wang, S. Y., Qin, L. P., Zhu, B. (2021). Isolation and identification of Atractylodes macrocephala endophytic bacteria AM14 and its biological control for host plant root-rot disease. Chin. Traditional Herbal Drugs (China) 52 (10), 3075–3080. doi: 10.7501/j.issn.0253-2670.2021.10.025
Wu, Z. F., Xie, L. Z., Li, Y. H., Wang, Y. Q., Wang, X. C., Wan, N., et al. (2019). A novel application of the vacuum distillation technology in extracting Origanum vulgare l. essential oils. Ind. Crops Prod. 139, 111516. doi: 10.1016/j.indcrop.2019.111516
Yan, D., Zhang, Y. J., Liu, L. G., Yan, H. (2016). Pesticide exposure and risk of alzheimer’s disease: a systematic review and meta-analysis. Sci. Rep. 6, 32222. doi: 10.1038/srep32222
Yuan, F., Fu, X., Yu, X., Yang, Q., Jin, H., Zhu, L. (2021). Comparative analysis and development of a flavor fingerprint for volatile compounds of vegetable soybean seeds based on headspace-gas chromatography-ion mobility spectrometry. Front. Plant Sci. 12. doi: 10.3389/fpls.2021.768675
Yuan, J., Wen, T., Zhang, H., Zhao, M. L., Penton, C. R., Thomashow, L. S., et al. (2020). Predicting disease occurrence with high accuracy based on soil macroecological patterns of Fusarium wilt. ISME J. 14, 2936–2950. doi: 10.1038/s41396-020-0720-5
Zhao, J. Q. (2018). The extraction and antibacterial affect of cupressus funebris and Sabina chinensis (Sichuan: Sichuan Agricultural University). Available at: https://d.wanfangdata.com.cn/thesis/Y3494278.
Keywords: Sabina chinensis, essential oil, response surface methodology, gas chromatography-mass spectrometry, antifungal activity, soil-borne disease
Citation: Zhang J, Zhao Z, Liang W, Bi J, Zheng Y, Gu X and Fang H (2022) Essential oil from Sabina chinensis leaves: A promising green control agent against Fusarium sp.. Front. Plant Sci. 13:1006303. doi: 10.3389/fpls.2022.1006303
Received: 29 July 2022; Accepted: 28 October 2022;
Published: 11 November 2022.
Edited by:
Monica Butnariu, Banat University of Agricultural Sciences and Veterinary Medicine, RomaniaReviewed by:
Ericsson Coy-Barrera, Universidad Militar Nueva Granada, ColombiaCopyright © 2022 Zhang, Zhao, Liang, Bi, Zheng, Gu and Fang. This is an open-access article distributed under the terms of the Creative Commons Attribution License (CC BY). The use, distribution or reproduction in other forums is permitted, provided the original author(s) and the copyright owner(s) are credited and that the original publication in this journal is cited, in accordance with accepted academic practice. No use, distribution or reproduction is permitted which does not comply with these terms.
*Correspondence: Xian Gu, Z3V4aWFuZXIxOEAxNjMuY29t; Huiyong Fang, aHVpeW9uZ2ZhbmdAMTI2LmNvbQ==
†These authors have contributed equally to this work and share first authorship
Disclaimer: All claims expressed in this article are solely those of the authors and do not necessarily represent those of their affiliated organizations, or those of the publisher, the editors and the reviewers. Any product that may be evaluated in this article or claim that may be made by its manufacturer is not guaranteed or endorsed by the publisher.
Research integrity at Frontiers
Learn more about the work of our research integrity team to safeguard the quality of each article we publish.