- Institute of Horticulture, Zhejiang Academy of Agricultural Sciences, Hangzhou, China
Periderm protects enlarged organs of most dicots and gymnosperms as a barrier to water loss and disease invasion during their secondary growth. Its development undergoes a complex process with genetically controlled and environmental stress-induced characters. Different development of periderm makes the full and partial russet of fruit skin, which diverges in inheritance with qualitative and quantitative characters, respectively, in pear pome. In addition to its specific genetics, fruit periderm has similar development and structure as that of stem and other organs, making it an appropriate material for periderm research. Recently, progress in histochemical as well as transcriptome and proteome analyses, and quantitative trait locus (QTL) mapping have revealed the regulatory molecular mechanism in the periderm based on the identification of switch genes. In this review, we concentrate on the periderm development, propose the conservation of periderm regulation between fruit and other plant organs based on their morphological and molecular characteristics, and summarize a regulatory network with the elicitors and repressors for the tissue development. Spontaneous programmed-cell death (PCD) or environmental stress produces the original signal that triggers the development of periderm. Spatio-temporal specific PCD produced by PyPPCD1 gene and its homologs can play a key role in the coordinated regulation of cell death related tissue development.
Introduction
Periderm replaces the cuticle to protect growing organs of most dicots and gymnosperms during their secondary growth (Wunderling et al., 2018). The formation of periderm is a common phenomenon in stems and roots of dicotyledons and gymnosperms that increase in thickness by the secondary growth (Campilho et al., 2020). The cork cambium is re-differentiated in the vertical direction with outwardly to cork layer and inwardly to endodermis (Graça, 2015; Macnee et al., 2020). The formation of cork layer includes rapid cell enlargement and suberized cell walls, followed by cell death (Daneva et al., 2016). Periderm development is extremely complex and it differs among diverse species, genotypes and organs, and it is also characterized by developmental stages, seasonal changes, and environmental stresses (Lulai and Corsini, 1998; Caritat et al., 2000; Neubauer et al., 2012; Thangavel et al., 2016). Because of its importance for plant performance and survival, periderm has noticeably attracted attention, and the resulting studies have concentrated on the chemical composition of cork and high-resolution transcript profilings that have provided a reliable basis for periderm development (Vishwanath et al., 2015; Ragni and Greb, 2018; Campilho et al., 2020; Macnee et al., 2020).
Periderm development has been reported to be significantly associated with regulation of switch genes, which could be triggered during plant secondary growth. The identification of switch genes is the key to reveal the molecular mechanism for controlling the trait formation (Wang et al., 2016; Wang et al., 2022). However, obtaining genetic material has been difficult as the PCD mutations are often fatal (Wang et al., 2022). In addition to acting as the secondary protective tissue, periderm also affects the quality of fruits, such as that of pear, apple, and kiwi, in which the outmost cork layer in fruit skin affects the fruit appearance, storage capability, and shelf life. The genetic differentiation in the periderm development of fruit makes it highly appropriate for the study of biochemical profiles and molecular regulation of periderm (Wang et al., 2016; Macnee et al., 2020). Except for histochemical analysis, integrative transcriptome and proteome analysis and quantitative trait locus (QTL) mapping have been carried out for the russet trait made by periderm development in the fruit skin (Yamamoto et al., 2014; Falginella et al., 2015; Lashbrooke et al., 2015; Heng et al., 2016; Legay et al., 2016; Shi et al., 2019; Wang et al., 2020). In particular, the switch genes for fruit periderm development and the functional conservation of the homologs among different species (Lashbrooke et al., 2015; Wang et al., 2022) showed to have multiple important implications to reveal the regulatory mechanisms underlying periderm development. In the present review, we concentrate on the progresses of periderm development research in fruit skin, with an emphasis on exploring the original signal and the underlying regulatory mechanisms.
Signals for fruit periderm development
Orchard factors that induce fruit periderm development to russet include the expansion-growth-induced strain and environmental stresses (Winkler et al., 2022). Partially russet development is stress inducible, and it can be repressed by rain sheltered cultivation and eliminated by fruit bagging (Wang et al., 2016; Shi et al., 2019; Zhang et al., 2021). Similar effect of bagging has been observed in other fruits (Winkler et al., 2022). These results suggest that expansion-growth-induced strain does not cause russeting independently in the absence of stress or certain environmental factors. Either fruit bagging or rain sheltered cultivation continuously provides an appropriate growing condition for fruits. However, when environmental changes exceed the allowable ranges that are required for the appropriate growth condition, stress is produced and periderm development is triggered. The dehydration caused by transpiration with inappropriate water supply can be the main environmental stress leading to the fruit russet formation (Athoo et al., 2020; Chen et al., 2020; Khanal et al., 2021).
In addition to the type of induction by environmental stress, russeting is also formed by periderm development in fruit of some pear cultivars, which shows a dominant gene control and cannot be eliminated by fruit bagging (Wang et al., 2016). Histochemical staining at different development stages of pear fruit skin has demonstrated clearly spontaneous fruit periderm formation, including the steps of cell suberization and PCD (Macnee et al., 2020; Wang et al., 2022). For a long time, the regulatory relationship between the cell suberization and the PCD in the periderm development was not clearly documented until the switch gene for the russet formation was cloned in pear fruit skin (Wang et al., 2022). A study suggested that PCD in pear fruit skin has knock-on effects in terms of exocarp integrity with regards to gas-liquid exchange, resulting in the response of periderm development to the stress or environmental changes. High levels of oxygen and moisture play an important role in the fruit russet formation (Winkler et al., 2022). The H2O2 generated during polyamine metabolism in pear fruit skin was supposed to contribute to the fruit russet formation (Heng et al., 2016). The spontaneous periderm development can also be repressed when the fruit surface is coated with Vaseline before the fruit russet formation (Figure 1). A difference of Vaseline coating to fruit bagging treatment is that it can prevent the direct contact of fruit skin to oxygen. Thus, oxygen is considered as the necessary environmental factor for response of periderm development triggered by stress or a change in the environment.
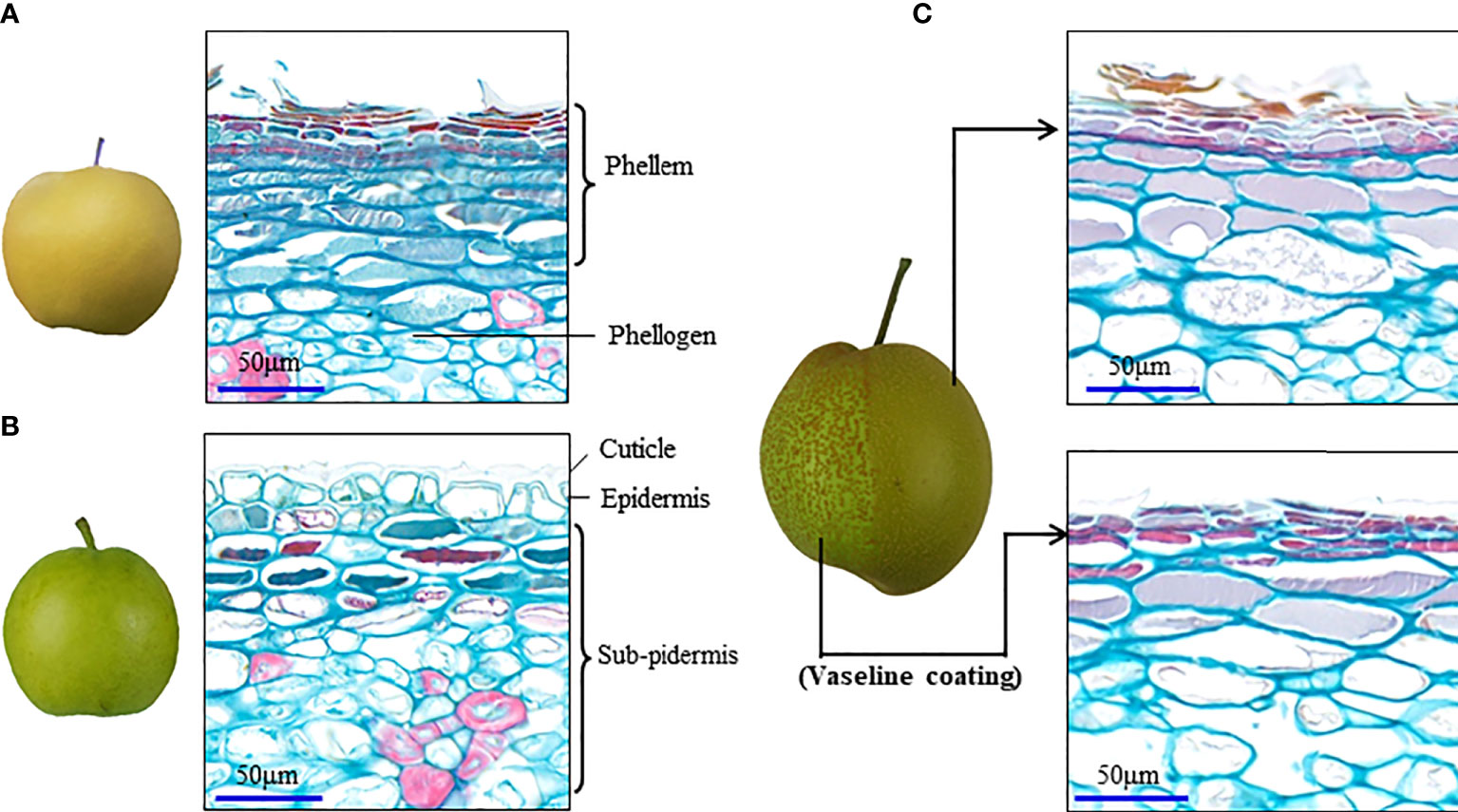
Figure 1 Vaseline coating repressed periderm development in the russet fruit skin of sand pear. (A, B) Naturally different periderm development between russet and green fruit skin of sand pear after 80 days of anthesis in normal growth conditions. (C) Vaseline coating on part of the fruit skin of sand pear that repressed the periderm development in the treatment position.
Wounding induces changes in cytokinin and auxin content in potato tuber, followed by the wound periderm formation (Lulai et al., 2016). In the cellular de-differentiation analysis, auxin and cytokinin induce reentry into S-phase and proliferation of the mesophyll cells, and auxin may induce re-differentiation (Valente et al., 1998). Stress induces plant somatic embryogenesis to acquire some features of stem cells, which has been discussed widely as a fundamental theme in a recent issue of Biochimica et Biophysica Acta (the Special Issue: Stress as a fundamental theme in cellplasticity. 2015). The fruit susceptibility to russeting varies with developmental stages (Knoche et al., 2011; Khanal et al., 2021; Winkler et al., 2022). The de-differentiation potential of somatic cells to meristem depends on cell types and cell developmental stages, which can explain why periderm or russeting is more easily induced in the early developmental stage.
Except for initiating periderm development with a pathway similar to that of stress, signaling molecules triggering PCD or produced during the process of PCD may directly regulate the secondary metabolism for spontaneous peridermogenesis in adjacent cells (Wang et al., 2022). As a major component of biological membranes, lipids also serve as substrates for generating numerous signaling molecules involved in tolerance of and responses to biotic and abiotic stresses (Ali et al., 2022; Haslam and Feussner, 2022). Lipid biosynthesis, metabolism, and oxidation-reduction have been reported to be regulated, and the structure of plasma membrane has been altered and disrupted during the PCD of pear fruit skin (Wang et al., 2020; Wang et al., 2022).
Genetic characteristics and gene mapping of fruit russet
As mentioned above, fruit russet made by periderm development is enriched in phenotypic variation. Such as that observed in pear, kiwifruit and apple, there exist both types of periderm that either completely or partially covers the fruit (Falginella et al., 2015; Lashbrooke et al., 2015; Wang et al., 2016; Macnee et al., 2020). The similar outmost periderm was also found in other storage organs as that of Potato (Solanum tuberosum), Sweet potato (Ipomea batatas), Cassava (Manihot esculenta), and Yam (Rhizoma Dioscoreae) (Figure 2). The inheritance of pear fruit russet has been studied for long. Early studies speculated two loci (R and I) controlling pear fruit skin russet, in which the R locus had the dominant effect on cork layer development, and the I locus had the dominant effect on cork layer suppression (Katayama et al., 2006; Song et al., 2010). Combined with normal distribution of the semi-russet in cross population and bi-directional russet mutations between smooth and russet exocarp, Wang et al. (2016) clustered pear peridermal fruits with qualitative characterization (full russet or complete peridermal skin) and quantitative characterization (semi-russet, partially peridermal skin). The two traits of full russet and semi-russet are inherited independently, while the former obscures the latter when they are co-inherited. A conservative dominant gene controlling the russet of pear fruit skin has been mapped at the end of the linkage group 8, which was also analyzed as a major QTL in several studies (Inoue et al., 2006; Song et al., 2010; Yamamoto et al., 2014; Kumar et al., 2017; Takeuchi et al., 2021; Wang et al., 2022). QTL mapping using different segregating progenies also led to the identification of one major determinant on the linkage group 12, and two loci in chromosomes 2 and 15 that could be associated with apple fruit russet (Falginella et al., 2015; Lashbrooke et al., 2015). QTL analysis in kiwifruit identified two types of gene loci that either associated with russet formation or were involved in cuticle integrity and coverage (Macnee et al., 2021). These results indicate the complexity of russet regulation and the presence of different determinants for the trait formation within and among different species.
Molecular triggers in fruit periderm development
Periderm development can be either repressed by a strong cuticle protection or advanced by a strong elicitor (Figure 1). Construction of cuticle membrane by multiple biopolymers suggests a complex regulation network underlying the development of the tissue. Previous studies have mapped different loci controlling cuticle strength or the propensity to russet in fruit skin (Lashbrooke et al., 2015; Macnee et al., 2021; Jiang et al., 2022). Orthologues of WAX INDUCER1/SHINE1 (SHN1/WIN1) have been shown to regulate cuticle formation in different species (Aharoni et al., 2004; Broun et al., 2004; Kannangara et al., 2007; Shi et al., 2011; Shi et al., 2013; Hen-Avivi et al., 2014). In apple, MdSHN3 was revealed to have an extremely low expression, which is in line with cuticle failure and russet formation, and could act as a putative negative regulator in apple fruit periderm development (Lashbrooke et al., 2015). PyPPCD1 has been identified as a strong elicitor dominantly controlling the periderm development in pear fruit skin by triggering an ARF1-dependent PCD and dedifferentiation of the parenchymal cells into cork cambium (Wang et al., 2022). PyPPCD1 has shown a preferential expression in pear fruit skin during the periderm development and its orthologues have been proved to be conservative with PCD function in different species (Wang et al., 2022). The environmental stress-inducible genes and quantitative traits suggest that there are more molecular regulators in the periderm development. Several key genes encoding the catalytic enzymes acyltransferases and P450s, and the transcription factor MYB1 and NAC for either suberin or cutin biosynthesis have been identified in other organs and species (Li et al., 2007; Höfer et al., 2008; Serra et al., 2009; Capote et al., 2018; Soler et al., 2020). These genes showed effect on the composition and structure of the periderm or cuticle. Further studies are still needed to determine whether these genes have a switch role in periderm development.
The common regulation in periderm
Suberized cells form the periderm. Suberins are complex polyesters which are highly variable in the relative proportion of the different families of monomers, and of the individual suberin acids depending on plant species and tissue location (reviewed by Graça, 2015; Woolfson et al., 2022). Although showing specific characters with a visible structure in the outmost cork layer (Figure 1), periderm shares the conserved developmental processes and conservative regulation among different species and organs (Lashbrooke et al., 2016; Fernández-Piñán et al., 2021; Serra et al., 2022). PCD is a process that causes an actively induced and tightly controlled cellular suicide, and different types of PCD play crucial roles in plant development, as well as in the reaction to environmental stresses (Daneva et al., 2016). Previous studies mainly described PCD as the result of environmental stress or as the destiny of the suberizing cells in the periderm development, while its regulatory role during the development of the tissue was not clearly indicated. Pear fruit is an annual organ, whereas its periderm development is similar to that of the perennial stem (Figure 3), which suggests the similar regulatory mechanisms underlying the development of the two types of the tissue. Thus, the regulatory role of PCD in pear fruit periderm development is supposed to be conservative in the development of other types of periderm.
In addition to the PCD-triggered type, uneven development of periderm also exists in some tree stems (Figure 4), which is similar to the semi-russet type periderm of pear fruit. For tree stem and other perennial organs, their periderm meristem typically shows perennial properties with seasonal rhythm and stress responsiveness, and the timing of the first periderm formation also varies among different species from the first year of growth to several years delayed (Serra et al., 2022). The cuticle serves as a primary protective layer to protect plant organs from environmental stresses that induce periderm development, while the genetic persistence of cuticle membrane is different among different species (Lashbrooke et al., 2015; Macnee et al., 2021), which leads to the protection variation and causes differences in the occurrence of the first periderm among different species. In order to better understand the regulation of periderm development, we summarized and plotted the regulatory relationship between periderm development and the regulatory factors based on the above-mentioned analysis (Figure 5), which is generally considered for the regulation of periderm development in different organs or species. The molecular triggers identified most recently in pear and apple showed functional conservation among their homologs (Lashbrooke et al., 2015; Wang et al., 2022), their certain roles for periderm development in different types of organs and species are still needed to be clarified in the next studies.
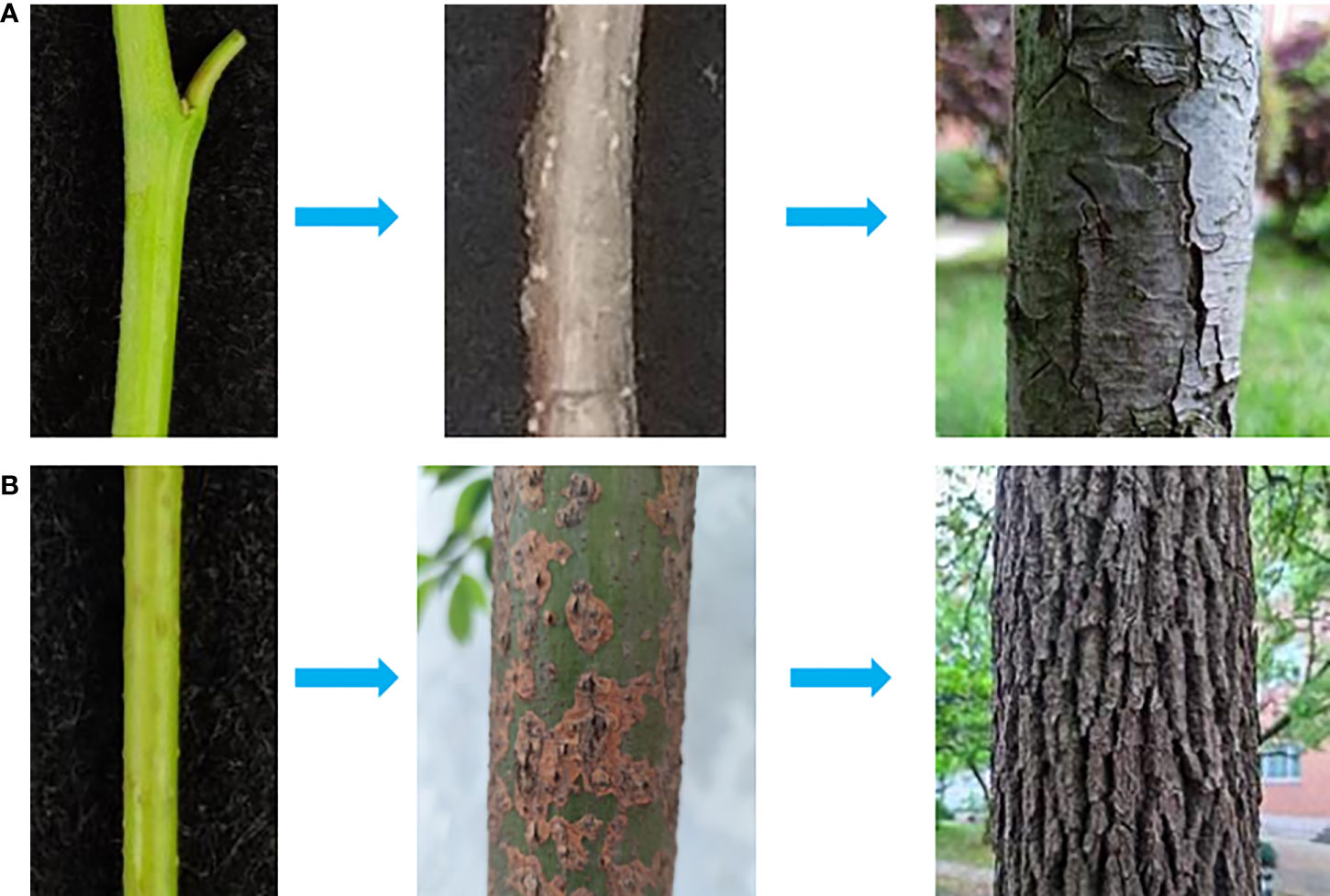
Figure 4 Different types of stem periderm. In the direction of the arrow, the new spring stem, biennial stem, and perennial stem of sand pear (Pyrus pyrifolia) (A) and camphor tree (Camphora officinarum) (B) could be observed.
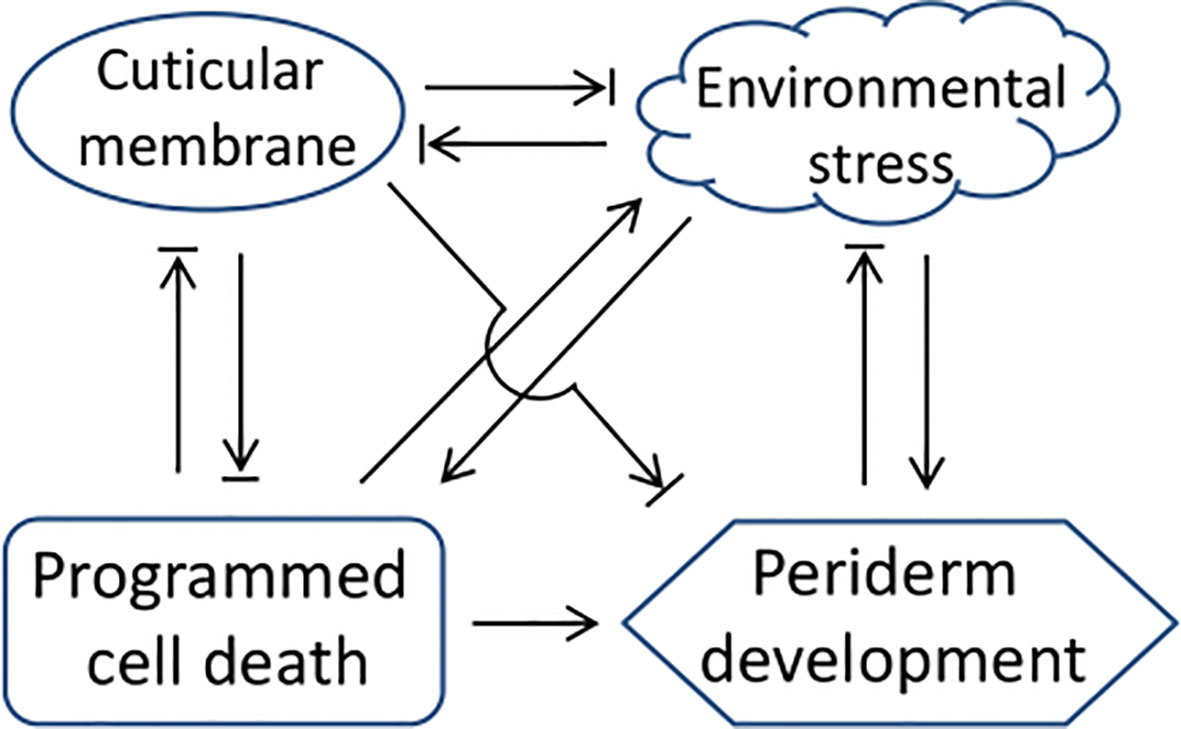
Figure 5 A network of the regulatory factors and periderm development. The arrow and termination arrow symbols indicate that one side of the link promotes or inhibits the development of the other side.
Conclusions
The present review summarized a putative common pathway for periderm development, i.e. either environmental stress or PCD could disrupt the structure of plasma membrane and other organelles, leading to the cells at the condition of oxygen exposure and water imbalance, followed by the stress response with activation of the synthesis of phytohormones to initiate phellogen and periderm development. PCD has been revealed to play a key role in the regulation of periderm development (Wunderling et al., 2018; Wang et al., 2022), which is also shared by the vascular cambium in its differentiation to xylem (Wunderling et al., 2018; Serra et al., 2022). The β-glucuronidase (GUS) fusion expression analysis indicated that the PyPPCD1 gene was also highly expressed in the vascular cambium of a poplar system (data were not published). Considering the role of PyPPCD1-guided PCD in cork cambium development regulation, this result suggests the similar regulation for the vascular cambium activity as that in cork cambium. The roles of PyPPCD1 gene and its homologs in the co-regulation of the development of these tissues need to be further explored. The regulation of periderm development consists of a complex regulatory network superimposed by multiple aspects of regulation, including the tissue or cell type specificity, seasonal and developmental stage specificity, and environmental stress response. More regulators of phellogen proliferation and differentiation are required to be functionally characterized to refine the framework of the molecular mechanisms. Robust tools and methods, including single-cell transcriptomics and genetic manipulation, will facilitate this process.
Author contributions
Y-ZW prepared the figures and wrote the manuscript. Z-BS, M-SD, and D-YC contributed their ideas and suggestions for the manuscript revision. All authors have read and agreed to the published version of the manuscript.
Funding
This work was partially funded by the Earmarked Fund for China Agriculture Research System (CARS) and the Key Project for New Agricultural Cultivar Breeding in Zhe-jiang Province, China (2021C02066-5).
Conflict of interest
The authors declare that the research was conducted in the absence of any commercial or financial relationships that could be construed as a potential conflict of interest.
Publisher’s note
All claims expressed in this article are solely those of the authors and do not necessarily represent those of their affiliated organizations, or those of the publisher, the editors and the reviewers. Any product that may be evaluated in this article, or claim that may be made by its manufacturer, is not guaranteed or endorsed by the publisher.
References
Aharoni, A., Dixit, S., Jetter, R., Thoenes, E., van Arkel, G., Pereira, A. (2004). The SHINE clade of AP2 domain transcription factors activates wax biosynthesis, alters cuticle properties, and confers drought tolerance when overexpressed in arabidopsis. Plant Cell 16, 2463–2480. doi: 10.1105/tpc.104.022897
Ali, U., Lu, S., Fadlalla, T., Iqbal, S., Yue, H., Yang, B., et al. (2022). The functions of phospholipases and their hydrolysis products in plant growth, development and stress responses. Prog. Lipid Res. 86, 101158. doi: 10.1016/j.plipres.2022.101158
Athoo, T. O., Winkler, A., Knoche, M. (2020). Russeting in 'Apple' mango: Triggers and mechanisms. Plants (Basel) 9, 898. doi: 10.3390/plants9070898
Broun, P., Poindexter, P., Osborne, E., Jiang, C. Z., Riechmann, J. L. (2004). WIN1, a transcriptional activator of epidermal wax accumulation in arabidopsis. Proc. Natl. Acad. Sciences U.S.A. 101, 4706–4711. doi: 10.1073/pnas.0305574101
Campilho, A., Nieminen, K., Ragni, L. (2020). The development of the periderm: The final frontier between a plant and its environment. Curr. Opin. Plant Biol. 53, 10–14. doi: 10.1016/j.pbi.2019.08.008
Capote, T., Barbosa, P., Usié, A., Ramos, A. M., Inácio, V., Ordás, R., et al. (2018). ChIP-seq reveals that QsMYB1 directly targets genes involved in lignin and suberin biosynthesis pathways in cork oak (Quercus suber). BMC Plant Biol. 18, 198. doi: 10.1186/s12870-018-1403-5
Caritat, A, Gutiérrez, E, Molinas, M. (2000). Influence of weather on cork-ring width. Tree Physiol. 20:893–900. doi: 10.1093/treephys/20.13.893
Chen, Y. H., Straube, J., Khanal, B. P., Knoche, M., Debener, T. (2020). Russeting in apple is initiated after exposure to moisture ends-I. Histological Evidence. Plants (Basel) 9, 1293. doi: 10.3390/plants9101293
Daneva, A., Gao, Z., Van Durme, M., Nowack, M. K. (2016). Functions and regulation of programmed cell death in plant development. Annu. Rev. Cell Dev. Biol. 32, 441. doi: 10.1146/annurev-cellbio-111315-124915
Falginella, L., Cipriani, G., Monte, C., Gregori, R., Testolin, R., Velasco, R., et al. (2015). A major QTL controlling apple skin russeting maps on the linkage group 12 of 'Renetta grigia di torriana'. BMC Plant Biol. 15, 150. doi: 10.1186/s12870-015-0507-4
Fernández-Piñán, S., Boher, P., Soler, M., Figueras, M., Serra, O. (2021). Transcriptomic analysis of cork during seasonal growth highlights regulatory and developmental processes from phellogen to phellem formation. Sci. Rep. 11 (1), 12053. doi: 10.1038/s41598-021-90938-5
Graça, J. (2015). Suberin: the biopolyester at the frontier of plants. Front. Chem. 3, 62. doi: 10.3389/fchem.2015.00062
Haslam, T. M., Feussner, I. (2022). Diversity in sphingolipid metabolism across land plants. J. Exp. Bot. 73, 2785–2798. doi: 10.1093/jxb/erab558
Hen-Avivi, S., Lashbrooke, J., Costa, F., Aharoni, A. (2014). Scratching the surface: genetic regulation of cuticle assembly in fleshy fruit. J. Exp. Bot. 65, 4653–4664. doi: 10.1093/jxb/eru225
Heng, W., Wang, Z., Jiang, X., Jia, B., Liu, P., Liu, L., et al. (2016). The role of polyamines during exocarp formation in a russet mutant of 'Dangshansuli' pear (Pyrus bretschneideri rehd.). Plant Cell Rep. 35, 1841–1852. doi: 10.1007/s00299-016-1998-7
Höfer, R., Briesen, I., Beck, M., Pinot, F., Schreiber, L., Franke, R. (2008). The arabidopsis cytochrome P450 CYP86A1 encodes a fatty acid omega-hydroxylase involved in suberin monomer biosynthesis. J. Exp. Bot. 59, 2347–2360. doi: 10.1093/jxb/ern101
Inoue, E., Kasumi, M., Sakuma, F., Anzai, H., Amano, K., Hara, H. (2006). Identification of RAPD marker linked to fruit skin colour in Japanese pear (Pyrus pyrifolia nakai). Sci. Hortic. 107, 254–258. doi: 10.1016/j.scienta.2005.07.009
Jiang, S., Luo, J., Wang, X., An, H., Zhang, J., Li, S. (2022). QTL mapping and transcriptome analysis to identify genes associated with green/russet peel in Pyrus pyrifolia. Scientia Hortic. 293, 110714. doi: 10.1016/j.scienta.2021.110714
Kannangara, R., Branigan, C., Liu, Y., Penfield, T., Rao, V., Mouille, G., et al. (2007). The transcription factor WIN1/SHN1 regulates cutin biosynthesis in arabidopsis thaliana. Plant Cell 19, 1278–1294. doi: 10.1105/tpc.106.047076
Khanal, B. P., Imoro, Y., Chen, Y. H., Straube, J., Knoche, M. (2021). Surface moisture increases microcracking and water vapour permeance of apple fruit skin. Plant Biol. 23, 74–82. doi: 10.1111/plb.13178
Kikuchi, A. (1924). On the origin of Japanese pear and the inheritance of the skin colors of their fruits. Japan. J. Genet. 3, 1–27. doi: 10.1266/jjg.3.1
Kikuchi, A. (1930). On skin color of the Japanese pear, and its inheritance. Contr. Inst. Plant Ind. 8, 1–50.
Knoche, M., Khanal, B. P., Stopar, M. (2011). Russeting and microcracking of ‘Golden delicious’ apple fruit concomitantly decline due to gibberellin a 4+7 application. J. Am. Soc Hortic. Sci. 136, 159–164. doi: 10.21273/JASHS.136.3.159
Kumar, S., Kirk, C., Deng, C., Wiedow, C., Knaebel, M., Brewer, L. (2017). Genotyping-by-sequencing of pear (Pyrus spp.) accessions unravels novel patterns of genetic diversity and selection footprints. Hortic. Res. 4, 17015. doi: 10.1038/hortres.2017.15
Lashbrooke, J., Aharoni, A., Costa, F. (2015). Genome investigation suggests MdSHN3, an APETALA2-domain transcription factor gene, to be a positive regulator of apple fruit cuticle formation and an inhibitor of russet development. J. Exp. Bot. 66, 6579–6589. doi: 10.1093/jxb/erv366
Lashbrooke, J., Cohen, H., Levy-Samocha, D., Tzfadia, O., Panizel, I., Zeisler, V., et al. (2016). MYB107 and MYB9 homologs regulate suberin deposition in angiosperms. Plant Cell 28, 2097–2116. doi: 10.1105/tpc.16.00490
Legay, S., Guerriero, G., André, C., Guignard, C., Cocco, E., Charton, S., et al. (2016). MdMyb93 is a regulator of suberin deposition in russeted apple fruit skins. New Phytol. 212, 977–991. doi: 10.1111/nph.14170
Li, Y., Beisson, F., Koo, A. J., Molina, I., Pollard, M., Ohlrogge, J. (2007). Identification of acyltransferases required for cutin biosynthesis and production of cutin with suberin-like monomers. Proc. Natl. Acad. Sci. U S A. 104, 18339–18344. doi: 10.1073/pnas.0706984104
Lulai, EC, Corsini, DL (1998). Differential deposition of suberin phenolic and aliphatic domains and their roles in resistance to infection during potato tuber (Solanum tuberosum L.) wound-healing. Physiol. Mol. Plant Pathol. 53, 209–222. doi: 10.1006/pmpp.1998.0179
Lulai, E. C., Suttle, J. C., Olson, L. L., Neubauer, J. D., Campbell, L. G., Campbell, M. A. (2016). Wounding induces changes in cytokinin and auxin content in potato tuber, but does not induce formation of gibberellins. J. Plant Physiol. 191, 22–28. doi: 10.1016/j.jplph.2015.11.006
Macnee, N. C., Rebstock, R., Hallett, I. C., Schaffer, R. J., Bulley, S. M. (2020). A review of current knowledge about the formation of native peridermal exocarp in fruit. Funct. Plant Biol. 47, 1019–1031. doi: 10.1071/FP19135
Macnee, N, Hilario, E, Tahir, J, Currie, A, Warren, B, Rebstock, R, et al (2021). Peridermal fruit skin formation in Actinidia sp. (kiwifruit) is associated with genetic loci controlling russeting and cuticle formation. BMC Plant Biol. 21, 334. doi: 10.1186/s12870-021-03025-2
Mori, H. (1953). Studies on the inheritance of the main characteristics of deciduous fruit trees (peach, Japanese pear and Japanese persimmon). Bull. Nat. Inst. Agric. Sci. E 2, 1–66.
Neubauer, JD, Lulai, EC, Thompson, AL, Suttle, JC, Bolton, MD (2012). Wounding coordinately induces cell wall protein, cell cycle and pectin methyl esterase genes involved in tuber closing layer and wound periderm development. J Plant Physiol. 169, 586–95. doi: 10.1016/j.jplph.2011.12.010.
Ragni, L., Greb, T. (2018). Secondary growth as a determinant of plant shape and form. Semin. Cell Dev. Biol. 79, 58–67. doi: 10.1016/j.semcdb.2017.08.050
Serra, O., Mähönen, A. P., Hetherington, A. J., Ragni, L. (2022). The making of plant armor: The periderm. Annu. Rev. Plant Biol. 73, 405–432. doi: 10.1146/annurev-arplant-102720-031405
Serra, O., Soler, M., Hohn, C., Sauveplane, V., Pinot, F., Franke, R., et al. (2009). CYP86A33-targeted gene silencing in potato tuber alters suberin composition, distorts suberin lamellae, and impairs the periderm's water barrier function. Plant Physiol. 149, 1050–1060. doi: 10.1104/pp.108.127183
Shi, J. X., Adato, A., Alkan, N., He, Y., Lashbrooke, J., Matas, A. J., et al. (2013). The tomato SlSHINE3 transcription factor regulates fruit cuticle formation and epidermal patterning. New Phytol. 197, 468–480. doi: 10.1111/nph.12032
Shi, J. X., Malitsky, S., De Oliveira, S., Branigan, C., Franke, R. B., Schreiber, L., et al. (2011). SHINE transcription factors act redundantly to pattern the archetypal surface of arabidopsis flower organs. PloS Genet. 7, e1001388. doi: 10.1371/journal.pgen.1001388
Shi, C., Qi, B., Wang, X., Shen, L., Luo, J., Zhang, Y. (2019). Proteomic analysis of the key mechanism of exocarp russet pigmentation of semi-russet pear under rainwater condition. Scientia Hortic. 254, 178–186. doi: 10.1016/j.scienta.2019.04.086
Soler, M., Verdaguer, R., Fernández-Piñán, S., Company-Arumí, D., Boher, P., Góngora-Castillo, E., et al. (2020). Silencing against the conserved NAC domain of the potato StNAC103 reveals new NAC candidates to repress the suberin associated waxes in phellem. Plant Sci. 291, 110360. doi: 10.1016/j.plantsci.2019.110360
Song, W., Wang, C., Tian, Y., Tian, W., Yin, H. (2010). SSR molecular markers linked to the fruit russet skin of pear. Acta Horticult Sinica. 37, 1325–1328.
Takeuchi, Y., Nishio, S., Terakami, S., Takada, N., Saito, T. (2021). Haplotype structure analysis of a locus associated with fruit skin type on chromosome 8 in japanese pear. Tree Genet. Genomes 17 (3). doi: 10.1007/s11295-020-01483-7
Thangavel, T., Tegg, R. S., Wilson, C. R. (2016). Toughing it out—disease-resistant potato mutants have enhanced tuber skin defenses. Phytopathology 106:474–483. doi: 10.1094/PHYTO-08-15-0191-R
Valente, O., Tao, W., Verbelen, J. P. (1998). Auxins and cytokinins control DNA endoreduplication and deduplication in single cells of tobacco. Plant Sci. 134, 207–215. doi: 10.1016/S0168-9452(98)00062-4
Vishwanath, S. J., Delude, C., Domergue, F., Rowland, O. (2015). Suberin: biosynthesis, regulation, and polymer assembly of a protective extracellular barrier. Plant Cell Rep. 34, 573–586. doi: 10.1007/s00299-014-1727-z
Wang, Y., Dai, M., Cai, D., Shi, Z. (2020). Proteome and transcriptome profile analysis reveals regulatory and stress-responsive networks in the russet fruit skin of sand pear. Hortic. Res. 7, 16. doi: 10.1038/s41438-020-0242-3
Wang, Y., Dai, M., Cai, D., Zhang, S., Shi, Z. (2016). A review for the molecular research of russet/semi-russet of sand pear exocarp and their genetic characters. Sci. Hortic. 210, 138–142. doi: 10.1016/j.scienta.2016.07.019
Wang, Y., Dai, M., Wu, X., Zhang, S., Shi, Z., Cai, D., et al. (2022). An ARF1-binding factor triggering programmed cell death and periderm development in pear russet fruit skin. Hortic. Res., uhab061. doi: 10.1093/hr/uhab061
Winkler, A, Athoo, T, Knoche, M. (2022). Russeting of Fruits: Etiology and Management. Horticulturae. 8, 231. doi: 10.3390/horticulturae8030231
Woolfson, KN, Esfandiari, M, Bernards, MA. (2022). Suberin Biosynthesis, Assembly, and Regulation. Plants (Basel). 11, 555. doi: 10.3390/plants11040555
Wunderling, A., Ripper, D., Barra-Jimenez, A., Mahn, S., Sajak, K., Targem, M. B., et al. (2018). A molecular framework to study periderm formation in arabidopsis. New Phytol. 219, 216–229. doi: 10.1111/nph.15128
Yamamoto, T., Terakami, S., Takada, N., Nishio, S., Onoue, N., Nishitani, C., et al. (2014). Identification of QTLs controlling harvest time and fruit skin color in japanese pear (Pyrus pyrifolia nakai). Breed. Sci. 64, 351–361. doi: 10.1270/jsbbs.64.351
Keywords: periderm, secondary development, abiotic stress, programmed cell death, fruit skin
Citation: Wang Y-z, Dai M-s, Cai D-y and Shi Z-b (2022) Solving the regulation puzzle of periderm development using advances in fruit skin. Front. Plant Sci. 13:1006153. doi: 10.3389/fpls.2022.1006153
Received: 29 July 2022; Accepted: 12 September 2022;
Published: 29 September 2022.
Edited by:
Abelardo Carlos Vegetti, CONICET Santa Fe, ArgentinaReviewed by:
Lixiang Miao, Fujian Agriculture and Forestry University, ChinaKaijie Qi, Nanjing Agricultural University, China
Copyright © 2022 Wang, Dai, Cai and Shi. This is an open-access article distributed under the terms of the Creative Commons Attribution License (CC BY). The use, distribution or reproduction in other forums is permitted, provided the original author(s) and the copyright owner(s) are credited and that the original publication in this journal is cited, in accordance with accepted academic practice. No use, distribution or reproduction is permitted which does not comply with these terms.
*Correspondence: Yue-zhi Wang, yzwang2010@mail.zaas.ac.cn; Ze-bin Shi, Shizebinszb@126.com