- 1Key Laboratory of Wheat Biology and Genetic Improvement on South Yellow and Huai River Valley, Ministry of Agriculture, Hefei, China
- 2College of Agronomy, Anhui Agricultural University, Hefei, China
Due to the frequent occurrence of extreme weather events, the area of wheat affected by continuous cloudy rainfall is increasing, with waterlogging becoming a major limiting factor of wheat yield. To alleviate the effect, spraying exogenous plant growth regulators is often used. In this study, two wheat cultivars, waterlogging-tolerant Yangmai 18 and waterlogging-sensitive Sumai 188, were selected for waterlogging and shading (WS) after anthesis for 7, 11, and 15 days respectively. Three concentrations of 6-benzylaminoadenine (6-BA) solution (15, 25, and 35 mg·L−1) were sprayed after WS treatment and water was sprayed as the control. Then, the effect of spraying 6-BA on photosynthetic characteristics, starch content, grain filling characteristics, and yield was explored under artificially stimulated continuous cloudy rainfall during anthesis. Compared with the control, the application of 6-BA caused a significant increase in grain plumpness throughout grain filling, as well as increases in the net photosynthetic rate (Pn), stomatal conductance (Gs), and transpiration rate (Tr), and a significant decrease in the intercellular CO2 concentration (Ci) of the flag leaves, all of which enhanced the photosynthetic capacity. The content of total starch, amylose, and amylopectin in the grains also increased significantly compared with the control. After WS for 15 days, the starch content increased by 3.81%–11.41% compared with the control. Spraying 6-BA also prolonged grain filling, increased the average grain filling rate, and significantly increased the 1000-grain weight and yield. The thousand-grain weight increased by 5.06%–43.28%, and wheat yield increased by 8.93%–64.27% after spraying 25 mg·L−1 of the 6-BA solution. These findings suggest that the application of 6-BA after WS stress could significantly improve the photosynthetic performance, which is propitious to the accumulation and transport of photosynthetic products after anthesis. Besides, spraying 6-BA can also increase the duration and rate of grain filling and starch accumulation content and improve grain weight, thereby alleviating the adverse effects of WS on wheat yield. Overall, spraying 25 mg·L−1 of the 6-BA solution had an optimal effect. These findings provide a theoretical basis for the exploration of cultivation techniques and measures aimed at alleviating damage caused by continuous rainfall during wheat anthesis.
1 Introduction
In the recent years, continuous or heavy rainfall caused by climatic factors, coupled with poor irrigation and drainage facilities as well as a low-lying terrain, has had widespread effects on global wheat production, with waterlogging becoming a major limiting factor of wheat yield (Boru et al., 2001; Singh and Setter, 2015). This is particularly apparent in the wheat-growing regions of the middle and lower reaches of the Yangtze River, China, where rice–wheat rotation has resulted in heavy soil with saturated water content (Shao et al., 2013). Moreover, much of the high rainfall in this region occurs in spring, a critical wheat growth period in terms of booting and anthesis. Studies have shown that the frequency of waterlogging in wheat-growing areas along the Yangtze River was as high as 60% during the 50-year period from 1961 to 2010, with detrimental effects on wheat yield (Wu et al., 2016; Wu H. et al., 2018). At the same time, the weak light effect caused by continuous precipitation has an additional effect during later growth stages, greatly reducing grain dry matter accumulation, which also affects yield (Mu et al., 2010). However, waterlogging stress and shading stress on the damage mechanism of wheat are different (Geigenberger, 2003; Gao et al., 2017). At present, there are many studies on the effects of single-factor stress such as waterlogging or shading, while there are few studies on the effect of compound stress on wheat yield. Under natural conditions, waterlogging is generally caused by continuous rainy weather, which leads to insufficient light. Therefore, the study of the combined stress of WS can better simulate the natural disaster situation of wheat. New cultivation techniques and measures aimed at effectively alleviating the damage caused by the combined stress of WS are therefore essential.
About 70% of wheat grain yield is associated with the accumulation of photosynthetic products after anthesis (Ziaei and Sepaskhah, 2003). Under waterlogging stress, the nitrogen content of wheat plants is significantly reduced, leaves become chlorotic, senescence is accelerated, and plants lose their ability to capture light and carboxylate (Ziaei and Sepaskhah, 2003; Wu W. et al., 2018). This has a direct effect on the rate of photosynthesis, dramatically slowing growth and development. Meanwhile, studies have also shown that while mild shading (88% light transmittance) delays the senescence of wheat leaves, increasing the Pn, canopy apparent photosynthetic rate, 1000-grain weight and yield, and moderate (67% light transmittance) and heavy shading (35% light transmittance) has significant negative effects on all of the above traits (Xu et al., 2015). The later stages of wheat growth and development center on the formation of grains, the main component of which is starch, which accounts for about 65% of the dry weight. Starch in the wheat endosperm is composed of amylose and amylopectin, with differences in the composition having an important impact on flour quality (Golay et al., 1991; Holm and Björck, 1992). Waterlogging during heading and anthesis causes damage to endosperm cell structure, resulting in the formation of irregular starch granules (Zhou Q. et al., 2018a), in addition, post-anthesis waterlogging affects the content of starch components by altering the activity and expression of enzymes related to starch synthesis (Zhou Q. et al., 2018b). Similarly, shading treatment in the wheat filling stage also causes a decrease in the starch content of wheat grains, significantly reducing overall quality (Andrade et al., 2018). In addition, shading also causes a reduction in dry matter accumulation before anthesis and wheat yield (Demotes-Mainard and Jeuffroy, 2004; Mu et al., 2010).
The exogenous application of plant growth regulators is often used to alleviate the impact of water or shading stress on crops. For example, studies have shown that the application of abscisic acid (ABA) before waterlogging can improve the antioxidant and photosynthetic capacity of waterlogged crops (Kim et al., 2018). Application of methyl jasmonate increased grain yield and biomass and water use efficiency under water stress during the vegetative stage (Ghasem et al., 2022). It was also shown that exogenous application of salicylic acid (SA) inhibited the uptake of Na and stimulated the uptake of N, P, and K in wheat under water stress, decreasing stomatal conductivity and increasing the grain number per spike and 1000-grain weight (Hafez and Farig, 2019). 6-Benzylaminoadenine (6-BA) promotes cell division and the transport and accumulation of photosynthetic products, enhancing stress resistance in plants. Moreover, in comparisons of five hormones (auxin, cytokinin, abscisic acid, gibberellin, and brassino steroid), 6-BA was found to have the greatest effect on duckweed biomass and, along with ABA, was most effective in enhancing biomass and starch accumulation (Liu et al., 2019). A previous study also revealed that after waterlogging during anthesis, foliar application of 6-BA significantly heightened the Pn of wheat leaves and the aboveground biomass and grain yield (Wang X. et al., 2020a). Evidence also suggests that spraying exogenous 6-BA was able to postpone leaf senescence and improve the chlorophyll content and photosynthetic capacity, thereby effectively lessening the harmful effects of flooding in summer maize (Ren et al., 2016). Furthermore, spraying the 6-BA solution before shading during anthesis was also found to delay the senescence of wheat flag leaves under shading treatment, as well as increase dry matter accumulation and reduce yield losses (Li et al., 2019).
At present, research into the mitigating effects of 6-BA application tends to focus on single stress events such as waterlogging or high temperatures, with the research content centering on photosynthetic characteristics and yield. However, few reports have examined the mitigating effects of exogenous 6-BA application on photosynthetic product transport, grain starch, and its starch component content in wheat under the combined stress of WS. This study aimed at ascertaining the effects of spraying several different concentrations of the 6-BA solution on photosynthetic characteristics, photosynthetic product transport, grain starch accumulation, grain filling characteristics, and yield under shading and waterlogging stress after anthesis. Furthermore, the physiological reasons for reducing the damage of WS to wheat yield after spraying the 6-BA solution were analyzed by studying the process of the photosynthetic performance and dry matter accumulation of wheat. This research could provide the theoretical foundation for effective cultivation techniques and measures to alleviate the damage of continuous rainfall after the anthesis of wheat.
2 Materials and methods
2.1 Experimental design
The experiment was carried out from November 2020 to June 2022 at Anhui Agricultural University Wanzhong Experimental Base, Guohe Town, Lujiang County, Hefei City (117°01′E, 30°57′N). The test site has a subtropical humid monsoon climate, with an average annual temperature of 15°C–16°C, average annual precipitation of 900–1000 mm, an annual sunshine duration of about 2000 h, and an average annual frost-free period of 228 days. The mean daily temperature and monthly cumulative rainfall from 2020 to 2022 during the wheat growing season is shown in Figure 1. The waterlogging-tolerant cultivar Yangmai 18 (bred by the Academy of Agricultural Sciences of Lixiahe District, Jiangsu Province) and the waterlogging-sensitive cultivar Sumai 188 (bred by Jiangsu Fengqing Seed Industry Technology Co., Ltd.) were used as test materials. Each box contained 150 kg of paddy soil taken from the 0–20 cm tillage layer. Before sowing in 2020 and 2021, the soil contains 22.3 and 15.8 g·kg−1 of organic matter, 1.4 and 0.8 g·kg−1 of total nitrogen, 106.5 and 104.4 mg·kg−1 of alkali-hydrolyzable nitrogen, 105.0 and 91.0 mg·kg−1 of available potassium, and 21.2 and 22.7 mg·kg−1 of available phosphorus, respectively. Plants were grown in boxes measuring 70 cm in length, 50 cm wide, and 43 cm high, with a volume of 120 L. Each box contained 150 kg of soil. Round drainage holes with a diameter of 2 cm were arranged around the sides of each box approximately 8 cm from the bottom, with eight along each long side and six on each end. During WS treatment, the drainage holes were plugged with rubber stoppers, while at all other times, they were kept open to ensure sufficient water permeability. The wheat plants were sown on 9 November 2020 and 2 November 2021, five rows per box at a row spacing of 15 cm. Before sowing, each box was supplied with 105 g organic fertilizer, 2.6 g phosphate fertilizer (P2O5), 5.2 g potassium fertilizer (K2SO4), and 15.98 g nitrogen fertilizer (46% urea), followed by 6.85 g nitrogen fertilizer as topdressing during the jointing stage. All other field management ways were the same as high-yield cultivation measures in the field.
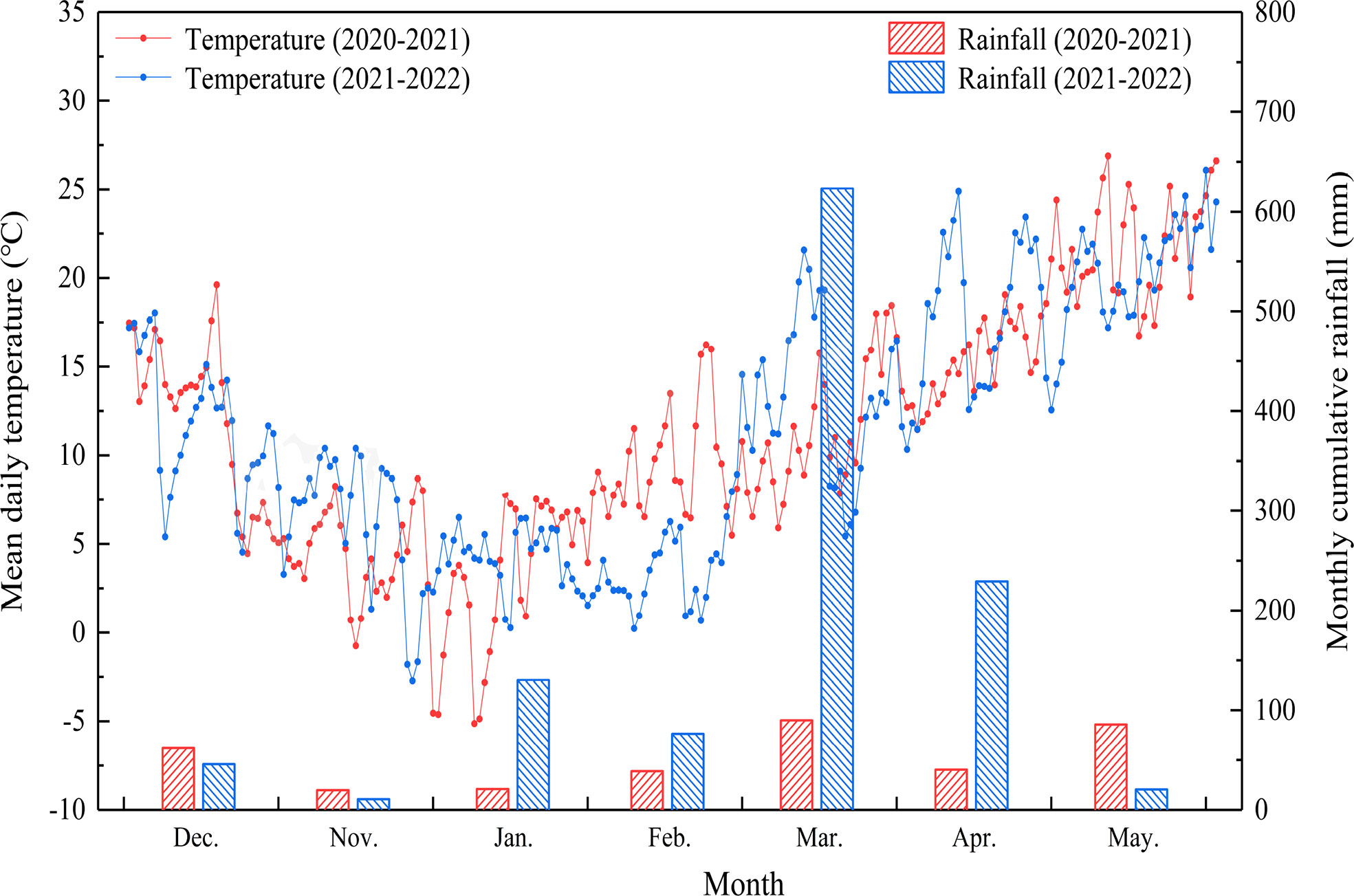
Figure 1 The mean daily temperature and monthly cumulative rainfall during wheat growing seasons (2020–2022).
WS were carried out during anthesis at durations of 7, 11, and 15 days. Shading and waterlogging treatment were carried out simultaneously, with shade nets used to create a shading rate of approximately 45%. The light intensity after shading is shown in Figure 2. Yangmai 18 underwent WS treatment at 19:00 on 10 April 2021 and 4 April 2022, while Sumai 188 began treatment at 19:00 on 12 April 2021 and 4 April 2022. The shade net was fixed to an open arched structure, the top of which was 1.8 m above the ground to allow sufficient ventilation. During waterlogging, the drainage holes were plugged to allow approximately 2 cm of water to accumulate on the soil surface. Rainproof measures were also taken during WS to mitigate the effect of external rainfall events. At 9:00 a.m. the day following WS treatment, plants were sprayed with one of three concentrations of the 6-BA solution (15, 25, and 35 mg·L−1). As a control, the same amount of distilled water was used. The spraying amount was 500 ml for each box, so as to ensure that each leaf was evenly covered with the reagent. Each treatment set consisted of 12 boxes.
2.2 Acquisition of samples
Single stems that bloomed on the same day and had similar-sized wheat ears were marked in each treatment to indicate anthesis. Following WS treatment, and starting from 10 days after anthesis, 20–25 of the marked wheat ears were then randomly selected from each treatment every 5 days (see Table 1 for sampling times). The grains were then collected and the remaining kernels were removed after heating at 105°C for 15 min. These samples were kiln-dried to a steady weight at 80°C for the analysis of starch and each starch component, and for grain dry matter accumulation.
2.3 Measurements
2.3.1 Morphology of grains and starch granules
Grain morphology was observed in samples obtained as described above and in Table 1, every 5 days from 10 days after anthesis. One to two seeds from the bottom of the intermediate spikelet were selected for observations using a SZX16 stereo microscope (OLYMPUS, Japan).
For observations of starch grain morphology, one to two wheat ears were randomly selected from the marked wheat plant after reaching maturity. One to two grains at the bottom of the middle spikelet were then selected and cut along the middle with a blade to provide cross-sections for observations of the endosperm. The samples were fixed on a copper column with double-sided carbon glue then plated with gold using an ion sputtering device then the ultrastructure of the starch granules was observed using an S-4800 scanning electron microscope (HITACHI, Japan).
2.3.2 Photosynthetic parameters of flag leaves
The LI-6400XT portable photosynthetic system (LI-COR Co., USA), with an open gas circuit, was used to set the rate of photosynthetically active radiation (PAR) to 1200 μmol·m−2·s−1. In each treatment, three flag leaves of wheat with the same growth and development process were measured from 9:00 a.m. to 11:00 a.m. When the net photosynthetic rate of wheat tended to be stable, the photosynthetic parameters at this time were recorded. Each flag leaf was measured three times then the average value was determined. Measurements were performed every 5 days from 10 to 25 days after anthesis.
2.3.3 Isotope δ13C analysis
After spraying with the 6-BA solution the day after WS, 10 flag leaves were randomly selected from the marked single stem of wheat from each treatment; then the flag leaves were then sealed with transparent PVC plastic bags, injected with 5 ml of 13CO2 gas into the plastic bags using a medical syringe, and assimilated for 60 min under natural light conditions at 10:00–11:00 a.m. on the same day. Three flag leaves were then removed, fixed at 105°C for 15 min, and kiln-dried at 80°C. After reaching maturation, three unsampled wheat ears were selected, the grains removed, fixed, and dried. They were then ground to a powder using an A11 basic analytical mill (IKA, Germany) and passed through a 100-mesh screen. The Sumai 188 after 11 days under WS was selected for analysis of δ13C using flag leaves and grains were treated with 25 mg·L−1 of the 6-BA solution. To do so, a Sercon Integra 2 Elemental Analysis-Stable Isotope Ratio Mass Spectrometer (EA-IRMS) was used with the 13CO2-labeled flag leaves and grains (SerCon, Britain).
δ13CTOC values were obtained using the PDB international standard as a reference, as follows:
where R (13C/12CVPDB) is the carbon isotope abundance ratio of the international standard Vienna Pee Dee Belemnite (VPDB). The analytical precision of the δ13CTOC values was set at ± 0.2‰.
2.3.4 Grain starch and its component content
The total starch content of the grains was determined by anthrone colorimetry (Jia and Zhang, 2008). After drying, the wheat kernels were ground and then passed through a 100-mesh sieve. Next, 8 ml of 80% ethanol was added to about 0.1 g (accurate to 1mg) of the sample, heated in a water bath at 80°C for 30 min, cooled, and then centrifuged at 5000×g for 15 min. The supernatant was then discarded, 2 ml of distilled water was added to the precipitate, shaken, and then boiled for 20 min before cooling and adding 2 ml of 9.2 mol·L−1 HCl4. The samples were then shaken for a further 10 min before adding 6 ml of distilled water and centrifuging at 5000×g for 15 min. The resulting supernatant was then poured into a 50 ml volumetric flask. The process was repeated three times, giving a total sample volume of 50 ml, respectively. Next, 0.1 ml of the extract solution was added to 4 ml of 0.2% anthrone, boiled for 15 min, cooled, and observed using a spectrophotometer at a wavelength of 620 nm (Agilent Cary 300, USA). The starch standard curve was then used to calculate the starch content of each sample.
The amylose content was determined using a coupled spectrophotometer (Zhang et al., 2009). After drying, the wheat grains were ground into a powder, passed through a 100-mesh sieve, and then degreased with ether. Next, 10 ml of 0.5 mol·L−1 KOH solution was added to about 0.1 g (accurate to 1 mg) of defatted sample, boiled for 10 min, then diluted to 50 ml with distilled water. Any foam was removed with ethanol then the sample was left to stand for 20 min. Next, 30 ml of distilled water was added to 2.5 ml of solution; the pH was adjusted to 3.5 using 0.1 mol·L−1 HCl, and 0.5 ml iodine reagent was added (diluted 2.0 g potassium iodide and 0.2 g iodine with distilled water to 100 ml). A blank solution without an iodine reagent was diluted to 50 ml then left to stand for 20 min for use as a control. Colorimetry was then performed using a spectrophotometer at wavelengths of 630 and 460 nm (Agilent Cary 300, USA). The amylose content was obtained using the amylose standard curve then the amylopectin content was determined as follows:
Amylopectin content (%) = total starch content (%)−amylose content (%).
2.3.5 Grain filling measurements
Every 5 days from 10 days after anthesis, 15–20 wheat ears showing consistent growth and development were sampled. The grains were removed then fixed at 105°C for 15 min, dried at 80°C to a constant weight, weighted, and then converted into the 1000-grain weight. The Logistic equation Y = K/[1+exp(A+Bt)] (Darroch and Baker, 1990) was used to fit the variation in 1000-grain weight with the number of days after anthesis, where Y is the 1000-grain weight of the observed grain (g), t is the number of days (d) from anthesis until observation, A and B are determined parameters, and K is the fitted maximum 1000-grain weight (g). Least-squares estimates of K, A, and B were determined by nonlinear regression. The first and second derivatives of this Logistic equation are obtained, and the following parameters were then determined:
Effective days of grain filling (D):
Duration of the gradual grain filling period (D1):
Duration of the rapid grain filling period (D2):
Duration of the slow grain filling period (D3):
Average grain-filling rate (Vmean):
Maximum grain-filling rate (Vmax):
Time point of the maximum grain-filling rate (Tmax):
2.3.6 Yield and contributing factors
After reaching maturity, three boxes of unsampled wheat were selected from each treatment for the analysis of spikes, kernel numbers per spike, thousand-grain weight, and grain yield.
2.4 Data processing and analysis
Data were analyzed using the SPSS 22.0 software to fit the logistic curve with related parameters of starch, each starch component, and grain dry matter data. Duncan’s method was used to perform multiple comparisons of the measured data. Origin 2017 was used for graphing and Photoshop was used for image annotation and processing.
3 Results
3.1 Effects of spraying 6-BA after WS on the morphology of grain and starch granules
3.1.1 Grain morphology
The degree of grain filling gradually deteriorated with increasing WS; however, the effect was alleviated after the application of 6-BA. Under each duration of WS, grain plumpness increased after the application of 6-BA, and the difference in grain fullness was obvious between the treatment and control groups. The grain ripening process was faster in the waterlogging-sensitive variety Sumai 188 (Figure 3B) than the waterlogging-tolerant Yangmai 18 (Figure 3A), while the degree of overall grain fullness was greater in Yangmai 18 than Sumai 188.
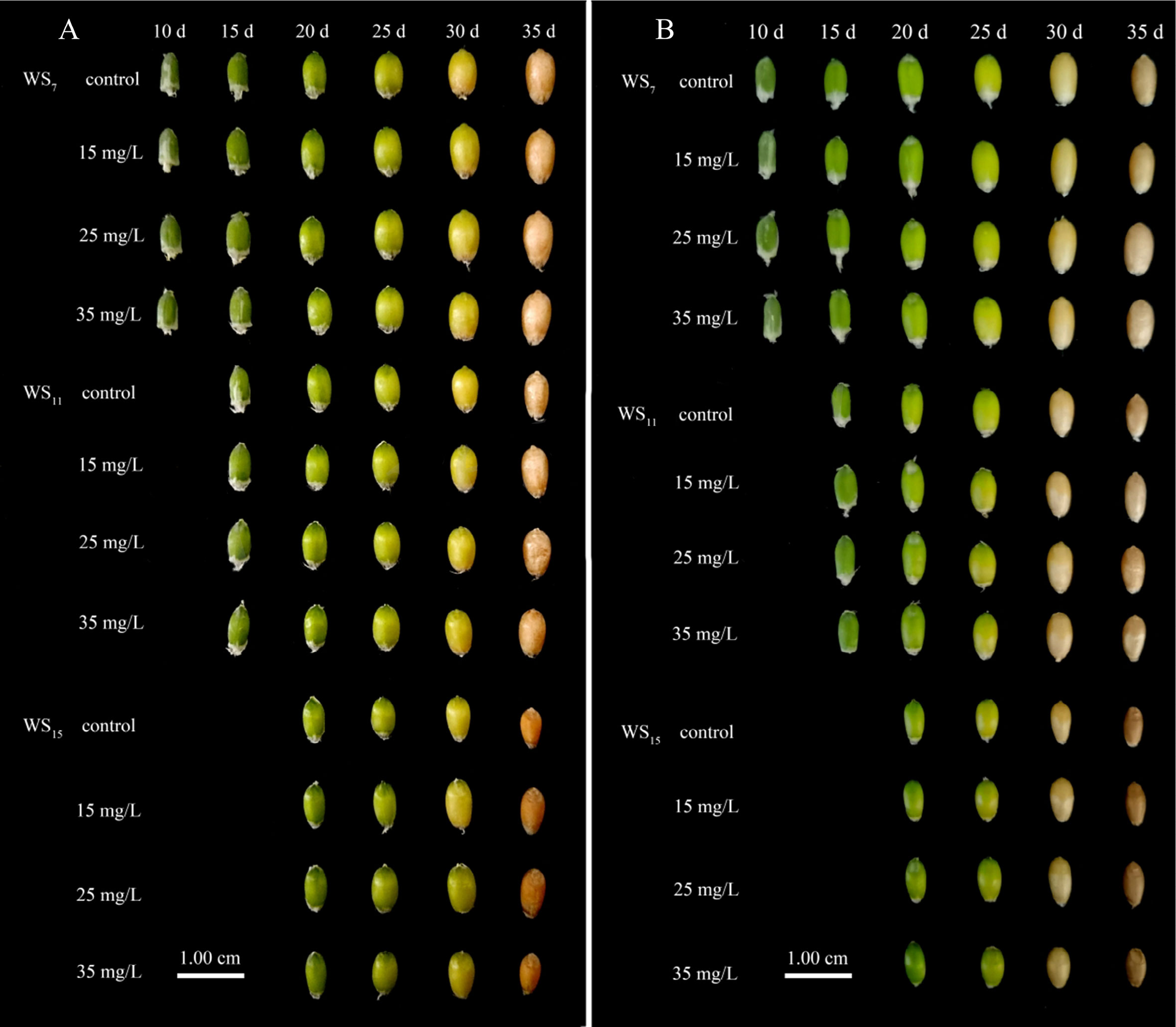
Figure 3 The effect of exogenous 6-BA on grain morphology of wheat under waterlogging and shading. The waterlogging-tolerant (Yangmai 18, 2021 A) and the waterlogging-sensitive (Sumai 188, 2021 B) were waterlogging and shading during anthesis. WS7, WS11, and WS15 respectively represent treatments under waterlogging and shading for 7, 11, and 15 days after anthesis. Shade nets with a shading rate of about 45% were used for shading, and waterlogging preserves about 2 cm of moisture on the surface of the soil. Control is the treatment of spraying the same amount of distilled water; 15 mg·L−1, 25 mg·L−1, and 35 mg·L−1 are the concentrations of 6-BA sprayed.
3.1.2 Morphology of starch granules
The ultrastructure of the wheat grains at maturity was observed in Sumai 188 using a scanning electron microscope (Figure 4). As shown, A- and B-type starch granules in the grain were closely arranged and filled the endosperm cells, with granular proteins in between. Meanwhile, with increasing WS, the cross-sectional area of the grains decreased; moreover, these grains became shrunken and deformed. In terms of starch granule morphology, the longer the duration of WS, the smaller the individual volume of A-type starch granules and the greater the number of deformed B-type granules. After WS for 11 days, some irregular B-type starch granules were observed, while after 15 days, most B-type granules formed irregular polygons. Compared with the control, the volume of A-type starch granules in the grains increased significantly after the application of 6-BA, and deformation of the B-type granules decreased.
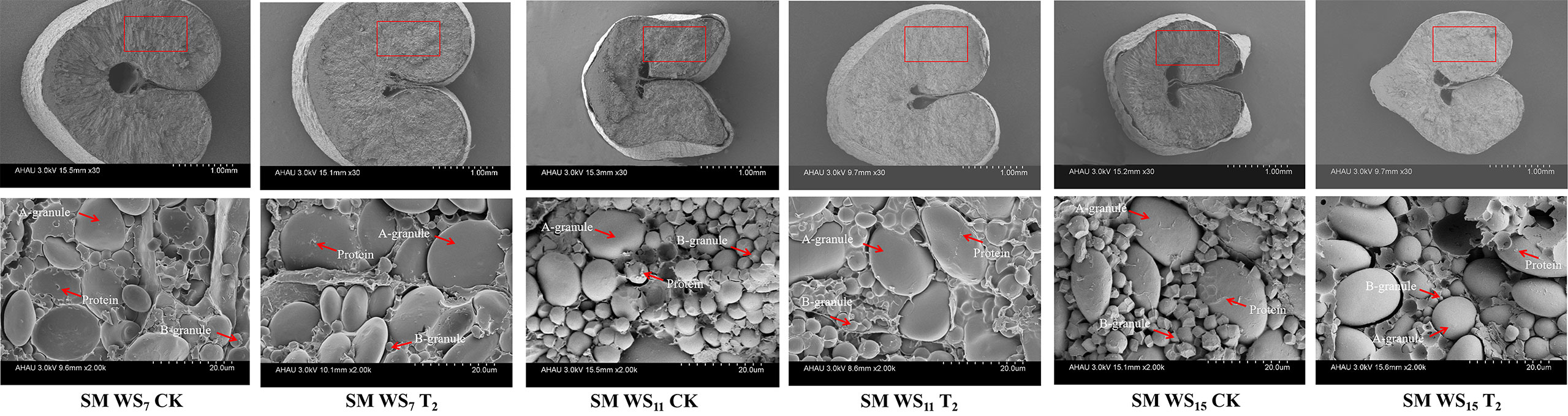
Figure 4 The effects of exogenous 6-BA on final starch grain morphology under waterlogging and shading. SM, Sumai 188, 2021. WS7, WS11, and WS15 respectively represent treatments under waterlogging and shading for 7, 11, and 15 days after anthesis. Shade nets with a shading rate of about 45% were used for shading, and waterlogging preserves about 2 cm of moisture on the surface of the soil. CK refers to the control sprayed with distilled water; T2: the concentration of 6-BA sprayed is 25 mg·L−1. The red rectangles in the graphs indicate the range of areas to be observed.
3.2 Effects of spraying 6-BA after WS on photosynthetic parameters of the flag leaves
As shown in Figure 5A, the Pn of the flag leaves continued to decrease with growth after anthesis, and this decrease increased with increased WS. The Pn of the flag leaves increased significantly after the application of the 6-BA solution (p< 0.05), and was highest at a concentration of 25 mg·L−1 of 6-BA, with significant differences compared with the remaining two treatments (p< 0.05). Taking Sumai 188 after 20 days after anthesis as an example, the Pn of the flag leaves increased by 19.74%, 34.13%, and 25.97% compared with the control under WS treatment for 7 days after spraying 15, 25, and 35 mg·L−1 of the 6-BA solution, respectively. Meanwhile, after 11 days under WS, increases of 28.57%, 37.66%, and 34.38% were observed, while after 15 days, increases of 22.80%, 50.83%, and 36.60% were noted, respectively.
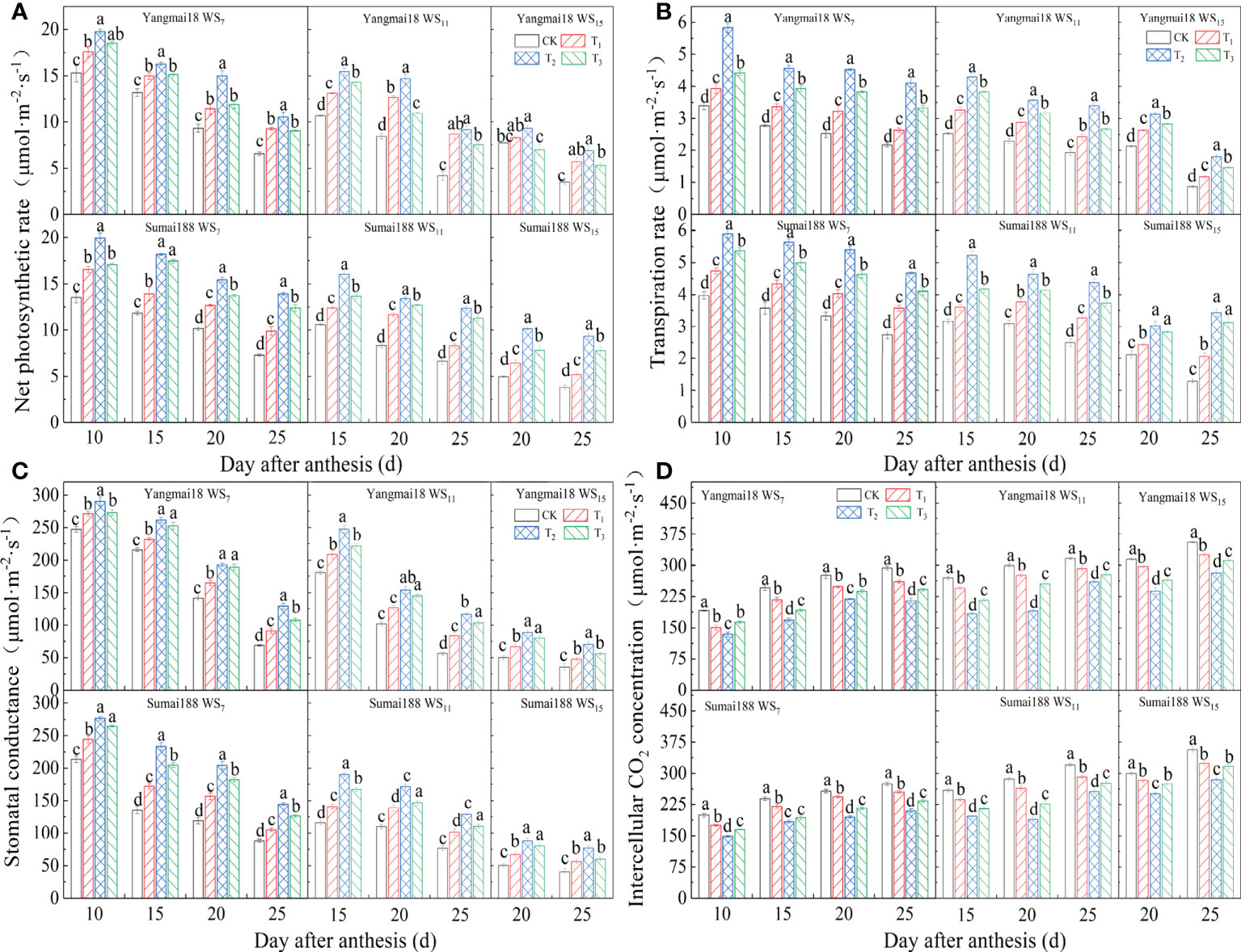
Figure 5 The effect of exogenous 6-BA on Pn (A), Tr (B), Gs (C), Ci (D) of wheat under waterlogging and shading in 2021. WS7, WS11, and WS15 respectively represent treatments under waterlogging and shading for 7, 11, and 15 days after anthesis. Shade nets with a shading rate of about 45% were used for shading, and waterlogging preserves about 2 cm of moisture on the surface of the soil. CK refers to the control sprayed with distilled water. T1, T2, and T3 refer to the concentrations of 6-BA sprayed (15, 25, and 35 mg·L−1). Each value is expressed as mean ± SE (n = 3). Different lowercase letters embedded at the top of the histogram express significant differences between treatments (p< 0.05).
As shown in Figures 5B, C the changes in Gs and Tr in the control flag leaves after anthesis were consistent with those of Pn, with a continual decrease with growth, and WS. In contrast, compared with the control, both Gs and Tr increased significantly after the application of 6-BA (p< 0.05). Meanwhile, as shown in Figure 5D, the Ci of the control flag leaves after anthesis increased gradually with growth and WS. In comparison, the application of 6-BA caused a significant decrease in the Ci of the flag leaves (p< 0.05).
3.3 Effect of spraying 6-BA after WS on the δ13C of the flag leaves and mature grains
As shown in Figure 6, the δ13C of the flag leaves increased significantly by 8.67% compared with the control after spraying 25 mg·L−1 of the 6-BA solution for 24 h, while that of the mature grains increased significantly by 10.77% (p< 0.05). The transport rate of δ13C from the flag leaves to the grains was 92.02% in the control group, while that spraying 25 mg·L−1 of the 6-BA solution was 94.18%. Overall, after application of each concentration of 6-BA, the transport of assimilates from the flag leaves to the grains was significantly improved.
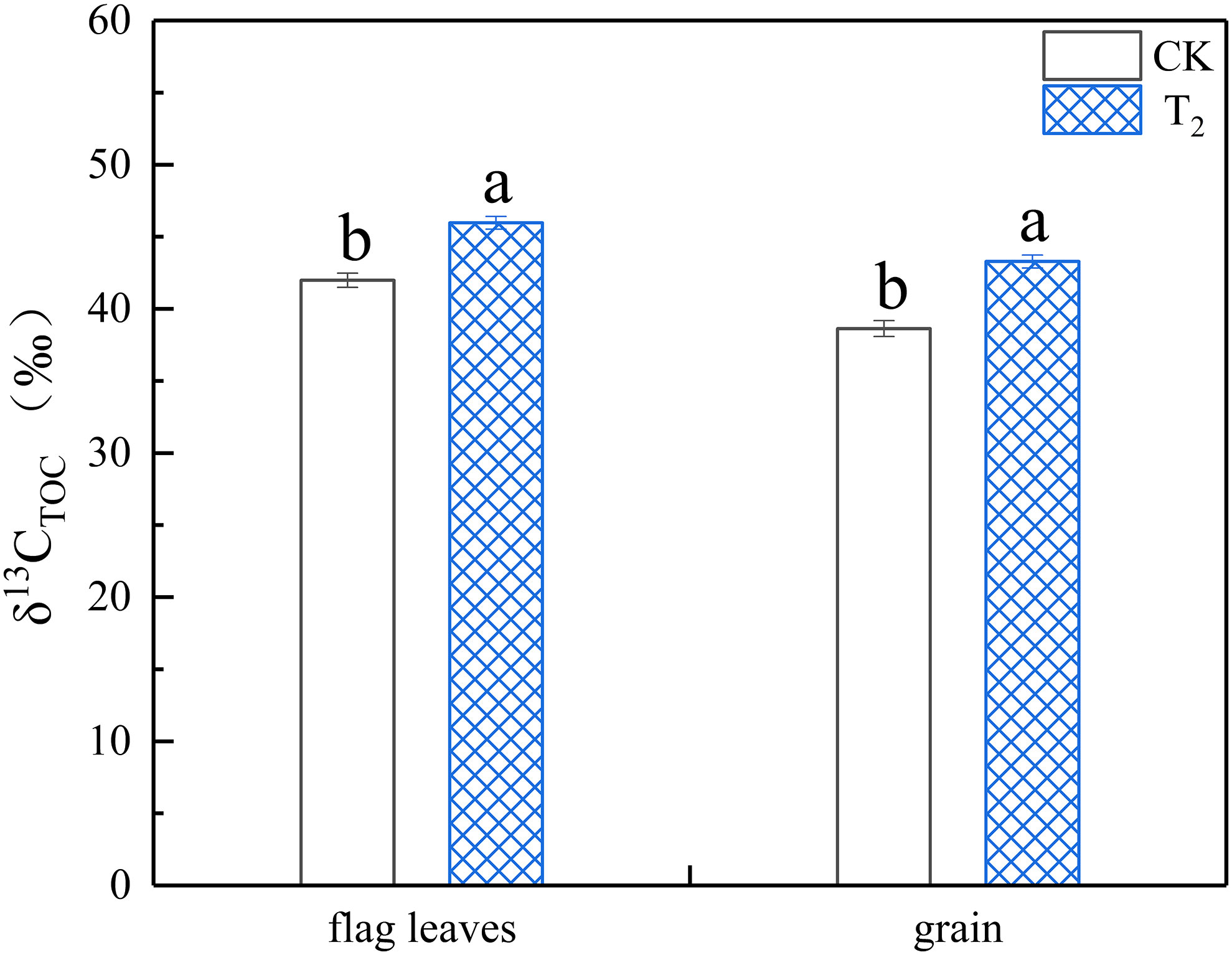
Figure 6 The effect of exogenous 6-BA on flag leaves and grain δ13C of wheat under waterlogging and shading treatment of 11 days in 2021. CK refers to the control sprayed with distilled water. T2: the concentration of 6-BA sprayed is 25 mg·L−1. Shade nets with a shading rate of about 45% were used for shading, and waterlogging preserves about 2 cm of moisture on the surface of the soil. Each value is expressed as mean ± SE (n = 3). Different lowercase letters embedded at the top of the histogram express significant differences among treatments (p< 0.05).
3.4 Effect of spraying 6-BA after WS on the content of grain starch and its component
3.4.1 Starch content
As shown in Figure 7, the growth of the grain starch showed a rapid increase from 15 to 25 days after anthesis followed by gradual stabilization from 25 to 35 days. The longer the duration of WS, the lower the final accumulation of starch; a significant increase was observed following the application of 6-BA during the entire grain-filling process compared with the control (Figures 7A, B, p< 0.05). Moreover, starch accumulation was significantly higher after spraying 25 mg·L−1 of the 6-BA solution compared with the remaining two concentrations. Take the 2021 year results as an example, after WS for 15 days, the final accumulation of starch in the Sumai 188 grains increased by 3.85%, 10.24%, and 5.28% compared with the control after spraying 15, 25, and 35 mg·L−1 of the 6-BA solution, respectively.
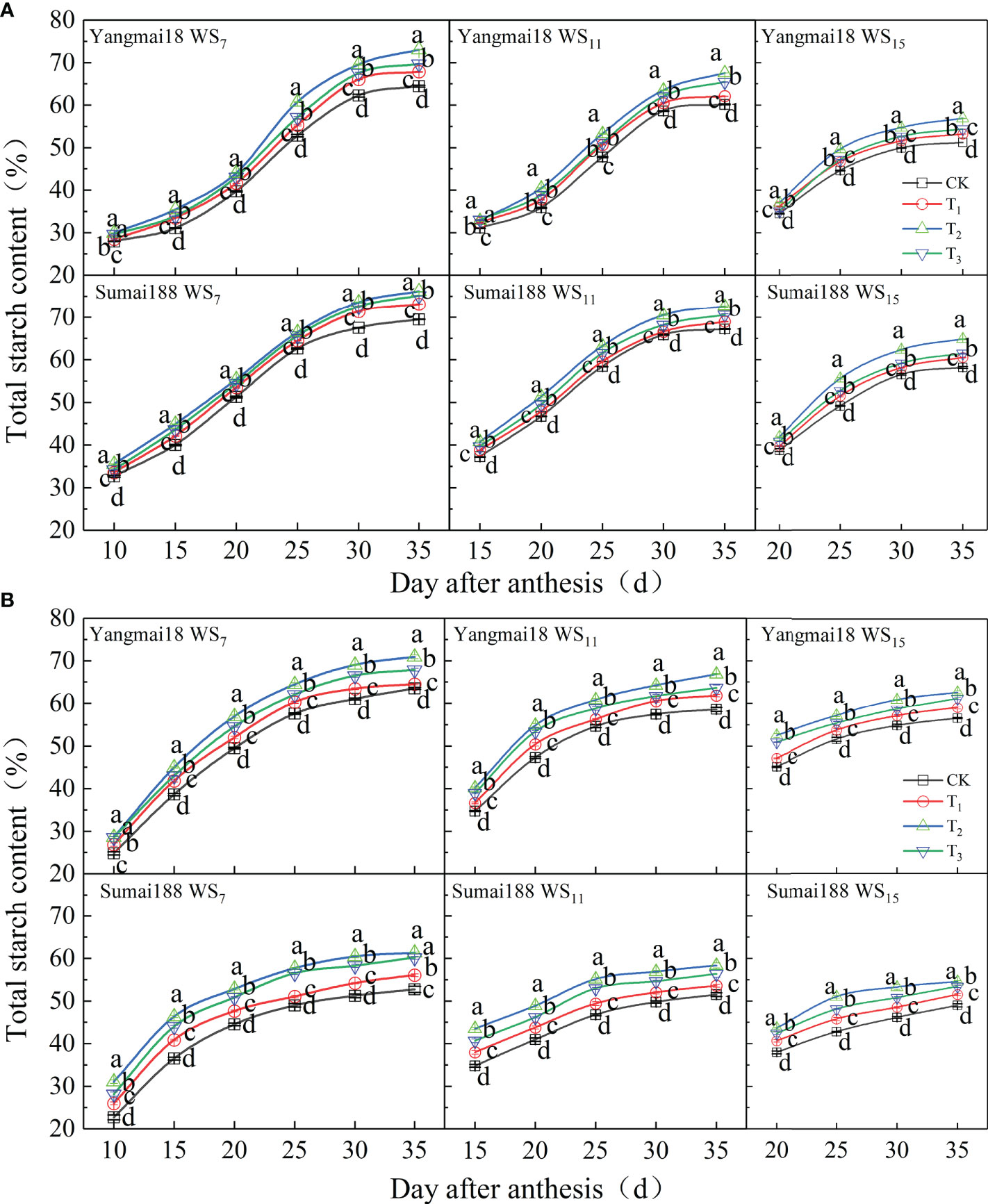
Figure 7 The effect of exogenous 6-BA on total starch accumulation of wheat under waterlogging and shading in 2021 (A) and 2022 (B). WS7, WS11, and WS15 respectively represent treatments under waterlogging and shading for 7, 11, and 15 days after anthesis. Shade nets with a shading rate of about 45% were used for shading, and waterlogging preserves about 2 cm of moisture on the surface of the soil. CK refers to the control sprayed with distilled water. T1, T2, and T3 refer to the concentration of 6-BA sprayed (15, 25, and 35 mg·L−1). Each value is expressed as mean ± SE (n = 3). Different lowercase letters embedded in the linear graph express significant differences among treatments (p< 0.05).
3.4.2 Amylose and amylopectin content
As shown in Tables 2, 3, the amylose and amylopectin content gradually increased with wheat grain development; however, the longer the duration of WS, the lower the final accumulation. The different cultivars, treatment durations of WS, and spraying concentrations of 6-BA significantly affected the final amylose and amylopectin contents from wheat grains. Under the same treatment duration of WS, except at 10 days after anthesis, the content of amylose and amylopectin was improved remarkably following the application of 6-BA in contrast with the control treatment during the grouting period (p< 0.05), suggesting that 6-BA improved the accumulation of amylose and amylopectin in the wheat grains. As an example, after WS for 15 days, the final amylose content of the Sumai 188 grains increased by 3.44%, 6.60%, and 3.15% compared with the control after spraying 15, 25, and 35 mg·L−1 of the 6-BA solution, while the final accumulation of amylopectin increased by 3.95%, 11.15%, and 5.82%, respectively.
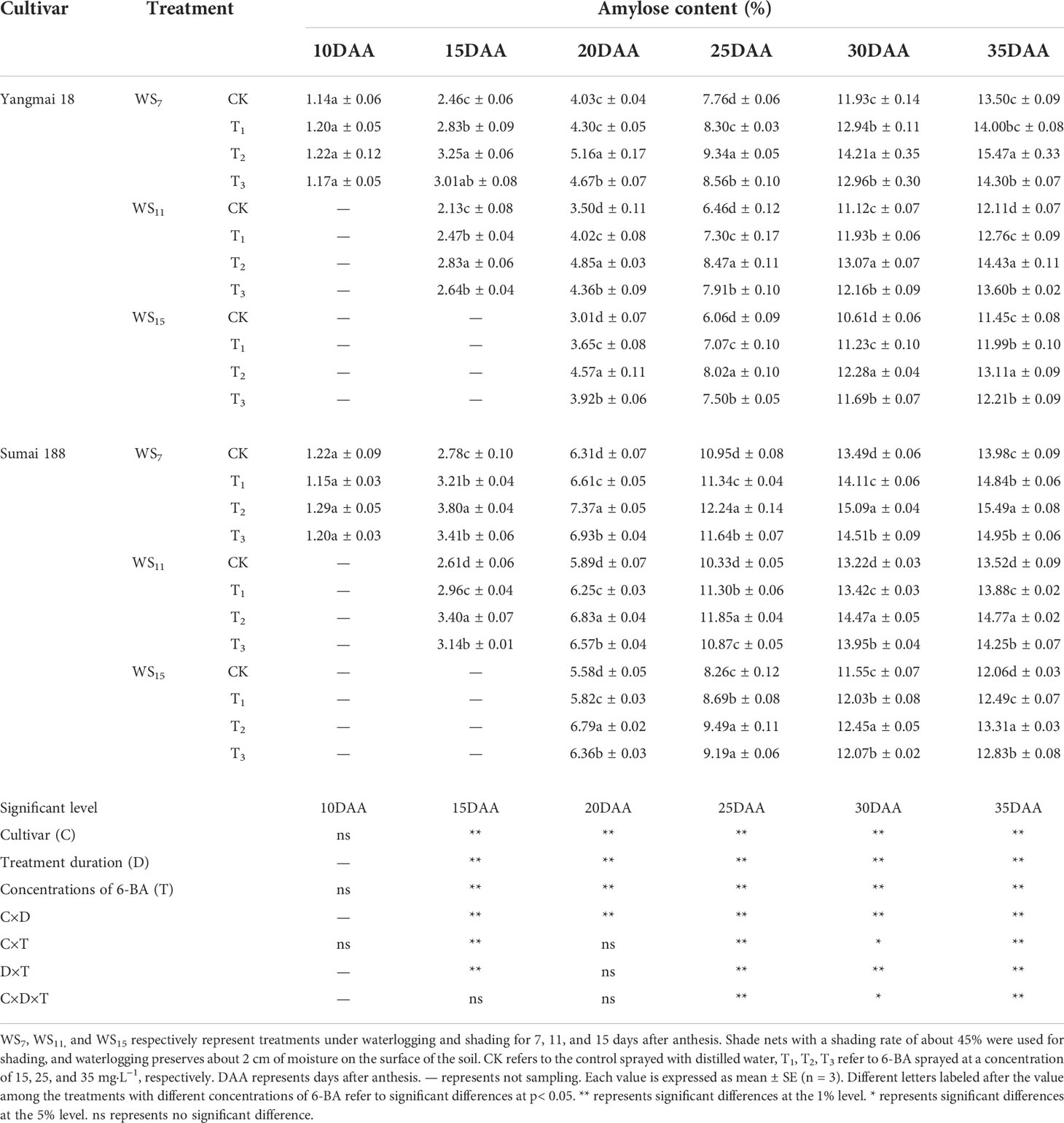
Table 2 The effect of exogenous 6-BA on amylose accumulation of wheat under waterlogging and shading in 2021.
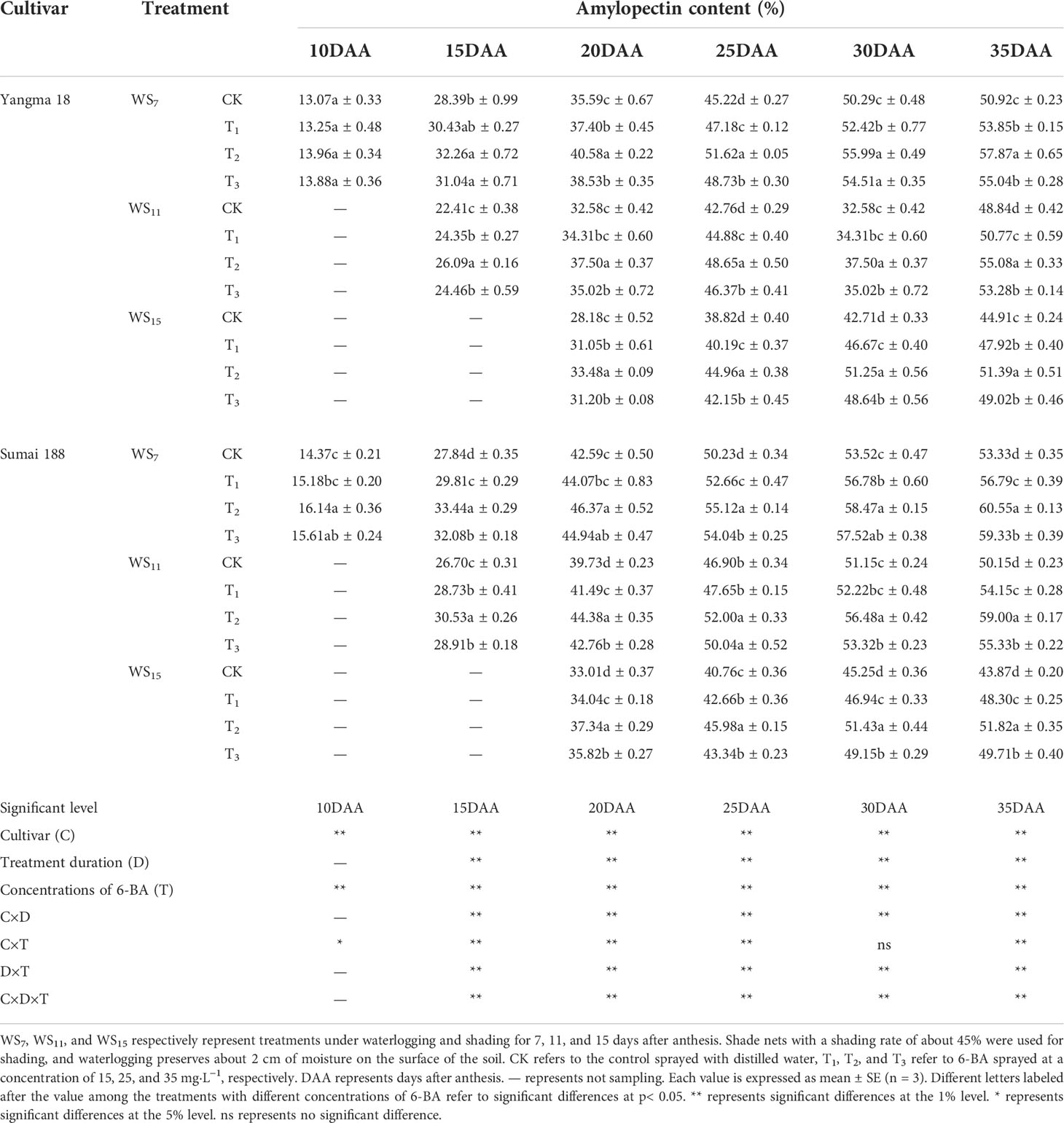
Table 3 The effect of exogenous 6-BA on amylopectin accumulation of wheat under waterlogging and shading in 2021.
3.5 Effect of spraying 6-BA after WS on grain filling
A logistic equation served to fit the grain-filling, with the coefficient factor of each equation reaching a notable level (Table 4). The maximum theoretical 1000-kernel weight was higher after the application of 6-BA compared with the control, and the number of effective days of grain filling (D) was prolonged in both cultivars (except in Yangmai 18 after WS for 15 days and Sumai 188 after WS for 11 days in 2021, and after WS for 7 days during in 2022). Moreover, under the same treatment duration of WS, the average grain filling rate (Vmean) increased significantly after spraying the 6-BA solution, and the longer the treatment duration of WS, the lower the average grain filling rate. Compared with the control, spraying the 6-BA solution increased the duration of gradual grain filling in Yangmai 18 following 7 days of WS in 2021, while the rapid and slow grain filling periods increased following 11 and 15 days of WS in 2021 and 2022. Of the three concentrations of 6-BA, spraying 25 mg·L−1 of the 6-BA solution had the greatest effect. Take the 2021 results as an example; in Yangmai 18, spraying 25 mg·L−1 of the 6-BA solution following 7, 11, and 15 days of WS resulted in an increase in Vmean by 6.27%, 5.59%, and 21.20% compared with the control, while in Sumai 188, increases of 7.84%, 5.08%, and 15.11% were observed, respectively.
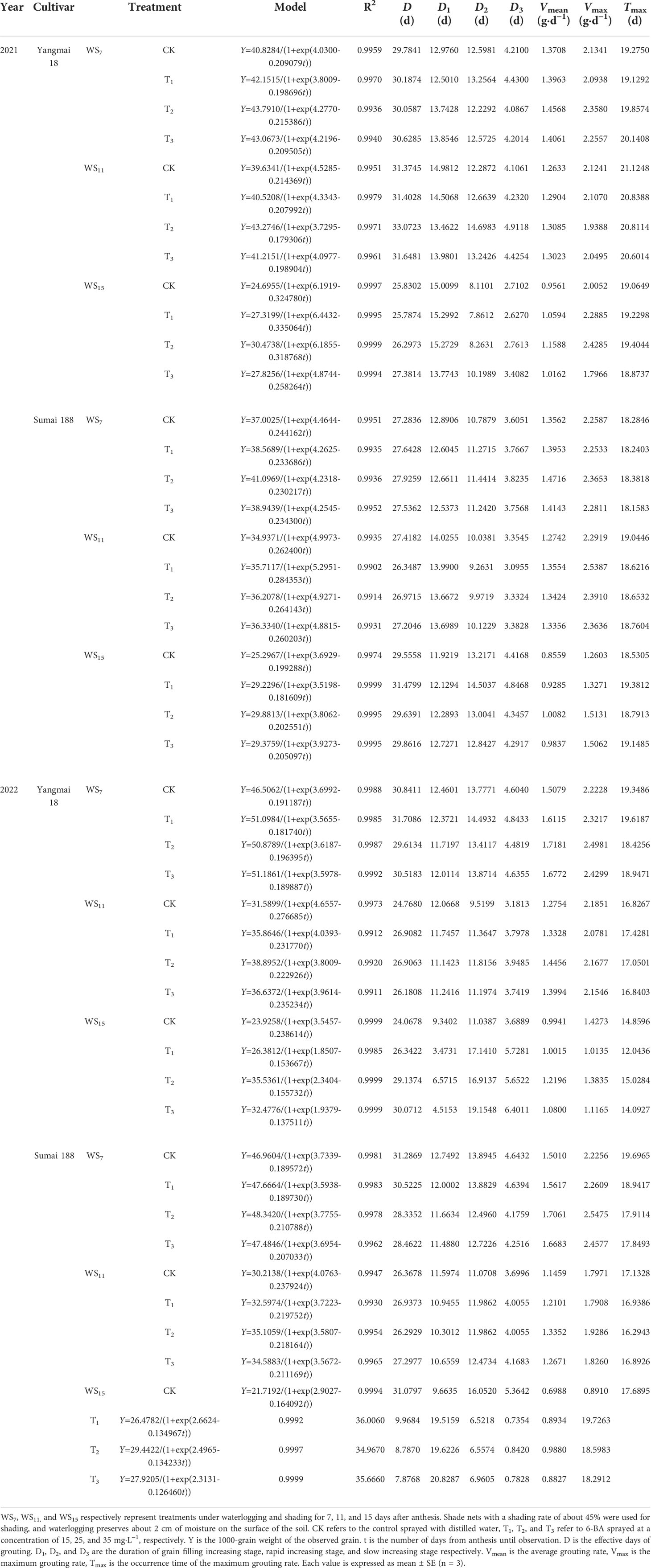
Table 4 The effect of exogenous 6-BA on the grout characteristic of wheat grain under waterlogging and shading.
3.6 Effect of spraying 6-BA after WS on yield and contributing factors
As shown in Table 5, the longer the duration of WS, the lower the number of grains per spike, the thousand-grain weight, and the yield. The different cultivars, treatment durations of WS, and spraying concentrations of 6-BA significantly affected the thousand-grain weight and yield from wheat. Moreover, under the same treatment duration of WS, compared with the control, the application of 6-BA after WS caused a remarkable improvement in the thousand-grain weight and yield in both varieties (p< 0.05); however, there were little difference in the spike numbers and kernel numbers per spike. Of the three 6-BA concentrations, spraying 25 mg·L−1 of the 6-BA solution had the greatest effect on the thousand-grain weight and yield. Take the results of the wheat growing season from 2020 to 2021 as an example; in Yangmai18, spraying 25 mg·L−1 of the 6-BA solution following 7, 11, and 15 days of WS caused an increase in the thousand-grain weight by 5.06%, 7.97%, and 30.52%, and an increase in yield of 9.43%, 18.23%, and 28.15% compared with the control, respectively. Meanwhile, in Sumai 188, the thousand-grain weight increased by 11.21%, 7.23%, and 17.73%, while yield increased by 15.64%, 8.93%, and 24.89%, respectively. Taken together, these findings suggest that the application of 6-BA had the greatest influence on yield after 15 days of WS.
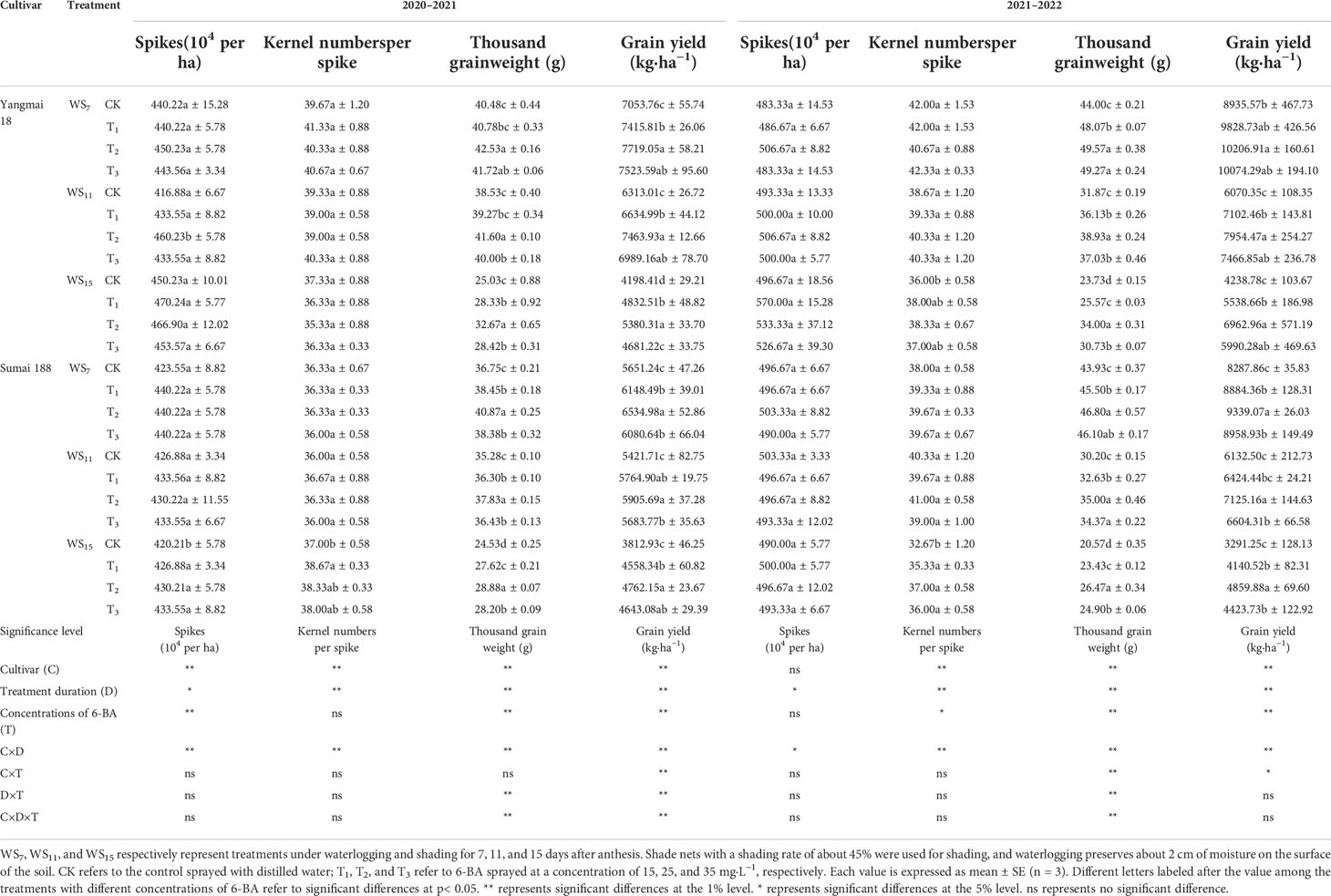
Table 5 The effect of exogenous 6-BA on wheat yield and its components under waterlogging and shading.
4 Discussion
4.1 Effects of spraying 6-BA after WS on photosynthetic parameters and photosynthetic product transport
Studies have shown that improvements in the photosynthetic area of functional leaves, the photosynthetic duration, and the photosynthetic capacity can improve the grain yield of crops (Parry et al., 2011). Studies have shown that waterlogging accelerates the leaf senescence in wheat, especially under soil compaction, thereby significantly reducing leaf SPAD values as well as Pn, Gs, and Tr, which affects the overall photosynthetic capacity (Wu W. et al., 2018b; Wu X. et al., 2018a). Similarly, insufficient light is also known to limit photosynthesis in wheat (Shimoda and Sugikawa, 2020). This study also found that the Pn of wheat flag leaves decreased rapidly with the increase of WS treatment duration, especially after 15 days of WS treatment. However, the self-regulation effect of wheat can only resist the damage caused by WS stress in a short time (Liu et al., 2007), thus taking flag leaf photosynthesis at a low level under the 15 days of WS. Meanwhile, studies have also shown that spraying the 6-BA solution before waterlogging can increase the photosynthetic rate of the flag leaves, slowing down the rate of plant senescence, and reducing yield losses (Wang X. et al., 2020a). Notably, maintaining the photosynthetic rate of flag leaves after anthesis was found to effectively increase biomass and yield (Luo et al., 2006; Chen et al., 2010). In this study, spraying the 6-BA solution after WS caused a significant increase in the Pn, Gs, and Tr of the flag leaves, and a reduction in the Ci. These findings suggest that the stomatal limitations of photosynthesis under WS was relieved by 6-BA, elevating the photosynthesis of wheat flag leaf. In line with this, maintaining a longer photosynthetically active period during grain filling, which allows the transfer of more assimilates into the grains, was previously found to improve resistance to abiotic stress after anthesis (Chen et al., 2010). Therefore, in this study, spraying 6-BA prolonged the photosynthetic activity period of wheat leaves after anthesis, especially under the treatment of WS for 7 days; and spraying 6-BA prolonged the duration of grain filling; the photosynthetic rate of flag leaves of wheat decreased slowly, which increased the amount of photosynthetic products produced by leaves into grains. Moreover, spraying 6-BA can alleviate the effects of waterlogging on the chloroplast and mitochondrial structure, thereby increasing the chlorophyll content and improving the photosynthetic performance of maize after waterlogging (Ren et al., 2017). These results confirmed that spraying 6-BA could improve the photosynthetic capacity of leaves.
After the wheat leaves absorbed 13CO2, we found that the ability of flag leaves to assimilate CO2 improved after spraying the 6-BA solution, and the accumulation of photosynthetic products in grains also increased. 6-BA improved the ability of the flag leaves to assimilate CO2, thereby increasing the transportation rate of assimilates. This may be because exogenous 6-BA reduces the damage to the photosynthetic system, improving the assimilation efficiency of CO2, which allows the generation of more carbohydrates, thus meeting the needs of plant growth (Ding et al., 2013). Therefore, the results of this study showed that spraying 6-BA could not only improve the photosynthetic capacity of flag leaves but also alleviate the transport process of carbohydrates generated by flag leaves to grains, and this process was also related to the transport of photosynthates in stem and stem sheath. It was found that 7 days after spraying the 6-BA solution, the contents of soluble sugar and starch in different organs of maize (leaves, sheaths, stems and young corn cobs) increased, and 6-BA could significantly improve the translocation rate of photosynthetic products from maize leaves to maize grains (Yang et al., 2019).
4.2 Effects of spraying 6-BA after WS on starch granules and starch content
Wheat grain starch exists as starch granules that differ in shape, size, composition, and properties (Shewry, 2010; Vamadevan, 2013; Guo et al., 2019; Yan et al., 2021). Studies have shown that WS reduces the number and volume of A-type starch granules (Li et al., 2020), with deformation of the granules under stress (Wang and Copeland, 2012). However, 6-BA can restore the adverse effects of ethephon on the number and size of starch granules and increase the storage capacity of grains (Liu et al., 2018). In this study, spraying 6-BA after WS could increase the volume of A-type starch granules and reduce the deformation of B-type starch granules, which indicated that spraying 6-BA can alleviate the formation and development of starch granules.
The enrichment process of wheat grains under WS is largely related to the accumulation of starch in the endosperm (Tomlinson and Denyer, 2003). The content and ratio of amylose to amylopectin has an effect on starch structure, gelatinization, and thermal properties (Wang H. et al., 2020b). 6-BA and ABA are the most potent phytohormones in terms of increased biomass and starch accumulation (Liu et al., 2019). In this study, in contrast with the control, the application of 6-BA significantly increased the starch content and starch accumulation rate in grains, and alleviated the effect of WS stress on starch accumulation. The enhanced photosynthetic capacity of wheat after spraying 6-BA increased the photosynthate produced by flag leaves, which improved the amount of carbohydrates transported to grains and resulted in the increase of starch accumulation. Moreover, the application of 6-BA was found to increase the content of starch in various organs, promoting the transport and subsequent accumulation of carbohydrates in the grains (Yang et al., 2019). The activity of starch-related enzymes is able to regulate the starch synthesis rate, thereby affecting carbohydrate synthesis (Ran et al., 2020; Wang et al., 2021); however, spraying the 6-BA solution can improve the activity of enzymes related to starch synthesis, which causes an increase in starch accumulation (Hsu et al., 2014; Luo et al., 2015; Wang et al., 2022).
4.3 Effects of spraying 6-BA after WS on grain filling and yield
The rate and duration of grain filling are the decisive factors of the final grain weight of wheat kernels (Duguid and Br Lé-Babel, 1994; Gelang et al., 2000). Previous studies revealed a significant increase in wheat grain weight and yield with increasing waterlogging (Arata et al., 2019; Ding et al., 2020), while shading treatment caused a significant drop in wheat grain yield (Yang et al., 2020). Meanwhile, studies have also shown that spraying the 6-BA solution before shading can delay the senescence process of flag leaves under shading treatment, improving dry matter accumulation and, ultimately, grain yield (Li et al., 2019). In this study, the application of 6-BA after WS stress could prolong the photosynthetic activity period of wheat leaves after anthesis, which is propitious to the accumulation and transport of photosynthetic products, and thus increase grain weight and grain yield (Figure 8). These findings are related to the fact that exogenous 6-BA can delay leaf senescence, increase the chlorophyll content, and improve photosynthetic performance, all of which effectively enhance the grain-filling characteristics and photosynthesis of crops under waterlogging stress, ultimately having a significant effect on yield (Ren et al., 2016).
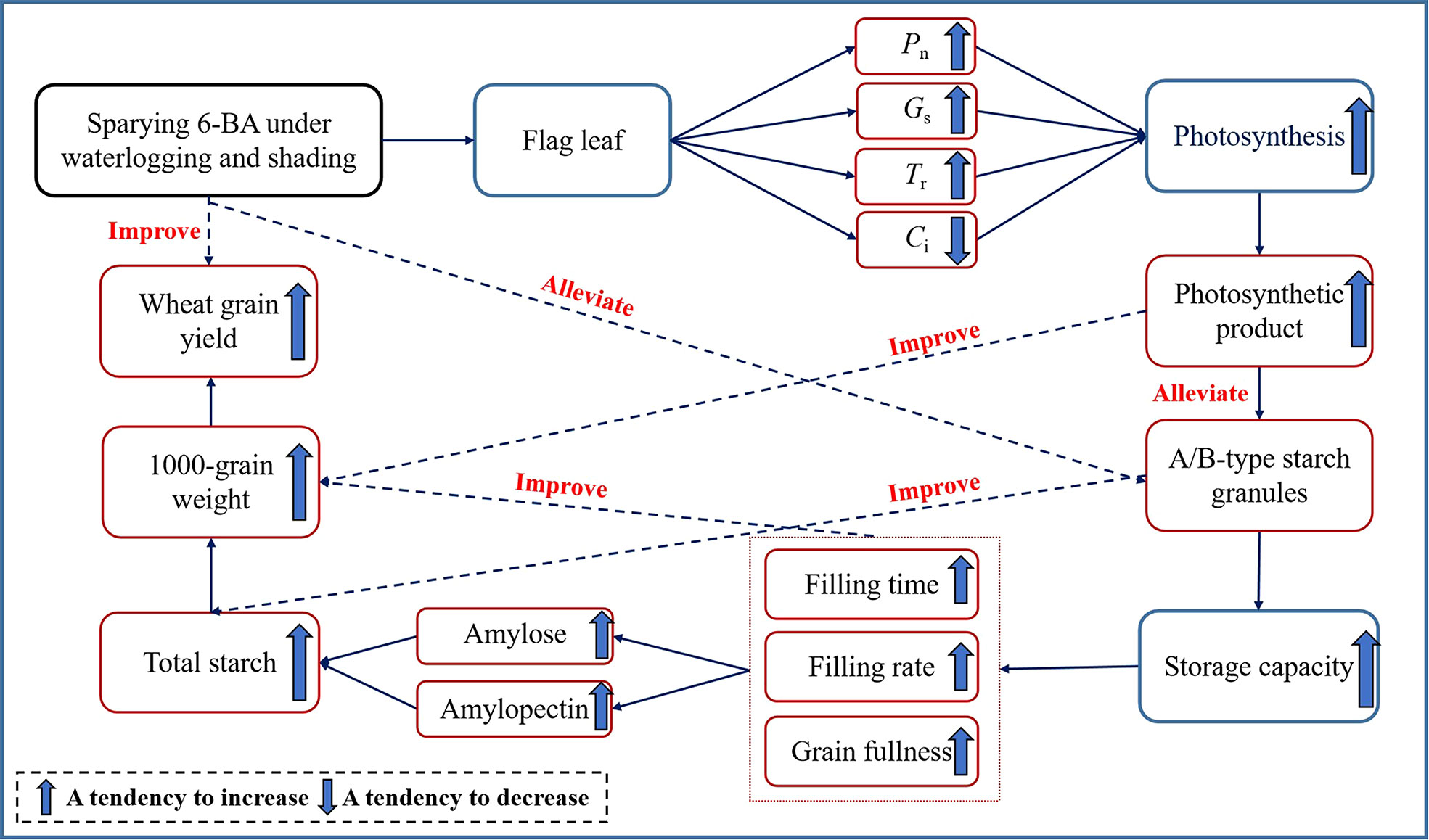
Figure 8 Analysis of the ways of spraying 6-BA to improve wheat yield under waterlogging and shading after anthesis.
The grain filling process is directly affected by the storage capacity, and the number of starch granule can affect the grain-filling, and finally affect the dry matter accumulation in the grain, thereby affecting the yield (Tetlow and Emes, 2017; Zheng et al., 2017; Xie et al., 2018). In this study, spraying 6-BA can alleviate the adverse influence of WS on the formation and development of wheat starch granules, to further expand the storage capacity of wheat grains after WS and increase the duration and rate of grain filling and the fullness degree of grains. However, the duration and rate of grain filling were positively correlated with the grain weight (Dias and Lidon, 2009), so 6-BA application eventually improved wheat grain weight and yield (Figure 8).
5 Conclusions
Exogenous spraying of the 6-BA solution could improve photosynthesis in the flag leaves and alleviate the negative influence on starch granules, increase the content of starch and its components, and improve the duration and rate of grain filling, thus resulting in improvement of grain weight. In addition, under the conditions of this study, spraying 25 mg·L−1 of the 6-BA solution had an optimal effect with three different spray concentrations.
Data availability statement
The original contributions presented in the study are included in the article/supplementary material. Further inquiries can be directed to the corresponding author.
Author contributions
WZ and ZH designed the experiment. WZ and BW initiated statistical analysis and drafted the manuscript. BW, AZ, and QZ performed the experiments and determined related data. YL and LL contributed to the experiments proceeding and data interpretation. SM and YF assisted in polishing the manuscript. All authors contributed to the article and approved the submitted version.
Funding
This work was supported by the Key Research and Development Plan of Anhui Province (grant numbers 202204c06020040), the University Natural Science Research Project of Anhui Province (grant numbers YJS20210251), and the National Key Research and Development Plan of China (grant numbers 2017YFD0300205).
Conflict of interest
The authors declare that the research was conducted in the absence of any commercial or financial relationships that could be construed as a potential conflict of interest.
Publisher’s note
All claims expressed in this article are solely those of the authors and do not necessarily represent those of their affiliated organizations, or those of the publisher, the editors and the reviewers. Any product that may be evaluated in this article, or claim that may be made by its manufacturer, is not guaranteed or endorsed by the publisher.
References
Andrade, C. A., Souza, K. R. D. D., Santos, M. D. O., Silva, D. M. D., Alves, J. D. (2018). Hydrogen peroxide promotes the tolerance of soybeans to waterlogging. Sci. Hortic. 232, 40–45. doi: 10.1016/j.scienta.2017.12.048
Arata, A. F., Dinolfo, M. I., Martínez, M., Lázaro, L. (2019). Effects of waterlogging during grain filling on yield components, nitrogen uptake and grain quality in bread wheat. Cereal Res. Commun. 47, 42–52. doi: 10.1556/0806.46.2018.058
Boru, G., van Ginkel, M., Kronstad, W. E., Boersma, L. (2001). Expression and inheritance of tolerance to waterlogging stress in wheat. Euphytica 117, 91–98. doi: 10.1023/A:1003929803920
Chen, J. B., Liang, Y., Hu, X. Y., Wang, X. X., Tan, F. Q., Zhang, H. Q., et al. (2010). Physiological characterization of ‘stay green’ wheat cultivars during the grain filling stage under field growing conditions. Acta Physiol. Plant 32, 875–882. doi: 10.1007/s11738-010-0475-0
Darroch, B. A., Baker, R. J. (1990). Grain filling in three spring wheat genotypes: Statistical analysis. Crop Sci. 30, 525–529. doi: 10.2135/cropsci1990.0011183X003000030009x
Demotes-Mainard, S., Jeuffroy, M. (2004). Effects of nitrogen and radiation on dry matter and nitrogen accumulation in the spike of winter wheat. Field Crops. Res. 87, 221–233. doi: 10.1016/j.fcr.2003.11.014
Dias, A. S., Lidon, F. C. (2009). Evaluation of grain filling rate and duration in bread and durum wheat, under heat stress after anthesis. J. Agron. Crop Sci. 195, 137–147. doi: 10.1111/j.1439-037X.2008.00347.x
Ding, J. F., Liang, P., Wu, P., Zhu, M., Li, C. Y., Zhu, X. K. (2020). And Identifying the critical stage near anthesis for waterlogging on wheat yield and its components in the Yangtze river basin, China. Agronomy 10, 130. doi: 10.3390/agronomy10010130
Ding, X. T., Wang, H., Jin, H. J., Zhang, H. M., Hong, C. C., Yu, J. Z. (2013). Effects of cytokinin on photosynthetic gas exchange, chlorophyll fluorescence parameters, antioxidative system and carbohydrate accumulation in cucumber (Cucumis sativus l.) under low light. Acta Physiol. Plant 35, 1427–1438. doi: 10.1007/s11738-012-1182-9
Duguid, S. D., Br Lé-Babel, A. L. (1994). Rate and duration of grain filling in five spring wheat (Triticum aestivum l.) genotypes. Can. J. Plant Sci. 74, 681–686. doi: 10.4141/cjps94-123
Gao, J., Shi, J. G., Dong, S. T., Liu, P., Zhao, B., Zhang, J. W. (2017). Response of endosperm cell proliferation and grain yield of summer maize to different light conditions. Acta Agron. Sin. 43, 1548–1558. doi: 10.3724/SP.J.1006.2017.01548
Geigenberger, P. (2003). Response of plant metabolism to too little oxygen. Curr. Opin. Plant Biol. 6, 247–256. doi: 10.1016/S1369-5266(03)00038-4
Gelang, J. G. U. S., Pliejel, H., Sild, E., Danielsson, H., Younis, S., Sellden, G. (2000). Rate and duration of grain filling in relation to flag leaf senescence and grain yield in spring wheat (Triticum aestivum) exposed to different concentrations of ozone. Physiol. Plant 110, 366–375. doi: 10.1111/j.1399-3054.2000.1100311.x
Ghasem, P., Ali, E., Masoud, H., Barbara, H. N., Carol, B., Soodabe, J. (2022). Plant growth regulators improve grain production and water use efficiency of Foeniculum vulgare mill. under water stress. Plants 11, 1718. doi: 10.3390/PLANTS11131718
Golay, A., Schneider, H., Temler, E., Felber, J. P. (1991). Effect of trestatin, an amylase inhibitor, incorporated into bread, on glycemic responses in normal and diabetic patients. Am. J. Clin. Nutr. 53, 61–65. doi: 10.1093/ajcn/53.1.61
Guo, L., Deng, Y. F., Lu, L., Zou, F. X., Cui, B. (2019). Synergistic effects of branching enzyme and transglucosidase on the modification of potato starch granules. Int. J. Biol. Macromol. 130, 499–507. doi: 10.1016/j.ijbiomac.2019.02.160
Hafez, E., Farig, M. (2019). Efficacy of salicylic acid as a cofactor for ameliorating effects of water stress and enhancing wheat yield and water use efficiency in saline soil. Int. J. Plant Prod. 13, 163–176. doi: 10.1007/s42106-019-00036-w
Holm, J., Björck, I. (1992). Bioavailability of starch in various wheat-based bread products: Evaluation of metabolic responses in healthy subjects and rate and extent of in vitro starch digestion. Am. J. Clin. Nutr. 55, 420–429. doi: 10.1016/0923-2508(92)90007-B
Hsu, Y. C., Tseng, M. C., Wu, Y. P., Lin, M. Y., Wei, F. J., Hwu, K. K., et al. (2014). Genetic factors responsible for eating and cooking qualities of rice grains in a recombinant inbred population of an inter-subspecific cross. Mol. Breed. 34, 655–673. doi: 10.1007/s11032-014-0065-8
Jia, W. S., Zhang, J. H. (2008). Stomatal movements and long-distance signaling in plants. Plant Signal. Behav. 3, 772–777. doi: 10.4161/PSB.3.10.6294
Kim, Y., Seo, C., Khan, A. L., Mun, B., Shahzad, R., Ko, J., et al. (2018). Exo-ethylene application mitigates waterlogging stress in soybean (Glycine max l.). BMC Plant Biol. 18, 254. doi: 10.1186/s12870-018-1457-4
Liu, Y., Chen, X. Y., Wang, X. H., Fang, Y., Zhang, Y., Huang, M. J., et al. (2019). The influence of different plant hormones on biomass and starch accumulation of duckweed: A renewable feedstock for bioethanol production. Renew. Energ. 138, 659–665. doi: 10.1016/j.renene.2019.01.128
Liu, J. H., Kitashiba, H., Wang, J., Ban, Y., Moriguchi, T. (2007). Polyamines and their ability to provide environmental stress tolerance to plants. Plant Biotechnol. 24, 117–126. doi: 10.5511/plantbiotechnology.24.117
Liu, X. Q., Zhan, Y. T., Wang, Y., Wang, P., Yin, S. K., Han, C. X., et al. (2018). Effect of some plant growth regulators used by stages treatments on endosperm cell and starch granule in maize endosperm development. Acta Agric. Boreali-Sin. 33, 137–144. doi: 10.7668/hbnxb.2018.06.019
Li, H. W., Wang, Z. S., Zhuo, Q. C., Zhang, B., Wang, F. H., Jiang, D. (2020). Starch granule size distribution and pasting characteristic response to post-anthesis combined stress of waterlogging and shading. Agric. (Basel). 10, 384. doi: 10.3390/agriculture10090384
Li, L. L., Wei, M. M., Li, X., Wang, X. Y. (2019). Effects of exogenous 6-BA on the yield of wheat after rice in the jianghan plain under different shading treatments. Chin. J. Appl. Ecol. 30, 3753–3761. doi: 10.13287/j.1001-9332.201911.024
Luo, J., Jobling, S. A., Millar, A., Morell, M. K., Li, Z. (2015). Allelic effects on starch structure and properties of six starch biosynthetic genes in a rice recombinant inbred line population. Rice (N.Y.). 8, 15. doi: 10.1186/s12284-015-0046-5
Luo, P. G., Ren, Z. L., Wu, X. H., Zhang, H. Y., Zhang, H. Q., Feng, J. (2006). Structural and biochemical mechanism responsible for the stay-green phenotype in common wheat. Chin. Sci. Bull. 51, 2595–2603. doi: 10.1007/s11434-006-2175-0
Mu, H., Jiang, D., Wollenweber, B., Dai, T. F., Qing, J., Cao, W. (2010). Long-term low radiation decreases leaf photosynthesis, photochemical efficiency and grain yield in winter wheat. J. Agron. Crop Sci. 196, 38–47. doi: 10.1111/j.1439-037X.2009.00394.x
Parry, M. A. J., Reynolds, M., Salvucci, M. E., Raines, C., Andralojc, P. J., Zhu, X., et al. (2011). Raising yield potential of wheat. II. increasing photosynthetic capacity and efficiency. J. Exp. Bot. 62, 453–467. doi: 10.1093/jxb/erq304
Ran, L. P., Yu, X. R., Li, Y. Q., Zou, J. C., Deng, J. W., Pan, J. Y., et al. (2020). Analysis of development, accumulation and structural characteristics of starch granule in wheat grain under nitrogen application. Int. J. Biol. Macromol. 164, 3739–3750. doi: 10.1016/j.ijbiomac.2020.08.192
Ren, B. Z., Zhang, J. W., Dong, S. T., Liu, P., Zhao, B. (2017). Regulations of 6-benzyladenine (6-BA) on leaf ultrastructure and photosynthetic characteristics of waterlogged summer maize. J. Plant Growth Regul. 36, 743–754. doi: 10.1007/s00344-017-9677-7
Ren, B. Z., Zhu, Y. L., Zhang, J. W., Dong, S. T., Liu, P., Zhao, B. (2016). Effects of spraying exogenous hormone 6-benzyladenine (6-BA) after waterlogging on grain yield and growth of summer maize. Field Crops Res. 188, 96–104. doi: 10.1016/j.fcr.2015.10.016
Shao, G., Lan, J., Yu, S., Liu, N., Guo, R., She, D. (2013). Photosynthesis and growth of winter wheat in response to waterlogging at different growth stages. Photosynthetica 51, 429–437. doi: 10.1007/s11099-013-0039-9
Shewry, P. R. (2010). Principles of cereal science and technology. J. Cereal Sci. 51, 415. doi: 10.1016/j.jcs.2010.01.001
Shimoda, S., Sugikawa, Y. (2020). Grain-filling response of winter wheat (Triticum aestivum l.) to post-anthesis shading in a humid climate. J. Agron. Crop Sci. 206, 90–100. doi: 10.1111/jac.12370
Singh, S. P., Setter, T. L. (2015). Effect of waterlogging on element concentrations, growth and yield of wheat varieties under farmer’s sodic field conditions. P. Natl. A. Sci. India. B. 87, 513–520. doi: 10.1007/s40011-015-0607-9
Tetlow, I. J., Emes, M. J. (2017). Starch biosynthesis in the developing endosperms of grasses and cereals. Agron. Basel 7, 81. doi: 10.3390/agronomy7040081
Tomlinson, K., Denyer, K. (2003). Starch synthesis in cereal grains. Adv. Bot. Res. 40, 1–61. doi: 10.1016/S0065-2296(05)40001-4
Vamadevan, V. (2013). Impact on molecular organization of amylopectin in starch granules upon annealing. Carbohydr. Polym. 98, 1045–1055. doi: 10.1016/j.carbpol.2013.07.006
Wang, S. J., Copeland, L. (2012). Effect of alkali treatment on structure and function of pea starch granules. Food Chem. 135, 1635–1642. doi: 10.1016/j.foodchem.2012.06.003
Wang, W. T., Cui, W. P., Xu, K., Gao, H. (2021). Effects of early- and late-sowing on starch accumulation and associated enzyme activities during grain filling stage in rice. Rice Sci. 28, 191–199. doi: 10.1016/j.rsci.2021.01.008
Wang, X. Y., Liu, D. M., Wei, M. M., Man, J. G. (2020a). Spraying 6-BA could alleviate the harmful impacts of waterlogging on dry matter accumulation and grain yield of wheat. PeerJ 8, e8193. doi: 10.7717/peerj.8193
Wang, H. W., Xu, K., Liu, X. L., Zhang, Y. Y., Xie, X. H., Zhang, H. (2020b). Understanding the structural, pasting and digestion properties of starch isolated from frozen wheat dough. Food Hydrocoll. 111, 106168. doi: 10.1016/j.foodhyd.2020.106168
Wang, B. B., Xu, X., Zhao, Y., Hou, J. Y., Yang, L., Hu, Z., et al. (2022). Effects of plant growth hormone on starch synthesis and dry matter accumulation of wheat grain under waterlogging and shading after anthesis. Jiangsu J. Agr. Sci. 38, 9–19. doi: 10.3969/j.issn.1000-4440.2022.01.002
Wu, H. Y., Cao, L., Li, J., Gao, P. (2016). Risk assessment on winter wheat suffering from spring wet damages in middle and lower Yangtze. Resour. Env. Yangtze Basin. 25, 1279–1285. doi: 10.11870/cjlyzyyhj201608015
Wu, X. L., Tang, Y. L., Li, C. S., McHugh, A. D., Li, Z., Wu, C. (2018a). Individual and combined effects of soil waterlogging and compaction on physiological characteristics of wheat in southwestern China. Field Crops. Res. 215, 163–172. doi: 10.1016/j.fcr.2017.10.016
Wu, W., Wang, S., Chen, H., Song, Y., Zhang, L., Peng, C., et al. (2018b). Optimal nitrogen regimes compensate for the impacts of seedlings subjected to waterlogging stress in summer maize. PloS One 13, e0206210. doi: 10.1371/journal.pone.0206210
Wu, H. Y., Zhang, P., Xu, M., Zhuang, L. W. (2018c). Spatial-temporal variations of the risk of winter wheat loss suffered from spring waterlogging disaster in the middle and lower Yangtze river reaches. Resour. Env. Yangtze Basin. 27, 1152–1158. doi: 10.11870/cjlyzyyhj201805022
Xie, G. N., Li, Z. X., Ran, Q. J., Wang, H., Zhang, J. R. (2018). Over-expression of mutated ZmDA1 or ZmDAR1 gene improves maize kernel yield by enhancing starch synthesis. Plant Biotechnol. J. 16, 234–244. doi: 10.1111/pbi.12763
Xu, C., Tao, H., Wang, P., Wang, Z. (2015). Slight shading after anthesis increases photosynthetic productivity and grain yield of winter wheat (Triticum aestivum l.) due to the delaying of leaf senescence. J. Integr. Agric. 15, 63–75. doi: 10.1016/S2095-3119(15)61047-4
Yang, H., Dong, B. D., Wang, Y. K., Qiao, Y. Z., Shi, C. H., Jin, L. L., et al. (2020). Photosynthetic base of reduced grain yield by shading stress during the early reproductive stage of two wheat cultivars. Sci. Rep-Uk. 10, 14353. doi: 10.1038/s41598-020-71268-4
Yang, X., Peng, J., Zhang, M. M., Zhang, Q., Liu, X. P. (2019). Effects of foliar spraying of 6-BA on carbohydrate transfer and allocation of maize at the reproductive stage. J. Northwest A&F Univ. (Nat. Sci. Ed.). 47, 62–70. doi: 10.13207/j.cnki.jnwafu.2019.07.009
Yan, H. L., Lu, Q. Y., Gui, J. (2021). Characteristics of A/B-type starch-wheat germ oil complexes and their effects on noodle texture. Lwt-Food Sci. Technol. 144, 111251. doi: 10.1016/J.LWT.2021.111251
Zhang, C. H., Jiang, D., Liu, F. L., Cai, J., Dai, T. B., Cao, W. X. (2009). Starch granules size distribution in superior and inferior grains of wheat is related to enzyme activities and their gene expressions during grain filling. J. Cereal Sci. 51, 226–233. doi: 10.1016/j.jcs.2009.12.002
Zheng, Y. K., Fei, X., Yu, X. R. (2017). Observation and investigation of starch granules within wheat pericarp and endosperm. Agric. Res. 6, 320–325. doi: 10.1007/s40003-017-0270-x
Zhou, Q., Huang, M., Huang, X., Liu, J., Wang, X., Cai, J., et al. (2018a). Effect of post-anthesis waterlogging on biosynthesis and granule size distribution of starch in wheat grains. Plant Physiol. Biochem. 132, 222–228. doi: 10.1016/j.plaphy.2018.08.035
Zhou, Q., Wu, X. J., Xin, L., Jiang, H. D., Wang, X., Cai, J., et al. (2018b). Waterlogging and simulated acid rain after anthesis deteriorate starch quality in wheat grain. Plant Growth Regul. 85, 257–265. doi: 10.1007/s10725-018-0390-8
Keywords: wheat (Triticum aestivum L.), waterlogging and shading, 6-benzylaminoadenine, photosynthetic performance, starch accumulation, grain filling, yield
Citation: Zhang W, Wang B, Zhang A, Zhou Q, Li Y, Li L, Ma S, Fan Y and Huang Z (2022) Exogenous 6-benzylaminopurine enhances waterlogging and shading tolerance after anthesis by improving grain starch accumulation and grain filling. Front. Plant Sci. 13:1003920. doi: 10.3389/fpls.2022.1003920
Received: 26 July 2022; Accepted: 10 October 2022;
Published: 27 October 2022.
Edited by:
Muhammad Rizwan, Government College University, Faisalabad, PakistanReviewed by:
Anis Ali Shah, University of Education Lahore, PakistanBaizhao Ren, Shandong Agricultural University, China
Aziz Khan Khan, Guangxi University, China
Copyright © 2022 Zhang, Wang, Zhang, Zhou, Li, Li, Ma, Fan and Huang. This is an open-access article distributed under the terms of the Creative Commons Attribution License (CC BY). The use, distribution or reproduction in other forums is permitted, provided the original author(s) and the copyright owner(s) are credited and that the original publication in this journal is cited, in accordance with accepted academic practice. No use, distribution or reproduction is permitted which does not comply with these terms.
*Correspondence: Zhenglai Huang, zhangwenjing79@ahau.edu.cn
†These authors have contributed equally to this work and share first authorship