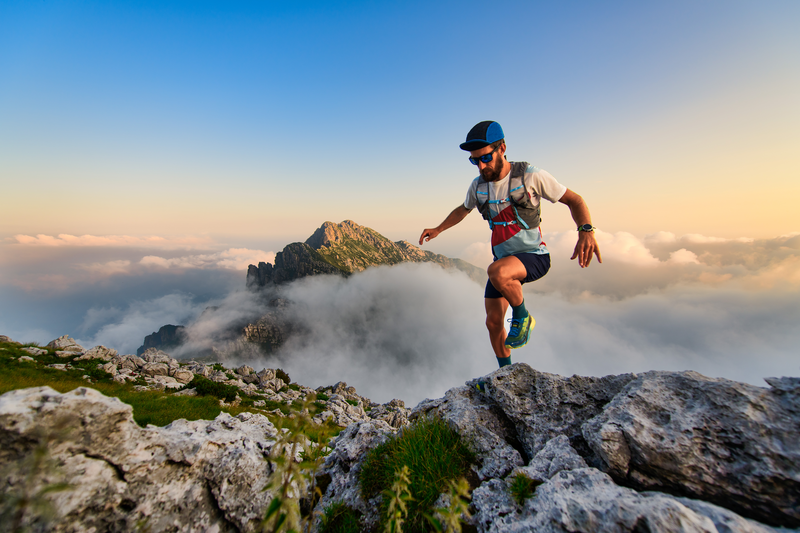
94% of researchers rate our articles as excellent or good
Learn more about the work of our research integrity team to safeguard the quality of each article we publish.
Find out more
PERSPECTIVE article
Front. Plant Sci. , 24 December 2021
Sec. Technical Advances in Plant Science
Volume 12 - 2021 | https://doi.org/10.3389/fpls.2021.805032
This article is part of the Research Topic Plant Transformation View all 20 articles
Most major crops are polyploid species and the production of genetically engineered cultivars normally requires the introgression of transgenic or gene-edited traits into elite germplasm. Thus, a main goal of plant research is the search of systems to identify dominant mutations. In this article, we show that the Tnt1 element can be used to identify dominant mutations in allogamous tetraploid cultivated alfalfa. Specifically, we show that a single allelic mutation in the MsNAC39 gene produces multifoliate leaves (mfl) alfalfa plants, a pivot trait of breeding programs of this forage species. Finally, we discuss the potential application of a combination of preliminary screening of beneficial dominant mutants using Tnt1 mutant libraries and genome editing via the CRISPR/Cas9 system to identify target genes and to rapidly improve both autogamous and allogamous polyploid crops.
Legume crops fix nitrogen symbiotically by interacting with soil nitrogen-fixing rhizobia, producing high-protein foods and reducing the use of nitrogen fertilizers derived from fossil fuel. The most important forage legume worldwide is alfalfa (Medicago sativa), also called the “Queen of forages” due to its high yield and quality and wide adaptation. However, traditional and modern alfalfa breeding programs are limited by the intrinsic features of the species itself.
Cultivated alfalfa is an allogamous perennial tetraploid species which displays high levels of self-incompatibility and extreme inbreeding depression (Dieterich Mabin et al., 2021). Because of this polyploid nature and particular reproductive behavior, an alfalfa cultivar commonly involves a large number of genetically and phenotypically heterozygous parental plants. Then, the production of genetically modified alfalfa cultivars needs the introgression of transgenic or gene-edited traits into elite heterogeneous populations, independently of the agronomic quality of the regenerative clone used in alfalfa transformation.
Empirically, only two introgression approaches have been able to bypass the intrinsic limitations of the polyploid and outcrossing nature of alfalfa: the dihomogenic and supertransgene process described by Forage Genetics Inc. (McCaslin et al., 2002) and that described by the research group of Dr. Gabriela Soto (Jozefkowicz et al., 2018). These rapid and low-cost introgression processes require the use of dominant traits (e.g., herbicide tolerance transgenes), excluding the use of beneficial recessive mutations such as some alfalfa mutant events generated via the CRISPR/Cas9 system (Gao et al., 2018).
In the last years, the increased efficiency of the CRISPR/Cas9 system in highly regenerative alfalfa germplasms has allowed full allelic knockout of an individual gene in the T0 generation (Chen et al., 2020; Wolabu et al., 2020; Bottero et al., 2021). Naturally, this optimized system can help to validate in alfalfa the strategies found in the model species Medicago truncatula. As an example of this transfer, null palm1 mutants produce pentafoliate leaves rather than wild-type trifoliate leaves in both M. truncatula (Chen et al., 2010) and alfalfa (Chen et al., 2020). Although the high expression of this type of beneficial traits (e.g., multifoliated leaves) obtained by the full allelic knockout of an individual alfalfa gene (e.g., palm1) in elite germplasms is not impossible, it requires an adaptation of the current introgression processes, a fact that increases the cost and time of production of a genetically modified alfalfa cultivar (Bottero et al., 2021).
Retrotransposon Tnt1, an effective mutagen, has been used as a tool to study gene function in both model (e.g., M. truncatula, Arabidopsis thaliana and wild potato) and non-model (e.g., soybean, lotus, cucumber and lettuce) autogamous species (Courtial et al., 2001; d’Erfurth et al., 2003; Mazier et al., 2007; Cui et al., 2013; Duangpan et al., 2013; Iantcheva et al., 2016). In this classical application of Tnt1 for functional genomics studies, homozygous mutant lines generated via Tnt1 insertions show complete loss of the function of target genes (full allelic knockout). In this article, we explore the use of Tnt1 for the identification of beneficial dominant mutations in alfalfa. Specifically, we focus on dominant alleles, which are those that express a trait even if there is only one copy.
Due to their high protein content and high digestibility, alfalfa leaves contribute the majority of the feeding value of this forage. Thus, increasing the proportion of leaves to stems is a pivotal target of alfalfa breeding programs (Odorizzi et al., 2015). During a screening of dominant mutations with visible phenotype and Mendelian inheritance within an alfalfa Tnt1 library performed at our lab (Supplementary Figure 1), we identified an alfalfa mutant with polyfoliated leaves. Contrary to its parental wild-type plant C23, which shows common trifoliate leaves (Figure 1A), this mutant (mutant 1–158) displays both trifoliate and polyfoliate stems (Figure 1B). These multifoliate leaves (mlf) plants have different numbers (3–7) of leaflets (Figure 1C) and leaf morphologies (Figures 1D–E). As expected, this mlf mutation produces a higher leaf-to-stem ratio (Figure 1F) and higher numbers of leaflets (Figure 1G), at least in the first generation. Moreover, around 50% of the mlf flowers show an increased number of petals (Figure 1H), an extremely unusual (<0.001%) trait in cultivated alfalfa. In biotechnological terms, these findings represent the first report of a dominant mutation conferring polyfoliate leaves in alfalfa and of the expanded use of Tnt1 for the identification of this type of dominant mutations in polyploid species.
Figure 1. Identification of a dominant mutation conferring multifoliate leaves in cultivated alfalfa via Tnt1. During a screening of dominant mutations with visible phenotype from an alfalfa Tnt1 insertion mutant library using the wild-type highly regenerative alfalfa clone C23 (García et al., 2014), we identified a mutant (1–158, mfl) plant displaying both usual trifoliate stems (A,B) and unusual polyfoliate leaves (B). These mutant plants show trifoliate to heptafoliate leaves (C), polyfoliate leaves with different number of leaflets (D), and tetrafoliate leaves with unusual morphologies (E). When comparing clonally propagated individual plants derived from the wild-type clone (C23), the original mutant clone 1–158 (G0) and its first generation offspring (G1), we observed that the mfl mutation induces a higher leaf-to-stem ratio (F) and higher numbers of leaflets (G). The mfl mutant also shows flowers with extra petals (H). In these experiments, the cuts were performed at 8 cm from the soil and plants were harvested periodically (every 60 days) to prevent their flowering and evaluate their leaf/stem ratio and number of leaflets for 2 years. The values are the average for this period. Wild-type and mutant plants were intercrossed by hand. Wild-type plants contains four different alleles of MsNAC39 and Tnt1 is inserted into this gene on chromosome 3.4 in mfl mutant plants (I). Multiple alignment of the partial amino acid sequences of MsNAC39 protein and its closely related proteins in M. truncatula (MtNAC77, MtNAC63, and MtNAC51) showing that the Tnt1 insertion is localized in the C-terminal hypervariable domain of the MsNAC39 protein (J). The microRNA target region of Arabidopsis NAC-like genes AT5G61430 and AT5G07680 is conserved in the MsNAC39 alleles (K). Values are mean ± SEM (n = 25). Statistical analysis was carried out with Student’s t-test (*p < 0.05).
Because of the extreme heterozygosity of alfalfa, an individual plant (e.g., regenerative clone C23) normally possesses four different alleles of the same locus, and it is thus possible to determine the exact localization of a mutation by segregation analysis (Bottero et al., 2021). Bioinformatic analysis of the Tnt1-flanking sequences of the mfl mutant plant and its progeny showed that the mfl mutation colocalized with the MsNAC39 gene in chromosome 3.4 (Figure 1I). The NAC protein family is a large group of plant-specific transcriptional factors involved in the control of gene expressions related to leaf development and adaptation to environmental conditions (Liu et al., 2014; Oda-Yamamizo et al., 2016; Trupkin et al., 2019; Min et al., 2020; Challa et al., 2021). NAC transcriptional factors contain a highly conserved N-terminal DNA-binding domain and a C-terminal hypervariable domain related to the activation or repression of the transcription of specific target genes (Yamaguchi-Shinozaki and Shinozaki, 2005; Le et al., 2011; He et al., 2016; Maugarny et al., 2016). Similarly, the MsNAC39 protein and its closely related proteins (>40% amino acid identity) in M. truncatula (MtNAC77, MtNAC63, and MtNAC51) show a completely different C-terminal region (Figure 1J). Interestingly, the Tnt1 element disrupts the particular C-terminal region of the NAC39 protein in mlf alfalfa (Figure 1J), suggesting that this mutation affects its transcriptional factor activity. In addition the Arabidopsis NAC-like proteins most related to MsNAC39 (AT5G61430 and AT5G07680; Supplementary Figure 2), together with the CUC1 and CUC2 genes (Supplementary Figure 2) are common targets of a microRNA controlling leaf and floral development (Mallory et al., 2004; Rubio-Somoza et al., 2014). An alteration in their expression leads to the development of striking floral organ phenotypes, including extra petals (Mallory et al., 2004; Baker et al., 2005) in agreement with the mfl alfalfa phenotype. Interestingly, the microRNA target region of AT5G61430 and AT5G07680 is highly preserved in the MsNAC39 alleles, sharing 19 of the 20 nucleotides (Figure 1K). Taken together, evolutionary and phenotypic studies suggest that MsNAC39 can play ancient and multiple roles in alfalfa ontogeny, including vegetative growth and reproductive development.
Although there is a legal framework for the deregulation of transgenic plants, including transgenic alfalfa cultivars (Samac and Temple, 2021), the deregulation of transgenic events are long-term and expensive procedures. Specifically, Tnt1 is a mobile element that can move around within a genome not only under regenerative conditions but also under abiotic stress conditions (Iantcheva et al., 2009), which is an undesirable trait in transgenic crops. Thus, the direct application of Tnt1 insertions (e.g., MsNAC39:Tnt1) in crop production is extremely limited. In contrast, engineering plant traits using the CRISPR/Cas9 system (e.g., the induction of small indels in MsNAC39 alleles) could be an attractive strategy to improve germplasms available worldwide. However, the current knowledge of beneficial dominant mutations is very scarce, thus limiting the rational design of the generation of this type of mutation via the CRISPR/Cas9 system in polyploid species. Most major crop species, including soybean, wheat, maize, potato, sugarcane, coffee, cotton, alfalfa, and tall fescue, are polyploid species. In this context, Tnt1 libraries can function as sources of beneficial dominant mutations that can be phenocopied using transgenic-free edited cultivars via the CRISPR/Cas9 system in these major crops with complex genetics (Figure 2). In the case of facultative autogamous and allogamous species, the editing machinery can be easily segregated during the introgression process, and then, the Agrobacterium transformation does not imply a limitation to produce transgenic-free commercial cultivars (Figure 2). In contrast, in the case of highly autogamous species, the use of the CRISPR/Cas9 system with economic purposes implies the search of alternative deliveries of the editing machinery to the target plant cell, such as the transfection of protoplasts with ribonucleoprotein complexes (Figure 2). The perspective of combining the Tnt1 element and the CRISPR/Cas9 system could lead to a synergism between applied research and breeding programs, in which breeders will rapidly convert beneficial dominant mutations in improved elite germplasm varieties.
Figure 2. Schematic representation of the possible combination of Tnt1 element and the CRISPR/Cas9 system to rapidly improve polyploidy crops. This picture is explained in the text.
The original contributions presented in the study are included in the article/Supplementary Material, further inquiries can be directed to the corresponding author/s.
GS, NA, CJ, AI, and PR designed and funded the experiments. CJ and CG performed the experiments. CJ, CG, AO, AI, PR, NA, and GS helped with the experiments and data analysis. GS wrote the manuscript. All authors contributed to the article and approved the submitted version.
This work was supported by Grants PICT 2017#0484, FVT 2017#39, and PICT-STARTUP 2020 #00004 to GS, Grant PICT 2017#1678 to CJ, and by PE I115 (Biotechnology Program of INTA). Alfalfa Tnt1 library is free to use.
The authors declare that the research was conducted in the absence of any commercial or financial relationships that could be construed as a potential conflict of interest.
All claims expressed in this article are solely those of the authors and do not necessarily represent those of their affiliated organizations, or those of the publisher, the editors and the reviewers. Any product that may be evaluated in this article, or claim that may be made by its manufacturer, is not guaranteed or endorsed by the publisher.
The Supplementary Material for this article can be found online at: https://www.frontiersin.org/articles/10.3389/fpls.2021.805032/full#supplementary-material
Baker, C. C., Sieber, P., Wellmer, F., and Meyerowitz, E. M. (2005). The early extra petals1 Mutant Uncovers a Role for MicroRNA miR164c in Regulating Petal Number in Arabidopsis. Curr. Biol. 15, 303–315. doi: 10.1016/j.cub.2005.02.017
Bottero, E., Massa, G., González, M., Stritzler, M., Tajima, H., Gómez, C., et al. (2021). Efficient CRISPR/Cas9 Genome Editing in Alfalfa Using a Public Germplasm. Front. Agron. 3:661526. doi: 10.3389/fagro.2021.661526
Challa, K. R., Rath, M., Sharma, A. N., Bajpai, A. K., Davuluri, S., Acharya, K. K., et al. (2021). Active suppression of leaflet emergence as a mechanism of simple leaf development. Nat. Plants 7, 1264–1275. doi: 10.1038/s41477-021-00965-3
Chen, H., Zeng, Y., Yang, Y., Huang, L., Tang, B., Zhang, H., et al. (2020). Allele-aware chromosome-level genome assembly and efficient transgene-free genome editing for the autotetraploid cultivated alfalfa. Nat. Commun. 11:2494. doi: 10.1038/s41467-020-16338-x
Chen, J., Yu, J., Ge, L., Wang, H., Berbel, A., Liu, Y., et al. (2010). Control of dissected leaf morphology by a Cys(2)His(2) zinc finger transcription factor in the model legume Medicago truncatula. Proc. Natl. Acad. Sci. U. S. A. 107, 10754–10759. doi: 10.1073/pnas.1003954107
Courtial, B., Feuerbach, F., Eberhard, S., Rohmer, L., Chiapello, H., Camilleri, C., et al. (2001). Tnt1 transposition events are induced by in vitro transformation of Arabidopsis thaliana, and transposed copies integrate into genes. Mol. Genet. Genomics 265, 32–42. doi: 10.1007/s004380000387
Cui, Y., Barampuram, S., Stacey, M. G., Hancock, C. N., Findley, S., Mathieu, M., et al. (2013). Tnt1 retrotransposon mutagenesis: a tool for soybean functional genomics. Plant Physiol. 161, 36–47. doi: 10.1104/pp.112.205369
d’Erfurth, I., Cosson, V., Eschstruth, A., Lucas, H., Kondorosi, A., and Ratet, P. (2003). Efficient transposition of the Tnt1 tobacco retrotransposon in the model legume Medicago truncatula. Plant J. 34, 95–106. doi: 10.1046/j.1365-313x.2003.01701.x
Dieterich Mabin, M. E., Brunet, J., Riday, H., and Lehmann, L. (2021). Self-Fertilization, Inbreeding, and Yield in Alfalfa Seed Production. Front. Plant Sci. 12:700708. doi: 10.3389/fpls.2021.700708
Duangpan, S., Zhang, W., Wu, Y., Jansky, S. H., and Jiang, J. (2013). Insertional mutagenesis using Tnt1 retrotransposon in potato. Plant Physiol. 163, 21–29. doi: 10.1104/pp.113.221903
Gao, R., Feyissa, B. A., Croft, M., and Hannoufa, A. (2018). Gene editing by CRISPR/Cas9 in the obligatory outcrossing Medicago sativa. Planta 247, 1043–1050. doi: 10.1007/s00425-018-2866-1
García, A. N., Ayub, N. D., Fox, A. R., Gómez, M. C., Diéguez, M. J., Pagano, E. M., et al. (2014). Alfalfa snakin-1 prevents fungal colonization and probably coevolved with rhizobia. BMC Plant Biol. 14:248. doi: 10.1186/s12870-014-0248-9
He, X., Zhu, L., Xu, L., Guo, W., and Zhang, X. (2016). GhATAF1, a NAC transcription factor, confers abiotic and biotic stress responses by regulating phytohormonal signaling networks. Plant Cell Rep. 35, 2167–2179. doi: 10.1007/s00299-016-2027-6
Iantcheva, A., Chabaud, M., Cosson, V., Barascud, M., Schutz, B., Primard-Brisset, C., et al. (2009). Osmotic shock improves Tnt1 transposition frequency in Medicago truncatula cv Jemalong during in vitro regeneration. Plant Cell Rep. 28, 1563–1572. doi: 10.1007/s00299-009-0755-6
Iantcheva, A., Revalska, M., Zehirov, G., Boycheva, I., Magne, K., Radkova, M., et al. (2016). Tnt1 retrotransposon as an efficient tool for development of an insertional mutant collection of Lotus japonicus. In Vitro Cell. Dev. Biol. Plant 52, 338–347.
Jozefkowicz, C., Frare, R., Fox, R., Odorizzi, A., Arolfo, V., Pagano, E., et al. (2018). Maximizing the expression of transgenic traits into elite alfalfa germplasm using a supertransgene configuration in heterozygous conditions. Theor. Appl. Genet. 131, 1111–1123. doi: 10.1007/s00122-018-3062-1
Le, D. T., Nishiyama, R., Watanabe, Y., Mochida, K., Yamaguchi-Shinozaki, K., Shinozaki, K., et al. (2011). Genome-Wide Survey and Expression Analysis of the Plant-Specific NAC Transcription Factor Family in Soybean During Development and Dehydration Stress. DNA Res. 18, 263–276. doi: 10.1093/dnares/dsr015
Liu, T., Song, X., Duan, W., Huang, Z., Liu, G., Li, Y., et al. (2014). Genome-Wide Analysis and Expression Patterns of NAC Transcription Factor Family Under Different Developmental Stages and Abiotic Stresses in Chinese Cabbage. Plant Mol. Biol. Rep. 32, 1041–1056.
Mallory, A. C., Dugas, D. V., Bartel, D. P., and Bartel, B. (2004). MicroRNA regulation of NAC-domain targets is required for proper formation and separation of adjacent embryonic, vegetative, and floral organs. Curr. Biol. 14, 1035–1046. doi: 10.1016/j.cub.2004.06.022
Maugarny, A., Gonçalves, B., Arnaud, N., and Laufs, P. (2016). “Chapter 15 - CUC Transcription Factors: To the Meristem and Beyond,” in Plant Transcription Factors, ed. D. H. Gonzalez (Boston: Academic Press), 229–247.
Mazier, M., Botton, E., Flamain, F., Bouchet, J. P., Courtial, B., Chupeau, M. C., et al. (2007). Successful gene tagging in lettuce using the Tnt1 retrotransposon from tobacco. Plant Physiol. 144, 18–31. doi: 10.1104/pp.106.090365
McCaslin, M. H., Temple, S. J., and Tofte, J. E. (2002). Methods for maximizing expression of transgenic traits in autopolyploid plants. Patent US 2002/0042928.
Min, X., Jin, X., Zhang, Z., Wei, X., Ndayambaza, B., Wang, Y., et al. (2020). Genome-Wide Identification of NAC Transcription Factor Family and Functional Analysis of the Abiotic Stress-Responsive Genes in Medicago sativa L. J. Plant Growth Regul. 39, 324–337. doi: 10.1007/s00344-019-09984-z
Oda-Yamamizo, C., Mitsuda, N., Sakamoto, S., Ogawa, D., Ohme-Takagi, M., and Ohmiya, A. (2016). The NAC transcription factor ANAC046 is a positive regulator of chlorophyll degradation and senescence in Arabidopsis leaves. Sci. Rep. 6:23609.
Odorizzi, A., Mamani, E. M. C., Sipowicz, P., Julier, B., Gieco, J., and Basigalup, D. (2015). Effect of phenotypic recurrent selection on genetic diversity of non-dormant multifoliolate lucerne (Medicago sativa L.) populations. Crop Pasture Sci. 66, 1190–1196.
Rubio-Somoza, I., Zhou, C. M., Confraria, A., Martinho, C., Von Born, P., Baena-Gonzalez, E., et al. (2014). Temporal control of leaf complexity by miRNA-regulated licensing of protein complexes. Curr. Biol. 24, 2714–2719. doi: 10.1016/j.cub.2014.09.058
Samac, D. A., and Temple, S. J. (2021). “Biotechnology Advances in Alfalfa,” in Compendium of Plant Genomes, eds X. Yu and C. Kole (Cham: Springer).
Trupkin, S. A., Astigueta, F. H., Baigorria, A. H., García, M. N., Delfosse, V. C., González, S. A., et al. (2019). Identification and expression analysis of NAC transcription factors potentially involved in leaf and petal senescence in Petunia hybrida. Plant Sci. 287:110195. doi: 10.1016/j.plantsci.2019.110195
Wolabu, T. W., Cong, L., Park, J.-J., Bao, Q., Chen, M., Sun, J., et al. (2020). Development of a Highly Efficient Multiplex Genome Editing System in Outcrossing Tetraploid Alfalfa (Medicago sativa). Front. Plant Sci. 11:1063. doi: 10.3389/fpls.2020.01063
Keywords: transformation, Tnt1, dominant mutation, polyploidy, alfalfa
Citation: Jozefkowicz C, Gómez C, Odorizzi A, Iantcheva A, Ratet P, Ayub N and Soto G (2021) Expanding the Benefits of Tnt1 for the Identification of Dominant Mutations in Polyploid Crops: A Single Allelic Mutation in the MsNAC39 Gene Produces Multifoliated Alfalfa. Front. Plant Sci. 12:805032. doi: 10.3389/fpls.2021.805032
Received: 29 October 2021; Accepted: 30 November 2021;
Published: 24 December 2021.
Edited by:
Horacio Esteban Hopp, University of Buenos Aires, ArgentinaReviewed by:
Maria Carelli, Council for Agricultural and Economics Research (CREA), ItalyCopyright © 2021 Jozefkowicz, Gómez, Odorizzi, Iantcheva, Ratet, Ayub and Soto. This is an open-access article distributed under the terms of the Creative Commons Attribution License (CC BY). The use, distribution or reproduction in other forums is permitted, provided the original author(s) and the copyright owner(s) are credited and that the original publication in this journal is cited, in accordance with accepted academic practice. No use, distribution or reproduction is permitted which does not comply with these terms.
*Correspondence: Gabriela Soto, c290by5nYWJyaWVsYWNpbnRoaWFAaW50YS5nb2IuYXI=
Disclaimer: All claims expressed in this article are solely those of the authors and do not necessarily represent those of their affiliated organizations, or those of the publisher, the editors and the reviewers. Any product that may be evaluated in this article or claim that may be made by its manufacturer is not guaranteed or endorsed by the publisher.
Research integrity at Frontiers
Learn more about the work of our research integrity team to safeguard the quality of each article we publish.