- 1Shenzhen Key Laboratory of Marine Bioresource and Eco-Environmental Sciences, College of Life Sciences and Oceanography, Shenzhen University, Shenzhen, China
- 2Instrument Analysis Center, Shenzhen University, Shenzhen, China
- 3College of Plant Protection, Fujian Agriculture and Forestry University, Fuzhou, China
- 4Department of Biology, Faculty of Applied Science, Umm Al-Qura University, Makkah, Saudi Arabia
- 5Department of Plant Sciences, College of Agricultural and Marine Sciences, Sultan Qaboos University, Muscat, Oman
- 6College of Agriculture, Fujian Agriculture and Forestry University, Fuzhou, China
- 7Faculty of Agricultural Sciences and Technologies, Sivas University of Science and Technology, Sivas, Turkey
- 8Research Center for Advanced Materials Science (RCAMS), King Khalid University, Abha, Saudi Arabia
- 9Unit of Bee Research and Honey Production, Faculty of Science, King Khalid University, Abha, Saudi Arabia
- 10Faculty of Science, King Khalid University, Abha, Saudi Arabia
- 11Department of Biotechnology, College of Science, Taif University, Taif, Saudi Arabia
- 12Botany & Microbiology Department, Science College, King Saud University, Riyadh, Saudi Arabia
The domain of the unknown function 221 proteins regulate several processes in plants, including development, growth, hormone transduction mechanism, and abiotic stress response. Therefore, a comprehensive analysis of the potato genome was conducted to identify the deafness-dystonia peptide (DDP) proteins’ role in potatoes. In the present study, we performed a genome-wide analysis of the potato domain of the unknown function 221 (DUF221) genes, including phylogenetic inferences, chromosomal locations, gene duplications, gene structures, and expression analysis. In our results, we identified 10 DDP genes in the potato genome. The phylogenetic analysis results indicated that StDDPs genes were distributed in all four clades, and clade IV was the largest clade. The gene duplication under selection pressure analysis indicated various positive and purifying selections in StDDP genes. The putative stu-miRNAs from different families targeting StDDPs were also predicted in the present study. Promoter regions of StDDP genes contain different cis-acting components involved in multiple stress responses, such as phytohormones and abiotic stress-responsive factors. The analysis of the tissue-specific expression profiling indicated the StDDPs gene expression in stem, root, and leaf tissues. We subsequently observed that StDDP4, StDDP5, and StDDP8 showed higher expressions in roots, stems, and leaves. StDDP5 exhibited high expression against heat stress response, and StDDP7 showed high transcript abundance against salt stress in potatoes. Under abscisic acid (ABA) and indole acetic acid (IAA) treatments, seven StDDP genes’ expressions indicated that ABA and IAA performed important roles in immunity response. The expression profiling and real-time qPCR of stems, roots, and leaves revealed StDDPs’ significant role in growth and development. These expression results of DDPs are primary functional analysis and present basic information for other economically important crops.
Introduction
Almost 70% of annual potential crop yield losses are due to variations in the environment (Leng et al., 2021). Abiotic factors are the major limiting stress factors that affect plants during their vegetative and reproductive growth, resulting in abrupt economic and agricultural losses worldwide (Sade et al., 2018; Hafeez et al., 2021; Li et al., 2021). Salt and drought stress factors are the chief abiotic stress factors affecting geographical plant distribution, threatening food security, and limiting crop production (Muhammad Ahmad et al., 2021). Generally, plants lack a structure that works directly with environmental impacts, but they can respond to climate change (Mahalingam and Fedoroff, 2003; Huang et al., 2021; Raza et al., 2021c). Plants exhibit extensive defense responses at molecular and cellular levels to oppose the cell damage caused by stress factors (Kim et al., 2014; Raza et al., 2021b).
Plant biologists have always been attracted to gene families’ structure, function, and evolutionary model. The interaction and adaptation between the environment and plants are well studied based on the information of these gene families (Zhao et al., 2021). Among them, the domain of unknown function (DUF) proteins are extensively distributed in different plants and restrain at least one extremely conserved domain of DUF (Bateman et al., 2010). Many studies on DUF genes have revealed the importance of DUFs in plants in various functions, one of which is abiotic stress tolerance (Zhang et al., 2021). However, there have been reports of other DUF gene families in many plants. These include the DUF221, DUF810, DUF866, DUF936, and DUF1618 gene families in rice and the DUF581 and DUF724 gene families in Arabidopsis (Zhao et al., 2021). Furthermore, DUF proteins act as integral membrane proteins and are associated with other membrane proteins (Rai et al., 2012). Resistance to DUF-mediated strains has been studied in various plants, while a comprehensive study of the DUF family gene remains to be conducted in other plants. Additionally, in transgenic Oryza sativa (rice) and Arabidopsis thaliana, OsSGL presents significant drought stress tolerance, whereas other diverse stress-responsive genes have been altered significantly in transgenic rice. Xin et al. (2007) reported that in A. thaliana, the AT3g55990 (ESK1 gene) is associated with the DUF283 family gene, considered a novel negative cold acclimation gene regulator. The AtCSC1 gene in Arabidopsis (Hou et al., 2014) and its homologous gene in rice are known OsCA1, responsible for osmotic regulation (Yuan et al., 2014), ERD4 is known as a dehydration-responsive gene in Brassica juncea (Rai et al., 2012), whereas DUF27 and DUF538 have chlorophyll-binding capabilities (Gholizadeh, 2016). Moreover, the superfamily of DUF283 is important for siRNA processing (in gene silencing) in Arabidopsis (Qin et al., 2010). Another study in A. thaliana illustrated the reduced effect of ABA mediation (drought stress gene) after inhibiting the gene expression of both ATRDUF2 (RING-DUF1117 E3 ubiquitin ligases) (Kim et al., 2012). From rice, two genes (DUF1644 and OsSIDP366) positively regulate the drought and salt stresses by overexpressing OsSIDP366 in transgenic rice, which enhances tolerance against drought and salinity factors (Guo et al., 2016). In O. sativa, other DUF family genes have been related to abiotic stress factors, including OsDUF810.7 (Li et al., 2018), DUF1644 (SIDP361) (Li et al., 2016), and DUF966 (OsDSR2) (Luo et al., 2014). Three family members of deafness-dystonia peptides (DDPs) in Medicago truncatula reported dehydration (MtST2 and MtST3) and modulation through nutritional status (MtST1) during development (Albornos et al., 2017).
Potato is an important food source and cash crop worldwide (Zaynab et al., 2021c). In 2018, about 368,168,914 tons of potatoes were harvested globally from 17,578,672 ha of land, and over one billion people consume them. Among the potato-producing countries, China holds the first position, producing about 90,321,442 tons of potatoes from 4,813,542 ha of land in 2018 (Rashid et al., 2021). Though, like other plants, potato yield is also at risk to abiotic and biotic stress factors (Zaynab et al., 2021f). The objective of the present research was to characterize the DDP genes family across the potato genomes. Thus, based on the putative role of DDPs against abiotic stress, we carried out a comprehensive analysis of DDP genes in the potato genome against abiotic stress. To date, DDP genes expression analysis against abiotic has not been reported in potatoes. We examine the expression profiling of StDDPs under phytohormones, salt, and heat stress. These data may potentially explain the validated functional foundation of potato DUF221 genes and their functions according to growth and development in potatoes under unfavorable/stressful conditions.
Materials and Methods
Identification and Phylogenetic Analysis of the StDDP Genes
The Arabidopsis DDPs sequences were attained from the TIAR1 and used as the query to search the potato DDP genes from the Phytozome site2. A local BLASTP similarity search was carried out to find the DDPs in the S. tuberosum genome. The collected gene sequences were subjected to PfamScan and Batch CDD-NCBI search to validate the presence of the Pfam domain (PF14703). The data redundancy was removed, and the identified genes were analyzed for further study. The protein physiochemical properties, including isoelectric point (PI), and molecular weight (MW), were forecasted by Expasy3 server.
A phylogenetic tree was constructed to observe evolutionary relationships among A. thaliana, Solanum tuberosum, and Solanum lycoperiscum by MEGA 7 software. The entire protein sequences were arranged by using MUSCLE through 16 iterations. Then, the protein sequence alignment was used to make the phylogenetic tree through the Neighbor-Joining method through 1,000 bootstrap values.
Chromosomal Location, Synteny, and Selection Pressure Analysis
The information about StDDPs was retrieved from the Potato Genome Sequencing Consortium (PGSC). The TBtools software4 was used to map the chromosomal positions of StDDPs. The comparative synteny analysis was executed to visualize the genome conservation through the Circoletto Tool (tools.bat.infspire.org/circoletto/). Further, the duplicated genes’ coding sequences were arranged by the Muscle program in MEGA 7. The synonymous and non synonymous substitution rates (Ka = No. of nonsynonymous substitution/nonsynonymous site; Ks = No. of synonymous substitution/synonymous site) were calculated by KaKs_ Calculator 2.0 software tool through the exchange rate (r = 2.6 × 10–9; Li et al., 2019).
Gene Structure and Conserved Motif Analysis
For the gene structure analysis, we used Gene Structure Display Server, and DDP proteins’ conserved motifs were determined by MEME tool5 through the following parameters: optimum width ranges: 6–200; no. of motifs: 20. TB tools software4 was used to figure out the distribution of motifs.
Cis-Elements Analysis miRNAs Prediction and Orthologous Genes Identification
To analyze the cis-regulatory elements of potato DDP genes, the promoter sequences (2,000 bp upstream of the ATG initiation codon) were taken from the S. tuberosum genome database in a generic file format. The promoter sequences were scanned with the PlantCARE database6. The coding sequence of StDDPs was used to identify potential miRNAs targeting the StDDPs using the psRNATarget database7 with default parameters. The orthologous DDPs proteins in A. thaliana, S. tuberosum, and S. lycoperiscum were identified using orthovenn2 https://orthovenn2.bioinfotoolkits.net/home. Protein sequences of three species were used for analysis. Each species was individually assessed with each other for the identification of orthologous gene clusters.
Expression Analysis of StDDP Genes
For the StDDP genes expression analysis, fragments per kilobase million (FPKM) values in root, stem, and leaf tissues were used. The data was collected and assembled with enormously expressed tissues, including leaves, stems, and roots. The FPKM values were used to illustrate the heat map by using TBtools. The log2 normalized values were used to construct the heat maps (Zaynab et al., 2021a).
Plant Sampling and Material Collection
Potato tubers were obtained from the NARC (National Agricultural Research Center), Islamabad, Pakistan, and planted in the glasshouse under controlled conditions at National Institute for Genomics and Advanced Biotechnology (NIGAB) NARC, Islamabad, Pakistan. Later, 25 days after germination, the roots, stems, and leaves were collected in replicates and for RNA extraction stored in liquid nitrogen.
RNA Extraction and RT-qPCR Analysis
The total RNA from roots, stems, and leaves was extracted by a quick isolation Ribonucleic acid (RNA) Kit (Huayueyang, Beijing, China) following the manufacture’s protocol. The quality of RNA was assessed through gel electrophoresis using 1% agarose gel. The first cDNA strand was prepared from 0.5 μg RNA. The qRT-PCR was executed in BioRad CFX96 RT-PCR Detection System instrument (BioRad Laboratories) with a 20 μL reaction mixture through SYBR ® Green RT-PCR Master (TOYOBO QPK-210, Shanghai, China) using gene-specific primers. The thermocycler was set according to the given protocol: denaturation at 95°C for 15 s, annealing occurs at 55°C for 15 s, and extension takes to play at 72°C for 15 s.
Target gene amplification was monitored with SYBR Green fluorescence in each cycle. In addition, the qRT-PCR amplification specificity was routinely checked with the melting curve. The data were observed through the 2–ΔΔCt method (Zaynab et al., 2018), while results were represented through relative gene expression level (Zaynab et al., 2021d). Through this analysis report, elongation factor 1 elongation factor 1 - α was considered housekeeping gene. In the whole experimental observation, four technical replicates were conducted (Zaynab et al., 2021b). The sequences of all primers used are mentioned in Supplementary Table 1.
Results
Identification and Phylogenetic Analysis of StDDP Genes
The results showed that 10 DDP genes were found after genome-wide identification in the potato genome. Similar genes with diverse transcripts were not considered in this study. Although all the identified genes contain conserved DDP domains with Pfam ID (PF14703). The details of 10 DDPs, including their molecular weight (MW), chromosome number, isoelectric point (PI), and protein length, are shown in Table 1. Moreover, the protein lengths ranged from 99 to 892 amino acids (aa), the PI was 8.86–9.74, and the MW was from 11.371 to 10.2546 kDa. The evolutionary relationship of DDPs was observed through a phylogenetic tree by MEGA7.0 software using the Neighbor-joining method with 1,000 bootstrap values. The phylogenetic tree was classified into four clades. The results of the phylogenetic analysis indicated that StDDPs genes were distributed in all four clades and clade IV was the largest clade (Figure 1).
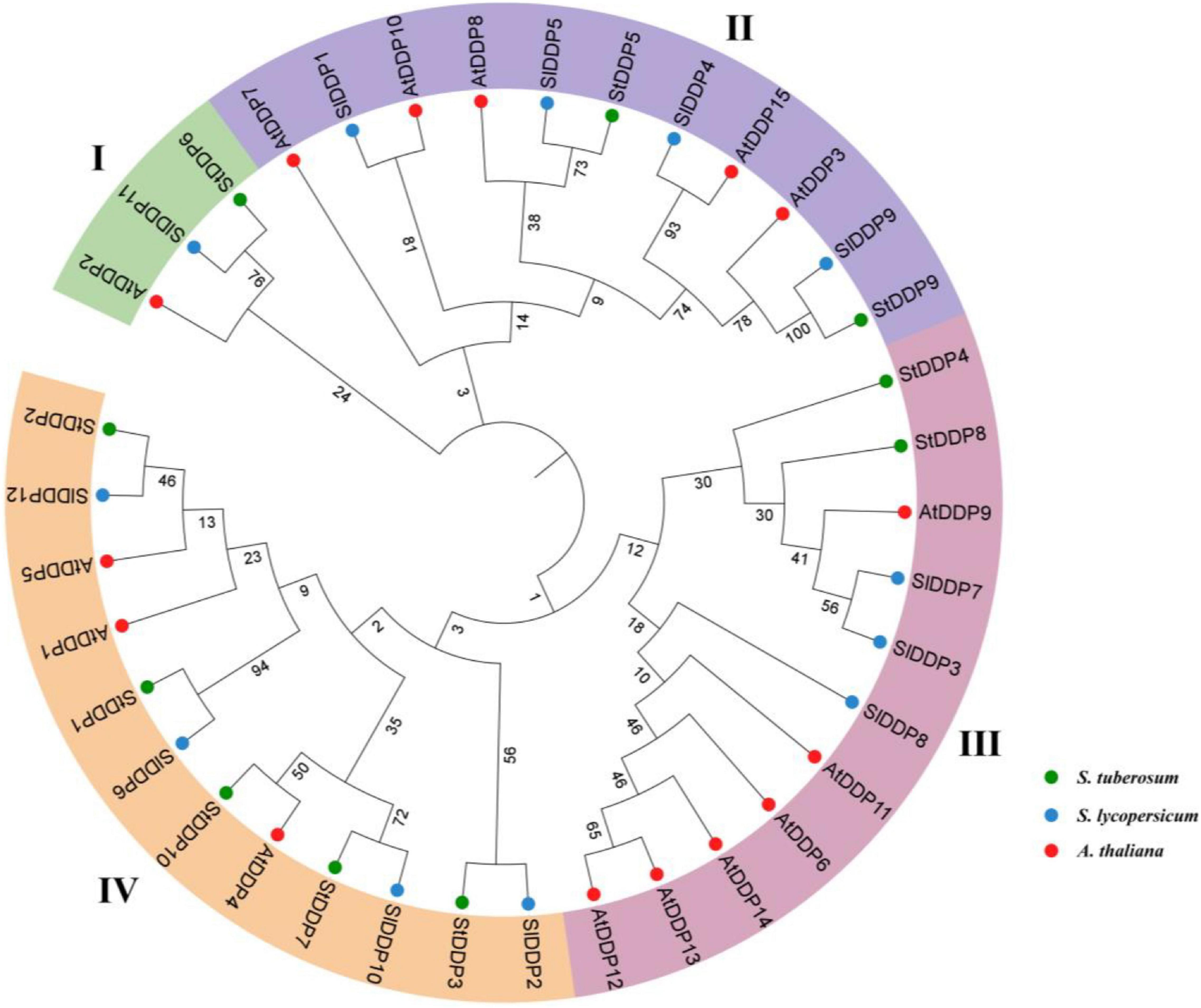
Figure 1. Phylogenetic tree of deafness-dystonia peptide (DDP) proteins from Arabidopsis, Solanum lycoperiscum, and Solanum tuberosum. The colored arcs indicate different groups. The stars’ colors, represent proteins of Arabidopsis S. lycoperiscum, and S. tuberosum.
Gene Structure and Conserved Motif Analysis
The genomic DNA and coding DNA sequences were used for exon-intron structure analysis in S. tuborosum. The number, length, and distribution of introns-exons were not the same among all genes. For instance, StDDP2 was the most extended, whereas StDDP9 was the smallest gene. The number of StDDP exons ranged from 1 to 13. In our results, StDDP8 has only one exon, whereas StDDP7 has a maximum of 13 exons. However, some genes, such as StDDP2, StDDP3, and StDDP6 have a similar number of exons. In addition, StDDP1 and StDDP5 have an equal number of exons but with different sequence lengths (Figure 2).
The architecture of StDDP proteins was also investigated using DDP amino acids sequences. The MEME motif analysis identified several common and unique motifs in StDDPs. Commonly shared motifs tended to cluster in the same groups, indicating similar functions. The first motifs were observed in all StDDPs, except StDDP10, whereas the second motif was observed in most of the proteins except StDDP8. Motif 3 was absent in StDDP8. In summary, some motifs were family-specific, group-specific, clade, and taxa-specific. The length of motifs also varied; for example, the first and ninth motifs had 49 amino acids, the second, third, sixth, and seventh motifs had 50 amino acids and the eighth, tenth, and fifth motifs had 29 amino acids (Figure 3).
Chromosomal Distribution and Cis Element Analysis
The numbers of StDDP genes were present unequally on chromosomes. The maximum number of DDP genes was observed on chromosome # 2 (four genes). A total of two DDP genes were present on chromosomes # 8 and 9. Only one gene was present on chromosomes # 4 and 10 (Table 1). The results of the cis-element analysis indicated that generally, the cis-acting elements were from growth and development, phytohormones, and stress-responsive classes. Light and MYB binding were the most enriched elements. Furthermore, anaerobic induction, stress-responsive components, and defense were also enriched in their promoters among stress-responsive components. The MeJRE and ABA response elements were enriched in phytohormones responsive elements. Consequently, it was observed that various cis-regulatory elements carried out DDP gene expression (Figure 4).
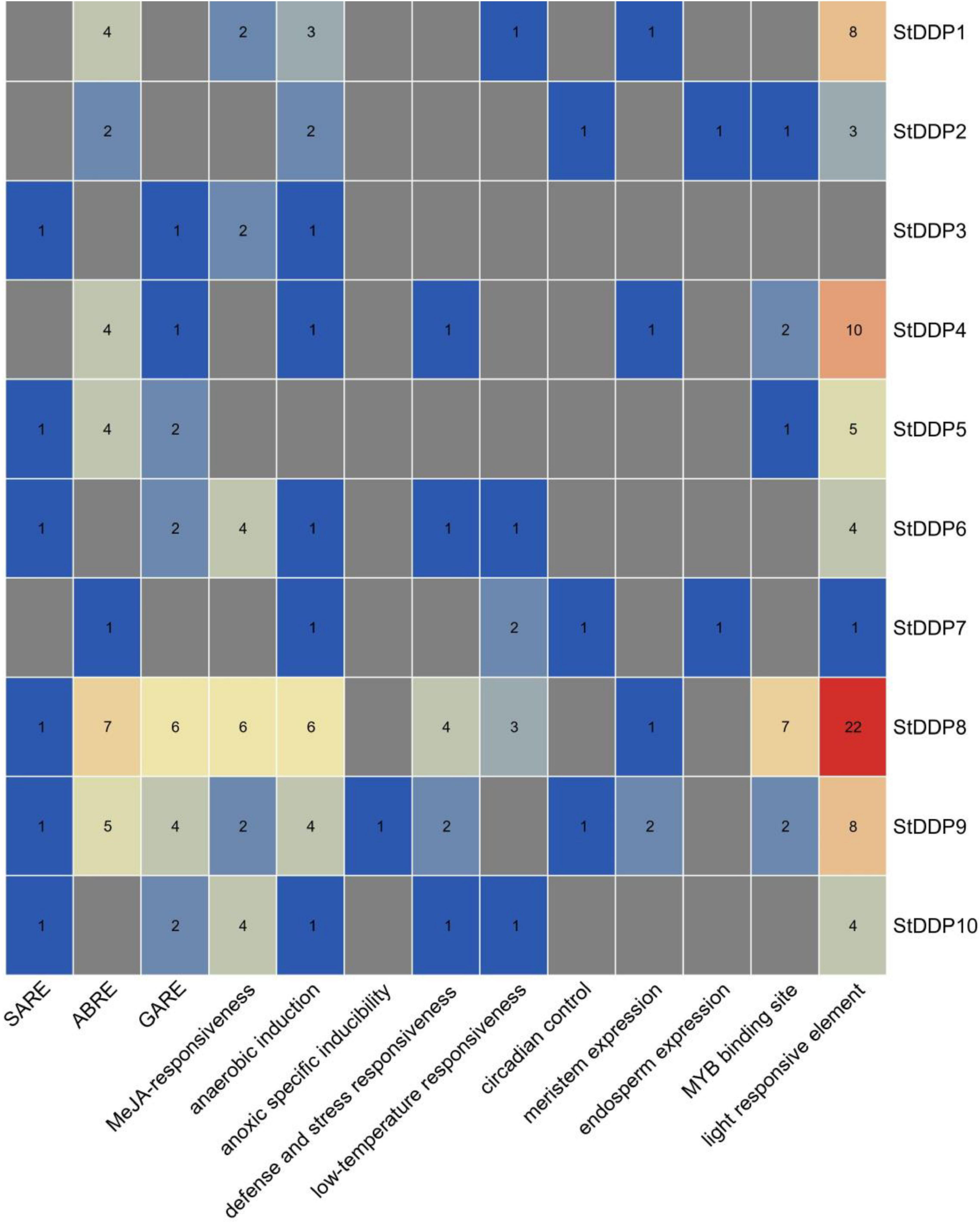
Figure 4. Predicted cis-acting elements in StDDP promoters. Promoter sequences (2,000 bp) of ten StDDP genes were analyzed by PlantCARE.
Genes Duplications and Identification of Orthologous Gene Clusters
The rate of molecular evolution was measured for entire duplicated gene pairs with estimating the rate of Ka/Ks. The value of Ka/Ks > 1 was a positive selection factor, whereas the Ks/Ks < 1 were indicated as a purifying selection factor. Ka/Ks = 1 was indicated a neutral selection amongst the duplicated genes. Our results illustrated that the majority of the DDP duplicated genes tolerated the pressure of purifying selection during the duplication process, implying that the role of the DDP duplicated genes may not alter comprehensively in successive evolutionary processes. The time deviation among duplicated gene pairs was also approximated. The cosmic majority of DDP genes illustrated the Ks > 0.52 value, although the resulting time deviation may be greater than 100 MYA (millions of years ago). Surprisingly, in the present study, the Ks value for the duplicated gene (StDDP1/StDDP2) was 0.614, while the deviation time was 118.17 MYA (Table 2).
The corresponding evaluation was carried out to determine orthologous clusters of DDPs in potato, tomato, and Arabidopsis genomes. This will aid in analyzing processes related to polyploidization throughout the evolutionary period of the DDP gene family in the genome of S. tuberosum. Spotted orthologous gene clusters and their imbricated regions of S. tuberosum are depicted in Figure 5. The highest number of in-paralogous genes was noted in S. lycopersicum. Seven in-paralogous genes were identified in S. tuberosum, and eight in-paralogous genes were identified in A. thaliana.
Comparative Synteny and miRNA Analysis
The synteny analysis in A. thaliana, S. lycopersicum, and S. tuberosum illustrated the significant relationship between gene evolution, duplication, triplication, expression, and function. The Solyc12g088230 gene sequence showed synteny in tomatoes with the StDDP2 gene sequence of potato. Likewise, the potato gene StDDP2 showed synteny with tomato Solyc02g036260. Potato StDDP2 presented synteny with tomato Solyc08g076310 (Figure 6).
In the previous few years, various studies have revealed miRNA-mediated regulation escorts stress responses in plants. The comprehensive information of entire miRNA targeted sites/genes is shown in Supplementary Table 2. These results illustrated that 10 members of the stu-miR395 targeted StDDP4. Three members of miR319-3p, stu-miR319a-3p, and stu-miR319b targeted two genes (StDDP1 and StDDP5). The stu-miR172a-5p targeted StDDP7, stu-miR172b-5p targeted StDDP2 and stu-miR172d-3p targeted StDDP1. Furthermore, stu-miR8033-3p targeted StDDP6. StDDP5, StDDP4, StDDP2, and StDDP1 genes were estimated to be targeted with more miRNAs. These results revealed that miRNAs have significant biological functions in the genome of the potato.
Tissue-Specific Expression Profiling
The expression profiling suggests that most DDP genes represented comparatively higher transcriptional abundance in roots than in stems and leaves. Though, some detected genes do not illustrate any expression, while others show a tissue-specific expression. Such as, StDDP8 was expressed in leaf, root, and stem tissues. In our results, the StDDP8 gene had high transcript abundance in the roots, and the StDDP9 gene was highly expressed in stems and leaves (Figure 7).
Expression Patterns of StDDP Genes in Response to Heat and Salt Stress
Based on the transcription levels of StDDPs in potatoes under heat stress, we inferred that StDDPs might take part against potato heat stress. Six StDDP genes were expressed against heat treatment, although the transcription levels of StDDP2, StDDP5, and StDDP7 are remarkably enhanced in potatoes after heat treatment (Figure 8). However, the expression level of StDDP5 was observed to be higher than those of StDDP2 and StDDP7. The expression of StDDP4 was lower compared with those of other genes. A total of 7 DDP genes were upregulated against salt treatment, although the transcription levels of StDDP5, StDDP7, and StDDP9 were remarkably enhanced in potatoes after salt treatment (Figure 8). The expression level of StDDP7 was observed to be higher than those of StDDP5 and StDDP9 in potatoes in response to salt treatment.
Expression Patterns of StDDP Genes in Response to Phytohormones
Indole acetic acid (IAA) and abscisic acid (ABA) were selected to identify the DDP genes’ transcriptional responses toward hormones treatments. Leaf tissues were treated with ABA, and the expression pattern of all 10 genes was observed. A total of seven DDP genes showed expression against ABA treatment, and StDDP9 showed higher expression compared to other StDDPs. Similarly, a total of seven DDP genes showed expression against IAA in treated leaves, and StDDP7 showed higher expression compared to other StDDPs (Figure 9). These results indicated a strong correlation between gene clusters or their expression in IAA and ABA treatments.
RT-qPCR Analysis
The RT-qPCR analysis also reported the expressions of the StDDP2, StDDP5, StDDP7, StDDP8, and StDDP9 genes in leaves, roots, and stems. The comparative expression analysis pattern illustrated that StDDP5, StDDP7, and StDDP8 have higher expressions in roots. Furthermore, StDDP2 and StDDP9 showed higher expression in stems than in roots and leaves. These results validate the RNA-Sequencing results. The higher expression in the roots demonstrates their strong association with the soil and environment (Figure 10).
Discussion
Most plants bear destruction from biotic and abiotic stress agents (Zaynab et al., 2020), which influence their productivity and survival rate throughout their life cycle (Zaynab et al., 2018). DDPs are implicated in several regulatory processes, including development, growth, biotic and abiotic stresses (Zaynab et al., 2021e). Plants have evolved tolerance systems to change their cellular biochemistry and physiology during stress by changing gene expression (Zaynab et al., 2017). One of the domains known as DUF221 is considered a more conserved membrane-associated protein and acts to stimulate calcium osmoregulation through the cellular membrane (Hou et al., 2014). Thus far, the status of DUF221 genes has been primarily unexplored. In this analysis, the DDP genes were observed in, salt, heat, and phytohormones stress, in keeping with previous identification in maize (Ding et al., 2019), rice (Li et al., 2015), and Arabidopsis (Yuan et al., 2014).
The structural identification of StDDPs is supportive during functional analysis.
All obtained StDDP genes were categorized into four phylogenetic groups. Similar phylogenetic grouping was also observed in other plant species. The comparative analysis of phylogeny illustrated that the organizations of A. thaliana, S. lycopersicum, and S. tuberosum proteins were relatively similar with each other in four clades, representing that all StDDP genes in representing groups may originate from a common ancestor. Previous studies have also reported the classification of DDPs into four clades in Arabidopsis (Yuan et al., 2014) and rice (Yuan et al., 2014; Li et al., 2015), and rice (Li et al., 2015).
Furthermore, evolutionary evidence found that exon-intron alignment affects gene family evolution (Moore and Purugganan, 2005). This is related to prior Scientific researches that several genes are subject to be kept in plants that could not have introns/short introns during the evolutionary process (Flagel and Wendel, 2009). In plants, gene expression levels are low with few and no introns (Mattick and Gagen, 2001). Moreover, a conserved gene configuration can allow a fast expression response toward an exogenous and endogenous gene stimulus (Jeffares et al., 2008). The structure analysis of the gene reported that the StDDP gene sequences illustrated a similarity in exon-intron number with analogous functional characters due to the origination of duplication course in evolutionary processes (Waqas et al., 2019). Some important factors, including gene distribution, genome size, and duplications, are involved in the genetic diversity of plants. However, the duplication factor of genetics has been extensively studied throughout the gene families’ expression origins, complexity, and evolutionary novelty. We also identified several duplication events in StDDPs, which played an important role in StDDP amplification. Gene duplication is an important event in expansion, diversification, and neofunctionalization (Lavin et al., 2005); correspondingly, the distribution and mapping of StDDP genes at the chromosomal level will support potato breeders in producing desired traits. Cis-element studies may illustrate an important foundation for functional analysis of the StDDP genes (Wen et al., 2021). Moreover, we also identified that all StDDP promoters hold multiple stress-responsive cis-elements, such as the low-temperature responsive elements, ABRE, the MeJA responsive elements, and the SA responsive elements (Raza et al., 2021a). These Cis-elements perform an essential function in stress response through stress-responsive gene regulation (Wu et al., 2014). Therefore, in StDDPs, these vital Cis-acting points propose their response to various environmental stress factors.
Over the past few years, through genome-wide examination, abundant miRNAs have been recognized in the potato to employ in different environmental factors. In addition obtained results reported that the DDPs in potatoes were targeted through miR172, miR8033, and miR319 members. These findings revealed that a particular gene may be regulated through multiple miRNAs. Some studies have reported the relationship between the ZmDDP (Ding et al., 2019) and AtDDP proteins (Zhao et al., 2015). The dynamic phytohormones and abiotic responsive expression patterns of StDDP genes are still ambiguous. The analysis of the expression pattern of StDDP genes supported us to understand their potential functions and propose a systematic base for future analysis. Therefore, StDDPs’ expression profiling and its validation are useful for deeper consideration of the potato genome. A current analysis presented a high transcript abundance of DDPs in plant roots; this finding was also established in a previous analysis (Yang et al., 2020) and helped in our results, in which StDDP4, StDDP5, and StDDP8 showed high expression in roots.
The results of RT-qPCR indicated that StDDP7 and StDDP8 were particularly upregulated in roots. This suggests that potato StDDP genes play a vital function in development, growth and, diverse stress responses. So far, an increasing number of studies have illustrated the importance of StDDPs against various stresses (Zhao et al., 2021). Furthermore, in potatoes, StDDP2, StDDP5, and StDDP7 were upregulated against heat stress. Though, the hormones can affect the physiological and biochemical reactions in plants during multiple signal transduction processes (Fatima et al., 2021). Moreover, IAA and ABA are important hormones in plant immunity. Numerous analyses have reported that DDPs are concerned with stress response and are involved in hormonal and developmental signaling (Waseem et al., 2021). To evaluate whether the StDDPs in potatoes were expressed by hormonal signaling, the potato leaves were treated with IAA and ABA, and gene expression was examined. After the ABA and IAA treatments, seven genes were influenced, indicating that various members of StDDPs played several roles in ABA- and IAA-induced immune response. During the ABA and IAA treatments, the upregulation of seven StDDP genes identified that ABA and IAA played important roles in the immune system, in accordance with previous studies. results (Waseem et al., 2021). The expression of genes and their clusters highlighted a strong correlation between gene groups and their analysis in various tissues during various stresses. This co-occurrence and co-expression illustrate their putative character related to plant adaptation under varied environmental stresses.
Conclusion
Overall, a total of 10 StDDP genes were identified in S. tuberosum. The relative evolutionary analysis illustrated the existence of four main groups in the DDP gene family. Furthermore, the conserved structural and functional motifs were identified in StDDPs, through a slight change between groups and members. The high expression of StDDPs in roots demonstrated their significant role in plant–soil associations. The existing consequences present a profound understanding of main potato development and growth challenges under different stresses. In potatoes, StDDP5 represented higher expression analysis in response to heat stress. During ABA and IAA treatments, the seven StDDP gene expressions demonstrated that ABA and IAA played essential roles in defense. Furthermore, the StDDP9 gene showed higher expression against ABA treatment, and the StDDP7 gene showed higher expression against IAA treatment.
Data Availability Statement
The datasets presented in this study can be found in online repositories. The names of the repository/repositories and accession number(s) can be found in the article/Supplementary Material.
Author Contributions
MZ, YS, and RA-Y performed the experiments and wrote the manuscript. HS, IA, MN, KK, and SA revised the manuscript. SL revised and supervised the manuscript. All authors contributed to the article and approved the submitted version.
Funding
This work was supported by the Shenzhen Special Project for Sustainable Development (KCXFZ20201221173211033). The authors appreciate the support of the Research Center for Advanced Materials Science (RCAMS) at King Khalid University Abha, Saudi Arabia through a grant KKU/RCAMS/G002-21. Taif University Researchers Supporting Project number (TURSP-2020/38), Taif University, Taif, Saudi Arabia. This project was also supported by the King Saud University, Deanship of Scientific Research, College of Science Research.
Conflict of Interest
The authors declare that the research was conducted in the absence of any commercial or financial relationships that could be construed as a potential conflict of interest.
Publisher’s Note
All claims expressed in this article are solely those of the authors and do not necessarily represent those of their affiliated organizations, or those of the publisher, the editors and the reviewers. Any product that may be evaluated in this article, or claim that may be made by its manufacturer, is not guaranteed or endorsed by the publisher.
Supplementary Material
The Supplementary Material for this article can be found online at: https://www.frontiersin.org/articles/10.3389/fpls.2021.804600/full#supplementary-material
Footnotes
- ^ http://www.arabidopsis.org/
- ^ https://phytozome.jgi.doe.gov/
- ^ https://web.expasy.org/protparam/
- ^ https://github.com/CJ-Chen/TBtools
- ^ http://meme-suite.org/
- ^ http://bioinformatics.psb.ugent.be/webtools/plantcare/html/
- ^ http://plantgrn.noble.org/psRNATarget/
References
Albornos, L., Martín, I., Labrador, E., and Dopico, B. (2017). Three members of Medicago truncatula ST family are ubiquitous during development and modulated by nutritional status (MtST1) and dehydration (MtST2 and MtST3). BMC Plant Biol. 17:117. doi: 10.1186/s12870-017-1061-z
Bateman, A., Coggill, P., and Finn, R. D. (2010). DUFs: families in search of function. Acta Crystallogr. Sect. F Struct. Biol. Cryst. Commun. 66, 1148–1152. doi: 10.1107/S1744309110001685
Ding, S., Feng, X., Du, H., and Wang, H. (2019). Genome-wide analysis of maize OSCA family members and their involvement in drought stress. PeerJ 7:e6765. doi: 10.7717/peerj.6765
Fatima, M., Ma, X., Zhou, P., Zaynab, M., and Ming, R. (2021). Auxin regulated metabolic changes underlying sepal retention and development after pollination in spinach. BMC Plant Biol. 21:166. doi: 10.1186/s12870-021-02944-4
Flagel, L. E., and Wendel, J. F. (2009). Gene duplication and evolutionary novelty in plants. New Phytol. 183, 557–564. doi: 10.1111/j.1469-8137.2009.02923.x
Gholizadeh, A. (2016). DUF538 protein superfamily is predicted to be chlorophyll hydrolyzing enzymes in plants. Physiol. Mol. Biol. Plants 22, 77–85. doi: 10.1007/s12298-015-0331-1
Guo, C., Luo, C., Guo, L., Li, M., Guo, X., Zhang, Y., et al. (2016). OsSIDP366, a DUF1644 gene, positively regulates responses to drought and salt stresses in rice. J. Integr. Plant Biol. 58, 492–502. doi: 10.1111/jipb.12376
Hafeez, M. B., Zahra, N., Zahra, K., Raza, A., Khan, A., Shaukat, K., et al. (2021). Brassinosteroids: molecular and physiological responses in plant growth and abiotic stresses. Plant Stress 2, 100029.
Hou, C., Tian, W., Kleist, T., He, K., Garcia, V., Bai, F., et al. (2014). DUF221 proteins are a family of osmosensitive calcium-permeable cation channels conserved across eukaryotes. Cell Res. 24, 632–635. doi: 10.1038/cr.2014.14
Huang, F., Abbas, F., Fiaz, S., Imran, M., Yanguo, K., Hassan, W., et al. (2021). Comprehensive characterization of guanosine monophosphate synthetase in Nicotiana tabacum. Mol. Biol. Rep. 1–8. doi: 10.1007/s11033-021-06718-x
Jeffares, D. C., Penkett, C. J., and Bahler, J. (2008). Rapidly regulated genes are intron poor. Trends Genet. 24, 375–378. doi: 10.1016/j.tig.2008.05.006
Kim, S. J., Ryu, M. Y., and Kim, W. T. (2012). Suppression of Arabidopsis RING-DUF1117 E3 ubiquitin ligases, AtRDUF1 and AtRDUF2, reduces tolerance to ABA-mediated drought stress. Biochem. Biophys. Res. Commun. 420, 141–147. doi: 10.1016/j.bbrc.2012.02.131
Kim, Y., Tsuda, K., Igarashi, D., Hillmer, R. A., Sakakibara, H., Myers, C. L., et al. (2014). Mechanisms underlying robustness and tunability in a plant immune signaling network. Cell Host Microbe 15, 84–94.
Lavin, M., Herendeen, P. S., and Wojciechowski, M. F. (2005). Evolutionary rates analysis of leguminosae implicates a rapid diversification of lineages during the tertiary. Syst. Biol. 54, 575–594. doi: 10.1080/10635150590947131
Leng, Z.-X., Liu, Y., Chen, Z.-Y., Guo, J., Chen, J., Zhou, Y.-B., et al. (2021). Genome-wide analysis of the DUF4228 family in soybean and functional identification of GmDUF4228–70 in response to drought and salt stresses. Front. Plant Sci. 12:628299. doi: 10.3389/fpls.2021.628299
Li, G., Hou, M., Liu, Y., Pei, Y., Ye, M., Zhou, Y., et al. (2019). Genome-wide identification, characterization and expression analysis of the non-specific lipid transfer proteins in potato. BMC Genomics 20:375. doi: 10.1186/s12864-019-5698-x
Li, L.-H., Lv, M.-M., Li, X., Ye, T.-Z., He, X., Rong, S.-H., et al. (2018). The rice OsDUF810 family: OsDUF810.7 may be involved in the tolerance to salt and drought. Mol. Biol. 52, 489–496. doi: 10.1134/S002689331804012X
Li, M., Guo, L., Guo, C., Wang, L., and Chen, L. (2016). Over-expression of a DUF1644 protein gene, SIDP361, enhances tolerance to salt stress in transgenic rice. J. Plant Biol. 59, 62–73. doi: 10.1007/s12374-016-0180-7
Li, W., Huai, X., Li, P., Raza, A., Mubarik, M. S., Habib, M., et al. (2021). Genome-wide characterization of glutathione peroxidase (GPX) gene family in rapeseed (Brassica napus L.) revealed their role in multiple abiotic stress response and hormone signaling. Antioxidants 10, 1481. doi: 10.3390/antiox10091481
Li, Y., Yuan, F., Wen, Z., Li, Yihao, Wang, F., et al. (2015). Genome-wide survey and expression analysis of the OSCA gene family in rice. BMC Plant Biol. 15:261. doi: 10.1186/s12870-015-0653-8
Luo, C., Guo, C., Wang, W., Wang, L., and Chen, L. (2014). Overexpression of a new stress-repressive gene OsDSR2 encoding a protein with a DUF966 domain increases salt and simulated drought stress sensitivities and reduces ABA sensitivity in rice. Plant Cell Rep. 33, 323–336. doi: 10.1007/s00299-013-1532-0
Mahalingam, R., and Fedoroff, N. (2003). Stress response, cell death and signalling: the many faces of reactive oxygen species. Physiol. Plant. 119, 56–68. doi: 10.1034/j.1399-3054.2003.00156.x
Mattick, J. S., and Gagen, M. J. (2001). The evolution of controlled multitasked gene networks: the role of introns and other noncoding RNAs in the development of complex organisms. Mol. Biol. Evol. 18, 1611–1630. doi: 10.1093/oxfordjournals.molbev.a003951
Moore, R. C., and Purugganan, M. D. (2005). The evolutionary dynamics of plant duplicate genes. Curr. Opin. Plant Biol. 8, 122–128. doi: 10.1016/j.pbi.2004.12.001
Muhammad Ahmad, H., Wang, X., Fiaz, S., Rahman, M. U., Azhar Nadeem, M., Aslam Khan, S., et al. (2021). Comprehensive genomics and expression analysis of eceriferum (CER) genes in sunflower (Helianthus annuus). Saudi J. Biol. Sci. 28, 6884–6896. doi: 10.1016/j.sjbs.2021.07.077
Qin, H., Chen, F., Huan, X., Machida, S., Song, J., and Yuan, Y. A. (2010). Structure of the Arabidopsis thaliana DCL4 DUF283 domain reveals a noncanonical double-stranded RNA-binding fold for protein–protein interaction. RNA 16, 474–481. doi: 10.1261/rna.1965310
Rai, A., Suprasanna, P., D’Souza, S. F., and Kumar, V. (2012). Membrane topology and predicted rna-binding function of the ‘early responsive to dehydration (ERD4)’ plant protein. PLoS One 7:e32658. doi: 10.1371/journal.pone.0032658
Rashid, M.-O., Li, J.-H., Liu, Q., Wang, Y., and Han, C.-G. (2021). Molecular detection and identification of eight potato viruses in Gansu province of China. Curr. Plant Biol. 25, 100184. doi: 10.1016/j.cpb.2020.100184
Raza, A., Tabassum, J., Kudapa, H., and Varshney, R. K. (2021c). Can omics deliver temperature resilient ready-to-grow crops? Crit. Rev. Biotechnol. 41, 1209–1232.
Raza, A., Su, W., Hussain, M. A., Mehmood, S. S., Zhang, X., Cheng, Y., et al. (2021b). Integrated analysis of metabolome and transcriptome reveals insights for cold tolerance in rapeseed (Brassica napus L.). Front. Plant Sci. 12:721681. doi: 10.3389/fpls.2021.721681
Raza, A., Su, W., Gao, A., Mehmood, S. S., Hussain, M. A., Nie, W., et al. (2021a). Catalase (CAT) gene family in rapeseed (Brassica napus L.): genome-wide analysis, identification, and expression pattern in response to multiple hormones and abiotic stress conditions. Int. J. Mol. Sci. 22:4281.
Sade, N., del Mar Rubio-Wilhelmi, M., Umnajkitikorn, K., and Blumwald, E. (2018). Stress-induced senescence and plant tolerance to abiotic stress. J. Exp. Bot. 69, 845–853. doi: 10.1093/jxb/erx235
Waqas, M., Azhar, M. T., Rana, I. A., Azeem, F., Ali, M. A., Nawaz, M. A., et al. (2019). Genome-wide identification and expression analyses of WRKY transcription factor family members from chickpea (Cicer arietinum L.) reveal their role in abiotic stress-responses. Genes Genomics 41, 467–481. doi: 10.1007/s13258-018-00780-9
Waseem, M., Aslam, M. M., and Shaheen, I. (2021). The DUF221 domain-containing (DDP) genes identification and expression analysis in tomato under abiotic and phytohormone stress. GM Crops Food 1–14. doi: 10.1080/21645698.2021.1962207
Wen, Y., Raza, A., Chu, W., Zou, X., Cheng, H., Hu, Q., et al. (2021). Comprehensive in silico characterization and expression profiling of TCP gene family in rapeseed. Front. Genet. 12:794297. doi: 10.3389/fgene2021.794297
Wu, J., Wang, J., Pan, C., Guan, X., Wang, Y., Liu, S., et al. (2014). Genome-wide identification of MAPKK and MAPKKK gene families in tomato and transcriptional profiling analysis during development and stress response. PLoS One 9:e103032. doi: 10.1371/journal.pone.0103032
Xin, Z., Mandaokar, A., Chen, J., Last, R. L., and Browse, J. (2007). Arabidopsis ESK1 encodes a novel regulator of freezing tolerance. Plant J. 49, 786–799. doi: 10.1111/j.1365-313X.2006.02994.x
Yang, Q., Niu, X., Tian, X., Zhang, X., Cong, J., Wang, R., et al. (2020). Comprehensive genomic analysis of the DUF4228 gene family in land plants and expression profiling of ATDUF4228 under abiotic stresses. BMC Genomics 21:12. doi: 10.1186/s12864-019-6389-3
Yuan, F., Yang, H., Xue, Y., Kong, D., Ye, R., Li, C., et al. (2014). OSCA1 mediates osmotic-stress-evoked Ca2+ increases vital for osmosensing in Arabidopsis. Nature 514, 367–371. doi: 10.1038/nature13593
Zaynab, M., Hussain, A., Sharif, Y., Fatima, M., Sajid, M., Rehman, N., et al. (2021c). Mitogen-activated protein kinase expression profiling revealed its role in regulating stress responses in potato (Solanum tuberosum). Plants 10, 1371. doi: 10.3390/plants10071371
Zaynab, M., Wang, Z., Hussain, A., Bahadar, K., Sajid, M., Sharif, Y., et al. (2021f). ATP-binding cassette transporters expression profiling revealed its role in the development and regulating stress response in Solanum tuberosum. Mol. Biol. Rep. 1–14.
Zaynab, M., Chen, H., Chen, Y., Ouyang, L., Yang, X., Hu, Z., et al. (2021a). Signs of biofilm formation in the genome of Labrenzia sp. PO1. Saudi J. Biol. Sci. 28, 1900–1912. doi: 10.1016/j.sjbs.2020.12.041
Zaynab, M., Pan, D., Fatima, M., Sharif, Y., Chen, S., and Chen, W. (2021d). Proteomics analysis of Cyclobalanopsis gilva provides new insights of low seed germination. Biochimie 180, 68–78. doi: 10.1016/j.biochi.2020.10.008
Zaynab, M., Chen, H., Chen, Y., Ouyang, L., Yang, X., Xu, W., et al. (2021b). Transcriptome analysis revealed transporter proteins role in the growth of Labrenzia sp. PO1 and SY1. J. King Saud Univ. Sci. 33:101433. doi: 10.1016/j.jksus.2021.101433
Zaynab, M., Sharif, Y., Abbas, S., Afzal, M. Z., Qasim, M., Khalofah, A., et al. (2021e). Saponin toxicity as key player in plant defense against pathogens. Toxicon 193, 21–27. doi: 10.1016/j.toxicon.2021.01.009
Zaynab, M., Fatima, M., Abbas, S., Sharif, Y., Umair, M., Zafar, M. H., et al. (2018). Role of secondary metabolites in plant defense against pathogens. Microb. Pathog. 124, 198–202. doi: 10.1016/j.micpath.2018.08.034
Zaynab, M., Kanwal, S., Furqan, M., Islam, W., Noman, A., Ali, G. M., et al. (2017). Proteomic approach to address low seed germination in Cyclobalnopsis gilva. Biotechnol. Lett. 39, 1441–1451. doi: 10.1007/s10529-017-2393-3
Zaynab, M., Sharif, Y., Fatima, M., Afzal, M. Z., Aslam, M. M., Raza, M. F., et al. (2020). CRISPR/Cas9 to generate plant immunity against pathogen. Microb. Pathog. 141:103996. doi: 10.1016/j.micpath.2020.103996
Zhang, L., Hou, D., Li, C., Li, X., Fan, J., Dong, Y., et al. (2021). Molecular characterization and function analysis of the rice OsDUF1664 family. Biotechnol. Biotechnol. Equip. 35, 53–60. doi: 10.1080/13102818.2020.1853606
Zhao, J., Wang, P., Gao, W., Long, Y., Wang, Y., Geng, S., et al. (2021). Genome-wide identification of the DUF668 gene family in cotton and expression profiling analysis of GhDUF668 in Gossypium hirsutum under adverse stress. BMC Genomics 22:395. doi: 10.1186/s12864-021-07716-w
Keywords: gene family, miRNA, expression, duplication, phylogeny
Citation: Zaynab M, Peng J, Sharif Y, Albaqami M, Al-Yahyai R, Fatima M, Nadeem MA, Khan KA, Alotaibi SS, Alaraidh IA, Shaikhaldein HO and Li S (2022) Genome-Wide Identification and Expression Profiling of DUF221 Gene Family Provides New Insights Into Abiotic Stress Responses in Potato. Front. Plant Sci. 12:804600. doi: 10.3389/fpls.2021.804600
Received: 29 October 2021; Accepted: 07 December 2021;
Published: 20 January 2022.
Edited by:
Shabir Hussain Wani, Sher-e-Kashmir University of Agricultural Sciences and Technology, IndiaReviewed by:
Shoaib Ur Rehman, Muhammad Nawaz Shareef University of Agriculture, PakistanSajid Fiaz, The University of Haripur, Pakistan
Copyright © 2022 Zaynab, Peng, Sharif, Albaqami, Al-Yahyai, Fatima, Nadeem, Khan, Alotaibi, Alaraidh, Shaikhaldein and Li. This is an open-access article distributed under the terms of the Creative Commons Attribution License (CC BY). The use, distribution or reproduction in other forums is permitted, provided the original author(s) and the copyright owner(s) are credited and that the original publication in this journal is cited, in accordance with accepted academic practice. No use, distribution or reproduction is permitted which does not comply with these terms.
*Correspondence: Shuangfei Li, sfli@szu.edu.cn
†These authors share first authorship