- 1State Key Laboratory of Tree Genetics and Breeding, Northeast Forestry University, Harbin, China
- 2College of Forest Resources and Environmental Science, Michigan Technological University, Houghton, MI, United States
Drought stress causes various negative impacts on plant growth and crop production. R2R3-MYB transcription factors (TFs) play crucial roles in the response to abiotic stress. However, their functions in Betula platyphylla haven’t been fully investigated. In this study, a R2R3 MYB transcription factor gene, BpMYB123, was identified from Betula platyphylla and reveals its significant role in drought stress. Overexpression of BpMYB123 enhances tolerance to drought stress in contrast to repression of BpMYB123 by RNA interference (RNAi) in transgenic experiment. The overexpression lines increased peroxidase (POD) and superoxide dismatase (SOD) activities, while decreased hydrogen peroxide (H2O2), superoxide radicals (O2–), electrolyte leakage (EL) and malondialdehyde (MDA) contents. Our study showed that overexpression of BpMYB123 increased BpLEA14 gene expression up to 20-fold due to BpMYB123 directly binding to the MYB1AT element of BpLEA14 promoter. These results indicate that BpMYB123 acts as a regulator via regulating BpLEA14 to improve drought tolerance in birch.
Introduction
Drought is an adverse environmental condition as sessile plants have to cope with it at some point degrees in the their life cycle. When drought occurs, cells and tissues in plants are deprived of water, resulting in shrinkage, collapses, attenuated growth and eventually loss of biomass and productivity (Eziz et al., 2017). With the explosion of the world’s population and million metric tons of greenhouse gases, the global climate change increases the odds of worsening of drought in the world. In addition, drought alone causes more annual loss in crop yield than all other environmental factors combined (Ciais et al., 2005; Nadeem et al., 2019). Short-term drought has a direct destructive effect on the growth and production of annual crops (Wang et al., 2020) and can cause dieback of perennial woody plants. Long-term drought escalate the damage to forests, ecosystems, and wildlife (Gomes and Bernardino, 2020), and has the potential to alter forest composition and provoke the occurrence of tree-damaging forest insects and pathogens. Therefore, it is of great significance to understand the molecular mechanism of improving drought tolerance in plant (Zandalinas et al., 2018).
MYB transcription factor family plays an important role in the adaptive response to drought stress (Baldoni et al., 2015). The DNA-binding domains of MYB gene family consists of 51-52 amino acids, including three repeated sequences of R1, R2 and R3, each of which contains an H-T-H three-dimensional structure (Ogata et al., 1996). MYB is subdivided into four subfamilies according to the number of adjacent repeat units repeats: 4R-MYB, R1R2R3-MYB (3R-MYB), R2R3-MYB, and 1R-MYB (MYB-related) (Dubos et al., 2010).
In plants, the majority of MYB proteins belong to the R2R3-MYB subfamily that play central roles in response to drought stress, including root growth, stomatal movement, signaling transduction, homeostasis, and metabolite biosynthesis. For instance, AtMYB96 plays a critical role in root growth under drought (Seo et al., 2009). MdSIMYB-overexpressing apple plant develops more robust root systems as compared to wild-type, and an improved tolerance to salt, drought and cold (Wang et al., 2014). Hypersensitivity to drought stress occurs to the transgenic overexpression plants of AtMYB60, a guard cell-specific gene (Oh et al., 2011), whereas the light-induced stomatal opening is repressed in the myb60 mutant, which enhances plant drought tolerance via less water loss (Cominelli et al., 2005). The silencing of GbMYB5 gene decreases the drought tolerance in cotton seedlings, while the stomatal size and stomatal opening rate are significantly reduced in GbMYB5 overexpression transgenic tobacco, resulting in the reduced the water loss and improved the survival rate under drought stress (Chen et al., 2015). The other example is Arabidopsis thaliana AtMYB96, whose overexpression transgenic lines exhibit normal growth and development and enhanced tolerance to drought. The deposition of epicuticular wax crystals increases significantly on the surfaces of transgenic leaves (Lee et al., 2014). GmMYB14, whose overexpression enhances the drought tolerance significantly through regulating plant architecture mediated by the brassinosteroid pathway. GmMYB14 binds to the promoter of GmBEN1 and up-regulates its expression, resulting in decreasing the content of brassinosteroids (BRs) (Chen et al., 2021a). Furthermore, AtMYB12 regulates the key enzyme genes involved in the biosynthesis of flavonoid in the AtMYB12-OE transgenic lines, leading to the significant increase of the abscisic acid (ABA), proline, superoxide dismatase (SOD) and peroxidase (POD), and reduction of hydrogen peroxide (H2O2) and malondialdehyde (MDA) levels (Wang et al., 2016). In comparison with wild-type, the ABA content in PtrMYB94 overexpression transgenic lines was significantly increased. PtrMYB94 coordinates with ABA signaling and improves drought tolerance in Populus (Fang et al., 2019). Phytohormone signaling as key for regulating the response to drought (Gupta et al., 2020). In rice, overexpression of a R2R3-MYB gene, SiMYB56, from foxtail millet significantly enhances tolerance to drought stress whereas the lower MDA content and higher lignin and ABA contents were observed (Xu et al., 2020b).
Although MYB transcription factor has been studied extensively, R2R3-MYB gene functions in Betula platyphylla haven’t been fully investigated. In this study, we characterized the roles of R2R3-MYB transcription factor gene, BpMYB123, from B. platyphylla in response to drought stress. We found that the overexpression of BpMYB123 in transgenic lines significantly improved the drought resistance of B. platyphylla, while down-regulation of BpMYB123 using RNA interference (RNAi) decreased stress tolerance in repression transgenic lines. We used the yeast one-hybrid (Y1H) and chromosomal immunoprecipitation (ChIP) to show that BpMYB123 regulated the expression of BpLEA14 by binding to the MYB1AT element in the promoter of BpLEA14, thereby improving the drought resistance of the plants. Overall, this study sheds new insights on drought tolerance mechanisms with broader implications for breeding drought tolerant varieties in B. platyphylla.
Materials and Methods
Vector Construction and Plant Transformation
We cloned the coding sequences (CDS) of BpMYB123 whose gene identifier is BPChr03G03353 in recently published B. platyphylla genome (Chen et al., 2021b). Primers with adaptors containing specific restriction sites were designed for further cloning. Using cDNA of B. platyphylla as template, PCR products were cloned into PMD19-T vector for cDNA sequence validation. The CDS of BpMYB123 was inserted into the binary vector pROK2 by using BamHI and KpnI double-enzyme digestion. Using the similar method, the CDS of BpMYB123 (XbaI and SalI) was cloned into binary vector of pBI121-GFP. The antisense fragments of BpMYB123 were amplified by PCR, and then cloned into the binary vector pROK2-RNAi. The primer sequences used are shown in Supplementary Table 1.
The three binary vectors, pROK2-BpMYB123, pBI121-BpMYB123-GFP and pROK2-RNAi-BpMYB123, were transformed into Agrobacterium strain EHA105 by freeze-thaw method (Weigel and Glazebrook, 2006). The B. platyphylla transgenic lines were generated by the leaf disc method (Lv et al., 2020a). To identify true transgenic lines, DNA was extracted from transgenic plants using a DNA extraction kit (TIANGEN, Beijing, China) and was used for PCR. RNA was extracted from transgenic lines using the CTAB method (Chang et al., 1993) and used for qRT-PCR to calculate the expression levels of BpMYB123 in transgenic lines. The primer sequences for PCR and qPCR are listed in Supplementary Table 1.
RNA-Seq Data Analysis
Three BpMYB123 overexpression lines were used for RNA-seq experiments. The RNA-Seq data was submitted to NCBI SRA database, and the BioProject ID was PRJNA776425. The clean reads were mapped to the B. platyphylla genome by using HISAT2 (Kim et al., 2019). The threshold of p-adjusted (padj) value <0.05 and fold change (FC) ≥2 were employed to identify the differentially expressed genes (DEGs). DEGs were annotated by eggNOG-mapper v2 (Cantalapiedra et al., 2021) and that were further analyzed by Gene Ontology (GO) enrichment analysis (Chen et al., 2020) to identify biological processes that were over-represented in DEGs.
Transcription Factor-Centered Yeast One-Hybrid and Yeast One-Hybrid
The TF-centered Y1H was used to identify the binding cis-elements of BpMYB123 following the previously described method (Ji et al., 2014). Positive colonies were chosen and sequenced to identify candidate cis-element sequences.
To do Y1H, the CDS of BpMYB123 was first cloned into the pGADT7 vector (Clontech), while the promoter of BpLEA14 was ligated into the pAbAi vector (Clontech). The construct, pAbAi-BpLEA14, was transformed into Y1HGold competent cells, and then cultured on SD/-Ura and SD/-Ura + AbA medium. Finally, pGADT7-BpMYB123 plasmids were transformed into pAbAi-BpLEA14 yeast competent cells, and cultured on SD/-Leu and SD/-Leu + AbA medium. The Y1H analysis was conducted using the Matchmaker Gold Yeast One-Hybrid Library Screening System Kit (Clontech). The primer sequences are listed in Supplementary Table 1.
Chromosomal Immunoprecipitation Experiment and Chromosomal Immunoprecipitation-PCR
pBI121-BpMYB123-GFP transgenic plants were subjected to ChIP experiments following a method as described previously (Li et al., 2014) using anti-GFP antibodies. The precipitated DNAs were used as the templates for ChIP-PCR. There were six MYB1AT cis-elements in the promoter of BpLEA14, we designed the primers that span at the six sites. The primer sequences used are shown in Supplementary Table 1.
Drought Stress Treatment
Birch seedlings were planted in soil in the greenhouse (16/8 h light/dark, 24°C) for two months. These seedlings were then used for drought treatment, the plants were placed in the greenhouse without watering for ten days. After that, the plants were irrigated for recovering. The phenotypes were the photographed.
To measure the physiological changes, the plants under drought treatment for seven days were harvested for measuring the activities of SOD and POD, the contents of H2O2, superoxide radicals (O2–), MDA and electrolyte leakage (EL). MDA, SOD and POD were measured following the methods as described previously (Wang et al., 2010), while EL was measured as described in our early publication (Lv et al., 2020b). H2O2 and O2– were measured using the kit (Hydrogen peroxide content kit, H2O2-2-Y, Superoxide anion kit, SA-2-G, Suzhou Comin Biotechnology).
B. platyphylla seedlings were cultured in bottles pre-filled with 1/2 MS + 0.02 mg/L NAA + 2% (w/v) sucrose media, and then placed in a tissue culture room pre-set to 16/8-h light/dark cycles and an average temperature of 25°C for two months. They were then subjected to drought stress, and the leaves of these stressed plants were harvested for nitroblue tetrazolium (NBT) and 3, 3′-diaminobenzidine (DAB) stainings, as described previously (Zhang et al., 2011).
Subcellular Localization of BpMYB123
In order to localize BpMYB123 proteins in the cells, the pBI121-BpMYB123-GFP plasmids were transferred into Agrobacterium strain EHA105, and then the agrobacteria were delivered into Nicotiana benthamiana epidermal cells using the injection method as described earlier (Pečenková et al., 2017). After the infection, the materials were placed at room temperature for 48 h. Fluorescence was observed and photographed under the confocal laser scanning microscope (LSM 800, Zeiss, Germany).
Statistical Analysis
The student’s t-test was employed to test the statistically significant differences of various measures between BpMYB123 transgenic lines and wild-type. The threshold for statistically significant differences was set to p-value <0.05.
Results
BpMYB123 Gene Sequence and Subcellular Localization
Based on Sanger sequencing, CDS of BpMYB123 is 915 base pairs (bp), which is 81 bp longer than 834bp of BPChr03G03353 from Betula platyphylla reference genome (Supplementary File 1). The subcellular localization experiment showed that BpMYB123 transcription factor was localized in the nucleus (Figure 1).
Drought Tolerance of BpMYB123 Transgenic Plants
Nine BpMYB123 overexpression (BpMYB123-OE) transgenic lines, and eight BpMYB123 repression (BpMYB123-RE) transgenic lines were obtained using leaf disc transformation method (Supplementary Figure 1). These transgenic lines and wild-type plants had been growing in the greenhouse for two months. After the drought treatment (without watering for 10-day) and a three-day watering recovery. Several leaves of BpMYB123-OE transgenic lines near the root shriveled and wilted, and half of them survived. In the wild-type, one of the third of the leaves survived, while all the leaves in the BpMYB123-RE transgenic lines withered (Figure 2A). We conclude that BpMYB123 improved drought tolerance of B. platyphylla. We harvested the leaves after drought treatment for about a week and measured the contents of proline, MDA and EL (Figures 2B–D). The content of proline in BpMYB123-OE was significantly higher than wild-type (Figure 2B), whereas BpMYB123-RE transgenic lines were lower than wild-type. Plant cells subjected to stress can lead to membrane lipid peroxidation, damage of the structure of biofilm and changes in the permeability of cell plasma membrane. MDA is one of the final products of membrane lipid peroxidation. The integrity of the plasma membranes of the cells can be determined by the results of EL. The results of MDA content and EL in transgenic plants and wild-type cells before and after stress were shown in Figures 2C,D. Before drought stress, the content of MDA and EL in cells of all transgenic lines were largely the same without a significant difference. After drought stress treatment, the content of MDA and EL in cells increased, indicating that the cell membranes were oxidized. MDA was produced and the permeability of cell membrane was enhanced under drought stress. While the contents of MDA and EL in BpMYB123-OE transgenic lines were significantly decreased, the contents of MDA and EL in BpMYB123-RE transgenic lines were significantly increased in comparison with wild-type.
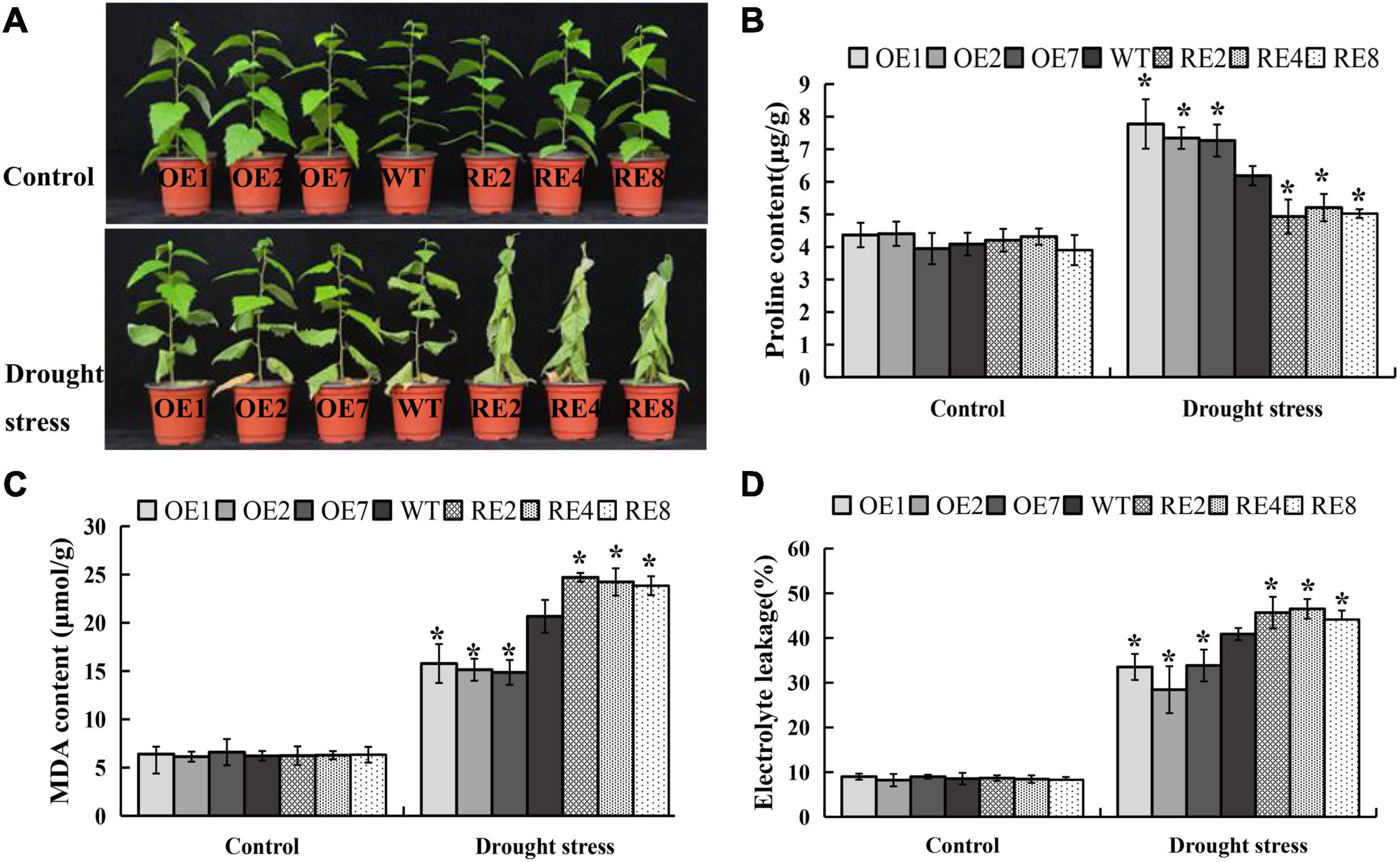
Figure 2. Overexpression of BpMYB123 conferred enhanced drought tolerance in its transgenic lines. (A) Phenotypic comparison of BpMYB123 transgenic lines and wild-type plants under drought stress. (B) Content of proline. (C) Content of malondialdehyde (MDA). (D) The result of electrolyte leakage. Asterisks indicate significant differences by t-test, p < 0.05.
Reactive Oxygen Species Scavenging in BpMYB123 Transgenic Lines
Biochemical and physiological traits were measured to determine if the gain- and loss-of-function in BpMYB123 transgenic lines under drought treatment. The DAB and NBT staining were used to measure H2O2 and O2– levels; and thus represented the activities of POD and SOD. This is because POD and SOD are the enzymes responsive for removing H2O2 and O2–. After drought treatment, all three BpMYB123-OE lines showed significantly reduced H2O2 and O2– levels compared to wild-type (Figures 3A,B). POD and SOD enzyme activities in the BpMYB123-OE transgenic lines were significantly higher than those in the wild-type (Figures 3C,D). Correspondingly, the contents of H2O2 and O2– were significantly lower than those in the wild-type (Figures 3E,F). Thus, overexpression transgenic plants could eliminate reactive oxygen species (ROS) and diminish the toxicity of ROS.
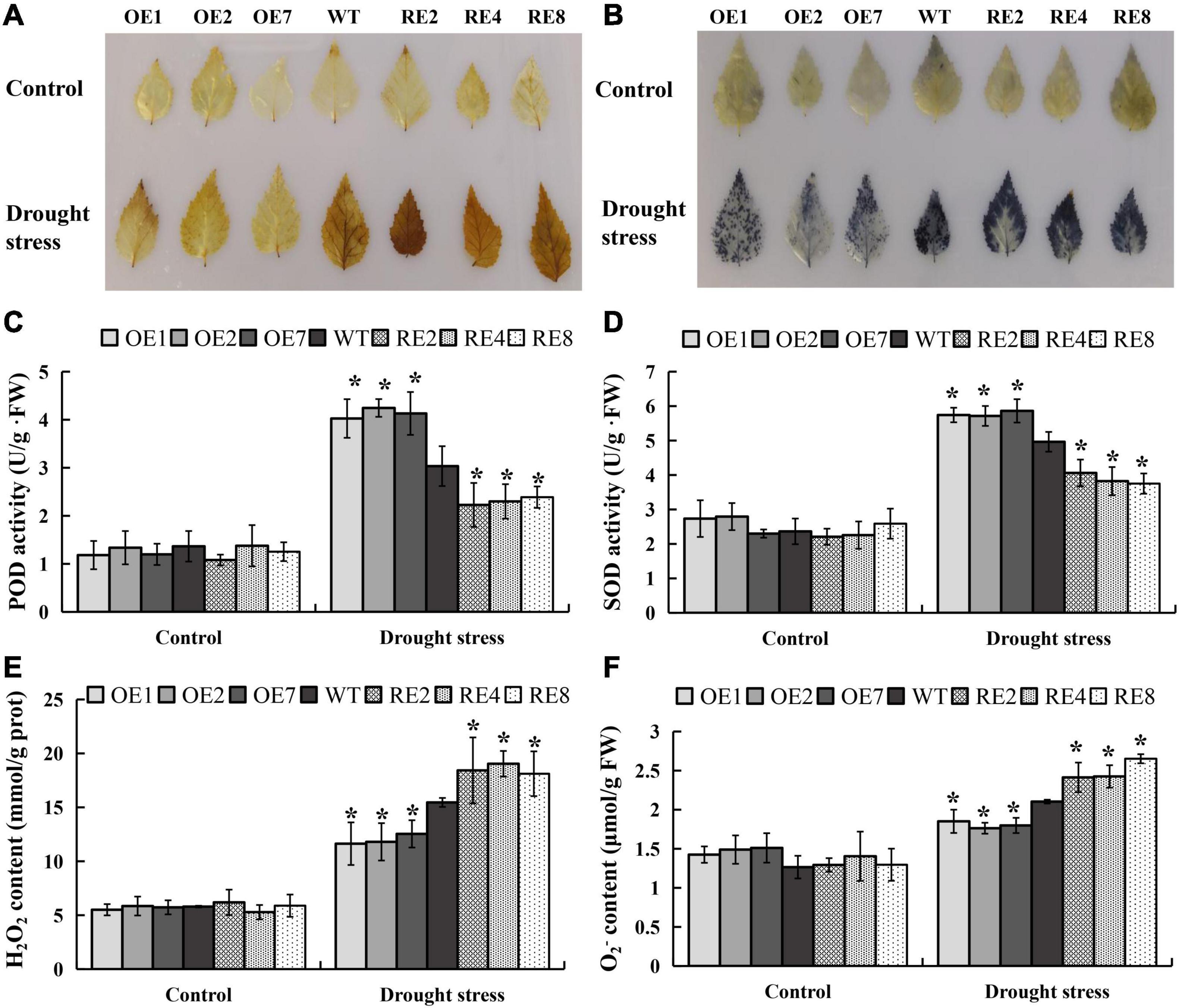
Figure 3. Reactive oxygen species (ROS) scavenging analyses in BpMYB123-overexpression (BpMYB123-OE) and BpMYB123-repression (BpMYB123-RE) transgenic lines and wild-type. (A) Content of hydrogen peroxide (H2O2) of BpMYB123 different lines under drought stress by 3,3′-diaminobenzidine (DAB) staining. (B) Content of superoxide radicals (O2–) of BpMYB123 different lines under drought stress by nitroblue tetrazolium (NBT) staining. (C) Content of peroxidase (POD). (D) Content of superoxide dismatase (SOD). (E) Content of H2O2. (F) Content of O2–. Asterisks indicate significant differences by t-test, p < 0.05.
Identification and Validation of BpMYB123-Binding Motifs Using Yeast One-Hybrid
Using a TF-centered Y1H method, we obtained multiple yeast colonies containing pHIS2 element library plasmids with candidate sequence motifs, which could potentially be bound by BpMYB123 protein. We used the sequencing results to search the PLACE database1 and identify cis-elements in the inserted sequences. Some motifs were identified. There are six MYB1AT motifs in the promoter of BpLEA14. Because MYB1AT (WAACCA) is an element closely related to abiotic stress, and we considered it was the candidate motif (Table 1).
RNA-Seq Data Analysis
1480 DEGs were identified in BpMYB123-OE lines as shown in Supplementary Table 2. Gene ontology (GO) enrichment analysis was performed using the DEGs form a pairwise comparison of BpMYB123-OE vs wild-type. The result showed a large number of DEGs responded to abiotic stress (Supplementary Table 3). There were 132 genes belonged to biological process of response to hormones (GO:0009725); 61 out of these 132 genes belong to biological process of respond to abscisic acid (GO:0009737), and many DEGs were responsive to metabolic process, such as cellular glucan metabolic process (GO:0006073); glycogen metabolic process (GO:0005977); energy reserve metabolic process (GO:0006112). According to the adjusted p-value, the top 20 GO terms were shown (Supplementary Figure 2).
BPChr06G29277 encodes a late embryogenesis abundant protein (LEA) and its protein sequence is highly homologous to AtLEA14 in A. thaliana; it was thus named BpLEA14. BpLEA14 was significantly up-regulated (Log2 based fold change = 4.35, adjusted p-value = 6.04E-51) in BpMYB123-OE lines. We believe that BpLEA14 plays an important role in enhancement of drought tolerance in BpMYB123 transgenic plants.
Identification of the Downstream Target Gene BpLEA14 of BpMYB123
To confirm that BpMYB123 could bind to BpLEA14, we performed Y1H (Figure 4B). The result confirmed the binding of BpMYB123 to the promoter of BpLEA14.
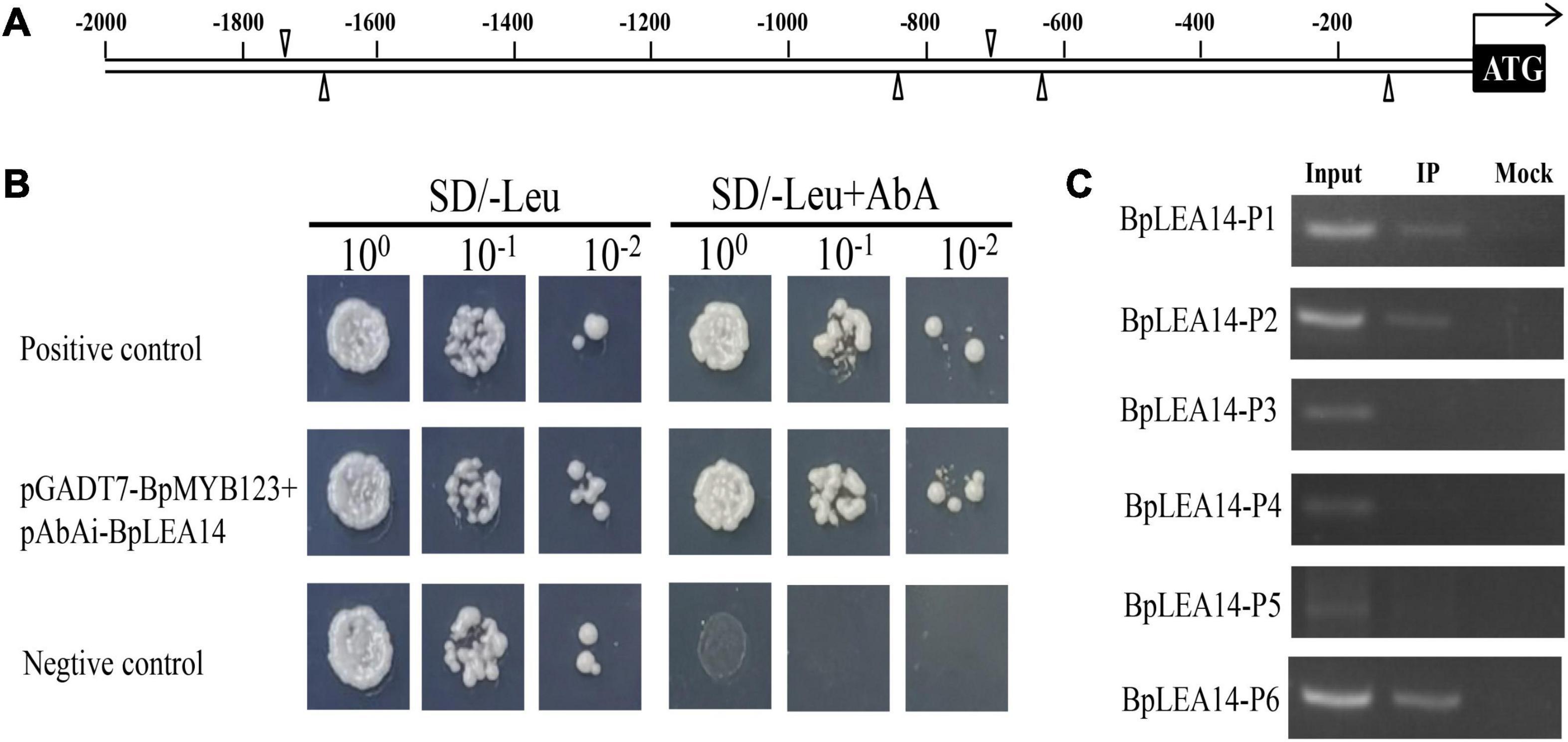
Figure 4. BpLEA14 was the target gene of BpMYB123. (A) Proximal locations of BpMYB123 binding cis-elements in BpLEA14 promoter sequence. (B) BpMYB123 could bind to the promoter of BpLEA14. (C) Chromosomal immunoprecipitation (ChIP)-PCR analysis proved the binding of BpMYB123 to BpLEA14 promoter in vivo using an anti-GFP tag antibody for ChIP.
ChIP-PCR was used to identify putative target gene of BpMYB123. There were six MYB1AT (WAACCA) cis-elements in the promoter of BpLEA14 (Figure 4A). To verify that BpMYB123 could bind to BpLEA14 by MYB1AT (WAACCA) motifs, we performed ChIP-PCR. After ChIP was done, the regions spanning the six MYB1AT motifs in BpLEA14 promoter were amplified using PCR and the results were shown in Figure 4C. These results substantiated the binding of BpMYB123 to three MYB1AT (WAACCA) motifs in the promoter of BpLEA14.
Drought Tolerance Assays of BpLEA14 Overexpression Transgenic Lines
The CDS of BpLEA14 (456bp) was cloned from B. platyphylla, 1611bp shorter than the reference genomic region of BPChr06G29277 (2067bp). The sequence was shown in the Supplementary File 1. The CDS of BpLEA14 was inserted into the binary vector pROK2. Seven transgenic lines were obtained by the leaf disc method, and three lines with the highest gene expression were selected for subsequent experiments (Supplementary Figure 3). Two-month-old B. platyphylla transgenic lines were grown in a greenhouse at 25°C under 16 h light/8 h dark period. Before the drought experiment was performed, all plants were fully irrigated. After 12 days without water, the plants were subjected to dehydration. The photos were taken three days later after the rehydration we initiated (Figure 5A). The content of MDA and EL in BpLEA14 overexpression (BpLEA14-OE) transgenic lines were significantly decreased compared with wild-type (Figures 5B,C). That indicates BpLEA14 improves drought tolerancein B. platyphylla.
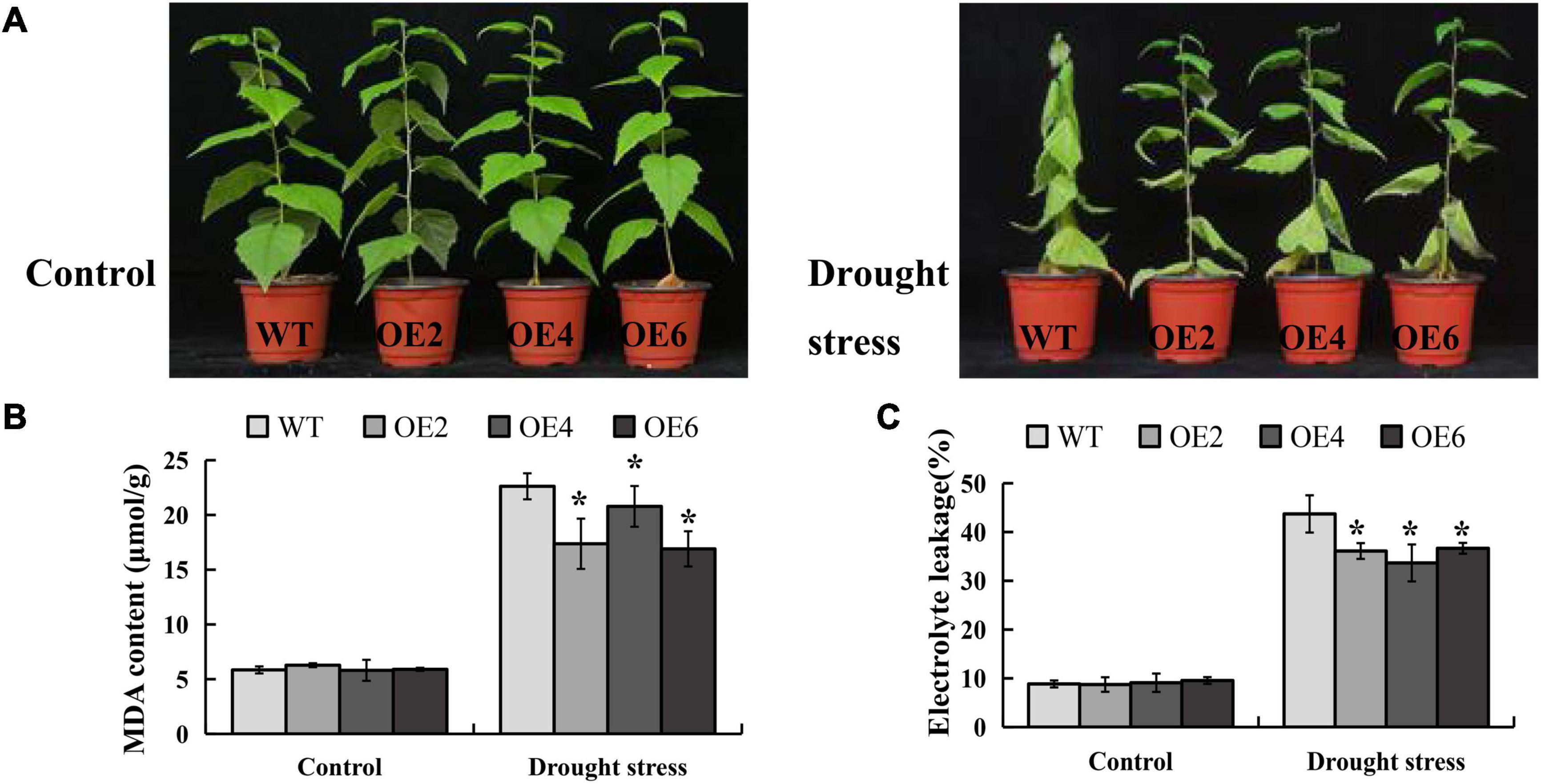
Figure 5. Overexpression of BpLEA14 conferred enhanced drought tolerance in its transgenic lines. (A) Phenotypic comparison of BpLEA14 overexpression (BpLEA14-OE) transgenic lines and wild-type (WT) plants under drought stress. (B) Content of malondialdehyde (MDA). (C) The result of electrolyte leakage. Asterisks indicate significant differences by t-test, p < 0.05.
Discussion
MYB transcription factors play key regulatory roles to improve plant drought tolerance. For example, overexpression of an apple R2R3 gene, MdoMYB121, in tomato leads to lower MDA and EL levels and higher proline content in response to drought (Cao et al., 2013). In addition, overexpression of rice MYB-related type gene, OsMYB48-1, improves the tolerance to mannitol, PEG and NaCl in rice (Xiong et al., 2014), consequently, the transgenic lines show the improved tolerance to drought and the high salinity stress. Moreover, the activities of SOD, the contents of proline and of chlorophyll in tobacco transgenic lines overexpressing sugarcane R2R3-MYB subfamily gene, SoMYB18, increase significantly, resulting in the salt and drought stress tolerance of transgenic plants (Shingote et al., 2015). Finally, overexpression of GmMYB118 in soybean improves tolerance to drought and salt stress through promoting expression of stress-associated genes and regulating osmotic and oxidizing substances to maintain cell homeostasis (Du et al., 2018).
In this study, we characterized the overexpression and repression transgenic lines of newly identified R2R3-MYB subfamily gene, BpMYB123, from Betula platyphylla. Under drought stress, the content of proline, activities of SOD and POD in BpMYB123-OE transgenic lines were significantly higher than those in wild-type, while the contents of H2O2 and O2– were significantly lower. On the contrary, the BpMYB123-RE repression lines exhibited some opposite physiological responses and changes as compared to overexpression. Notably, the ability to remove reactive oxygen species of BpMYB123-OE lines were augmented under drought stress. These changes are largely aligned with the functions of some of above mentioned R2R3 MYB subfamily genes reported in other species.
Plant hormones and metabolites play an important role in stress response and tolerance in plants. Metabolic pathways could coordinately alleviate oxidative stress (Naderi et al., 2020), and hormone accumulation affects plant response to drought (Xu et al., 2017). In this study many DEGs associated with hormone and metabolic pathways (Supplementary Table 3). Gene ontology enrichment analysis suggests that BpMYB123 regulates the hormone signaling and metabolic biosynthesis pathway under drought stress. This indicates that BpMYB123 may modulate the levels of hormones and metabolites.
LEA protein is a large group of hydrophilic, glycine-rich protein members that exist in many plants (Amara et al., 2014), and play a key role in a variety of stresses (Gao and Lan, 2016). The accumulation of LEA protein has been reported to augment the tolerance of multiple stresses including cold (Xu et al., 2020a), salt (Aduse Poku et al., 2020) and drought (Yang et al., 2018). LEA genes play a determinant role in drought tolerance in rice (Kamarudin et al., 2019); for example, over-expression of OsLEA3-1 in rice improves drought resistance (Xiao et al., 2007). In this study, over-expression of BpMYB123 caused more than 20-fold increase of BpLEA14, and BpLEA14 enhanced drought tolerance presumably by inhibiting cell membrane damage in B. platyphylla because over-expression of BpLEA14 reduced the relative electrical conductivity, and malondialdehyde content under drought stress in BpLEA14-OE transgenic lines.
Based on the above discussions and analyses, a schematic diagram sketching the molecular mechanism of how BpMYB123 enhanced stress tolerance in B. platyphylla was drawn (Figure 6). Under drought stress, BpMYB123 could enhance the ability of scavenging activity by boosting the activities of POD and SOD in transgenic plants. On the other hand, BpMYB123 bound to the MYB1AT cis-elements in the BpLEA14 promoter to regulate the expression of BpLEA14, improving the stability of cell membrane and enhancing the drought resistance of the plants.
Data Availability Statement
The original contributions presented in the study are publicly available. This data can be found here: https://www.ncbi.nlm.nih.gov/bioproject/, PRJNA776425.
Author Contributions
KL and GL designed the study. KL performed most experiments. KL, HW, and GL wrote the manuscript. All authors contributed to the article and approved the submitted version.
Funding
This work was supported by the China National Key R&D Programme during the 14th Five-year Plan Period “Forest Tree Genome Editing Technology” (2021YFD2200006) and Heilongjiang Touyan Innovation Team Program (Tree Genetics and Breeding Innovation Team).
Conflict of Interest
The authors declare that the research was conducted in the absence of any commercial or financial relationships that could be construed as a potential conflict of interest.
Publisher’s Note
All claims expressed in this article are solely those of the authors and do not necessarily represent those of their affiliated organizations, or those of the publisher, the editors and the reviewers. Any product that may be evaluated in this article, or claim that may be made by its manufacturer, is not guaranteed or endorsed by the publisher.
Supplementary Material
The Supplementary Material for this article can be found online at: https://www.frontiersin.org/articles/10.3389/fpls.2021.791390/full#supplementary-material
Supplementary Figure 1 | Identification of BpMYB123 transgenic lines. (A) The PCR identification of BpMYB123-overexpression (BpMYB123-OE) transgenic lines, M: DL2000 DNA Marker, N1: negative control (ddH2O), N2: negative control (wild-type of Betula platyphylla), P: positive plasmid, 1-9: different transgenic lines. (B) The qRT-PCR identification of BpMYB123-OE lines. (C) The PCR identification of BpMYB123-repression (BpMYB123-RE) lines. (D) The qRT-PCR identification of BpMYB123-RE lines. Asterisks indicate significant differences by t-test, p < 0.05.
Supplementary Figure 2 | Gene ontology (GO) enrichment analysis. According to the adjusted p-value, the top 20 GO terms were shown.
Supplementary Figure 3 | Identification of BpLEA14 transgenic lines. (A) The PCR identification of overexpression transgenic plants of BpMYB14, M: DL2000 DNA Marker, N1: negative control (ddH2O), N2: negative control (wild-type of Betula platyphylla), P: positive plasmid, 1-7: different transgenic lines. (B) The qRT-PCR identification of BpLEA14 overexpression (BpLEA14-OE) transgenic plants. Asterisks indicate significant differences by t-test, p < 0.05.
Footnotes
References
Aduse Poku, S., Nkachukwu Chukwurah, P., Aung, H. H., and Nakamura, I. (2020). Over-expression of a melon Y3SK2-Type LEA gene confers drought and salt tolerance in transgenic tobacco plants. Plants 9:9121749. doi: 10.3390/plants9121749.
Amara, I., Zaidi, I., Masmoudi, K., Ludevid, M., and Brini, F. (2014). Insights into late embryogenesis abundant (LEA) proteins in plants: from structure to the functions. Am. J. Plant Sci. 5, 3440–3455. doi: 10.4236/ajps.2014.522360
Baldoni, E., Genga, A., and Cominelli, E. (2015). Plant MYB transcription factors: their role in drought response mechanisms. Int. J. Mol. Sci. 16, 15811–15851. doi: 10.3390/ijms160715811
Cantalapiedra, C. P., Hernández-Plaza, A., Letunic, I., Bork, P., and Huerta-Cepas, J. (2021). eggNOG-mapper v2: functional annotation, orthology assignments, and domain prediction at the metagenomic scale. bioRxiv 2021:446934. doi: 10.1101/2021.06.03.446934
Cao, Z.-H., Zhang, S.-Z., Wang, R.-K., Zhang, R.-F., and Hao, Y.-J. (2013). Genome wide analysis of the apple MYB transcription factor family allows the identification of MdoMYB121 gene confering abiotic stress tolerance in plants. PloS One 8:e69955. doi: 10.1371/journal.pone.0069955
Chang, S., Puryear, J., and Cairney, J. (1993). A simple and efficient method for isolating RNA from pine trees. Plant Mol. Biol. Rep. 11, 113–116. doi: 10.1007/BF02670468
Chen, C., Chen, H., Zhang, Y., Thomas, H. R., Frank, M. H., He, Y., et al. (2020). TBtools: An integrative toolkit developed for interactive analyses of big biological data. Mol. Plant 13, 1194–1202. doi: 10.1016/j.molp.2020.06.009
Chen, L., Yang, H., Fang, Y., Guo, W., Chen, H., Zhang, X., et al. (2021a). Overexpression of GmMYB14 improves high-density yield and drought tolerance of soybean through regulating plant architecture mediated by the brassinosteroid pathway. Plant Biotechnol. J. 19, 702–716. doi: 10.1111/pbi.13496
Chen, S., Wang, Y., Yu, L., Zheng, T., Wang, S., Yue, Z., et al. (2021b). Genome sequence and evolution of Betula platyphylla. Horticult. Res. 8:37. doi: 10.1038/s41438-021-00481-7
Chen, T., Li, W., Hu, X., Guo, J., Liu, A., and Zhang, B. (2015). A Cotton MYB transcription factor, GbMYB5, is positively involved in plant adaptive response to drought stress. Plant Cell Physiol. 56, 917–929. doi: 10.1093/pcp/pcv019
Ciais, P., Reichstein, M., Viovy, N., Granier, A., Ogée, J., Allard, V., et al. (2005). Europe-wide reduction in primary productivity caused by the heat and drought in 2003. Nature 437, 529–533. doi: 10.1038/nature03972
Cominelli, E., Galbiati, M., Vavasseur, A., Conti, L., Sala, T., Vuylsteke, M., et al. (2005). A guard-cell-specific MYB transcription factor regulates stomatal movements and plant drought tolerance. Curr. Biol. 15, 1196–1200. doi: 10.1016/j.cub.2005.05.048
Du, Y.-T., Zhao, M.-J., Wang, C.-T., Gao, Y., Wang, Y.-X., Liu, Y.-W., et al. (2018). Identification and characterization of GmMYB118 responses to drought and salt stress. BMC Plant Biol. 18:320. doi: 10.1186/s12870-018-1551-7
Dubos, C., Stracke, R., Grotewold, E., Weisshaar, B., Martin, C., and Lepiniec, L. C. (2010). MYB transcription factors in Arabidopsis. Trends Plant Sci. 15, 573–581. doi: 10.1016/j.tplants.2010.06.005
Eziz, A., Yan, Z., Tian, D., Han, W., Tang, Z., and Fang, J. (2017). Drought effect on plant biomass allocation: a meta-analysis. Ecol. Evol. 7, 11002–11010. doi: 10.1002/ece3.3630
Fang, Q., Wang, X., Wang, H., Tang, X., Liu, C., Yin, H., et al. (2019). The poplar R2R3 MYB transcription factor PtrMYB94 coordinates with abscisic acid signaling to improve drought tolerance in plants. Tree Physiol. 40, 46–59. doi: 10.1093/treephys/tpz113
Gao, J., and Lan, T. (2016). Functional characterization of the late embryogenesis abundant (LEA) protein gene family from Pinus tabuliformis (Pinaceae) in Escherichia coli. Sci. Rep. 6:19467. doi: 10.1038/srep19467
Gomes, L. E. O., and Bernardino, A. F. (2020). Drought effects on tropical estuarine benthic assemblages in Eastern Brazil. Sci. Total Environ. 703:135490. doi: 10.1016/j.scitotenv.2019.135490
Gupta, A., Rico-Medina, A., and Cao-Delgado, A. I. (2020). The physiology of plant responses to drought. Science 368, 266–269. doi: 10.1126/science.aaz7614
Ji, X., Wang, L., Nie, X., He, L., Zang, D., Liu, Y., et al. (2014). A novel method to identify the DNA motifs recognized by a defined transcription factor. Plant Mol. Biol. 86, 367–380. doi: 10.1007/s11103-014-0234-5
Kamarudin, Z. S., Yusop, M. R., Ismail, M. R., Tengku Muda, Mohamed, M., Harun, A. R., et al. (2019). LEA gene expression assessment in advanced mutant rice genotypes under drought stress. Int. J. Genomics 2019:8406036. doi: 10.1155/2019/8406036
Kim, D., Paggi, J. M., Park, C., Bennett, C., and Salzberg, S. L. (2019). Graph-based genome alignment and genotyping with HISAT2 and HISAT-genotype. Nat. Biotechnol. 37, 907–915. doi: 10.1038/s41587-019-0201-4
Lee, S. B., Kim, H. R., and Kim, R. J. (2014). Overexpression of Arabidopsis MYB96 confers drought resistance in Camelina sativa via cuticular wax accumulation. Plant Cell Rep. 33, 1535–1546. doi: 10.1007/s00299-014-1636-1
Li, W., Lin, Y. C., Li, Q., Shi, R., Lin, C. Y., Chen, H., et al. (2014). A robust chromatin immunoprecipitation protocol for studying transcription factor-DNA interactions and histone modifications in wood-forming tissue. Nat. Protoc. 9, 2180–2193. doi: 10.1038/nprot.2014.146
Lv, K. W., Wei, H. R., and Jiang, J. (2020b). Overexpression ofBplERD15Enhances drought tolerance inbetula platyphyllaSuk. Forests 11:978. doi: 10.3390/f11090978
Lv, K., Li, J., Zhao, K., Chen, S., Nie, J., Zhang, W., et al. (2020a). Overexpression of an AP2/ERF family gene, BpERF13, in birch enhances cold tolerance through upregulating CBF genes and mitigating reactive oxygen species. Plant Sci. 292:110375. doi: 10.1016/j.plantsci.2019.110375
Nadeem, M., Li, J., Yahya, M., Sher, A., Ma, C., Wang, X., et al. (2019). Research progress and perspective on drought stress in Legumes: a review. Int. J. Mol. Sci. 20:2541. doi: 10.3390/ijms20102541
Naderi, S., Fakheri, B. A., Maali-Amiri, R., and Mahdinezhad, N. (2020). Tolerance responses in wheat landrace Bolani are related to enhanced metabolic adjustments under drought stress. Plant Physiol. Biochem. 150, 244–253. doi: 10.1016/j.plaphy.2020.03.002
Ogata, K., Kanei-Ishii, C., Sasaki, M., Hatanaka, H., Nagadoi, A., Enari, M., et al. (1996). The cavity in the hydrophobic core of Myb DNA-binding domain is reserved for DNA recognition and trans-activation. Nat. Struct. Biol. 3, 178–187. doi: 10.1038/nsb0296-178
Oh, J. E., Kwon, Y., Kim, J. H., Noh, H., Hong, S. W., and Lee, H. (2011). A dual role for MYB60 in stomatal regulation and root growth of Arabidopsis thaliana under drought stress. Plant Mol. Biol. 77, 91–103. doi: 10.1007/s11103-011-9796-7
Pečenková, T., Pleskot, R., and Žárský, V. (2017). Subcellular localization of arabidopsis pathogenesis-related 1 (PR1) Protein. Int. J. Mol. Sci. 18:825. doi: 10.3390/ijms18040825
Seo, P. J., Xiang, F., Qiao, M., Park, J.-Y., Lee, Y. N., Kim, S.-G., et al. (2009). The MYB96 transcription factor mediates abscisic acid signaling during drought stress response in arabidopsis. Plant Physiol. 151, 275–289. doi: 10.1104/pp.109.144220
Shingote, P. R., Kawar, P. G., Pagariya, M. C., Kuhikar, R. S., Thorat, A. S., and Babu, K. H. (2015). SoMYB18, a sugarcane MYB transcription factor improves salt and dehydration tolerance in tobacco. Acta Physiol. Plant. 37:217. doi: 10.1007/s11738-015-1961-1
Wang, C., Linderholm, H. W., Song, Y., Wang, F., Liu, Y., Tian, J., et al. (2020). Impacts of drought on Maize and Soybean production in northeast China during the past five decades. Int. J. Environ. Res. Public Health 17:2459. doi: 10.3390/ijerph17072459
Wang, F., Kong, W., Wong, G., Fu, L., Peng, R., Li, Z., et al. (2016). AtMYB12 regulates flavonoids accumulation and abiotic stress tolerance in transgenic Arabidopsis thaliana. Mol. Genet. Genomics 291, 1545–1559. doi: 10.1007/s00438-016-1203-2
Wang, R. K., Cao, Z. H., and Hao, Y. J. (2014). Overexpression of a R2R3 MYB gene MdSIMYB1 increases tolerance to multiple stresses in transgenic tobacco and apples. Physiol. Plant 150, 76–87. doi: 10.1111/ppl.12069
Wang, Y., Gao, C., Liang, Y., Wang, C., Yang, C., and Liu, G. (2010). A novel bZIP gene from Tamarix hispida mediates physiological responses to salt stress in tobacco plants. J. Plant Physiol. 167, 222–230. doi: 10.1016/j.jplph.2009.09.008
Weigel, D., and Glazebrook, J. (2006). Transformation of agrobacterium using the freeze-thaw method. Csh Protocol. 2006:4666. doi: 10.1101/pdb.prot4666
Xiao, B., Huang, Y., Tang, N., and Xiong, L. (2007). Over-expression of a LEA gene in rice improves drought resistance under the field conditions. Theor. Appl. Genet. 115, 35–46. doi: 10.1007/s00122-007-0538-9
Xiong, H., Li, J., Liu, P., Duan, J., Zhao, Y., Guo, X., et al. (2014). Overexpression of OsMYB48-1, a novel MYB-related transcription factor, enhances drought and salinity tolerance in rice. PloS One 9:e92913. doi: 10.1371/journal.pone.0092913
Xu, M., Tong, Q., Wang, Y., Wang, Z., Xu, G., Elias, G. K., et al. (2020a). Transcriptomic Analysis of the Grapevine LEA gene family in response to osmotic and cold stress reveals a key role for VamDHN3. Plant Cell Physiol. 61, 775–786. doi: 10.1093/pcp/pcaa004
Xu, W., Tang, W., Wang, C., Ge, L., Sun, J., Qi, X., et al. (2020b). SiMYB56 confers drought stress tolerance in transgenic Rice by regulating lignin biosynthesis and ABA signaling pathway. Front. Plant Sci. 11:785. doi: 10.3389/fpls.2020.00785
Xu, Y., Burgess, P., and Huang, B. (2017). Transcriptional regulation of hormone-synthesis and signaling pathways by overexpressing cytokinin-synthesis contributes to improved drought tolerance in creeping bentgrass. Physiol. Plant 161:12588. doi: 10.1111/ppl.12588
Yang, J., Zhao, S., Zhao, B., and Li, C. (2018). Overexpression of TaLEA3 induces rapid stomatal closure under drought stress in Phellodendron amurense Rupr. Plant Sci. 277, 100–109. doi: 10.1016/j.plantsci.2018.09.022
Zandalinas, S. I., Mittler, R., Balfagón, D., Arbona, V., and Gómez-Cadenas, A. (2018). Plant adaptations to the combination of drought and high temperatures. Physiol. Plant 162, 2–12. doi: 10.1111/ppl.12540
Keywords: abiotic stress, drought stress, Betula platyphylla, transcription factor, BpMYB123
Citation: Lv K, Wei H and Liu G (2021) A R2R3-MYB Transcription Factor Gene, BpMYB123, Regulates BpLEA14 to Improve Drought Tolerance in Betula platyphylla. Front. Plant Sci. 12:791390. doi: 10.3389/fpls.2021.791390
Received: 08 October 2021; Accepted: 18 November 2021;
Published: 10 December 2021.
Edited by:
Luis E. Hernandez, Autonomous University of Madrid, SpainReviewed by:
Bijayalaxmi Mohanty, National University of Singapore, SingaporeWenjing Yao, Nanjing Forestry University, China
Copyright © 2021 Lv, Wei and Liu. This is an open-access article distributed under the terms of the Creative Commons Attribution License (CC BY). The use, distribution or reproduction in other forums is permitted, provided the original author(s) and the copyright owner(s) are credited and that the original publication in this journal is cited, in accordance with accepted academic practice. No use, distribution or reproduction is permitted which does not comply with these terms.
*Correspondence: Guifeng Liu, liuguifeng@126.com