- Ecology and Conservation Biology, Institute of Plant Sciences, University of Regensburg, Regensburg, Germany
Seed persistence in the soil is crucial for population dynamics. Interspecific differences in soil seed mortality could be a mechanism that may stimulate species coexistence in herbaceous plant communities. Therefore, understanding the levels and causes of seed persistence is vital for understanding community composition and population dynamics. In this study, we evaluated the burial depth as a significant predictor of the temporal dynamics of soil seed persistence. We suppose that species differ in this temporal dynamics of soil seed persistence according to burial depth. Furthermore, we expected that burial depth would affect soil seed persistence differently concerning the species-specific type of dormancy, light, and fluctuating temperature requirements for germination. Seeds of 28 herbaceous species of calcareous grasslands were buried in the field into depths of 1, 5, and 10 cm under the soil surface. Seed viability was tested by germination and tetrazolium tests several times for three years. Species-specific seed traits—a type of dormancy, light requirements and alternating temperature requirements for germination, and longevity index—were used for disentangling the links behind species-specific differences in soil seed persistence. Our study showed differences in soil seed persistence according to the burial depth at the interspecific level. Generally, the deeper the buried seeds, the longer they stayed viable, but huge differences were found between individual species. Species-specific seed traits seem to be an essential determinant of seed persistence in the soil. Seeds of dormant species survived less and only dormant seeds stayed viable in the soil. Similarly, seeds of species without light or alternating temperature requirements for germination generally remained viable in the soil in smaller numbers. Moreover, seeds of species that require light for germination stayed viable longer in the deeper soil layers. Our results help understand the ecosystem dynamics caused by seed reproduction and highlight the importance of a detailed long-term investigation of soil seed persistence. That is essential for understanding the fundamental ecological processes and could help restore valuable calcareous grassland habitats.
Introduction
Soil seed bank formation is one of the bed-hedging mechanisms for many species because more or less part of their seeds stay ungerminated in the soil for the future years (Venable and Brown, 1988), especially in ecosystems where opportunities for seedling establishment are unpredictable (Wijayratne and Pyke, 2012; Bhatt et al., 2019). At the same time, species-specific differences in the soil seed persistence are known to promote species diversity and co-existence in herbaceous plant communities, as referred to in the storage effect concept (Chesson and Warner, 1981; Warner and Chesson, 1985; Facelli et al., 2005). Understanding the soil seed bank persistence is crucial for understanding the community dynamics in both short- and long-term perspectives (Basto et al., 2018), can improve predictions of species distribution under a changing environment (Ooi, 2012), and has direct implications for successful management and restoration of endangered ecosystems (Richter and Stromberg, 2005; Tatár, 2010; Chapman et al., 2019; Funk, 2021).
One widely used method to study soil seed persistence is determining the depth distribution of germinable seeds in the soil. The depth distribution of seeds is considered indirect evidence of seed longevity (Thompson, 1993; Thompson et al., 1997). Based on these measurements, Thompson et al. (1998) classified soil seed persistence as “transient” or “persistent” and calculated the longevity index to summarize seed-soil persistence data from different studies. On the other hand, direct evidence of seed longevity (i.e., the time elapsed since a species was the last present on the locality or long-term field burial experiments) is rarely used (but see Schwienbacher et al., 2010; Moravcová et al., 2018), although it is known that site-specific conditions can affect both the soil seed persistence (Schafer and Kotanen, 2003; Long et al., 2015) and burial depth (Benvenuti, 2007; Egawa and Tsuyuzaki, 2013). Furthermore, results may be confounded by seed size—seed number trade-off. Smaller seeds enter easier deeper soil layers (Benvenuti, 2007) and are often overrated according to their persistence than larger seeds during indirect longevity estimation (Saatkamp et al., 2009).
Moreover, the deeper the seeds are buried, the lower the chance of seedling reaching the surface (Pearson et al., 2002; Grundy et al., 2003). Different mechanisms which allow seeds to stay ungerminated in the deeper soil layers were evolved (Milberg et al., 2000; El-Keblawy et al., 2018). Three main aspects of germination traits are considered the most important for soil seed persistence, germination timing, and hitting the gap of favorable conditions after disturbances or during the season (Grubb, 1977; Fenner and Thompson, 2005; Saatkamp et al., 2011b). First, delayed germination via dormancy mechanisms (Baskin and Baskin, 2014). Second, light requirements for germination, since light can penetrate only an upper layer of soil (Kasperbauer and Hunt, 1988; Mandoli et al., 1990), so seeds can persist deeper until disturbances occur (Baskin and Baskin, 2014; Milberg et al., 2000). Third, alternating temperature requirements for germination may also serve as detection of burial depth and for a gap detection (Thompson and Grime, 1983).
It is unclear whether and how burial depth affects soil seed persistence during a time, and comparison among a higher number of species is almost missing (but see Rivera et al., 2012). Therefore, we investigated the soil seed persistence concerning the burial depth. We address these hypotheses: (i) the deeper the seeds are buried, the longer they will remain viable, (ii) seeds of non-dormant species will survive shorter in the soil without respect to the burial depth, and (iii) seeds of species with light and/or alternating temperature requirements for germination will survive in higher proportions in deeper layers where light is not available and temperature conditions are more constant.
Materials and Methods
Study Site and Species Selection
The experiment was located in Baden-Wuerttemberg, Germany. We chose four localities of calcareous grassland—Teck (48.59N, 9.47E), Eichhalde (48.58N, 9.49E), Eselsrain (48.51N, 9.06E), and Surrlesrain (48.84N, 9.05E). All localities are situated on White Jurassic rubble with rendzina soil type. Mild and dry climate and species-rich vegetation are typical. We selected 28 species concerning germinability (Beier, 1991) and aspects of seed-soil bank dynamics (Poschlod and Jackel, 1993). We collected seeds and performed burial experiments between June 1991 and October 1996 (see Table 1 for details about species, localities, and experiment timing).
Burial Experiment
For the burial experiment, the homogeneous site at each of the localities was selected. We collected diaspores at the same locality where the burial experiment was performed. Harvest was timed to the moment of full maturity, i.e., it was possible to separate seeds from the mother plant with a light touch (except for Carlina sp., whose entire inflorescences were collected in mid-October before the achenes were blown off). Random selection of harvested seeds and inflorescences was made to obtain the broadest possible natural spectrum of diaspores (Maas, 1989); maternal effects on the dominant structure of diaspores were not taken into account (Gutterman, 1992). Immediately after harvest, we placed 50 seeds into nylon bags (4 cm × 4 cm, mesh size 300 μm). We made a borehole 10 cm deep and put three nylon bags with seeds of the same species inside the soil core in the depth of 1, 5, and 10 cm. We placed the soil core into a nylon bag (mesh size 2 mm) and inserted it back to the soil. We made five replicates for each species for each excavation time (except for Festuca ovina, Cirsium acaule, and Anthericum ramosum with two, two, and four replicates, respectively because there were not enough seeds available for these species). The position of replicates was randomized inside the site.
We performed several excavations of seeds during the next few years. The first excavation took place at the end of October in the same year as seeds were buried (except for late species whose seeds ripen during September or later). The subsequent excavation was performed during April following the burial (after winter freezing), then during October (after one whole season). The remaining replicates were excavated during April in the following years (see Table 1 for details).
We took all seeds which were not germinated or molded in the soil, treated them by 2% solution of sodium hypochlorite for 2 min, placed them in the Petri dish with filter paper and sufficient moisture, and kept them in the growing chamber (22°C/14°C at 14 h/10 h light/dark) for 6 weeks. We counted as viable those in which the radicle emerged through the seed testa. We stratified ungerminated seeds in dark conditions at a temperature of 3°C for 6 weeks and then put them again in the growing chamber with the same settings for the next 6 weeks. We tested the viability of remaining ungerminated seeds using the tetrazolium test to distinguish between viable (but dormant) and death seeds.
Seed Traits
We used the longevity index from the LEDA database (Kleyer et al., 2008; Poschlod et al., 2020; unpublished data) and information about the type of dormancy, light requirements for germination, and alternating temperature requirements from literature (Kawatani et al., 1976; Grime et al., 1981; Thompson and Grime, 1983; Jones and Turkington, 1986; Pegtel, 1988; Maas, 1989; Beier, 1991; Milberg, 1994; Poschlod et al., 2003; McDavid, 2012; ten Brink et al., 2013; Baskin and Baskin, 2014; Lang et al., 2014; Tudela-Isanta et al., 2018; Leipold et al., 2019; Lopez del Egido et al., 2019; Rosbakh et al., 2020; Holländer and Jäger, n.d.) and online databases ENSCOBASE,1 Seed Information Database2 (see Table 1 for details).
Data Analysis
We used a set of mixed-effect linear models with the proportion of viable seeds (all viable seeds regardless of dormancy) and the proportion of dormant seeds (seeds viable according to the tetrazolium test) as the dependent variable. We used species and plant families as random effects. To avoid the model overfitting, we performed individual analysis for each seed trait and used time, burial depth, one of the seed traits (longevity index, dormancy, light requirements, and alternating temperature requirements), and interaction of respective seed trait with time and burial depth as fixed effects. Furthermore, we performed a model with time, burial depth, and its interaction as fixed effects for investigation of the time × depth interaction. Explanatory variables were standardized and log-transformed to meet the assumptions of normality and homogeneity of variance and take into account the right-skewed distribution of these variables. We classified species with the morphological, morphophysiological, physiological, and physical types of dormancy together as dormant species. Mixed-effect models were performed using the lmer function in R package lme4 (Bates et al., 2015). We tested the random effects using the ranova function from the lmerTest package. We calculated R2 using Nakagawa and Schielzeth’s R2GLMM (Johnson, 2014) as implemented in the r.squaredGLMM function from the R package MuMIn. For better understanding the behavior of individual species and at the same time for not to overparametrize the model, we preferred to redo the analysis for each species individually. We fitted linear models for individual species for burial depth, time, and interaction and performed a multiway ANOVA. We used R software (R Core Team, 2021) for performing all analyses.
Results
Time and Burial Depth
The mixed-effect model indicated no very strong main effect of burial depth or time on the proportion of viable seeds. Unsurprisingly, the proportion of viable seeds decreased during the time and increased with burial depth. No significant interaction between time and burial depth was detected (Table 2 and Figure 1A).
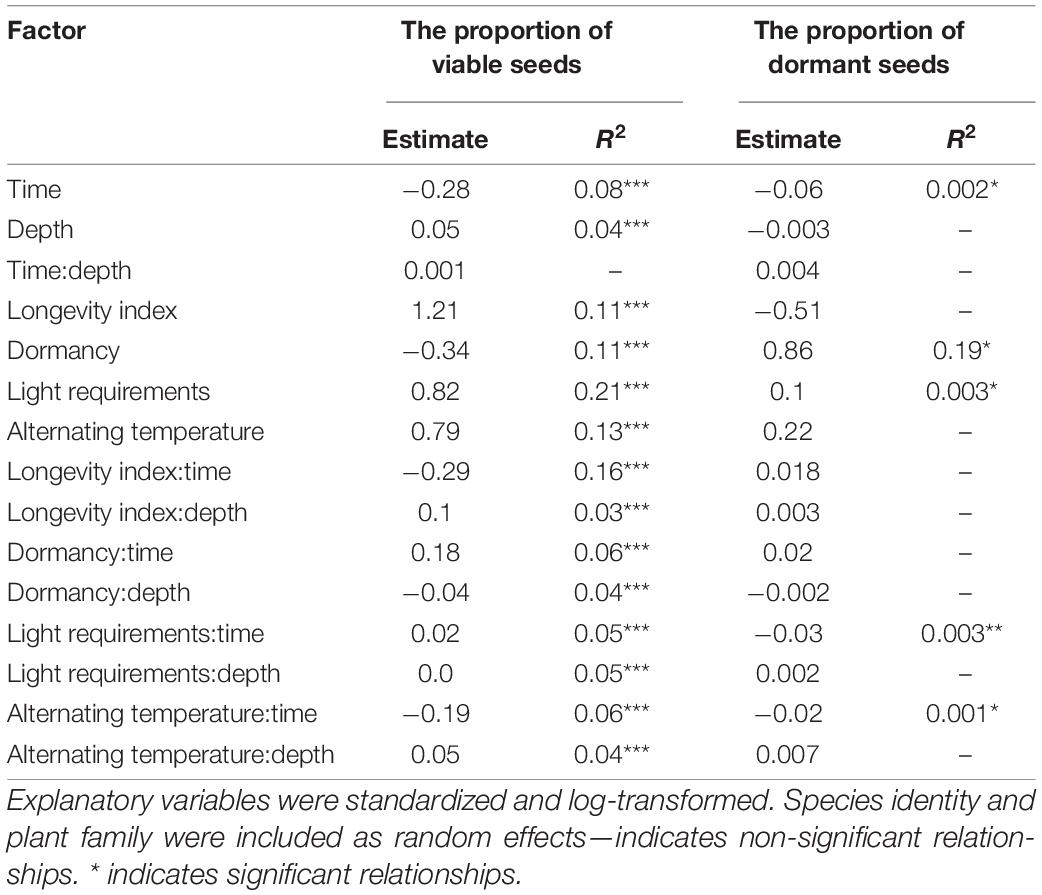
Table 2. Results of set of mixed-effect linear models with the proportion of viable seeds and proportion of dormant seeds as the dependent variable.
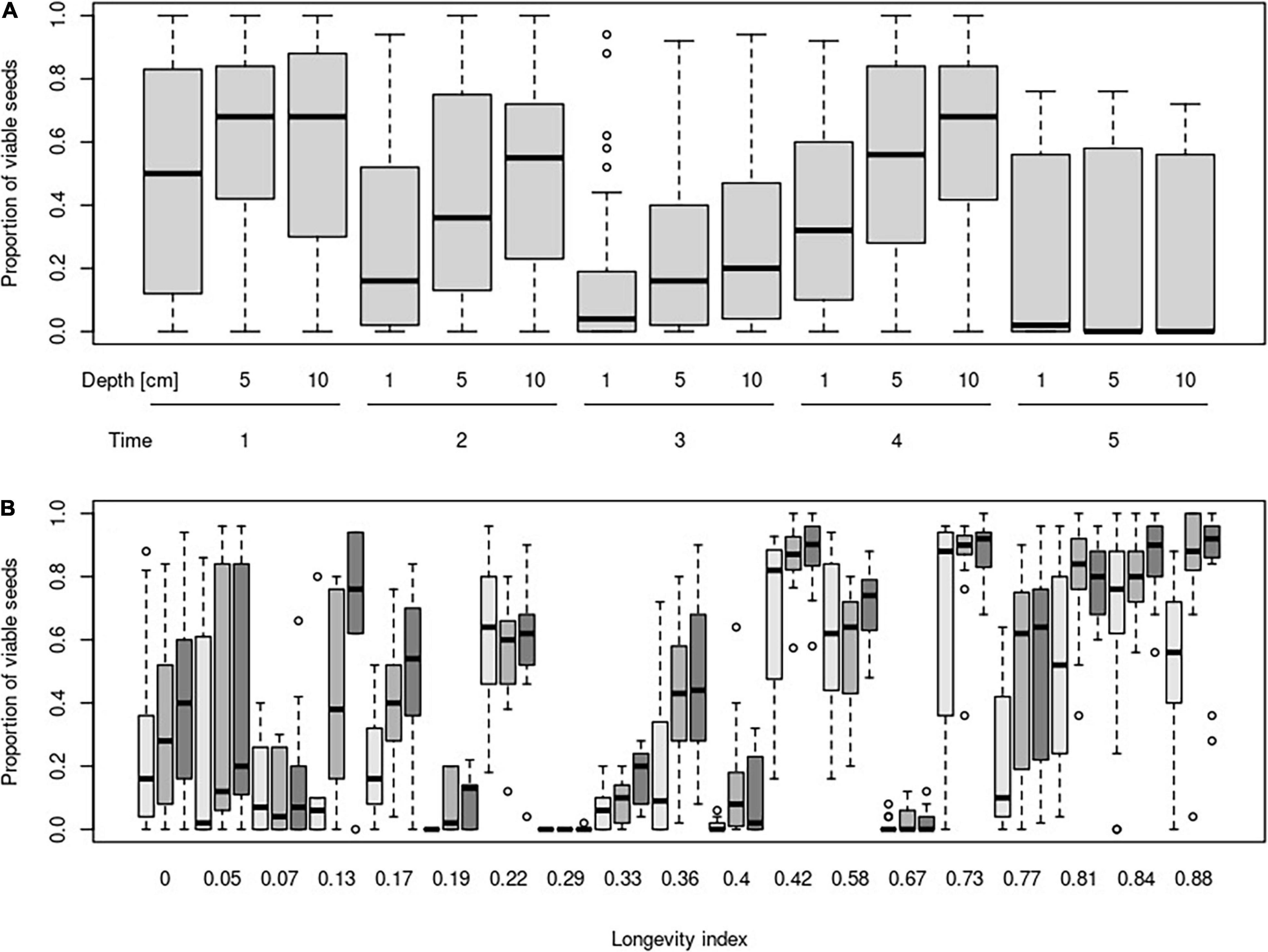
Figure 1. Proportion of viable seeds in different burial depth and their changes according to time (A) and longevity index (B). Time steps indicate: 1—October in the same year as seeds were buried; 2—April of the year following the burial; 3—October of the year following the burial; 4—April of the second year following the burial; and 5—April of the third year following the burial. Darkness of boxes indicates burial depth (light gray = 1 cm, middle gray = 5 cm, and dark gray = 10 cm).
We found only a very low negative effect of time on the proportion of dormant seeds; all other investigated factors—burial depth and interaction between time and burial depth—did not affect the proportion of dormant seeds (Table 2).
For random effects, we found a strong significant effect of species on the proportion of viable seeds (p < 0.001), indicating that the interspecific differences are the most important for the soil seed persistence. We found no effect of a family (p = 0.14) on the proportion of viable seeds. On the other hand, both random effects—species and family—affected the proportion of dormant seeds (p < 0.001, p = 0.026, respectively).
Seed Traits
The proportion of viable seeds was significantly related to all investigated seed traits—longevity index, dormancy, light requirements for germination, and alternating temperature requirements for germination and interacted with both time and burial depth (Table 2 and Figures 1B, 2 for details). The proportion of dormant seeds was significantly related to the dormancy but time and burial depth did not modify it. Furthermore, we found a significant interaction between time and light requirement for germination and time and alternating temperature requirement for germination in the case of the proportion of dormant seeds. Namely, species with some type of dormancy showed a lower proportion of viable seeds than species without dormancy. We determined the opposite pattern in the case of the proportion of dormant seeds (Figure 3A).
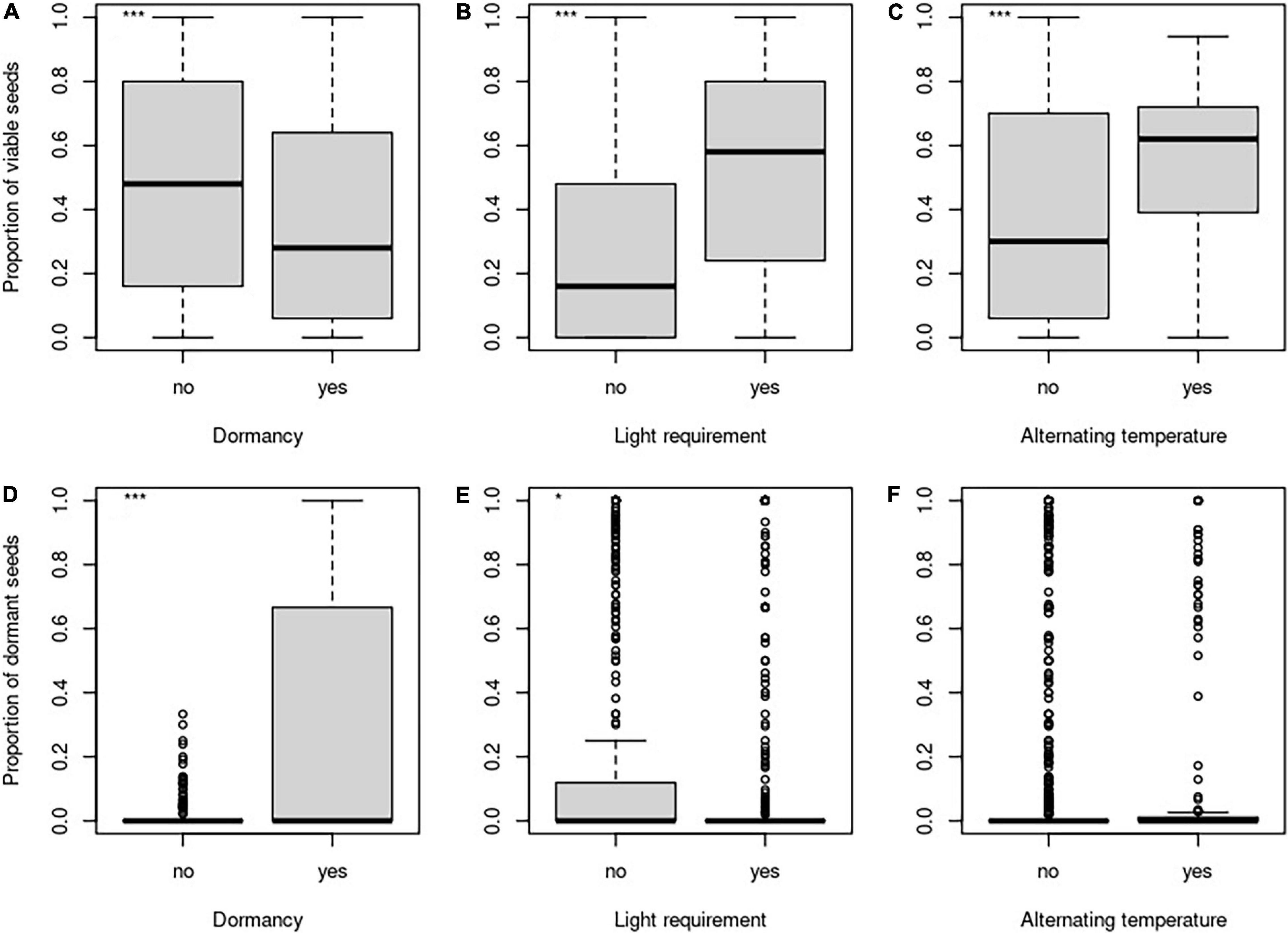
Figure 2. Differences in the proportion of viable seeds (A–C) and the proportion of dormant seeds (D–F) concerning the dormancy (A,D), light requirements for germination (B,E), and alternating temperature requirements (C,F). *Indicates significant relationship.
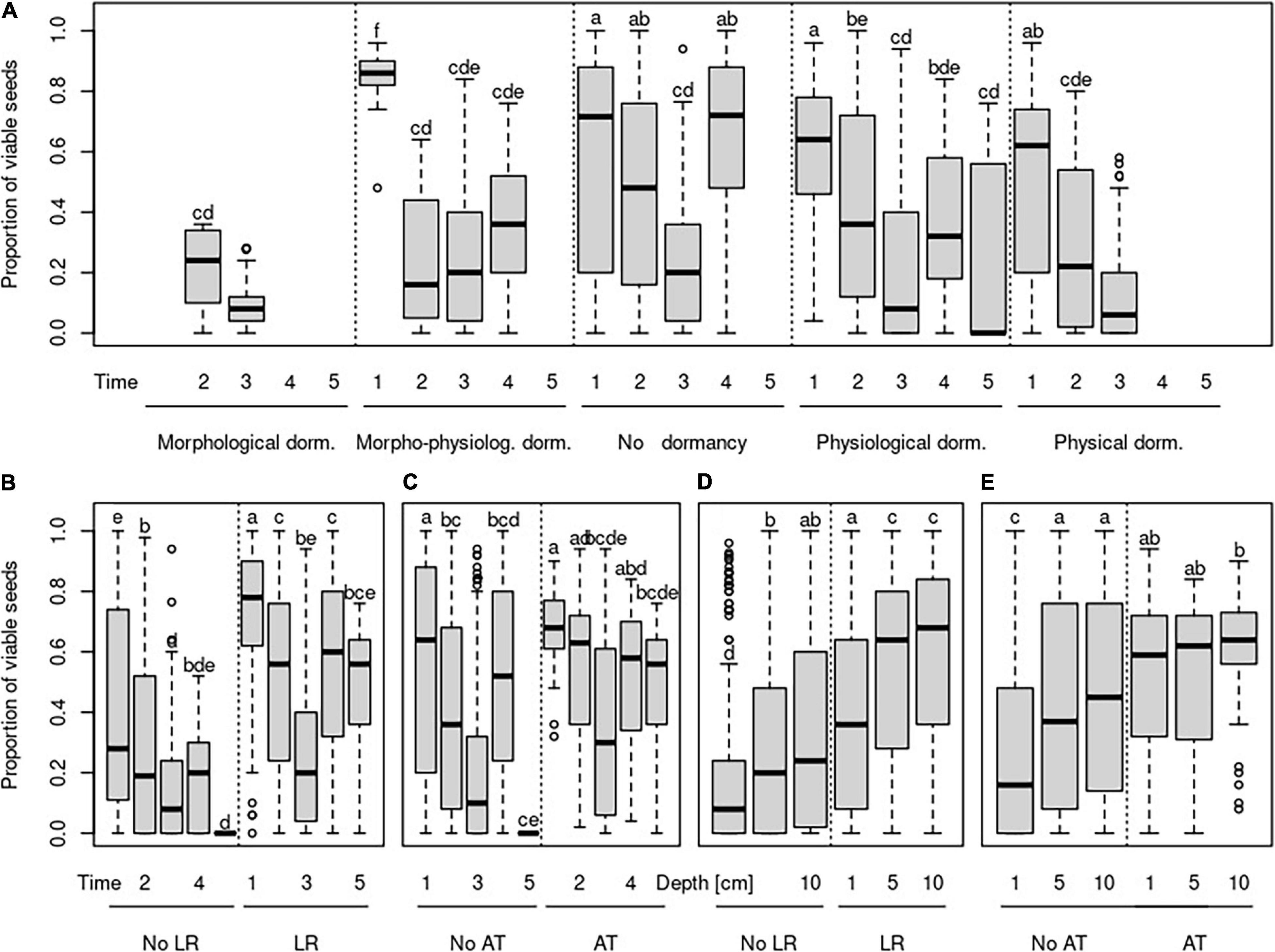
Figure 3. Changes in the proportion of viable seeds over time (A–C) and over burial depth (D,E) concerning the species-specific type of dormancy (A), species-specific germination requirements to light (B,D), and species-specific germination requirements to alternating temperature (C,E). Time steps indicate: 1—October in the same year as seeds were buried; 2—April of the year following the burial; 3—October of the year following the burial; 4—April of the second year following the burial; 5—April of the third year following the burial. No LR—species without light requirements for germination, LR—species with requirements for germination, No AT—species without alternating temperature requirements for germination, and AT—species with alternating requirements for germination.
Surprisingly, a significant negative relationship between the proportion of viable seeds and interaction between longevity index and time was detected. The relationship of the proportion of viable seeds with the interaction between longevity index and burial depth was significantly positive.
The species without the requirements to both—light and alternating temperature for germination—had a generally lower proportion of viable seeds (Figures 3B–E). Significant interactions between light requirements for germination with both time and burial depth, on the proportion of viable seeds, showed that seeds of species that require light for germination stayed viable longer in the deeper layer of soil (Figures 3B,D). We also found a significant interaction between alternating temperature requirements and time and burial depth on the proportion of viable seeds. The proportion of viable seeds increased with the burial depth for species with the requirements of alternating temperature and decreased over time for this species (Figure 3E).
Species-Specific Behavior
For most species (21 from 28), the proportion of viable seeds significantly decreased during the time (e.g., Brachypodium pinnatum). We also found the relationship between the proportion of viable seeds and the burial depth—the proportion of viable seeds decreased with the burial depth for four species (e.g., Origanum vulgare) and increased for 14 species (e.g., Gentianella germanica). There was a significant positive interaction between burial depth and time for three species (Daucus carota, Hypericum perforatum, and O. vulgare) and a significant negative interaction for one species (Bupleurum falcatum). See Table 3 and Supplementary Figure 1 for species-specific details.
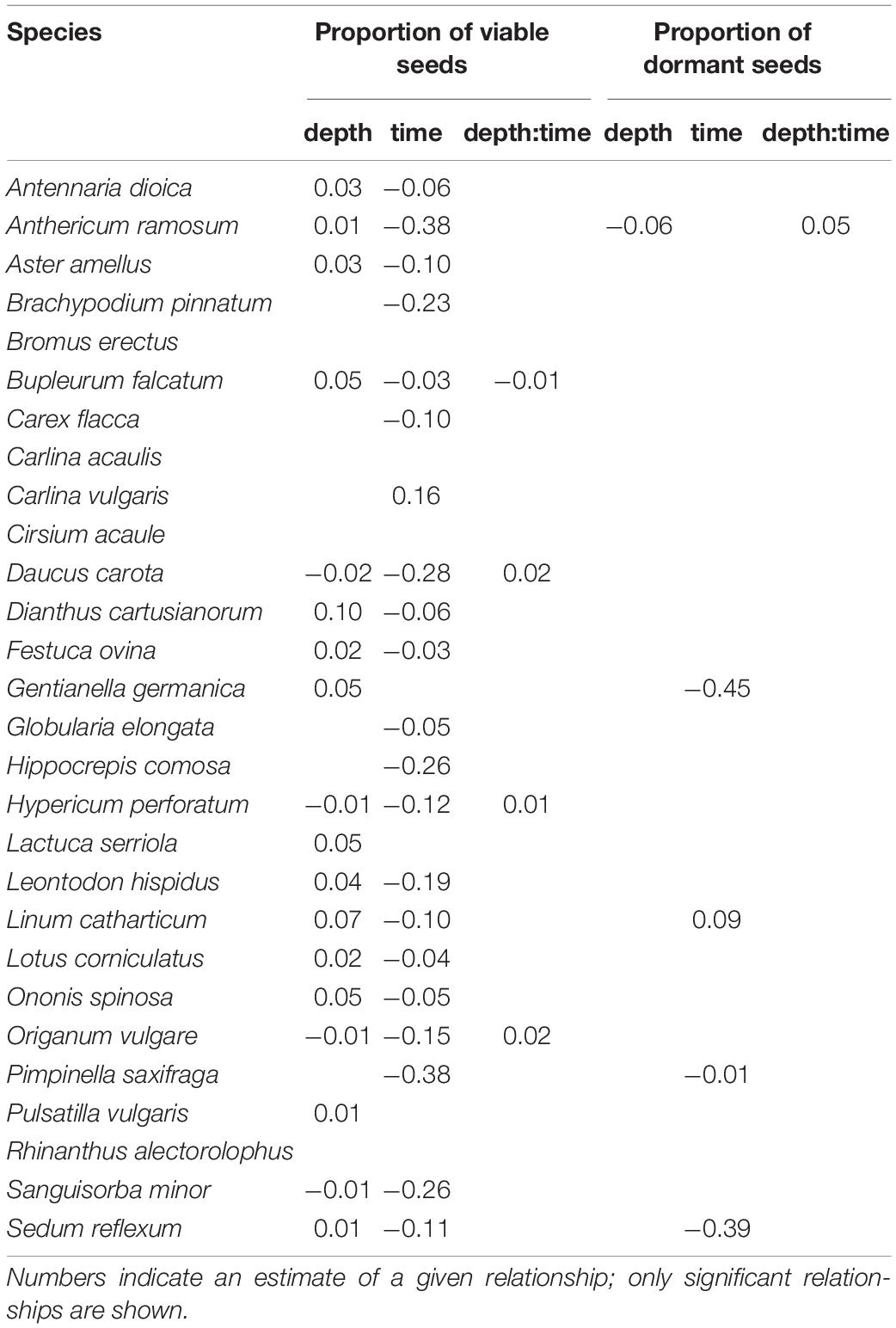
Table 3. Results of linear models for individual species between the proportion of viable seeds and proportion of dormant seeds and burial depth, time, and its interaction, performed as a multiway ANOVA.
Only for A. ramosum (the only non-legume species with physical dormancy in our dataset), we found a significant relationship between the proportion of dormant seeds and the burial depth and its significant interaction with time—the proportion of dormant seeds declined during the time in the upper layer but increased in the deeper layer. For three species (Pimpinella saxifraga, G. germanica, and Sedum reflecum), the proportion of dormant seeds significantly decreased during the time (e.g., G. germanica) and for Linum catharcticum significantly increased during the time. All these four species have the physiological or morphophysiological types of dormancy—however, another five species with the physiological type of dormancy did not show this pattern. See Table 3 and Supplementary Figure 2 for species-specific details.
Discussion
Our burial experiment with seeds of 28 species from calcareous grassland habitat demonstrates the complexity of the soil seed bank and clearly shows that results of indirect investigation of seed longevity have to be interpreted carefully. Although we found significantly better seed persistence in deeper soil layers for species with a higher longevity index, this correlation was not strong. Our findings confirm the previous investigation of Saatkamp et al. (2009) which found no relationship between soil seed persistence in the burial experiment and seed bank persistence and therefore recommended different use of soil seed abundance and experimental soil seed persistence. Moreover, site-specific conditions, such as rainfall or soil texture, affected both natural seed vertical movement (Benvenuti, 2007; Egawa and Tsuyuzaki, 2013) and soil seed persistence (Schafer and Kotanen, 2003; Long et al., 2015). Therefore, long-term direct investigation under the given environmental conditions is necessary for a precise understanding of the community dynamics.
We found substantial species-specific differences in the pattern of soil seed persistence both over time and depending on the burial depth. These differences were explained mainly on the species level for the proportion of viable seeds and the family level for the proportion of dormant seeds, which is in agreement with our knowledge of seed dormancy as the earliest trait in plant life history (Carta et al., 2016; Liu et al., 2017). Previous investigation in calcareous grassland communities showed essentially similar patterns in species-specific differences for constant burial depth (Pons, 1991). On the other hand, our results emphasized the importance of burial depth for particular species.
In our experiment, we cannot separate if seeds detected as non-viable after the given time of burial germinated in the soil before excavation or were destroyed due to pathogens attack. Mortality via fungi attack is both site-specific (Schafer and Kotanen, 2003) and species-specific (Gardarin et al., 2010). Nevertheless, the reason why seeds did not stay viable in the soil has not high importance for answering our questions about soil seed persistence. In both cases, such seeds do not play a role as seed supply in the soil and do not affect the long-term community dynamics.
As we expected, seeds of species with light requirements for germination stay viable longer in the deeper layer. Although light can penetrate only a tiny upper layer of soil (Kasperbauer and Hunt, 1988; Mandoli et al., 1990), seedlings, especially of large-seeded species, can emerge successfully from much greater depth (Bond et al., 1999). Generally, germination in light conditions is one of the mechanisms to detect the burial depth. It was shown before that light requirement is essential to keep seeds ungerminated just after entering the soil (Saatkamp et al., 2011b). Our findings of the longer persistence of seeds in deeper soil layers for species with light requirements for germination support this idea. Huge differences between individual species were found, and we can agree with Saatkamp et al. (2011a) that burial depth detection is a highly species-specific mechanism. Different species with light requirements for germination showed different patterns in soil seed persistence. For example, seeds of B. falcatum and Linum catharticum did not stay viable in the upper soil layer, which suggests that they germinate immediately after burial in light conditions. Seeds of these species stay viable during one season in deeper soil layers, and after this time, the number of viable seeds decreased to the same number as in the upper soil layer. Conditions in deeper soil layers postponed the decline of a count of viable seeds, but they did not guarantee their long-term survival.
Furthermore, we found the group of species with light requirements for germination, namely, C. acaule, D. carota, H. perforatum, O. vulgare, and Leontodon hispidus, which showed another pattern in soil seed persistence. Seeds of these species survived in a similar amount after the first winter in all burial depths. Later, the number of viable seeds decreased substantially in the upper soil layer. In contrast, at deeper burial depth, their number remained constant throughout the experiment. On the other hand, high seed persistence in the deeper soil layer throughout the experiment and at the same time, constantly low number of viable seeds in the upper soil layer was found for species G. germanica and Lactuca serriola. That indicates that detecting the burial depth is crucial for these species immediately after burial; they are not able to germinate from deeper soil layers. They can stay viable in the soil seed bank for a long time, although both are often classified as transient soil seed banks (i.e., their seeds should persist in the soil less than one year) in the literature (Pons, 1991; Kleyer et al., 2008). We found around 50% of seeds viable after two winters in the deep soil layer and around 20% of seeds viable in the upper soil layer for G. germanica. This inconsistency between classification as transient soil seed bank from literature and our finding of viable seeds after two years of burial could result from the long dormancy, so classical germination experiments cannot detect it, as reported by Fischer and Matthies (1998).
Our results of differences between species with and without alternating temperature requirements for germination match our expectations. The proportion of viable seeds changes with the burial depth and during the time for species with such requirements. This relationship was weak, and we see the main reason for the unbalanced design of our dataset. We have only three species out of 28 with alternating temperature requirements for germination, which differ in other seed traits, so it is challenging to generalize them. Alternating temperature requirements are known as the mechanism which can serve for detection of burial depth but simultaneously also for detection of disturbances (Thompson and Grime, 1983; Saatkamp et al., 2011a). Nevertheless, its role in soil seed persistence in some environments seems to be negligible (Rivera et al., 2012). Therefore, further investigation with the precise selection of species according to this seed trait and careful setup of the experiment is needed to disentangle the role of alternating temperature for soil seed persistence.
Our work showed a broad range of soil seed persistence strategies under the different burial depths. This diversity can potentially promote species coexistence by the storage effect (Chesson, 1994; Facelli et al., 2005) and thereby maintaining a species-rich community that can withstand temporal fluctuations in environmental conditions. On the other hand, it is known that current climatic changes, such as changing temperature and rainfall regimes, can accelerate the decline of seed viability (Chen et al., 2021), compromising the persistence of plant populations dependent on long-lived seed banks (Ooi, 2012) or dry habitats (Basto et al., 2018). Our results from the long-term burial experiment also proved that seeds of some species could survive in the soil much longer than expected from the indirect measurement of seed longevity by the seedling establishment from soil samples. It points out the importance of further direct long-term investigation.
Data Availability Statement
The raw data supporting the conclusions of this article will be made available by the authors, without undue reservation.
Author Contributions
PP designed the study and performed the experiments. TM analyzed the data. TM and PP interpreted results. TM wrote the text with contributions of PP. All authors approved the final version of the manuscript.
Conflict of Interest
The authors declare that the research was conducted in the absence of any commercial or financial relationships that could be construed as a potential conflict of interest.
The handling Editor declared a past co-authorship with one of the authors PP.
Publisher’s Note
All claims expressed in this article are solely those of the authors and do not necessarily represent those of their affiliated organizations, or those of the publisher, the editors and the reviewers. Any product that may be evaluated in this article, or claim that may be made by its manufacturer, is not guaranteed or endorsed by the publisher.
Acknowledgments
We want to thank Julia Hahn and Sabine Kiefer for data collection, Judith Lang for help with seed traits investigation, and Sabine Fischer and Roberta Dayrell for providing additional information.
Supplementary Material
The Supplementary Material for this article can be found online at: https://www.frontiersin.org/articles/10.3389/fpls.2021.790867/full#supplementary-material
Supplementary Figure 1 | The species-specific pattern in the proportion of viable seeds during the time and different burial depths.
Supplementary Figure 2 | The species-specific pattern in the proportion of dormant seeds during the time and different burial depths.
Footnotes
References
Baskin, C. C., and Baskin, J. M. (2014). Seeds: Ecology, Biogeography, and Evolution of Dormancy and Germination, 2nd Edn. London: Academic Press.
Basto, S., Thompson, K., Grime, J. P., Fridley, J. D., Calhim, S., Askew, A. P., et al. (2018). Severe effects of long-term drought on calcareous grassland seed banks. NPJ Clim. Atmospher. Sci. 1:1. doi: 10.1038/s41612-017-0007-3
Bates, D., Mächler, M., Bolker, B., and Walker, S. (2015). Fitting linear mixed-effects models using lme4. J. Stat. Softw 67, 1–48. doi: 10.18637/jss.v067.i01
Beier, B. (1991). Keimungsbiologische Untersuchungen an Kalkmagerrasenpflanzen und ihre Bedeutung für die Interpretation der Samenbank. Stuttgart: Universität Hohenheim.
Benvenuti, S. (2007). Natural weed seed burial: effect of soil texture, rain and seed characteristics. Seed Sci. Res. 17, 211–219. doi: 10.1017/S0960258507782752
Bhatt, A., Bhat, N. R., Lozano-Isla, F., Gallacher, D., Santo, A., Batista-Silva, W., et al. (2019). Germination asynchrony is increased by dual seed bank presence in two desert perennial halophytes. Botany 97, 639–649. doi: 10.1139/cjb-2019-0071
Bond, W. J., Honig, M., and Maze, K. E. (1999). Seed size and seedling emergence: an allometric relationship and some ecological implications. Oecologia 120, 132–136. doi: 10.1007/s004420050841
Carta, A., Hanson, S., and Müller, J. V. (2016). Plant regeneration from seeds responds to phylogenetic relatedness and local adaptation in Mediterranean Romulea (Iridaceae) species. Ecol. Evol. 6, 4166–4178. doi: 10.1002/ece3.2150
Chapman, T., Miles, S., and Trivedi, C. (2019). Capturing, protecting and restoring plant diversity in the UK: RBG Kew and the Millennium Seed Bank. Plant Divers. 41, 124–131. doi: 10.1016/j.pld.2018.06.001
Chen, D., Chen, X., Jia, C., Wang, Y., Yang, L., and Hu, X. (2021). Effects of precipitation and microorganisms on persistence of buried seeds: a case study of 11 species from the Loess Plateau of China. Plant Soil 467:3. doi: 10.1007/s11104-021-04990-1
Chesson, P. (1994). Multispecies competition in variable environments. Theor. Popul. Biol. 45, 227–276. doi: 10.1006/tpbi.1994.1013
Chesson, P., and Warner, R. R. (1981). Environmental variability promotes coexistence in lottery competitive systems. Am. Nat. 117, 923–943. doi: 10.1111/ele.12672
Egawa, C., and Tsuyuzaki, S. (2013). The effects of litter accumulation through succession on seed bank formation for small- and large-seeded species. J. Veg. Sci. 24, 1062–1073. doi: 10.1111/jvs.12037
El-Keblawy, A., Shabana, H. A., and Navarro, T. (2018). Seed mass and germination traits relationships among different plant growth forms with aerial seed bank in the sub-tropical arid Arabian deserts. Plant Ecol. Divers. 11, 393–404. doi: 10.1080/17550874.2018.1496365
Facelli, J. M., Chesson, P., and Barnes, N. (2005). Differences in seed biology of annual plants in arid lands: a key ingredient of the storage effect. Ecology 86, 2998–3006. doi: 10.1890/05-0304
Fischer, M., and Matthies, D. (1998). Experimental demography of the rare Gentianella germanica: seed bank formation and microsite effects on seedling establishment. Ecography 21, 269–278. doi: 10.1111/j.1600-0587.1998.tb00564.x
Funk, J. L. (2021). Revising the trait-based filtering framework to include interacting filters: lessons from grassland restoration. J. Ecol. 109, 3466–3472. doi: 10.1111/1365-2745.13763
Gardarin, A., Dürr, C., Mannino, M. R., Busser, H., and Colbach, N. (2010). Seed mortality in the soil is related to seed coat thickness. Seed Sci. Res. 20, 243–256. doi: 10.1017/S0960258510000255
Grime, J. P., Mason, G., Curtis, A. V., Rodman, J., and Band, S. R. (1981). A comparative study of germination characteristics in a local flora. J. Ecol. 69:1017. doi: 10.2307/2259651
Grubb, P. J. (1977). The maintenance of species-richness in plant communities: the importance of the regeneration niche. Biol. Rev. 52, 107–145. doi: 10.1111/j.1469-185X.1977.tb01347.x
Grundy, A. C., Mead, A., and Burston, S. (2003). Modelling the emergence response of weed seeds to burial depth: interactions with seed density, weight and shape. J. Appl. Ecol. 40, 757–770.
Gutterman, Y. (1992). “Maternal effects on seeds during development,” in Seeds. The Ecology of Regeneration in Plant Communities, ed. M. Fenner (Wallingford: CAB International), 27–59.
Holländer, K., and Jäger, E. J. (n.d.). Wuchsform und Lebensgeschichte von Globularia bisnagarica L. (G. punctata LAPEYR., Globulariaceae). Hercynia 31, 143–171.
Johnson, P. C. D. (2014). Extension of Nakagawa & Schielzeth’s R 2 GLMM to random slopes models. Methods Ecol. Evol. 5, 944–946. doi: 10.1111/2041-210X.12225
Jones, D. A., and Turkington, R. (1986). Lotus Corniculatus L. J. Ecol. 74:1185. doi: 10.2307/2260243
Kasperbauer, M. J., and Hunt, P. G. (1988). Biological and photometric measurement of light transmission through soils of various colors. Bot. Gaz. 149, 361–364. doi: 10.1086/337726
Kawatani, T., Kaneki, Y., and Momonoki, Y. (1976). Studies on the germination of seeds of Bupleurum falcatum L.: I. influence of the time elapse after seed harvest and light conditions on the germination. Proc. Crop Sci. Soc. JPN. 45, 243–247. doi: 10.1626/jcs.45.243
Kleyer, M., Bekker, R. M., Knevel, I. C., Bakker, J. P., Thompson, K., Sonnenschein, M., et al. (2008). The LEDA traitbase: a database of life-history traits of the Northwest European flora. J. Ecol. 96, 1266–1274. doi: 10.1111/j.1365-2745.2008.01430.x
Lang, J., Listl, D., Glaab, P., Reisch, C., and Poschlod, P. (2014). “Qualität und Keimungseigenschaften von Saatgut in der Genbank,” in Handbuch Genbank WEL. Hoppea. Denkschriften der Regensburgischen Botanischen Gesellschaft, ed. D. O. Mills (Sonderband: BHL Consortium), 99–132.
Leipold, M., Tausch, S., Reisch, C., and Poschlod, P. (2019). Genbank für Wildpflanzen-Saatgut – Bayern Arche zum Erhalt der Floristischen Artenvielfalt. UmweltSpezial Augsbg. Augsburg: Bayerisches Landesamt für Umwelt.
Liu, Y., Barot, S., El-Kassaby, Y. A., and Loeuille, N. (2017). Impact of temperature shifts on the joint evolution of seed dormancy and size. Ecol. Evol. 7, 26–37. doi: 10.1002/ece3.2611
Long, R. L., Gorecki, M. J., Renton, M., Scott, J. K., Colville, L., Goggin, D. E., et al. (2015). The ecophysiology of seed persistence: a mechanistic view of the journey to germination or demise: The ecophysiology of seed persistence. Biol. Rev. 90, 31–59. doi: 10.1111/brv.12095
Lopez del Egido, L., Toorop, P. E., and Lanfermeijer, F. C. (2019). Seed enhancing treatments: comparative analysis of germination characteristics of 23 key herbaceous species used in European restoration programmes. Plant Biol. 21, 398–408. doi: 10.1111/plb.12937
Maas, D. (1989). Germination characteristics of some plant species from calcareous fens in Southern Germany and their implications for the seed bank. Holarct. Ecol. 12, 337–344.
Mandoli, D. F., Ford, G. A., Waldron, L. J., Nemson, J. A., and Briggs, W. R. (1990). Some spectral properties of several soil types: implications for photomorphogenesis*. Plant Cell Environ. 13, 287–294. doi: 10.1111/j.1365-3040.1990.tb01313.x
McDavid, K. L. (2012). Utilizing Sedum Seeds as an Installation Method for Green Roofs through Seed Enhancement Techniques. University Park, PA: The Pennsylvania State University.
Milberg, P. (1994). Germination ecology of the grassland biennial Linum catharticum. Acta Bot. Neerland. 43, 261–269. doi: 10.1111/j.1438-8677.1994.tb00751.x
Milberg, P., Andersson, L., and Thompson, K. (2000). Large-seeded spices are less dependent on light for germination than small-seeded ones. Seed Sci. Res. 10, 99–104. doi: 10.1017/S0960258500000118
Moravcová, L., Pyšek, P., Krinke, L., Müllerová, J., Perglová, I., and Pergl, J. (2018). Long-term survival in soil of seed of the invasive herbaceous plant Heracleum mantegazzianum. Preslia 90, 225–234. doi: 10.23855/preslia.2018.225
Ooi, M. K. J. (2012). Seed bank persistence and climate change. Seed Sci. Res. 22, S53–S60. doi: 10.1017/S0960258511000407
Pearson, T. R. H., Burslem, D. F. R. P., Mullins, C. E., and Dalling, J. W. (2002). Germination ecology of neotropical pioneers: interacting effects of environmental conditions and seed size. Ecology 83, 2798–2807. doi: 10.1890/0012-9658(2002)083[2798:geonpi]2.0.co;2
Pegtel, D. M. (1988). Germination in declining and common herbaceous plant populations co-occurring in an acid peaty heathland. Acta Bot. Neerland. 37, 215–223. doi: 10.1111/j.1438-8677.1988.tb02130.x
Pons, T. L. (1991). Dormancy, germination and mortality of seeds in a chalk-grassland flora. J. Ecol. 79, 765. doi: 10.2307/2260666
Poschlod, P., Glick, F., Krickl, P., and Sattler, J. (2020). Diasporenbanken in Böden – Kenntnisstand und Forschungslücken. Germany: Universität Osnabrück.
Poschlod, P., and Jackel, A.-K. (1993). Untersuchungen zur Dynamik von generativen Diasporenbanken von Samenpflanzen in Kalkmagerrasen. Flora 188, 49–71. doi: 10.1016/S0367-2530(17)32247-8
Poschlod, P., Kleyer, M., Jackel, A.-K., Dannemann, A., and Tackenberg, O. (2003). BIOPOP: A database of plant traits and internet application for nature conservation. Folia Geobot. 38, 263–271.
R Core Team (2021). A Language and Environment for Statistical Computing. R Foundation for Statistical Computing. Vienna: R Core Team.
Richter, R., and Stromberg, J. C. (2005). Soil seed banks of two montane riparian areas: implications for restoration. Biodivers. Conserv. 14, 993–1016. doi: 10.1007/s10531-004-7844-1
Rivera, D., Jáuregui, B. M., and Peco, B. (2012). The fate of herbaceous seeds during topsoil stockpiling: restoration potential of seed banks. Eco. Eng. 44, 94–101. doi: 10.1016/j.ecoleng.2012.03.005
Rosbakh, S., Baskin, C. C., and Baskin, J. M. (2020). Nikolaeva et al.’s reference book on seed dormancy and germination. Ecology 101: e03049. doi: 10.1002/ecy.3049
Saatkamp, A., Affre, L., Baumberger, T., Dumas, P., Gasmi, A., Gachet, S., et al. (2011a). Soil depth detection by seeds and diurnally fluctuating temperatures: different dynamics in 10 annual plants. Plant Soil 349, 331–340.1.
Saatkamp, A., Affre, L., Dutoit, T., and Poschlod, P. (2009). The seed bank longevity index revisited: limited reliability evident from a burial experiment and database analyses. Ann. Bot. 104, 715–724. doi: 10.1093/aob/mcp148
Saatkamp, A., Affre, L., Dutoit, T., and Poschlod, P. (2011b). Germination traits explain soil seed persistence across species: the case of Mediterranean annual plants in cereal fields. Ann. Bot. 107, 415–426. doi: 10.1093/aob/mcq255
Schafer, M., and Kotanen, P. M. (2003). The influence of soil moisture on losses of buried seeds to fungi. Acta Oecol. 24, 255–263. doi: 10.1016/j.actao.2003.09.001
Schwienbacher, E., Marcante, S., and Erschbamer, B. (2010). Alpine species seed longevity in the soil in relation to seed size and shape – A 5-year burial experiment in the Central Alps. Flora Morphol. Distrib. Funct. Ecol. Plants 205, 19–25. doi: 10.1016/j.flora.2008.10.007
Tatár, S. (2010). Seed longevity and germination characteristics of six fen plant species. Acta Biol. Hung. 61, 197–205. doi: 10.1556/ABiol.61.2010.Suppl.19
ten Brink, D.-J., Hendriksma, H. P., and Bruun, H. H. (2013). Habitat specialization through germination cueing: a comparative study of herbs from forests and open habitats. Ann. Bot 111, 283–292. doi: 10.1093/aob/mcs253
Thompson, K. (1993). “Seed persistence,” in Methods in Comparative Plant Ecology, eds G. A. F. Hendry and J. P. Grime (London: Chapman & Hall), 199–202.
Thompson, K., Bakker, J. P., and Bekker, R. M. (1997). Soil Seed Banks of North-West Europe Methodology, Density and Longevity. Cambridge: Cambridge University Press.
Thompson, K., Bakker, J. P., Bekker, R. M., and Hodgson, J. G. (1998). Ecological correlates of seed persistence in soil in the north-west European flora. J. Ecol. 86, 163–169. doi: 10.1046/j.1365-2745.1998.00240.x
Thompson, K., and Grime, J. P. (1983). A comparative study of germination responses to diurnally-fluctuating temperatures. J. Appl. Ecol. 20:141. doi: 10.2307/2403382
Tudela-Isanta, M., Fernández-Pascual, E., Wijayasinghe, M., Orsenigo, S., Rossi, G., Pritchard, H. W., et al. (2018). Habitat-related seed germination traits in alpine habitats. Ecol. Evol 8, 150–161. doi: 10.1002/ece3.3539
Venable, D. L., and Brown, J. S. (1988). The selective interactions of dispersal, dormancy and seed size as adaptations for reducing risks in variable environments. Am. Nat. 131, 306–384.
Warner, R. R., and Chesson, P. L. (1985). Coexistence mediated by recruitment fluctuations: a field guide to the storage effect. Am. Nat. 125, 769–787. doi: 10.1086/284379
Keywords: calcareous grasslands, dormancy, longevity index, soil seed bank, light requirements for germination, alternating temperature requirements for germination, burial depth
Citation: Mašková T and Poschlod P (2022) Soil Seed Bank Persistence Across Time and Burial Depth in Calcareous Grassland Habitats. Front. Plant Sci. 12:790867. doi: 10.3389/fpls.2021.790867
Received: 07 October 2021; Accepted: 13 December 2021;
Published: 04 February 2022.
Edited by:
Péter Török, University of Debrecen, HungaryReviewed by:
Judit Sonkoly, University of Debrecen, HungaryJennifer Lesley Silcock, The University of Queensland, Australia
Arne Saatkamp, Aix Marseille Université, France
Copyright © 2022 Mašková and Poschlod. This is an open-access article distributed under the terms of the Creative Commons Attribution License (CC BY). The use, distribution or reproduction in other forums is permitted, provided the original author(s) and the copyright owner(s) are credited and that the original publication in this journal is cited, in accordance with accepted academic practice. No use, distribution or reproduction is permitted which does not comply with these terms.
*Correspondence: Tereza Mašková, VGVyZXphLk1hc2tvdmFAYmlvbG9naWUudW5pLXJlZ2Vuc2J1cmcuZGU=