- 1Department of Plant Sciences, University of Cambridge, Cambridge, United Kingdom
- 2Institute of Integrative Plant Biology, School of Life Science, Jiangsu Normal University, Xuzhou, China
Extracellular ATP (eATP) is now held to be a constitutive damage-associated molecular pattern (DAMP) that is released by wounding, herbivory or pathogen attack. The concentration of eATP must be tightly regulated as either depletion or overload leads to cell death. In Arabidopsis thaliana, sensing of eATP is by two plasma membrane legume-like lectin serine–threonine receptor kinases (P2K1 and P2K2), although other receptors are postulated. The transcriptional response to eATP is dominated by wound- and defense-response genes. Wounding and pathogen attack can involve the cyclic nucleotides cyclic AMP (cAMP) and cyclic GMP (cGMP) which, in common with eATP, can increase cytosolic-free Ca2+ as a second messenger. This perspective on DAMP signaling by eATP considers the possibility that the eATP pathway involves production of cyclic nucleotides to promote opening of cyclic nucleotide-gated channels and so elevates cytosolic-free Ca2+. In silico analysis of P2K1 and P2K2 reveals putative adenylyl and guanylyl kinase sequences that are the hallmarks of “moonlighting” receptors capable of cAMP and cGMP production. Further, an Arabidopsis loss of function cngc mutant was found to have an impaired increase in cytosolic-free Ca2+ in response to eATP. A link between eATP, cyclic nucleotides, and Ca2+ signaling therefore appears credible.
Introduction
Extracellular ATP (eATP) is now recognized as a plant cell regulator, with the ability to affect growth, development, and stress responses (reviewed by Matthus et al., 2019a). With resting levels thought to be in the nanomolar range, eATP concentration must be tightly regulated as either depletion or overload leads to cell death (Chivasa et al., 2005; Sun et al., 2012; Deng et al., 2015). This extracellular nucleotide is now thought to be involved in the response to wounding and microbial attack, such that it is classified as a constitutive damage-associated molecular pattern (DAMP; Choi et al., 2014b; Tanaka and Heil, 2021). It is readily envisaged that a breach of the plasma membrane would allow release of cytosolic ATP to the apoplast. Increased eATP concentration has indeed been measured from Arabidopsis thaliana roots, plus Arabidopsis and kidney bean (Phaseolus vulgaris L) leaves in response to mechanical wounding (Song et al., 2006; Weerasinghe et al., 2009; Dark et al., 2011; Wang et al., 2019a). Wounding can also result in accumulation of extracellular glutamate, which in turn can cause eATP accumulation (Dark et al., 2011; Toyota et al., 2018). Non-damaging mechanical disturbance generated by touch can lead to increased eATP of roots (Weerasinghe et al., 2009), suggesting that microbial growth that does not breach the plasma membrane could cause eATP increase by putting pressure on walls. If the plasma membrane were to remain intact, studies suggest that ATP release mechanisms include exocytosis and a diversity of transport proteins (Kim et al., 2006; Choi et al., 2014b; Matthus et al., 2019a).
Microbial presence has indeed been found to influence eATP levels, which in turn may affect colonization. The early phase of root colonization of both Arabidopsis and barley (Hordeum vulgare) by the endophytic fungus Serendipita indica is associated with significant eATP increase (Nizam et al., 2019). However, secretion of an eATP hydrolyzing ecto-5'-nucleotidase by the fungus lowers eATP and allows greater colonization (Nizam et al., 2019). Arabidopsis roots expressing the S. indica nucleotidase became more susceptible to colonization by the pathogenic fungus Colletotrichum incanum, suggestive of a role for eATP depletion (Nizam et al., 2019). The presence of Pseudomonas syringae (and to a lesser extent flg22) results in increased eATP levels in Arabidopsis leaf, particularly around guard cells. The high eATP causes stomatal closure, limiting bacterial entry (Chen et al., 2017). The mycotoxin beauvericin (BEA) increases eATP of wheat (Triticum aestivum) coleoptiles and causes cell death (Šrobárova et al., 2009). BEA is most notably produced by Fusarium species and acts in wheat head blight (Šrobárova et al., 2009). As a Ca2+ ionophore (Caloni et al., 2020), BEA could act to increase Ca2+ influx into cells. In Arabidopsis roots, promoting Ca2+ influx results in increased eATP most likely by stimulating exocytotic ATP release (Kim et al., 2006), which may help explain BEA’s ability to elevate wheat eATP. In contrast, the mycotoxin Fumonisin B1 (FB1) causes eATP depletion in Arabidopsis leading to cell death (Chivasa et al., 2005). Delineating how cells sense and respond to varying eATP levels is therefore critical to understanding the role of eATP in regulating what may be a continuum between growth, immunity, and death (Choi et al., 2014b).
The first angiosperm eATP receptor was identified through an Arabidopsis forward genetic screen as a plasma membrane legume-like lectin serine–threonine receptor kinase, “DOes not Respond to Nucleotides1” (DORN1), now also known as P2K1 (consistent with animal eATP receptor terminology) and formerly known as LecRK1.9 (Gouget et al., 2006; Bouwmeester et al., 2011; Choi et al., 2014a; Balagué et al., 2017). It is thought to form part of the plasma membrane–cell wall continuum (Bouwmeester et al., 2011). Structurally, DORN1/P2K1 differs markedly from the animal eATP receptors, which are eATP-binding Ca2+ channels or G-protein-coupled receptors (Choi et al., 2014a,b; Nguyen et al., 2016; Verkhratsky, 2021). DORN1/P2K1 is important for defense against Botrytis cinerea (necrotrophic fungus: Tripathi et al., 2018), Phytophthora brassicae and Phytophthora infestans (biotrophic oomycetes: Gouget et al., 2006; Bouwmeester et al., 2011, 2014), P. syringae (hemibiotrophic bacterium: Balagué et al., 2017; Chen et al., 2017), Rhizoctonia solani (necrotrophic fungus: Kumar et al., 2020) and S. indica (Nizam et al., 2019). A second Arabidopsis eATP receptor, P2K2, was identified by complementation of a p2k1 mutant. P2K2 is also a plasma membrane legume-like lectin receptor kinase which is trans-phosphorylated by P2K1 and is involved in defense against P. syringae (Pham et al., 2020). There is now evidence that DORN1/P2K1 and P2K2 are unlikely to be the only Arabidopsis eATP receptors (Zhu et al., 2017, 2020; Matthus et al., 2019a; Smith et al., 2021). Nevertheless, activation of DORN1/P2K1 by eATP leads to phosphorylation of mitogen-activated protein kinase 3 (MPK3; Choi et al., 2014a). MPK3 can phosphorylate calmodulin-binding transcription activator 3 (CAMTA3) in flg22 signaling, which results in CAMTA3 breakdown and the release of its repression of defense gene transcription (Jiang et al., 2020). It is not clear whether this also occurs in eATP signaling, but an estimated 99.8% of seedling eATP-responsive transcriptome requires DORN1/P2K1 and the CAM-box CAMTA3-binding target is highly enriched in promoters of eATP-regulated genes (Jewell et al., 2019). The eATP transcriptome is enriched in wound response and defense-related genes, with some also requiring regulation by the ethylene, jasmonate, and salicylic acid pathways (Choi et al., 2014a; Tripathi et al., 2018; Jewell et al., 2019).
While receptor characterization and analyses of receptor mutants have established eATP as a DAMP, the signaling systems downstream of receptors remain less well understood. A transient increase in cytosolic-free Ca2+ ([Ca2+]cyt) as a second messenger is one of the earliest detectable changes downstream of eATP (Demidchik et al., 2003; Choi et al., 2014a; Behera et al., 2018). Mechanical wounding and insect feeding also increase [Ca2+]cyt (Hander et al., 2019; Malabarba et al., 2021), suggesting the involvement of eATP. It can be noted in passing that saliva from insect herbivores can contain eATP hydrolyzing enzymes such as ecto-apyrase, suggesting that eATP is an important target to control (Su et al., 2012; Wu et al., 2012). Studies on how eATP increases [Ca2+]cyt in Arabidopsis have focused on plasma membrane Ca2+-permeable channels. Pharmacological block of such channels prevents [Ca2+]cyt elevation (Demidchik et al., 2003; Behera et al., 2018) and adversely affects transcription of jasmonate-dependent genes involved in wounding and defense (Tripathi et al., 2018). Patch clamp electrophysiological analyses of root epidermis have revealed eATP- and DORN1/P2K1-dependent Ca2+ influx channel conductances that would be competent to elevate [Ca2+]cyt (Demidchik et al., 2009; Zhu et al., 2017; Wang et al., 2018, 2019b). eATP-activated Ca2+ influx conductances have also been found in tobacco pollen tube and Vicia faba guard cell plasma membranes (Wang et al., 2014; Wu et al., 2018). The identity of the underpinning Ca2+ channels in all of these cell types remains under investigation. To date, the plasma membrane NADPH oxidase RBOHC (respiratory burst oxidase homologue C; Demidchik et al., 2009) and the heterotrimeric G protein alpha subunit (GPA1; Zhu et al., 2017) are implicated in regulating Arabidopsis root eATP-activated channels. Annexin1 (which supports a hydroxyl radical-activated Ca2+ channel activity; Laohavisit et al., 2012) is implicated in eATP-induced [Ca2+]cyt increase of Arabidopsis roots and transcriptional regulation of wound- and defense-response genes, but its mode of action has not been determined (Mohammad-Sidik et al., 2021). It also underpins wounding- and herbivory-induced [Ca2+]cyt increases (Malabarba et al., 2021). Annexin4 supports an eATP-induced [Ca2+]cyt increase when expressed in Xenopus oocytes, but its in planta activity has not yet been reported (Ma et al., 2019). Recently, a patch clamp study on Arabidopsis pollen grains identified two cyclic nucleotide-gated channel (CNGC) subunits (CNGC2 and CNGC4) as underpinning a plasma membrane eATP-activated Ca2+ influx conductance that may be relevant to germination (Wu et al., 2021). The conductance was lost in the dorn1 loss of function mutants suggesting the channels act downstream of this receptor although it was not checked whether CNGC2 and CNGC4 expression were downregulated in dorn1. The CNGC2 and CNGC4 channel subunits form a heterotetrameric plasma membrane Ca2+ influx channel in flg22 pathogen-associated molecular pattern-triggered immunity (PAMP; Tian et al., 2019), indicating a possible common point in DAMP and PAMP signaling. Furthermore, the results of Wu et al. (2021) imply that the cyclic nucleotides 3',5'-cyclic adenosine monophosphate (cAMP) or 3',5'-cyclic guanosine monophosphate (cGMP) act downstream of eATP and DORN/P2K1. Although eATP has long been known to increase animal cell cAMP (Choi and Kim, 1997; Castro et al., 1998), there appear to be no reports to date of eATP’s having an effect on cAMP or cGMP levels in plants, but these nucleotides can increase [Ca2+]cyt through CNGC activation (reviewed by Jarratt-Barnham et al., 2021). This perspective will consider the possibility that cyclic nucleotides and CNGCs act in eATP-DAMP [Ca2+]cyt signaling.
Cyclic Nucleotide Production by Moonlighting Proteins in Response to Pathogens and Wounding
cAMP and cGMP are involved in growth and development, abiotic stress responses, stomatal kinetics, control of photorespiration and photosynthesis, and immunity (reviewed by Jarratt-Barnham et al., 2021). Production of cAMP and cGMP is now held to be by adenylyl cyclase (AC) and guanylyl cyclase (GC) centers embedded in a diverse range of soluble and membrane-integral proteins (Ludidi and Gehring, 2003; Ruzvidzo et al., 2019; Al-Younis et al., 2021; Turek and Irving, 2021). There is clear evidence supporting cytosolic cyclic nucleotide production by a “moonlighting” function of members of the receptor-like kinase (RLK) superfamily, relevant to response to pathogens, where the catalytic center is present in the cytosolic kinase domain (Qi et al., 2010; Kwezi et al., 2011; Turek and Irving, 2021). For example in Arabidopsis, LLRAC1 (leucine-rich repeat adenylyl cyclase 1) is implicated in defense against P. syringe and the biotrophic fungus Golovinomyces orontii (Bianchet et al., 2019). Wall-associated kinase-like (WAKL) proteins in Arabidopsis, wheat and Brassica napus are implicated in cGMP production and pathogen defense (reviewed by Turek and Irving, 2021). Most recently, expression of rice WAKL21.2 (which has GC activity) in Arabidopsis resulted in transcription of SA-related defense genes and conferred resistance to P. syringae (Malukani et al., 2020).
In terms of DAMP signaling, wounding was found to result in a rapid, fivefold increase in Arabidopsis leaf cAMP and cGMP by van Damme et al. (2014). In this instance, the 2',5' isomers were detected but the mode of production and downstream consequences remain unknown. Larval oral secretions from the moth Spodoptera littoralis can increase cAMP content of Arabidopsis leaves within 3 min, which may relate to herbivory damage (Kumar et al., 2020). An initial decreased transcription followed by longer-term increased transcription of Hippeastrum hybridum Guanylyl Cyclase 1 (HpGC1, most likely encoding a soluble enzyme) was found in wounded and infected scales (fungal infection by Peyronellaea curtisii). Although a causal relationship was not demonstrated, HpGC1 activity may relate to downstream production of cGMP in the challenged scales (Świeżawska et al., 2015). Since then, triphosphate tunnel metalloenzyme 3 (TTM3) from Brachypodium distachyon has been found to have both ATP hydrolyzing and AC activities, with upregulation of transcription by wounding (Świeżawska et al., 2019). There remains a significant knowledge gap when it comes to whether eATP as a DAMP (or in its role as growth regulator) has an effect on cAMP or cGMP levels. However, increasing cAMP through expression of an AC increases transcription of wound-related genes (Xu et al., 2021) which could place eATP (as a DAMP governing wound-related transcription) upstream of cAMP production. A key question is whether the eATP receptors could themselves generate cAMP or cGMP.
Could eATP Receptors Generate cAMP or cGMP?
To date neither DORN1/P2K1 nor P2K2 has been identified as harboring AC/GC domains. However, the ACPred AC prediction tool sequence [KSR]X[DE]X{10}[KR]X{0,3}[DE] (Xu et al., 2018b; http://gcpred/acpred/; Figure 1A) does reveal possible sequences. Two overlapping sequences are present in DORN1/P2K1 (Figure 1B) that harbor an aspartic acid residue ([D]) at position 3 that should confer ATP-binding specificity, but the sequences are extracellular, lying close to the transmembrane domain and beyond the residues already implicated in eATP binding in the lectin domain (Nguyen et al., 2016; Cho et al., 2017; Figure 1C). The first sequence starting at S259 has an aspartic acid residue predicted for cation binding that should follow the 14 amino acid catalytic center motif (Al-Younis et al., 2021) but after an interval of 2 amino acids. The second sequence (starting at R267) lacks a cation-binding residue but overlaps at position 4 with the AC catalytic center motif used recently by Al-Younis et al. (2021; [KSR][YFW][DE][VIL]X{4}[Y]X{4}[KR]X{0,3}[DE]; Figure 1A). Curiously, this second sequence contains the PHPR found previously to be an RGD-binding sequence, relevant to the RGD-containing IPI-O protein secreted by P. infestans (Gouget et al., 2006). Potentially, therefore, DORN1/P2K1 has more than one way to bind eATP. P2K2 harbors a putative AC sequence in its cytosolic serine–threonine kinase domain and this sequence conserves an aspartic acid residue for cation binding following the catalytic center motif (Figure 1D). The GCPred GC prediction tool sequence [KS]X[SCG]X{10}[KR]X{0,3}[DHSE] (Xu et al., 2018a; http://gcpred; Figure 1A) revealed two intracellular sequences in DORN1/P2K1, with one harbored in its serine–threonine kinase domain (Figure 1C). For P2K2, two sequences were found in the extracellular lectin domain and one in the intracellular serine–threonine kinase domain (Figure 1D). All of these have the conserved [KS]X[SCG]X{10}[KR] sequence that confers GC function (Wong and Gehring, 2013) and is present in the Pharbitis nil GC1 enzyme which has been shown to catalyze cGMP production in vitro (Szmidt-Jaworska et al., 2009). The [DHSE] sequence was absent or present after a 2 amino acid gap. The intracellular sequence and proximal extracellular sequence also had limited conservation with the more detailed GC motif [RKS][YFW][CTGH][VIL][FV]G[DNA]X[VIL]X{4}[KR] used to identify AtGC1 (Ludidi and Gehring, 2003; Wong et al., 2018). Both eATP receptors therefore appear to have the requisite sequences to generate cyclic nucleotides, and this now requires experimental validation.
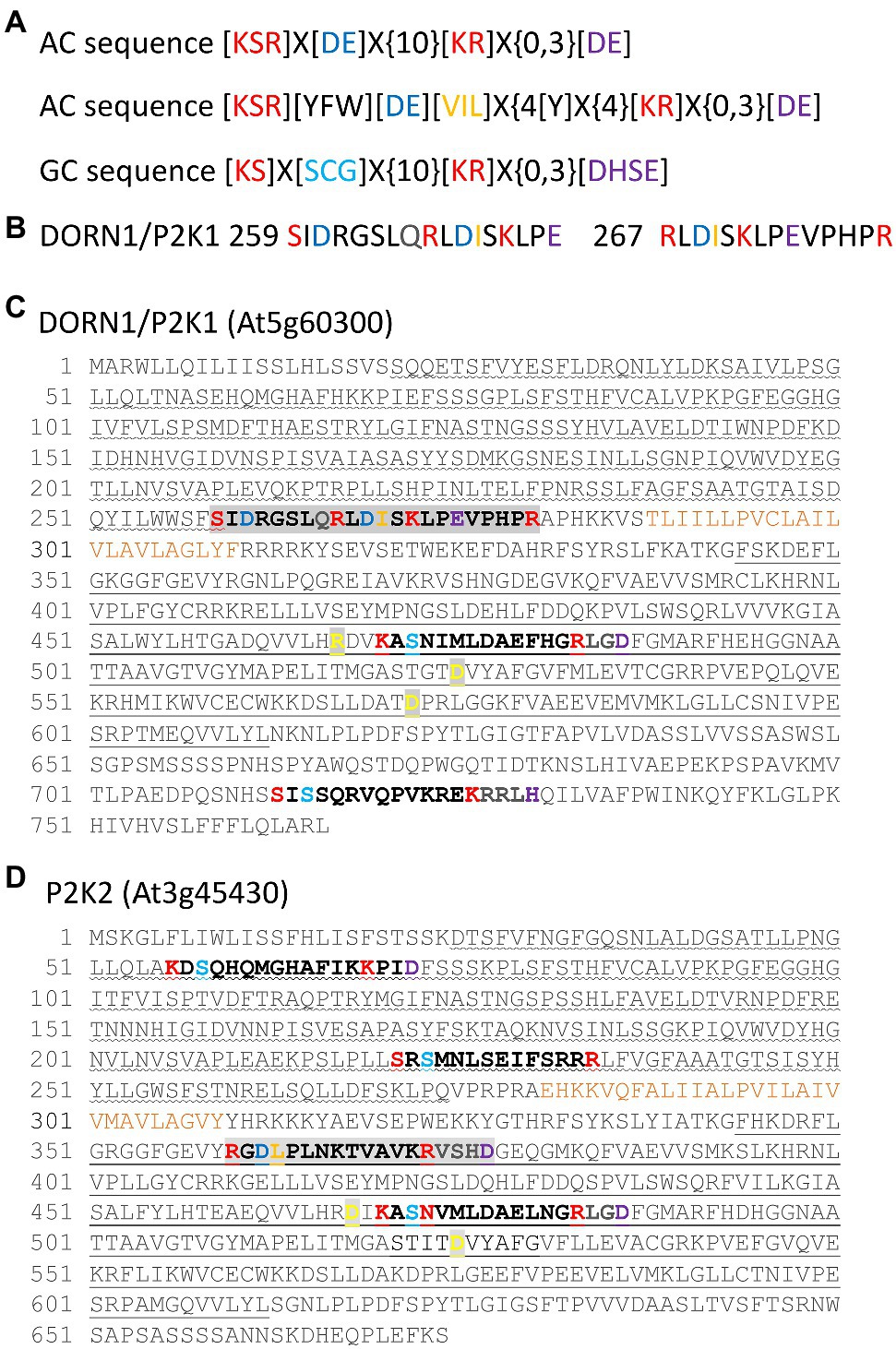
Figure 1. Putative AC and GC sequences in the extracellular ATP (eATP) receptors DORN1/P2K1 and P2K2. (A) Top: The ACPred AC prediction tool sequence (Xu et al., 2018b). “X” denotes any amino acid, and “{}” denotes the number of undetermined amino acids. Centre: The AC catalytic center sequence used by Al-Younis et al. (2021). Bottom: The GCPred GC prediction tool sequence (Xu et al., 2018a). (B) The two extracellular, overlapping putative AC sequences from DORN1/P2K1 shown separately for clarity. (C) Putative AC and GC sequences of DORN1/P2K1. Overlapping AC sequences are highlighted in light gray. The extracellular lectin domain is underlined with a wave, and the intracellular serine–threonine kinase domain is denoted with a straight underline. The transmembrane domain is annotated in ochre. Amino acids found to be important for kinase activity are bold in yellow on a gray background (Choi et al., 2014b; Nguyen et al., 2016; Cho et al., 2017). (D) Putative AC and GC sequences of P2K2. The AC sequence is highlighted in light gray. Domains are identified as for (B). Amino acids found to be important for kinase activity are bold in yellow on a gray background (Pham et al., 2020).
CNGC2 is Implicated in Cotyledon eATP-Induced [Ca2+]CYT Increase
While there remains a question mark between eATP and cyclic nucleotides, critically there is a precedent of a DAMP-cytosolic cGMP-CNGC2 pathway. Wounding of Arabidopsis increases [Ca2+]cyt resulting in activation of metacaspase 4. The latter then catalyzes production of plant elicitor peptide1 (Pep1) from its inactive precursor PROPEP1 (Hander et al., 2019). Pep1 can be perceived as a DAMP in a neighboring cell by its cognate plasma membrane receptors PEPR1 and PEPR2. Both of these LRR RLKs are predicted to have cytosolic GC activity, with PEPR1 confirmed experimentally. The cytosolic cGMP activates CNGC2 to effect a [Ca2+]cyt increase and downstream defense transcriptional response (Qi et al., 2010). eATP is now reported to act upstream of CNGC2. Wu et al. (2021) reported that eATP (0.1 mM) failed to elicit a plasma membrane Ca2+-permeable influx conductance in Arabidopsis pollen grain protoplasts of a cngc2 mutant. This concentration of eATP stimulated pollen germination in the wild type but not cngc2 (Wu et al., 2021), but it remains unknown whether activation of CNGC2 by eATP would increase [Ca2+]cyt. Here, the cngc2 loss of function mutant defense not death 1 (dnd1; which constitutively expresses cytosolic (apo)aequorin as a luminescent [Ca2+]cyt reporter, Qi et al., 2010) was tested for impaired eATP-induced [Ca2+]cyt increase. The dnd1 mutant of CNGC2 has a single point mutation causing a stop codon in the third exon that would result in a truncated protein lacking the pore region for ion conduction. dnd1 has a dwarf rosette phenotype that was also observed here (Supplementary Figure S1). Addition of control solution alone to single, excised cotyledons of Col-0 and dnd1 only induced the transient “touch response” caused by mechanical disturbance, which did not differ between genotypes (Figures 2A,B). In contrast, addition of 0.1 mM eATP caused a monophasic increase in [Ca2+]cyt after the touch response (Figure 2C). A similar pattern was observed in a previous study by Mohammad-Sidik et al. (2021). The response of dnd1 to 0.1 mM eATP was significantly lower than the Col-0 wild type, both for the maximum [Ca2+]cyt increase and estimated total [Ca2+]cyt mobilized [estimated as area under the curve (AUC), Figure 2D]. Increasing eATP to 1 mM (Figures 2E,F) also revealed a significant impairment in the response of dnd1 (Figure 2G) although clearly at both eATP concentrations loss of CNGC2 function allows the majority of the [Ca2+]cyt increase to occur. Overall these results show that CNGC2 could be involved in an eATP-induced [Ca2+]cyt increase in leaf tissue.
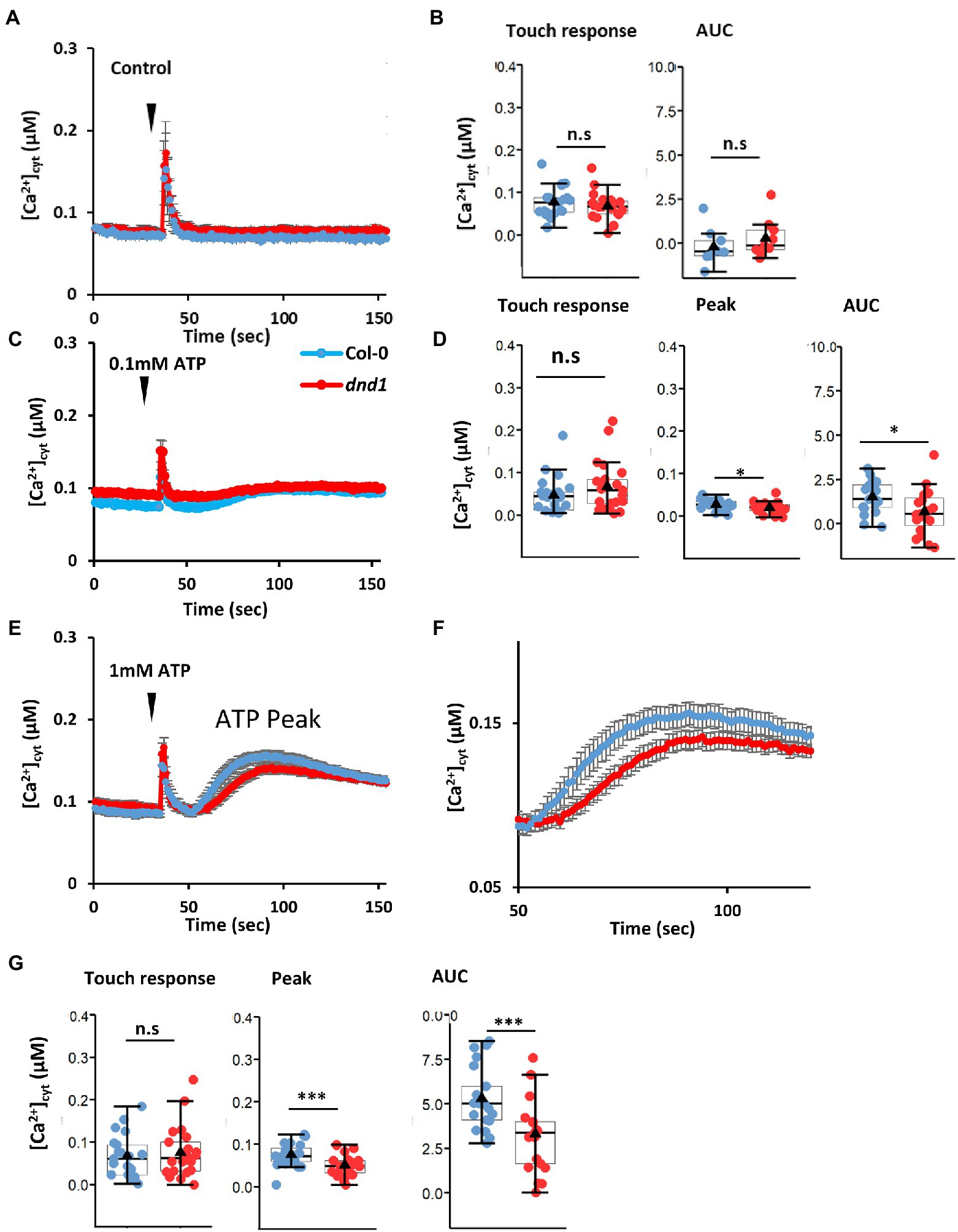
Figure 2. The defense not death 1 (dnd1) mutant has an impaired [Ca2+]cyt response to eATP. Cotyledons were excised from 14-day-old Col-0 and dnd1; cytosolic aequorin was reconstituted with coelenterazine (in 10 mM CaCl2, 0.1 mM KCl, 2 mM Tris/MES, pH 5.8) as described by Mohammad-Sidik et al. (2021). Luminescence was recorded from individual samples bathed in 10 mM CaCl2, 0.1 mM KCl, 2 mM Tris/MES, pH 5.8 as described by Mohammad-Sidik et al. (2021). As with flg22, ATP causes pronounced acidification of solutions, and so in this study, pH of eATP test solutions was maintained by buffers to avoid acidic pH artefactual responses which can impair interpretation (Westphal et al., 2019). [Ca2+]cyt values were calculated as described by Matthus et al. (2019b). (A) Mean ± SEM time course of [Ca2+]cyt response of Col-0 and dnd1 to control solution (10 mM CaCl2, 0.1 mM KCl, 2 mM Tris/MES, pH 5.8) added at 35s (inverted triangle). (B) Touch-induced [Ca2+]cyt increase from pre-stimulus baseline and estimated total [Ca2+]cyt mobilized [area under the curve (AUC), after baseline subtraction]. (C) Mean ± SEM time course of [Ca2+]cyt response of Col-0 and dnd1 to 0.1 mM eATP added at 35s (inverted triangle). (D) Touch-induced [Ca2+]cyt increase from pre-stimulus baseline, peak eATP-induced [Ca2+]cyt increase from baseline, and estimated AUC. (E) As (C) but with 1 mM eATP. (F) Mean ± SEM time course of the eATP response shown in (E). (G) As (D) but for 1 mM eATP. Results are from 3 independent trials with 9 samples in total per genotype in control trials and 19–21 samples in total per genotype in eATP trials. Each dot in box plots represents an individual recording. The middle line and the triangle in the box plot are the median and mean, respectively. Student’s t-test was used for analyzing statistical difference (***p < 0.001; *p < 0.05; n.s, not significant).
Discussion
At present, it can only be inferred from existing studies that eATP could increase cAMP or cGMP synthesis to act as second messengers in [Ca2+]cyt-based DAMP signaling. However, it is now timely to assess experimentally whether eATP does elicit an increase in cyclic nucleotides and whether this is driven by known eATP receptors. Verification of the putative AC/GC sequences in DORN1/P2K1 and P2K2 would mean that phosphorylation of cytosolic targets is not the only way these receptors can relay the eATP signal. Increasing [Ca2+]cyt inhibits the kinase activity of the Arabidopsis plasma membrane phytosulphokine receptor 1 (PSKR1) and promotes its GC activity (Muleya et al., 2014). It is therefore feasible that the receptors’ initial function is to relay by phosphorylation (which could activate plasma membrane Ca2+ channels to increase [Ca2+]cyt), but increasing [Ca2+]cyt switches the receptors’ mode to cyclic nucleotide production. A further layer of regulation could be effected by levels of H2O2, given the findings of Kuzakova et al. (2020) of dose-dependent stimulation or inhibition of pea (Pisum sativum) AC activity. The finding here of putative extracellular AC sequences in DORN1/P2K1 begs several questions. How does the affinity of eATP binding compare to that of the legume lectin domain (Kd 46 nM; Choi et al., 2014a)? Under what circumstances would extracellular cAMP be synthesized and to what purpose? Here, it should be noted that plant plasma membrane moonlighting ACs and GCs have low productivity and are held to be within distinct membrane domains containing their target in order to be efficient (Wong et al., 2018; Turek and Irving, 2021). Could the plant plasma membrane harbor a receptor for extracellular cAMP in close proximity to those for eATP? Two decades ago, there were no signs of a receptor for eATP in the Arabidopsis genome, but if a measurable output for sensing extracellular cyclic nucleotides could be established, then there is a way forward through forward genetic screens. Similar questions and future directions can also be posed for the extracellular putative cGMP synthesis sites of P2K2.
The finding here that a small but significant component of the eATP-induced [Ca2+]cyt elevation requires CNGC2 (at eATP levels consistent with wounding) helps fill a gap in our knowledge on how [Ca2+]cyt elevation is achieved and helps indirectly make the case for a role for cAMP or cGMP in DAMP signaling by eATP (summarized in Supplementary Figure S2). However, it remains feasible that CNGC2 could be phosphorylated by DORN1/P2K1 or P2K2 (if downstream of these) to promote Ca2+ influx. CNGC2 is already known to act downstream of PEPR1 and PEPR2 in DAMP signaling (Qi et al., 2010) and downstream of flg22 in PAMP signaling (Tian et al., 2019). CNGC2 therefore could be a critical component in coordinating the response to pathogens, which requires both damage and PAMPs to elicit the strongest immunogenic transcriptional outcome (Zhou et al., 2020). CNGC2 has recently been reported to be involved in auxin signaling (Chakraborty et al., 2021) so it could possibly play a part in the restorative growth response of cells surrounding damage sites as these sustain an increase in auxin as a longer-term response (Hoermayer et al., 2020). Much will depend on which cells harbor CNGC2, what its possible sub-unit partners in heterotetrameric channels are to help generate specific [Ca2+]cyt signals and their position in the membrane relative to receptors. There are another nineteen CNGCs in Arabidopsis that now warrant consideration as components of eATP DAMP signaling, although CNGC14 appears not to be involved in the root’s [Ca2+]cyt elevation by eATP (Shih et al., 2015).
Data Availability Statement
The raw data supporting the conclusions of this article will be made available by the authors, without undue reservation.
Author Contributions
JS and KW designed and performed the aequorin experiments. YN and LW analyzed the data. LW designed, performed, and analyzed the biomass determinations. JD analyzed sequences, conceived the project, and wrote the manuscript with contributions from the other authors. All authors contributed to the article and approved the submitted version.
Funding
Funding was from the UKRI BBSRC (BB/K009869/1), the framework of the 3rd call of the ERA-NET for Coordinating Action in Plant Sciences with funding from the BBSRC (BB/S004637/1), the University of Cambridge and Jiangsu University.
Conflict of Interest
The authors declare that the research was conducted in the absence of any commercial or financial relationships that could be construed as a potential conflict of interest.
Publisher’s Note
All claims expressed in this article are solely those of the authors and do not necessarily represent those of their affiliated organizations, or those of the publisher, the editors and the reviewers. Any product that may be evaluated in this article, or claim that may be made by its manufacturer, is not guaranteed or endorsed by the publisher.
Acknowledgments
We thank G. Berkowitz for the dnd1 line. We apologize to authors whose work we have not cited due to manuscript length restriction.
Supplementary Material
The Supplementary Material for this article can be found online at: https://www.frontiersin.org/articles/10.3389/fpls.2021.788514/full#supplementary-material
References
Al-Younis, I., Moosa, B., Kwiatkowski, M., Jaworski, K., Wong, A., and Gehring, C. (2021). Functional crypto-adenylate cyclases operate in complex plant proteins. Front. Plant Sci. 12:711749. doi: 10.3389/fpls.2021.711749
Balagué, C., Gouget, A., Bouchez, O., Souriac, C., Haget, N., Boutet-Mercey, S., et al. (2017). The Arabidopsis thaliana lectin receptor kinase LecRK-1.9 is required for full resistance to Pseudomonas syringae and affects jasmonate signalling. Mol. Plant Pathol. 18, 937–948. doi: 10.1111/mpp.12457
Behera, S., Xu, Z., Luoni, L., Bonza, C., Doccula, F. G., DeMichelis, M. I., et al. (2018). Cellular Ca2+ signals generate defined pH signatures in plants. Plant Cell 30, 2704–2719. doi: 10.1105/tpc.18.00655
Bianchet, C., Wong, A., Quaglia, M., Alqurashi, M., Gehring, C., Ntoukakis, V., et al. (2019). An Arabidopsis thaliana leucine-rich repeat protein harbors an adenylyl cyclase catalytic center and affects responses to pathogens. J. Plant Physiol. 232, 12–22. doi: 10.1016/j.jplph.2018.10.025
Bouwmeester, K., de Sain, M., Weide, R., Gouget, A., Klamer, S., Canut, H., et al. (2011). The lectin receptor kinase LecRK-1.9 is a novel Phytophthora resistance component and a potential host target for a RXLR effector. PLoS Pathog. 7:e1001327. doi: 10.1371/journal.ppat.1001327
Bouwmeester, K., Han, M., Blanco-Portales, R., Song, W., Weide, R., Guo, L. Y., et al. (2014). The Arabidopsis lectin receptor kinase LecRK-I.9 enhances resistance to Phytophthora infestans in Solanaceous plants. Plant Biotechnol. J. 12, 10–16. doi: 10.1111/pbi.12111
Caloni, F., Fossati, P., Anadón, A., and Bertero, A. (2020). Beauvericin: the beauty and the beast. Environ. Toxicol. Pharmacol. 75:103349. doi: 10.1016/j.etap.2020.103349
Castro, A. F., Amorena, C., Müller, A., Ottaviano, G., Tellez-Iñon, M. T., and Taquini, A. C. (1998). Extracellular ATP and bradykinin increase cGMP in vascular endothelial cells via activation of PKC. Am. J. Physiol. 275, C113–C119. doi: 10.1152/ajpcell.1998.275.1.C113
Chakraborty, S., Toyota, M., Moeder, W., Chin, K., Fortuna, A., Champigny, M., et al. (2021). Cyclic nucleotide-gated ion channel 2 modulates auxin homeostasis and signaling. Plant Physiol. doi: 10.1093/plphys/kiab332 [Epub ahead of print]
Chen, D., Cao, Y., Li, H., Kim, D., Ahsan, N., Thelen, J., et al. (2017). Extracellular ATP elicits DORN1-mediated RBOHD phosphorylation to regulate stomatal aperture. Nat. Commun. 8:2265. doi: 10.1038/s41467-017-02340-3
Chivasa, S., Ndimba, B. K., Simon, W. J., Lindsey, K., and Slabas, A. R. (2005). Extracellular ATP functions as an endogenous external metabolite regulating plant cell viability. Plant Cell 17, 3019–3034. doi: 10.1105/tpc.105.036806
Cho, S.-H., Nguyen, C. T., Choi, J., and Stacey, G. (2017). Molecular mechanism of plant recognition of extracellular ATP. Adv. Exp. Med. Biol. 1051, 233–253. doi: 10.1007/5584_2017_110
Choi, S. Y., and Kim, K. T. (1997). Extracellular ATP-stimulated increase of cytosolic cAMP in HL-60 cells. Biochem. Pharmacol. 53, 429–432. doi: 10.1016/S0006-2952(96)00719-8
Choi, J., Tanaka, K., Cao, Y., Qi, Y., Qiu, J., Liang, Y., et al. (2014a). Identification of a plant receptor for extracellular ATP. Science 343, 290–294. doi: 10.1126/science.343.6168.290
Choi, J., Tanaka, K., Liang, Y., Cao, Y. R., Lee, S. Y., and Stacey, G. (2014b). Extracellular ATP, a danger signal, is recognized by DORN1 in Arabidopsis. Biochem. J. 463, 429–437. doi: 10.1042/BJ20140666
Dark, A., Demidchik, V., Richards, S. L., Shabala, S., and Davies, J. M. (2011). Release of extracellular purines from plant roots and effect on ion fluxes. Plant Signal. Behav. 6, 1855–1857. doi: 10.4161/psb.6.11.17014
Demidchik, V., Nichols, C., Oliynyk, M., Dark, A., Glover, B. J., and Davies, J. M. (2003). Is ATP a signaling agent in plants? Plant Physiol. 133, 456–461. doi: 10.1104/pp.103.024091
Demidchik, V., Shang, Z., Shin, R., Thompson, E., Rubio, L., Laohavisit, A., et al. (2009). Plant extracellular ATP signalling by plasma membrane NADPH oxidase and Ca2+ channels. Plant J. 58, 903–913. doi: 10.1111/j.1365-313X.2009.03830.x
Deng, S., Sun, J., Zhao, R., Ding, M., Zhang, Y., Sun, Y., et al. (2015). Populus euphratica APYRASE2 enhances cold tolerance by modulating vesicular trafficking and extracellular ATP in Arabidopsis plants. Plant Physiol. 169, 530–548. doi: 10.1104/pp.15.00581
Gouget, A., Senchou, V., Govers, F., Sanson, A., Barre, A., Rougé, P., et al. (2006). Lectin receptor kinases participate in protein-protein interactions to mediate plasma membrane-cell wall adhesions in Arabidopsis. Plant Physiol. 140, 81–90. doi: 10.1104/pp.105.066464
Hander, T., Fernández-Fernández, Á. D., Kumpf, R. P., Willems, P., Schatowitz, H., Rombaut, D., et al. (2019). Damage on plants activates Ca2+-dependent metacaspases for release of immunomodulatory peptides. Science 363:eaar7486. doi: 10.1126/science.aar7486
Hoermayer, L., Montesinos, J. C., Marhava, P., Benková, E., Yoshida, S., and Friml, J. (2020). Wounding-induced changes in cellular pressure and localized auxin signalling spatially coordinate restorative divisions in roots. Proc. Natl. Acad. Sci. U. S. A. 117, 15322–15331. doi: 10.1073/pnas.2003346117
Jarratt-Barnham, E., Wang, L., Ning, Y., and Davies, J. M. (2021). The complex story of plant cyclic nucleotide-gated channels. Int. J. Mol. Sci. 22:874. doi: 10.3390/ijms22020874
Jewell, J. B., Sowders, J. M., He, R., Willis, M. A., Gang, D. R., and Tanaka, K. (2019). Extracellular ATP shapes a defense-related transcriptome both independently and along with other defense signaling pathways. Plant Physiol. 179, 1144–1158. doi: 10.1104/pp.18.01301
Jiang, X. Y., Hoehenwarter, W., Scheel, D., and Lee, J. (2020). Phosphorylation of the CAMTA3 transcription factor triggers its destabilization and nuclear export. Plant Physiol. 184, 1056–1071. doi: 10.1104/pp.20.00795
Kim, S. Y., Sivaguru, M., and Stacey, G. (2006). Extracellular ATP in plants. Visualization, localization, and analysis of physiological significance in growth and signaling. Plant Physiol. 142, 984–992. doi: 10.1104/pp.106.085670
Kumar, S., Tripathi, D., Okubara, P. A., and Tanaka, K. (2020). Purinoceptor P2K1/DORN1 enhances plant resistance against a soilborne fungal pathogen, Rhizoctonia solani. Front. Plant Sci. 11:572920. doi: 10.3389/fpls.2020.572920
Kuzakova, O. V., Lomovatskaya, L. A., Romanenko, A. S., and Goncharova, A. M. (2020). Regulation of the activity of adenylate cyclases by hydrogen peroxide in pea root cells infected with pathogens and a mutualist. Izvestiya Vuzov-Prikladnaya Khimiya i Biotekhnologiya 10, 450–458. doi: 10.21285/2227-2925-2020-10-3-450-458
Kwezi, L., Ruzvidzo, O., Wheeler, J. I., Govender, K., Iacuone, S., Thompson, P. E., et al. (2011). The phytosulfokine (PSK) receptor is capable of guanylate cyclase activity and enabling cyclic GMP-dependent signaling in plants. J. Biol. Chem. 286, 22580–22588. doi: 10.1074/jbc.M110.168823
Laohavisit, A., Shang, Z., Rubio, L., Cuin, T. A., Véry, A. A., Wang, A., et al. (2012). Arabidopsis annexin1 mediates the radical-activated plasma membrane Ca2+-and K+-permeable conductance in root cells. Plant Cell 24, 1522–1533. doi: 10.1105/tpc.112.097881
Ludidi, N. N., and Gehring, C. (2003). Identification of a novel protein with guanylyl cyclase activity in Arabidopsis thaliana. J. Biol. Chem. 278, 6490–6494. doi: 10.1074/jbc.M210983200
Ma, L., Ye, J., Yang, Y., Lin, H., Yue, L., Luo, J., et al. (2019). The SOS2-SCaBP8 complex generates and fine-tunes an AtANN4-dependent calcium signature under salt stress. Dev. Cell 48, 697.e5–709.e5. doi: 10.1016/j.devcel.2019.02.010
Malabarba, J., Meents, A., Reichelt, M., Scholz, S., Peiter, E., Rachowka, J., et al. (2021). ANNEXIN1 mediates calcium-dependent systemic defense in Arabidopsis plants upon herbivory and wounding. New Phytol. 231, 243–254. doi: 10.1111/nph.17277
Malukani, K. K., Ranjan, A., Hota, S. J., Patel, H. K., and Sonti, R. V. (2020). Dual activities of receptor-like kinase OsWAKL21.2 induce immune responses. Plant Physiol. 183, 1345–1363. doi: 10.1104/pp.19.01579
Matthus, E., Sun, J., Wang, L., Bhat, M. G., Mohammad-Sidik, A. B., Wilkins, K. A., et al. (2019a). DORN1/P2K1 and purino-calcium signaling in plants: making waves with extracellular ATP. Ann. Bot. 124, 1227–1242. doi: 10.1093/aob/mcz135
Matthus, E., Wilkins, K. A., Swarbreck, S. M., Doddrell, N. H., Doccula, F. G., Costa, A., et al. (2019b). Phosphate starvation alters abiotic-stress-induced cytosolic free calcium increase in roots. Plant Physiol. 179, 1754–1767. doi: 10.1104/pp.18.01469
Mohammad-Sidik, A., Sun, J., Shin, R., Song, Z., Ning, Y., Matthus, E., et al. (2021). Annexin1 is a component of eATP-induced cytosolic calcium elevation in Arabidopsis thaliana roots. Int. J. Mol. Sci. 22:494. doi: 10.3390/ijms22020494
Muleya, V., Wheeler, J. I., Ruzvidzo, O., Freihat, L., Manallack, D. T., Gehring, C., et al. (2014). Calcium is the switch in the moonlighting dual function of the ligand-activated receptor kinase phytosulfokine receptor 1. Cell Commun. Signal. 12:60. doi: 10.1186/s12964-014-0060-z
Nguyen, C. T., Tanaka, K., Cao, Y., Cho, S. H., Xu, D., and Stacey, G. (2016). Computational analysis of the ligand binding site of the extracellular ATP receptor DORN1. PLoS One 11:e0161894. doi: 10.1371/journal.pone.0161894
Nizam, S., Qiang, X., Wawra, S., Nostadt, R., Getzke, F., Schwanke, F., et al. (2019). Serendipita indica E50NT modulates extracellular nucleotide levels in the plant apoplast and affects fungal colonization. EMBO Rep. 20:e47430. doi: 10.15252/embr.201847430
Pham, A. Q., Cho, S.-H., Nguyen, C. T., and Stacey, G. (2020). Arabidopsis lectin receptor kinase P2K2 is a second plant receptor for extracellular ATP and contributes to innate immunity. Plant Physiol. 183, 1364–1375. doi: 10.1104/pp.19.01265
Qi, Z., Verma, R., Gehring, C., Yamaguchi, Y., Zhao, Y., Ryan, C. A., et al. (2010). Ca2+ signaling by plant Arabidopsis thaliana pep peptides depends on AtPepR1, a receptor with guanylyl cyclase activity, and cGMP-activated Ca2+ channels. Proc. Natl. Acad. Sci. U. S. A. 107, 21193–21198. doi: 10.1073/pnas.1000191107
Ruzvidzo, O., Gehring, C., and Wong, A. (2019). New perspectives on plant adenylyl cyclases. Front. Mol. Biosci. 6:136. doi: 10.3389/fmolb.2019.00136
Shih, H. W., DePew, C. L., Miller, N. D., and Monshausen, G. B. (2015). The cyclic nucleotide-gated channel CNGC14 regulates root gravitropism in Arabidopsis thaliana. Curr. Biol. 25, 3119–3125. doi: 10.1016/j.cub.2015.10.025
Smith, S. J., Goodman, H., Kroon, J. T. M., Brown, A. P., Simon, W. J., and Chivasa, S. (2021). Isolation of Arabidopsis extracellular ATP binding proteins by affinity proteomics and identification of PHOSPHOLIPASE C-LIKE 1 as an extracellular protein essential for fumonisin B1 toxicity. Plant J. 106, 1387–1400. doi: 10.1111/tpj.15243
Song, C. J., Steinebrunner, I., Wang, X., Stout, S. C., and Roux, S. J. (2006). Extracellular ATP induces the accumulation of superoxide via NADPH oxidases in Arabidopsis. Plant Physiol. 140, 1222–1232. doi: 10.1104/pp.105.073072
Šrobárova, A., Teixeira da Silva, J. A., Kogan, G., Ritieni, A., and Santini, A. (2009). Beauvericin decreases cell viability of wheat. Chem. Biodivers. 6, 1208–1215. doi: 10.1002/cbdv.200800158
Su, Y.-L., Li, J.-M., Li, M., Luan, J.-B., Ye, X.-D., Wang, X.-W., et al. (2012). Transcriptomic analysis of the salivary glands of an invasive whitefly. PLoS One 7:e39303. doi: 10.1371/journal.pone.0039303
Sun, J., Zhang, C. L., Deng, S. R., Lu, C. F., Shen, X., Zhou, X. Y., et al. (2012). An ATP signalling pathway in plant cells: extracellular ATP triggers programmed cell death in Populus euphratica. Plant Cell Environ. 35, 893–916. doi: 10.1111/j.1365-3040.2011.02461.x
Świeżawska, B., Duszyn, M., Kwiatowski, M., Jaworski, K., Pawełek, A., and Szmidt-Jaworska, A. (2019). Brachypodium distachyon triphosphatase tunnel metalloenzyme 3 is both a triphosphatase and an adenylyl cyclase upregulated by mechanical wounding. FEBS Lett. 594, 1101–1111. doi: 10.1002/1873-3468.13701
Świeżawska, B., Jaworski, K., Szewczuk, P., Pawełek, A., and Szmidt-Jaworska, A. (2015). Identification of a Hippeastrum hybridum guanylyl cyclase responsive to wounding and pathogen infection. J. Plant Physiol. 189, 77–86. doi: 10.1016/j.jplph.2015.09.014
Szmidt-Jaworska, A., Jaworski, K., Pawełek, A., and Kocewicz, J. (2009). Molecular cloning and characterization of a guanylyl cyclase, PNGC-1, involved in light signaling in Pharbitis nil. J. Plant Growth Regul. 28, 367–380. doi: 10.1007/s00344-009-9105-8
Tanaka, K., and Heil, M. (2021). Damage-associated molecular patterns (DAMPs) in plant innate immunity: applying the danger model and evolutionary perspectives. Annu. Rev. Phytopathol. 59, 53–75. doi: 10.1146/annurev-phyto-082718-100146
Tian, W., Hou, C., Ren, Z., Wang, C., Zhao, F., Dahlbeck, D., et al. (2019). A calmodulin-gated calcium channel links pathogen patterns to plant immunity. Nature 572, 131–135. doi: 10.1038/s41586-019-1413-y
Toyota, M., Spencer, D., Sawai-Toyota, S., Jiaqi, W., Zhang, T., Koo, A. J., et al. (2018). Glutamate triggers long-distance, calcium-based plant defense signaling. Science 361, 1112–1115. doi: 10.1126/science.aat7744
Tripathi, D., Zhang, T., Koo, A. J., Stacey, G., and Tanaka, K. (2018). Extracellular ATP acts on jasmonate signaling to reinforce plant defense. Plant Physiol. 176, 511–523. doi: 10.1104/pp.17.01477
Turek, I., and Irving, H. (2021). Moonlighting proteins shine new light on molecular signaling niches. Int. J. Mol. Sci. 22:1367. doi: 10.3390/ijms22031367
van Damme, T., Blancquaert, D., Couturon, P., van der Staeten, D., Sandra, P., and Lynen, F. (2014). Wounding stress causes rapid increase in concentration of the naturally occurring 2',3'-isomers of cyclic guanosine- and cyclic adenosine monophosphate (cGMP and cAMP) in plant tissues. Phytochemistry 103:59066. doi: 10.1016/j.phytochem.2014.03.013
Verkhratsky, A. (2021). Early evolutionary history (from bacteria to the hemichordate) of the omnipresent purinergic signaling: a tribute to Geoff Burnstock’s inquisitive mind. Biochem. Pharmacol. 187:114261. doi: 10.1016/j.bcp.2020.114261
Wang, Q. W., Jia, L. Y., Shi, D. L., Wang, R. F., Lu, L. N., Xie, J. J., et al. (2019a). Effects of extracellular ATP on local and systemic responses of bean (Phaseolus vulgaris L) leaves to wounding. Biosci. Biotechnol. Biochem. 83, 417–428. doi: 10.1080/09168451.2018.1547623
Wang, F., Jia, J., Wang, Y., Wang, W., Chen, Y., Liu, T., et al. (2014). Hyperpolarization-activated Ca2+ channels in guard cell plasma membrane are involved in extracellular ATP-promoted stomatal opening in Vicia faba. J. Plant Physiol. 171, 1241–1247. doi: 10.1016/j.jplph.2014.05.007
Wang, L., Stacey, G., Leblanc-Fournier, N., Legué, V., Moulia, B., and Davies, J. M. (2019b). Early extracellular ATP signaling in Arabidopsis root epidermis: a multi-conductance process. Front. Plant Sci. 10:1064. doi: 10.3389/fpls.2019.01064
Wang, L., Wikins, K. A., and Davies, J. M. (2018). Arabidopsis DORN1 extracellular ATP receptor; activation of plasma membrane K+-and Ca2+-permeable conductances. New Phytol. 218, 1301–1304. doi: 10.1111/nph.15111
Weerasinghe, R. R., Swanson, S. J., Okada, S. F., Garrett, M. B., Kim, S.-Y., Stacey, G., et al. (2009). Touch induces ATP release in Arabidopsis roots that is modulated by the heterotrimeric G-protein complex. FEBS Lett. 583, 2521–2526. doi: 10.1016/j.febslet.2009.07.007
Westphal, L., Strehmel, N., Eschen-Lippold, L., Bauer, N., Westermann, B., Rosahl, S., et al. (2019). pH effects on plant calcium fluxes: lessons from acidification-mediated calcium elevation induced by the gamma-glutamyl-leucine dipeptide identified from Phytophthora infestans. Sci. Rep. 9:4733. doi: 10.1038/s41598-019-41276-0
Wong, A., and Gehring, C. (2013). The Arabidopsis thaliana proteome harbors undiscovered multi-domain molecules with functional guanylyl cyclase catalytic centers. Cell Commun. Signal. 11:48. doi: 10.1186/1478-811X-11-48
Wong, A., Tian, X., Gehring, C., and Marondedze, C. (2018). Discovery of novel functional centers with rationally designed amino acid motifs. Comput. Struct. Biotechnol. J. 16, 70–76. doi: 10.1016/j.csbj.2018.02.007
Wu, S., Peiffer, M., Luthe, D. S., and Felton, G. W. (2012). ATP hydrolyzing salivary enzymes of caterpillars suppress plant defenses. PLoS One 7:e41947. doi: 10.1371/journal.pone.0041947
Wu, Y., Qin, B., Feng, K., Yan, R., Kang, E., Liu, T., et al. (2018). Extracellular ATP promoted pollen germination and tube growth of Nicotiana tabacum through promoting K+ and Ca2+ absorption. Plant Reprod. 31, 399–410. doi: 10.1007/s00497-018-0341-6
Wu, Y., Yin, H., Liu, X., Xu, J., Qin, B., Feng, K., et al. (2021). P2K1 receptor, heterotrimeric Gα protein and CNGC2/4 are involved in extracellular ATP-promoted ion influx in the pollen of Arabidopsis thaliana. Plan. Theory 10:1743. doi: 10.3390/plants10081743
Xu, N., Fu, D. F., Li, S., Wang, Y. X., and Wong, A. (2018a). GCPred: a web tool for guanylyl cyclase functional centre prediction from amino acid sequence. Bioinformatics 34, 2134–2135. doi: 10.1093/bioinformatics/bty067
Xu, R., Guo, Y., Peng, S., Liu, J., Li, P., Jia, W., et al. (2021). Molecular targets and biological functions of cAMP signaling in Arabidopsis. Biomol. Ther. 11:688. doi: 10.3390/biom11050688
Xu, N., Zhang, C., Lim, L. L., and Wong, A. (2018b). “Bioinformatic analysis of nucleotide cyclase functional centers and development of ACPred webserver.” in Proceedings of the 2018 ACM International Conference on Bioinformatics, Computational Biology, and Health Informatics; August 29–September 1, 2018; Washington, DC, USA, 122–129.
Zhou, F., Emonet, A., Tendon, D., Marhavy, P., Wu, D., Lahaye, T., et al. (2020). Co-incidence of damage and microbial patterns controls localized immune responses in roots. Cell 180, 440–453. doi: 10.1016/j.cell.2020.01.013
Zhu, R., Dong, X., Hao, W., Gao, W., Zhang, W., Xia, S., et al. (2017). Heterotrimeric G protein-regulated Ca2+ influx and PIN2 asymmetric distribution are involved in Arabidopsis thaliana roots’ avoidance response to extracellular ATP. Front. Plant Sci. 8:1522. doi: 10.3389/fpls.2017.01522
Keywords: calcium, CNGC, cyclase, cyclic nucleotide, DAMP, ATP
Citation: Sun J, Ning Y, Wang L, Wilkins KA and Davies JM (2021) Damage Signaling by Extracellular Nucleotides: A Role for Cyclic Nucleotides in Elevating Cytosolic Free Calcium? Front. Plant Sci. 12:788514. doi: 10.3389/fpls.2021.788514
Edited by:
Yuree Lee, Seoul National University, South KoreaReviewed by:
Sarah J. Swanson, University of Wisconsin System, United StatesStéphane Bourque, Université de Bourgogne, France
Copyright © 2021 Sun, Ning, Wang, Wilkins and Davies. This is an open-access article distributed under the terms of the Creative Commons Attribution License (CC BY). The use, distribution or reproduction in other forums is permitted, provided the original author(s) and the copyright owner(s) are credited and that the original publication in this journal is cited, in accordance with accepted academic practice. No use, distribution or reproduction is permitted which does not comply with these terms.
*Correspondence: Julia M. Davies, am1kMzJAY2FtLmFjLnVr