- 1State Key Laboratory of North China Crop Improvement and Regulation, Key Laboratory of Crop Growth Regulation of Hebei Province, College of Agronomy, Hebei Agricultural University, Baoding, China
- 2National Engineering Laboratory for Crop Molecular Breeding, National Center of Space Mutagenesis for Crop Improvement, Institute of Crop Sciences, Chinese Academy of Agricultural Sciences, Beijing, China
- 3Shijiazhuang Academy of Agricultural and Forestry Sciences, Shijiazhuang, China
Melatonin (MT) is a small molecule indole hormone that plays an important role in the regulation of biological processes and abiotic stress resistance. Previous studies have confirmed that MT promotes the normal development of plants under stress by mediating physiological regulation mechanisms. However, the physiological mechanism of exogenous MT regulating seed germination and seedling growth of wheat under salt stress is still unclear. In this study, NaCl stress decreased germination rate and inhibited seedling growth of wheat, but shoot length, root length, and plant weight of SM15 did not change significantly. The addition of 300 μM MT in the cultivation solution directly promoted the germination rate of SM15 and ZM18, and lateral root production, but decreased the germination rate of JM22 and inhibited the length of germ and radicle of three varieties under salt stress. For wheat seedling, application of MT could increase proline content, soluble protein, soluble sugar, Ca2+ content, and vital amino acid content in leaves to keep high water content, low level of H2O2 content, and low [K+]/[Na+] ratio. MT increased root vigor and [K+]/[Na+] ratio and decreased H2O2 content in root induced by salt stress. In conclusion, MT enhanced salt tolerance in wheat seeds and seedlings by regulating the synthesis of soluble protein and sugar, ion compartmentation in roots and leaves, enhancement of enzymatic systems, and changes in amino acid levels. Salt resistance varied with different varieties under the same environmental condition. SM15 was a higher salt-resistant variety and JM22 was a salt-sensitive one. In wheat production, the application of exogenous MT should consider the differences among varieties of wheat during the sowing and seedling stages.
Introduction
Soil salinization, a geologically environmental problem all over the world, is one of the main factors resulting in the decrease in grain production (Liang et al., 2018). According to incomplete statistics, the salinization of arable land globally is increasing at a rate of 0.3–1.5 million hectares year–1 (Harper et al., 2021). The total area of salinized soil in China is about 99.13 × 106 hm2, which accounts for 1.03% of the total Chinese land area (Yang and Wang, 2015). It is expected that 50% of arable land will be under saline-alkali stress by 2050 due to environmental pollution, lack of freshwater, improper irrigation methods, and other factors (Dou et al., 2019; Yang et al., 2021). Wheat (Triticum aestivum L.), one of the medium salt-tolerant crops and the second-largest food crop in the world, also faces soil salinization in its growing area. Salt stress leads to the significant inhibition of seed germination and seedling growth (Shahi et al., 2015). Under salt stress, excessive reactive oxygen species (ROS) are accumulated which will cause cell damage, DNA damage, and oxidative stress to plants, consequently disrupting the physiological balance and leading to reduced photosynthesis and production yield (Zhang et al., 2016; Tripathi et al., 2020). High Na+ accumulation in plant cells leads to osmotic stresses and limits uptake and utilization of other nutrition ions, which causes moisture loss and electrolytic leaching due to cell membrane damage (Abbas et al., 2015; Ahmed et al., 2015; Rebey et al., 2017).
Melatonin (MT), an amine hormone, has been confirmed extensively for enhancing plant tolerance to various abiotic stresses (Zhang et al., 2013; Turk et al., 2014; Ren et al., 2020). There are multiple mechanisms verified in MT helping plants to enhance abiotic stress tolerance (Huangfu et al., 2021; Sun et al., 2021). The application of MT has shown stimulating antioxidant enzymes and scavenging ROS in plants under stress conditions (Park et al., 2013; Li et al., 2018), preventing chlorophyll degradation and increasing photosynthetic efficiency, reducing ion toxicity (e.g., heavy metals, Na+, and so on) and increasing osmotic substances to maintain water status (Gao et al., 2016; Cai et al., 2017; Siddiqui et al., 2019a,2020; Al-Huqail et al., 2020), and enhancing secondary metabolite biosynthesis and upregulating defense genes to decrease cell injury (Li H. et al., 2017; Zhao et al., 2017; Elsayed et al., 2021). Under salt stress, MT alleviates directly ROS burst and cell damage for bermudagrass (Shi et al., 2015). Also, MT alleviates the inhibitory effects of NaCl stress on germination of cucumber seeds mainly by increasing antioxidant enzymes, affecting phytohormone biosynthesis and catabolism, and regulating storage protein degradation to vital amino acids during seed germination (Zhang H. J. et al., 2014; Zhang et al., 2017). MT has been reported to extend longevity by significantly reducing chlorophyll degradation, delaying the leaf senescence, and preventing cell death in rice plant and tomato seedlings under salt stress (Siddiqui et al., 2019b; Zhao et al., 2021). During wheat seedling growth, MT accelerates the transformation from arginine and methionine to polyamines in wheat seedlings (Ke et al., 2018). Salt tolerance enhanced in maize with MT is most likely due to the improvement in photosynthetic capacity, antioxidative capacity, and ion homeostasis (increased K+ contents and K+/Na+ ratios) in leaves (Jiang C. et al., 2016). Also, Liu et al. (2020) demonstrate that MT improves salt tolerance in rice by reducing K+ efflux induced by high NaCl concentrations in roots. Subsequent researches have partly revealed the mechanisms of MT, which affects directly or indirectly salt resistance in many plants. However, MT application had no effects on the growth of wheat and maize under normal conditions (Ke et al., 2018; Ren et al., 2020). It has not fully elucidated a universal pathway on whether or how MT launches crosstalk with vital regulators under stress.
Different concentrations of MT may have differential effects in mediating abiotic stress defense. MT at 200 μmol L–1 can effectively inhibit ROS production, improve the biomass and chlorophyll content, and regulate the photosynthetic characteristics of cotton seedlings under salt stress (Jiang et al., 2021). MT at 300 μmol L–1 alleviates the negative effect of water stress on wheat germination and promotes morphological development including radicle length, radicle number, and plumule length of germinated seeds (Li et al., 2020). About 800 μmol L–1 MT used for priming seed significantly improves germination energy, germination percentage, proline content, and total phenolic content of maize (Jiang X. et al., 2016). Application of exogenous MT (100 μmol L–1) alleviates ROS burst and protects the photosynthetic activity in maize seedlings under salt stress (Chen et al., 2018). For some other reports, MT at a concentration of 100 μmol L–1 inhibits seed germination and seedling growth and enhances the toxic effect of copper on seedlings, but 1 or 10 μmol L–1 MT can eliminate the inhibitory effect of copper on the fresh weight of red cabbage seedlings (Posmyk et al., 2008). Pretreatment with 1 μmol L–1 MT was also found to partially mitigate the inhibition of shoot dry weight induced by salt stress (Ke et al., 2018). Li X. et al. (2017) showed that 10–500 μmol L–1 MT could help to recover seed germination potential, germination index, and vigor index on two varieties of rice treated with 120 mM NaCl to control levels. It seems that MT can regulate and enhance stress resistance by multiple mechanisms in a concentration-dependent manner.
In wheat production, different varieties show different resistances, adaptive areas, and production yields. So far, the roles of MT in mediating salt stresses on physiological regulation still need to be explored in different wheat varieties. In this study, seed germination and seedling growth of winter wheat experiments were conducted in a hydroponic solution with NaCl (100 mM) or without NaCl (100 mM), and the addition of MT. The purposes of this study were to (1) verify that MT enhances seed germination rate and antioxidant activities of seedling, (2) elucidate the regulatory effect of MT on ion exchange in the organelles, and (3) compare the regulatory effects of MT on the different varieties of wheat under salt stress. The results of this experiment provide theoretical support to promote the application of exogenous MT on saline-alkali wheat to increase arable land reserve and improve food security.
Materials and Methods
Plant Material
The experiment was conducted in 2021 at the Hebei Agricultural University (38° 85′ N, 115° 30′ E), Hebei Province, China. Three commercial wheat (T. aestivum L.) cultivars, ‘Jimai 22’ (JM22), ‘Shimai 15’ (SM15), and ‘Zhoumai 18’ (ZM18), were used in this study. All varieties are high-yielding and extensively cultivated in Huang-Huai-Hai region. ZM18 is suitable for planting in south section of the Huanghuai winter wheat area, and JM22 is suitable for planting in north section of the Huanghuai winter wheat area. SM15 has comprehensive resistance and good adaptability.
Experimental Design
This experiment was performed in a growth chamber.
Seed Germination
In total, 450 seeds of each wheat variety were surface-sterilized with 75% ethanol for five minutes. After that, all surface-sterilized seeds were washed thoroughly with distilled water and were transferred into a glass plate for germination. Totally, two layers of filter paper saturated with three different treatment solutions: CK (distilled water), NaCl (100 mM), and NaCl-MT (100 mM NaCl + 300 μM MT) were placed in each plate. The concentrations of NaCl and MT solutions were selected referring to Li X. et al. (2017), Ke et al. (2018), and our former study (Li et al., 2020). The germination conditions were set at a light/dark shift (12/12h). The day/night temperature was 20/15°C. Light intensity was 600 μmol/m2/s1. Relative humility was 60%. The number of seed germinated was recorded daily till the 10th day. Water and other solutions were added into the germination plates to keep suitable humidity.
Hydroponic Experiments
At first, some other healthy seeds of three wheat varieties were germinated in a hole tray with moist vermiculite. The indoor environmental conditions were the same as those described above for the germination plate experiment. When the second leaf appeared, seedlings were removed out of hole tray gently and washed off vermiculite attached to the roots in tap water several times. Then, seedlings were transferred into Hoagland nutrient solution in plastic boxes covered with black film to avoid light. After about 1 week of recovery growth, seedlings were treated with fresh Hoagland nutrient solution as control, 100 mM NaCl solution as salt stress, and 100 mM NaCl + 300 μM MT. Each treatment was repeated three times. After 24 h, leaf and root samples were collected and quickly frozen using liquid nitrogen and then stored in a −80°C freezer until the measurements were taken.
Measurement of Morphological Parameters
Germinated seeds were recorded daily when radicle length exceeded 2 mm according to Liu et al. (2016). Germ and radicle length and radicle number of 10 randomly selected grains from each treatment were recorded with a ruler for 24 h after treatment.
Shoot and root length of five randomly selected seedlings from each replication were measured 24 h after treatment. Seedlings of each treatment with triplicates were dissected into roots, stems, and leaf and their fresh weights were recorded immediately. Then, the weighed fresh samples were kept in the paper bags to be dried in an oven at 105°C for 30 min and then at 75°C until the consistent weight was obtained.
Na+, K+, and Ca2+ Contents
Na+, K+, and Ca2+ contents of the whole-plant tissues were determined using a previous method with minor modifications (Chen et al., 2017). After the measurement of dry weight, samples were digested in a mixed solution of perchloric acid or concentrated nitric acid (volume ratio, HClO4:HNO3 = 1:5, v:v) in a glass test tube. More solution has replenished the solution to 12 mL. The ion content of a solution in a glass test tube was extracted in boiling water bath for 5 h and measured using an atomic absorption spectrophotometer (ZA-3000; Hitachi Instruments, Tokyo, Japan).
Hydrogen Peroxide Content and Detection of H2O2 and O2–
Hydrogen peroxide (H2O2) content in the leaf and root samples was determined using a H2O2 assay kit (A064, Nanjing Jiancheng Bioengineering Institute, Nanjing, China) according to the manufacturer’s user manual. H2O2 accumulation in root samples was visualized according to what Liu (2020) described. About 1.5 cm root tip was soaked and stained in 1% (w/v) 3,3′-diaminobenzidine staining (DAB, dissolved with 10 mM MES buffer on pH value = 6.5) and incubated in dark at 25°C for 8 h. Then, the samples were washed several times and observed using an optical microscope (Leica, Wetzlar, Germany).
Superoxide anion (O2–) accumulation in root samples (about 1.5 cm) was also visualized according to Liu’s (2020) method. Root samples were stained in 100 μM nitro blue tetrazolium chloride (NBT) dissolved with 50 mM phosphate buffer (pH value = 6.4) for 15 min and observed using an optical microscope (Leica, Wetzlar, Germany).
Antioxidant Enzymes, Soluble Sugar, Soluble Protein, and Malondialdehyde Content
Fresh leaf sample (0.5 g each) was triturated in 50 mM phosphate buffer (pH = 6.4). Homogenate solution was centrifuged at 20,000 g at 4°C for 15 min. Superoxide dismutase (SOD), peroxidase (POD), catalase (CAT) activity, soluble sugar, soluble protein, and malondialdehyde (MDA) contents were determined using assay kits (A064, Nanjing Jiancheng Bioengineering Institute, Nanjing, China) according to the manufacturer’s instructions. Soluble sugar and protein contents were determined using a soluble sugar assay kit (A145-1-1, Nanjing Jiancheng Bioengineering Institute) and a BCA assay kit (BCAP-1-W, Suzhou Comin Biotechnology Co., Ltd.) according to the manufacturer’s user manual.
Extraction and Measurement of Amino Acid Content
Sample Preparation
The leaf and root samples (0.20 g) were accurately weighed and placed in the sample bottle (12 mL), into which 10 mL 6 mol/L hydrochloric acid (containing 0.1% phenol) was added. The mixed solution was homogenized by ultrasound. The samples were hydrolyzed in the oven at 110°C for 24 h. After cooling, the filtrate was mixed and filtered by a 0.45 μm water membrane. One milliliter of the filtrate was put into a rotary evaporator and dried at 80°C. Finally, 2.00 mL 0.1 mol/L hydrochloric acid was added to evaporated samples in the rotary evaporator and then mixed evenly by the whirlpool and transferred to the sample bottle for further use.
Standard Curve Development
Seventeen amino acid standards including aspartic acid (Asp), glutamic (Glu), histidine (His), serine (Ser), arginine (Arg), glycine (Gly), threonine (Thr), proline (Pro), alanine (Ala), valine (Val), methionine (Met), cysteine (Cys), isoleucine (Ile), leucine (leu), phenylalanine (Phe), lysine (Lys), and tyrosine (Tyr) were calculated and accurately weighed in a volumetric flask. The mixed standard was diluted using 0.1 mol/L hydrochloric acid to obtain a final concentration of 500 mg/L of each amino acid. An appropriate amount of 500 mg/L mixed standard was diluted to make 10, 25, 50, 100, 150, 200, 300, and 400 mg/L mixed standard solution. The gradient elution procedures are shown in Table 1.
Aminoacyl Derivatization
Three solutions including 100 μL mixed standards, 200 μL buffer solution (0.5 mol/L sodium bicarbonate solution, 0.5 mol/L sodium carbonate solution, pH value = 9.0), and 100 μL 2,4-dinitrochlorobenzene (100 mg/mL) were swirly mixed and then reacted at 90°C for 90 min in dark. Thereafter, 50 μL of 10% acetic acid solution and 550 μL ultrapure water were added to 1.00 mL. The mixed solution was swirled and mixed and then filtered with 0.45-μm organic film and filter liquor prepared for measuring.
Chromatographic Analysis
An high-performance liquid chromatography (HPLC, Agilent 1200, United States) equipped with a C18 column (4.6 mm × 250 mm, 5 μm; Kromat Universil, catalog no. 35D5) and a diode array detector was used. The column temperature was 40°C. Mobile phase A was pure acetonitrile, and mobile phase B was acetic acid – sodium acetate buffer solution (2.50 g/L sodium acetate, 1.17 mL/L glacial acetic acid, 1.50 mL/L triethylamine, pH value = 5.25 ± 0.05). The flow rate was 1 mL/min. The sample injection amount was 10 μL with 10- to15-min intervals. The detection wavelength was 360 nm.
Statistical Analyses
All data were processed using analysis of variance (ANOVA) in triplicate in Excel 2003 and IBM SPSS Statistics 17.0 (IBM Corp., Armonk, NY, United States). Duncan’s new multiple range (DMR) test at a 5% probability level was used to test the differences among the means. Significant differences were labeled based on DMR.
Results
Exogenous Melatonin Promotes Wheat Germination Trait
The effect of MT on wheat germination rate under salt stress is shown in Figure 1. The germination rate of CK reached the highest value on the third day and then showed a stable performance. The germination rate of wheat treated with NaCl increased rapidly in the first 3 days and then showed differences: JM22 increased steadily, and SM15 and ZM 18 were basically stable. The germination rate of wheat decreased significantly under salt stress, especially for Jimai22, which was 11.09% lower than CK (p < 0.05), but there was no significant difference between Shimai 15 and Zhoumai18.
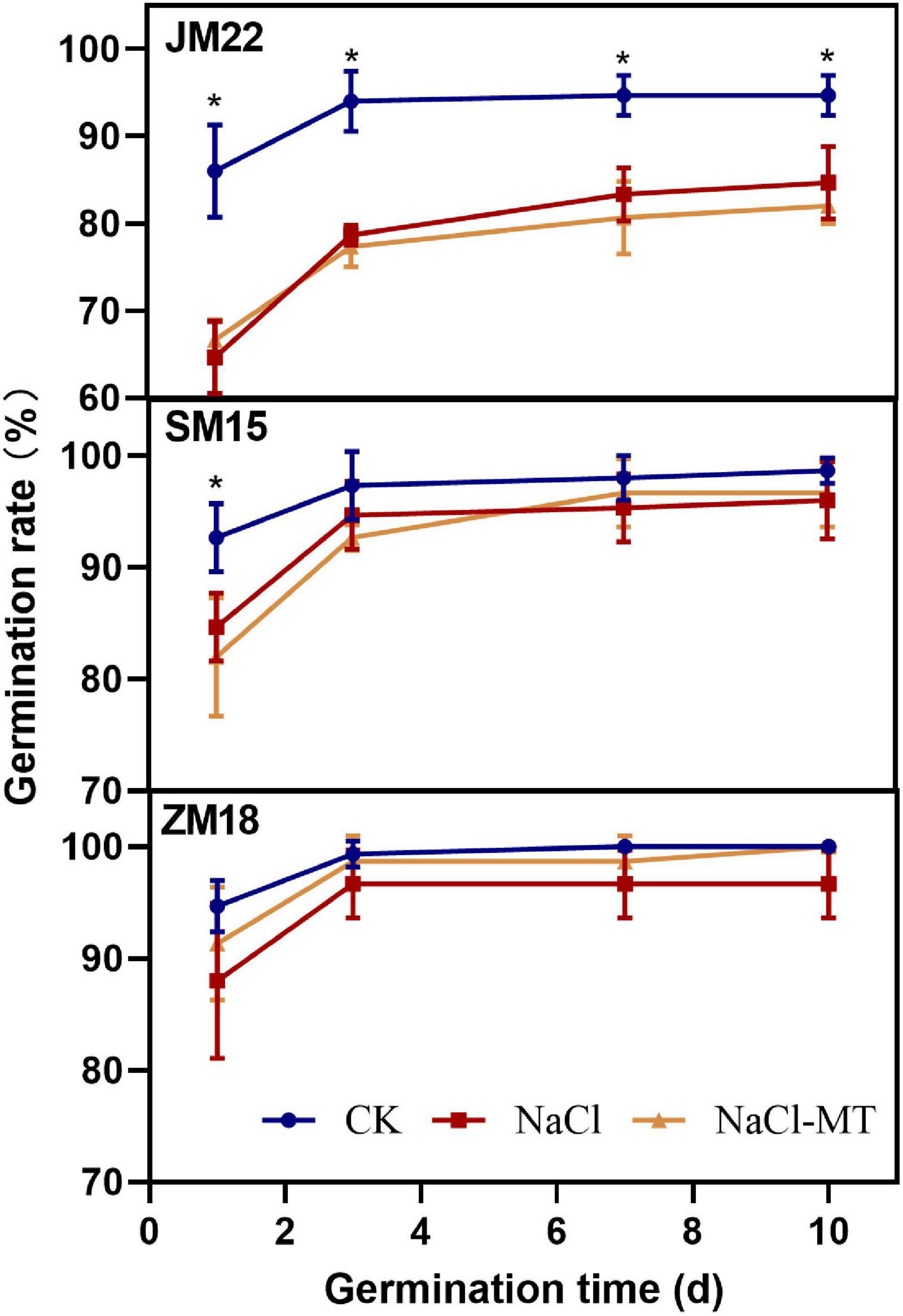
Figure 1. Effects of MT on germination trait under salt stress. For each trait, asterisks represent significantly different according to Duncan’s test at a p < 0.05 threshold.
Exogenous Melatonin Inhibits Root Length and Germ Length and Promotes Radicle Number
Radicle number, root length, and germ length were also affected by salt stress significantly (Table 2). NaCl stress significantly decreased the length of germ and root of JM22, SM15, and ZM18 by 23.99 and 40.76, 27.97 and 44.79, and 16.79 and 33.43%, respectively. The radicle number did not change significantly under the NaCl treatment than that under CK. MT application further significantly decreased the length of germ and root of JM22, SM15, and ZM18 by 30.98 and 68.69, 45.41 and 75.29, and 37.66 and 70.27%, respectively. Meantime, the radicle number was significantly increased under NaCl-MT than the other two treatments.
Melatonin Application Relieve Inhibited Effect of NaCl on Shoot and Root Length
Figure 2 shows that shoot and root lengths of JM22 and ZM18 were both decreased significantly under NaCl stress compared with CK. MT application increased those indexes to some degrees, in which the shoot length of ZM18 and root length of JM22 were significantly increased under NaCl treatment than those under the NaCl-MT. Additionally, shoot and root lengths of ZM18 did not change significantly under NaCl and NaCl-MT treatments. These results indicated that SM15 showed higher salt resistance compared with the other two varieties.
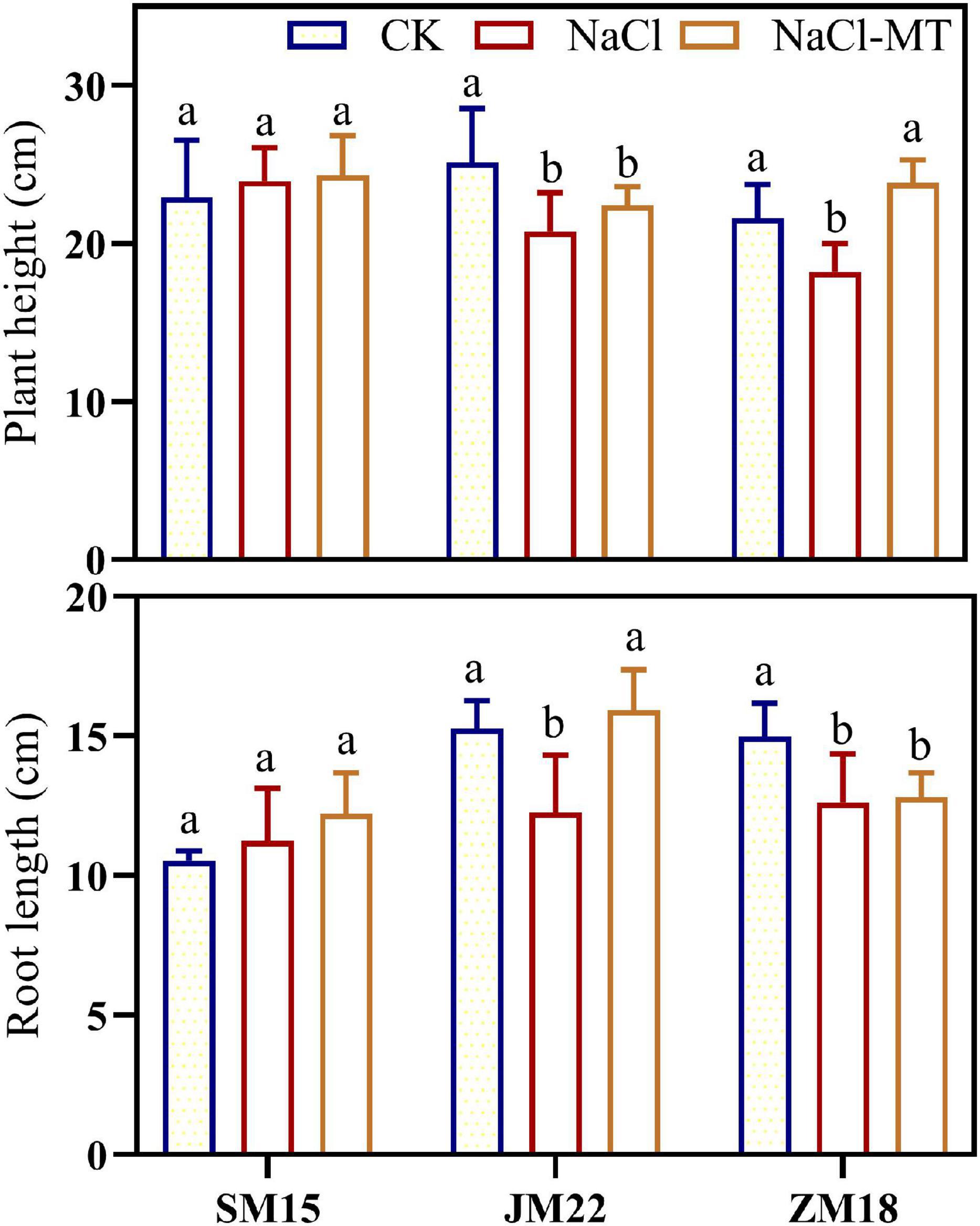
Figure 2. Effects of MT on plant height and root length under salt stress. For each trait, bars with the same letter are not significantly different according to Duncan’s test at a p < 0.05 threshold.
Melatonin Application Increased Water Content in Plant Under NaCl Stress
Table 3 shows that fresh weight, dry weight, and water content in root, stem, and leaf of JM22 and ZM18 significantly decreased under NaCl stress. MT application had increased those indexes to the level of CK. However, for SM15, the fresh weight, dry weight, and water content in plants did not change significantly under the NaCl and NaCl-MT treatments compared with CK. These results also indicated that SM15 contained a good ability on salt resistance.
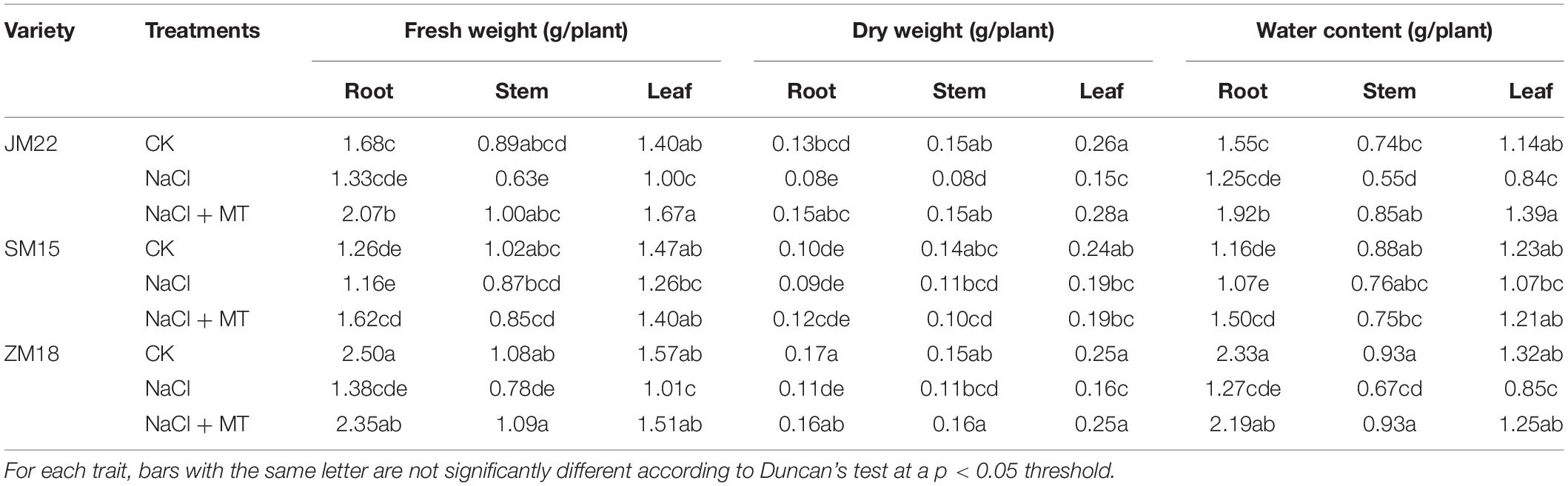
Table 3. Effects of MT on fresh weight and dry weight of root, stem, and leaf of wheat under salt stress.
Melatonin Application Regulates Na+, K+, Ca2+ Compartmentation, and [K+]/[Na+] at Root and Leaf of Plant
Table 4 shows that K+, Ca2+, and Na+ contents in different organelles and varieties followed different changing patterns. Under NaCl stress, compared with CK, Na+ content significantly increased in root, stem, and leaf of three wheat varieties; K+ and Ca2+ contents were both significantly decreased in root and stem (Ca2+ content in the stem of SM15 and ZM18 exception); when K+ content increased, Ca2+ contents decreased significantly in the leaf of JM 22, but Ca2+ increased in the leaf of SM15; both K+ and Ca2+ in leaf were not obviously changed in ZM18. NaCl-MT treatment significantly decreased Na+ contents in root compared with that treated by NaCl alone, but increased in stem and leaf. This suggested that MT took effect first in the root, a higher Na+ concentration formed in stem and leaf. Also, MT application further decreased K+ content in stem and leaf and increased significantly Ca2+ content in the leaf of JM22; it decreased K content in the leaf of SM15 and decreased significantly K+ content in stem and increased Ca2+ in root and leaf of ZM18. [K+]/[Na+] ratio in root and stem + leaf was significantly decreased under NaCl stress compared with CK. MT application could increase [K+]/[Na+] ratio in the root, but decrease this value in stem + leaf.
Melatonin Application Enhance Activity of Antioxidant Enzymes
Figure 3 shows that NaCl stress-induced increase of activity for SOD, POD, and CAT in different degrees compared with CK. MT application could enhance further the activity of SOD and CAT in the leaves of three wheat varieties under NaCl treatments; POD activity was recovered to the level of CK. It is noted that SOD activity of JM22 was significantly decreased under NaCl stress, which was possibly explained by the fact that JM22 was salt-sensitive and biochemical characteristics experienced serious damage.
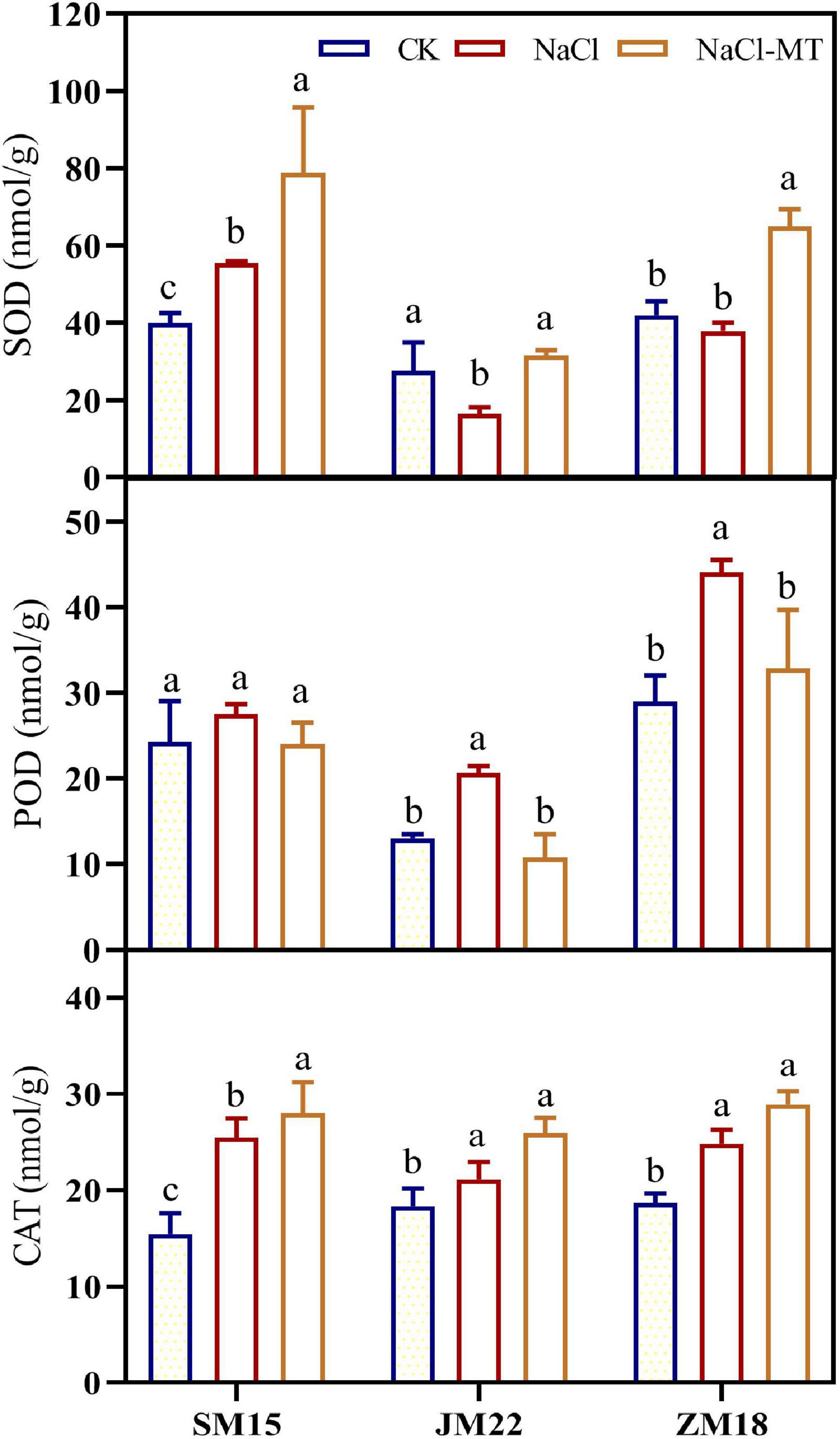
Figure 3. Effects of MT on superoxide dismutase (SOD), peroxidase (POD), and catalase (CAT) under salt stress. For each trait, bars with the same letter are not significantly different according to Duncan’s test at a p < 0.05 threshold.
Melatonin Application Decreased Malondialdehyde Content and Increased Proline Content
As shown in Figure 4, MDA content and proline content in the leaves of three wheat varieties were both increased under NaCl stress. MT application decreased MDA content under NaCl condition, suggesting that the cell membrane structure had been improved significantly. Additionally, MT further increased the proline content in the leaf of SM15 significantly under NaCl + MT compared with that under the NaCl treatment alone.
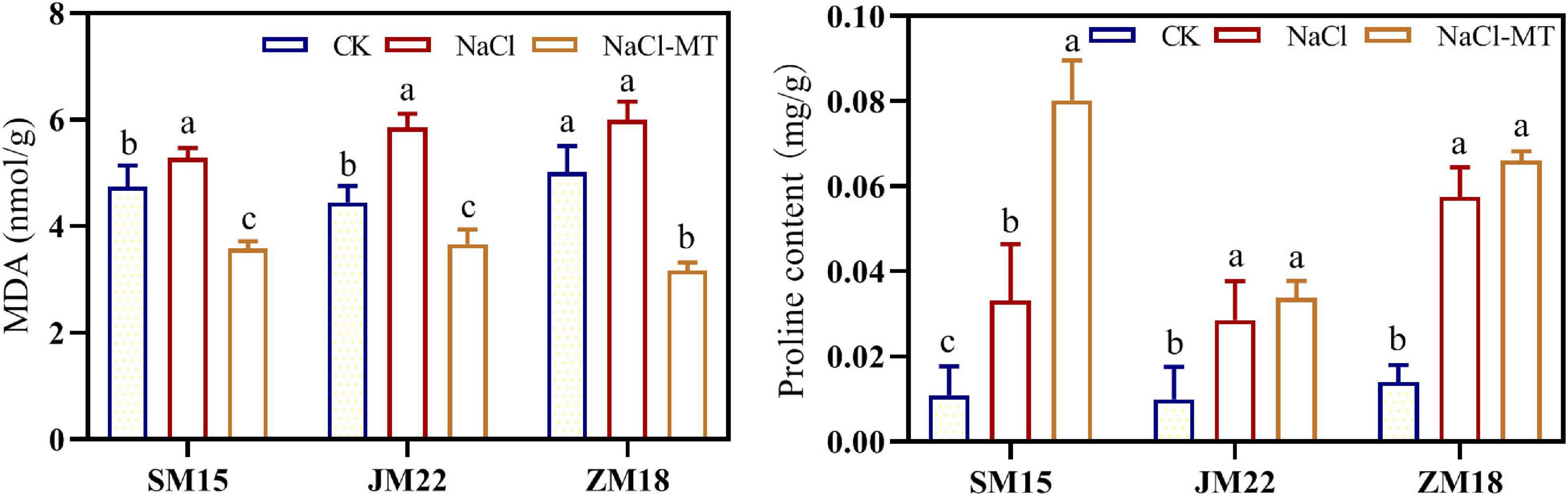
Figure 4. Effects of MT on malondialdehyde (MDA) and proline content under salt stress. For each trait, bars with the same letter are not significantly different according to Duncan’s test at a p < 0.05 threshold.
Melatonin Application Increased the Contents of Soluble Protein and Soluble Sugar
As shown in Figure 5, the contents of soluble protein and soluble sugar were decreased by different degrees under NaCl stress compared with CK. MT application significantly increased the contents of soluble protein and soluble sugar, which helped plants keep good water status under salt stress. Soluble protein content in the leaf of ZM18 did not change significantly under different treatments.
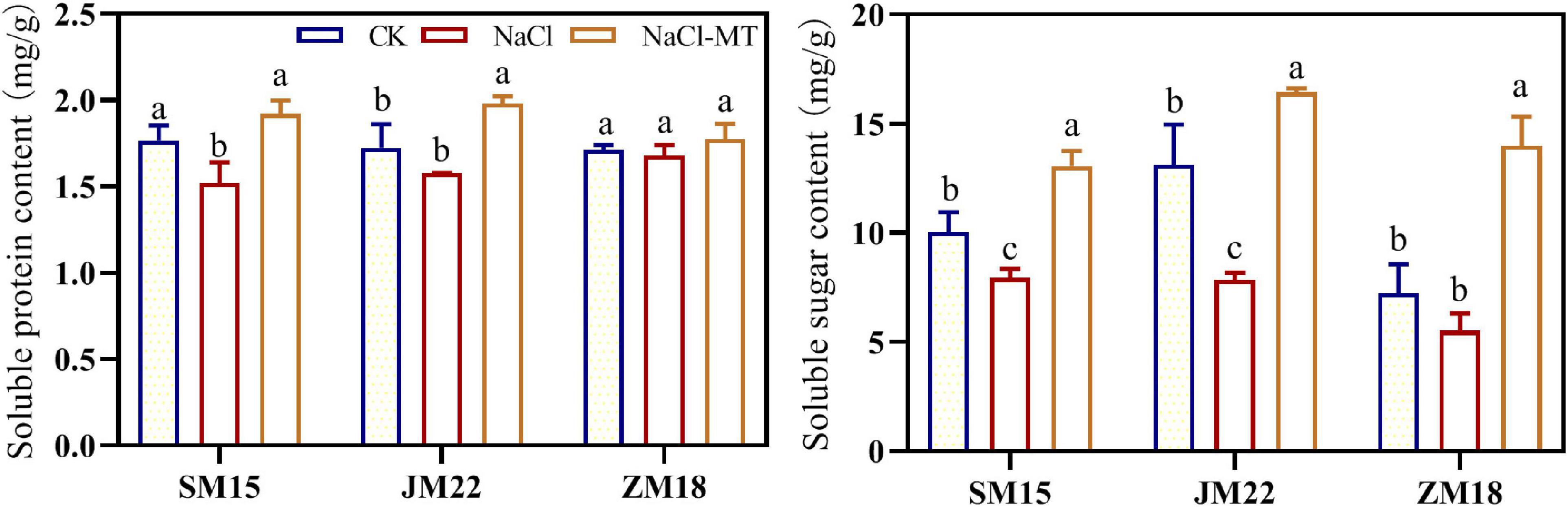
Figure 5. Effects of MT on soluble protein and sugar content under salt stress. For each trait, bars with the same letter are not significantly different according to Duncan’s test at a p < 0.05 threshold.
Melatonin Application Increased the Root Vigor Under NaCl Stress
Figure 6 shows that the root vigor of JM22 and ZM18 decreased significantly under NaCl stress compared with CK. However, the root vigor of SM15 was increased significantly under NaCl conditions, suggesting higher salt resistance for this variety. MT application had increased the root vigor of JM22 and ZM18 significantly to alleviate the salt damage.
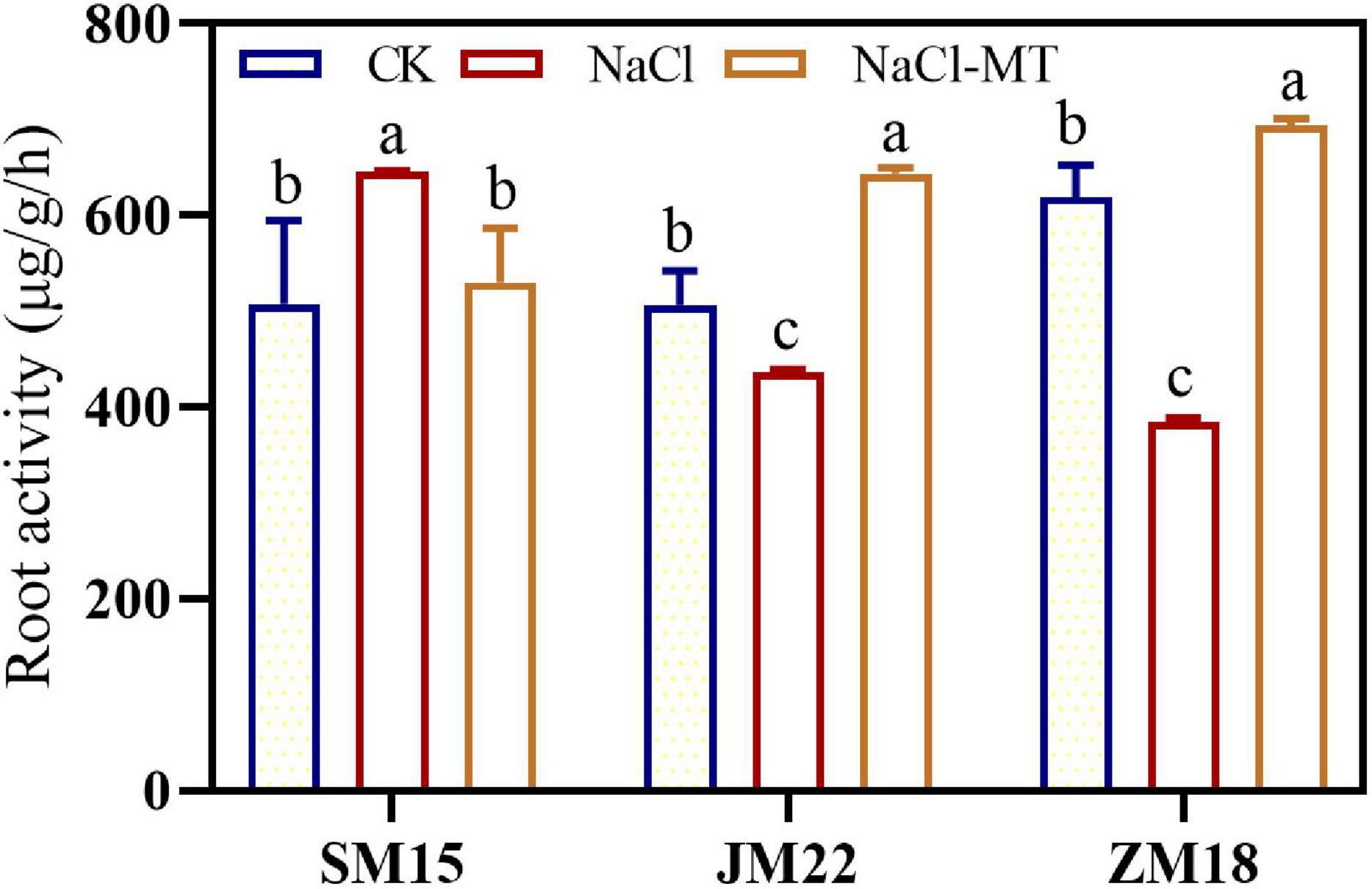
Figure 6. Effects of MT on root activity under salt stress. For each trait, bars with the same letter are not significantly different according to Duncan’s test at a p < 0.05 threshold.
Melatonin Application Decreased H2O2 Content in Root and Displayed Less H2O2 and O2– Accumulation in Root
Figure 7A shows that H2O2 contents in root of JM22, SM15, and ZM18 were significantly higher under NaCl stress than that under CK by 23.74, 33.89, and 20.24%, respectively. MT application reduced H2O2 contents in the roots of three varieties under NaCl stress to the level of CK. H2O2 and O2– accumulation in roots of three varieties with three treatments were visualized by DAB and NBT staining. As expected, the detached roots of MT-treated wheat seedlings displayed less H2O2 and O2– accumulation than those of NaCl-treated seedlings (Figure 7B). However, H2O2 contents in the leaf of JM22, SM15, and ZM18 were decreased significantly compared with CK. MT application maintained a low level of H2O2 contents in the leaves of three varieties.
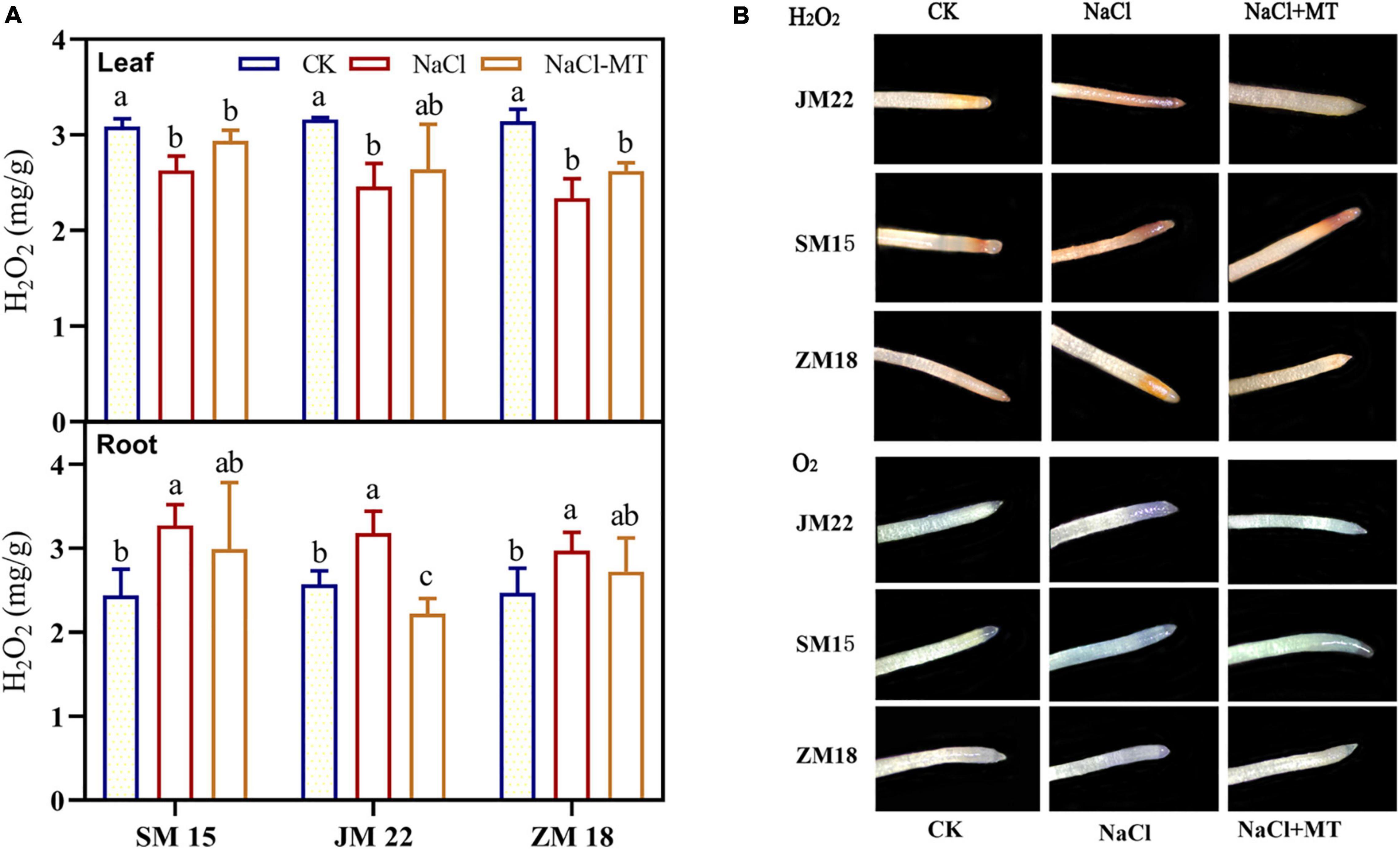
Figure 7. Effects of MT on hydrogen peroxide (H2O2) content in plant (A) and H2O2 and upper-oxide anions (O2–) distribution in root (B) under salt stress. For each trait, bars with the same letter are not significantly different according to Duncan’s test at a p < 0.05 threshold.
Melatonin on Cluster Heatmap of Amino Acid Content of Wheat Seedlings Under Salt Stress
Figure 8 shows the variations in amino acid content in plants of three wheat varieties under different treatments. Principal component analysis extracted two major components that together accounted for 97.0% of the variance in the dataset. Principal component 1 (PC1, x-axis) explained 94.0% of the variation among the individual samples, and principal component 2 (PC2, y-axis) explained 3.0% of the variation. Under NaCl stress, the amino acid content in the roots and leaves of three cultivars shifted greatly along PC1 axis (Figure 8A). Glu content showed high content, but Met, Cys, His, and Tyr content were low in plants. Cys and Met content in the root of JM 22 and Cys content in the root of SM15 and ZM18 decreased under salt stress, but increased when MT application. Lys and Tyr content in leaf both increased under salt stress and further increased with MT application; His content in leaf increased significantly under both NaCl and NaCl-MT treatments. Other amino acids increased under NaCl and decreased under NaCl-MT without recovering to the level of control (Figure 8B and Supplementary Table 1).
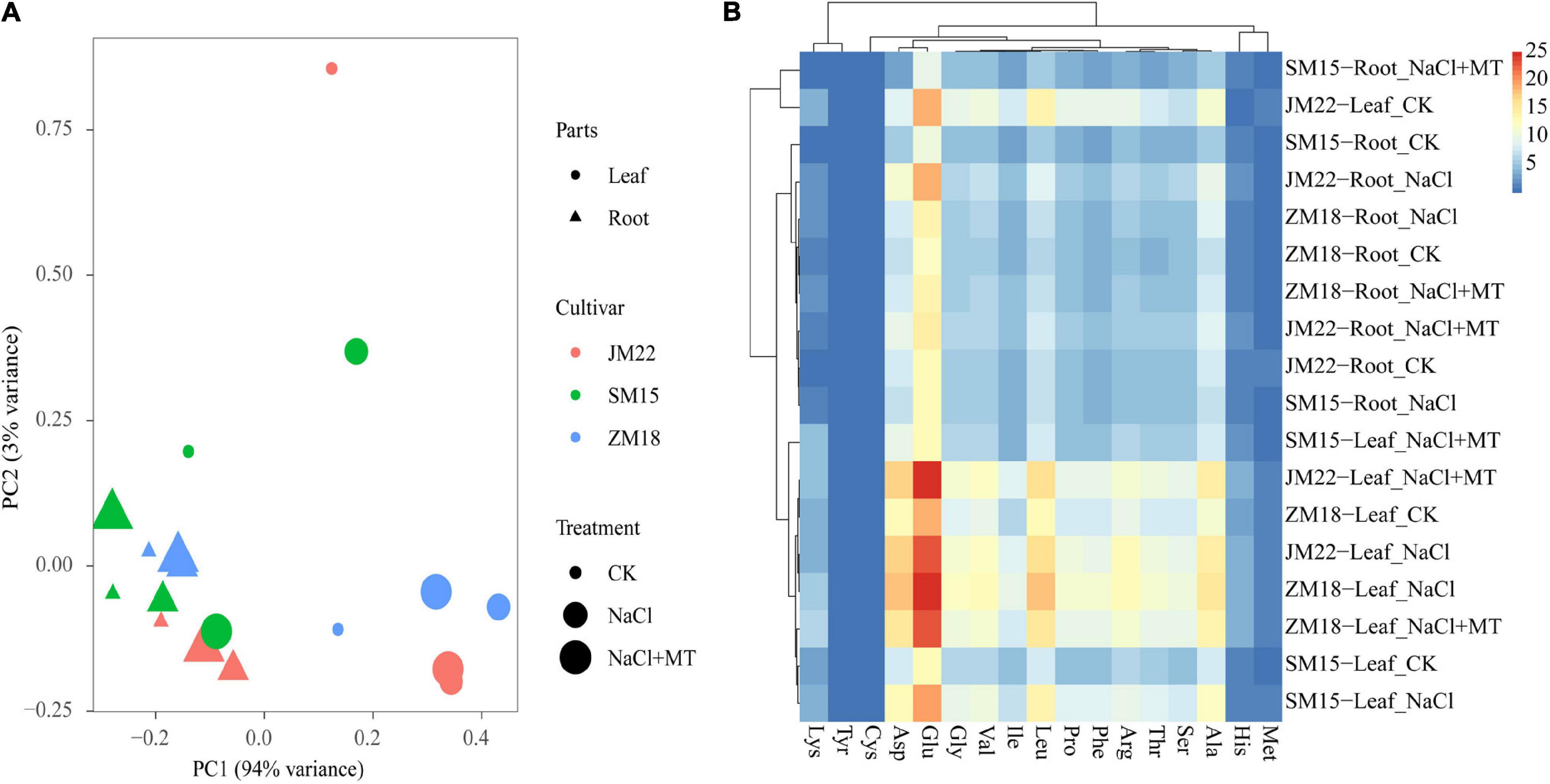
Figure 8. The principal component analysis (PCA) (A) and cluster heatmap (B) of amino acid content of wheat seedlings under different treatments. Asp, aspartic acid; Glu, glutamic; His, histidine; Ser, serine; Arg, arginine; Gly, glycine; Thr, threonine; Pro, proline; Ala, alanine; Val, valine; Met, methionine; Cys, cysteine; Ile, isoleucine; Leu, leucine; Phe, phenylalanine; Lys, lysine; Tyr, tyrosine.
Discussion
Salinity, one of the major abiotic stresses, limits the growth and productivity of many field crops. For increasingly extreme climate events, it is becoming the most important scientific research and agricultural practices in improving the salt tolerance of crops and exploiting arable areas of saline soils (Zhang et al., 2011; Himabindu et al., 2016). MT plays significant roles in antisenescence and antistress (Arnao and Hernández-Ruiz, 2014). It is well documented that exogenous MT can also improve the salinity resistance of wheat seedlings (Ke et al., 2018; Liu et al., 2020). During seed germination, exogenous MT (20 and 1 μM) pretreatment enhanced the germination rate of cotton, wheat, cucumber, and so on (Zhang N. et al., 2014; Ke et al., 2018; Chen et al., 2021). In our result, however, the germination rate showed somewhat improvement on ZM18 and SM15, no improvement on JM22, and furtherly inhibited the length of radical and germ under salt stress (Table 2 and Figure 9). On the one hand, different MT concentrations for different crops might be partly reasons. However, concentration was not the main and only reason because MT (0–500 μM) pretreatment recovered root vigor and growth of two maize varieties and decreased relative electrolytic leakage in roots and leaves during the period of seed germination and seedling cultivation under NaCl stress (Li X. et al., 2017). This is likely for different treatment modes, plant pretreated with MT often shows better stress resistance even under lower concentrations, but MT was added directly to the culture solution especially in high concentrations, the seed germination was inhibited, and the growth of radical and radicle appeared retardation because plants would trigger a defensive response as external stress elements. On the other hand, the radicle number increased significantly under salt and MT condition, which suggested that MT promoted lateral root produced and growth of plants under NaCl stress. This result was consistent with the newest report that MT promotes lateral root under salt stress (Javeed et al., 2021).
Exogenous MT partially mitigated the salt stress-induced inhibition of whole-plant growth by reducing the accumulation of H2O2 in wheat leaf and increasing endogenous MT content and polyamine contents (Ke et al., 2018). Rice and cotton plants pretreated with MT also reduced H2O2 contents in leaf and roots, which accumulate high H2O2 concentration induced by salt stress (Li X. et al., 2017; Jiang et al., 2021). In our research, the changing rule of H2O2 content in root was also decreased with salt and MT treatments compared with NaCl alone treatment. However, H2O2 content in wheat leaf was significantly decreased under salt stress alone compare with CK, and MT application did not significantly recover H2O2 content to the level of control (Figure 7). The possible reason was that roots receive stress signals to turn on the defense system including recognizing and appointing exogenous MT as a stress regulator in a short time; for leaf, there was delayed effects for that MT has been reported as a potent long-distance signal to translocate stress message from roots to leaf (Li H. et al., 2017). Whether seeds of rice and wheat were pretreated with MT for 3–7 days, or the cotton seedling was treated with foliar spray MT every 24 h for 12 days, plants have been finished antistress training (Li X. et al., 2017; Ke et al., 2018; Jiang et al., 2021). Besides playing a vital signaling role during stress responses, H2O2 was induced by respiratory burst oxidase homologs (RBOH) to convert eventually into other ROS (OH) by the Fenton reaction and Haber–Weiss reaction (Liu et al., 2020; Michard and Simon, 2020). Additionally, MT treatment enhanced significantly the activities of antioxidant enzymes (especially SOD and CAT) and decreased MDA content in leaves resulted in a decrease in H2O2 level, which was conformed to this conclusion of Li X. et al. (2017). Not only does MT act as an endogenous antioxidant that enhanced the antioxidant capacity, but also MT was applied exogenously to modulate subcellular antioxidant systems in barley (Li et al., 2016). The possible response mechanism is still unknown comprehensively considering systematic reaction and crosstalk between organs.
Another effective strategy to resist salt stress employed by plants is to keep ion homeostasis and relieve ionic toxicity. One important approach that increases salt tolerance is to maintain a high level of [K+]/[Na+] ratio in cells (Flowers and Läuchli, 1983). Many of glycophytes are subjected to NaCl stress which showed that Na+ in roots and leaves significantly increased, and K+ content clearly decreased compared with those of the control (Li X. et al., 2017; Ren et al., 2020). Na+ is sensed possibly by Na+ sensor glucuronosyltransferase, activates Ca2+ channels, and increases Ca2+ influx into the cytosol (Jiang et al., 2019). In our study, MT application significantly decreased Na+ contents, increased [K+]/[Na+] ratio in root induced by NaCl stress alone, but further increased Na+ content, and decreased [K+]/[Na+] in stem and leaf. This suggested that MT may take ameliorating effect first on the root, and meantime, a higher Na ion concentration formed along with nutrient solution flows and circulates in stem and leaf. It is known that the ion transport system including ionic equilibrium of [K+] and [Na+] and [K+]/[Na+] is often considered in a signaling network involving H2O2 and Ca2+. Kaya et al. (2019) reported that MT treatments increase plant growth attributes to increased Ca2+ and K+ in the leaves and reduced MDA, H2O2 in Cd-stressed wheat plants. MT also helps cold-stress plants that possessed higher Ca2+-ATPase, which are important for the ATP formation (Sun et al., 2018). In our results, SM15 also showed a higher Ca2+ keeping ability in stem and leaf under salt stress alone; ZM 18 was more easily adjusted by MT to increase significantly Ca2+ content in root and leaf under salt stress. These results conform to that the increase in Ca2+ content in cytosol that triggers RBOH activity directly induces the formation of H2O2 that is eventually converted into other ROS (Michard and Simon, 2020), which is also interpreted as why H2O2 content decreased in leaves under salt treatment (Figure 7).
Salt stress promoted storage protein degradative, and thus, amino acid content changed accordingly (Zhang et al., 2017). Lysine (Lys) content is decreased with MT and transforms into other substances to raise the level of stress tolerance in wheat seeds during germination under drought stress (Li et al., 2020). MT also accelerates the metabolic flow from the precursor amino acids arginine and methionine to polyamines and decreases the degradation of salt-induced polyamines (Ke et al., 2018). From our study, the consistent results showed that salt stress increased Arg and Met contents, which decreased with MT application to some degrees. Additionally, Cys and Met contents in root of JM 22 and Cys content in the root of SM15 and ZM18 decreased under salt stress, but increased when MT application. This suggested that Cys is likely to participate in the H2S-Cys cycle, which enhances its roles in the regulation of the antioxidant system (Huang et al., 2021). In our study, Lys and Tyr contents in leaf both increased under salt stress and further increased with MT application; His content in leaf increased significantly under both NaCl and NaCl-MT treatments. Other amino acids increased under NaCl and decreased under NaCl-MT without recovering to the level of control. It is known that MT often helps to induce amino acid accumulation in root and leaf to enhance the cellular osmotic potential (Cui et al., 2018). Lysine has been reported to be transformed into proline, aminobutyric acid, and polyamines during drought resisting processes (Hare and Cress, 1997; Klessig et al., 2000). So, our results also provided the evidence that MT increases the ability for the leaves of different wheat varieties to keep high water status and accumulate high soluble protein, soluble sugar, and proline content under NaCl stress (Table 3 and Figures 4, 5). On the other hand, lysine residues on histone terminus are deacetylated by histone deacetylase 14, which is involved in the biosynthesis of MT in Arabidopsis thaliana (Zhao et al., 2018). Both Lys and His contents increased in leaf under NaCl and NaCl + MT which indicated a complex biochemical process, during which the MT content changing the interaction between MT and amino acid, and involved mechanism would be the next important research point.
It is noted that MT application methods (pretreated coating or soaking, leaf pray, root or rhizospheric application, mixed application with other growth regulators) and varied experimental elements (MT concentration, plant species, varieties, and adversity types) appear to have different regulatory effects during stress-resistance process (Kostopoulou et al., 2015; Jiang C. et al., 2016; Li X. et al., 2017). In our research, different wheat varieties showed different salt-sensitivities. JM22, a widely adaptive super-high-yield variety, was not salt-resistant and shows an obvious decrease on germination rate and activity of SOD and CAT under NaCl stress. SM15 and ZM18 showed higher salt-resistant, considering that SOD activity of SM15 did not decrease but increased significantly and higher germination rate and proline accumulation under NaCl stress alone. MT at a concentration of 300 μmol L–1 played roles as regulated antioxygen to keep the physiological equilibrium of wheat seedling and to promote germination and lateral roots, but it took an inhibitory effect on the length of radicle and germ. The optimal concentration of MT on wheat seed germination and growth of wheat seedling and the dominant varieties of wheat for coping with salt stress should be further researched for meeting future production reality needs.
Conclusion
Exogenous MT promoted germination rate of SM15 and ZM 18 recovering control level under salt stress, but had no effect on that of JM22. SM15 and ZM 18 showed higher salt resistance than that of JM22, especially for SM15 that had higher proline content, root vigor, and higher antioxygen system under salt stress. JM22 was salt-sensitive. MT at 300 ’μmol L–1 inhibited the length of germ and radicle, but promoted lateral root production of seed embryo, and increased shoot and root length of wheat seedling under NaCl stress. Salt stress-induced antioxygen system activity enhancing and H2O2 content increasing, decreased soluble protein, soluble sugar content, [K+]/[Na+] in leaf and root vigor, and increased Na+ content in root and leaf. MT application could increase proline content, soluble protein, soluble sugar, Ca2+ content, and vital amino acid content in leaf to keep high water status and maintain a low level of H2O2 content and [K+]/[Na+] ratio in leaf. MT increased root vigor, [K+]/[Na+] ratio, and decreased H2O2 content in root induced by salt stress. The findings of this study showed that the root as the first defense organization responded to salt stress was rapidly and earlier than the leaf in a short time (24 h), and the signal transmission and interaction between roots and leaf maintained to reduce stress injury as much as possible. So, in wheat production, the optimal concentration, varieties, and MT application method should be considered comprehensively at the sowing and seedling stages.
Data Availability Statement
The raw data supporting the conclusions of this article will be made available by the authors, without undue reservation.
Author Contributions
DL and RL initiated and designed the research. ZZ, HL, XF, and SZ performed the experiments and collected the data. XZ, NY, and JS wrote the code and tested the methods. LL, ZZ, and DL analyzed the data and wrote the manuscript. All authors contributed to the article and approved the submitted version.
Funding
This work was supported by the Hebei Province Natural Science Foundation for Youth (C2019204358), the Scientific Research Project of Hebei Education Department (QN2019046), the National Natural Science Foundation of China (no. 31871569), the Science and Technology Program of Baoding (1911ZN010), the National System of Modern Agriculture Industrial Technology Project (CARS-03-05), and the National Key Research and Development Program of China (2017YFD0300909 and 2021YFD1901004-2).
Conflict of Interest
The authors declare that the research was conducted in the absence of any commercial or financial relationships that could be construed as a potential conflict of interest.
Publisher’s Note
All claims expressed in this article are solely those of the authors and do not necessarily represent those of their affiliated organizations, or those of the publisher, the editors and the reviewers. Any product that may be evaluated in this article, or claim that may be made by its manufacturer, is not guaranteed or endorsed by the publisher.
Acknowledgments
The authors thank Yifen Wang, Auburn University, for his linguistic assistance during the preparation of this manuscript. The authors are grateful to Zhilian Liu, Yong Zhao, and Ruihui Wang of Hebei Agricultural University provided wheat seeds.
Supplementary Material
The Supplementary Material for this article can be found online at: https://www.frontiersin.org/articles/10.3389/fpls.2021.787062/full#supplementary-material
References
Abbas, G., Saqib, M., Akhtar, J., and Haq, M. (2015). Interactive effects of salinity and iron deficiency on different rice genotypes. J. Plant Nutr. Soil Sc. 178, 306–311. doi: 10.1002/jpln.201400358
Ahmed, I. M., Nadira, U. A., Bibi, N., Cao, F., He, X., Zhang, G., et al. (2015). Secondary metabolism and antioxidants are involved in the tolerance to drought and salinity, separately and combined, in Tibetan wild barley. Environ. Exp. Bot. 111, 1–12. doi: 10.1016/j.envexpbot.2014.10.003
Al-Huqail, A. A., Khan, M. N., Ali, H. M., Siddiqui, M. H., Al-Humaid, A. A., AlZuaibr, F. M., et al. (2020). Exogenous melatonin mitigates boron toxicity in wheat. Ecotox. Environ. Safe. 201:110822. doi: 10.1016/j.ecoenv.2020.110822
Arnao, M. B., and Hernández-Ruiz, J. (2014). Melatonin: plant growth regulator and/or biostimulator during stress? Trends Plant Sci. 19, 789–797. doi: 10.1016/j.tplants.2014.07.006
Cai, S. Y., Zhang, Y., Xu, Y. P., Qi, Z. Y., Li, M. Q., Ahammed, G. J., et al. (2017). Hsfa1a upregulates melatonin biosynthesis to confer cadmium tolerance in tomato plants. J. Pineal Res. 62:e12387. doi: 10.1111/jpi.12387
Chen, L., Lu, B., Liu, L., Duan, W., Jiang, D., Li, J., et al. (2021). Melatonin promotes seed germination under salt stress by regulating ABA and GA3 in cotton (Gossypium hirsutum L.). Plant Physiol. Bioch. 162, 506–516. doi: 10.1016/j.plaphy.2021.03.029
Chen, Y. E., Mao, J. J., Sun, L. Q., Huang, B., Ding, C. B., Gu, Y., et al. (2018). Exogenous melatonin enhances salt stress tolerance in maize seedlings by improving antioxidant and photosynthetic capacity. Physiol. Plantarum 164, 349–363. doi: 10.1111/ppl.12737
Chen, Z., Xie, Y., Gu, Q., Zhao, G., Zhang, Y., Cui, W., et al. (2017). The Atrbohf-dependent regulation of ROS signaling is required for melatonin-induced salinity tolerance in Arabidopsis. Free Radic. Biol. Med. 108, 465–477. doi: 10.1016/j.freeradbiomed.2017.04.009
Cui, G., Sun, F., Gao, X., Xie, K., Zhang, C., Liu, S., et al. (2018). Proteomic analysis of melatonin-mediated osmotic tolerance by improving energy metabolism and autophagy in wheat (Triticum aestivum L.). Planta Daninha 248, 1–19. doi: 10.1007/s00425-018-2881-2
Dou, X., Shi, H. B., Miao, Q. F., Tian, F., Yu, D. D., Zhou, L. Y., et al. (2019). Spatial-temporal variation characteristics of soil water and salt and the influence of groundwater depth on soil salinity in salinized irrigation area. J. Soil Water Conserv. 3, 246–253.
Elsayed, A. I., Rafudeen, M. S., Gomaa, A. M., and Hasanuzzaman, M. (2021). Exogenous melatonin enhances the ROS metabolism, antioxidant defense-related gene expression and photosynthetic capacity of phaseolus vulgaris L. to confer salt stress tolerance. Physiol. Plant. 173, 1369–1381. doi: 10.1111/ppl.13372
Flowers, T. J., and Läuchli, A. (1983). Sodium versus potassium: substitution and compartmentation. Plant Physiol. 15, 651–681.
Gao, Q. H., Jia, S. S., Miao, Y. M., Xiao-Min, L. U., and Hui-Min, L. I. (2016). Effects of exogenous melatonin on nitrogen metabolism and osmotic adjustment substances of melon seedlings under sub-low temperature. J. Appl. Ecol. 27, 519–524.
Hare, D., and Cress, W. A. (1997). Metabolic implications of stress-induced proline accumulation in plants. J. Plant Growth Regul. 21, 79–102.
Harper, R. J., Dell, B., Ruprecht, J. K., Sochacki, S. J., and Smettem, K. (2021). “Salinity and the reclamation of salinized lands,” in Soils and Landscape Restoration, ed. E. Thomson (Cambridge, MA: Academic Press), 193–208. doi: 10.1016/b978-0-12-813193-0.00007-2
Himabindu, Y., Chakradhar, T., Reddy, M. C., Kanygin, A., Redding, K. E., and Chandrasekhar, T. (2016). Salt-tolerant genes from halophytes are potential key players of salt tolerance in glycophytes. Environ. Exp. Bot. 124, 39–63. doi: 10.1111/pce.13907
Huang, D., Huo, J., and Liao, W. (2021). Hydrogen sulfide: roles in plant abiotic stress response and crosstalk with other signals. Plant Sci. 302:110733. doi: 10.1016/j.plantsci.2020.110733
Huangfu, L., Zhang, Z., Zhou, Y., Zhang, E., Chen, R., Fang, H., et al. (2021). Integrated physiological, metabolomic and transcriptomic analyses provide insights into the roles of exogenous melatonin in promoting rice seed germination under salt stress. Plant Growth Regul. 95, 19–31. doi: 10.1007/s10725-021-00721-9
Javeed, H. M. R., Ali, M., Skalicky, M., Nawaz, F., Qamar, R., Rehman, A. Ur, et al. (2021). Lipoic Acid Combined with Melatonin Mitigates Oxidative Stress and Promotes Root Formation and Growth in Salt-Stressed Canola Seedlings (Brassica napus L). Molecules 26, 3147–3147. doi: 10.3390/molecules26113147
Jiang, C., Cui, Q., Feng, K., Xu, D., Li, C., and Zheng, Q. (2016). Melatonin improves antioxidant capacity and ion homeostasis and enhances salt tolerance in maize seedlings. Acta Physiol. Plant 38, 1–9.
Jiang, D., Lu, B., Liu, L., Duan, W., Meng, Y., Li, J., et al. (2021). Exogenous melatonin improves the salt tolerance of cotton by removing active oxygen and protecting photosynthetic organs. BMC. Plant Biol. 21:331. doi: 10.1186/s12870-021-03082-7
Jiang, X., Li, H., and Song, X. (2016). Seed priming with melatonin effects on seed germination and seedling growth in maize under salinity stress. Pak. J. Bot. 48, 1345–1352.
Jiang, Z., Zhou, X., Tao, M., Fang, Y., Liu, L., Wu, F., et al. (2019). Plant cell-surface GIPC sphingolipids sense salt to trigger Ca2 + influx. Nature 572, 341–346. doi: 10.1038/s41586-019-1449-z
Kaya, C., Okant, M., Ugurlar, F., Alyemeni, M. N., Ashraf, M., and Ahmad, P. (2019). Melatonin-mediated nitric oxide improves tolerance to cadmium toxicity by reducing oxidative stress in wheat plants. Chemosphere 225, 627–638. doi: 10.1016/j.chemosphere.2019.03.026
Ke, Q., Ye, J., Wang, B., Ren, J., Yin, L., Deng, X., et al. (2018). Melatonin mitigates salt stress in wheat seedlings by modulating polyamine metabolism. Front. Plant Sci. 9:914. doi: 10.3389/fpls.2018.00914
Klessig, D. F., Durner, J., Noad, R., and Navarre, D. A. (2000). Nitric oxide and salicylic acid signaling in plant defense. P. Natl. A. Sci. 97, 8849–8855. doi: 10.1073/pnas.97.16.8849
Kostopoulou, Z., Therios, I., Roumeliotis, E., Kanellis, A. K., and Molassiotis, A. (2015). Melatonin combined with ascorbic acid provides salt adaptation in Citrus aurantium L. seedlings. Plant Physiol. Biochem. 86C, 155–165. doi: 10.1016/j.plaphy.2014.11.021
Li, D. X., Batchelor, W. D., Zhang, Di, Miao, H. X., Li, H. Y., Song, S. J., et al. (2020). Analysis of melatonin regulation of germination and antioxidant metabolism in different wheat cultivars under polyethylene glycol stress. PLoS One 15:e0237536. doi: 10.1371/journal.pone.0237536
Li, H., Chang, J., Zheng, J., Dong, Y., Liu, Q., Yang, X., et al. (2017). Local melatonin application induces cold tolerance in distant organs of Citrullus lanatus L. via long distance transport. Sci. Rep. 7:40858. doi: 10.1038/srep40858
Li, X., Yu, B., Cui, Y., and Yin, Y. (2017). Melatonin application confers enhanced salt tolerance by regulating Na+ and Cl– accumulation in rice. J. Plant Growth Regul. 83, 441–454.
Li, J., Arkorful, E., Cheng, S., Zhou, Q., Li, H., Chen, X., et al. (2018). Alleviation of cold damage by exogenous application of melatonin in vegetatively propagated tea plant (Camellia sinensis (L.) o. kuntze). Sci. Hortic Ams. Terdam. 238, 356–362.
Li, X., Tan, D. X., Jiang, D., and Liu, F. (2016). Melatonin enhances cold tolerance in drought-primed wild-type and abscisic acid-deficient mutant barley. J. Pineal. Res. 61, 328–339. doi: 10.1111/jpi.12350
Liang, W., Ma, X., Wan, P., and Liu, L. (2018). Plant salt-tolerance mechanism: a review. Biochem. Bioph. Res. Co. 495, 286–291.
Liu, Y., Sun, J., Tian, Z., Hakeem, A., Wang, F., Jiang, D., et al. (2016). Physiological responses of wheat (Triticum aestivum L.) germination to elevated ammonium concentrations: reserve mobilization, sugar utilization, and antioxidant metabolism. Plant Growth Regul. 81, 209–220. doi: 10.1007/s10725-016-0198-3
Liu, J., Zhang, J., Ma, G., Chen, D., and Shabala, S. (2020). Melatonin improves rice salinity stress tolerance by nadph oxidase-dependent control of the plasma membrane K+ transporters and K+ homeostasis. Plant Cell Environ. 43, 2591–2605. doi: 10.1111/pce.13759
Liu, W. J. (2020). Physiological Mechanisms of Heat Shock-Induced Adaption to Aluminum Toxicity in Wheat (Triticum aestivum L.). Doctor’s thesis. Zhejiang: Zhejiang University.
Michard, E., and Simon, A. A. (2020). Melatonin’s antioxidant properties protect plants under salt stress. Plant Cell Environ. 43, 2587–2590. doi: 10.1111/pce.13900
Park, S., Lee, D. E., Jang, H., Byeon, Y., Kim, Y. S., and Back, K. (2013). Melatonin-rich transgenic rice plants exhibit resistance to herbicide-induced oxidative stress. J. Pineal Res. 54, 258–263. doi: 10.1111/j.1600-079X.2012.01029.x
Posmyk, M. M., Kuran, H., Marciniak, K., and Janas, K. M. (2008). Presowing seed treatment with melatonin protects red cabbage seedlings against toxic copper ion concentrations. J. Pineal Res. 45, 24–31. doi: 10.1111/j.1600-079X.2007.00552.x
Rebey, I. B., Bourgou, S., Rahali, F. Z., Msaada, K., Ksouri, R., and Marzouk, B. (2017). Relation between salt tolerance and biochemical changes in cumin (Cuminum cyminum L.) seeds. J. Food Drug Anal. 25, 391–402. doi: 10.1016/j.jfda.2016.10.001
Ren, J., Ye, J., Yin, L., Li, G., Deng, X., and Wang, S. (2020). Exogenous melatonin improves salt tolerance by mitigating osmotic, ion, and oxidative stresses in maize seedlings. Agronomy Basel 10:663. doi: 10.3390/agronomy10050663
Shahi, C., Vibhuti Bargali, K., and Bargali, S. S. (2015). Influence of seed size and salt stress on seed germination and seedling growth of wheat (Triticum aestivum). Indian J. Agr. Sci. 85, 1134–1137.
Shi, H., Jiang, C., Ye, T., Tan, D., Reiter, R. J., Zhang, H., et al. (2015). Comparative physiological, metabolomic, and transcriptomic analyses reveal mechanisms of improved abiotic stress resistance in bermudagrass [Cynodon dactylon (L). Pers.] by exogenous melatonin. J. Environ. Exp. Bot. 66, 681–694. doi: 10.1093/jxb/eru373
Siddiqui, M. H., Alamri, S., Alsubaie, Q. D., Ali, H. M., Ibrahim, A. A., et al. (2019a). Potential roles of melatonin and sulfur in alleviation of lanthanum toxicity in tomato seedlings. Ecotox. Environ. Safe 180, 656–667. doi: 10.1016/j.ecoenv.2019.05.043
Siddiqui, M. H., Alamri, S., Al-Khaishany, M. Y., Khan, M. N., Al-Amri, A., Ali, H. M., et al. (2019b). Exogenous melatonin counteracts NaCl-induced damage by regulating the antioxidant system, proline and carbohydrates metabolism in tomato seedlings. Int. J. Mol. Sci. 20:353. doi: 10.3390/ijms20020353
Siddiqui, M. H., Alamri, S., Nasir Khan, M., Corpas, F. J., Al-Amri, A. A., Alsubaie, Q. D., et al. (2020). Melatonin and calcium function synergistically to promote the resilience through ROS metabolism under arsenic-induced stress. J. Hazard. Mater. 398:122882. doi: 10.1016/j.jhazmat.2020.122882
Sun, C. L., Liu, L. J., Wang, L. X., Li, B. H., Jin, C. W., and Lin, X. Y. (2021). Melatonin: A master regulator of plant development and stress responses. J. Integr. Plant Biol. 63, 126–145. doi: 10.1111/jipb.12993
Sun, L., Li, X., Wang, Z., Sun, Z., Zhu, X., Liu, S., et al. (2018). Cold priming induced tolerance to subsequent low temperature stress is enhanced by melatonin application during recovery in wheat. Molecules 23:1091. doi: 10.3390/molecules23051091
Tripathi, D., Nam, A., Oldenburg, D. J., and Bendich, A. J. (2020). Reactive oxygen species, antioxidant agents, and dna damage in developing maize mitochondria and plastids. Front. Plant Sci. 11:596. doi: 10.3389/fpls.2020.00596
Turk, H., Erdal, S., Genisel, M., Atici, O., Demir, Y., and Yanmis, D. (2014). The regulatory effect of melatonin on physiological, biochemical and molecular parameters in cold-stressed wheat seedlings. J. Plant Growth Regul. 74, 139–152.
Yang, Y., Han, X., Ma, L., Wu, Y., Liu, X., Fu, H., et al. (2021). Dynamic changes of phosphatidylinositol and phosphatidylinositol 4-phosphate levels modulate H+-ATPase and Na+/H+antiporter activities to maintain ion homeostasis in arabidopsis under salt stress. Mol. Plant 14, 2000–2014. doi: 10.1016/j.molp.2021.07.020
Yang, Z., and Wang, B. S. (2015). Present situation of saline soil resources in China and countermeasures for improvement and utilization. Shandong Agr. Sci. 47, 125–130.
Zhang, H. J., Zhang, N., Yang, R. C., Wang, L., Sun, Q. Q., Li, D. B., et al. (2014). Melatonin promotes seed germination under high salinity by regulating antioxidant systems, ABA and GA4 interaction in cucumber (Cucumis sativus L.). J Pineal Res. 57, 269–279. doi: 10.1111/jpi.12167
Zhang, N., Jiang, Q., Li, D. B., Cai, L. T., Zhang, H. J., Si, W. J., et al. (2014). Effects of exogenous melatonin on seed germination and related physiological indexes of Pennisetum alopecuroides under NaCl stress. J. China Agr. U. 4, 54–60.
Zhang, N., Zhang, H. J., Sun, Q. Q., Cao, Y. Y., Li, X., Zhao, B., et al. (2017). Proteomic analysis reveals a role of melatonin in promoting cucumber seed germination under high salinity by regulating energy production. Sci. Rep Uk 7, 1–15. doi: 10.1038/s41598-017-00566-1
Zhang, N., Zhao, B., Zhang, H. G., Weeda, S., Yang, C., Yang, Z. C., et al. (2013). Melatonin promotes water-stress tolerance, lateral root formation, and seed germination in cucumber (Cucumis sativus L.). J. Pineal Res. 54, 15–23. doi: 10.1111/j.1600-079X.2012.01015.x
Zhang, X., Shi, Z., Tian, Y., Zhou, Q., Cai, J., Dai, T., et al. (2016). Salt stress increases content and size of glutenin macropolymers in wheat grain. Food Addit. Contam. A 197, 516–521. doi: 10.1016/j.foodchem.2015.11.008
Zhang, X. K., Zhou, Q. H., Cao, J. H., and Yu, B. J. (2011). Differential cl-/salt tolerance and nacl-induced alternations of tissue and cellular ion fluxes in glycine max, glycine soja and their hybrid seedlings. J. Agron. Crop Sci. 197, 329–339. doi: 10.1111/j.1439-037x.2011.00467.x
Zhao, H., Kai, Z., Zhou, X., Xi, L., Wang, Y., Xu, H., et al. (2017). Melatonin alleviates chilling stress in cucumber seedlings by up-regulation of cszat12 and modulation of polyamine and abscisic acid metabolism. Scientfic Rep. 7, 36–42. doi: 10.1038/s41598-017-05267-3
Zhao, M. L., Wang, W., Nie, H., Cao, S. S., and Du, L. F. (2018). In silico structure prediction and inhibition mechanism studies of athda14 as revealed by homology modeling, docking, molecular dynamics simulation. Comput. Biol. Chem. 75, 120–130. doi: 10.1016/j.compbiolchem.2018.05.006
Keywords: salt stress, melatonin, wheat, germination, antioxidative activity
Citation: Zhang Z, Liu L, Li H, Zhang S, Fu X, Zhai X, Yang N, Shen J, Li R and Li D (2022) Exogenous Melatonin Promotes the Salt Tolerance by Removing Active Oxygen and Maintaining Ion Balance in Wheat (Triticum aestivum L.). Front. Plant Sci. 12:787062. doi: 10.3389/fpls.2021.787062
Received: 30 September 2021; Accepted: 17 December 2021;
Published: 31 January 2022.
Edited by:
Lauren A. E. Erland, The University of British Columbia – Okanagan Campus, CanadaReviewed by:
Manzer H. Siddiqui, King Saud University, Saudi ArabiaXiangnan Li, Northeast Institute of Geography and Agroecology, Chinese Academy of Sciences (CAS), China
Copyright © 2022 Zhang, Liu, Li, Zhang, Fu, Zhai, Yang, Shen, Li and Li. This is an open-access article distributed under the terms of the Creative Commons Attribution License (CC BY). The use, distribution or reproduction in other forums is permitted, provided the original author(s) and the copyright owner(s) are credited and that the original publication in this journal is cited, in accordance with accepted academic practice. No use, distribution or reproduction is permitted which does not comply with these terms.
*Correspondence: Dongxiao Li, lidongxiao.xiao@163.com; Ruiqi Li, wheatlrq@163.com
†These authors have contributed equally to this work