- 1Laboratory for Plant Genetic Breeding (LMGV), State University of the North Fluminense Darcy Ribeiro, Rio de Janeiro, Brazil
- 2Department of Exact, Environmental and Technological Sciences (CEATEC), Pontifical Catholic University of Campinas, Campinas, Brazil
- 3Business School and Polytechnic School, MBA in Business Technology, Data Science and Big Data, Pontifical Catholic University of Rio Grande do Sul, Porto Alegre, Brazil
- 4Center of Agricultural, Natural and Literary Sciences, State University of the Tocantina Region of Maranhão (UEMASUL), Estreito, Maranhão, Brazil
Increases in water use efficiency (WUE) and the reduction of negative impacts of high temperatures associated with high solar radiation are being achieved with the application of fine particle film of calcined and purified kaolin (KF) on the leaves and fruits of various plant species. KF was applied on young Coffea arabica and Coffea canephora plants before their transition from nursery to full sunlight during autumn and summer. The effects of KF were evaluated through the responses of leaf temperature (Tleaf), net CO2 assimilation rate (A), stomatal conductance (gs), transpiration (E), WUE, crop water stress index (CWSI), index of relative stomatal conductance (Ig), initial fluorescence (F0), and photosynthetic index (PI) in the first 2–3 weeks after the plant transitions to the full sun. All measurements were performed at midday. In Coffea plants, KF decreased the Tleaf up to 6.7°C/5.6°C and reduced the CWSI. The plants that were not protected with KF showed lower A, gs, E, and Ig than those protected with KF. C. canephora plants protected with KF achieved higher WUE compared with those not protected by 11.23% in autumn and 95.58% in summer. In both Coffea sp., KF application reduced F0, indicating reduced physical dissociation of the PSII reaction centers from the light-harvesting system, which was supported with increased PI. The use of KF can be recommended as a management strategy in the transition of Coffea seedlings from the nursery shade to the full sunlight, to protect leaves against the excessive solar radiation and high temperatures, especially in C. canephora during the summer.
Introduction
The fine particle film of calcined and purified kaolin (KF) application is a technological product-oriented to sustainable use of water resources in various agricultural crops (Boari et al., 2014; Brito et al., 2018; Faghih et al., 2019). The use of KF is certified by the Organic Materials Review Institute (OMRI, 2021) as an authorized substance in organic food production, in the context of rising health and environmental concerns, especially for organically growing agricultural and horticultural crops (Sharma et al., 2015; Mphande et al., 2020). Adding to improved crop water management, KF can be applied to reduce the impact of heat and excessive light (Steiman et al., 2007; Santos et al., 2021) and also help in pest (Amalin et al., 2015) and pathogen control (Glenn et al., 2001; Tubajika et al., 2007). Fine kaolin films reduce negative UV impacts by increasing light reflection in apple, Malus domestica (Glenn et al., 2002); reduce leaf temperature by 1.1°C in tomato, Solanum lycopersicum (Boari et al., 2014); reduce water stress in pepper, Capsicum annuum (Creamer et al., 2005); increase water use efficiency (WUE) by 26% in tomato (AbdAllah, 2019), and stomatal conductance (gs) in well-watered grapevines, Vitis vinifera (Glenn et al., 2010); increase leaf photosynthesis (A) in apple trees (Glenn et al., 2001); increase the sucrose biosynthesis in grapevines (Conde et al., 2018); increase height and diameter growth in young eucalyptus hybrid plants, Eucalyptus grandis × Eucalyptus urophylla (Santos et al., 2021). In tomato cultivation, the KF application results in a 23% increase of a marketable yield (average of 3 years) with significant net profit gain for the tomato producer of 600 € ha–1 on non-saline soils, and 900 € ha–1 in saline soils (Boari et al., 2014).
Coffee is one of the most consumed world beverages, and its production is made possible by the work of approximately 100 million coffee growers worldwide (Davis et al., 2019). Among 124 species of wild coffees, the global coffee trade relies on two species, namely Arabica (Coffea arabica) and Robusta coffee (Coffea canephora). The world’s two largest coffee producers, Brazil, and Vietnam are geographically distant from the African centers of coffee origin (Anthony et al., 2011). The environmental pressure of monoculture, the predominant Brazilian coffee-growing system (DaMatta et al., 2019) is far from deep forest environmental conditions in the centers of coffee origin, which causes their classification into shade-tolerant species (Ayalew, 2018). The C. arabica is an understory tree and an endemic species originated at the Ethiopian rainforests at altitudes above 1,500 m characterized with an average annual temperature of 20°C; C. canephora originated at West and Central Africa of altitudes from 0 to 1,200 m, characterized with average annual temperature between 24 and 26°C, where it evolved as a medium-sized tree (Charrier and Berthaud, 1985).
The expectation of the life of a coffee tree in their natural habitat is up to 100 years, while in plantations it is about 30–40 years (Gokavi et al., 2019). In plantations, tree production and yield cycles are regulated by diverse systems of training and renovations (Taugourdeau et al., 2014; Rakocevic et al., 2021a). In Brazil, about 300,000 ha are planted every year for orchard renovation or for the new area’s plantation, which corresponds to 13% of the areas under coffee crops (CONAB, 2021).
The coffee seedlings are produced in nurseries, normally covered with plastic mesh, which can block about 50 to 75% of photosynthetically active radiation (PAR), attaining up to 600–700 μmol m–2 s–1 at midday of one tropical sunny day (Matiello et al., 2010). Under actual air CO2 concentration, this PAR range is considered as a light saturation point for C. arabica (Rodrigues et al., 2016; Rakocevic et al., 2021b). High light intensities provoke photoinhibition in both C. arabica and C. canephora, decreasing maximum photochemical efficiency (Fv/Fm) because of an increased initial and a quenched maximum fluorescence (DaMatta and Maestri, 1997; Martins et al., 2014). In coffee, generally limited and low A is explained by stomatal factor limitations, followed by the mesophyll and biochemical constraints (Martins et al., 2014). Moreover, coffee plants showed significant sensibility to UV radiation, mainly C. canephora, which displayed reduced root and total biomass, number of leaves and leaf area, increased leaf elongation rate under ambient compared with reduced UV radiation (Bernado et al., 2021).
The optimal development of Coffea seedlings in the nursery is dependent on water supply, with an average of 4.5 mm day–1, summing about 600 mm (Vallone et al., 2010). When the seedlings formed in the nursery reach 4 to 6 pairs of leaves, they are subjected to a gradual increase of light and eventually gradual water reduction for about 30 days in a process of acclimatization. Afterward, seedlings are planted to the field, which is recommended to occur in the rainy spring in not irrigated fields (Mesquita et al., 2016). Until they are 1 year old, the young plants in the field need to be irrigated (Gervásio and Lima, 1998), otherwise without the irrigation, the mortality of transplanted coffee seedlings attains up to 73% after 6–8 months (Oliveira et al., 2015).
In the post-planting period, under the combination of elevated air temperatures and elevated solar irradiance, especially its UV bands (Ulm and Jenkins, 2015; Bernado et al., 2021), one physiological disorder called leaf sunburn can occur in coffee seedlings (Santos et al., 2016). Sunburn is expressed by symptoms of chlorosis and necrosis in a great number of species (Racskó et al., 2010). Among deleterious effects of sunburn, the reduction of leaf gas exchange, reduction in plant height, leaf area, shortened internode, and branch length, and sunburn browning of fruits were reported in apple (Racskó et al., 2010) and coffee trees (Santos et al., 2016). Recently, KF application is shown to be the best method to prevent sunburn in fruits of pomegranate, Punica granatum (Yazici and Kaynak, 2006; Sharma et al., 2018).
Despite the great importance of the coffee crops in Brazil, and the continuous necessity of new sustainable strategies for agricultural production, natural resource conservation, and to guarantee the maximum setting of seedlings in the transition from the nursery to the field, no research was performed to test the use of KF on this crucial stage of the seedling’s establishment in the full sunlight. It was hypothesized that KF leaf protection from high light intensities and temperature stress can avoid the photoinhibition, improving leaf gas exchanges and WUE in young coffee plants, having more pronounced impacts on C. canephora than in C. arabica. Thus, the aim of this work was to assess the effects of KF technology on chlorophyll a fluorescence and net photosynthesis rate of young coffee plants in two seasons, autumn and summer, in their transition from nursery to full sun under the well-watered conditions, contributing to the conservation of local water resources, environmental sustainability, and increasing plant abilities to cope with abiotic stresses.
Materials and Methods
Experimental Site, Species Description, and Fine Calcined Kaolin-Based Particle Films Application
The experiment was conducted at the State University of Northern Rio de Janeiro, in Campos dos Goytacazes (21° 44′ 47″ S and 41° 18′ 24″ W, at 14 m altitude), Southeastern Brazil, using important cropped genotypes in Brazil: C. arabica L. cv. Catuaí Vermelho IAC 44, and C. canephora Pierre ex. A. Froehner, cl. Al. Seedlings of C. canephora and C. arabica were cultivated in 800-mL plastic bags in a nursery for 5 months, covered with a plastic mesh, which were blocked with 50–70% of PAR (Braun et al., 2007). When seedlings reached five pairs of leaves, we simulated the commercial planting by transplanting young plants into 25 L pots under the shade of plastic mesh and then under full sunlight. At the bottom of each pot, 3 L of gravel was placed before adding the substrate to facilitate the water drainage. The substrate in both plastic bags and 25 L pots was composed of sieved oxisol, sand, and fermented cattle manure in a 7:1:2 ratio. Two kilograms of dolomitic limestone and 7 kg of simple superphosphate were added to 1,000 L of the substrate.
The KF used in the experiment was produced from calcinate purified kaolin (Surround® WP; TK Inc., Phoenix, AZ., United States), enriched and with a low abrasive compound of aluminum silicate [Al4Si4O10(OH)8], which is chemically inert and highly soluble in water (Glenn et al., 2010). The Surround® WP was mixed in a 2-L beaker, in the proportion of 50 g of product to 1 L of water, which resulted, when applied on the leaves, in a coverage of 646 mg m–2 of leaves, predominantly on their adaxial side. The foliar application was performed with one 1.2 L capacity precompression sprayer. The amount of KF applied per meter square of leaves was obtained from the arrangement of Petri dishes, of known mass and area, positioned at the same angle as the leaves of Coffea sp. in the moment of KF application. After application, the Petri dishes were collected and left in an oven at a temperature of 85°C for 24 h to complete a water evaporation, and after that Petri dishes were weighed.
The experiment was carried out in two seasons, autumn of 2018 and summer of 2019. For ecophysiological measurements performed in autumn of 2018, seedlings were firstly transplanted into 25-L pots on March 7th and remained under the shade of nursery for 78 days. KF application was performed on May 20th. The shade was removed (simulating transplanting to the full sunlight of the field) on May 22nd at 8 p.m., and afterward the plants were exposed to full sunlight for 22 days. For ecophysiological measurements in the summer of 2019, the seedlings were transplanted into 25-L pots on March 10th and remained under the shade of nursery for two more days. The KF application was performed on March 10th. The shade was removed on March 12th at 8 p.m., afterward the plants were exposed to full sunlight for 14 days.
Microclimate Description
The microclimate and ecophysiological measurements were conducted from the day 0 (representing seedling responses under shade nursery with 50% of PAR) to the 1st, 2nd, 7th, and 22nd/14th (autumn/summer) days of exposure to full sunlight (DFS), respectively.
Micrometeorological conditions, such as PAR (μmol m–2 s–1), air temperature (Tair, °C), relative humidity (RH,%), and air vapor pressure deficit (VPD, kPa) were monitored using a miniautomatic climatological station (Model 2475, WatchDog Spectrum Technologies, Aurora, Illinois, United States) installed between the plants during the experiment. The data were recorded every 30 min, and data are presented as an average of three readings (i.e., readings shown for 8 a.m. were calculated as an average of those registered at 7:30, 8:00, and 8:30 a.m., Table 1).
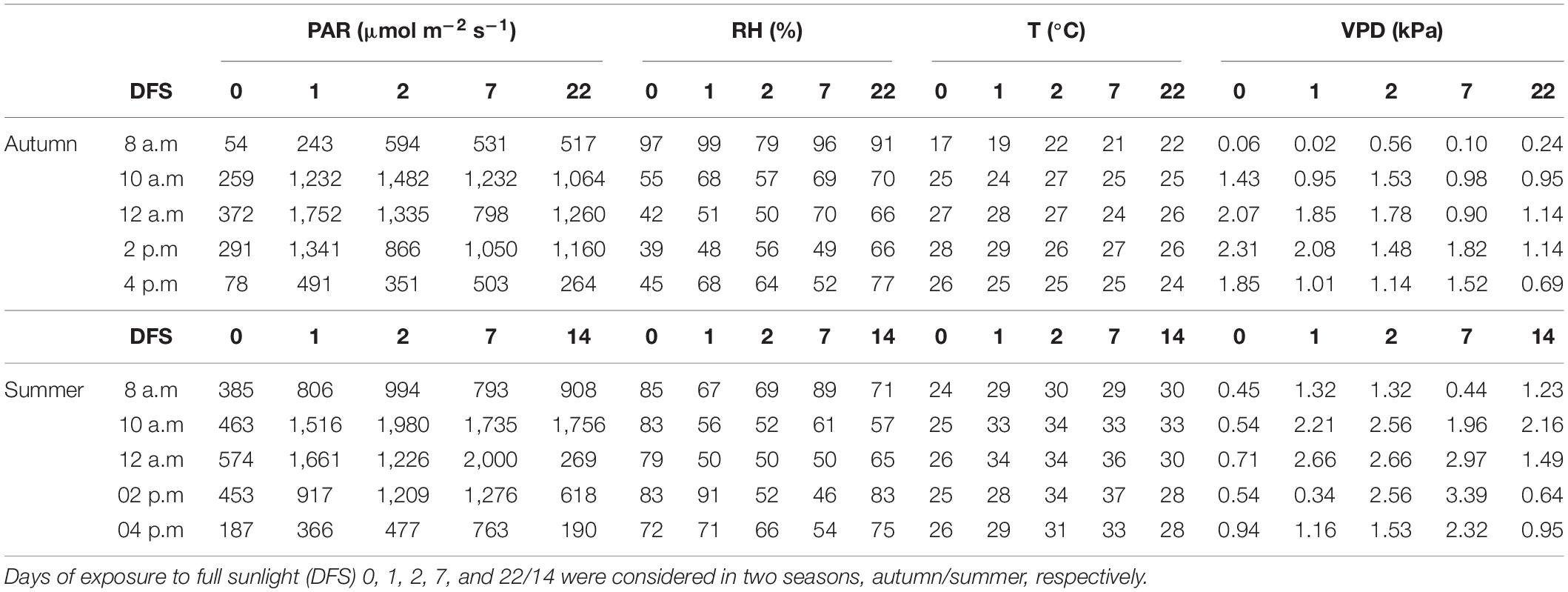
Table 1. Photosynthetic active radiation (PAR), relative humidity (RH), air temperature (Tair), and vapor pressure deficit (VPD) recorded for days of ecophysiological measurements of young coffee plants transferred from nursery to full sunlight.
On DFS 0, the PAR was extremely low, attaining the maximum values of 372 and 574 μmol m–2s–1 in autumn and summer, respectively, due to nursery shade conditions (Table 1). After exposure to full sunlight, in both seasons (autumn and summer), elevated PAR above 1,200 μmol m–2 s–1 occurred mainly between 12 a.m. and 2 p.m. The PAR recorded at 8 a.m. and 4 p.m. remained below 1,000 μmol m–2 s–1, in both seasons.
In autumn, at 8:00 a.m., RH always remained above 79%, decreasing over the diurnal cycle (Table 1). The lowest values of RH were registered at 12 a.m. and 2 p.m. In summer, RH remained above 46%, even in full sunlight. At 8 a.m., RH remained above 67%. On DFS 1 and 14 at 2 p.m., the presence of clouds contributed to the RH staying high, about 91 and 83%, respectively.
In autumn, the air temperatures remained between 17° and 30°C, whereas in summer they were higher, reaching 37°C (Table 1). In both the seasons, the hottest diurnal periods were at 12 a.m. and 2 p.m., with the highest temperatures registered on DFS 7 in summer, occurring together with the highest PAR registered (2,000 μmol m–2 s–1).
In autumn, VPD was between 0.90 and 2.31 kPa in the diurnal period of ecophysiological measurements, 12 a.m. to 2 p.m. (Table 1). In summer, the highest VPD was registered in the DFS 7 (3.39 kPa) in the same diurnal period.
Thermography, Water Stress Index, and Index of Relative Stomatal Conductance
Thermography measurements were performed in totally expanded, most recently emitted leaves, in dates defined at 2.1 when all ecophysiological measurements were performed. They were effectuated at midday (12 a.m. to 2 p.m), which corresponded to the diurnal period of the highest PAR and the highest air temperature (Table 1). On experimental plants, one pair of recently matured leaves was used: one leaf was wetted with water on its adaxial face 5 min before the image was recorded, to reduce leaf temperature (Tleaf) due to water evaporation from the leaf surface, representing Twet. On the second leaf, the Vaseline was applied (Costa et al., 2013) on its abaxial face (stomata are at abaxial side in coffee), 30 min before the image was registered, to attain the maximum Tleaf, because of transpiration blockage, representing Tdry (Figure 1). The reference leaf temperature (Tcanopy) was measured on plants used for leaf gas exchange measurements. Leaves that received the Vaseline usually dropped after 5 days. For this reason, the thermography analyses were performed with additional 20 plants (n = 5) on each evaluation day, summing 100 plants for each season.
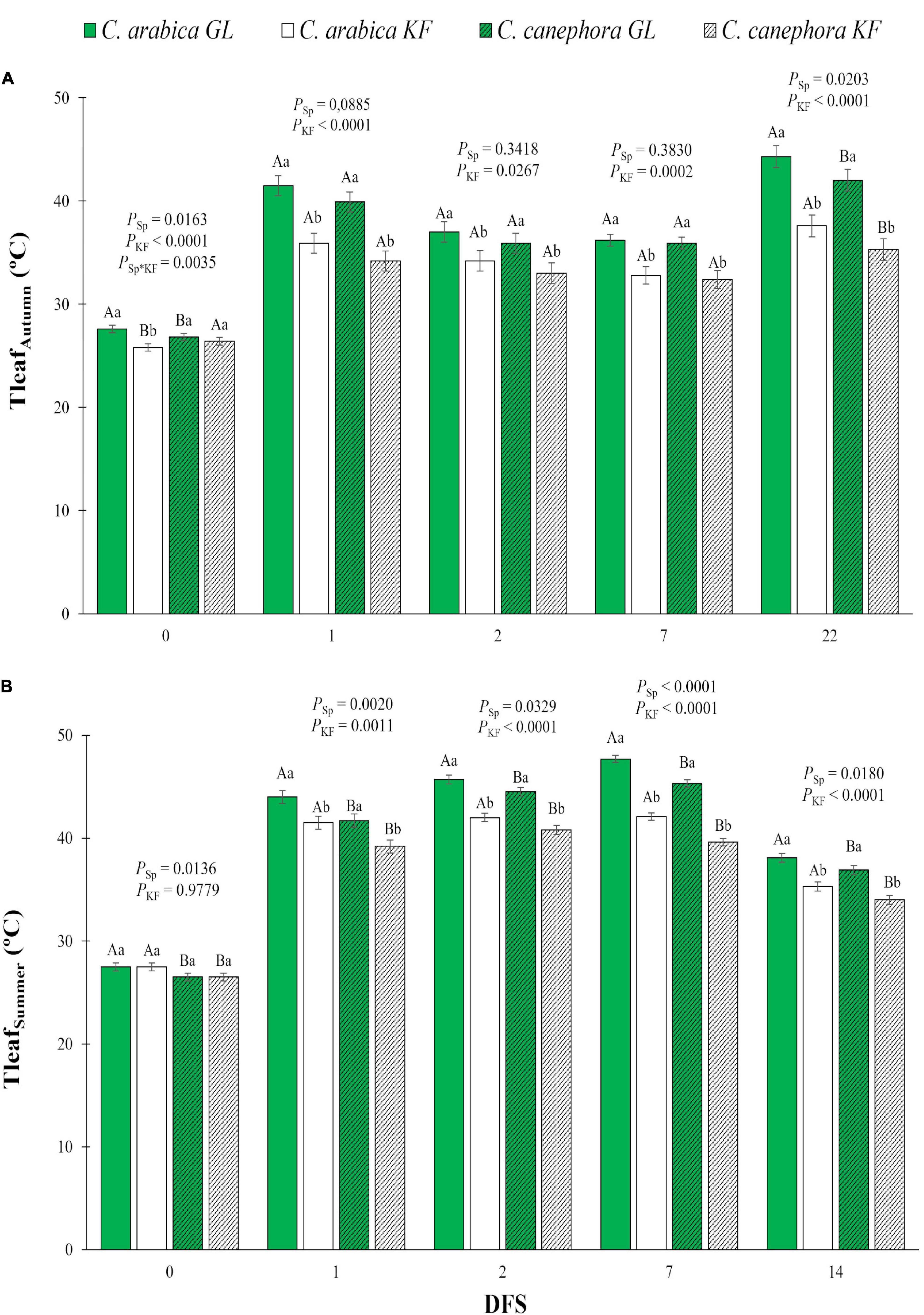
Figure 1. Leaf temperature (Tleaf) estimated by thermography in young plants of two species (Sp), Coffea arabica and C. canephora, protected with kaolin film (KF) and not protected (GL), during the transference of coffee seedlings from nursery to full sunlight in (A) autumn (Tleaf Autumn) and (B) summer (Tleaf Summer). Means ± S.E. followed by different lowercase letters indicate statistically different values between kaolin treatments within the same species, while uppercase letters indicate differences between two coffee species within the same kaolin treatment, detected by the ANOVA and Tukey test (n = 5). P-values for effects of species kaolin and their interactions are indicated for days of exposure to full sunlight (DFS).
Thermal images were obtained with a Flir i50 mid-wave infrared camera (Flir Systems, Billerica, MA, United States) with camera emissivity set to 0.96. With a focal plane array detector, images with a resolution of 140 × 140 pixels (19,600 pixels, circles at Figure 1) were produced with an accuracy of ± 2%. For thermography measurements, the equipment was approached approximately 0.50 m above the plant. The captured images were stored in the equipment’s memory, and all image processing and analysis were undertaken in Flir Tools software version 5.2.15161 in the temperature range 20–50°C. Thermographic images were treated with the iron palette, using a circle, to calculate Tdry, Twet, and Tcanopy temperatures.
From the Tcanopy, Tdry, and Twet, the canopy CWSI was calculated. CWSI concept was developed by Idso et al. (1981), relating the observed temperature to the minimum (non-stressed) and maximum (non-transpiring) temperatures of a reference crop under similar environmental conditions. Its adaptation by Jones (2018), mitigates the downfalls of the original CWSI to the Equation 1:
The thermal index of relative stomatal conductance (Ig) was calculated on according to Jones (2018) using Eq 2:
Leaf Gas Exchange and Chlorophyll a Fluorescence Measurements
The instantaneous leaf gas exchanges measurement included net CO2 assimilation rate (A, μmol CO2 m–2 s–1), transpiration (E, mmol H2O m–2 s–1), stomatal conductance (gs, mol m–2 s–1), instantaneous WUE (μmol mmol–1, calculated as the linear regression of A/E), and intrinsic water use efficiency (iWUE, μmol mol–1, calculated as linear regression of A/gs). They were performed on the same leaves as thermography, at midday (12 a.m.–2 p.m.), corresponding to the diurnal period of the highest PAR (Table 1) and the highest air temperature. The measurements were performed with an infrared gas analyzer LI-6400 (LI-COR, Lincoln, Nebraska, United States), with an external (CO2) supply of 400 μL L–1 and PAR of 1,500 μmol m–2 s–1 (from a 6400-02B, LED source composed on 80% red and 20% blue light), to attain leaf light saturation. The temperature and relative humidity inside the chamber were defined at 25°C and 60%, respectively.
In the KF treatment, immediately before the leaf-gas measurements, at the assessment site on the leaf blade, the kaolin particles were gently removed with cotton puffs to prevent their transition to the equipment pipes, avoiding undesirable modifications in the reading, and to assess the appropriate effect damage to the leaf mesophyll. Immediately after the readings, the leaves of the KF treatment received KF application, avoiding the exposure of the leaf tissue to the sunlight.
Chlorophyll a fluorescence measurements were performed in the same leaves and dates used for thermography and leaf gas exchange analyses at midday (12 a.m.–2 p.m.), using a non-modulated fluorimeter model Pocket PEA (Plant Efficiency Analyzer, Hansatech, King’s Lynn, Norfolk, United Kingdom). Leaves were previously dark-adapted for about 30 min, using Hansatech leaf clips. This premeasure ensures that all reaction centers of photosystem II (PSII) acquired an “open” status, and heat loss is minimalized (Strasser et al., 2000). Thereafter, the dark-adapted leaf parts were exposed to saturating irradiance of red light (650 nm, 3,500 μmol m–2 s–1, which is the technical limit of Pocket PEA) to obtain the fast chlorophyll a fluorescence transient of PSII, usually used to detect the stress impact affecting photosynthetic processes (Oukarroum et al., 2009). Subsequently, the collected data were submitted to the JIPtest (Strasser et al., 2004). Some variables generated by the JIPtest were used, such as the maximum PSII quantum yield (Fv/Fm), and the photosynthetic index (PI) (Strasser et al., 2004).
Statistical Analyses
The analyses of the effects of KF (KF for kaolin film application or GL for green leaves), genotype (C. arabica and C. canephora), and their interactions in leaf gas exchange responses, chlorophyll a fluorescence, and thermography were performed via two-way analysis of variance (ANOVA) using R software (R Core Team, 2021). The “nlme” (Pinheiro et al., 2021), “emmeans” (Searle et al., 1980), and “agricolae” (De Mendiburu and Simon, 2015) packages were used. All data were previously evaluated for homogeneity of variance by the Bartlett’s test (Snedecor and Cochran, 1989). A linear mixed-effects model (LME) was used to perform ANOVA and the Tukey test for comparison of treatment means, at each of the two studied seasons (autumn or summer). Models were compared by the likelihood ratio test and, when appropriate, reduced models were adopted. The estimated means and standard errors (s.e.) are represented in tables and charts.
Results
Thermography Responses
After exposure to full sunlight, young plants protected with KF showed lower Tleaf than GL plants (Figure 1). KF caused a decrease in Tleaf up to 6.7°C for both species during autumn (Figure 1A). On DFS 22 in autumn, C. arabica showed Tleaf greater than C. canephora in both treatments. In summer, C. canephora plants managed to keep its leaves cooler than C. arabica (Figure 1B). KF impacted a Tleaf decrease of 5.6°C for C. arabica and 5.7°C for C. canephora compared with GL in summer.
Leaf Gas Exchange Responses
Coffea sp. protected with KF reached higher A than GL on days 1, 2, and 7 DFS in both the seasons, whereas only in summer DFS 14 in C. canephora (Table 2). In summer, A was higher in C. canephora than in C. arabica on DFS 0, 2, 7, and 14. The average A increases in C. arabica protected with KF was 53 and 281% when compared with GL, whereas in C. canephora it was about 42 and 101% in autumn and summer, respectively. This means that C. arabica increased A more than C. canephora when protected with kaolin.
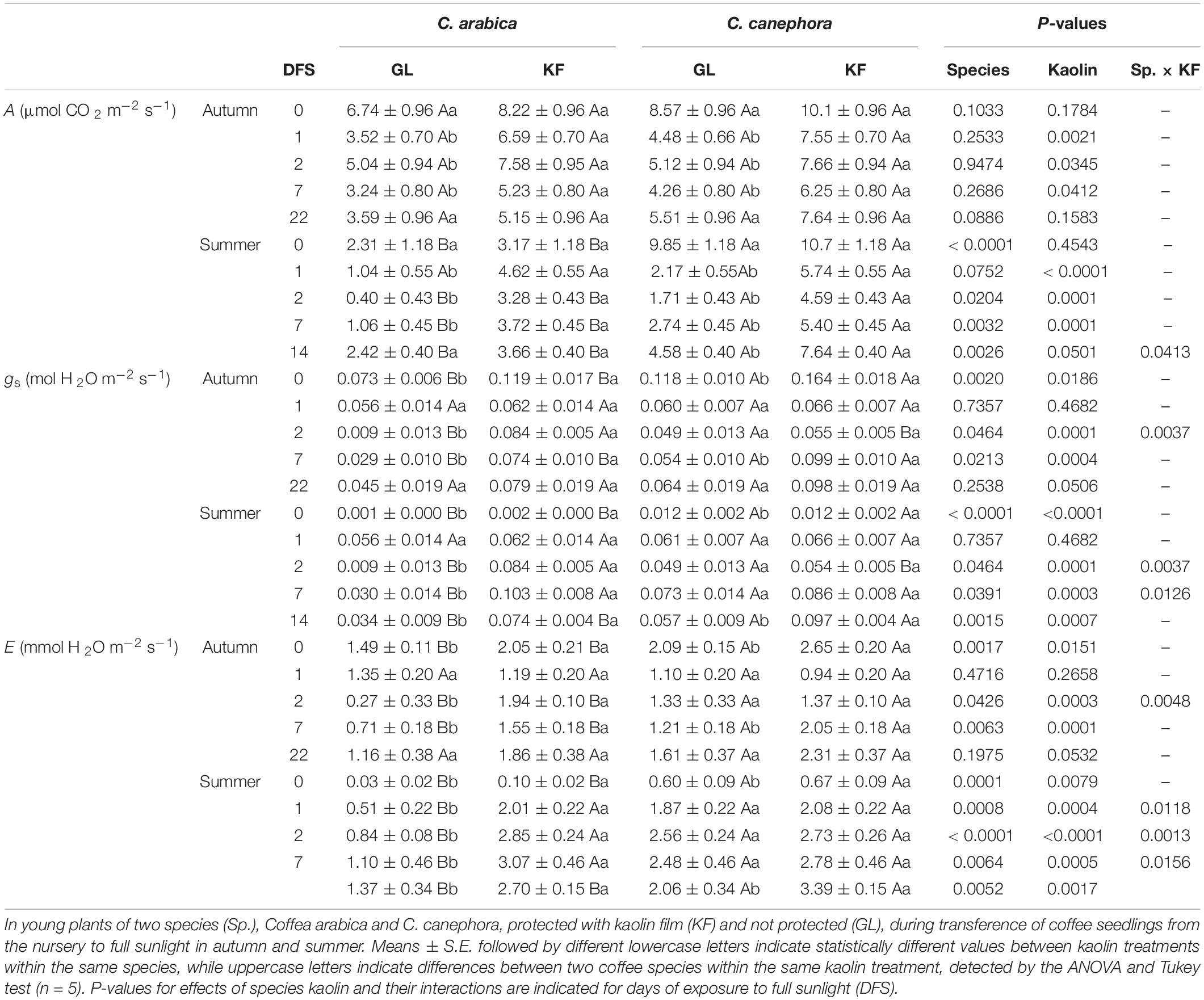
Table 2. The instantaneous leaf gas exchanges measurement included net CO2 assimilation rate (A, μmol CO2 m–2 s–1), stomatal conductance (gs, mmol H2O m–2 s–1), transpiration rate (E, mmol H2O m–2 s–1).
During autumn, KF increased gs on DFS 0, 2, and 7 in C. arabica, whereas only on DFS 0 and 7 in C. canephora (Table 2). During summer, higher gs in KF than in GL treatment was observed on DFS 0, 2, 7, and 14 in C. arabica, whilst only on DFS 0 and 14 in C. canephora. Furthermore, C. canephora plants had higher gs than C. arabica on DFS 0 and 7 during autumn, and on DFS 0 and 14 during summer, regardless of the kaolin treatment. Interestingly, C. canephora leaves protected with KF had lower gs than C. arabica on autumn and summer DFS 2, whereas the opposite situation was observed on the not protected leaves on the same DFS. With the KF protection, C. arabica increased the gs for 229 and 264% compared with GL, whereas C. canephora for 39 and 24%, in autumn and summer, respectively. This means that the gs increases in C. arabica were much higher than in C. canephora.
The impact of KF on E in C. arabica was greater in summer than in autumn (Table 2). In autumn, C. arabica plants protected with KF had higher E than GL treatment on DFS 0, 2, and 7, whereas in summer this positive effect on elevated E was observed during the whole observed period. C. canephora protected with KF maintained higher E than GL treatment on DFS 0 and 7 in autumn, and on DFS 0 and 14 in summer. On DFS 2 of autumn only in C. arabica increased E by KF, whereas this response was repeated on DFS 1, 2, and 7 of summer in a range of 179–620% (interaction Sp. × KF). C. canephora showed generally higher E values than C. arabica, regardless of the kaolin treatment, especially in summer. With the KF protection, C. arabica increased the E for 171 and 207% compared with GL, whilst C. canephora for 26 and 21%, in autumn and summer, respectively. This means that the E increases in C. arabica were up to tenfolds higher than in C. canephora in summer.
Protection with KF increased A, E, and gs in both species (Table 2). In C. arabica the increase in E was proportionally greater than the increase in A, resulting in WUE lower in KF plants than in GL plants by 9.83% in autumn and 28.56% in summer (Figures 2A,B). Similarly, the increase in gs in C. arabica was proportionally greater than the increase in A, resulting in iWUE lower by 14.32% in autumn and 8.78% in summer compared with GL (Supplementary Figures 2A,B). C. canephora plants protected with KF maintained higher WUE than GL plants by 11.23% in autumn and 95.58% in summer (Figures 2C,D), and higher iWUE by 1.79% in autumn and 75.55% in summer, compared with GL plants (Supplementary Figures 2C,D). Higher iWUE and WUE responses with KF protection in C. canephora were the consequence of generally lower relative increases in gs and E in this species when compared with C. arabica.
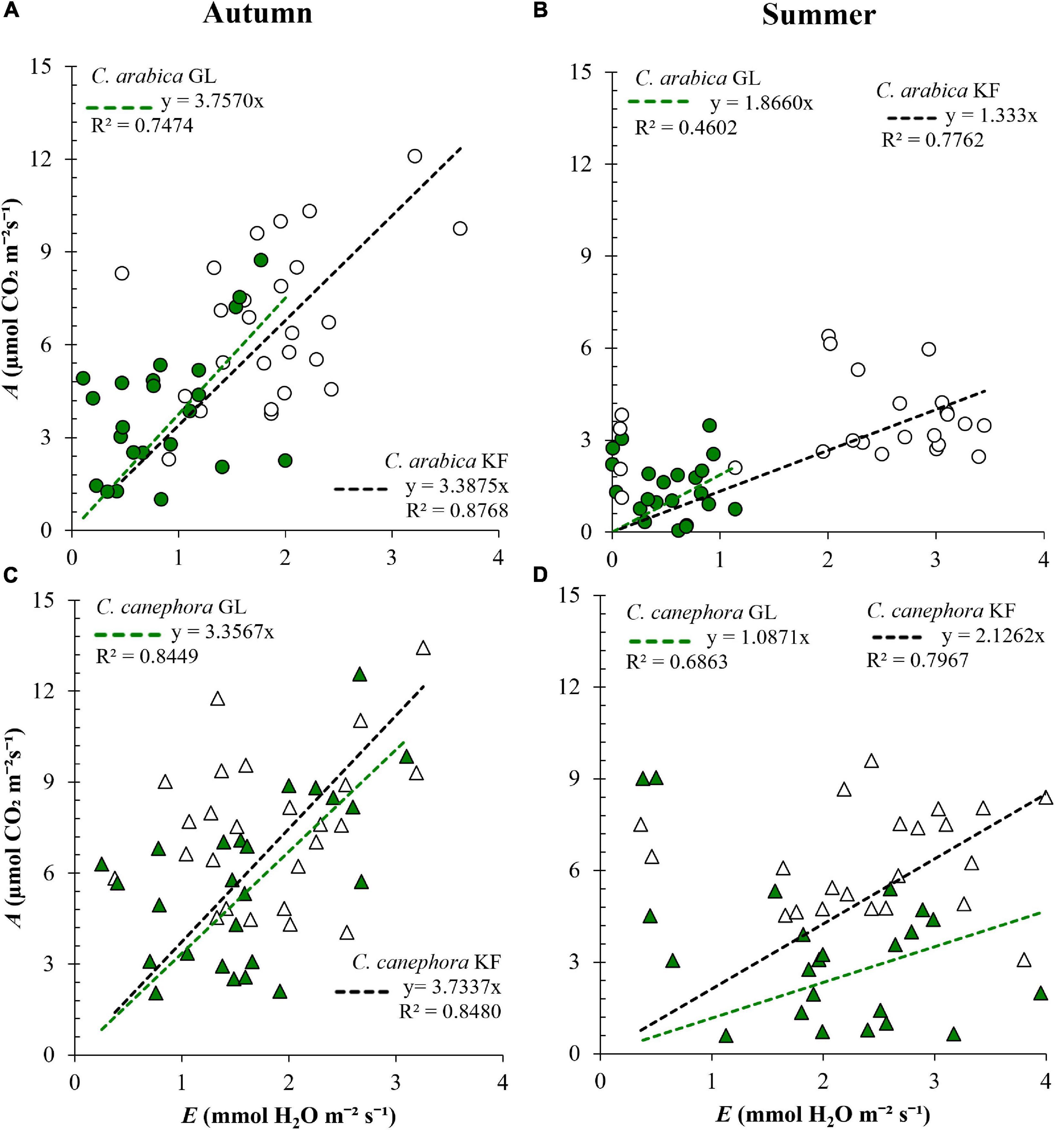
Figure 2. Instantaneous water use efficiency (WUE, μmol mmol– 1) in young plants of (A,B) Coffea arabica and (C,D) C. canephora protected with kaolin film (KF) and not protected (GL), during a transference of coffee seedlings from nursery to the full sunlight in autumn and summer.
Crop Water Stress Index and Index of Relative Stomatal Conductance
The general response to KF was CWSI reduction in both C. arabica and C. canephora plants in autumn and summer on all DFS (Figure 3). In spite of that CWSI on DFS 0 in C. arabica estimated in autumn was lower in a group of plants destinated to KF application compared with the GF group, and the general tendency was CWSI increase in DFS 1 in all treatments (Figure 3A). C. canephora maintained lower CWSI than C. arabica on DFS 1 during autumn (Figure 3A) and on DFS 1 and 7 during summer (Figure 3B), regardless of the kaolin treatment.
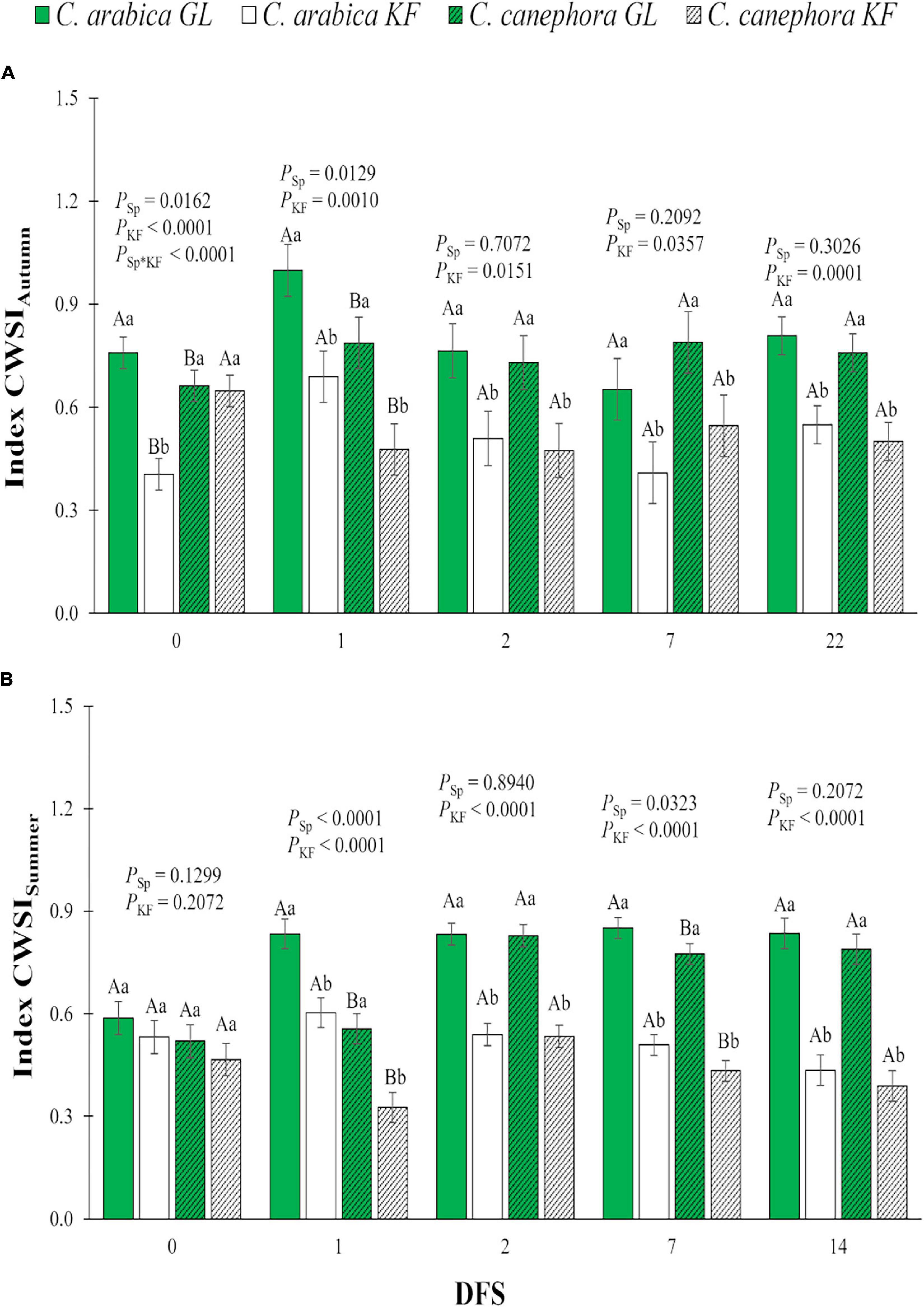
Figure 3. Water stress index (CWSI) in young plants of two species (Sp.), Coffea arabica and C. canephora, protected with kaolin film (KF) and not protected (GL), during transference of coffee seedlings from the nursery to the field in (A) autumn (CWSIAutumn) and (B) summer (CWSISummer). Means ± S.E. followed by different lowercase letters indicate statistically different values between kaolin treatments within the same species, while uppercase letters indicate differences between two coffee species within the same kaolin treatment, detected by the ANOVA and Tukey test (n = 5). P-values for effects of species kaolin and their interactions are indicated for days of exposure to full sunlight (DFS).
The application of KF increased the Ig in both species on DFS 1, 2, 7, and 22 during the autumn (Figure 4A) and on DFS 0, 1, 2, 7, and 14 during the summer (Figure 4B). On DFS 7 in autumn, C. arabica had higher Ig than C. canephora (Figure 4A), whereas on DFS 1 in summer, C. arabica had lower Ig than C. canephora (Figure 4B), regardless of the kaolin treatment.
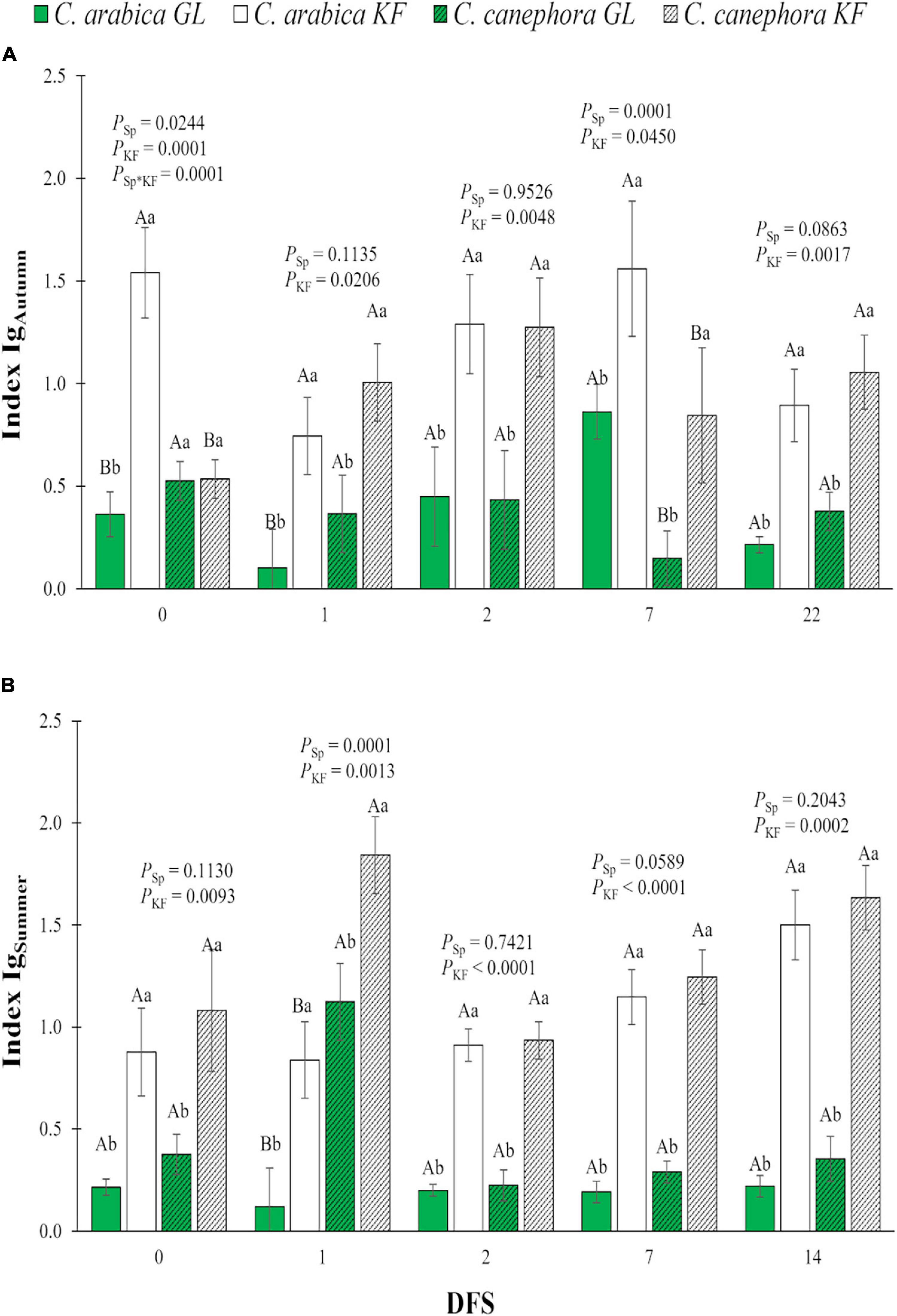
Figure 4. Index of relative stomatal conductance (Ig) in young plants of two species (Sp.), Coffea arabica and C. canephora, protected with kaolin film (KF) and not protected (GL), during transference of coffee seedlings from the nursery to full sunlight in (A) autumn (IgAutumn) and (B) summer (IgSummer). Means ± S.E. followed by different lowercase letters indicate statistically different values between kaolin treatments within the same species, while uppercase letters indicate differences between two coffee species within the same kaolin treatment, detected by the ANOVA and Tukey test (n = 5). P-values for effects of species kaolin and their interactions are indicated for days of exposure to full sunlight (DFS).
Chlorophyll a Fluorescence
Protection with KF reduced the initial fluorescence (F0) when compared with GL on DFS 0, 1, 2, and 22 during autumn (Table 3), indicating better functioning of the PSII reaction centers in this treatment than in GL. In summer, this situation was observed on DFS 2 in both species and in C arabica on DFS 7. C. arabica had higher F0 than C. canephora on DFS 2 and 7 during autumn, and on DFS 14 during the summer, regardless of the kaolin treatment.
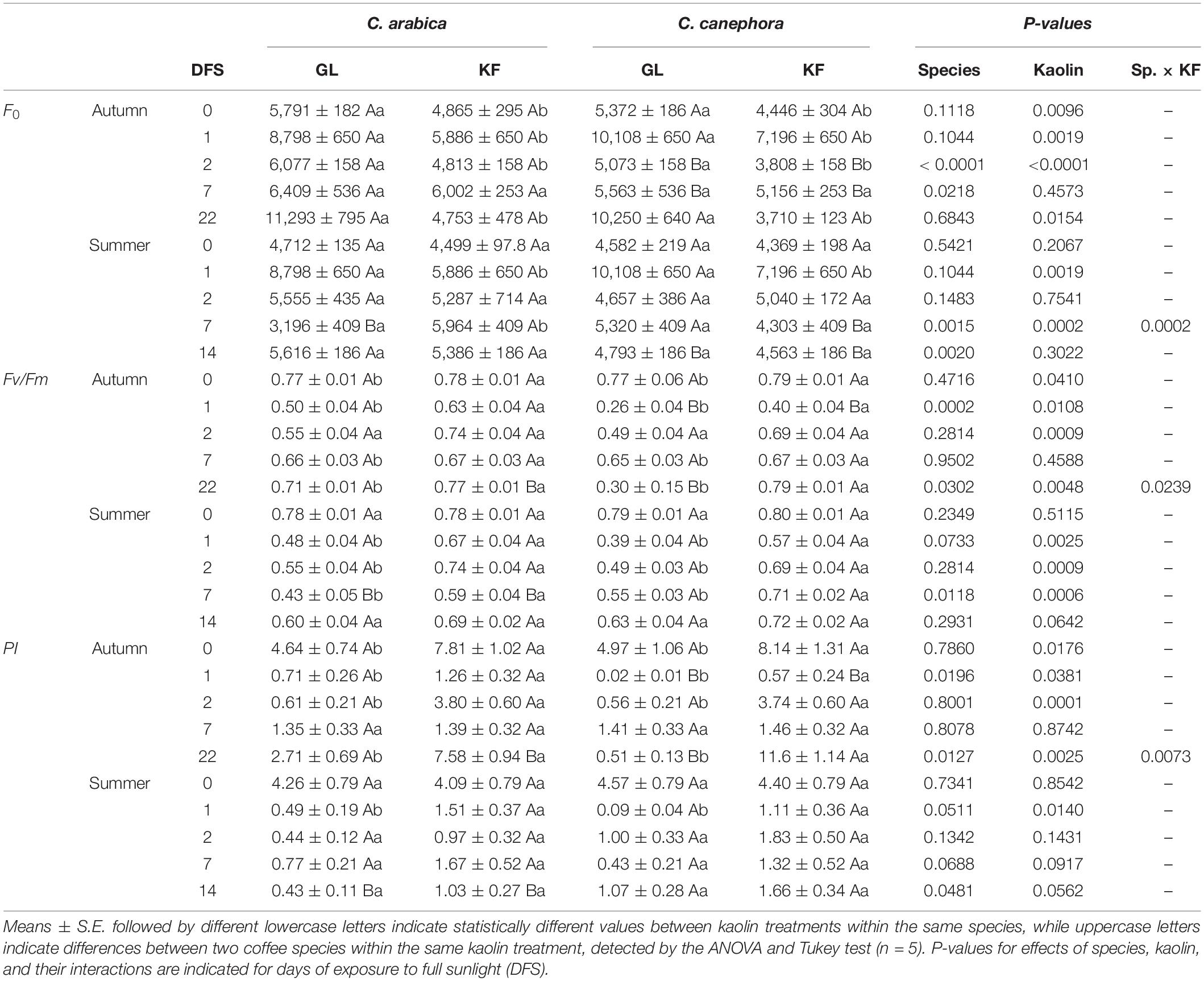
Table 3. The initial fluorescence (F0), maximum PSII quantum yield (Fv/Fm), and the photosynthetic index (PI) in young plants of two species (Sp), Coffea arabica and C. canephora, protected with kaolin film (KF) and not protected (GL), during transference of seedlings from the nursery to full sunlight, in autumn and summer.
Kaolin increased Fv/Fm on DFS 0, 1, 2, and 22 in both species during autumn, whereas on DFS 1, 2, and 7 during summer (Table 3). On DFS 1 and 22 during autumn, the Fv/Fm was higher in C. canephora compared with C. arabica, as on DFS 7 during the summer, regardless of the kaolin treatment.
Protection with KF increased PI in both species on DFS 0, 1, 2, and 22 during autumn and on DFS 1 during summer (Table 3). C. arabica plants had higher PI than C. canephora in the DFS 1 during the autumn regardless of the kaolin treatment, with no significant species impact in summer.
Discussion
We first showed that during the transference of young Coffea plants from nursery shade to full sunlight, KF application decreased the stressed conditions of the new environment characterized by high light and elevated temperatures. Both species decreased Tleaf, which impacted a general increase of leaf gas exchange parameters (gs, E, A) and Ig. In both Coffea sp., KF application reduced F0, indicating reduced physical dissociation of the PSII reaction centers from the light-harvesting system (Sundby et al., 1986), which was supported with increased PI. Interestingly, only C. canephora leaves protected with KF achieved higher WUE compared with not-protected ones, which was one specific species response.
In cascade of plant responses during the transition from nursery to the sunlight, the KF application in young Coffea plants turned the leaf surfaces white (increasing PAR reflection), which firstly reduced Tleaf at the hottest daylight period up to 6.7°C during autumn, and up to 5.6°C during summer. Considering the effects of KF in other species, the reduction of Tleaf by 3°C is observed in grapefruit, Citrus paradisi (Jifon and Syvertsen, 2003), or by 2.5°C in rose, Rosa sp. (Sotelo-Cuitiva et al., 2011). The reduction in Tleaf is explained by the ability of KF to create a modified leaf/plant microclimate by the reflective nature of kaolin particles (Glenn et al., 2002; Steiman et al., 2007). The KF white color and formulation increase albedo on the fruit or leaf surfaces (Shellie and King, 2013), increasing radiation reflection on the canopy, impacting on Tleaf reduction (Campostrini et al., 2010), as was observed in both Coffee species, with high efficiency in midday.
The reduction in Tleaf of the KF-protected plants in Coffea sp. occurred in parallel with the increase in gs, E, and Ig, and a reduction in CWSI. The microclimate created by the application of KF reduced the possible negative environmental effects of high PAR and high Tair, minimizing the partial or total closure of the coffee stomata, as happened without KF technology application (Martins et al., 2014; DaMatta et al., 2019). Without KF, C. canephora kept the leaves cooler at midday than C. arabica, which was likely related primarily to the higher E values linked to higher gs, knowing that the increased transpiration rate results in increased latent heat loss and reduced leaf temperature (Ainsworth and Rogers, 2007; Jones, 2018). Additionally, overall, non-KF treated leaves from C. canephora have showed higher stomatal density than C. arabica (Rodrigues et al., 2018; Bernado et al., 2021), helping to understand the species-specific responses, i.e., could allow C. canephora leaves to respond more rapidly to changing environmental cues. C. arabica also showed increased gs and E values when treated with KF, but the difference in gs and E between the two coffee species was reduced with KF spraying, resulting from the reduction in C. canephora efforts to acclimatize on high light and temperature.
The A was fluctuated in two seasons, in well-watered Coffee plantlets, with generally higher assimilation in autumn than in summer as previously observed in Rodrigues et al. (2016). The season in adult Coffee plants grown in field conditions without irrigation can produce the opposite effect, showing higher assimilation in rainy summer than in dry autumn (Rakocevic et al., 2021b). Generally, A was higher in C. canephora than in C. arabica in plants protected with KF than in not protected, which was associated with increases in gs. In fact, kaolin reduces abscisic acid accumulation in grapevine leaves, helping in the faster recovery of leaf gas exchanges under high light and temperature (Dinis et al., 2018), which was probably the mechanism of biochemical action in young coffee plants.
Photosynthetic carbon assimilation increased in C. canephora and C. arabica when protected with KF, but in C. arabica the increases in E and gs were proportionally greater than the increases in A, and therefore, the WUE and iWUE were reduced compared with GL plants. In summer, C. canephora protected with KF increased A more than E, resulting in elevated WUE when compared with GL plants, or to C. arabica protected with KF. A similar response is observed in grapevine, where KF application reduces canopy temperature and the thermal stress, impacting on increased WUE and productivity (Glenn et al., 2010). On the other hand, C. arabica decreased WUE and iWUE in both seasons due to relatively higher increases in gs and E than in A, when compared with C. canephora. Kaolin applied at high doses acts as an antitranspirant, impacting the direction of leaf A and E reductions in some stages of grapevines (Frioni et al., 2019, 2020). Two coffee species differ in anatomical leaf characteristics: C. arabica is characterized by a greater thickness of the abaxial epidermis and the spongy parenchyma, and by the lower thickness of the palisade parenchyma and reduced stomatal density than C. canephora (Bernado et al., 2021). Considering those anatomical species specificities and their differential responses in leaf gas exchanges with KF application, the question is: Could kaolin spraying dose be different between C. arabica and C. canephora species to provoke a positive response in water savings? In future research, reduced doses could be tested in C. arabica to promote WUE elevation, and water savings. The elevated WUE in C. canephora in the summer period can lead to water savings. In fact, the KF application in other species, such as in strawberry (Fragaria ananassa) seedlings during transplanting, allowed savings between 20 and 40% of the water volume without affecting plant growth and green intensity (Santos et al., 2012). The protective effect of KF places this technology as a sustainable development tool to mitigate the effects of ongoing global warming and allows water economy (Roy et al., 2018).
When leaves are submitted to heat stress, the increase of chlorophyll fluorescence (F0 parameter) is observed (Smillie and Nott, 1979). KF influenced the chlorophyll fluorescence emission and minimized damage to the photochemical apparatus before the appearance of visual symptoms in these two coffee species, C. canephora and C arabica (data not shown). Coffea sp. plants protected with KF had lower F0, higher Fv/Fm, and higher PI than those not protected. This effect presumably reflects the physical dissociation of the PS II reaction centers from the light-harvesting system (Sundby et al., 1986). Fv/Fm values less than 0.75 indicate a photo-inhibitory effect of the PSII-associated photosynthetic apparatus (Bolhar-Nordenkampf et al., 1989), which occurred in coffee exposed to full sunlight, regardless of KF treatment. The PI values of coffee seedlings not protected with KF indicated that the activity of PS I and PS II was compromised during the transition of the seedlings from a nursery shade to full sunlight. In C. canephora protected with KF, PI values were upto 25-fold higher than those not protected with KF on DFS 2 during the summer. Results about coffee seedlings not protected with KF may suggest some destabilization of membranes and proteins, production of reactive oxygen species, and cell death, as observed in apples (Gindaba and Wand, 2007).
In conclusion, the application of KF on coffee leaves would reduce Tleaf under high PAR and high Tair during the sensitive agronomic management of young Coffea plants, confirming the initial hypothesis. KF impacted on F0, Fv/Fm, and PI modifications in Coffea sp., minimizing possible damages of the photochemical apparatus, preventing the stomatal closure, and permitting higher net CO2 assimilation. For the C. arabica, it seems that autumn can be considered as the best season for planting, although KF application improved the plant acclimatization to elevated light and temperatures at midday. On the other hand, C. canephora showed greater plasticity than C. arabica related to the planting season. Observing the species-specific responses in water management efficiency with KF applications, C. canephora showed higher WUE and iWUE than C. arabica, indicating water savings in C. canephora cultivations, from the practical point of view. The second practical point of view could be related to diminished costs and risks, where the dilution of 1 kg of KF (the commercial price of Surround WP is about 4–5 USD) in 20 L of water (5% w/v) can protect 1,550 m2 of nursery bed or around 300,000 to 450,000 young coffee plantlets. In the field, 1 kg of KF in 5% w/v protects 1 hectare, i.e., 3,000–5,000, of newly planted coffee plants. Thus, the processed-kaolin particle film technology is important in the transition of seedlings from the nursery to the field planting condition, given that the young plant price is 0.12–0.20 USD, whereas the KF cost per plant is less than 0.002 USD. The use of KF can be used as a management strategy to protect leaves from the two coffee species against excess solar radiation, elevated temperatures, and excess water spend, especially in summer and in C. canephora.
Data Availability Statement
The raw data supporting the conclusions of this article will be made available by the authors, without undue reservation.
Author Contributions
DA: investigation, data curation, and writing original draft. NR: resources and conceptualization. GA: software and data curation. WB: investigation. WR: methodology. EC: resources, conceptualization, definition, and validation. MR: conceptualization, validation, reviewing, and editing. All authors read and approved the final manuscript.
Funding
This work was supported by CNPq fellowships (02.09.20.008.00.00 and 02.13.02.042.00.00 for DA; Visiting Researcher fellowship, process 312959/2019-2 for MR, and PQ fellowship 303166/2019-3 for EC); Fundação Carlos Chagas de Apoio à Pesquisa do Estado do Rio de Janeiro (FAPERJ) grants (E-26/202.759/2018, E-26/210.309/2018, and E-26/210.037/2020, EC); and by Tessenderlo Kerley, Inc.
Conflict of Interest
The authors declare that the research was conducted in the absence of any commercial or financial relationships that could be construed as a potential conflict of interest.
Publisher’s Note
All claims expressed in this article are solely those of the authors and do not necessarily represent those of their affiliated organizations, or those of the publisher, the editors and the reviewers. Any product that may be evaluated in this article, or claim that may be made by its manufacturer, is not guaranteed or endorsed by the publisher.
Supplementary Material
The Supplementary Material for this article can be found online at: https://www.frontiersin.org/articles/10.3389/fpls.2021.784482/full#supplementary-material
Abbreviations
Tair, Air temperature; VPD, air vapor pressure deficit; CWSI, canopy water stress index; DFS, days of exposure to full sunlight; KF, fine particle film based on calcined and purified kaolin; F0, initial fluorescence; WUE, instantaneous water use efficiency; iWUE, intrinsic water use efficiency; Tleaf, leaf temperature; Fv/Fm, maximum photochemical efficiency; A, net CO2 assimilation rate; PI, photosynthetic index; PS I and PS II, photosystems I and II; PAR, photosynthetically active radiation; RH, relative humidity; gs, stomatal conductance; Ig, thermal index of relative stomatal conductance; E, transpiration.
References
AbdAllah, A. (2019). Impacts of kaolin and pinoline foliar application on growth. yield and water use efficiency of tomato (Solanum lycopersicum L.) grown under water deficit: a comparative study. J. Saudi Soc. Agric. Sci. 18, 256–268. doi: 10.1016/j.jssas.2017.08.001
Ainsworth, E. A., and Rogers, A. (2007). The response of photosynthesis and stomatal conductance to rising [CO2]: mechanisms and environmental interactions. Plant Cell Environ. 30, 258–270.
Amalin, D. M., Averion, L., Bihis, D., Legaspi, J. C., and David, E. F. (2015). Effectiveness of kaolin clay particle film in managing Helopeltis collaris (Hemiptera: Miridae). a major pest of cacao in the Philippines. Fla. Entomol. 98, 354–355. doi: 10.1653/024.098.0156
Anthony, F., Bertrand, B., Etienne, H., and Lashermes, P. (2011). “Coffea and psilanthus,” in Wild Crop Relatives: Genomic And Breeding Resources, Plantation And Ornamental Crops, ed. C. Kole (Berlin: Springer-Verlag Heidelberg), 41–61. doi: 10.1007/978-3-642-21201-7_3
Ayalew, B. (2018). Impact of shade on morpho-physiological characteristics of coffee plants, their pests and diseases: a review. Afr. J. Agric. Res. 13, 2016–2024. doi: 10.5897/AJAR2018.13408
Bernado, W. P., de Rakocevic, M., Santos, A. R., Ruas, K. F., Baroni, D. F., Abraham, A. C., et al. (2021). Biomass and leaf acclimations to ultraviolet solar radiation in juvenile plants of Coffea arabica and C. csanephora. Plants 10:640. doi: 10.3390/plants10040640
Boari, F., Cucci, G., Donadio, A., Schiattone, M. I., and Cantore, V. (2014). Kaolin influences tomato response to salinity: physiological aspects. Acta Agric. Scand. B Soil Plant Sci. 64, 559–571. doi: 10.1080/09064710.2014.930509
Bolhar-Nordenkampf, H. R., Long, S. P., Baker, N. R., Oquist, G., Schreiber, U. L. E. G., and Lechner, E. G. (1989). Chlorophyll fluorescence as a probe of the photosynthetic competence of leaves in the field: a review of current instrumentation. Funct. Ecol. 3, 497–514. doi: 10.2307/2389624
Braun, H., Zonta, J. H., Lima, J. S. S., and Reis, E. F. (2007). “Conilon” Coffee Seedling Production At Different Shading Levels Idesia, Vol. 25. Arica, CI: IDESIA, 85–91. doi: 10.4067/S0718-34292007000300009
Brito, C., Dinis, L.-T., Ferreira, H., Rocha, L., Pavia, I., Moutinho-Pereira, J., et al. (2018). Kaolin particle film modulates morphological, physiological and biochemical olive tree responses to drought and rewatering. Plant Physiol. Biochem. 133, 29–39. doi: 10.1016/j.plaphy.2018.10.028
Campostrini, E., Reis, F. O., Souza, M. A., and Yamanishi, O. K. (2010). Processed-kaolin particle film on papaya leaves: a study related to gas exchange, leaf temperature and light distribution in canopy. Acta Hortic. 864, 195–200. doi: 10.17660/ActaHortic.2010.864.25
Charrier, A., and Berthaud, J. (1985). “Botanical classification of coffee,” in Coffee, eds M. N. Clifford and K. C. Willson (Boston, MA: Springer), 13–47. doi: 10.1007/978-1-4615-6657-1_2
CONAB (2021). Café Brasil: O Acompanhamento Da Safra Brasileira De Café, Vol. 8. Available online at: http://www.conab.gov.br (accessed May 29, 2021).
Conde, A., Neves, A., Breia, R., Pimentel, D., Dinis, L.-T., Bernardo, S., et al. (2018). Kaolin particle film application stimulates photoassimilate synthesis and modifies the primary metabolome of grape leaves. J. Plant Physiol. 223, 47–56. doi: 10.1016/j.jplph.2018.02.004
Costa, J. M., Grant, O. M., and Chaves, M. M. (2013). Thermography to explore plant–environment interactions. J. Exp. Bot. 64, 3937–3949. doi: 10.1093/jxb/ert029
Creamer, R., Sanogo, S., El-Sebai, O. A., Carpenter, J., and Sanderson, R. (2005). Kaolin-based foliar reflectant affects physiology and incidence of beet curly top virus but not yield of Chile pepper. J. Am. Soc. Hortic. Sci. 40, 574–576. doi: 10.21273/HORTSCI.40.3.574
DaMatta, F. M., and Maestri, M. (1997). Photoinhibition and recovery of photosynthesis in Coffea arabica and C. canephora. Photosynthetica 34, 439–446. doi: 10.1023/A:1006824404141
DaMatta, F. M., Rahn, E., Läderach, P., Ghini, R., and Ramalho, J. C. (2019). Why could the coffee crop endure climate change and global warming to a greater extent than previously estimated. Clim. Change 152, 167–178. doi: 10.1007/s10584-018-2346-4
Davis, A. P., Chadburn, H., Moat, J., O’Sullivan, R., Hargreaves, S., and Lughadha, E. N. (2019). High extinction risk for wild coffee species and implications for coffee sector sustainability. Sci. Adv. 5:eaav3473. doi: 10.1126/sciadv.aav3473
De Mendiburu, F., and Simon, R. (2015). Agricolae - Ten years of an open-source statistical tool for experiments in breeding, agriculture and biology. Peer J. 3:e1404v1. doi: 10.7287/peerj.preprints.1404v1
Dinis, L.-T., Bernardo, S., Luzio, A., Pinto, G., Meijon, M., Pinto-Marijuan, M., et al. (2018). Kaolin modulates ABA and IAA dynamics and physiology of grapevine under Mediterranean summer stress. J. Plant Physiol. 220, 181–192. doi: 10.1016/j.jplph.2017.11.007
Faghih, S., Zamani, Z., Fatahi, R., and Liaghat, A. (2019). Effects of deficit irrigation and kaolin application on vegetative growth and fruit traits of two early ripening apple cultivars. Biol. Res. 52:43. doi: 10.1186/s40659-019-0252-5
Frioni, T., Saracino, S., Squeri, C., Tombesi, S., Palliotti, A., Sabbatini, P., et al. (2019). Understanding kaolin effects on grapevine leaf and whole-canopy physiology during water stress and re-watering. J. Plant Physiol. 242:153020. doi: 10.1016/j.jplph.2019.153020
Frioni, T., Tombesi, S., Sabbatini, P., Squeri, C., Lavado Rodas, N., Palliotti, A., et al. (2020). Kaolin reduces ABA biosynthesis through the inhibition of neoxanthin synthesis in grapevines under water deficit. Int. J. Mol. Sci. 21:4950. doi: 10.3390/ijms21144950
Gervásio, E. S., and Lima, L. A. (1998). Efeito de diferentes lâminas de água no desenvolvimento do cafeeiro (Coffea arabica L.) em fase inicial de formação da lavoura. Rev. Bras. Eng. Agríc. Ambient. 2, 68–74. doi: 10.1590/1807-1929/agriambi.v2n1p68-74
Gindaba, J., and Wand, S. J. E. (2007). Do fruit sunburn control measures affect leaf photosynthetic rate and stomatal conductance in “Royal Gala” apple? Environ. Exp. Bot. 59, 160–165.
Glenn, D. M., Cooley, N., Walker, R., Clingeleffer, P., and Shellie, K. (2010). Impact of kaolin particle film and water deficit on wine grape water use efficiency and plant water relations. HortScience 45, 1178–1187. doi: 10.21273/HORTSCI.45.8.1178
Glenn, D. M., Prado, E., Erez, A., McFerson, J. R., and Puterka, G. J. (2002). A reflective processed kaolin particle film affects fruit temperature, radiation reflection and solar injury in apple. J. Am. Soc. Hort. Sci. 127, 188–193. doi: 10.21273/JASHS.127.2.188
Glenn, D. M., van der Zwet, T., Puterka, G., Gundrum, P., and Brown, E. (2001). Efficacy of kaolin-based particle films to control apple diseases. Plant Health Prog. 2, 1–14. doi: 10.1094/PHP-2001-0823-01-RS
Gokavi, N., Mukharib, D. S., Mote, K., Manjunatha, A. N., and Raghuramulu, Y. (2019). Studies on planting geometry and pruning methods for improving productivity and reduce labour drudgery in Arabica coffee cv. Chandragiri. J. Crop. Weed. 15, 58–64.
Idso, S. B., Jackson, R. D., Pinter, P. J., Reginato, R. J., and Hatfield, J. L. (1981). Normalizing the stress-degree-day parameter for environmental variability. Agric. Meteorol. 24, 45–55. doi: 10.1016/0002-1571(81)90032-7
Jifon, J. L., and Syvertsen, J. P. (2003). Kaolin particle film applications can increase photosynthesis and water use efficiency of ruby red grapefruit leaves. J. Am. Soc. Hortic. Sci. 128, 107–112. doi: 10.21273/JASHS.128.1.0107
Jones, H. G. (2018). “Thermal imaging and infrared sensing in plant ecophysiology,” in Advances in plant ecophysiology techniques, eds A. M. Sánchez-Moreiras and M. J. Reigosa (Berlin: Springer International Publishing) 135–151. doi: 10.1007/978-3-319-93233-0_8
Martins, S. C., Galmes, J., Cavatte, P. C., Pereira, L. F., Ventrella, M. C., and DaMatta, F. M. (2014). Understanding the low photosynthetic rates of sun and shade coffee leaves: bridging the gap on the relative roles of hydraulic, diffusive and biochemical constraints to photosynthesis. PLoS One 9:e95571. doi: 10.1371/journal.pone.0095571
Matiello, J. B., Santinato, R., Garcia, A. W. R., Almeida, S. R., and Fernandes, D. R. (2010). Cultura De Café No Brasil: Manual De Recomendações. Rio de Janeiro: MAPA/Procafé, 435.
Mesquita, C. M., Melo, E. M., de. Rezende, J. E., de. Carvalho, J. S., Fabri-Júnior, M. A., Moraes, N. C., et al. (2016). Manual do café. Implantação de cafezais. Belo horizonte. EMATER-MG, 50.
Mphande, W., Kettlewell, P. S., Grove, I. G., and Farrell, A. D. (2020). The potential of antitranspirants in drought management of arable crops: a review. Agric. Water Manag. 236:106143. doi: 10.1016/j.agwat.2020.106143
Oliveira, L. P. V., de Dominghetti, A. W., Guimarães, R. J., Rezende, T. T., and Ferreira, M. M. (2015). Planting season and hydro retainer polymer on initial growth coffee. Coffee Sci. 10, 507–515.
OMRI (2021). Available online at: https://www.omri.org/mfg/tki (accessed September 3, 2021).
Oukarroum, A., Schansker, G., and Strasser, R. J. (2009). Drought stress effects on photosystem I content and photosystem II thermotolerance analyzed using Chl a fluorescence kinetics in barley varieties differing in their drought tolerance. Physiol. Plant 137, 188–199. doi: 10.1111/j.1399-3054.2009.01273.x
Pinheiro, J., Bates, D., DebRoy, S., and Sarkar, D. (2021). R Core Team nlme: Linear and Nonlinear Mixed Effects Models. R package version 3.1-153. Available online at: https://CRAN.R-project.org/package=nlme (accessed May 15, 2021).
R Core Team (2021). Available online at: https://www.r-project.org (accessed May 15, 2021).
Racskó, J., Szabó, T., Nyéki, J., Soltész, M., Nagy, P. T., Miller, D. D., et al. (2010). Characterization of sunburn damage to apple fruits and leaves. Int. J. Hortic. Sci. 16, 15–20.
Rakocevic, M., Matsunaga, F. T., Baroni, D. F., Campostrini, E., and Costes, E. (2021a). Multiscale analyses of growth and berry distributions along four branching orders and vertical profile of Coffea arabica L. cultivated under high-density planting systems. Sci. Hortic. 281:109934. doi: 10.1016/j.scienta.2021.109934
Rakocevic, M., Batista, E. R., Pazianotto, R. A. A., Scholz, M. B. S., Souza, G. A. R., Campostrini, E., et al. (2021b). Leaf gas exchange and bean quality fluctuations over the whole canopy vertical profile of Arabic coffee cultivated under elevated CO2. Funct. Plant Biol. 48, 469–482. doi: 10.1071/FP20298
Rodrigues, W. P., Machado Filho, J. A., Figueiredo, F. A. M. M. A., Ferraz, T. M., Ferreira, L. S., Bezerra, L. B. S., et al. (2016). Whole-canopy gas exchange in Coffea sp. is affected by supra-optimal temperature and light distribution within the canopy: the insights from an improved multi-chamber system. Sci. Hortic. 211, 194–202. doi: 10.1016/j.scienta.2016.08.022
Rodrigues, W. P., Silva, J. R., Ferreira, L. S., Filho, J. A. M., Figueiredo, F. A. M. M. A., Ferraz, T. M., et al. (2018). Stomatal and photochemical limitations of photosynthesis in coffee (Coffea spp.) plants subjected to elevated temperatures. Crop Pasture Sci. 69, 317–325. doi: 10.1071/cp17044
Roy, J., Tscharket, P., Waisman, H., Abdul Halim, S., Antwi-Agyei, P., Dasgupta, P., et al. (2018). Sustainable Development, Poverty Eradication And Reducing Inequalities. Available online at: https://www.ipcc.ch/sr15/ (accessed September 6, 2021).
Santos, B. M., Salame-Donoso, T. P., and Whidden, A. J. (2012). Reducing sprinkler irrigation volumes for strawberry transplant establishment in Florida. HortTechnology 22, 224–227. doi: 10.21273/HORTTECH.22.2.224
Santos, D. P., da Rocha Sobrinho, M., de Castro Oliveira, M. D. F., Costa, N. B., Ferraz, T. M., Reis, O. F., et al. (2021). Effect of applying a calcined kaolin-based particle film on the photosynthetic capacity and growth of young eucalyptus plants. J. For. Res. 1, 1–12. doi: 10.1007/s11676-021-01345-5
Santos, L. A., Lorenzetti, E. R., Souza, P. E., de Paula, P. V. A. A., and Luz, A. L. F. (2016). Scalding in the coffee: exposure face and photosynthetic damage. Technol. Ciên. Agropec. 10, 13–17.
Searle, S. R., Speed, F. M., and Milliken, G. A. (1980). Population marginal means in the linear model: an alternative to least squares means. Am. Stat. 34, 216–221. doi: 10.1080/00031305.1980.10483031
Sharma, R. R., Datta, S. C., and Varghese, E. (2018). Effect of Surround® WP a kaolin-based particle film on sunburn. fruit cracking and postharvest quality of “Kandhari”. Pomegranates. Crop Prot. 114, 18–22. doi: 10.1016/j.cropro.2018.08.009
Sharma, R. R., Reddy, S. V. R., and Datta, S. C. (2015). Particle films and their applications in horticultural crops. Appl. Clay Sci. 116, 54–68.
Shellie, K. C., and King, B. A. (2013). Kaolin particle film and water deficit influence red winegrape color under high solar radiation in an arid climate. Am. J. Enol. Vitic. 64, 214–222. doi: 10.5344/ajev.2013.12067
Smillie, R. M., and Nott, R. (1979). Heat injury in leaves of alpine, temperate and tropical plants. Aust. J. Plant Physiol. 6, 135–141. doi: 10.1071/PP9790135
Snedecor, G. W., and Cochran, W. G. (1989). Statistical Methods, Ed. 8 Edn. Ames, IA: Iowa State Univ. Press Iowa, 491.
Sotelo-Cuitiva, Y. M., Restrepo-Díaz, H., García-Castro, A., Ramírez-Godoy, A., and Flórez-Roncancio, V. J. (2011). Effect of kaolin film particle applications (Surround® WP) and water deficit on physiological characteristics in rose cut plants (Rose spp. L.). Am. J. Plant Sci. 2, 354–358. doi: 10.4236/ajps.2011.23040
Steiman, S. R., Bittenbender, H. C., and Idol, T. W. (2007). Analysis of kaolin particle film use and its application on coffee. Hortic. Sci. 42, 1605–1608. doi: 10.21273/HORTSCI.42.7.1605
Strasser, R. J., Srivastava, A., and Tsimilli-Michael, M. (2000). “The fluorescence transient as a tool to characterize and screen photosynthetic samples,” in Probing Photosynthesis: Mechanisms, Regulation And Adaptation, 445–483. Available online at: https://www.researchgate.net/ (accessed July 10, 2021).
Strasser, R. J., Tsimilli-Michael, M., and Srivastava, A. (2004). “Analysis of the chlorophyll a fluorescence transient,” in Chlorophyll a Fluorescence, ed. G. C. Papageorgiou (Dordrecht: Springer), 321–362. doi: 10.1007/978-1-4020-3218-9_12
Sundby, C., Melis, A., Maenpää, P., and Andersson, B. (1986). Temperature dependent changes in the antenna size of photosystem II. Reversible conversion of Photosystem IIα to Photosystem IIβ. Biochim. Biophys. Acta 851, 475–483. doi: 10.1016/0005-2728(86)90084-8
Taugourdeau, S., Le Maire, G., Avelino, J., Jones, J. R., Ramirez, L. G., Quesada, M. J., et al. (2014). Leaf area index as an indicator of ecosystem services and management practices: an application for coffee agroforestry. Agric. Ecosyst. Environ. 192, 19–37.
Tubajika, K. M., Civerolo, E. L., Puterka, G. J., Hashim, J. M., and Luvisi, D. A. (2007). The effects of kaolin, harpin and imidacloprid on development of Pierce’s disease in grape. Crop Prot. 26, 92–99. doi: 10.1016/j.cropro.2006.04.006
Ulm, R., and Jenkins, G. I. (2015). QandA: how do plants sense and respond to UV-B radiation? BMC Biol. 13:45. doi: 10.1186/s12915-015-0156-y
Vallone, H. S., Guimarães, R. J., Mendes, A. N. G., Souza, C. A. S., Cunha, R. L. D., and Dias, F. P. (2010). Diferentes recipientes e substratos na produção de mudas de cafeeiros. Ciênc. Agrotechnol. 34, 55–60. doi: 10.1590/S1413-70542010000100006
Keywords: chlorophyll fluorescence, leaf photosynthesis, leaf transpiration, thermography, water management
Citation: de Abreu DP, Roda NM, de Abreu GP, Bernardo WP, Rodrigues WP, Campostrini E and Rakocevic M (2022) Kaolin Film Increases Gas Exchange Parameters of Coffee Seedlings During Transference From Nursery to Full Sunlight. Front. Plant Sci. 12:784482. doi: 10.3389/fpls.2021.784482
Received: 27 September 2021; Accepted: 09 December 2021;
Published: 07 January 2022.
Edited by:
Ep Heuvelink, Wageningen University and Research, NetherlandsReviewed by:
Vitor L. Nascimento, Universidade Federal de Lavras, BrazilLuisa C. Carvalho, University of Lisbon, Portugal
Copyright © 2022 de Abreu, Roda, de Abreu, Bernado, Rodrigues, Campostrini and Rakocevic. This is an open-access article distributed under the terms of the Creative Commons Attribution License (CC BY). The use, distribution or reproduction in other forums is permitted, provided the original author(s) and the copyright owner(s) are credited and that the original publication in this journal is cited, in accordance with accepted academic practice. No use, distribution or reproduction is permitted which does not comply with these terms.
*Correspondence: Eliemar Campostrini, campostrini@uenf.br; Miroslava Rakocevic, mimarako@unicamp.br