- 1Department of Plant Protection, College of Agriculture, Ferdowsi University of Mashhad, Mashhad, Iran
- 2Department of Behavioral and Cognitive Biology, University of Vienna, Vienna, Austria
Plants mediate interactions between below- and above-ground microbial and animal communities. Microbial communities of the rhizosphere commonly include mutualistic symbionts such as mycorrhizal fungi, rhizobia and free-living plant growth-promoting rhizobacteria (PGPR) that may influence plant growth and/or its defense system against aboveground pathogens and herbivores. Here, we scrutinized the effects of three PGPR, Azotobacter chroococcum, Azospirillum brasilense, and Pseudomonas brassicacearum, on life history and population dynamics of two-spotted spider mites, Tetranychus urticae, feeding on aboveground tissue of strawberry plants, and examined associated plant growth and physiology parameters. Our experiments suggest that these three species of free-living rhizobacteria strengthen the constitutive, and/or induce the direct, anti-herbivore defense system of strawberry plants. All three bacterial species exerted adverse effects on life history and population dynamics of T. urticae and positive effects on flowering and physiology of whole strawberry plants. Spider mites, in each life stage and in total, needed longer time to develop on PGPR-treated plants and had lower immature survival rates than those fed on chemically fertilized and untreated plants. Reduced age-specific fecundity, longer developmental time and lower age-specific survival rates of mites feeding on rhizobacteria treated plants reduced their intrinsic rate of increase as compared to mites feeding on chemically fertilized and control plants. The mean abundance was lower in spider mite populations feeding on PGPR-treated strawberries than in those feeding on chemically fertilized and untreated plants. We argue that the three studied PGPR systemically strengthened and/or induced resistance in above-ground plant parts and enhanced the level of biochemical anti-herbivore defense. This was probably achieved by inducing or upregulating the production of secondary plant metabolites, such as phenolics, flavonoids and anthocyanins, which were previously shown to be involved in induced systemic resistance of strawberry plants. Overall, our study emphasizes that PGPR treatment can be a favorable strawberry plant cultivation measure because providing essential nutrients needed for proper plant growth and at the same time decreasing the life history performance and population growth of the notorious herbivorous pest T. urticae.
Introduction
In terrestrial ecosystems, plants are the link between below- and above-ground communities, which may in consequence either indirectly, by altering plant traits, or directly, by moving up or down in the plant, interact with each other. During the last two decades, research on below-aboveground interactions has immensely intensified and many studies have focused on effects that cascade up and down between the communities of both subsystems (Van der Putten et al., 2001; Pineda et al., 2010; Hoffmann et al., 2011a,b; Friman et al., 2021b). Plant-mediated below-aboveground interactions involving micro-organisms of the rhizosphere may occur between plant-associated and/or free-living microorganisms (Bezemer and van Dam, 2005; Coskun et al., 2017; Dellagi et al., 2020), between micro-organisms and herbivores (Hoffmann et al., 2009; Patiño-Ruiz and Schausberger, 2014; Disi et al., 2019; Friman et al., 2021a) and, in turn, between micro-organisms and the herbivores’ natural enemies (Hoffmann et al., 2011a,b; Pineda et al., 2013; Gadhave et al., 2016). The rhizosphere microbial community may consist of a huge diversity of pathogenic, neutral and/or mutualistic micro-organisms. Among the usually mutualistic symbionts, it is especially mycorrhizal fungi, rhizobia and plant growth-promoting rhizobacteria (PGPR) that may influence aboveground plant growth and defense against pathogens and herbivores (Hoffmann et al., 2009, 2011a,b; Hoffmann and Schausberger, 2012; Khaitov et al., 2015; Pangesti et al., 2015; Nunes and Kotanen, 2017; Friman et al., 2021b). Here, we studied plant-mediated interactions between free-living soil bacteria that are commonly considered PGPR and aboveground herbivorous spider mites on strawberry plants.
Free-living soil bacteria such as Azotobacter spp., Pseudomonas spp., Azospirillum spp., and Bacillus spp. are well adapted to establish and thrive in competitive rhizosphere environments. Among other activities, these bacteria are well-known for using different kinds of inorganic nitrogen (N) from the soil and/or fixing atmospheric nitrogen (N2) (Steenhoudt and Vanderleyden, 2000; Dellagi et al., 2020), and thereby provide for nitrogen availability for uptake by the plants. Hence, free-living rhizobacteria can support plant growth and development via optimizing uptake of nitrogen and other nutrients such as phosphorus, iron and other micronutrients from the soil (Kempel et al., 2009; Gamalero and Glick, 2011). Due to the globally increasing demand for high agricultural productivity and the awareness of the disadvantages of using synthetic chemical pesticides and inorganic chemical fertilizers, ever growing efforts have been dedicated to sustainable agriculture (Gamalero and Glick, 2011). Application of biofertilizers such as PGPR to supply all or parts of nutritional requirements of crops is becoming more and more popular (Erturk and Çakmakçı, 2012; Conti et al., 2014; Andrade et al., 2019).
In addition to, and/or as a consequence of, promoting nutrient uptake by plants, PGPR may influence plant defense against below- and above-ground pathogens and herbivores. The phenomenon of belowground micro-organisms priming plants for defense against later occurring threats, or inducing a defense response against biotic stressors of aboveground plant parts, has been dubbed induced systemic resistance (ISR) (Dam, 2009; Glick, 2013; Pieterse et al., 2014). ISR is elicited by beneficial microbes in the rhizosphere and within-plants regulated by a network of endogenous signaling pathways, in which phytohormones play a major role (Pineda et al., 2010; Pieterse et al., 2014). Several studies have addressed indirect plant-mediated interactions between rhizobacteria and above-ground herbivores’ performance (Zehnder et al., 1997; Khan et al., 2018). These interactions are highly system- and context-specific and may range from positive (Khaitov et al., 2015) to neutral (Valenzuela-Soto et al., 2009) to negative (Zamioudis and Pieterse, 2012) effects on the herbivores, among others depending on the species of bacteria, plant and herbivore, the defensive array of the plant and the abiotic environmental conditions (Shah et al., 2021). Free-living rhizobacteria may increase the abundance of herbivores by enhancing the plant’s nutritional state but may simultaneously enhance growth and biomass of vegetative shoot and root tissue (Khaitov et al., 2015). Thus, rhizobacteria may affect plant tolerance to herbivore infestation by enhanced regrowth following herbivore injury (Disi et al., 2019). Free-living rhizobacteria can also trigger or enhance biosynthesis of defense-related chemical compounds in plants and, by this way, decrease life history performance and population growth of herbivores attacking aboveground plant tissue (Fahimi et al., 2014; Khoshfarman-Borji et al., 2020; Pourya et al., 2020).
Strawberry, Fragaria × ananassa Duchesne (Rosaceae), is one of the most frequently cultivated fruit crops around the world (Todeschini et al., 2018). Several studies documented that treatment of strawberry plants with PGPR may improve plant growth and reproduction (Martín et al., 2007; Pırlak and Köse, 2009; Roussos et al., 2012). Additionally, PGPR application may increase the concentration of some polyphenolic compounds in strawberry plants (Lingua et al., 2013; Todeschini et al., 2018), which may protect the plants against biotic stressors. However, the effects of PGPR on aboveground herbivores of strawberry have not yet been investigated. Two-spotted spider mites Tetranychus urticae Koch (Acari: Tetranychidae) are ubiquitous herbivores and highly destructive pests of a wide variety of agricultural and horticultural crops and may cause significant yield loss in field- and greenhouse-grown strawberry plants (Wermelinger et al., 1985; Migeon et al., 2010; Alizade et al., 2016). Severe spider mite infestations may strongly reduce strawberry fruit quality and quantity (Attia et al., 2013). While the effects of rhizosphere fungi on aboveground-feeding spider mite performance have been investigated in various plants such as bean (Hoffmann et al., 2009, 2011a; Hoffmann and Schausberger, 2012; Patiño-Ruiz and Schausberger, 2014), tomato (Pappas et al., 2018), and pepper (Pappas et al., 2021), it is unknown whether the presence of PGPR in the soil influences the defense system of strawberry plants against spider mites. This is an important question because spider mites usually thrive and are favored by high N-contents of plants (Wermelinger et al., 1985, 1991) such as presumably provided by PGPR (Khaitov et al., 2015 for common bean Phaseolus vulgaris L.).
Accordingly, we investigated whether treatment of strawberry plants with the PGPR Azotobacter chroococcum Beijerinck, Azospirillum brasilense Tarrand, Krieg & Döbereiner and Pseudomonas brassicacearum Achouak et al. positively affects the nutritional state of strawberry plants, which could either make them more prone to attack by two-spotted spider mites and enhance their performance and/or, additionally or alternatively, induce and/or strengthen the strawberry defense system against herbivory and thereby decrease the performance of spider mites. In detail, we scrutinized the effects of PGPR treatments on strawberry plant growth and physiology and the life history and population dynamics of T. urticae feeding on strawberry leaves and plants growing in substrates treated with PGPR, as compared to plants treated with chemical fertilizer and untreated plants (control).
Materials and Methods
Bacteria Preparation
The bacterial strains of Azotobacter chroococcum (Ac), Azospirillum brasilense (Ab), and Pseudomonas brassicacearum (Pb) used in the experiments were obtained from the Plant Pathology Laboratory, Department of Plant Protection, Faculty of Agriculture, Ferdowsi University of Mashhad, Iran. Bacteria were grown on nutrient agar (NA) for routine use, and maintained in nutrient broth (NB) with 15% glycerol at −80°C for long-term storage (Merck KGaA, Darmstadt, Germany). For each experiment, cultures were taken out from storage and grown on NA until use. A loop full of bacteria was transferred to 1-litre flasks containing NB, and grown aerobically in flasks on a rotating shaker with 150 rpm for 48 h at 24°C. The bacterial suspension was then diluted in sterile distilled water to a final concentration of 2 × 109 CFUs (colony forming units) per ml, and the resulting suspensions were used to treat the strawberry plants (Zehnder et al., 1997). All three strains of the rhizobacteria species used in our study have previously revealed plant growth promoting effects on wheat (Mirzamohammadi et al., personal communication).
Strawberry Culturing and Treatments
Seedlings of strawberry (Fragaria × ananassa cv. Selva) were periodically singly grown in 1-litre plastic pots (20 cm height × 17 cm diameter) filled with a substrate mixture of coconut peat (Kumari Coir Products, Singapore), clay and sand (1:1:1 by volume). The seedlings and pots were covered by transparent cylindrical fine-mesh cages (70 cm height × 30 cm diameter) and kept in a greenhouse at 26 ± 1°C, 65 ± 5% RH under natural lighting at the Agricultural Faculty, Ferdowsi University of Mashhad. The photoperiod was about 13 to 14/11 to 10 h L/D when the study was conducted in Mashhad, Khorasan-e-Razavi, Iran from early April to mid-May 2020.
We conducted two experiments on life history and population growth of T. urticae feeding on strawberry leaves and plants and one experiment on strawberry plant growth and physiology. The five treatments assigned to each experiment included (1) inoculation of strawberry roots with A. chroococcum, (2) with P. brassicacearum, and (3) with A. brasilense (no chemical fertilizer application for all three PGPR treatments) as well as (4) chemical fertilization (no PGPR application), and (5) control (no PGPR and chemical fertilizer application). For the PGPR treatments (1), (2), and (3), prior to planting, the roots of the seedlings were dipped in watery suspensions (density 2 × 109 CFU/ml) of either Ac, or Ab, or Pb for 40 min. After that, the seedlings were air-dried for 30 min and then transplanted into the pots. The pots were tap-watered once every 2 days. For the chemical fertilization treatment, the recommended levels of nitrogen (N), potassium (K) and phosphorus (P) applied for conventionally grown strawberry plants were 170, 140, and 100 kg/ha (Hochmuth et al., 1996; Tabatabaei et al., 2006). Pots were fertilized three times per week with N and Ca (0.6 g/pot as calcium nitrate with total amount of 16.88 g/pot for 8 weeks), K and P (0.2 g/pot as di-potassium hydrogen phosphate with a total amount of 3.6 g/pot for 6 weeks) as well as Fe (0.1 g/pot as iron-chelate EDDHA with a total amount of 2.4 g/pot for 8 weeks) dissolved in 100 ml of distilled water.
Tetranychus urticae Rearing
To initiate a strawberry-reared population of T. urticae (green form) specimens were collected from a population reared on whole common bean plants Phaseolus vulgaris kept under the above-described environmental conditions in the greenhouse. For rearing, strawberry plants (non-inoculated and fertilized) in the 3- to 4-leaf stage were periodically infested with mixed mobile stages of T. urticae, taken from other infested strawberry plants, and the plants covered by mesh cages (70 cm height × 30 cm diameter) to avoid any contamination by other herbivores. The strawberry-reared stock population was left to grow for at least four generations (∼45 days) before use in the experiments.
Experiment 1. Life History and Life Table Analysis of Tetranychus urticae
This experiment was performed in a completely randomized design with leaves detached from 20 singly-potted strawberry plants, for each of the five treatments. About 1 month after seedling transplanting, leaves were clipped from clean strawberry plants that were in the 6- to 8-leaf stage and used to examine the life history of T. urticae in whole leaf tests (Alizade et al., 2016). To this end, the youngest fully developed leaf (average size 8.3 × 6.5 cm) of each strawberry plant was placed upside down on water-saturated cotton in a Petri dish (Ø 9 cm) and the Petri dishes were stored in a climate-controlled incubator at 25 ± 1.8°C, 70 ± 10% RH, and 16:8 h (L: D) photoperiod. Water was added daily to the Petri dishes to maintain cotton moisture. Every 3 to 4 days, the experimental mites were transferred to freshly detached leaves placed inside new Petri dishes.
To study the life history of T. urticae, an adult female/male pair was randomly withdrawn, using a fine camel-hair brush, from the strawberry-reared stock population, placed on a detached leaf from one of the five plant treatments and the female was allowed to lay eggs for 24 h. Subsequently, the adult mites and all but one egg were removed. Survival and developmental stage of each individual mite were checked twice daily at 10–12 h intervals until emergence of the adult. After reaching adulthood, a male from the same treatment was added to the female. If there were not enough males within the same treatment, additional males were collected from the strawberry-reared stock population and added to the leaf harboring the female; those males were not considered for life table analysis. Survival, longevity and fecundity of adult females and males were monitored once per day until natural death. The number of eggs laid by the females were daily counted and then removed. The number of replicates was 38, 40, 40, 45, 40 for Ac, Ab, Pb, chemical fertilizer and control, respectively.
From the survivorship, longevity and oviposition data of T. urticae, age-stage, two-sex life tables (Chi and Liu, 1985; Chi, 1988) were constructed using the computer program TWOSEX-MSChart (Chi, 2020). This program is freely available at http://140.120.197.173/Ecology/ (National Chung Hsing University, Taiwan) and http://nhsbig.inhs.uiuc.edu/wes/chi.html (Illinois Natural History Survey, IL, United States). Using this method, we calculated the age-stage specific survival rate (Sxj), where x is the age and j is the stage; the age-stage specific fecundity (fx); the age-specific survival rate (lx); the age-specific fecundity (mx); the parameter Sxj represents the probability that an egg survives to age x and stage j; lx, the probability that an egg survives to age x; the net reproductive rate (R0), which is the mean number of offspring that a female mite can produce during her lifetime; the intrinsic rate of natural increase (rm) and the finite rate of increase (λ), which are the per capita rates of increase per time unit assuming unlimited and limited population growth; and the mean generation time (T), which is the average time required for the population to complete a full life cycle from egg to production of new eggs.
Experiment 2. Strawberry Growth and Physiological Parameters
To characterize the effects of rhizobacteria treatments on strawberry growth and physiology, the number of flowers and leaves, leaf chlorophyll content and stomatal conductivity of the potted strawberry plants (6 weeks old, in the 6- to 8-leaf stage) were measured; these measurements were taken before releasing T. urticae to start the population dynamics experiment (experiment 3). To this end, the number of flowers and fully expanded trifoliate leaves (each composed of three leaflets) of the strawberry plants were counted, and the leaf chlorophyll content and stomatal conductivity were measured by a SPAD portable leaf chlorophyll meter (SPAD-502, Minolta Camera, Co., Japan) and a leaf porometer system (Sc-1), respectively. In both measurements, one newly expanded sunlit leaflet was randomly selected from each sampled plant. Each leaflet was one replication and measured once, and there were six replications for each treatment.
Experiment 3. Population Dynamics of Tetranychus urticae
This experiment aimed at evaluating the effects of rhizobacteria and chemical fertilizer treatments as well as control treatment on the population dynamics of T. urticae on whole strawberry plants. To this end, a set of mixed T. urticae life stages including 3 adult females, 3 adult males and 3 protonymphs were gently transferred from the strawberry-reared stock population (reared on non-inoculated fertilized plants) to the abaxial surface of the youngest fully developed leaf of each potted strawberry plant (6 weeks old, in the 6- to 8-leaf stage) of each of the five treatments (8 replicate plants each per treatment). To make sure that the mites had successfully settled, we visually checked their fate after release. To prevent movement of T. urticae between pots, each pot was covered by an acrylic cylinder (70 × 30 cm) closed on top by an organdy-mesh and kept under the afore-mentioned environmental conditions of the greenhouse. Population development of T. urticae on the potted strawberry plants was assessed once per week by counting the number of mites on one strawberry leaf/plant; sampling started 1 week after mite infestation on 6 April and ended on 18 May 2020 for six consecutive samplings. On each sampling date, the youngest fully developed leaf of each strawberry plant was detached, placed into a labeled polyethylene bag, transferred to the laboratory and there all mite life stages (except eggs) were counted using a stereo microscope. The youngest fully developed leaf was chosen to standardize sampling among treatments. The detached leaves were subsequently returned to the experimental units they came from to let the mites reintegrate on their host plant.
Statistical Analyses
For the data of experiment 1, the bootstrap technique, with 200,000 bootstrap samples, was used to estimate the means, variances, and standard errors of the life history parameters of T. urticae in each treatment. The bootstrap subroutine is included in the TWOSEX-MSChart (Chi, 2020) program. Paired bootstrap tests were used to compare the differences in T. urticae reproduction and life table among treatments (Mou et al., 2014) using TWOSEX-MSChart (Chi, 2020).
To assess the effect of rhizobacteria inoculation on growth and physiological measurements of strawberry plants (experiment 2), the data were analyzed by one-way ANOVA. Before analysis, the data were checked for normality and homogeneity of variance using Kolmogorov-Smirnov and Bartlett tests, respectively. Significant differences between treatment pairs were determined (P < 0.05) by post hoc Tukey’s test.
For the data of experiment 3, mite counting was repeated over time on the same strawberry plants; hence, the data were analyzed using repeated measures analysis of variance (RepANOVA), with sampling date as the repeated (within-subject) factor, and treatment being the between-subject factor. Statistical analyses of growth and physiological measurements of strawberry plants (experiment 2) and population dynamics of T. urticae (experiment 3) were performed using IBM SPSS 21 (IBM Corp, 2012).
Results
Experiment 1. Life History and Life Table Analysis of Tetranychus urticae
Immature Survival and Development, Adult Longevity and Reproduction
Development from egg to adult differed among the five treatments of strawberry (Table 1). Overall and for each life stage and total development, both females and males needed the shortest time on chemically fertilized plants and the longest on Ac-treated plants. In neither treatment, there was a significant difference in the developmental times of females and males except for longer total development of females than males reared on Ab-treated leaves (Table 1). The longest pre-oviposition period (POP) was observed in mites reared on Ac-treated plants. Fecundity was the highest on chemically fertilized plants and the lowest on Ac- and Ab-treated plants. Adult females and males reared on chemically fertilized plants lived the longest. In all treatments, adult longevity was significantly longer for females than for males (Table 1).
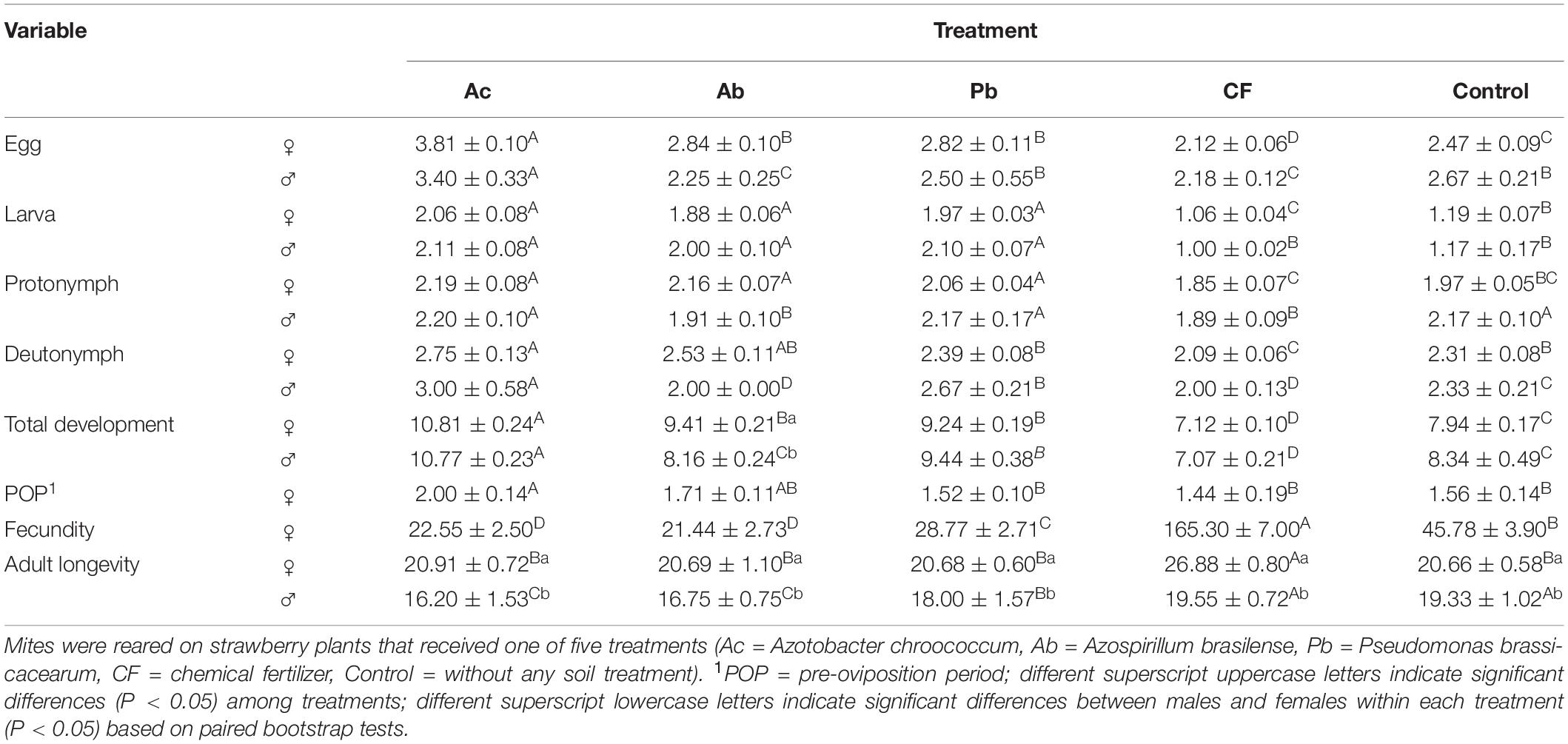
Table 1. Mean (± SE) developmental times of life stages and phases (in days) and fecundity (number of eggs/female) of T. urticae calculated using the age-stage, two-sex life table.
Age-Specific Survivorship and Fecundity
The age-stage specific survival curves (sxj), i.e., the probability that a newborn will survive to age x and develop to stage j, of T. urticae reared on leaves from strawberry plants differed significantly among treatments (Figure 1). PGPR application to the substrate of strawberry plants decreased the immature survival rate (sa) in Ac, Ab and Pb treatments in comparison to the chemical fertilizer and control treatments (Figure 1). In all treatments, females survived longer than males. Females in the chemical fertilizer treatment survived longer than females in the other treatments. The overlapping curves of the different life stages result from varying developmental times of immature mites (Figure 1).
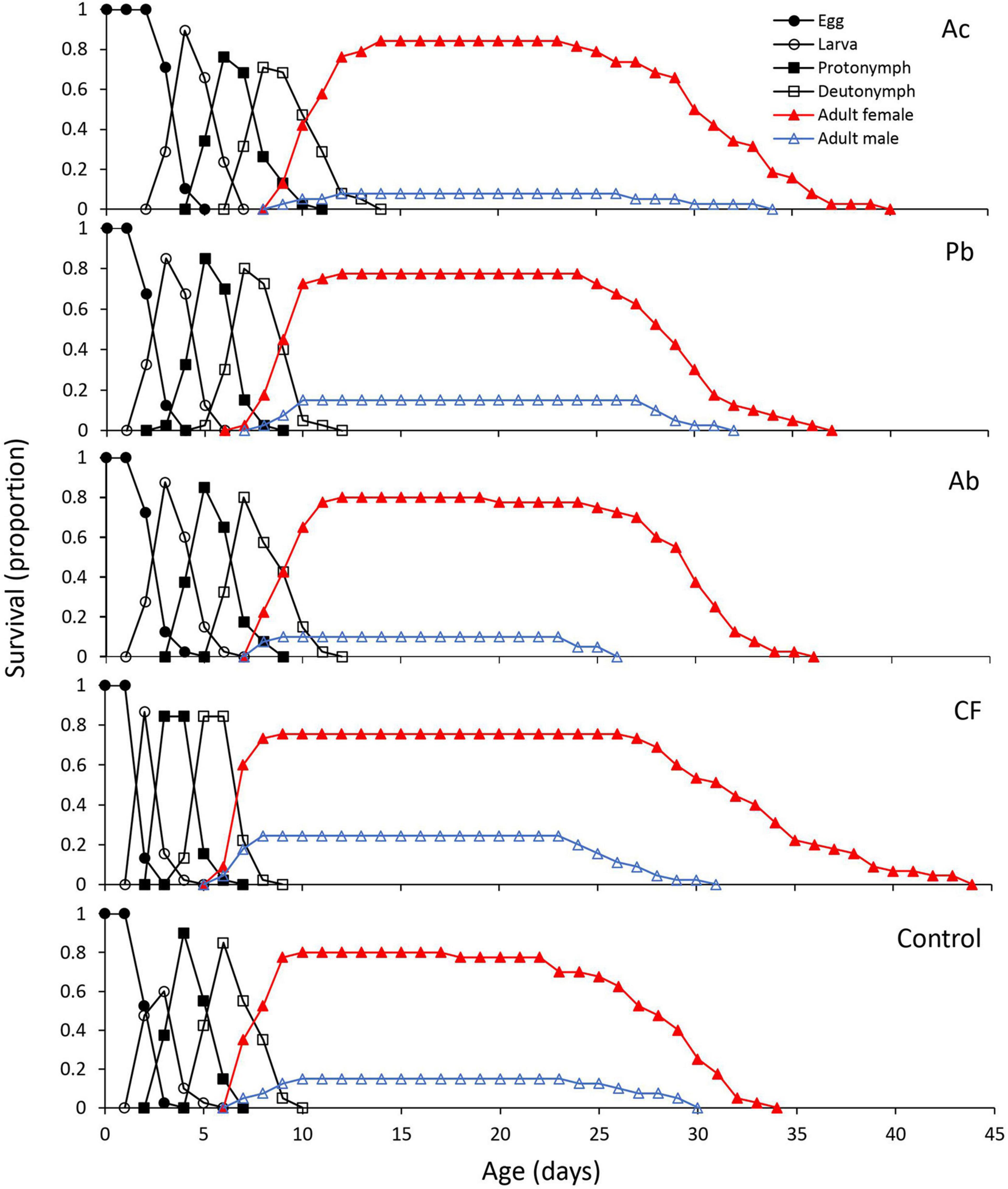
Figure 1. Age-stage specific survival curve (sxj) of T. urticae reared on leaves from strawberry plants subjected to one of five treatments, Ac (Azotobacter chroococcum), Ab (Azospirillum brasilense), Pb (Pseudomonas brassicacearum), CF (chemical fertilizer), and control (untreated).
The age-specific survival rate (lx) represents the probability that an individual will survive to age x (Figure 2). Mites feeding on chemically fertilized plants had the longest survival times; no individual died before the age of 24 days. Also, mites reared on leaves of chemically fertilized strawberry plants started oviposition earlier (at 6 days age) than mites from the other four treatments. The age-specific fecundity (mx; mean number of eggs produced by each female at age x) and the age-stage specific fecundity (fx; mean number of eggs produced by each female at age x and stage j) curves show that the mean daily oviposition was pronouncedly higher in females feeding on leaves from chemically fertilized plants than in females from the other treatments; females feeding on chemically fertilized plants produced eggs over longer time than females in the other treatments (Figure 2).
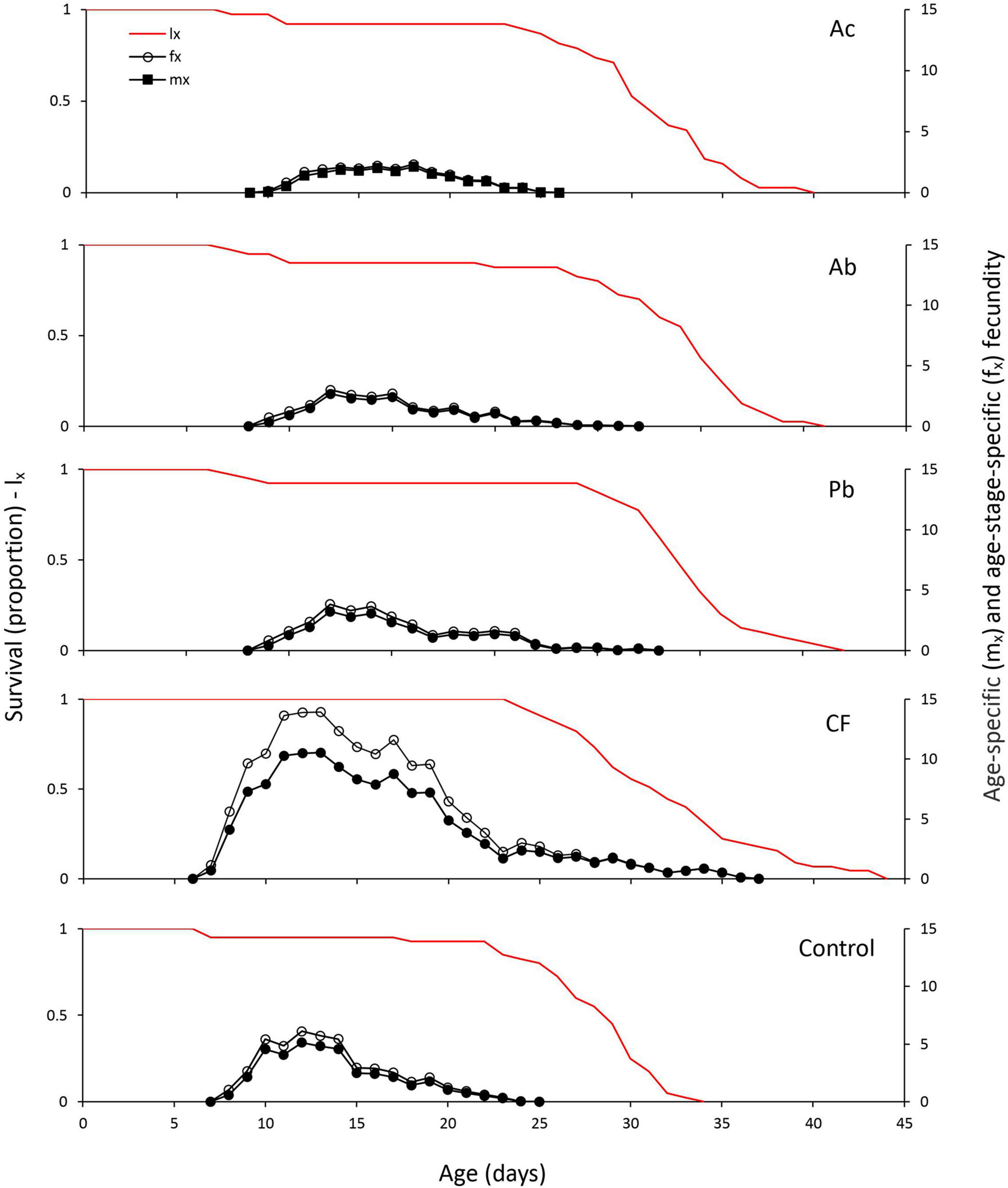
Figure 2. Age-specific survival (lx) and fecundity (mx, fx) curves of T. urticae reared on leaves of strawberry plants subjected to one of five treatments, Ac (Azotobacter chroococcum), Ab (Azospirillum brasilense), Pb (Pseudomonas brassicacearum), CF (chemical fertilizer), and control (untreated).
Demographic Parameters
Demographic parameters of T. urticae differed among rhizobacteria-treated strawberry plants, and chemical fertilizer and control treatments of strawberry plants (Table 2). The highest intrinsic rate of increase (rm), net reproductive rate (R0) and finite rate of increase (λ) were observed in mites feeding on chemically fertilized plants and the lowest in mites feeding on Ac-treated plants. Mites reared on leaves of rhizobacteria-treated strawberry plants, especially those of Ac-treated plants, had longer mean generation times than mites feeding of chemically fertilized and control plants (Table 2).
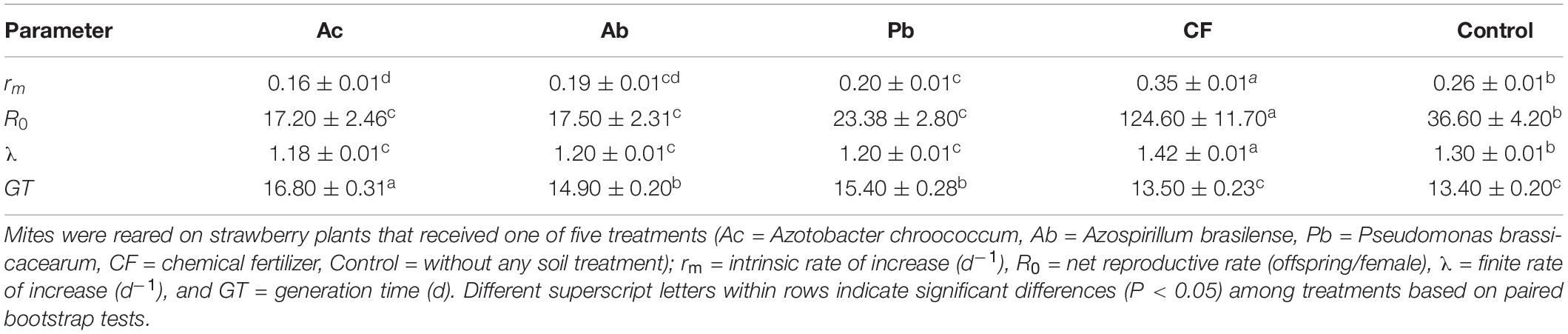
Table 2. Mean (± SE) demographic parameters of T. urticae calculated using the age-stage, two-sex life table.
Experiment 2. Strawberry Growth and Physiological Parameters
The number of flowers produced by young 6-week-old plants was significantly greater in Ac, Ab and Pb treatments than in the chemical fertilizer and control treatments (F4,29 = 7.5,P < 0.05;Table 3). The number of leaves did not differ among treatments (F4,29 = 0.70,P > 0.05). The highest leaf chlorophyll content was observed in Pb- and Ab-treated as well as chemically fertilized plants and the lowest in the Ac-treated and control plants (F4,29 = 17,P < 0.05). Stomatal conductivity was significantly enhanced in plants inoculated with rhizobacteria in comparison to chemically fertilized as well as control plants and was the greatest in the Pb treatment (F4,29 = 4.89,P < 0.05).

Table 3. Mean (± SE) growth parameters (N = 20 plants for flowers and leaflets) and physiological parameters (N = 6) of clean young strawberry plants (6 weeks after transplanting) that received one of five treatments (Ac = Azotobacter chroococcum, Ab = Azospirillum brasilense, Pb = Pseudomonas brassicacearum, CF = chemical fertilizer, Control = without any soil treatment).
Experiment 3. Population Dynamics of Tetranychus urticae
In addition to significant main effects of time (six sampling dates) and treatment (three species of PGPR application, chemical fertilizer and control) on the abundance of T. urticae, also the interaction between time and treatment was statistically significant (RepANOVA: time: Greenhouse–Geisser adjusted F5,75 = 29.1, P < 0.01; treatment: Greenhouse–Geisser adjusted F4,75 = 56.9, P < 0.01; time by treatment: Greenhouse–Geisser adjusted F20,75 = 29.1, P < 0.01). In all treatments, a marked increase in spider mite abundance was observed 1 week after release (Figure 3); the population developing on the Ac-treated strawberries increased the least and had the lowest abundance; also the populations developing on the two other PGPR-treated plants (Ab and Pb) grew less than those on chemically fertilized and control plants (F4,19 = 12.7, P < 0.01; Figure 3). On chemically fertilized plants, the mite population dropped to very low levels in weeks 4 and 5, after an initial increase from week 1 to 2, but then steeply increased to 3- to 4-times higher abundances in weeks 6 and 7 than the populations in the other treatments (Figure 3). Overall, the population fluctuations of T. urticae were less pronounced on PGPR-treated plants than on chemically fertilized and control plants.
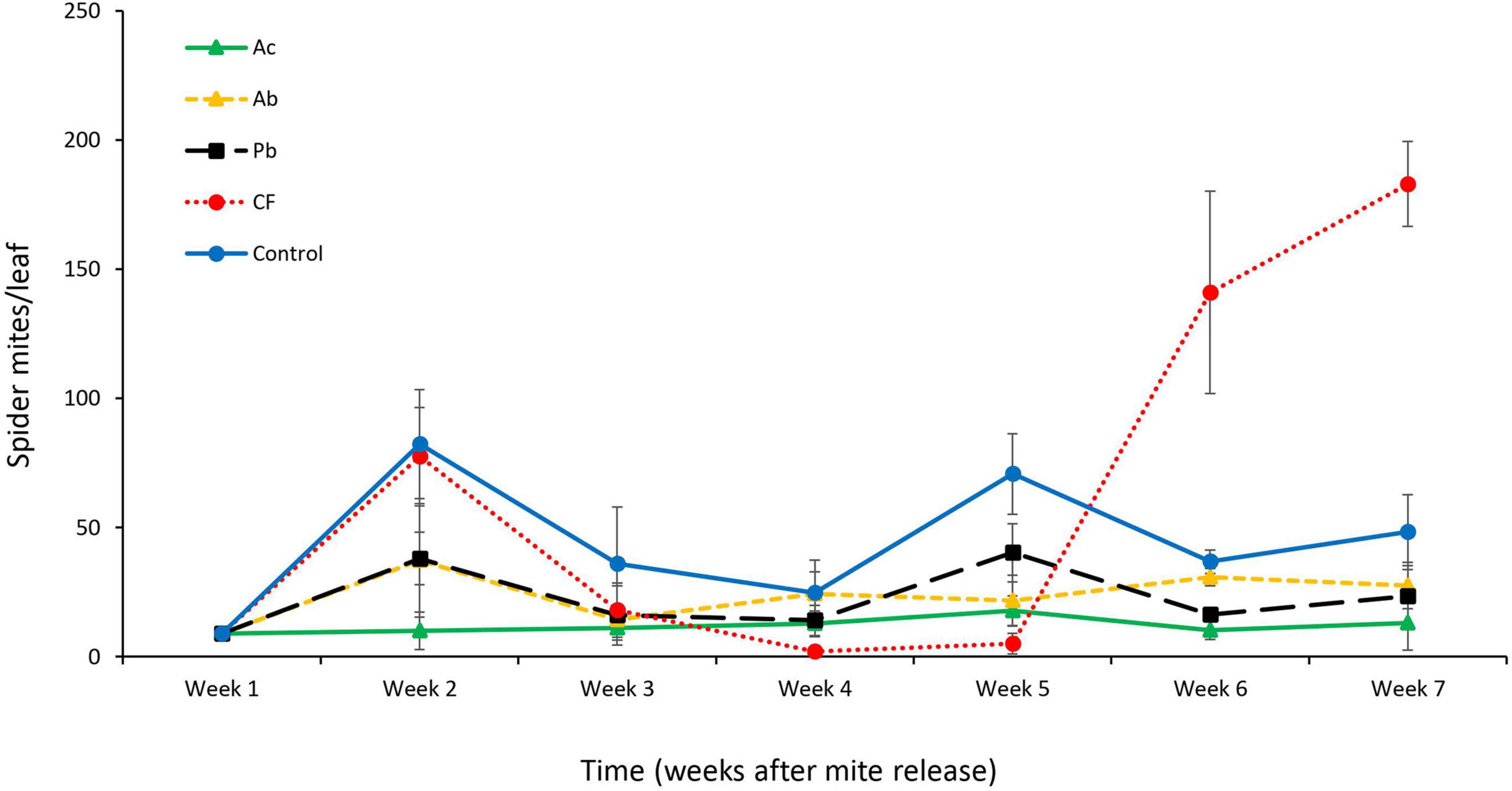
Figure 3. Mean (± SE) number of T. urticae (including all mobile developmental stages and adult males and females) per leaf at each weekly sampling interval (n = 6) on whole strawberry plants receiving one of five treatments, Ac (Azotobacter chroococcum), Ab (Azospirillum brasilense), Pb (Pseudomonas brassicacearum), CF (chemical fertilizer), and control (untreated).
Discussion
Below-ground beneficial micro-organisms may affect the performance of above-ground herbivores through plant-mediated mechanisms that may involve changes in plant physiology including enhancement of the plant’s nutritional state and/or induction of systemic resistance (ISR). The latter often results involves the production of secondary plant metabolites (Dean et al., 2009; Hoffmann et al., 2009; Kempel et al., 2009; Khaitov et al., 2015; Ruiu, 2020; Friman et al., 2021a). There exists ample evidence documenting that below-ground interactions with beneficial micro-organisms enhance nutrition and growth of strawberry plants (e.g., Pırlak and Köse, 2009; Todeschini et al., 2018; Andrade et al., 2019); however, the effects of these belowground interactions on life history performance and population dynamics of the cosmopolitan and most serious herbivorous pest of aboveground strawberry plant tissue, T. urticae, have not yet been studied. Here, we document adverse plant-mediated effects of three species of free-living rhizobacteria, Azotobacter chroococcum (Ac), Azospirillum brasilense (Ab) and Pseudomonas brassicacearum (Pb), on life history and population dynamics of T. urticae feeding on strawberry leaves and plants, and positive effects of these rhizobacteria on flowering and physiology of whole strawberry plants. Spider mites feeding on rhizobacteria-treated strawberry plants had longer developmental times and lower fecundity in comparison to those feeding on untreated and chemically fertilized plants. Overall, the intrinsic rate of increase (rm) of T. urticae was the lowest on Ac- and Ab-treated strawberry leaves and the highest on chemically fertilized strawberry leaves. Young rhizobacteria-treated clean plants produced more flowers than untreated and chemically fertilized plants but had similar numbers of leaves; stomatal conductivity tended to be higher in bacteria (especially Pb)-treated plants than in untreated and chemically fertilized plants whereas chlorophyll content was similar in Ac-treated and untreated plants and higher in chemically fertilized, and Ab- and Pb-treated plants.
Population dynamics of spider mites growing on PGPR-treated, chemically fertilized and untreated whole strawberry plants was in accordance with the adverse PGPR effects on spider mite life history. The mean abundance was lower in spider mite populations feeding on PGPR-treated strawberries than in those feeding on chemically fertilized and untreated plants. Time-dependent population development fluctuated similarly in all treatments except for the chemical fertilizer treatment. Mite abundance dropped to relatively low levels in the chemical fertilizer treatment in week 4 but grew exponentially from week 5 onward (corresponding to the 2nd and 3rd spider mite generation after transfer to the treated plants), which was expected considering the high intrinsic rate of increase. The drop in abundance in week 4 was probably caused by the higher nutrient content of leaf tissue of chemically fertilized plants making the mites staying longer on older leaves, and dispersing later to the newest leaf, than in the other treatments (weekly sampling concerned always the youngest fully developed leaf).
Beneficial below-ground microorganisms may influence the performance of herbivores via two major functional pathways, that is, enhancement of nutrition promoting plant growth and development, which in turn may favor the herbivores, or induced systemic resistance (ISR), which may disfavor the herbivores, or the combination thereof (Kempel et al., 2009; Pineda et al., 2010; Gamalero and Glick, 2011; Friman et al., 2021b). Many studies of above-belowground interactions showed that beneficial soil microorganisms such as free-living rhizobacteria can induce systemic resistance in above-ground plant parts and enhance the level of protection against herbivorous attackers (Zehnder et al., 1997; Koricheva et al., 2009; Khoshfarman-Borji et al., 2020; Ruiu, 2020; Friman et al., 2021a). Regarding interaction between the three species of rhizobacteria used in our study and aboveground herbivores, nothing has been known for P. brassicacearum (Pb). A. brasilense (Ab) had no effects on Hessian flies, Mayetiola destructor, in wheat (Prischmann-Voldseth et al., 2020) and soybean aphids Aphis glycines (Brunner et al., 2015) and positive effects on armyworm Mythimna separata in maize (Li et al., 2018). A. chroococcum (Ac) exerted positive effects on spider mites T. urticae in common bean (Khaitov et al., 2015) and both negative and positive effects (context- and plant genotype-dependent) on armyworm M. separata in maize (Li et al., 2018; Song et al., 2020).
In general, ISR may involve changes in the secondary metabolite profile such as alkaloids, phenols, terpenes, and flavonoids, some of which may inhibit feeding or digestion or have other toxic activity against herbivores (Pineda et al., 2010; Gadhave and Gange, 2013; Disi et al., 2019; Ruiu, 2020). For instance, reduced larval feeding and development of the beet armyworm Spodoptera exigua (Hübner) on Bacillus spp.-treated cotton plants was mediated by increased biosynthesis of gossypol, a phenolic aldehyde with insecticidal property, as compared to untreated plants (Zebelo et al., 2016). Our study revealed that both male and female spider mites, and in each life stage, needed longer time to develop on PGPR-treated strawberry plants and had lower immature survival rates than those fed on chemically fertilized and untreated plants. Reduced age-specific fecundity, longer developmental time and lower age-specific survival rates (as estimators of increased intrinsic rate of increase; Vehrsi et al., 1992) of the mites feeding on rhizobacteria-treated plants reduced their intrinsic rate of increase as compared to mites feeding on chemically fertilized and control plants. Collectively, these results suggest that rhizobacteria inoculation induces phytohormonal signaling (jasmonic acid – JA – and ethylene) that regulates the concentration of secondary plant metabolites (Stout et al., 2002; Pineda et al., 2010, 2013; Pieterse et al., 2014). For example, Steinite and Levinsh (2002, 2003) showed that induced resistance in strawberry, indicated by increased polyphenol oxidase, which is an important JA-inducible marker, negatively affected reproduction of T. urticae. Mouden et al. (2021) reported that induction of the JA pathway enhanced strawberry plant defense against T. urticae via increased production of phenolic acids, flavonoid compounds and anthocyanins. Similarly, Luczynski et al. (1990) observed that the development of T. urticae correlated negatively with foliar concentrations of phenolics. Therefore, we assume that in our study system increased biosynthesis of phenolic compounds and other secondary metabolites in rhizobacteria-treated strawberry plants acted as feeding deterrents, digestion inhibitors and/or toxins for the spider mites. Population dynamics of T. cinnabarinus Boisduval was inhibited in response to increased tannin levels (a polyphenol compound) in cotton (Ma et al., 2021). Similar to our study, Alizade et al. (2016) showed enhanced life history performance and population growth of T. urticae on N-fertilized strawberry plants. Increasing N content of the host plant tissue via chemical N fertilization improved the availability of dietary proteins, which typically benefits herbivores such as two-spotted spider mites (Alizade et al., 2016; Hosseini et al., 2018). Beneficial soil bacteria commonly also improve plant nutrition via increasing the uptake and concentration of a variety of nutrients, including nitrogen, and might also enhance the production of phytohormones that promote growth indicators like the rate of photosynthesis activity (Zhang et al., 2008; Pineda et al., 2010). In our study, application of rhizobacteria increased photosynthesis and growth compared to control plants, resulting in leaf chlorophyll content of Ab- and Pb-treated strawberry plants being similar to that of chemically fertilized plants and PGPR treatments having higher stomatal conductivity than chemically fertilized plants; while vegetative growth, indicated by the number of leaves, did not differ among treatments, reproductive growth, measured as the number of flowers, was significantly enhanced by rhizobacteria application, relative to chemically fertilized and untreated control plants.
Due to possible trade-offs between investment in growth and defense, the positive effects of mutualistic belowground microorganisms–plant interactions on plant growth do not necessarily and not always result in a net fitness benefit for the plant if the fitness of plant-associated herbivores is also enhanced and third trophic level natural enemies are left out (Gehring and Whitham, 2003). We did not assess the net effect of the rhizobacteria on plant growth at simultaneous defense against herbivores, because we just measured the plant characteristics of clean plants; however, our study documents that rhizobacteria can principally enhance both reproductive growth and defense of strawberry plants. Accordingly, we assume that rhizobacteria application may help attacked strawberry plants to limit the damage caused by spider mites, via inducing or increasing the production of secondary metabolites interfering with mite proliferation (Alizade et al., 2016), and to alleviate the deleterious effects of mite damage, via enhancing plant tolerance through vegetative or reproductive regeneration of herbivore-injured plant tissue, as a result of an improved nutritional state (McNaughton, 1983; Disi et al., 2019).
Overall, our results stress the importance of soil microbe-plant interactions on life history and population dynamics of aboveground herbivores. Rhizobacteria inoculation was more favorable than chemical fertilization in terms of decreasing the life history performance of T. urticae and limiting its population growth and improving reproductive growth of strawberry plants. Our study suggests that in crops with well-developed direct anti-herbivore defense systems, such as strawberry, PGPR can efficiently contribute to dampen herbivore infestation and provide for an improved nutritional state of the host plant. Future investigations should explore the consequences of PGPR application on aboveground tri-trophic interactions.
Data Availability Statement
The raw data supporting the conclusions of this article will be made available by the authors, without undue reservation.
Author Contributions
AH and MH conceived the study idea and designed the experiments. AH conducted the experiments, analyzed the data, and wrote the first draft of the manuscript. MH contributed to methods development and managed the project locally. PS gave advice on experimental design and contributed to results analysis and presentation. MH and PS contributed to writing and wrote sections of the manuscript. All authors contributed to manuscript revision, read, and approved the submitted version.
Funding
The authors are grateful to the Iran National Science Foundation for providing financial support for this research (grant no. 98015271). Open access funding was provided by the University of Vienna.
Conflict of Interest
The authors declare that the research was conducted in the absence of any commercial or financial relationships that could be construed as a potential conflict of interest.
Publisher’s Note
All claims expressed in this article are solely those of the authors and do not necessarily represent those of their affiliated organizations, or those of the publisher, the editors and the reviewers. Any product that may be evaluated in this article, or claim that may be made by its manufacturer, is not guaranteed or endorsed by the publisher.
Acknowledgments
We thank Nature Biotechnology Company (Biorun) for the provision of the initial culture of bacterial strains. The research deputy of the Ferdowsi University of Mashhad is acknowledged for their facilities.
References
Alizade, M., Hosseini, M., Modarres Awal, M., Goldani, M., and Hosseini, A. (2016). Effects of nitrogen fertilization on population growth of two-spotted spider mite. Syst. Appl. Acarol. 21, 947–956. doi: 10.11158/saa.21.7.8
Andrade, F., De Assis Pereira, T., Souza, T., Henrique, P., Martins, A., Schwan, R., et al. (2019). Beneficial effects of inoculation of growth-promoting bacteria in strawberry. Microbiol. Res. 223-225, 120–128. doi: 10.1016/j.micres.2019.04.005
Attia, S., Grissa, K., Lognay, G., Bitume, E., Hance, T., and Mailleux, A. C. (2013). A review of the major biological approaches to control the worldwide pest Tetranychus urticae (Acari: Tetranychidae) with special reference to natural pesticides. J. Pest Sci. 86, 361–386. doi: 10.1007/s10340-013-0503-0
Bezemer, T. M., and van Dam, N. M. (2005). Linking aboveground and belowground interactions via induced plant defenses. Trends Ecol. Evol. 20, 617–624. doi: 10.1016/j.tree.2005.08.006
Brunner, S., Goos, R. J., Swenson, S. J., Foster, S., Schatz, B., Lawley, Y., et al. (2015). Impact of nitrogen fixing and plant growth-promoting bacteria on a phloem-feeding soybean herbivore. Appl. Soil Ecol. 86, 71–81. doi: 10.1016/j.apsoil.2014.10.007
Chi, H. (1988). Life-table analysis incorporating both sexes and variable development rates among individuals. Environ. Entomol. 17, 26–34. doi: 10.1093/ee/17.1.26
Chi, H. (2020). TWOSEX-MSChart: A Computer Program for the Age-Stage, Two-Sex Life Table analysis. Available online at: http://140.120.197.173/ecology/ (accessed January 11, 2021).
Chi, H., and Liu, H. S. I. (1985). Two new methods for study of insect population ecology. Bull. Inst. Zool. Acad. Sin. 24, 225–240.
Conti, S., Villari, G., Faugno, S., Melchionna, G., Somma, S., and Caruso, G. (2014). Effects of organic vs. conventional farming system on yield and quality of strawberry grown as an annual or biennial crop in southern Italy. Sci.Hortic. 180, 63–71. doi: 10.1016/j.scienta.2014.10.015
Coskun, D., Britto, D. T., Shi, W., and Kronzucker, H. J. (2017). How plant root exudates shape the nitrogen cycle. Trends Plant Sci. 22, 661–673. doi: 10.1016/j.tplants.2017.05.004
Dam, N. (2009). Belowground herbivory and plant defenses. Annu. Rev. Ecol. Evol. Syst. 40, 373–391. doi: 10.1146/annurev.ecolsys.110308.120314
Dean, J. M., Mescher, M. C., and De Moraes, C. M. (2009). Plant–rhizobia mutualism influences aphid abundance on soybean. Plant Soil 323, 187–196. doi: 10.1007/s11104-009-9924-1
Dellagi, A., Quillere, I., and Hirel, B. (2020). Beneficial soil-borne bacteria and fungi: a promising way to improve plant nitrogen acquisition. J. Exp. Bot. 71, 4469–4479. doi: 10.1093/jxb/eraa112
Disi, J., Simmons, J., and Zebelo, S. (2019). “Plant growth-promoting rhizobacteria-induced defense against insect herbivores,” in Field Crops: Sustainable Management by PGPR. Sustainable Development and Biodiversity, eds D. Maheshwari and S. Dheeman (Cham: Springer), 385–410. doi: 10.1007/978-3-030-30926-8_14
Erturk, Y., and Çakmakçı, R. (2012). Yield and growth response of strawberry to plant growth-promoting rhizobacteria inoculation. J. Plant Nutr. 35, 817–826. doi: 10.1080/01904167.2012.663437
Fahimi, A., Ashouri, A., Ahmadzadeh, M., Hoseini Naveh, V., Asgharzadeh, A., Maleki, F., et al. (2014). Effect of PGPR on population growth parameters of cotton aphid. Arch. Phytopathol. Plant Prot. 47, 1274–1285. doi: 10.1080/03235408.2013.840099
Friman, J., Pineda, A., Gershenzon, J., Dicke, M., and Van Loon, J. J. A. (2021a). Differential effects of the rhizobacterium Pseudomonas simiae on above- and belowground chewing insect herbivores. J. Appl. Entomol. 145, 250–260. doi: 10.1111/jen.12842
Friman, J., Pineda, A., Van Loon, J. J. A., and Dicke, M. (2021b). Bidirectional plant-mediated interactions between rhizobacteria and shoot-feeding herbivorous insects: a community ecology perspective. Ecol. Entomol. 46, 1–10. doi: 10.1111/een.12966
Gadhave, K., Finch, P., Gibson, T., and Gange, A. (2016). Plant growth-promoting Bacillus suppress Brevicoryne brassicae field infestation and trigger density-dependent and density-independent natural enemy responses. J. Pest Sci. 89, 985–992. doi: 10.1007/s10340-015-0721-8
Gadhave, K., and Gange, A. (2016). Plant-associated Bacillus spp. alter life-history traits of the specialist insect Brevicoryne brassicae L. Agric. Forest Entomol. 18, 35–42.
Gamalero, E., and Glick, B. (2011). “Mechanisms used by plant gowth-promoting bacteria,” in Bacteria Agrobiology: Plant Nutrient Management, ed. D. Maheshwari (Berlin: Springer), 17–46. doi: 10.1007/978-3-642-21061-7_2
Gehring, C. A., and Whitham, T. G. (2003). “Mycorrhizae-herbivore interactions: population and community consequences,” in Mycorrhizal Ecology, eds M. G. A. Van Der Heijden and I. R. Sanders (Berlin: Springer), 295–320. doi: 10.1007/978-3-540-38364-2_12
Glick, B. (2013). Bacteria with ACC deaminase can promote plant growth and help to feed the world. Microbiol. Res. 169, 30–39. doi: 10.1016/j.micres.2013.09.009
Hochmuth, G. J., Albregts, E. E., Chandler, C. C., Cornell, J., and Harrison, J. (1996). Nitrogen fertigation requirements of drip-irrigated strawberries. J. Amer. Soc. Hort. Sci. 121, 660–665. doi: 10.17660/ActaHortic.2006.708.12
Hoffmann, D., and Schausberger, P. (2012). Plant-mediated aboveground-belowground interactions: the spider mite perspective. Acarologia 52, 17–27. doi: 10.1051/acarologia/20122040
Hoffmann, D., Vierheilig, H., Riegler, P., and Schausberger, P. (2009). Arbuscular mycorrhizal symbiosis increases host plant acceptance and population growth rates of the two-spotted spider mite Tetranychus urticae. Oecologia 158, 663–671. doi: 10.1007/s00442-008-1179-7
Hoffmann, D., Vierheilig, H., Peneder, S., and Schausberger, P. (2011a). Mycorrhiza modulates aboveground tri-trophic interactions to the fitness benefit of its host plant. Ecol. Entomol. 36, 574–581. doi: 10.1111/j.1365-2311.2011.01298.x
Hoffmann, D., Vierheilig, H., and Schausberger, P. (2011b). Mycorrhiza-induced trophic cascade enhances fitness and population growth of an acarine predator. Oecologia 166, 141–149. doi: 10.1007/s00442-010-1821-z
Hosseini, A., Hosseini, M., Michaud, J. P., Modarres Awal, M., and Ghadamyari, M. (2018). Nitrogen fertilization increases the nutritional quality of Aphis gossypii (Hemiptera: Aphididae) as prey for Hippodamia variegata (Coleoptera: Coccinellidae) and alters predator foraging behavior. J. Econ. Entomol. 111, 2059–2068. doi: 10.1093/jee/toy205
Kempel, A., Brandl, R., and Schädler, M. (2009). Symbiotic soil microorganisms as players in aboveground plant–herbivore interactions–the role of rhizobia. Oikos 118, 634–640. doi: 10.1111/j.1600-0706.2009.17418.x
Khaitov, B., Patiño-Ruiz, J. D., Pina, T., and Schausberger, P. (2015). Interrelated effects of mycorrhiza and free-living nitrogen fixers cascade up to aboveground herbivores. Ecol. Evol. 5, 3756–3768. doi: 10.1002/ece3.1654
Khan, N., Zandi, P., Ali, S., Mehmood, A., and Adnan Shahid, M. (2018). Impact of salicylic acid and PGPR on the drought tolerance and phytoremediation potential of Helianthus annus. Front. Microbiol. 9:2507. doi: 10.3389/fmicb.2019.02222
Khoshfarman-Borji, H., Pahlavan Yali, M., and Bozorg-Amirkalaee, M. (2020). Induction of resistance against Brevicoryne brassicae by Pseudomonas putida and salicylic acid in canola. Bull. Entomol. Res. 110, 597–610. doi: 10.1017/S0007485320000097
Koricheva, J., Gange, A., and Jones, T. (2009). Effects of mycorrhizal fungi on insect herbivores: a meta-analysis. Ecology 90, 2088–2097. doi: 10.1890/08-1555.1
Li, Z., Parajulee, M., and Chen, F. (2018). Influence of elevated CO2 on development and food utilization of armyworm Mythimna separata fed on transgenic Bt maize infected by nitrogen-fixing bacteria. PeerJ 6:e5138. doi: 10.7717/peerj.5138
Lingua, G., Bona, E., Manassero, P., Marsano, F., Todeschini, V., Cantamessa, S., et al. (2013). Arbuscular mycorrhizal fungi and plant growth-promoting pseudomonads increases anthocyanin concentration in strawberry fruits (Fragaria x ananassa var. Selva) in conditions of reduced fertilization. Int. J. Mol. Sci. 14, 16207–16225. doi: 10.3390/ijms140816207
Luczynski, A., Isman, M. B., and Raworth, D. A. (1990). Strawberry foliar phenolics and their relationship to development of the twospotted spider mite. J. Econ. Entomol. 83, 557–563. doi: 10.1093/jee/83.2.557
Ma, H., Xin, C., Xu, Y., Wang, D., Lin, X., and Chen, Z. (2021). Effect of salt stress on secondary metabolites of cotton and biological characteristics and detoxification enzyme activity of cotton spider mites. Crop Prot. 141:105498. doi: 10.1016/j.cropro.2020.105498
Martín, L., Velázquez, E., Rivas, R., Mateos, P. F., Martínez, M. E., Rodríguez, B., et al. (2007). “Effect of inoculation with a strain of Pseudomonas fragi in the growth and phosphorous content of strawberry plants,” in Developments in Plant and Soil Sciences, eds E. Velázquez and C. Rodríguez-Barrueco (Dordrecht: Springer), 309–315. doi: 10.1007/978-1-4020-5765-6_49
McNaughton, S. J. (1983). Compensatory plant growth as a response to herbivory. Oikos 40, 329–336. doi: 10.2307/3544305
Migeon, A., Nouguier, E., and Dorkeld, F. (2010). “Spider mites web: a comprehensive database for the Tetranychidae,” in Trends in Acarology, eds M. W. Sabelis and J. Bruin (Netherlands: Springer), 557–560.
Mou, D. F., Lee, C. C., Smith, C. L., and Chi, H. (2014). Using viable eggs to accurately determine the demographic and predation potential of Harmonia dimidiata (Coleoptera: Coccinellidae). J. Appl. Entomol. 139, 579–591. doi: 10.1111/jen.12202
Mouden, S., Bac-Molenaar, J. A., Kappers, I. F., Beerling, E. A. M., and Leiss, K. A. (2021). Elicitor application in strawberry results in long-term increase of plant resilience without yield loss. Front. Plant Sci. 12:1321. doi: 10.3389/fpls.2021.695908
Nunes, K. A., and Kotanen, P. M. (2017). Comparative impacts of aboveground and belowground enemies on an invasive thistle. Ecol. Evol. 8, 1430–1440. doi: 10.1002/ece3.3751
Pangesti, N., Weldegergis, B., Langendorf, B., Van Loon, J., Dicke, M., and Pineda, A. (2015). Rhizobacterial colonization of roots modulates plant volatile emission and enhances the attraction of a parasitoid wasp to host-infested plants. Oecologia 178, 1169–1180. doi: 10.1007/s00442-015-3277-7
Pappas, M. L., Liapoura, M., Papantoniou, D., Avramidou, M., Kavroulakis, N., Weinhold, A., et al. (2018). The beneficial endophytic fungus Fusarium solani strain K alters tomato responses against spider mites to the benefit of the plant. Front. Plant Sci. 9:1603. doi: 10.3389/fpls.2018.01603
Pappas, M. L., Samaras, K., Koufakis, I., and Broufas, G. D. (2021). Beneficial soil microbes negatively affect spider mites and aphids in pepper. Agronomy 11:1831.
Patiño-Ruiz, J. D., and Schausberger, P. (2014). Spider mites adaptively learn recognizing mycorrhiza induced changes in host plant volatiles. Exp. Appl. Acarol. 64, 455–463. doi: 10.1007/s10493-014-9845-4
Pieterse, C. M. J., Zamioudis, C., Berendsen, R. L., Weller, D. M., Wees, S. C. M. V., and Bakker, P. A. H. M. (2014). Induced systemic resistance by beneficial microbes. Annu.Rev. Phytopathol. 52, 347–375. doi: 10.1146/annurev-phyto-082712-102340
Pineda, A., Soler, R., Weldegergis, B., Shimwela, M., Van Loon, J., and Dicke, M. (2013). Non-pathogenic rhizobacteria interfere with the attraction of parasitoids to aphid-induced plant volatiles via jasmonic acid signalling. Plant Cell Environ. 36, 393–404. doi: 10.1111/j.1365-3040.2012.02581.x
Pineda, A., Zheng, S.-J., Van Loon, J. J. A., Pieterse, C. M. J., and Dicke, M. (2010). Helping plants to deal with insects: the role of beneficial soil-borne microbes. Trends Plant Sci. 15, 507–514. doi: 10.1016/j.tplants.2010.05.007
Pırlak, L., and Köse, M. (2009). Effects of plant growth promoting rhizobacteria on yield and some fruit properties of strawberry. J. Plant Nutr. 32, 1173–1184. doi: 10.1080/01904160902943197
Pourya, M., Shakarami, J., Mardani-Talaee, M., Sadeghi, A., and Serrão, J. E. (2020). Induced resistance in wheat Triticum aestivum L. by chemical- and bio- fertilizers against english aphid Sitobion avenae (Fabricius) (Hemiptera: Aphididae) in greenhouse. Int. J. Trop. Insect Sci. 40, 1043–1052. doi: 10.1007/s42690-020-00164-1
Prischmann-Voldseth, D. A., Özsisli, T., Aldrich-Wolfe, L., Anderson, K., and Harris, M. O. (2020). Microbial inoculants differentially influence plant growth and biomass allocation in wheat attacked by gall-inducing Hessian Fly (Diptera: Cecidomyiidae). Environ. Entomol. 49, 1214–1225. doi: 10.1093/ee/nvaa102
Roussos, P., Triantafillidis, A., and Kepolas, E. (2012). Strawberry fruit production and quality under conventional, integrated and organic management. Acta Hortic. 926, 541–546. doi: 10.17660/ActaHortic.2012.926.76
Ruiu, L. (2020). Plant-growth-promoting bacteria (PGPB) against insects and other agricultural pests. Agronomy 10:861. doi: 10.3390/agronomy10060861
Shah, A., Azari, M., Antar, M., Msimbira, L. A., Naamala, J., Lyu, D., et al. (2021). PGPR in agriculture: a sustainable approach to increasing climate change resilience. Front. Plant Sci. 5:667546. doi: 10.3389/fsufs.2021.667546
Song, Y., Liu, J., and Chen, F. (2020). Azotobacter chroococcum inoculation can improve plant growth and resistance of maize to armyworm, Mythimna separata even under reduced nitrogen fertilizer application. Pest Manag. Sci. 76, 4131–4140. doi: 10.1002/ps.5969
Steenhoudt, O., and Vanderleyden, J. (2000). Azospirillum, a free-living nitrogen-fixing bacterium closely associated with grasses: genetic, biochemical and ecological aspects. FEMS Microbiol. Rev. 24, 487–506. doi: 10.1111/j.1574-6976.2000.tb00552.x
Steinite, I., and Levinsh, G. (2002). Wound-induced responses in leaves of strawberry cultivars differing in susceptibility to spider mite. J. Plant Physiol. 159, 491–497.
Steinite, I., and Levinsh, G. (2003). Possible role of trichomes in resistance of strawberry cultivars against spider mite. Acta Univ. Latviensis 662, 59–65.
Stout, M., Zehnder, G., and Baur, M. (2002). Potential for the use of elicitors of plant resistance in arthropod management programs. Arch. Insect Biochem. Physiol. 51, 222–235. doi: 10.1002/arch.10066
Tabatabaei, S. J., Fatemi, L. S., and Fallahi, E. (2006). Effect of ammonium: nitrate ratio on yield, calcium concentration, and photosynthesis rate in strawberry. J. Plant Nutr. 29, 1273–1285. doi: 10.1080/01904160600767575
Todeschini, V., Aitlahmidi, N., Mazzucco, E., Marsano, F., Gosetti, F., Robotti, E., et al. (2018). Impact of beneficial microorganisms on strawberry growth, fruit production, nutritional quality, and volatilome. Front. Plant Sci. 9:1611. doi: 10.3389/fpls.2018.01611
Valenzuela-Soto, J. H., Estrada-Hernández, M. G., Ibarra-Laclette, E., and Délano-Frier, J. P. (2009). Inoculation of tomato plants (Solanum lycopersicum) with growth-promoting Bacillus subtilis retards whitefly Bemisia tabaci development. Planta 231:397. doi: 10.1007/s00425-009-1061-9
Van der Putten, W. H., Vet, L. E. M., Harvey, J. A., and Wäckers, F. L. (2001). Linking above- and belowground multitrophic interactions of plants, herbivores, pathogens, and their antagonists. Trends Ecol. Evol. 16, 547–554. doi: 10.1016/S0169-5347(01)02265-0
Vehrsi, S., Walker, G., and Parrella, M. (1992). Comparison of population growth rate and within-plant distribution between Aphis gossypii and Myzus persicae (Homoptera: Aphididae) reared on potted chrysanthemums. J. Econ. Entomol. 85, 799–807. doi: 10.1093/jee/85.3.799
Wermelinger, B., Oertli, J. J., and Baumgärtner, J. (1991). Environmental factors affecting the life-tables of Tetranychus urticae (Acari: Tetranychidae). III. Host-plant nutrition. Exp. Appl. Acarol. 12, 259–274. doi: 10.1007/BF01193472
Wermelinger, B., Oertli, J. J., and Delucchi, V. (1985). Effect of host plant nitrogen fertilization on the biology of the two-spotted spider mite, Tetranychus urticae. Entomol. Exp. Appl. 38, 23–28. doi: 10.1111/j.1570-7458.1985.tb03493.x
Zamioudis, C., and Pieterse, C. (2012). Modulation of host immunity by beneficial microbes. Mol. Plant Microbe Interact. 25, 139–150. doi: 10.1094/MPMI-06-11-0179
Zebelo, S., Song, Y., Kloepper, J. W., and Fadamiro, H. (2016). Rhizobacteria activates (C)-d-cadinene synthase genes and induces systemic resistance in cotton against beet armyworm (Spodoptera exigua). Plant Cell Environ. 39, 935–943. doi: 10.1111/pce.12704
Zehnder, G., Kloepper, J., Yao, C., and Wei, G. (1997). Induction of systemic resistance in cucumber against cucumber beetles (Coleoptera: Chrysomelidae) by plant growth-promoting rhizobacteria. J. Econ. Entomol. 90, 391–396. doi: 10.1093/jee/90.2.391
Keywords: below-above ground interactions, plant growth-promoting rhizobacteria, plant growth, plant physiology, spider mite, life table, population dynamics
Citation: Hosseini A, Hosseini M and Schausberger P (2022) Plant Growth-Promoting Rhizobacteria Enhance Defense of Strawberry Plants Against Spider Mites. Front. Plant Sci. 12:783578. doi: 10.3389/fpls.2021.783578
Received: 26 September 2021; Accepted: 08 December 2021;
Published: 06 January 2022.
Edited by:
Katharina Pawlowski, Stockholm University, SwedenReviewed by:
Simon Zebelo, University of Maryland Eastern Shore, United StatesMaria L. Pappas, Democritus University of Thrace, Greece
Vladimir Zhurov, Western University, Canada
Copyright © 2022 Hosseini, Hosseini and Schausberger. This is an open-access article distributed under the terms of the Creative Commons Attribution License (CC BY). The use, distribution or reproduction in other forums is permitted, provided the original author(s) and the copyright owner(s) are credited and that the original publication in this journal is cited, in accordance with accepted academic practice. No use, distribution or reproduction is permitted which does not comply with these terms.
*Correspondence: Mojtaba Hosseini, m.hosseini@um.ac.ir; Peter Schausberger, peter.schausberger@univie.ac.at