- 1School of Agriculture and Biology, Shanghai Jiao Tong University, Shanghai, China
- 2Department of Laboratory Medicine and Pathology, Mayo Clinic, Rochester, MN, United States
Flavonoids belong to the family of polyphenolic secondary metabolites and contribute to fruit quality traits. It has been shown that MBW complexes (MYB-bHLH-WD40) regulate the flavonoids biosynthesis in different plants, but only a limited number of MBW complexes have been identified in strawberry species in general. In this study, we identified 112 R2R3-MYB proteins in woodland strawberry; 12 of them were found to have potential functions in regulating flavonoids biosynthesis by phylogenetic analysis. qRT-PCR assays showed that FvMYB3, FvMYB9, FvMYB11, FvMYB22, FvMYB64, and FvMYB105 mostly expressed at green stage of fruit development, aligned with proanthocyanidins accumulation; FvMYB10 and FvMYB41 showed higher expression levels at turning and ripe stages, aligned with anthocyanins accumulation. These results suggest that different MYBs might be involved in flavonoids biosynthesis at specific stages. Furthermore, FvMYB proteins were demonstrated to interact with FvbHLH proteins and induce expression from the promoters of CHS2 and DFR2 genes, which encode key enzymes in flavonoids biosynthesis. The co-expression of FvMYB and FvbHLH proteins in strawberry fruits also promoted the accumulation of proanthocyanidins. These findings confirmed and provided insights into the biofunction of MBW components in the regulation of flavonoid biosynthesis in woodland strawberry.
Introduction
Strawberry is favored by consumers mainly because of its unique aroma, sweet taste, bright color and nutritional value. These quality traits are largely determined by the secondary metabolites in the fruit. Flavonoids, which are natural polyphenol compounds, consist of six major subgroups, including chalcones, flavones, flavonols, flavandiols, anthocyanins, and proanthocyanidins (Li, 2014). Flavonoids not only give plants their attractive colors for pollen and seed dispersal, but also protect plants against ultraviolet radiation (Winkel-Shirley, 2001; Emiliani et al., 2013). In addition, flavonoids may act as antioxidants or signaling molecules to exert potential beneficial effects on human health (He and Giusti, 2010), as well as being antimicrobial agents in plant-microbe interactions and plant defense response (Nijveldt et al., 2001; Battino et al., 2009).
Flavonoids are synthesized from malonyl-CoA produced by the fatty acid metabolic pathway and 4-coumaroyl-CoA produced by the phenylpropanoid metabolic pathway with the catalysis by a variety of enzymes. In general, three molecules of malonyl-CoA and one molecule of 4-coumaroyl-CoA were converted into one anthocyanin under a successive catalytic action of many enzymes, including chalcone synthase (CHS), chalcone isomerase (CHI), flavonoid 3-hydroxylase (F3H), flavonol synthase (FLS), flavonoid 3′-hydroxylase (F3′H), dihydroflavonol-4-reductase (DFR), anthocyanidin synthase (ANS), leucoanthocyanidin reductase (LAR), anthocyanidin reductase (ANR), and 3-glycosyltransferase (3-GT). In the synthesis pathway of anthocyanins, the intermediate metabolites dihydrokaempferol and dihydroquercetin, products of F3H, can be oxidized by FLS to produce flavonols. Similarly, the products of DFR and ANS, leucocyanidin, and cyanidin, can also be converted into proanthocyanidins precursors by LAR and ANR, respectively.
Flavonoids biosynthesis has been well studied in many plants and is largely regulated at the transcriptional level by MYB-bHLH-WD40 (MBW) complex. The MYB protein family is one of the largest families of transcription factors in plants. According to the number of R motifs contained in a MYB gene, it could be divided into four subfamilies, namely 1R-MYB with one R domain, 2R-MYB (R2R3-MYB) with two R domains, and 3R-MYB or 4R-MYB with three or four R domains. Among the four subfamilies, R2R3-MYB is the most abundant, which is widely involved in regulating plant growth and development, responses to biotic and abiotic stresses, as well as environmental factors (Dubos et al., 2010). MBW comprises three classes of regulatory proteins, including R2R3-MYBs, bHLHs and TRANSPARENT TESTA GLABROUS1 (TTG1; also termed WD40) (Li, 2014). In Arabidopsis, it has been reported that the three regulatory proteins, namely AtTT2/AtMYB123 (Nesi et al., 2001; Dubos et al., 2010), AtTT8/AtbHLH042 (Nesi et al., 2000), and AtTTG1 (WD40-repeat protein) (Walker et al., 1999; Gonzalez et al., 2009), act together as a MBW complex to promote the production of proanthocyanidins in seed coat, by activating the expression of target gene BAN/ANR (Baudry et al., 2004). The genes involved in the synthesis and accumulation of anthocyanins are also regulated by MBW complexes, comprise at least one R2R3-MYB protein from AtMYB75, AtMYB90, AtMYB113 and AtMYB114, one bHLH protein from TT8, GLABROUS3 (GL3) and ENHANCER OF GLABRA3 (EGL3), and one WD40 protein, AtTTG1 (Dubos et al., 2010; Xu et al., 2015).
For fruit crops, such as grapevine, apple, peach and strawberry, the identification of key MYB proteins have significantly contributed to the understanding of the regulation of fruit flavonoids synthesis (Azuma et al., 2008; Terrier et al., 2009; Zhang et al., 2014; Zoratti et al., 2014; Tuan et al., 2015). Schaart et al. (2013) identified FaMYB9 and FaMYB11 as regulators of proanthocyanidins in octoploid strawberry by a strategy combining yeast-two-hybrid screening and agglomerative hierarchical clustering of transcriptomic and metabolomic data. FvMYB10 has been well studied for its function in the accumulation of anthocyanin in woodland strawberry (Lin-Wang et al., 2014; Castillejo et al., 2020). In the MBW complex, MYB protein is the decisive component for the regulation of flavonoids synthesis. The R2R3 motif at the N-terminal of MYB is responsible for the specific binding to the promoter of target genes, while the C-terminal is responsible for the activation or inhibition of the expression of target genes (Mehrtens et al., 2005; Prouse and Campbell, 2012; Franco-Zorrilla et al., 2014; Kelemen et al., 2015). bHLH protein functions by interacting with MYB (Grotewold et al., 1994). WD40 protein seems to have a more general role in the regulatory complex (Hichri et al., 2010). bHLH proteins in MBW complexes also play important roles in anthocyanin accumulation. There are 133 bHLHs in Arabidopsis, three of which have been proven to be related to anthocyanin formation. AtTT8 is a key regulator of anthocyanin and proanthocyanidins biosynthesis. AtEGL3 and AtGL3 mainly act in vegetative tissues (Ramsay and Glover, 2005; Gonzalez et al., 2008). In petunia, the bHLH protein AN1 and JAF13 can interact with AN2 (a R2R3-MYB protein) and WD40 protein AN11, thereby activating anthocyanin biosynthesis genes (Quattrocchio et al., 1993; deVetten et al., 1997; Spelt et al., 2000). In grape, apple and pear, several bHLH proteins, such as VvMYC1, MdbHLH3, MdbHLH33, PpbHLH3, and PpbHLH33, have been found to promote anthocyanin accumulation, and these proteins can form MBW complexes with MYB proteins (Espley et al., 2007; Hichri et al., 2010; Lin-Wang et al., 2014). Similarly, FvbHLH33 can help FvMYB10 to activate the expression of FvDFR and FvUFGT, key enzyme genes of anthocyanin synthesis. However, there is still limited information available on the transcriptional regulation of the flavonoids biosynthesis by the MBW complexes, especially in the fruits of woodland strawberry.
To identify additional regulatory proteins involved in the flavonoids biosynthesis pathways in woodland strawberry, we first identified the R2R3-MYB gene family using latest genome database version 4.0.2a. Novel MYB and bHLH genes that are possibly related to flavonoids biosynthesis were identified through homology alignment and comprehensive phylogenetic analysis. The corresponding MYB and bHLH proteins were confirmed to form functional complexes using molecular and biochemical experiments. The results from our study contribute to the understanding of flavonoids biosynthesis in the fruits of woodland strawberry at molecular levels.
Materials and Methods
Plant Materials and Growth Conditions
Fragaria vesca seeds of RG (Ruegen, runnerless, everbearing, and red fruited) were grown in flowerpots and cultivated in photoperiodic conditions of 16 h of light and 8 h of dark at a temperature of 22°C, and a relative humidity of 50%.
Identification of R2R3-MYB Proteins in Woodland Strawberry
All the protein sequences of F. vesca were downloaded from GDR database1 and the genome database version 4.0.2a was used. An HMM search with the MYB DNA-binding domain HMM profile (PF000249) was used to blast against strawberry protein database and the e-value was set as 1.0 × 10––3. Because of the different splicing models for many genes in version 4.0.2a, a total of 499 MYB gene transcripts were obtained as a result. For the different splicing of each MYB gene, the longest transcript was preserved and the remaining was removed, resulting in 242 MYB transcripts obtained. To confirm the presence of MYB DNA-binding domain, the protein sequences of the 242 MYB transcripts were used as queries to search against Pfam2, SMART3, and HMMER4 database, the e-value was set as 1.0 × 10––5. In the end, a total of 118 MYB protein sequences containing more than two MYB DNA-binding domains were obtained. The isoelectric points and molecular weights of the corresponding MYB proteins were obtained from the online database of ExPASY5.
Phylogenetic Analysis
The 125 R2R3-MYB genes identified in Arabidopsis were obtained from a previous study (Dubos et al., 2010). The MYB protein sequences were downloaded from The Arabidopsis Information Resource (TAIR)6. The sequences of MYB proteins from Arabidopsis and strawberry were aligned using ClustalW (Thompson et al., 1994), and the BLOSUM matrix was used. The parameters of gap opening and extension penalties were 25 and 1, respectively, and the other parameters were default. The phylogenetic tree was constructed using the Neighbour Joining Tree Method in Mega5 (Tamura et al., 2011). The bootstrap was 1,000 replicates. Evolutionary distances were computed with P-distance method and the positions containing gaps and missing data were eliminated with partial deletion option (95%). For the MYB and bHLH proteins, and other corresponding proteins from different species, the phylogenetic tree was constructed as above.
Yeast Two-Hybrid Assay
Full CDS of bHLH and MYB genes were amplified and then inserted into BD vector pLexA/pGBKT7 and AD vector pB42AD/pGADT7, respectively, between EcoR I and Xho I using ClonExpress II One Step Cloning Kit (Vazyme Biotech Co., Ltd.). All sequences of the recombinant plasmids were verified by sequencing (Sangon Biotech Co., Ltd. at Shanghai). The LexA yeast two-hybrid was performed as described previously (Xu et al., 2019). For the GAL4 yeast two-hybrid assay, the bait and prey vectors were co-transformed into AH109 yeast cells as described by Lu et al. (2015). The primers used were listed in Supplementary Table 1.
Yeast One-Hybrid Assay
The 2.0 kb upstream sequences before the ATG of FvCHS2 and FvDFR2 genes were amplified from woodland strawberry of RG and inserted into the reporter vector pLacZ using homologous recombination method as above. The recombinant effector plasmids with inserted MYB or bHLH genes and the reporter plasmids were co-transformed into EGY48 yeast cells and grown at 30°C for 3 days. Then the DNA-binding activity were detected as described in the previous study (Li et al., 2020). To investigate whether bHLH can affect the bioactivity of MYB protein, the cDNA encoding the full-length bHLH was inserted into a modified pLexA vector, which lacks the BD domain. The two effector plasmids harboring bHLH and MYB genes, respectively, were co-expressed with the reporter plasmid in EGY48 yeast cells. Transformed colonies were selected on SD-Trp-His-Ura medium plus X-gal as described previously (Li et al., 2020). The sequences of the CHS2 and DFR2 promoters were listed in Supplementary Table 2 and the potential cis-elements of the CHS2 and DFR2 promoters for MBW binding were analyzed by PlantCARE7 (Lescot et al., 2002). The primers used were listed in Supplementary Table 1.
Quantitative RT-PCR Analysis
Strawberry fruits at green stage (about 15 days post anthesis, DPA), white stage (about 22 DPA), pre-turning stage (white receptacles with red achenes), turning stage (2 or 3 days after the pre-turning stage), and ripen stage (3 or 4 days after the turning stage) were harvested. The achenes were removed from all the fruits. and then the total RNA was extracted following the manufacturer’s instructions of RNA Plant Plus Reagent (Tiangen, Beijing, China, Cat.DP437). DNase I was used to remove the genomic DNA contamination. First-strand cDNA was synthesized using EasyScript® One-Step gDNA Removal and cDNA Synthesis SuperMix (Transgen, China). Real-time qPCR was performed using the CFX96 TouchTM Real-Time PCR System (Bio-Rad, United States) with 2 × M5 HiPer SYBR Premix EsTaq (with Tli RNaseH) (Meibio, China, Cat. MF787-01). The qPCR reaction was performed by pre-denaturing at 95°C for 1 min, followed by 40 cycles of denaturing at 95°C for 5 s, annealing at 60°C for 15 s, and extension at 72°C for 15 s. Melting curve detection from 65 to 95°C was done after the cyclic reactions. Transcript abundance of target genes were calculated using 2–ΔCt method in comparison with the internal control gene, Fv26S or FvActin. Three biological replicates were used in each assay. The experiments were repeated at least three times. The primer sequences were listed in Supplementary Table 3.
BiFC Assay
The vectors used to produce constructs for the BiFC assays were pXY104 and pXY106, which carry fragments encoding the C- and N-terminal halves of YFP (cYFP and nYFP), respectively. The full-length cDNAs of MYB and bHLH genes were cloned into pXY104 and/or pXY106 vector to generate corresponding recombinant plasmids. All constructs were transformed into Agrobacterium tumefaciens strain GV3101 and infiltrated into tobacco (Nicotiana benthamiana) epidermal leaves in the given combinations as described previously (Lu et al., 2015; Xu et al., 2016). After incubation in the dark for 36–40 h, the tobacco leaf samples were examined by confocal microscopy (Leica TCS SP5II) to detect the expression of various fluorescent proteins. The intensity of the laser was set at 30%, the wavelength at 514 nm, and the voltage at 700 v. All the primers used were listed in Supplementary Table 1.
Luciferase Reporter Assay
The pGreen0800-ProDFR2:Luc vector was described previously (Li et al., 2020). The full length CDS of MYB and bHLH genes including FvMYB22, FvMYB64, FvMYB105, and FvbHLH33 were cloned into pGreen62SK vector and served as effectors. The reporter strain GV3101 harboring ProDFR2:Luc was mixed with each of the effector strain harboring pGreen62SK, pGreen62SK-bHLH33 or one of the pGreen62SK-FvMYB plasmids (pGreen62SK-FvMYB22, pGreen62SK-FvMYB64, or pGreen62SK-FvMYB105) individually, as well as combinations of plasmids indicated. The mixed culture of each combination was infiltrated into tobacco leaves as described in the results of this article. After incubation in the dark for 36–40 h, the N. benthamiana leaves were treated with 1 mM l-luciferin sodium salt (Yeasen, Shanghai, China, Cat. 40903ES02) and kept in the dark for an additional 10 min before the bioluminescence signal were imaged and the intensity measured by a luminescent imaging workstation (5200; Tanon, Shanghai, China). The primers used for luciferase reporter assay are listed in Supplementary Table 1.
Detection of Anthocyanin and Proanthocyanidin
The method of anthocyanin detection was described in previous studies (Xu et al., 2018; Li et al., 2020). The method of proanthocyanidins detection was described in previous study (Li et al., 2018). DMACA, (−)-epicatechin and 4-hydroxy-3-methoxybenzaldehyde used in the experiments were from Bide Pharmatech. Ltd. (Shanghai, China).
Transient Overexpression in Strawberry Fruit
The construct of pGreen62SK, pGreen62SK-bHLH33 or one of the pGreen62SK-FvMYB plasmids (pGreen62SK-FvMYB22, pGreen62SK-FvMYB64, or pGreen62SK-FvMYB105) was used for transient overexpression. The method of injection was described in previous study (Zhang et al., 2020). The octoploid strawberry of “HongYan” was used for transient overexpression. The fruit were collected at 7 days after transfection and the measurement of proanthocyanidins was described in the same previous study (Xu et al., 2018). The expression of FvbHLH33, FvMYB22, FvMYB64, FvMYB105, FvCHS2, and FvDFR2 was confirmed by qRT-PCR assay.
Results
Genome Wide Identification of R2R3-MYB Genes in Fragaria vesca
In order to identify R2R3-MYB genes in strawberry, the HMM profile (Pfam:00249) of MYB DNA-binding domain was used to blast against the woodland strawberry genome database (F. vesca Whole Genome v4.0.a2) from GDR (see Text Footnote 1). The blast search resulted in a total of 242 MYB genes after removing repetitive redundant sequences. The protein products of 118 MYB genes out of the 242 were verified to contain conserved MYB DNA-binding domains through searching Pfam, SMART and HMMER databases. All 118 MYB gene products contain more than two MYB repeat units. Among them, one contains four MYB repeat units, five contain three MYB repeat units. The remaining 112 contain two MYB repeat units, which are R2R3-MYBs (Supplementary Table 4). Through gene sequence alignment analysis, we found that FvH4_1G22020 shares the same sequence with FvMYB10, thus annotated as FvMYB10. Blast results showed that the sequences of FvH4_2g31100 and FvH4_6g34650 were almost consistent with FaMYB9 and FaMYB11, respectively. We therefore named FvH4_2g31100 and FvH4_6g34650 as FvMYB9 and FvMYB11. The remaining R2R3-MYB genes were named according to the order of their chromosomal locations in the strawberry genome browser (Supplementary Table 4). The physical and chemical property prediction analysis was performed for the 112 R2R3-MYB proteins using ExPASy database. The results showed that the sizes of these MYB proteins are significantly different. For example, the protein product of FvH4_7g19850 is composed of 1703 amino acids, while other MYB proteins are much smaller, containing amino acids of a number ranging from 127 to 562. The isoelectric points of MYB proteins have been predicted to range from 4.82 to 10.10, and the presumed molecular weights are in a range of 15–62.7 kD, except for the protein product of FvH4_7g19850, the molecular weight of which is 185 kD (Supplementary Table 4).
Identification of Potential MYB and bHLH Proteins Involved in Regulating Flavonoids Biosynthesis
To identify which R2R3-MYB genes in strawberry are possibly involved in flavonoids biosynthesis, the sequences of the 112 strawberry R2R3-MYB genes identified by us and the 125 R2R3-MYB genes from Arabidopsis were phylogenetically analyzed. The results showed that a total of 17 strawberry MYB genes clustered with AtMYBs that are involved in flavonoids biosynthesis (highlighted in black solid circles in Figure 1). We also included R2R3-MYB genes from other species with known functions in flavonoids synthesis for comparison, including those from grape, apple, cotton, tomato, maize and freesia. These R2R3-MYB genes were also phylogenetically analyzed together with the 17 strawberry MYB genes. Taken together,12 strawberry R2R3-MYB genes were identified as potentially involved in flavonoids biosynthesis (highlighted in black solid circles, Supplementary Figure 1). Specifically, FvMYB10 and FvMYB75 clustered with MdMYB10 and MdMYB1, which promote anthocyanin biosynthesis (Espley et al., 2007). FvMYB3, FvMYB9, and FvMYB11 clustered with FaMYB9 and FaMYB11, which were found to complement the phenotype of transparent testa in Arabidopsis tt2-1 mutant (Schaart et al., 2013), suggesting a function of regulating proanthocyanidins biosynthesis. FvMYB64 and FvMYB105 were homologous to VvMYBPA1, which is specific to the regulation of proanthocyanidins biosynthesis in seeds (Bogs et al., 2007). FvMYB45 and FvMYB77 were similar to maize P (Grotewold et al., 1994) and AtMYB111 (Stracke et al., 2007), respectively, suggesting that both of them might be related to flavonols synthesis. FvMYB41 clustered with VvMYB5b, which contributes to the regulation of anthocyanin and proanthocyanidins biosynthesis in the developing grape fruits (Deluc et al., 2008). AtMYB123 did not explicitly cluster with any known genes, but when AtMYB123 was used as a query to blast against strawberry genome, FvMYB21 and FvMYB22 was also among the results, displaying high similarity to AtMYB123, suggesting that FvMYB21 and FvMYB22 are potentially related to proanthocyanidins biosynthesis.
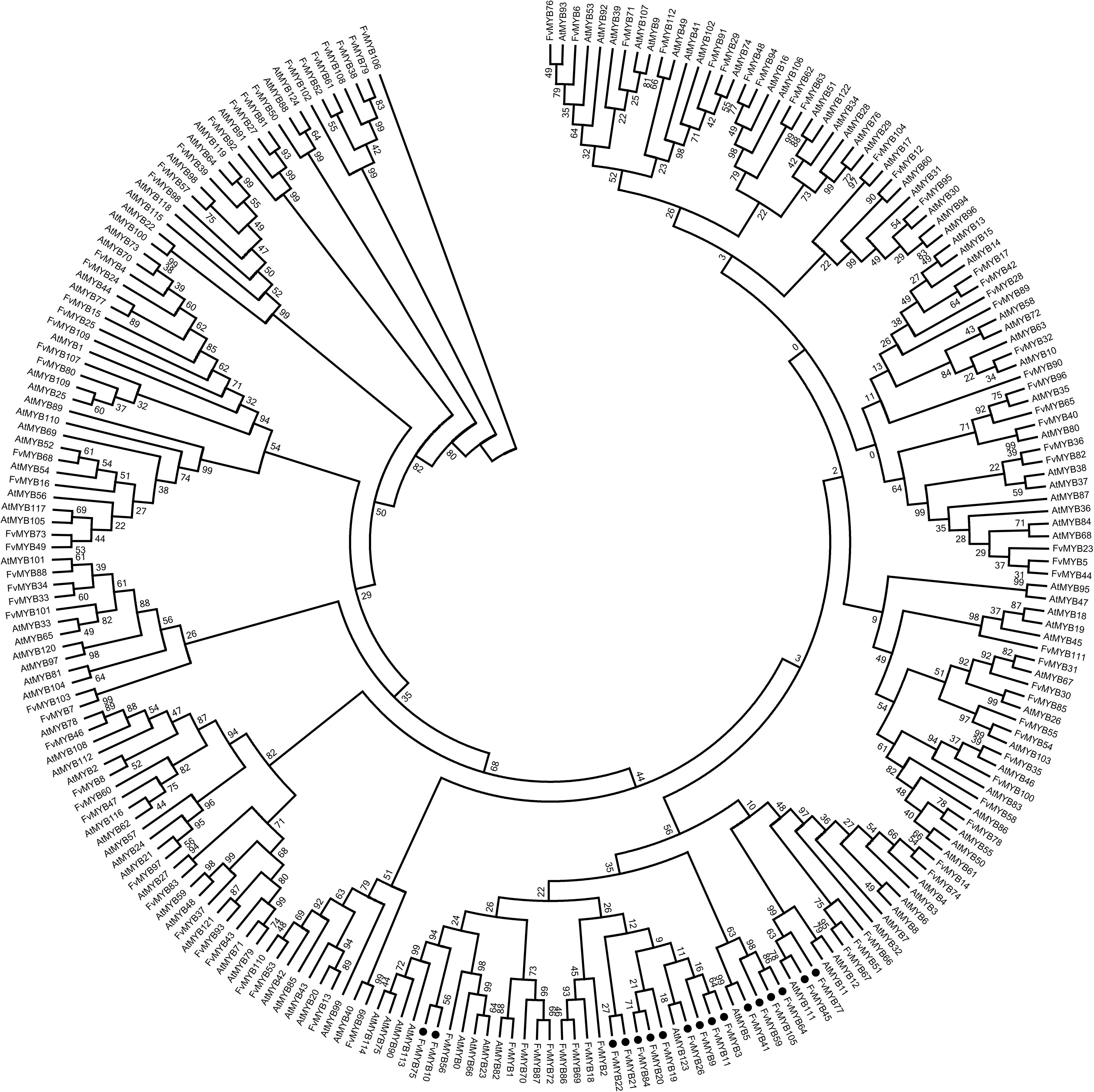
Figure 1. Phylogenetic analysis of R2R3-MYB proteins of Arabidopsis and woodland strawberry. The numbers above the line are the bootstrap values. Black solid circles indicate those related to flavonoid biosynthesis.
To screen bHLH components of MBW complexes, we used three Arabidopsis bHLH proteins as queries to perform BLASTP searches against F. vesca protein database. The three Arabidopsis bHLH proteins, including AtTT8, AtEGL3, and AtGL3, have been shown to have partially overlapping expression patterns and redundant functions in flavonoids biosynthesis. By the searches, we found that no matter which one of AtTT8, AtEGL3, and AtGL3 was used for blasting, the matching proteins with higher similarity were always the products of FvH4_2g23700, FvH4_7g14230, and FvH4_5g02520 (Supplementary Table 5). A phylogenetic analysis of these three strawberry bHLH proteins and those from other 16 plants was performed, and we found that FvH4_2g23700, FvH4_7g14230, and FvH4_5g02520 clustered with FabHLH3, FabHLH33 and FaMYC1 of Fragaria × ananassa, respectively (Supplementary Figure 2). Consequently, the bHLH proteins from woodland strawberry were named as FvbHLH3, FvbHLH33, and FvMYC1, and considered potential components of MBW complexes for flavonoids biosynthesis.
The Expression Pattern of R2R3-MYB and bHLH Genes Correlates With Anthocyanin and Proanthocyanidin Accumulation in Strawberry Fruits
We studied the transcript levels of MYBs and bHLHs, and their effects on the accumulation of flavonoids in strawberry fruits at five stages of fruit development, including green stage, white stage, pre-turning stage, turning stage and ripe stage. qRT-PCR results showed that the transcript level of a bHLH gene (FvMYC1) and nine MYB genes (FvMYB3, FvMYB9, FvMYB11, FvMYB21, FvMYB22, FvMYB45, FvMYB64, FvMYB77, and FvMYB105) was higher at green stage, but significantly decreased at the other four stages (Figure 2 and Supplementary Figure 3), matching proanthocyanidins biosynthesis pattern, which accumulated mainly at the early stages, especially at the green stage (Figure 3A). Specifically, the expression of FvMYB11, FvMYB21, FvMYB77, and FvMYB105 were not detected during turning stage and ripe stage. By contrast, the expression of FvMYB10 was extremely low in green and white stages, but significantly increased during the late development stages, especially the turning stage, resembling anthocyanin biosynthesis pattern, which accumulated in a large amount during turning and maturation stages (Figure 3B). The expression of FvMYB41 and FvbHLH3 gradually increased accompanying the development of fruit and peaked at ripe stage (Figure 2 and Supplementary Figure 3), also aligned with anthocyanins accumulation (Figure 3B). The expression of FvMYB75 and FvbHLH33, however, was detected at all five stages, with higher expression level at early stages from green to pre-turning than turning and ripe stages (Figure 2 and Supplementary Figure 3). Taken together, the bHLH gene FvMYC1, and the nine MYB genes including FvMYB3, FvMYB9, FvMYB11, FvMYB21, FvMYB22, FvMYB45, FvMYB64, FvMYB77, and FvMYB105, might be involved in proanthocyanidins biosynthesis at early stages of fruit development. FvMYB10, FvMYB41, and FvbHLH3 might be associated with the accumulation of anthocyanins at later stages. The proposed functions of woodland strawberry MYB and bHLH genes are consistent with the phylogenetical analysis (Supplementary Figure 1).
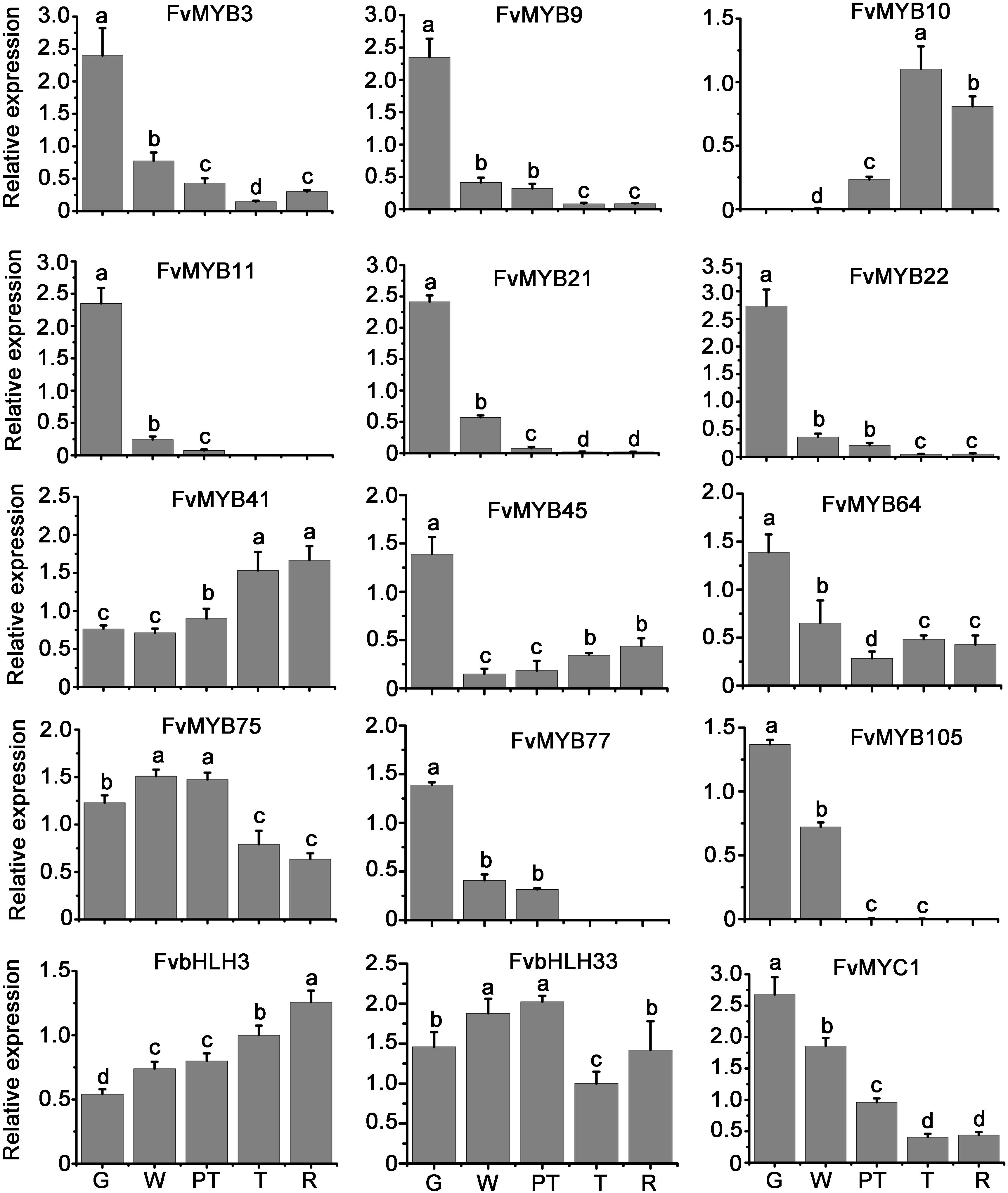
Figure 2. Relative expression levels of MYB and bHLH genes in fruits of different development stages. Values were normalized to the expression level of an internal control, Fv26S. Data are presented as the mean of biological replicates ± SD (n = 3). G, green stage; W, white stage; PT, pre-turning stage; T, turning stage; R, ripe stage. The letters a–d indicate statistically significant differences, as determined by Tukey’s LSD test (P ≤ 0.05).
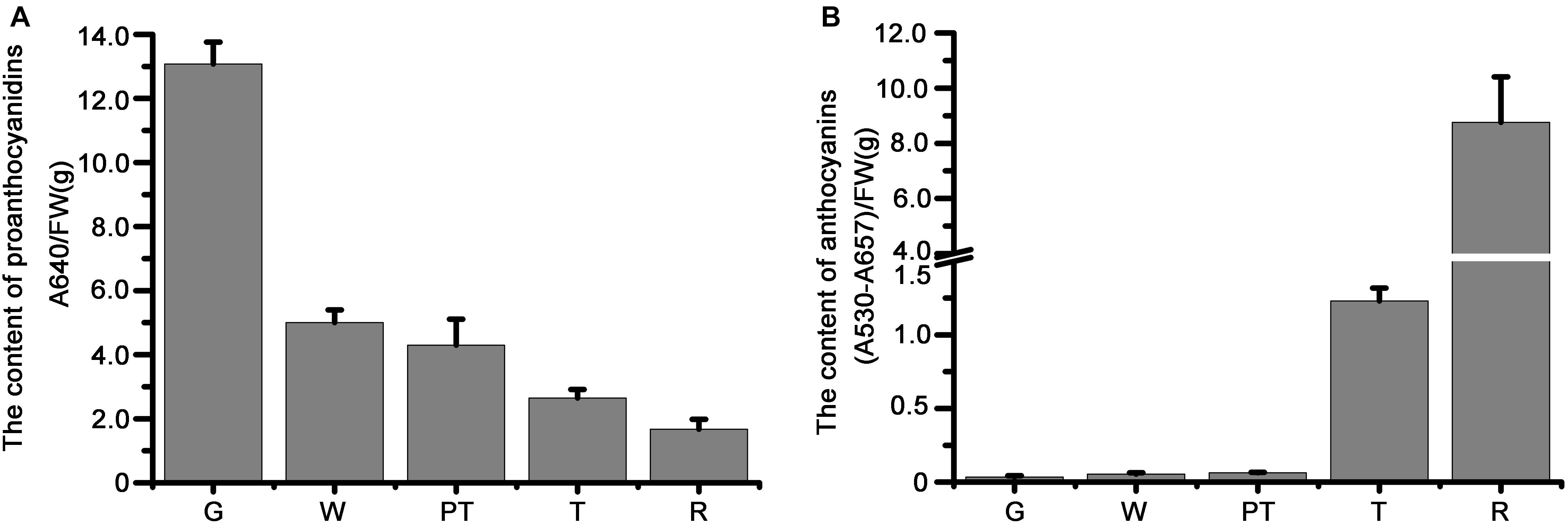
Figure 3. The measurement of anthocyanins and proanthocyanidins in fruits of different development stages. (A) Proanthocyanidins; (B) anthocyanins. G, green stage; W, white stage; PT, pre-turning stage; T, turning stage; R, ripe stage.
Interaction Between the Putative Components of Woodland Strawberry MBW Complexes in vitro and in vivo
To study whether these potential MYB and bHLH proteins can form MBW complexes, we first investigated the interaction among them in vitro using LexA yeast two-hybrid system. When FvbHLH3 or FvMYC1 were used as a bait (fused with DNA-binding domain, BD), they both could interact with every MYB protein tested (fused with Activation domain, AD) except for FvMYB45 or FvMYB77 (Figure 4). FvbHLH33, however, displayed strong self-activation when fused to LexA BD as a bait. This is also true when only the N-terminal fragment of FvbHLH33 was used (Supplementary Figure 4). Considering that 3-AT can be used to inhibit self-activation in GAL4 yeast two-hybrid system, the interaction between FvbHLH33 and MYB proteins were studied in GAL4 system instead. 20 mM 3-AT was introduced to inhibit self-activation using FvbHLH33 as a bait, and we were able to detect interactions between FvbHLH33 and 10 individual MYB proteins, including FvMYB3, FvMYB9, FvMYB10, FvMYB11, FvMYB21, FvMYB22, FvMYB41, FvMYB75, FvMYB77, and FvMYB105, but not FvMYB45 or FvMYB64 (Supplementary Figure 5).
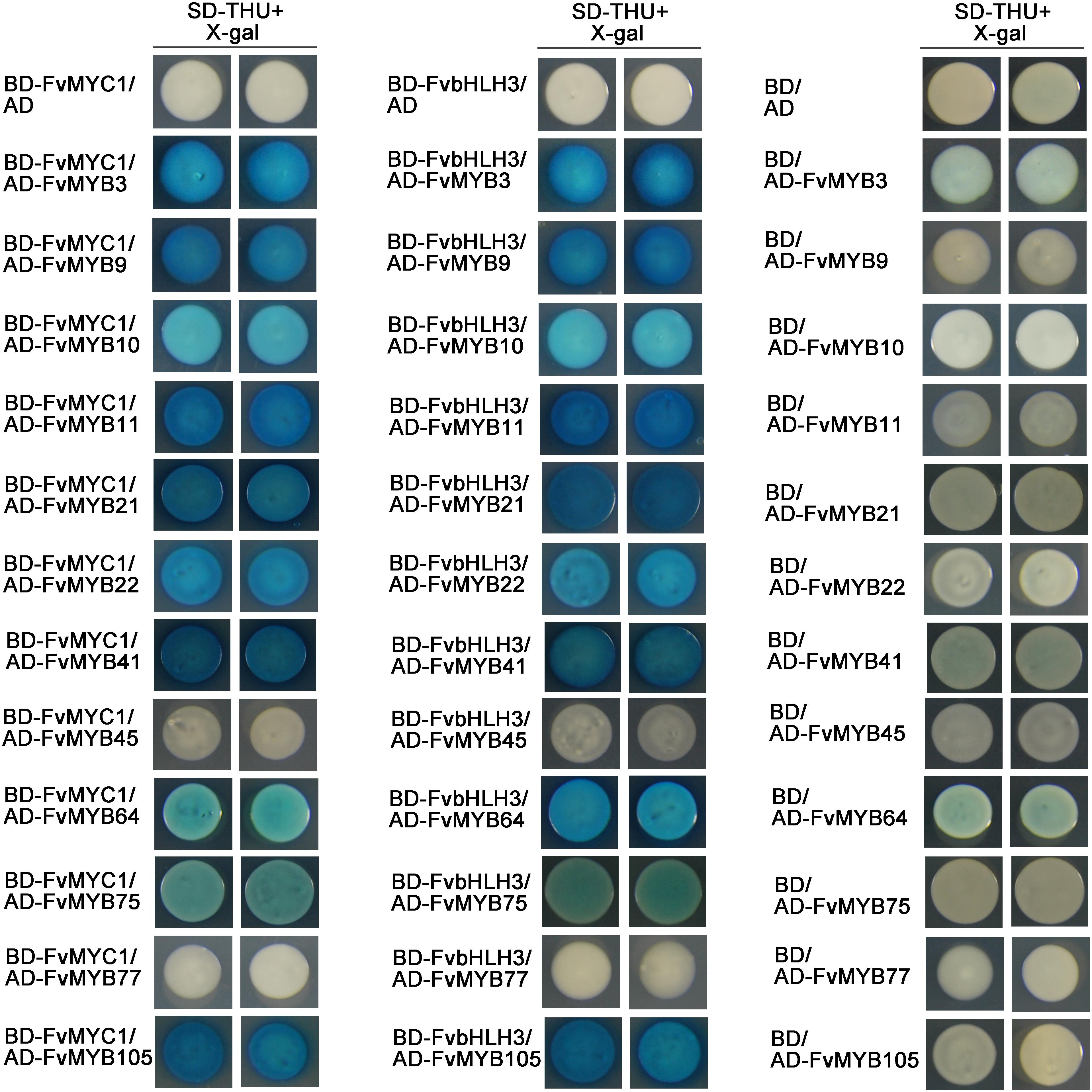
Figure 4. The interaction of MYB and bHLH proteins in yeast cells. LexA yeast two-hybrid assays showing interactions between FvMYB proteins and FvbHLH proteins. Yeast cells were grown on selective medium (SD-Trp, -His, -Ura; SD-THU) with 80 mg/L X-gal. Blue precipitates represent cumulative β-galactosidase activity resulting from the activation of the LacZ reporter gene by interacting proteins. Two representative colonies were shown for each interaction.
We also performed BiFC experiments to furtherly investigate the interactions between bHLH and MYB proteins in vivo. As shown in Figure 5, when MYBs or FvbHLH3 were expressed individually, no over-lapping fluorescent signal of YFP was seen. When FvbHLH3 and any of the 10 MYB proteins, including FvMYB3, FvMYB9, FvMYB10, FvMYB11, FvMYB21, FvMYB22, FvMYB41, FvMYB64, FvMYB75, and FvMYB105, were co-expressed in tobacco cells, strong fluorescent signal of YFP was observed. However, when FvbHLH3 and FvMYB45 or FvMYB77 were co-expressed, no YFP signal was detected. Similarly, FvMYC1 or FvbHLH33 was observed to interact with the same 10 MYB proteins, but not FvMYB45 or FvMYB77 (Supplementary Figure 6). The interaction between FvMYB64 and FvbHLH33 was not detected in yeast two-hybrid, but the interaction was detected in BiFC assay, likely due to the difference between the in vitro and in vivo experimental systems. Taken together, the results in vitro and in vivo suggested that the 10 MYB proteins, except for FvMYB45 and FvMYB77, could possibly form functional MBW complexes with FvbHLH3, FvbHLH33, or FvMYC1.
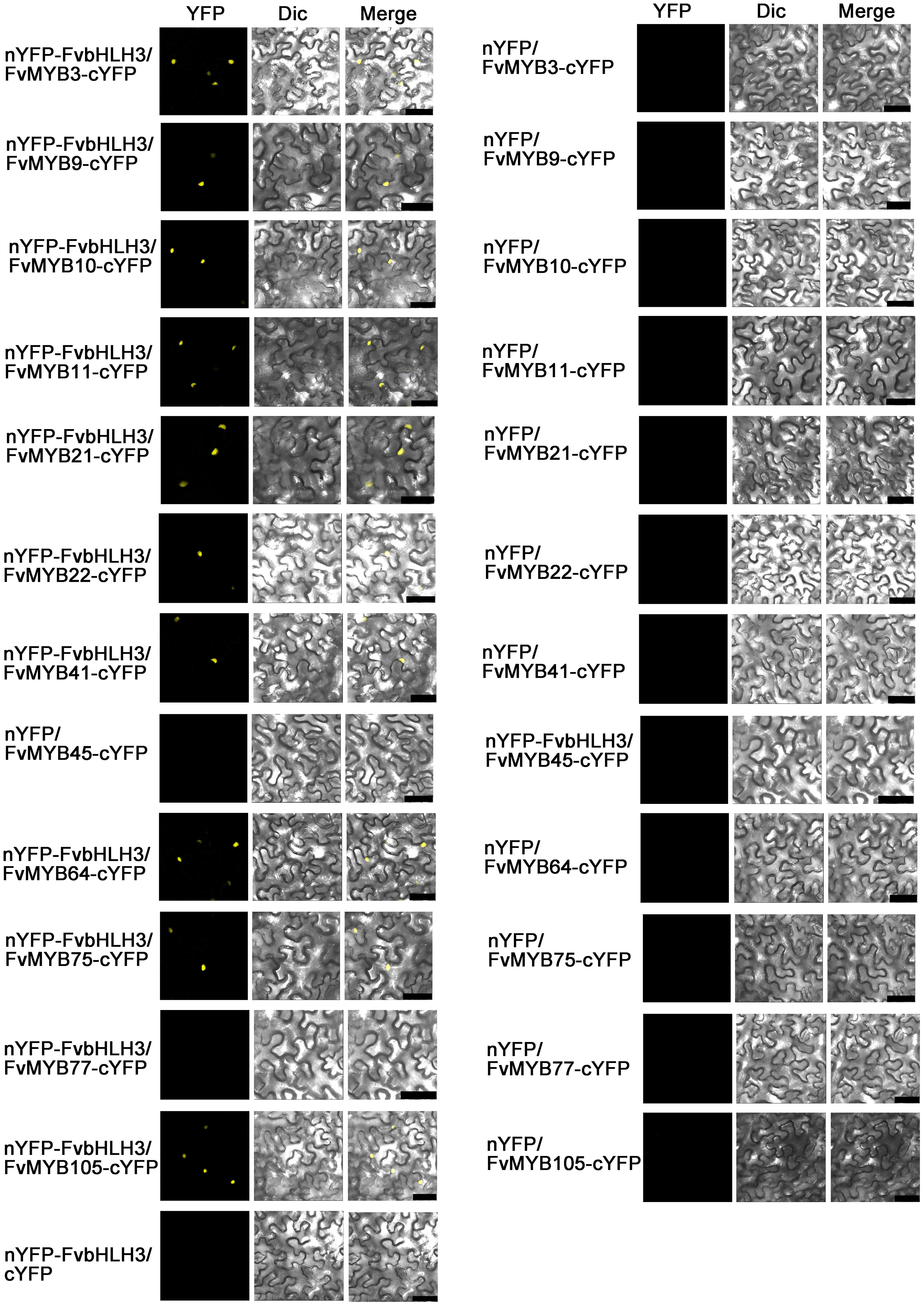
Figure 5. BiFC assay showing interactions between FvMYB proteins and FvbHLH proteins in tobacco cells. Combinations of constructs encoding the indicated proteins were co-transformed into tobacco leaf epidermal cells. Overlays of fluorescence and bright field were shown. Dic, differential interference contrast. Bars, 50 μm.
Most MYB Proteins Bind to the Promoters of Key Enzyme Genes in Flavonoids Biosynthesis
In a MBW complex, MYB protein is the decisive factor for the regulation of specific flavonoids synthesis genes. To confirm whether the identified MYB proteins have a potential role in regulating genes involved in flavonoids biosynthesis, we first analyzed whether the promoters of CHS2 and DFR2 contained cis-elements bound by MBW complexes. As shown in Supplementary Figure 7, the promoter regions of CHS2 and DFR2 contain binding elements of MYB proteins. Then, we tested in yeast one-hybrid system to see whether these MYB proteins are able to bind to the promoters of CHS2 and DFR2 genes, which encode key enzymes related to the flavonoids synthesis pathways. As shown in Figure 6, all tested MYB proteins, except for FvMYB75, displayed binding activity to the promoter of CHS2 gene. However, only FvMYB11 and FvMYB21 bound to the DFR2 promoter.
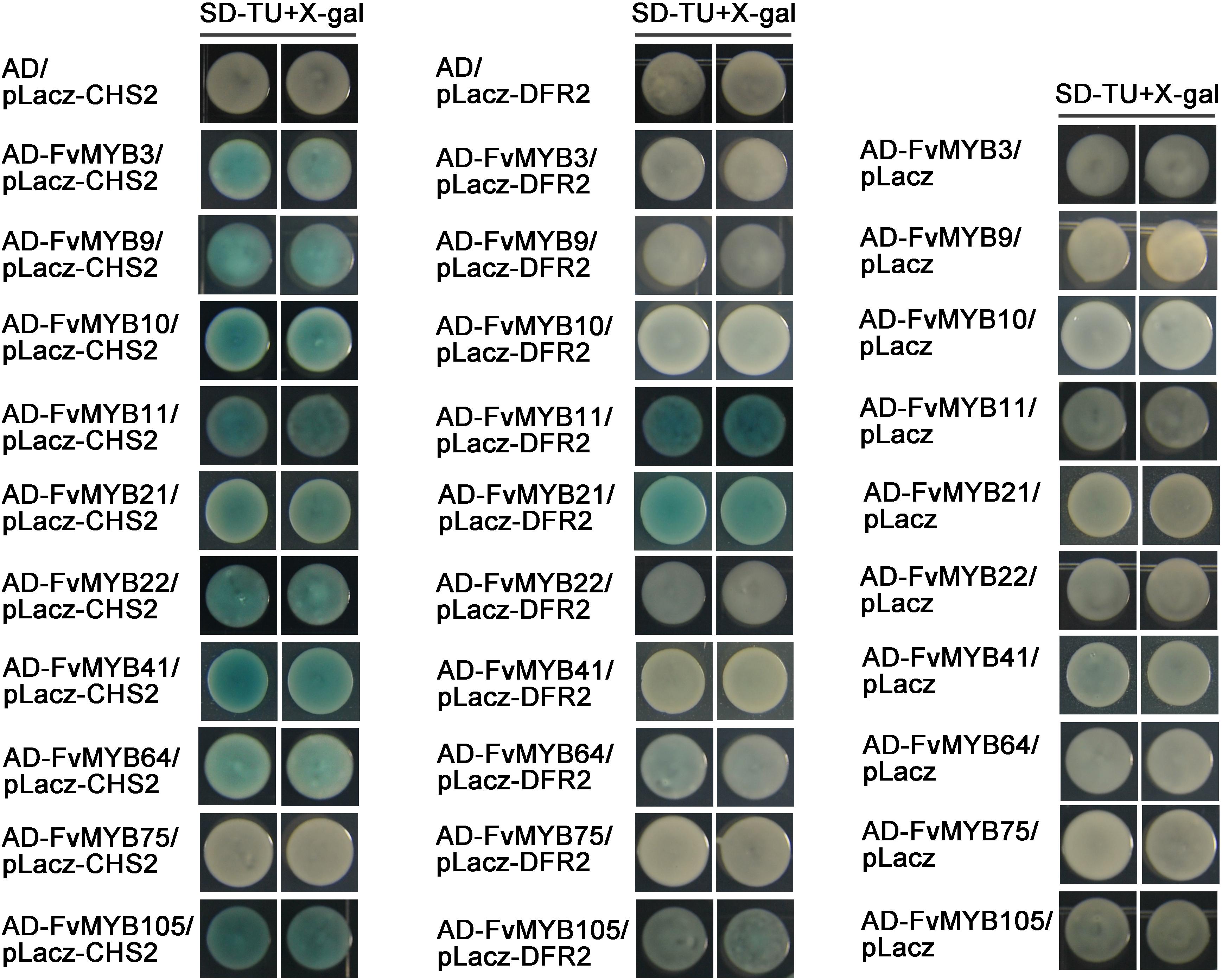
Figure 6. Yeast one-hybrid assays showing the binding of MYB proteins to the promoters of CHS2 and DFR2. Yeast cells were grown on selective medium (SD-Trp, -Ura; SD-TU) with 80 mg/L X-gal. Blue precipitates represent cumulative β-galactosidase activity resulting from the activation of the LacZ reporter gene by binding. Two representative colonies were shown for each binding.
The Heterodimers Formed by MYBs and bHLHs Activate the Expression From DFR2 Promoter
We observed that most of the MYB proteins (except for FvMYB11 and FvMYB21) in strawberry could not bind to the promotor of DFR2 in yeast one-hybrid assay (Figure 6), nor the three bHLH proteins (FvbHLH3, FvbHLH33, or FvMYC1) (Supplementary Figure 8). To explore whether bHLH proteins are in need for those MYBs to bind to the promotor of DFR2, we test the DNA-binding activity of heterodimers formed between five FvMYB proteins (FvMYB10, FvMYB22, FvMYB64, FvMYB75, and FvMYB105) and three FvbHLH proteins (FvbHLH3, FvbHLH33, or FvMYC1) in yeast one-hybrid assay. As shown in Figure 7, transformation of yeast cells with either one of the five MYB proteins fused to AD did not show positive DFR2 promotor binding activity. However, when either one of the three bHLH proteins, FvbHLH3, FvbHLH33 or FvMYC1, was co-expressed with AD-FvMYB22, AD-FvMYB64, or AD-FvMYB105, the report gene of LacZ was activated and the yeast cells displayed blue on media containing X-gal, demonstrating the binding of MYB proteins to the promotor of DFR2 in the presence of bHLH proteins. For FvMYB75 and FvMYB10, the reporter gene LacZ can only be activated in the presence of FvMYC1, but not FvbHLH3 and FvbHLH33 (Supplementary Figure 9), suggesting a requirement of specific bHLH protein for the DNA-binding activity of FvMYB75 or FvMYB10.
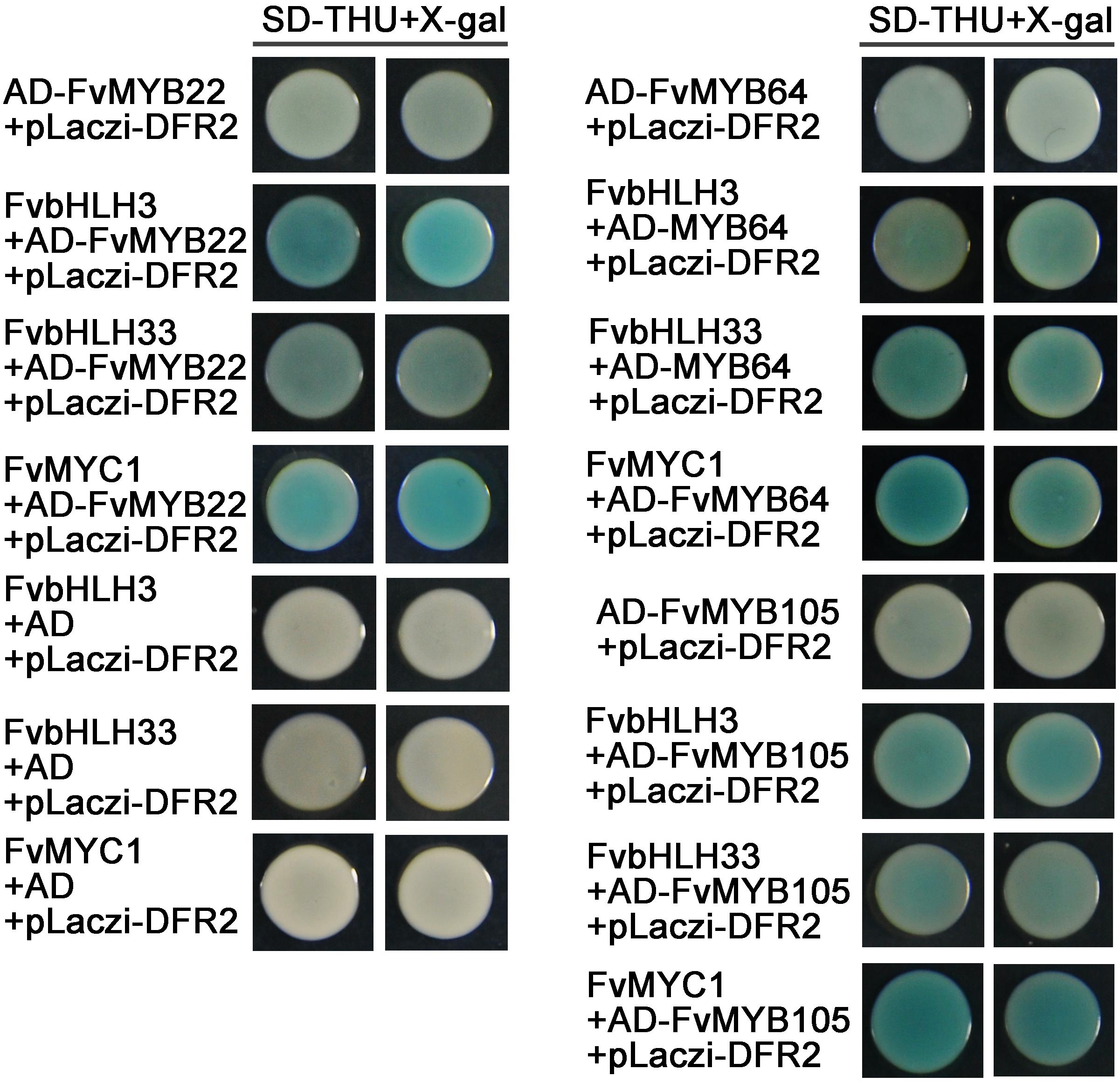
Figure 7. The effect of interactions between MYB and bHLH proteins on the DFR2 promoter fused with LacZ reporter gene. Yeast cells were grown on selective medium (SD-Trp, -His, -Ura; SD-THU) with 80 mg/L X-gal. Blue precipitates represent cumulative β-galactosidase activity resulting from the activation of the LacZ reporter gene by binding. Two representative colonies were shown for each binding.
To further confirm the requirement of FvbHLH proteins for the DNA-Binding activity of FvMYB proteins in vivo, we transiently expressed FvbHLH33 and three FvMYB proteins (FvMYB22, FvMYB64, and FvMYB105) separately, or as a combination of FvbHLH33 with one of the three FvMYB proteins in N. benthamiana leaves, along with a reporter luciferase gene under the control of DFR2 promoter. As shown in Figures 8A,B, when FvMYB22, FvMYB64, or FvMYB105 was expressed alone, the reporter gene was not significantly induced, whereas these MYB proteins induced the reporter gene in the presence of FvbHLH33, leading to a strong luminescence signal when the substrate of luciferin was added. These results, together with results from yeast one-hybrid suggested that the heterodimers between FvMYBs and FvbHLHs were necessary for activating some flavonoids synthesis genes.
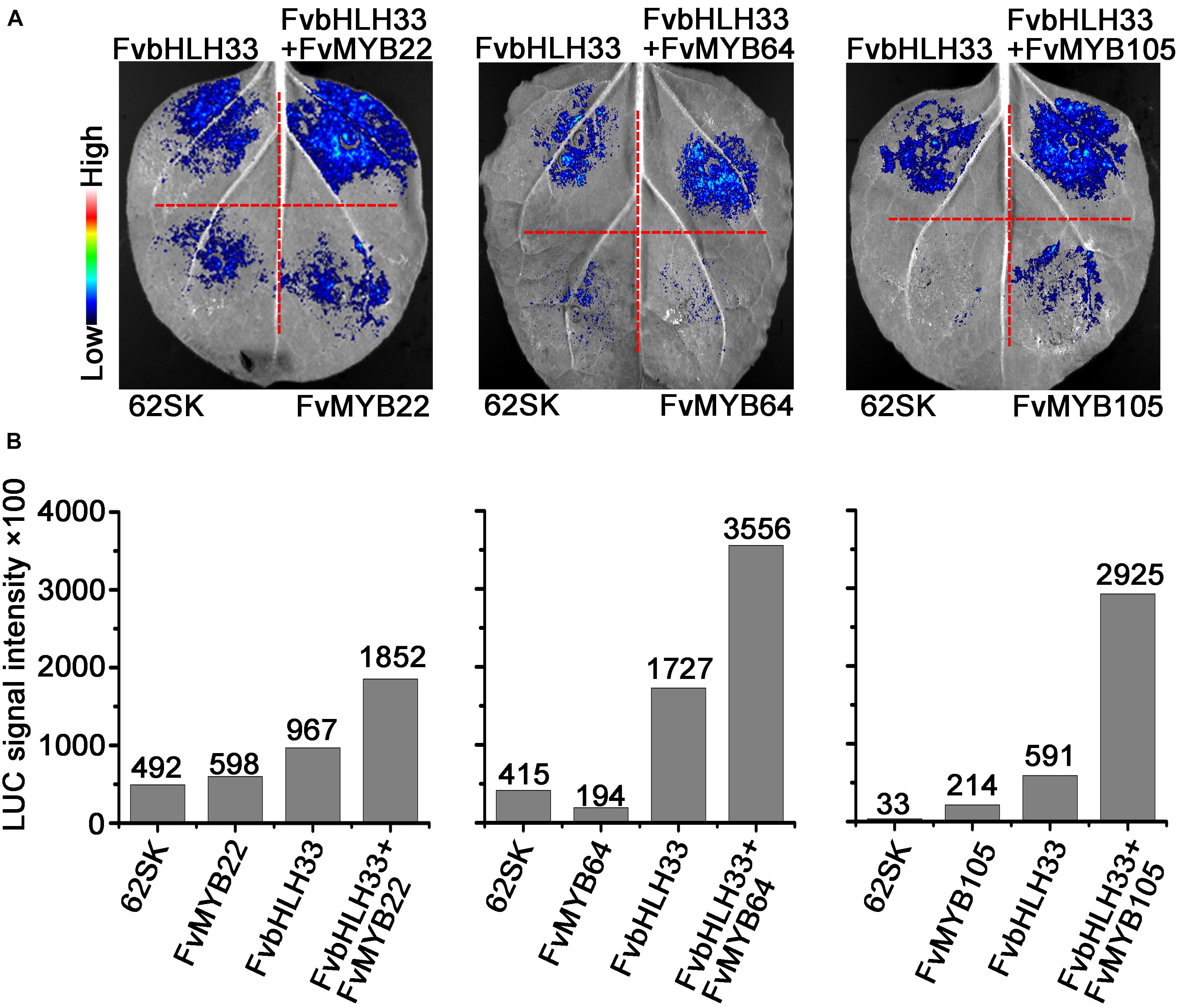
Figure 8. The effect of interactions between MYB and bHLH proteins on the DFR2 promoter fused with luciferase reporter gene. The constructs encoding the indicated combinations of proteins were co-transformed into tobacco leaf epidermal cells, and the luminescence images were captured using a CCD imaging system (A), the intensity of luminescence was indicated in (B).
Co-expression of FvMYB and FvbHLH Proteins Promotes the Accumulation of Proanthocyanidins
To confirm the biofunction of strawberry MBW complexes in flavonoids synthesis, we co-expressed FvMYB and FvbHLH proteins in the fruits and measured the flavonoids accumulation. FvMYB22, FvMYB64, and FvMYB105 are homologs of MYB proteins in other plants that regulate proanthocyanidin synthesis (Supplementary Figure 1) and mainly expressed at green stage (Figure 2 and Supplementary Figure 3). FvbHLH33 has been shown to interact with all three FvMYB proteins in yeast and tobacco cells. We transiently expressed FvbHLH33 and the three FvMYB proteins individually, or a combination of FvbHLH33 and one of the three FvMYB proteins in green stage strawberry fruit. The accumulation of proanthocyanidins was measured following the expression. As shown in Figures 9A,B and Supplementary Figure 10, the accumulation of procyanidins did not significantly increase following the expression of either FvbHLH33 or any of the three FvMYB proteins alone. However, the expression levels of flavonoid biosynthesis related genes CHS2 and DFR2 was significantly enhanced and the accumulation of proanthocyanidins was significantly increased in strawberry fruits when FvbHLH33 and one of the three FvMYB proteins were co-expressed (Figures 9A,B and Supplementary Figure 10). These results confirmed the function of the identified MBW components in strawberry flavonoids biosynthesis.
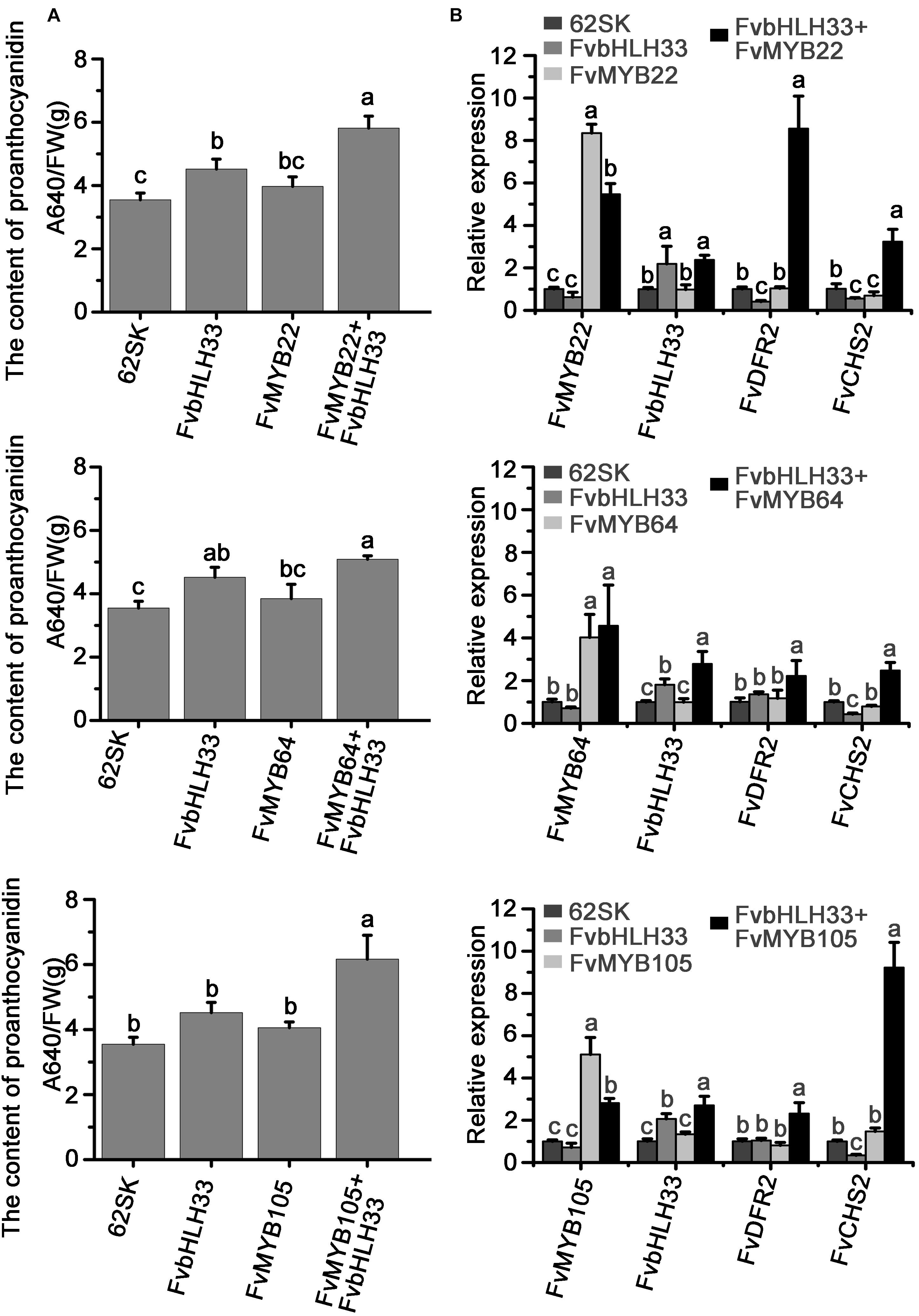
Figure 9. The complex of FvMYB and FvbHLH33 can promote the accumulation of proanthocyanidin in strawberry fruits. The Agrobacterium containing the indicated combinations of constructs were injected into strawberry fruits and the content of proanthocyanidins was detected at 7 days after injection. (A) Measurement of proanthocyanidin related to the transient expression of FvMYB and FvbHLH proteins. (B) Relative expression levels of FvMYBs, FvbHLH33, FvCHS2, and FvDFR2 genes from transient expression. Fv26S served as an internal control. Data are presented as the mean ± SD (n = 3). The letters a–c indicate statistically significant differences, as determined by Tukey’s LSD test (P ≤ 0.05).
Discussion
In this study, we identified 112 R2R3-MYB genes in woodland strawberry by using HMM profile of MYB DNA-binding domain to blast strawberry genome database and further verified the presence of the MYB DNA-binding domain in Pfam, SMART and HHMER (Supplementary Table 4). Recently, Liu et al. (2019) have identified R2R3-MYB genes in six Rosaceae species including strawberry (F. vesca), but they identified 105 R2R3-MYB genes which was seven genes fewer than what we found. The difference was likely because they used the 125 R2R3-MYB from Arabidopsis to perform repetitive BLASTP search against the strawberry genome version 1.1.a2, in which there were still many scaffolds (Darwish et al., 2015). In our study, we used HMM profile of MYB DNA-binding domain to search against the strawberry genome version 4.0.a2, which contains 34,007 genes, 511 more genes than the 33,496 genes in version 1.1.a2 (Li et al., 2019). We speculate that some R2R3-MYB genes might have been omitted or wrongly identified due to the annotation errors in the version 1.1.a2. For example, mrna32181, mrna16655, mrna01311 and mrna28755 were identified as R2R3-MYB genes in version 1.1.a2 by Li et al. (2019). When using these genes to blast against version 4.0.a2, each of these four genes was annotated as two or three different genes in version 4.0.a2 (Supplementary Figure 11), indicating that there were wrong annotations in version 1.1.2a. Given that genomic information in version 4.0 is at chromosomal level, 112 R2R3-MYB identified in our study have higher confidence levels.
It was proposed that in a MBW complex, MYB protein is the decisive factor for the regulation of specific flavonoids synthesis (Baudry et al., 2004). In our study, through phylogenetic tree analysis of R2R3-MYB genes from different plants including Arabidopsis, grape, pear, strawberry, etc., we obtained 12 potential MYB genes that regulate flavonoids synthesis in strawberry. Among these 12 MYB genes, FvMYB10 and FvMYB75 clustered with MdMYB1 and MdMYB10 (Supplementary Figure 1), which were known to promote anthocyanin accumulation in apple (Espley et al., 2007; Gonzalez et al., 2008). It has been reported that over-expression of FvMYB10 or FaMYB10 (homolog of FvMYB10 in cultivated strawberry Fragaria × ananassa) resulted in elevated concentrations of anthocyanin in root, leaves, petioles, stigmas, petals and fruits (Lin-Wang et al., 2014; Medina-Puche et al., 2014; Wang et al., 2020). Additionally, Hawkins et al. (2016) identified an SNP (a specific SNP mutation of G to C, leading to W12S variant) in FvMYB10 resulting in abolished accumulation of anthocyanins in the fruits of the woodland strawberry Yellow Wonder (YW). Furthermore, in the yellow and red-fruited varieties tested, the C (W12S) in FvMYB10 is 100% associated with the yellow color of the fruit, while the G is 100% associated with the red color (Hawkins et al., 2016; Zhang et al., 2017). Interestingly in YW, there is no accumulation of anthocyanins in fruits, but the accumulation is normal in petioles (Xu et al., 2014). In addition, two Fragaria pentaphylla subvarieties displayed red and white skin color, the relatively low expression level of FpMYB10 might have contributed to the white fruit variety. The achenes of both varieties, however, are red (Bai et al., 2019). These results suggested that other factors besides FvMYB10 or FpMYB10 might be involved in the regulation of anthocyanin accumulation. In our study, the expression of FvMYB41 displayed expression pattern similar to FvMYB10 during the five development stages (Figure 2 and Supplementary Figure 3), resembling anthocyanin biosynthesis pattern (Figure 3B), suggesting that FvMYB41 might have a role in promoting anthocyanin accumulation. Through the phylogenetic tree analysis, we found that FvMYB41 was most genetically related to VvMYB5b from grape and FhMYB5 from Freesia, both of which have been proven to promote the accumulation of anthocyanin and proanthocyanidins (Deluc et al., 2008; Li et al., 2018). By comparing the sequences of VvMYB5b, FhMYB5, and FvMYB41, we found that the similarity of all three was greater than 45% (Supplementary Table 6). The most conserved sequences were within the DNA-binding R2R3 domain as well as the C1 and C3 motifs in the C-terminal regions of the three genes (Supplementary Figure 12). Thus, it was speculated that FvMYB41 likely serves as a homologous protein of VvMYB5b and FhMYB5 in strawberry that promotes flavonoids biosynthesis. However, this notion needs to be tested in future study.
It has been known that MBW complexes composed of MYB-bHLH-WD40 play key roles in regulating flavonoids biosynthesis in plants. In this study, we confirmed the interactions between potential components of MBW in woodland strawberry. We detected the interaction between twelve MYB proteins (FvMYB3, FvMYB9, FvMYB10, FvMYB11, FvMYB21, FvMYB22, FvMYB41, FvMYB45, FvMYB64, FvMYB75, FvMYB77, and FvMYB105) and three bHLH proteins (FvbHLH3, FvbHLH33, and FvMYC1). Using the yeast two hybrid and BiFC assays, we found that except for FvMYB45 and FvMYB77, all the other MYBs can interact with either one of FvbHLH3, FvbHLH33, or FvMYC1 (Figures 4, 5 and Supplementary Figures 5, 6). In addition, we found that FvTTG1, a WD40 protein, interacted with all the three bHLH proteins (Supplementary Figure 13). All these results indicated that the strawberry MBW component proteins identified in this study could form MBW complexes. Interestingly, in octoploid strawberry, FabHLH3, FabHLH33, and FaMYC1 can interact with FaTTG1, but only FabHLH3 can interact with FaMYB9, FaMYB11 (Schaart et al., 2013). Whereas, we found that both FvMYB9 and FvMYB11 interacted with FvbHLH33 and FvMYC1 in woodland strawberry (Figure 4 and Supplementary Figure 5). For these paradoxical results between woodland strawberry and octoploid strawberry, it is likely because that Schaart et al. (2013) used the C terminal of FvMYC1 and FvbHLH33 but not the full length of CDS for yeast two-hybrid assays (Supplementary Figures 14, 15). Only the full-length protein of FvbHLH33, but not the C-terminus fragment, interacted with MYB proteins in our assay (Supplementary Figures 5, 16). Even though Schaart et al. (2013) had demonstrated the direct interactions between FabHLH3 and the two FaMYB proteins (FaMYB9 and FaMYB11), they only did so in yeast cells, not in plant cells (Schaart et al., 2013). In our study, we provided evidence to support the interactions between FvMYB proteins and FvbHLH proteins in both yeast (Figure 4 and Supplementary Figure 5) and plant cells (Figure 5 and Supplementary Figure 6).
To further test whether FvMYB proteins or FvbHLH proteins are involved in the regulation of flavonoids biosynthesis-related genes, we examined the DNA-binding activity of FvMYB proteins, as well as the requirement of FvbHLH proteins, using reporter genes (LacZ and Luciferase) under the control of the promoters of CHS2 and DFR2, two key enzyme genes related to the flavonoids biosynthesis pathways. Most of the FvMYB proteins except for FvMYB75 displayed DNA-binding activity to CHS2 promoter. However, most of the FvMYB proteins and three bHLH proteins were not able to bind to DFR2 promoter except for FvMYB11 and FvMYB21 (Figure 6 and Supplementary Figure 8). It has been reported that in Arabidopsis, AtTT2 (AtMYB123) can’t bind by itself to the promoter of BAN, a gene involved in proanthocyanidins production. AtTT8 (AtbHLH042) must be present for AtTT2 to bind to the BAN promoter in yeast (Baudry et al., 2004). Considering that MYB proteins often form complexes with bHLH proteins to regulate flavonoids biosynthesis, we further examined whether co-expression of FvbHLH proteins could promote the binding of FvMYB proteins to the promoter of DFR2. Our results showed that co-expression of either one of the three FvbHLH proteins (FvbHLH3, FvbHLH33, and FvMYC1) with FvMYB22, FvMYB64, or FvMYB105 in yeast cells significantly increased the expression of the reporter gene LacZ from DFR2 promotor, suggested that FvbHLHs can promote the binding activity of FvMYBs to the promoter of DFR2 (Figure 7). The requirement of FvbHLH protein for the gene induction function of FvMYB proteins was also demonstrated in tobacco cells. FvMYB22, FvMYB64, or FvMYB105 induced the expression of luciferase from DFR2 promoter when FvbHLH33 was co-expressed (Figure 8). This was further confirmed by the accumulation of flavonoids in strawberry fruits. When FvbHLH33 was co-expressed with either one of FvMYB22, FvMYB64, or FvMYB105 in green stage strawberry fruits, the accumulation of proanthocyanidins was significantly increased (Figure 9A). But for FvMYB75 or FvMYB10, only FvMYC1 could help increase their DNA-binding activity, leading to the induction of LacZ expression (Supplementary Figure 9). These results suggested that some FvMYB proteins only form complexes with specific FvbHLH proteins. It has been reported that the maize R2R3-MYB protein C1 co-worked with the bHLH protein R to activate the expression of anthocyanin biosynthesis genes (Grotewold et al., 2000). Later studies showed that the dimerization domain of R behaves as a switch that permits distinct configurations of the regulatory complex to be tethered to different promoters of target genes (Feller et al., 2006; Kong et al., 2012). These studies suggested that bHLH protein may determines the target gene of MBW complexes. The biofunctions of the three FvbHLH proteins in woodland strawberry, including FvbHLH3, FvbHLH33, and FvMYC1, remain to be further investigated.
Since FvMYB22, FvMYB64, and FvMYB105 clustered with VvMYBPA1, FaMYB9, and FaMYB11, which were reported to promote proanthocyanidins biosynthesis. We tested whether these three MYBs can bind to the promoter of FvLAR, a key enzyme gene regulating the proanthocyanidins synthesis. Our results showed that FvMYB22, FvMYB64, and FvMYB105 bound to FvLAR promoters only in the presence of FvMYC1 (Supplementary Figure 17). Considering the interaction with other FvMYB proteins in activating CHS2 and DFR2 promoters, FvMYC1 might be a more universal bHLH partner for FvMYB proteins and play more extensive roles in flavonoids biosynthesis than the other two bHLH proteins, FvbHLH3 and FvbHLH33. In fact, even when the transcripts of FvbHLH33 was significantly reduced in FvbHLH33 RNAi lines, the pigmentation was not significantly altered in those lines when compared with WT (Lin-Wang et al., 2014). This also indicated that other bHLH proteins, especially FvMYC1, might compensate for the lack of FvbHLH33 in this case. Recently, it was found that FvbHLH9 can promote anthocyanin accumulation in strawberry fruits, but FvMYB10 could not promote the expression of downstream target genes when only bHLH9 was present (Li et al., 2020), likely because the lack of WD40 protein. The results of existing studies strongly suggest that there are other regulatory MBW proteins in strawberry that control the synthesis and accumulation of anthocyanins.
Conclusion
In this study, we identified novel MBW components in woodland strawberry utilizing phylogenetic analysis, and confirmed the biofunctions of several members in flavonoids accumulation using molecular and biochemical methods. This work provided not only the basis for study of flavonoids synthesis in strawberry, but also potential economic benefit in strawberry cultivation. As transcriptional regulators, further studies of MBW complexes and their biological role in other important physiological processes and regulatory mechanism will contribute to better understanding of strawberry biology.
Data Availability Statement
The datasets presented in this study can be found in online repositories. The names of the repository/repositories and accession number(s) can be found in the article/Supplementary Material.
Author Contributions
PX and HL designed the experiments. PX, MC, and YL carried out the experiments. PX, MC, and KX collected the data. PX, LW, and HL analyzed the data. PX, LW, CM, YL, and HL wrote the manuscript with input from all other authors. All authors contributed to the article and approved the submitted version.
Funding
This work was supported by The National Natural Science Foundation of China grants to PX (32002003) and HL (32172536 and 31570282), the China Postdoctoral Science Foundation (2019M661488 and 2020T130415 to PX), the National Natural Science Foundation of Shanghai (20ZR1426000 to HL), and Shanghai Pujiang Program (Grant: 19PJ1406700).
Conflict of Interest
The authors declare that the research was conducted in the absence of any commercial or financial relationships that could be construed as a potential conflict of interest.
Publisher’s Note
All claims expressed in this article are solely those of the authors and do not necessarily represent those of their affiliated organizations, or those of the publisher, the editors and the reviewers. Any product that may be evaluated in this article, or claim that may be made by its manufacturer, is not guaranteed or endorsed by the publisher.
Supplementary Material
The Supplementary Material for this article can be found online at: https://www.frontiersin.org/articles/10.3389/fpls.2021.774943/full#supplementary-material
Footnotes
- ^ https://www.rosaceae.org/
- ^ https://www.ebi.ac.uk/Tools/pfa/pfamscan/
- ^ http://smart.embl-heidelberg.de/
- ^ https://www.ebi.ac.uk/Tools/hmmer/
- ^ https://www.expasy.org/
- ^ http://www.arabidopsis.org/
- ^ http://bioinformatics.psb.ugent.be/webtools/plantcare/html/
References
Azuma, A., Kobayashi, S., Mitani, N., Shiraishi, M., Yamada, M., Ueno, T., et al. (2008). Genomic and genetic analysis of Myb-related genes that regulate anthocyanin biosynthesis in grape berry skin. Theor. Appl. Genet. 117, 1009–1019. doi: 10.1007/s00122-008-0840-1
Bai, L., Chen, Q., Jiang, L., Lin, Y., Ye, Y., Liu, P., et al. (2019). Comparative transcriptome analysis uncovers the regulatory functions of long noncoding RNAs in fruit development and color changes of Fragaria pentaphylla. Hortic. Res-England 6:42. doi: 10.1038/s41438-019-0128-4
Battino, M., Beekwilder, J., Denoyes-Rothan, B., Laimer, M., McDougall, G. J., and Mezzetti, B. (2009). Bioactive compounds in berries relevant to human health. Nutr. Rev. 67(Suppl. 1), S145–S150.
Baudry, A., Heim, M. A., Dubreucq, B., Caboche, M., Weisshaar, B., and Lepiniec, L. (2004). TT2, TT8, and TTG1 synergistically specify the expression of BANYULS and proanthocyanidin biosynthesis in Arabidopsis thaliana. Plant J. 39, 366–380. doi: 10.1111/j.1365-313X.2004.02138.x
Bogs, J., Jaffe, F. W., Takos, A. M., Walker, A. R., and Robinson, S. P. (2007). The grapevine transcription factor VvMYBPA1 regulates proanthocyanidin synthesis during fruit development. Plant Physiol. 143, 1347–1361. doi: 10.1104/pp.106.093203
Castillejo, C., Waurich, V., Wagner, H., Ramos, R., Oiza, N., Munoz, P., et al. (2020). Allelic variation of MYB10 is the major force controlling natural variation in skin and flesh color in strawberry (Fragaria spp.) fruit. Plant Cell 32, 3723–3749. doi: 10.1105/tpc.20.00474
Darwish, O., Shahan, R., Liu, Z. C., Slovin, J. P., and Alkharouf, N. W. (2015). Re-annotation of the woodland strawberry (Fragaria vesca) genome. BMC Genom. 16:29. doi: 10.1186/s12864-015-1221-1
Deluc, L., Bogs, J., Walker, A. R., Ferrier, T., Decendit, A., Merillon, J. M., et al. (2008). The transcription factor VvMYB5b contributes to the regulation of anthocyanin and proanthocyanidin biosynthesis in developing grape berries. Plant Physiol. 147, 2041–2053. doi: 10.1104/pp.108.118919
deVetten, N., Quattrocchio, F., Mol, J., and Koes, R. (1997). The an11 locus controlling flower pigmentation in petunia encodes a novel WD-repeat protein conserved in yeast, plants, and animals. Genes Dev. 11, 1422–1434. doi: 10.1101/gad.11.11.1422
Dubos, C., Stracke, R., Grotewold, E., Weisshaar, B., Martin, C., and Lepiniec, L. (2010). MYB transcription factors in Arabidopsis. Trends Plant Sci. 15, 573–581.
Emiliani, J., Grotewold, E., Falcone Ferreyra, M. L., and Casati, P. (2013). Flavonols protect Arabidopsis plants against UV-B deleterious effects. Mol. Plant 6, 1376–1379. doi: 10.1093/mp/sst021
Espley, R. V., Hellens, R. P., Putterill, J., Stevenson, D. E., Kutty-Amma, S., and Allan, A. C. (2007). Red colouration in apple fruit is due to the activity of the MYB transcription factor. MdMYB10. Plant J. 49, 414–427.
Feller, A., Hernandez, J. M., and Grotewold, E. (2006). An ACT-like domain participates in the dimerization of several plant basic-helix-loop-helix transcription factors. J. Biol. Chem. 281, 28964–28974. doi: 10.1074/jbc.M603262200
Franco-Zorrilla, J. M., Lopez-Vidriero, I., Carrasco, J. L., Godoy, M., Vera, P., and Solano, R. (2014). DNA-binding specificities of plant transcription factors and their potential to define target genes. Proc. Natl. Acad. Sci. U S A. 111, 2367–2372.
Gonzalez, A., Mendenhall, J., Huo, Y., and Lloyd, A. (2009). TTG1 complex MYBs, MYB5 and TT2, control outer seed coat differentiation. Dev. Biol. 325, 412–421. doi: 10.1016/j.ydbio.2008.10.005
Gonzalez, A., Zhao, M., Leavitt, J. M., and Lloyd, A. M. (2008). Regulation of the anthocyanin biosynthetic pathway by the TTG1/bHLH/Myb transcriptional complex in Arabidopsis seedlings. Plant J. 53, 814–827. doi: 10.1111/j.1365-313X.2007.03373.x
Grotewold, E., Drummond, B. J., Bowen, B., and Peterson, T. (1994). The myb-homologous P gene controls phlobaphene pigmentation in maize floral organs by directly activating a flavonoid biosynthetic gene subset. Cell 76, 543–553. doi: 10.1016/0092-8674(94)90117-1
Grotewold, E., Sainz, M. B., Tagliani, L., Hernandez, J. M., Bowen, B., and Chandler, V. L. (2000). Identification of the residues in the Myb domain of maize C1 that specify the interaction with the bHLH cofactor R. Proc. Natl. Acad. Sci. U S A. 97, 13579–13584. doi: 10.1073/pnas.250379897
Hawkins, C., Caruana, J., Schiksnis, E., and Liu, Z. (2016). Genome-scale DNA variant analysis and functional validation of a SNP underlying yellow fruit color in wild strawberry. Sci. Rep. 6:29017. doi: 10.1038/srep29017
He, J. A., and Giusti, M. M. (2010). Anthocyanins: natural colorants with health-promoting properties. Annu. Rev. Food Sci. T 1, 163–187.
Hichri, I., Heppel, S. C., Pillet, J., Leon, C., Czemmel, S., Delrot, S., et al. (2010). The basic helix-loop-helix transcription factor MYC1 is involved in the regulation of the flavonoid biosynthesis pathway in grapevine. Mol. Plant 3, 509–523. doi: 10.1093/mp/ssp118
Kelemen, Z., Sebastian, A., Xu, W. J., Grain, D., Salsac, F., Avon, A., et al. (2015). Analysis of the DNA-binding activities of the Arabidopsis R2R3-MYB transcription factor family by one-hybrid experiments in yeast. PLoS One 10:e0141044. doi: 10.1371/journal.pone.0141044
Kong, Q., Pattanaik, S., Feller, A., Werkman, J. R., Chai, C. L., Wang, Y. Q., et al. (2012). Regulatory switch enforced by basic helix-loop-helix and ACT-domain mediated dimerizations of the maize transcription factor R. Proc. Natl. Acad. Sci. U S A. 109, E2091–E2097. doi: 10.1073/pnas.1205513109
Lescot, M., Dehais, P., Thijs, G., Marchal, K., Moreau, Y., Van De Peer, Y., et al. (2002). PlantCARE, a database of plant cis-acting regulatory elements and a portal to tools for in silico analysis of promoter sequences. Nucleic Acids Res. 30, 325–327. doi: 10.1093/nar/30.1.325
Li, S. (2014). Transcriptional control of flavonoid biosynthesis: fine-tuning of the MYB-bHLH-WD40 (MBW) complex. Plant Signal. Behav. 9:e27522. doi: 10.4161/psb.27522
Li, Y., Shan, X., Zhou, L., Gao, R., Yang, S., Wang, S., et al. (2018). The R2R3-MYB factor FhMYB5 from freesia hybrida contributes to the regulation of anthocyanin and proanthocyanidin biosynthesis. Front.Plant Sci. 9:1935. doi: 10.3389/fpls.2018.01935
Li, Y., Xu, P., Chen, G., Wu, J., Liu, Z., and Lian, H. (2020). FvbHLH9, functions as a positive regulator of anthocyanin biosynthesis, by forming HY5-bHLH9 transcription complex in strawberry fruits. Plant Cell Physiol. 61, 826–837. doi: 10.1093/pcp/pcaa010
Li, Y. P., Pi, M. T., Gao, Q., Liu, Z. C., and Kang, C. Y. (2019). Updated annotation of the wild strawberry Fragaria vesca V4 genome. Hortic. Res-England 6:61. doi: 10.1038/s41438-019-0142-6
Lin-Wang, K., McGhie, T. K., Wang, M., Liu, Y., Warren, B., Storey, R., et al. (2014). Engineering the anthocyanin regulatory complex of strawberry (Fragaria vesca). Front. Plant Sci. 5:651. doi: 10.3389/fpls.2014.00651
Liu, H., Xiong, J. S., Jiang, Y. T., Wang, L., and Cheng, Z. M. (2019). Evolution of the R2R3-MYB gene family in six Rosaceae species and expression in woodland strawberry. J. Integr. Agr. 18, 2753–2770.
Lu, X.-D., Zhou, C.-M., Xu, P.-B., Luo, Q., Lian, H.-L., and Yang, H.-Q. (2015). Red-light-dependent interaction of phyB with SPA1 promotes COP1–SPA1 dissociation and photomorphogenic development in Arabidopsis. Mol. Plant 8, 467–478. doi: 10.1016/j.molp.2014.11.025
Medina-Puche, L., Cumplido-Laso, G., Amil-Ruiz, F., Hoffmann, T., Ring, L., Rodriguez-Franco, A., et al. (2014). MYB10 plays a major role in the regulation of flavonoid/phenylpropanoid metabolism during ripening of Fragaria x ananassa fruits. J. Exp. Bot. 65, 401–417. doi: 10.1093/jxb/ert377
Mehrtens, F., Kranz, H., Bednarek, P., and Weisshaar, B. (2005). The Arabidopsis transcription factor MYB12 is a flavonol-specific regulator of phenylpropanoid biosynthesis. Plant Physiol. 138, 1083–1096.
Nesi, N., Debeaujon, I., Jond, C., Pelletier, G., Caboche, M., and Lepiniec, L. (2000). The TT8 gene encodes a basic helix-loop-helix domain protein required for expression of DFR and BAN genes in Arabidopsis siliques. Plant Cell 12, 1863–1878. doi: 10.1105/tpc.12.10.1863
Nesi, N., Jond, C., Debeaujon, I., Caboche, M., and Lepiniec, L. (2001). The Arabidopsis TT2 gene encodes an R2R3 MYB domain protein that acts as a key determinant for proanthocyanidin accumulation in developing seed. Plant Cell 13, 2099–2114. doi: 10.1105/tpc.010098
Nijveldt, R. J., van Nood, E., van Hoorn, D. E., Boelens, P. G., van Norren, K., and van Leeuwen, P. A. (2001). Flavonoids: a review of probable mechanisms of action and potential applications. Am. J. Clin. Nutr. 74, 418–425. doi: 10.1093/ajcn/74.4.418
Prouse, M. B., and Campbell, M. M. (2012). The interaction between MYB proteins and their target DNA binding sites. Bba-Gene Regul. Mech. 1819, 67–77. doi: 10.1016/j.bbagrm.2011.10.010
Quattrocchio, F., Wing, J. F., Leppen, H., Mol, J., and Koes, R. E. (1993). Regulatory genes controlling anthocyaninpigmentation are functionally conserved among plant species and have distinct sets of target genes. Plant Cell 5, 1497–1512. doi: 10.1105/tpc.5.11.1497
Ramsay, N. A., and Glover, B. J. (2005). MYB-bHLH-WD40 protein complex and the evolution of cellular diversity. Trends Plant Sci. 10, 63–70. doi: 10.1016/j.tplants.2004.12.011
Schaart, J. G., Dubos, C., Fuente, I. R. D. L., Houwelingen, A. M. M. L. V., Vos, R. C. H. D., Jonker, H. H., et al. (2013). Identification and characterization of MYB-bHLH-WD40 regulatory complexes controlling proanthocyanidin biosynthesis in strawberry. New Phytol. 197, 454–467. doi: 10.1111/nph.12017
Spelt, C., Quattrocchio, F., Mol, J. N. M., and Koes, R. (2000). Anthocyanin1 of petunia encodes a basic helix-loop-helix protein that directly activates transcription of structural anthocyanin genes. Plant Cell 12, 1619–1631. doi: 10.1105/tpc.12.9.1619
Stracke, R., Ishihara, H., Barsch, G. H. A., Mehrtens, F., Niehaus, K., and Weisshaar, B. (2007). Differential regulation of closely related R2R3-MYB transcription factors controls flavonol accumulation in different parts of the Arabidopsis thaliana seedling. Plant J. 50, 660–677. doi: 10.1111/j.1365-313X.2007.03078.x
Tamura, K., Peterson, D., Peterson, N., Stecher, G., Nei, M., and Kumar, S. (2011). MEGA5: molecularevolutionary genetics analysis using maximum likelihood, evolutionary distance, and maximumparsimony methods. Mol. Biol. Evol. 28, 2731–2739. doi: 10.1093/molbev/msr121
Terrier, N., Torregrosa, L., Ageorges, A., Vialet, S., Verries, C., Cheynier, V., et al. (2009). Ectopic expression of VvMYBPA2 promotes proanthocyanidin biosynthesis in grapevine and suggests additional targets in the pathway. Plant Physiol. 149, 1028–1041. doi: 10.1104/pp.108.131862
Thompson, J. D., Higgins, D. G., and Gibson, T. J. (1994). CLUSTAL W: improving the sensitivity of progressive multiple sequence alignment through sequence weighting, position-specific gap penalties and weight matrix choice. Nucleic Acids Res. 22, 4673–4680. doi: 10.1093/nar/22.22.4673
Tuan, P. A., Bai, S. L., Yaegaki, H., Tamura, T., Hihara, S., Moriguchi, T., et al. (2015). The crucial role of PpMYB10.1 in anthocyanin accumulation in peach and relationships between its allelic type and skin color phenotype. BMC Plant Biol. 15:280. doi: 10.1186/s12870-015-0664-5
Walker, A. R., Davison, P. A., Bolognesi-Winfield, A. C., James, C. M., Srinivasan, N., Blundell, T. L., et al. (1999). The TRANSPARENT TESTA GLABRA1 locus, which regulates trichome differentiation and anthocyanin biosynthesis in Arabidopsis, encodes a WD40 repeat protein. Plant Cell 11, 1337–1349. doi: 10.1105/tpc.11.7.1337
Wang, H., Zhang, H., Yang, Y., Li, M., Zhang, Y., Liu, J., et al. (2020). The control of red colour by a family of MYB transcription factors in octoploid strawberry (Fragaria x ananassa) fruits. Plant Biotechnol. J. 18, 1169–1184. doi: 10.1111/pbi.13282
Winkel-Shirley, B. (2001). Flavonoid biosynthesis. a colorful model for genetics, biochemistry, cell biology, and biotechnology. Plant Physiol. 126, 485–493. doi: 10.1104/pp.126.2.485
Xu, F., Li, T., Xu, P. B., Li, L., Du, S. S., Lian, H. L., et al. (2016). DELLA proteins physically interact with CONSTANS to regulate flowering under long days in Arabidopsis. FEBS Lett. 590, 541–549. doi: 10.1002/1873-3468.12076
Xu, P., Lian, H., Xu, F., Zhang, T., Wang, S., Wang, W., et al. (2019). Phytochrome Band AGB1 coordinately regulate photomorphogenesis by antagonistically modulating PIF3 stability in Arabidopsis. Mol. Plant 12, 229–247. doi: 10.1016/j.molp.2018.12.003
Xu, P., Zawora, C., Li, Y., Wu, J., Liu, L., Liu, Z., et al. (2018). Transcriptome sequencing reveals role of light in promoting anthocyanin accumulation of strawberry fruit. Plant Growth Regul. 86, 121–132. doi: 10.1007/s10725-018-0415-3
Xu, W., Dubos, C., and Lepiniec, L. (2015). Transcriptional control of flavonoid biosynthesis by MYB-bHLH-WDR complexes. Trends Plant Sci. 20, 176–185. doi: 10.1016/j.tplants.2014.12.001
Xu, W., Peng, H., Yang, T., Whitaker, B., Huang, L., Sun, J., et al. (2014). Effect of calcium on strawberry fruit flavonoid pathway gene expression and anthocyanin accumulation. Plant Physiol. Biochem. 82, 289–298. doi: 10.1016/j.plaphy.2014.06.015
Zhang, J. X., Zhang, Y. C., Dou, Y. J., Li, W. J., Wang, S. M., Shi, W. J., et al. (2017). Single nucleotide mutation in FvMYB10 may lead to the yellow fruit in Fragaria vesca. Mol. Breed. 37:35.
Zhang, Y., Butelli, E., and Martin, C. (2014). Engineering anthocyanin biosynthesis in plants. Curr. Opin. Plant Biol. 19, 81–90. doi: 10.1016/j.pbi.2014.05.011
Zhang, Z., Shi, Y., Ma, Y., Yang, X., Yin, X., Zhang, Y., et al. (2020). The strawberry transcription factor FaRAV1 positively regulates anthocyanin accumulation by activation of FaMYB10 and anthocyanin pathway genes. Plant Biotechnol. J. 18, 2267–2279. doi: 10.1111/pbi.13382
Keywords: woodland strawberry, MBW complex, flavonoid biosynthesis, MYB, bHLH
Citation: Xu P, Wu L, Cao M, Ma C, Xiao K, Li Y and Lian H (2021) Identification of MBW Complex Components Implicated in the Biosynthesis of Flavonoids in Woodland Strawberry. Front. Plant Sci. 12:774943. doi: 10.3389/fpls.2021.774943
Received: 13 September 2021; Accepted: 20 October 2021;
Published: 08 November 2021.
Edited by:
Fangyuan Zhang, Southwest University, ChinaReviewed by:
Yu-Qing He, Zhejiang Academy of Agricultural Sciences, ChinaAndrea Moglia, University of Turin, Italy
Copyright © 2021 Xu, Wu, Cao, Ma, Xiao, Li and Lian. This is an open-access article distributed under the terms of the Creative Commons Attribution License (CC BY). The use, distribution or reproduction in other forums is permitted, provided the original author(s) and the copyright owner(s) are credited and that the original publication in this journal is cited, in accordance with accepted academic practice. No use, distribution or reproduction is permitted which does not comply with these terms.
*Correspondence: Hongli Lian, hllian@sjtu.edu.cn