- 1Neiker-BRTA, Centro de Arkaute, Campus Agroalimentario de Arkaute, Arkaute, Spain
- 2Laboratório de Fisiologia do Desenvolvimento e Genética Vegetal, Universidade Federal de Santa Catarina, Florianópolis, Brazil
- 3Departamento de Botânica, Universidade Federal de Santa Catarina, Florianópolis, Brazil
Changes in the chemical environment at the maturation stage in Pinus spp. somatic embryogenesis will be a determinant factor in the conversion of somatic embryos to plantlets. Furthermore, the study of biochemical and morphological aspects of the somatic embryos could enable the improvement of somatic embryogenesis in Pinus spp. In the present work, the influence of different amino acid combinations, carbohydrate sources, and concentrations at the maturation stage of Pinus radiata D. Don and Pinus halepensis Mill. was analyzed. In P. radiata, the maturation medium supplemented with 175 mM of sucrose and an increase in the amino acid mixture (1,100 mgL–1 of L-glutamine, 1,050 mgL–1 of L-asparagine, 350 mgL–1 of L-arginine, and 35 mgL–1 of L-proline) promoted bigger embryos, with a larger stem diameter and an increase in the number of roots in the germinated somatic embryos, improving the acclimatization success of this species. In P. halepensis, the maturation medium supplemented with 175 mM of maltose improved the germination of somatic embryos. The increase in the amount of amino acids in the maturation medium increased the levels of putrescine in the germinated somatic embryos of P. halepensis. We detected significant differences in the amounts of polyamines between somatic plantlets of P. radiata and P. halepensis; putrescine was less abundant in both species. For the first time, in P. radiata and P. halepensis somatic embryogenesis, we detected the presence of cadaverine, and its concentration changed according to the species.
Introduction
Somatic embryogenesis has been suggested as the most promising method for vegetative propagation for a large number of conifers (Gupta et al., 1993), and it can help to capture the greatest benefits from traditional breeding programs by multiplying trees with desirable characteristics for plantation forestry (Pullman and Bucalo, 2014).
Pinus radiata D. Don is one of the most planted pine species in the world, and it is widely used to produce quality wood (Escandon et al., 2017; Fuentes-Sepulveda et al., 2020). Alternatively, Pinus halepensis Mill. is a species with high forest importance for reforestation because it can survive under low precipitation levels, and it has higher tolerance to thermal and drought stress (Gazol et al., 2017; Manrique-Alba et al., 2020; Quinto et al., 2021).
Previous studies carried out in our laboratory have demonstrated that P. radiata and P. halepensis showed different responses to abiotic stress such as drought and high temperature (do Nascimento et al., 2020). Also, in previous experiments, we have focused on the optimization of somatic embryogenesis (SE) processes. In this sense, the optimization of initiation and proliferation stages of SE in P. radiata (Montalbán et al., 2010, 2012) and P. halepensis (Montalbán et al., 2013) has been our objective in recent years. Recently, some studies focused on promoting and improving the embryogenic process in terms of quality and quantity of somatic embryos (ses) obtained by reduction of water availability in P. radiata (Garcia-Mendiguren et al., 2016; Castander-Olarieta et al., 2020) and P. halepensis (Pereira et al., 2016, 2017; do Nascimento et al., 2020) have been carried out. However, the rate of germination (%) and the subsequent conversion into plantlets is sometimes low (do Nascimento et al., 2020), and additional research is needed to increase somatic plantlet regeneration (Montalbán and Moncaleán, 2019).
The source of organic nitrogen is an important component of the culture media for the success of the SE process, and in this sense, amino acids are commonly used to improve the maturation of ses, modifying its type and concentration (Gerdakaneh et al., 2011; Satish et al., 2016; Dahrendorf et al., 2018). As Maruyama et al. (2021) reported, the presence of organic nitrogen improved the production of ses. Casein and glutamine are usually used in the SE of conifers (Afele and Saxena, 1995; Pullman and Bucalo, 2014; Dahrendorf et al., 2018), but, in some species, such as Pinus patula Schiede ex Schltdl. and Cham. or P. radiata, it has been seen that mixtures of amino acids can be more beneficial for the process (Malabadi and Van Staden, 2005; Montalbán et al., 2010).
The beneficial effect of chemical environment modification in a certain stage of the process can be witnessed not only in that stage but also in the following ones (Dahrendorf et al., 2018; Castander-Olarieta et al., 2019; do Nascimento et al., 2020). In this case, the presence of a carbon source in the culture medium is necessary due to the heterotrophy conditions in which the cells grow (Yaseen et al., 2013). In addition, the carbohydrates used in the SE increase the osmolality of the culture medium (Smeekens et al., 2010; Kube et al., 2014). The most common source of carbohydrates in the culture medium is sucrose, but other carbohydrates such us maltose have been used in other Pinus species such as Pinus nigra Arn. (Salaj et al., 2019). Therefore, due to the different responses observed in the SE of various species, it is necessary to carry out a study about the effect of different carbohydrates and concentrations (Yang et al., 2020).
Polyamines are considered to be a class of compounds related to cellular proliferation (Bais and Ravishankar, 2002; Jimenez, 2005) and play an important role in the regulation of SE (Silveira et al., 2004; Jin et al., 2020; Sundararajan et al., 2021). Moreover, polyamines (PAs) could be associated with the stress caused in plant cells, protecting them and upregulating stress-related genes (Alcazar et al., 2020; Kielkowska and Adamus, 2021). PAs levels vary according to each stage of SE, for example, during the intense cell division observed in the proliferation phase; higher diamine putrescine (Put) contents were reported (Minocha et al., 2004), whereas, in the maturation stage, where intense cell differentiation occurs, higher levels of triamine spermidine (Spd) and tetraamine spermine (Spm) were observed (Minocha et al., 2004; Gemperlova et al., 2009). In conifers, the accumulation of high levels of PAs was correlated also with the conversion of ses in plants (Gemperlova et al., 2009). Moreover, PAs have been considered as markers of SE competence in several species, such as Oryza sativa L. (Shoeb et al., 2001), Medicago sativa L. (Huang et al., 2001), and Panax ginseng C.A. Meyer (Monteiro et al., 2002).
In plants, the three most common PAs are Put, Spd, Spm (Bouchereau et al., 1999; Minocha et al., 2014), and cadaverine (Cad), which are rarely reported (Jancewicz et al., 2016). Additionally, as the amino acids are precursors of PAs biosynthesis, the exogenous addition of amino acids in the medium culture can directly influence PA profiles (Bagni and Tassoni, 2001; Pullman and Bucalo, 2014). The biosynthesis of Put, Spd, and Spm is directly related to arginine and ornithine (de Oliveira et al., 2018), while the biosynthesis of Cad is associated with lysine and ornithine (Liu et al., 2014). In Araucaria angustifolia Bert. O. Ktze., the ratio of PAs determined the transition from embryonal masses (EMs) to ses (Farias-Soares et al., 2014).
The aim of this work was to evaluate the influence of the different amino acid mixtures and carbohydrate sources, applied during the maturation stage of the SE, on the number and morphology of the ses obtained, their PAs profile, and the morphological characteristics of the P. radiata and P. halepensis plantlets developed.
Materials and Methods
Plant Material
Embryonal masses (Ems) were obtained from immature cones of P. radiata, collected from four mother trees in a seed orchard set-up by Neiker-BRTA in Deba (Spain; latitude: 43°16′59″N, longitude: 2°17′59″W, elevation: 50 m) and of P. halepensis, collected from five mother trees in Berantevilla (Spain; latitude: 42°40′57.14″N, longitude: 2°51′29.95″W, elevation: 473 m). The immature seeds of both species were extracted and surface sterilized, following Montalbán et al. (2010, 2012). Seed coats were removed, and intact megagametophytes were excised out aseptically and cultured on initiation media for both species; for P. radiata, the basal medium was Embryo Development Medium (EDM; Walter et al., 2005), and for P. halepensis, the basal medium used was the DCR medium (Gupta and Durzan, 1985). Initiation and proliferation were carried out following the protocols described by Montalbán et al. (2012, 2013) for P. radiata and P. halepensis, respectively. Five established cell lines (ECLs) were used for each experiment and species.
Amino Acid Supplementation Experiment
The maturation of P. halepensis and P. radiata EMs was carried out following the method described in Montalbán et al. (2010, 2013), respectively. Briefly, the EMs were suspended in a liquid basal medium without plant growth regulators and then filtered on a filter paper in a Büchner funnel. Aliquots containing 0.08 g of EMs on the filter papers were placed on each maturation media. The basal media (DCR for P. halepensis and EDM for P. radiata) were supplemented with 175 mM of sucrose, 9 gL–1 of Gelrite®, and 75 μM of abscisic acid (ABA) for P. halepensis or 60 μM of ABA for P. radiata; these maturation media were supplemented after autoclaving with different amino acid mixtures as described in Table 1: MIX I (Control) (Walter et al., 2005), MIX II – two times the concentration in the MIX I of the L-glutamine (Gln), L-asparagine (Asn), L-arginine (Arg), and L-proline (Pro); and MIX III – 4-fold the concentration in the MIX I of the Gln (Table 1).
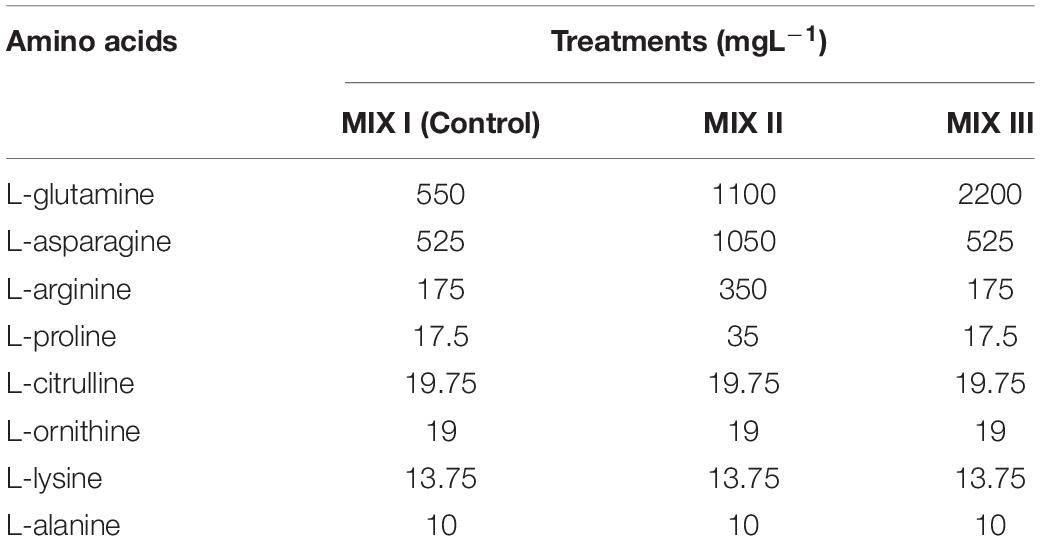
Table 1. Different combinations of amino acids used in the maturation media (EDM and DCR) for Pinus radiata D. Don and Pinus halepensis Mill.
Both, P. radiata and P. halepensis cultures were kept at 23°C and in darkness for 16 weeks on maturation media.
The germination of cotyledonary ses and the acclimatization of plantlets were performed according to Montalbán and Moncaleán (2019). Briefly, the ses were germinated for 8 weeks on half macronutrients LP [Quoirin and Lepoivre (1977), modified by Aitken-Christie et al. (1988)], supplemented with 2 gL–1 of activated charcoal and 9 gL–1 of Difco Agar granulated (Becton and Dickinson). First, petri dishes (90 mm × 15 mm) were used as containers, with the root caps of the ses pointing downward at an angle of approximately 60°. After this, the germinated plantlets were subcultured in the same medium for another month, but, in EcoBox® (Eco2Box/green filter: a polypropylene vessel with a “breathing” hermetic cover, 125 mm × 65 mm × 80 mm, Duchefa). The cultures were kept at 23°C under 16-h photoperiod at 120 μmolm–2 s–1 provided by cool white fluorescent tubes (TFL 58 W/33; Philips, France) (Montalbán and Moncaleán, 2019).
After the germination, the plantlets were acclimatized in 43-cm3 pots, containing blond peat moss (Pindstrup, Ryomgård, Denmark): vermiculite (8:2, v/v) in the greenhouse under controlled conditions.
Carbohydrate Supplementation Experiment
Pinus radiata and P. halepensis EMs obtained from the same materials and methods as in the amino acid supplementation experiment were used. The basal media described in amino acid supplementation experiment were supplemented with the MIX I (Table 1), and two different carbohydrate sources at two concentrations (four treatments) were assayed: 175 mM of sucrose (175 suc – control); 175 mM of maltose (175 mal); 350 mM of sucrose (350 suc), or 350 mM of maltose (350 mal). Cultures were kept at 23°C and in darkness for 16 weeks. Germination and acclimatization followed the same methodology described in the material and methods of the amino acid supplementation experiment.
Osmolality of the Maturation Media
The osmolality (mosmkg–1) of all maturation media was measured at the onset of the experiment using a Micro-Osmometer Automatic (Löser Messtechnik, Berlin, Germany) according to the manufacturer’s instructions. Aliquots containing 100 μl of each maturation medium without Gelrite® were measured, and the measurements were replicated three times (Montalbán et al., 2010).
Free Polyamine Content Determination
Germinated ses obtained after 2 weeks in germination media were analyzed for free PA content for P. radiata (amino acid supplementation experiment) and P. halepensis (amino acid supplementation experiment and carbohydrate supplementation experiment). Germinated ses of P. radiata were not analyzed for the carbohydrate supplementation experiment, because we only obtained viable germinated ses for the control treatment. PA quantification was carried out according to Silveira et al. (2004) with some modifications. Samples (200 mg of fresh weight) were briefly grounded in 1.4 ml of 5% perchloric acid (v/v) in a Precellys® shaker. After 1 h, the extraction solution was centrifuged for 20 min (15,000 g, 4°C), and supernatant was collected. The pellet was once again suspended in 0.2 ml of perchloric acid and centrifuged for 20 min (15,000 g, 4°C). Both extraction solutions were merged, homogenized, and frozen at −20°C for future analysis. Derivatization was performed according to Silveira et al. (2004), where 40 μl of each sample was mixed with 20 μl of diaminoheptane 0.05 mM, 50 μl of saturated sodium carbonate solution, and 100 μl of dansyl chloride in acetone 1.8 mM. After 50 min of incubation in the dark at 70°C, 25 μl of proline was added to the solution, followed by another 30-min incubation at room temperature. After that, 200 μl of toluene was added, the solution was vigorously shaken, and 175 μl of the superior organic phase with PAs was taken to a SpeedVac freeze dryer for 40 min at 40°C. Finally, pellets were suspended in 175 μl of acetonitrile and were ready for high-performance liquid chromatography (HPLC) quantification. Mobile phases were composed of (A) 10% (v/v) acetonitrile/ultrapure water pH 3.5 adjusted with HCl and (B) 100% of acetonitrile. The gradient started at 65% of B and lasted for 11 min; it was raised to 100% B for up to 25 min, maintained in 100% B until 35.5 min and then returned to 65% B until the end at 44 min. The flow rate was constantly 1 ml min–1, and oven temperature was maintained at 40°C. The stationary phase was a 5-μm Shim-pack CLC-ODS (M) 100 Å column with 250 mm × 4.6 mm equipped with a pre-column, both from Shimadzu®. The analyses were carried out in a Shimadzu Prominence HPLC equipped with a fluorescent detector configured to excitation of 340 nm and emission of 510-nm wavelengths. To determine PA concentration, peak areas of 20 μl samples were compared to triplicates of peak areas of correspondent standards of Put, Spd, Cad, and Spm, bought from Sigma-Merck®. The 1,7-diaminoheptane (DAH) (Sigma-Merck®) was used as an internal standard. Total free PAs were obtained by the sum of the individual PAs. Moreover, based on the fact that Put is a precursor to Spd and Spm (Mustafavi et al., 2018), the Put/(Spd + Spm) ratio was calculated. The final concentration of PAs was expressed in mmolμg–1.
Data Collection and Statistical Analysis
For both species P. radiata and P. halepensis, the number of normal (NNE) (white to yellowish, non-germinating, with a distinct hypocotyl region, and at least three cotyledons) and abnormal mature ses (NAE) (germinating precociously, with fewer than three cotyledons or bearing abnormally shaped cotyledons) (Montalbán et al., 2010) per gram of embryogenic tissue was recorded. Also, the length (mm) (LE) and width (mm) (WE) of 240 (Amino acid supplementation experiment on each species) and 320 (Carbohydrate supplementation experiment on each species) NNE, and the LE/WE ratio of NNE were calculated. Embryos were obtained in three of the five maturated lines in both experiments and species. From the total of ses obtained, one half of them was germinated for the morphological analysis and the other half was germinated for the determination of free PA content. After 2 months in a germination medium, the germination rate for the NNE was calculated. Before ex vitro planting, the length of plantlets (mm), the length of aerial part (mm), the width of needles (mm), the stem diameter (mm), and the root length (mm) were measured with a digital caliber, and the number of secondary roots was counted in 10 plantlets per treatment. The percentage of acclimatization was calculated after 2 months in ex vitro conditions.
For Amino acid supplementation experiment, three different amino acid mixtures were tested in five ECLs for each species (R2, R9, R54, R82, and R137 for P. radiata and H48, H51, H149, H153, and H204 for P. halepensis) in a factorial with four repetitions (plates) per maturation condition and embryogenic cell line. In carbohydrate supplementation experiment, four different carbohydrates sources and concentrations were tested in five ECLs for P. radiata (R2, R9, R54, R82, and R137) and for P. halepensis (H48, H51, H149, H153, and H204) in a factorial with four repetitions.
For the effect of the ECLs on each of the variables of this study, an analysis of deviance was performed, followed by a Tukey post hoc test (α = 0.05), adjusted for multiple comparisons. To obtain robust conclusions, ECL factors were introduced into all the models as a block variable to reduce variability and analyze the effect of the culture medium more accurately.
In Amino acid supplementation experiment, the width of needles and stem diameter, for P. radiata, and P. halepensis, respectively, were square root transformed, and an ANOVA was performed. Multiple comparisons were made using the Tukey post hoc tests (α = 0.05). The same occurred in the carbohydrate supplementation experiment, for P. halepensis, in length of plantlets, width of needles, and the stem diameter.
In the amino acid supplementation experiment, for P. radiata, the NNE, length of plantlets, stem diameter, root part length, and number of secondary roots did not fulfill homoscedasticity and normality assumptions for ANOVA, and therefore, a Kruskal–Wallis was performed. The same occurred for the characteristics: P. halepensis—NNE, NAE, length of normal embryo, width of normal embryo, width of needles, and number of secondary roots in the amino acid supplementation experiment; P. radiata—length of normal embryo and the LE/WE ratio of ses in the carbohydrate supplementation experiment; and P. halepensis—NNE, NAE, length of normal embryo, width of normal embryo, the LE/WE ratio of ses, root part length, and number of secondary roots in the carbohydrate supplementation experiment.
For free PA content determination, the data obtained were log transformed, and an ANOVA was performed, followed by Tukey post hoc tests (α = 0.05), adjusted for multiple comparisons.
The data were analyzed using R software®, version 3.6.1. (R Core Team, 2017).
Results
Osmolality of the Maturation Medium
Statistically significant differences in the osmolality were observed between all the culture media with different compositions of amino acids and carbohydrates (Table 2). The osmolalities of the maturation media (EDM and DCR) were significantly higher when they contained 350 mM of sucrose compared with other carbohydrate and amino acid treatments (Table 3). Both of the maturation media supplemented with 175 mM of maltose showed the lowest values of osmolality (Table 3). Statistically significant differences were observed when comparing the osmolality of MIX I (control) with the media supplemented with MIX II and MIX III (Table 3).
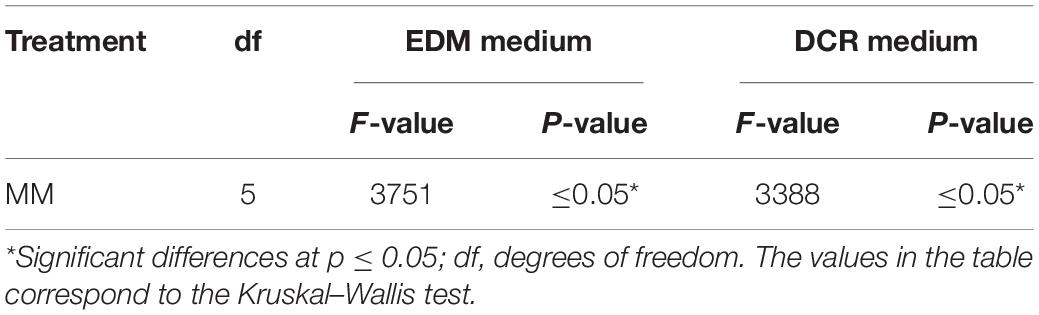
Table 2. Analysis of deviance for the osmolality (mosmkg–1 water) of the different maturation media (MM) for Pinus radiata D. Don (EDM medium) and Pinus halepensis Mill (DCR medium).
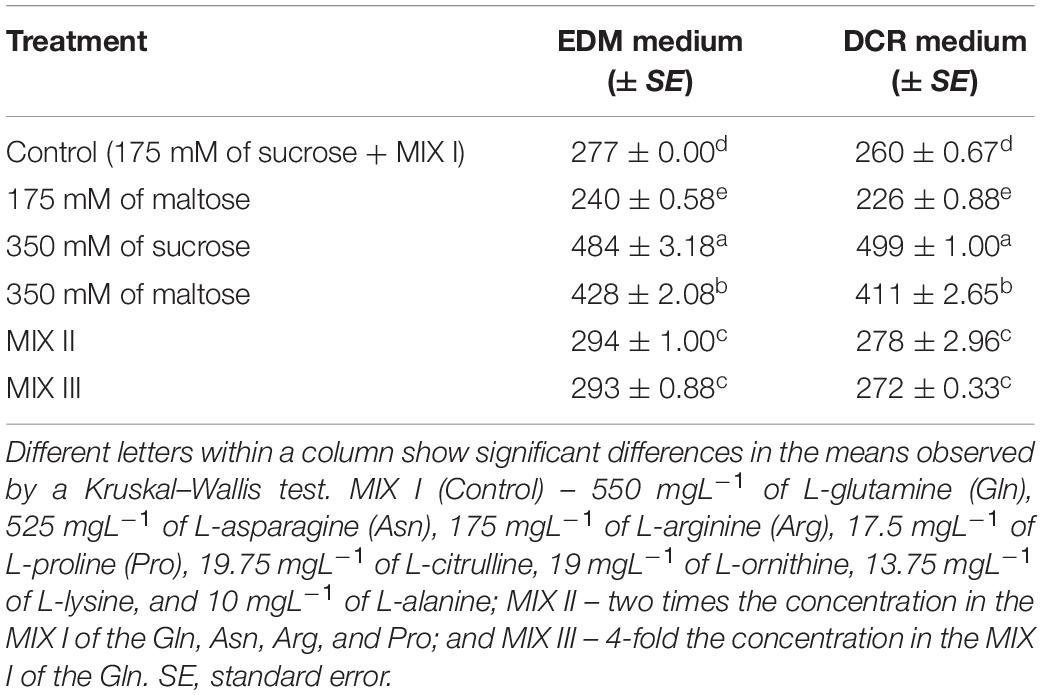
Table 3. Osmolality (mosmkg–1 water) of the maturation media (M ± SE) for Pinus radiata D. Don (EDM medium) and Pinus halepensis Mill. (DCR medium).
Amino Acid Supplementation Experiment
Regarding the amino acid mixture used in the maturation media, statistically significant differences were found for all the parameters evaluated in P. radiata (NAE, LE, WE, germination of ses, and acclimatization of plantlets) except for the NNE and LE/WE ratio (Table 4).
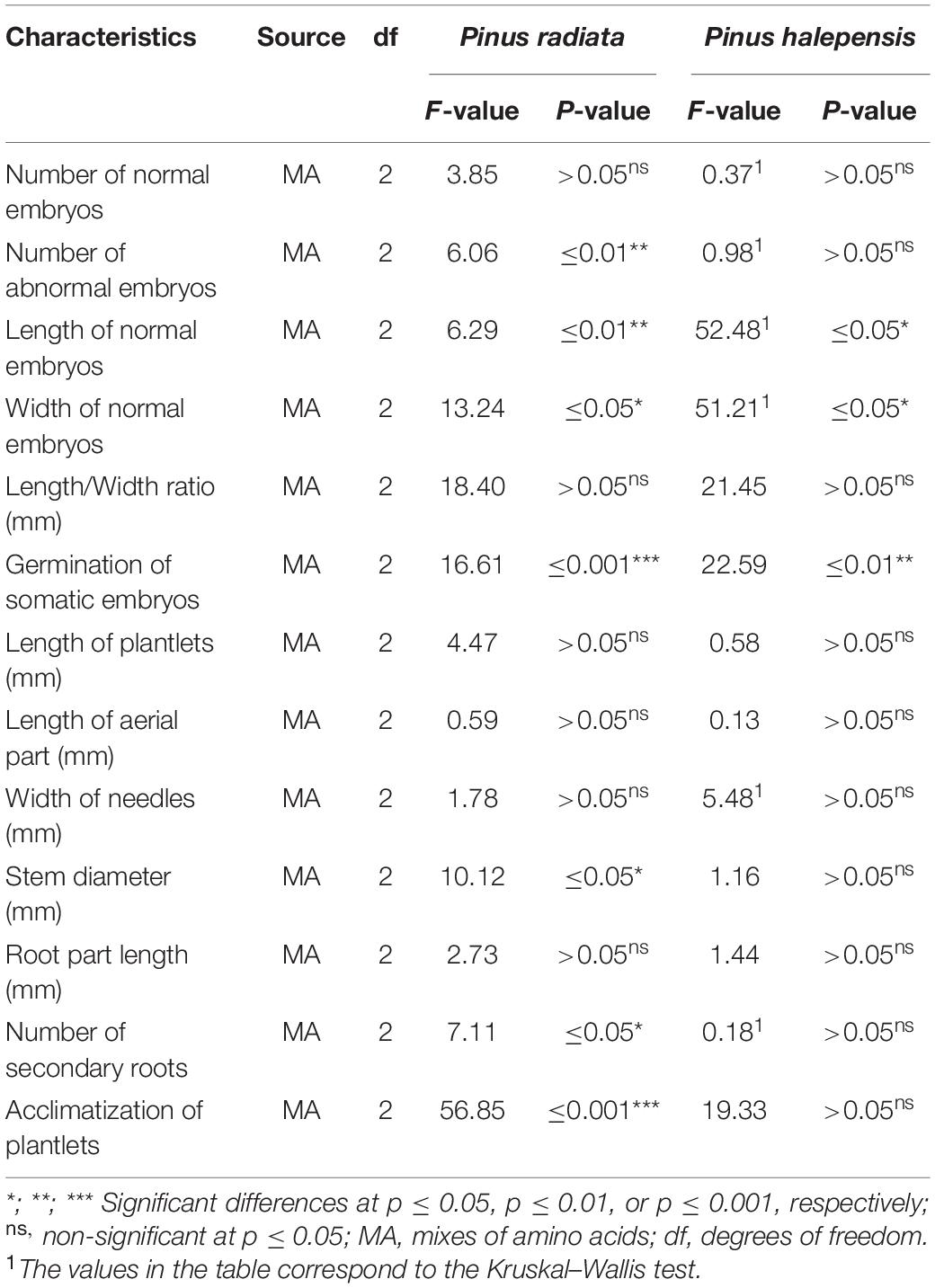
Table 4. Analysis of deviance for the effect of the different mixes of amino acids (MA) in the maturation stage in: the number of normal and abnormal somatic embryos per 0.08 g of embryonal masses, the morphological characteristics of normal embryos, the germination of somatic embryos, the morphological characteristics, and the acclimatization of plantlets of Pinus radiata D. Don and Pinus halepensis Mill., respectively.
In the case of P. halepensis, the different mixtures of amino acids produced statistically significant differences for the LE, WE, and germination rate of NNE (Table 4). However, the mixture of different amino acids did not show statistically significant differences for the NNE, NAE, the LE/WE ratio, and acclimatization of plantlets (Table 4).
Figures 1A,B show normal and aberrant morphologies of ses of P. radiata, respectively. For P. radiata, although non-significant differences were found among the different combinations of amino acids when compared with the production of NNE (Table 4), an increase of the NNE in MIX II and MIX III (240 and 317 sesg–1 fresh weight, respectively), compared with the control (MIX I – 157 sesg–1 fresh weight), was observed (Figure 2A). The same tendency was found for the NAE, with a statistically significant increase for NAE in media supplemented with MIX II and MIX III when compared to MIX I (Figure 2A). Moreover, we observed that treatments MIX II and MIX III induced an increase in LE and WE when compared to control treatment (MIXI) (Figures 2B,D,E,F). With respect to the LE/WE ratio, non-significant differences were found between the different combinations of amino acids (Figure 2C).
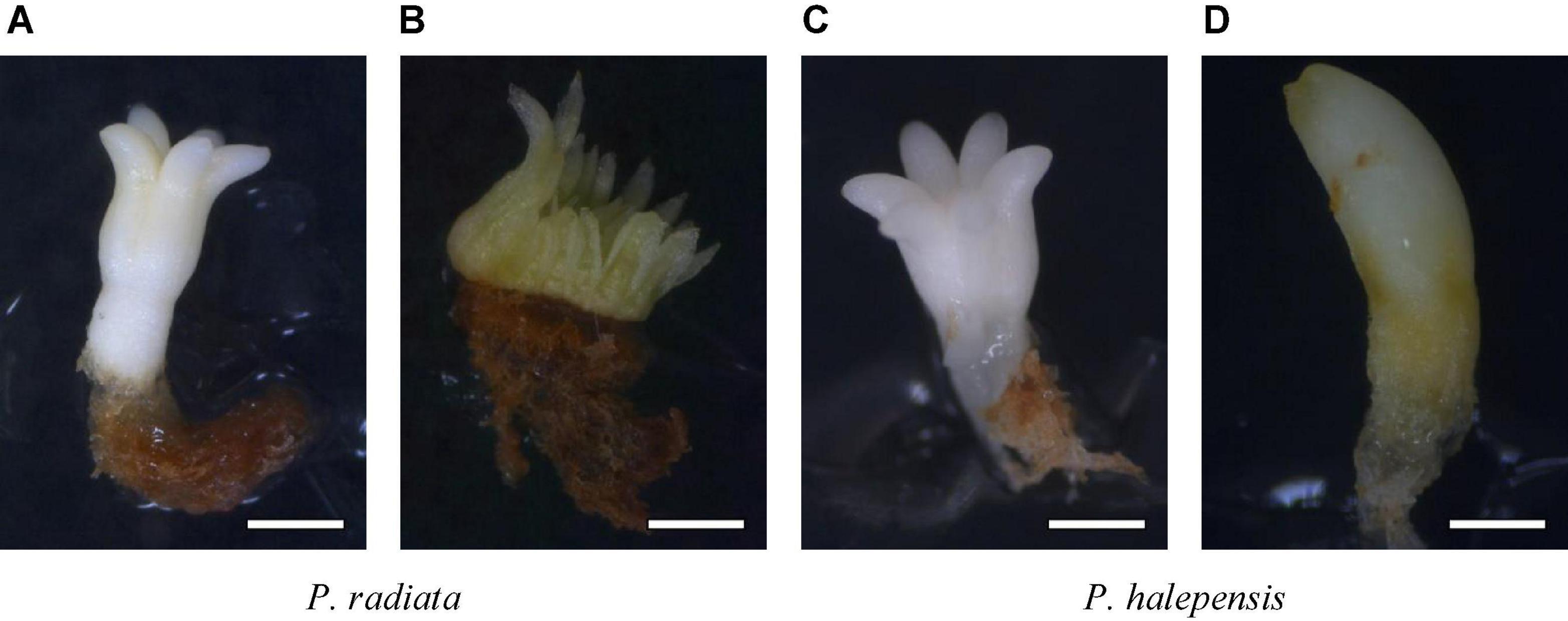
Figure 1. Somatic embryos showing different morphologies: Pinus radiata D. Don normal somatic (A), bar = 1.44 mm; P. radiata abnormal somatic embryo (B), bar = 1.85 mm; Pinus halepensis Mill. normal somatic embryos (C), bar = 0.84 mm; P. halepensis abnormal somatic embryos (D), bar = 0.60 mm.
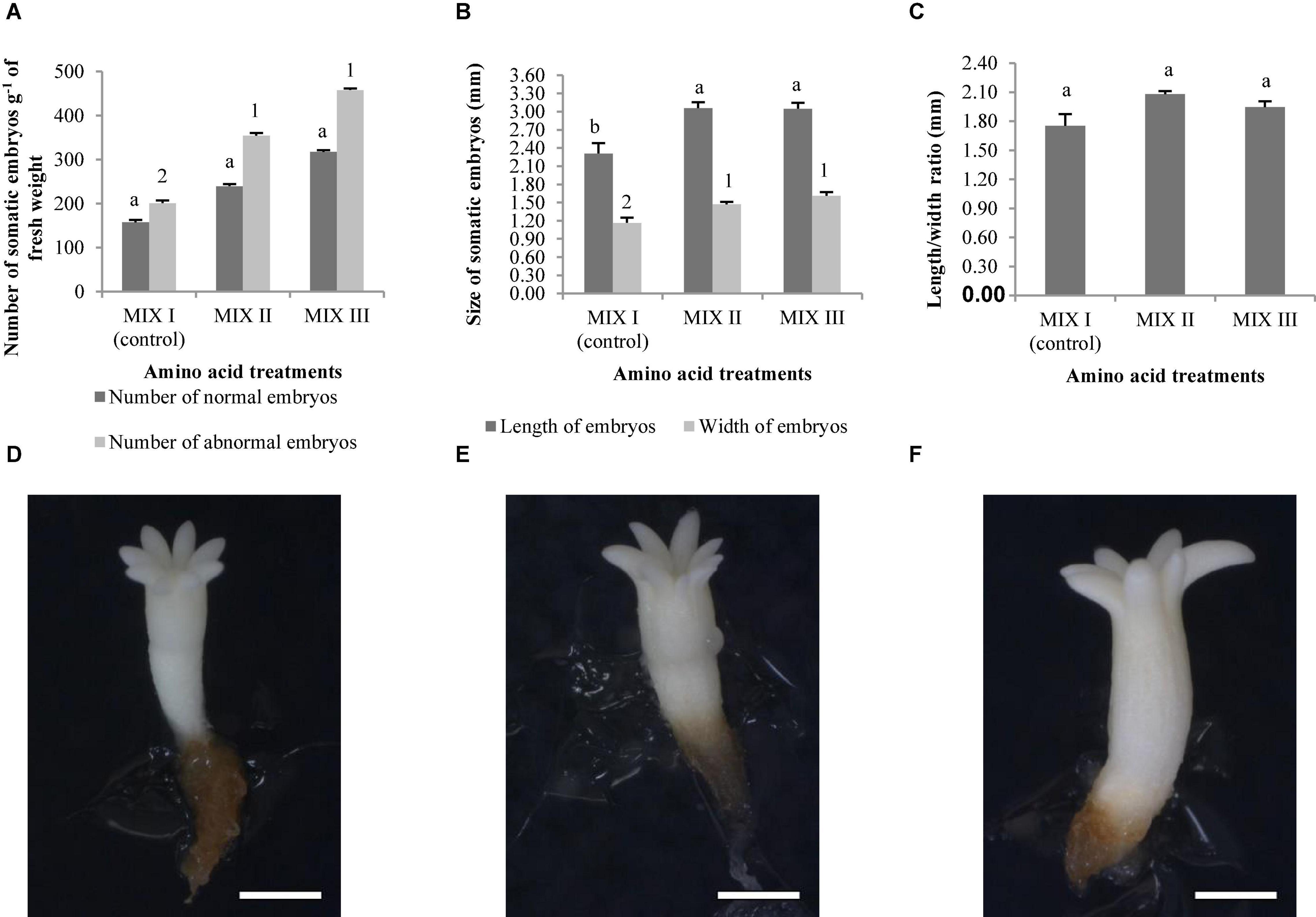
Figure 2. Pinus radiata D. Don somatic embryos of maturation media supplemented with different mixes of amino acids. Number of normal and abnormal somatic embryos (A); the length and width of normal embryos (B); and the length/width ratio of normal embryos (C). Bars indicate standard errors. Significant differences at p ≤ 0.05 are indicated by different letters or numbers. Pinus radiata normal somatic obtained from maturation media supplemented with different mixes of amino acids. MIX I (D), bar = 1.85 mm; MIX II (E), bar = 1.85 mm; and MIX III (F), bar = 1.85 mm. MIX I (Control) – 550 mgL–1 of L-glutamine (Gln), 525 mgL–1 of L-asparagine (Asn), 175 mgL–1 of L-arginine (Arg), 17.5 mgL–1 of L-proline (Pro), 19.75 mgL–1 of L-citrulline, 19 mgL–1 of L-ornithine, 13.75 mgL–1 of L-lysine, and 10 mgL–1 of L-alanine; MIX II – two times the concentration in the MIX I of the Gln, Asn, Arg, and Pro; and MIX III – 4-fold the concentration in the MIX I of the Gln.
Figures 1C,D show normal and aberrant morphologies of ses of P. halepensis, respectively. For P. halepensis, although non-significant differences were found among the different mixtures of amino acids for NNE and NAE (Table 4), the highest NNE and NAE were recorded in control conditions (68 sesg–1 fresh weight for NNE and 178 sesg–1 fresh weight for NAE); MIX II showed intermediate values, and MIX III presented the lowest values (23 sesg–1 fresh weight for NNE and 109 sesg–1 fresh weight for NAE) (Figure 3A).
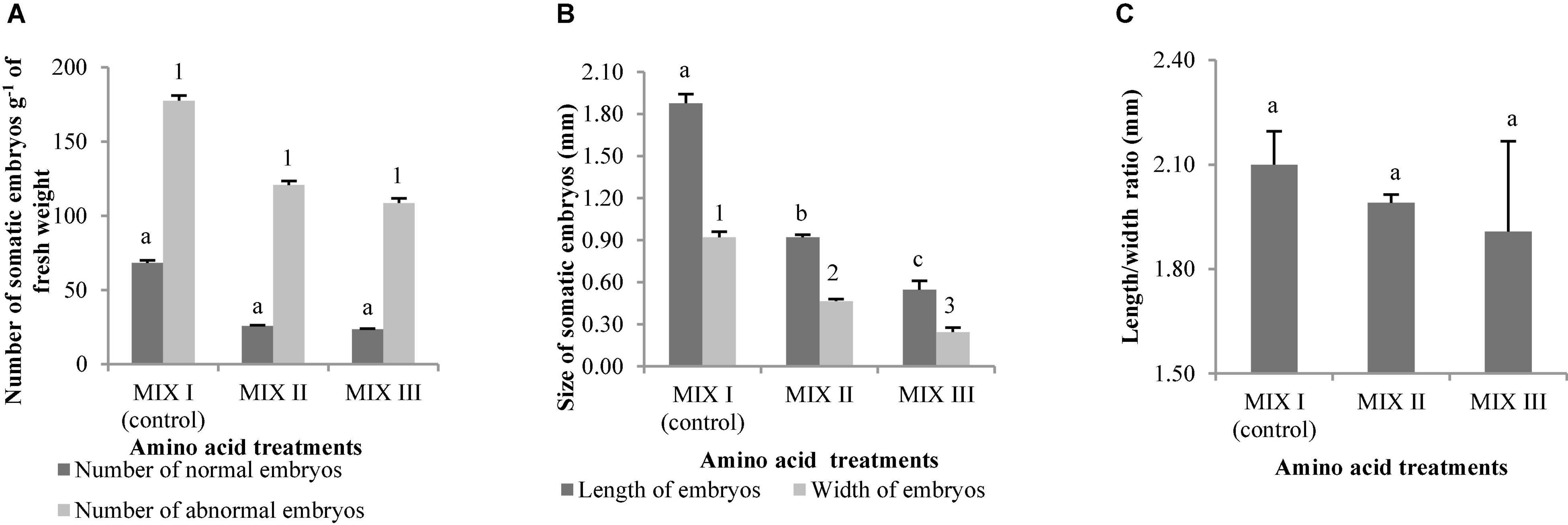
Figure 3. Pinus halepensis Mill. somatic embryos obtained per gram of embryonal masses matured in different mixes of amino acids. Number of normal and abnormal somatic embryos (A); the length and width of normal embryos (B); the length/width ratio of normal embryos (C). Bars indicate standard errors. Significant differences at p ≤ 0.05 are indicated by different letters or numbers. MIX I (Control) – 550 mgL–1 of L-glutamine (Gln), 525 mgL–1 of L-asparagine (Asn), 175 mgL–1 of L-arginine (Arg), 17.5 mgL–1 of L-proline (Pro), 19.75 mgL–1 of L-citrulline, 19 mgL–1 of L-ornithine, 13.75 mgL–1 of L-lysine, and 10 mgL–1 of L-alanine; MIX II – two times the concentration in the MIX I of the Gln, Asn, Arg, and Pro; and MIX III – 4-fold the concentration in the MIX I of the Gln.
Pinus halepensis ses developed in control conditions (MIX I) were significantly wider and longer than those obtained in MIX II and MIX III. Significantly lower LE and WE values were obtained when the maturation medium was supplemented with a 4-fold concentration of Gln (MIX III) (Figure 3B), although the LE/WE ratio did not show significant differences (Figure 3C).
As observed in Figure 4A, the best germination percentage of P. radiata was obtained in those embryos coming from ECLs maturated on a medium supplemented with double the concentration of Gln, Asn, Arg, and Pro (MIX II), but not in relation to control treatment (MIX I). However, the treatment with 4-fold of Gln (MIX III) promoted a significant decrease of the ses germination with respect to MIX II (Figure 4A). Plantlets of P. radiata survived after acclimatization with significantly higher percentage of acclimatization when coming from ses maturated on a culture medium supplemented with MIX II (99%) and MIX III (89.29%) than compared with control (MIX I) (49.21%) (Figures 4B, 5).
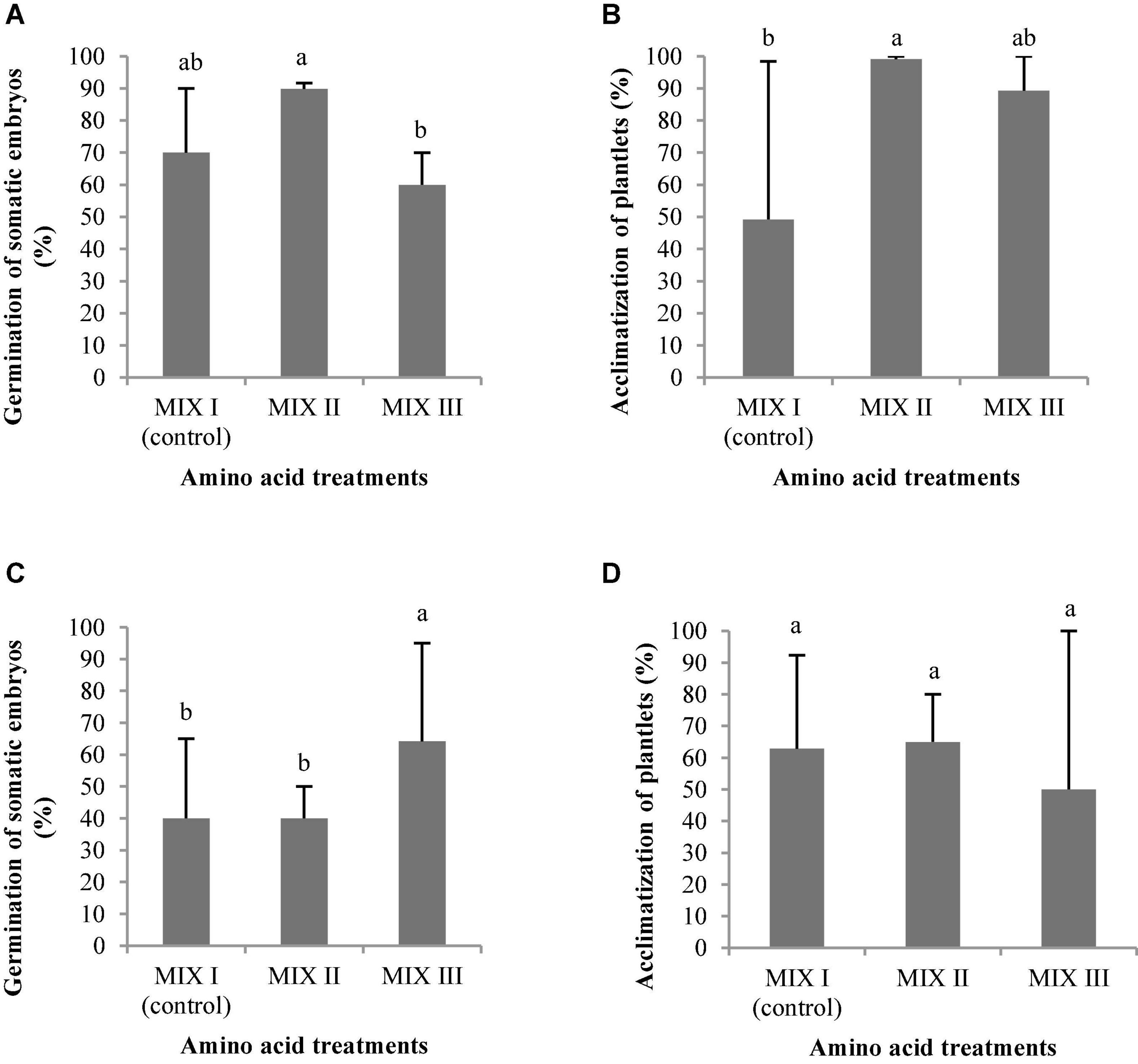
Figure 4. Pinus radiata D. Don germination (%) of somatic embryos maturated in a medium supplemented with different mixes of amino acids (A). Acclimatization (%) of plantlets of P. radiata obtained from somatic embryos maturated in a medium supplemented with different mixes of amino acids (B). Germination (%) of somatic embryos of Pinus halepensis Mill. maturated in a medium supplemented with different mixes of amino acids (C). Acclimatization (%) of plantlets of P. halepensis obtained from somatic embryos maturated in a medium supplemented with different mixes of amino acids (D). Bars indicate standard errors. Significant differences at p ≤ 0.05 are indicated by different letters. MIX I (Control) – 550 mgL–1 of L-glutamine (Gln), 525 mgL–1 of L-asparagine (Asn), 175 mgL–1 of L-arginine (Arg), 17.5 mgL–1 of L-proline (Pro), 19.75 mgL–1 of L-citrulline, 19 mgL–1 of L-ornithine, 13.75 mgL–1 of L-lysine, and 10 mgL–1 of L-alanine; MIX II – two times the concentration in the MIX I of the Gln, Asn, Arg, and Pro; and MIX III – 4-fold the concentration in the MIX I of the Gln.
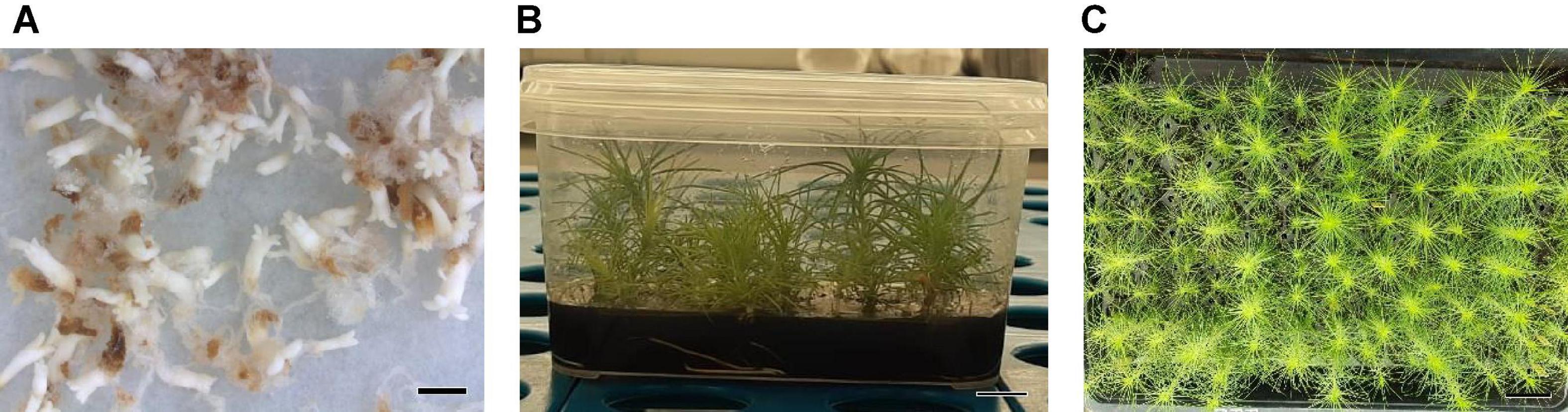
Figure 5. Maturation, germination, and acclimatization of somatic embryos from Pinus radiata D. Don embryogenic cell lines. Somatic embryos after 4 months in a maturation medium (A), bar = 3 mm; somatic plantlets after 3 months in a germination medium (B), bar = 1.4 cm; plantlets derived from normal cotyledonary somatic embryos growing in the greenhouse (C), bar = 2.35 cm. The maturation medium was supplemented with MIX I (Control) – 550 mgL–1 of L-glutamine (Gln), 525 mgL–1 of L-asparagine (Asn), 175 mgL–1 of L-arginine (Arg), 17.5 mgL–1 of L-proline (Pro), 19.75 mgL–1 of L-citrulline, 19 mgL–1 of L-ornithine, 13.75 mgL–1 of L-lysine, and 10 mgL–1 of L-alanine; MIX II – two times the concentration in the MIX I of the Gln, Asn, Arg, and Pro; and MIX III – 4-fold the concentration in the MIX I of the Gln.
Pinus halepensis germination significantly increased in those embryos coming from ECLs maturated in the presence of 4-fold the concentration of Gln (MIX III) (64%) when compared to results obtained with other amino acid combinations (Figure 4C). Significant differences were not observed for the survival of plantlets in the greenhouse (62.82, 65, and 50% for MIX I, MIX II, and MIX III, respectively) (Figure 4D).
Statistically significant differences for the stem diameter and the number of secondary roots were observed in P. radiata (Table 4). The mean length of the plantlets ranged from 38.13 to 47.66 mm, and this value was divided into the aerial part and the root part (Table 5). In this sense, the width of the needle varied between 0.27 and 0.32 mm (Table 5). The MIX II and MIX III promoted a significant increase of the stem diameter when compared to the control (Figure 6A). The same tendency was observed with the number of secondary roots where the MIX II promoted a significant increase in the number of secondary roots (Figures 6B,C).
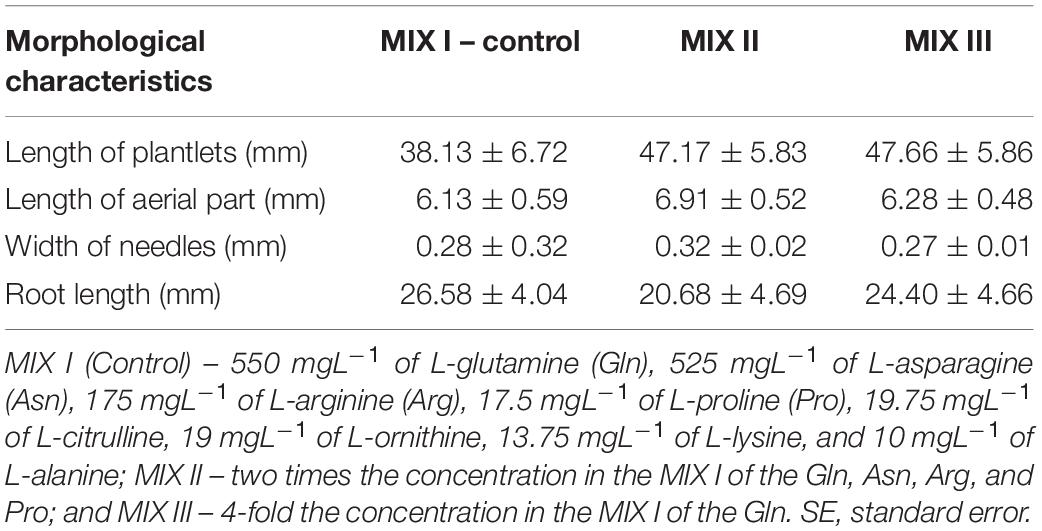
Table 5. Morphological characteristics of P. radiata D. Don somatic plantlets developed from ECLs maturated in a culture medium supplemented with different amino acid compositions (M ± SE).
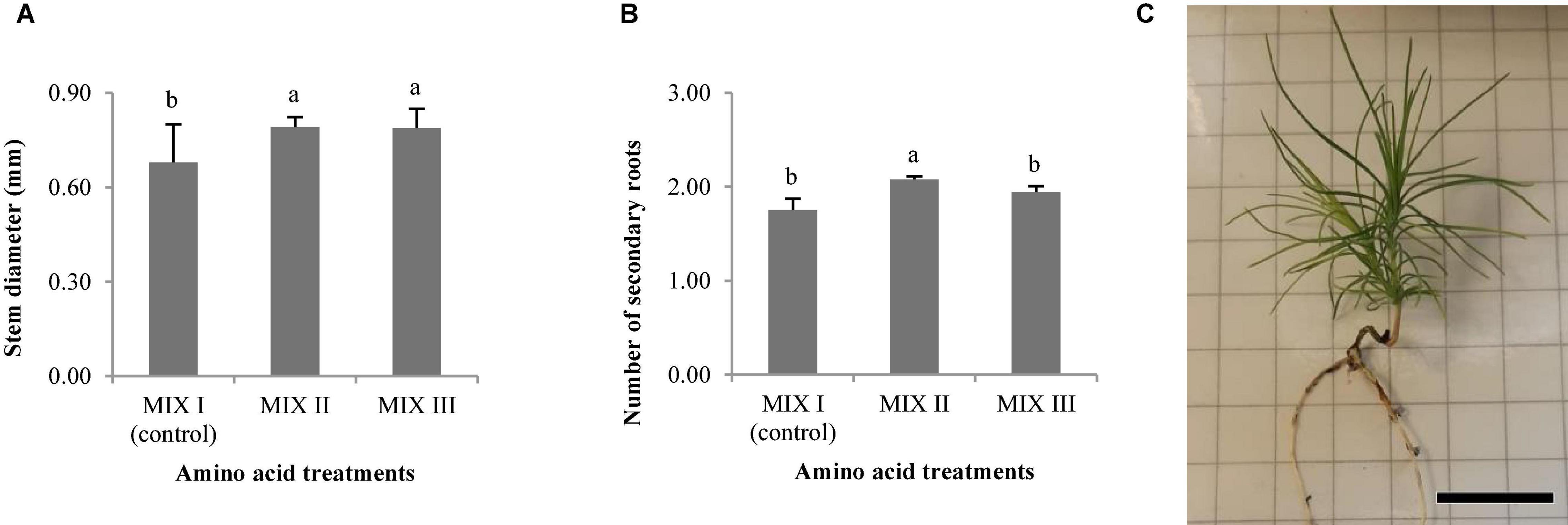
Figure 6. Morphological characteristics of Pinus radiata D. Don plantlets, stem diameter (mm) (A), and number of secondary roots (B). Bars indicate standard errors. Significant differences at p ≤ 0.05 are indicated by different letters or numbers. Plantlet obtained from somatic embryos maturated in EDM medium with MIX II (C), bar = 2 cm. MIX I (Control) – 550 mgL–1 of L-glutamine (Gln), 525 mgL–1 of L-asparagine (Asn), 175 mgL–1 of L-arginine (Arg), 17.5 mgL–1 of L-proline (Pro), 19.75 mgL–1 of L-citrulline, 19 mgL–1 of L-ornithine, 13.75 mgL–1 of L-lysine and 10 mgL–1 of L-alanine; MIX II, twice the concentration in the MIX I of the Gln, Asn, Arg, and Pro; MIX III, fourfold the concentration in the MIX I of the Gln.
When evaluating the morphological characteristics of the germinated ses developed from ECLs of P. halepensis maturated in the presence of different combinations of amino acids, no statistically significant differences were observed (Tables 4, 6).
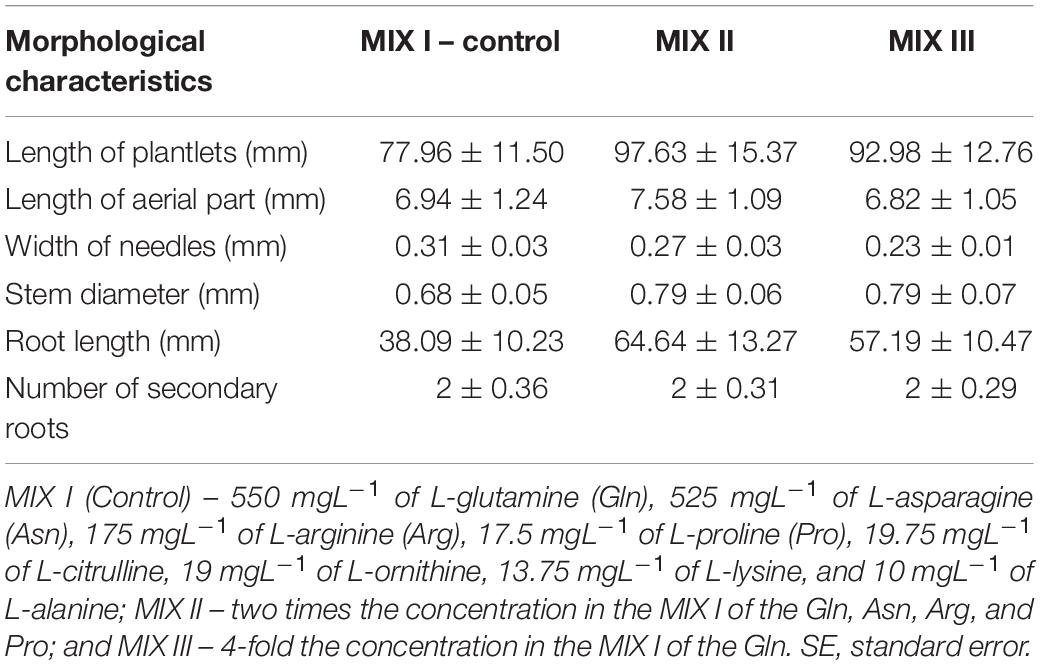
Table 6. Morphological characteristics of Pinus halepensis Mill. somatic plantlets developed from ECLs maturated in a culture medium supplemented with different amino acid compositions (M ± SE).
Carbohydrate Supplementation Experiment
The carbohydrate sources significantly affected the NNE, the NAE, and the LE (Table 7). For NAE, WE, and the LE/WE ratio, a significant interaction between the carbohydrate sources and concentrations was observed (Table 7). Finally, the carbohydrate source and the carbohydrate concentration had a significant effect on the germination rates, but there was no interaction between the previously mentioned factors (Table 7).
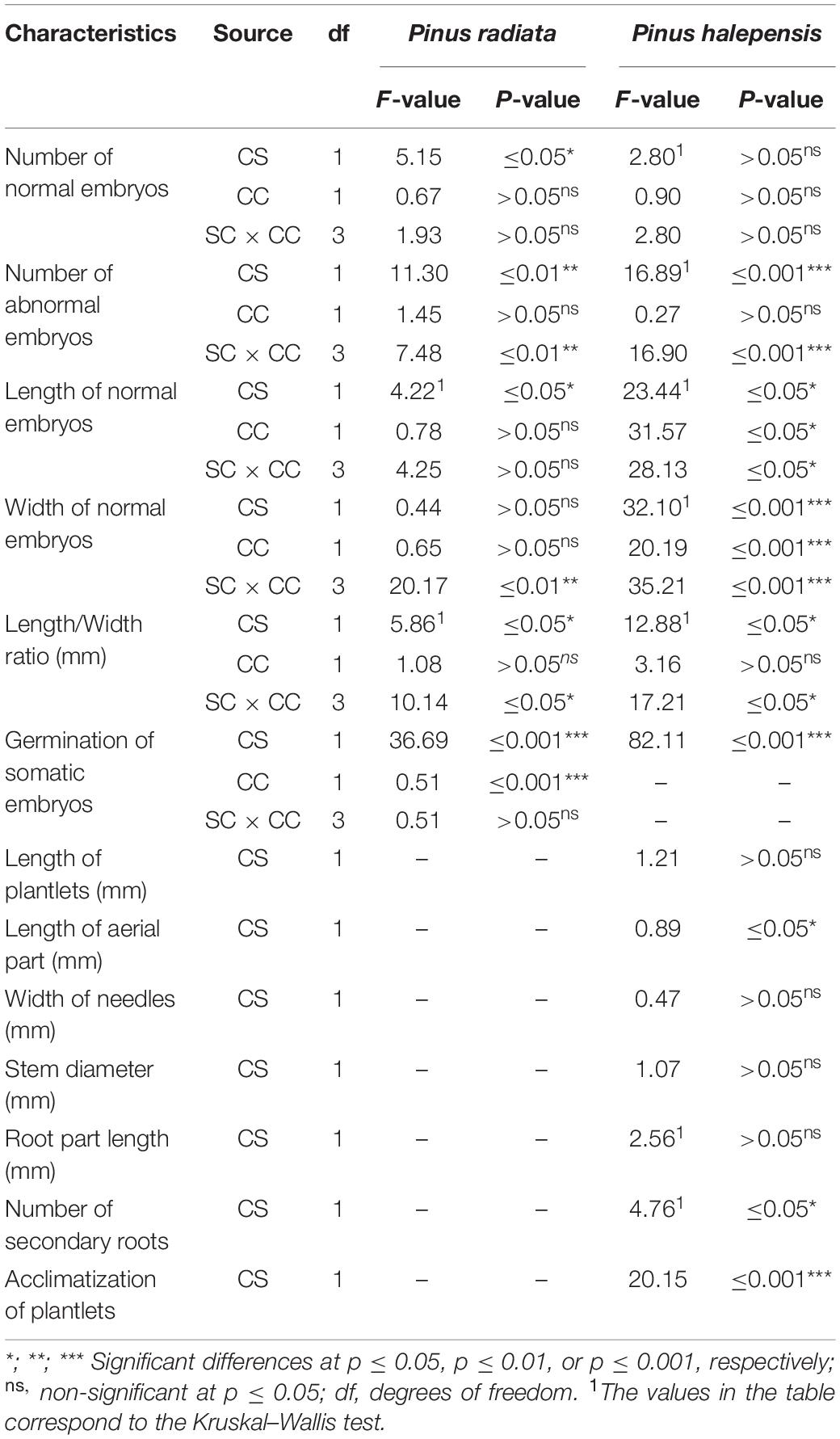
Table 7. Analysis of deviance for the effect of the different carbohydrate sources (CS) and concentrations (CC) in the maturation stage in the number of normal and abnormal somatic embryos per 0.08 g of embryonal masses; the morphological characteristics of normal embryos; the germination of somatic embryos of Pinus radiata D. Don and Pinus halepensis Mill., respectively; and the effect of the different CS in the germination of somatic embryos, the morphological characteristics, and the acclimatization of plantlets P. halepensis.
Two types of carbohydrates in both concentrations produced normal and aberrant embryos in P. radiata. However, the use of sucrose in the maturation medium, regardless of the concentration, resulted in a significantly higher NNE (Figure 7A). In this regard, we also observed the highest NAE in the medium of maturation with sucrose but only at a concentration of 175 suc (control) in relation to maltose (Figure 7A). In addition, the same tendency was observed for LE and WE (Figure 7B), with the development of longer and wider ses in the maturation medium with sucrose, regardless of the concentration. The ses maturated in media with sucrose presented a significantly higher LE/WE ratio than those obtained from a medium with 350 mal (Figure 7C).
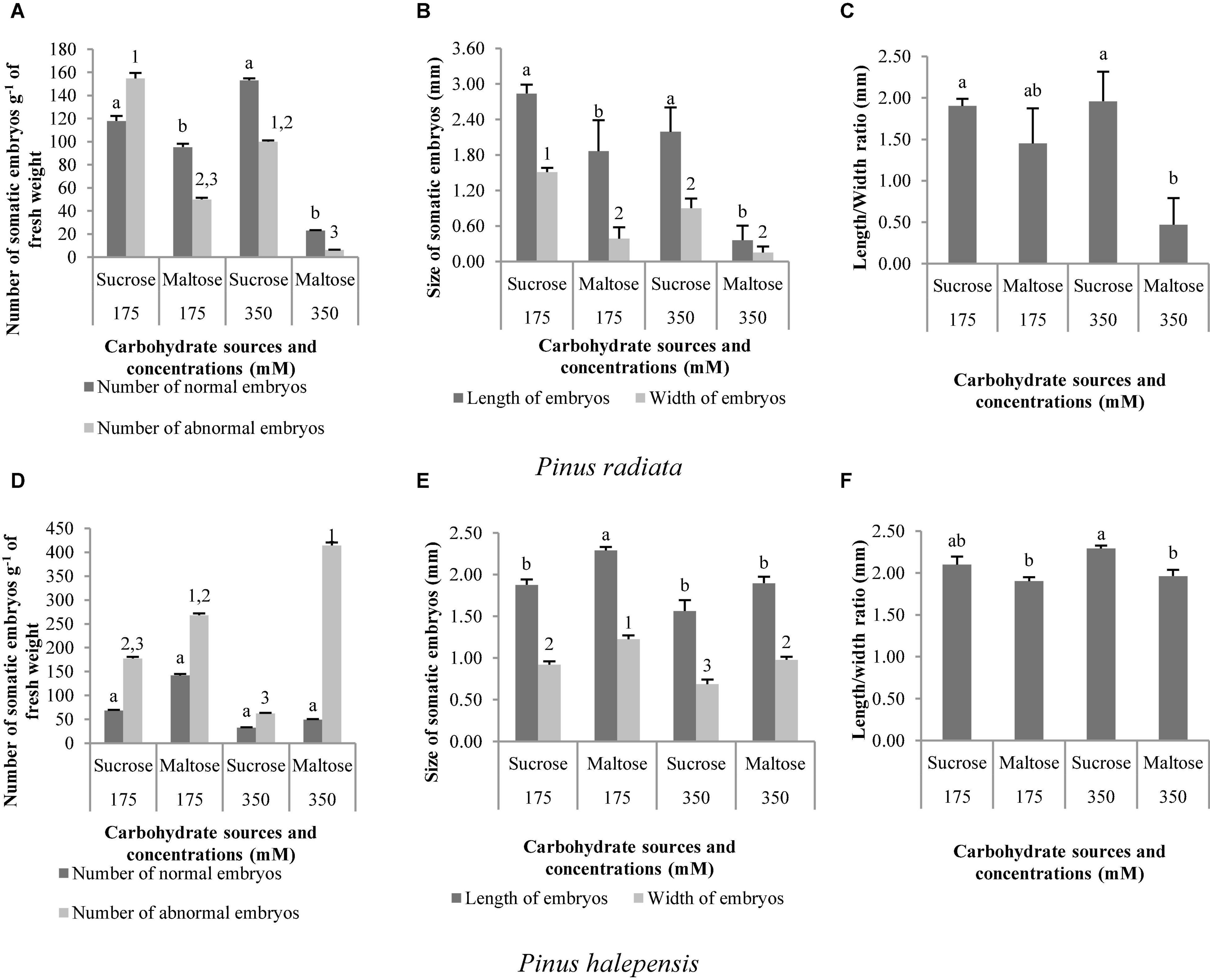
Figure 7. Pinus radiata D. Don and Pinus halepensis Mill. somatic embryos obtained per gram of embryonal masses matured in different carbohydrate sources and concentrations. Number of normal and abnormal somatic embryos (A,D); the length and width of normal embryos (B,E); and the length/width ratio of normal embryos (C,F). Bars indicate standard errors. Significant differences at p ≤ 0.05 are indicated by different letters or numbers.
In P. halepensis, the NNE was not affected by the different carbohydrate treatments tested (Table 7 and Figure 7D). However, NAE, LE, WE, and the LE/WE ratio were significantly affected by the interaction between the carbohydrate source and the concentration (Table 7). The medium with the highest maltose concentration produced a significantly higher number of NAE than the sucrose media (Figure 7D).
The LE and the WE of P. halepensis obtained in a maturation medium supplemented with 175 mal were significantly higher than the LE obtained after maturation with other carbohydrate sources or concentrations (Figure 7E). As noted in Figure 7F, a significantly higher LE/WE ratio was observed in ses maturated with the highest concentration of 350 suc when compared to results obtained in ses from media supplemented with maltose. Thus, higher concentrations of carbohydrates caused the formation of less developed embryos (Figure 8).
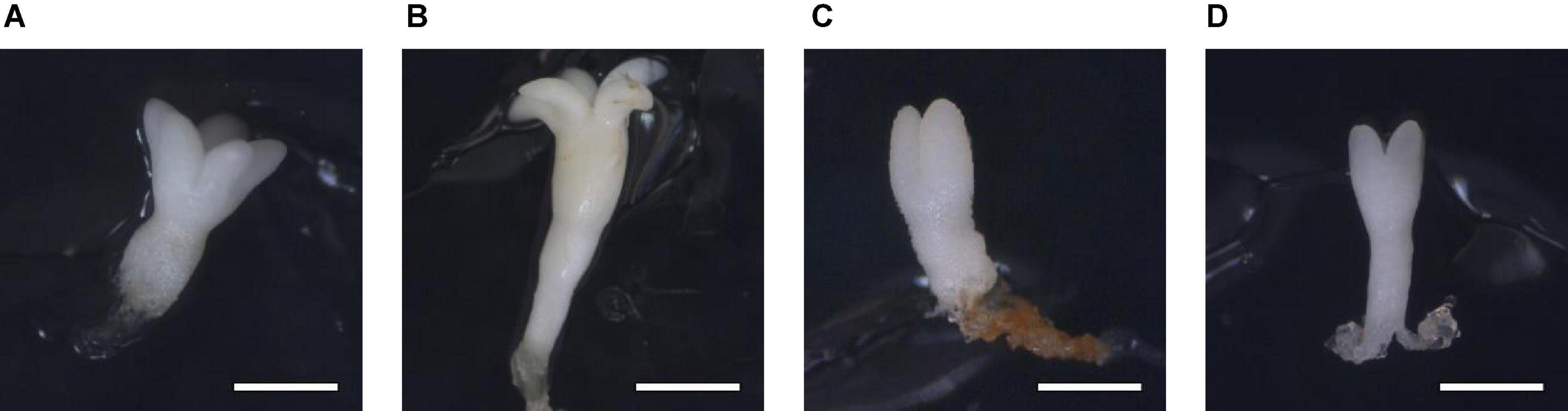
Figure 8. Somatic embryos of Pinus halepensis Mill. maturated in the DCR medium supplemented with: 175 mM of sucrose (A), bar = 1 mm; 175 mM of maltose (B), bar = 1.57 mm; 350 mM of sucrose (C), bar = 0.57 mm; and 350 mM of maltose (D), bar = 1 mm.
The use of 175 suc promoted a germination percentage of 70%, while the other treatments promoted a percentage lower than 15% for ses of P. radiata (Figure 9A). However, the ses maturated at a maturation medium with a high concentration of maltose did not promote the germination of ses (Figure 9A). Viable plantlets were only obtained in those embryos germinated in culture media with 175 suc where the acclimatization percentage was 49.21%.
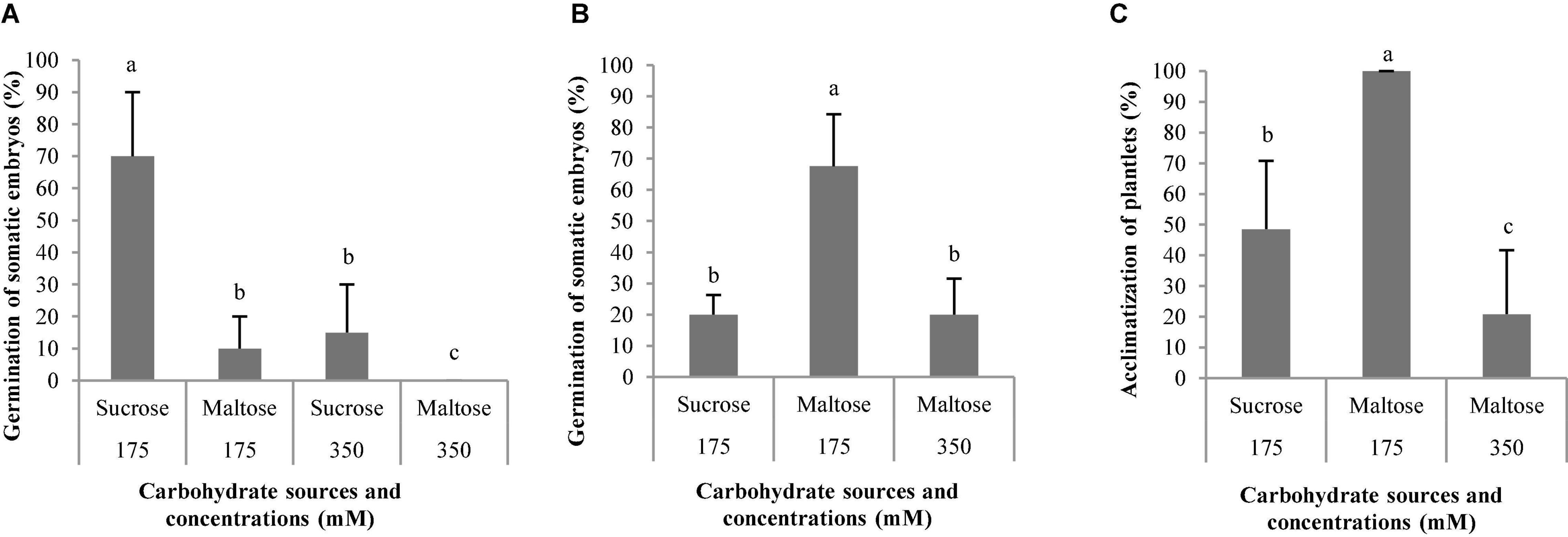
Figure 9. Germination (%) of somatic embryos of Pinus radiata D. Don maturated in a medium supplemented with different carbohydrate sources and concentrations (A). Germination (%) of somatic embryos of Pinus halepensis Mill. maturated in a medium supplemented with different carbohydrate sources and concentrations (B). Acclimatization (%) of plantlets of P. halepensis obtained from somatic embryos maturated in a medium supplemented with different carbohydrate sources and concentrations (C). Bars indicate standard errors. Significant differences at p ≤ 0.05 are indicated by different letters.
The ses of P. halepensis maturated with 175 mal showed a germination percentage significantly (Table 7) higher than the rest of the treatments assayed (Figure 9B). However, the ses maturated with 350 suc did not germinate. The plantlets of P. halepensis from the maturation treatment with 175 mM of maltose survived after acclimatization in a significantly higher percentage (100%) than those that came from a treatment with 175 suc (control) (Table 7 and Figures 9C, 10). In contrast, the worst results were obtained in the treatment with a higher concentration of maltose (Figure 9C).
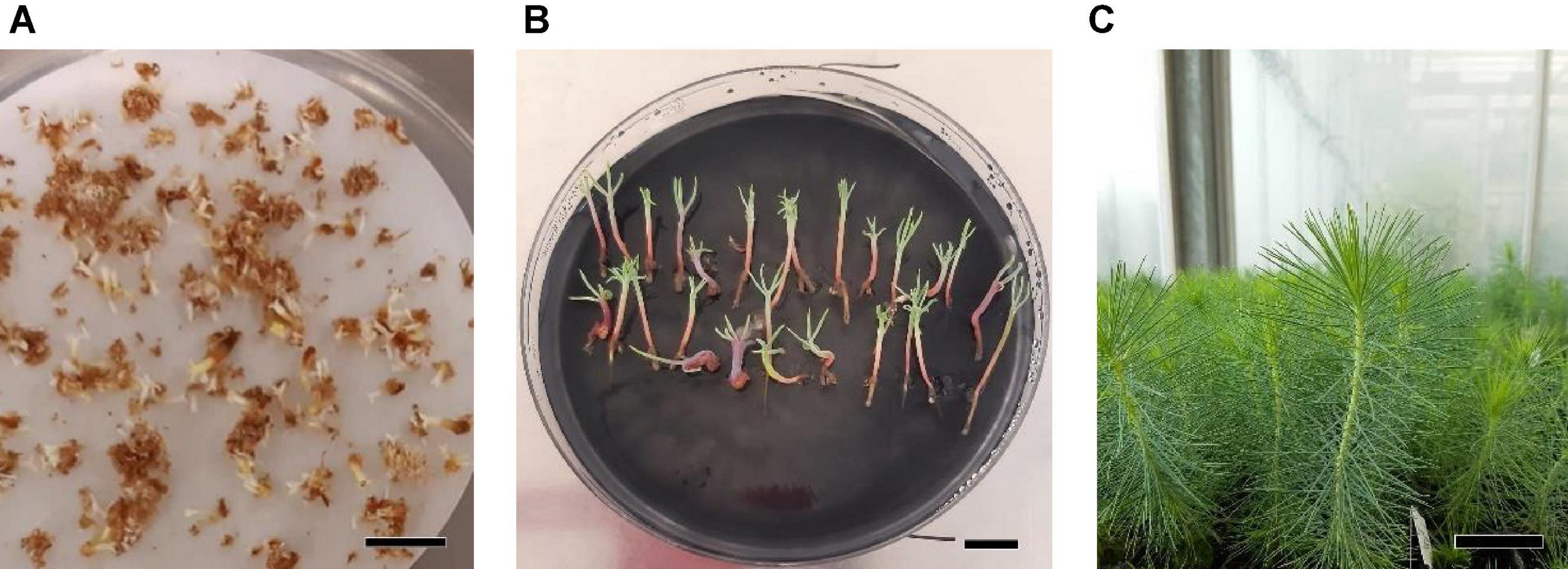
Figure 10. Maturation, germination, and acclimatization of somatic embryos from Pinus halepensis Mill. embryogenic cell lines. Somatic embryos after 4 months in a maturation medium (A), bar = 1 cm; somatic plantlets after 3 months cultivated in a germination medium (B), bar = 1 cm; plantlets derived from normal cotyledonary somatic embryos growing in the greenhouse (C), bar = 3 cm. The maturation medium was supplemented with MIX I (Control) – 550 mgL–1 of L-glutamine (Gln), 525 mgL–1 of L-asparagine (Asn), 175 mgL–1 of L-arginine (Arg), 17.5 mgL–1 of L-proline (Pro), 19.75 mgL–1 of L-citrulline, 19 mgL–1 of L-ornithine, 13.75 mgL–1 of L-lysine, and 10 mgL–1 of L-alanine; MIX II – two times the concentration in the MIX I of the Gln, Asn, Arg, and Pro; and MIX III – 4-fold the concentration in the MIX I of the Gln.
The length of aerial part and number of secondary roots were significantly affected by the different carbohydrate sources, and no differences were observed for the other morphological characteristics studied in P. halepensis (Table 7). It was observed that 175 mal promoted an increase in the aerial part and number of secondary roots in comparison with 175 suc (Figures 11A,B). Although significantly statistical differences were not found for the other morphological characteristics, an increase in the length of plantlets was observed in those obtained from the ses developed in a maturation medium supplemented with 175 mal (Table 8 and Figure 11C). The same results were observed for a stem diameter and the root length; but the largest width of the needle was observed in 175 suc (Table 8).
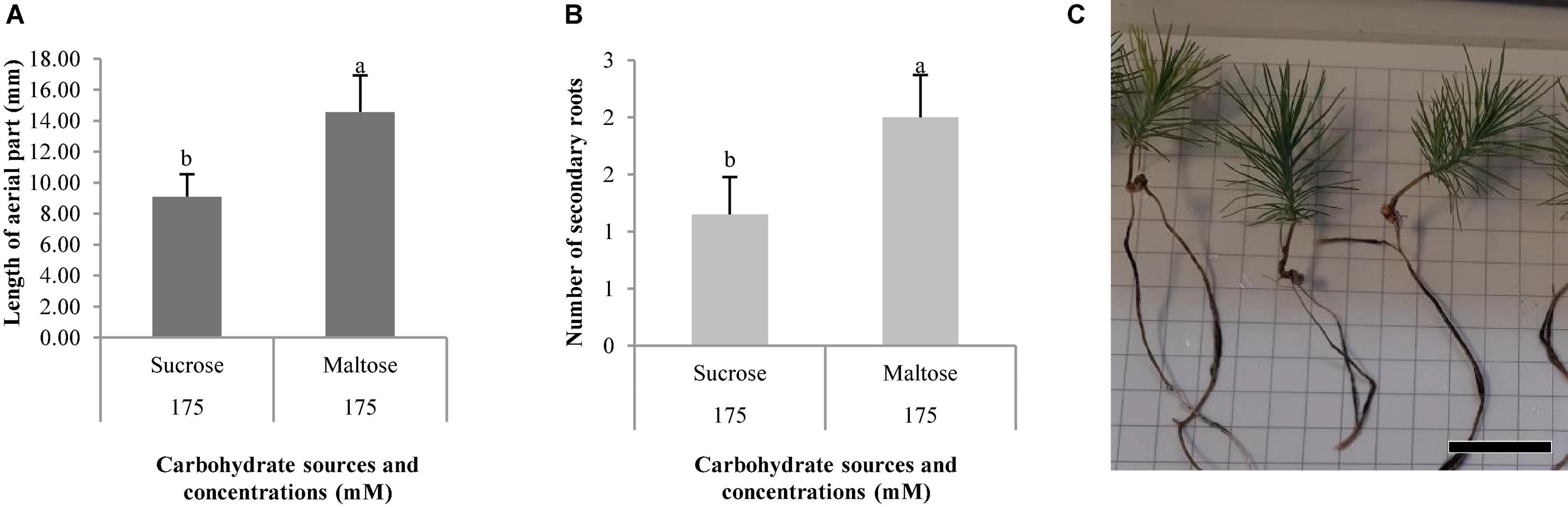
Figure 11. Morphological characteristics of Pinus halepensis Mill. plantlets, length of aerial part (mm) (A), and number of secondary roots (B). Bars indicate standard errors. Significant differences at p ≤ 0.05 are indicated by different letters or numbers. Plantlets of Pinus halepensis Mill. obtained from somatic embryos maturated in the EDM medium supplemented with 175 mM of maltose (C), bar = 3 cm.
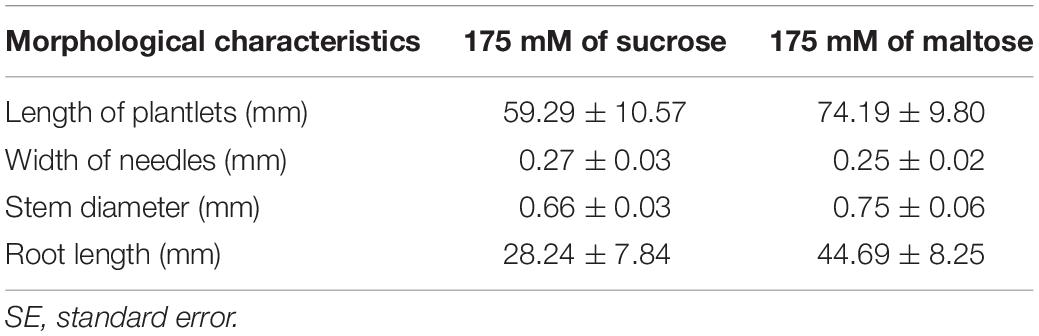
Table 8. Morphological characteristics of Pinus halepensis Mill. somatic plantlets developed from ECLs maturated in a culture medium supplemented with different carbohydrate sources.
Free Polyamine Content Determination
The addition of different combinations of amino acids in the maturation medium did not provoke statistically significant differences in the ses germination of P. radiata, as well as no statistically significant differences were observed for the interaction between amino acid mixtures and the amounts of PAs (Table 9). However, the only statistically significant differences were found when the content of individual Put, Cad, Spd, and Spm was analyzed (Table 9). Conversely, total free PAs and the ratio Put/(Spd + Spm) did not show significant differences (Table 9 and Figures 12A,B). A higher amount of total free PAs was observed, with means ranging from 300.09 to 368.80 mmolμg–1 (Figure 12A). Within the total Free PAs, Cad accounted for half the amount of the total quantity followed by Spd (Figure 12C). However, the ses germinated from P. radiata had three times less Spm when compared with the other PAs previously mentioned, while the amount of Put was the lowest free PAs detected (Figure 12C). Plantlets of P. radiata contained large amounts of Cad and Spd, intermediate amounts of Spm, and low amounts of Put (Figure 12C).
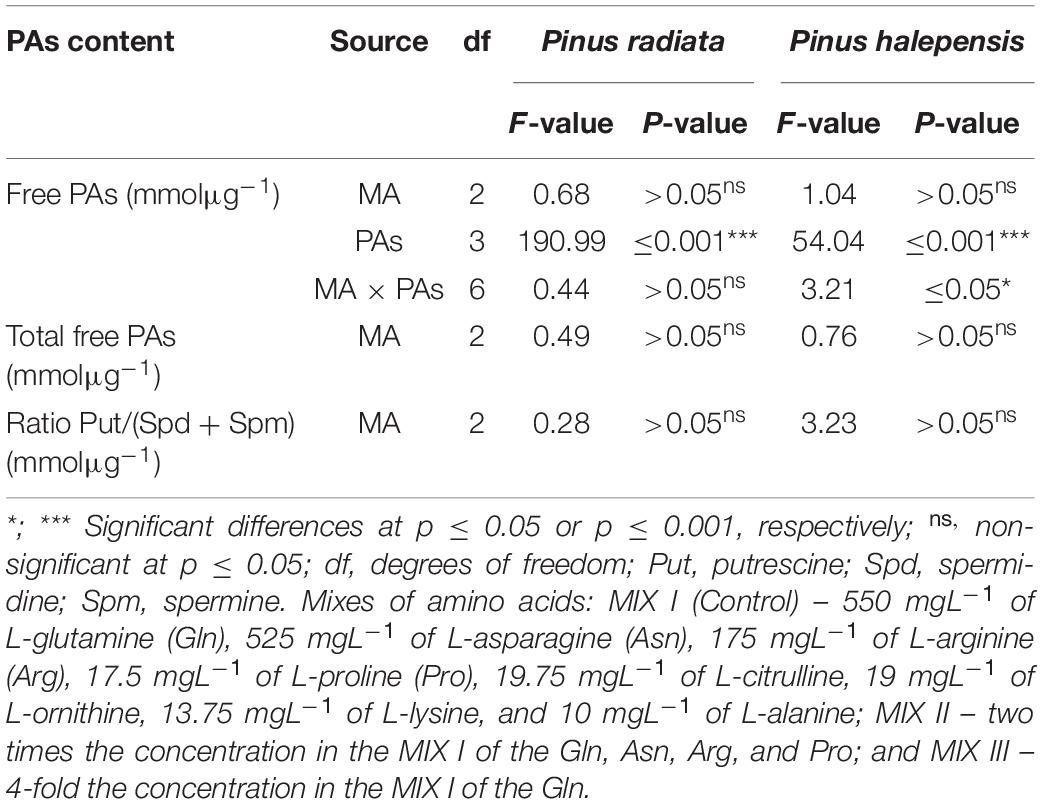
Table 9. Analysis of variance for the effect of the mixes of amino acids (MA) and the free individual polyamine levels (PAs); and for the effect of the mixes of MA on the total free Pas and the ratio Put/(Spd + Spm) in germinated somatic embryos of Pinus radiata D. Don and Pinus halepensis Mill.
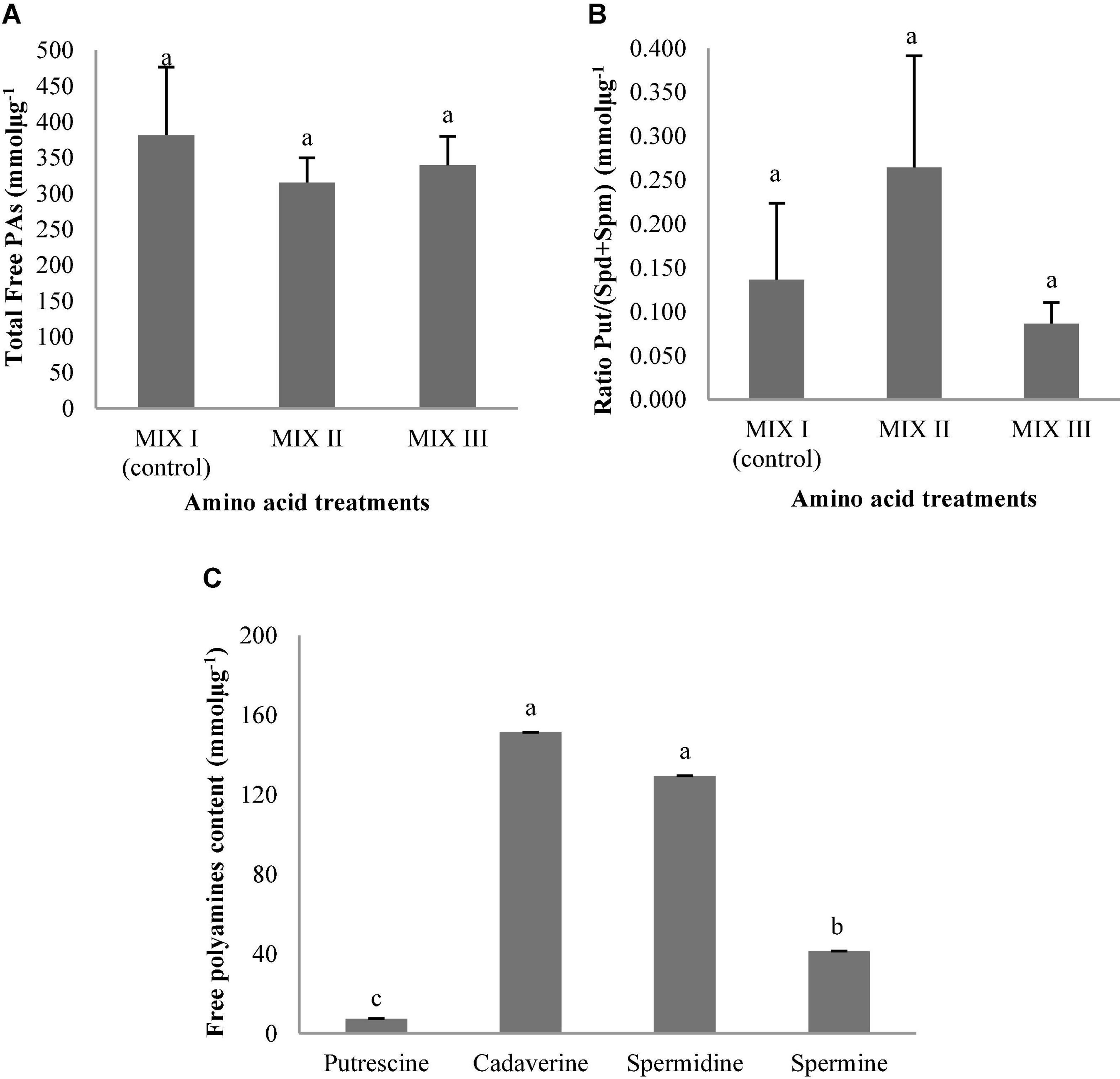
Figure 12. Polyamine (PA) levels (mmolμg–1) in germinated somatic embryos of Pinus radiata D. Don obtained from somatic embryos maturated in media supplemented with different mixes of amino acids. Total Free PAs (A); the ratio Put/(Spd + Spm) (B); and the individual free PAs (C). Bars indicate standard errors. Significant differences at p ≤ 0.05 are indicated by different letters. MIX I (Control) – 550 mgL–1 of L-glutamine (Gln), 525 mgL–1 of L-asparagine (Asn), 175 mgL–1 of L-arginine (Arg), 17.5 mgL–1 of L-proline (Pro), 19.75 mgL–1 of L-citrulline, 19 mgL–1 of L-ornithine, 13.75 mgL–1 of L-lysine, and 10 mgL–1 of L-alanine; MIX II – two times the concentration in the MIX I of the Gln, Asn, Arg, and Pro; and MIX III – 4-fold the concentration in the MIX I of the Gln.
Statistically significant differences were observed in PAs and the interaction between the amino acid mixtures and free PAs in germinated ses of P. halepensis (Table 9). However, no statistically significant differences were observed for the total free PAs and the ratio Put/(Spd + Spm) (Table 9 and Figures 13A,B). The highest concentrations of total free PAs were observed in ses from MIX II treatment (Figure 13A). The amounts of Cad, Spd, and Spm did not change during the germination of ses obtained from maturation media supplemented with different combinations of amino acids (Figure 13C). The level of these three PAs exhibited a slight increase in MIX I compared to the other treatments (Figure 13C). In this case, the amount of Spd increased slightly, followed by Cad (Figure 13C). In contrast, the Put concentration exhibited a considerable significant decrease in MIX I and MIX III (Figure 13C). Exogenous application of amino acids of MIX II in the maturation medium promoted similar amounts of four Pas analyzed in the germinated ses (Figure 13C).
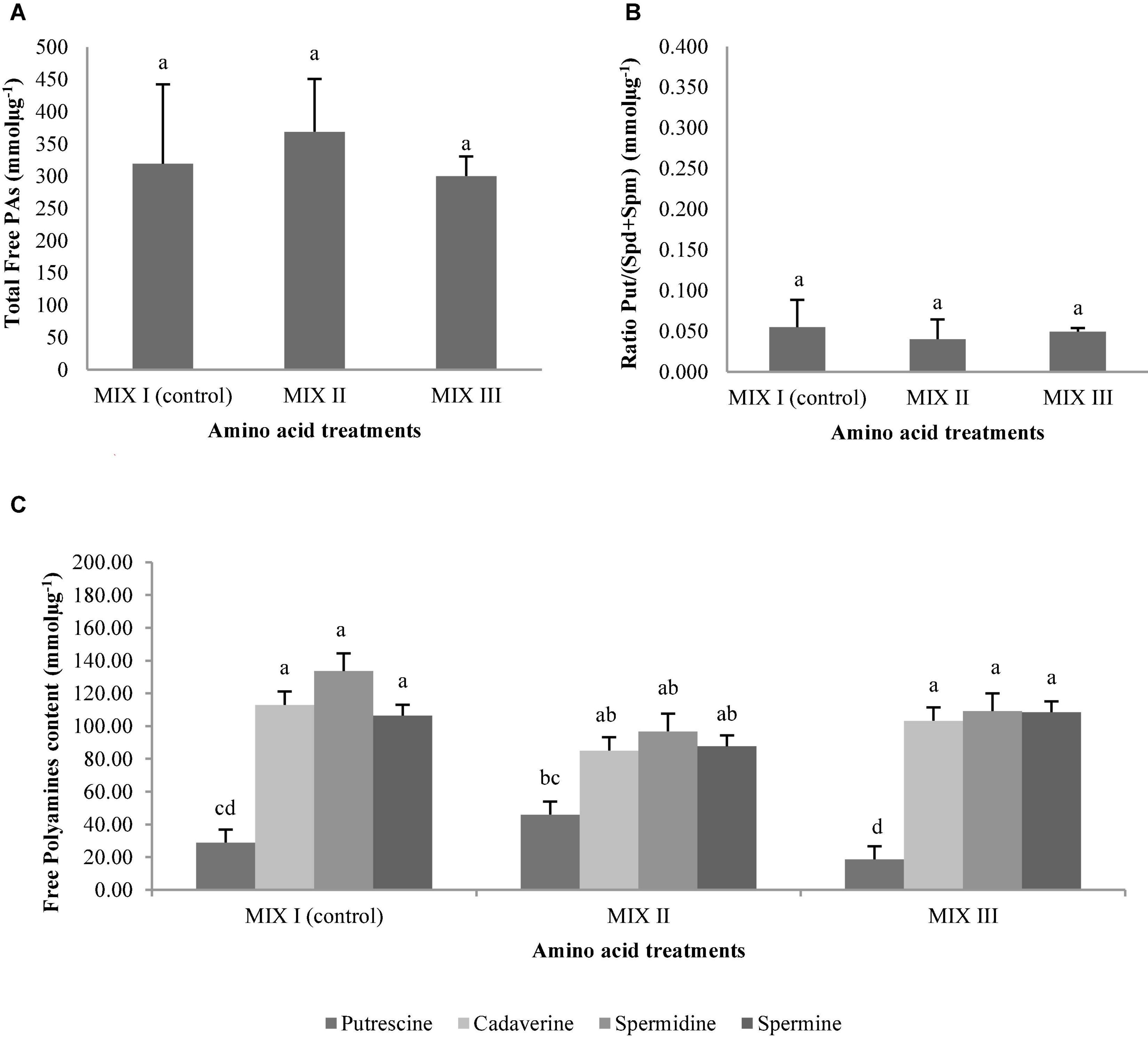
Figure 13. Polyamine (PAs) levels (mmolμg–1) in germinated somatic embryos of Pinus halepensis Mill. obtained from somatic embryos maturated in media supplemented with different mixes of amino acids. Total Free PAs (A); the ratio Put/(Spd + Spm) (B); and the individual free PAs (C). Bars indicate standard errors. Significant differences at p ≤ 0.05 are indicated by different letters. MIX I (Control) – 550 mgL–1 of L-glutamine (Gln), 525 mgL–1 of L-asparagine (Asn), 175 mgL–1 of L-arginine (Arg), 17.5 mgL–1 of L-proline (Pro), 19.75 mgL–1 of L-citrulline, 19 mgL–1 of L-ornithine, 13.75 mgL–1 of L-lysine, and 10 mgL–1 of L-alanine; MIX II – two times the concentration in the MIX I of the Gln, Asn, Arg, and Pro; and MIX III – 4-fold the concentration in the MIX I of the Gln.
For P. halepensis, statistically significant differences were observed for the different sources of carbohydrates, PAs, the interaction between carbohydrate sources, and carbohydrate concentrations and the triple interaction carbohydrate sources, carbohydrate concentrations, and PAs (Table 10).
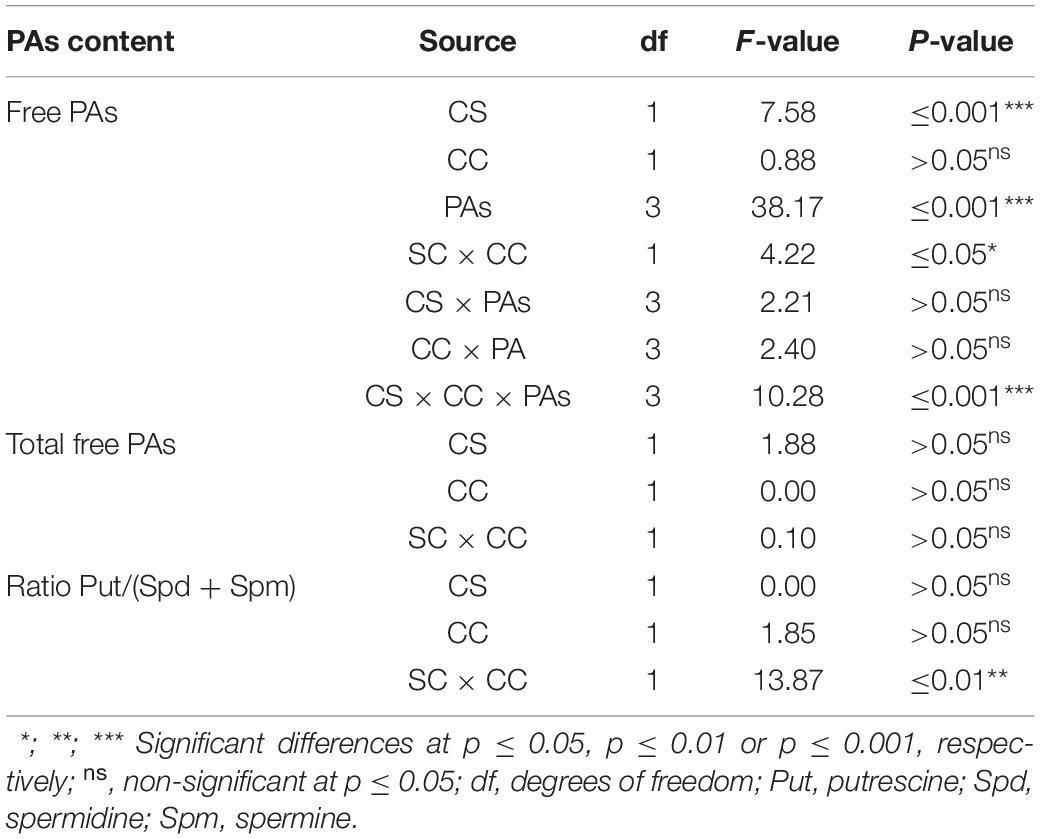
Table 10. Analysis of variance for the effect of the different carbohydrates sources (CS) and concentration (CC), and the free polyamine levels (PAs) (mmolμg–1); and for the effect of the different carbohydrate sources (CS) and concentration (CC) in the total free polyamine (PAs) (mmolμg–1) and the ratio Put/(Spd + Spm) in germinated somatic embryos of Pinus halepensis Mill.
The total free PAs were not affected by the carbohydrate sources, carbohydrate concentrations, or the interaction between these factors (Table 10 and Figure 14A). On the contrary, the ratio Put/(Spd + Spm) was affected by the interaction carbohydrate sources × carbohydrate concentration (Table 10). The P. halepensis germinated ses maturated in media with 350 suc or 175 mal showed significantly higher Put/(Spd + Spm) ratios than those obtained in a medium with the lowest maltose concentration (Figure 14B). Additionally, an intermediate value for Put/(Spd + Spm) ratios was observed in the germinated ses maturated in control conditions (175 suc) (Figure 14B). Germinated ses coming from maturation media with different carbohydrate sources and concentrations showed statistical higher values of all PAs, except Put (Figure 14C). In this case, levels of Put presented a marked decrease in germinated ses maturated in maturation media 350 mal when compared with the germinated ses maturated in maturation media 175 mal and 350 suc (Figure 14C). Cad, Spd, and Spm detected in germinated ses maturated in different carbohydrate sources and concentrations presented a similar behavior, but with an increase in the Cad amount in the germinated ses maturated in maturation media with 350 suc (Figure 14C).
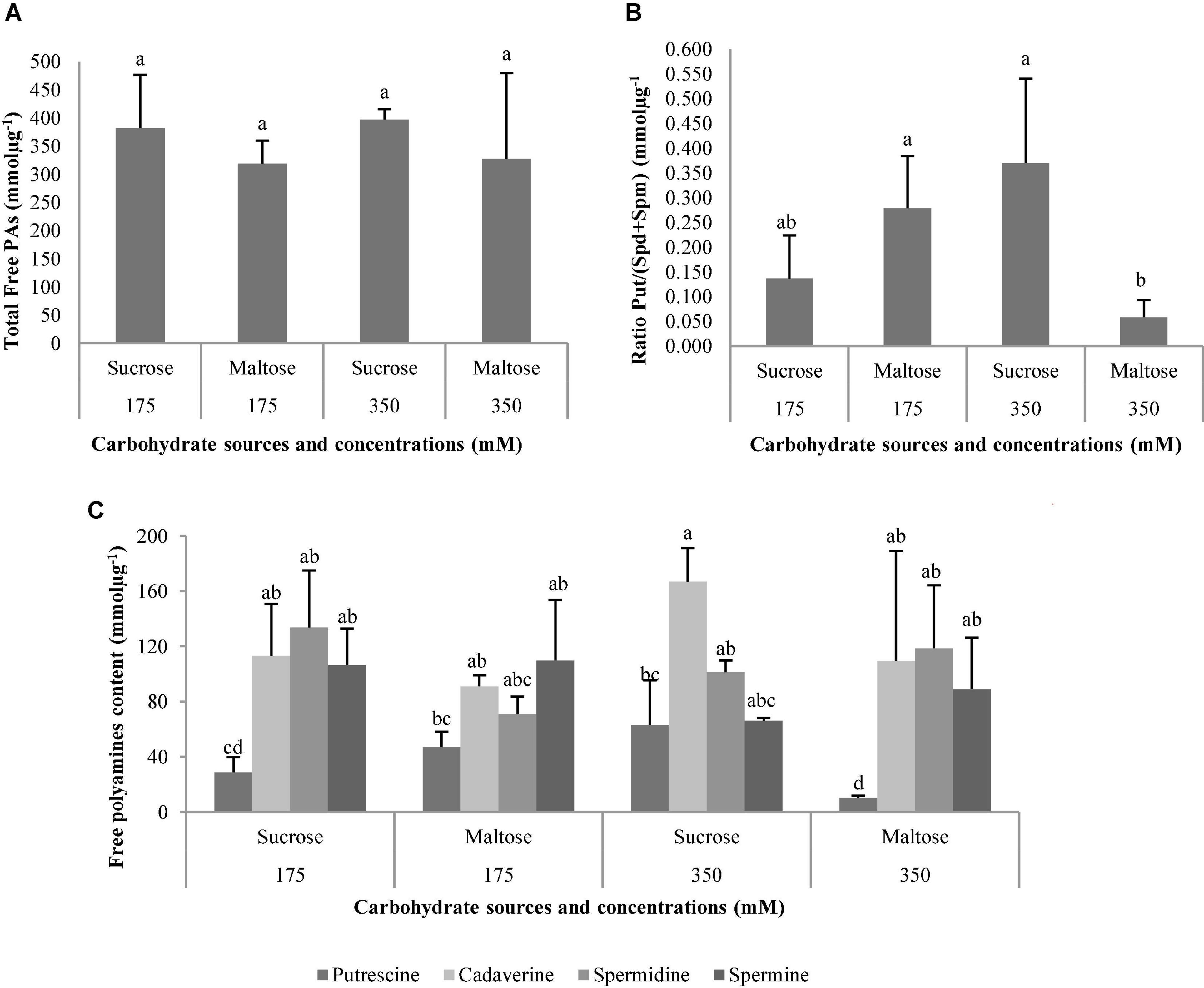
Figure 14. Polyamine (PAs) levels (mmolμg–1) in plantlets of Pinus halepensis Mill. obtained from germinated somatic embryos maturated in media supplemented with different carbohydrate sources and concentrations. Total Free Pas (A); the ratio Put/(Spd + Spm) (B); ant the Free Pas (C). Bars indicate standard errors. Significant differences at p ≤ 0.05 are indicated by different letters.
Discussion
Different amino acid or carbohydrate combinations significantly affected the osmolality of the maturation culture medium; it is common in the conifers SE process to restrict the water availability of the medium (increasing gellan gum concentration), modifying the osmolality in order to cause a shift in the developmental program of EMs and promote the formation of ses (Kvaalen and Johnsen, 2008; Garcia-Mendiguren et al., 2016). In our experiments, the best environment to achieve proper conversion of ses in plantlets was observed in maturation media with osmolalities between 277 (175 mM of sucrose) and 294 mosmkg–1 water (MIX II) for P. radiata and between 226 (175 mM of maltose) and 272 mosmkg–1 water (MIX III) for P. halepensis. However, and in agreement with Montalbán et al. (2010), who obtained good results at osmolalities around 270 mosmkg–1 water, the medium osmolality alone did not explain the results obtained because, in other media with similar osmolalities, such good results were not obtained in both species.
In our work, the highest osmolalities (above 400 mosmkg–1 water) did not increase the maturation success; in contrast, in Pseudotsuga menziesii (Mirb.) Franco raising medium osmolality to 450 mosmkg–1 water provoked an improvement in the vigor and morphology of ses developed (Gupta and Pullman, 1991). In this sense, the improvement in the number of ses in P. radiata and in Cryptomeria japonica D. Don was obtained in lower water availability in maturation media caused by 10 gL–1 of gellan gum and 17.5% of polyethylene glycol (PEG), respectively (Moncaleán et al., 2018; Maruyama et al., 2021). However, in Pinus taeda L., the maturation medium with 13% of PEG 8,000 and the osmolality ranging from 227 to 233 mmolkg–1 allowed an increase in the number of ses (Pullman et al., 2003).
When the size of the P. radiata somatic embryos was analyzed, we found that the maturation with MIX I (550 mgL–1 of Gln, 525 mgL–1 of Asn, 175 mgL–1 of L-Arg, 17.5 mgL–1 of Pro, 19.75 mgL–1 of L-citrulline, 19 mgL–1 of L-ornithine, 13.75 mgL–1 of L-lysine, and 10 mgL–1 of L-alanine) promoted the formation of smaller ses, showing lower acclimatization success months later (Figure 4B). In contrast, germinated ses maturated on MIX II (1,100 mgL–1 of Gln, 1,050 mgL–1 of Asn, 350 mgL–1 of L-Arg, 35 mgL–1 of Pro, 19.75 mgL–1 of L-citrulline, 19 mgL–1 of L-ornithine, 13.75 mgL–1 of L-lysine, and 10 mgL–1 of L-alanine) promoted larger embryos, with a larger stem diameter and an increase in the number of roots in the germinated ses, improving the acclimatization to ex vitro conditions of this species (Figure 4B). Similar to our results with P. radiata, Afele and Saxena (1995) reported an increase in the size of the mature somatic embryos and plantlet development of Picea pungens Engelm. in the culture medium supplemented with Asn. The combination of several amino acids (Gln, Asn, Arg, L-citrulline, L-ornithine, L-lysine, L-alanine, and Pro) was also beneficial in C. japonica SE, and the increase of concentration of several amino acids (2 gL–1 of Gln, 1 gL–1 of Asn, and 0.5 gL–1 of Arg) was essential to improve an efficient maturation of ses (>1,000 sesg–1 fresh weight) (Maruyama et al., 2021). In addition, the inclusion of a mixture of amino acid solution with 17 amino acids (Gln, alanine, Pro, lysine, glycine, Arg, leucine, phenylalanine, serine, isoleucine, valine, histidine, threonine, tyrosine, Asn, tryptophan, and cysteine) in the maturation medium of P. patula tended to increase the production of ses and the later conversion of ses in plantlets (Malabadi and Van Staden, 2005). P. halepensis ses developed in culture media with MIX III (2,200 mgL–1 of Gln, 525 mgL–1 of Asn, 175 mgL–1 of L-Arg, 17.5 mgL–1 of Pro, 19.75 mgL–1 of L-citrulline, 19 mgL–1 of L-ornithine, 13.75 mgL–1 of L-lysine, and 10 mgL–1 of L-alanine) were smaller and showed better germination rates (Figure 4C). These results are in agreement with those found in Pinus strobus L. where an increase in the concentration of Gln in the maturation medium for an embryogenic line resulted in an increase in the number of ses (Garin et al., 2000). Our findings show the important role of Gln in the maturation of P. halepensis, enhancing the germination of ses obtained from the maturation medium with a 4-fold increase in this amino acid concentration. This result coincides with some authors who report the use of Gln as one of the main sources of organic nitrogen for SE in Plinia peruviana Poir. Govaerts, Pinus koraiensis Siebold, and Zucc. and Viola canescens Wall. Ex. Roxb. (Silveira et al., 2020; Gao et al., 2021; Khajuria et al., 2021). In agreement with the improvements observed in the germination of P. halepensis ses, it was reported that the Gln also influenced the growth of pro-embryogenic masses in Picea abies L. Karst. (Carlsson et al., 2017) and P. peruviana (Silveira et al., 2020), increasing production of ses and consequent conversion to viable plants in date palm (El-Dawayati et al., 2018), as well as induction of secondary ses in Quercus suber L. (Rahmouni et al., 2020).
In relation to the carbohydrate source in a maturation medium, our results showed a different response in P. radiata and P. halepensis. In this sense, production, germination, and acclimatization of P. radiata ses were better in the presence of sucrose than in maltose in agreement with results obtained in Pinus pinaster Ait. (Ramarosandratana et al., 2001; Klimaszewska et al., 2007). Although, in our results, we did not see an improvement in the NNE at high carbohydrate concentrations in P. radiata, in P. strobus, the increase in its concentration (350 mM) produced a high amount of ses (Garin et al., 2000). Unlike P. radiata, the presence of 175 mM of maltose in P. halepensis maturation media promoted an increase in the size of ses, with a significant improvement in their germination rates. In this sense, plantlets obtained from P. halepensis ses developed in maturation media with 175 mM of maltose had an increase in the aerial part, and number of secondary roots and 100% of acclimatization was observed. Our results for P. halepensis are in agreement with those found in other Pinus species such as P. patula (Malabadi and Van Staden, 2005), Pinus thunbergii Parl. (Sun et al., 2021), and Pinus elliottii Engelm. (Yang et al., 2020).
Scott et al. (1995), studying the metabolism of maltose and sucrose in the embryogenesis of microspores isolated from Hordeum vulgare L., reported a higher assimilation of carbon in the culture medium, containing sucrose than in those containing maltose, probably because sucrose is well assimilated in both gymnosperms and angiosperms (Ameri et al., 2020; De Sousa et al., 2020; Peng et al., 2020; Shirin et al., 2020). However, different authors have shown controversial results; in this sense, Scott et al. (1995) associated the induction of embryogenesis with lower rates of substrate accumulation obtained from culture media containing maltose, and Carrion Pereira et al. (2019) described maltose as more efficient than sucrose to regenerate Urochloa brizantha cv. “Marandu” plants. Even when there are no reports describing morphological characteristics of ses with the concentration of maltose besides ours for P. halepensis, an increase in the production of the number of ses in Pinus massoniana Lamb. and P. elliottii has been reported (Yang et al., 2020; Xia et al., 2021). Similar to our results for P. halepensis, Salaj et al. (2019) reported that the presence of maltose in the maturation medium was essential for the formation ses with a consequent conversion of P. nigra plantlets.
In addition to morphological characteristics, the changes in the maturation medium also affected the PAs contents in germinated ses. PAs are extremely important for plants and are related to abiotic and biotic stress tolerance (Zou et al., 2021). Also, the Pas vary between species and the developmental stages of SE (Minocha et al., 1999; Silveira et al., 2004; Kuznetsov and Shevyakova, 2007; de Oliveira et al., 2020; Botini et al., 2021). In our work, the amount of PAs did not change with different treatments assayed in P. radiata. On the contrary, we observed that the amount of Put was more affected by the different treatments during the maturation stage than other Pas in P. halepensis. Moreover, this is the first report about the presence of endogenous Cad in Pinus spp. In this sense, concentrations of Cad were observed in the germinated ses obtained in both species and regardless of the treatments assayed. Cad is a lysine decomposition product, rare in plants, and associated with the accumulation of other Pas (Liu et al., 2014; Jancewicz et al., 2016). In our studies, the presence of Cad could not be associated with worse acclimatization, although, in Pinus sylvestris L., the root formation decreased in the presence of exogenous Cad (Niemi et al., 2002). In Arabidopsis thaliana L. Heynh., the presence of exogenous Cad was associated with the alterations in the morphological characteristics that modulate plant development, as well as environmental stress responses (Liu et al., 2014). In addition, in the same species, Cad inhibited primary root growth, and it was related to skewing, waving, and lateral root formation (Strohm et al., 2015). Moreover, it is possible to study the relationship of the Cad content with future stress experiments in Pinus, knowing that the Cad can improve the tolerance of abiotic stress in several species with an overexpression of the genes responsible for stress tolerance (Rajpal and Tomar, 2020).
Furthermore, differences between PAs contents were reported as important in SE. The reduction in Put content and the increase in other PAs (Spd, Spm, and Cad) during SE enabled the correct development of ses; for this reason, those differences have been used as important biochemical parameters for the selection of cell lines with embryogenic potential in A. angustifolia and P. radiata (Minocha et al., 1999; Jo et al., 2014; de Oliveira et al., 2015). In this work, regardless of the treatment of amino acids mixture, we observed an increase of Cad in relation to Put and Spm, but not in relation to Spd in the germinated P. radiata ses. Also, levels of Put were the lowest of all PAs detected in germinated ses; a similar profile was reported by Mikula et al. (2021) in Cyathea delgadii Sternb. SE process in which profiles of Cad and Spd increased in relation to other PAs. In agreement with these results and in opposition to ours, Minocha et al. (1999) reported that the most abundant PAs during the development of zygotic and ses in P. radiata was Spd in relation to Put and Spm. In contrast to our results, in the maturation of Picea glauca (Moench) Voss, the amount of Put was higher than Spd (Kong et al., 1998).
In P. halepensis, in MIX I, there were higher amounts of Cad, Spd, and Spm than in the remaining combinations, although there were no significant differences. In this sense, Vicia faba L. was reported that foliar application with different concentrations of amino acids mixture [Arg (5.2–6.2%), Pro (2.23–3.5%), Tiroanine (3.05–3.56%), Aspartic acid (3.2–3.45%), Serine (3.76–4.49%), Glutamic acid (7.24–9.12%), Lysine (1.87–2.45%), Alanine (2.16–2.20%), Cysteine (1.87–2.45%), Valine (2.8–3.1%), Methionine (0.23–0.3%), Isoleucine (1.26–1.7%), Leucine (1.98–2.8%), Tyrosine (0.48–1.02%), Phenylalanine (1.03–1.78%), lysine (1.39–2.3%), and Histidine (0.42–0.9%)] improved the PAs contents in the plants grown under seawater salinity stress (Sadak and Abdelhamid, 2015). Similar levels of Put and the other PAs were observed in germinated ses of P. halepensis from a maturation medium with an increase of 350 mgL–1 of L-Arg content (MIX II). However, with 2,200 mgL–1 of Gln (MIX III) in the maturation medium, a decrease in Put was observed in the germinated ses for P. halepensis. Our results are in agreement with those reported by Nieves et al. (2008), which showed that the addition of Arg in the culture medium of Saccharum spp. is related to the changes in the amount of PAs, especially Put. The observed levels of Put in our results can be explained due to PAs derived from amino acids through decarboxylation, and the Put can be synthesized from the arginine (Bagni and Tassoni, 2001; de Oliveira et al., 2015). Furthermore, the Put is a direct substrate for Spd and Spm (Mustafavi et al., 2018). For example, in embryogenic masses of P. sylvestris, the highest detected PA was Put compared to Spd and Spm (Salo et al., 2016). High amounts of Put appeared both in embryogenic lines that produced ses and in those that did not produce ses, while Spd was directly associated with the embryogenic lines and was able to produce ses in P. sylvestris (Salo et al., 2016). In P. nigra, the increase in the total amounts of free PAs was related with a lower embryogenic potential (Noceda et al., 2009). Meanwhile, the Spm was crucial to regulate the stress responses in A. thaliana, Rosa damascene Mill., and Citrus reticulata L., among others (Yamaguchi et al., 2007; Shi et al., 2010; Hassan et al., 2018; Hasan et al., 2021).
Conclusion
An improvement in the germination of ses, conversion of ses in plantlets, and the acclimatization of plantlets were obtained in P. radiata and P. halepensis with alterations in the amino acid mixture or carbohydrate sources/concentrations in the maturation medium. Furthermore, changes in the maturation medium were not affected by the amount of PA levels in the ses of P. radiata but changed in P. halepensis. Cadaverine was detected in germinated ses of P. radiata and P. halepensis.
Data Availability Statement
The raw data supporting the conclusions of this article will be made available by the authors, without undue reservation.
Author Contributions
PM, IM, and AN conceived and planned the experiments. AN performed the experiments and wrote the manuscript. AC-O and AN carried out the statistical analyses for the amino acid supplementation experiment, and the carbohydrate supplementation experiment, and the characterization of the plantlets. AN, LP, FB, MG, and NS carried out the polyamines analysis. All authors provided critical feedback and helped shape the manuscript.
Funding
This research was funded by the MINECO (Spanish Government) project (AGL2016-76143-C4-3R), CYTED (P117RT0522), and MINECO (BES-2017-081249, “Ayudas para contratos predoctorales para la formación de doctores”). MULTIFOREVER [Project MULTIFOREVER is supported under the umbrella of ERA-NET co-founded through Forest Value via ANR (FR), FNR (DE), MINCyT (AR), MINECO-AEI (ES), MMM (FI), and VINNOVA (SE)]. Forest value has received funding from the European Union’s Horizon 2020 research and innovation programmed under agreement No. 773324.
Conflict of Interest
The authors declare that the research was conducted in the absence of any commercial or financial relationships that could be construed as a potential conflict of interest.
Publisher’s Note
All claims expressed in this article are solely those of the authors and do not necessarily represent those of their affiliated organizations, or those of the publisher, the editors and the reviewers. Any product that may be evaluated in this article, or claim that may be made by its manufacturer, is not guaranteed or endorsed by the publisher.
References
Afele, J. C., and Saxena, P. K. (1995). “Somatic embryogenesis in blue spruce (Picea pungens Engelmann),” in Somatic Embryogenesis in Woody Plants, eds S. M. Jain, P. K. Gupta, and R. J. Newton (New York: Springer), 99–109.
Aitken-Christie, J., Singh, A. P., and Davies, H. (1988). “Multiplication of meristematic tissue: a new tissue culture system for radiata pine,” in Genetic Manipulation of Woody Plants, eds J. W. Hanover and D. E. Keathley (New York: Springer), 413–432.
Alcazar, R., Bueno, M., and Tiburcio, A. (2020). Polyamines: small amines with large effects on plant abiotic stress tolerance. Cells 9:2373. doi: 10.3390/cells9112373
Ameri, A., Davarynejad, G., Moshtaghi, N., and Tehranifar, A. (2020). The Role of carbohydrates on the induction of somatic embryogenesis and the biochemical state of the embryogenic callus in Pyrus communis L. Cv. ‘Dar Gazi’. Erwerbs-Obstbau 62, 411–419. doi: 10.1007/s10341-020-00518-6
Bagni, N., and Tassoni, A. (2001). Biosynthesis, oxidation and conjugation of aliphatic polyamines in higher plants. Amino Acids 20, 301–317. doi: 10.1007/s007260170046
Bais, H., and Ravishankar, G. (2002). Role of polyamines in the ontogeny of plants and their biotechnological applications. Plant Cell Tissue Organ Cult. 69, 1–34.
Botini, N., Almeida, F., Cruz, K., Reis, R., Vale, E., Garcia, A., et al. (2021). Stage-specific protein regulation during somatic embryo development of Carica papaya L. ‘Golden’. Biochim. Biophys. Acta Proteins Proteom. 1869:140561. doi: 10.1016/j.bbapap.2020.140561
Bouchereau, A., Aziz, A., Larher, F., and Martin-Tanguy, J. (1999). Polyamines and environmental challenges: recent development. Plant Sci. 140, 103–125. doi: 10.1016/S0168-9452(98)00218-0
Carlsson, J., Svennerstam, H., Moritz, T., Egertsdotter, U., and Ganeteg, U. (2017). Nitrogen uptake and assimilation in proliferating embryogenic cultures of Norway spruce-Investigating the specific role of glutamine. PLoS One 12:e0181785. doi: 10.1371/journal.pone.0181785
Carrion Pereira, A. V., Midori Takamori, L., Harumi Yaguinuma, D., Mendonça de Oliveira, A., and Ferreira Ribas, A. (2019). Maltose in culture media improves the in vitro regeneration of Urochloa brizantha cv. Marandu plants. Biotecnol. Veg. 19, 205–213.
Castander-Olarieta, A., Montalban, I., Oliveira, E., Dell’Aversana, E., D’Amelia, L., Carillo, P., et al. (2019). Effect of thermal stress on tissue ultrastructure and metabolite profiles during initiation of radiata pine somatic embryogenesis. Front. Plant Sci. 9:2004. doi: 10.3389/fpls.2018.02004
Castander-Olarieta, A., Pereira, C., Sales, E., Meijon, M., Arrillaga, I., Canal, M., et al. (2020). Induction of Radiata Pine Somatic Embryogenesis at High Temperatures Provokes a Long-Term Decrease in DNA Methylation/Hydroxymethylation and Differential Expression of Stress-Related Genes. Plants-Basel 9:1762. doi: 10.3390/plants9121762
Dahrendorf, J., Clapham, D., and Egertsdotter, U. (2018). Analysis of nitrogen utilization capability during the proliferation and maturation phases of Norway spruce (Picea abies (L.) H.Karst.) Somatic Embryogenesis. Forests 9:288. doi: 10.3390/f9060288
de Oliveira, L., dos Santos, A., and Floh, E. (2020). Polyamine and amino acid profiles in immature Araucaria angustifolia seeds and their association with embryogenic culture establishment. Trees Struct. Funct. 34, 845–854. doi: 10.1007/s00468-019-01938-y
de Oliveira, L., Macedo, A., dos Santos, A., and Floh, E. (2015). “Polyamine levels, arginine and ornithine decarboxylase activity in embryogenic cultures of Araucaria angustifolia (Bert.) O. Kuntze,” in VIII International Symposium on In Vitro Culture and Horticultural Breeding 1083), eds J. M. Canhoto and S. I. Correia (Leuven: International Society for Horticultural Science), 419–425.
de Oliveira, L., Navarro, B., Cerruti, G., Elbl, P., Minocha, R., Minocha, S., et al. (2018). Polyamine- and amino acid-related metabolism: the roles of arginine and ornithine are associated with the embryogenic potential. Plant Cell Physiol. 59, 1084–1098. doi: 10.1093/pcp/pcy049
De Sousa, P. C. A., Souza, S. S. S. E., Meira, F. S., Meira, R. D. O., Gomes, H. T., Silva-Cardoso, I. M. D. A., et al. (2020). Somatic embryogenesis and plant regeneration in Piper aduncum L. In Vitro Cell. Dev. Biol. Plant 56, 618–633. doi: 10.1007/s11627-020-10110-y
do Nascimento, A., Barroso, P., do Nascimento, N., Goicoa, T., Ugarte, M., Montalban, I., et al. (2020). Pinus spp. somatic embryo conversion under high temperature: effect on the morphological and physiological characteristics of plantlets. Forests 11:1181. doi: 10.3390/f11111181
El-Dawayati, M. M., Ghazzawy, H. S., and Munir, M. (2018). Somatic embryogenesis enhancement of date palm cultivar Sewi using different types of polyamines and glutamine amino acid concentration under in-vitro solid and liquid media conditions. Int. J. Biosci. 12, 149–159.
Escandon, M., Valledor, L., Pascual, J., Pinto, G., Canal, M., and Meijon, M. (2017). System-wide analysis of short-term response to high temperature in Pinus radiata. J. Exp. Bot. 68, 3629–3641. doi: 10.1093/jxb/erx198
Farias-Soares, F., Steiner, N., Schmidt, E., Pereira, M., Rogge-Renner, G., Bouzon, Z., et al. (2014). The transition of proembryogenic masses to somatic embryos in Araucaria angustifolia (Bertol.) Kuntze is related to the endogenous contents of IAA, ABA and polyamines. Acta Physiol. Plant. 36, 1853–1865. doi: 10.1007/s11738-014-1560-6
Fuentes-Sepulveda, R., Garcia-Herrera, C., Vasco, D., Salinas-Lira, C., and Ananias, R. (2020). Thermal characterization of Pinus radiata wood vacuum-impregnated with octadecane. Energies 13:942. doi: 10.3390/en13040942
Gao, F., Peng, C., Wang, H., Shen, H., and Yang, L. (2021). Selection of culture conditions for callus induction and proliferation by somatic embryogenesis of Pinus koraiensis. J. For. Res. 32, 483–491. doi: 10.1007/s11676-020-01147-1
Garcia-Mendiguren, O., Montalban, I., Correia, S., Canhoto, J., and Moncalean, P. (2016). Different environmental conditions at initiation of radiata pine somatic embryogenesis determine the protein profile of somatic embryos. Plant Biotechnol. 33, 143–152. doi: 10.5511/plantbiotechnology.16.0520a
Garin, É, Bernier-Cardou, M., Isabel, N., Klimaszewska, K., and Plourde, A. (2000). Effect of sugars, amino acids, and culture technique on maturation of somatic embryos of Pinus strobuson medium with two gellan gum concentrations. Plant Cell Tissue Organ Cult. 62, 27–37. doi: 10.1023/A:1006402215457
Gazol, A., Ribas, M., Gutierrez, E., and Camarero, J. (2017). Aleppo pine forests from across Spain show drought-induced growth decline and partial recovery. Agric. For. Meteorol. 232, 186–194. doi: 10.1016/j.agrformet.2016.08.014
Gemperlova, L., Fischerova, L., Cvikrova, M., Mala, J., Vondrakova, Z., Martincova, O., et al. (2009). Polyamine profiles and biosynthesis in somatic embryo development and comparison of germinating somatic and zygotic embryos of Norway spruce. Tree Physiol. 29, 1287–1298. doi: 10.1093/treephys/tpp063
Gerdakaneh, M., Mozafari, A., Sioseh-mardah, A., and Sarabi, B. (2011). Effects of different amino acids on somatic embryogenesis of strawberry (Fragaria x ananassa Duch.). Acta Physiol. Plant. 33, 1847–1852. doi: 10.1007/s11738-011-0725-9
Gupta, P., Pullman, G., Timmis, R., Kreitinger, M., Carlson, W., Grob, J., et al. (1993). Forestry in the 21st century. Nat. Biotechnol. 11, 454–459. doi: 10.1038/nbt0493-454
Gupta, P. K., and Durzan, D. J. (1985). Shoot multiplication from mature trees of Douglas-fir (Pseudotsuga menziesii) and sugar pine (Pinus lambertiana). Plant Cell Rep. 4, 177–179. doi: 10.1007/BF00269282
Gupta, P. K., and Pullman, G. S. (1991). Method for Reproducing Coniferous Plants By Somatic Embryogenesis Using Abscisic Acid And Osmotic Potential Variation. Alexandria: United States Patent and Trademark Office.
Hasan, M., Skalicky, M., Jahan, M., Hossain, M., Anwar, Z., Nie, Z., et al. (2021). Spermine: its emerging role in regulating drought stress responses in plants. Cells 10:261. doi: 10.3390/cells10020261
Hassan, F., Ali, E., and Alamer, K. (2018). Exogenous application of polyamines alleviates water stress-induced oxidative stress of Rosa damascena Miller var. trigintipetala Dieck. S. Afr. J. Bot. 116, 96–102. doi: 10.1016/j.sajb.2018.02.399
Huang, X., Li, X., Li, Y., and Huang, L. (2001). The effect of AOA on ethylene and polyamine metabolism during early phases of somatic embryogenesis in Medicago sativa. Physiol. Plant. 113, 424–429. doi: 10.1034/j.1399-3054.2001.1130317.x
Jancewicz, A., Gibbs, N., and Masson, P. (2016). Cadaverine’s functional role in plant development and environmental response. Front. Plant Sci. 7:870. doi: 10.3389/fpls.2016.00870
Jimenez, V. (2005). Involvement of plant hormones and plant growth regulators on in vitro somatic embryogenesis. Plant Growth Regul. 47, 91–110. doi: 10.1007/s10725-005-3478-x
Jin, L., Yarra, R., Zhou, L., Zhao, Z., and Cao, H. (2020). miRNAs as key regulators via targeting the phytohormone signaling pathways during somatic embryogenesis of plants. 3 Biotech. 10:495. doi: 10.1007/s13205-020-02487-9
Jo, L., Dos Santos, A., Bueno, C., Barbosa, H., and Floh, E. (2014). Proteomic analysis and polyamines, ethylene and reactive oxygen species levels of Araucaria angustifolia (Brazilian pine) embryogenic cultures with different embryogenic potential. Tree Physiol. 34, 94–104. doi: 10.1093/treephys/tpt102
Khajuria, A., Hano, C., and Bisht, N. (2021). Somatic embryogenesis and plant regeneration in Viola canescens Wall. Ex. Roxb.: an endangered himalayan herb. Plants-Basel 10:761. doi: 10.3390/plants10040761
Kielkowska, A., and Adamus, A. (2021). Exogenously applied polyamines reduce reactive oxygen species, enhancing cell division and the shoot regeneration from Brassica oleracea L. var. capitata protoplasts. Agron. Basel 11:735. doi: 10.3390/agronomy11040735
Klimaszewska, K., Trontin, J.-F., Becwar, M. R., Devillard, C., Park, Y.-S., and Lelu-Walter, M.-A. (2007). Recent progress in somatic embryogenesis of four Pinus spp. Tree For. Sci. Biotechnol. 1, 11–25.
Kong, L., Attree, S., and Fowke, L. (1998). Effects of polyethylene glycol and methylglyoxal bis(guanylhydrazone) on endogenous polyamine levels and somatic embryo maturation in white spruce (Picea glauca). Plant Sci. 133, 211–220. doi: 10.1016/S0168-9452(98)00040-5
Kube, M., Drazna, N., Konradova, H., and Lipavska, H. (2014). Robust carbohydrate dynamics based on sucrose resynthesis in developing Norway spruce somatic embryos at variable sugar supply. In Vitro Cell. Dev. Biol. Plant 50, 45–57. doi: 10.1007/s11627-013-9589-6
Kuznetsov, V. V., and Shevyakova, N. I. (2007). Polyamines and stress tolerance of plants. Plant Stress 1, 50–71.
Kvaalen, H., and Johnsen, O. (2008). Timing of bud set in Picea abies is regulated by a memory of temperature during zygotic and somatic embryogenesis. New Phytologist 177, 49–59. doi: 10.1111/j.1469-8137.2007.02222.x
Liu, T., Dobashi, H., Kim, D., Sagor, G., Niitsu, M., Berberich, T., et al. (2014). Arabidopsis mutant plants with diverse defects in polyamine metabolism show unequal sensitivity to exogenous cadaverine probably based on their spermine content. Physiol. Mol. Biol. Plants 20, 151–159. doi: 10.1007/s12298-014-0227-5
Malabadi, R., and Van Staden, J. (2005). Role of antioxidants and amino acids on somatic embryogenesis of Pinus patula. In Vitro Cell. Dev. Biol. Plant 41, 181–186. doi: 10.1079/IVP2004623
Manrique-Alba, A., Begueria, S., Molina, A., Gonzalez-Sanchis, M., Tomas-Burguera, M., del Campo, A., et al. (2020). Long-term thinning effects on tree growth, drought response and water use ef ficiency at two Aleppo pine plantations in Spain. Sci. Total Environ. 728:138536. doi: 10.1016/j.scitotenv.2020.138536
Maruyama, T. E., Ueno, S., Mori, H., Kaneeda, T., and Moriguchi, Y. (2021). Factors influencing somatic embryo maturation in Sugi (Japanese Cedar, Cryptomeria japonica (Thunb. ex Lf) D. Don). Plants 10:874.
Mikula, A., Tomaszewicz, W., Dziurka, M., Kazmierczak, A., Grzyb, M., Sobczak, M., et al. (2021). The Origin of the Cyathea delgadii Sternb. somatic embryos is determined by the developmental state of donor tissue and mutual balance of selected metabolites. Cells 10:1388. doi: 10.3390/cells10061388
Minocha, R., Majumdar, R., and Minocha, S. (2014). Polyamines and abiotic stress in plants: a complex relationship. Front. Plant Sci. 5:175. doi: 10.3389/fpls.2014.00175
Minocha, R., Minocha, S., and Long, S. (2004). Polyamines and their biosynthetic enzymes during somatic embryo development in red spruce (Picea rubens Sarg.). In Vitro Cell. Dev. Biol. Plant 40, 572–580. doi: 10.1079/IVP2004569
Minocha, R., Smith, D., Reeves, C., Steele, K., and Minocha, S. (1999). Polyamine levels during the development of zygotic and somatic embryos of Pinus radiata. Physiol. Plant. 105, 155–164. doi: 10.1034/j.1399-3054.1999.105123.x
Moncaleán, P., Garcia-Mendiguren, O., Novak, O., Strnad, M., Goicoa, T., Ugarte, M., et al. (2018). Temperature and water availability during maturation affect the cytokinins and auxins profile of radiata pine somatic embryos. Front. Plant Sci. 9:1898. doi: 10.3389/fpls.2018.01898
Montalbán, I. A., De Diego, N., and Moncaleán, P. (2010). Bottlenecks in Pinus radiata somatic embryogenesis: improving maturation and germination. Trees 24, 1061–1071. doi: 10.1007/s00468-010-0477-y
Montalbán, I. A., De Diego, N., and Moncaleán, P. (2012). Enhancing initiation and proliferation in radiata pine (Pinus radiata D. Don) somatic embryogenesis through seed family screening, zygotic embryo staging and media adjustments. Acta Physiol. Plant. 34, 451–460. doi: 10.1007/s11738-011-0841-6
Montalbán, I. A., and Moncaleán, P. (2019). Rooting of Pinus radiata somatic embryos: factors involved in the success of the process. J. For. Res. 30, 65–71. doi: 10.1007/s11676-018-0618-5
Montalbán, I. A., Setién-Olarra, A., Hargreaves, C. L., and Moncaleán, P. (2013). Somatic embryogenesis in Pinus halepensis Mill.: an important ecological species from the Mediterranean forest. Trees 27, 1339–1351. doi: 10.1007/s00468-013-0882-0
Monteiro, M., Kevers, C., Dommes, J., and Gaspar, T. (2002). A specific role for spermidine in the initiation phase of somatic embryogenesis in Panax ginseng CA Meyer. Plant Cell Tissue Organ Cult. 68, 225–232. doi: 10.1023/A:1013950729576
Mustafavi, S., Badi, H., Sekara, A., Mehrafarin, A., Janda, T., Ghorbanpour, M., et al. (2018). Polyamines and their possible mechanisms involved in plant physiological processes and elicitation of secondary metabolites. Acta Physiol. Plant. 40:102. doi: 10.1007/s11738-018-2671-2
Niemi, K., Haggman, H., and Sarjala, T. (2002). Effects of exogenous diamines on the interaction between ectomycorrhizal fungi and adventitious root formation in Scots pine in vitro. Tree Physiol. 22, 373–381. doi: 10.1093/treephys/22.6.373
Nieves, N., Sagarra, F., Gonzalez, R., Lezcano, Y., Cid, M., Blanco, M., et al. (2008). Effect of exogenous arginine on sugarcane (Saccharum sp.) somatic embryogenesis, free polyamines and the contents of the soluble proteins and proline. Plant Cell Tissue Organ Cult. 95, 313–320. doi: 10.1007/s11240-008-9445-2
Noceda, C., Salaj, T., Perez, M., Viejo, M., Canal, M., Salaj, J., et al. (2009). DNA demethylation and decrease on free polyamines is associated with the embryogenic capacity of Pinus nigra Arn. cell culture. Trees-Struct. Funct. 23, 1285–1293. doi: 10.1007/s00468-009-0370-8
Peng, C., Gao, F., Wang, H., Shen, H., and Yang, L. (2020). Physiological and biochemical traits in Korean pine somatic embryogenesis. Forests 11:577. doi: 10.3390/f11050577
Pereira, C., Montalban, I., Garcia-Mendiguren, O., Goicoa, T., Ugarte, M., Correia, S., et al. (2016). Pinus halepensis somatic embryogenesis is affected by the physical and chemical conditions at the initial stages of the process. J. For. Res. 21, 143–150. doi: 10.1007/s10310-016-0524-7
Pereira, C., Montalban, I., Goicoa, T., Ugarte, M., Correia, S., Canhoto, J., et al. (2017). The effect of changing temperature and agar concentration at proliferation stage in the final success of Aleppo pine somatic embryogenesis. Forest Syst. 26:eSC05. doi: 10.5424/fs/2017263-11436
Pullman, G., and Bucalo, K. (2014). Pine somatic embryogenesis: analyses of seed tissue and medium to improve protocol development. New Forests 45, 353–377. doi: 10.1007/s11056-014-9407-y
Pullman, G., Johnson, S., Peter, G., Cairney, J., and Xu, N. (2003). Improving loblolly pine somatic embryo maturation: comparison of somatic and zygotic embryo morphology, germination, and gene expression. Plant Cell Rep. 21, 747–758. doi: 10.1007/s00299-003-0586-9
Quinto, L., Navarro-Cerrillo, R., Palacios-Rodriguez, G., Ruiz-Gomez, F., and Duque-Lazo, J. (2021). The current situation and future perspectives of Quercus ilex and Pinus halepensis afforestation on agricultural land in Spain under climate change scenarios. New Forests 52, 145–166. doi: 10.1007/s11056-020-09788-0
Quoirin, M., and Lepoivre, P. (1977). Etude de milieux adaptes aux cultures in vitro de Prunus. Acta Hortic. 78, 437–442.
Rahmouni, S., El Ansari, Z. N., Badoc, A., Martin, P., El Kbiach, M. L. B., and Lamarti, A. (2020). Effect of amino acids on secondary somatic embryogenesis of moroccan cork oak (Quercus suber L.) Tree. Am. J. Plant Sci. 11, 626–641.
Rajpal, C., and Tomar, P. C. (2020). Cadaverine: a potent modulator of plants against abiotic stresses: cadaverine: a potent modulator. J. Microbiol. Biotechnol. Food Sci. 10, 205–210. doi: 10.15414/jmbfs.2020.10.2.205-210
Ramarosandratana, A., Harvengt, L., Bouvet, A., Calvayrac, R., and Pâques, M. (2001). Effects of carbohydrate source, polyethylene glycol and gellan gum concentration on embryonal-suspensor mass (ESM) proliferation and maturation of maritime pine somatic embryos. In Vitro Cell. Dev. Biol. Plant 37, 29–34.
Sadak, M., and Abdelhamid, M. (2015). Influence of amino acids mixture application on some biochemical aspects, antioxidant enzymes and endogenous polyamines of Vicia faba plant grown under seawater salinity stress. Gesunde Pflanzen 67, 119–129. doi: 10.1007/s10343-015-0344-2
Salaj, T., Klubicova, K., Matusova, R., and Salaj, J. (2019). Somatic embryogenesis in selected conifer trees Pinus nigra Arn. and Abies Hybrids. Front. Plant Sci. 10:13. doi: 10.3389/fpls.2019.00013
Salo, H., Sarjala, T., Jokela, A., Haggman, H., and Vuosku, J. (2016). Moderate stress responses and specific changes in polyamine metabolism characterize Scots pine somatic embryogenesis. Tree Physiol. 36, 392–402. doi: 10.1093/treephys/tpv136
Satish, L., Rathinapriya, P., Ceasar, S., Rency, A., Pandian, S., Rameshkumar, R., et al. (2016). Effects of cefotaxime, amino acids and carbon source on somatic embryogenesis and plant regeneration in four Indian genotypes of foxtail millet (Setaria italica L.). In Vitro Cell. Dev. Biol. Plant 52, 140–153. doi: 10.1007/s11627-015-9724-7
Scott, P., Lyne, R., and Aprees, T. (1995). Metabolism of maltose and sucrose by microspores isolated from barley (Hordeum vulgare L.). Planta 197, 435–441.
Shi, J., Fu, X.-Z., Peng, T., Huang, X.-S., Fan, Q.-J., and Liu, J.-H. (2010). Spermine pretreatment confers dehydration tolerance of Citrusin vitro plants via modulation of antioxidative capacity and stomatal response. Tree Physiol. 30, 914–922. doi: 10.1093/treephys/tpq030
Shirin, F., Bhadrawale, D., Mishra, J., Sonkar, M., and Maravi, S. (2020). Evaluation of biochemical changes during different stages of somatic embryogenesis in a vulnerable timber treeDalbergia latifolia(Indian rosewood). In Vitro Cell. Dev. Biol. Plant 56, 894–902. doi: 10.1007/s11627-020-10099-4
Shoeb, F., Yadav, J., Bajaj, S., and Rajam, M. (2001). Polyamines as biomarkers for plant regeneration capacity: improvement of regeneration by modulation of polyamine metabolism in different genotypes of indica rice. Plant Sci. 160, 1229–1235. doi: 10.1016/S0168-9452(01)00375-2
Silveira, S. S., Sant Anna-Santos, B. F., Degenhardt-Goldbach, J., and Quoirin, M. (2020). Somatic embryogenesis from mature split seeds of jaboticaba (Plinia peruviana (Poir) Govaerts). Acta Sci. Agron. 42:e43798.
Silveira, V., Floh, E., Handro, W., and Guerra, M. (2004). Effect of plant growth regulators on the cellular growth and levels of intracellular protein, starch and polyamines in embryogenic suspension cultures of Pinus taeda. Plant Cell Tissue Organ Cult. 76, 53–60. doi: 10.1023/A:1025847515435
Smeekens, S., Ma, J., Hanson, J., and Rolland, F. (2010). Sugar signals and molecular networks controlling plant growth. Curr. Opin. Plant Biol. 13, 274–279. doi: 10.1016/j.pbi.2009.12.002
Strohm, A., Vaughn, L., and Masson, P. (2015). Natural variation in the expression of ORGANIC CATION TRANSPORTER 1 affects root length responses to cadaverine in Arabidopsis. J. Exp. Bot. 66, 853–862. doi: 10.1093/jxb/eru444
Sun, T., Wang, Y., Zhu, L., Liu, X., Wang, Q., and Ye, J. (2021). Evaluation of somatic embryo production during embryogenic tissue proliferation stage using morphology, maternal genotype, proliferation rate and tissue age of Pinus thunbergii Parl. J. For. Res. doi: 10.1007/s11676-021-01311-1 [Epub ahead of print].
Sundararajan, S., Sivakumar, H., Nayeem, S., Rajendran, V., Subiramani, S., and Ramalingam, S. (2021). Influence of exogenous polyamines on somatic embryogenesis and regeneration of fresh and long-term cultures of three elite indica rice cultivars. Cereal Res. Commun. 49, 245–253. doi: 10.1007/s42976-020-00098-x
Walter, C., Find, J. I., and Grace, L. J. (2005). “Somatic Embryogenesis and Genetic Transformation in Pinus radiata,” in Protocol for Somatic Embryogenesis in Woody Plants, eds S. M. Jain and P. K. Gupta (Dordrecht: Springer), 11–24.
Xia, X., Yang, F., Ke, X., Chen, Y., Ye, J., and Zhu, L. (2021). Somatic embryogenesis of masson pine (Pinus massoniana): initiation, maturation and genetic stability analysis at SSR loci. Plant Cell Tissue Organ Cult. 145, 667–677. doi: 10.1007/s11240-021-02036-z
Yamaguchi, K., Takahashi, Y., Berberich, T., Imai, A., Takahashi, T., Michael, A., et al. (2007). A protective role for the polyamine spermine against drought stress in Arabidopsis. Biochem. Biophys. Res. Commun. 352, 486–490. doi: 10.1016/j.bbrc.2006.11.041
Yang, F., Xia, X.-R., Ke, X., Ye, J., and Zhu, L.-H. (2020). Somatic embryogenesis in slash pine (Pinus elliottii Engelm): improving initiation of embryogenic tissues and maturation of somatic embryos. Plant Cell Tissue Organ Cult. 143, 159–171. doi: 10.1007/s11240-020-01905-3
Yaseen, M., Ahmad, T., Sablok, G., Standardi, A., and Hafiz, I. (2013). Review: role of carbon sources for in vitro plant growth and development. Mol. Biol. Rep. 40, 2837–2849. doi: 10.1007/s11033-012-2299-z
Keywords: Pinus halepensis, Pinus radiata, sugars, amino acids, osmolality
Citation: do Nascimento AMM, Polesi LG, Back FP, Steiner N, Guerra MP, Castander-Olarieta A, Moncaleán P and Montalbán IA (2021) The Chemical Environment at Maturation Stage in Pinus spp. Somatic Embryogenesis: Implications in the Polyamine Profile of Somatic Embryos and Morphological Characteristics of the Developed Plantlets. Front. Plant Sci. 12:771464. doi: 10.3389/fpls.2021.771464
Received: 06 September 2021; Accepted: 28 October 2021;
Published: 26 November 2021.
Edited by:
Jonny E. Scherwinski-Pereira, Brazilian Agricultural Research Corporation (EMBRAPA), BrazilReviewed by:
Rodrigo J. Hasbun, University of Concepción, ChilePedro Alfonso Sansberro, Instituto de Botánica del Nordeste (IBONE), Consejo Nacional de Investigaciones Científicas y Técnicas (CONICET), Argentina
Copyright © 2021 do Nascimento, Polesi, Back, Steiner, Guerra, Castander-Olarieta, Moncaleán and Montalbán. This is an open-access article distributed under the terms of the Creative Commons Attribution License (CC BY). The use, distribution or reproduction in other forums is permitted, provided the original author(s) and the copyright owner(s) are credited and that the original publication in this journal is cited, in accordance with accepted academic practice. No use, distribution or reproduction is permitted which does not comply with these terms.
*Correspondence: Paloma Moncaleán, cG1vbmNhbGVhbkBuZWlrZXIuZXVz; Itziar Aurora Montalbán, aW1vbnRhbGJhbkBuZWlrZXIuZXVz
†These authors have contributed equally to this work