- 1Irrigated Agriculture Research and Extension Center, Washington State University, Prosser, WA, United States
- 2Department of Plant Sciences, North Dakota State University, Fargo, ND, United States
- 3Grain Legume Genetics and Physiology Research Unit, USDA-ARS, Prosser, WA, United States
Bean common mosaic virus (BCMV) is a major disease in common bean (Phaseolus vulgaris L.). Host plant resistance is the most effective strategy to minimize crop damage against BCMV and the related Bean common mosaic necrosis virus (BCMNV). To facilitate breeding for resistance, we sought to identify candidate genes and develop markers for the bc-2 gene and the unknown gene with which it interacts. Genome-wide association study (GWAS) of the Durango Diversity Panel (DDP) identified a peak region for bc-2 on chromosome Pv11. Haplotype mapping narrowed the bc-2 genomic interval and identified Phvul.011G092700, a vacuolar protein-sorting 4 (Vps4) AAA+ ATPase endosomal sorting complexes required for transport (ESCRT) protein, as the bc-2 candidate gene. The race Durango Phvul.011G092700 gene model, bc-2[UI 111], contains a 10-kb deletion, while the race Mesoamerican bc-2[Robust] consists of a single nucleotide polymorphism (SNP) deletion. Each mutation introduces a premature stop codon, and they exhibit the same interaction with the pathogroups (PGs) tested. Phvul.005G125100, another Vps4 AAA+ ATPase ESCRT protein, was identified as the candidate gene for the new recessive bc-4 gene, and the recessive allele is likely an amino acid substitution in the microtubule interacting and transport (MIT) domain. The two Vps4 AAA+ ATPase ESCRT proteins exhibit high similarity to the Zym Cucsa.385040 candidate gene associated with recessive resistance to Zucchini yellow mosaic virus in cucumber. bc-2 alone has no resistance effect but, when combined with bc-4, provides resistance to BCMV (except PG-V) but not BCMNV, and, when combined with bc-ud, provides resistance to BCMV (except BCMV PG-VII) and BCMNV. So instead of different resistance alleles (i.e., bc-2 and bc-22), there is only bc-2 with a differential reaction based on whether it is combined with bc-4 or bc-ud, which are tightly linked in repulsion. The new tools and enhanced understanding of this host-virus pathogen interaction will facilitate breeding common beans for resistance to BCMV and BCMNV.
Introduction
Bean common mosaic virus (BCMV) and Bean common mosaic necrosis virus (BCMNV) are closely related positive-stranded RNA potyviruses that limit common bean (Phaseolus vulgaris L.) production worldwide. BCMV and BCMNV are transmitted by aphids, mechanically, or through infected seeds. Genetic resistance is the most effective means to control BCMV and BCMNV. For a comprehensive review of these viruses and their interactions with the common bean, refer to Worrall et al. (2015).
The long-standing host-pathogen interaction model developed by Drijfhout (1978) for these two viruses and common bean was based on six host recessive resistance alleles (i.e., bc-1 and bc-12 alleles, bc-2 and bc-22 alleles, bc-3, and bc-u) distributed across four loci and the dominant I gene. The presence of the bc-u “ubiquitous” gene was necessary for the expression of the other recessive resistance genes, in the absence of the I gene. Isolate diversity was classified into seven pathogroups (PGs), i.e., PG-I to PG-VII, based on interactions with 11 host groups (HGs), i.e., HG-1 to HG-11, which possessed different combinations of resistance genes. Recent adjustments to the model include as follows: (i) a new PG (PG-VIII) with a BCMV isolate that overcomes bc-3 gene in the HG-7 genotype IVT-7214 (Feng et al., 2015); (ii) a new HG-12 with I and bc-3 resistance genes (Larsen et al., 2005); (iii) only one resistance allele “bc-1” for the Bc-1 locus (Soler-Garzón et al., 2021); and (iv) absence of the bc-u “helper” gene in HG-2, -4, -5, and -7 where presence was expected. Soler-Garzón et al. (2021) used the new symbol “bc-ud” to designate the presence of bc-u in HG-3, -6, -10, and -11. They observed that bc-ud may “help” bc-22 to condition resistance to BCMV and BCMNV in genotypes assigned to HG-6 and HG-11. However, those interactions need to be validated, and a bc-u-like gene for bc-2 in genotypes assigned to HG-4 and HG-5 has not been identified.
Candidate genes were recently characterized for bc-1 (i.e., receptor-like protein kinases) on chromosome 3 (Pv03) and bc-ud (i.e., basic leucine zipper transcription factor protein) on Pv05 (Soler-Garzón et al., 2021). A eukaryotic translation initiation factor 4E protein (eIF4E) with a reported role in potyviral infection (Naderpour et al., 2010) was identified as the candidate gene for bc-3 on Pv06. The I gene on Pv02, which induces an immune response to BCMV or temperature-dependent hypersensitive response (HR) to select a few BCMV strains as well as temperature-independent HR to BCMNV infection, was associated with a cluster of genes encoding nucleotide-binding site-leucine-rich repeat (NBS-LRR) proteins (Vallejos et al., 2006). The Bc-2 locus and the unknown gene that interacts with bc-2 in HG-4 and HG-5 have been neither associated with candidate genes nor mapped yet, limiting the utilization of the bc-2 and bc-22 alleles in breeding for resistance to BCMV and BCMNV.
To facilitate breeding for resistance to BCMV and BCMNV and to gain a better understanding of the host-pathogen model, our objectives were to map, identify candidate genes, and develop markers for bc-2 and the unknown “helper” gene it interacts with, in HG-4 and HG-5 categorized lines.
Materials and Methods
Plant Materials
A total of 182 dry bean lines from the Durango Diversity Panel (DDP), described in a previous study by Soler-Garzón et al. (2021), were used in this study to map and target candidate genes for bc-2/bc-22 resistance alleles and the unknown gene that interacts with bc-2. The Othello/VAX 3 (OV3) F6:7 recombinant inbred line (RIL) population (n = 100) (Viteri et al., 2014) was used to narrow the bc-22 genomic interval. Othello pinto bean cultivar (NW-410/2/Victor/Aurora; Burke et al., 1995) possesses the bc-1/bc-22/bc-ud resistance genes (Soler-Garzón et al., 2021), which places it in HG-6. Othello is only susceptible to BCMV PG-VII strains such as US-6 and NL-4. VAX 3 is a germplasm release derived from a multiple-parent interspecific cross between common bean and tepary bean (Phaseolus acutifolius). VAX 3 has the I gene for resistance to BCMV (Singh et al., 2001).
Seven F2 populations were generated from crosses among DDP lines selected for presence vs. absence of the bc-2 allele based on phenotypic response to different PGs, as reported in the literature, or from inoculations conducted by us. Furthermore, two HG-4 navy bean cultivars, namely, Sanilac and Michelite-62 with bc-2, were crossed with Poncho (DDP-041), Beryl (DDP055), and Matterhorn (DDP-033), which lack bc-2 to generate six additional F2 populations segregating for the bc-2 gene originating from race Mesoamerica (Supplementary Table 1).
Phenotypic Evaluation
The DDP lines were evaluated for reaction to US-6 (PG-VII) BCMV strain (Silbernagel, 1969). DDP reactions to NL-8 (PG-III) and NL-3 (PG-VI) BCMNV strains were reported by Soler-Garzón et al. (2021). The OV3 RILs were evaluated for reaction to US-6, NL-8, and NL-3 strains and the BCMV US-4 (PG-IV) strain (Skotland and Burke, 1961) was used to inoculate a subset of OV3 RILs. The subsets of individual plants from F2 populations and F2:3 families (i.e., progeny lines) were evaluated for reaction to one or more strains, which included US-4 (PG-IV) and US-6 (PG-V11) BCMV strains as well as NL-8 (PG-III) and NL-3 (PG-VI) BCMNV strains.
All virus strains were maintained in the universal susceptible HG-1 differential cultivar “Sutter Pink,” with the youngest leaves with mosaic symptoms used as inoculum. All inoculations were conducted in the USDA-ARS greenhouses at Prosser, WA, United States, under controlled conditions (22–28°C temperature range and 14-h photoperiod using artificial lights as necessary). Test plants were sown in 9 cm3 pots (three plants maximum per pot) containing a commercial potting mix (Sun Gro Horticulture, Bellevue, WA, United States). About 10 days after planting, the two primary leaves of each plant were mechanically inoculated with the same strain using the procedure described by Drijfhout (1978).
Disease reactions were observed every week until 5 weeks post-inoculation (wpi). The symptoms for each plant, as reported by Soler-Garzón et al. (2021), were recorded as follows: NS = no apparent symptoms on inoculated leaves and no systemic symptoms; M = leaf curling and plant stunting with severe systemic chlorotic mosaic symptoms; mM = mild systemic chlorotic mosaic symptoms; dM = delayed severe systemic chlorotic mosaic symptoms observed 2–4 wpi; VN = restricted vein necrosis on inoculated leaves, no systemic symptoms; VN+ = restricted vein necrosis on inoculated leaves, with some small patches (10 mm2) of systemic restricted vein necrosis on upper trifoliolate leaves observed from 3 to 5 wpi; NLL = local necrotic pinpoint lesions on inoculated leaves, no systemic symptoms; TN = lethal systemic top necrosis by 7–10 days post-inoculation (dpi), resulting in plant death; and dTN = delayed top necrosis beginning >11 dpi, most often resulting in plant death. Host differentials with specific reactions to BCMV and BCMNV strains in parenthesis (US-4/US-6/NL-8/NL-3): HG-1, Sutter pink (M/M/M/M); HG-4, UI-34 (NS/NS/M/M); HG-6, Othello (NS/M/NS/NS); HG-9, Topcrop (NS/NS/VN/TN); HG-10, Beryl (NS/NS/VN/VN); and HG-9b, Jubila (TN/NS/VN/VN+) were included as controls.
DNA Extraction and Genotyping
Genomic DNA was extracted from 20 mg of leaf tissue from an individual plant for each DDP accession, check line, and HG differential cultivar using a QIAGEN DNeasy 96 Plant Kit (Hilden, Germany). For F2 and F3 plants and single plants of OV3 RILs, DNA was extracted from four-leaf disks (approximately 30 mm2) according to the alkaline extraction method described by Xin et al. (2003) with modifications (Soler-Garzón et al., 2021). A 1:7 dilution of the extracted DNA was placed in a 96-well plate with a final volume of 100 μl. Finally, 5 μl of diluted DNA template was used for PCR.
The DDP was genotyped, as reported by Soler-Garzón et al. (2021), with a filtered set of 1,269,044 biallelic single-nucleotide polymorphisms (SNPs) obtained by whole-genome resequencing. The linkage map for OV3, which was generated with polymorphic SNPs from the BARCBean6K_3 BeadChip assay (Song et al., 2015), was obtained from Viteri et al. (2014). The DDP and OV3 RILs were assayed for the I-, bc-1-, bc-3-, and bc-ud-linked SNP markers described by Soler-Garzón et al. (2021). The individual F2 plants from each population were similarly assayed with the same resistance gene-linked markers. Eventually, the abovementioned materials were assayed with the new putative markers for bc-2 and unknown gene (described below). Notably, 35 select F2:3 progenies from individual F2 plants, either fixed or heterozygous for one or more of the resistance gene-linked markers, were genotyped using this same set of SNP markers. Eight true-breeding F2:3 families were used as controls for each gene in a homozygous state, and the remaining 27 families had at least 1 gene in a heterozygous state (Supplementary Table 1). A total of 217 F2 plants and 1,345 F2:3 plants were assayed for the I, bc-1, bc-3, bc-ud, and new bc-2 and unknown gene, i.e., gene-linked SNP markers (Supplementary Table 2).
Genome-Wide Association Study Analysis
The phenotypic reactions to US-6, NL-8, and NL-3 strains and the genotypic SNP data for the DDP were integrated for the genome-wide association study using a multi-locus random-SNP-effect mixed linear model (mrMLM) described by Wang et al. (2016) and implemented in the “mrMLM” R package (Wen et al., 2018). A kinship matrix was generated using the efficient mixed-model association (EMMA) algorithm implemented in the Genome Association and Prediction Integrated Tool (GAPIT) R package (Lipka et al., 2012) with corrections for kinship and population structure. Five principal components (PCs) generated from GAPIT were included as covariates. The Bonferroni test was implemented to control the experiment-wise type I error rate at 0.05. The GWAS results were plotted using CMPlot v3.62 (Yin, 2016), and IntAssoPlot v3 (He et al., 2020) was used to represent regional-based marker-trait associations graphically.
Toward Candidate Gene Markers for bc-2 and Unknown Gene
Candidate genes located within the identified genomic intervals were obtained by perusing available scientific literature and aligning their sequences to the Andean G19833 v2.1 and Middle-American UI 111 v1.1 P. vulgaris reference genomes.1 Subsequently, exon sequences of each candidate gene were amplified across select genotypes using PCR primers designed with Primer3 software (Koressaar and Remm, 2007; Untergasser et al., 2012; Supplementary Table 3).
Two standard PCRs, for each sample, were replicated in a volume of 25 μl containing 1.8 mM MgCl2, 0.4 mM of deoxynucleoside triphosphate (dNTP) Mix (Promega™, Madison, WI, United States), 0.25 μM of each primer (forward and reverse), 25 ng of genomic DNA, and 1 unit Taq DNA polymerase (Promega), in 1× PCR buffer (Promega), under the following amplification conditions: 2 min at 95°C, followed by 38 cycles at 94°C for 20 s, specific annealing temperature for each primer set for 30 s and 72°C for 90 s, and a final extension at 72°C for 5 min. All amplifications were performed in a PCR Eppendorf Mastercycler (Eppendorf AG, Hamburg, Germany). PCR fragments were visualized by gel electrophoresis on 2% (w + v) agarose and then purified and Sanger sequenced by Eurofins MWG Operon (Louisville, KY, United States). Sequence trimming, alignment, and polymorphism discovery were performed with Geneious 9.1.2 software (Kearse et al., 2012).
Candidate Single-Nucleotide Polymorphism Marker Verification
For each polymorphic variant identified in coding regions of the sequenced candidate genes, a set of allele-specific primers, with GC tail of unequal length attached to their 5′ end, were designed using the Primer3 software (Koressaar and Remm, 2007; Untergasser et al., 2012), according to melting temperature (Tm)-shift SNP genotyping method developed by Wang et al. (2005). Fragments were amplified by PCR on an Eppendorf Mastercycler using a volume of 20-μl master-mix containing 1.5 mM MgCl2, 0.2 mM of dNTP Mix (Promega), 0.15 μM of each primer, 1X EvaGreen™ (Biotium, Fremont, CA, United States), 20 ng of genomic DNA, 1X Taq buffer, and 0.1 μl Taq1 polymerase (Promega) under the following profile: 94°C for 2 min, then 38 cycles of denaturation at 92°C for 20 s, annealing for 20 s (i.e., the temperature was specific to each primer trio), extension at 72°C for 20 s, and a final extension at 72°C for 5 min. The melting point analysis for the allele determination of the template DNA was performed with a fluorescence-detecting thermocycler (LightCycler™ 4890 Instrument II, Roche, Basel, Switzerland) with EvaGreen™ Fluorescent Dye (Biotium). The fluorescent detection profile was for 1 min at 95°C, and the melting curve step was ramping from 65 to 95°C in increments of 1°C for every 20 s.
Results
Identification of the bc-2 Locus by Genome-Wide Association Study
The GWAS was performed with 23 DDP lines with bc-2 or bc-22 alleles [i.e., 9 lines with bc-2 (NS to US-6 but M against NL-3) and 14 lines with bc-22 (12 lines with M against US-6 but NS to NL-3, and 2 lines with NLL to NL-3)] compared to 146 DDP lines absent the bc-2 and bc-22 alleles as indicated by M to both US-6 and NL-3, or mM, TN, or VN to NL-3. One significant peak interval (α = 0.05; p-value = 8.53E-26 to 4.33E-31) was detected between 9,117,985 and 10,468,271 based on Pv11 in G19833 v2.1 reference genome (Figure 1A and Supplementary Table 4a).
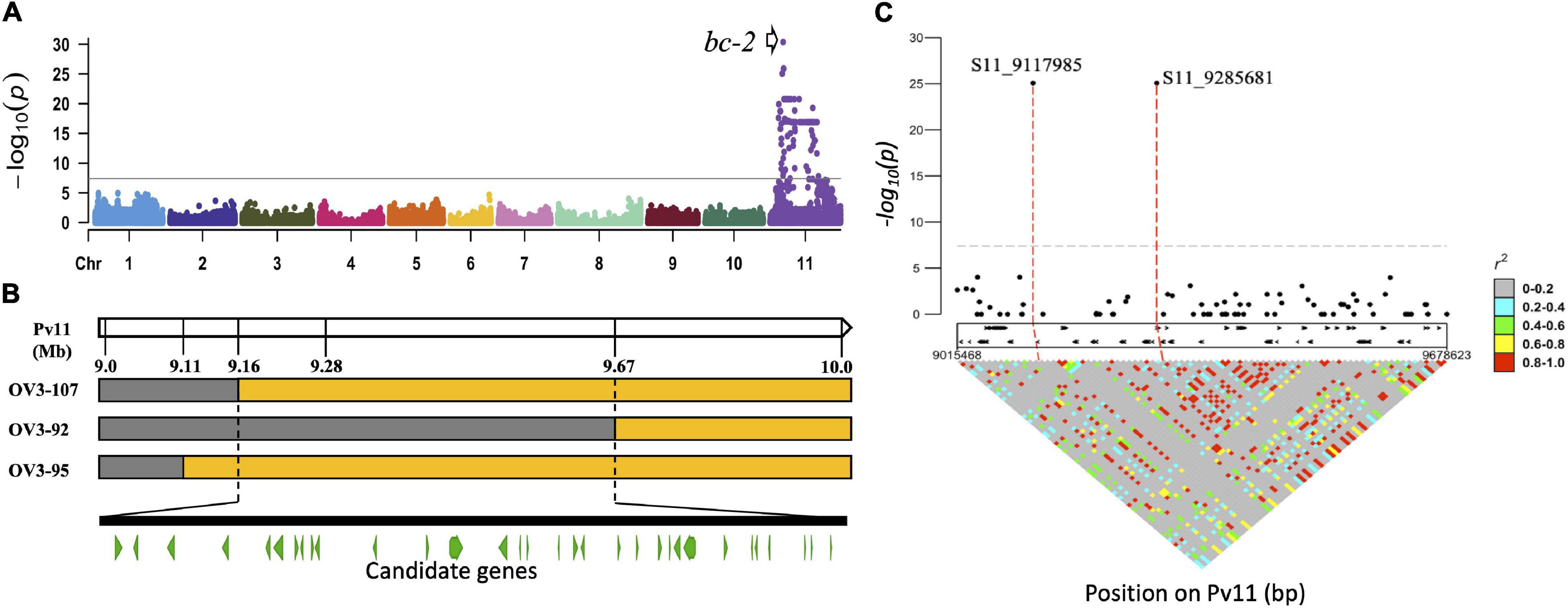
Figure 1. Detection and physical position of bc-2 gene on Pv11. (A) Genome-wide association study (GWAS) between Durango Diversity Panel (DDP) lines with no symptoms when inoculated with either NL-3 or US-6 strains vs. DDP lines exhibiting mosaic to both strains or top necrosis (TN), mild mosaic (mM), or vein necrosis (VN) to NL-3. (B) Othello/VAX 3 (OV3) RILs with recombinant haplotypes in the bc-2 region (orange color = “Othello” segment and gray = VAX-3 segment). (C) Marker-trait associations and linkage disequilibrium (LD) plots for the bc-2 region.
The interval for bc-22 was narrowed from 9.16 to 9.67 Mb in the OV3 RIL population, based on three RILs (i.e., OV3-92 with VN, OV3-107 with TN, and OV3-95 with NLL reactions to NL-3) with recombinant SNP haplotypes (Figure 1B and Supplementary Table 5). The narrowed region for bc-22, when applied to the whole-genome resequencing data for the DDP, revealed a low level of linkage disequilibrium (LD) between 74 single-nucleotide variants (SNVs), 31 insertions/deletions (InDel), and 11 short tandem repeats (STRs) polymorphic variants, distributed across 32 gene models (based on the G19833 v2.1 reference genome) (Figure 1C and Supplementary Table 6).
Additional alignment of the bc-2 region between G19833 v2.1 and UI 111 v1.1 reference genomes revealed a gap of 10,084 bases in “UI 111,” which just so happens to possess the bc-2 allele based on NS reaction to US-6 strain herein and from published reports (Singh et al., 2007, pp. 987). This 10-kb deletion in “UI 111” spans the Phvul.011G092600 and Phvul.011G092700 gene models of G19833 v2.1 reference genome (Figure 2A). The Phvul.011G092600 model, a subtilisin-like serine protein with endopeptidase activity, is completely missing, and the 3′ end of Phvul.011G092700, a vacuolar protein-sorting 4 (Vps4) AAA+ ATPase endosomal sorting complexes required for transport (ESCRT) protein involved in multivesicular endosome function, is truncated (Figures 2B,C). Amano et al. (2013) identified a Vps4 AAA+ ATPase ESCRT protein as a candidate gene (Cucsa.385040 in Phytozome v13) for the recessive zymA192– 18 gene conferring resistance to Zucchini yellow mosaic virus (ZYMV) in cucumber (Cucumis sativus L.). Using BLASTp, the Vps4 AAA+ ATPase ESCRT protein in cucumber had 92.2% identity with the Phvul.011G092700 gene model. Based on functional similarity to zymA192– 18, Phvul.011G092700 was chosen as the most likely candidate gene for bc-2.
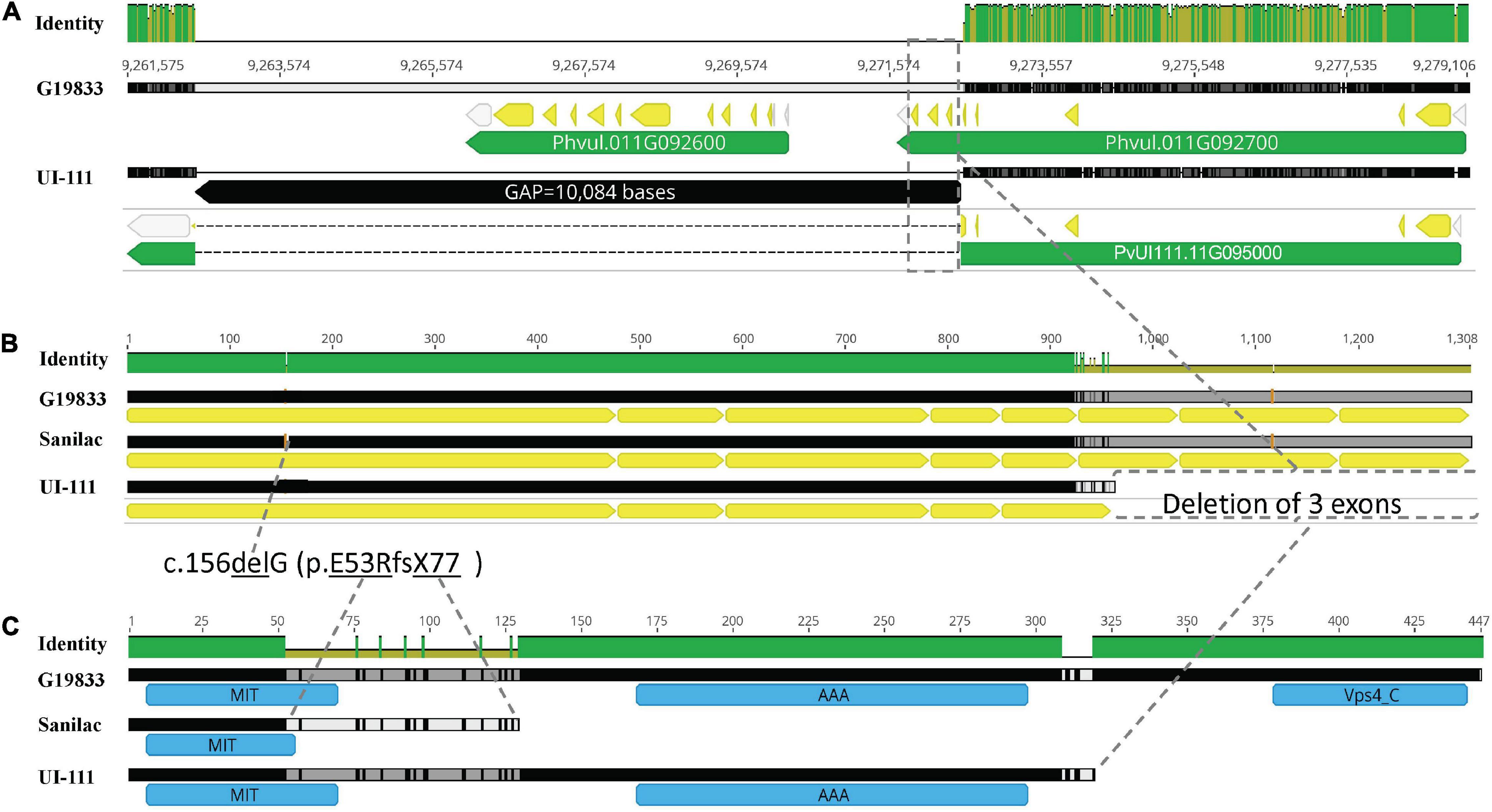
Figure 2. Genomic and protein representation of the candidate gene (Phvul.011G092700 gene in G19833 v2.1 is synonymous with PvUI111.11G095000 gene in UI 111 v1) for bc-2. (A) Candidate gene comparison between G19833 and UI 111 reference genomes depicting a deletion in the 3′ region of the candidate gene PvUI111.11G095000 (perpendicular rectangular box outlined by dashed line). (B) The Coding Sequence (CDS) of Phvul.011G092700 gene model in a reverse alignment of G19833 (wild-type Bc-2), Sanilac (bc-2[Robust]), and UI 111 (bc-2[UI 111]) genotypes. (C) Protein prediction in silico of Phvul.011G092700 in G19833, Sanilac, and UI 111.
Identification of the “Unknown” Gene (bc-4) Revealed by Genome-Wide Association Study and BLASTp
To identify the unknown gene that interacts with bc-2, GWAS was conducted with 9 DDP lines with bc-2 as detected by NS to US-6 and M to NL-3 strain compared to 160 DDP lines without bc-2 as determined by M, mM, NS, TN, and VN reactions against NL-3 strain. Notably, DDP lines without bc-2 included 12 lines with NS and 2 lines with NLL to NL-3 strain due to the presence of bc-22. The Manhattan plot revealed one significant peak (α = 0.05; p-value = 6.74E-43 to 2.62E-32) on Pv05, with an interval between 35,471,758 and 36,225,550 bases (Figure 3A and Supplementary Table 4b), which is putatively associated with the “unknown” gene that assists the expression of bc-2.
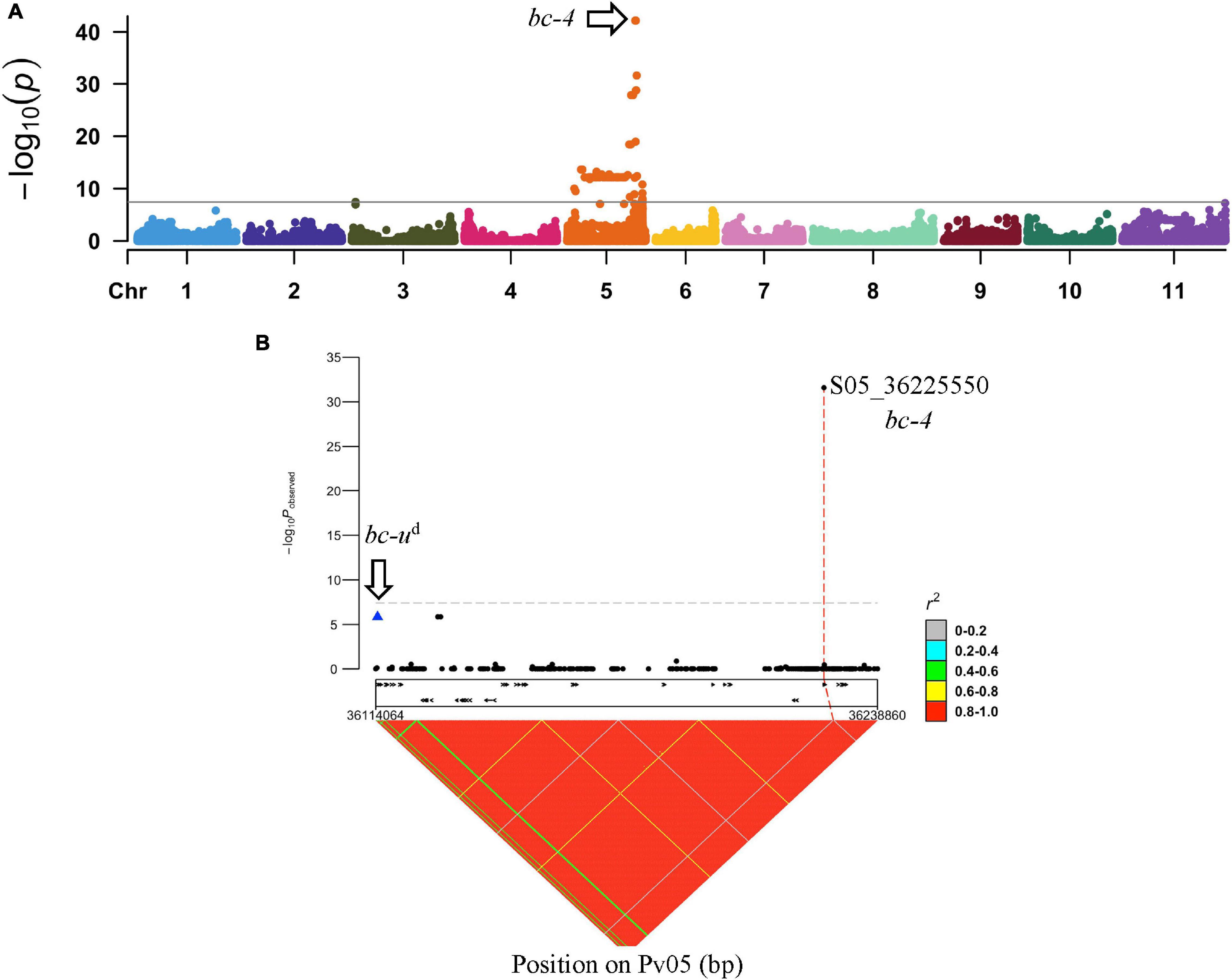
Figure 3. GWAS between DDP lines with no symptoms to US-6 strain vs. DDP lines exhibiting mosaic (M), TN, mM, or VN against NL-3. (A) Manhattan plot from GWAS in DDP lines exhibiting a significant peak on Pv05. (B) bc-4 regional association plot showing linkage (in repulsion) between bc-ud and bc-4 (S05_36225550).
The Vps4 AAA+ ATPase ESCRT protein of the Phvul.011G092700 candidate gene for the bc-2 resistance gene was blasted against the G19833 v2.1 reference genome, and the most significant hit (i.e., 94.9% identity) was Phvul.005G125100, a Vps4 AAA+ ATPase ESCRT homolog. Phvul.005G125100 was within the 35,471,758- to 36,225,550-bp GWAS interval. A significant SNP S05_36225550 (p-value = 2.62E-32), a missense variant, from the GWAS analysis was located within Phvul.005G125100. Furthermore, SNP S05_36225550 was present in all nine DDP lines with the unknown gene and absent in all DDP lines without the unknown gene. Interestingly, among DDP lines, the SNP S05_36225550 was found to be 111,034 bases upstream and in high LD with the bc-ud gene that interacts with bc-1 to confer resistance to BCMNV (Soler-Garzón et al., 2021; Figure 3B). Given these preliminary results, this new gene was assigned the preliminary gene symbol bc-4.
Sequencing the Candidate Genes for bc-2 and bc-4
A total of 16 lines were chosen according to BCMV and BCMNV reactions, gene combinations, and origin, for the exon sequencing of the bc-2 candidate gene Phvul.011G092700 and the bc-4 candidate gene Phvul.005G125100.
The Phvul.011G092700 sequences were blasted to the G19833 v2.1 reference genome (which has the Bc-2 wild-type genotype), revealing eight exon regions (Table 1 and Figure 2A). The Phvul.011G092700 sequences in the race Durango genotypes with either bc-2 (i.e., UI-111 and UI129) or bc-22 (i.e., Othello and 92US-1006) recessive resistance alleles all possessed the same 10,084-bp deletion that truncates three exons from the 3′ end (Figure 2B), providing initial evidence of a single resistance allele. For the race Mesoamerican genotypes Robust, Michelite-62, and Sanilac, also with the bc-2 resistance allele, there was a single deletion-frameshift mutation (Pv11: 9,278,764 bases) in the first exon of Phvul.011G092700. To track these different bc-2 mutations, they were assigned a superscript in brackets: bc-2[UI 111] for the large 10-kb deletion of three exons found in race Durango lines, and bc-2[Robust] for the deletion-frameshift found in navy beans.
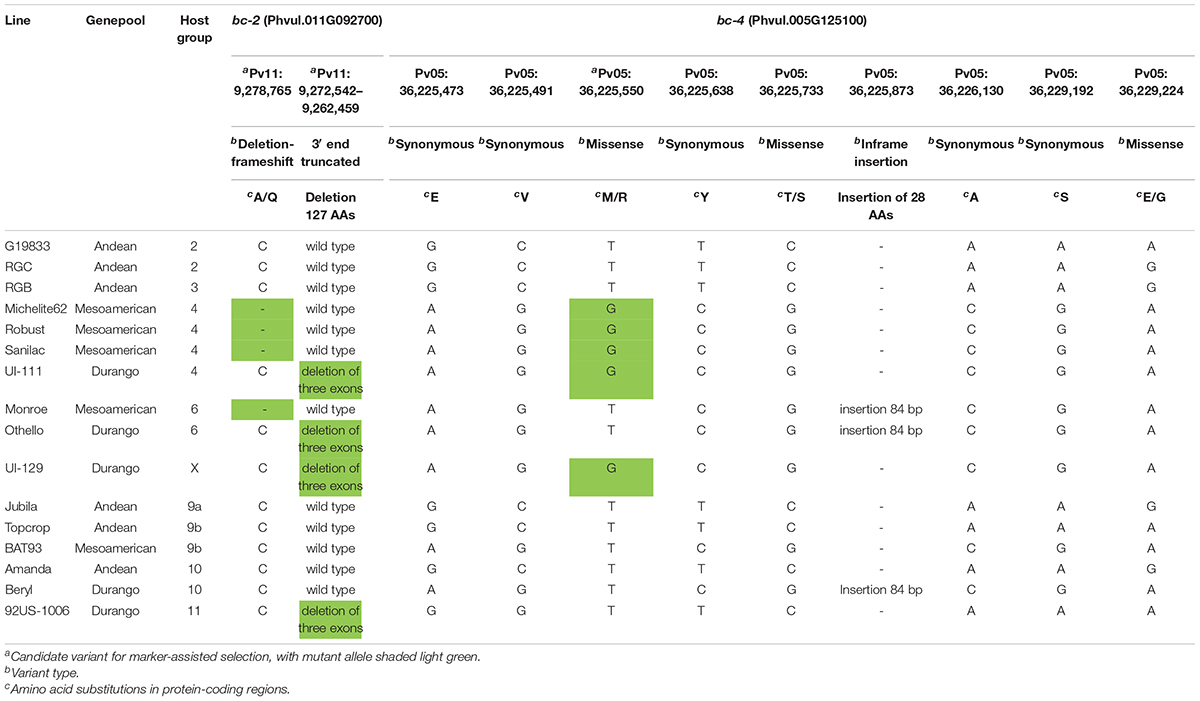
Table 1. Polymorphisms detected within the exon regions for the candidate genes Phvul.011G092700 and Phvul.005G125100 for bc-2 and bc-4, respectively, among 16 genotypes.
The G19833 Phvul.011G092700 protein is 446 amino acids (aa) in length and contains three domains, namely, (i) microtubule interacting and transport (MIT) domain, (ii) AAA-type ATPase (AAA+) domain, and (iii) Vps4 domain (Figure 2C). The same protein homolog PvUI111.11G095000 (but with a different code) in reference genome “UI 111” (which has bc-2 genotype) has only 319 aa and 2 domains, i.e., MIT and AAA+, due to the 10,084-bp deletion. The Sanilac (or Robust) homolog has only 128 aa due to 1 deletion-frameshift mutation generating a single aa replacement in aa position 53 (p.E53R) and a premature stop codon in position 77 (fsX77), resulting in an incomplete protein with only the MIT domain.
The in silico-translated Vps4 AAA+ ATPase ESCRT protein of sequenced open reading frame (ORF) for bc-4 candidate gene Phvul.005G125100 did not reveal disruption among the MIT, AAA+, and Vps4 domains. The SNP S05_36225550 missense variant was found to be a transversion T > G, which causes the substitution of a non-polar, neutral aa, Methionine (M), with a basic polar, positively charged aa, Arginine (R), at position 33 of the MIT domain (i.e., p.M33R) (Table 1 and Figure 4).
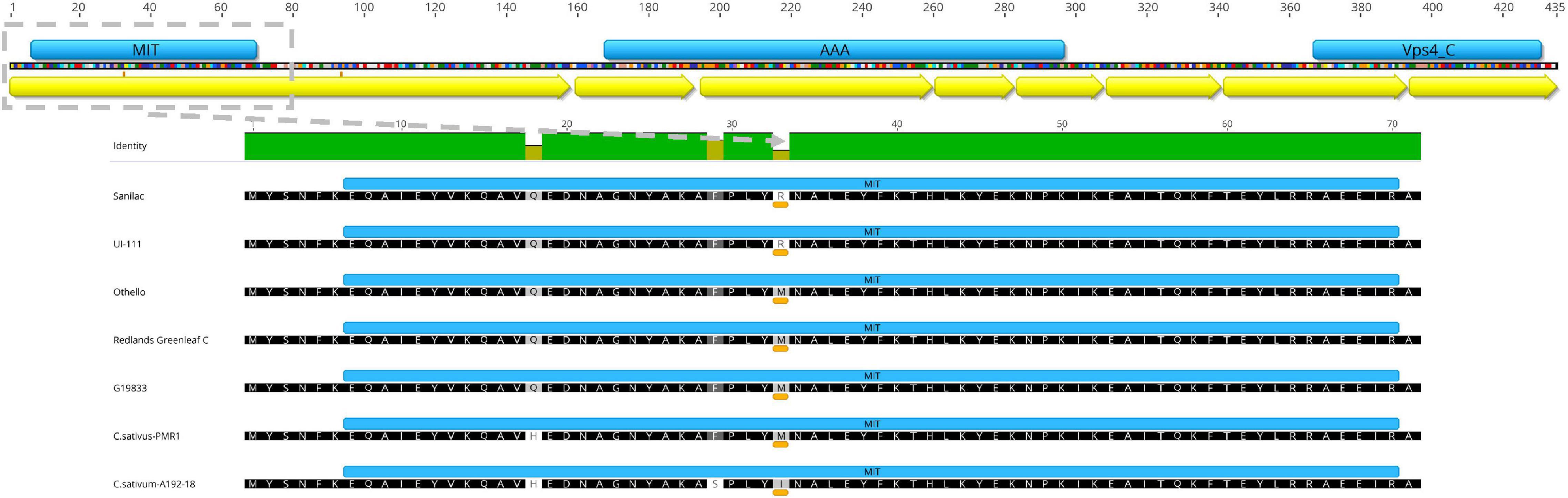
Figure 4. Phvul.005G125100 candidate gene for bc-4. (Top) Phvul.005G125100 shows three domains (blue bars) and eight exons (yellow arrows). (Bottom) Microtubule interacting and transport (MIT) domain (blue bar) zoomed in for five Phaseolus vulgaris lines and two Cucumis sativus lines (Amano et al., 2013), depicting the amino acid substitution for position 33 underlined by an orange-colored bar.
Tm-Shift Genotyping With Candidate Single-Nucleotide Polymorphism Markers
Tm-shift assays were developed to detect the polymorphisms in the bc-2 and bc-4 candidate genes (Table 2). Two distinct assays were designed for the different bc-2 candidate gene mutations. The bc-2[UI 111] assay was developed using the first forward primer aligned to the “UI 111” gene (PvUI111.11G095000) sequence and the second forward primer aligned to the G19833 gene (Phvul.011G092700) sequence and a common reverse primer (Supplementary Material 1). The technical name for this marker assay is “Pvvps4_del.” For better amplification efficiency, no GC tails were added to “Pvvps4_del” forward primers. The assay for bc-2[Robust] flanking the S11_9278764 in-frame-deletion in the navy beans Sanilac and Michelite-62 is named “Pvmit-2_C_del.”
The assay for bc-4 was developed using the S05_36225550, i.e., missense SNP, in the Phvul.005G125100 candidate gene. The technical name for this marker is “Pvmit-1_T_G.” These bc-2 and bc-4 markers were added to I, bc-1, bc-3, and bc-ud markers developed by Soler-Garzón et al. (2021) for genotyping the lines and populations described below.
Durango Diversity Panel and Othello/VAX 3 Population Assays
The DDP was assayed for both “Pvmit-2_C_del” and “Pvvps4_del” markers for bc-2, but only the latter bc-2[UI 111]-linked marker was detected. All DDP lines with bc-2 (NS to US-6 and M to NL-3) or bc-22 resistance alleles (M to US-6 and NS to NL-3) possessed the “Pvvps4_del” marker for bc-2[UI 11], and all other DDP lines possessed the wild-type Bc-2 allele. The DDP lines with bc-2 possessed the bc-4 marker, and those with bc-22 possessed the bc-ud marker, including those DDP lines in HG-11 (92US-1006 and Quincy) with NLL reaction to NL-3 conditioned by I, bc-22, and bc-ud genes (Supplementary Table 7). These results further support that only one resistance allele, i.e., bc-2, exists at the Bc-2 locus. This bc-2 allele exhibits a differential effect based on the presence of bc-4 or bc-ud.
Four genes (i.e., I, bc-1, bc-2, and bc-ud) were segregated in the OV3 RIL population. Of the four genes, only the I gene had distorted (df = 1.0, p ≤ 0.05: X2 = 5.99) segregation, 3:1, in favor of the dominant I gene, whereas 1:1 segregation was expected. Altogether, there were six distinct phenotypes to NL-3, seven phenotypes to NL-8, two phenotypes to US-6, and 16 distinct genotypes (Supplementary Table 8). The phenotypic reactions of all RILs to US-6, NL-8, and NL-3 strains matched the genotypes predicted by the resistance gene-linked markers. The most relevant results for this study were that RILs with bc-2 and bc-ud expressed M to US-6 and NS to NL-3 when I gene was absent (∼HG-6) and NLL when I gene was present (∼HG-11), whereas the bc-2 allele had no discernible effect against any of the strains in the absence of bc-ud. Additionally, OV3 RILs with unique genotypes were phenotyped with US-4 (PG-IV) to further validate their use as additional differential cultivars in HGs 1, 2, 6, 8, and 9b (Table 3).
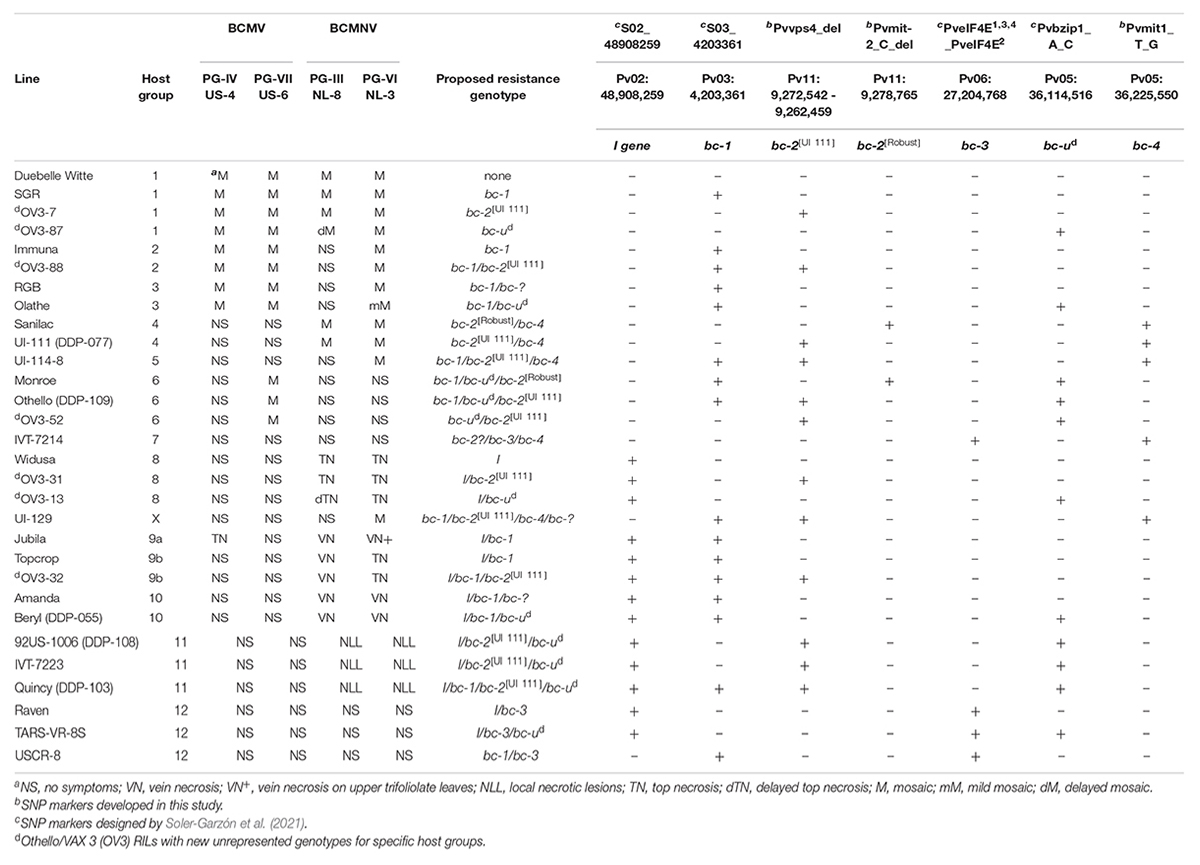
Table 3. Single-nucleotide polymorphism (SNP) genotyping of host differential genotypes, including OV RILs with new unrepresented genotypes within specific host groups, with reactions to select Bean common mosaic virus (BCMV) and Bean common mosaic necrosis virus (BCMNV) strains.
Host Group Assays
Differential genotypes were distributed across 12 HGs for BCMV/BCMNV, and one unassigned genotype “UI 129” was assayed for the I, bc-1, bc-3, bc-ud, bc-2[UI 111], bc-2[Robust], and bc-4 markers (Table 3). Only genotypes in HG-4 and HG-5, previously reported to possess bc-2, possessed the bc-4 gene. In addition, HG-4 (NS to US-6 and M to NL-3) had a mix of genotypes with the two distinct bc-2 mutations, namely, Michelite-62 and Sanilac with bc-2[Robust], and “UI 111” and “UI 34” with bc-2[UI 111]. Moreover, all HG-4 genotypes exhibited NS to US-6 and M to NL-3 strains, regardless of the mutation.
The HG-5 genotype, “UI 114,” possesses bc-1, bc-2[UI 111], and bc-4. “UI 114,” similar to HG-4 genotypes, exhibits NS to US-6 and M to NL-3; however, it also possesses resistance to NL-8 strain, whereas HG-4 genotypes are susceptible. Soler-Garzón et al. (2021) showed that bc-1 alone provides protection to NL-8, and the addition of this gene in HG-5 lines explains the differential reaction between HG-4 and HG-5 genotypes to NL-8. Unassigned genotype “UI 129” also carries bc-1, bc-2[UI 111], and bc-4 like HG-5, but unlike HG-5 lines, it exhibits resistance to PG-V strains. Therefore, the hypothesis that “UI 129” has another unknown gene that contributes to its resistance against PG-V strains should be tested.
The HG-6 genotypes (bc-2 and bc-ud) exhibit resistance to all BCMV and BCMNV PGs except PG-VII with BCMV strains such as NL-4 and US-6 (Drijfhout, 1978). However, most HG-6 genotypes possess bc-1, in addition to bc-2[UI 111] and bc-ud (e.g., Othello, UI-31, and UI-35). Furthermore, Monroe with bc-2[Robust], bc-1, and bc-ud is also classified as an HG-6 differential cultivar (Miklas et al., 2000), which indicates that the bc-2[Robust] and bc-2[UI 111] mutations react similarly with bc-ud. Finally, the OV3 RILs with bc-2 and bc-ud but without bc-1 were resistant to PG-III, IV, VI, and VII strains in our screenings, indicating that the combination of bc-2 and bc-ud conditions resistance to PG-III and PG-V strains in the absence of bc-1.
The HG-7 cultivar IVT-7214 possessed the bc-3 and bc-4 genes, based on the marker assays, indicating that bc-4, not bc-ud as previously considered, may be interacting with bc-3 to confer broad resistance against all PGs, except for the newly discovered PG-VIII (Feng et al., 2015). Therefore, we are in the process of developing a differential cultivar with bc-3 and bc-ud to test whether bc-ud may help to extend the range of effectiveness for bc-3 to condition resistance to this new PG-VIII as well. However, we did not observe our bc-2 gene-linked markers in IVT-7214, whereas its presence was reported by Drijfhout (1978). Perhaps IVT-7214 is heterogeneous, and the line we happened to test was absent bc-2. This is the best explanation because the results for the “Michelite-62 (bc-2/bc-4) x IVT-7214 (bc-2/bc-3/bc-4)” F2 population from the study by Drijfhout (1978) support the segregation of a single gene, i.e., bc-3, with bc-2 and bc-4 genes present in both parents.
For the HGs 8–12 with I gene, bc-4 was absent in all, and bc-2 was only detected in HG-11. The HG-11 lines all possessed I, bc-2[UI 111], and bc-ud genes. As was reported by Drijfhout (1978) and validated by Soler-Garzón et al. (2021), the bc-ud and bc-2[UI 111] gene combination is needed to condition NLL to BCMNV (PG-III and PG-VI) in the presence of the I gene. OV3 RILs with I, bc-1, bc-2[UI 111], and bc-ud also expressed NLL to BCMNV, indicating no additional phenotypic effect from the presence of bc-1.
Allelism Tests in F2 Populations and F2:3 Families
Fixed Genotypes
The observed genotypic and phenotypic segregation ratios for BCMV and BCMNV reactions for each F2 population and F2:3 family are summarized in Supplementary Tables 9, 10, respectively. The resistant gene-linked SNP and InDel markers for I, bc-1, bc-ud, bc-2 (both mutations), and bc-4 in F2 and F2:3 families, in most cases, fit 1:2:1 (df = 2.0, p ≤ 0.05: 5.99) segregation ratios, as expected for codominant markers.
As expected, F2 individuals homozygous for gene-linked markers bred true in the F3 generation. The true-breeding F2:3 families for one or more markers matched the phenotypes of the parents and other DDP lines with the same marker genotypes (Supplementary Table 9). F2:3 families with bc-2[UI 111] or bc-2[Robust] + bc-ud fixed exhibited M to US-6 and NS to NL-3. Conversely, families with bc-2[UI 111] or bc-2[Robust] + bc-4 fixed exhibited NS to US-6 and M to NL-3. Finally, families with I + bc-ud + bc-2[UI 111] or bc-2[Robust] exhibited NLL reaction, whereas those with I + bc-2[UI 111] or bc-2[Robust] + bc-4 exhibited TN to NL-8 and NL-3. These results further support a single allele at the bc-2 locus with a differential reaction to PG-VI and PG-VII strains based on which epistatic gene, bc-ud or bc-4, is present.
Segregating Families: US-6 and NL-3 Comparison Reactions
The F2:3 families with bc-2[UI 111] fixed and segregating for bc-ud and bc-4, which are linked in repulsion, showed 1 NS to 3 M segregation ratio to US-6 and NL-3 strains. A low recombination frequency (6 of 552 plants = 1.1 cM) was observed for bc-ud and bc-4, as expected for loci 111,034 bases apart. The recombinant F2:3 plants with bc-2[UI 111]bc-2[UI 111]/bc-udbc-ud/Bc-4bc-4 genotype exhibited M to US-6 and NS to NL-3, and plants with bc-2[UI 111]bc-2[UI 111]/Bc-udbc-ud/bc-4bc-4 exhibited NS to US-6 and M to NL-3. No F2 or F2:3 plants, which were homozygous recessive for both bc-ud and bc-4 genes, were observed.
For F2:3 families with bc-1 fixed and segregating for bc-2[UI 111], bc-ud, and bc-4, the phenotypic ratios of 3 M to 1 NS against US-6 and 12 M to 3 mM to 1 NS against NL-3 were observed. Thus, unlike bc-1 and bc-ud, which interact to confer partial mM reaction to NL-3, the bc-1 and bc-4 combination does not exhibit partial resistance to NL-3. Again, no F2 or F2:3 plants, which were homozygous recessive for both bc-ud and bc-4 genes, were observed.
The F2 population “Michelite-62 x Poncho” segregating for bc-2[Robust], bc-ud, and bc-4 exhibited 15 M to 1 NS segregation ratios to US-6 and NL-3. In this case, F2 plants with Bc-udBc-ud/bc-4bc-4/bc-2[Robust]bc-2[Robust] genotype had NS to US-6 and M to NL-3, and plants with bc-udbc-ud/Bc-4Bc-4/bc-2[Robust]bc-2[Robust] were M to US-6 and NS to NL-3. These results further support that the two bc-2 mutations, namely, bc-2[UI 111] and bc-2[Robust], have the same genetic and phenotypic effects.
The F2:3 families, with I gene and bc-1 fixed and bc-ud and bc-4 segregating, exhibited a 1 TN to 2 dTN to 1 VN segregation ratio to NL-3, where plants with II/bc-1bc-1/Bc-udBc-ud/bc-4bc-4 genotype showed TN, and plants heterozygous for bc-ud and bc-4 (II/bc-1bc-1/Bc-udbc-ud/Bc-4bc-4) exhibited dTN reaction 2 wpi. Plants with II/bc-1bc-1/bc-udbc-ud/Bc-4Bc-4 genotype, as was reported by Soler-Garzón et al. (2021), exhibited VN. Additionally, F2:3 families with I gene and bc-2[UI 111] fixed and segregating for bc-ud showed a 3 TN to 1 NLL segregation ratio to NL-3. The F2 population “Beryl x Sanilac” segregating for I, bc-1, bc-2[Robust], bc-ud, and bc-4 exhibited six distinct phenotypes to NL-3, revealing one plant genotype Ii/bc-2[Robust]bc-2[Robust]/bc-udbc-ud with NLL reaction, and one plant genotype II/Bc-1bc-1/bc-2[Robust]bc-2[Robust]/bc-4bc-4 with TN. Therefore, both genotypes provide evidence that bc-2[Robust] behaves similarly as bc-2[UI 111] in the presence of I and bc-ud or bc-4 genes.
Segregating Families: US-4 Reactions
The F2 population “Sanilac x Poncho” exhibited a 14 (M or dM) to 2 (NS) segregation ratio against US-4 with bc-2[Robust]bc-2[Robust]/Bc-udBc-ud/bc-4bc-4 and bc-2[Robust]bc-2[Robust]/bc-udbc-ud/Bc-4Bc-4 genotypes exhibiting NS. Furthermore, F2:3 plants from “NW-63 x Gloria,” i.e., “NW-63 x UI 114” and “Gemini x UI-126” crosses with bc-2[UI 111]bc-2[UI 111]/bc-udbc-ud/Bc-4Bc-4 or bc-2[UI 111]bc-2[UI 111]/Bc-udBc-ud/bc-4bc-4 genotypes, exhibited no symptoms to US-4, regardless of the allelic state for bc-1.
Segregating Families: NL-8 Reactions
The F2:3 families fixed for the I and bc-1 genes (II/bc-1bc-1) all exhibited VN to NL-8, regardless of the allelic state for bc-ud or bc-4. When the I and bc-ud genes were fixed and bc-2[UI 111] was segregating, a 3 dTN to 1 NLL phenotypic ratio was observed. When both bc-2[UI 111] and bc-ud were segregating and I gene fixed, a 1 TN to 2 dTN to 1 NLL segregation ratio was observed, which fit with the segregation ratios observed above for each gene. Thus, the bc-1 allele exhibited dominant inheritance in the presence of the I gene, as F2:3 individuals with II/Bc-1bc-1 genotype exhibited VN to NL-8, as reported by Soler-Garzón et al. (2021). Conversely, genotypes with the I and bc-2[UI 111] genes fixed, but absent bc-1 or bc-ud, exhibited TN.
Discussion
In this study, we reported on the genomic characterization of the recessive bc-2 gene and its genetic interactions with other genes to confer resistance to BCMV and BCMNV. GWAS with the DDP was used to locate bc-2 to Pv11. Haplotyping in the OV3 RIL population was then used to narrow the bc-2 region. The Vps4 AAA+ ATPase ESCRT gene, Phvul.011G092700, was identified, within the narrowed interval, as the candidate gene for bc-2. Another GWAS with the DDP combined with BLASTp of the Phvul.011G092700 was used to discover the new recessive bc-4 gene on Pv05. The bc-4 candidate gene, Phvul.005G125100, is also a Vps4 AAA+ ATPase ESCRT protein with 95% identity to the bc-2 candidate gene.
Numerous polymorphic variants were observed for the two candidate genes. Two polymorphic variants, resulting in the translation of incomplete proteins for Phvul.011G092700, in silico, had different evolutionary origins. A 10-kb deletion eliminated the 3′ exons in race Durango lines, such as the “Common Red” landrace, which we believed is the progenitor for this variant. A single “missense” SNP deletion, in the first exon, was found in Robust navy bean, a landrace selection from the Michigan State University bean program released in 1915 (Kelly, 2010). The variant is also found in Michelite-62 navy with Robust as a progenitor, released in 1938, Monroe navy from “Michelite-62 x UI-1” (a selection from great northern landrace) released in 1953, and Sanilac navy bean released in 1956. Dr. Bill Dean, a bean breeder for many years, postulated that the bc-2 and bc-22 genes (now just bc-2) were hidden in “Common Red” and did not surface until crosses were made with great northern landrace selections, which possessed the bc-12 (now bc-1) and bc-u (now bc-ud) genes (Dean, 1994). Dr. Bill Dean was absolutely correct in his assumption.
A single missense SNP S05_36225550 in the first exon of Phvul.005G125100 was the variant identified by comparing ORF sequences across genotypes as the most likely causal mutation for bc-4. Furthermore, this same variant was the peak SNP for the bc-4 interval identified by GWAS.
The Tm-shift assay markers for the two InDel variants named bc-2[UI 111] for the 10-kb deletion of race Durango origin and bc-2[Robust] for the SNP deletion of navy bean origin, as well as missense SNP for bc-4, were developed to track the bc-2 and bc-4 genotypes underlying phenotypic segregations in F2 and F2:3 families and for genotyping lines in the DDP, OV3 population, and HGs. In addition, the same populations were assayed for the I, bc-1, and bc-ud gene-linked markers developed by Soler-Garzón et al. (2021). Across populations, the different variants for bc-2[UI 111] and bc-2[Robust] did not exhibit differential reactions against the PGs (i.e., III, IV, VI, and VII) tested in this study. Thus, we bracketed the superscripts “UI 111” and “Robust” for the different bc-2 gene mutations with the same effect to distinguish them from alleles with different genetic effects that are normally noted by unbracketed superscripts.
The bc-2 and bc-4 genes exhibited recessive inheritance across the segregating populations. Across all populations (i.e., F2, F2:3, DDP, OV3, and HGs), bc-2 required the presence of either bc-4 or bc-ud for expression. Drijfhout (1978), and the literature since then, described two recessive resistance alleles bc-2 and bc-22 for the Bc-2 locus with HG-4, HG-5, and HG-7 lines possessing bc-2 allele and HG-6 and HG-11 lines possessing the bc-22 allele. Conversely, we observed no alleles for bc-2. The sequencing data for the bc-2 Vps4 AAA+ ATPase ESCRT candidate gene did not reveal any polymorphic variant between bc-2 (e.g., “UI 111”) and bc-22 (e.g., Othello) in dry bean genotypes. Consequently, bc-2 in HG-4 and HG-5 lines interacted with bc-4 to confer resistance to all BCMV PGs except PG-V, and bc-2 in HG-6 and HG-11 lines interacted with bc-ud to confer resistance to both BCMV and BCMNV. bc-2 was not observed in the HG-7 genotype IVT-7214 plant we sampled, but it did possess bc-4. Interestingly, IVT-7214 was susceptible to a Clover yellow vein virus (ClYVV) strain from Wisconsin (Larsen et al., 2008) and to BCMV “1755a” strain forming the new PG-VIII (Feng et al., 2015). Meanwhile, the USCR-8 germplasm line (Miklas and Hang, 1998), with bc-1 and bc-3, was resistant to all ClYVV strains (Hart and Griffiths, 2014) and all BCMV and BCMNV strains, except NL-3 K strain to which it exhibits mild mosaic symptoms (Larsen et al., 2005). Perhaps bc-4 negatively affects bc-3 resistance against these potyviruses. Whether bc-4 interacts with bc-3 in IVT-7214 (HG-7) will require further investigation. At present, F2 populations with IVT-7214 as a parent are lacking in our studies because it is photoperiod sensitive and does not produce flowers in our greenhouse.
The tight linkage of bc-4 and bc-ud in the repulsion phase likely contributed to the study by Drijfhout (1978) describing bc-u as the helper gene for bc-2 in HG-4 and HG-5 lines. In addition, bc-4 did not exhibit any effect by itself or in combination with any other gene besides bc-2, whereas bc-ud alone delays the onset of symptoms by about 1 week and interacts with multiple genes, e.g., bc-1 and bc-2, to confer resistance to BCMV and BCMNV (Soler-Garzón et al., 2021).
Overall, bc-2 helped by bc-4 provides resistance against all BCMV PGs except PG-V, while bc-2 helped by bc-ud provides resistance against all BCMV and BCMNV PGs except BCMV PG-VII. This results in a major differential reaction for the bc-2 and bc-4 vs. bc-2 and bc-ud combinations, as the former is resistant to BCMV strain US-6 (PG-VII) and susceptible to BCMNV strain NL-3 (PG-VI), and the latter is susceptible to US-6 and resistant to NL-3. In addition, the bc-2 and bc-4 combination does not protect the I gene (TN, leading to plant death) against BCMNV strains, whereas the bc-2 and bc-ud combination does protect the I gene (NLL restricted to the inoculated leaf). Thus, in the absence of the I gene, if BCMV strains from PG-VII are prominent in a region, then the bc-2 and the bc-4 combination is preferable for protection. If that PG is absent, then the combination of bc-2 and bc-ud is the better combination to deploy.
The studies below support Vps4 AAA+ ATPase ESCRT proteins as the candidate genes for the recessive bc-2 and bc-4 genes, which interact to condition resistance to BCMV, which like TBSV and ZYMV, is a +ssRNA virus. The Vps4 AAA+ ATPase ESCRT candidate gene proteins for bc-2 and bc-4 have a key function in various activities related to endosomal traffic to lysosomes, organelle biogenesis, DNA replication, protein folding, and proteolysis (Obita et al., 2007). Barajas et al. (2014) documented a non-canonical role for Vps4 AAA+ ATPase ESCRT during Tomato bushy stunt virus (TBSV) replication, whereby the virus recruits the cellular ESCRT machinery for replication, evading recognition by the host defenses and preventing viral RNA destruction. For example, a study in C. sativus revealed Vps4 AAA+ ATPase ESCRT as a candidate gene for zym, a recessive gene resistant to ZYMV (Amano et al., 2013). A critical difference between Vps4 AAA+ ATPase ESCRT-mediated resistance to ZYMV is that only one gene model encodes this protein in cucumber. In contrast, there are two such gene models in common bean, and both need to be disrupted to confer resistance to BCMV. Moreover, Feng et al. (2018) observed that the bc-2 (and bc-4 combination) had no effect on cell-to-cell movement and replication of BCMV but did affect systemic spread of BCMV in common bean, which fits the phenotypic reactions observed in this study and the mode of action for Vps4 AAA+ ATPase ESCRT described by Barajas et al. (2014).
The in silico analysis of the transcribed proteins for the bc-2[UI 111] and bc-2[Robust] frameshift mutations showed non-functional truncated Vps4 AAA+ ATPase ESCRT proteins due to premature termination. For bc-4, a missense SNP mutation (S05_36225550) at codon M33R (Methionine to Arginine) in the MIT domain of the Vps4 AAA+ ATPase ESCRT protein from Phvul.005G125100 gene model was predicted by in silico protein analysis. Similarly, Amano et al. (2013) found variants at codons F29S (Phenylalanine to Serine) and M33I (Methionine to Isoleucine) in the MIT domain of the candidate protein for zym resistance gene. The latter variant is in the same codon position as our variant for bc-4. They suggested that the codon variants found in the MIT domain are the key elements responsible for viral-host protein-protein interactions. It is clear from the literature, as well as the results for bc-2 and bc-4 in this study, that host proteins that are used by the virus for replication and transport, which become non-functional or less functional due to frameshift or missense mutations, will result in recessive resistance genes in the host for combatting the virus. Candidate genes encoding altered or non-functional proteins due to missense or frameshift mutations underlie the recessive bc-3 (Naderpour et al., 2010) and bc-ud (Soler-Garzón et al., 2021) genes conditioning resistance to BCMV and BCMNV. The candidate gene for bc-ud (Pvbzip1) contains a stop-gained mutation that results in a premature termination codon and non-functional protein. For eIF4E, the missense coding variants that contributed to bc-3, aligned with missense coding variants for eIF4E associated with virus resistance in other species, similar to the same missense codon 33 variant in the Vps4 AAA+ ATPase ESCRT candidates for bc-4 and zym.
Conclusion
The findings that were obtained herein enhance our understanding of host-virus pathogen interactions and provide new tools and information to facilitate breeding for resistance to BCMV and BCMNV in common beans.
Data Availability Statement
The datasets presented in this study can be found in online repositories. The names of the repository/repositories and accession number(s) can be found in the article/Supplementary Material.
Author Contributions
AS-G and PNM conceived and designed the experiments and wrote the manuscript. AS-G conducted the genomics analyses, genotyping, and phenotyping. PEM generated SNP data. All authors contributed to the article and approved the submitted version.
Conflict of Interest
The authors declare that the research was conducted in the absence of any commercial or financial relationships that could be construed as a potential conflict of interest.
Publisher’s Note
All claims expressed in this article are solely those of the authors and do not necessarily represent those of their affiliated organizations, or those of the publisher, the editors and the reviewers. Any product that may be evaluated in this article, or claim that may be made by its manufacturer, is not guaranteed or endorsed by the publisher.
Supplementary Material
The Supplementary Material for this article can be found online at: https://www.frontiersin.org/articles/10.3389/fpls.2021.769247/full#supplementary-material
Footnote
References
Amano, M., Mochizuki, A., Kawagoe, Y., Iwahori, K., Niwa, K., Svoboda, J., et al. (2013). High-resolution mapping of zym, a recessive gene for Zucchini yellow mosaic virus resistance in cucumber. Theor. Appl. Genet. 126, 2983–2993. doi: 10.1007/s00122-013-2187-5
Barajas, D., Martín, I. F. C., Pogany, J., Risco, C., and Nagy, P. D. (2014). Noncanonical role for the host Vps4 AAA+ ATPase ESCRT protein in the formation of Tomato bushy stunt virus replicase. PLoS Pathog. 10:e1004087. doi: 10.1371/journal.ppat.1004087
Burke, D. W., Silbernagel, M. J., Kraft, J. M., and Koehler, H. H. (1995). Registration of ‘Othello’ pinto bean. Crop Sci. 35, 943–943. doi: 10.2135/cropsci1995.0011183X003500030064x
Drijfhout, E. (1978). Genetic interaction between Phaseolus vulgaris and bean common mosaic virus with implications for strain identification and breeding for resistance. Versl. van Landbouwkd. Onderz. 1978, 1–89.
Feng, X., Myers, J. R., and Karasev, A. V. (2015). Bean common mosaic virus isolate exhibits a novel pathogenicity profile in common bean, overcoming the bc-3 resistance allele coding for the mutated eIF4E translation initiation factor. Phytopathology 105, 1487–1495. doi: 10.1094/PHYTO-04-15-0108-R
Feng, X., Orellana, G. E., Myers, J. R., and Karasev, A. V. (2018). Recessive resistance to Bean common mosaic virus conferred by the bc-1 and bc-2 genes in common bean (Phaseolus vulgaris) affects long-distance movement of the virus. Phytopathology 108, 1011–1018. doi: 10.1094/PHYTO-01-18-0021-R
Hart, J. P., and Griffiths, P. D. (2014). Resistance to Clover yellow vein virus in common bean germplasm. Crop Sci. 54, 2609–2618. doi: 10.2135/cropsci2014.03.0263
He, F., Ding, S., Wang, H., and Qin, F. (2020). IntAssoPlot: an R package for integrated visualization of genome-wide association study results with gene structure and linkage disequilibrium matrix. Front. Genet. 11:260. doi: 10.3389/fgene.2020.00260
Kearse, M., Moir, R., Wilson, A., Stones-Havas, S., Cheung, M., Sturrock, S., et al. (2012). Geneious Basic: an integrated and extendable desktop software platform for the organization and analysis of sequence data. Bioinformatics 28, 1647–1649. doi: 10.1093/bioinformatics/bts199
Kelly, J. D. (2010). The Story of Bean Breeding White Paper Prepared for BeanCAP & PBG Works on the Topic of Dry Bean Production and Breeding Research in the U.S. Available online at: http://www.hrt.msu.edu/pbgp/Links/plantbreedingintro.html (accessed July 21, 2021).
Koressaar, T., and Remm, M. (2007). Enhancements and modifications of primer design program Primer3. Bioinformatics 23, 1289–1291. doi: 10.1093/bioinformatics/btm091
Larsen, R. C., Miklas, P. N., Druffel, K. L., and Wyatt, S. D. (2005). NL-3 K strain is a stable and naturally occurring interspecific recombinant derived from Bean common mosaic necrosis virus and Bean common mosaic virus. Phytopathology 95, 1037–1042. doi: 10.1094/PHYTO-95-1037
Larsen, R. C., Miklas, P. N., Eastwell, K. C., and Grau, C. R. (2008). A strain of Clover yellow vein virus that causes severe pod necrosis disease in snap bean. Plant Dis. 92, 1026–1032. doi: 10.1094/PDIS-92-7-1026
Lipka, A. E., Tian, F., Wang, Q., Peiffer, J., Li, M., Bradbury, P. J., et al. (2012). GAPIT: genome association and prediction integrated tool. Bioinformatics 28, 2397–2399. doi: 10.1093/bioinformatics/bts444
Miklas, P. N., and Hang, A. N. (1998). Release of cranberry dry bean germplasm lines USCR-7 and USCR-8 with resistance to bean common mosaic and necrosis viruses. Annu. Rep. Bean Improv. Coop. 41, 227–228.
Miklas, P. N., Larsen, R. C., Riley, R., and Kelly, J. D. (2000). Potential marker- assisted selection for bc-12 resistance to bean common mosaic potyvirus in common bean. Euphytica 3, 211–219.
Naderpour, M., Lund, O. S., Larsen, R., and Johansen, E. (2010). Potyviral resistance derived from cultivars of Phaseolus vulgaris carrying bc-3 is associated with the homozygotic presence of a mutated eIF4E allele. Mol. Plant Pathol. 11, 255–263.
Obita, T., Saksena, S., Ghazi-Tabatabai, S., Gill, D. J., Perisic, O., Emr, S. D., et al. (2007). Structural basis for selective recognition of ESCRT-III by the AAA ATPase Vps4. Nature 449, 735–739. doi: 10.1038/nature06171
Silbernagel, M. J. (1969). Mexican strain of bean common mosaic virus. Phytopathology 59, 1809–1812.
Singh, S. P., Munoz, C. G., and Teran, H. (2001). Registration of common bacterial blight resistant dry bean germplasm VAX 1, VAX 3, and VAX 4. Crop Sci. 41, 275–276. doi: 10.2135/cropsci2001.411275x
Singh, S. P., Terán, H., Lema, M., Webster, D. M., Strausbaugh, C. A., Miklas, P. N., et al. (2007). Seventy-five years of breeding dry bean of the western USA. Crop Sci. 47, 981–989. doi: 10.2135/cropsci2006.05.0322
Skotland, C. B., and Burke, D. W. (1961). A seed-borne bean virus of wide host-range. Phytopathology 51, 565–568.
Soler-Garzón, A., McClean, P. E., and Miklas, P. N. (2021). Genome-wide association mapping of bc-1 and bc-u reveals candidate genes and new adjustments to the host-pathogen interaction for resistance to Bean common mosaic necrosis virus in common bean. Front. Plant Sci. 12:699569. doi: 10.3389/fpls.2021.699569
Song, Q., Jia, G., Hyten, D. L., Jenkins, J., Hwang, E.-Y., Schroeder, S. G., et al. (2015). SNP assay development for linkage map construction, anchoring whole genome sequence and other genetic and genomic applications in common bean. G3 Genes| Genomes| Genet. 5, 2285–2290. doi: 10.1534/g3.115.020594
Untergasser, A., Cutcutache, I., Koressaar, T., Ye, J., Faircloth, B. C., Remm, M., et al. (2012). Primer3–new capabilities and interfaces. Nucleic Acids Res. 40:e115. doi: 10.1093/nar/gks596
Vallejos, C. E., Astua-Monge, G., Jones, V., Plyler, T. R., Sakiyama, N. S., and Mackenzie, S. A. (2006). Genetic and molecular characterization of the I locus of Phaseolus vulgaris. Genetics 172, 1229–1242.
Viteri, D. M., Cregan, P. B., Trapp, J. J., Miklas, P. N., and Singh, S. P. (2014). A new common bacterial blight resistance QTL in VAX 1 common bean and interaction of the new QTL, SAP6, and SU91 with bacterial strains. Crop Sci. 54, 1598–1608. doi: 10.2135/cropsci2014.01.0008
Wang, J., Chuang, K., Ahluwalia, M., Patel, S., Umblas, N., Mirel, D., et al. (2005). High-throughput SNP genotyping by single-tube PCR with Tm-shift primers. Biotechniques 39, 885–893. doi: 10.2144/000112028
Wang, S. B., Feng, J. Y., Ren, W. L., Huang, B., Zhou, L., Wen, Y. J., et al. (2016). Improving power and accuracy of genome-wide association studies via a multi-locus mixed linear model methodology. Sci. Rep. 6:19444. doi: 10.1038/srep19444
Wen, Y.-J., Zhang, H., Ni, Y.-L., Huang, B., Zhang, J., Feng, J.-Y., et al. (2018). Methodological implementation of mixed linear models in multi-locus genome-wide association studies. Brief. Bioinform. 19, 700–712. doi: 10.1093/bib/bbw145
Worrall, E. A., Wamonje, F. O., Mukeshimana, G., Harvey, J. J. W., Carr, J. P., and Mitter, N. (2015). Bean common mosaic virus and bean common mosaic necrosis virus: relationships, biology, and prospects for control. Adv. Virus Res. 93, 1–46. doi: 10.1016/bs.aivir.2015.04.002
Xin, Z., Velten, J. P., Oliver, M. J., and Burke, J. J. (2003). High-throughput DNA extraction method suitable for PCR. Biotechniques 34, 820–826. doi: 10.2144/03344rr04
Yin, L. (2016). CMplot. Available online at: https://github.com/YinLiLin/CMplot (accessed March 17, 2021).
Keywords: genome-wide association study, gene homology, host-pathogen interaction, Phaseolus vulgaris, potyvirus, marker-assisted selection
Citation: Soler-Garzón A, McClean PE and Miklas PN (2021) Coding Mutations in Vacuolar Protein-Sorting 4 AAA+ ATPase Endosomal Sorting Complexes Required for Transport Protein Homologs Underlie bc-2 and New bc-4 Gene Conferring Resistance to Bean Common Mosaic Virus in Common Bean. Front. Plant Sci. 12:769247. doi: 10.3389/fpls.2021.769247
Received: 01 September 2021; Accepted: 02 November 2021;
Published: 13 December 2021.
Edited by:
Zuhua He, Center for Excellence in Molecular Plant Sciences, Chinese Academy of Sciences (CAS), ChinaReviewed by:
Alexander Karasev, University of Idaho, United StatesPrakit Somta, Kasetsart University, Thailand
Copyright © 2021 Soler-Garzón, McClean and Miklas. This is an open-access article distributed under the terms of the Creative Commons Attribution License (CC BY). The use, distribution or reproduction in other forums is permitted, provided the original author(s) and the copyright owner(s) are credited and that the original publication in this journal is cited, in accordance with accepted academic practice. No use, distribution or reproduction is permitted which does not comply with these terms.
*Correspondence: Phillip N. Miklas, phil.miklas@usda.gov; orcid.org/0000-0002-6636-454X