- 1Instituto de Agrobiotecnología y Biología Molecular, UEDD INTA-CONICET, Hurlingham, Argentina
- 2Laboratorio de Agrobiotecnología, IPADS (INTA – CONICET), Balcarce, Argentina
- 3Facultad de Ciencias Agrarias, Universidad Nacional de Mar del Plata, Balcarce, Argentina
- 4Facultad de Ciencias Exactas y Naturales, Universidad de Buenos Aires, Buenos Aires, Argentina
Potato (Solanum tuberosum L.) is a crop of world importance that produces tubers of high nutritional quality. It is considered one of the promising crops to overcome the challenges of poverty and hunger worldwide. However, it is exposed to different biotic and abiotic stresses that can cause significant losses in production. Thus, potato is a candidate of special relevance for improvements through conventional breeding and biotechnology. Since conventional breeding is time-consuming and challenging, genetic engineering provides the opportunity to introduce/switch-off genes of interest without altering the allelic combination that characterize successful commercial cultivars or to induce targeted sequence modifications by New Breeding Techniques. There is a variety of methods for potato improvement via genetic transformation. Most of them incorporate genes of interest into the nuclear genome; nevertheless, the development of plastid transformation protocols broadened the available approaches for potato breeding. Although all methods have their advantages and disadvantages, Agrobacterium-mediated transformation is the most used approach. Alternative methods such as particle bombardment, protoplast transfection with polyethylene glycol and microinjection are also effective. Independently of the DNA delivery approach, critical steps for a successful transformation are a rapid and efficient regeneration protocol and a selection system. Several critical factors affect the transformation efficiency: vector type, insert size, Agrobacterium strain, explant type, composition of the subculture media, selective agent, among others. Moreover, transient or stable transformation, constitutive or inducible promoters, antibiotic/herbicide resistance or marker-free strategies can be considered. Although great efforts have been made to optimize all the parameters, potato transformation protocols are highly genotype-dependent. Genome editing technologies provide promising tools in genetic engineering allowing precise modification of targeted sequences. Interestingly, transient expression of genome editing components in potato protoplasts was reported to generate edited plants without the integration of any foreign DNA, which is a valuable aspect from both a scientific and a regulatory perspective. In this review, current challenges and opportunities concerning potato genetic engineering strategies developed to date are discussed. We describe their critical parameters and constrains, and the potential application of the available tools for functional analyses or biotechnological purposes. Public concerns and safety issues are also addressed.
Importance of Biotechnology Application in Potato
Potato (Solanum tuberosum L.) is a worldwide important crop plant that produces high nutritional quality tubers. It is the fourth most important staple crop (after wheat, corn, and rice) in terms of production and demand, with around 378 million tons produced annually (Campos and Ortiz, 2020). The tuber, which serves the plant as a storage organ and as a vegetative propagation system, is an excellent source of complex carbohydrates, proteins, and vitamins (Banerjee et al., 2006; Barrell et al., 2013). Therefore, it is considered one of the promising crops to overcome the challenges of poverty and hunger worldwide (Bakhsh, 2020). In addition, potato is largely used in industry to make processed food products, alcohol, animal feed, and for bioenergy production substrates like biofuel (Ahmed et al., 2018). Moreover, given the physicochemical properties of refined starch it is used as a thickening and stabilizing agent in food, and as a raw material in paper, textile, cosmetic, adhesive, and plastics industries (Craze et al., 2018). Altogether, the importance of potato cultivation lies not only in its use as a basic food crop but also as a source of compounds of interest. While wide climate adaptability has facilitated potato to be extensively distributed in the world, several factors like climate change, industrialization, and urbanization have overburdened the existing agriculture lands and food resources (Badami and Ramankutty, 2015; Tiwari et al., 2020). Moreover, potato production faces important challenges such as biotic (viruses, bacteria, fungal, and insect pests) and abiotic stresses (drought, flooding, salinity, heat, and cold), and postharvest problems (accumulation of reducing sugars during cold storage, injury-induced enzymatic browning). Due to its large negative impacts on yield and tuber quality, improving resistance to disease and pests and/or abiotic factors, as well as quality traits is of significant economic importance (Rooke and Lindsey, 1998; Halterman et al., 2016).
Potato is a relevant candidate for improvement through conventional breeding and biotechnology. However, given that most of cultivated potatoes have tetraploid genomes, a high level of genetic heterozygosity and a narrow genetic base, conventional breeding is time-consuming and challenging (Chakravarty et al., 2007).
Therefore, genetic engineering provides the opportunity to introduce genes of interest without altering the allelic combinations that characterize successful commercial cultivars. Moreover, transgene-mediated post-transcriptional gene silencing using RNA interference (RNAi) is a strategy used to inactivate one or several genes and it has also proven its potential to obtain a desirable phenotype for potato improvement (Bradshaw, 2021). More recently, the so-called New Breeding Techniques (NBTs), which include genome editing, enabled the induction of targeted sequence modifications within a genome reducing off target effects.
Interestingly, several of potato genotypes are amenable to propagation through tissue culture enabling the application of available biotechnology techniques (Han et al., 2015; Halterman et al., 2016; Nadakuduti et al., 2018). Through genetic engineering approaches, different traits related to stress tolerance, nutritional quality, and/or compounds of interest were incorporated. Genetically engineered (GE) potato plants, obtained by classical genetic transformation strategies or genome editing tools, with increased resistance to insects, bacteria, fungi, viruses, herbicides, abiotic factors, and/or improved nutritional and post-harvest quality were developed. Also, the production of compounds such as biopharmaceuticals, biopolymers, polyhydroxyalkanoates, spiderweb, freeze-thaw-stable potato starch, increased synthesis of lipids and even vaccines, and human proteins were reported (for reviews see Pribylova et al., 2006; Halterman et al., 2016; Hameed et al., 2018; Rakosy-Tican and Molnar, 2021). Otherwise, the elimination of antinutritional or allergenic molecules such as alkaloids, glycoproteins, acrylamide, or patatin was also addressed (Zaheer and Akhtar, 2016; Hameed et al., 2018).
The large number of scientific reports and the diversity of engineered traits successfully transferred to potato reflect the ease of this crop to be biotechnologically improved. In this regard, the availability of potato genome sequence (Xu et al., 20111) has facilitated the development of comparative genomic analyses and functional studies of candidate genes to improve several important traits.
In summary, genetic engineering methods are essential tools in plant science research, not only for applied but also for basic purposes, providing invaluable tools for the characterization and validation of gene function to better understand plant physiology and development. In this review, several biotechnological strategies applied to potato are discussed, from classic genetic transformation to genome editing.
Strategies for Potato Transformation
Successful plant transformation requires a suitable DNA delivery system, an efficient plant regeneration protocol and an optimized selection method to recognize transgenic cells (Bakhsh, 2020). There is a wide variety of DNA delivery systems for the genetic transformation of potato. Most of them incorporate the genes of interest into the nuclear genome. However, in the recent years, the development of plastid transformation protocols has been reported, broadening the available approaches. A comprehensive view of the evidence reviewed, suggests that although all systems have their advantages and disadvantages, Agrobacterium tumefaciens-mediated transformation is the most used. Potato was one of the first crops to be successfully transformed with this method (Ooms et al., 1986) and since then, numerous genetic transformation protocols based on A. tumefaciens have been developed. However, effective alternative direct DNA uptake methods have been also reported (Halterman et al., 2016). The A. tumefaciens method is more efficient than others and results in a higher proportion of plants with a single transgene copy insertion, minimizing the potential side effects (Gelvin, 2017). Since the modification of traits based on multiple genes via A. tumefaciens is time-consuming and laborious (Romano et al., 2001), particle bombardment is the method of choice for transforming potato with several genes (Zhang et al., 2020). However, the stacking of transgenes via A. tumefaciens was successfully achieved through different approaches: crossing of individual transgenic plants, re-transformation with independent genetic constructs, use of gene combining constructs, co-transformation with double constructs, and the use of polyprotein systems, among others (Romano et al., 2001; Asurmendi, 2002; Rivero et al., 2012; Fernandez Bidondo et al., 2019). In addition, another biotechnological approach successfully applied in potatoes for stacking resistance genes against viruses is RNAi technology (Missiou et al., 2004; Chung et al., 2013; Hameed et al., 2017). It is important to note that RNAi approach has been successfully employed in a great number of reports for disease and pest resistance (Waterhouse et al., 1998; Missiou et al., 2004; Chung et al., 2013; Dinh et al., 2015; Sun et al., 2016; Hameed et al., 2017), improved processing quality (Rommens et al., 2006; Llorente et al., 2010; Chawla et al., 2012; Zhu et al., 2016; Hameed et al., 2018), and improved nutritional value (Van Eck et al., 2007; Itkin et al., 2013; Sawai et al., 2014). In fact, the effectiveness of this technology is illustrated in the several commercially approved GE potatoes based on RNAi (ISAAA, 20212).
Generation of transgenic potato using other bacteria species, such as Sinorhizobium meliloti, Rhizobium sp. NGR234, Mesorhizobium loti, Sinorhizobium adhaerens, and Agrobacterium rhizogenes, has also been reported (Wendt et al., 2011, 2012; Butler et al., 2020). A. rhizogenes is often applied for obtaining transgenic roots faster than using A. tumefaciens, since plant regeneration is not needed, representing an alternative tool for potato functional studies and characterization of root genes (Fernández-Piñán et al., 2019).
Lastly, DNA delivery can be achieved by direct DNA uptake, like microinjection, particle bombardment, protoplast transfection with polyethylene glycol (PEG), and protoplasts electroporation (Fehér et al., 1991; Romano et al., 2001, 2003; Valkov et al., 2011). These methodologies would facilitate the coordinated integration and expression of several genes and the manipulation of metabolic pathways in potato (Craig et al., 2005). Even though these methods are effective (Weiland, 2003), disadvantages such as complex integration patterns, high copy numbers, transgene rearrangements, and gene silencing have also been reported (Sawahel, 2002). New methodologies for genetic engineering such as nanoparticle-mediated approaches for passive delivery of genetic cargo were successfully employed in potato (Abdel-Razik et al., 2017).
Once DNA was delivered, transient or stable transformation can occur. Virus induced gene silencing is an attractive approach to generate transient loss-of-function assays to assess the role of genes in a short time, as an alternative to stable transformation (Brigneti et al., 2004; Faivre-Rampant et al., 2004; Dobnik et al., 2016). In addition, A. tumefaciens-mediated infiltration without involving a viral based system has been reported to study gene function or protein localization in potato (Bhaskar et al., 2009). Although there are some reports using these tools in potato, their applications are far from routine and currently more limited to model plant species.
Other strategies involving DNA delivery to organelles have been employed. Plastid transformation has some potential advantages in comparison to nuclear transformation for both plant breeding and molecular farming. In contrast to the random nature of nuclear transformation, the gene of interest is integrated into the plastome through homologous recombination, which avoids negative effects associated with transgene insertion in transcriptionally silent regions or with the disruption of host genes or regulatory regions (Gelvin, 2017). Moreover, mechanisms of gene silencing are not present in plastids, so expression of the transgene is stable in progeny of transplastomic plants (Sidorov et al., 1999; Thanh et al., 2005). In addition, other advantages include high expression levels of transgenes and protein accumulation, the opportunity of expressing several genes in operons and the inherent confinement of transgenes and recombinant products in plastids (Valkov et al., 2011, 2014; Segretin et al., 2012). The availability of the complete chloroplast genome sequence of S. tuberosum in 2005, allowed the construction of species-specific vectors by increasing homology and the improvement of potato plastid transformation efficiency (Scotti et al., 2011; Valkov et al., 2011). Even though it has attractive advantages and potential applications in potato biotechnology, low transformation frequencies and the reduced levels of transgene expression registered in tubers limit a wider use of potato plastid transformation (Sidorov et al., 1999; Thanh et al., 2005; Segretin et al., 2012).
In sum, DNA delivery methods based on biological vectors –such as bacteria or virus–, physical agents –like electroporation, microinjection, or particle bombardment–, and chemical agents –such as PEG– are available for potato transformation. Even direct strategies are effective A. tumefaciens-mediated transformation is the most established and preferred approach for potato. The choice of the potato transformation strategy is based on the experimental objective, such as transient or stable integration and/or nuclear or plastid expression, and will determine the most suitable DNA delivery method.
Main Parameters Influencing Transformation Efficiency
There is not a consensus in the definition of transformation efficiency in the bibliography, but we consider the most suitable estimation as follows: Transformation efficiency (%) = (Total number of PCR positive lines/Total number of inoculated explants) × 100. It is important to note that to calculate the exact efficiency only independent lines must be considered. According to the reviewed reports the most relevant parameters that affect potato transformation efficiency are detailed below.
Potato Genotypes
There are more than 4,000 landraces of native potatoes, mostly found in the Andean Region and over 180 wild potato species (source: International Potato Center). Despite the great number of potato varieties available, few cultivars are commercialized, chosen for their viability to be marketed and stored (Yang et al., 2015). Cultivars ‘Desiree’ and ‘Bintje’ were early favorites for transformation assays but nowadays, most potato cultivars are amenable to tissue culture and nearly all commercially important varieties can be successfully transformed with modifications of the standard protocols (Vinterhalter, 2008; Han et al., 2015; Halterman et al., 2016; Nadakuduti et al., 2018; Bruce and Shoup Rupp, 2019; Kaur et al., 2020). In 1988, it was reported the development of a genotype-independent method to transform four potato cultivars (‘Bintje’, ‘Berolina’, ‘Desiree’, and ‘Russet Burbank’) using leaf discs (De Block, 1988). However, subsequent evidence showed that the transformation efficiency is dependent on genotypes. There are several reports comparing the regeneration and transformation efficiencies of different genotypes using the same protocol and the results indicate that the effectiveness in obtaining transgenic plants is variable (Conner et al., 1992; Dale and Hampson, 1995; Kumar et al., 1995; Heeres et al., 2002; Han et al., 2015; Bakhsh, 2020). Moreover, Heeres et al. (2002) determined that regeneration and transformation efficiency are two different genetically controlled factors. So, for a given genotype, the success of transformation depends on several critical factors including vector, Agrobacterium strain, infection time, mode of injury, pre-culture period, cocultivation time, explant type, composition of the subculture media, selection markers, among others (Kaur and Devi, 2019; Bakhsh, 2020). Thus, standard protocols can be considered to transform a new genotype but then all parameters should be optimized to achieve adequate efficiency.
Agrobacterium tumefaciens Strains
Once the use of a bacterial delivery method has been defined, the next parameter to determine is the strain to use since it affects the efficiency of the genetic transformation. In this sense, LBA4404 A. tumefaciens strain is the most used in potato transformation protocols (Stiekema et al., 1988; Tavazza et al., 1989; Trujillo et al., 2001; Heeres et al., 2002; Bakhsh, 2020; Mollika et al., 2020). However, alternative strains such as EHA105, GV2260, GV3101, and C58C1 have also been successfully used (Kumar et al., 1995; Banerjee et al., 2006; Han et al., 2015; Ahmed et al., 2018; Craze et al., 2018; Bruce and Shoup Rupp, 2019; Décima Oneto et al., 2020). Despite the existence of numerous reports, there is not a clear association between the strain and the genotype to be transformed; this means that for a particular genotype the most suitable strain must be experimentally tested.
Once the strain was selected there are three principal factors that affect transformation efficiency: the optical density of the bacterial suspension, the addition of a centrifugation step of the bacterial culture before inoculation and the length of the co-cultivation period. Considering the evidence reviewed, it seems that the optimal optical density values are between 0.5 and 0.8, depending on the construct and the growth medium. Some protocols indicate that centrifugation of the bacterial culture before inoculation may affect the viability of the bacteria, causing a drastic reduction of transformation efficiency (Beaujean et al., 1998; Banerjee et al., 2006; Décima Oneto et al., 2020). Co-cultivation period should be long enough to enable proper T-DNA transfer, but prolonged periods should be avoided to reduce tissue damage and somaclonal variation. Therefore, the optimal time is between 24 and 96 h, being 48 h the most reported co-cultivation period (Millam, 2006). Nonetheless, for each genotype is necessary to determine empirically not only the optimal strain but also the co-cultivation conditions to obtain the best transformation efficiency.
Vectors
Another important choice is the vector to employ based on the transformation strategy selected. The type, the size, the regulatory elements, the selectable marker gene, the cloning efficiency, the cost and the availability, among other factors, must be considered.
The type and the size of the vector are directly related to the transformation strategy selected, mainly whether a shuttle (see Hellens et al., 2000) or a not binary vector is necessary. Regarding the regulatory elements, the constitutive and ubiquitous CaMV35S promoter is commonly used to express transgenes in potato; however, there are many reports of promoters successfully employed for specific objectives (Yevtushenko et al., 2004; Pino et al., 2007; Li et al., 2015; Nahirñak et al., 2019). Moreover, regarding a cisgenic approach, well characterized tissue-specific or inducible promoters have been reported from potato (Martini et al., 1993; Naumkina et al., 2007; Almasia et al., 2010). Another point to consider in the vector design is to avoid repeated regulatory sequences to prevent possible silencing effects.
The selectable markers generally used to identify transformed plant cells are genes encoding resistance to antibiotics or herbicides (Bruce and Shoup Rupp, 2019). The neomycin phosphotransferase II (nptII) gene, which confers resistance to the antibiotic kanamycin, is the most used in potato transformation protocols (Barrell et al., 2013). Barrell et al. (2002) evaluated the efficiency of different selectable markers using identical vectors for potato transformation. The effectiveness of recovery of transgenic lines was ranked as follows: kanamycin resistance > hygromycin resistance > phosphinothricin resistance > phleomycin resistance > methotrexate resistance (Barrell et al., 2002).
Other markers have been reported for potato transformation that do not involve the use of herbicides or antibiotics and use xylose or galactose as selective agents instead (Haldrup et al., 1998; Joersbo et al., 2003). These systems offer alternatives to the conventional ones, although their use requires further optimization. Visual markers genes such as glucuronidase, luciferase and green fluorescent protein have also been adopted, although to a lesser extent (Sidorov et al., 1999; Verhees et al., 2002; Rakosy-Tican et al., 2007). Alternatively, marker-free transformation of potato has also been reported. This approach is based on the recovery of transformed plants by PCR screening of plants regenerated without the use of a selection system (De Vetten et al., 2003; Ahmad et al., 2008). However, this approach resulted in the recovery of transgenic potato lines at low frequencies, with most of lines displaying insertions of undesirable vector backbone sequences and only a few lines containing the desirable single T-DNA insertion, which is another important criterion for commercialization of GE crops (Kondrák et al., 2006).
Concerning A. tumefaciens binary vectors, the arrangement of expression cassettes in the plasmid should also be contemplated. The selectable/screenable markers should be near the left border to facilitate the selection of events containing the entire cassette since the transference of the T-DNA is directed from right border to left border. Also, the inwards opposite orientation of the cassettes should be avoided preventing possible silencing effects.
Finally, it is important to check the vector through DNA sequencing before starting the transformation protocol.
Explants
For a genetic transformation system to be effective, it is essential to develop a rapid and efficient regeneration protocol. Leaves, stems, tubers, petiole, protoplasts, and micro-tubers have been used as explants to develop transgenic potato lines.
The source of explant tissue for potato transformation is frequently derived from in vitro plants (Barrell et al., 2013). The main advantage of using them is the supply of uniform and pathogen-free material for transformation (Newell et al., 1991). In vitro micropropagation of shoot cultures makes possible the accessibility of healthy and vigorously growing plant material throughout the year (Visser, 1991). Since it is sterile and already acclimatized to grow under in vitro conditions, surface sterilization of the plant tissue is not required, reducing both handling time and the possibility of contamination, and avoiding plant stress due to chemical treatments (Conner et al., 1992; Kumar, 1995).
Leaves and stem internodes from in vitro plants are the most widely used explants since they are readily available and easy to use. It has been reported that stem pieces are relatively robust and can be handled easily in larger numbers comparing to leaf explants, which are delicate and can be injured during the manipulation reducing the frequency of transformation and regeneration (De Block, 1988; Newell et al., 1991).
The main disadvantage of employing leaf and stem explants in transformation assays is the somaclonal variation which can occur in the callus phase (Visser, 1991). The use of tubers is advantageous because the possibility of somaclonal variation is reduced (Ishida et al., 1989). In vitro grown microtubers have several advantages over soil-grown tubers since they are derived from virus-free, aseptically grown potato shoots, they can be produced conveniently at any time in large quantities, and they take up less storage (Kumar, 1995).
It is important to note that a critical factor influencing the frequency of callus and regeneration is the physiological state of the starting material (Chakravarty and Wang-Pruski, 2010). To ensure a successful transformation, the selection of young and healthy explants from stock plant is preferred while damaged tissue will negatively affect regeneration potential (Ahmed et al., 2018; Craze et al., 2018). Moreover, the size of the explant should be large enough to resist Agrobacterium co-cultivation or biolistic bombardment without losing moisture during transformation procedures. Frequently the explant chosen for transformation depends on the cultivar or is determined by the experience of each laboratory (Barrell et al., 2013).
In conclusion, there are many options regarding tissue amenable for potato transformation, in any case it must be a healthy material, sterile, appropriate for manipulation and with regenerative capacity to obtain good and reproducible results.
Tissue Culture Media
Most protocols employ a two-step regeneration procedure, with a callus induction step followed by a shoot growth step. In particular, the de-differentiation and redifferentiation of explants is one of the main points of an effective plant transformation (Zhang et al., 2020). There are many types of plant tissue culture media, most based on Murashige and Skoog’s MS Media (Murashige and Skoog, 1962). MS media contains the major salts, a variety of minor salts, sucrose, vitamins, and plant growth regulators, which include cytokinins, auxins, gibberellins, abscisic acid, and ethylene (for details about regulators used in potato tissue culture see Bruce and Shoup Rupp, 2019). The callus induction stage is often facilitated by treatment of explants with zeatin or zeatin riboside with low levels of auxin, while the shoot induction stage often has a reduction of zeatin and auxin, plus the addition of gibberellin to stimulate shoot outgrowth. Regeneration rates per explant are usually high and the first shoots appear after 4–6 weeks (Millam, 2006), depending on the genotype used. For each potato genotype, it is necessary to adjust and determine the ratio of growth regulators most suitable for dedifferentiation and form callus, which also depends on the explant used (Zhang et al., 2020). Furthermore, another factor that influences the different transformation steps is light types, intensity and photoperiod (Gelaye, 2014).
On the other hand, the success of genetic transformation relies not only on the DNA delivery approach and a rapid and effective regeneration protocol, but also on the selection system to identify the transgenic cells. An efficient selection system results from a delicate balance between the inhibition of growth of wild-type cells and the preservation of the regeneration capacity of transformed cells. It is important to determine empirically the lowest level of the selective agent that prevent the development of non-transformed cells under selective conditions to reduce the recovery of false positives (also named “escapes” since they have escaped the selection process). Moreover, if the selection pressure is too high it may result in false negatives because of the loss of transformed plants (Barrell et al., 2002). Even though several selection strategies have been reported for potato transformation, kanamycin resistance is the most used selectable system and was shown to be more effective in the rapid recovery of large numbers of independently derived transgenic lines (Barrell et al., 2002). It has also a history of safe use in 122 transgenic crops approved for cultivation, food, or feed (ISAAA, 2021).
The freshness of the media and growth regulators is relevant so they must be replaced regularly. Moreover, experiments controls are very important since they allow to identify problems, for example, non-inoculated pieces of leaves placed with a selection agent to demonstrate their functionality, and without selection to ensure that the shoots can regenerate. The experience acquired to identify the putative transgenics trough visual selection is another main point in potato transformation protocols, for example, the formation of roots in the selective medium is an excellent indicator of the existence of transformed tissues.
As it was already mentioned, one of the main constrain in potato genetic engineering, is the somaclonal variation, which consists of phenotypic changes observed when plants are regenerated from cultured somatic cells. The observed phenotypic variations among somaclonal potato lines involve physiological, epigenetic, and/or genetic changes (Barrell et al., 2013). Maintaining potato lines in culture for prolonged periods can result in these variations (Craze et al., 2018). Genotype, explant origin and the culture conditions are others critical variables contributing to somaclonal variation (Meiyalaghan et al., 2011). The success of gene transfer techniques also depends on minimizing these variations (Stiekema et al., 1988), especially by decreasing the callus induction stage (Millam, 2006) or the period of in vitro culture in general (Han et al., 2015).
As it is shown in Table 1 even though there are many potato transformation protocols reported for different genotypes most of them are based on A. tumefaciens, employ nptII as selective marker, and use leaves and stems as tissue explant.
Genome Editing in Potato
In recent years, genome editing technologies have emerged as novel biotechnological approaches for crop breeding and have received considerable attention due to their simplicity and accuracy in introducing targeted modifications that result in desired traits (Arora and Narula, 2017; Baltes et al., 2017; Scheben et al., 2017; Gao, 2018; Chen et al., 2019; Zhu et al., 2020). Genome editing is based on the employment of site-specific nucleases (SSNs) to induce modifications at specific genomic sites (Gao, 2021). SSNs such as Zinc Finger Nucleases (ZFNs), Transcription Activator-Like Effector Nucleases (TALENs), and the more recently developed Clustered Regularly Interspaced Short Palindromic Repeats (CRISPR) and CRISPR associated proteins (CRISPR/Cas) introduce double stranded breaks (DSBs) at a specific target site in the host genome, which led to targeted modifications via endogenous DNA repair mechanisms (Schmidt et al., 2019). In somatic plant cells, DSBs are mainly repaired by the error-prone non-homologous end joining (NHEJ) pathway, which occasionally results in the introduction of small insertions or deletions at the repaired site, producing the disruption of specific genes and/or regulatory regions of the plant genome (Puchta, 2005). Although less frequent than NHEJ, the homology-directed repair (HDR) pathway can be triggered in plant cells to repair the induced DSBs (Schmidt et al., 2019). A requirement for the HDR pathway to take place is the availability of a homologous DNA fragment, which can be exploited for targeted integration of sequences of interest into the plant genome (Huang and Puchta, 2019).
The availability of potato genome sequence and the development of highly efficient transformation systems make potato a perfect candidate for the application of genome editing technologies to improve important traits leading to a more sustainable potato production (Hameed et al., 2018; Nadakuduti et al., 2018). Initial genome editing platforms, i.e., ZFN and TALEN, were developed through the fusion of a programmable DNA-binding domain (Zinc Fingers and Transcription Activator-Like Effectors for ZFN and TALEN, respectively) and the catalytic domain of the type II restriction enzyme FokI (Voytas, 2013). Both platforms are based on protein-DNA interactions to recognize the target sequences, which represented a drawback in the widespread adoption of these technologies, due to the complexity in the design of the DNA-binding domains to recognize new sequences, their synthesis and activity validation (Isalan, 2012; Doudna and Charpentier, 2014). Nevertheless, TALEN technology has been successfully used for potato genome editing in several applications, including both basic potato research and the improvement of important traits (Table 2).
In contrast to ZFN and TALEN, the CRISPR/Cas systems utilize a short and programmable guide RNA molecule to recognize the target site, which represent a more simple, versatile and efficient platform to mediate genome editing (Chen et al., 2019). In particular, the components of the CRISPR/Cas9 system from Streptococcus pyogenes were the first to be adapted as a programmable genome-editing tool, consisting of a Cas9 nuclease directed by an easily re-programmable single guide RNA (sgRNA) (Jinek et al., 2012). Cas9 can induce DSBs at specific sites determined by both base complementary between the sgRNA and the target sequence and the presence of a 5′-NGG-3′ motif adjacent to the complementary region in the target sequence (PAM, protospacer adjacent motif) (Jiang and Doudna, 2017). The simplicity of CRISPR/Cas9 system made it the most widely applied technology for potato genome editing, as well as for the rest of plant species (Gao, 2021). Since the first report on a CRISPR/Cas9-mediated genome editing in potato in 2015 (Wang et al., 2015), this system has been applied in number of basic research studies and in improving important traits in potato, including its nutritional quality, modification of tuber starch composition, post-harvest quality enhancement, biotic stress tolerance, and elimination of reproductive self-incompatibility. Such applications have been extensively reviewed in recently published articles (Hameed et al., 2018; Nadakuduti et al., 2018; Dev et al., 2021) and are summarized in Table 2, together with more recently published reports.
Genome Editing Reagents Delivery in Potato
Like in all plant species, delivery of the genome editing reagents in potato is based on previously established transformation methods (Ran et al., 2017). Therefore, A. tumefaciens-mediated transformation has been the most widely used approach to deliver either TALEN or CRISPR/Cas systems in potato (Table 2). Genomic integration of transgenes encoding the genome-editing reagents is effective in producing a sustained expression of the components that led to the intended modification of the target site(s).
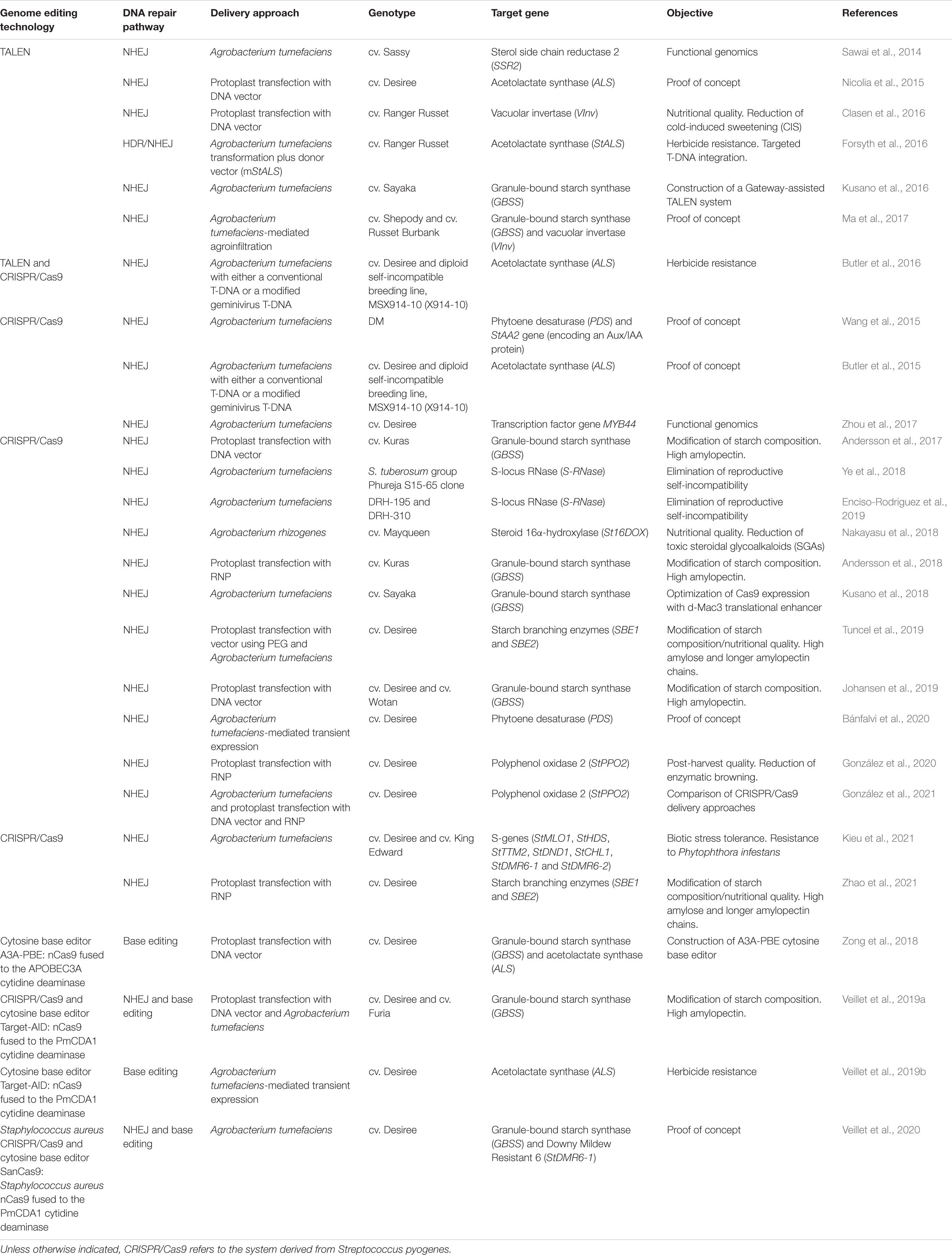
Table 2. Applications of TALEN or CRISPR/Cas systems for both basic research and agronomic/agroindustrial traits improvement in potato.
Transient expression of the genome editing reagents is a promising alternative delivery method to obtain transgene-free edited plants (Chen et al., 2019). This is of a special importance in a vegetatively propagated and highly heterozygous crop like potato since the elimination of the transgenes by crossing or self-crossing is not suitable. Transient expression of genome editing reagents has been achieved in potato using different strategies. Bánfalvi et al. (2020) have obtained edited potato plants of the tetraploid cultivar Desiree trough transient expression of a CRISPR/Cas9-coding vector upon A. tumefaciens-mediated infection by using a selection protocol consisting in 3 days-kanamycin treatments. Edited lines (2–10%) were screened to check the binary vector incorporation in their genomes by PCR amplification of specific vector fragments, with negative results in all analyzed cases (Bánfalvi et al., 2020). Nicolia et al. (2015) had developed a pipeline based on the transient expression of TALEN in potato by protoplast transfection with DNA vector followed by whole plant regeneration without selective agents, obtaining 10% of the regenerated lines with mutations in the expected region (Nicolia et al., 2015). In addition to the transient expression of DNA vectors, protoplasts allow the delivery of CRISPR/Cas9 components as pre-assembled ribonucleoprotein (RNP) complexes (Woo et al., 2015). This represents a promising alternative, with the potential of both reducing to a minimum (or even eliminating) the possibility of foreign DNA insertions in the plant genome and minimizing the probabilities of unwanted off-target effects. Such an alternative may result of great interest in the application of this technology to obtain potato commercial cultivars, since the obtained edited plants could be indistinguishable from those containing naturally or conventionally induced mutations (Kumlehn et al., 2018). The first application of RNP complexes to obtain potato edited lines was reported by Andersson et al. (2018). Authors transfected protoplasts isolated from the cultivar Kuras, with two different types of RNP complexes targeting the GBSSI gene and obtained a frequency of 1–25% of regenerated edited lines, varying with the transfection conditions and the origin of RNP complexes (Andersson et al., 2018). Furthermore, for one transfection up to 3% of the total analyzed lines displayed mutations in all four alleles of the target gene and no DNA insertions. Since this first report, RNPs have been successfully used to mediate potato genome editing using the protoplasts transfection and regeneration system, with editing efficiencies ranging from 27 to 68% (González et al., 2020) and 52 to 72% (Zhao et al., 2021), varying with the targeted genes and the design of RNP components. Protoplasts provide a suitable platform for genome-editing reagents delivery and may in some cases represent even a more efficient strategy when compared to Agrobacterium-mediated transformation (González et al., 2021). Even though it represents a promising strategy to mediate transgene-free genome editing in potato, the use of RNP in protoplasts present some important aspects to consider. For instance, DNA traces remaining in the assembled RNPs may led to unintended foreign DNA insertions in the regenerated plants, with different frequencies (Andersson et al., 2018; González et al., 2021; Zhao et al., 2021). A possible origin of such traces is the DNA molecules employed for the in vitro transcription of sgRNAs, before RNP assembly. To solve this issue, the use of synthetically produced sgRNAs to obtain the RNP complexes completely eliminates the foreign DNA insertions (Andersson et al., 2018). On the other hand, regeneration of complete potato plants from protoplasts has been associated with somaclonal variation and chromosome instability (Barrell et al., 2013; Fossi et al., 2019). Therefore, the selection of strategies that enhance the number of edited lines after protoplasts regeneration, ensures a vast number of individuals to select the best candidates for further phenotypic analysis (Andersson et al., 2017).
New Clustered Regularly Interspaced Short Palindromic Repeats-Related Tools for Potato Genome Editing
Clustered Regularly Interspaced Short Palindromic Repeats/Cas systems have been rapidly expanding and new CRISPR-related tools have been created that greatly broaden the scopes of genome editing and/or made it more precise.
Base editing technology is based on the fusion of a catalytically inactive (or partially inactive) Cas nuclease, fused to a cytosine or adenosine deaminase domain capable to convert one base to another (Mishra et al., 2020). Cytosine base editors (CBEs) convert a C to a G, while adenosine base editors (ABEs) mediate the conversion of A to G. CBEs have been successfully used to mediate potato base-editing (Table 2). Moreover, CBE consisting in a Cas9 nickase (nCas9) fused to either a human (Zong et al., 2018) or a Petromyzon marinus cytidine deaminase (Veillet et al., 2019a,b) were reported to obtain loss-of-functions or gain-of-function mutations related to important traits in potato.
The use of CRISPR/Cas systems originated from other bacteria species than S. pyogenes provide new benefits, including but not limited to, the expansion of the putative target sites within a given genome via different PAM requirements and the generation of different cutting patterns to introduce the DSB (Huang and Puchta, 2021). In a proof-of-concept publication, Veillet et al. (2020) demonstrated that Cas9 nuclease derived from Staphylococcus aureus (SaCas9) is efficient in inducing both genome editing via the NHEJ repair pathway and precise base editing in potato. Since SaCas9 recognize a more complex PAM (5′-NNGRRT-3′) it could additionally represent a highly specific nuclease by limiting the off-target activity, particularly for highly conserved genomic sequences in polyploidy species, such as potato (Veillet et al., 2020). This and other CRISPR/Cas orthologs systems are available for potato genome editing and can expand the toolbox for trait improvement (Huang and Puchta, 2021).
The recently developed prime editing technology allows the creation of different types of genomic changes with high precision, which represents potentially a new technological breakthrough (Gao, 2021). Prime editors are composed of an nCas9 fused to a reverse transcriptase, guided to the target site via a modified prime-editing guide RNA. In addition to define the target site, the prime-editing guide RNA serves as a template for reverse transcription, carrying a primer binding site and a sequence to be copied in the genome at its 3′end. Once nCas9 nicks the DNA, the released ssDNA can hybridize with the primer binding site and be used as a primer for the reverse transcriptase, which transfers the sequence encoded in the prime-editing guide RNA to the DNA strand (Huang and Puchta, 2021). The new sequence is incorporated later into the target site, through the DNA repair. Although prime editing is still inefficient in plant cells and no reports with this technology has been published in potato so far, its applications in a few crop species and its continuous optimization rise high hopes to incorporate it to the potato genome editing toolbox (Lin et al., 2021).
Public Concerns About Genetically Engineered Potato
As we already stated in this review, genetic engineering includes genetic transformation and genome editing. Genetic transformation comprises the traditional tools for introduction of a gene of interest randomly integrated into plant genomes. Genome editing techniques have been developed as an alternative to introduce precise and predictable genome modifications into plants without adding foreign DNA. For a GE potato to become a market success it must be accepted by government regulatory system, producers, and consumers (Halterman et al., 2016).
Nowadays, most countries practicing commercial agriculture have established regulations, with different degrees of stringency, for field experimentation and later larger scale cultivation of GE crops. These regulations take into consideration food, feed, and environmental safety risks. Some countries have a process-oriented regulation and have established that the regulations that apply to genetically modified organism should be also applied to genome editing developments (European Union, New Zealand) (Nadakuduti et al., 2018). Other countries have pronounced in favor of regulating the varieties obtained by genome editing as conventional ones, if the developed varieties lack from any foreign genetic material (Argentina, United States, Brazil, etc.) (Feingold et al., 2018; Lema, 2019).
After more than 20 years of biosafety analysis of different GE potatoes, the main concern still regards the potential genetic flow between cultivated and wild relatives plants, particularly in the centers of origin. In most of the major potato producing countries, the chance of gene flow to interfertile species is virtually non-existent due to the lack of wild relatives. In South America, the Andean region is the center of origin of potato and represents a valuable source of diversity, being an important genetic resource. Even though intercrossings between cultivated potato and wild relatives have been reported, it is commonly restricted by reproductive barriers (Hawkes and Hjerting, 1969; Rabinowitz et al., 1990). Thus, the risk associated with a commercial released of GE potato in regions where interfertile species coexist should be evaluated case by case. In Argentina, an interspecific out-crossing trial between a S. tuberosum cv. Spunta transgenic line and the only wild relative species in the Pampean region that could possibly cross with it (Solanum chacoense), failed to detect any event of transgene transfer under natural field conditions (Bravo-Almonacid et al., 2012). In addition to safety, public concern also focuses on patents, plant breeders’ rights and the concentration of intellectual property in a small number of corporations (Haverkort et al., 2016). These are some points, together with the economic cost of risk assessment and regulatory framework process, that the political leadership should include in their schedule to facilitate the social access of these technologies to their farmers.
Regarding the producers, they are who probably see, more directly, the great benefits of the use of GE potatoes in their production. Recently, Bangladesh started a field trial of two late blight resistant transgenic potato lines, the success of which will allow 20% less yield loss and farmers will save around $ 12 million spent on fungicides (source PotatoPro3). In addition, the avoidance of agrochemical uses and its impact on carbon balance that may affect climate change will lead to important environmental benefits. Also, in Uganda, another transgenic variety that resists late blight infection without the use of fungicides is being evaluated. This would improve the safety of farmers and their families, and in particular the small farmers, who have limited access to fungicides, could reduce their production losses by up to 60% (Potato News Today, 2021). According to Ghislain et al. (2021) delaying adoption of this potato will lead to continued pesticide use and significant losses that will affect the most vulnerable farmers. On the other hand, the improvement of GE plant resistance to abiotic stresses such as salinity, drought, or temperature allows potato cultivation in less fertile agricultural lands (Hameed et al., 2018). Nevertheless, looking closely at the history of approved GE potato it can be noticed that producers may be reluctant to incorporate improved GE varieties because the industry fears mixing them with conventional ones and thus losing markets (Grafius and Douches, 2008; Haverkort et al., 2016; Tagliabue, 2018). This industry decision responds to a pre-judgment of consumers reactions, or to avoid negative publicity of their products. However, a science based potential risks and benefits communication campaign should be undertaken to comply with consumers’ right to be informed.
Consumer apprehension toward GE technology exists even though the cultivation area of these crops in 2020 was around 190 million hectares all over the world. According to Mullins et al. (2006), consumer concerns toward these technologies focuses on the public’s desire for unbiased information about potential risks. This leads us to think about the need to communicate clear, precise, and comprehensible information about GE technologies to the public, particularly showing the great advantages on its use. Public debate on the release of GE crops has led to questions regarding their environmental safety; however, it is important to clearly transmit that the deregulation of GE crops involves risk assessments and a complex regulatory framework. Concerning ecological compatibility of GE potato, many studies focused to address biosafety aspects and risk assessment did not find undesired ecological side-effects (Griffiths et al., 2000; Heuer et al., 2002; Rasche et al., 2006; Bravo-Almonacid et al., 2012; Fernandez Bidondo et al., 2019; van der Voet et al., 2019). In addition, as we mentioned before, GE potatoes resistant to biotic factors allow a radical reduction of pesticide applications, which are harmful to the environment and human health. Other advantage of GE potato directly relevant for consumers is the elimination of anti-nutritional or allergens to improve the nutrition quality. Recently, the potato variety Z6, engineered for low reducing sugars, low acrylamide potential, reduced black spot bruising and late blight protection, was deregulated in United States (source: USDA, 2021). It is interesting to discover if this GE potato with direct nutritional benefits will be well received by consumers. According to the international database, there are currently 51 events recorded on the potato list (ISAAA, 2021) and hundreds in the regulatory or pre-commercialization pipeline. The public concerns of consumers must be allayed with the information necessary to understand that GE potato are important for the sustainability of potato production, food security, income generation, and environmental protection. Therefore, it is worth taking a proactive approach to consumer apprehension in which all actors involved in the generation and adoption of these technologies provide that accurate information.
The Figure 1 summarizes the different strategies available for potato genetic engineering, including both genetic transformation and genome editing focusing on the common steps and the differences between them.
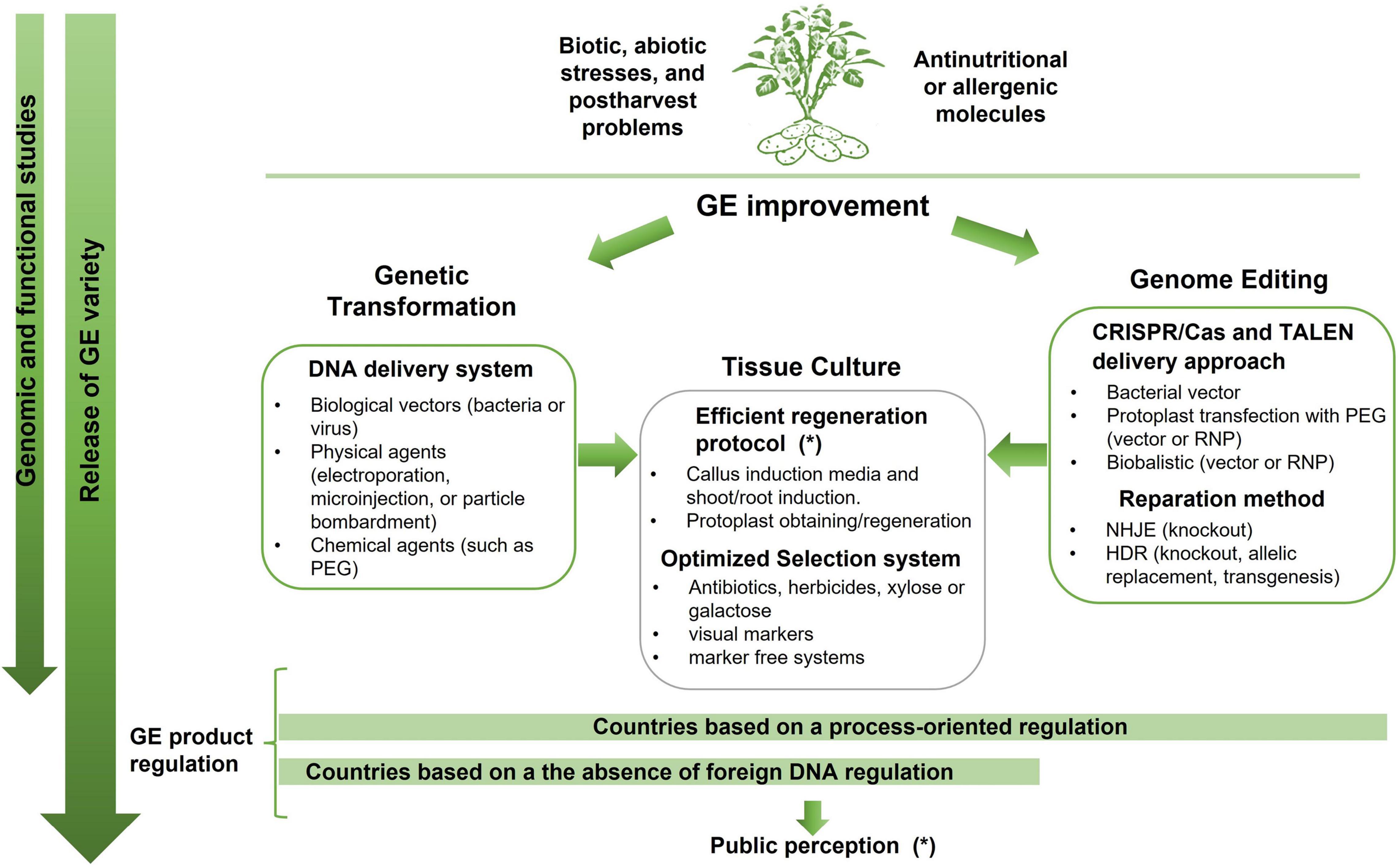
Figure 1. Simplified diagram of the strategies for genetic engineering improvements: genetic transformation or genome editing and common steps of vegetal culture to achieve it. Genetic transformation includes the traditional tools for introduction of a gene of interest randomly integrated into plant genomes. Genome editing techniques have been developed as an alternative to introduce precise and predictable genome modifications into plants to obtain desired traits. Those technologies were refinements of transformation whose final purpose is the obtaining a modified plant without foreign DNA. It is important to note that the regulations governing these developments vary from country to country. Some countries have a process-oriented regulation and apply the same regulation for all the GE products. Others consider the presence of foreign DNA as a mandatory requirement to be regulated and only in that case the product should be subjected to government regulations. Despite the differences between the classical genetic transformation strategies and the genome editing tools, both relies on tissue culture to regenerate and select GE plants. The symbol (*) represent the main constrains: the regeneration protocol for recalcitrant genotypes and the public perception regarding the release of GE varieties.
Future Perspectives and Concluding Remarks
Potato is relatively easy to improve by genetic modification and genome editing because it benefits from a good amenability to regenerate shoots from in vitro tissue and protoplast culture. However, difficulties due to recalcitrance to transformation for some genotypes remain given that the limited regeneration capability in vitro restricts the recovery of transformed lines. The regeneration step is often the biggest bottleneck in the transformation process and is one of the most important factors explaining the low success in obtaining GE plants from recalcitrant genotypes. The entire plant regeneration from transformed explants involves tissue-culture procedures that can be time-consuming and can result in undesirable somaclonal variation.
The development and application of new technologies to overcome these drawbacks, especially bypassing tissue culture methods, are invaluable. In the recent years, some strategies to improve the regeneration efficiency in several plant species have been reported. These approaches involve the overexpression of genes encoding developmental regulators that can potentially be applied to improve plant transformation technologies (Debernardi et al., 2020; Maher et al., 2020; Kong et al., 2020). Interestingly, Maher et al. (2020) showed that the ectopic expression of specific transcription factors in somatic cells has the potential to induce meristems avoiding the use of traditional tissue culture. They demonstrated the induction of de novo meristems and the consequently shoot regeneration in various plant species including potato. Debernardi et al. (2020) reported that the expression of one Growth-Regulating Factor (GRF4) and its cofactor (GIF1) substantially increases regeneration efficiency in both monocotyledonous and dicotyledonous species; they even developed a protocol inducing embryogenesis in the absence of cytokinins. Finally, Kong et al. (2020) showed that the overexpression of GRF5 enhances regeneration and genetic transformation in various crop species. These advances represent a promising tool to overcome the problems associated with low regeneration capacities of certain genotypes improving the transformation process. However, the constitutive expression of developmental regulators not only enhances transformation efficiency but can also result in abnormal growth, so it is necessary to restrict/eliminate their expression in the plant after transformation. Even though these strategies would facilitate plant transformation in a broad range of recalcitrant genotypes new tools are needed such as the use of suitable promoters to control tissue- and timing-specific expression of these developmental regulators. In vegetatively propagated and highly heterozygous crops like potato, the procedure for developmental transgenes removal in subsequent generations through segregation is a challenge. We speculate that this last could be an option for some recalcitrant wild potatoes since most of them are diploid, so apart from asexual reproduction (by stolons and tubers), they have the alternative modes of sexual reproduction (by seeds) hence diploid progenies segregate for genes. In sum, the use of new technologies, would overcome the problems and limitations related to the classical methodology of potato transformation for some recalcitrant genotype.
There are many reports concerning successfully gene transfer of economically important genes in potato and GE varieties have been developed for a wide range of traits (Pribylova et al., 2006; Halterman et al., 2016; Zaheer and Akhtar, 2016; Hameed et al., 2018; Nadakuduti et al., 2018; Dev et al., 2021; Rakosy-Tican and Molnar, 2021). However, the use of biotechnology for potato improvement has been ultimately constrained by the regulation process and the public perception (Ghislain and Douches, 2020). Unfortunately, the consumer apprehension affects the field cultivation of the GE varieties and their adoption in the food chain. Looking closely at the history of approved potato GE, there are examples of deregulated potatoes whose field cultivation was discontinued due to lack of acceptance (Rakosy-Tican and Molnar, 2021; Bradshaw, 2021).
Along all the process from the development to the release of GE varieties, plant transformation and regeneration are the limiting factors for several crops, nonetheless for potato this does not seem to be the main constrain. The public perception is possibly the most difficult issue regarding the production and marketing a GE potato. The use of GE potato would allow reducing the use of pesticides, increasing yields, reducing production costs, lowering undesirable characters and/or providing a better nutrition quality, which would guarantee an adequate intake of vitally important foods. Taking everything into consideration and pondering the demographic expansion that is coming, it would be desirable that technical, ethical, and social/public constraints should be overcome in a relatively short time.
Author Contributions
VN, NA, CV provided the outlines of the review and contributed the key ideas. VN, NA, MG, and GM wrote the manuscript and prepared the tables. CV, HH, GM, CD, and SF worked on and improved the original draft. All co-authors approved the manuscript.
Funding
FONCyT: PICT Start-up 2018 0899: Variedades de papa editadas para mayor calidad y nutrición and PICT 2017-2878: Producción del péptido antimicrobiano Snakin-1 en cuerpos de oclusión de baculovirus y estudio de su potencial aplicación en el control de enfermedades vegetales; PICT 2018-02926 “Caracterización y utilización de genes candidatos para conferir resistencia al enverdecimiento (greening o HLB) y otras enfermedades bacterianas de cítricos mediante ingeniería genética”. INTA grants: 2019-PE-E6-I509-001 Mejoramiento genético de especies hortícolas de uso semi extensivo: PAPA y BATATA; 2019-PE-E6-I115-001 “Edición génica, transgénesis y mutagénesis como generadores de nueva variabilidad en especies de interés agropecuario”; 2019-PD-E4-I085-001 Determinación de los mecanismos de resistencia a enfermedades mediante la caracterización de las interacciones moleculares en sistemas planta-patógeno; 2019-PD-E6-I116-001 Identificación y análisis funcional de genes o redes génica s de interés biotecnológico con fin agropecuario, forestal, agroalimentario y/o agroindustrial; INTA-Fondo de Valorización Tecnológica (FVT-63): Variedades de papa editadas con mayor calidad industrial y nutricional. CONICET: P-UE 2018: “Estrategias de mejoramiento de nueva generación en cultivos agrícolas y forestales”.
Conflict of Interest
The authors declare that the research was conducted in the absence of any commercial or financial relationships that could be construed as a potential conflict of interest.
Publisher’s Note
All claims expressed in this article are solely those of the authors and do not necessarily represent those of their affiliated organizations, or those of the publisher, the editors and the reviewers. Any product that may be evaluated in this article, or claim that may be made by its manufacturer, is not guaranteed or endorsed by the publisher.
Abbreviations
ABEs, adenosine base editors; bar, herbicide bialaphos; CBEs, cytosine base editors; CRISPR, Clustered Regularly Interspaced Short Palindromic Repeats; DSBs, double stranded breaks; GE, genetically engineered; HDR, homology-directed repair; HPT, hygromycin phosphotransferase; NHEJ, non-homologous end joining; nptII, neomycin phosphotransferase II; PAM, protospacer adjacent motif; PEG, polyethylene glycol; RNP, assembled ribonucleoprotein; SSNs, site-specific nucleases; ZFNs, Zinc Finger Nucleases.
Footnotes
- ^ http://spuddb.uga.edu/pgsc_download.shtml
- ^ https://www.isaaa.org/gmapprovaldatabase/default.asp
- ^ https://www.potatopro.com/es
References
Abdel-Razik, A. B., Hammad, I. A., and Tawfik, E. (2017). Transformation of thionin genes using chitosan nanoparticle into potato plant to be resistant to fungal infection. IOSR J. Biotechnol. Biochem. 03, 1–13. doi: 10.9790/264X-03030113
Ahmad, R., Kim, Y.-H., Kim, M. D., Phung, M.-N., Chung, W.-I., Lee, H.-S., et al. (2008). Development of selection marker-free transgenic potato plants with enhanced tolerance to oxidative stress. J. Plant Biol. 51, 401–407. doi: 10.1007/BF03036060
Ahmed, H. A., Barpete, S., Akdogan, G., Aydin, G., Sancak, C., and Ozcan, S. (2018). Efficient regeneration and agrobacterium tumefaciens mediated genetic transformation of potato (Solanum Tuberosum L.). Fresenius Environ. Bull. 27, 3020–3027.
Almasia, N. I., Narhirñak, V., Hopp Esteban, H., and Vazquez-Rovere, C. (2010). Isolation and characterization of the tissue and development-specific potato snakin-1 promoter inducible by temperature and wounding. Electron. J. Biotechnol. 13, 8–9. doi: 10.2225/vol13-issue5-fulltext-12
An, G., Watson, B. D., and Chiang, C. C. (1986). Transformation of tobacco, tomato, potato, and arabidopsis thaliana using a binary ti vector system. Plant Physiol. 81, 301–305. doi: 10.1104/pp.81.1.301
Andersson, M., Turesson, H., Nicolia, A., Fält, A. S., Samuelsson, M., and Hofvander, P. (2017). Efficient targeted multiallelic mutagenesis in tetraploid potato (Solanum tuberosum) by transient CRISPR-Cas9 expression in protoplasts. Plant Cell Rep. 36, 117–128. doi: 10.1007/s00299-016-2062-3
Andersson, M., Turesson, H., Olsson, N., Fält, A. S., Ohlsson, P., Gonzalez, M. N., et al. (2018). Genome editing in potato via CRISPR-Cas9 ribonucleoprotein delivery. Physiol. Plant. 164, 378–384. doi: 10.1111/ppl.12731
Arora, L., and Narula, A. (2017). Gene editing and crop improvement using CRISPR-Cas9 system. Front. Plant Sci. 8:1932. doi: 10.3389/fpls.2017.01932
Asurmendi, S. (2002). Desarrollo Y Aplicación De Un Sistema De Expresión De Poliproteínas Autoclivables Basado En La Proteasa Nia Del Virus Tev Para La Obtención De Plantas Transgénicas Con Capacidad De Procesar, Secretar Y Dirigir Subcelularmente Las Proteínas Expresadas. Argentina: Universidad de Buenos Aires.
Badami, M. G., and Ramankutty, N. (2015). Urban agriculture and food security: a critique based on an assessment of urban land constraints. Glob. Food Sec. 4, 8–15. doi: 10.1016/j.gfs.2014.10.003
Bakhsh, A. (2020). Development of efficient, reproducible and stable agrobacterium-mediated genetic transformation of five potato cultivars. Food Technol. Biotechnol. 58, 57–63. doi: 10.17113/ftb.58.01.20.6187
Baltes, N. J., Gil-Humanes, J., and Voytas, D. F. (2017). Genome Engineering and Agriculture: opportunities and Challenges. Prog. Mol. Biol. Transl. Sci. 149, 1–26. doi: 10.1016/bs.pmbts.2017.03.011
Banerjee, A. K., Prat, S., and Hannapel, D. J. (2006). Efficient production of transgenic potato (S. tuberosum L. ssp. andigena) plants via Agrobacterium tumefaciens-mediated transformation. Plant Sci. 170, 732–738. doi: 10.1016/j.plantsci.2005.11.007
Bánfalvi, Z., Csákvári, E., Villányi, V., and Kondrák, M. (2020). Generation of transgene-free PDS mutants in potato by Agrobacterium-mediated transformation. BMC Biotechnol. 20:25. doi: 10.1186/s12896-020-00621-2
Barrell, P. J., Meiyalaghan, S., Jacobs, J. M. E., and Conner, A. J. (2013). Applications of biotechnology and genomics in potato improvement. Plant Biotechnol. J. 11, 907–920. doi: 10.1111/pbi.12099
Barrell, P. J., Yongjin, S., Cooper, P. A., and Conner, A. J. (2002). Alternative selectable markers for potato transformation using minimal T-DNA vectors. Plant Cell. Tissue Organ Cult. 70, 61–68. doi: 10.1023/A:1016013426923
Beaujean, A., Sangwan, R. S., Lecardonnel, A., and Sangwan-Norreel, B. S. (1998). Agrobacterium-mediated transformation of three economically important potato cultivars using sliced internodal explants: an efficient protocol of transformation. J. Exp. Bot. 49, 1589–1595. doi: 10.1093/jxb/49.326.1589
Bhaskar, P. B., Venkateshwaran, M., Wu, L., Ané, J. M., and Jiang, J. (2009). Agrobacterium-mediated transient gene expression and silencing: a rapid tool for functional gene assay in potato. PLoS One 4:e5812. doi: 10.1371/journal.pone.0005812
Bradshaw, J. E. (2021). Potato Breeding: Theory and Practice. Cham: Springer, doi: 10.1007/978-3-030-64414-7
Bravo-Almonacid, F., Rudoy, V., Welin, B., Segretin, M. E., Bedogni, M. C., Stolowicz, F., et al. (2012). Field testing, gene flow assessment and pre-commercial studies on transgenic Solanum tuberosum spp. tuberosum (cv. Spunta) selected for PVY resistance in Argentina. Transgenic Res. 21, 967–982. doi: 10.1007/s11248-011-9584-9
Brigneti, G., Martín-Hernández, A. M., Jin, H., Chen, J., Baulcombe, D. C., Baker, B., et al. (2004). Virus-induced gene silencing in Solanum species. Plant J. 39, 264–272. doi: 10.1111/j.1365-313X.2004.02122.x
Bruce, M. A., and Shoup Rupp, J. L. (2019). “Agrobacterium-mediated transformation of Solanum tuberosum L., Potato,” in Transgenic Plants: Methods and Protocols, eds S. Kumar, P. Barone, and M. Smith (New York: Springer), 203–223. doi: 10.1007/978-1-4939-8778-8
Butler, N. M., Atkins, P. A., Voytas, D. F., and Douches, D. S. (2015). Generation and inheritance of targeted mutations in potato (Solanum tuberosum L.) Using the CRISPR/Cas System. PLoS One 10:e0144591. doi: 10.1371/journal.pone.0144591
Butler, N. M., Baltes, N. J., Voytas, D. F., and Douches, D. S. (2016). Geminivirus-mediated genome editing in potato (Solanum tuberosum l.) using sequence-specific nucleases. Front. Plant Sci. 7:1045. doi: 10.3389/fpls.2016.01045
Butler, N. M., Jansky, S. H., and Jiang, J. (2020). First-generation genome editing in potato using hairy root transformation. Plant Biotechnol. J. 18, 2201–2209. doi: 10.1111/pbi.13376
Campos, H., and Ortiz, O. (2020). The Potato Crop. New York: Springer International Publishing, doi: 10.1007/978-3-030-28683-5
Chakravarty, B., and Wang-Pruski, G. (2010). Rapid regeneration of stable transformants in cultures of potato by improving factors influencing Agrobacterium-mediated transformation. Adv. Biosci. Biotechnol. 01, 409–416. doi: 10.4236/abb.2010.15054
Chakravarty, B., Wang-Pruski, G., Flinn, B., Gustafson, V., and Regan, S. (2007). Genetic transformation in potato: approaches and strategies. Am. J. Potato Res. 84, 301–311. doi: 10.1007/BF02986242
Chawla, R., Shakya, R., and Rommens, C. M. (2012). Tuber-specific silencing of asparagine synthetase-1 reduces the acrylamide-forming potential of potatoes grown in the field without affecting tuber shape and yield. Plant Biotechnol. J. 10, 913–924. doi: 10.1111/j.1467-7652.2012.00720.x
Chen, K., Wang, Y., Zhang, R., Zhang, H., and Gao, C. (2019). CRISPR/Cas Genome Editing and Precision Plant Breeding in Agriculture. Annu. Rev. Plant Biol. 70, 667–697. doi: 10.1146/annurev-arplant-050718-100049
Chung, B. N., Yoon, J.-Y., and Palukaitis, P. (2013). Engineered resistance in potato against potato leafroll virus, potato virus A and potato virus Y. Virus Genes 47, 86–92. doi: 10.1007/s11262-013-0904-4
Clasen, B. M., Stoddard, T. J., Luo, S., Demorest, Z. L., Li, J., Cedrone, F., et al. (2016). Improving cold storage and processing traits in potato through targeted gene knockout. Plant Biotechnol. J. 14, 169–176. doi: 10.1111/pbi.12370
Conner, A. J., Williams, M. K., Deroles, S. C., Deroles, S. C., Shaw, M. L., and Lancaster, J. E. (1992). Agrobacterium-mediated transformation of New Zealand potato cultivars. New Zeal. J. Crop Hortic. Sci. 19, 1–8. doi: 10.1080/01140671.1991.10418098
Craig, W., Gargano, D., Scotti, N., Nguyen, T. T., Lao, N. T., Kavanagh, T. A., et al. (2005). Direct gene transfer in potato: a comparison of particle bombardment of leaf explants and PEG-mediated transformation of protoplasts. Plant Cell Rep. 24, 603–611. doi: 10.1007/s00299-005-0018-0
Craze, M., Bates, R., Bowden, S., and Wallington, E. J. (2018). Highly efficient agrobacterium-mediated transformation of potato (Solanum tuberosum) and production of transgenic microtubers. Curr. Protoc. plant Biol. 3, 33–41. doi: 10.1002/cppb.20065
Dale, P. J., and Hampson, K. K. (1995). An assessment of morphogenic and transformation efficiency in a range of varieties of potato (Solanum tuberosum L.). Euphytica Int. J. Plant Breed. 85, 101–108. doi: 10.1007/BF00023936
De Block, M. (1988). Genotype-independent leaf disc transformation of potato (Solanum tuberosum) using Agrobacterium tumefaciens. Theor. Appl. Genet. 76, 767–774. doi: 10.1007/BF00303524
De Vetten, N., Wolters, A. M., Raemakers, K., Van der Meer, I., Ter Stege, R., Heeres, E., et al. (2003). A transformation method for obtaining marker-free plants of a cross-pollinating and vegetatively propagated crop. Nat. Biotechnol. 21, 439–442. doi: 10.1038/nbt801
Debernardi, J. M., Tricoli, D. M., Ercoli, M. F., Hayta, S., Ronald, P., Palatnik, J. F., et al. (2020). A GRF–GIF chimeric protein improves the regeneration efficiency of transgenic plants. Nat. Biotechnol. 38, 1274–1279. doi: 10.1038/s41587-020-0703-0
Décima Oneto, C. A., Coronel, J., Storani, L., González, M. N., Feingold, S. E., and Massa, G. A. (2020). Transformación Eficiente de Solanum Tuberosum cv. Spunta Mediada Por Agrobacterium Tumefaciens Utilizando Higromicina Como Agente Selectivo. Rev. Ria - inta 46. Available Online at: https://repositorio.inta.gob.ar/bitstream/handle/20.500.12123/7970/RIA_VOLUMEN46_No2_p.249-257.pdf?sequence=1&isAllowed=y (accessed October 26, 2021).
Dev, S. S., Joseph, J., and D’Rosario, L. L. (2021). “Prospects for genome editing of potato,” in Solanum tuberosum-a Promising Crop for Starvation Problem, eds M. Yildiz and Y. Ozgen (London: IntechOpen). doi: 10.5772/intechopen.99278
Dinh, P. T., Zhang, L., Mojtahedi, H., Brown, C. R., and Elling, A. A. (2015). Broad meloidogyne resistance in potato based on RNA interference of effector gene 16D10. J. Nematol. 47, 71–78.
Dobnik, D., Lazar, A., Stare, T., Gruden, K., Vleeshouwers, V. G. A. A., and Žel, J. (2016). Solanum venturii, a suitable model system for virus-induced gene silencing studies in potato reveals StMKK6 as an important player in plant immunity. Plant Methods 12:29. doi: 10.1186/s13007-016-0129-3
Doudna, J. A., and Charpentier, E. (2014). Genome editing. The new frontier of genome engineering with CRISPR-Cas9. Science 346:1258096. doi: 10.1126/science.1258096
Enciso-Rodriguez, F., Manrique-Carpintero, N. C., Nadakuduti, S. S., Buell, C. R., Zarka, D., and Douches, D. (2019). Overcoming self-incompatibility in diploid potato using CRISPR-cas9. Front. Plant Sci. 10:376. doi: 10.3389/fpls.2019.00376
Faivre-Rampant, O., Gilroy, E. M., Hrubikova, K., Hein, I., Millam, S., Loake, G. J., et al. (2004). Potato virus X-induced gene silencing in leaves and tubers of potato. Plant Physiol. 134, 1308–1316. doi: 10.1104/pp.103.037507
Fehér, A., Felföldi, K., Preiszner, J., and Dudits, D. (1991). PEG-mediated transformation of leaf protoplasts of Solanum tuberosum L. cultivars. Plant Cell. Tissue Organ Cult. 27, 105–114. doi: 10.1007/BF00048214
Feingold, S. E., Bonnecarrere, V., Nepomuceno, A., Hinrichsen, P., Cardozo Tellez, L., Molinari, H., et al. (2018). Edición génica: una oportunidad para la región. Rev. Investig. Agrop. 44, 424–427.
Fernandez Bidondo, L., Almasia, N., Bazzini, A., Colombo, R., Hopp, E., Vazquez-Rovere, C., et al. (2019). The overexpression of antifungal genes enhances resistance to rhizoctonia solani in transgenic potato plants without affecting arbuscular mycorrhizal symbiosis. Crop Prot. 124:104837. doi: 10.1016/j.cropro.2019.05.031
Fernández-Piñán, S., López, J., Armendariz, I., Boher, P., Figueras, M., and Serra, O. (2019). Agrobacterium tumefaciens and Agrobacterium rhizogenes-mediated transformation of potato and the promoter activity of a suberin gene by GUS Staining. J. Vis. Exp. 145, 1–9. doi: 10.3791/59119
Forsyth, A., Weeks, T., Richael, C., and Duan, H. (2016). Transcription activator-like effector nucleases (TALEN)-mediated targeted DNA insertion in potato plants. Front. Plant Sci. 7:1572. doi: 10.3389/fpls.2016.01572
Fossi, M., Amundson, K., Kuppu, S., Britt, A., and Comai, L. (2019). Regeneration of solanum tuberosum plants from protoplasts induces widespread genome instability. Plant Physiol. 180, 78–86. doi: 10.1104/pp.18.00906
Gao, C. (2018). The future of CRISPR technologies in agriculture. Nat. Rev. Mol. Cell Biol. 19, 275–276. doi: 10.1038/nrm.2018.2
Gao, C. (2021). Genome engineering for crop improvement and future agriculture. Cell 184, 1621–1635. doi: 10.1016/j.cell.2021.01.005
Gelvin, S. B. (2017). Integration of Agrobacterium T-DNA into the Plant Genome. Annu. Rev. Genet. 51, 195–217. doi: 10.1146/annurev-genet-120215-035320
Ghislain, M., and Douches, D. (2020). “The Genes and Genomes of the Potato,” in The Potato Crop, eds H. Campos and O. Ortiz (Cham: Springer), 139–162. doi: 10.1007/978-3-030-28683-5_5
Ghislain, M., Goodman, R., and Barekye, A. (2021). Defeating Late Blight Disease Of Potato in sub-Saharan Africa. Available Online at: https://www.openaccessgovernment.org/late-blight-disease/108180/ (accessed October 26, 2021).
González, M. N., Massa, G. A., Andersson, M., Décima Oneto, C. A., Turesson, H., Storani, L., et al. (2021). Comparative potato genome editing: agrobacterium tumefaciens-mediated transformation and protoplasts transfection delivery of CRISPR/Cas9 components directed to StPPO2 gene. Plant Cell. Tissue Organ Cult. 145, 291–305. doi: 10.1007/s11240-020-02008-9
González, M. N., Massa, G. A., Andersson, M., Turesson, H., Olsson, N., Fält, A. S., et al. (2020). Reduced enzymatic browning in potato tubers by specific editing of a polyphenol oxidase gene via ribonucleoprotein complexes delivery of the CRISPR/Cas9 System. Front. Plant Sci. 10:1649. doi: 10.3389/fpls.2019.01649
Grafius, E. J., and Douches, D. S. (2008). “The present and future role of insect-resistant genetically modified potato cultivars in IPM,” in Integration of Insect-Resistant Genetically Modified Crops within IPM Programs, eds J. Romeis, A. M. Shelton, and G. G. Kennedy (Dordrecht: Springer), 195–221. doi: 10.1007/978-1-4020-8373-0_7
Griffiths, B. S., Geoghegan, I. E., and Robertson, W. M. (2000). Testing genetically engineered potato, producing the lectins GNA and Con A, on non-target soil organisms and processes. J. Appl. Ecol. 37, 159–170. doi: 10.1046/j.1365-2664.2000.00481.x
Gustafson, V., Mallubhotla, S., MacDonnell, J., Sanyal-Bagchi, M., Chakravarty, B., Wang-Pruski, G., et al. (2006). Transformation and plant regeneration from leaf explants of Solanum tuberosum L. cv. “Shepody.”. Plant Cell. Tissue Organ Cult. 85, 361–366. doi: 10.1007/s11240-006-9085-3
Haldrup, A., Petersen, S. G., and Okkels, F. T. (1998). Positive selection: a plant selection principle based on xylose isomerase, an enzyme used in the food industry. Plant Cell Rep. 18, 76–81. doi: 10.1007/s002990050535
Halterman, D., Guenthner, J., Collinge, S., Butler, N., and Douches, D. (2016). Biotech potatoes in the 21st century: 20 years since the first biotech potato. Am. J. Potato Res. 93, 1–20. doi: 10.1007/s12230-015-9485-1
Hameed, A., Tahir, M. N., Asad, S., Bilal, R., Van Eck, J., Jander, G., et al. (2017). RNAi-Mediated simultaneous resistance against three RNA viruses in potato. Mol. Biotechnol. 59, 73–83. doi: 10.1007/s12033-017-9995-9
Hameed, A., Zaidi, S. S., Shakir, S., and Mansoor, S. (2018). Applications of new breeding technologies for potato improvement. Front. Plant Sci. 9:925. doi: 10.3389/fpls.2018.00925
Han, E. H., Goo, Y. M., Lee, M. K., and Lee, S. W. (2015). An efficient transformation method for a potato (Solanum tuberosum L. var. Atlantic). J. Plant Biotechnol. 42, 77–82. doi: 10.5010/JPB.2015.42.2.77
Haverkort, A. J., Boonekamp, P. M., Hutten, R., Jacobsen, E., Lotz, L. A. P., Kessel, G. J. T., et al. (2016). Durable late blight resistance in potato through dynamic varieties obtained by cisgenesis: scientific and societal advances in the DuRPh project. Potato Res. 59, 35–66. doi: 10.1007/s11540-015-9312-6
Hawkes, J. G., and Hjerting, J. P. (1969). The Potatoes Of Argentina, Brazil, Paraguay And Uruguay: A Biosystematic Study. Oxford: Clarendon P.
Heeres, P., Schippers-Rozenboom, M., Jacobsen, E., and Visser, R. G. F. (2002). Transformation of a large number of potato varieties: genotype-dependent variation in efficiency and somaclonal variability. Euphytica 124, 13–22. doi: 10.1023/A:1015689112703
Hellens, R., Mullineaux, P., and Klee, H. (2000). A guide to Agrobacterium binary Ti vectors. Trends Plant Sci. 5, 446–451. doi: 10.1016/S1360-1385(00)01740-4
Heuer, H., Kroppenstedt, R. M., Lottmann, J., Berg, G., and Smalla, K. (2002). Effects of T4 lysozyme release from transgenic potato roots on bacterial rhizosphere communities are negligible relative to natural factors. Appl. Environ. Microbiol. 68, 1325–1335. doi: 10.1128/AEM.68.3.1325-1335.2002
Huang, T. K., and Puchta, H. (2019). CRISPR/Cas-mediated gene targeting in plants: finally a turn for the better for homologous recombination. Plant Cell Rep. 38, 443–453. doi: 10.1007/s00299-019-02379-0
Huang, T. K., and Puchta, H. (2021). Novel CRISPR/Cas applications in plants: from prime editing to chromosome engineering. Transgenic Res. 30, 529–549. doi: 10.1007/s11248-021-00238-x
Isalan, M. (2012). Zinc-finger nucleases: how to play two good hands. Nat. Methods 9, 32–34. doi: 10.1038/nmeth.1805
Ishida, B. K., Snyder, G. W., and Belknap, W. R. (1989). The use of in vitro-grown microtuber discs inAgrobacterium-mediated transformation of Russet Burbank and Lemhi Russet potatoes. Plant Cell Rep. 8, 325–328. doi: 10.1007/BF00716665
ISAAA (2021). GM Approval Database. Available online at: https://www.isaaa.org/gmapprovaldatabase/default.asp
Itkin, M., Heinig, U., Tzfadia, O., Bhide, A. J., Shinde, B., Cardenas, P. D., et al. (2013). Biosynthesis of antinutritional alkaloids in solanaceous crops is mediated by clustered genes. Science 341, 175–179. doi: 10.1126/science.1240230
Jiang, F., and Doudna, J. A. (2017). CRISPR – Cas9 structures and mechanisms. Annu. Rev. Biophys. 46, 505–529. doi: 10.1146/annurev-biophys-062215-010822
Jinek, M., Chylinski, K., Fonfara, I., Hauer, M., Doudna, J. A., and Charpentier, E. (2012). A Programmable Dual-RNA – Guided DNA endonuclease in adaptive bacterial immunity. Science 337, 816–821. doi: 10.1126/science.1225829
Joersbo, M., Jørgensen, K., and Brunstedt, J. (2003). A selection system for transgenic plants based on galactose as selective agent and a UDP-glucose:galactose-1-phosphate uridyltransferase gene as selective gene. Mol. Breed. 11, 315–323. doi: 10.1023/A:1023402424215
Johansen, I. E., Liu, Y., Jørgensen, B., Bennett, E. P., Andreasson, E., Nielsen, K. L., et al. (2019). High efficacy full allelic CRISPR/Cas9 gene editing in tetraploid potato. Sci. Rep. 9:17715. doi: 10.1038/s41598-019-54126-w
Kaur, A., Guleria, S., Reddy, M. S., and Kumar, A. (2020). A robust genetic transformation protocol to obtain transgenic shoots of Solanum tuberosum L. cultivar ‘Kufri Chipsona 1.’. Physiol. Mol. Biol. Plants 26, 367–377. doi: 10.1007/s12298-019-00747-4
Kaur, R. P., and Devi, S. (2019). In planta transformation in plants: a review. Agric. Rev. 40, 159–174. doi: 10.18805/ag.r-1597
Khatun, A., Hasan, M. M., Bachchu, M. A. A., Moniruzzaman, M., and Nasiruddin, K. M. (2012). Agrobacterium-mediated Genetic Transformation of Potato (Solanum tuberosum L.) var. Cardinal Heera. Agric. 10, 81–86. doi: 10.3329/agric.v10i1.11068
Kieu, N. P., Lenman, M., Wang, E. S., Petersen, B. L., and Andreasson, E. (2021). Mutations introduced in susceptibility genes through CRISPR/Cas9 genome editing confer increased late blight resistance in potatoes. Sci. Rep. 11:4487. doi: 10.1038/s41598-021-83972-w
Kondrák, M., van der Meer, I. M., and Bánfalvi, Z. (2006). Generation of marker- and backbone-free transgenic potatoes by site-specific recombination and a bi-functional marker gene in a non-regular one-border agrobacterium transformation vector. Transgenic Res. 15, 729–737. doi: 10.1007/s11248-006-9021-7
Kong, J., Martin-Ortigosa, S., Finer, J., Orchard, N., Gunadi, A., Batts, L. A., et al. (2020). Overexpression of the Transcription Factor GROWTH-REGULATING FACTOR5 Improves Transformation of Dicot and Monocot Species. Front. Plant Sci. 11:572319. doi: 10.3389/fpls.2020.572319
Kumar, A. (1995). “Agrobacterium-Mediated Transformation of Potato Genotypes,” in Methods in Molecular Biology: Agrobacterium Protocols, eds K. M. A. Gartland and M. R. Davey (Totowa: Springer), 121–128. doi: 10.1385/0-89603-302-3:121
Kumar, A., Miller, M., Whitty, P., Lyon, J., and Davie, P. (1995). Agrobacterium-mediated transformation of five wild Solanum species using in vitro microtubers. Plant Cell Rep. 14, 324–328. doi: 10.1007/BF00232037
Kumlehn, J., Pietralla, J., Hensel, G., Pacher, M., and Puchta, H. (2018). The CRISPR/Cas revolution continues: from efficient gene editing for crop breeding to plant synthetic biology. J. Integr. Plant Biol. 60, 1127–1153. doi: 10.1111/jipb.12734
Kusano, H., Ohnuma, M., Mutsuro-Aoki, H., Asahi, T., Ichinosawa, D., Onodera, H., et al. (2018). Establishment of a modified CRISPR/Cas9 system with increased mutagenesis frequency using the translational enhancer dMac3 and multiple guide RNAs in potato. Sci. Rep. 8:13753. doi: 10.1038/s41598-018-32049-2
Kusano, H., Onodera, H., Kihira, M., Aoki, H., Matsuzaki, H., and Shimada, H. (2016). A simple Gateway-assisted construction system of TALEN genes for plant genome editing. Sci. Rep. 6:30234. doi: 10.1038/srep30234
Lema, M. A. (2019). Regulatory aspects of gene editing in Argentina. Transgenic Res. 28, 147–150. doi: 10.1007/s11248-019-00145-2
Li, M., Xie, C., Song, B., Ou, Y., Lin, Y., Liu, X., et al. (2015). Construction of efficient, tuber-specific, and cold-inducible promoters in potato. Plant Sci. 235, 14–24. doi: 10.1016/j.plantsci.2015.02.014
Lin, Q., Jin, S., Zong, Y., Yu, H., Zhu, Z., Liu, G., et al. (2021). High-efficiency prime editing with optimized, paired pegRNAs in plants. Nat. Biotechnol. 39, 923–927. doi: 10.1038/s41587-021-00868-w
Llorente, B., Rodríguez, V., Alonso, G. D., Torres, H. N., Flawiá, M. M., and Bravo-Almonacid, F. F. (2010). Improvement of Aroma in Transgenic Potato As a Consequence of Impairing Tuber Browning. PLoS One 5:e14030. doi: 10.1371/journal.pone.0014030
Ma, J., Xiang, H., Donnelly, D. J., Meng, F. R., Xu, H., Durnford, D., et al. (2017). Genome editing in potato plants by agrobacterium-mediated transient expression of transcription activator-like effector nucleases. Plant Biotechnol. Rep. 11, 249–258. doi: 10.1007/s11816-017-0448-5
Maher, M. F., Nasti, R. A., Vollbrecht, M., Starker, C. G., Clark, M. D., and Voytas, D. F. (2020). Plant gene editing through de novo induction of meristems. Nat. Biotechnol. 38, 84–89. doi: 10.1038/s41587-019-0337-2
Martini, N., Egen, M., Rüntz, I., and Strittmatter, G. (1993). Promoter sequences of a potato pathogenesis-related gene mediate transcriptional activation selectively upon fungal infection. Mol. Gen. Genet. 236, 179–186. doi: 10.1007/BF00277110
Meiyalaghan, S., Barrell, P. J., Jacobs, J. M. E., and Conner, A. J. (2011). Regeneration of multiple shoots from transgenic potato events facilitates the recovery of phenotypically normal lines: assessing a cry9Aa2 gene conferring insect resistance. BMC Biotechnol. 11:93. doi: 10.1186/1472-6750-11-93
Millam, S. (2006). “Potato (Solanum tuberosum L.),” in Agrobacterium Protocols Volume 2, ed. K. Wang (Totowa: Humana Press), 25–35. doi: 10.1385/1-59745-131-2:25
Mishra, R., Joshi, R. K., and Zhao, K. (2020). Base editing in crops: current advances, limitations and future implications. Plant Biotechnol. J. 18, 20–31. doi: 10.1111/pbi.13225
Missiou, A., Kalantidis, K., Boutla, A., Tzortzakaki, S., Tabler, M., and Tsagris, M. (2004). Generation of transgenic potato plants highly resistant to potato virus Y (PVY) through RNA silencing. Mol. Breed. 14, 185–197. doi: 10.1023/B:MOLB.0000038006.32812.52
Mollika, S. R., Sarker, R. H., and Hoque, M. I. (2020). In vitro Regeneration and Agrobacterium-mediated genetic transformation of a cultivated potato variety using marker genes. Plant Tissue Cult. Biotechnol. 30, 149–160. doi: 10.3329/ptcb.v30i1.47800
Mullins, E., Milbourne, D., Petti, C., Doyle-Prestwich, B. M., and Meade, C. (2006). Potato in the age of biotechnology. Trends Plant Sci. 11, 254–260. doi: 10.1016/j.tplants.2006.03.002
Murashige, T., and Skoog, F. (1962). A Revised medium for rapid growth and bio assays with tobacco tissue cultures. Physiol. Plant. 15, 473–497. doi: 10.1111/j.1399-3054.1962.tb08052.x
Nadakuduti, S. S., Buell, C. R., Voytas, D. F., Starker, C. G., and Douches, D. S. (2018). Genome editing for crop improvement – applications in clonally propagated polyploids with a focus on potato (solanum tuberosum L.). Front. Plant Sci. 871:1607. doi: 10.3389/fpls.2018.01607
Nahirñak, V., Rivarola, M., Almasia, N. I., Barón, M. P. B., Hopp, H. E., Vile, D., et al. (2019). Snakin-1 affects reactive oxygen species and ascorbic acid levels and hormone balance in potato. PLoS One 14:e0214165. doi: 10.1371/journal.pone.0214165
Nakayasu, M., Akiyama, R., Lee, H. J., Osakabe, K., Osakabe, Y., Watanabe, B., et al. (2018). Generation of α-solanine-free hairy roots of potato by CRISPR/Cas9 mediated genome editing of the St16DOX gene. Plant Physiol. Biochem. 131, 70–77. doi: 10.1016/j.plaphy.2018.04.026
Naumkina, E., Bolyakina, Y., and Romanov, G. (2007). Organ-specificity and inducibility of patatin class I promoter from potato in transgenic arabidopsis plants. Russ. J. plant Physiol. 54, 350–359. doi: 10.1134/S1021443707030090
Newell, C. A., Rozman, R., Hinchee, M. A., Lawson, E. C., Haley, L., Sanders, P., et al. (1991). Agrobacterium-mediated transformation of Solanum tuberosum L. ev. “Russet Burbank”. Plant Cell Rep. 10, 30–34.
Nicolia, A., Proux-Wéra, E., Åhman, I., Onkokesung, N., Andersson, M., Andreasson, E., et al. (2015). Targeted gene mutation in tetraploid potato through transient TALEN expression in protoplasts. J. Biotechnol. 204, 17–24. doi: 10.1016/j.jbiotec.2015.03.021
Ooms, G., Bossen, M. E., Burrell, M. M., and Karp, A. (1986). Genetic manipulation in potato with Agrobacterium rhizogenes. Potato Res. 29, 367–379. doi: 10.1007/BF02359965
Pino, M.-T., Skinner, J. S., Park, E.-J., Jeknić, Z., Hayes, P. M., Thomashow, M. F., et al. (2007). Use of a stress inducible promoter to drive ectopic AtCBF expression improves potato freezing tolerance while minimizing negative effects on tuber yield. Plant Biotechnol. J. 5, 591–604. doi: 10.1111/j.1467-7652.2007.00269.x
Potato News Today (2021). A Blight-Resistant GMO Potato Variety Help Farmers in Uganda to Defeat Late Blight and Change Their Fortunes. Available online at: https://www.potatonewstoday.com/2021/07/31/a-blight-resistant-gmo-potato-variety-help-farmers-in-uganda-to-defeat-late-blight-and-change-their-fortunes/
Pribylova, R., Pavlik, I., and Bartos, M. (2006). Genetically modified potato plants in nutrition and prevention of diseases in humans and animals: a review. Vet. Med. (Praha). 51, 212–223. doi: 10.17221/5540-VETMED
Puchta, H. (2005). The repair of double-strand breaks in plants: mechanisms and consequences for genome evolution. J. Exp. Bot. 56, 1–14. doi: 10.1093/jxb/eri025
Rabinowitz, D., Linder, C. R., Ortega, R., Begazo, D., Murguia, H., Douches, D. S., et al. (1990). High levels of interspecific hybridization betweensolanum sparsipilum ands. stenotomum in experimental plots in the andes. Am. Potato J. 67, 73–81. doi: 10.1007/BF02990957
Rakosy-Tican, E., Aurori, C. M., Dijkstra, C., Thieme, R., Aurori, A., and Davey, M. R. (2007). The usefulness of the gfp reporter gene for monitoring Agrobacterium- mediated transformation of potato dihaploid and tetraploid genotypes. Plant Cell Rep. 26, 661–671. doi: 10.1007/s00299-006-0273-8
Rakosy-Tican, E., and Molnar, I. (2021). “Biotechnological strategies for a resilient potato crop,” in Solanum tuberosum - a Promising Crop for Starvation Problem, eds M. Yildiz and Y. Ozgen (London: IntechOpen). doi: 10.5772/intechopen.98717
Ran, Y., Liang, Z., and Gao, C. (2017). Current and future editing reagent delivery systems for plant genome editing. Sci. China Life Sci. 60, 490–505. doi: 10.1007/s11427-017-9022-1
Rasche, F., Marco-Noales, E., Velvis, H., van Overbeek, L. S., López, M. M., van Elsas, J. D., et al. (2006). Structural characteristics and plant-beneficial effects of bacteria colonizing the shoots of field grown conventional and genetically modified T4-lysozyme producing potatoes. Plant Soil 289, 123–140. doi: 10.1007/s11104-006-9103-6
Rivero, M., Furman, N., Mencacci, N., Picca, P., Toum, L., Lentz, E., et al. (2012). Stacking of antimicrobial genes in potato transgenic plants confers increased resistance to bacterial and fungal pathogens. J. Biotechnol. 157, 334–343. doi: 10.1016/j.jbiotec.2011.11.005
Romano, A., Raemakers, K., Bernardi, J., Visser, R., and Mooibroek, H. (2003). Transgene organisation in potato after particle bombardment-mediated (co-)transformation using plasmids and gene cassettes. Transgenic Res. 12, 461–473. doi: 10.1023/A:1024267906219
Romano, A., Raemakers, K., Visser, R., and Mooibroek, H. (2001). Transformation of potato (Solanum tuberosum) using particle bombardment. Plant Cell Rep. 20, 198–204. doi: 10.1007/s002990000314
Rommens, C. M., Ye, J., Richael, C., and Swords, K. (2006). Improving potato storage and processing characteristics through all-native DNA transformation. J. Agric. Food Chem. 54, 9882–9887. doi: 10.1021/jf062477l
Rooke, L., and Lindsey, K. (1998). Potato transformation. Methods Mol. Biol. 81, 353–358. doi: 10.1385/0-89603-385-6:353
Sawahel, W. (2002). The production of transgenic potato plants expressing human a-interferon using lipofectin-mediated transformation. Cell. Mol. Biol. Lett. 7, 19–29.
Sawai, S., Ohyama, K., Yasumoto, S., Seki, H., Sakuma, T., Yamamoto, T., et al. (2014). Sterol side chain reductase 2 is a key enzyme in the biosynthesis of cholesterol, the common precursor of toxic steroidal glycoalkaloids in potato. Plant Cell 26, 3763–3774. doi: 10.1105/tpc.114.130096
Scheben, A., Wolter, F., Batley, J., Puchta, H., and Edwards, D. (2017). Towards CRISPR/CAS crops – Bringing together genomics and genome editing. New Phytol. 216, 682–698. doi: 10.1111/nph.14702
Schmidt, C., Pacher, M., and Puchta, H. (2019). DNA break repair in plants and its application for genome engineering. Methods Mol. Biol. 1864, 237–266. doi: 10.1007/978-1-4939-8778-8_17
Scotti, N., Valkov, V. T., and Cardi, T. (2011). Improvement of plastid transformation efficiency in potato by using vectors with homologous flanking sequences. GM Crops 2, 89–91. doi: 10.4161/gmcr.2.2.17504
Segretin, M. E., Lentz, E. M., Wirth, S. A., Morgenfeld, M. M., and Bravo-Almonacid, F. F. (2012). Transformation of Solanum tuberosum plastids allows high expression levels of β-glucuronidase both in leaves and microtubers developed in vitro. Planta 235, 807–818. doi: 10.1007/s00425-011-1541-6
Sheerman, S., and Bevan, M. W. (1988). A rapid transformation method for Solanum tuberosum using binary Agrobacterium tumefaciens vectors. Plant Cell Rep. 7, 13–16. doi: 10.1007/BF00272967
Si, H., Xie, C. H., and Liu, J. (2003). An efficient protocol for Agrobacterium-mediated transformation with microtuber and the induction of an antisense class I patatin gene into potato. Acta Agron. Sin. 29, 801–805.
Sidorov, V. A., Kasten, D., Pang, S. Z., Hajdukiewicz, P. T. J., Staub, J. M., and Nehra, N. S. (1999). Stable chloroplast transformation in potato: use of green fluorescent protein as a plastid marker. Plant J. 19, 209–216. doi: 10.1046/j.1365-313X.1999.00508.x
Stiekema, W. J., Heidekamp, F., Louwerse, J. D., Verhoeven, H. A., and Dijkhuis, P. (1988). Introduction of foreign genes into potato cultivars Bintje and Désirée using an Agrobacterium tumefaciens binary vector. Plant Cell Rep. 7, 47–50. doi: 10.1007/BF00272976
Sun, K., Wolters, A. M. A., Vossen, J. H., Rouwet, M. E., Loonen, A. E. H. M., Jacobsen, E., et al. (2016). Silencing of six susceptibility genes results in potato late blight resistance. Transgenic Res. 25, 731–742. doi: 10.1007/s11248-016-9964-2
Tagliabue, G. (2018). Counterproductive consequences of anti-GMO activism. Ethics Sci. Environ. Polit. 18, 61–74. doi: 10.3354/esep00185
Tavazza, R., Tavazza, M., Ordas, R. J., Ancora, G., and Benvenuto, E. (1989). Genetic transformation of potato (Solanum tuberosum): an efficient method to obtain transgenic plants. Plant Sci. 59, 175–181. doi: 10.1016/0168-9452(89)90135-0
Thanh, T. N., Nugent, G., Cardi, T., and Dix, P. J. (2005). Generation of homoplasmic plastid transformants of a commercial cultivar of potato (Solanum tuberosum L.). Plant Sci. 168, 1495–1500. doi: 10.1016/j.plantsci.2005.01.023
Tiwari, J. K., Challam, C., Chakrabarti, S. K., and Feingold, S. E. (2020). “Climate-smart potato: an integrated breeding, genomics, and phenomics approach,” in Genomic Designing of Climate-Smart Vegetable Crops, ed. C. Kole (Cham: Springer International Publishing), 1–46. doi: 10.1007/978-3-319-97415-6_1
Trujillo, C., Rodríguez-Arango, E., Jaramillo, S., Hoyos, R., Orduz, S., and Arango, R. (2001). One-step transformation of two andean potato cultivars (Solanum tuberosum L. subsp. andigena). Plant Cell Rep. 20, 637–641. doi: 10.1007/s002990100381
Tuncel, A., Corbin, K. R., Ahn-Jarvis, J., Harris, S., Hawkins, E., Smedley, M. A., et al. (2019). Cas9-mediated mutagenesis of potato starch-branching enzymes generates a range of tuber starch phenotypes. Plant Biotechnol. J. 17, 2259–2271. doi: 10.1111/pbi.13137
USDA (2021). USDA Announces Deregulation Extension of Potato Developed Using Genetic Engineering. Available online at: https://www.aphis.usda.gov/aphis/newsroom/stakeholder-info/stakeholder-messages/biotechnology-news/ge-potato
Valkov, V. T., Gargano, D., Manna, C., Formisano, G., Dix, P. J., Gray, J. C., et al. (2011). High efficiency plastid transformation in potato and regulation of transgene expression in leaves and tubers by alternative 5′ and 3′ regulatory sequences. Transgenic Res. 20, 137–151. doi: 10.1007/s11248-010-9402-9
Valkov, V. T., Gargano, D., Scotti, N., and Cardi, T. (2014). Plastid transformation in potato: Solanum tuberosum. Methods Mol. Biol. 1132, 295–303. doi: 10.1007/978-1-62703-995-6_18
van der Voet, H., Goedhart, P. W., Lazebnik, J., Kessel, G. J. T., Mullins, E., van Loon, J. J. A., et al. (2019). Equivalence analysis to support environmental safety assessment: using nontarget organism count data from field trials with cisgenically modified potato. Ecol. Evol. 9, 2863–2882. doi: 10.1002/ece3.4964
Van Eck, J., Conlin, B., Garvin, D. F., Mason, H., Navarre, D. A., and Brown, C. R. (2007). Enhancing beta-carotene content in potato by rnai-mediated silencing of the beta-carotene hydroxylase gene. Am. J. Potato Res. 84:331. doi: 10.1007/BF02986245
Veillet, F., Chauvin, L., Kermarrec, M. P., Sevestre, F., Merrer, M., Terret, Z., et al. (2019a). The Solanum tuberosum GBSSI gene: a target for assessing gene and base editing in tetraploid potato. Plant Cell Rep. 38, 1065–1080. doi: 10.1007/s00299-019-02426-w
Veillet, F., Perrot, L., Chauvin, L., Kermarrec, M. P., Guyon-Debast, A., Chauvin, J. E., et al. (2019b). Transgene-free genome editing in tomato and potato plants using Agrobacterium-mediated delivery of a CRISPR/Cas9 cytidine base editor. Int. J. Mol. Sci. 20:402. doi: 10.3390/ijms20020402
Veillet, F., Kermarrec, M. P., Chauvin, L., Chauvin, J. E., and Nogué, F. (2020). CRISPR-induced indels and base editing using the Staphylococcus aureus Cas9 in potato. PLoS One 15:e0235942. doi: 10.1371/journal.pone.0235942
Verhees, J., van der Krol, A. R., Vreugdenhil, D., and van der Plas, L. H. W. (2002). Characterization of gene expression during potato tuber development in individuals and populations using the luciferase reporter system. Plant Mol. Biol. 50, 653–665. doi: 10.1023/A:1019922329081
Vinterhalter, D. (2008). Potato In Vitro Culture Techniques and Biotechnology. Available online at: http://globalsciencebooks.info/Online/GSBOnline/images/0812/FVCSB_2(SI1)/FVCSB_2(SI1)16-45o.pdf
Visser, R. G. F. (1991). “Regeneration and transformation of potato by Agrobacterium tumefaciens,” in Plant Tissue Culture Manual, ed. K. Lindsey (Dordrecht: Springer), 301–309. doi: 10.1007/978-94-009-0103-2_16
Visser, R. G. F., Jacobsen, E., Hesseling-Meinders, A., Schans, M. J., Witholt, B., and Feenstra, W. J. (1989). Transformation of homozygous diploid potato with an Agrobacterium tumefaciens binary vector system by adventitious shoot regeneration on leaf and stem segments. Plant Mol. Biol. 12, 329–337. doi: 10.1007/BF00043210
Voytas, D. F. (2013). Plant genome engineering with sequence-specific nucleases. Annu. Rev. Plant Biol. 64, 327–350. doi: 10.1146/annurev-arplant-042811-105552
Wang, S., Zhang, S., Wang, W., Xiong, X., Meng, F., and Cui, X. (2015). Efficient targeted mutagenesis in potato by the CRISPR/Cas9 system. Plant Cell Rep. 34, 1473–1476. doi: 10.1007/s00299-015-1816-7
Waterhouse, P. M., Graham, M. W., and Wang, M. B. (1998). Virus resistance and gene silencing in plants can be induced by simultaneous expression of sense and antisense RNA. Proc. Natl. Acad. Sci. U. S. A. 95, 13959–13964. doi: 10.1073/pnas.95.23.13959
Weiland, J. J. (2003). Transformation of Pythium aphanidermatum to geneticin resistance. Curr. Genet. 42, 344–352. doi: 10.1007/s00294-002-0359-y
Wendt, T., Doohan, F., and Mullins, E. (2012). Production of Phytophthorainfestans-resistant potato (Solanum tuberosum) utilising Ensifer adhaerens OV14. Transgenic Res. 21, 567–578. doi: 10.1007/s11248-011-9553-3
Wendt, T., Doohan, F., Winckelmann, D., and Mullins, E. (2011). Gene transfer into Solanum tuberosum via Rhizobium spp. Transgenic Res. 20, 377–386. doi: 10.1007/s11248-010-9423-4
Woo, J. W., Kim, J., Kwon, S. Il, Corvalán, C., Cho, S. W., Kim, H., et al. (2015). DNA-free genome editing in plants with preassembled CRISPR-Cas9 ribonucleoproteins. Nat. Biotechnol. 33, 1162–1164. doi: 10.1038/nbt.3389
Xu, X., Pan, S., Cheng, S., Zhang, B., Mu, D., Ni, P., et al. (2011). Genome sequence and analysis of the tuber crop potato. Nature 475, 189–195. doi: 10.1038/nature10158
Yang, Y., Achaerandio, I., and Pujola, M. (2015). Classification of potato cultivars to establish their processing aptitude. J. Sci. Food Agricul. 96. doi: 10.1002/jsfa.7104
Ye, M., Peng, Z., Tang, D., Yang, Z., Li, D., Xu, Y., et al. (2018). Generation of self-compatible diploid potato by knockout of S-RNase. Nat. Plants 4, 651–654. doi: 10.1038/s41477-018-0218-6
Yevtushenko, D. P., Sidorov, V. A., Romero, R., Kay, W. W., and Misra, S. (2004). Wound-inducible promoter from poplar is responsive to fungal infection in transgenic potato. Plant Sci. 167, 715–724. doi: 10.1016/j.plantsci.2004.04.023
Zaheer, K., and Akhtar, M. H. (2016). Potato Production, Usage, and Nutrition—A Review. Crit. Rev. Food Sci. Nutr. 56, 711–721. doi: 10.1080/10408398.2012.724479
Zhang, C., Wang, D., Yang, Y., and Chen, Q. (2020). Construction of potato DM1-3-516-R44 (DM) transgenic system based on Agrobacterium transformation. Res. Square [Preprint]. doi: 10.21203/rs.2.21523/v1
Zhao, X., Jayarathna, S., Turesson, H., Fält, A. S., Nestor, G., González, M. N., et al. (2021). Amylose starch with no detectable branching developed through DNA-free CRISPR-Cas9 mediated mutagenesis of two starch branching enzymes in potato. Sci. Rep. 11:4311. doi: 10.1038/s41598-021-83462-z
Zhou, X., Zha, M., Huang, J., Li, L., Imran, M., and Zhang, C. (2017). StMYB44 negatively regulates phosphate transport by suppressing expression of PHOSPHATE1 in potato. J. Exp. Bot. 68, 1265–1281. doi: 10.1093/jxb/erx026
Zhu, H., Li, C., and Gao, C. (2020). Applications of CRISPR–Cas in agriculture and plant biotechnology. Nat. Rev. Mol. Cell Biol. 21, 661–677. doi: 10.1038/s41580-020-00288-9
Zhu, X., Gong, H., He, Q., Zeng, Z., Busse, J. S., Jin, W., et al. (2016). Silencing of vacuolar invertase and asparagine synthetase genes and its impact on acrylamide formation of fried potato products. Plant Biotechnol. J. 14, 709–718. doi: 10.1111/pbi.12421
Keywords: potato, genetic engineering, biotechnology, Agrobacterium, New Breeding Techniques, genome editing
Citation: Nahirñak V, Almasia NI, González MN, Massa GA, Décima Oneto CA, Feingold SE, Hopp HE and Vazquez Rovere C (2022) State of the Art of Genetic Engineering in Potato: From the First Report to Its Future Potential. Front. Plant Sci. 12:768233. doi: 10.3389/fpls.2021.768233
Received: 31 August 2021; Accepted: 09 December 2021;
Published: 10 January 2022.
Edited by:
Marcos Egea-Cortines, Universidad Politécnica de Cartagena, SpainReviewed by:
Allah Bakhsh, University of the Punjab, PakistanSatoko Non-aka, University of Tsukuba, Japan
Copyright © 2022 Nahirñak, Almasia, González, Massa, Décima Oneto, Feingold, Hopp and Vazquez Rovere. This is an open-access article distributed under the terms of the Creative Commons Attribution License (CC BY). The use, distribution or reproduction in other forums is permitted, provided the original author(s) and the copyright owner(s) are credited and that the original publication in this journal is cited, in accordance with accepted academic practice. No use, distribution or reproduction is permitted which does not comply with these terms.
*Correspondence: Cecilia Vazquez Rovere, dmF6cXVlei5jZWNpbGlhQGludGEuZ29iLmFy
†These authors have contributed equally to this work and share first authorship