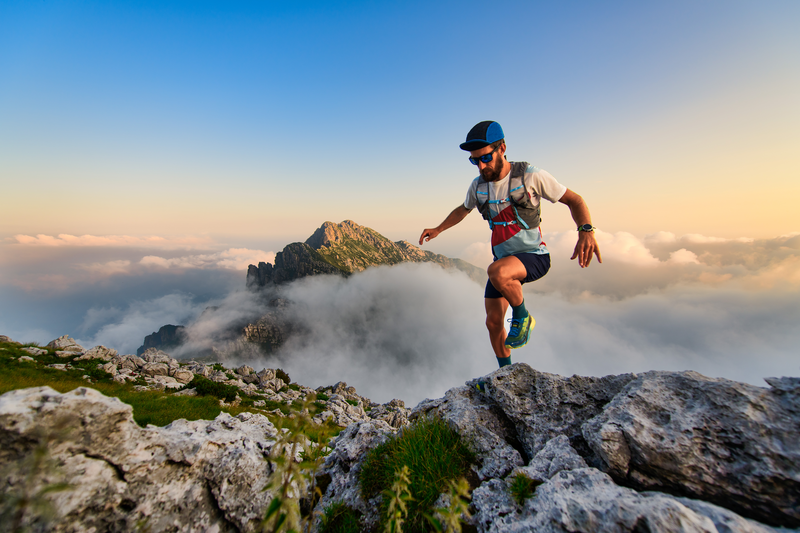
94% of researchers rate our articles as excellent or good
Learn more about the work of our research integrity team to safeguard the quality of each article we publish.
Find out more
ORIGINAL RESEARCH article
Front. Plant Sci. , 22 October 2021
Sec. Technical Advances in Plant Science
Volume 12 - 2021 | https://doi.org/10.3389/fpls.2021.765292
This article is part of the Research Topic Plant Transformation View all 20 articles
Coffea spp. are tropical plants used for brewing beverages from roasted and grounded seeds, the favorite drink in the world. It is the most important commercial crop plant and the second most valuable international commodity after oil. Global coffee trade relies on two Coffea species: C. arabica L. (arabica coffee) comprising 60% and C. canephora (robusta) comprising the remaining 40%. Arabica coffee has lower productivity and better market price than robusta. Arabica coffee is threatened by disease (i.e., coffee leaf rust), pests [i.e., Hypothenemus hampei or coffee berry borer (CBB) and nematodes], and susceptibility to climate change (i.e., drought and aluminum toxicity). Plant biotechnology by means of tissue culture inducing somatic embryogenesis (SE) process, genetic transformation, and genome editing are tools that can help to solve, at least partially, these problems. This work is the continuation of a protocol developed for stable genetic transformation and successful plant regeneration of arabica coffee trees expressing the Bacillus thuringiensis (Bt) toxin Cry10Aa to induce CBB resistance. A highly SE line with a high rate of cell division and conversion to plants with 8-month plant regeneration period was produced. To validate this capability, gene expression analysis of master regulators of SE, such as BABY BOOM (BBM), FUS3, and LEC1, embryo development, such as EMB2757, and cell cycle progression, such as ETG1 and MCM4, were analyzed during induction and propagation of non-competent and highly competent embryogenic lines. The particle bombardment technique was used to generate stable transgenic lines after 3 months under selection using hygromycin as selectable marker, and 1 month in plant regeneration. Transgenic trees developed fruits after 2 years and demonstrated expression of the Bt toxin ranging from 3.25 to 13.88 μg/g fresh tissue. Bioassays with transgenic fruits on CBB first instar larvae and adults induced mortalities between 85 and 100% after 10 days. In addition, transgenic fruits showed a seed damage lower than 9% compared to 100% of control fruits and adult mortality. This is the first report on stable transformation and expression of the Cry10Aa protein in coffee plants with the potential to control CBB.
Coffee (Coffea arabica L. and C. canephora Pierre ex A. Froehner) is the most valuable tropical export crop in the world, with an annual global coffee production of around 7.7 million tons of golden coffee, in an area of 10.5 million hectares in more than 50 countries (International Coffee Organization, 2019).
This production has been registered in spite of the susceptibility of C. arabica to diseases and insect pests, being the coffee berry borer (CBB), Hypothenemus hampei (Ferrari) (Coleoptera: Curculionidae: Scolytinae), one of the main pests in the world. CBB larvae in coffee seeds cause yield losses up to 80%, equivalent to US$500 million annually and affecting more than 25 million rural households involved in coffee production worldwide, due to reduction in grain weight, lower product quality (Moore and Prior, 1988; Vega et al., 2003), premature fruit fall due to early infestations (Schmitz and Crisinel, 1957; Kraker, 1988; Mairena-Ortíz et al., 1991), and physical damage to the fruit, which allows infestation and attacks by other pests (Leefmans, 1923; Penados and Ochoa, 1979).
Coffee berry borer control depends on the use of chemicals (i.e., endosulfan) and some biological agents, such as bethylid parasitoids (Cephalonomia stephanoderis and Prorops nasuta) and entomopathogenic fungi (Beauveria bassiana and Metarhizium anisopliae). These agents show their greater efficiency on the surface of the berry, having an effect mainly on adults (Gingerich et al., 1996; Bustillo et al., 1998).
Bacillus thuringiensis (Bt) has contributed globally to insect pest control since the 1960s (Heimpel and Angus, 1960). Currently, more than 800 Cry protein sequences have been recorded, grouped into 78 different classes, and are specifically active mainly against insects and nematodes (Crickmore et al., 1998). The optimized nucleotide sequences, which code for the active fragment of the Cry proteins of Bt in plants, have been successfully cloned, integrated, and expressed through the use of genetic engineering in different crops.
The Bt-protected crops, such as corn, cotton, soybeans, and potatoes, have shown significant benefits since their introduction in 1996. These materials provide a level of protection against insects that is generally higher as compared to conventional chemical pesticides. As a result, Bt crops require fewer synthetic pesticide applications (if any), which avoid exposure to toxic compounds; in addition, they help to preserve the population of beneficial insects, such as parasitoids and predators (Klotz-Ingram et al., 1999; Betz et al., 2000).
Transgenic coffee plants expressing Cry proteins were first developed by Leroy et al. (2000) and evaluated under field conditions by Perthuis et al. (2015). These plants express the Cry1Ac protein that confers resistance to the coffee leaf miner, Leucoptera coffeella (Lepidoptera: Lyonetiidae), with successful damage inhibition. Most of the characterized Cry toxins are active against Lepidoptera, and to a lower extent, to coleopteran species (De Maagd et al., 2001; James et al., 2009; Shah et al., 2016). Méndez-López et al. (2003) demonstrated that B. thuringiensis serovar israelensis (Bti), which contains the Cry10Aa protein at very low expression levels, exhibits high toxic levels against CBB. Subsequently, the specific and efficient activity of Cry10Aa was demonstrated in vitro toward the cotton boll weevil, Anthonomus grandis Boheman (Coleoptera: Curculionidae) (de Souza-Aguiar et al., 2012) and in transgenic cotton plants under greenhouse conditions, showing high levels of toxicity against Anthonomus grandis (Ribeiro et al., 2017).
Most genetic transformation protocols are based on the integration of the genes of interest into the plant genome in undifferentiated plant tissues, such as somatic embryos (SEMs). However, arabica coffee varieties have been demonstrated to be more difficult to transform than robusta coffee.
Long-term and fast-growing SE with a high rate of plant conversion is a very important requirement to obtain stable transgenic trees and seed development. The induction and maturation of C. arabica var. Typica SEMs were generated under osmotic stress conditions (Valencia-Lozano et al., 2019, 2021). Highly competent (HC) embryogenic lines were generated and used for genetic transformation with Bt cry10A toxin coding gene.
To understand basic molecular mechanisms affecting SE in C. arabica and genetic transformation competence, genes involved in SE, such as BABY BOOM (BBM), FUS3, and LEC1; embryo development, such as EMB2757; and cell cycle progression, such as ETG1 and MCM4, were analyzed during induction and propagation of non-competent (NC) and HC embryogenic lines.
In this study, we followed an efficient and reliable protocol for genetic transformation of C. arabica var. Typica. Fruits from transgenic trees showed expression of the Bt toxin. Bioassays with transgenic fruits induced high CBB mortality and very low seed damage. Stable transformation and expression of the Cry10Aa protein in coffee plants show potential to control the CBB.
Plant material was obtained from the protocol developed by Valencia-Lozano et al. (2019). SE of C. arabica var. Typica was induced from leaf explants collected from 8-month-old trees in Tapachula, Chiapas, Mexico. Explants were superficially disinfected according to Cabrera-Ponce et al. (2015), and the protocol for induction and propagation of SEMs was adapted from traditional medium by Van Boxtel and Berthouly (1996) and modified CP2 medium by Valencia-Lozano et al. (2019). The SE lines derived from C. arabica var. Typica after 2 months of subculture in globular stage on traditional and modified CP2 media, 24-h mannitol 0.15-M–Sorbitol 0.15-M treatment before and after bombardment, were used.
To select genes involved in the SE development process, a gene network with a high confidence (0.700) was performed with the software STRING v11.0 (http://string-db.org; Szklarczyk et al., 2019) based on C. arabica homologous genes present in the Arabidopsis thaliana genome.
The selected genes were those involved as gene master regulators of SE (BBM, FUS3, and LEC1), embryo development (EMB2757), and cell cycle (ETG1 and MCM4). These genes were analyzed during the induction and propagation process.
Gene identifier was made according to UniProt (http://www.uniprot.org), NCBI (http://www.ncbi.nlm.nih.gov), and Phytozome database (Goodstein et al., 2012). Sequences of all genes were analyzed from A. thaliana using blastN and blastP in the coffee genome homologous sequences.
Homologous sequences in C. arabica genome >40% in protein sequence with A. thaliana were considered. Proteins of C. arabica homologous with A. thaliana aligned by blastP were identified as: XP_027062561 (BBM), XP_027089900.1 (EMB2757), XP_027110645.1 (ETG1), XP_027102113.1 (FUS3), XP_027085797 (LEC1), and XP_027112176.1 (MCM4) (Table 1). Oligonucleotides were designed (Table 1) to use them in qPCR (2−ΔΔCT) analysis.
Total RNA from SEMs cultured in traditional medium (NC) and modified (CP2) medium (HC) was isolated using TRIzol (Invitrogen, Carlsbad, CA, USA) (UniProt Consortium, 2014). RNA concentration was measured by its absorbance at 260 nm (ratio 260 nm/280 nm was assessed), and its integrity confirmed by electrophoresis in agarose 2% (w/v) gels.
The cDNA samples were amplified by PCR using the SYBR Green qPCR System (Bio-Rad, Hercules, CA, USA) in Real-Time PCR Systems (CFX96 Bio-Rad). The reference genes in this work were ACT, 24S, and RPL39, according to Freitas et al. (2017) applied for the qPCR analysis of coffee SEMs.
Retfinder, NormFinder, Bestkeeper, and Delta-Ct were used in this analysis by triplicate. Relative expression was calculated, and weighted ct, and next, a delta ct in each gene was analyzed and relative amount of target gene expression using the 2−ΔΔCT method (Livak et al., 2013). The qPCR analysis was based on at least three biological replicates for each sample with three technical replicates and control treatment.
The SEMs maturation and plant regeneration of transgenic and wild-type SEMs were made according to Valencia-Lozano et al. (2021). Three transgenic events and wild-type plants were successfully acclimatized for 8 months in growth chambers. Transgenic and wild-type plants were transferred to experimental greenhouses at the IMBIOTECA (Xalapa, Veracruz, Mexico) and developed under controlled agroclimatic conditions (relative humidity >75% and temperature between 22 ± 2°C).
Flowering occurred after 12 months, and fruits were evaluated 125 days after anthesis until they showed 20% dry matter. These are the optimal conditions for the CBB infestation, required to check for the efficiency in the control of H. hampei in the transformed plants.
Transgenic fruits showed similar dimensions, within the range of commercial arabic varieties (e.g., Var. Typica and Var. Colombia) and comparable with the non-transformed wild type.
Total genomic DNA was isolated from fruits of three transgenic tree lines established under greenhouse conditions and including wild-type plants, as described by Valencia-Lozano et al. (2019). Aliquots of 20 μg of genomic DNA were digested with EcoRI and HindIII (10 U/μg each), which fragmented the cry10Aa sequence into three bands (963, 598, and 410 bp). Products were then electrophoresed in a 1.0% agarose gel and transferred to positively charged nylon membranes (Hybond-N+, Amersham, Little Chalfont, UK) using 2× SC, as described earlier (Sambrook et al., 1989). Membranes were prehybridized for 24 h at 60°C in 2 × SCP, 0.5% BSA, and hybridized overnight at 60°C (Sambrook et al., 1989). The hybridized biotin-labeled probes were detected with streptavidin antibody conjugated with alkaline phosphatase (AP) and revealed using an AP Conjugate Substrate Kit (Thermo Fisher, Vilnius, Lithuania).
To demonstrate the insecticidal efficiency of the cry10Aa gene in transgenic coffee plants, a laboratory colony of H. hampei was established from individuals collected in coffee fields (Xalapa, Veracruz, Mexico), and females were recovered (flying and walking) from each fruit (Figure 1A). As known, sibling male and female mate within the berry; males die, and the fertilized females leave the berry to find a new berry in which to deposit their eggs (Baker et al., 1992).
Figure 1. Bioassay using first instar larvae of Hypothenemus hampei. (A) Females (1.6–1.9 mm long) recovered from a laboratory colony. (B) Individualization of H. hampei females in 24-well titration dishes. (C) Close-up of coffee berry borer (CBB) drills in green coffee seed pieces (0.7 mm). (D) First instar larvae (0.019 mm) and egg (0.001 mm) after 20 days of infestation. (E) First instar larvae fed with 1 μl of transgenic plant extracts. (F) First instar larvae fed with 1 μl of control plant extract. Bars: 0.5 mm.
Females were isolated in humid chambers, disinfected with 1.2 g/L of Benomyl (Bustillo Pardey, 2006), and incubated at >75% RH, 8/16 h photoperiod, and temperature of 22 ± 3°C until complete the cycle (30 days) to have a homogeneous population. F1 females obtained under laboratory conditions were transferred to 24- and 96-well titration plates on green coffee seed pieces. Eggs were selected for the bioassay after 15 ± 5 days (Figures 1B–D).
Proteins extracted as described earlier (Valencia-Lozano et al., 2019) from three SEMs and three-leaf samples of transgenic lines, showing differential expression of the Cry10Aa among them, were quantified by ELISA. The six concentrations obtained were reported by Valencia-Lozano et al. (2019) corresponding to events 1, 2, and 3 (Table 2).
Table 2. Cry 10Aa concentrations found in SEMs and leaves from three transformation events of coffee.
Each first instar larva (2 days after hatching) was fed individually with 1 μl of total protein extract (200 mg/ml) dissolved in water with 20 μl of food dye to observe the intake of the toxin (Figure 1E). Negative control was fed with water and food dye (Figure 1F). All food dyes (red, blue, green, and yellow) were previously tested negative for any toxic effect. After 2 h, larvae were washed with distilled water to remove surface dye residues and transferred to 24-well plates, with green coffee seed pieces, under dark conditions at 25°C and 70% RH. Each experiment was performed in triplicate for a total of 60 individuals per dose. The toxic activity was evaluated daily for 10 days.
The Cry10Aa concentrations found in each transformation event were used as a dose to carry out a preliminary Probit analysis for the estimation of an LC50 (concentration that generates 50% mortality in test insects), as a statistical parameter that reflects the level of toxicity (Ibarra and Federici, 1987). A regression line was established between the Cry10Aa concentrations and the mortality percentages, both transformed to logarithms and Probit units, respectively. The main estimated parameters were: the slope, the chi-square value, and LC50.
Fruits harvested 125 days after flowering from three transgenic events of coffee were inoculated with CBB-fertilized females. The bioassay was made in closed plastic containers, where each fruit was infested with a female and incubated in a growth chamber at 25°C and RH 70%.
Female penetration to fruits, mortality, and emergence of new adults were evaluated daily. When the emergence of the first adults in control fruits was observed, a cross-section was made in all tested fruits and percent damage, development of CBB larvae, and resistance to fruits by H. hampei infestation was quantified.
Understanding the basic molecular mechanisms affecting SE in C. arabica is keys to establish genetic transformation protocols, based on the integration of the genes of interest into the plant genome in undifferentiated plant tissues, such as SEMs. A line with high SE capabilities and high cell division rate, followed by the conversion into plants in 8-month, was generated under osmotic stress conditions. This HC embryogenic lines were generated and used for genetic transformation with the cry10A gene, which showed efficient expression of the Bt toxin.
To understand the molecular mechanisms involved in SEMs development and to validate this capability, a STRING-based bioinformatic analysis with high confidence (0.700) based on C. arabica homologous sequences in the A. thaliana genome was performed. As expected, upregulation during induction and propagation of embryogenic lines, cultured in traditional medium (NC) and modified (CP2) medium (HC), was found in genes involved in SE: BABY-BOOM (XP_027062561), FUS3 (XP_027102113.1), and LEC1(XP_027085797), embryo development EMB2757 (XP_027089900.1), and cell cycle progression ETG1 (XP_02711061.1) and MCM4 (XP_027112176.1) (Figure 2).
Figure 2. Gene expression analysis by qPCR during SE induction in highly competent embryogenic line (HC-SE) and non-competent line (NC-SE). Relative expression levels were plotted based on Log2 values, normalized with RPL39 (ribosomal protein L39), ACT (β-actin), and 24S (Ribosomal protein 24S).
As expected, the expression level of LEC1, a nuclear transcription factor Y subunit B-9, was upregulated 3.17 times in the HC-SE line as compared to the regulated expression in the control of 1.65 times in NC-SE line. BBM, an AP2-like ethylene-responsive transcription factor, was upregulated 3.19 times in HC-SE line as compared with the control of 0.9 times in NC-SE. FUS3, a regulator of gene expression during late embryogenesis, was upregulated 3.84 times in HC-SE line, as compared to the control of 2.74 in NC-SE line. EMB2757/TAIMEN, which encodes a WD repeat protein with seven WD repeat motifs, was upregulated 3.39 times in HC-SE line, as compared to the control of 1.83 in NC-SE line. ETG1, an associated component of the mini-chromosome maintenance complex-binding protein that acts as a regulator of DNA replication, was also upregulated 3.57 times in HC-SE line, as compared to the control of 0.68 in NC-SE line. MCM4 acts as component of the MCM2-7 complex (MCM complex), which is the putative replicative helicase essential for DNA replication initiation and elongation, and was upregulated 3.48 times in HC-SE line, as compared in the control of 0.85 times in NC-SE line (Figure 2).
Transgenic and wild-type plants regenerated according to Valencia-Lozano et al. (2021) developed robust plants with a prominent root and leaf area (Figure 3A), and the length of the internodes. Flowering and seed and fruit production occurred after 12 months (Figure 3B). Fruits were evaluated until day 125 after anthesis (Figures 3C,D), when they showed 20% dry matter. These are the optimal conditions for CBB infestation, and therefore the optimal time to perform the final check of the efficiency in the control of H. hampei in the transformed plants. Fruits were selected from three different clones corresponding to three independent transformation events with similar dimensions within the range of commercial arabic varieties and comparable with the non-transformed wild type.
Figure 3. (A) Transgenic Coffea arabica plants grown under greenhouse conditions after 24 months. (B) Flowering of transgenic coffee trees after 18 months under greenhouse conditions. (C,D) Fruit development from transgenic coffee plants after 22 months under greenhouse conditions.
Fruits from three different transgenic events grown under greenhouse conditions were analyzed by Southern blot hybridization analysis to confirm the sexual segregation of cry10Aa gene in C. arabica var. Typica.
Expected hybridization signals of 963, 598, and 410 bp, according to the cry10Aa gene sequence digested EcoRI/HindIII, were observed in all transgenic events. The presence of positive hybridization signals of the predicted size showed that gene integration and sexual segregation of at least one foreign gene copy (cry10Aa) occurred in coffee plants derived from our highly competent SE line. No signal was detected in wild-type plants (Figure 4).
Figure 4. Southern blot and hybridization analysis of transgenic coffee fruits. (A) Electrophoresis of DNA digest of transgenic fruits and SEM of Coffea arabica digested with EcoR1/HindIII. (B) Southern blot analysis hybridized with cry10Aa-biotin of 1,993 bp probes. Lane 1, DNA from wild-type fruits; lane 2, wild-type SEMs; lane 3, transgenic fruits, event E1; lane 4, transgenic SEM event E1; lane 5, transgenic fruits, event E2; lane 6, partially digested transgenic SEM, event E2; lane 7, transgenic fruits, event E3; lane 8, partially digested transgenic SEM, event E3.
Tested transgenic clones displayed unique hybridization patterns, indicating that these transgenic plants were derived from independent transformation events. In all transgenic lines, bands with molecular weights different from expected were found. This indicates either that multiple independent insertions occurred, or that the integrated fragments are long tandem repeats resulting from re-arrangements.
The CBB bioassays were evaluated with six different concentrations of the toxin Cry10Aa quantified by ELISA from extracts (total protein) from leaves of transformed plants and embryogenic lines, using first instar larvae of H. hampei. Toxic activity of Cry10Aa against larvae of H. hampei began with mobility loss and turgor change and ended up showing body darkening after 2–5 days due to the onset of septicemia (Figure 5A).
Figure 5. Mortality of coffee berry borer (CBB) first instar larvae. (A) Average morphology of first instar larvae in 24-well titration plates at 10 days. R1, R2, R3, and R4: Replicates of bioassays with larvae fed with Cry10Aa extract. Vertical gray line, water-fed larvae. Bar: 1 mm. (B) Mortality curve for Cry 10Aa concentrations found in SEMs and leaves from three transformation events of coffee (μg/g fresh weight).
Mortality of CBB was observed after 24 h in the higher concentrations (25%), reaching 96% at day 7. After 10 days, mortality raised to 87% with a Cry10Aa concentration of 3.25 μg/g fresh weight, 92% with 4.6 μg/g, 95% with 7.8 μg/g, 97% with 8.67 μg/g, and 100% with 12.7 and 13.88 μg/g (Figure 5B).
It should be noticed that CBB larvae normally show a living range of 20–26 days; however, and in the bioassays, we observed mortalities of 100% in the middle of that period. In addition, the amount of expressed Cry10Aa guarantees a 25% immediate control (24 h), and in a period of 10 days, total control is achieved inside the fruit in transformed coffee plants (Figure 5).
As the quantification of expressed Cry10Aa varied from 3.25 to 13.88 μg/g fresh weight among the different transformation events, a relationship dose/mortality was analyzed by Probit analysis to estimate a preliminary LC50 for transgenically expressed Cry10Aa. The chi-square value was estimated at 2.34 (lower than the number of doses tested), the slope was 2.39 (it should be between 1.5 and 6), the LC50 was 1.202 (0.56 ± 2.56) μg/g, and the LC95 was 5.82 (4.55 ± 7.44) μg/fresh weight. The natural mortality in the negative control was 1.6%, which is within the recommended limits.
The lethal effect of the transgenically expressed Cry10Aa protein on H. hampei was evaluated, starting with the infestation of transgenic fruits with CBB adults, during a period of 30 days, a period in which the emergence of the first adults of the next generation was observed in control fruits.
We quantified four steps of colonization levels by the female within the berry: (1) the female initiated the penetration of the exocarp before dying; (2) the female penetrated the endocarp, and only part of the abdomen is visible before dying; (3) the female is no longer visible and has bored into the endosperm where it died; and (4) the female constructed galleries and oviposited within the seed, where emerging larvae eventually died.
Each fruit was individualized in a container with a fertilized (flying) female. In the first 24 h, 100% of control fruits were perforated, and 67% of clone 8 (Event 2) (Figure 6). About 67% of fruits of clone 9 (Event 3) were perforated 48 h later, and fruits from clone 6 (Event 1), only 33% were partially perforated until after 20 days.
Figure 6. Fruits 125 days after anthesis from Cry10Aa transgenic plants infested with coffee berry borer (CBB) adults. Three transformation events. Events 1, 2, and 3 and a wild-type plant (A–D) were selected for the performance of bioassays. At the right-hand side, the evaluation of damage by cross section of fruits of F1 in the three events and the wild-type fruits after 30 days.
Scrapings on the exocarp near the micropyle and excrement were observed on the fruit, and on dead females, which indicates that the insect fed on the fruit, but was unsuccessful to penetrate. In 67% of fruits of this clone, the average female survival was 5 days, but after day 20 the rest of the females were able to drill the fruit but unable to penetrate it.
Fruits from clone 8 (Event 2) showed perforations in the peduncle and in the micropyle; however, 50% of females were dead on day 10. These qualitative evaluations indicated the activity of adults within the fruit from day 1 to day 10, shown by the presence of excrement outside the micropyle. This was more evident in the wild-type fruits, whereas some other events showed fewer excrement such as in clone 9 (Event 3) and clone 8 (Event 2), but a complete absence was detected in fruits from clone 6 (Event 1). Surveillance kept going until day 30, when the emergence of the first adults was observed in the control fruits. No adults emerged from any of the transgenic fruits. At this point, fruits were cross-sectioned to evaluate larval survival and levels of infestations (Figure 6).
About clone 6, female penetration in the transformed fruits was <12%. Some adults were able to drill to the interior of the fruit, causing no damage. In no case, larvae were found in transgenic fruits. In 33% of fruits (Figure 6), the presence of an egg was observed for a period of 10 days, but most females died, and no damage was detected on the seed. This clone showed the highest expression level of the Cry10Aa toxin.
Conversely, 70% of clone 8 fruits showed boring of multiple entrances and exits of females, with an average of only three adults, and two pupae, within a period of 30 days. Seed damage was much lower than that shown by the control fruits (Figure 6D).
About 30% of seeds showed saprophytic damage due to the penetration of bacteria into the galleries, but the presence of progeny development stages was not observed, and the female was dead (Figure 6B1)
Clone 9 presented contamination by fungus. Although the CBB could survive under these conditions, no progeny development was observed, nor was the fruit damage through galleries (Figure 6C1).
The results mentioned above demonstrate the toxic activity of the Cry10Aa protein on the adults and first instar larvae. Given the damage observed in the fruits of clone 8 and the absence of larvae, it could be attributed to a toxic activity directly to the infesting adults or to first instar larvae in the first days of the evaluation.
SEMs in plants can be the result of a natural (Garcês et al., 2007) or an artificial process (plant tissue culture medium), and it occurs when a somatic cell becomes into a totipotent embryonic stem cell to differentiate in an embryo with the potential to become a new plant. SEMs lack the development of an embryo sac, endosperm, and seed coat. SEMs play a critical role in clonal propagation, synthetic seed production, cryopreservation, genetic transformation, and genome editing.
In coffee, SEMs induced from leaf explants have been the most widely used target tissue in genetic transformation (Cortleven et al., 2019). SE induction and proliferation in coffee is time-consuming, ranging from 9 to 15 months until they can be used for stable genetic transformation (Barry-Etienne et al., 2002; Campos et al., 2017). In this work, we have induced a long-term induction and plant regeneration of SE lines of C. arabica var. Typica, previously used for stable transformation of coffee with a Bt toxin (Valencia-Lozano et al., 2019).
To understand the basic molecular mechanisms of SE induction in our embryogenic line, a set of genes derived from a network with a high confidence (0.700) performed in STRING (v11.0, http://string-db.org), based on C. arabica homologous genes present in A. thaliana genome, were evaluated.
The master regulators of SE, BBM, LEC1, and FUS3 were upregulated in our competent SE line (HC-SE) (5.0-, 5.9-, and 7.3-fold change, respectively) over the NC-SE line. Repression of complete SE competence was observed in double (lec1 lec2, lec1 fus3, and lec2 fus3) and triple (fus3 lec1 lec2) mutants in Arabidopsis. These mutants were able to regenerate plants via organogenesis derived from roots (Gaj et al., 2005). In cassava, MeLEC1 and MeLEC2 were highly upregulated in SEM cells in contrast with mature tissues. Increased levels were at early SE induction in the transition from somatic to embryogenic state (Brand et al., 2019). BBM promotes cell proliferation, differentiation, and morphogenesis, specifically during embryogenesis. BBM-induced embryogenesis relies on transcriptional activation of LEC1 and LEC2. LEC1 is a transcriptional activator required for both embryo maturation and cellular differentiation and FUS3, a regulator of gene expression during late embryogenesis (Horstman et al., 2017).
On the other hand, TANMEI/EMB2757 was 7-fold upregulated in our HC-SE line over the NC-SE line. It encodes a regulatory WD repeat protein required for both early and late phases of zygotic embryo development (Yamagishi et al., 2005) and SEM (Baster et al., 2009). In Arabidopsis, tanmei/emb2757 (tan) mutation resulted in a total loss of embryogenic and organogenic capacity of cultured tissues, suggesting the involvement of TAN gene in basic cellular processes related to cell growth and differentiation. TANMEI/EMB2757 is a cell cycle checkpoint regulator, as it triggers the halt of cell cycle progression in the presence of DNA cross-linking agents.
Additionally, ETG1 and MCM4 genes were upregulated (9.3- and 10.3-fold) in the HC-SE line over the NC-SE one. They are involved in the cell cycle and maintenance of the genome. ETG1 binds to the MCM complex during late S phase and acts by promoting the disassembly of the MCM complex from chromatin. MCM4 double mutation in Arabidopsis impairs pollen development and is essential for embryo development (Long et al., 2019). MCM4 has a role in the mitotic cycle, consequently in cell proliferation, which is key for plant growth. Mutations induce different degrees of reduction in rosette size (González et al., 2020). ETG1 mutants are macroscopically normal, but an increase in cell size and endoreduplication occurs. Analysis of etg1, tert, and mid mutants was involved in DNA stress checkpoint activation indispensable for correct morphogenesis and survival (Cools and De Veylder, 2009).
Genetic transformation of plants can stall at several key points, including the acquisition of a stem cell-like state in cells as they re-enter the cell cycle, progression between the G1 and S phases of the cycle, and the differentiation of the transformed cell into a new embryo (Arias et al., 2006). Plant tissue culture medium (chemical formulation, plant growth regulators, and different stressing factors) and environmental signals (light and temperature) are essential for correct activation of the cell cycle during in vitro culture of plants. It will also depend on personal perception about plant growth regulators as the most important activators of differentiation of SEMs under in vitro conditions.
The upregulation of LEC1, FUS3, BBM, TANMEI/EMB2757, ETG1, and MCM4 in our HC-SE line reveals that the molecular mechanism for SE induction, maturation, and plant regeneration is partially due to the expression of these genes. Further experiments of transcriptomic and proteomic are required to elucidate these molecular mechanisms.
Worldwide, H. hampei is the most damaging insect pest of coffee, causing an annual loss of around US$500 million. Global warming is driving CBB invasion and spread worldwide to warmer producer areas. CBB is the only insect pest of coffee that feeds and completes its life cycle in the coffee seeds (Johnson et al., 2020).
The major components of CBB integrated pest management (IPM) strategies used in most coffee-growing regions around the world include: monitoring of populations (traps and tree sampling), cultural control (preharvest and post-harvest sanitation), biological control (use of natural enemies), chemical control (use of insecticides and repellents), and physical control (exclusion netting and border crops) (Cabrera-Ponce et al., 2019).
Transgenic plants with insect resistance traits (IRTs) have contributed significantly to the agricultural industry on a commercial basis. The development of plants that undermine insect attack has been achieved by the expression of genes that encode toxins normally produced as Cry proteins in the soil bacterium Bt.
Genes for different Bt endotoxins are derived from assorted strains of the bacterium, and each insecticidal protein has different activity spectrum for various insect pests within the orders Lepidoptera and Coleoptera. As a toxic mechanism, Cry proteins bind to specific receptors on the membranes of mid-gut epithelial cells, resulting in the rupture of those cells. Any organism that lacks the appropriate gut receptors cannot be affected by the Cry proteins expressed in plants (Dorsch et al., 2002).
The toxic activity of Cry10Aa protein against coleopterans, such as CBB, when they tested individually all the Bti crystal components, except the Cry10A, and no toxicity was detected. The mortality of 52% was obtained using spore–crystal complex and 63% pure crystal with an estimated LC50 for the CBB of 219.5 ng/cm2 of diet.
This estimated LC50 is within the range reported for Anthonomus grandis, when using the same toxin transgenically expressed in cotton (Ribeiro et al., 2017). The importance of this work lies in the fact that it is the first time that the action of a coffee plant, transformed with the cry10Aa gene, shows efficient control of first instar larvae of CBB. Moreover, the 100% mortality shown by the two highest expression levels among the transformed events showed the great potential of these approaches to efficiently control this pernicious pest.
A successful protocol of genetic transformation of C. arabica var. Typica to integrate cry10Aa gene of Bt and be expressed was developed by Valencia-Lozano et al. (2019). These plants were grown in a greenhouse until fruit and seed sets were achieved. Different protein concentration was found in each transgenic line analyzed, ranged from 3.25 to 13.88 μg/g fresh weight. In cotton transgenic plants expressing Cry10Aa protein, a concentration of 6.35 μg/ml was found to be efficient to control Anthonomus grandis (Ribeiro et al., 2017).
As expected, variation of Cry10Aa expression was found in fruits of the T1 generation derived from three different events of transformation. Bioassays with first instar larvae and adults found that 75% of seeds was able to control CBB, yielding a 3:1 ratio, as a mendelian inheritance. This ratio was obtained on the T1 generation. Once homozygous lines are obtained, we expect a 90% efficiency or higher.
Evaluations made on fruits of transgenic plants revealed a direct effect on the infestation capacity of CBB, showing for the first time, the toxic effect of a Bt toxin on adults and larvae of an insect species, and an efficient control of H. hampei.
Physical damage shown by transgenic fruits varied from absolutely no damage to small holes in coffee seeds. Wild-type fruits showed that when challenged with one female CBB, they not only penetrated but they also built galleries for oviposition, reproduction, and larval feeding on the endosperm.
These results showed that the expression of the Cry10Aa protein in C. arabica plants is an efficient alternative to control CBB. It is important to notice that recombinant Cry10Aa protein has no apparent toxic effect in mice derived from genotoxic and hepatotoxic assays, highlighting its biosafety potential for use in transgenic crops (de Souza-Freire et al., 2014).
Transgenic plants evaluated in this work represent IRTs that potentially could have an impact on the environment by avoiding massive insecticide use and saving in carbon dioxide emissions (Brookes and Barfoot, 2016, 2017). In addition, these plants also have several agronomic traits that potentially can be used to resolve several problems in coffee farming (Valencia-Lozano et al., 2021).
We demonstrated that the expression of Cry10Aa in elite embryogenic line allowed the transformed coffee plants to be toxic toward adults and larvae of H. hampei. This is the first report of coffee plants resistant to CBB.
Fruits of genetically transformed plants showed inhibition on the development and infestation capacity of H. hampei females, showing a successful and applicable control of this pest of global economic importance in C. arabica.
A significant improvement of SE resulted in solving important problems such as germination, time, and efficiency of genetic transformation.
The raw data supporting the conclusions of this article will be made available by the authors, without undue reservation.
EV-L conceived and designed the research, performed the experiments, analyzed the data, and wrote the manuscript. JC-P supervised the study, analyzed the data, and wrote the manuscript. JN-C involved in the supervision of greenhouse plants and analyzed the manuscript. JI conceived the research, supervision, analysis of data, and wrote the manuscript. All authors read and approved the manuscript.
The authors declare that the research was conducted in the absence of any commercial or financial relationships that could be construed as a potential conflict of interest.
All claims expressed in this article are solely those of the authors and do not necessarily represent those of their affiliated organizations, or those of the publisher, the editors and the reviewers. Any product that may be evaluated in this article, or claim that may be made by its manufacturer, is not guaranteed or endorsed by the publisher.
The authors thank Rosalia Vuelvas-Nolasco and Victoria Estefania Ruiz Molina for their help in the field collection and bioassays and Javier Luevano Borroel and Gabriel Ordoñez for their technical assistance in insectary and laboratory.
Arias, R. S., Filichkin, S. A., and Strauss, S. H. (2006). Divide and conquer: development and cell cycle genes in plant transformation. Trends Biotechnol. 24, 267–273. doi: 10.1016/j.tibtech.2006.04.007
Baker, P. S., Barrera, J. F., and Rivas, A. (1992). Life-history studies of the coffee berry borer (Hypothenemus hampei, Scolytidae) on coffee trees in southern Mexico. J. Appl. Ecol. 29, 656–662. doi: 10.2307/2404473
Barry-Etienne, D., Bertrand, B., Vasquez, N., and Etienne, H. (2002). Comparison of somatic embryogenesis-derived Coffee (Coffea arabica L.) plantlets regenerated in vitro or ex vitro: morphological, mineral and water characteristics. Ann. Bot. 90, 77–85. doi: 10.1093/aob/mcf149
Baster, P., Ledwoń, A., Gliwicka, M., Trojanowska, A., and Gaj, M. D. (2009). Arabidopsis tanmei/emb2757 embryo mutant is defective for in vitro plant morphogenesis. Plant Cell Tissue Organ Cult. 99, 305–312. doi: 10.1007/s11240-009-9605-z
Betz, F. S., Hammond, B. G., and Fuchs, R. L. (2000). Safety and advantages of Bacillus thuringiensis-protected plants to control insect pests. Regul. Toxicol. Pharmacol. 32, 156–173. doi: 10.1006/rtph.2000.1426
Brand, A., Quimbaya, M., Tohme, J., and Chavarriaga-Aguirre, P. (2019). Arabidopsis LEC1 and LEC2 orthologous genes are key regulators of somatic embryogenesis in Cassava. Front. Plant Sci. 10:673. doi: 10.3389/fpls.2019.00673
Brookes, G., and Barfoot, P. (2016). Global income and production impacts of using GM crop technology 1996–2014. GM Crops Food 7, 38–77. doi: 10.1080/21645698.2016.1176817
Brookes, G., and Barfoot, P. (2017). GM Crops: Global Socio-Economic and Environmental Impacts 1996-2015. Dorchester: PG Economics Ltd., 1–201.
Bustillo Pardey, A. E. (2006). A review of the coffee berry borer, Hypothenemus hampei (Coleoptera: Curculionidae: Scolytinae), in Colombia. Revista Colombiana de Entomología 32, 101–116.
Bustillo, A. E., Cárdenas, R., Villalba, D. A., Benavides, P., Orozco, J., and Posada, F. J. (1998). Manejo integrado de la broca del café: Hypothenemus hampei Ferrari en Colombia. Available online at: http://hdl.handle.net/10778/848 (accessed September 11, 2019).
Cabrera-Ponce, J. L., López, L., León-Ramírez, C. G., Jofre-Garfias, A. E., and Verver-y-Vargas, A. (2015). Stress induced acquisition of somatic embryogenesis in common bean Phaseolus vulgaris L. Protoplasma 252, 559–570. doi: 10.1007/s00709-014-0702-4
Cabrera-Ponce, J. L., Valencia-Lozano, E., and Trejo-Saavedra, D. L. (2019). “Genetic modifications of Corn,” in Corn, ed Serna-Saldivar (Cambridge, MA: AACC International Press), 43–85. doi: 10.1016/B978-0-12-811971-6.00003-6
Campos, N. A., Panis, B., and Carpentier, S. C. (2017). Somatic embryogenesis in coffee: the evolution of biotechnology and the integration of omics technologies offer great opportunities. Front. Plant Sci. 8:1460. doi: 10.3389/fpls.2017.01460
Cools, T., and De Veylder, L. (2009). DNA stress checkpoint control and plant development. Curr. Opin. Plant Biol. 12, 23–28. doi: 10.1016/j.pbi.2008.09.012
Cortleven, A., Leuendorf, J. E., Frank, M., Pezzetta, D., Bolt, S., and Schmülling, T. (2019). Cytokinin action in response to abiotic and biotic stresses in plants. Plant Cell Environ. 42, 998–1018. doi: 10.1111/pce.13494
Crickmore, N., Zeigler, D. R., Feitelson, J., Schnepf, E., Van Rie, J., Lereclus, D., et al. (1998). Revision of the nomenclature for the Bacillus thuringiensis pesticidal crystal proteins. Microbiol. Mol. Biol. Rev. 62, 807–813. doi: 10.1128/MMBR.62.3.807-813.1998
De Maagd, R. A., Bravo, A., and Crickmore, N. (2001). How Bacillus thuringiensis has evolved specific toxins to colonize the insect world. Trends Genet. 17, 193–199. doi: 10.1016/S0168-9525(01)02237-5
de Souza-Aguiar, R. W., Martins, E. S., Ribeiro, B. M., and Monnerat, R. G. (2012). Cry10Aa protein is highly toxic to Anthonomus grandis Boheman (Coleoptera: Curculionidae), an important insect pest in Brazilian cotton crop fields. Bt Res. 3, 21–23. doi: 10.5376/bt.2012.03.0004
de Souza-Freire, I., Miranda-Vilela, A. L., Barbosa, L. C. P., Martins, E. S., Monnerat, R. G., and Grisolia, C. K. (2014). Evaluation of cytotoxicity, genotoxicity and hematotoxicity of the recombinant spore-crystal complexes Cry1Ia, Cry10Aa and Cry1Ba6 from Bacillus thuringiensis in Swiss mice. Toxins 6, 2872–2885 doi: 10.3390/toxins6102872
Dorsch, J. A., Candas, M., Griko, N. B., Maaty, W. S. A., Midboe, E. G., Vadlamudi, R. K., et al. (2002). Cry1A toxins of Bacillus thuringiensis bind specifically to a region adjacent to the membrane-proximal extracellular domain of BT-R1 in Manduca sexta: involvement of a cadherin in the entomopathogenicity of Bacillus thuringiensis. Insect Biochem. Mol. Biol. 32, 1025–1036. doi: 10.1016/S0965-1748(02)00040-1
Freitas, N. C., Barreto, H. G., Fernandes-Brum, C. N., Moreira, R. O., Chalfun-Junior, A., and Paiva, L. V. (2017). Validation of reference genes for qPCR analysis of Coffea arabica L. somatic embryogenesis-related tissues. Plant Cell Tissue Organ Cult. 128, 663–678. doi: 10.1007/s11240-016-1147-6
Gaj, M. D., Zhang, S., Harada, J. J., and Lemaux, P. G. (2005). Leafy cotyledon genes are essential for induction of somatic embryogenesis of Arabidopsis. Planta 222, 977–988. doi: 10.1007/s00425-005-0041-y
Garcês, H. M., Champagne, C. E., Townsley, B. T., Park, S., Malhó, R., Pedroso, M. C., et al. (2007). Evolution of asexual reproduction in leaves of the genus Kalanchoë. Proc. Natl. Acad. Sci. U.S.A. 104, 15578–15583. doi: 10.1073/pnas.0704105104
Gingerich, D. P., Borsa, P., Suckling, D. M., and Brun, L. O. (1996). Inbreeding in the coffee berry borer, Hypothenemus hampei (Coleoptera: Scolytidae) estimated from endosulfan resistance phenotype frequencies. Bull. Entomol. Res. 86, 667–674. doi: 10.1017/S0007485300039183
González, R., Butković, A., Rivarez, M. P. S., and Elena, S. F. (2020). Natural variation in Arabidopsis thaliana rosette area unveils new genes involved in plant development. Sci. Rep. 10:17600. doi: 10.1038/s41598-020-74723-4
Goodstein, D. M., Shu, S., Howson, R., Neupane, R., Hayes, R. D., Fazo, J., et al. (2012). Phystozome: a comparative platform for green plant genomics. Nuclec Acids Res. 40, D1178–D1186. doi: 10.1093/nar/gkr944
Horstman, A., Li, M., Heidmann, I., Weemen, M., Chen, B., Muino, J. M., et al. (2017). The BABY BOOM transcription factor activates the LEC1-ABI3-FUS3-LEC2 network to induce somatic embryogenesis. Plant Physiol. 175, 848–857. doi: 10.1104/pp.17.00232
Ibarra, J. E., and Federici, B. A. (1987). An alternative bioassay employing neonate larvae for determining the toxicity of suspended particles to mosquitoes. J. Am. Mosquito Control Assoc. 3, 187–192.
International Coffee Organization (2019). Trade Statistics International Coffee Organization. Available online at: http://www.ico.org/trade_statistics.asp (accessed April 30, 2019).
James, B. L., Kret, J., Patrick, J. E., Kearns, D. B., and Fall, R. (2009). Growing Bacillus subtilis tendrils sense and avoid each other. FEMS Microbiol. Lett. 298, 12–19. doi: 10.1111/j.1574-6968.2009.01665.x
Johnson, M. A., Ruiz-Diaz, C. P., Manoukis, N. C., and Verle Rodrigues, J. C. (2020). Coffee berry borer (Hypothenemus hampei), a global pest of coffee: perspectives from historical and recent invasions, and future priorities. Insects 11:882. doi: 10.3390/insects11120882
Klotz-Ingram, C., Jans, S., Fernandez-Cornejo, J., and McBride, W. (1999). Farm-Level Production Effects Related to the Adoption of Genetically Modified Cotton for Pest Management. Available online at: http://hdl.handle.net/10355/1203 (accessed January 2, 2021).
Kraker, J. D. (1988). The Coffee Berry Borer Hypothenemus hampei (Ferr.): Factors Affecting Emergence and Early Infestation. Report of field experiments. Centro de Investigaciones Ecológicas del Sureste, Tapachula.
Leefmans, S. (1923). De Koffiebessenboeboek (Setephanoderes hampei Ferrari: coffeae Hagedorn). I. Levenswijze en oecologie. Mededeelingen van het Instituut voor Plantenziekten 57:93.
Leroy, T., Henry, A. M., Royer, M., Altosaar, I., Frutos, R., Duris, D., et al. (2000). Genetically modified coffee plants expressing the Bacillus thuringiensis cry 1Ac gene for resistance to leaf miner. Plant Cell Rep. 19, 382–385. doi: 10.1007/s002990050744
Livak, K. J., Wills, Q. F., Tipping, A. J., Datta, K., Mittal, R., Goldson, A. J., et al. (2013). Methods for qPCR gene expression profiling applied to 1440 lymphoblastoid single cells. Methods 59, 71–79. doi: 10.1016/j.ymeth.2012.10.004
Long, Y. P., Xie, D. J., Zhao, Y. Y., Shi, D. Q., and Yang, W. C. (2019). BICELLULAR POLLEN 1 is a modulator of DNA replication and pollen development in Arabidopsis. N. Phytol. 222, 588–603. doi: 10.1111/nph.15610
Mairena-Ortíz, M., Rodríguez, M. A., Ubeda Herrera, R., and Cruz Mora, N. (1991). Diagnóstico del cultivo del café (Coffea arabica L.) en áreas del Proyecto Denis Gutiérrez, Pancasan. Ministerio de Agricultura y Ganadería, Managua (Nicaragua) Centro Nacional de Investigación del Café, Matagalpa (Nicaragua). Departamento de Protección Vegetal.
Méndez-López, I., Basurto-Ríos, R., and Ibarra, J. E. (2003). Bacillus thuringiensis serovar israelensis is highly toxic to the coffee berry borer, Hypothenemus hampei Ferr. (Coleoptera: Scolytidae). FEMS Microbiol. Lett. 226, 73–77. doi: 10.1016/S0378-1097(03)00557-3
Moore, D., and Prior, C. (1988). “Present status of biological control of the coffee berry borer Hypothenemus hampei,” in Brighton Crop Protection Conference. Pests and Diseases-1988, Vol. 3 (British Crop Protection Council), 1119–1124. Available online at: https://www.cabdirect.org/cabdirect/abstract/19911151695 (accessed July 6, 2021).
Penados, R. R., and Ochoa, M. H. (1979). La consistencia del fruto del café y su importancia en el control de broca, Hypothenemus hampei Ferr. Revista Cafetalera (Guatemala, Guatemala), 10, 12, 14–16, 32–36, 50.
Perthuis, B., Vassal, J. M., Fenouillet, C., and Leroy, T. (2015). Cry1Ac insecticidal protein levels in genetically modified Coffea canephora Pierre coffee plants were negatively correlated with the growth speed in a field experiment. Euphytica 202, 373–383. doi: 10.1007/s10681-014-1258-2
Ribeiro, T. P., Arraes, F. B. M., Lourenço-Tessutti, I. T., Silva, M. S., Lisei-de-Sá, M. E., Lucena, W. A., et al. (2017). Transgenic cotton expressing Cry10Aa toxin confers high resistance to the cotton boll weevil. Plant Biotechnol. J. 15, 997–1009. doi: 10.1111/pbi.12694
Sambrook, J., Fritsch, E. F., and Maniatis, T. (1989). Molecular Cloning: A Laboratory Manual, 2nd Edn. New York, NY: Cold Spring Harbor Laboratory Press.
Schmitz, G., and Crisinel, P. (1957). La lutte contre Stephanoderes hampei Ferr. Bélgica: Série Scientifique-Institut National pour l'Etude Agronomique du Congo Belge, 156.
Shah, A. T., Favaro, L., Alibardi, L., Cagnin, L., Sandon, A., Cossu, R., et al. (2016). Bacillus sp. strains to produce bio-hydrogen from the organic fraction of municipal solid waste. Appl. Energy 176, 116–124. doi: 10.1016/j.apenergy.2016.05.054
Szklarczyk, D., Gable, A. L., Lyon, D., Junge, A., Wyder, S., Huerta-Cepas, J., et al. (2019). STRING v11: protein–protein association networks with increased coverage, supporting functional discovery in genome-wide experimental datasets. Nucleic Acids Res. 47, D607–D613. doi: 10.1093/nar/gky1131
UniProt Consortium (2014). Activities at the universal protein resource (UniProt). Nucleic Acids Res. 42, D191–D198. doi: 10.1093/nar/gkt1140
Valencia-Lozano, E., Cabrera-Ponce, J. L., Gómez-Lim, M. A., and Ibarra, J. E. (2019). Development of an efficient protocol to obtain transgenic coffee, Coffea arabica L., expressing the Cry10Aa toxin of Bacillus thuringiensis. Int. J. Mol. Sci. 20:5334. doi: 10.3390/ijms20215334
Valencia-Lozano, E., Ibarra, J. E., Herrera-Ubaldo, H., De Folter, S., and Cabrera-Ponce, J. L. (2021). Osmotic stress-induced somatic embryo maturation of coffee Coffea arabica L., shoot and root apical meristems development and robustness. Sci. Rep. 11:9661. doi: 10.1038/s41598-021-88834-z
Van Boxtel, J., and Berthouly, M. (1996). High frequency somatic embryogenesis from coffee leaves. Plant Cell Tissue Organ Cult. 44, 7–17. doi: 10.1007/BF00045907
Vega, F. E., Rosenquist, E., and Collins, W. (2003). Global project needed to tackle coffee crisis. Nature 425, 343–343. doi: 10.1038/425343a
Keywords: Cry10Aa, Bacillus thuringiensis, coffee transformation, coffee berry borer, somatic embryogenesis
Citation: Valencia-Lozano E, Cabrera-Ponce JL, Noa-Carrazana JC and Ibarra JE (2021) Coffea arabica L. Resistant to Coffee Berry Borer (Hypothenemus hampei) Mediated by Expression of the Bacillus thuringiensis Cry10Aa Protein. Front. Plant Sci. 12:765292. doi: 10.3389/fpls.2021.765292
Received: 26 August 2021; Accepted: 21 September 2021;
Published: 22 October 2021.
Edited by:
Horacio Esteban Hopp, University of Buenos Aires, ArgentinaReviewed by:
Laura Mabel Radonic, National Institute of Agricultural Technology (INTA), ArgentinaCopyright © 2021 Valencia-Lozano, Cabrera-Ponce, Noa-Carrazana and Ibarra. This is an open-access article distributed under the terms of the Creative Commons Attribution License (CC BY). The use, distribution or reproduction in other forums is permitted, provided the original author(s) and the copyright owner(s) are credited and that the original publication in this journal is cited, in accordance with accepted academic practice. No use, distribution or reproduction is permitted which does not comply with these terms.
*Correspondence: Jorge E. Ibarra, am9yZ2UuaWJhcnJhQGNpbnZlc3Rhdi5teA==
Disclaimer: All claims expressed in this article are solely those of the authors and do not necessarily represent those of their affiliated organizations, or those of the publisher, the editors and the reviewers. Any product that may be evaluated in this article or claim that may be made by its manufacturer is not guaranteed or endorsed by the publisher.
Research integrity at Frontiers
Learn more about the work of our research integrity team to safeguard the quality of each article we publish.