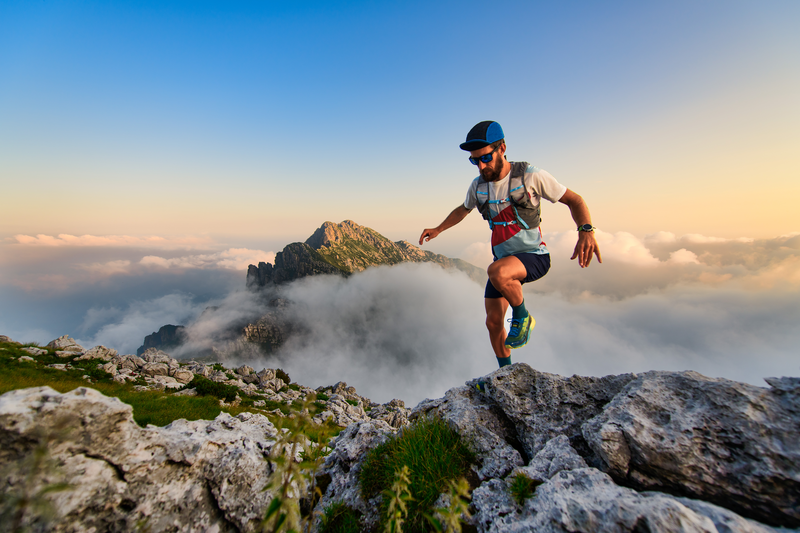
95% of researchers rate our articles as excellent or good
Learn more about the work of our research integrity team to safeguard the quality of each article we publish.
Find out more
ORIGINAL RESEARCH article
Front. Plant Sci. , 20 October 2021
Sec. Plant Biotechnology
Volume 12 - 2021 | https://doi.org/10.3389/fpls.2021.757186
Diterpene biosynthesis commonly originates with the methylerythritol phosphate (MEP) pathway in chloroplasts, leading to the C20 substrate, geranylgeranyl pyrophosphate (GGPP). The previous work demonstrated that over-expression of genes responsible for the first and last steps in the MEP pathway in combination with GERANYLGERANYL PYROPHOSPHATE SYNTHASE (GGPPS) and CASBENE SYNTHASE (CAS) is optimal for increasing flux through to casbene in Nicotiana benthamiana. When the gene responsible for the last step in the MEP pathway, 4-HYDROXY-3-METHYLBUT-2-ENYL DIPHOSPHATE REDUCTASE (HDR), is removed from this combination, casbene is still produced but at lower amounts. Here, we report the unexpected finding that this reduced gene combination also results in the production of 16-hydroxy-casbene (16-OH-casbene), consistent with the presence of 16-hydroxy-geranylgeranyl phosphate (16-OH-GGPP) in the same material. Indirect evidence suggests the latter is formed as a result of elevated levels of 4-hydroxy-3-methyl-but-2-enyl pyrophosphate (HMBPP) caused by a bottleneck at the HDR step responsible for conversion of HMBPP to dimethylallyl pyrophosphate (DMAPP). Over-expression of a GERANYLLINALOOL SYNTHASE from Nicotiana attenuata (NaGLS) produces 16-hydroxy-geranyllinalool (16-OH-geranyllinalool) when transiently expressed with the same reduced combination of MEP pathway genes in N. benthamiana. This work highlights the importance of pathway flux control in metabolic pathway engineering and the possibility of increasing terpene diversity through synthetic biology.
Many of the gem-dimethylcyclopropyl class of bioactive casbene-derived diterpenoids from plants have been shown to have pharmacological activities. Low abundance in their natural host (Hohmann et al., 2000; Johnson et al., 2008) along with their structural complexity (Jorgensen et al., 2013; Kawamura et al., 2016; Hashimoto et al., 2017) has led to efforts to engineer alternative microbial (Hill et al., 1996; Callari et al., 2018; Wong et al., 2018), algal (Mehrshahi et al., 2020), or plant-based production platforms (Forestier et al., 2021). The majority of diterpenes in plants are biosynthesized in chloroplasts (Rohmer et al., 1993; McGarvey and Croteau, 1995; Lichtenthaler et al., 1997) from the five-carbon building blocks, isopentyl pyrophosphate (IPP) and dimethylallyl pyrophosphate (DMAPP), provided by the MEP pathway and combined together in a three-to-one ratio to form the C20 precursor, GGPP (Gershenzon and Croteau, 1993).
We recently developed a Nicotiana benthamiana platform optimized for production of casbene and derivatives by engineering flux through the MEP pathway (Forestier et al., 2021). We demonstrated how this platform could be used for production of the lathyrane jolkinol C by introduction of functionally characterized P450 oxidases (Forestier et al., 2021). The elucidation of the biosynthetic steps from casbene to the tigliane (Kulkosky et al., 2001; Kissin and Szallasi, 2011; De Ridder et al., 2021), ingenane (Siller et al., 2010) and jatrophane (Corea et al., 2009; Hadi et al., 2013) classes of diterpenoids has also been investigated but remains to be resolved.
In our work on N. benthamiana to optimize flux through the MEP pathway to GGPP – the substrate for casbene production – we unexpectedly detected the novel compound, 16-hydroxy-casbene. The design of our experiments suggested that this metabolite did not arise from a hydroxylation downstream of GGPP and we therefore hypothesized that 16-hydroxy-casbene could derive from an alternative substrate. Herein, we present results leading us to conclude that 16-hydroxy-GGPP can act as a novel precursor for diterpene biosynthesis.
Arabidopsis thaliana cDNAs of 1-DEOXY-D-XYLULOSE-5-PHOSPHATE SYNTHASE (DXS, AT4G15560.1), 4-HYDROXY-3-METHYLBUT-2-ENYL DIPHOSPHATE REDUCTASE (HDR, AT4G34350.1, Phillips et al., 2008), and GERANYLGERANYL PYROPHOSPHATE SYNTHASE 11 (GGPPS11, AT4G36810.1, Beck et al., 2013) coding for plastidial enzymes referenced in TAIR (The Arabidopsis Information Resource) and cDNA of CASBENE SYNTHASE (CAS) from Jatropha curcas (King et al., 2014) were cloned into pEAQ-HT (Sainsbury et al., 2009) vector as described in Forestier et al. (2021). A codon optimized cDNA of NaGLS based on the sequence accession number XM_019410085 was synthesized by gBlock IDT with extensions allowing to clone it directly with In-Fusion® in AgeI/StuI linearized pEAQ-HT vector. Transient expression in wild-type N. benthamiana was performed as described in Forestier et al. (2021).
We detected both casbene and 16-OH-casbene in transiently expressed plants by extracting around 200mg of dry material with 5ml of hexane containing 100μg/ml of β-caryophyllene, then sonicating for 15min. We quantified the compounds by GC–MS as detailed in Forestier et al. (2021). For geranyllinalool and its derivatives, we extracted around 150mg of dry weigh (DW) of infiltrated tobacco with 1ml of ethyl acetate containing 100mg/L of β-caryophyllene. The samples were shaken overnight at 2000rpm on a IKA Vibrax VXR basic shaker and then centrifuged, and 100μl of the supernatant was used directly for GC–MS.
We isolated GGPP and 16-OH-GGPP by adapting the protocol described by Nagel et al. (2014). Approximately 750mg of ground dry material was extracted with 15ml of methanol/H2O (7:3, v/v) and sonicated for 30min. We then added 5ml of water to the mixture, centrifuged for 3min at 2000g, and filtered through Whatman filter paper grade 1 and cotton. The cleared extracts were passed through Chromabond HX RA columns and pre-conditioned with 5ml of methanol and 5ml of water, and compounds were eluted with 3ml of ammonium formate 1M in methanol. Each eluate was dried under a stream of nitrogen and re-dissolved in 250 μl of water/methanol (1:1). We transferred 100 μl into glass HPLC vials, and 2 μl aliquots were analyzed by LC–MS as described by Catania et al. (2018). Additional high-resolution mass spectral data were obtained on a parallel LC interfaced to a Thermo Orbitrap Fusion mass spectrometer, operating in ESI mode at 500000 (FWHM) resolution for MS1 data, with MS2 data collected at 120000 resolution using stepped collision energies between 20 and 60units in both HCD and CID modes.
To identify 16-OH-casbene, we ground 4.9g of dry material obtained from 10 full-grown plants infiltrated with DXS, GGPPS, and CAS, and extracted with 100ml of hexane. After 1h of sonication and 2days shaking, the extract was centrifuged for 3min at 2000g, filtered through Whatman paper grade 1 and cotton, and evaporated to obtain 350mg of oily residue. The residue was re-suspended in 10ml of hexane/ethyl acetate (70:30, v/v) and purified through a 40g Buchi silica column on a PuriFlash® 4,250 system (Interchim). We used the same method of flash chromatography as described in King et al. (2014) to fractionate the extract into 80 samples. GC–MS was used to identify the fraction containing our compound of interest, and 2.6mg of this was obtained after evaporation, at sufficient purity for direct 1H NMR analysis on a Bruker AVIII 700MHz instrument equipped with a cryoprobe.
For 16-OH-geranyllinalool, we infiltrated 40 young plants with DXS, GGPPS, and NaGLS, which provided 10g of dry material after freeze-drying and grinding. We extracted this with 150ml of ethyl acetate and left to shake for 5days on a rotary shaker. After centrifugation and filtration as detailed above, we reduced the volume down to 1ml before re-suspending in 9ml of hexane/ethyl acetate and purifying with the same column and method as described above. The fractions of interest were combined and dried to obtain 16.4mg of extract that was further purified with a reverse phase column [C18-HQ 5μm 250 mm×10 mm (Interchim)] to remove the pigment content. The reverse phase column was first equilibrated with solvent A – mix of water/acetonitrile (95:5, v/v) – for eight column volumes (CV), before injecting the extract, diluted in 2.5ml of the same solvent, into a 5ml injection loop. The separation method consisted of one CV of solvent A, followed by a gradient of nine CV, to reach 100% acetonitrile (solvent 0B). This solvent was maintained for a further 10 CV, and the entire run was carried out at a flow rate of 3ml/min. We used an in-line connected Advion Expression compact mass spectrometer (CMS), which enabled product isolation guided by mass spectra. To evaluate the fragmentation of 16-OH-geranyllinalool, we additionally ran the extracts on UPLC-MS, allowing us to determine two main ions at m/z 271 and m/z 289, which we used to select our compound of interest on the Puriflash-CMS. We collected one fraction, which after evaporation contained 4.6mg of sufficiently pure metabolite for NMR identification.
In the previous work, we tested the transient co-expression of different MEP pathway genes and GGPPS from A. thaliana with CAS from Jatropha curcas to evaluate the best combination for the highest production of casbene. We determined that the combination of DXS (catalyzing the first step in the MEP pathway), HDR (4-hydroxy-3-methylbut-2-enyl diphosphate reductase, catalyzing the last step), GGPPS, and CAS resulted in an up to 5-fold increase in casbene production, compared to CAS expression alone (Forestier et al., 2021). Omitting HDR from this combination resulted in lower production of casbene (Forestier et al., 2021). Further inspection of the total ion chromatograms of plant extracts from this reduced gene combination identified three additional peaks (Figure 1A), compared to co-expression of DXS, HDR, GGPPS, and CAS which only produced casbene (Figure 1B).
Figure 1. GC–MS total ion chromatograms (TIC) showing the diterpene profile of N. benthamiana infiltrated with casbene synthase (CAS) and different precursor genes. (A) Co-expression of DXS+GGPPS+CAS. (B) Co-expression of DXS+HDR+GGPPS+CAS. (C) Simplified diagram of the MEP pathway plus geranylgeranyl pyrophosphate synthase (GGPPS). MEP pathway enzymes are DXS (1-deoxy-D-xylulose 5-phosphate synthase), DXR (1-deoxy-D-xylulose 5-phosphate reductoisomerase), MCT (2-C-methyl-D-erythritol 4-phosphate cytidylyltransferase), CMK (4-(cytidine 5’diphospho)-2-C-methyl-D-erythritol kinase), MDS (2-C-methyl-D-erythritol 2,4-cyclodiphosphate synthase), HDS (4-hydroxy-3-methylbut-2-enyl-diphosphate synthase), HDR (4-hydroxy-3-methylbut-2-enyl diphosphate reductase), and IPPI (isopentenyl diphosphate Δ-isomerase). 1, casbene; 2, 16-hydroxy-casbene; and 3 and 4, undetermined compounds.
The largest of the three additional peaks was present in sufficient amount to allow its identification as 16-hydroxy-casbene (16-OH-casbene) by NMR spectroscopy (Supplementary Figure S1). 16-OH-casbene was present at approximately 30% of casbene levels when HDR was absent from the gene combination (Supplementary Figure S2).
The fact that 16-OH-casbene is only produced when HDR is omitted from the gene combination used to increase flux into the C20 GGPP precursor for casbene production, led us to investigate the possibility that the hydroxyl group at the 16-position of the precursor is also hydroxylated. GGPP is formed by head-to-tail condensations between one molecule of DMAPP and three molecules of IPP (Ogura and Koyama, 1998), with the chain-starter DMAPP ending up distal to the pyrophosphate group (Figure 2A). In the MEP pathway, the immediate precursor to DMAPP is (E)-4-hydroxy-3-methyl-but-2-enyl pyrophosphate (HMBPP; Figure 1C), which has the same structure as DMAPP, but with a hydroxyl functionality at position 4 (Figures 2A,B). This hydroxyl group would appear at the 16-position of GGPP after chain-extension by IPP if HMBPP is accepted by GGPPS as an alternative chain starter to DMAPP (Figure 2B). We considered therefore that 16-OH-GGPP could be formed if there were an excess of HMBPP, caused by the over-expression of DXS and GGPPS with insufficient conversion to DMAPP and/or IPP due to this being dependent on an endogenous HDR. 16-OH-GGPP could then be further incorporated into the casbene backbone assuming that CAS accepts 16-OH-GGPP as substrate for production of 16-OH-casbene (Figure 2B).
Figure 2. Theoretical formation of GGPP/casbene and OH-GGPP/16-OH-casbene. (A) Condensation of dimethylallyl pyrophosphate (DMAPP) and isopentyl pyrophosphate (IPP) to form GGPP and cyclisation by casbene synthase to form casbene. (B) Condensation of HMBPP and IPP to form OH-GGPP and cyclisation by casbene synthase to form 16-OH-casbene.
To establish whether 16-OH-GGPP accumulates depending on the gene combination, either DXS+GGPPS or DXS+HDR+GGPPS were transiently expressed in N. benthamiana and C20 prenyl diphosphate intermediates were extracted as described by Nagel et al. (2014). UPLC-MS/MS negative mode analysis of methanolic extracts was used to detect GGPP by selecting the m/z range 449–450 and by comparison with an authentic GGPP standard (Figures 3A–C). In the absence of a 16-OH-GGPP standard, we predicted that since m/z 449.2 represents the [M-H]- ion for GGPP (Figure 3F), hydroxylated forms should be detectable at an added mass of 16; i.e at 465.2. Hydroxylated forms of prenyl diphosphates would be more hydrophilic and therefore elute at an earlier retention time compared to GGPP in reverse phase chromatographic separation. A clear peak at m/z 465.2, was detected at the earlier retention time of 2.5 min, in the extract of material over-expressing DXS+GGPPS (Figures 3D, F), consistent with 16-OH-GGPP. High-resolution mass spectrometry analysis revealed a m/z of 449.1869 for GGPP ([M-H]− theoretical 449.1864; error 1.11ppm) and m/z of 465.1819 for 16-OH-GGPP ([M-H]− theoretical 465.1813; error 1.29ppm). Both peaks generated a common 158.9252m/z MS2 fragment, identified as the diagnostic pyrophosphate group ion, [P2O6H]−. We were unable to detect the m/z 465.2 peak in the gene combination of DXS+HDR+GGPPS (Figure 3E), consistent with the hypothesis that OH-GGPP is produced when HMBPP reduction is limiting due to lack of HDR activity.
Figure 3. UPLC-MS chromatograms showing the prenyl diphosphate profile of N. benthamiana infiltrated with different diterpene precursor genes. (A) Authentic standard of GGPP, m/z 449–450. (B) Co-expression of DXS+GGPPS, m/z 449–450. (C) Co-expression of DXS+HDR+GGPPS, m/z 449–450. (D) Co-expression of DXS+GGPPS, m/z 465–466. (E) Co-expression of DXS+HDR+GGPPS, m/z 465–466. (F) Mass spectrum of GGPP (1) and putative OH-GGPP (2).
To further explore whether 16-OH-GGPP could be used by other diterpene synthases, we transiently expressed the Nicotiana attenuata geranyllinalool synthase (NaGLS) in N. benthamiana, alone or in combination with DXS+HDR+GGPPS (Figure 4 and Supplementary Figure S3). This resulted in accumulation of geranyllinalool in both cases. Co-expression of NaGLS+DXS+GGPPS produced geranyllinalool but also two additional peaks with retention times (Rt) of 30.0 and 31.6min (Figure 4A and Supplementary Figure S3E). We used UPLC-MS (Supplementary Figure S4) and flash chromatography to purify the compound giving rise to the larger peak at Rt 30.0 min in sufficient quantities to permit its identification by NMR spectroscopy as 16-OH-geranyllinalool (Supplementary Figure S5) This novel natural product represented up to 25% of the geranyllinalool peak (Figure 4C). This is comparable amount of 16-OH-casbene to casbene (30%) with the same reduced gene combination (Supplementary Figure S2).
Figure 4. NaGLS produces both geranyllinalool and 16-hydroxy-geranyllinalool in Nicotiana benthamiana (A) GC–MS TIC of extract from N. benthamiana infiltrated with DXS+GGPPS+NaGLS. (B) Extract from material infiltrated with DXS+HDR+GGPPS+NaGLS. (C) Quantities of compounds 1, 2, and 3 in extracts from the different gene combinations (ng/mg DW±SD, n=5). Symbols show significant differences between treatment means (p<0.05, F-test and T-test). 1, geranyllinalool; 2, 16-hydroxy-geranyllinalool; and 3, undetermined.
Despite obvious parallels with the regio-isomeric 17-OH-geranyllinalool (Supplementary Figure S6), the precursor of the insecticidal diterpene glycosides in many Nicotiana species (Snook et al., 1997; Jassbi et al., 2010; Falara et al., 2014), there is no indication that 16-OH-geranyllinalool could be involved in the biosynthetic pathway to 17-OH-diterpenes. A recent work actually demonstrated that two cytochrome P450s from N. attenuata are responsible for the 17-hydroxylation of geranyllinalool (Li et al., 2021).
We did not detect any other hydroxy geranyllinalool compounds apart from 16-OH-geranyllinalool with the DXS+GGPPS+NaGLS gene combination, providing further evidence that 16-OH-geranyllinalool is derived from a direct conversion of 16-OH-GGPP.
This work provides evidence for the formation of 16-OH-GGPP when the flux through the MEP pathway in N. benthamiana is altered. Both casbene synthase from Jatropha curcas and geranyllinalool synthase from N. attenuata result in production of 16-hydroxylated versions of their usual diterpene products when transiently expressed in N. benthamiana producing 16-OH-GGPP. The detection of additional minor compounds from both enzymes suggests that additional products may also arise when 16-OH-GGPP is used as substrate. We hypothesize that 16-OH-GGPP is formed through the action of A. thaliana GGPP synthase when HMBPP levels are elevated due to increased flux through the MEP pathway and a bottleneck exists at the HDR step. When the HDR enzyme, which reduces the hydroxy group in HMBPP to make DMAPP, is co-expressed with DXS and GGPPS, neither 16-OH-GGPP nor 16-OH-diterpenes are detected. Taken together the evidence presented supports formation of 16-OH-casbene or 16-OH-geranyllinalool by promiscuous diterpene synthases acting on 16-OH-GGPP rather than P450-based hydroxylation of casbene or geranyllinalool. The fact that formation of these 16-hydroxylated compounds is exclusively associated with over-expression of the first step in the MEP pathway combined with omission of the last step points to the bottleneck at the HDR step giving rise to 16-OH-GGPP via a plausible route. Interestingly, in Escherichia coli, overproduction of HMBPP is cytotoxic and removal of this effect is achieved by activation of IspG, the gene encoding the HDR equivalent in plants (Li et al., 2017). The transient expression approach we use in planta may have by-passed such regulation if indeed it is important in N. benthamiana.
There are precedents from the terpenoid literature for the formation of more highly oxidized precursors, which are then accepted as alternatives to the normal substrate in a known biosynthetic pathway. Thus, 2,3-oxidosqualene, the usual precursor of triterpenes and sterols, can undergo a second oxidization by the endogenous squalene epoxidase to form dioxidosqualene, when it accumulates in yeast (Salmon et al., 2016). Research has shown that a mutated triterpene synthase actually prefers this double-oxygenated substrate to the normal 2,3-oxidosqualene, leading to the production of unusual triterpenes that incorporate an additional oxygen atom in the fifth ring (Salmon et al., 2016). There are also examples of synthetic chemistry work focusing on obtaining analogues of the sesquiterpene precursor farnesyl pyrophosphate (Dolence and Dale Poulter, 1996; Placzek and Gibbs, 2011) or even (Z,E,E)-geranylgeranyl pyrophosphate (Minutolo et al., 2006), demonstrating the interest of alternative substrates for terpenoid production.
It is perhaps unlikely that 16-OH-GGPP is a significant substrate in nature when the MEP and diterpene biosynthetic pathways are subject to their normal mechanisms of regulation. However, the substantial level of 16-hydroxylated diterpenes with native diterpenes synthases in transient expression systems might suggest that 16-OH-diterpenes can become more biologically relevant under abnormal circumstances, when such regulation is compromised.
In terms of engineering biology, this work demonstrates the importance of regulating flux through biosynthetic pathways to ensure intermediates do not accumulate as the promiscuity of substrate specificity can result in the production of unexpected end products. On the other hand, this example shows that the generation of a novel GGPP substrate can open the possibility of entirely new diterpenes that could be further modified and evaluated in terms of their bioactivity.
The original contributions presented in the study are included in the article/Supplementary Material; further inquiries can be directed to the corresponding author.
EF designed experiments, performed experiments, and analyzed data. GB, DH, and TL performed experiments, analyzed data, and contributed to the writing of the manuscript. EF and IG wrote the manuscript. IG contributed to the design and analysis of the study. All authors contributed to the article and approved the submitted version.
This research was funded by the BBSRC and Innovate UK under grant number BB/M018210/01. High-resolution mass spectrometry was performed using equipment within the Centre of Excellence in Mass Spectrometry (University of York), funded by Science City York (Yorkshire Forward, EP/K039660/1, EP/M028127/1).
The authors declare that the research was conducted in the absence of any commercial or financial relationships that could be construed as a potential conflict of interest.
All claims expressed in this article are solely those of the authors and do not necessarily represent those of their affiliated organizations, or those of the publisher, the editors and the reviewers. Any product that may be evaluated in this article, or claim that may be made by its manufacturer, is not guaranteed or endorsed by the publisher.
GB would like to thank the Chemical Analysis Facility (CAF) at the University of Reading for the provision of the 700MHz NMR spectrometer used in these studies.
The Supplementary Material for this article can be found online at: https://www.frontiersin.org/articles/10.3389/fpls.2021.757186/full#supplementary-material
Beck, G., Coman, D., Herren, E., Ruiz-Sola, M. Á., Rodríguez-Concepción, M., Gruissem, W., et al. (2013). Characterization of the GGPP synthase gene family in Arabidopsis thaliana. Plant Mol. Biol. 82, 393–416. doi: 10.1007/s11103-013-0070-z
Callari, R., Meier, Y., Ravasio, D., and Heider, H. (2018). Dynamic control of ERG20 and ERG9 expression for improved casbene production in Saccharomyces cerevisiae. Front. Bioeng. Biotechnol. 6:160. doi: 10.3389/fbioe.2018.00160
Catania, T. M., Branigan, C. A., Stawniak, N., Hodson, J., Harvey, D., Larson, T. R., et al. (2018). Silencing amorpha-4,11-diene synthase genes in Artemisia annua leads to FPP accumulation. Front. Plant Sci. 9:547. doi: 10.3389/fpls.2018.00547
Corea, G., Di Pietro, A., Dumontet, C., Fattorusso, E., and Lanzotti, V. (2009). Jatrophane diterpenes from Euphorbia spp. as modulators of multidrug resistance in cancer therapy. Phytochem. Rev. 8, 431–447. doi: 10.1007/s11101-009-9126-8
De Ridder, T. R., Campbell, J. E., Burke-Schwarz, C., Clegg, D., Elliot, E. L., Geller, S., et al. (2021). Randomized controlled clinical study evaluating the efficacy and safety of intratumoral treatment of canine mast cell tumors with tigilanol tiglate (EBC-46). J. Vet. Intern. Med. 35, 415–429. doi: 10.1111/jvim.15806
Dolence, J. M., and Dale Poulter, C. (1996). Synthesis of analogs of farnesyl diphosphate. Tetrahedron 52, 119–130. doi: 10.1016/0040-4020(95)00909-R
Falara, V., Alba, J. M., Kant, M. R., Schuurink, R. C., and Pichersky, E. (2014). Geranyllinalool synthases in solanaceae and other angiosperms constitute an ancient branch of diterpene synthases involved in the synthesis of defensive compounds. Plant Physiol. 166, 428–441. doi: 10.1104/pp.114.243246
Forestier, E. C. F., Czechowski, T., Cording, A. C., Gilday, A. D., King, A. J., Brown, G. D., et al. (2021). Developing a Nicotiana benthamiana transgenic platform for high-value diterpene production and candidate gene evaluation. Plant Biotechnol. J. 19, 1614–1623. doi: 10.1111/pbi.13574
Gershenzon, J., and Croteau, R. B. (1993). “Terpenoid biosynthesis: the basic pathway and formation of monoterpenes, sesquiterpenes, and diterpenes,” in Lipid Metabolism in Plants, 376–380.
Hadi, V., Hotard, M., Ling, T., Salinas, Y. G., Palacios, G., Connelly, M., et al. (2013). Evaluation of Jatropha isabelli natural products and their synthetic analogs as potential antimalarial therapeutic agents. Eur. J. Med. Chem. 65, 376–380. doi: 10.1016/j.ejmech.2013.04.030
Hashimoto, S., Katoh, S., Kato, T., Urabe, D., and Inoue, M. (2017). Total synthesis of resiniferatoxin enabled by radical-mediated three-component coupling and 7- endo cyclization. J. Am. Chem. Soc. 139, 16420–16429. doi: 10.1021/jacs.7b10177
Hill, A. M., Cane, D. E., Mau, C. J. D., and West, C. A. (1996). High level expression of ricinus communis casbene synthase in Escherichia coliand characterization of the recombinant enzyme. Arch. Biochem. Biophys. 336, 283–289. doi: 10.1006/abbi.1996.0559
Hohmann, J., Evanics, F., Berta, L., and Bartók, T. (2000). Diterpenoids from Euphorbia peplus. Planta Med. 66, 291–294. doi: 10.1055/s-2000-8568
Jassbi, A. R., Zamanizadehnajari, S., and Baldwin, I. T. (2010). 17-Hydroxygeranyllinalool glycosides are major resistance traits of Nicotiana obtusifolia against attack from tobacco hornworm larvae. Phytochemistry 71, 1115–1121. doi: 10.1016/j.phytochem.2010.04.014
Johnson, H. E., Banack, S. A., and Cox, P. A. (2008). Variability in content of the anti-AIDS drug candidate prostratin in samoan populations of Homalanthus nutans. J. Nat. Prod. 71, 2041–2044. doi: 10.1021/np800295m
Jorgensen, L., McKerrall, S. J., Kuttruff, C. A., Ungeheuer, F., Felding, J., and Baran, P. S. (2013). 14-step synthesis of (+)-ingenol from (+)-3-carene. Science 341, 878–882. doi: 10.1126/science.1241606
Kawamura, S., Chu, H., Felding, J., and Baran, P. S. (2016). Nineteen-step total synthesis of (+)-phorbol. Nature 532, 90–93. doi: 10.1038/nature17153
King, A. J., Brown, G. D., Gilday, A. D., Larson, T. R., and Graham, I. A. (2014). Production of bioactive diterpenoids in the euphorbiaceae depends on evolutionarily conserved gene clusters. Plant Cell 26, 3286–3298. doi: 10.1105/tpc.114.129668
Kissin, I., and Szallasi, A. (2011). Therapeutic targeting of TRPV1 by resiniferatoxin, from preclinical studies to clinical trials. Curr. Top. Med. Chem. 11, 2159–2170. doi: 10.2174/156802611796904924
Kulkosky, J., Culnan, D. M., Roman, J., Dornadula, G., Schnell, M., Boyd, M. R., et al. (2001). Prostratin: activation of latent HIV-1 expression suggests a potential inductive adjuvant therapy for HAART. Blood 98, 3006–3015. doi: 10.1182/blood.V98.10.3006
Li, Q., Fan, F., Gao, X., Yang, C., Bi, C., Tang, J., et al. (2017). Balanced activation of IspG and IspH to eliminate MEP intermediate accumulation and improve isoprenoids production in Escherichia coli. Metab. Eng. 44, 13–21. doi: 10.1016/j.ymben.2017.08.005
Li, J., Halitschke, R., Li, D., Paetz, C., Su, H., Heiling, S., et al. (2021). Controlled hydroxylations of diterpenoids allow for plant chemical defense without autotoxicity. Science 371, 255–260. doi: 10.1126/science.abe4713
Lichtenthaler, H. K., Schwender, J., Disch, A., and Rohmer, M. (1997). Biosynthesis of isoprenoids in higher plant chloroplasts proceeds via a mevalonate-independent pathway. FEBS Lett. 400, 271–274. doi: 10.1016/S0014-5793(96)01404-4
Mehrshahi, P., Nguyen, G. T. D., Gorchs Rovira, A., Sayer, A., Llavero-Pasquina, M., Lim Huei Sin, M., et al. (2020). Development of Novel Riboswitches for Synthetic Biology in the Green Alga Chlamydomonas. ACS. Synth. Biol. 9, 1406–1417. doi: 10.1021/acssynbio.0c00082
McGarvey, D. J., and Croteau, R. (1995). Terpenoid metabolism. Plant Cell 7, 1015–1026. doi: 10.1105/tpc.7.7.1015
Minutolo, F., Bertini, S., Betti, L., Danesi, R., Gervasi, G., Giannaccini, G., et al. (2006). Synthesis of stable analogues of geranylgeranyl diphosphate possessing a (Z,E,E)-geranylgeranyl side chain, docking analysis, and biological assays for prenyl protein transferase inhibition. ChemMedChem 1, 218–224. doi: 10.1002/cmdc.200500010
Nagel, R., Berasategui, A., Paetz, C., Gershenzon, J., and Schmidt, A. (2014). Overexpression of an isoprenyl diphosphate synthase in spruce leads to unexpected terpene diversion products that function in plant defense. Plant Physiol. 164, 555–569. doi: 10.1104/pp.113.228940
Ogura, K., and Koyama, T. (1998). Enzymatic aspects of isoprenoid chain elongation. Chem. Rev. 98, 1263–1276. doi: 10.1021/cr9600464
Phillips, M., Leon, P., Boronat, A., and Rodríguez-concepción, M. (2008). The plastidial MEP pathway: unified nomenclature and resources. Trends Plant Sci. 13, 619–623. doi: 10.1016/j.tplants.2008.09.003
Placzek, A. T., and Gibbs, R. A. (2011). New synthetic methodology for the construction of 7-substituted farnesyl diphosphate analogs. Org. Lett. 13, 3576–3579. doi: 10.1021/ol201069x
Rohmer, M., Knani, M., Simonin, P., Sutter, B., and Sahm, H. (1993). Isoprenoid biosynthesis in bacteria: a novel pathway for the early steps leading to isopentenyl diphosphate. Biochem. J. 295, 517–524. doi: 10.1042/bj2950517
Sainsbury, F., Thuenemann, E. C., and Lomonossoff, G. P. (2009). pEAQ: versatile expression vectors for easy and quick transient expression of heterologous proteins in plants. Plant Biotechnol. J. 7, 682–693. doi: 10.1111/j.1467-7652.2009.00434.x
Salmon, M., Thimmappa, R. B., Minto, R. E., Melton, R. E., Hughes, R. K., O’Maille, P. E., et al. (2016). A conserved amino acid residue critical for product and substrate specificity in plant triterpene synthases. Proc. Natl. Acad. Sci. U. S. A. 113, E4407–E4414. doi: 10.1073/pnas.1605509113
Siller, G., Rosen, R., Freeman, M., Welburn, P., Katsamas, J., and Ogbourne, S. M. (2010). PEP005 (ingenol mebutate) gel for the topical treatment of superficial basal cell carcinoma: results of a randomized phase IIa trial. Australas. J. Dermatol. 51, 99–105. doi: 10.1111/j.1440-0960.2010.00626.x
Snook, M. E., Johnson, A. W., Severson, R. F., Teng, Q., White, R. A., Sisson, V. A., et al. (1997). Hydroxygeranyllinalool glycosides from tobacco exhibit antibiosis activity in the tobacco budworm [Heliothis virescens (F.)]. J. Agric. Food Chem. 45, 2299–2308. doi: 10.1021/jf960748u
Keywords: plant diterpenes, Nicotiana benthamiana, GGPP, hydroxy-GGPP, metabolic engineering
Citation: Forestier ECF, Brown GD, Harvey D, Larson TR and Graham IA (2021) Engineering Production of a Novel Diterpene Synthase Precursor in Nicotiana benthamiana. Front. Plant Sci. 12:757186. doi: 10.3389/fpls.2021.757186
Received: 11 August 2021; Accepted: 15 September 2021;
Published: 20 October 2021.
Edited by:
Qi Chen, Kunming University of Science and Technology, ChinaReviewed by:
Feng Ge, Kunming University of Science and Technology, ChinaCopyright © 2021 Forestier, Brown, Harvey, Larson and Graham. This is an open-access article distributed under the terms of the Creative Commons Attribution License (CC BY). The use, distribution or reproduction in other forums is permitted, provided the original author(s) and the copyright owner(s) are credited and that the original publication in this journal is cited, in accordance with accepted academic practice. No use, distribution or reproduction is permitted which does not comply with these terms.
*Correspondence: Ian A. Graham, aWFuLmdyYWhhbUB5b3JrLmFjLnVr
Disclaimer: All claims expressed in this article are solely those of the authors and do not necessarily represent those of their affiliated organizations, or those of the publisher, the editors and the reviewers. Any product that may be evaluated in this article or claim that may be made by its manufacturer is not guaranteed or endorsed by the publisher.
Research integrity at Frontiers
Learn more about the work of our research integrity team to safeguard the quality of each article we publish.