- MOA Key Laboratory of Integrated Management of Pests on Crops in Southwest China, Institute of Plant Protection, Sichuan Academy of Agricultural Sciences, Chengdu, China
Plant hormones have a prominent place in the plant immune and defense mechanism. To gain more information about the plant hormone pathways involved in rice defense against nematodes, here, we studied the roles of three core hormones, namely, salicylic acid (SA), jasmonate (JA), and ethylene (ET) in rice defense to Aphelenchoides besseyi by using the susceptible variety, Nipponbare as well as the resistant variety Tetep. The data showed that Tetep exhibited pre- and post-invasion with suppression of nematode infection, development, and reproduction. The quantitative real-time (qRT)-PCR analysis of plant hormone marker genes in the two cultivars clearly revealed that all the SA-related genes were downregulated in susceptible Nipponbare plants but were significantly upregulated in resistant Tetep plants at the flowering stage. The exogenous application of the SA analog, benzo-1,2,3-thiadiazole-7-carbothioic acid S-methyl ester (BTH), methyl jasmonate (MeJA), and ethephon did induce rice resistance to A. besseyi, and the rice plants treated by hormone inhibitors increased susceptibility to A. besseyi. Similarly, corresponding transgenic biosynthesis or signaling mutants of those hormones also showed an increased susceptibility. Collectively, these results suggest that SA, JA, and ET play important defense roles in rice against A. besseyi.
Introduction
Rice (Oryza sativa) is a major food source that feeds more than one-third of the world population. During the growth of rice plants, they are potential targets for more than 100 species of plant parasitic nematodes (Fortuner and Merny, 1979); Aphelenchoides besseyi, known as the rice white tip nematode, is one of the most important parasitic nematodes of rice (Duncan and Moens, 2013). As a seed-borne nematode, A. besseyi can survive on stored grain in anhydrobiosis for several years (Tiwari and Khare, 2003). After sowing, anabiotic A. besseyi rapidly become active and are attracted to rice meristematic areas. The nematodes migrate in the leaf or young tissue of rice as ecto- and endoparasites and cause a typical symptom of whitening and withering at the leaf tips (Togashi and Hoshino, 2001; Duncan and Moens, 2013). A. besseyi can be found in low numbers at all green tissues during early growth stages of rice; later, the nematodes enter spikelets before anthesis, and a rapid increase in the number of nematodes takes place at the flowering stage (Goto and Fukatsu, 1952). Around 30–70% reduction in yield caused by A. besseyi has been reported (Tikhonova, 1966; Muthukrishnan et al., 1974; Lin et al., 2004; Tulek and Cobanoglu, 2010); therefore, A. besseyi was listed in the top 10 plant-parasitic nematodes (Jones et al., 2013). To control this nematode, resistant cultivars offer the most favorable and durable options (De Waele, 2002), as has been proven in the United States (Prot and Rahman, 1994). In China, A. besseyi was first found in the 1940s and widely spread throughout the rice-growing regions (Pei et al., 2010; Wang et al., 2017); it causes 30–50% yield losses in flooded rice (Wang et al., 2014). Notably, 27 rice cultivars have been assessed to determine the resistance against A. besseyi in the field, it was found that 7 cultivars were resistant, and Tetep (i.e., an indica rice) showed high resistance to A. besseyi (Feng et al., 2014).
To minimize the damage caused by pathogens, plants have evolved an arsenal of immune and defense mechanisms, in which plant hormones play important signaling roles. Salicylic acid (SA), jasmonate (JA), and ethylene (ET) are the three classical hormones that generate and transmit signals to help host plants fight against pathogens (Grant and Jones, 2009; Robert-Seilaniantz et al., 2011). SA is usually activated when plants encounter threats from biotrophic and hemi-biotrophic pathogens, while JA and ET play key roles in the defense against necrotrophic pathogens, insects, and root-knot nematodes (Glazebrook, 2005; Howe and Jander, 2008; Nahar et al., 2011). During defense signaling, these three hormones can act synergistically or antagonistically (Koornneef and Pieterse, 2008; Pieterse et al., 2009). In general, JA and ET signaling pathways are believed to be synergistical in dicotyledon plants, while SA and JA/ET defense pathways are often found mutually antagonistic, but synergistic interactions between these two hormones have also been reported (Rojo et al., 2003; Lorenzo and Solano, 2005; Beckers and Spoel, 2006; Van Loon et al., 2006). Among others, SA, JA, or ET accumulation leads to the expression of pathogenesis-related (PR) genes such as PR1, PR2, and PR3 in various tissues (López et al., 2008; Bari and Jones, 2009).
Recently, some studies have reported on the roles of SA, JA, and ET in rice defense toward plant-parasitic nematodes. For instance, it has been reported that JA pathway plays a crucial role in rice defense against the root-knot nematode Meloidogyne graminicola, while SA, JA, and ET biosynthesis pathways are equally important for defense against the migratory root nematode Hirschmanniella oryzae and rice stem nematode Ditylenchus angustus (Kyndt et al., 2012; Nahar et al., 2012; Khanam et al., 2018). There is little knowledge about the role of plant hormones in the interaction of rice plants and A. besseyi, which has a different strategy of multiplication and dispersal to root nematodes such as root-knot nematode or cyst nematode and rice stem nematode D. angustus.
To gain more information about the roles of plant hormone pathways involved in rice defense against A. besseyi, we first characterized the reaction of two rice cultivars upon A. besseyi infection, and then further clarified the role of SA, JA, or ET in the interaction of rice and A. besseyi by several approaches.
Materials and Methods
Plant Materials
The NahG line was a gift from Prof. Dr. Cheng-Cai Chu (Chinese Academy of Sciences, Beijing, China). The Coi1-13 line was provided by Prof. Dr. Dong-Lei Yang (Nanjing Agricultural University, Nanjing, China). The OsEin2b-RNAi line was provided by Yi-Nong Yang (Pennsylvania State University, United States), and the OsWRKY45-RNAi transgenic line was a gift from H. Takatsuji (Plant Disease Resistance Research Unit, National Institute of Agrobiological Sciences, Ibaraki, Japan); all the above four lines were all with a background of Nipponbare. The mutant hebiba and its corresponding wild-type cv. Nihonmasari were generously provided by P. Nick (Karlsruhe University, Karlsruhe, German). Cultivar Tetep and Nipponbare were maintained in the lab, which were initially obtained from rice breeders.
Rice cultivar Tetep and Nipponbare were used to detect the level of resistance/susceptibility to A. besseyi and analyze the expression of plant hormone-related genes. Rice cultivar Nipponbare was also used to be sprayed by hormones or hormone inhibitors to clarify the roles of hormones in nematode defense. Five rice mutants or transgenic lines including an SA-deficient transgenic NahG line, a JA response-deficient mutant line Coi1-13, a transgenic line OsEin2b-RNAi, which is with a silenced expression of ET signaling gene OsEin2b, an OsWRKY45-RNAi transgenic line, and a JA biosynthesis mutant hebiba were used to further obtain a detailed role of the three hormone pathways in the interaction of rice and A. besseyi.
Growth Conditions
All seeds used in this research were first soaked in hot water at 56°C for 15 min to kill the seed-borne nematodes (Fortuner and Orton Williams, 1975), then germinated on wet filter paper for 3 days at 28°C, subsequently transferred to autoclaved sand mixed with 0.2% (m/v) superabsorbent polymer (SAP) substrate (Reversat et al., 1999) in 10 ml centrifuge tubes, and further were grown at 26°C under a 16/8 h light regime. To harvest the rice grains and further count the nematodes, the seedlings were transplanted into a greenhouse plot at 25 days after inoculation (dai) with a density of 250 mixed infective stages of A. besseyi per plant and grown at approximately 28–30°C and 60–70% relative humidity (RH). Plots were 1.5 m × 2.0 m and caged with nylon nets to protect against insects. An earth levee of 25 cm height was made around each plot to maintain the water level and to isolate each plot.
Nematode Culture and Inoculation
Aphelenchoides besseyi was isolated from infected rice seeds of AnHui1 (O. sativa subsp. japonica) by soaking seeds in tap water for 72 h. Then, nematodes were collected and surface-sterilized with 0.1% streptomycin sulfate for 10 min, washed three times with sterile double-distilled water, and subsequently cultured on carrot discs at 25°C for 30 days as described in the study by Tulek et al. (2009).
The nematodes were then collected in tap water, and approximately 250 nematodes were inoculated to each plant by using water flotation method described by Xie et al. (2019). Briefly, hot water-treated seeds were germinated for 3 days at 28°C and planted individually into the center of a 10 ml centrifuge tube containing 4 ml SAP substrate; the tubes were capped and the plants were grown for 3 days at 26°C under a 16 h/8 h light regime. Subsequently, 2 ml of nematode suspensions in tap water containing 250 mixed infective stages of nematodes were added to each plant to submerge the growth site of the seedling. The tubes were capped for another 5 days to retain the moisture. Clean tap water without nematodes was used as the mock control.
Chemical Treatment
The hormone analogs benzo-1,2,3-thiadiazole-7-carbothioic acid S-methyl ester (BTH), methyl jasmonate (MeJA), and ethephon were purchased from Sigma-Aldrich (Sigma, St. Louis, United States). Diethyldithiocarbamic acid [DIECA (Farmer et al., 1994)], a JA biosynthesis inhibitor; aminooxyacetic acid [AOA (Iwai et al., 2006; Mao et al., 2006)], an inhibitor of ET biosynthesis, and L-2-aminooxy-3-phenylpropionic acid [AOPP (Amrhein and Gödeke, 1977)], an inhibitor of phenylalanine ammonia-lyase (PAL) activity were bought from FUJIFILM Wako Pure Chemical Corporation (Neuss, Deutschland) and Sigma-Aldrich (Sigma, St. Louis, United States). Two concentrations of hormones or analogs were used in the study, i.e., MeJA (250 and 500 μM), BTH (125 and 250 μM), and ethephon (250 and 500 μM). The following concentrations of the hormone inhibitor were used in this study: DIECA (100 μM), AOA (20 mM), and AOPP (100 μM). All chemicals were dissolved in water containing 0.02% (v/v) Tween 20.
The chemical treatment was examined at two stages in Nipponbare. For the analysis of the effects of chemicals on nematode infection at the seedling stage, the prepared chemicals or water control containing 0.02% (v/v) Tween 20 were sprayed with a perfume sprayer onto the leaves of six-day-old seedlings; the nematode of mixed infective stages with a density of 250 per plant were inoculated at 24 h after spraying, and a number of nematodes were collected from above ground parts at 25 dai. For the analysis of the effects of chemicals in nematode infection at flowering stage, 250 of mixed infective stages nematodes were inoculated individually onto seven-day-old seedlings and then transplanted into a greenhouse plot at 25 dai for further growth. The prepared chemicals or water control containing 0.02% (v/v) Tween 20 were sprayed onto the leaves and panicles at day one of flowering, and the number of nematodes were collected from grains at the ripen stage.
Nematode Counting
To analyze the nematode reproduction dynamics in Nipponbare and Tetep, nematodes were collected from the above ground parts at 15 dai, tillering stage (about 30 dai), jointing stage (about 45 dai), from panicles at booting stage (about 60 dai) and flowering stage (about 70 dai), and from grains at ripen stage (about 100 dai). To confirm the effects of plant hormones on nematode infection, nematodes on mutant lines or chemical-treated plants were collected at 25 dai and grains. The white-tip symptoms were observed and recorded at tillering stage. Symptom rates (%) = The number of plants showing white-tip symptoms/the number of inoculated plants × 100%.
All the counts in this study were performed according to Xie et al. (2019). Briefly, the above ground part of seedlings or panicles was cut into approximately 1 cm long pieces, immersed in 0.01% Tween 20, placed on a shaker at 40 rpm for 24 h, and then, the water was collected and the materials were rinsed twice; all the water was collected into a counting dish, and the nematodes in water were counted under a stereomicroscope. Each experiment or treatment had three biological replicates, and each replicate contained 15 plants. For evaluation of the nematode population in grains, a total of randomly selected 100 mixed seeds from one replicate of one treatment were collected and were gently ground to separate glumes and seeds, and then the glumes and grains were soaked in tap water in a Petri dish on a shaker at 40 rpm for 8 h and washed twice. Nematode observation was performed the same as described above under a stereomicroscope. Each replicate contains five randomly mixed 100 grains; three independent biological replicates were recorded.
RNA Extraction, cDNA Synthesis, and Quantitative Real-Time-PCR
For quantitative real-time (qRT)-PCR, the above-ground tissues (including all the leaves and stem) of Tetep and Nipponbare were separately collected at 3, 5, 7, and 14 dai, and the panicles were collected at the flowering stage after nematode inoculation. The tissues of clean tap water inoculation were served as the corresponding control. RNA was extracted using the TRIzol reagent (Invitrogen, Breda, Netherlands), and the extracted RNA was treated with DNaseI (Thermo Fisher Scientific) to remove all contaminating DNA as follows: added 1 μl (1 U) DNase I, 1 μg extracted RNA, 1 μl of 10× reaction buffer with 25 mM MgCl2, and DEPC-treated water into RNase-free tube to a final volume of 10 μl and then incubated at 37°C for 30 min; later, 1 μl 50 mM EDTA was added and incubated at 65°C for 10 min. The RNA concentration and purity were measured using a NanoDrop 2000 (Thermo Scientific). The first-strand cDNA synthesis was generated using RevertAid First Strand cDNA Synthesis Kit (Thermo Fisher Scientific, Lithuania). The PowerUp™ SYBR™ Green Master Mix (Thermo Fisher Scientific, Lithuania) was used to perform qRT-PCR: Green Master Mix (2×) 10 μl, forward primer 1 μl (10 ng/μl), reverse primer 1 μl (10 ng/μl), cDNA 1 μl (20 ng/μl), and nuclease-free water 7 μl. qRT-PCR was performed under the following conditions: 5 min at 95°C and 45 cycles of (10 s at 95°C and 30 s at 59°C). After the PCR reaction, a melting curve was generated by gradually increasing the temperature to 95°C to test for amplicon specificity. All reactions were performed in three technical replicates on Bio-Rad CFX connect (Bio-Rad, United States) and analyzed using R version 3.3.0 (R Core Team, 2016). Each biological replicate composed of a pool of eight individual plants or panicles. The experiment was independently repeated three times. Expression data were normalized using two reference genes. Primer pairs for each target or reference gene are listed in Table 1.
Data Analysis
The data were analyzed using the R version 3.3.0 software. Normality and equality of variances were tested with Shapiro–Wilk normality test (α = 0.05) and Bartlett test (α = 0.05), respectively. Normally distributed data were analyzed using an independent samples t-test or ANOVA followed by individual comparisons with the Tukey test (α = 0.05). Non-parametric testing was carried out with the Kruskal–Wallis test with α = 0.05. All data except qRT-PCR in the same treatment were not significantly different between replicates in this research; therefore, the data presented in all the results are the means of three replicates.
Results
The Reproduction Dynamics of Aphelenchoides besseyi in Nipponbare and Tetep
The number of nematodes per plant in Nipponbare was significantly higher than the ones in Tetep at all the stages tested (Figure 1). There were no significant differences between the number of nematodes per plant at 15 dai, tillering stage, and jointing stage either in Nipponbare or in Tetep. However, a 13- to 26-fold increase of nematode numbers was observed in Nipponbare at booting and flowering stages, and the number reached 160.0 and 310.1 per plant, respectively. The number of nematodes only slightly increased in Tetep at these two stages, with a four- to eightfold increase up to 14.2 and 24.8 nematodes per plant. Besides, there were, on average, 10.6 nematodes in 100 grains for Tetep, which was significantly lower than the average of 92.7 nematodes in 100 grains for Nipponbare.
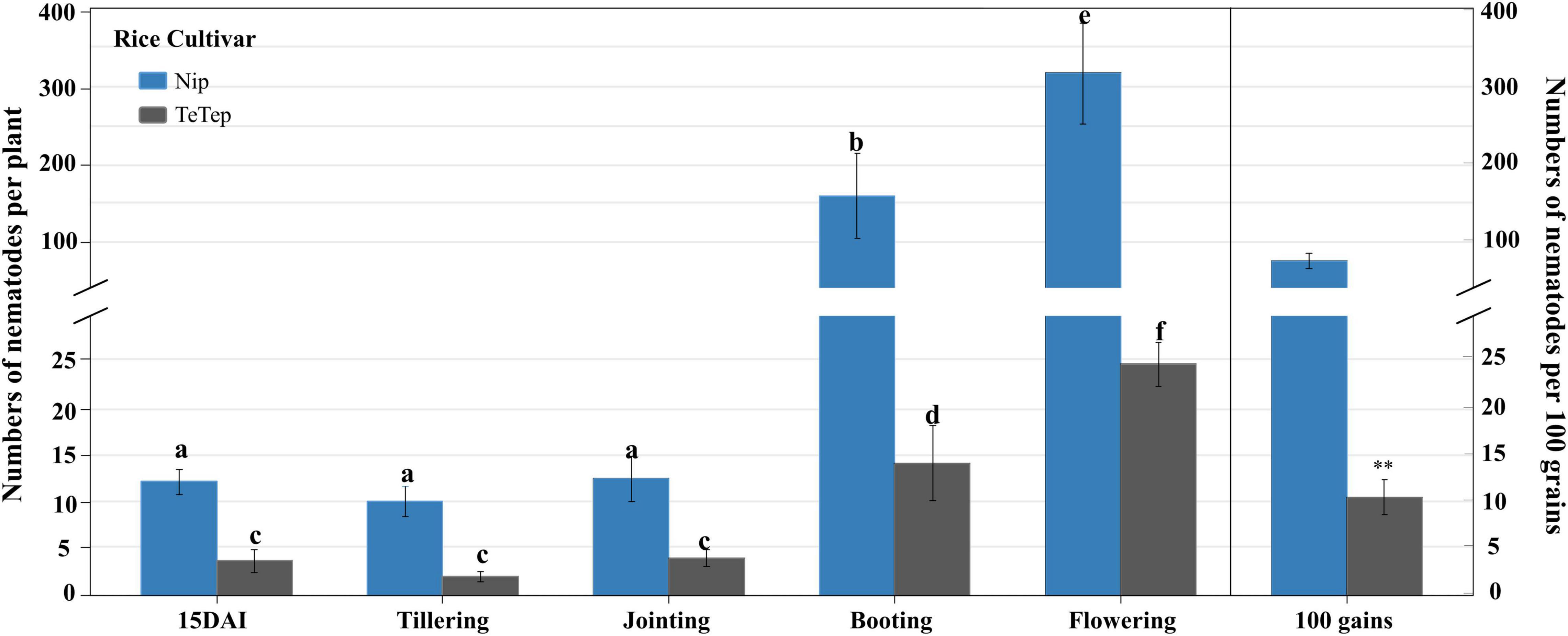
Figure 1. Number of Aphelenchoides besseyi of two rice cultivars Nipponbare and Tetep at different stages. Six-day-old rice plants were inoculated with 250 mixed stages of A. besseyi. The number of nematodes was observed at 15 dai, tillering, jointing, booting, flowering stages, and mature grains. Bars in the left part are represented as the means ± SE from 3n = 45 plants. Bars in the right part were represented as the means ± SE from 3n = 15 in 100 grains. Different letters indicate statistically significant differences (Tukey test with α = 0.05). The symbol “**” indicates statistically significant differences with t-test at α = 0.01.
The typical white tip symptom is the production of chlorotic tips on flag leaves starting at tillering, which may subsequently necrotize. This symptom was recorded in both Nipponbare and Tetep at the tillering stage, at a rate of 72.9% for Nipponbare, while in Tetep, no typical symptom was observed at the whole growth stages (Supplementary Figure 1).
The Expression of Plant Hormone Marker Genes at Seedling Stages in Rice Plants Under Attack by Aphelenchoides besseyi
The possible roles of plant hormones during the interactions of rice and A. besseyi were investigated by evaluating the expression levels of 13 selected SA, JA, or ET marker genes and 2 PR genes in Nipponbare and Tetep at early seedling stages (Figure 2).
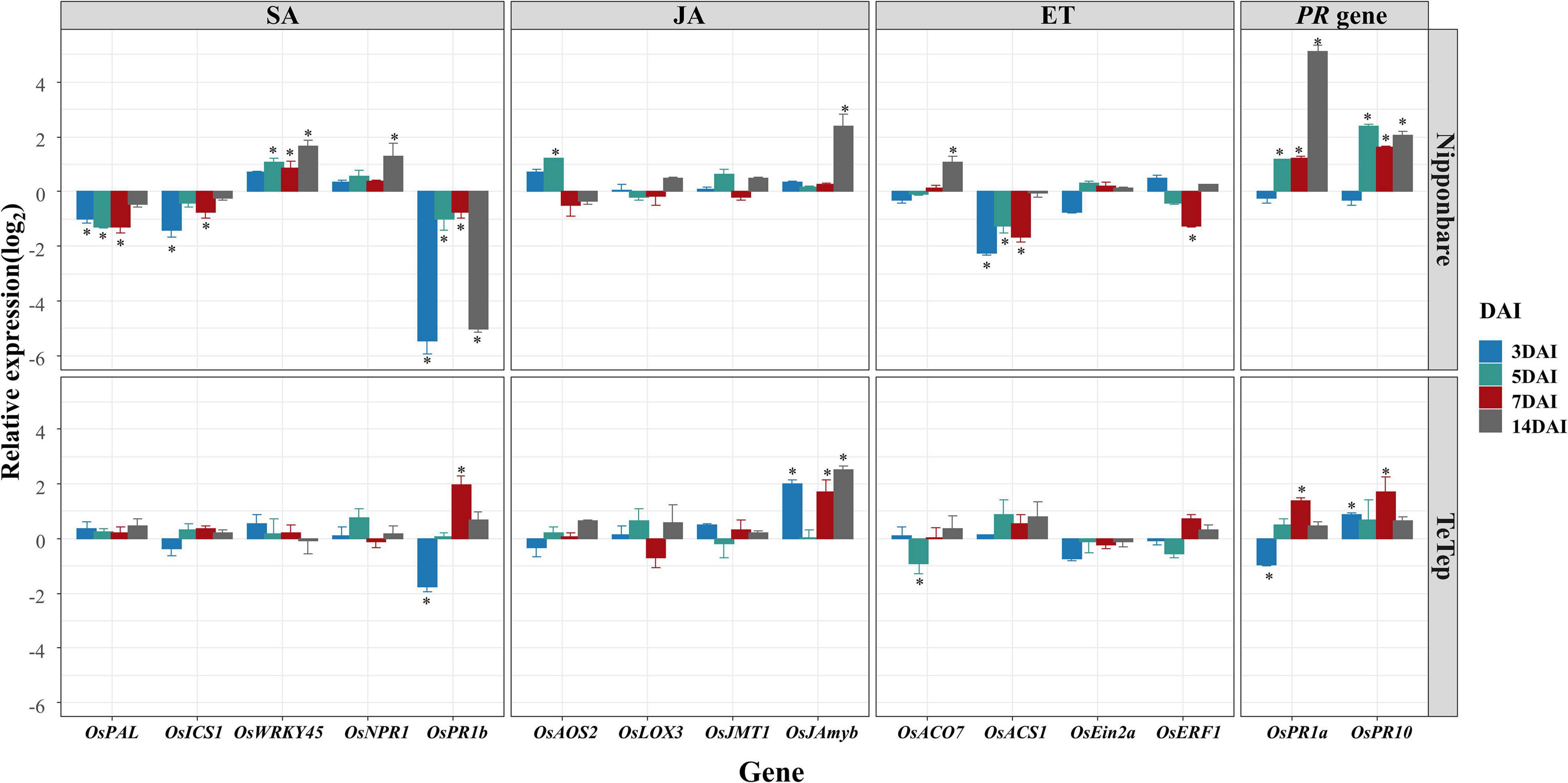
Figure 2. Quantitative real-time (qRT)-PCR analysis on the selected defense-related genes in shoot tissues in two cultivars at seedling stage. Bars represent mean expression levels and SE from four technical replicates of one biological replicate, containing a pool of eight plants. The other two biological replicates show similar results. Data are shown as relative expression levels of infected tissues in comparison with the control tissue (uninfected tissue). Gene expression levels were normalized using two internal reference genes, OsEXP and OsEXPNarsai. Asterisks indicate statistically significant (α = 0.05) differential expression in comparison with control plants, performed using R version 3.3.0.
For SA signaling pathway, two biosynthesis genes OsICS1 and OsPAL1, two SA response genes OsWRKY45 and OsNPR1, and one SA-inducible PR gene OsPR1b were analyzed. During the interaction of Nipponbare and A. besseyi, the expression levels of OsPAL1 and OsICS1 were consistently downregulated at all time points compared with those in mock control. However, the transcript level of OsWRKY45 was slightly increased in shoot tissues at 3 dai but was significantly upregulated at 5, 7, and 14 dai. The expression of OsNPR1 was not significantly affected by A. besseyi at the most of stages; however, the transcription level of OsNPR1 was significantly upregulated at 14 dai. The OsPR1b expression levels were downregulated at all times, especially at 3 and 14 dai. During the interaction of Tetep and A. besseyi, OsICS1, OsPAL1, OsWRKY45, and OsNPR1 showed no significant changes upon A. besseyi inoculation at all time points, while downregulation at 3 dai and upregulation at 7 and 14 dai were observed in OsPR1b.
To investigate the JA response, OsAOS2 (a key enzyme in JA biosynthesis), OsLOX3 (a JA synthesis pathway-related gene), OsJMT1 (an enzyme that converts JA to the volatile component MeJA), and OsJAmyb (a JA-inducible Myb transcription factor) were analyzed. The expression levels of OsAOS2 at 5 dai and OsJAmyb at 14 dai were significantly upregulated in Nipponbare, while the other genes had no significant changes at all time points. In contrast, during the interaction of Tetep and A. besseyi, the expression of OsJAmyb was significantly upregulated at 3, 7, and 14 dai, while all the other genes showed similar expression levels compared with control plants.
Genes such as OsACO7, OsACS1, OsEin2b, and OsERF1 that play key roles in ET biosynthesis or signaling were analyzed by using qRT-PCR. The expression of OsACO7 was significantly upregulated at 14 dai in Nipponbare but was downregulated at 5 dai in Tetep. The expression of OsACS1 was significantly downregulated in Nipponbare at 3, 5, and 7 dai, but only minor upregulation was detected in Tetep at the same stages. No significant differences were detected for OsEin2b between the inoculated and control plants. Nevertheless, ET-inducible gene OsERF1 appeared to be significantly down-regulated at 7 dai in Nipponbare, but no significant transcription alteration was detected in Tetep at these stages.
To assess the general defense responses in these two cultivars, after A. besseyi inoculation, the expressions of two PR genes OsPR1a and OsPR10 were analyzed. OsPR1a and OsPR10 were both significantly upregulated at all the time points except at 3 dai in Nipponbare. In contrast, the mRNA level of OsPR1a was dramatically downregulated at 3 dai and upregulated at 7 dai in Tetep; the expression levels of OsPR10 were significantly induced at 3 and 7 dai.
The Expression of Plant Hormone Marker Genes at Flowering Stage During the Infection of Aphelenchoides besseyi
As shown in Figure 1, the number of nematodes is massively increasing at the flowering stage in rice panicles. Therefore, the expression levels of the same plant hormone marker genes in panicles were analyzed to investigate the role of plant hormones during A. besseyi infection at the flowering stage (Figure 3).
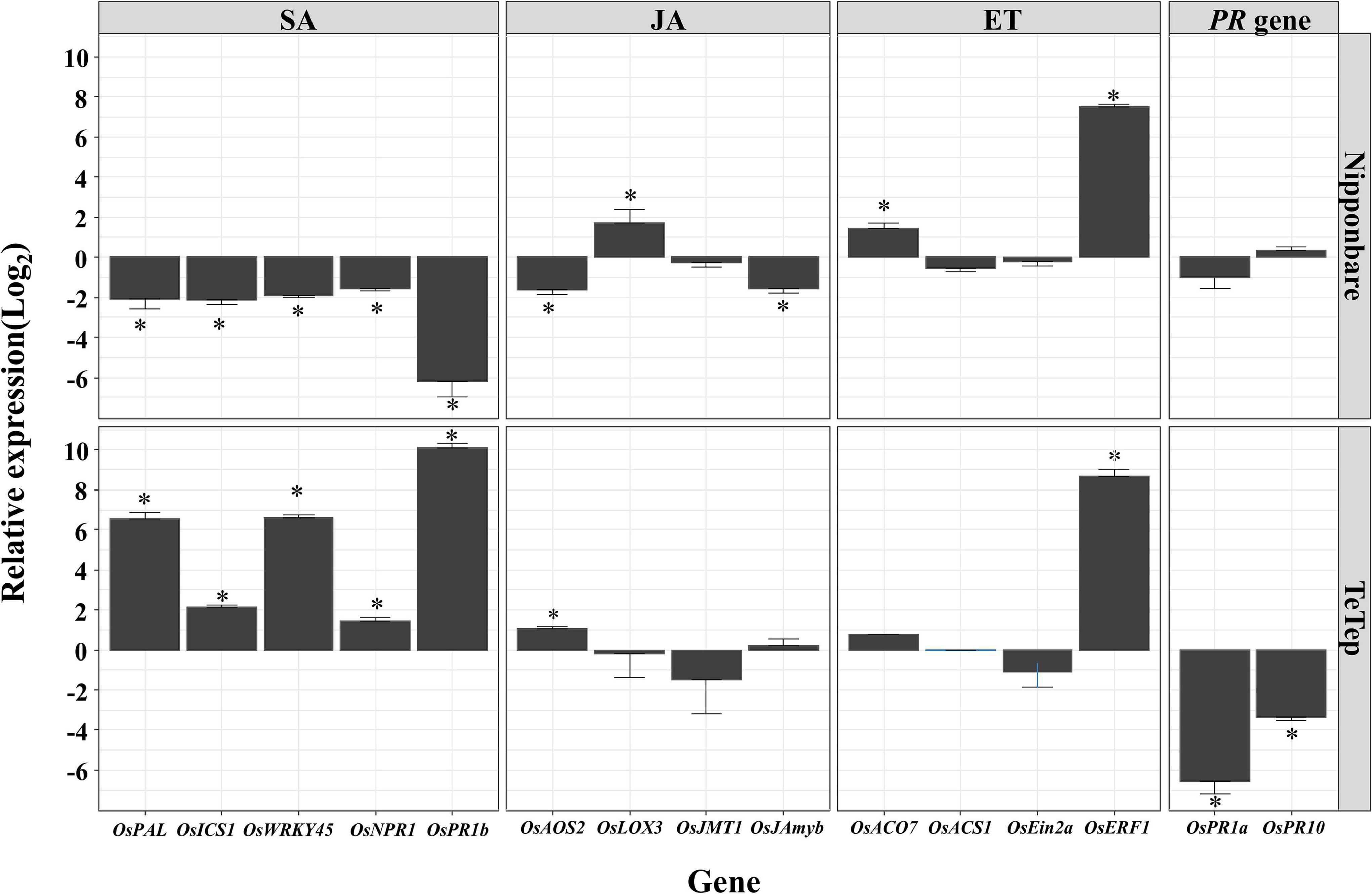
Figure 3. Quantitative real-time (qRT)-PCR analysis on the selected defense-related genes in panicle tissues in two cultivars at flowering stage. Bars represent mean expression levels and SE from four technical replicates of one biological replicate, containing a pool of eight panicles. The other two biological replicates show similar results. Data are shown as relative expression levels of infected tissues in comparison with the control tissue (uninfected tissue). Gene expression levels were normalized using two internal reference genes, OsEXP and OsEXPNarsai. Asterisks indicate statistically significant (α = 0.05) differential expression in comparison with control plants, performed using R version 3.3.0.
During the interaction of Nipponbare and A. besseyi, the SA marker genes were significantly downregulated at this stage compared with corresponding control. On the contrary, all these genes showed significantly upregulated expression levels in Tetep upon A. besseyi infection at this time points compared with those in the control.
For the key genes involved in JA response, the expressions of OsAOS2 and OsJAmyb were down-regulated, while OsLOX3 was upregulated at the flowering stage in Nipponbare. In contrast, in Tetep, only the expression of OsAOS2 was upregulated by A. besseyi infection, and all the other genes showed similar expression level compared with the control.
For ET signaling marker genes, OsERF1 appeared to be significantly upregulated both in Nipponbare and Tetep. OsACO7 was significantly upregulated in Nipponbare, but this gene was not affected by A. besseyi infection in Tetep. Moreover, the other two genes showed no significant differences in the transcription level in both cultivars compared with the corresponding controls.
OsPR1a and OsPR10 were not significantly affected in Nipponbare but were both significantly downregulated in Tetep at the flowering stage after nematode infection.
The Effect of the Application of Hormones or Hormone Inhibitors on Nipponbare Susceptibility to Aphelenchoides besseyi
To analyze the role of SA, JA, and ET in rice defense against A. besseyi, the number of nematodes was examined at two stages in Nipponbare. The results indicated that both in plants of 25 dai and in grains, the number of A. besseyi significantly reduced in hormone-treated plants when compared with the corresponding control in two concentrations (Figure 4 and Supplementary Figure 2), and the results showed the two concentrations had similar effects on nematode infection. As the results showed in Figure 4, in BTH-, MeJA-, and ethephon-treated plants, the number of nematodes exhibited decrease of 79.91, 77.58, and 71.47% at 25 dai, respectively. While in grains, BTH-, MeJA-, and ethephon-treated plants showed 63.71, 73.96, and 70.39% nematode reductions, respectively.
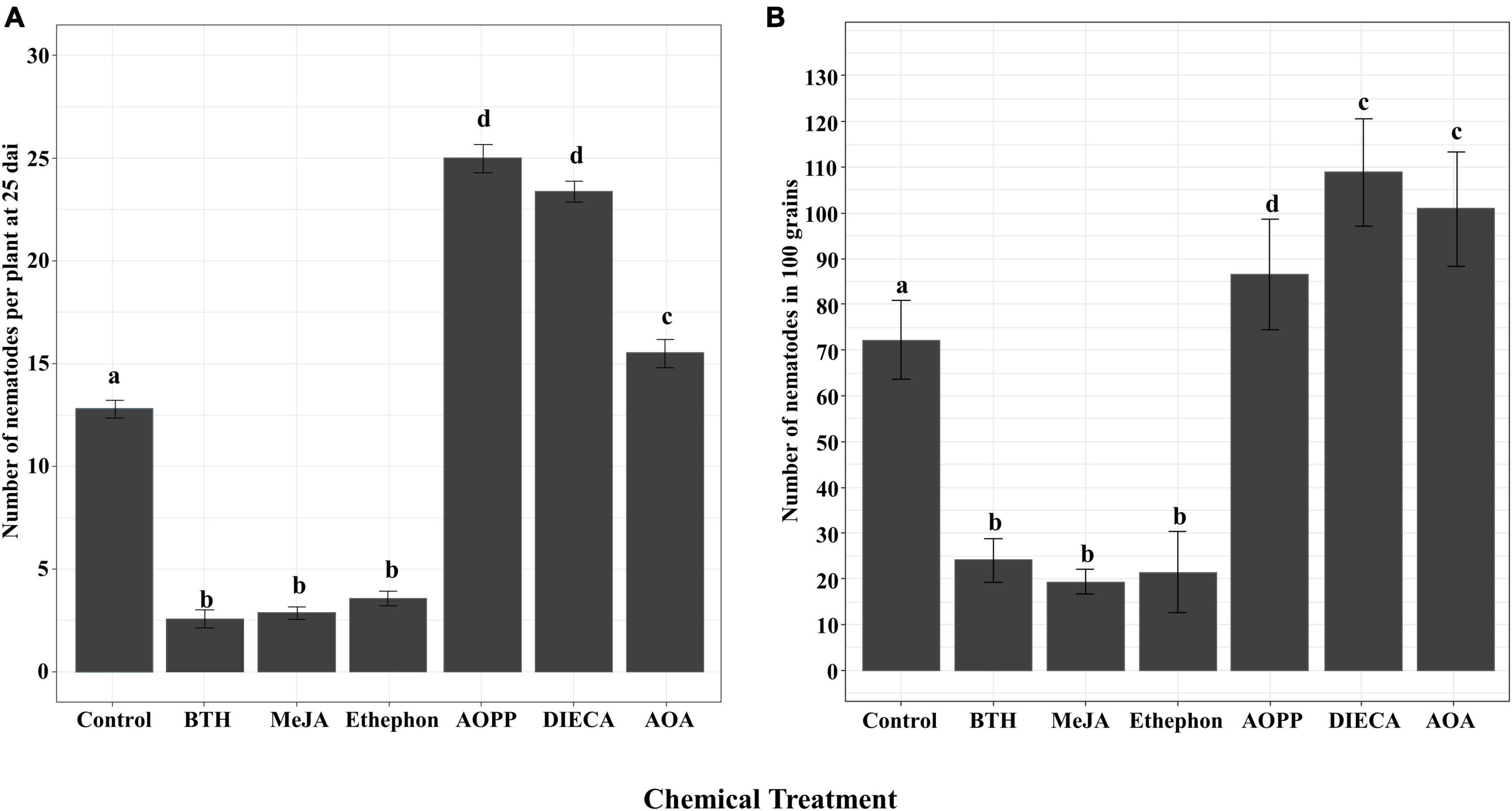
Figure 4. Effects of foliar application of plant hormones and hormone biosynthesis inhibitors on rice defense against Aphelenchoides besseyi infection. Shoots of six-day-old plants were sprayed until runoff with 125 μM BTH, 250 μM MeJA, 250 μM ethephon, 100 μM DIECA, 100 μM AOPP, and 20 mM AOA or water. At 24 h after treatment, plants were inoculated with 250 mixed stages of A. besseyi. (A) The number of nematodes per plant at 25 dai. Bars represent means ± SE from 3n = 45 plants. (B) The number of nematodes in 100 mature grains. Bars represent means ± SE from 3n = 15 in 100 grains. Different letters indicate statistically significant differences (Tukey test with α = 0.05). MeJA, methyl jasmonate; ethephon (converted to ET in the plant); BTH, benzathiadiazole (SA analog); AOA, aminooxyacetic acid (ET biosynthesis inhibitor); DIECA, diethyldithiocarbamic acid (JA biosynthesis inhibitor); AOPP, L-2-aminooxy-3-phenylpropionic acid (inhibitor of biosynthesis of phenylpropanoids, including SA).
In contrast, chemical inhibition of the phenylpropanoid pathway with AOPP, JA biosynthesis with DIECA, or ET production with AOA all resulted in a significantly increased susceptibility toward A. besseyi both in 25 dai plants and grains (Figure 4). In 25 dai plants and grains, compared with those in control, AOPP-, DIECA-, and AOA-treated plants showed an increase of 91.51 and 19.76%, 83.84 and 50.78%, and 16.43 and 39.70% nematodes, respectively.
Susceptibility of Rice Hormone Pathway Mutants to Aphelenchoides besseyi
To obtain a more detailed understanding of the role of these defense hormone pathways against A. besseyi, the number of nematodes was evaluated in the mutants or transgenic lines impaired in hormone pathways. Both in 25 dai plants and grains, the SA-signaling deficient mutant WRKY45-RNAi, the SA-deficient mutant NahG, and the JA insensitive mutant Coi1-13 all showed a significant increase in the number of nematodes compared with the corresponding control of Nipponbare. Nevertheless, the ET-insensitive mutant EIN2b-RNAi did not have a significant change in the number of nematodes compared with that in control either in 25 dai plants or grains. The nematode number in the JA biosynthesis mutant hebiba had no significant change in 25 dai plants but was significantly increased in grains in comparison with control Nihonmasari (Figure 5).
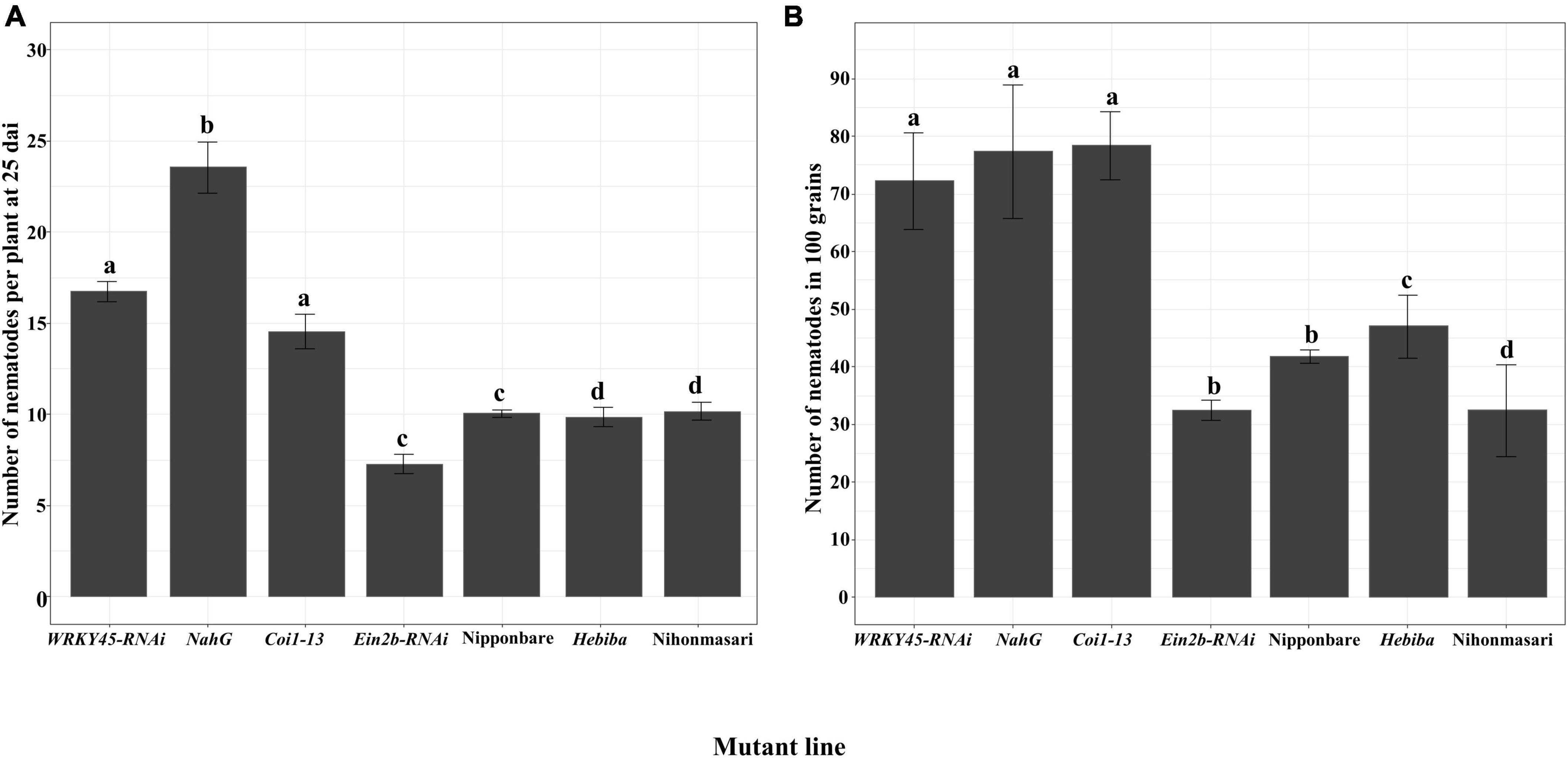
Figure 5. The number of A. besseyi on hormone biosynthesis or signaling deficient lines. Seven-day-old plants were inoculated with 250 mixed stages of Aphelenchoides besseyi. (A) The number of nematodes per plant at 25 dai. Bars represent means ± SE from 3n = 45 plants. (B) The number of nematodes in 100 mature grains. Bars represent means ± SE from 3n = 15 in 100 grains. Different letters indicate statistically significant differences (Tukey test with α = 0.05). WRKY45-RNAi, SA signaling deficient line; NahG, SA biosynthesis deficient line; Ein2b-RNAi, ET-insensitive line; Coi1-13, JA-insensitive line and their corresponding wild-type Nipponbare; Hebiba, JA biosynthesis mutant and its corresponding wild-type Nihonmasari.
Discussion
It has been reported that Tetep is highly resistant to A. besseyi according to the few nematodes in 100 grains and the lack of “white tip” symptom, but the underlying mechanism remains largely unknown (Feng et al., 2014). To gain insights into the defense mechanisms of Tetep against A. besseyi, in this study, the number of nematodes and the expression levels of plant hormone marker genes were investigated in cultivar Tetep and Nipponbare. The number of nematodes in Tetep were significantly lower at all the time points as well as in 100 grains compared with Nipponbare, which indicates that the resistance in Tetep may be associated with the reduced numbers of invasion, development, and reproduction of A. besseyi. The same resistance mechanisms were also observed in previous studies on cultivars resistant to other nematodes (Linsell et al., 2014; Khanam et al., 2018; Lahari et al., 2020; Nzogela et al., 2020).
Several studies have been conducted to explore the role of SA, JA, and ET during the infection of parasitic nematodes in rice or dicotyledonous plants (Bhattarai et al., 2008; Uehara et al., 2010; Nahar et al., 2011, 2012; Khanam et al., 2018). Our study aims to provide a characterization of the role of SA, JA, or ET in rice defense against the foliar nematode A. besseyi, which has a different strategy of multiplication and dispersal compared with root nematodes or stem nematodes (Huang and Huang, 1972; Nandakumar et al., 1975).
The plant hormone SA plays an important role as a signaling molecule during the defense reaction on pathogen infection (Lefevere et al., 2020). SA is generally linked with defense against biotrophic pathogens and usually activated in systemic acquired resistance (SAR), which is also accompanied by the induction of a set of PR genes (Van Loon et al., 2006; Vlot et al., 2009). It is widely accepted that SA in plants is synthesized by both isochorismate synthase (ICS) and PAL, which starts from chorismate (Lefevere et al., 2020), and SA-signaling pathway in rice branches into WRKY45-dependent and NPR1-dependent sub-pathways (Shimono et al., 2007; Sugano et al., 2010). It has been found that foliar application of BTH to rice plants only resulted in a minor induction of systemic defense against the root-knot nematode M. graminicola; however, BTH could induce stronger defense in rice against migratory root nematode H. oryzae and rice stem nematode D. angustus (Nahar et al., 2012; Khanam et al., 2018) as well as other sedentary nematodes (Owen et al., 2002; Nandi et al., 2003; Branch et al., 2004; Wubben et al., 2008). Our expression analyses of SA biosynthesis in Nipponbare and Tetep showed that the related genes were downregulated in infected susceptible cultivar Nipponbare but were hardly affected in resistant cultivar Tetep at seedling stages. However, SA signaling genes such as OsWRKY45 and OsNPR1 were upregulated at early growth stage of rice in above-ground tissue of Nipponbare, which may be due to the mechanical wounding upon nematode feeding as found in the rice infested by brown planthopper (Huangfu et al., 2016). At the flowering stage, a sudden burst of A. besseyi happened along with the significant downregulation of SA-related genes in Nipponbare, but the significantly upregulation of SA-related genes was observed in Tetep. Further results showed that exogenous application of SA analog, BTH, strongly decreased the rice susceptibility to A. besseyi, and corresponding transgenic biosynthesis/signaling defective lines or inhibitor-treated plants showed increased susceptibility. Based on the data provided here, we can conclude that the SA-dependent defense pathway in rice induces a strong defense against the rice foliar nematode A. besseyi.
Jasmonates enable plants to defend themselves against attacks by a wide variety of herbivores (Pieterse et al., 2012; Campos et al., 2014), and JA has also been shown to mediate defense against some biotrophic pathogens such as M. graminicola and Xanthomonas oryzae (Nahar et al., 2011; De Vleesschauwer et al., 2012). Our data showed that exogenous application of MeJA resulted in a strong reduction of nematode number in plants or grains; this observation is in line with previous findings in other nematodes (Nahar et al., 2011, 2012; Khanam et al., 2018). However, previous studies in dicotyledonous plants showed that MeJA application on roots of oat (Avena sativa) and spinach (Spinacia oleracea) enhanced resistance to the root-knot nematodes due to the stimulated toxic compounds such as 20-hydroxyecdysone and flavone-C-glycosides after MeJA treatment. These compounds are toxic to nematodes in vitro and have been implicated in plant defense against plant-parasitic nematodes (Soriano et al., 2004a,b). Our results reveal that JA plays a positive role in rice resistance against A. besseyi rather than the toxic effect, which is exemplified by the much higher number of nematodes in JA inhibitor-treated or mutant plants in either seedlings or grains. However, the nematode number in the JA biosynthesis mutant hebiba had no significant changes at 25 dai compared with its control Nihonmasari, this probably due to the relatively smaller number of nematodes in the plants at seedling stage.
Generally, the ET pathway synergistically activates JA pathway against necrotrophic pathogens and herbivore insects (Kessler and Baldwin, 2002; Rojo et al., 2003; Howe and Jander, 2008; Pieterse et al., 2012). However, it largely depends on the pathogen lifestyle, infection stage, as well as infected tissues. Our data showed that exogenous application of ethephon leads to a significantly decreased number of nematodes in plants or grains than control, and inhibited ET biosynthesis resulted in an increased susceptibility of the rice plants toward A. besseyi. However, mutants with impaired ET signaling (Ein2b-RNAi) did not show a significant change in the nematode number neither in plants nor in grains. Considering this result, we deduced that ET biosynthesis, rather than ET signaling, is responsible for ET-mediated systemic defense against A. besseyi. Our case is in contrast to the defense pathway of ET involved in rice root innate immunity against M. graminicola, in which ET signaling is in charge of ET-mediated systemic defense against M. graminicola.
In this study, we presented the responses of two rice cultivars to the foliar nematode A. besseyi infection either in nematode number or gene expression. Furthermore, we clarified the roles of SA, JA, and ET pathways in the interaction of rice and A. besseyi. Our data show that the resistance in Tetep resulted from the suppression of nematode infection, development, and reproduction. The defense mechanisms in Tetep at least in part were achieved by activating the expression of SA-related defense genes, especially at the flowering stage. Exogenous application of hormones, their analogs, or inhibitors, as well as the analyses of the expression of the marker genes, shows that SA, JA, or ET is involved in rice defense against A. besseyi. Nevertheless, the cross talk between the three hormones and other plant hormones in the interaction of rice and A. besseyi needs to be further investigated.
Data Availability Statement
The original contributions presented in the study are included in the article/Supplementary Material, further inquiries can be directed to the corresponding author.
Author Contributions
HJ and YP initiated and designed the research. JX performed most of the experiments, analyzed the data, and wrote the manuscript. FY and XX helped to conduct the qRT-PCR experiments and analyzed the data. All authors read and approved the final manuscript.
Funding
This project was supported by the financial support of the National Natural Science Foundation of China (Grant No. 31701769), the financial support from Sichuan Youth Science and Technology Foundation (Grant No. 2017JQ0004), the Agricultural Science and Technology Project of Chengdu (Grant No. 2015-NY02-00400-NC), Earmarked Fund for China Agriculture Research System (Grant No. CARS-01-41), and Sichuan Science and Technology Programs (Grant Nos. 2018JY0628 and 2019YFH0115).
Conflict of Interest
The authors declare that the research was conducted in the absence of any commercial or financial relationships that could be construed as a potential conflict of interest.
Publisher’s Note
All claims expressed in this article are solely those of the authors and do not necessarily represent those of their affiliated organizations, or those of the publisher, the editors and the reviewers. Any product that may be evaluated in this article, or claim that may be made by its manufacturer, is not guaranteed or endorsed by the publisher.
Acknowledgments
The authors would like to thank Cheng-Cai Chu (Chinese Academy of Sciences, China), Dong-Lei Yang (Nanjing Agricultural University, China), Yi-Nong Yang (Pennsylvania State University, United States), H. Takatsuji (Plant Disease Resistance Research Unit, National Institute of Agrobiological Sciences, Ibaraki, Japan), and P. Nick (Karlsruhe University, Karlsruhe, German) for providing the transgenic or mutant lines of rice. The authors would also like to thank Tina Kyndt for the careful reading of the manuscript.
Supplementary Material
The Supplementary Material for this article can be found online at: https://www.frontiersin.org/articles/10.3389/fpls.2021.755802/full#supplementary-material
Supplementary Figure 1 | White tip symptoms observed in Nipponbare and Tetep at the tillering stage. (A) The typical white tip symptoms can be observed in Nipponbare. Left below: White tip symptoms in detail (B). No typical white tip symptoms in Tetep.
Supplementary Figure 2 | Effects of foliar application of plant hormones at high concentrations on rice defense against Aphelenchoides besseyi infection. Shoots of six-day-old plants were sprayed until runoff with 250 μM BTH, 500 μM MeJA, and 500 μM ethephon. At 24 h after treatment, plants were inoculated with 250 mixed stages of A. besseyi. (A) The number of nematodes per plant at 25 dai. Bars represent means ± SE from 3n = 45 plants. (B) The number of nematodes in 100 mature grains. Bars represent means ± SE from 3n = 15 in 100 grains. Different letters indicate statistically significant differences (Tukey test with α = 0.05). MeJA, methyl jasmonate; ethephon (converted to ET in the plant); BTH, benzathiadiazole (SA analog).
References
Amrhein, N., and Gödeke, K.-H. (1977). α-Aminooxy-β-phenylpropionic acid—a potent inhibitor of Lphenylalanine ammonia-lyase in vitro and in vivo. Plant Sci. Lett. 8, 313–317. doi: 10.1016/0304-4211(77)90148-1
Bari, R., and Jones, J. (2009). Role of plant hormones in plant defence responses. Plant Mol. Biol. 69, 473–488. doi: 10.1007/s11103-008-9435-0
Beckers, G. J. M., and Spoel, S. H. (2006). Fine-tuning plant defence signaling: salicylate versus jasmonate. Plant Biol. 8, 1–10. doi: 10.1055/s-2005-872705
Bhattarai, K. K., Xie, Q. G., Mantelin, S., Bishnoi, U., Girke, T., Navarre, D. A., et al. (2008). Tomato susceptibility to root-knot nematodes requires an intact jasmonic acid signaling pathway. Mol. Plant Microbe Interact. 21, 1205–1214. doi: 10.1094/MPMI-21-9-1205
Branch, C., Hwang, C. F., Navarre, D. A., and Williamson, V. M. (2004). Salicylic acid is part of the Mi-1-mediated defence response to root-knot nematode in tomato. Mol. Plant Microbe Interact. 17, 351–356. doi: 10.1094/MPMI.2004.17.4.351
Campos, M. L., Kang, J. H., and Howe, G. A. (2014). Jasmonate-triggered plant immunity. J. Chem. Ecol. 40, 657–675. doi: 10.1007/s10886-014-0468-3
De Vleesschauwer, D., Van Buyten, E., Satoh, K., Balidion, J., Mauleon, R., Choi, I., et al. (2012). Brassinosteroids antagonize gibberellin- and salicylate-mediated root immunity in rice. Plant Physiol. 158, 1833–1846. doi: 10.1104/pp.112.193672
De Waele, D. (2002). “Foliar nematodes: Aphelenchoides species,” in Plant Resistance to Parasitic Nematodes, eds J. L. Starr, R. Cook, and J. Bridge (Wallingford, UK: CAB International), 141–151.
Duncan, L. W., and Moens, M. (2013). “Migratory endoparasitic nematodes,” in Plant Nematology, 2nd Edn, eds R. N. Perry and M. Moens (Wallingford, UK: CAB International), 144–178.
Farmer, E. E., Caldelari, D., Pearce, G., Walker-Simmons, M. K., and Ryan, C. A. (1994). Diethyldithiocarbamic acid inhibits the octadecanoid signaling pathway for the wound induction of proteinase inhibitors in tomato leaves. Plant Physiol. 106, 337–342. doi: 10.1104/pp.106.1.337
Feng, H., Wei, L. H., Lin, M. S., and Zhou, Y. J. (2014). Assessment of rice cultivars in China for field resistance to Aphelenchoides besseyi. J. Integr. Agric. 13, 2221–2228. doi: 10.1016/S2095-3119(13)60608-5
Fortuner, R., and Merny, G. (1979). Root-parasitic nematodes of rice. Revue de Nematologie 2, 79–102.
Fortuner, R., and Orton Williams, K. J. (1975). Review of the literature on Aphelenchoides besseyi Christie, 1942, the nematode causing “white tip” disease in rice. Plant Nematol. 44, 1–40.
Glazebrook, J. (2005). Contrasting mechanisms of defence against biotrophic and necrotrophic pathogens. Annual Rev. Phytopathol. 43, 205–227. doi: 10.1146/annurev.phyto.43.040204.135923
Goto, K., and Fukatsu, R. (1952). Studies on white tip of rice plant caused by Aphelenchoides oryzae YOKOO II. Japanese J. Phytopathol. 16, 57–60.
Grant, M. R., and Jones, J. D. G. (2009). Hormone (dis)harmony moulds plant health and disease. Science 324, 750–752. doi: 10.1126/science.1173771
Howe, G. A., and Jander, G. (2008). Plant immunity to insect herbivores. Annual Rev. Plant Biol. 59, 41–66. doi: 10.1146/annurev.arplant.59.032607.092825
Huang, C. S., and Huang, S. P. (1972). Bionomics of white-tip nematode. Bot. Bull. Acad. Sinica 13, 1–10.
Huangfu, J., Li, J., Li, R., Ye, M., Kuai, P., Zhang, T., et al. (2016). The transcription factor OsWRKY45 negatively modulates the resistance of rice to the brown planthopper Nilaparvata lugens. International J. Mol. Sci. 17:697. doi: 10.3390/ijms17060697
Iwai, T., Miyasaka, A., Seo, S., and Ohashi, Y. (2006). Contribution of ethylene biosynthesis for resistance to blast fungus infection in young rice plants. Plant Physiol. 142, 1202–1215. doi: 10.1104/pp.106.085258
Jones, J. T., Haegema, A., Danchin, E. G., Gaur, H. S., Helder, J., Jones, M. G. K., et al. (2013). Top 10 plant-parasitic nematodes in molecular plant pathology. Mol. Plant Pathol. 14, 946–961. doi: 10.1111/mpp.12057
Kessler, A., and Baldwin, I. T. (2002). Plant responses to insect herbivory: the emerging molecular analysis. Annual Rev. Plant Biol. 53, 299–328. doi: 10.1146/annurev.arplant.53.100301.135207
Khanam, S., Bauters, L., Singh, R. R., Verbeek, R., Haeck, A., Sultan, S. M., et al. (2018). Mechanisms of resistance in the rice cultivar Manikpukha t the rice stem nematode Ditylenchus angustus. Mole. Plant Pathol. 19, 1391–1402. doi: 10.1111/mpp.12622
Koornneef, A., and Pieterse, C. M. J. (2008). Cross talk in defence signaling. Plant Physiol. 146, 839–844. doi: 10.1104/pp.107.112029
Kyndt, T., Nahar, K., Haegeman, A., De Vleesschauwer, D., Hofte, M., and Gheysen, G. (2012). Comparing systemic defence-related gene expression changes upon migratory and sedentary nematode attack in rice. Plant Biol. 14, 73–82. doi: 10.1111/j.1438-8677.2011.00524.x
Lahari, Z., Nkurunziza, R., Bauters, L., and Gheysen, G. (2020). Analysis of Asian Rice (Oryza sativa) genotypes reveals a new source of resistance to the root-knot nematode Meloidogyne javanica and the root-lesion nematode Pratylenchus zeae. Phytopathology 110, 1572–1577. doi: 10.1094/PHYTO-11-19-0433-R
Lefevere, H., Bauters, L., and Gheysen, G. (2020). Salicylic acid biosynthesis in plants. Front. Plant Sci. 11:338. doi: 10.3389/fpls.2020.00338
Lin, M., Ding, X., Wang, Z., Zhou, F., and Lin, N. (2004). Description of Aphelenchoides besseyi from abnormal rice with ‘small grains and erect panicles’ symptom in China. Rice Sci. 12, 289–294.
Linsell, K. J., Riley, I. T., Davies, K. A., and Oldach, K. H. (2014). Characterization of resistance to Pratylenchus thornei (Nematoda) in wheat (Triticum aestivum): attraction, penetration, motility, and reproduction. Phytopathology 104, 174–187. doi: 10.1094/PHYTO-12-12-0345-R
López, M. A., Bannenberg, G., and Castresana, C. (2008). Controlling hormone signaling is a plant and pathogen challenge for growth and survival. Curr. Opin. Plant Biol. 11, 420–427. doi: 10.1016/j.pbi.2008.05.002
Lorenzo, O., and Solano, R. (2005). Molecular players regulating the jasmonate signalling network. Curr. Opin. Plant Biol. 8, 532–540. doi: 10.1016/j.pbi.2005.07.003
Mao, C., Wang, S., Jia, Q., and Wu, P. (2006). OsEIL1, a rice homolog of the Arabidopsis EIN3 regulates the ethylene response as a positive component. Plant Mol. Biol. 61, 141. doi: 10.1007/s11103-005-6184-1
Muthukrishnan, T. S., Rajendran, G., and Chandrasekaran, J. (1974). Studies on the white-tip nematode of rice. Indian J. Nematol. 4, 188–193.
Nahar, K., Kyndt, T., De Vleesschauwer, D., Hofte, M., and Gheysen, G. (2011). The jasmonate pathway is a key player in systemically induced defence against root knot nematodes in rice. Plant Physiol. 157, 305–316. doi: 10.1104/pp.111.177576
Nahar, K., Kyndt, T., Nzogela, Y. B., and Gheysen, G. (2012). Abscisic acid interacts antagonistically with classical defence pathways in rice–migratory nematode interaction. New Phytol. 196, 901–913. doi: 10.1111/j.1469-8137.2012.04310.x
Nandakumar, C., Prasad, J. S., Rao, Y. S., and Rao, J. (1975). Investigation on the white-tip nematode (Aphelenchoides besseyi Christie, 1942) of rice (Oryza sativa L). Indian J. Nematol. 5, 62–69.
Nandi, B., Kundu, K., Banerjee, N., and Babu, S. P. S. (2003). Salicylic acid-induced suppression of Meloidogyne incognita infestation of okra and cowpea. Nematology 5, 747–752. doi: 10.1163/156854103322746922
Nzogela, Y. B., Luzi-Kihupi, A., De Waele, D., and Gheysen, G. (2020). Comparison of the penetration, development and reproduction of Meloidogyne javanica and M. graminicola on partially resistant Oryza sativa cultivars from East Africa. Nematology 22, 381–399. doi: 10.1163/15685411-00003312
Owen, K. J., Green, C. D., and Deverall, B. J. (2002). A benzothiadiazole applied to foliage reduces development and egg deposition by Meloidogyne spp. in glasshouse-grown grapevine roots. Australas. Plant Pathol. 31, 47–53. doi: 10.1071/AP01068
Pei, Y., Luo, A., Xie, H., Yang, Z., Cheng, X., and Xu, C. (2010). Reproductive fitness of 15 Aphelenchoides besseyi populations from China. J. Northwest A F Univ. 38, 165–170.
Pieterse, C. M. J., Leon-Reyes, A., der Ent, S. V., and Van Wees, S. C. M. (2009). Networking by small-molecule hormones in plant immunity. Nature Chem. Biol. 5, 308–316. doi: 10.1038/nchembio.164
Pieterse, C. M. J., Van der Does, D., Zamioudis, C., Leon-Reyes, A., and Van Wees, S. C. (2012). Hormonal modulation of plant immunity. Annual Rev. Cell Dev. Biol. 28, 489–521. doi: 10.1146/annurev-cellbio-092910-154055
Prot, J. C., and Rahman, M. L. (1994). “Nematode ecollogy, economic importance, and management in rice ecosystems in South and Southeast Asia,” in Rice Pest Science and Management, eds P. S. Teng, K. L. Heong, and K. Moody (Philippines: IRRI, Manila), 129–144.
R Core Team (2016). R foundation for Statistical Computing. Austria: R Foundation for Statistical Computing.
Reversat, G., Boyer, J., Sannier, C., and Pando-Bahuon, A. (1999). Use of a mixture of sand and water-absorbent synthetic polymer as substrate for the xenic culturing of plant-parasitic nematodes in the laboratory. Nematology 1, 209–212. doi: 10.1163/156854199508027
Robert-Seilaniantz, A., Grant, M., and Jones, J. D. G. (2011). Hormone crosstalk in plant disease and defence: more than just jasmonate-salicylate antagonism. Annual Rev.Phytopathol. 49, 317–343. doi: 10.1146/annurev-phyto-073009-114447
Rojo, E., Solano, R., and Sanchez-Serrano, J. J. (2003). Interactions between signaling compounds involved in plant defence. J. Plant Growth Regulat. 22, 82–98. doi: 10.1007/s00344-003-0027-6
Shimono, M., Sugano, S., Nakayama, A., Jiang, C. J., Ono, K., Toki, S., et al. (2007). Rice WRKY45 plays a crucial role in benzothiadiazole-inducible blast resistance. Plant Cell 19, 2064–2076. doi: 10.1105/tpc.106.046250
Soriano, I. R., Asenstorfer, R. E., Schmidt, O., and Riley, I. T. (2004a). Inducible flavone in oats (Avena sativa) is a novel defence against plant-parasitic nematodes. Phytopathology 94, 1207–1214. doi: 10.1094/PHYTO.2004.94.11.1207
Soriano, I. R., Riley, I. T., Potter, M. J., and Bowers, W. S. (2004b). Phytoecdysteroids: a novel defence against plant-parasitic nematodes. J. Chem. Ecol. 30, 1885–1899. doi: 10.1023/B:JOEC.0000045584.56515.11
Sugano, S., Jiang, C. J., Miyazawa, S. I., Masumoto, C., Yazawa, K., Hayashi, N., et al. (2010). Role of OsNPR1 in rice defence program as revealed by genome-wide expression analysis. Plant Mol. Biol. 74, 549–562. doi: 10.1007/s11103-010-9695-3
Tikhonova, L. V. (1966). Aphelenchoides besseyi Christie, 1942 (Nematoda). Zool. Zhurnal 45, 1759–1766.
Tiwari, S. P., and Khare, M. N. (2003). “White tip caused by Aphelenchoides besseyi, an important seed borne disease of rice,” in Advances in Nematology, ed. P. C. Trivedi (Jodhpur: Scientific Publishers), 103–114.
Togashi, K., and Hoshino, S. (2001). Distribution pattern and mortality of the white tip nematode. Nematology 3, 17–24. doi: 10.1163/156854101300106847
Tulek, A., and Cobanoglu, S. (2010). Distibution of the rice white tip nematode. Nematol. Mediterr. 38, 215–217.
Tulek, A., Kepenekci, I., Cobanoglu, S., Hekimhan, H., Devran, Z., Melik, B., et al. (2009). A new culturing method for the rice white tip nematode, Aphelenchoides besseyi Christie, 1942, on carrot discs. Russian J. Nematol. 17, 143–144.
Uehara, T., Sugiyama, S., Matsuura, H., Arie, T., and Masuta, C. (2010). Resistant and susceptible responses in tomato to cyst nematode are differentially regulated by salicylic acid. Plant Cell Physiol. 51, 1524–1536. doi: 10.1093/pcp/pcq109
Van Loon, L. C., Geraats, B. P. J., and Linthorst, H. J. M. (2006). Ethylene as a modulator of disease resistance in plants. Trends Plant Sci. 11, 184–191. doi: 10.1016/j.tplants.2006.02.005
Vlot, A. C., Dempsey, D. A., and Klessig, D. F. (2009). Salicylic acid, a multifaceted hormone to combat disease. Annual Rev. Phytopathol. 47, 177–206. doi: 10.1146/annurev.phyto.050908.135202
Wang, F., Li, D., Wang, Z., Dong, A., Liu, L., Wang, B., et al. (2014). Transcriptomic analysis of the rice white tip nematode. PLoS One 9:e91591. doi: 10.1371/journal.pone.0091591
Wang, Y., Yang, F., Zhang, Z. M., Ni, J., Kang, X., Peng, Y., et al. (2017). Current status and sources of infestation of commercial rice seeds by Aphelenchoides besseyi in China. Southwest China J. Agric. Sci. 30, 1772–1776.
Wubben, M. J. E., Jin, J., and Baum, T. J. (2008). Cyst nematode parasitism of Arabidopsis thaliana is inhibited by salicylic acid (SA) and elicits uncoupled SA-independent pathogenesis-related gene expression in roots. Mol. Plant Microbe Interact. 21, 424–432. doi: 10.1094/MPMI-21-4-0424
Keywords: plant hormones, rice, Tetep, Nipponbare, rice white tip nematode
Citation: Xie J, Yang F, Xu X, Peng Y and Ji H (2022) Salicylic Acid, Jasmonate, and Ethylene Contribute to Rice Defense Against White Tip Nematodes Aphelenchoides besseyi. Front. Plant Sci. 12:755802. doi: 10.3389/fpls.2021.755802
Received: 09 August 2021; Accepted: 20 December 2021;
Published: 20 January 2022.
Edited by:
Xiaojie Wang, Northwest A&F University, ChinaReviewed by:
Abolfazl Hajihassani, University of Georgia, United StatesDeliang Peng, Institute of Plant Protection, Chinese Academy of Agricultural Sciences (CAAS), China
Copyright © 2022 Xie, Yang, Xu, Peng and Ji. This is an open-access article distributed under the terms of the Creative Commons Attribution License (CC BY). The use, distribution or reproduction in other forums is permitted, provided the original author(s) and the copyright owner(s) are credited and that the original publication in this journal is cited, in accordance with accepted academic practice. No use, distribution or reproduction is permitted which does not comply with these terms.
*Correspondence: Hongli Ji, SG9uZ2xpLkppQEppaG9uZ2xpLmNvbQ==