- Plant Molecular Biology and Biotechnology Laboratory, Faculty of Veterinary and Agricultural Sciences, The University of Melbourne, Parkville, VIC, Australia
Drought is one of the significant abiotic stresses threatening crop production worldwide. Soybean is a major legume crop with immense economic significance, but its production is highly dependent on optimum rainfall or abundant irrigation. Also, in dry periods, it may require supplemental irrigation for drought-susceptible soybean varieties. The effects of drought stress on soybean including osmotic adjustments, growth morphology and yield loss have been well studied. In addition, drought-resistant soybean cultivars have been investigated for revealing the mechanisms of tolerance and survival. Advanced high-throughput technologies have yielded remarkable phenotypic and genetic information for producing drought-tolerant soybean cultivars, either through molecular breeding or transgenic approaches. Further, transcriptomics and functional genomics have led to the characterisation of new genes or gene families controlling drought response. Interestingly, genetically modified drought-smart soybeans are just beginning to be released for field applications cultivation. In this review, we focus on breeding and genetic engineering approaches that have successfully led to the development of drought-tolerant soybeans for commercial use.
Introduction
Soybean, an important legume, is one of the most widely grown food crops in the world due to its valuable seed composition. In 2019, the annual global soybean production was estimated to be above 333 million tones (Faostat, 2019). Soybean provides an inexpensive source of protein and fats, and natural nitrogen fertilisation for the soil (Foyer et al., 2016). Interestingly, the economic benefits derived from soybean cultivation are not just limited to the food supply; it is also an important industrial crop utilised in producing edible oils, wax, paints, dyes and fibre (Rezaei et al., 2002; Raghuvanshi and Bisht, 2010). Also, meat substitutes based on soybean are extensively used by vegan and vegetarian consumers (Messina and Messina, 2010; Raghuvanshi and Bisht, 2010; Tang, 2017).
Soybean is grown mainly in tropical, subtropical and temperate regions (Fao, 2021). It is a water-intensive crop, requiring substantial water to grow and reproduce (Bhardwaj, 1986). Consequently, rising global temperatures and changing precipitation patterns pose a significant threat to soybean production, especially in under-irrigated or rainfed areas (Jin et al., 2017; Cotrim et al., 2021). It is known that under dry conditions or drought, soybean yield can reduce by more than 50%, causing substantial financial losses to farmers and growers (Wei et al., 2018). Hence, drought is a significant climatic risk that calls for effective mitigation strategies to sustain the supply of soybeans worldwide.
Soybean varieties are classified into maturity groups according to their response to the photoperiod. Early maturing varieties belong to groups 0 to 3, whereas late-maturing varieties fall in groups 6 and onwards (Zhang et al., 2007; Yang et al., 2019). Drought impacts soybeans cultivars differently, as some cultivars are more susceptible than others (Oya et al., 2004; Maleki et al., 2013; Du et al., 2020; Dayoub et al., 2021). Also, the timing of drought stress, whether at the vegetative or the reproductive phase, is important in determining yield loss. Desclaux et al. (2000) investigated the drought-induced phenotypes of early maturity soybean varieties grown in France. They reported that drought stress at vegetative stages led to reduced plant height and a decline in seed number in the early reproductive stages and reduced seed weight in late reproductive stages. The water scarcity between flowering and early seed filling stages can affect branches’ vegetative growth, resulting in decreased branch seed number and reduced branch seed yield (Frederick et al., 2001). A report on the effect of drought on soybeans grown in the semi-arid and semi-humid regions of Huaibei regions of China reported a 73–82% decline in yield when drought stress was applied at flowering and seed filling stages (Wei et al., 2018). Recently, Du et al. (2020) reported that long-term drought stress in reproductive stages decreases biomass allocation to reproductive organs, thereby reducing seed weight in soybean. In addition, drought also impacts the symbiotic nitrogen-fixing ability of soybeans by disturbing nitrogenase activity, which can cause carbon shortage and oxygen limitation leading to poor growth and yield (Arrese-Igor et al., 2011; Collier and Tegeder, 2012; Kunert et al., 2016).
Plants use diverse mechanisms to overcome the adverse effects of drought, and the ability of crops to adjust using adaptive traits is termed ‘drought tolerance’ (Basu et al., 2016). Decreased stomatal opening associated with reduced photosynthesis is a typical drought response observed in plants (Liu et al., 2003; Mak et al., 2014). Abscisic acid (ABA) plays a vital role in reducing water loss under dry conditions (Wilkinson and Davies, 2002; Kim, 2014; Wang et al., 2016). Further drought-tolerant soybeans exhibit higher ABA levels than drought-susceptible varieties (Mutava et al., 2015). ABA, synthesised in plant roots, is transported to the guard cells of leaves, where it induces closure of stomatal openings to reduce water loss (Wilkinson and Davies, 2002). Further, there is evidence that ABA synthesised in leaf xylem also contributes to this process (Malcheska et al., 2017). However, reduction in the stomatal opening leads to reduced CO2 assimilation and photosynthesis, affecting growth and development (Cornic and Briantais, 1991; Ohashi et al., 2006; Mutava et al., 2015; Cohen et al., 2021).
Maintenance of cell turgidity is another essential adjustment to survive drought. Under dehydration conditions, cells induce biochemical changes by synthesising necessary metabolites called osmoprotectants (Silvente et al., 2012). These include soluble and complex sugars, sugar alcohols, organic acids and free amino acids (Ashraf and Iram, 2005; Silvente et al., 2012; Kido et al., 2013). Osmoprotectant accumulation in the cell balances the osmotic difference between cell exteriors and the cytosol help to retain water and maintain the integrity of the cell membrane (Yancey, 2005; Basu et al., 2016). An increase in soluble sugars, such as sucrose and fructose, improves homeostasis under stressed conditions. Further, soluble sugars are required for enhanced carbohydrate metabolism, signal transduction and synthesising enzymes and hormones needed to survive under drought (Gupta and Kaur, 2005; Mak et al., 2014; Du et al., 2020). Metabolite profiling of biochemical compounds synthesised during drought stress revealed an increase in pinitol in the leaves of a drought-susceptible cultivar (Silvente et al., 2012). Pinitol is a common sugar alcohol that acts as an osmoprotectant in legumes (Ford, 1984; Streeter et al., 2001; Dumschott et al., 2019). Further, Silvente et al. (2012) reported increased levels of amino acids, such as proline, during drought stress in flowering stages. Proline helps retain water by adjusting the intracellular osmotic potential of the cells (Heerden van and Krüger, 2002). In addition, reactive oxygen species (singlet oxygen) produced in stressed cells can cause severe oxidative damage under prolonged drought conditions. Alia et al. (2001) suggested that proline acts as a scavenger of singlet oxygen. However, the role of proline in quenching singlet oxygen in stressed plants remains debated as Signorelli et al. (2013) demonstrated that proline could not quench singlet oxygen in an aqueous buffer. Figure 1A summarises the effects of drought on soybean.
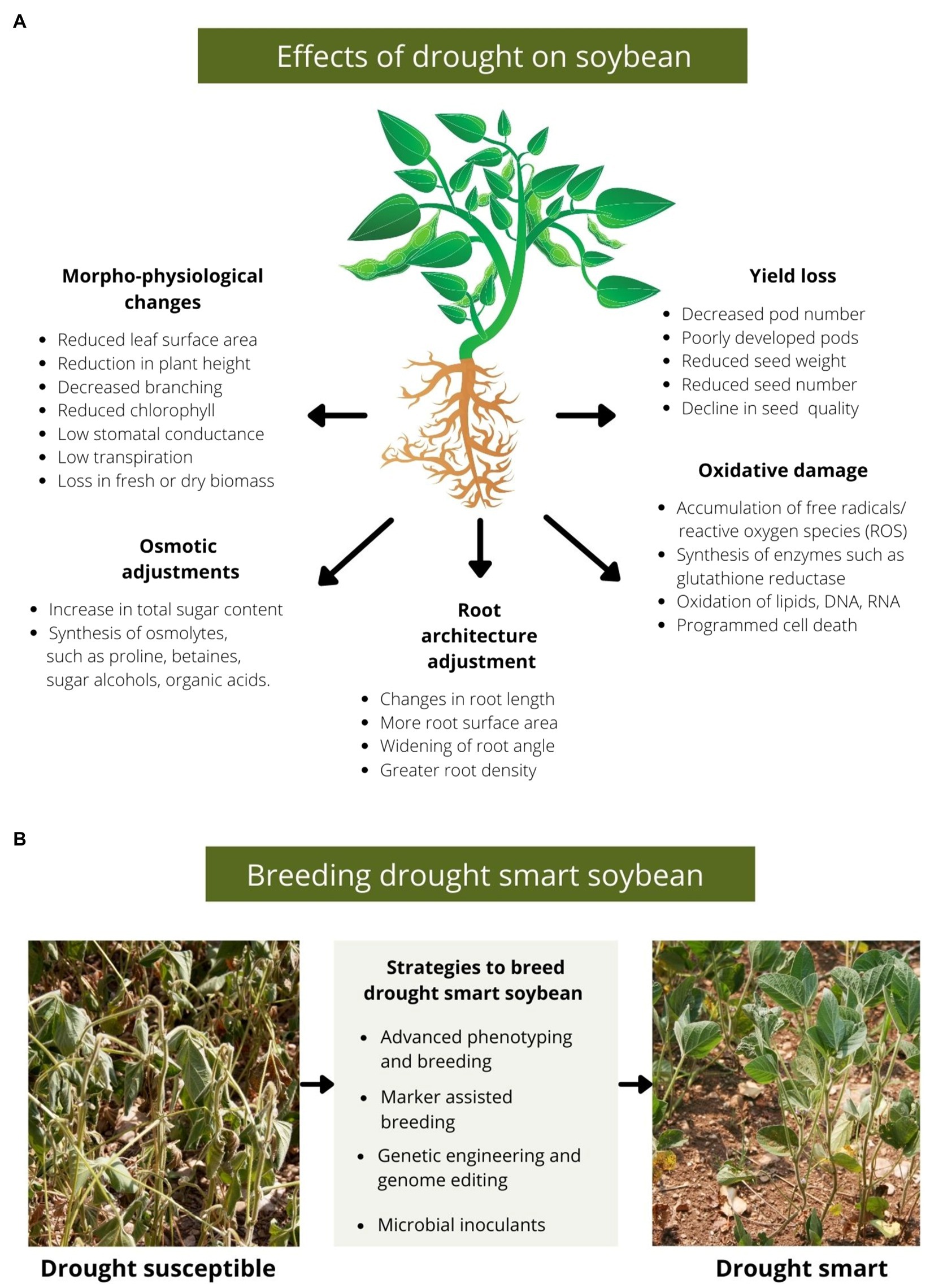
Figure 1. (A) A diagram depicting various effects of drought on soybean. Drought induces morphological changes, such as loss in vegetative biomass, accompanied by a reduction in pod number, seed number, seed weight and altered biochemical composition of seeds. Further, cells regulate the effect of drought, synthesising osmolytes, such as proline and sugar alcohols, to balance the osmotic potential for maintaining cell membrane integrity. Also, in many drought-resistant soybean cultivars, roots adjust their architecture in response to water-deficit conditions by changing root length, branching and other phenotypes to absorb more soil moisture. Further, severe drought stress leads to ROS accumulation which can cause cell and tissue damage by oxidising biomolecules. (B) An outline of strategies to breed drought-smart soybeans.
On the cellular level, largely overlapping signalling mechanisms and phytohormone cross-talks mediate drought response in plants (Basu et al., 2016). With the advent of high-throughput sequencing techniques, several genes or gene families have been identified and characterised in soybean (Wong et al., 2013; Zhang et al., 2020, 2021). Among these, the CUC (NAC; Hussain et al., 2017), MYB (Chen L. et al., 2021), WRKY (Shi et al., 2018), ABA-responsive element binding (AREB; Fuganti-Pagliarini et al., 2017) and dehydration response element-binding proteins (DREB; Nguyen et al., 2019) transcription factor families are some of the prime regulators that control drought response by regulating the synthesis of drought-responsive hormones, such as ABA, ethylene and other drought signalling compounds including Brassinosteroids (Nguyen et al., 2019; Chen L. et al., 2021).
Comparative transcriptomics have further elucidated the molecular mechanisms underlying drought response in soybean (Ha et al., 2015; Hussain et al., 2017). For example, Hussain et al. (2017) identified 28 drought-responsive GmNAC genes in soybean and reported that only eight GmNAC genes showed high expression levels in drought-tolerant soybean variety; with drought-sensitive cultivars exhibiting lower expression levels. WRKY transcription factors have been highlighted to play vital roles in plant abiotic stress tolerance (Ning et al., 2017; Shi et al., 2018). Shi et al. (2018) identified a drought-responsive soybean WRKY gene, GmWRKY12, whose over-expression in a transgenic hairy root assay led to increased proline levels under drought stress. Further, Wei et al. (2019) characterised another WRKY gene, GmWRKY54, which mediates drought tolerance via ABA and Ca+2 signalling pathways. The over-expression of GmWRKY54 conferred drought tolerance in soybean (Wei et al., 2019). Other recently identified gene families participating in soybean drought response are AT-hook motif (Wang et al., 2021b), P-type ATPases (Zhao et al., 2021), CCT family (Mengarelli and Zanor, 2021) and GRAS (Wang et al., 2020). Identifying new drought-responsive genes is vital for developing drought-smart soybeans. The term ‘drought-smart’ refers to soybean cultivars that can adapt to diverse types of drought, such as early drought, middle-stage drought, later-drought and seasonal drought, by combining different mechanisms of drought resistance, such as drought avoidance, drought tolerance and drought recovery. Drought avoidance is generally achieved by retaining water in plant tissues, either by restricting water loss or using water judiciously to support different plant functions. However, drought tolerance is achieved using adaptive traits, such as biochemical adjustments to maintain cell turgidity and minimise photosynthetic damage (Basu et al., 2016). Further, cultivars with drought avoidance and drought tolerance features can recover fast upon rehydration after a short-term or seasonal drought (Dong et al., 2019). Hence, smart soybeans are able to produce a stable yield in drought-prone areas. Traits, such as improved water retention, restricted transpiration and stable photosynthesis, are desirable to sustain biomass and yield under water-deficit conditions (Wei et al., 2019; Kunert and Vorster, 2020; Yang et al., 2020). A combination of such traits would make a cultivar suitable for cultivation in arid or semi-arid regions (Liu et al., 2005; Abdel-Haleem et al., 2012; Carter et al., 2016). Also, as genome sequencing of soybean cultivars gains momentum, the new genetic diversity resources will aid in developing drought-smart varieties using advanced breeding or genetic engineering methods (Fuganti-Pagliarini et al., 2017; Golicz et al., 2018; Kajiya-Kanegae et al., 2021). Here, we review genetic improvement approaches for improving drought tolerance in soybean.
Breeding for Drought-Smart Soybean
A combination of advanced phenotyping, molecular breeding and genetic engineering approaches can be employed to breed drought-smart soybeans (Figure 1B). Using conventional breeding techniques, pre-selected soybean germplasms (donor cultivars) with desired drought-responsive traits can be crossed to introduce favourable alleles in the resulting populations. However, extensive screening of subsequent generations is required to select a line with stable characteristics for continued cultivation (Carter et al., 2016). Further, genetic transformation offers the possibility of targeted gene expression under constitutive or inducible promoters (Ribichich et al., 2020). For example, Arabidopsis thaliana AtMYB44 gene was transformed in soybean using Agrobacterium-mediated transformation which resulted in improved soybeans with better yield under water-deficit conditions in the field (Seo et al., 2012). Improvements in tissue culture regeneration of commercial soybean cultivars and optimization of Agrobacterium-mediated transformation methods will also facilitate engineering soybeans for drought tolerance (Raza et al., 2017, 2019). Recently, more precise gene editing technique, CRISPR/cas9, has shown promising results in modifying soybean’s genome in a targetted manner to obtain more specific gene modifications. CRISPR/cas9 has been successfully employed to characterise soybean drought-responsive genes using knock-down approaches. For example, CRISPR/cas9-mediated mutagenesis of soybean circadian rhythm genes (GmLCLs) generated mutant plants with decreased water loss under dehydration stress conditions (Yuan et al., 2021). Interestingly, breeding and genetic transformation methods have successfully delivered improved soybeans that have been tested in laboratory, glasshouse or field conditions and a few of these varieties have also been approved for commercial production. In the sections below, we review recent examples of soybeans improved for drought tolerance via breeding or transgenic approaches.
Breeding and High-Throughput Phenotyping
Molecular breeding approaches for enhancing drought tolerance, such as marker-assisted selection, quantitative trait loci (QTL) mapping, GWAS and genomic selection, depend upon the extent of existing genetic diversity for the desirable traits. High genetic diversity of soybean makes it feasible to select cultivars with drought tolerance properties (Kajiya-Kanegae et al., 2021). Some of the traits to address water deficit are slow canopy wilting, water-conserving transpiration response, dense root surface area and low stomatal conductance (Kim, 2014; Basu et al., 2016).
Using phenotypic trait selection approaches, a high-yield and drought-tolerant cultivar was bred by the US Department of Agriculture and North Carolina State University researchers. This study involved extensive screening of soybean germplasm collected from around the world. Fifteen years of rigorous selection led to discovering a slow-wilting landrace PI 416937 from Japan and another resistant cultivar from Nepal, PI 471938. Continuous breeding efforts led to the development of a new drought-tolerant soybean variety released as ‘USDA-N8002’ (maturity group VIII) for field use (Carter et al., 2016).
Recently, high-throughput phenotyping of plant populations has widened trait identification by reducing the timeline and physical labour involved in the manual screening processes (Crusiol et al., 2021; Zhou et al., 2021). For example, remote sensing has shown great potential in analysing the genotypes of plants in a non-destructive way. These remote sensing mechanisms of phenotyping involve thermal, spectral and hyperspectral imaging techniques (Li et al., 2014). A simple example of spectral measurement is the estimation of chlorophyll content using the soil plant analysis development metre which measures red vs. infra-red light (Yuan et al., 2016). Hyperspectral imaging is an advanced imaging method used to detect material across the entire electromagnetic spectrum. It works on the principle that certain elements have unique spectral fingerprints that can be used to identify materials by analysing the image of the scanned object (Crusiol et al., 2021).
Crusiol et al. (2021) employed a leaf-based hyperspectral reflectance method to distinguish soybean genotypes under different moisture conditions and at different phenological stages. Leaf-based hyperspectral reflectance was collected from soybean genotypes growing under different watering conditions over multiple cropping seasons. Short-wave infra-red wavelength (1300–2,500nm) was critical in these measurements, as it can effectively detect vegetation water status. Principal component analysis of spectral datasets of soybean genotypes showed 94% variance in the first three components, indicating that spectral data could successfully distinguish the soybean genotypes.
Multispectral and visible RGB camera imaging was also performed by Zhou et al. (2021) to estimate the yield of soybean genotypes under drought stress. They employed the unmanned aerial vehicle technique for collecting image data to develop a method of yield estimation for large breeding populations. Images of 972 breeding lines were captured at vegetative (R6), early and late reproductive phases (R1 and R6-R8). By assessing the image features related to plant height, canopy colour and canopy texture, they built a deep learning model which could explain the yield to up to 78%. The yield of slow- and fast-wilting plants belonging to three genotypes (maturity group 3, 4 and 5) was assessed, and it was found that the fast-wilting group produced less average yield (986.5kg/ha) as compared to the slow-wilting group (1,395kg/ha). Hence, high-throughput imaging for phenotyping has a remarkable potential for assessing large populations to identify plants with drought-tolerant traits.
Marker-Assisted Breeding
Marker-assisted breeding is another promising approach for developing drought-tolerant soybeans. It relies on identifying variations in chromosomal regions, known as QTLs. QTLs that make a genotype more robust than others for drought tolerance are particularly valuable for breeding. Marker-assisted breeding uses DNA markers linked to specific QTLs for selecting genotypes with the desired alleles (Zhang et al., 2015). For example, Chen H. et al. (2021) identified QTLs related to primary root length on chromosome 16 of soybean. This QTL accounts for 30.25% variation in phenotype and will assist in developing of markers for root-length selection, which is an important trait for drought tolerance. Dhungana et al. (2021) identified QTLs associated with flooding stress at the V1-V2 stage of soybean. They analysed a recombinant inbred line (RIL) population derived from crossing a drought-susceptible (NTS116) and drought-tolerant (Danbaekkong) soybean cultivar. Using a composite interval mapping technique, they identified 10 QTLs related to flood tolerance at the V1-V2 stage of soybean. These QTLs can cause up to 30.7% phenotypic variations and can be useful for future soybean improvement programmes. Table 1 shows a list of major QTLs associated with soybean drought response. Further, other QTLs identified with soybean drought tolerance can be found at www.soybase.org, one of the prime repositories of soybean genetic resources.
Genetic Engineering
Genetic engineering approaches offer viable opportunities for accelerated crop improvement (Khan et al., 2020; Lohani et al., 2020; Arya et al., 2021). Genes controlling traits, such as flowering time, disease resistance and lipid profile, have been identified and used as targets for soybean improvement (Haun et al., 2014; Arya et al., 2018; Ngaki et al., 2021). Similarly, high-throughput genome and transcriptome sequencing have led to identification of key transcriptional regulators of soybean drought response. Among these, the DREB (Nguyen et al., 2019; Zhou et al., 2020), AREB (Fuganti-Pagliarini et al., 2017), NAC (Hussain et al., 2017; Yang et al., 2020), MYB (Chen L. et al., 2021) and WRKY (Ning et al., 2017; Shi et al., 2018; Wei et al., 2019) are the prime transcription factor families mediating abiotic stress responses. Gain of function and gene-knock-down approaches, such as RNAi and CRISPR/cas9, have yielded valuable information on how complex gene networks regulate dehydration stress physiology in soybean. For example, Yang et al. (2020) characterised GmNAC8 transcription factor as a positive regulator of soybean drought stress. NAC transcription factor family is primarily involved in plant growth and stress response. GmNAC8 was cloned under the control of 35s promoter for gene over-expression, and CRISPR/Cas9 was used to knock down GmNAC8. The performance of over-expression and knock-down lines was analysed under drought stress by withholding water supply for 14days. Interestingly, GmNAC8 over-expression lines had significantly high superoxide dismutase levels and proline content, which are both indicators of drought tolerance in plants. Further, as the water supply was restored, the recovery rate of over-expression lines was relatively high (up to 96%) compared to WT lines which showed only a 40% recovery rate. Interestingly, the GmNAC8 knock-down lines only had a 5 to 14% recovery rate.
MYB transcription factors regulate the biosynthesis of secondary metabolites for stress responses. Recently, Chen H. et al. (2021) characterised GmMYB14, which participates in drought tolerance and high-density soybean yield by affecting plant architecture through the Brassinosteroid pathway. GmMYB14 over-expression lines were compact with decreased plant height, internodal length, leaf surface area and petiole angle. However, the transgenic plant showed an increase in node number on the main stem and increased branch number, which contributed to enhanced yield under high-density cropping (20cm intervals) conditions. Further, under drought stress, pod number, seed number and seed weight per plant were significantly improved in soybeans over-expressing GmMYB14 compared to WT. Hence, as we face the challenge of producing more food from our limited arable land, plants that can withstand drier conditions and generate better yield under high-density cropping have the potential of ensuring future food security. Table 2 shows a list of genes or gene families recently identified to soybean drought response.
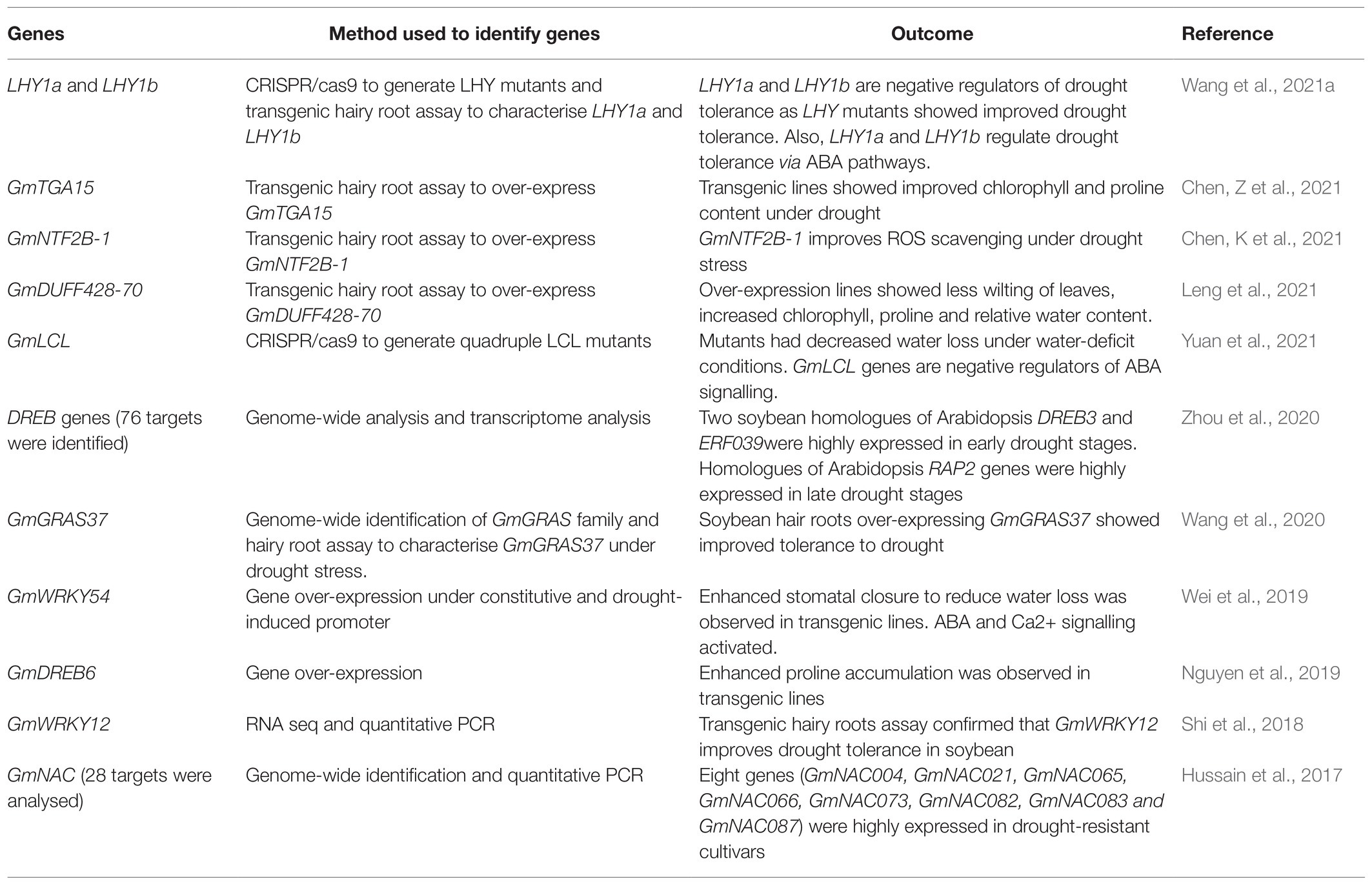
Table 2. A summary of recently characterised gene targets for engineering drought tolerance in soybean.
Dehydration responsive element binding (DREB) and AREB transcription factor family genes are known to mediate drought inducible gene expression (Fuganti-Pagliarini et al., 2017; Zhou et al., 2020). The transgenic soybean lines over-expressing transcription factors DREB1A, DREB2A and AREB1 have been field tested for the agronomic and physiological performance under-irrigated and non-irrigated conditions (Fuganti-Pagliarini et al., 2017). Improved water use efficiency and leaf area index were reported in 35S: AtAREB1FL lines compared to the control lines. Also, 35S: AtAREB1FL lines had the highest yield under non-irrigated conditions, similar to the yield of 35S: AtAREB1FL lines under-irrigated conditions. Interestingly, in non-irrigated conditions, the oil and protein contents of seeds were not affected by the insertion of DREB1A or DREB2A or AREB1 transcription factors.
Recently, soybeans expressing the sunflower (Helianthus annus) transcription factor, HaHB4, were approved for production by the US Department of Agriculture. HaHB4 is a water-deficit responsive sunflower transcription factor whose over-expression in Arabidopsis led to improved drought tolerance (Manavella et al., 2008). HaHB4 was cloned under constitutive 35S promoter and inducible HaHB4 promoter. Soybeans expressing HaHB4 were studied under glasshouse and field conditions by Ribichich et al. (2020). Transgenic lines designated as b10H performed best under field trials when genotype to environment interaction (G×E) was analysed. The b10H soybeans had better yield (seed number) under warm and dry conditions, not compensated by a decrease in seed weight. Under water-deficit conditions, b10H produced 43.4% more yield compared to WT (Williams 82). Also, the diameter of epicotyls, internode and xylem was wider in b10H soybeans as compared to WT plants. Further, b10H plants had a significantly high photosynthetic rate (at R5 and R6 stage) under warm field conditions. Molecular analysis showed that transcripts of heat shock proteins homologous to Arabidopsis thaliana HSC70-1(At5G02500), HSFB2A (At5G62020), Hsp81.4(At5G56000) and HOT5 (At5G43940) were differentially regulated in soybeans expressing HaHB4 (Ribichich et al., 2020). Due to its exceptional field performance, the trait HaHB4 has received regulatory approvals in Argentina, Brazil, Paraguay and Canada (Businesswire, 2021).
Microbial Inoculants for Drought-smart Soybean
Symbiotic rhizobium species associated with soybean root nodules benefit plant growth via mediating biological N fixation (Jaiswal et al., 2021). Reduced photosynthesis under drought conditions disturbs oxygen balance in nodules triggering premature nodule senescence (Arrese-Igor et al., 2011; Kunert et al., 2016). Symbiotic association of soybean plants with arbuscular mycorrhizal fungi, Glomus mosseae and G. intraradices, has been reported to alleviate drought-induced nodule senescence (Porcel and Ruiz-Lozano, 2004; Takács et al., 2018). Further, it has been demonstrated that extenuation of premature nodule senescence is mediated by the induction of high glutathione reductase in soybean roots and nodules. Glutathione reductase activity likely exerts its influence by reducing oxidative damage to biomolecules (Porcel and Ruiz-Lozano, 2004; Prabha and Sharadamma, 2019; Meena et al., 2021). Hence, arbuscular mycorrhizal fungi can play important roles in alleviating the impact of drought at root-nodule interfaces in soybeans. Recently, co-inoculation of rhizobia and mycorrhizal fungi has shown enhanced soybean tolerance to drought stress providing a cost-effective strategy for improving soybean productivity (Igiehon et al., 2021).
Conclusion
Soybean is a crop of immense economic importance (Johnson and Myers, 1995; Messina and Messina, 2010). Vast genetic diversity has been reported in soybean germplasm, and the increasing availability of soybean genetic resources has instigated the development of drought-smart soybeans (Carter et al., 2016; Ribichich et al., 2020; Kajiya-Kanegae et al., 2021; Chen L. et al., 2021). Modern breeding and advanced biotechnology methods have shown promising results, and market-ready drought-tolerant soybeans have been released in some parts of the world (Carter et al., 2016; Ribichich et al., 2020). However, soybean production is still dependent on adequate irrigation facilities in many regions, especially in under-developed and developing nations (Droppers et al., 2021; Suriadi et al., 2021). As genetic and non-genetic improvement methods are tested on more cultivars, the dependency of soybean production on rainfall or heavy irrigation should reduce. With changing precipitation patterns and a hotter climate, drought-tolerant soybeans will play a significant role in ensuring our future food security.
Author Contributions
All authors contributed to the article and approved the submitted version.
Conflict of Interest
The authors declare that the research was conducted in the absence of any commercial or financial relationships that could be construed as a potential conflict of interest.
Publisher’s Note
All claims expressed in this article are solely those of the authors and do not necessarily represent those of their affiliated organizations, or those of the publisher, the editors and the reviewers. Any product that may be evaluated in this article, or claim that may be made by its manufacturer, is not guaranteed or endorsed by the publisher.
Acknowledgments
We thank the reviewers for the suggestions to improve the manuscript. The research support from the ARC Discovery grant DP0988972 is gratefully acknowledged.
References
Abdel-Haleem, H., Carter, T. E., Purcell, L. C., King, C. A., Ries, L. L., Chen, P., et al. (2012). Mapping of quantitative trait loci for canopy-wilting trait in soybean (Glycine max L. Merr). Theor. Appl. Genet. 125, 837–846. doi: 10.1007/s00122-012-1876-9
Alia,, Mohanty, P., and Matysik, J. (2001). Effect of proline on the production of singlet oxygen. Amino Acids 21, 195–200. doi: 10.1007/s007260170026
Arrese-Igor, C., González, E., Marino, D., Ladrera, R., Larrainzar, E., and Gil-Quintana, E. (2011). Physiological response of legume nodules to drought. Plant. Stress. 5, 24–31.
Arya, H., Singh, M. B., and Bhalla, P. L. (2018). Genomic and molecular analysis of conserved and unique features of soybean PIF4. Sci. Rep. 8, 1–11. doi: 10.1038/s41598-018-30043-2
Arya, H., Singh, M. B., and Bhalla, P. L. (2021). Overexpression of PIF4 affects plant morphology and accelerates reproductive phase transitions in soybean. Food. Energy. Secur. 10:e291. doi: 10.1002/fes3.291
Ashraf, M., and Iram, A. (2005). Drought stress induced changes in some organic substances in nodules and other plant parts of two potential legumes differing in salt tolerance. Flora-Morphol. Distrib. Funct. Ecol. Plants. 200, 535–546. doi: 10.1016/j.flora.2005.06.005
Basu, S., Ramegowda, V., Kumar, A., and Pereira, A. (2016). Plant adaptation to drought stress. F1000Research 5:1554. doi: 10.12688/f1000research.7678.1
Bhardwaj, S. F. (1986). Consumptive use and water requirement of soybeans. J. Irrig. Drain. Eng. 112, 157–163. doi: 10.1061/(ASCE)0733-9437(1986)112:2(157)
Businesswire (2021). Bioceres Crop Solutions Receives Approval of HB4® Drought Tolerant Soybean in Canada [Online]. Available at: https://www.businesswire.com/news/home/20210601005303/en/Bioceres-Crop-Solutions-Receives-Approval-of-HB4%C2%AE-Drought-Tolerant-Soybean-in-Canada (Accessed 12, August 2021).
Carter, T. E., Todd, S. M., and Gillen, A. M. (2016). Registration of ‘USDA-N8002’ soybean cultivar with high yield and abiotic stress resistance traits. J. Plant. Regist. 10, 238–245. doi: 10.3198/jpr2015.09.0057crc
Charlson, D. V., Bhatnagar, S., King, C. A., Ray, J. D., Sneller, C. H., Carter, T. E., et al. (2009). Polygenic inheritance of canopy wilting in soybean [Glycine max (L.) Merr]. Theor. Appl. Genet. 119, 587–594. doi: 10.1007/s00122-009-1068-4
Chen, H., Kumawat, G., Yan, Y., Fan, B., and Xu, D. (2021). Mapping and validation of a major QTL for primary root length of soybean seedlings grown in hydroponic conditions. BMC Genomics 22, 1–9. doi: 10.1186/s12864-021-07445-0
Chen, K., Su, C., Tang, W., Zhou, Y., Xu, Z., Chen, J., et al. (2021). Nuclear transport factor GmNTF2B-1 enhances soybean drought tolerance by interacting with oxidoreductase GmOXR17 to reduce reactive oxygen species content. Plant. J. 107, 740–759. doi: 10.1111/tpj.15319
Chen, L., Yang, H., Fang, Y., Guo, W., Chen, H., Zhang, X., et al. (2021). Overexpression of GmMYB14 improves high-density yield and drought tolerance of soybean through regulating plant architecture mediated by the brassinosteroid pathway. Plant Biotechnol. J. 19:33098207, 702–716. doi: 10.1111/pbi.13496
Chen, Z., Fang, X., Yuan, X., Zhang, Y., Li, H., Zhou, Y., et al. (2021). Overexpression of transcription factor GmTGA15 enhances drought tolerance in transgenic soybean hairy roots and Arabidopsis plants. Agronomy 11:170. doi: 10.3390/agronomy11010170
Cohen, I., Zandalinas, S. I., Fritschi, F. B., Sengupta, S., Fichman, Y., Azad, R. K., et al. (2021). The impact of water deficit and heat stress combination on the molecular response, physiology, and seed production of soybean. Physiol. Plant. 172, 41–52. doi: 10.1111/ppl.13269
Collier, R., and Tegeder, M. (2012). Soybean ureide transporters play a critical role in nodule development, function and nitrogen export. Plant J. 72, 355–367. doi: 10.1111/j.1365-313X.2012.05086.x
Cornic, G., and Briantais, J.-M. (1991). Partitioning of photosynthetic electron flow between CO2 and O2 reduction in a C3 leaf (Phaseolus vulgaris L.) at different CO2 concentrations and during drought stress. Planta 183, 178–184. doi: 10.1007/BF00197786
Cotrim, M. F., Gava, R., Campos, C. N. S., De David, C. H. O., Reis, I. D. A., Teodoro, L. P. R., et al. (2021). Physiological performance of soybean genotypes grown under irrigated and rainfed conditions. J. Agron. Crop Sci. 207, 34–43. doi: 10.1111/jac.12448
Crusiol, L. G. T., Nanni, M. R., Furlanetto, R. H., Sibaldelli, R. N. R., Cezar, E., Sun, L., et al. (2021). Classification of soybean genotypes assessed Under different water availability and at different Phenological stages using leaf-based hyperspectral reflectance. Remote Sens. 13:172. doi: 10.3390/rs13020172
Dayoub, E., Lamichhane, J. R., Schoving, C., Debaeke, P., and Maury, P. (2021). Early-stage phenotyping of root traits provides insights into the drought tolerance level of soybean cultivars. Agronomy 11:188. doi: 10.3390/agronomy11010188
Desclaux, D., Huynh, T. T., and Roumet, P. (2000). Identification of soybean plant characteristics that indicate the timing of drought stress. Crop Sci. 40, 716–722. doi: 10.2135/cropsci2000.403716x
Dhungana, S. K., Kim, H.-S., Kang, B.-K., Seo, J.-H., Kim, H.-T., Shin, S.-O., et al. (2021). Identification of QTL for tolerance to flooding stress at seedling stage of soybean (Glycine max L. Merr.). Agronomy 11:908. doi: 10.3390/agronomy11050908
Dong, S., Jiang, Y., Dong, Y., Wang, L., Wang, W., Ma, Z., et al. (2019). A study on soybean responses to drought stress and rehydration. Saudi Journal of Biological Sciences. 26, 2006-2017. doi: 10.1016/j.sjbs.2019.08.005
Droppers, B., Supit, I., Van Vliet, M. T. H., and Ludwig, F. (2021). Worldwide water constraints on attainable irrigated production for major crops. Environ. Res. Lett. 16:055016. doi: 10.1088/1748-9326/abf527
Du, W., Wang, M., Fu, S., and Yu, D. (2009). Mapping QTLs for seed yield and drought susceptibility index in soybean (Glycine max L.) across different environments. J. Genet. Genomics 36, 721–731. doi: 10.1016/S1673-8527(08)60165-4
Du, Y., Zhao, Q., Chen, L., Yao, X., and Xie, F. (2020). Effect of drought stress at reproductive stages on growth and nitrogen metabolism in soybean. Agronomy 10:302. doi: 10.3390/agronomy10020302
Dumschott, K., Dechorgnat, J., and Merchant, A. (2019). Water deficit elicits a transcriptional response of genes governing d-pinitol biosynthesis in soybean (Glycine max). Int. J. Mol. Sci. 20:2411. doi: 10.3390/ijms20102411
Fao, (2021). Land & Water [Online]. Available at: http://www.fao.org/land-water/databases-and-software/crop-information/soybean/en/ (Accessed 12, August 2021).
Faostat (2019). Crops and livestock products [Online]. Available at: http://www.fao.org/faostat/en/#data/QCL (Accessed 12, August 2021).
Ford, C. W. (1984). Accumulation of low molecular weight solutes in water-stressed tropical legumes. Phytochemistry 23, 1007–1015. doi: 10.1016/S0031-9422(00)82601-1
Foyer, C. H., Lam, H.-M., Nguyen, H. T., Siddique, K. H., Varshney, R. K., Colmer, T. D., et al. (2016). Neglecting legumes has compromised human health and sustainable food production. Nature. plants. 2, 1–10. doi: 10.1038/nplants.2016.112
Frederick, J. R., Camp, C. R., and Bauer, P. J. (2001). Drought-stress effects on branch and mainstem seed yield and yield components of determinate soybean. Crop Sci. 41, 759–763. doi: 10.2135/cropsci2001.413759x
Fuganti-Pagliarini, R., Ferreira, L. C., Rodrigues, F. A., Molinari, H. B. C., Marin, S. R. R., Molinari, M. D. C., et al. (2017). Characterization of soybean genetically modified for drought tolerance in field conditions. Front. Plant Sci. 8:448. doi: 10.3389/fpls.2017.00448
Golicz, A. A., Singh, M. B., and Bhalla, P. L. (2018). The long intergenic noncoding RNA (LincRNA) landscape of the soybean genome. Plant Physiol. 176, 2133–2147. doi: 10.1104/pp.17.01657
Gupta, A. K., and Kaur, N. (2005). Sugar signalling and gene expression in relation to carbohydrate metabolism under abiotic stresses in plants. J. Biosci. 30, 761–776. doi: 10.1007/BF02703574
Ha, C., Watanabe, Y., Tran, U., Le, D., Tanaka, M., Nguyen, K., et al. (2015). Comparative analysis of root transcriptomes from two contrasting drought-responsive Williams 82 and DT2008 soybean cultivars under normal and dehydration conditions. Front. Plant Sci. 6:551. doi: 10.3389/fpls.2015.00551
Haun, W., Coffman, A., Clasen, B. M., Demorest, Z. L., Lowy, A., Ray, E., et al. (2014). Improved soybean oil quality by targeted mutagenesis of the fatty acid desaturase 2 gene family. Plant Biotechnol. J. 12, 934–940. doi: 10.1111/pbi.12201
Hussain, R. M., Ali, M., Feng, X., and Li, X. (2017). The essence of NAC gene family to the cultivation of drought-resistant soybean (Glycine max L. Merr.) cultivars. BMC. Plant. biol. 17, 1–11. doi: 10.1186/s12870-017-1001-y
Igiehon, N. O., Babalola, O. O., Cheseto, X., and Torto, B. (2021). Effects of rhizobia and arbuscular mycorrhizal fungi on yield, size distribution and fatty acid of soybean seeds grown under drought stress. Microbiol. Res. 242:126640. doi: 10.1016/j.micres.2020.126640
Jaiswal, S. K., Mohammed, M., Ibny, F. Y. I., and Dakora, F. D. (2021). Rhizobia as a source of plant growth-promoting molecules: potential applications and possible operational mechanisms. Front. Sustainable. Food. Sys. 4:311. doi: 10.3389/fsufs.2020.619676
Jin, Z., Zhuang, Q., Wang, J., Archontoulis, S. V., Zobel, Z., and Kotamarthi, V. R. (2017). The combined and separate impacts of climate extremes on the current and future US rainfed maize and soybean production under elevated CO2. Glob. Chang. Biol. 23, 2687–2704. doi: 10.1111/gcb.13617
Johnson, L. A., and Myers, D. J. (1995). “Chapter 21 - Industrial Uses for Soybeans,” in Practical Handbook of Soybean Processing and Utilization. ed. D. R. Erickson (AOCS press), 380–427.
Kajiya-Kanegae, H., Nagasaki, H., Kaga, A., Hirano, K., Ogiso-Tanaka, E., Matsuoka, M., et al. (2021). Whole-genome sequence diversity and association analysis of 198 soybean accessions in mini-core collections. DNA Res. 28:dsaa032. doi: 10.1093/dnares/dsaa032
Khan, M. I. R., Palakolanu, S. R., Chopra, P., Rajurkar, A. B., Gupta, R., Iqbal, N., et al. (2020). Improving drought tolerance in rice: ensuring food security through multi-dimensional approaches. Physiol. Plant. 172, 645–668. doi: 10.1111/ppl.13223
Kido, E. A., Ferreira Neto, J. R. C., Silva, R. L. O., Belarmino, L. C., Bezerra Neto, J. P., Soares-Cavalcanti, N. M., et al. (2013). Expression dynamics and genome distribution of osmoprotectants in soybean: identifying important components to face abiotic stress. BMC. Bioinf. 14, 1–11. doi: 10.1186/1471-2105-14-S1-S7
Kim, T.-H. (2014). Mechanism of ABA signal transduction: agricultural highlights for improving drought tolerance. J. Plant. Biol. 57, 1–8. doi: 10.1007/s12374-014-0901-8
Heerden Van, P. D. R., and Krüger, H. J. G. (2002). Separately and simultaneously induced dark chilling and drought stress effects on photosynthesis, proline accumulation and antioxidant metabolism in soybean. J. Plant Physiol. 159, 1077–1086. doi: 10.1078/0176-1617-00745
Kunert, K., and Vorster, B. J. (2020). In search for drought-tolerant soybean: is the slow-wilting phenotype more than just a curiosity? J. Exp. Bot. 71, 457–460. doi: 10.1093/jxb/erz235
Kunert, K. J., Vorster, B. J., Fenta, B. A., Kibido, T., Dionisio, G., and Foyer, C. H. (2016). Drought stress responses in soybean roots and nodules. Front. Plant Sci. 7:1015. doi: 10.3389/fpls.2016.01015
Leng, Z.-X., Liu, Y., Chen, Z.-Y., Guo, J., Chen, J., Zhou, Y.-B., et al. (2021). Genome-wide analysis of the DUF4228 family in soybean and functional identification of GmDUF4228-70 in response to drought and salt stresses. Front. Plant Sci. 12:628299. doi: 10.3389/fpls.2021.628299
Li, L., Zhang, Q., and Huang, D. (2014). A review of imaging techniques for plant phenotyping. Sensors 14, 20078–20111. doi: 10.3390/s141120078
Liu, F., Andersen, M. N., Jacobsen, S.-E., and Jensen, C. R. (2005). Stomatal control and water use efficiency of soybean (Glycine max L. Merr) during progressive soil drying. Environ. Exp. Bot. 54, 33–40. doi: 10.1016/j.envexpbot.2004.05.002
Liu, F., Jensen, C. R., and Andersen, M. N. (2003). Hydraulic and chemical signals in the control of leaf expansion and stomatal conductance in soybean exposed to drought stress. Funct. Plant Biol. 30, 65–73. doi: 10.1071/FP02170
Lohani, N., Jain, D., Singh, M. B., and Bhalla, P. L. (2020). Engineering multiple abiotic stress tolerance in canola Brassica napus. Front. Plant. Sci. 11:3. doi: 10.3389/fpls.2020.00003
Mak, M., Babla, M., Xu, S.-C., O’carrigan, A., Liu, X.-H., Gong, Y.-M., et al. (2014). Leaf mesophyll K+, H+ and Ca2+ fluxes are involved in drought-induced decrease in photosynthesis and stomatal closure in soybean. Environ. Exp. Bot. 98, 1–12. doi: 10.1016/j.envexpbot.2013.10.003
Malcheska, F., Ahmad, A., Batool, S., Müller, H. M., Ludwig-Müller, J., Kreuzwieser, J., et al. (2017). Drought-enhanced xylem sap Sulfate closes stomata by affecting ALMT12 and guard cell ABA synthesis. Plant Physiol. 174, 798–814. doi: 10.1104/pp.16.01784
Maleki, A., Naderi, A., Naseri, R., Fathi, A., Bahamin, S., and Maleki, R. (2013). Physiological performance of soybean cultivars under drought stress. Bull. Env. Pharmacol. Life Sci. 2, 38–44.
Manavella, P. A., Dezar, C. A., Ariel, F. D., Drincovich, M. F., and Chan, R. L. (2008). The sunflower HD-zip transcription factor HAHB4 is up-regulated in darkness, reducing the transcription of photosynthesis-related genes. J. Exp. Bot. 59, 3143–3155. doi: 10.1093/jxb/ern170
Meena, S. K., Pandey, R., Sharma, S., Gayacharan, V. K., Dikshit, H. K., Siddique, K. H. M., et al. (2021). Cross tolerance to phosphorus deficiency and drought stress in mungbean is regulated by improved antioxidant capacity, biological N2-fixation, and differential transcript accumulation. Plant Soil, 466, 337–356. doi: 10.1007/s11104-021-05062-0
Mengarelli, D. A., and Zanor, M. I. (2021). Genome-wide characterization and analysis of the CCT motif family genes in soybean (Glycine max). Planta 253, 1–17. doi: 10.1007/s00425-020-03537-5
Messina, M., and Messina, V. (2010). The role of soy in vegetarian diets. Nutrients 2, 855–888. doi: 10.3390/nu2080855
Mian, R., Bailey, M., Ashley, D., Wells, R., Carter, T., Parrott, W., et al. (1996). Molecular markers associated with water use efficiency and leaf ash in soybean. Crop. Sci., 36, 1252–1257. doi: 10.2135/cropsci1996.0011183X003600050030x
Mutava, R. N., Prince, S. J. K., Syed, N. H., Song, L., Valliyodan, B., Chen, W., et al. (2015). Understanding abiotic stress tolerance mechanisms in soybean: a comparative evaluation of soybean response to drought and flooding stress. Plant Physiol. Biochem. 86, 109–120. doi: 10.1016/j.plaphy.2014.11.010
Ngaki, M. N., Sahoo, D. K., Wang, B., and Bhattacharyya, M. K. (2021). Overexpression of a plasma membrane protein generated broad-spectrum immunity in soybean. Plant Biotechnol. J. 19, 502–516. doi: 10.1111/pbi.13479
Nguyen, Q. H., Vu, L. T. K., Nguyen, L. T. N., Pham, N. T. T., Nguyen, Y. T. H., Van Le, S., et al. (2019). Overexpression of the GmDREB6 gene enhances proline accumulation and salt tolerance in genetically modified soybean plants. Sci. Rep. 9, 1–8. doi: 10.1038/s41598-019-55895-0
Ning, W., Zhai, H., Yu, J., Liang, S., Yang, X., Xing, X., et al. (2017). Overexpression of Glycine soja WRKY20 enhances drought tolerance and improves plant yields under drought stress in transgenic soybean. Mol. Breed. 37:19. doi: 10.1007/s11032-016-0614-4
Ohashi, Y., Nakayama, N., Saneoka, H., and Fujita, K. (2006). Effects of drought stress on photosynthetic gas exchange, chlorophyll fluorescence and stem diameter of soybean plants. Biol. Plant. 50, 138–141. doi: 10.1007/s10535-005-0089-3
Oya, T., Nepomuceno, A. L., Neumaier, N., Farias, J. R. B., Tobita, S., and Ito, O. (2004). Drought tolerance characteristics of Brazilian soybean cultivars—evaluation and characterization of drought tolerance of various Brazilian soybean cultivars in the field. Plant. Prod. Sci. 7, 129–137. doi: 10.1626/pps.7.129
Porcel, R., and Ruiz-Lozano, J. M. (2004). Arbuscular mycorrhizal influence on leaf water potential, solute accumulation, and oxidative stress in soybean plants subjected to drought stress. J. Exp. Bot. 55, 1743–1750. doi: 10.1093/jxb/erh188
Prabha, C., and Sharadamma, N. (2019). Arbuscular mycorrhizal influence on oxidative stress in French bean (Phaseolus vulgaris) under drought conditions. GSC. biol. Pharm. Sci. 7, 021–029. doi: 10.30574/gscbps.2019.7.3.0092
Raghuvanshi, R. S., and Bisht, K. (2010). “Chapter 18- Uses of Soybean: Products and Preparation,” in The soybean: botany, production and uses. ed. G. Singh (CAB International), 404–426.
Raza, G., Singh, M. B., and Bhalla, P. L. (2017). In vitro plant regeneration from commercial cultivars of soybean. Biomed. Res. Int. 2017:7379693. doi: 10.1155/2017/7379693
Raza, G., Singh, M. B., and Bhalla, P. L. (2019). Somatic embryogenesis and plant regeneration from commercial soybean cultivars. Plants 9:38. doi: 10.3390/plants9010038
Rezaei, K., Wang, T., and Johnson, L. A. (2002). Hydrogenated vegetable oils as candle wax. J. Am. Oil Chem. Soc. 79, 1241–1247. doi: 10.1007/s11746-002-0634-z
Ribichich, K. F., Chiozza, M., Ávalos-Britez, S., Cabello, J. V., Arce, A. L., Watson, G., et al. (2020). Successful field performance in warm and dry environments of soybean expressing the sunflower transcription factor HB4. J. Exp. Bot. 71, 3142–3156. doi: 10.1093/jxb/eraa064
Seo, J. S., Sohn, H.-B., Noh, K., Jung, C., An, J., Donovan, C., et al. (2012). Expression of the Arabidopsis AtMYB44 gene confers drought/salt-stress tolerance in transgenic soybean. Mol. Breed. 29, 601–608. doi: 10.1007/s11032-011-9576-8
Shi, W.-Y., Du, Y.-T., Ma, J., Min, D.-H., Jin, L.-G., Chen, J., et al. (2018). The WRKY transcription factor GmWRKY12 confers drought and salt tolerance in soybean. Int. J. Mol. Sci. 19:4087. doi: 10.3390/ijms19124087
Signorelli, S., Arellano, J. B., Melø, T. B., Borsani, O., and Monza, J. (2013). Proline does not quench singlet oxygen: evidence to reconsider its protective role in plants. Plant Physiol. Biochem. 64, 80–83. doi: 10.1016/j.plaphy.2012.12.017
Silvente, S., Sobolev, A. P., and Lara, M. (2012). Metabolite adjustments in drought tolerant and sensitive soybean genotypes in response to water stress. PLoS One 7:e38554. doi: 10.1371/journal.pone.0038554
Streeter, J., Lohnes, D., and Fioritto, R. (2001). Patterns of pinitol accumulation in soybean plants and relationships to drought tolerance. Plant Cell Environ. 24, 429–438. doi: 10.1046/j.1365-3040.2001.00690.x
Suriadi, A., Zulhaedar, F., Nazam, M., and Hipi, A. (2021). “Optimal Irrigation at Various Soil Types for Soybean Production”, in: IOP Conference Series: Earth and Environmental Science: IOP Publishing), 012081.
Takács, T., Cseresnyés, I., Kovács, R., Parádi, I., Kelemen, B., Szili-Kovács, T., et al. (2018). Symbiotic effectivity of dual and tripartite associations on soybean (Glycine max L. Merr) cultivars inoculated With Bradyrhizobium japonicum and AM fungi. Front. plant. sci. 9:1613. doi: 10.3389/fpls.2018.01631
Tang, C.-H. (2017). Emulsifying properties of soy proteins: a critical review with emphasis on the role of conformational flexibility. Crit. Rev. Food Sci. Nutr. 57, 2636–2679. doi: 10.1080/10408398.2015.1067594
Wang, K., Bu, T., Cheng, Q., Dong, L., Su, T., Chen, Z., et al. (2021a). Two homologous LHY pairs negatively control soybean drought tolerance by repressing the abscisic acid responses. New Phytol. 229, 2660–2675. doi: 10.1111/nph.17019
Wang, M., Chen, B., Zhou, W., Xie, L., Wang, L., Zhang, Y., et al. (2021b). Genome-wide identification and expression analysis of the AT-hook motif nuclear localized gene family in soybean. BMC Genomics 22, 1–26. doi: 10.1186/s12864-021-07687-y
Wang, H., Yang, L., Li, Y., Hou, J., Huang, J., and Liang, W. (2016). Involvement of ABA-and H2O2-dependent cytosolic glucose-6-phosphate dehydrogenase in maintaining redox homeostasis in soybean roots under drought stress. Plant Physiol. Biochem. 107, 126–136. doi: 10.1016/j.plaphy.2016.05.040
Wang, T.-T., Yu, T.-F., Fu, J.-D., Su, H.-G., Chen, J., Zhou, Y.-B., et al. (2020). Genome-wide analysis of the GRAS gene family and functional identification of GmGRAS37 in drought and salt tolerance. Front. Plant Sci. 11:2024. doi: 10.3389/fpls.2020.604690
Wei, Y., Jin, J., Jiang, S., Ning, S., and Liu, L. (2018). Quantitative response of soybean development and yield to drought stress during different growth stages in the Huaibei plain, China. Agronomy 8:97. doi: 10.3390/agronomy8070097
Wei, W., Liang, D. W., Bian, X. H., Shen, M., Xiao, J. H., Zhang, W. K., et al. (2019). GmWRKY54 improves drought tolerance through activating genes in abscisic acid and Ca2+ signaling pathways in transgenic soybean. Plant J. 100, 384–398. doi: 10.1111/tpj.14449
Wilkinson, S., and Davies, W. J. (2002). ABA-based chemical signalling: the co-ordination of responses to stress in plants. Plant Cell Environ. 25, 195–210. doi: 10.1046/j.0016-8025.2001.00824.x
Williams, B., Kantartzi, S., Meksem, K., Grier, R., Barakat, A., Lightfoot, D., et al. (2012). Genetic analysis of root and shoot traits in the ‘Essex’ By ‘Forrest’ recombinant inbred line (RIL) population of soybean [Glycine max (L.) Merr.]. J. Plant. Genome Sci. 1, 1–9. doi: 10.5147/jpgs.2012.0051
Wong, C. E., Singh, M. B., and Bhalla, P. L. (2013). The dynamics of soybean leaf and shoot apical meristem transcriptome undergoing floral initiation process. PLoS One 8:e65319. doi: 10.1371/journal.pone.0065319
Yancey, P. H. (2005). Organic osmolytes as compatible, metabolic and counteracting cytoprotectants in high osmolarity and other stresses. J. Exp. Biol. 208, 2819–2830. doi: 10.1242/jeb.01730
Yang, C., Huang, Y., Lv, W., Zhang, Y., Bhat, J. A., Kong, J., et al. (2020). GmNAC8 acts as a positive regulator in soybean drought stress. Plant Sci. 293:110442. doi: 10.1016/j.plantsci.2020.110442
Yang, W., Wu, T., Zhang, X., Song, W., Xu, C., Sun, S., et al. (2019). Critical photoperiod measurement of soybean genotypes in different maturity groups. Crop Sci. 59, 2055–2061. doi: 10.2135/cropsci2019.03.0170
Ye, H., Song, L., Schapaugh, W. T., Ali, M. L., Sinclair, T. R., Riar, M. K., et al. (2019). The importance of slow canopy wilting in drought tolerance in soybean. J. Exp. Bot. 71, 642–652. doi: 10.1093/jxb/erz150
Yuan, Z., Cao, Q., Zhang, K., Ata-Ul-Karim, S. T., Tian, Y., Zhu, Y., et al. (2016). Optimal leaf positions for SPAD meter measurement in Rice. Front. Plant Sci. 7:719. doi: 10.3389/fpls.2016.00719
Yuan, L., Xie, G. Z., Zhang, S., Li, B., Wang, X., Li, Y., et al. (2021). GmLCLs negatively regulate ABA perception and signalling genes in soybean leaf dehydration response. Plant Cell Environ. 44, 412–424. doi: 10.1111/pce.13931
Zhang, Z., Ali, S., Zhang, T., Wang, W., and Xie, L. (2020). Identification, evolutionary and expression analysis of PYL-PP2C-SnRK2s gene families in soybean. Plan. Theory 9:1356. doi: 10.3390/plants9101356
Zhang, L. X., Kyei-Boahen, S., Zhang, J., Zhang, M. H., Freeland, T. B., Watson, C. E., et al. (2007). Modifications of optimum adaptation zones for soybean maturity groups in the USA. Crop. Manage. 6, 1–11. doi: 10.1094/CM-2007-0927-01-RS
Zhang, Y. H., Liu, M. F., He, J. B., Wang, Y. F., Xing, G. N., Li, Y., et al. (2015). Marker-assisted breeding for transgressive seed protein content in soybean [Glycine max (L.) Merr.]. Theor. Appl. Genet. 128, 1061–1072. doi: 10.1007/s00122-015-2490-4
Zhang, S., Singh, M. B., and Bhalla, P. L. (2021). Molecular characterization of a soybean FT homologue, GmFT7. Sci. Rep. 11, 1–11. doi: 10.1038/s41598-021-83305-x
Zhao, B., Wu, H., Xu, W., Zhang, W., Chen, X., Zhu, Y., et al. (2021). Genome-wide identification, characterization, and expression analyses of P-type ATPase superfamily genes in soybean. Agronomy 11:71. doi: 10.3390/agronomy11010071
Zhou, Y., Zhou, W., Liu, H., Liu, P., and Li, Z. (2020). Genome-wide analysis of the soybean DREB gene family: identification, genomic organization and expression profiles in response to drought stress. Plant Breed. 139, 1158–1167. doi: 10.1111/pbr.12867
Keywords: soybean, legume, water stress, abiotic stress, drought, drought-tolerant soybean
Citation: Arya H, Singh MB and Bhalla PL (2021) Towards Developing Drought-smart Soybeans. Front. Plant Sci. 12:750664. doi: 10.3389/fpls.2021.750664
Edited by:
Santiago Signorelli, Universidad de la República, UruguayReviewed by:
Maria Martha Sainz, Universidad de la República, UruguayHui Xia, Shanghai Agrobiological Gene Center, China
Copyright © 2021 Arya, Singh and Bhalla. This is an open-access article distributed under the terms of the Creative Commons Attribution License (CC BY). The use, distribution or reproduction in other forums is permitted, provided the original author(s) and the copyright owner(s) are credited and that the original publication in this journal is cited, in accordance with accepted academic practice. No use, distribution or reproduction is permitted which does not comply with these terms.
*Correspondence: Prem L. Bhalla, cHJlbWxiQHVuaW1lbGIuZWR1LmF1