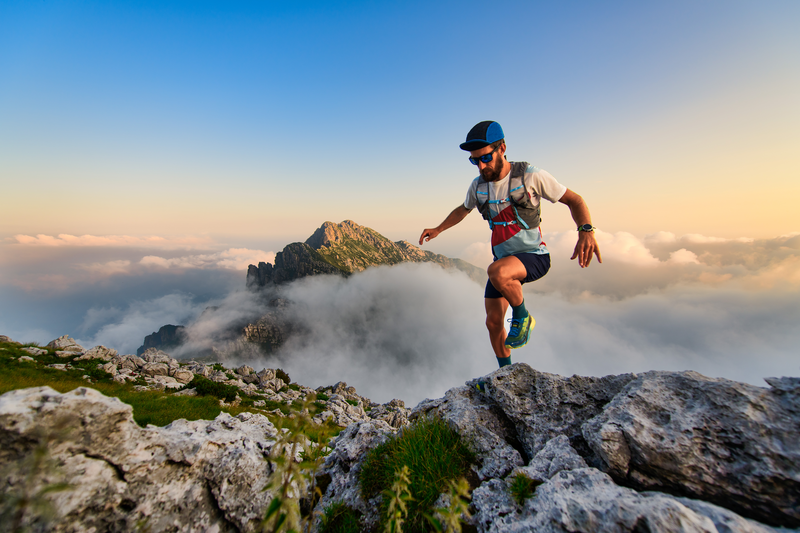
94% of researchers rate our articles as excellent or good
Learn more about the work of our research integrity team to safeguard the quality of each article we publish.
Find out more
ORIGINAL RESEARCH article
Front. Plant Sci. , 09 September 2021
Sec. Plant Pathogen Interactions
Volume 12 - 2021 | https://doi.org/10.3389/fpls.2021.749186
NAC transcriptional factors constitute a large family in rice and some of them have been demonstrated to play crucial roles in rice immunity. The present study investigated the function and mechanism of ONAC066 in rice immunity. ONAC066 shows transcription activator activity that depends on its C-terminal region in rice cells. ONAC066-OE plants exhibited enhanced resistance while ONAC066-Ri and onac066-1 plants showed attenuated resistance to Magnaporthe oryzae. A total of 81 genes were found to be up-regulated in ONAC066-OE plants, and 26 of them were predicted to be induced by M. oryzae. Four OsWRKY genes, including OsWRKY45 and OsWRKY62, were up-regulated in ONAC066-OE plants but down-regulated in ONAC066-Ri plants. ONAC066 bound to NAC core-binding site in OsWRKY62 promoter and activated OsWRKY62 expression, indicating that OsWRKY62 is a ONAC066 target. A set of cytochrome P450 genes were found to be co-expressed with ONAC066 and 5 of them were up-regulated in ONAC066-OE plants but down-regulated in ONAC066-Ri plants. ONAC066 bound to promoters of cytochrome P450 genes LOC_Os02g30110, LOC_Os06g37300, and LOC_Os02g36150 and activated their transcription, indicating that these three cytochrome P450 genes are ONAC066 targets. These results suggest that ONAC066, as a transcription activator, positively contributes to rice immunity through modulating the expression of OsWRKY62 and a set of cytochrome P450 genes to activate defense response.
During their lifespan, plants are always attacked by numerous potential pathogenic microbes including fungi, bacteria and viruses. To survive, plants have evolved to possess a sophisticated innate immune system, which includes two layered immune responses, known as pathogen/microbe/damage-associated molecular pattern (PAMP/MAMP/DAMP)-triggered immunity (PTI) and effector-triggered immunity (ETI) (Jones and Dangl, 2006; Boller and He, 2009; Dangl et al., 2013; Zhang et al., 2020). Upon perception and recognition of pathogen-derived signals, plants often effectively initiate a complicated signaling network (Peng et al., 2018; Zhou and Zhang, 2020), leading to a large-scale transcriptional reprogramming of gene expression in timely and coordinately manners (Tsuda and Somssich, 2015; Li et al., 2016; Birkenbihl et al., 2017; Chen et al., 2020). Transcriptional reprogramming in activation of immune response requires concerted and fine-tuned temporal and spatial functions of numerous transcription factors (TFs) belonging to different families (Buscaill and Rivas, 2014; Birkenbihl et al., 2017; Ng et al., 2018). During the last two decades, extensive genetic and biochemical studies have revealed the importance of TFs belonging to families of WRKY, AP2/ERF, NAC (NAM, ATAF1/2, and CUC2), MYB, bZIP, homeodomain, bHLH, NF-Y, and CAMTA in plant immunity (Nuruzzaman et al., 2013; Buscaill and Rivas, 2014; Dey and Vlot, 2015; Huang et al., 2016; Noman et al., 2017; Ng et al., 2018; Yuan et al., 2019a; Bian et al., 2020; Wani et al., 2021).
NAC family is plant-specific and represents one of the largest plant TF families. NAC TFs are characterized by the presence of highly conserved NAC domains at N-terminal, which determine DNA binding activity, and of variable domains at C-terminal, which are responsible for transcription activity (Olsen et al., 2005). Most NAC TFs exhibit binding activities to NAC recognition sequence (NACRS) cis-element with the sequence of CATGT or CACG (Tran et al., 2004), which are frequently present in promoters of certain defense genes. Functional studies using knockout/knockdown mutants and/or overexpression transgenic lines have demonstrated that NAC TFs play significant roles in plant growth, development, and response to biotic and abiotic stress (Puranik et al., 2012; Shao et al., 2015; Kim et al., 2016; Yuan et al., 2019a; Diao et al., 2020; Forlani et al., 2021; Kou et al., 2021).
Genome-wide transcriptome analysis revealed that a set of TF genes including NAC genes is activated by infection of Magnaporthe oryzae, the causal agent of blast disease (Kawahara et al., 2012; Sun et al., 2015). A total of 151 members in rice NAC TF family have been identified (Ooka et al., 2003; Fang et al., 2008; Nuruzzaman et al., 2010) and some of the rice NAC TFs have been shown to be involved in rice immunity, acting as either positive or negative regulators. OsNAC6, belonging to ATAF subfamily (Yuan et al., 2019a), positively regulates resistance to M. grisea and two genes for a cationic peroxidase (LOC_Os01g73200) and a protein containing a conserved DUF26 domain (LOC_Os04g25060) were identified as putative target genes of OsNAC6 (Nakashima et al., 2007). OsNAC111-overexpressing plants showed increased resistance to M. oryzae and constitutively expressed several defense genes, suggesting that OsNAC111, a member of TERN subfamily (Yuan et al., 2019a), positively regulates the promoter activity of a specific set of defense genes including PR2 and PR8 and contributes to rice immunity (Yokotani et al., 2014). Mutation in OsNAC60, whose transcript abundance is regulated by miR164a, and silencing of ONAC122 or ONAC131, coding for NAC TFs belonging to NAP subfamily (Yuan et al., 2019a), increased susceptibility to M. oryzae (Sun et al., 2013; Wang et al., 2018), while overexpression of OsNAC58 increased resistance to Xanthomonas oryzae pv. oryzae, the causal agent of bacterial blight disease (Park et al., 2017). ONAC066, which was induced by the blast fungus, positively regulates resistance to M. oryzae and X. oryzae pv. oryzae through modulating of abscisic acid (ABA) signaling pathway (Liu et al., 2018). RIM1, belonging to the NTL subfamily (Yuan et al., 2019a), negatively regulates rice immunity to Rice dwarf virus by acting as a host factor that is required for multiplication of the virus in host plants and functions as a molecular link in jasmonic acid signaling (Yoshii et al., 2009, 2010). OsNAC4 was found to be up-regulated during non-host defense response and regulate the occurrence of hypersensitive cell death, accompanied by loss of plasma membrane integrity, nuclear DNA fragmentation and typical morphological changes (Kaneda et al., 2009). Therefore, the NAC TFs play critical roles in different aspects of rice immunity; however, the molecular mechanism for these NAC TFs in rice immunity remains largely unknown.
We previously identified ONAC066 (LOC_Os03g56580) as one of the M. oryzae-responsive NAC TF genes (Sun et al., 2015). ONAC066 is classified into the ONAC022 subgroup of group I in NAC TF family (Ooka et al., 2003) and its Arabidopsis homologous ANAC042/AtJUB1 has been shown to be involved in pathogen-induced defense response (Wu et al., 2012; Shahnejat-Bushehri et al., 2016). ONAC066 has been shown to function as a positive regulator of drought and oxidative stress tolerance as well as disease resistance against M. oryzae and X. oryzae pv. oryzae (Liu et al., 2018; Yuan et al., 2019b). The present study aimed to elucidate the function of ONAC066 in rice immunity and to identify the putative targets that are regulated by ONAC066. Our data demonstrated that ONAC066 acts as a positive regulator of rice immunity against M. oryzae through modulating expression of OsWRKY62, a WRKY TF that is involved in rice immunity (Fukushima et al., 2016; Liu et al., 2016), and three cytochrome P450 genes.
ONAC066-OE and ONAC066-RNAi transgenic lines in background of rice (Oryza sativa L.) subsp. japonica cv. Zhonghua 11 (ZH11) were identified as previously described (Yuan et al., 2019b). Seeds of T-DNA insertion mutant onac066-1 (1C-13513) in japonica cv. Hwayoung (HY) background were obtained from POSTECH RISD (Rice T-DNA Insertion Sequence Database) (Jeon et al., 2000; An et al., 2003). Homozygous mutants for T-DNA insertion were identified by genomic DNA PCR with gene-specific and T-DNA-specific (2707RB) primers (Supplementary Table S1). Rice plants were grown in a growth room with a cycle of 14 h of light (28°C) and 10 h of darkness (24°C), as previously described (Hong et al., 2017).
Magnaprothe oryzae isolate RB22 was grown on complete medium [10 g glucose, 2 g peptone, 1 g yeast extract, 1 g casamino acids, 0.l% (vol/vol) 1000× trace elements (2.2 g ZnSO4∙7H2O, 1.1 g H3BO3, 0.5 g MnCl2∙4H2O, 0.5 g FeSO4∙7H2O, 0.16 g CoCl2∙6H2O, 0.15 g NaMoO4∙5H2O, 5 g NaEDTA, 100 mL ddH2O), 0.l% (v/v) 1000× vitamin supplement (10 mg biotin, 10 mg pyridoxin, 10 mg thiamine, 10 mg riboflavin, 10 mg p-aminobenzonic acid, 10 mg nicotinic acid, 100 mL ddH2O), 6 g NaNO3, 0.5 g KCl, 0.5 g MgSO4, 1.5 g KH2PO4, pH6.5, 1L] (Talbot et al., 1993) at 25°C for 10 days and spores were collected to prepare spore suspension inoculum (5 × 105 spores/ml containing 0.02% Tween-20). For whole plant inoculation assays, 4-week-old plants were inoculated by foliar spraying with spore suspension inoculum, as described previously (Hong et al., 2016). For detached leaf inoculation assays, fully expanded leaves from 4-week-old plants were inoculated by dropping 5 ml spore suspension inoculum on leaf surface. The inoculated plants and leaves were kept in dark for 24 h at 25°C with 100% relative humidity and then moved to the growth room with normal growth condition. Disease phenotypes were evaluated and samples were collected at 5 days post inoculation (dpi). Fungal growth in inoculated leaves was quantified by measuring M. oryzae genomic DNA relative to rice genomic DNA by qPCR (Zellerhoff et al., 2006). Total genomic DNA was extracted from leaves in extraction buffer containing 2% (wt/vol) CTAB, 20 mM EDTA, 1.4 M NaCl, and 100 mM Tris-HCl (pH8.0), and purified with chloroform/isoamyl alcohol (24:1, vol/vol), followed by isopropyl alcohol precipitation. Genomic DNA of M. oryzae and rice was determined using specific primers for M. oryzae MoPot2 DNA and rice OsUbi DNA, respectively, by qPCR, as previously described (Li et al., 2017). The primers used were listed in Supplementary Table S1. Relative fungal growth was presented as ratios obtained by comparison of the fungal MoPot2 genomic level with rice OsUbi genomic DNA level.
Total RNA was extracted using RNA Isolater reagent (Vazyme, Nanjing, China) and treated with genome DNA wiper mix (Vazyme, Nanjing, China) at 42°C for 2 min to remove the remaining genomic DNA. First-strand cDNA was synthesized with 2 μg purified total RNA using the reverse transcription system (Vazyme, Nanjing, China). qRT-PCR was performed on a CFX96 real-time PCR system (Bio-Rad, Hercules, CA, United States) with AceQ qPCR SYBR Green Master Mix (Vazyme, Nanjing, China). PCR conditions were as follow: 95°C for 5 min, then 40 cycles of 95°C for 10 s, 60°C for 15 s, and 72°C for 30 s. Rice 18s rRNA gene was used as an internal control to normalize data (Jain et al., 2006). Quantitative gene expression was analyzed by the 2–ΔΔCT method and all primers used were listed in Supplementary Table S1.
Coding region of ONAC066 was fused to GAL4 activation domain in effector vector pGADT7-Rec2 (Clontech, Mountain View, CA, United States). The promoter regions (1,500 bp upstream from ATG) of the genes of interest were amplified and cloned into reporter vector pHis2. pGADT7-Rec2-ONAC066 in combination with a reporter vector harboring the promoter of genes of interest were co-transformed into yeast strain Y187. DNA-protein interactions were verified by growth performance of yeasts grown on medium of SD/-Trp-Leu-His/3-amino-1,2,4-triazole (3-AT, a competitive inhibitor of His3 protein) (Clontech, Mountain View, CA, United States). All primers used for vector construction were listed in Supplementary Table S1.
Leave of 3-week-old ONAC066-OE11 and wild type (WT) plants grown in basal nutrient soil mixture under a 16 h light/8 h dark photoperiod at 28°C were collected and frozen in liquid nitrogen. Total RNA extraction, cDNA synthesis, purification, labeling, and chip hybridization were performed by CapitalBio Technology (Beijing, China) using the Affymetrix Rice GeneChip. Three independent biological replicates were used for each of the genotypes. After log2 transformation and normalization, genes whose expression showed fold change >2 (up-regulated) or <0.5 (down-regulated) with q-Value (a multiple-test corrected p-Value) (Benjamini and Hochberg, 1995) <5% were considered as differentially expressed genes. GO enrichment analysis of differentially expressed or co-expressed genes were performed using AgriGO website1 (Tian et al., 2017).
Promoter regions (1500 bp from ATG codon) of OsWRKY62, LOC_Os02g30110, LOC_Os06g37300 and LOC_Os02g36150 were amplified and cloned into reporter vector pGreenII. pA7-GAL4BD and pA7-GAL4BD::ONAC066 were used as the effectors. Plasmids were extracted using CompactPrep Plasmid Midi Kit (Qiagen, Valencia, CA, United States). Protoplasts were prepared from rice mesophyll cells as previously described (Sun et al., 2012). Briefly, leaf pieces were infiltrated in a vacuum and gently rotated in a cell wall degrading enzyme mixture (0.4 M mannitol, 1.5% cellulose, and 0.3% macerozyme). After 4 h digestion, protoplasts were collected by filtering through 100 mm mesh. For co-transfection assays, 2 μg of the reporter plasmid and 2 μg of the effector plasmid were mixed and introduced into freshly prepared rice protoplasts under 20% PEG solution. After incubated for 12 h at 22°C in dark, LUC assays were performed using the Dual-Luciferase reporter assay system (Promega, Madison, WI, United States) according to the manufacturer’s instructions. The firefly luciferase (LUC) activity was normalized according to the humanized renilla LUC activity in each assay, and the relative ratio was determined by comparing with that of the empty vector. Averages of the relative ratios were calculated from three independent experiments and the primers used for LUC assay are listed in Supplementary Table S1.
Chromatin immuno-precipitation-PCR assays were performed as described previously (Chung et al., 2018). Briefly, chromatin was isolated from ∼3 g of leaves from 2-week-old ONAC066-OE11 plants. After fragment sonication, the DNA/protein complex was immune-precipitated with ChIP-grade antibody against GFP (Roche, Switzerland). After reverse cross-linking, the immunoprecipitated DNA was extracted with phenol/chloroform. The chromatin samples incubated with pre-immune (Pre) serum (GenScript, Nanjing, China) and before immunoprecipitation were used as negative controls and input controls, respectively. PCR was performed using specific primers (Supplementary Table S1) and the products were separated on 1.5% agarose gels and visualized by Goldview staining.
The co-expression data were downloaded from CREP (Collections of Rice Expression Profiling2) and PLANEX (Plant co-expression database3) databases comprising a total of 190 microarray experiments from diverse Chinese cultivated rice varieties and the publicly available GEO data from NCBI, respectively. The permutation test was done to determine the optimal threshold of the Pearson’s correlation coefficients (PCCs) (Butte et al., 2000; Carter et al., 2004). Absolute value of PCC>0.75 or 0.55 for CREP and PLANEX database, respectively, were considered as co-expressed genes with ONAC066 (Aoki et al., 2007). Statistical significance for candidate genes in co-expression network construction was further determined by using a student t-test.
To further explore the biological function of ONAC066, transgenic rice lines with overexpression of ONAC066 or RNAi-mediated suppression of ONAC066 were generated and two independent lines for overexpression (ONAC066-OE11 and ONAC066-OE12) and RNAi-mediated suppression (ONAC066-Ri1 and ONAC066-Ri21) were selected for this study (Yuan et al., 2019b). Involvement of ONAC066 in rice immunity was examined by analyzing M. oryzae-caused disease phenotype on leaves of ONAC066-OE and ONAC066-Ri plants using whole plant inoculation and detached leaf inoculation assays. In whole plant inoculation assays, the ONAC066-OE11 and ONAC066-OE12 showed small, scattered lesions while ONAC066-Ri1 and ONAC066-Ri21 plants developed big and dark brown lesions, as compared to WT plants, which displayed typical blast lesions (Figure 1A). Fungal growth measurement results revealed a 22∼23% of reduction in ONAC066-OE plants and a 263∼396% of increase in ONAC066-Ri plants in comparison to that in WT plants (Figure 1B). In detached leaf inoculation assays, smaller lesions with a reduction of 37∼44% in lesion length were seen on detached leaves from ONAC066-OE plants while larger lesions with an increase of 28∼53% in lesion length were observed when compared with those in WT plants (Figures 1C,D). Similarly, the ONAC066-OE plants supported less fungal growth, accounting for 18∼33% of that in WT plants, while the ONAC066-Ri plants provided more fungal growth, representing 207∼403% of that in WT plants (Figure 1E). A mutant line onac066-1, in which T-DNA is inserted in the third exon of ONAC066 gene (Figure 1F), was identified from RISD (Jeon et al., 2000; An et al., 2003) and homozygous plants were characterized by genotyping (Figure 1G). Transcript of ONAC066 was undetectable in onac066-1 plants (Figure 1H), suggesting that onac066-1 is a null mutant. Disease assays indicated that the onac066-1 plants showed more and larger disease lesions and supported a 1.5-fold higher level of fungal growth, as compared with WT plants (Figures 1I,J). Taken together, these results suggest that ONAC066 acts as a positive regulator of rice immunity against M. oryzae.
Figure 1. ONAC066 positively regulates rice resistance against Magnaporthe oryzae. (A,B) Representative disease phenotype (A) and relative fungal growth (B) in leaves of ONAC066-OE and ONAC066-Ri plants. (C–E) Representative disease phenotype (C), lesion length (D), and relative fungal growth (E) in detached leaves of ONAC066-OE and ONAC066-Ri plants. (F) Gene structure of ONAC066 and location of T-DNA. Open boxes indicate the exons while lines indicate introns. Primers used for genotyping are indicated. (G) Genotyping of onac066-1 mutant by genome DNA PCR. F1 and R1 were used as gene-specific primers and R2/2707RB was a T-DNA-specific primer. onac066-h and onac066-1 stand for heterozygous and homozygous rice plants, respectively. (H) Detection of ONAC066 transcript in onac066-1 mutant plants. (I,J) Representative disease phenotype (I) and relative fungal growth (J) in leaves of onac066 plants. 4-week-old plants of different genotypes were inoculated by foliar spraying of spore suspension of M. oryzae (A,I) or detached leaves from 4-week-old plants were inoculated by dropping 5 μl spore suspension of M. oryzae (C). Photographs and leaf samples were taken at 5 days post inoculation. Relative fungal growth was quantified by genomic qRT-RCR analyzing of the M. oryzae MoPot2 and rice OsUbi genes and shown as ratios of MoPot2/OsUbi. Experiments were repeated at least three times with similar results, and results from one representative experiment are shown in panels (A,C,I). Data presented in panels (B,D,E,J) are the means ± SD from three independent experiments and **/* indicated significant difference at p < 0.01 and p < 0.05 levels, respectively, by Student’s t-test, in comparison to WT.
We previously showed that ONAC066 has the binding ability to NACRS, AtJUB1 binding site (JBS) and JUB-like sequence and is a transcription activator in Y1H assays (Yuan et al., 2019b). The transcription activator activity of ONAC066 was further examined using rice protoplast transient expression system. The effector vectors used for this experiment contain either GAL4-BD alone (GDBD), or ONAC066 (GONAC066), ONAC066-N (GONAC066-N, containing the 1-178 aa N-terminal region), ONAC066-C (GONAC066-C, containing the 179-362 aa C-terminal region), or ONAC022 (GONAC022), closely related to ONAC066 (Hong et al., 2016; Yuan et al., 2019b), fused to GDBD (Figure 2A). In the rice protoplasts co-transfected with the effector and reporter vectors, the LUC activity of the effector GONAC066 or GONAC022 was increased by approximately 1.25- and 2.21-fold of the empty vector GDBD control (Figure 2B). Furthermore, the LUC activity of the effector GONAC066-N was similar to the empty vector GDBD control while the LUC activity of the effector GONAC066-C showed 1.64-fold higher than that of the empty vector GDBD control (Figure 2B). These results demonstrate that ONAC066 has transactivation activator activity in rice cells and also indicate that the C-terminus is critical for the transcriptional activator activity of ONAC066.
Figure 2. Transactivation activity of ONAC066 in rice protoplasts. (A) Diagrams of the effector and reporter constructs used in panel (B). Amino acid positions in ONAC066 are indicated above the GONAC066 construct. (B) Luciferase (LUC) activity assay of GAL4DBD and GAL4 element-mediated LUC activity by full length or truncated ONAC066 in rice protoplasts. Data represented in panel (B) are the means ± SD from three independent experiments and ** indicated significant difference at p < 0.01 by two-tailed unpaired Student’s t-test.
To explore the molecular mechanism of ONAC066 in rice immunity and identify candidates for the ONAC066 target genes, gene expression profiling was performed and compared between ONAC066-OE11 and WT plants. After data processing, a total of 81 (ONAC066-OE11/WT fold changes >2 and q-Value < 5%) and 28 (ONAC066-OE11/WT fold changes <0.5 and q-Value < 5%) genes were found to be up- and down-regulated in ONAC066-OE11 plants grown under normal condition (Table 1 and Supplementary Table S2). The up-regulated and down-regulated genes in ONAC066-OE plants were clustered into 29 and four major categories, respectively (Figure 3A and Supplementary Table S3). The 7 main categories for up-regulated genes in ONAC066-OE plants belong to biological processes, cellular components, and molecular functions (Figure 3A). Notably, three genes for calmodulin-related proteins, LOC_Os03g59770, LOC_Os12g36910, and LOC_Os01g04280, were identified as ONAC066-up-regulated, and LOC_Os01g04280 shows 43.3% identity to Arabidopsis SARD1, which plays a critical role in salicylic acid (SA)-mediated defense signaling (Wang L. et al., 2011). LOC_Os02g50460 (OsPUB40) and LOC_Os03g13740 (OsPUB41) have 43.5 and 43.8% of identity, respectively, to tomato CMPG1, which is required for efficient activation of defense mechanisms in tomato (González-Lamothe et al., 2006). Among the up-regulated genes belonging to the DNA binding category, four WRKY TFs, including OsWRKY45 (Shimono et al., 2007, 2012; Cheng et al., 2015), OsWRKY62 (Peng et al., 2008; Fukushima et al., 2016; Liu et al., 2016), and OsWRKY76 (Yokotani et al., 2013; Liu et al., 2016), which have been shown to be involved in rice immunity, were identified (Table 1). In addition, three defense genes encoding for PR1, PR2 (β-1,3-glucanase), and PR8 (chitinase) were also up-regulated in ONAC066-OE plants (Table 1).
Figure 3. (A,B) Differentially expression of selected defense- and signaling-related genes in ONAC066-OE and ONAC066-Ri plants. Data represented are the means ± SD from three independent experiments and **/* indicated significant difference at p <0.01 and p < 0.05 levels, respectively, by Student’s t-test, in comparison to WT.
Publicly available microarray data GSE7256 (Ribot et al., 2008), which was generated from 2-week-old cv. Nipponbare plants infected with M. grisea virulent isolate FR13, was used to examine whether the up-regulated genes in ONAC066-OE11 plants are also responsive to M. oryzae. Among the 81 up-regulated genes in ONAC066-OE plants, 26 genes (32% of the up-regulated genes), were up-regulated in rice plants at 3 and/or 4 dpi after infection by M. oryzae isolate FR13 (Table 1). It is thus likely that overexpression of ONAC066 activates the expression of a set of genes that are responsive to M. oryzae.
To verify the expression patterns of the up-regulated genes in ONAC066-OE plants, we further compared the expression levels of 12 genes (9 ONAC066-up-regulated genes and 3 previously identified defense genes) among WT, ONAC066-OE and ONAC066-Ri plants by qRT-PCR. The expression levels of the ONAC066-up-regulated genes OsPR1, OsPR2, OsPR8, OsWRKY45, OsWRKY62, OsWRKY79, Myb (LOC_Os03g04760), POD (LOC_Os04g59200), and OsLOX7 (LOC_Os08g39840) as well as of two well-known defense genes OsPR1b and OsPAL1 (Inui et al., 1997; Agrawal et al., 2001) were significantly up-regulated in ONAC066-OE plants but markedly down-regulated in ONAC066-Ri plants (Figure 3B). Expression of another well-known defense gene OsPBZ1 (Midoh and Iwata, 1996) was significantly down-regulated in ONAC066-Ri plants but not changed in ONAC066-OE plants (Figure 3B). In the transcriptomic analyses, no expression data for OsPR1b were obtained and OsPBZ1 and OsPAL1 were not considered as differentially expressed genes as their expression was up-regulated by ∼1 fold with q-Value > 5%. The difference in expression change of OsPAL1 in ONAC066-OE plants, as revealed by qRT-PCR and transcriptomic analyses, may be due to different techniques used. Collectively, these data indicate that ONAC066 plays a role in activation of defense signaling and response in rice immunity.
The up-regulation pattern of OsWRKY45, OsWRKY62, OsWRKY76, and OsWRKY79 in ONAC066-OE plants led to examine whether these WRKY genes were ONAC066 targets. The binding ability of ONAC066 to the promoters of these WRKY genes was examined by Y1H assays. For this purpose, the 1500 bp promoter regions from start codons of these WRKY genes were amplified and cloned into pHis2 vectors (Figure 4A) and were then co-transformed with vector Rec2-ONAC066 into yeast strain Y187. Yeast co-transformed with Rec2-ONAC066 and pHis2-pWRKY62 grew normally on 3-AT containing medium, while yeast co-transformed with empty Rec2 and pHis2 vectors did not grow well at a high concentration of 3-AT (Figure 4B), suggesting that ONAC066 could directly bind to the promoter of OsWRKY62. However, growth performance of yeasts co-transformed with Rec2-ONAC066 and pHis2-pWRKY45, pHis2-pWRKY76, or pHis2-pWRKY79 was indistinguishable to yeast co-transformed with empty Rec2 and pHis2 vectors on 3-AT containing medium (Supplementary Figure S1), indicating that ONAC066 did not bind to the promoters of OsWRKY45, OsWRKY76, and OsWRKY79.
Figure 4. ONAC066 activates OsWRKY62 promoter. (A) Diagram of yeast expression construct Rec2-ONAC066 and reporter construct pHis2-pWRKY62. (B) Growth performance of transformants on SD/-Leu-/Trp/-His medium containing 50 or 100 mM 3-AT. (C) Diagram of putative NAC core-binding sites in OsWRKY62 promoter. P1-P4 were the probes used in ChIP-PCR assays. (D) ChIP-PCR analysis of ONAC066 binding to OsWRKY62 promoter. ChIP of ONAC066-GFP transgenic line using GFP antibody (α-GFP) or pre-immune (Pre) serum was performed and precipitated DNA fragments were subject to PCR analysis using OsWRKY62 promoter primers. 10% of chromatin amount before IP was used as positive controls (input) and IP sample with pre-immune serum was used as a negative control. (E,F) Activation of OsWRKY62 promoter by ONAC066. (E) Diagrams of the effector and reporter constructs. A 1500 bp from start codon was used as the OsWRKY62 promoter. (F) Luciferase activity in protoplasts co-transfected with the reporter and different combinations of effectors was determined. Experiments were repeated at least three times with similar results, and results from one representative experiment are shown in panels (B,D). Data presented in panel (F) are the means ± SD from three independent experiments and ** indicated significant difference at p < 0.01 by Student’s t-test, in comparison to empty vector.
The binding site of ONAC066 in OsWRKY62 promoter was further mapped by ChIP-PCR. Four NAC core-binding sites (CACG) (Tran et al., 2004) were identified within the 1500 bp promoter region of the OsWRKY62 gene and four probe regions were chosen for ChIP-PCR assays (Figure 4C). No PCR amplicon was detected in the four probe regions when pre-immune serum was used in IP (Figure 4D). A clear amplicon was observed in P4 probe region and no amplicon was detected in P1, P2, and P3 probe regions, when anti-GFP antibody was used in IP (Figure 4D), demonstrating that ONAC066 binds to P4 region, which contains three NAC core-binding sites (Figure 4C), in the OsWRKY62 promoter.
The ability of ONAC066 to drive the transcription of OsWRKY62 was further explored by using the rice protoplast transient expression system with the reporter vector harboring OsWRKY62 promoter, and the effector vector expressing ONAC066 (Figure 4E). In rice protoplasts co-transfected with the effector vector ONAC066 and reporter vector pOsWRKY62, the LUC activity was significantly higher than that in rice protoplasts co-transfected with the empty effector vector and reporter vector pOsWRKY62 (Figure 4F). These results indicate that ONAC066 transcriptionally drives the OsWRKY62 promoter. Taken together, it is clear that ONAC066 can bind to the NAC core-binding sites in OsWRKY62 promoter and thus drives the expression of OsWRKY62.
To further characterize putative ONAC066 targets, ONAC066 was used as a guide gene to identify its co-expressed genes using expression profiling data from 190 datasets in CREP and NCBI GEO-based PLANEX databases. As results, 86 and 114 genes with PCC values greater than 0.75 or 0.55 for CREP and PLANES databases, respectively, were identified as tightly co-expressed genes with ONAC066 (Supplementary Tables S4, S5). Enriched GO annotations were particularly clustered into categories of biological process and molecular functions, which are tightly associated with catalytic activity, binding, electron carrier activity, transcription regulator and transporter activity (Figure 5A).
Figure 5. Enriched GO terms of genes co-expressed with ONAC066 and expression of the selected cytochrome P450 genes and DPF in ONAC066-OE and ONAC066-Ri plants. (A) Enriched GO terms of genes co-expressed with ONAC066. (B) Expression of selected cytochrome P450 genes. (C) Expression of DPF. Data represented in panels (B,C) are the means ± SD from three independent experiments and **/* indicated significant difference at p < 0.01 and p < 0.05 levels, respectively, by Student’s t-test, in comparison to WT.
A total of 15 co-expressed genes were simultaneously identified in CREP and PLANEX databases (Table 2), among which 5 belong to the cytochrome P450 gene family. In fact, 14 cytochrome P450 genes were found to be co-expressed with ONAC066 in CREP and PLANEX databases and five of them were also induced by M. oryzae FR13 (Table 3). To verify the co-expression of these cytochrome P450 genes with ONAC066, we compared their expression patterns of 5 cytochrome P450 genes among WT, ONAC066-OE and ONAC066-Ri plants by qRT-PCR. The expression levels of these 5 cytochrome P450 genes, including LOC_Os01g41820 (CYP72A33), LOC_Os02g30110 (CYP81L6), LOC_Os03g25480 (CYP709B2), LOC_Os06g37300 (CYP701A8/OsKOL4), and LOC_Os02g36150 (CYP71Z6), were significantly up-regulated in ONAC066-OE plants but markedly down-regulated in ONAC066-Ri plants (Figure 5B), further confirming the co-expression feature of these cytochrome P450 genes with ONAC066. However, the expression patterns of the remaining co-expressed cytochrome P450 genes in ONAC066-OE and ONAC066-Ri plants were similar to WT (Supplementary Figure S2). Moreover, DPF, encoding a basic helix-loop-helix transcription factor acting as a master transcription factor in biosynthesis of diterpenoid phytoalexins (Yamamura et al., 2015), was also significantly up-regulated in ONAC066-OE plants but markedly down-regulated in ONAC066-Ri plants (Figure 5C).
The co-expression feature of the cytochrome P450 genes with ONAC066 raised the possibility that they were ONAC066 targets. The binding ability of ONAC066 to promoters of the co-expressed cytochrome P450 genes was therefore examined by Y1H assays. For this purpose, the 1500 bp promoter regions from start codons of these cytochrome P450 genes were cloned into pHis2 vectors and were then co-transformed with vector Rec2-ONAC066 into yeast strain Y187. Yeast co-transformed with Rec2-ONAC066 and pHis2-pLOC_Os02g30110 or pHis2-pLOC_Os06g37300 or pHis2-pLOC_Os02g36150 grew well on SD/-Trp/-Leu/-His medium containing 100 mM 3-AT, while yeasts co-transformed with Rec2-ONAC066 and pHis2-pLOC_Os01g41820 or pHis2-pLOC_Os03g25480 did not grow on the same medium (Figure 6A). These results indicate that ONAC066 could bind to the promoters of LOC_Os02g30110, LOC_Os06g37300, and LOC_Os02g36150 to activate the reporter gene expression in yeasts.
Figure 6. ONAC066 activates promoters of three cytochrome P450 genes. (A) Growth performance of transformants on SD/-Leu-/Trp/-His medium containing 100 mM 3-AT. (B) Diagrams of putative NAC core-binding sites in the promoters of the selected cytochrome P450 genes. P5-P16 represent the probes used in ChIP-PCR assays. (C) ChIP-PCR analysis of ONAC066 binding to promoters of the selected cytochrome P450 genes. ChIP of ONAC066-GFP transgenic line using GFP antibody (α-GFP) or pre-immune (Pre) serum was performed and precipitated DNA fragments were subject to PCR analysis using different primers for promoters of the selected cytochrome P450 genes. 10% of chromatin amount before IP was used as positive controls (input) and IP sample with pre-immune serum was used as a negative control. (D) Activation of promoters of the selected cytochrome P450 genes by ONAC066. Fragments of 1500 bp from start codon were used as promoters of the selected cytochrome P450 genes. Luciferase activity in protoplasts co-transfected with the reporter and different combinations of effectors was determined. Experiments were repeated at least three times with similar results, and results from one representative experiment are shown in panels (A,C). Data presented in panel (D) are the means ± SD from three independent experiments and ** indicated significant difference at p < 0.01 by Student’s t-test, in comparison to empty vector.
The binding site of ONAC0066 in the promoters of LOC_Os02g30110, LOC_Os06g37300, and LOC_Os02g36150 was further mapped by ChIP-PCR. One, three and five NAC core-binding sites (CACG) (Tran et al., 2004) were identified within the 1500 bp promoter regions of LOC_Os02g30110, LOC_Os06g37300 and LOC_Os02g36150, respectively, and four probe regions for each promoter of the genes were chosen for ChIP-PCR assays (Figure 6B). No PCR amplicon was detected in the four probe regions for each promoter of the genes tested when pre-immune serum was used in IP (Figure 6C). Amplicons were observed in P5 and P6 probe regions in LOC_Os02g30110 promoter, P12 probe region in LOC_Os06g37300 promoter and P13 and P14 probe regions in LOC_Os02g36150 promoter, when anti-GFP antibody was used in IP (Figure 6C). Notably, a clear amplicon was detected in P5 probe region of LOC_Os02g30110 promoter, which does not contain a NAC core-binding site (Figure 6C). Together, these results suggest that ONAC066 binds to the LOC_Os02g30110, LOC_Os06g37300, and LOC_Os02g36150 promoters.
The ability of ONAC066 to drive the transcription of LOC_Os02g30110, LOC_Os06g37300, and LOC_Os02g36150 genes was further explored by using the rice protoplast transient expression system with the effector vector expressing ONAC066 and the reporter vector harboring the promoters of LOC_Os02g30110, LOC_Os06g37300, or LOC_Os02g36150. In rice protoplasts co-transfected with the effector vector ONAC066 and reporter vector pOs02g30110, pOs06g37300, or pOs02g36150, the LUC activity was significantly higher than that in rice protoplasts co-transfected with the empty effector vector and reporter vectors (Figure 6D). These results indicate that ONAC066 transcriptionally drives the promoters of the LOC_Os02g30110, LOC_Os06g37300 and LOC_Os02g36150 genes. Collectively, it is clear that ONAC066 can bind to the promoters of LOC_Os02g30110, LOC_Os06g37300 and LOC_Os02g36150 and thus drives their expression.
The present study further investigated the function and explored the molecular mechanism of ONAC066 in rice immunity. ONAC066 belongs to ONAC022 subgroup (Ooka et al., 2003) and its Arabidopsis homologous ANAC042 was found to suppress defense responses to a bacterial pathogen (Shahnejat-Bushehri et al., 2016). ONAC066 transcriptionally responded to M. oryzae infection and abiotic stress factors (Liu et al., 2018; Yuan et al., 2019b) and thus is a stress-responsive NAC TF that can respond to multiple abiotic and biotic factors in rice. A recent study revealed that ONAC066 positively regulates resistance to blast and bacterial blight diseases through ABA-dependent signaling pathway (Liu et al., 2018). In this study, ONAC066-OE plants exhibited enhanced resistance while ONAC066-Ri and onac066-1 plants showed attenuated resistance to M. oryzae (Figure 1), clearly demonstrating that ONAC066 plays a positive role in rice immunity against M. oryzae. Furthermore, we previously demonstrated that ONAC066 functions as a positive regulator of drought and oxidative stress response in rice (Yuan et al., 2019b). Collectively, these findings enable ONAC066 as a promising factor to be used in creating novel rice germplasm with improved abiotic stress tolerance and disease resistance.
ONAC066 is a nucleus localized TF with an ability to bind to a canonical NAC recognition sequence (Tran et al., 2004) and a newly identified cis-element JUB1 binding site (Wu et al., 2012) in yeasts (Liu et al., 2018; Yuan et al., 2019b). ONAC066 has transcription activator activity that depends on its C-terminal in yeast (Yuan et al., 2019b). This is further demonstrated in the present study that ONAC066 has transcription activator activity in rice cells (Figure 2). Therefore, ONAC066 acts as a transcription activator and exerts its biological function through activating downstream target genes. Notably, the C-terminal region of ONAC066 was capable of initiating the transcription in rice cells (Figure 2), which is similar to the previous observations on ONAC022 and OsNAP, whose C-terminal regions showed abilities to activate the transcription in yeast (Liang et al., 2014; Hong et al., 2016). Generally, the N-terminals of NAC proteins recognize their cis-element sequences while the C-terminals is responsible to initiate the transcription of the downstream target genes (Olsen et al., 2005). Whether the C-terminal of ONAC066 affect or abolish its interactions with the cis-elements in OsWRKY62 promoter is unknown. However, our previous study showed that the full ONAC022 with its C-terminal could bind to the NACRS element in electrophoretic mobility shift assays (Hong et al., 2016), implying that the C-terminals may not affect the interactions of NAC proteins with the cis-elements in promoters of their target genes. On the other hand, ONAC066 was found to bind to probe regions harboring NAC core-binding sites in the promoters of OsWRKY62, LOC_Os02g30110, LOC_Os06g37300, and LOC_Os02g36150 genes (Figures 4, 6). However, ONAC066 also bound to a probe region without a NAC core-binding site in LOC_Os02g30110 promoter (Figure 6), suggesting that ONAC066 can bind to other unknown cis-elements in the promoters of its target genes. This is partially supported by our previous observation that ONAC066 not only bound to NACRS and JBS cis-elements in Y1H assays but also bound to a JBS-like cis-element in OsDREB2A promoter in ChIP-PCR and EMSA assays (Yuan et al., 2019b). Although the interaction of ONAC066 with OsWRKY62 promoter was validated by different experiments in the present study, including Y1H, ChIP-PCR and rice protoplast transient expression assays, the detailed cis-element(s) in OsWRKY62 promoter for ONAC066 need to be further identified.
Because ONAC066 has transcription activator activity in rice cells (Figure 2), we thus sought for genes that were up-regulated in ONAC066-OE plants by gene expression profiling analyses between ONAC066-OE11 and WT plants. Surprisingly, only 81 up-regulated genes were identified in ONAC066-OE plants (Table 1). The number of the up-regulated genes in ONAC066-OE plants is comparable to the numbers of co-expressed genes identified in two different databases (Supplementary Tables S4, S5). The up-regulated expression of genes for PRs, WRKYs and homologues of immunity-related factors (SARD1 and CMPG1) demonstrates the activation of defense response in ONAC066-OE plants. Importantly, 26 of these up-regulated genes were predicted to be induced by M. oryzae infection (Table 1), suggesting that ONAC066 may modulate a group of genes that are responsive to M. oryzae. Defense and signaling genes were significantly up-regulated in ONAC066-OE plants but down-regulated in ONAC066-Ri plants (Figure 3), indicating that the expression of these defense and signaling genes depends largely on ONAC066 function. This is partially similar to OsNAC111, which positively contributes to disease resistance by regulating the expression of a specific set of defense genes in disease response (Yokotani et al., 2014). Unlike OsNAC111 whose suppression did not affect rice immunity, ONAC066-Ri plants showed increased susceptibility to M. oryzae infection (Figure 1), implying that ONAC066 is required for basal immunity against M. oryzae.
Although the immunity-related functions of rice NAC TFs have been well documented (Nakashima et al., 2007; Kaneda et al., 2009; Yoshii et al., 2009, 2010; Sun et al., 2013; Yokotani et al., 2014; Park et al., 2017; Liu et al., 2018; Wang et al., 2018), the molecular mechanisms of these NAC TFs to regulate rice immunity are largely unknown yet. ONAC066 was previously found to directly bind to the promoter of OsNCED4 to modulate its expression (Liu et al., 2018). OsNCED4 is one of the 9-cis-epoxycarotenoid dioxygenases acting as a rate-limiting enzyme for abscisic acid biosynthesis (Huang et al., 2018, 2019; Hwang et al., 2018). Considering that ONAC066 is a transcription activator (Figure 2), the binding of ONAC066 to OsNCED4 promoter should drive the expression of OsNCED4, which in turn leads to ABA biosynthesis. However, the expression level of OsNCED4 was significantly down-regulated and ABA level was also markedly reduced in ONAC066-overexpressing plants (Liu et al., 2018). Therefore, whether OsNCED4 is a true target of ONAC066 and whether ABA signaling is involved in ONAC066-mediated regulation of rice immunity need to be further examined. On the other hand, SARD1 has been demonstrated to play a critical role in SA signaling through regulating pathogen-induced biosynthesis of SA in Arabidopsis (Zhang et al., 2010; Wang L. et al., 2011). The up-regulated expression of LOC_Os01g04280, which shows 43.3% of identity to Arabidopsis SARD1, in ONAC066-OE plants raises a possibility that ONAC066 may regulate rice immunity through SA signaling via modulating LOC_Os01g04280 expression.
In the present study, immunity-related WRKY genes including OsWRKY45 and OsWRKY62 (Shimono et al., 2007, 2012; Peng et al., 2008; Yokotani et al., 2013; Cheng et al., 2015; Fukushima et al., 2016; Liu et al., 2016) were significantly up-regulated in ONAC066-OE plants but down-regulated in ONAC066-Ri plants (Figure 3 and Table 1). Y1H assays revealed ONAC066 could bind the OsWRKY62 promoter but not the promoters of OsWRKY45, OsWRKY76 and OsWRKY79 (Figure 4A and Supplementary Figure S1). ChIP-PCR and rice protoplast transient expression assays demonstrated that ONAC066 bound to the NAC core-binding site in OsWRKY62 promoter and activated OsWRKY62 expression (Figure 4). It is thus likely that OsWRKY62 is a target of ONAC066 and ONAC066 modulates the expression of OsWRKY62 through direct binding to NAC core-binding site in OsWRKY62 promoter. Among these four WRKY genes up-regulated in ONAC066-OE plants, only OsWRKY62 was identified as one of the ONAC066 targets (Figure 4). The portion between verified ONAC066 binding and up-regulated expression of the genes in ONAC066-OE plants was 25%, which is similar to values reported for other plant TFs such as Arabidopsis WRKY33 (Liu et al., 2015). The altered expression of OsWRKY45 and OsWRKY76 in ONAC066-OE and ONAC066-Ri plants may be affected indirectly by the ONAC066-activated defense signaling pathway. OsWRKY45, a central component of the SA signaling pathway, is a positive regulator of rice immunity (Shimono et al., 2007, 2012; Goto et al., 2015, 2016). However, inconsistent conclusions regarding the function of OsWRKY62 in rice immunity were previously reported; for example, OsWRKY62 and OsWRKY76 are negative regulators of rice immunity against blast and bacterial leaf blight diseases (Peng et al., 2008, 2010; Liu et al., 2016; Liang et al., 2017) while OsWRKY62 was reported to play a positive role in rice immunity (Fukushima et al., 2016). OsWRKY62 can form homodimers and heterodimers with OsWRKY45, and the OsWRKY45-OsWRKY62 heterodimer acts as a strong activator while the OsWRKY62 homodimer acts as a repressor of rice immunity (Fukushima et al., 2016). Simultaneous up-regulation of OsWRKY45 and OsWRKY62 in ONAC066-OE plants implies the formation of the OsWRKY45-OsWRKY62 heterodimers, which leads to the activation of defense response.
Due to limited number of up-regulated genes was identified in ONAC066-OE plants, we tried to mine putative target genes of ONAC066 through co-expression analysis, which has been successfully used to establish the transcriptional regulatory network and identify target genes for given TFs (Fang et al., 2015; Smita et al., 2015). Hundreds of functional genes were found to be co-expressed with ONAC066 through two different online tools CREP and PLANEX (Supplementary Tables S4, S5). Among 15 co-expressed genes simultaneously identified in two different databases, 5 encode cytochrome P450s (Table 3). Cytochromes P450s constitute one of the largest families of enzymatic proteins with diverse functions from critical structural components to key signaling molecules (Mizutani and Ohta, 2010; Nelson and Werck-Reichhart, 2011; Pandian et al., 2020). Rice genome harbors 326 genes coding for 355 cytochrome P450s (Wei and Chen, 2018). Some of rice cytochrome P450s are involved in biosynthesis of diterpenoid phytoalexins and therefore play roles in resistance against fungal and bacterial pathogens (Wang Q. et al., 2011; Li et al., 2013, 2015; Maeda et al., 2019; Shen et al., 2019; Kitaoka et al., 2021; Liang et al., 2021). In our gene expression profiling and co-expression analysis, a set of cytochrome P450 genes were identified to be co-expressed with ONAC066 (Table 3). The co-expression feature of 5 cytochrome P450 genes was confirmed by their up-regulated expressions in ONAC066-OE plants and down-regulated expressions in ONAC066-Ri plants (Figure 5). ONAC066 directly bound to the promoters of 3 co-expressed cytochrome P450 genes, LOC_Os02g30110, LOC_Os06g37300 and LOC_Os02g36150, and activated their transcription (Figure 6), demonstrating that the LOC_Os02g30110, LOC_Os06g37300, and LOC_Os02g36150 cytochrome P450 genes are ONAC066 targets. The fact that DPF, a positive regulator of biosynthesis of diterpenoid phytoalexins (Yamamura et al., 2015), was up-regulated in ONAC066-OE plants but down-regulated in ONAC066-Ri plants (Figure 5) imply that ONAC066 may play a role in regulating biosynthesis of diterpenoid phytoalexins in rice. Another, among the co-expressed cytochrome P450 genes, OsCAld5H1 (LOC_Os10g36848) has been shown to be involved in regulating the structure of cell wall lignin (Takeda et al., 2017, 2019), which is related to rice immunity against M. oryzae and X. oryzae pv. oryzae (Liu et al., 2017; Li et al., 2020; Onohata and Gomi, 2020; Shamsunnaher et al., 2020). It is likely that ONAC066 contributes positively to rice immunity through direct regulation of a set of cytochrome P450s with distinct functions in biosynthesis of diterpenoid phytoalexins, cell wall lignin and other secondary metabolisms. However, the involvement of these co-expressed cytochrome P450s in rice immunity needs further investigation.
In the present study, we further demonstrated that ONAC066 is a positive regulator of rice immunity against M. oryzae and identified OsWRKY62 and three cytochrome P450 genes as ONAC066 targets. Therefore, ONAC066 contributes positively to rice immunity through regulating OsWRKY62 expression to activate defense response and/or activating a set of cytochrome P450 genes to promote the biosynthesis of defense compounds including phytoalexins. Together with our previous study on the function of ONAC066 in abiotic stress response (Yuan et al., 2019b), we concluded that ONAC066 acts as a positive regulator of abiotic and biotic stress response in rice and thus can be used in creating novel rice germplasm with improved abiotic stress tolerance and disease resistance.
The original contributions presented in the study are included in the article/Supplementary Material, further inquiries can be directed to the corresponding author/s.
XY, DL, and FS conceived the project, designed the experiments, analyzed the data, and drafted the manuscript. XY generated all material used in this study (cloning, vector, transformations, and transgenic plants). XY, HW, YB, YY, YG, XX, and JW performed the experiments and collected the data. All authors commented on the manuscript.
This study was supported by the National Key Research and Development Program of China (No. 2016YFD0100600) and the National Natural Science Foundation of China (Nos. 31871945 and 32072403).
The authors declare that the research was conducted in the absence of any commercial or financial relationships that could be construed as a potential conflict of interest.
All claims expressed in this article are solely those of the authors and do not necessarily represent those of their affiliated organizations, or those of the publisher, the editors and the reviewers. Any product that may be evaluated in this article, or claim that may be made by its manufacturer, is not guaranteed or endorsed by the publisher.
The Supplementary Material for this article can be found online at: https://www.frontiersin.org/articles/10.3389/fpls.2021.749186/full#supplementary-material
Supplementary Figure S1 | ONAC066 could not bind the promoters of OsWRKY45, OsWRKY76 and OsWRKY79.
Supplementary Figure S2 | Expression patterns of identified co-expressed cytochrome P450 genes in ONAC066-OE and ONAC066-Ri plants.
Supplementary Table S1 | List of primers used in this study.
Supplementary Table S2 | Down-regulated genes in ONAC066-OE plants.
Supplementary Table S3 | GO terms for up- and down-regulated genes in ONAC066-OE plants.
Supplementary Table S4 | A 84 genes co-expressed with ONAC066 from CREP database.
Supplementary Table S5 | A 114 genes co-expressed with ONAC066 from PALNEX database.
Agrawal, G. K., Rakwalm, R., Jwa, N. S., and Agrawal, V. P. (2001). Signalling molecules and blast pathogen attack activates rice OsPR1a and OsPR1b genes: a model illustrating components participating during defence/stress response. Plant Physiol. Biochem. 39, 1095–1103. doi: 10.1016/S0981-9428(01)01333-X
An, S., Park, S., Jeong, D. H., Lee, D. Y., Kang, H. G., Yu, J. H., et al. (2003). Generation and analysis of end sequence database for T-DNA tagging lines in rice. Plant Physiol. 133, 2040–2047. doi: 10.1104/pp.103.030478
Aoki, K., Ogata, Y., and Shibata, D. (2007). Approaches for extracting practical information from gene co-expression networks in plant biology. Plant Cell Physiol. 48, 381–390. doi: 10.1093/pcp/pcm013
Benjamini, Y., and Hochberg, Y. (1995). Controlling the false discovery rate: a practical and powerful approach to multiple testing. J. R. Stat. Soc. B 57, 289–300. doi: 10.1111/j.2517-6161.1995.tb02031.x
Bian, Z., Gao, H., and Wang, C. (2020). NAC transcription factors as positive or negative regulators during ongoing battle between pathogens and our food crops. Int. J. Mol. Sci. 22:81. doi: 10.3390/ijms22010081
Birkenbihl, R. P., Liu, S., and Somssich, I. E. (2017). Transcriptional events defining plant immune responses. Curr. Opin. Plant Biol. 38, 1–9. doi: 10.1016/j.pbi.2017.04.004
Boller, T., and He, S. Y. (2009). Innate immunity in plants: an arms race between pattern recognition receptors in plants and effectors in microbial pathogens. Science 324, 742–744. doi: 10.1126/science.1171647
Buscaill, P., and Rivas, S. (2014). Transcriptional control of plant defence responses. Curr. Opin. Plant Biol. 20, 35–46. doi: 10.1016/j.pbi.2014.04.004
Butte, A. J., Tamayo, P., Slonim, D., Golub, T. R., and Kohane, I. S. (2000). Discovering functional relationships between RNA expression and chemotherapeutic susceptibility using relevance networks. Proc. Natl. Acad. Sci. U.S.A. 97, 12182–12186. doi: 10.1073/pnas.220392197
Carter, S. L., Brechbuhler, C. M., Griffin, M., and Bond, A. T. (2004). Gene co-expression network topology provides a framework for molecular characterization of cellular state. Bioinformatics 20, 2242–2250. doi: 10.1093/bioinformatics/bth234
Chen, J., Clinton, M., Qi, G., Wang, D., Liu, F., and Fu, Z. Q. (2020). Reprogramming and remodeling: transcriptional and epigenetic regulation of salicylic acid-mediated plant defense. J. Exp. Bot. 71, 5256–5268. doi: 10.1093/jxb/eraa072
Cheng, H., Liu, H., Deng, Y., Xiao, J., Li, X., and Wang, S. (2015). The WRKY45-2 WRKY13 WRKY42 transcriptional regulatory cascade is required for rice resistance to fungal pathogen. Plant Physiol. 167, 1087–1099. doi: 10.1104/pp.114.256016
Chung, P. J., Jung, H., Do Choi, Y., and Kim, J. K. (2018). Genome-wide analyses of direct target genes of four rice NAC-domain transcription factors involved in drought tolerance. BMC Genomics 9:40. doi: 10.1186/s12864-017-4367-1
Dangl, J. L., Horvath, D. M., and Staskawicz, B. J. (2013). Pivoting the plant immune system from dissection to deployment. Science 341, 746–751. doi: 10.1126/science.1236011
Dey, S., and Vlot, A. C. (2015). Ethylene responsive factors in the orchestration of stress responses in monocotyledonous plants. Front. Plant Sci. 6:640. doi: 10.3389/fpls.2015.00640
Diao, P., Chen, C., Zhang, Y., Meng, Q., Lv, W., and Ma, N. (2020). The role of NAC transcription factor in plant cold response. Plant Signal Behav. 15:1785668. doi: 10.1080/15592324.2020.1785668
Fang, Y., Liao, K., Du, H., Xu, Y., Song, H., Li, X., et al. (2015). A stress-responsive NAC transcription factor SNAC3 confers heat and drought tolerance through modulation of reactive oxygen species in rice. J. Exp. Bot. 66, 6803–6817. doi: 10.1093/jxb/erv386
Fang, Y., You, J., Xie, K., Xie, W., and Xiong, L. (2008). Systematic sequence analysis and identification of tissue-specific or stress-responsive genes of NAC transcription factor family in rice. Mol. Gen. Genomics 280, 547–563. doi: 10.1007/s00438-008-0386-6
Forlani, S., Mizzotti, C., and Masiero, S. (2021). The NAC side of the fruit: tuning of fruit development and maturation. BMC Plant Biol. 21:238. doi: 10.1186/s12870-021-03029-y
Fukushima, S., Mori, M., Sugano, S., and Takatsuji, H. (2016). Transcription factor WRKY62 plays a role in pathogen defense and hypoxia-responsive gene expression in rice. Plant Cell Physiol. 57, 2541–2551. doi: 10.1093/pcp/pcw185
González-Lamothe, R., Tsitsigiannis, D. I., Ludwig, A. A., Panicot, M., Shirasu, K., and Jones, J. D. (2006). The U-box protein CMPG1 is required for efficient activation of defense mechanisms triggered by multiple resistance genes in tobacco and tomato. Plant Cell 18, 1067–1083. doi: 10.1105/tpc.106.040998
Goto, S., Sasakura-Shimoda, F., Suetsugu, M., Selvaraj, M. G., Hayashi, N., Yamazaki, M., et al. (2015). Development of disease-resistant rice by optimized expression of WRKY45. Plant Biotechnol. J. 13, 753–765. doi: 10.1111/pbi.12303
Goto, S., Sasakura-Shimoda, F., Yamazaki, M., Hayashi, N., Suetsugu, M., Ochiai, H., et al. (2016). Development of disease-resistant rice by pathogen-responsive expression of WRKY45. Plant Biotechnol. J. 14, 1127–1138. doi: 10.1111/pbi.12481
Hong, Y., Yang, Y., Zhang, H., Huang, L., Li, D., and Song, F. (2017). Overexpression of MoSM1, encoding for an immunity-inducing protein from Magnaporthe oryzae, in rice confers broad-spectrum resistance against fungal and bacterial diseases. Sci. Rep. 7:41037. doi: 10.1038/srep41037
Hong, Y., Zhang, H., Huang, L., Li, D., and Song, F. (2016). Overexpression of a stress-responsive NAC transcription factor gene ONAC022 improves drought and salt tolerance in rice. Front. Plant Sci. 7:4. doi: 10.3389/fpls.2016.00004
Huang, P. Y., Catinot, J., and Zimmerli, L. (2016). Ethylene response factors in Arabidopsis immunity. J. Exp. Bot. 67, 1231–1241. doi: 10.1093/jxb/erv518
Huang, Y., Guo, Y., Liu, Y., Zhang, F., Wang, Z., Wang, H., et al. (2018). 9-cis-Epoxycarotenoid dioxygenase 3 regulates plant growth and enhances multi-abiotic stress tolerance in rice. Front. Plant Sci. 9:162. doi: 10.3389/fpls.2018.00162
Huang, Y., Jiao, Y., Xie, N., Guo, Y., Zhang, F., Xiang, Z., et al. (2019). OsNCED5, a 9-cis-epoxycarotenoid dioxygenase gene, regulates salt and water stress tolerance and leaf senescence in rice. Plant Sci. 287, 110188. doi: 10.1016/j.plantsci.2019.110188
Hwang, S. G., Lee, C. Y., and Tseng, C. S. (2018). Heterologous expression of rice 9-cis-epoxycarotenoid dioxygenase 4 (OsNCED4) in Arabidopsis confers sugar oversensitivity and drought tolerance. Bot. Stud. 59:2. doi: 10.1186/s40529-018-0219-9
Inui, H., Yamaguchi, Y., and Hirano, S. (1997). Elicitor actions of N-acetylchitooligosaccharides and laminarioligosaccharides for chitinase and L-phenylalanine ammonia-lyase induction in rice suspension culture. Biosci. Biotechnol. Biochem. 61, 975–978. doi: 10.1271/bbb.61.975
Jain, M., Nijhawan, A., Tyagi, A. K., and Khurana, J. P. (2006). Validation of housekeeping genes as internal control for studying gene expression in rice by quantitative real-time PCR. Biochem. Biophys. Res. Commun. 345, 646–651. doi: 10.1016/j.bbrc.2006.04.140
Jeon, J. S., Lee, S., Jung, K. H., Jun, S. H., Jeong, D. H., Lee, J., et al. (2000). T-DNA insertional mutagenesis for functional genomics in rice. Plant J. 22, 561–570. doi: 10.1046/j.1365-313x.2000.00767.x
Jones, J. D. G., and Dangl, J. L. (2006). The plant immune system. Nature 444, 323–329. doi: 10.1038/nature05286
Kaneda, T., Taga, Y., Takai, R., Iwano, M., Matsui, H., Takayama, S., et al. (2009). The transcription factor OsNAC4 is a key positive regulator of plant hypersensitive cell death. EMBO J. 28, 926–936. doi: 10.1038/emboj.2009.39
Kawahara, Y., Oono, Y., Kanamori, H., Matsumoto, T., Itoh, T., and Minami, E. (2012). Simultaneous RNA-seq analysis of a mixed transcriptome of rice and blast fungus interaction. PLoS One 7:e49423. doi: 10.1371/journal.pone.0049423
Kim, H. J., Nam, H. G., and Lim, P. O. (2016). Regulatory network of NAC transcription factors in leaf senescence. Curr. Opin. Plant Biol. 33, 48–56. doi: 10.1016/j.pbi.2016.06.002
Kitaoka, N., Zhang, J., Oyagbenro, R. K., Brown, B., Wu, Y., Yang, B., et al. (2021). Interdependent evolution of biosynthetic gene clusters for momilactone production in rice. Plant Cell 33, 290–305. doi: 10.1093/plcell/koaa023
Kou, X., Zhou, J., Wu, C. E., Yang, S., Liu, Y., Chai, L., et al. (2021). The interplay between ABA/ethylene and NAC TFs in tomato fruit ripening: a review. Plant Mol. Biol. 106, 223–238. doi: 10.1007/s11103-021-01128-w
Li, B., Meng, X., Shan, L., and He, P. (2016). Transcriptional regulation of pattern-triggered immunity in plants. Cell Host Microbe 19, 641–650. doi: 10.1016/j.chom.2016.04.011
Li, W., Shao, M., Yang, J., Zhong, W., Okada, K., Yamane, H., et al. (2013). Oscyp71Z2 involves diterpenoid phytoalexin biosynthesis that contributes to bacterial blight resistance in rice. Plant Sci. 207, 98–107. doi: 10.1016/j.plantsci.2013.02.005
Li, W., Wang, F., Wang, J., Fan, F., Zhu, J., Yang, J., et al. (2015). Overexpressing CYP71Z2 enhances resistance to bacterial blight by suppressing auxin biosynthesis in rice. PLoS One 10:e0119867. doi: 10.1371/journal.pone.0119867
Li, W., Wang, K., Chern, M., Liu, Y., Zhu, Z., Liu, J., et al. (2020). Sclerenchyma cell thickening through enhanced lignification induced by OsMYB30 prevents fungal penetration of rice leaves. New Phytol. 226, 1850–1863. doi: 10.1111/nph.16505
Li, W., Zhu, Z., Chern, M., Yin, J., Yang, C., Ran, L., et al. (2017). A natural allele of a transcription factor in rice confers broad-spectrum blast resistance. Cell 170, 114–126 e115. doi: 10.1016/j.cell.2017.06.008
Liang, C., Wang, Y., Zhu, Y., Tang, J., Hu, B., Liu, L., et al. (2014). OsNAP connects abscisic acid and leaf senescence by fine-tuning abscisic acid biosynthesis and directly targeting senescence-associated genes in rice. Proc. Natl. Acad. Sci. U.S.A. 111, 10013–10018. doi: 10.1073/pnas.1321568111
Liang, J., Shen, Q., Wang, L., Liu, J., Fu, J., Zhao, L., et al. (2021). Rice contains a biosynthetic gene cluster associated with production of the casbane-type diterpenoid phytoalexin ent-10-oxodepressin. New Phytol. 231, 85–93. doi: 10.1111/nph.17406
Liang, X., Chen, X., Li, C., Fan, J., and Guo, Z. (2017). Metabolic and transcriptional alternations for defense by interfering OsWRKY62 and OsWRKY76 transcriptions in rice. Sci. Rep. 7:2474. doi: 10.1038/s41598-017-02643-x
Liu, H., Guo, Z., Gu, F., Ke, S., Sun, D., Dong, S., et al. (2017). 4-Coumarate-CoA ligase-like gene OsAAE3 negatively mediates the rice blast resistance, floret development and lignin biosynthesis. Front. Plant Sci. 7:2041. doi: 10.3389/fpls.2016.02041
Liu, J., Chen, X., Liang, X., Zhou, X., Yang, F., Liu, J., et al. (2016). Alternative splicing of rice WRKY62 and WRKY76 transcription factor genes in pathogen defense. Plant Physiol. 171, 1427–1442. doi: 10.1104/pp.15.01921
Liu, Q., Yan, S., Huang, W., Yang, J., Dong, J., Zhang, S., et al. (2018). NAC transcription factor ONAC066 positively regulates disease resistance by suppressing the ABA signaling pathway in rice. Plant Mol. Biol. 98, 289–302. doi: 10.1007/s11103-018-0768-z
Liu, S., Kracher, B., Ziegler, J., Birkenbihl, R. P., and Somssich, I. E. (2015). Negative regulation of ABA signaling by WRKY33 is critical for Arabidopsis immunity towards Botrytis cinerea 2100. Elife 4, e07295. doi: 10.7554/eLife.07295
Maeda, S., Dubouzet, J. G., Kondou, Y., Jikumaru, Y., Seo, S., Oda, K., et al. (2019). The rice CYP78A gene BSR2 confers resistance to Rhizoctonia solani and affects seed size and growth in Arabidopsis and rice. Sci. Rep. 9:587. doi: 10.1038/s41598-018-37365-1
Midoh, N., and Iwata, M. (1996). Cloning and characterization of a probenazole-inducible gene for an intracellular pathogenesis-related protein in rice. Plant Cell Physiol. 37, 9–18. doi: 10.1093/oxfordjournals.pcp.a028918
Mizutani, M., and Ohta, D. (2010). Diversification of P450 genes during land plant evolution. Annu. Rev. Plant Biol. 61, 291–315. doi: 10.1146/annurev-arplant-042809-112305
Nakashima, K., Tran, L. S., Van Nguyen, D., Fujita, M., Maruyama, K., Todaka, D., et al. (2007). Functional analysis of a NAC-type transcription factor OsNAC6 involved in abiotic and biotic stress-responsive gene expression in rice. Plant J. 51, 617–630. doi: 10.1111/j.1365-313X.2007.03168.x
Nelson, D., and Werck-Reichhart, D. (2011). A P450-centric view of plant evolution. Plant J. 66, 194–211. doi: 10.1111/j.1365-313X.2011.04529.x
Ng, D. W., Abeysinghe, J. K., and Kamali, M. (2018). Regulating the regulators: the control of transcription factors in plant defense signaling. Int. J. Mol. Sci. 19:3737. doi: 10.3390/ijms19123737
Noman, A., Liu, Z., Aqeel, M., Zainab, M., Khan, M. I., Hussain, A., et al. (2017). Basic leucine zipper domain transcription factors: the vanguards in plant immunity. Biotechnol. Lett. 39, 1779–1791. doi: 10.1007/s10529-017-2431-1
Nuruzzaman, M., Manimekalai, R., Sharoni, A. M., Satoh, K., Kondoh, H., Ooka, H., et al. (2010). Genome-wide analysis of NAC transcription factor family in rice. Gene 465, 30–44. doi: 10.1016/j.gene.2010.06.008
Nuruzzaman, M., Sharoni, A. M., and Kikuchi, S. (2013). Roles of NAC transcription factors in the regulation of biotic and abiotic stress responses in plants. Front. Microbiol. 4:248. doi: 10.3389/fmicb.2013.00248
Olsen, A. N., Ernst, H. A., Leggio, L. L., and Skriver, K. (2005). NAC transcription factors: structurally distinct, functionally diverse. Trends Plant Sci. 10, 79–87. doi: 10.1016/j.tplants.2004.12.010
Onohata, T., and Gomi, K. (2020). Overexpression of jasmonate-responsive OsbHLH034 in rice results in the induction of bacterial blight resistance via an increase in lignin biosynthesis. Plant Cell Rep. 39, 1175–1184. doi: 10.1007/s00299-020-02555-7
Ooka, H., Satoh, K., Doi, K., Nagata, T., Otomo, Y., Murakami, K., et al. (2003). Comprehensive analysis of NAC family genes in Oryza sativa and Arabidopsis thaliana. DNA Res. 10, 239–247. doi: 10.1093/dnares/10.6.239
Pandian, B. A., Sathishraj, R., Djanaguiraman, M., Prasad, P. V. V., and Jugulam, M. (2020). Role of cytochrome P450 enzymes in plant stress response. Antioxidants (Basel) 9:454. doi: 10.3390/antiox9050454
Park, S. R., Kim, H. S., Lee, K. S., Hwang, D. J., Bae, S. C., Ahn, I. P., et al. (2017). Overexpression of rice NAC transcription factor OsNAC58 on increased resistance to bacterial leaf blight. J. Plant Biotechnol. 44, 149–155. doi: 10.5010/JPB.2017.44.2.149
Peng, Y., Bartley, L. E., Canlas, P., and Ronald, P. C. (2010). OsWRKY IIa transcription factors modulate rice innate immunity. Rice (N Y) 3, 36–42. doi: 10.1007/s12284-010-9039-6
Peng, Y., Bartley, L. E., Chen, X. W., Dardick, C., Chern, M. S., Ruan, R., et al. (2008). OsWRKY62 is a negative regulator of basal and Xa21-mediated defense against Xanthomonas oryzae pv. oryzae in rice. Mol. Plant 1, 446–458. doi: 10.1093/mp/ssn024
Peng, Y., van Wersch, R., and Zhang, Y. (2018). Convergent and divergent signaling in PAMP-triggered immunity and effector-triggered immunity. Mol. Plant-Microbe Interact. 31, 403–409. doi: 10.1094/MPMI-06-17-0145-CR
Puranik, S., Sahu, P. P., Srivastava, P. S., and Prasad, M. (2012). NAC proteins: regulation and role in stress tolerance. Trends Plant Sci. 17, 369–381. doi: 10.1016/j.tplants.2012.02.004
Ribot, C., Hirsch, J., Balzergue, S., Tharreau, D., Nottéghem, J. L., Lebrun, M. H., et al. (2008). Susceptibility of rice to the blast fungus, Magnaporthe grisea. J. Plant Physiol. 165, 114–124. doi: 10.1016/j.jplph.2007.06.013
Shahnejat-Bushehri, S., Nobmann, B., Devi Allu, A., and Balazadeh, S. (2016). JUB1 suppresses Pseudomonas syringae-induced defense responses through accumulation of DELLA proteins. Plant Signal Behav. 11:e1181245. doi: 10.1080/15592324.2016.1181245
Shamsunnaher, Chen, X., Zhang, X., Wu, X. X., Huang, X., and Song, W. Y. (2020). Rice immune sensor XA21 differentially enhances plant growth and survival under distinct levels of drought. Sci. Rep. 10:16938. doi: 10.1038/s41598-020-73128-7
Shao, H., Wang, H., and Tang, X. (2015). NAC transcription factors in plant multiple abiotic stress responses: progress and prospects. Front. Plant Sci. 6:902. doi: 10.3389/fpls.2015.00902
Shen, Q., Pu, Q., Liang, J., Mao, H., Liu, J., and Wang, Q. (2019). CYP71Z18 overexpression confers elevated blast resistance in transgenic rice. Plant Mol. Biol. 100, 579–589. doi: 10.1007/s11103-019-00881-3
Shimono, M., Koga, H., Akagi, A., Hayashi, N., Goto, S., Sawada, M., et al. (2012). Rice WRKY45 plays important roles in fungal and bacterial disease resistance. Mol. Plant Pathol. 13, 83–94. doi: 10.1111/j.1364-3703.2011.00732.x
Shimono, M., Sugano, S., Nakayama, A., Jiang, C. J., Ono, K., Toki, S., et al. (2007). Rice WRKY45 plays a crucial role in benzothiadiazole-inducible blast resistance. Plant Cell 19, 2064–2076. doi: 10.1105/tpc.106.046250
Smita, S., Katiyar, A., Chinnusamy, V., Pandey, D. M., and Bansal, K. C. (2015). Transcriptional regulatory network analysis of MYB transcription factor family genes in rice. Front. Plant Sci. 6:1157. doi: 10.3389/fpls.2015.01157
Sun, H., Huang, X., Xu, X., Lan, H., Huang, J., and Zhang, H. S. (2012). ENAC1, a NAC transcription factor, is an early and transient response regulator induced by abiotic stress in rice (Oryza sativa L.). Mol. Biotechnol 52, 101–110. doi: 10.1007/s12033-011-9477-4
Sun, L. J., Huang, L., Hong, Y. B., Zhang, H. J., Song, F. M., and Li, D. Y. (2015). Comprehensive analysis suggests overlapping expression of rice ONAC transcription factors in abiotic and biotic stress responses. Int. J. Mol. Sci. 16, 4306–4326. doi: 10.3390/ijms16024306
Sun, L., Zhang, H., Li, D., Huang, L., Hong, Y., Ding, X. S., et al. (2013). Functions of rice NAC transcriptional factors, ONAC122 and ONAC131, in defense responses against Magnaporthe grisea. Plant Mol. Biol. 81, 41–56. doi: 10.1007/s11103-012-9981-3
Takeda, Y., Koshiba, T., Tobimatsu, Y., Suzuki, S., Murakami, S., Yamamura, M., et al. (2017). Regulation of CONIFERALDEHYDE 5-HYDROXYLASE expression to modulate cell wall lignin structure in rice. Planta 246, 337–349. doi: 10.1007/s00425-017-2692-x
Takeda, Y., Suzuki, S., Tobimatsu, Y., Osakabe, K., Osakabe, Y., Ragamustari, S. K., et al. (2019). Lignin characterization of rice CONIFERALDEHYDE 5-HYDROXYLASE loss-of-function mutants generated with the CRISPR/Cas9 system. Plant J. 97, 543–554. doi: 10.1111/tpj.14141
Talbot, N. J., Ebbole, D. J., and Hamer, J. E. (1993). Identification and characterization of MPG1, a gene involved in pathogenicity from the rice blast fungus Magnaporthe grisea. Plant Cell 5, 1575–1590. doi: 10.1105/tpc.5.11.1575
Tian, T., Liu, Y., Yan, H., You, Q., Yi, X., Du, Z., et al. (2017). agriGO v2.0: a GO analysis toolkit for the agricultural community, 2017 update. Nucleic Acids Res. 45, W122–W129. doi: 10.1093/nar/gkx382
Tran, L. S., Nakashima, K., Sakuma, Y., Simpson, S. D., Fujita, Y., Maruyama, K., et al. (2004). Isolation and functional analysis of Arabidopsis stress-inducible NAC transcription factors that bind to a drought-responsive cis-element in the early responsive to dehydration stress 1 promoter. Plant Cell 16, 2481–2498. doi: 10.1105/tpc.104.022699
Tsuda, K. M., and Somssich, I. E. (2015). Transcriptional networks in plant immunity. New Phytol. 206, 932–947. doi: 10.1111/nph.13286
Wang, L., Tsuda, K., Truman, W., Sato, M., Nguyen le, V., Katagiri, F., et al. (2011). CBP60g and SARD1 play partially redundant critical roles in salicylic acid signaling. Plant J. 67, 1029–1041. doi: 10.1111/j.1365-313X.2011.04655.x
Wang, Q., Hillwig, M. L., and Peters, R. J. (2011). CYP99A3: functional identification of a diterpene oxidase from the momilactone biosynthetic gene cluster in rice. Plant J. 65, 87–95. doi: 10.1111/j.1365-313X.2010.04408.x
Wang, Z., Xia, Y., Lin, S., Wang, Y., Guo, B., Song, X., et al. (2018). Osa-miR164a targets OsNAC60 and negatively regulates rice immunity against the blast fungus Magnaporthe oryzae. Plant J. 95, 584–597. doi: 10.1111/tpj.13972
Wani, S. H., Anand, S., Singh, B., Bohra, A., and Joshi, R. (2021). WRKY transcription factors and plant defense responses: latest discoveries and future prospects. Plant Cell Rep. 40, 1071–1085. doi: 10.1007/s00299-021-02691-8
Wei, K., and Chen, H. (2018). Global identification, structural analysis and expression characterization of cytochrome P450 monooxygenase superfamily in rice. BMC Genomics 19:35. doi: 10.1186/s12864-017-4425-8
Wu, A., Allu, A. D., Garapati, P., Siddiqui, H., Dortay, H., Zanor, M. I., et al. (2012). JUNGBRUNNEN1, a reactive oxygen species-responsive NAC transcription factor, regulates longevity in Arabidopsis. Plant Cell 24, 482–506. doi: 10.1105/tpc.111.090894
Yamamura, C., Mizutani, E., Okada, K., Nakagawa, H., Fukushima, S., Tanaka, A., et al. (2015). Diterpenoid phytoalexin factor, a bHLH transcription factor, plays a central role in the biosynthesis of diterpenoid phytoalexins in rice. Plant J. 84, 1100–1113. doi: 10.1111/tpj.13065
Yokotani, N., Sato, Y., Tanabe, S., Chujo, T., Shimizu, T., Okada, K., et al. (2013). WRKY76 is a rice transcriptional repressor playing opposite roles in blast disease resistance and cold stress tolerance. J. Exp. Bot. 64, 5085–5097. doi: 10.1093/jxb/ert298
Yokotani, N., Tsuchida-Mayama, T., Ichikawa, H., Mitsuda, N., Ohme-Takagi, M., Kaku, H., et al. (2014). OsNAC111, a blast disease-responsive transcription factor in rice, positively regulates the expression of defense-related genes. Mol. Plant Microbe Interact. 27, 1027–1034. doi: 10.1094/MPMI-03-14-0065-R
Yoshii, M., Shimizu, T., Yamazaki, M., Higashi, T., Miyao, A., Hirochika, H., et al. (2009). Disruption of a novel gene for a NAC-domain protein in rice confers resistance to Rice dwarf virus. Plant J. 57, 615–625. doi: 10.1111/j.1365-313X.2008.03712.x
Yoshii, M., Yamazaki, M., Rakwal, R., Kishi-Kaboshi, M., Miyao, A., and Hirochika, H. (2010). The NAC transcription factor RIM1 of rice is a new regulator of jasmonate signaling. Plant J. 61, 804–815. doi: 10.1111/j.1365-313X.2009.04107.x
Yuan, X., Wang, H., Cai, J., Li, D., and Song, F. (2019a). NAC transcription factors in plant immunity. Phytopathol. Res. 1:3. doi: 10.1186/s42483-018-0008-0
Yuan, X., Wang, H., Cai, J. T., Bi, Y., Li, D. Y., and Song, F. M. (2019b). Rice NAC transcription factor ONAC066 functions as a positive regulator of drought and oxidative stress response. BMC Plant Biol. 19:278. doi: 10.1186/s12870-019-1883-y
Zellerhoff, N., Jarosch, B., Groenewald, J. Z., Crous, P. W., and Schaffrath, U. (2006). Nonhost resistance of barley is successfully manifested against Magnaporthe grisea and a closely related Pennisetum-infecting lineage but is overcome by Magnaporthe oryzae. Mol. Plant-Microbe Interact. 19, 1014–1022. doi: 10.1094/MPMI-19-1014
Zhang, J., Coaker, G., Zhou, J. M., and Dong, X. (2020). Plant immune mechanisms: from reductionistic to holistic points of view. Mol. Plant 13, 1358–1378. doi: 10.1016/j.molp.2020.09.007
Zhang, Y., Xu, S., Ding, P., Wang, D., Cheng, Y. T., He, J., et al. (2010). Control of salicylic acid synthesis and systemic acquired resistance by two members of a plant-specific family of transcription factors. Proc. Natl. Acad. Sci. U.S.A. 107, 18220–18225. doi: 10.1073/pnas.1005225107
Keywords: NAC (NAM, ATAF, and CUC), ONAC066, rice immunity, OsWRKY62, cytochrome P450s
Citation: Yuan X, Wang H, Bi Y, Yan Y, Gao Y, Xiong X, Wang J, Li D and Song F (2021) ONAC066, A Stress-Responsive NAC Transcription Activator, Positively Contributes to Rice Immunity Against Magnaprothe oryzae Through Modulating Expression of OsWRKY62 and Three Cytochrome P450 Genes. Front. Plant Sci. 12:749186. doi: 10.3389/fpls.2021.749186
Received: 29 July 2021; Accepted: 20 August 2021;
Published: 09 September 2021.
Edited by:
Youfu “Frank” Zhao, University of Illinois at Urbana-Champaign, United StatesReviewed by:
Xiaochen Yuan, Michigan State University, United StatesCopyright © 2021 Yuan, Wang, Bi, Yan, Gao, Xiong, Wang, Li and Song. This is an open-access article distributed under the terms of the Creative Commons Attribution License (CC BY). The use, distribution or reproduction in other forums is permitted, provided the original author(s) and the copyright owner(s) are credited and that the original publication in this journal is cited, in accordance with accepted academic practice. No use, distribution or reproduction is permitted which does not comply with these terms.
*Correspondence: Fengming Song, Zm1zb25nQHpqdS5lZHUuY24=
Disclaimer: All claims expressed in this article are solely those of the authors and do not necessarily represent those of their affiliated organizations, or those of the publisher, the editors and the reviewers. Any product that may be evaluated in this article or claim that may be made by its manufacturer is not guaranteed or endorsed by the publisher.
Research integrity at Frontiers
Learn more about the work of our research integrity team to safeguard the quality of each article we publish.