- 1Jiangsu Key Laboratory of Crop Genetics and Physiology, College of Horticulture and Plant Protection, Yangzhou University, Yangzhou, China
- 2Co-innovation Center for Sustainable Forestry in Southern China, Jiangsu Province Key Laboratory of Soil and Water Conservation and Ecological Restoration, Nanjing Forestry University, Nanjing, China
- 3College of Mining Engineering, North China University of Science and Technology, Tangshan, China
- 4Institute of International Rivers and Eco-Security, Yunnan University, Kunming, China
Spartina alterniflora is a perennial herb native to the American Atlantic coast and is the dominant plant in coastal intertidal wetlands. Since its introduction to China in 1979, it has quickly spread along the coast and has caused various hazards. To control the further spread of S. alterniflora in China, we first reconstructed the history of the spread of S. alterniflora in its invasion and origin countries. We found that S. alterniflora spreads from the central coast to both sides of the coast in China, while it spreads from the west coast to the east coast in America. Furthermore, by comparing 19 environmental variables of S. alterniflora in its invasion and origin countries, it was found that S. alterniflora is more and more adaptable to the high temperature and dry environment in the invasion country. Finally, we predicted the suitable areas for this species in China and America using the maximum entropy (MaxEnt) model and ArcGIS. Overall, through analysis on the dynamic and trend of environmental characteristics during the invasion of S. alterniflora and predicting its suitable area in the invasion area, it guides preventing its reintroduction and preventing its further spread of the species has been found. It has reference significance for studying other similar alien plants and essential enlightening relevance to its invasion and spread in similar areas.
Introduction
Biological invasion is considered the second-largest factor leading to the reduction of biodiversity after habitat fragmentation (Vitousek et al., 1996; Wilcove et al., 1998). Plant invasion is an important branch of biological invasion research, which refers to the phenomenon where an alien plant is introduced into other new areas by human or natural factors; then, after some time, it can grow and reproduce wildly and threaten the local ecosystem, biodiversity, and even human health (Mack et al., 2000; Sakai et al., 2001; Richardson and Van Wilgen, 2004). Meanwhile, this plant is called an invasive plant. Invasive alien plants can quickly replace native species, change the structure and function of native communities, and affect ecosystem processes (Tilman, 2000; Raghubanshi and Tripathi, 2009). China is one of the critical victims of biological invasions (Raghubanshi and Tripathi, 2009; Zhu, 2012). China has more than 30 invasive species among the 100 most threatened invasive species around the world as announced by the International Union for Conservation of Nature (IUCN) in 2000 (Tilman, 2000; Luque et al., 2013). The plant invasion in China has the characteristics of rapid spread, a wide hazard area, and an increasing number of new invasive species, which has caused huge economic losses to Chinese agriculture, forestry, animal husbandry, and fishery (Ding et al., 2008). Statistics showed that China needs to invest a lot of money to control these invasive plants (Lin et al., 2007; Weber et al., 2008).
A series of global changes caused by human activities have brought extremely favorable conditions for introducing and invading invasive plants (Walther et al., 2009). Several studies have considered global change and biological invasion in a comprehensive manner. Among them, changes in atmospheric composition (such as increased concentrations of CO2, O3, etc.), climate change, and nitrogen deposition have pronounced and more direct effects on the invasion process of alien plants (Stachowicz et al., 2002; Bradley et al., 2009). Simultaneously, based on the different stages of biological invasion, global changes may affect biological invasion (Catford et al., 2012). For example, the increase in temperature has caused some alien species to successfully survive the winter in invading areas, and the mortality rate has been dramatically reduced (Walther et al., 2007; Kiritani, 2011). Furthermore, changes in the interrelationships between invading species, such as rising sea levels, increase the surrounding salinity, so invasive species with stronger salt-resistance and flooding resistance gradually become dominant in the inter-species competition process (Perry and Atkinson, 2009). In short, global change will not only promote pest invasion but also change its distribution pattern. The systematic investigation of the dynamics of the distribution of important invasive species in global climate change is of great significance for managing invasive species.
Spartina alterniflora Loisel. is a perennial herb native to the American Atlantic coast and is the dominant plant in coastal intertidal wetlands (Bertness, 1991; Feist and Simenstad, 2000; Ayres, 2004). It has a large root system, dense plant growth, and a good siltation-promoting effect. It can effectively slow down and control coastal erosion in its place of origin and provide rich food sources and habitats for fish, birds, and other animals (Simenstad and Thom, 1995). S. alterniflora has an excellent ability to promote the land formation, countries worldwide have introduced and planted it. It has become one of the most successful invasive plants in the estuarine salt marsh wetland ecosystem. Furthermore, S. alterniflora has strong adaptability and spreading ability (Daehler and Strong, 1994, 1996), and it is widely distributed in wetlands along the beach. At present, its distribution area has expanded from the Atlantic coast of North and South America to Europe, the west coast of North America, New Zealand, and the coast of China (Baumel et al., 2001), resulting in changes in the structure and function of the gulf ecosystem (Simenstad and Thom, 1995). In the areas invaded by S. alterniflora, local species are repelled, with strong inter-species competitiveness. It rapidly spreads to occupy the empty niche in the intertidal zone (Feist and Simenstad, 2000), resulting in the reduction of the beach area and the change of ecological environment, thereby reducing the distribution number and species of benthic organisms (Dumbauld et al., 1995), changing the original habitats of birds, and affecting the diversity of local birds (Evans, 1986). The proliferation of S. alterniflora in the invaded area has directly or indirectly reduced the biodiversity of the invaded area, which has brought significant losses to local biodiversity protection management and economic development (Chen et al., 2004; Chung et al., 2004; Li and Zhang, 2008). In particular, S. alterniflora was introduced into coastal wetlands of the China as an ecological project in 1979. It has strong adaptability in the coastal wetlands of the China, strong inter-species competition ability, rapid diffusion ability, and transmission power mechanism. Now, it is widely distributed in the coastal wetlands in China (Huang et al., 2008; Li and Zhang, 2008). Therefore, the large-scale spread of S. alterniflora in the coastal wetlands of China has caused losses to local environmental protection and economic development and has become one of the most invasive species in the coastal wetlands of China.
Biological invasion is essentially a dynamic process of species distribution (Dormann et al., 2012). The entire process of biological invasion is closely related to expanding the distribution area of alien species. The study of the dynamic diffusion of its distribution area has always been a critical issue in biological invasion. Therefore, more and more studies have been conducted on the historical patterns and potential ecologically suitable distributions of alien biological invasions (Salo, 2005; Wang and Wang, 2006). These studies further explore the possible routes of their spread from the reconstruction process of the history of alien species invasions. It is helpful to formulate effective prevention and control measures through the dynamic historical reconstruction of alien species distribution areas and the prediction of the spatiotemporal diffusion characteristics of species in potential distribution areas. As the niche shift of species changes with time and space, the phenomenon of a niche shift in alien species is particularly prominent (Müller-Schärer et al., 2004; Eastwood et al., 2007). Therefore, before predicting the potential ecologically suitable distributions of alien species, it is first necessary to determine whether the niche of the alien species in the invaded area has changed (Mitchell and Power, 2003; Dietz and Edwards, 2006). The current methods for the quantitative assessment of a niche overlap are increasingly becoming widely used in studying species niche changes over time and space, such as in Nylanderia fulva and Pueraria montana (Callen and Miller, 2015; Kumar et al., 2015). Therefore, studying the niche of the S. alterniflora origin and invasion countries is helpful to grasp the factors and thresholds that affect its distribution, as it is important to predict the potential distribution of S. alterniflora.
The species distribution model (SDM) is a modeling method that predicts the potential distribution of species based on the niche theory (Gelviz-Gelvez et al., 2015; Moor et al., 2015). There are many statements about the definition of a niche, the most famous of which is the multi-dimensional hypervolume niche model proposed by Hutchinson in 1957. In this study, it has been found that organisms in the environment result from the combined action of multiple environmental resource factors. Species have a specific suitable range for each environmental element, and the adaptation range of all ecological factors constitutes the ecological niche of the species in the environment (Perutz, 1967). Among the many species distribution models (Bioclim, Climex, Domain, Garp, and MaxEnt), the maximum entropy (MaxEnt) model is a density estimation and distribution prediction model based on the maximum entropy theory (Elith et al., 2006; Phillips et al., 2009). Its simulation accuracy is higher than other models, its prediction effect is better, and it is widely used (Phillips et al., 2009). The MaxEnt model calculates a prediction model based on the current distribution points of the species and the current climate data and then uses this model to simulate the distribution of the species in future climate states. Niche conservation is a theoretical premise that makes the simulation of species distribution in future climate valuable (Petitpierre et al., 2012). The ecologically suitable distributions for the most invasive species have been predicted using the MaxEnt model (O'donnell et al., 2012; Kelly et al., 2014).
At present, the research carried out on S. alterniflora has mainly focused on biology, ecological characteristics, competitive substitution mechanisms, and chemical control. However, it is not clear how S. alterniflora is introduced into China and its spread route and speed. In this study, we used MaxEnt modeling and bioclimatic data to characterize the niche shift of S. alterniflora between native and non-native countries. Our objectives were to: (1) understand the diffusion regularity of S. alterniflora in China and America and the stage of invasion in China; (2) compare the niche of S. alterniflora in the invasion and origin countries; (3) map the potential suitable distribution of S. alterniflora in China and America.
Materials and Methods
Species Occurrence and Reconstruction Historical Expansion Process of S. alterniflora
The distribution data for S. alterniflora in China were derived from the Global Biodiversity Information Facility (GBIF) database (http://www.gbif.org) and China Digital Herbarium (CVH, http://www.cvh.ac.cn/), supplemented with recent field surveys and literature records from China National Knowledge Infrastructure (http://www.cnki.net/) and Google Scholar. The distribution data for S. alterniflora in America were derived from the GBIF database (http://www.gbif.org) and literature records from Google Scholar. Duplicate latitude and longitude specimen information with unknown geographic coordinates were removed, and then the plant catalog of the sample plots was checked. For the available records that lacked latitude and longitude information, we used Google Earth to query the latitude and longitude information corresponding to the sample plots according to the place names. In this study, the limited number of distribution points were retained within a certain distance according to the spatial screening method, and systematic sampling was performed again according to the principle of uniformity and randomness to ensure that the geographic autocorrelation of the data points is minimized (Tang et al., 2021a). The distribution information of S. alterniflora in China (113 points) and in America (287 points) can be obtained and saved as a.csv file for later use. The climate variable data for this study were downloaded from the WorldClim database (http://www.worldclim.org/) from 1950 to 2000. We used the 19 bioclimatic variables at 30 arc seconds (about 1 km2) (Hijmans et al., 2005). The bioclimatic variables are shown in Table 1.
The administrative division maps of China and America were downloaded from the National Science and Technology Infrastructure Platform (http://www.geodata.cn/). We used the geographic information system software ArcGIS to map the space-time diffusion process of S. alterniflora in the invading area of China and the country of origin, America.
Selection and Comparison of Climate Variables
The results of the niche analysis and the prediction of the final ecologically suitable distributions directly impacted the selection of environmental variables. Therefore, the selection of appropriate environmental variables was a key link in the analysis of climate niches and the prediction of ecologically suitable distribution areas (Holm, 1979). To remove the high correlation between variables and reduce the over-fitting of the model, 19 environmental variables were extracted from all distribution points, and the correlation functions (ecospat.cor.plot, vifstep, princomp), and performed a correlation analysis (Pearson correlation coefficient, | r | ≥ 0.8). Meanwhile, we loaded 19 bioclimatic variables and species distribution points into the MaxEnt model and performed a modeling analysis based on default parameters. The contribution of the environmental variables was obtained from the MaxEnt model with default parameters. According to correlation analysis and the variable contribution rate, the variables with a small contribution in the high-correlation variable group should be removed (Li et al., 2020). In addition, the biological significance of the variables was fully considered. This process resulted in a set of environmental data containing seven environmental variables (bio2, bio3, bio5, bio8, bio12, bio14, and bio16) for America and six environmental variables (bio1, bio2, bio9, bio15, bio18, and bio19) for China. Note that, when studying the niche characteristics of an alien species during the invasion process, we usually choose to fix the native niche as a reference.
To roughly judge the similarities of the climatic conditions of China and the USA, we used SPSS 25 (IBM, Armonk, New York, USA) to compare the mean values of the 19 bioclimatic variables with a two-factor ANOVA without replication. We approximately distinguished the similarities and differences of the climatic conditions between the native and invasive countries. We used a bootstrapping procedure to determine whether differences in sample size affected univariate comparisons (Lee and Rodgers, 1998). We randomly sampled the same number of invasion distribution samples as the distribution points of origin and recalculated the mean and variance for each variable. This process was repeated 1,000 times, yielding a 95% CI for each environmental variable (a = 0.05) (Holm, 1979). The results of the comparison between the native and invasive countries were presented using a violin diagram.
Bioclimatic Niche Shift
The distribution data in this study showed the current geographic distribution of S. alterniflora in China and America. Based on the geospatial distribution data of S. alterniflora, to make the selected background data reflect the actual distribution of the species, we used R and the ArcGIS 10.5 mapping software (Environmental Systems Research Institute, ESRI, Redlands, California, United States) R language 3.6.1 to apply the kernel density distribution map along with the diffusion rate of S. alterniflora. Around each distribution point, a buffer zone with a diameter of 1.5° was selected, and 10,000 points in each province buffer zone were chosen as its background data. Then, we extracted the climate data for each background point. The “ecospat” package was used to perform a principal component analysis (PCA)-env in R language.
We used the species niche climate spatial comparison method constructed by Broennimann to compare the niche characteristics of S. alterniflora in the countries of origin and invasion (Broennimann et al., 2012). The selected six available bioclimatic variables in America (bio2, bio3, bio5, bio8, bio12, bio14, and bio16) were used to perform the PCA-env method implemented by Broennimann. In addition, the quantified niche of a species in climate space was represented by some niche indices, such as the overlap index D, niche equivalence, and niche similarity. The niche overlap index D represented the overlap rate of species niche between two regions (Schoener, 1970), and its value ranged from 0 (the niche of the two regions does not overlap at all) to 1 (the niche of the two areas overlap entirely). The test of niche equivalence was conducted by collecting all distribution points, randomly reselecting distribution points equal to the initial number of origin and invasion sites in two regions, respectively, and calculating the D value. The random calculation was repeated 100 times, and the statistical test was performed for the niche overlap index D value (a = 0.05). The niche similarity test evaluated whether the degree of overlap between the observed niche in two regions is higher than the overlap between the experimental niche in one area and a randomly selected niche in the other region. The calculation was repeated 100 times randomly. Through the analysis of the niche stability, unfilled and expansion indicators in the entire background environment and the marginal climate of 75–100% background environment were found. This method automatically removed the sampling bias through a smooth kernel density function (Broennimann et al., 2012).
Each species was modeled to 10 times, and an average niche model was used to generate the predicted niche occupancy (PNO) profiles following Evans et al. (Evans et al., 2009). These profiles were estimated using single bioclimatic variables that summarize the annual conditions of the habitats of the species. We used the selected variables of America: mean diurnal range (Bio2), isothermality (Bio3), the maximum temperature of the warmest month (Bio5), mean temperature of the wettest quarter (Bio8), annual precipitation (Bio12), precipitation of driest month (Bio14), and precipitation of wettest quarter (Bio16). These analyses were performed with the R package ecospat.
Predicting Ecologically Suitable Distributions of S. alterniflora in China and America
Based on the environmental variables of the actual distribution of S. alterniflora in China and America, the MaxEnt model was used to analyze the species niche demand and predict its potential distribution area (Yang et al., 2013). The specific operation method is as follows: First, the distribution occurrence data and selected environmental variables were loaded into the MaxEnt model. To estimate the capacity of the modeling, the running parameters were set to randomly select 25% of the distribution points as the test data and 75% of the distribution points as the training data for a model accuracy analysis. The maximum number of iterations is 500. Feature combination (FC) and regularization multiplier (RM) were optimized through calling the ENMeval packet in the R software, and other parameters were set as the default (Muscarella et al., 2014). There were many ways to divide ecological habitat suitability areas. The ecological habitat suitability areas of S. alterniflora were divided into four levels, namely, low habitat suitability (existence probability is <0.3), medium habitat suitability (existence probability is 0.3–0.5), high habitat suitability (existence probability is 0.5–0.7), and excellent habitat suitability (existence probability is >0.7).
The receiver operating characteristic (ROC) curve of the knife-cut method is an analytical method to evaluate the reliability of the model (Dorfman et al., 1992). The area under the ROC curve (AUC) is a widely used metric in the evaluation of species distribution models (Townsend Peterson et al., 2007; Jiménez-Valverde, 2012). The AUC is generally in the range of 0.5 to 1. The larger the value, the more accurate the prediction. Usually, a value between 0.9 and 1 is very good (Swets, 1988). For future climate projections, we used two representative concentration pathways (RCPs) of the IPCC-RCP 2.6 and 8.5 (GCM: BCC-CSM2-MR) for two future periods, 2050 and 2070. To explore the direction of the core distributional shifts between current and future ranges, we summarized the distribution to a single point, the centroid of suitable distribution (Vanderwal et al., 2013; Brown, 2014). We determined the migration direction of the geographical centroids for S. alterniflora under different RCP conditions and different periods by ArcGIS 10.4.1 (Yu et al., 2019; Tang et al., 2021b).
Results
The Historical Expansion Process of S. alterniflora
Spartina alterniflora was produced on the southeastern coast of America. It was introduced to China as early as 1979 and then spread to the whole country. After more than 30 years of development, S. alterniflora has spread throughout the coastal areas of China. It can be seen from Figures 1A–D that S. alterniflora extends from the central coast to both sides of the coast in China, while it extends from the west coast to the east coast in America (Figures 1E–H). From the expansion trend of the two countries, S. alterniflora grows along the coastline and is the dominant plant in the coastal intertidal zone.
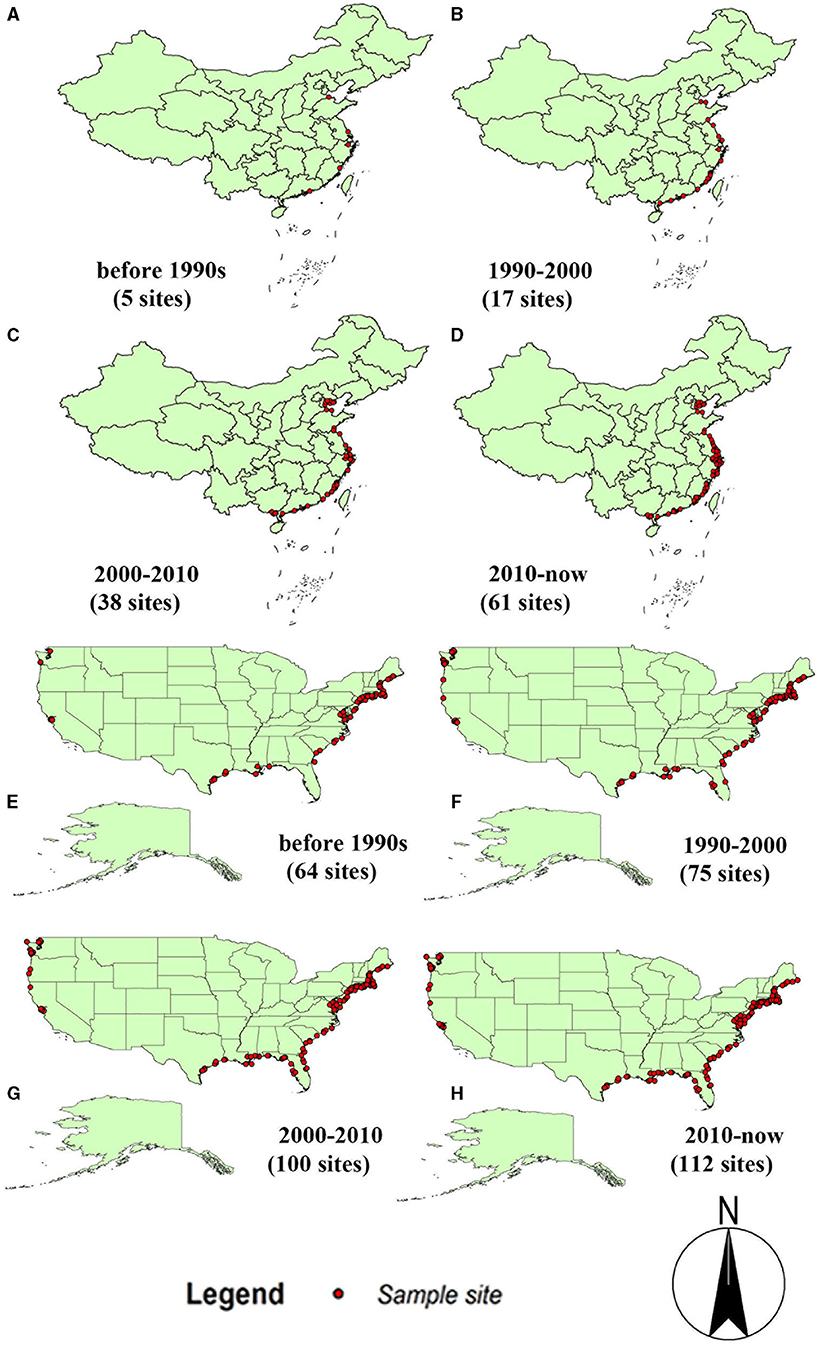
Figure 1. The dynamic map of Spartina alterniflora in China and America. (A) Before the 1990s in China; (B) 1990–2000 in China; (C) 2000–2010 in China; (D) 2010–now in China; (E) before the 1990s in America; (F) 1990–2000 in America; (G) 2000–2010 in America; (H) 2010–now in America.
When invasive alien species enter a new area and period after establishing the population, there is often little or no spread. This stage is called the lag period. After the lag period, the distribution area of invasive species will spread on a large scale. Only when a natural barrier (such as mountains, oceans, etc.) appears in the space where the population spreads, the spread will slow down and gradually approach a specific level, which has reached the saturation period (Shigesada and Kawasaki, 1997). The S. alterniflora spread in China satisfies this viewpoint. Prior to the year 2000, S. alterniflora had been in a lag period, and after 2000, it showed a large-scale spread. In Figures 1B–D, it can be seen that S. alterniflora occurred in 17 sites in China from 1990 to 2000, and its occurrence increased to 38 sites from 2000 to 2010, and up to 61 sites from 2010 to now.
Direct Comparison of Environmental Variables Between Origin and Invasion Countries
As shown in Figure 2, the average values of the 19 environmental variables of S. alterniflora in the origin and invasion areas are significantly different. Compared with the climatic variables of the origin and invasion areas, all climate variables except bio4, bio7, and bio12 have changed significantly (P < 0.001). The annual average temperature (bio1) of S. alterniflora in China is 5.083°C, which is higher than that of the country of origin, and the adaptable temperature of S. alterniflora in the invasion country is higher than that in the origin during the coldest year. Compared with the origin country, the minimum temperature of the coldest month (bio6) and mean temperature of the coldest quarter (bio11) increased by 5.555 and 4.393°C in the invasion country, respectively. At the hottest time of the year, S. alterniflora can adapt to higher temperatures than the place of origin, wherein the maximum temperature of the warmest month (bio5), the mean temperature of the wettest quarter (bio8), and the mean temperature of the warmest quarter (bio10) of the invasive population were 3.853, 3.561, and 5.497°C higher than those in the origin country, respectively. When the precipitation is the lowest in the year, the S. alterniflora invasion country can adapt to the drier environment compared with the origin country, wherein the humidity of the invasion country is 1.92–2.32 times lower than the origin in the precipitation of the driest month (bio14), the precipitation of the driest quarter (bio17), and the precipitation of the coldest quarter (bio19). Overall, S. alterniflora is more and more adapted to the high temperature and dry environment in the invasion country.
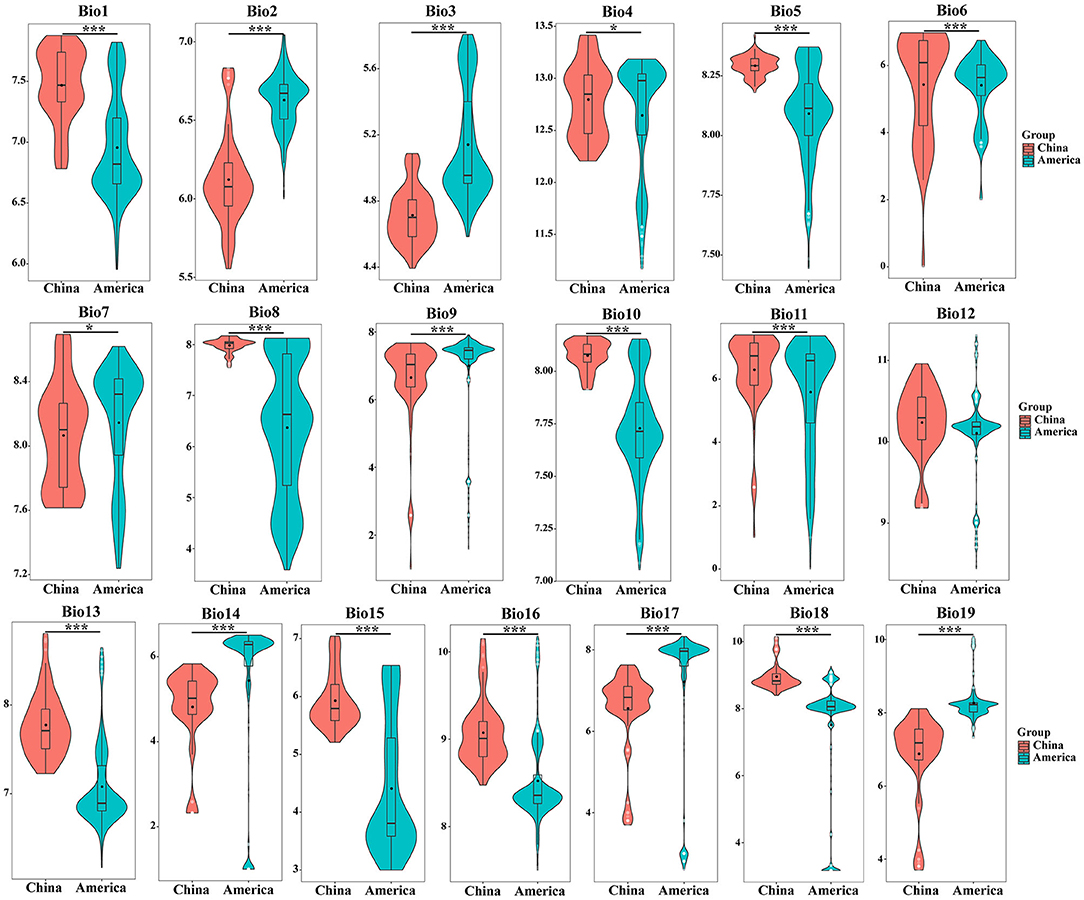
Figure 2. The comparison of S. alterniflora environmental variables between origin and invasion countries. The asterisk shows the level of significance between environmental variables in China and America. * means P < 0.05, ** means P < 0.01, *** means P < 0.001.
Climatic Niche Overlap, Equivalency, and Similarity
The results of the comparative analysis of the climatic niche of S. alterniflora in the invasion country, China, and the origin country. America, are shown in Figures 3, 4. The PCA results of the climate variables selected by the MaxEnt model showed that the first two principal components can explain 59.42% of each parameter variable (PC1 = 32.92%, PC2 = 26.5%), and the realized climatic niche of S. alterniflora may have shifted and expanded in the invasive regions examined. The first axis (32.92%) was best explained by a negative correlation with climate variations, while a positive correlation best explained the second axis (26.5%) with climate variations. The direction of the center shift between the climate niche of the origin country, America, and the invasion country, China, is different from the background climate change of its two regions, indicating that the actual distribution of S. alterniflora in China and America occupies a more significant proportion than that of the origin country, as the center of the realized climatic niche moved toward warmer temperatures and lower humidity. There was only a 0.4% niche overlap between native and invaded ranges. The PNO profiles indicated differences in the climatic requirements of S. alterniflora between native and non-native countries (Figure 4). The potential distribution of S. alterniflora in the invaded range showed more excellent suitability in drier areas (Figures 4E,F), with a small mean diurnal range (Figure 4B). On the contrary, the potential distribution in the native range noted more excellent suitability in wetter environments.
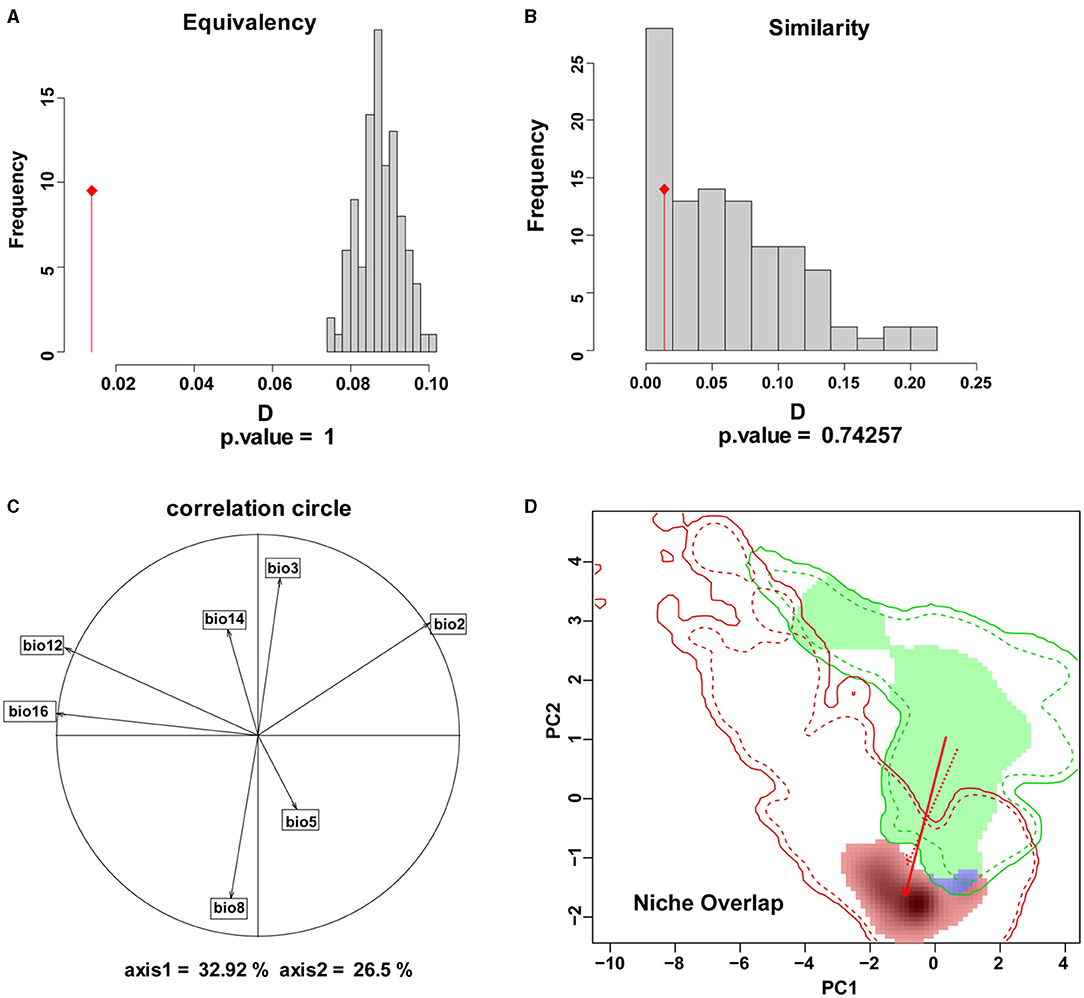
Figure 3. The niches of S. alterniflora in China and America under climatic ecological space. (A) Represents the contribution of each variable to the principal component axis, (B, C) represent the histograms representing the niche equivalence (D) test and niche similarity test of the two regions. (D) Indicates the overlapping of the species origin and invasion countries. Blue indicates niche overlap, green represents unfilling, and red represents expansion.
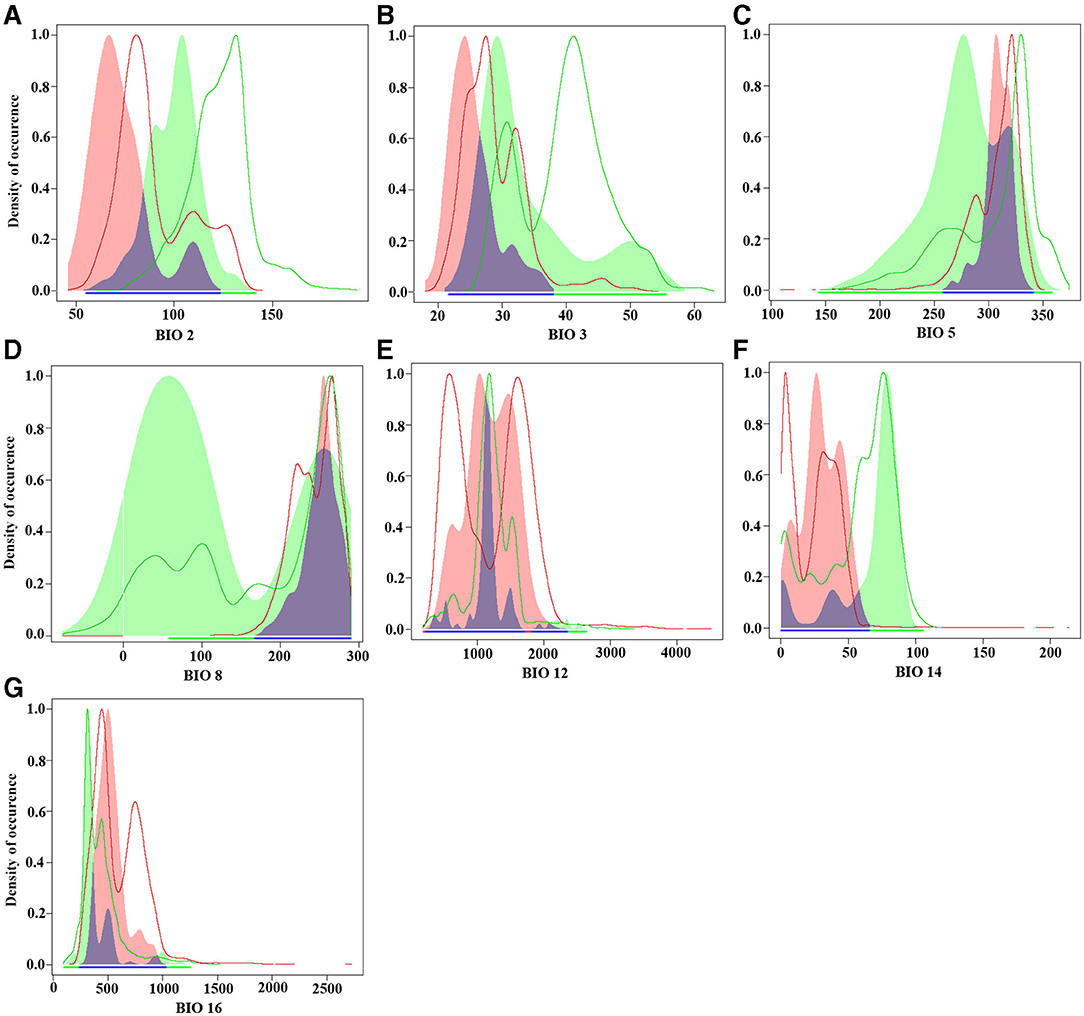
Figure 4. Predicted niche occupancy (PNO) profiles. Blue indicates niche overlap, green represents the origin country, and red represents the invasion country. (A) Mean diurnal range (Bio2); (B) isothermality (Bio3); (C) max temperature of the warmest month (Bio5); (D) mean temperature of the wettest quarter (Bio8); (E) annual precipitation (Bio12); (F) precipitation of the driest month (Bio14); (G) precipitation of the wettest quarter (Bio16).
The niche overlap indicates the similarity of the use of different environmental resources by the same species: the size of the niche overlap reflects the similarity of plant utilization resources; a large niche overlap indicates that their ecological requirements are close, the utilization of resources is similar, and the biological characteristics are similar under certain environmental conditions. However, the niche overlap between China and America is only 0.4%, which indicates that there is a significant difference between the native and invaded niches of S. alterniflora. The biological characteristics of S. alterniflora are not similar in the two countries, the requirements for the ecological environment are also not close, and the niche shift is significant.
Potential Ecologically Suitable Distribution of S. alterniflora in Invasion and Origin Countries Under Current Climate Conditions
The area under the ROC curve of the MaxEnt model has an AUC value of 0.981, indicating that the constructed model has a good predictive ability and can reasonably predict the potential habitat of S. alterniflora in China and America. The predicted value of the model is divided according to the operation data to generate the ecologically suitable level distribution map. Red indicates the area that is the most suitable for S. alterniflora to grow. As shown in Figure 5A, the potential ecological suitable distribution of S. alterniflora in the United States is mainly distributed on the east and west coasts. The northeast coast along the Gulf of St. Lawrence and the southern Mexico Gulf and a small amount of the California coast exist as the most suitable distribution areas. Suitable distribution areas also exist along the coasts of Washington and Oregon. As shown in Figure 5B, the prediction results of S. alterniflora in the ecological suitable distribution of China show that, under current climatic conditions, the Jiangsu, Shanghai, Zhejiang, and Fujian coastal areas and the northern Taiwan provinces all have ecological distribution areas most suitable for S. alterniflora.
Potential Ecologically Suitable Distribution of S. alterniflora in Invasion and Origin Countries Under Future Climate Conditions
The analysis and prediction of the potential suitable distribution of S. alterniflora under the two greenhouse gas emission scenarios (RCP 2.6 and RCP 8.5) in the 2050s and 2070s are shown in Figure 6. In China and America, under the greenhouse gas emission scenarios of RCP 2.6 and RCP 8.5, the ecologically suitable distribution areas for S. alterniflora will be mainly concentrated in coastal areas. By 2070, the suitable area of S. alterniflora in the inland areas of China will increase and be concentrated in the Sichuan–Chongqing junction and Hunan provinces. Under RCP 2.6, the ecologically suitable distribution areas of S. alterniflora in China are growing slowly until 2050, and will be rapidly growing to 2070. Under RCP 8.5, the ecological suitable distribution areas of S. alterniflora in China are expected to grow slowly from now to 2070. Furthermore, S. alterniflora ecological suitable distribution areas are relatively rare in America, only existing in the junction of Wisconsin and Iowa under RCP 2.6 in 2050. From the changes in the area of the invasion and origin countries, even if the area of the invasion country increases, it will always be smaller than the area of the country of origin. The trends of the area increase and decrease of the invasion country, China, and the origin country, America, are consistent (Table 2). The change of centroids reflects the directional shift of future ranges (Figure 7). Under different climate change scenarios, the direction changes of S. alterniflora are simulated to be quite different. In America, its range is expected to shift to the southeast under RCP 2.6 and RCP 8.5. In China, its range is expected to change to the north under RCP 2.6, but under RCP 8.5, the centroids shift to the east and then shift to the west.
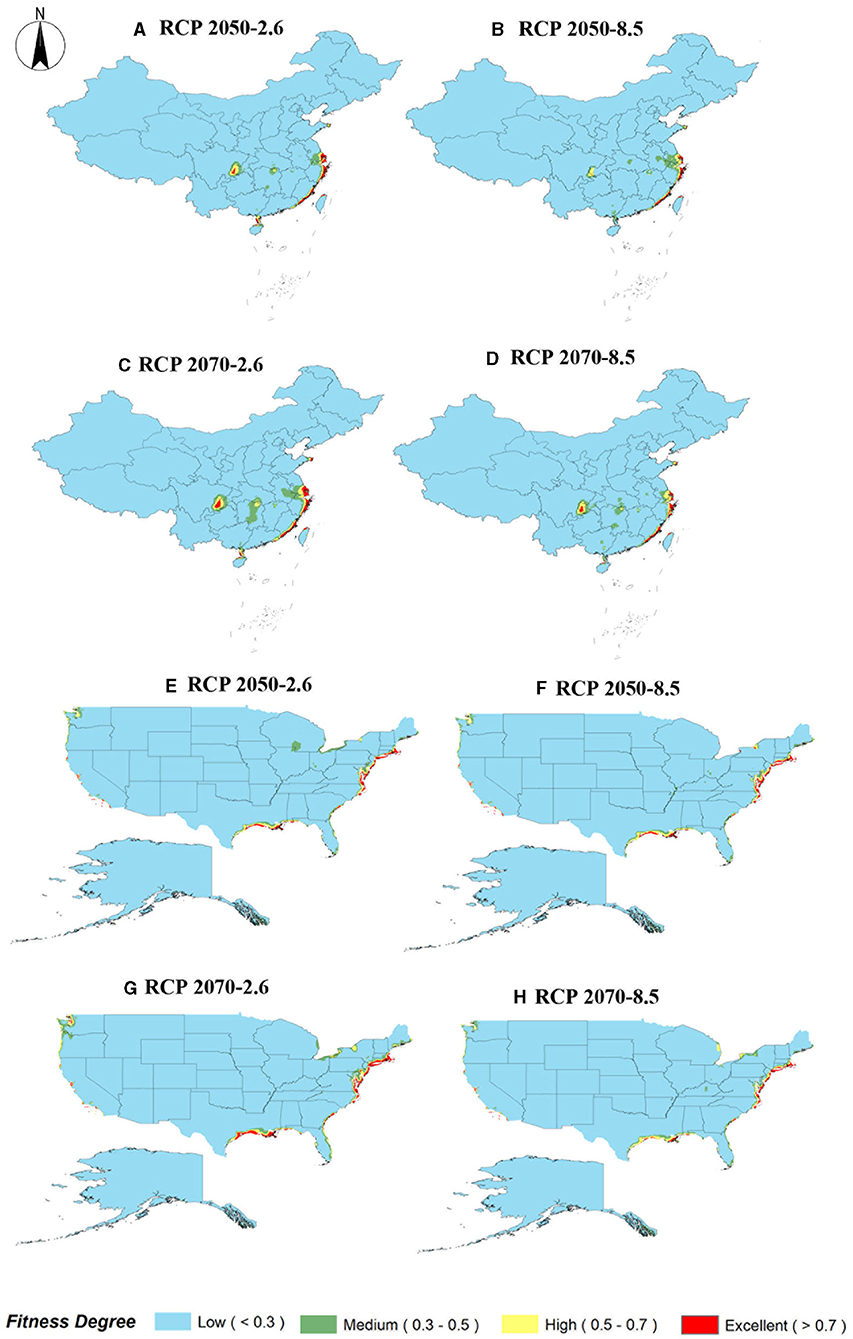
Figure 6. Future species distribution models of S. alterniflora in China and America under different climate scenarios predicted by MaxEnt. (A–D) RCP 2050-2.6 in China; RCP 2050-8.5 in China; RCP 2070-2.6 in China; RCP 2070-8.5 in China; (E–H) RCP 2050-2.6 in America; RCP 2050-8.5 in America; RCP 2070-2.6 in America; RCP 2070-8.5 in America.
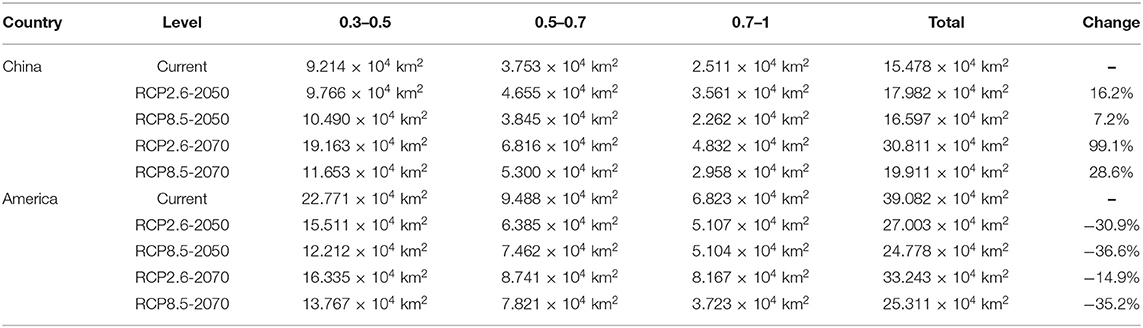
Table 2. The total area in China and America with S. alterniflora ecological suitable distribution levels > 0.3 under RCP 2.6 and 8.5.
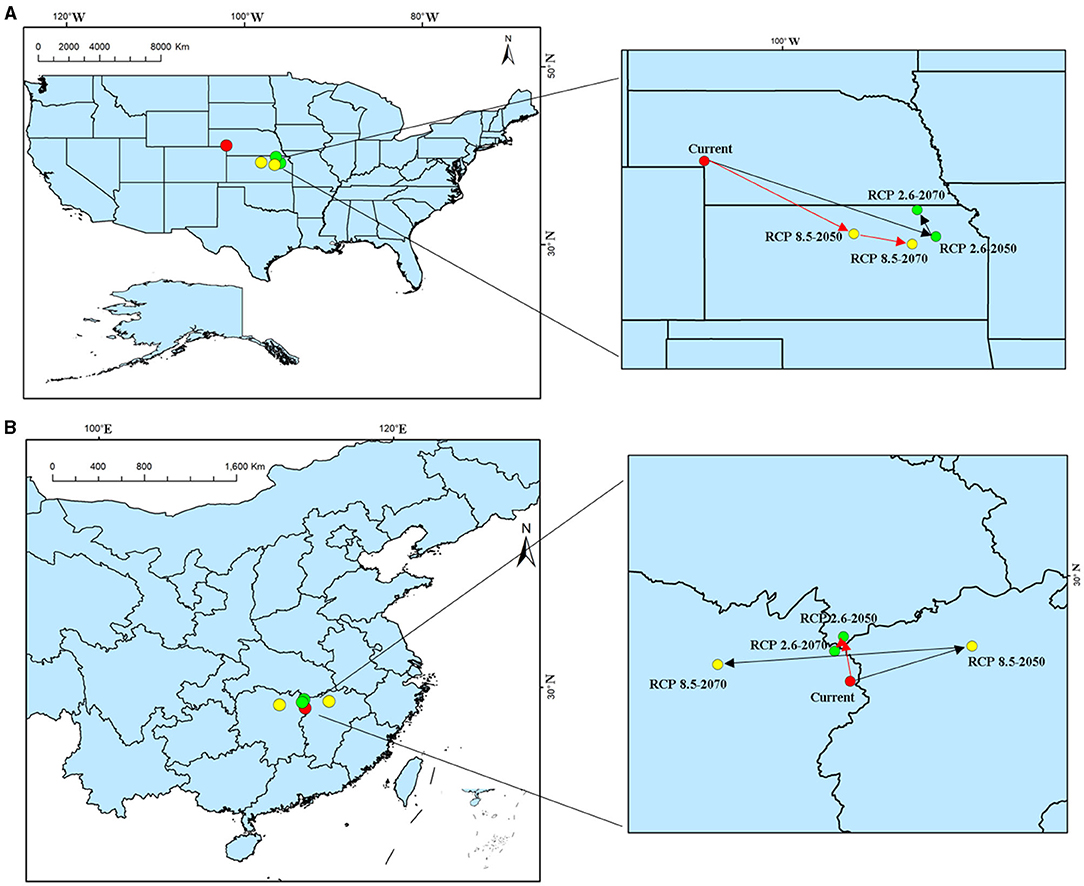
Figure 7. Geometric central migration of S. alterniflora habitat suitability areas in 2050 and 2070 under two distinct climate change scenarios. (A) America; (B) China.
Discussion
Spartina alterniflora was introduced to China in 1979 and then spread rapidly along the coastal beach. So far, the distribution of S. alterniflora spans 19 latitudes, from Liaoning in the north to Guangdong and Guangxi in the south (An et al., 2007; Zuo et al., 2012). China has become the country with the largest outbreak of S. alterniflora. The extensive spread of S. alterniflora has threatened the coastal wetland ecosystem of China. According to the S. alterniflora invasion and diffusion dynamics, the S. alterniflora spread in China can be divided into two stages: The first stage was from 1979 to 2000, which was the sporadic stage of S. alterniflora. Spartina alterniflora was concentrated in the coastal areas of Jiangsu, Zhejiang, and Fujian with earlier invasion times. At this stage, S. alterniflora invaded, successfully colonized, and spread to the surrounding areas of the invaded area. The second stage is from 2000 to the present. It is the rapid diffusion stage of S. alterniflora, showing a gradual spread from the coastal areas to the coastal provinces, and the distribution range gradually expanded.
The S. alterniflora invasion and spread pattern in China is from the central coast to the north–south coast. It may be because S. alterniflora was first introduced in the Fujian province, and gradually spread from the middle to the north and south. According to the literature, S. alterniflora has a robust sexual reproduction ability in North America, which is related to its geographical distribution and plays an important role in population spread and invasion (Callaway and Josselyn, 1992; Plyler and Carrick, 1993). In addition, S. alterniflora seeds have extremely strong salt tolerance characteristics and maintain high activity after soaking in 2–4°C seawater for 3 months, and their germination ability reaches the maximum (Mooring et al., 1971). This is an excellent adaptation to the salinity environment of the coastal wetland, which increases the opportunities for competition and spread and the invasion and spread of populations (Wijte and Gallagher, 1996). Studies in Willapa and San Francisco Bay in the United States have confirmed that the establishment and spread of S. alterniflora populations in this area are mainly through the propagation and spread of seeds. The propagation of germs is the most essential way for S. alterniflora populations to spread (Daehler, 1999; Davis et al., 2004).
Using S. alterniflora environmental variable data in China to compare with the origin country in America, it is found that the niche overlap between the climate niche of the origin country and the niche of the invasion country is small, indicating a relative lack of analog climates. Therefore, the species in the invaded area occupy non-native niches because the background in China is different than in America. The stability value reflects the conservation of the ecological niche of the species, while the expansion value reflects the new environment occupied by species (Broennimann et al., 2014; Callen and Miller, 2015). During the invasion of China, its niche needed to be changed significantly in the climate space. It shows that the niche demand of S. alterniflora in the invasion country has changed dramatically. As it is introduced into China, its niche demand is significantly different from the origin in the climate space. During the invasion process, several factors will lead to a niche shift. In turn, these factors will promote the colonization of new combinations of environmental conditions, causing species to exceed their native niche limits and occupy different non-native niches (Nunes et al., 2015). These factors include abiotic differences, biological interactions, decentralized barriers, or human activities (Wan et al., 2017). Climate differences can play an important role in ecological differences by promoting species to adapt to the new climate environment (Graham et al., 2004; Rissler and Apodaca, 2007).
The geographical and climatic conditions of the invaded areas and their spread patterns can often be used as an essential reference for predicting the suitable habitat of alien invasive plants (Kriticos and Randall, 2001). A species can cause the same damage in two places with very similar ecological conditions, so the case for the species itself is the best predictor. To predict the potential distribution of an alien species, it is necessary to analyze their current distribution and the climatic and environmental conditions of the invasion area (Kriticos and Randall, 2001). Therefore, this study attempted to predict the potential distribution of S. alterniflora in China and America by using the niche model software based on the invasion and diffusion patterns of S. alterniflora during historical reconstruction.
Based on the data obtained from specimen records and literature reports, we obtained the potential distribution area of S. alterniflora in China using the MaxEnt model. It can be seen that S. alterniflora is suitable for growing in coastal areas. The range of suitable habitats currently distributed in China is smaller than that predicted by MaxEnt. Therefore, S. alterniflora has room and possibility to continue to spread in China. However, the predictions in the United States were different. Regardless of whether they were under RCP 2.6 or RCP 8.5, S. alterniflora was predicted to have a smaller suitable area in the United States than currently; therefore, it is less likely that S. alterniflora will continue to expand in the United States. Climate is an essential factor affecting the distribution of S. alterniflora on a large scale. Spartina alterniflora exhibits different adaptive characteristics under different latitudes and climatic conditions, and its distribution range also changes (Zhao et al., 2015). This showed that S. alterniflora has strong climate adaptability. Therefore, S. alterniflora could still have a significant expansion trend in China in the case of small niche overlap between China and the United States.
Conclusions
Our study provided strong evidence of a shift in the realized climatic niche of S. alterniflora during its spread through China. This study analyzed the primary process and the earliest invasion area of S. alterniflora in China by reconstructing the historical process of its spread. Furthermore, we compared the climate adaptability of the S. alterniflora origin and invasion countries and analyzed the climate adaptability characteristics of S. alterniflora after it invaded China. Finally, based on the climate adaptability of S. alterniflora in China and its climatic adaptability during the invasion, the MaxEnt model and ArcGIS were used to predict its suitable ecological distribution in China and America, and monitoring and early warning were conducted to control the further spread of S. alterniflora in China. The results showed that S. alterniflora spreads from the middle coast to both sides of the coast in China, while it spreads from the west coast to the east coast in America. By comparing 19 environmental variables of S. alterniflora in the invasion and origin countries, it was found that S. alterniflora is more and more adaptable to the high temperature and dry environment in the invasion country. Moreover, a comparison between the niches of China and America found that the niche overlap is small, indicating that the requirements for the ecological environment of S. alterniflora are not close, and the niche shift is large.
Data Availability Statement
The original contributions presented in the study are included in the article/supplementary material, further inquiries can be directed to the corresponding author.
Author Contributions
YY and XT: conception and design of the research. XT: acquisition of data. YY: analysis and interpretation of data. ML and XL: statistical analysis. YY and JT: drafting the manuscript. All authors contributed to the article and approved the submitted version.
Funding
This project was supported by the National Natural Science Foundation of China (Grant No. 41901375), Hebei Natural Science (Grant No. D2019209322), and Program for the Youth talent of Higher Learning Institutions of Hebei National Special Fund for Forestry Scientific (Grant No. BJ2020058).
Conflict of Interest
The authors declare that the research was conducted in the absence of any commercial or financial relationships that could be construed as a potential conflict of interest.
Publisher's Note
All claims expressed in this article are solely those of the authors and do not necessarily represent those of their affiliated organizations, or those of the publisher, the editors and the reviewers. Any product that may be evaluated in this article, or claim that may be made by its manufacturer, is not guaranteed or endorsed by the publisher.
References
An, S., Gu, B., Zhou, C., Wang, Z., Deng, Z., Zhi, Y., et al. (2007). Spartina invasion in China: implications for invasive species management and future research. Weed Res. 47, 183–191. doi: 10.1111/j.1365-3180.2007.00559.x
Ayres, D. (2004). Spread of exotic cordgrasses and hybrids (Spartina sp.) in the tidal marshes of San Francisco Bay, California, USA. Biol. Invas. 6, 221–231. doi: 10.1023/B:BINV.0000022140.07404.b7
Baumel, A., Ainouche, M. L., and Levasseur, J. E. (2001). Molecular investigations in populations of Spartina anglica C.E. Hubbard (Poaceae) invading coastal Brittany (France). Mol. Ecol. 10, 1689–1701. doi: 10.1046/j.1365-294X.2001.01299.x
Bertness, M. D. (1991). Zonation of Spartina patens and Spartina alterniflora in New England Salt Marsh. Ecology 72, 138–148. doi: 10.2307/1938909
Bradley, B. A., Blumenthal, D. M., Wilcove, D. S., and Ziska, L. H. (2009). Predicting plant invasions in an era of global change. Trends Ecol. Evol. 25, 310–318. doi: 10.1016/j.tree.2009.12.003
Broennimann, O., Fitzpatrick, M. C., Pearman, P. B., Petitpierre, B., Pellissier, L., Yoccoz, N. G., et al. (2012). Measuring ecological niche overlap from occurrence and spatial environmental data. Glob. Ecol. Biogeogr. 21, 481–497. doi: 10.1111/j.1466-8238.2011.00698.x
Broennimann, O., Mráz, P., Petitpierre, B., Guisan, A., and Müller-Schärer, H. (2014). Contrasting spatio-temporal climatic niche dynamics during the eastern and western invasions of spotted knapweed in North America. J. Biogeogr. 41, 1126–1136. doi: 10.1111/jbi.12274
Brown, J. L. (2014). SDMtoolbox: a python-based GIS toolkit for landscape genetic, biogeographic and species distribution model analyses. Methods Ecol. Evol. 5, 694–700. doi: 10.1111/2041-210X.12200
Callaway, J. C., and Josselyn, M. N. (1992). The introduction and spread of smooth cordgrass (Spartina alterniflora) in South San Francisco Bay. Estuaries 15, 218–226. doi: 10.2307/1352695
Callen, S. T., and Miller, A. J. (2015). Signatures of niche conservatism and niche shift in the North American kudzu (Pueraria montana) invasion. Divers. Distrib. 21, 853–863. doi: 10.1111/ddi.12341
Catford, J. A., Vesk, P. A., Richardson, D. M., and Pyšek, P. (2012). Quantifying levels of biological invasion: towards the objective classification of invaded and invasible ecosystems. Glob. Change Biol. 18, 44–62. doi: 10.1111/j.1365-2486.2011.02549.x
Chen, Z., Li, B., Zhong, Y., and Chen, J. (2004). Local competitive effects of introduced Spartina alterniflora on Scirpus mariqueter at Dongtan of Chongming Island, the Yangtze River estuary and their potential ecological consequences. Hydrobiologia 528, 99–106. doi: 10.1007/s10750-004-1888-9
Chung, C., Zhuo, R., and Xu, G. (2004). Creation of Spartina plantations for reclaiming Dongtai, China, tidal flats and offshore sands. Ecol. Eng. 23, 135–150. doi: 10.1016/j.ecoleng.2004.07.004
Daehler, C. C. (1999). Inbreeding depression in smooth cordgrass (Spartina alterniflora, Poaceae) invading San Francisco Bay. Am. J. Bot. 86, 131–139. doi: 10.2307/2656962
Daehler, C. C., and Strong, D. R. (1994). Variable reproductive output among clones of Spartina alterniflora (Poaceae) invading San Francisco Bay, California: the influence of herbivory, pollination, and establishment site. Am. J. Bot. 81, 307–313. doi: 10.1002/j.1537-2197.1994.tb15448.x
Daehler, C. C., and Strong, D. R. (1996). Status, prediction and prevention of introduced cordgrass Spartina spp. invasions in Pacific estuaries, USA. Biol. Conserv. 78, 51–58. doi: 10.1016/0006-3207(96)00017-1
Davis, H. G., Taylor, C. M., Civille, J. C., and Strong, D. R. (2004). An Allee effect at the front of a plant invasion: Spartina in a Pacific estuary. J. Ecol. 92, 321–327. doi: 10.1111/j.0022-0477.2004.00873.x
Dietz, H., and Edwards, P. J. (2006). Recognition that causal processes change during plant invasion helps explain conflicts in evidence. Ecology 87, 1359–1367. doi: 10.1890/0012-9658(2006)87[1359:RTCPCD]2.0.CO;2
Ding, J., Mack, R. N., Lu, P., Ren, M., and Huang, H. (2008). China's booming economy is sparking and accelerating biological invasions. BioScience 58, 317–324. doi: 10.1641/B580407
Dorfman, D. D., Berbaum, K. S., and Metz, C. E. (1992). Receiver operating characteristic rating analysis: generalization to the population of readers and patients with the jackknife method. Investig. Radiol. 27, 723–731. doi: 10.1097/00004424-199209000-00015
Dormann, C. F., Schymanski, S. J., Cabral, J., Chuine, I., Graham, C., Hartig, F., et al. (2012). Correlation and process in species distribution models: bridging a dichotomy. J. Biogeogr. 39, 2119–2131. doi: 10.1111/j.1365-2699.2011.02659.x
Dumbauld, B., Peoples, M., Holcomb, L., Tagart, J., and Ratchford, S. (1995). The potential influence of cordgrass Spartina alterniflora on clam resources in Willapa Bay, Washington. J. Shellfish Res. 14, 1–278.
Eastwood, M. M., Donahue, M. J., and Fowler, A. E. (2007). Reconstructing past biological invasions: niche shifts in response to invasive predators and competitors. Biol. Invas. 9, 397–407. doi: 10.1007/s10530-006-9041-5
Elith, J. H., Graham, C. P., Anderson, R., Dudík, M., Ferrier, S., Guisan, A., et al. (2006). Novel methods improve prediction of species' distributions from occurrence data. Ecography 29, 129–151. doi: 10.1111/j.2006.0906-7590.04596.x
Evans, M. E. K., Smith, S. A., Flynn, R. S., and Donoghue, M. J. (2009). Climate, niche evolution, and diversification of the “BirdCage” evening primroses (Oenothera, Sections Anogra and Kleinia). Am. Nat. 173, 225–240. doi: 10.1086/595757
Evans, P. (1986). Use of the herbicide “Dalapon” for control of Spartina encroaching on intertidal mudflats: beneficial effects on shorebirds. Colon. Waterbirds 9, 171–175. doi: 10.2307/1521210
Feist, B. E., and Simenstad, C. A. (2000). Expansion rates and recruitment frequency of exotic smooth cordgrass, Spartina alterniflora (Loisel), colonizing unvegetated littoral flats in Willapa Bay, Washington. Estuaries 23, 267–274. doi: 10.2307/1352832
Gelviz-Gelvez, S. M., Pavón, N. P., Illoldi-Rangel, P., and Ballesteros-Barrera, C. (2015). Ecological niche modeling under climate change to select shrubs for ecological restoration in Central Mexico. Ecol. Eng. 74, 302–309. doi: 10.1016/j.ecoleng.2014.09.082
Graham, C. H., Ron, S. R., Santos, J. C., Schneider, C. J., and Moritz, C. (2004). Integrating phylogenetics and environmental niche models to explore speciation mechanisms in dendrobatid frogs. Evolution 58, 1781–1793. doi: 10.1111/j.0014-3820.2004.tb00461.x
Hijmans, R. J., Cameron, S. E., Parra, J. L., Jones, P. G., and Jarvis, A. (2005). Very high resolution interpolated climate surfaces for global land areas. Int. J. Climatol. 25, 1965–1978. doi: 10.1002/joc.1276
Huang, H.-M., Zhang, L.-Q., Guan, Y.-J., and Wang, D.-H. (2008). A cellular automata model for population expansion of Spartina alterniflora at Jiuduansha Shoals, Shanghai, China. Estuar. Coast. Shelf Sci. 77, 47–55. doi: 10.1016/j.ecss.2007.09.003
Jiménez-Valverde, A. (2012). Insights into the area under the receiver operating characteristic curve (AUC) as a discrimination measure in species distribution modelling. Glob. Ecol. Biogeogr. 21, 498–507. doi: 10.1111/j.1466-8238.2011.00683.x
Kelly, R., Leach, K., Cameron, A., Maggs, C. A., and Reid, N. (2014). Combining global climate and regional landscape models to improve prediction of invasion risk. Divers. Distrib. 20, 884–894. doi: 10.1111/ddi.12194
Kiritani, K. (2011). Impacts of global warming on Nezara viridula and its native congeneric species. J. Asia-Pac. Entomol. 14, 221–226. doi: 10.1016/j.aspen.2010.09.002
Kriticos, D. J., and Randall, R. P. (2001). A comparison of systems to analyse potential weed distributions. Weed Risk Assess. 61–79.
Kumar, S., Lebrun, E. G., Stohlgren, T. J., Stabach, J. A., Mcdonald, D. L., Oi, D. H., et al. (2015). Evidence of niche shift and global invasion potential of the Tawny Crazy ant, Nylanderia fulva. Ecol. Evol. 5, 4628–4641. doi: 10.1002/ece3.1737
Lee, W.-C., and Rodgers, J. L. J. P.M. (1998). Bootstrapping correlation coefficients using univariate and bivariate sampling. Psychol. Methods 3:91. doi: 10.1037/1082-989X.3.1.91
Li, H., and Zhang, L. (2008). An experimental study on physical controls of an exotic plant Spartina alterniflora in Shanghai, China. Ecol. Eng. 32, 11–21. doi: 10.1016/j.ecoleng.2007.08.005
Li, J., Fan, G., and He, Y. (2020). Predicting the current and future distribution of three Coptis herbs in China under climate change conditions, using the MaxEnt model and chemical analysis. Sci. Total Environ. 698:134141. doi: 10.1016/j.scitotenv.2019.134141
Lin, W., Zhou, G., Cheng, X., and Xu, R. (2007). Fast economic development accelerates biological invasions in China. PLoS ONE 2:e1208. doi: 10.1371/journal.pone.0001208
Luque, G. M., Bellard, C., Bertelsmeier, C., Bonnaud, E., and Courchamp, F. (2013). The 100th of the world's worst invasive alien species. Biol. Invas. 16, 981–985. doi: 10.1007/s10530-013-0561-5
Mack, R. N., Simberloff, D., Mark Lonsdale, W., Evans, H., Clout, M., and Bazzaz, F. A. (2000). Biotic invasions: causes, epidemiology, global consequences, and control. Ecol. Appl. 10, 689–710. doi: 10.1890/1051-0761(2000)010[0689:BICEGC]2.0.CO;2
Mitchell, C. E., and Power, A. G. (2003). Release of invasive plants from fungal and viral pathogens. Nature 421, 625–627. doi: 10.1038/nature01317
Moor, H., Hylander, K., and Norberg, J. (2015). Predicting climate change effects on wetland ecosystem services using species distribution modeling and plant functional traits. Ambio 44, 113–126. doi: 10.1007/s13280-014-0593-9
Mooring, M. T., Cooper, A. W., and Seneca, E. D. (1971). Seed germination response and evidence for height ecophenes in Spartina alterniflora from North Carolina. Am. J. Bot. 58, 48–55. doi: 10.1002/j.1537-2197.1971.tb09944.x
Müller-Schärer, H., Schaffner, U., and Steinger, T. (2004). Evolution in invasive plants: implications for biological control. Trends Ecol. Evol. 19, 417–422. doi: 10.1016/j.tree.2004.05.010
Muscarella, R., Galante, P. J., Soley-Guardia, M., Boria, R. A., Kass, J. M., Uriarte, M., Anderson, RPJMIE Evolution (2014). ENM eval: an R package for conducting spatially independent evaluations and estimating optimal model complexity for Maxent ecological niche models. 5, 1198–1205. doi: 10.1111/2041-210X.12261
Nunes, A. L., Tricarico, E., Panov, V. E., Cardoso, A. C., and Katsanevakis, S. (2015). Pathways and gateways of freshwater invasions in Europe. Aquat. Invas. 10, 359–370. doi: 10.3391/ai.2015.10.4.01
O'donnell, J., Gallagher, R. V., Wilson, P. D., Downey, P. O., Hughes, L., and Leishman, M. R. (2012). Invasion hotspots for non-native plants in A ustralia under current and future climates. Glob. Change Biol. 18, 617–629. doi: 10.1111/j.1365-2486.2011.02537.x
Perry, J. E., and Atkinson, R. B. (2009). York River tidal marshes. J. Coast. Res. 10057, 40–49. doi: 10.2112/1551-5036-57.sp1.40
Perutz, M. F. (1967). Concluding remarks. Proc. R. Soc. Lond. Ser. B. Biol. Sci. 167, 448–448. doi: 10.1098/rspb.1967.0046
Petitpierre, B., Kueffer, C., Broennimann, O., Randin, C., Daehler, C., and Guisan, A. (2012). Climatic niche shifts are rare among terrestrial plant invaders. Science 335, 1344–1348. doi: 10.1126/science.1215933
Phillips, S. J., Dudík, M., Elith, J., Graham, C. H., Lehmann, A., Leathwick, J., et al. (2009). Sample selection bias and presence-only distribution models: implications for background and pseudo-absence data. Ecol. Appl. 19, 181–197. doi: 10.1890/07-2153.1
Plyler, D. B., and Carrick, K. M. (1993). Site-specific seed dormancy in Spartina alterniflora (POACEAE). Am. J. Bot. 80, 752–756. doi: 10.1002/j.1537-2197.1993.tb15290.x
Raghubanshi, A., and Tripathi, A. (2009). Effect of disturbance, habitat fragmentation and alien invasive plants on floral diversity in dry tropical forests of Vindhyan highland: a review. Trop. Ecol. 50, 57–69.
Richardson, D. M., and Van Wilgen, B. W. (2004). Invasive alien plants in South Africa: how well do we understand the ecological impacts? Working for water. South Afric. J. Sci. 100, 45–52.
Rissler, L. J., and Apodaca, J. J. (2007). Adding more ecology into species delimitation: ecological niche models and phylogeography help define cryptic species in the black salamander (Aneides flavipunctatus). Syst. Biol. 56, 924–942. doi: 10.1080/10635150701703063
Sakai, A. K., Allendorf, F. W., Holt, J. S., Lodge, D. M., Molofsky, J., With, K. A., et al. (2001). The population biology of invasive species. Annu. Rev. Ecol. Syst. 32, 305–332. doi: 10.1146/annurev.ecolsys.32.081501.114037
Salo, L. F. (2005). Red brome (Bromus rubens subsp. madritensis) in North America: possible modes for early introductions, subsequent spread. Biol. Invas. 7, 165–180. doi: 10.1007/s10530-004-8979-4
Schoener, T. W. (1970). Nonsynchronous spatial overlap of lizards in patchy habitats. Ecology 51, 408–418. doi: 10.2307/1935376
Shigesada, N., and Kawasaki, K. (1997). Biological Invasions: Theory and Practice. Oxford: Oxford University Press.
Simenstad, C., and Thom, R. (1995). Spartina alterniflora(smooth cordgrass) as an invasive halophyte in Pacific northwest estuaries. Hortus Northwest Pac. Northwest Native Plant Directory J. 6, 9–13.
Stachowicz, J. J., Terwin, J. R., Whitlatch, R. B., and Osman, R. W. (2002). Linking climate change and biological invasions: ocean warming facilitates nonindigenous species invasions. Proc. Natl. Acad. Sci. USA. 99, 15497–15500. doi: 10.1073/pnas.242437499
Swets, J. A. (1988). Measuring the accuracy of diagnostic systems. Science 240, 1285–1293. doi: 10.1126/science.3287615
Tang, X., Yuan, Y., Li, X., and Zhang, J. (2021a). Maximum entropy modeling to predict the impact of climate change on pine wilt disease in China. Front. Plant Sci. 12:764. doi: 10.3389/fpls.2021.652500
Tang, X., Yuan, Y., Liu, X., and Zhang, J. J. E. E. (2021b). Potential range expansion and niche shift of the invasive Hyphantria cunea between native and invasive countries. Ecol. Entomol. 46, 910–925. doi: 10.1111/een.13028
Tilman, D. (2000). Causes, consequences and ethics of biodiversity. Nature 405, 208–211. doi: 10.1038/35012217
Townsend Peterson, A., Pape,ş, M., and Eaton, M. (2007). Transferability and model evaluation in ecological niche modeling: a comparison of GARP and Maxent. Ecography 30, 550–560. doi: 10.1111/j.0906-7590.2007.05102.x
Vanderwal, J., Murphy, H. T., Kutt, A. S., Perkins, G. C., and Reside, A. E. (2013). Focus on poleward shifts in species' distribution underestimates the fingerprint of climate change. Nat. Clim. Change 3, 239–243. doi: 10.1038/nclimate1688
Vitousek, P. M., D'antonio, C. M., Loope, L. L., and Westbrooks, R. (1996). Biological invasions as global environmental change. https://pubag.nal.usda.gov/?f%5Bjournal_name%5D%5B%5D=American+scientist&f%5Bpublication_year_rev%5D%5B%5D=8004-1996&f%5Bsource%5D%5B%5D=1996+v.84+no.5 Am. Sci. 84:46.8
Walther, G.-R., Roques, A., Hulme, P. E., Sykes, M. T., Pyšek, P., Kühn, I., et al. (2009). Alien species in a warmer world: risks and opportunities. Trends Ecol. Evol. 24, 686–693. doi: 10.1016/j.tree.2009.06.008
Walther, G. R., Gritti, E. S., Berger, S., Hickler, T., Tang, Z., and Sykes, M. T. (2007). Palms tracking climate change. Glob. Ecol. Biogeogr. 16, 801–809. doi: 10.1111/j.1466-8238.2007.00328.x
Wan, J. Z., Wang, C. J., Tan, J. F., and Yu, F. H. (2017). Climatic niche divergence and habitat suitability of eight alien invasive weeds in China under climate change. Ecol. Evol. 7, 1541–1552. doi: 10.1002/ece3.2684
Wang, R., and Wang, Y. Z. (2006). Invasion dynamics and potential spread of the invasive alien plant species Ageratina adenophora (Asteraceae) in China. Divers. Distrib. 12, 397–408. doi: 10.1111/j.1366-9516.2006.00250.x
Weber, E., Sun, S.-G., and Li, B. (2008). Invasive alien plants in China: diversity and ecological insights. Biol. Invas. 10, 1411–1429. doi: 10.1007/s10530-008-9216-3
Wijte, A. H., and Gallagher, J. L. (1996). Effect of oxygen availability and salinity on early life history stages of salt marsh plants. I. Different germination strategies of Spartina alterniflora and Phragmites australis (Poaceae). Am. J. Bot. 83, 1337–1342. doi: 10.1002/j.1537-2197.1996.tb13919.x
Wilcove, D. S., Rothstein, D., Dubow, J., Phillips, A., and Losos, E. (1998). Quantifying threats to imperiled species in the United States. BioScience 48, 607–615. doi: 10.2307/1313420
Yang, X.-Q., Kushwaha, S., Saran, S., Xu, J., and Roy, P. (2013). Maxent modeling for predicting the potential distribution of medicinal plant, Justicia adhatoda L. in Lesser Himalayan foothills. Ecol. Eng. 51, 83–87. doi: 10.1016/j.ecoleng.2012.12.004
Yu, F., Wang, T., Groen, T. A., Skidmore, A. K., Yang, X., Ma, K., et al. (2019). Climate and land use changes will degrade the distribution of Rhododendrons in China. Sci. Total Environ. 659, 515–528. doi: 10.1016/j.scitotenv.2018.12.223
Zhao, X., Zhao, C., Liu, X., Gong, L., Deng, Z., and Li, J. (2015). Growth characteristics and adaptability of Spartina alterniflora in different latitude area along China coast. Ecol. Sci. 34, 119–128.
Zhu, Y. (2012). Current status, influence and countermeasure of biological invasion on ecological security in China. Plant Dis. Pests 3, 64–67.
Keywords: Spartina alterniflora, invasive country, origin country, MaxEnt model, niche shift
Citation: Yuan Y, Tang X, Liu M, Liu X and Tao J (2021) Species Distribution Models of the Spartina alterniflora Loisel in Its Origin and Invasive Country Reveal an Ecological Niche Shift. Front. Plant Sci. 12:738769. doi: 10.3389/fpls.2021.738769
Received: 09 July 2021; Accepted: 08 September 2021;
Published: 12 October 2021.
Edited by:
Lars Hendrik Wegner, Foshan University, ChinaReviewed by:
Neus Nualart, Consejo Superior de Investigaciones Científicas (CSIC), SpainEduardo Cires, University of Oviedo, Spain
Copyright © 2021 Yuan, Tang, Liu, Liu and Tao. This is an open-access article distributed under the terms of the Creative Commons Attribution License (CC BY). The use, distribution or reproduction in other forums is permitted, provided the original author(s) and the copyright owner(s) are credited and that the original publication in this journal is cited, in accordance with accepted academic practice. No use, distribution or reproduction is permitted which does not comply with these terms.
*Correspondence: Jun Tao, dGFvanVuJiN4MDAwNDA7eXp1LmVkdS5jbg==
†These authors share first authorship