- 1School of Life Sciences and Food Engineering, Huaiyin Institute of Technology, Huai’an, China
- 2Huaiyin Institute of Agricultural Sciences of Xuhuai Region, Huai’an, China
- 3Institute of Botany, Jiangsu Province and Chinese Academy of Sciences, Nanjing, China
Auxin/indoleacetic acid (Aux/IAA) proteins play an important regulatory role in the developmental process of plants and their responses to stresses. A previous study has shown that constitutive expression of OsIAA18, an Aux/IAA transcription factor gene of rice improved salt and osmotic tolerance in transgenic Arabidopsis plants. However, little work is known about the regulatory functions of the OsIAA18 gene in regulating the abiotic stress tolerance of rice. In this study, the OsIAA18 gene was introduced into the rice cultivar, Zhonghua 11 and the OsIAA18 overexpression in rice plants exhibited significantly enhanced salt and drought tolerance compared to the wild type (WT). Moreover, overexpression of OsIAA18 in rice increased endogenous levels of abscisic acid (ABA) and the overexpression of OsIAA18 in rice plants showed hypersensitivity to exogenous ABA treatment at both the germination and postgermination stages compared to WT. Overexpression of OsIAA18 upregulated the genes involved in ABA biosynthesis and signaling pathways, proline biosynthesis pathway, and reactive oxygen species (ROS)-scavenging system in the overexpression of OsIAA18 in rice plants under salt and drought stresses. Proline content, superoxide dismutase (SOD), and peroxidase (POD) activities were significantly increased, whereas malonaldehyde (MDA), hydrogen peroxide (H2O2), and superoxide anion radical (O2–) content were significantly decreased in the transgenic plants under salt and drought stresses. Taken together, we suggest that OsIAA18 plays a positive role in drought and salt tolerance by regulating stress-induced ABA signaling. The OsIAA18 gene has a potential application in genetically modified crops with enhanced tolerance to abiotic stresses.
Introduction
Environmental abiotic stresses, such as salt and drought, impact the growth, development, and productivity of agricultural crops and are becoming serious threats to provide and satisfy the needs of a rapidly growing population worldwide (Munns and Tester, 2008; Wang et al., 2016; Zhu et al., 2020). Plant adaptation to salt and drought stresses is dependent on the activation of cascades of molecular networks involved in stress perception, signal transduction, and the expression of specific stress-related genes and metabolites (Huang et al., 2012; Zhang et al., 2014). Rice is one of the most important grain crops and its production has been significantly affected by salt and drought stresses (Joo et al., 2019). Rice establishes a complex defense network in the process of resisting and adapting to abiotic stresses. It is important to understand abiotic stress responses of rice for enhancing salt and drought tolerance.
Auxin plays a very important role in a wide variety of plant developmental and physiological processes (Friml, 2003; Song et al., 2009; Li G. et al., 2020; Li W. et al., 2020). Auxin signaling can regulate the expression of downstream genes and perform the required responses by recruiting specific transcription factors (Vogler and Kuhlemeier, 2003). Auxin/indoleacetic acid (Aux/IAA) and auxin response factor (ARF) family proteins, as two important protein families of plants, play important roles in the developmental process and responses to phytohormones and stress treatments by means of controlling auxin-responsive transcription (Hagen and Guilfoyle, 2002; Liscum and Reed, 2002; Berleth et al., 2004; Song et al., 2009; Song and Xu, 2013). Aux/IAA proteins are short-lived transcription factors that are characterized by the presence of four conserved domains, known as domains I, II, III, and IV. Domain I, which is similar to the conserved domain of ethylene response factors functioning as transcription repressors, can inactivate ARF function, thereby repressing auxin-responsive transcription (Hiratsu et al., 2003; Tiwari et al., 2003). Domain II has been shown to be essential for auxin signaling by interacting with a component of the ubiquitin-proteasome protein degradation pathway (Gray et al., 2001; Ramos et al., 2001; Song and Xu, 2013). Domains III and IV of the C-terminal dimerization mediate homodimerization and heterodimerization among Aux/IAA and ARF proteins (Ulmasov et al., 1997; Hardtke et al., 2004; Song and Xu, 2013).
In order to determine the molecular mechanism of auxin signaling, the genes encoding Aux/IAA proteins have been cloned and identified in mung bean (Yamamoto, 1994), Arabidopsis (Reed, 2001), rice (Thakur et al., 2001; Nakamura et al., 2006), and grapevine (Çakir et al., 2013; Li G. et al., 2020). Many members of the Aux/IAA gene families in a variety of plant species are induced in response to auxin (Guilfoyle and Hagen, 2001; Thakur et al., 2001, 2005; Kepinski and Leyser, 2004). It has been reported that thirty-one Aux/IAA (OsIAA) genes of rice were identified and their sequences were analyzed (Jain et al., 2006; Wang et al., 2007). The first rice OsIAA gene, named OsIAA13, was cloned in the original reports (Thakur et al., 2001, 2005), while the first Aux/IAA protein characterized in rice was OsIAA31 (Nakamura et al., 2006). The OsIAA23 gene played an important role in the postembryonic maintenance of a quiescent center (Jun et al., 2011). OsIAA13 was involved in lateral root initiation (Kitomi et al., 2012). OsIAA11 affected lateral root development (Zhu et al., 2012). Song et al. (2009) found that most of the OsIAA genes were responsive to various abiotic stresses, indicating an interaction between plant growth and abiotic stresses. However, the functions of most OsIAA genes in rice remain to be elucidated.
These are few reports on the overexpression of the related IAA genes in plants. The OsIAA31 overexpression in rice plants showed insensitivity to auxin and gravitropic stimuli and exhibited short leaf blades, reduced crown root formation, and abnormal leaf formation (Nakamura et al., 2006). Overexpression of OsIAA4 in rice induced morphological changes and reduced responsiveness to auxin (Song and Xu, 2013). Overexpression of OsIAA6/OsIAA20 enhanced the tolerance to salt and drought stress in rice (Jung et al., 2015; Zhang et al., 2021). The VvIAA18 gene was cloned from grapevine and it was found that the VvIAA18-overexpressing tobacco plants exhibited improved salt tolerance (Li W. et al., 2020). The OsIAA18 gene was induced by salt, drought, IAA, and abscisic acid (ABA) treatments in rice (Song et al., 2009). Li G. et al. (2020) reported that heterologous overexpression of OsIAA18 enhanced the tolerance to salt and osmotic stresses in transgenic Arabidopsis plants. However, little work is known about the regulatory functions of the OsIAA18 gene in regulating the abiotic stress tolerance of rice.
In this study, the OsIAA18 gene was further introduced into rice to characterize its functions. The functional analysis of OsIAA18 was determined by investigating ABA sensitivity and salt and drought tolerance of OsIAA18 overexpression in rice plants. Our results indicate that the OsIAA18 gene is a positive regulator in rice salt and drought stress responses by ABA signaling and has potential application in genetically modified crops with enhanced tolerance to abiotic stresses.
Materials and Methods
Plant Materials and Transformation of Rice With OsIAA18
Rice (Oryza sativa L.) plants were grown in an experimental field at the Huaiyin Institute of Technology under natural conditions. Zhonghua 11 was used as the wild type (WT). EHA105, the Agrobacterium tumefaciens strain harboring a binary vector, plasmid pCAMBIA1301, was used in this study (Li G. et al., 2020). This binary vector contains the OsIAA18 gene under the control of CaMV 35S promoter and nopaline synthase terminator (NOS) terminator of the expression box. This vector also contained gusA and hptIIgenes driven by a CaMV 35S promoter. Then the recombinant vector was transformed into Zhonghua11 via the A. tumefaciens-mediated method (Hiei et al., 1994). After transformation, the callus was selected from 1/2 Murashige and Skoog (MS) medium containing 100 μg/mL hygromycin. Seedlings with hygromycin resistance were transplanted to soil in a growth chamber.
DNA Extraction and PCR Detection
The DNA was extracted from rice leaves according to the instructions of EasyPure Plant Genomic DNA Kit (Transgen, Beijing, China). The presence of the OsIAA18 expression construct in positive plants was assessed by PCR analysis using specific primers (Supplementary Table 1). PCR amplifications were performed with an initial denaturation at 94°C for 3 min, followed by 35 cycles at 94°C for 30 s, 55°C for 30 s, 72°C for 1 min, and a final extension at 72°C for 10 min. PCR products were separated by electrophoresis on a 1.0% (w/v) agarose gel.
Salt and Drought Tolerance Assays
According to the methods of Li et al. (2017), the seeds of overexpressed OsIAA18 and WT lines were germinated on 1/2 medium under 16-h light (28°C)/8-h dark (25°C) photoperiod conditions for 1 week and transplanted to 1/2 MS medium with no stress, 200 mM of NaCl, and 200 mM of mannitol, respectively. Plant length and fresh weight of OsIAA18 overexpression and WT lines were measured after 1 week. The 4-week-old seedlings of OsIAA18 overexpressed rice and WT lines were grown in 9-cm diameter pots containing a mixture of soil and vermiculite (1:1, v/v) in a greenhouse. All pots were irrigated sufficiently with water for 2 weeks under optimum growth conditions. Each pot was then irrigated with 100 m of 200 mM NaCl solution once every 2 days for 2 weeks, or subjected to drought stress for 1 week by stopping irrigation and recovery with rewatering for 1 week. Seedlings were regarded as survivals if the fresh and green young leaves emerged after water supply. The survival rates of these plants were observed immediately. All treatments were performed in triplicate.
With respect to the salt and drought stresses, the assays of the isolated leaves, leaf sections of approximately 5 cm in length from overexpressed OsIAA18 rice and WT lines at seedling stages were removed and immersed in MS liquid media solutions with concentrations of 200 mM of NaCl and 200 mM of mannitol for 96 h, respectively. Disks that floated on sterile distilled water served as an experimental control. All the leaves were subsequently cultured under 16-h light (28°C)/8-h dark (25°C) photoperiod conditions. Each treatment was repeated three times, and 10 leaves were selected for each treatment. The total chlorophyll content of the rice leaves was measured according to the methods of Patra et al. (2010).
RNA Extraction, Complementary DNA Synthesis, and Real-Time Quantitative Reverse Transcription -PCR Assay
Total leaf RNA was extracted from the transgenic plants and WT using the RNAprep Pure Plant Kit (Tiangen Biotech, Beijing, China). RNA samples were reverse-transcribed using Quantscript Reverse Transcriptase Kit (Tiangen Biotech, Beijing, China). The complementary DNA (cDNA) solution was used as a template for PCR amplification with specific primers (Supplementary Table 1). The expression of genes related to IAA signaling pathway, ABA biosynthesis, and signaling pathways, proline biosynthesis, stress responses, and ROS scavenging in the leaves of transgenic rice plants and WT grown in pots and incubated for 1 week under optimum growth condition, for 1 week under 200 mM of NaCl stress or for 1 week under drought stress were analyzed by real-time quantitative reverse transcription (qRT)-PCR as described by Wang et al. (2016). The OsActin gene of rice was used as an internal control, and it was amplified by specific primers (Supplementary Table 1). Quantification of the gene expression was done with the comparative CT method (Schmittgen and Livak, 2008). All treatments were performed in triplicate.
Abscisic Acid Content and Sensitivity Assays
Endogenous ABA levels in the leaves of transgenic rice plants and WT grown in pots and incubated for 1 week under optimum growth condition, for 1 week under 200 mM of NaCl stress or for 1 week under drought stress were performed by indirect ELISA as described by Wang et al. (2016). The ABA sensitivity at germination and postgermination stages was determined according to the methods of Li et al. (2017). The seeds of the overexpressed OsIAA18 and WT lines were germinated on 1/2 MS medium with 2.5 μM of ABA, and the germination rates of the treated seeds were calculated after 5 days. The seeds of overexpressed OsIAA18 and WT lines germinated on 1/2 MS medium with no stress were transplanted to 1/2 MS medium with 2.5μM of ABA; then, the shoot and root lengths and fresh weight of all lines were measured after 1 week. All treatments were performed in triplicate.
3,3′-Diaminobenzidine and Nitro Blue Tetrazolium Staining
The 3,3′-diaminobenzidine (DAB) staining and nitro blue tetrazolium (NBT) staining for hydrogen peroxide (H2O2) and superoxide anion radical (O2–) detection in the leaves of transgenic rice plants and WT grown in pots and incubated for 1 week under optimum growth condition, for 1 week under 200 mM of NaCl stress or for 1 week under drought stress, respectively, were performed as described by Jiang et al. (2016). All treatments were performed in triplicate.
Measurements of IAA, Proline, H2O2, and Malondialdehyde Content
The IAA content in the leaves of transgenic rice plants and WT grown in pots and incubated for 1 week under normal growth conditions was analyzed based on the methods of Liu et al. (2012). The levels of proline, H2O2, and malondialdehyde (MDA) in the leaves of transgenic rice plants and WT grown in pots and incubated for 1 week under optimum growth condition, for 1 week under 200 mM of NaCl stress or for 1 week under drought stress, respectively, were measured as described (Wang et al., 2016). All treatments were performed in triplicate.
Analyses of 9-Cis-Epoxycarotenoid Dioxygenase, Superoxide Dismutase, and Peroxidase Activities
The 9-cis-epoxycarotenoid dioxygenase (NCED) activity in the leaves of transgenic rice plants and WT grown in pots and incubated for 1 week under optimum growth condition, for 1 week under 200 mM of NaCl stress or for 1 week under drought stress, was measured with Plant NCED ELISA Kit (Uscn Life Science Inc., Shanghai, China). The activities of superoxide dismutase (SOD) and peroxidase (POD) were measured according to the method of Wang et al. (2016) and Li G. et al. (2020), respectively. All treatments were performed in triplicate.
Statistical Analysis
The experiments were repeated three times and the data were presented as the mean ± standard error (SE). Wherever applicable, the data were analyzed by Student’s t-test in a two-tailed analysis. Values of P < 0.05 or < 0.01 were considered to be statistically significant.
Results
Overexpression of OsIAA18 in Transgenic Rice Lines
In our previous study, we confirmed that constitutive expression of OsIAA18 significantly enhanced tolerance to salt and osmotic stresses in Arabidopsis plants (Li G. et al., 2020). To explore whether OsIAA18 plays an important role in improving the agronomic traits through gene manipulation approaches, we introduced this gene to rice (Oryza sativa L. ssp. japonica cv. Zhonghua 11). The ORF of OsIAA18 was overexpressed in rice cv. Zhonghua 11 (WT) using the binary vector pCAMBIA1301-OsIAA18 (Figure 1A). Multiple lines were obtained from Hyg resistance selection. Seven independent transgenic lines of overexpressing OsIAA18 gene in rice (T1 generation), named OE1, OE2, OE3, OE4, OE5, OE6, and OE7 were obtained, and their progenies (T3 generation) were generated. PCR analysis of genomic DNA indicated that they were transgenic (Figure 1B). These transgenic plants were further confirmed to have higher expression of the OsIAA18 gene by qRT-PCR (Figure 1C), especially OE3, OE5, and OE6 plants. Therefore, the transgenic lines OE3, OE5, and OE6 were selected for further experiments.
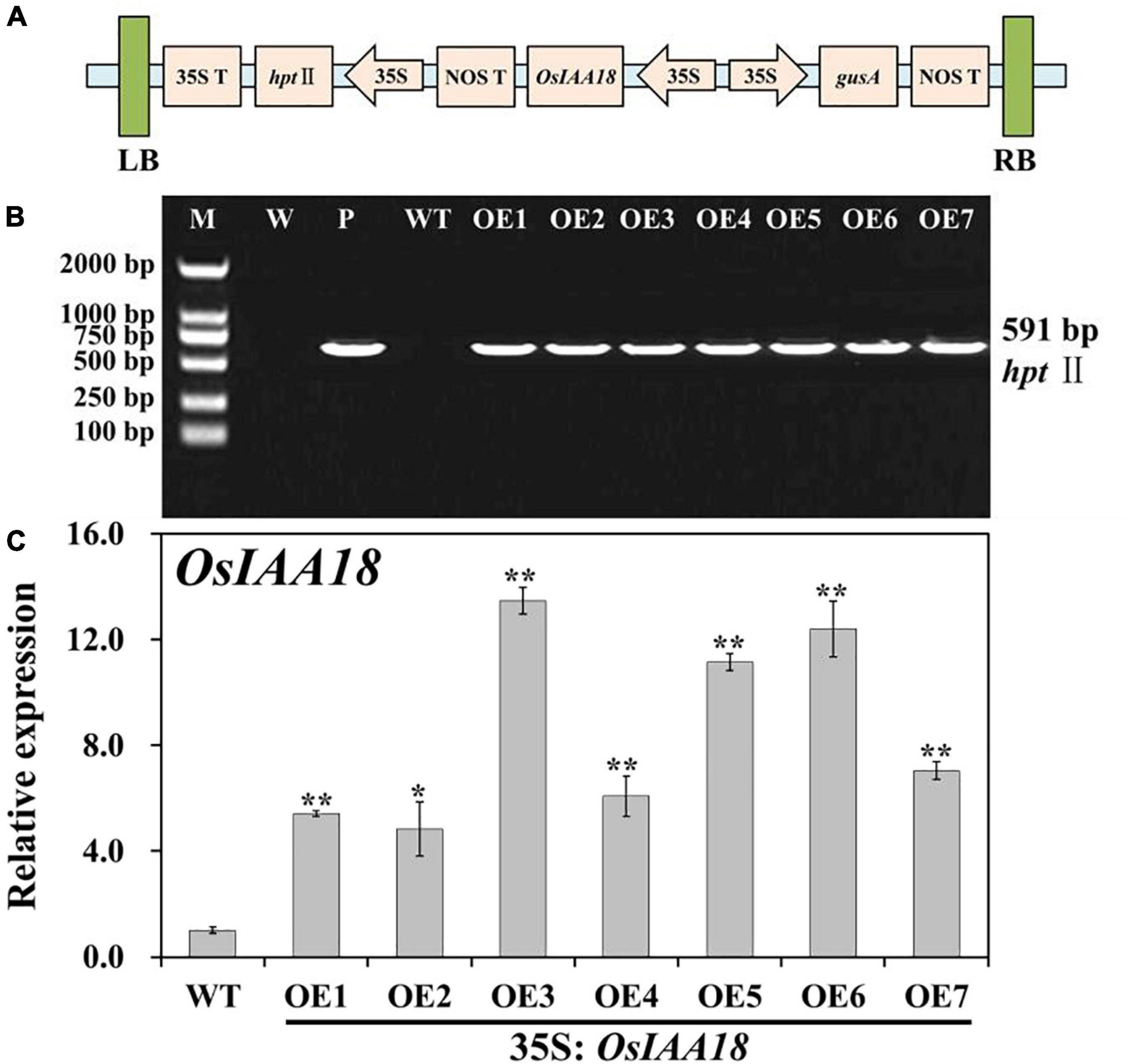
Figure 1. Molecular analyses of the OsIAA18-overexpressing rice plants. (A) Schematic diagram of the T-DNA region of binary plasmid pCAMBIA1301-OsIAA18. LB left border; RB right border; hptII hygromycin phosphotransferase II gene; OsIAA18 rice auxin/indoleacetic acid (Aux/IAA) transcription factor gene; gusA β-glucuronidase gene; 35S cauliflower mosaic virus (CaMV) 35S promoter; 35S T CaMV 35S terminator; NOS T nopaline synthase terminator. (B) PCR analysis of transgenic rice plants. Lane M, DL2000 DNA marker; Lane W, water as negative control; Lane P, plasmid pCAMBIA1301-OsIAA18 as positive control; Lane WT, wild type; Lanes OE1, OE2, OE3, OE4, OE5, OE6, and OE7, overexpressing OsIAA18 rice plants. (C) Expression level of OsIAA18 in the overexpressing OsIAA18 rice plants. The rice OsActin gene was used as an internal control. The results are expressed as relative values based on WT as reference sample set to 1.0. Data are presented as means ± SE (n = 3). The values for * and ** indicate a significant difference from that of WT at P < 0.05 and < 0.01, respectively, by Student’s t-test.
Under normal conditions, there were no significant differences in plant height and root length, and fresh weight between the overexpressing and WT lines grown at seedling stages (Figure 2). At the tillering stages, there were no developmental differences between OsIAA18 overexpression and WT plants at normal irrigation (Figure 3). Furthermore, endogenous IAA levels and the expression levels of the genes related to IAA signaling pathway were analyzed in the leaves of overexpressed OsIAA18 and WT plants under control growth conditions. The results showed that no obvious difference of IAA content was found under normal conditions (Supplementary Figure 1). There were no significant differences between overexpressing OsIAA18 and WT plants in the expression levels of IAA biosynthesis-related YUCCA-like family gene OsYUCCA6 and IAA responsive genes, OsIAA6 and OsIAA8 (Supplementary Figure 1).
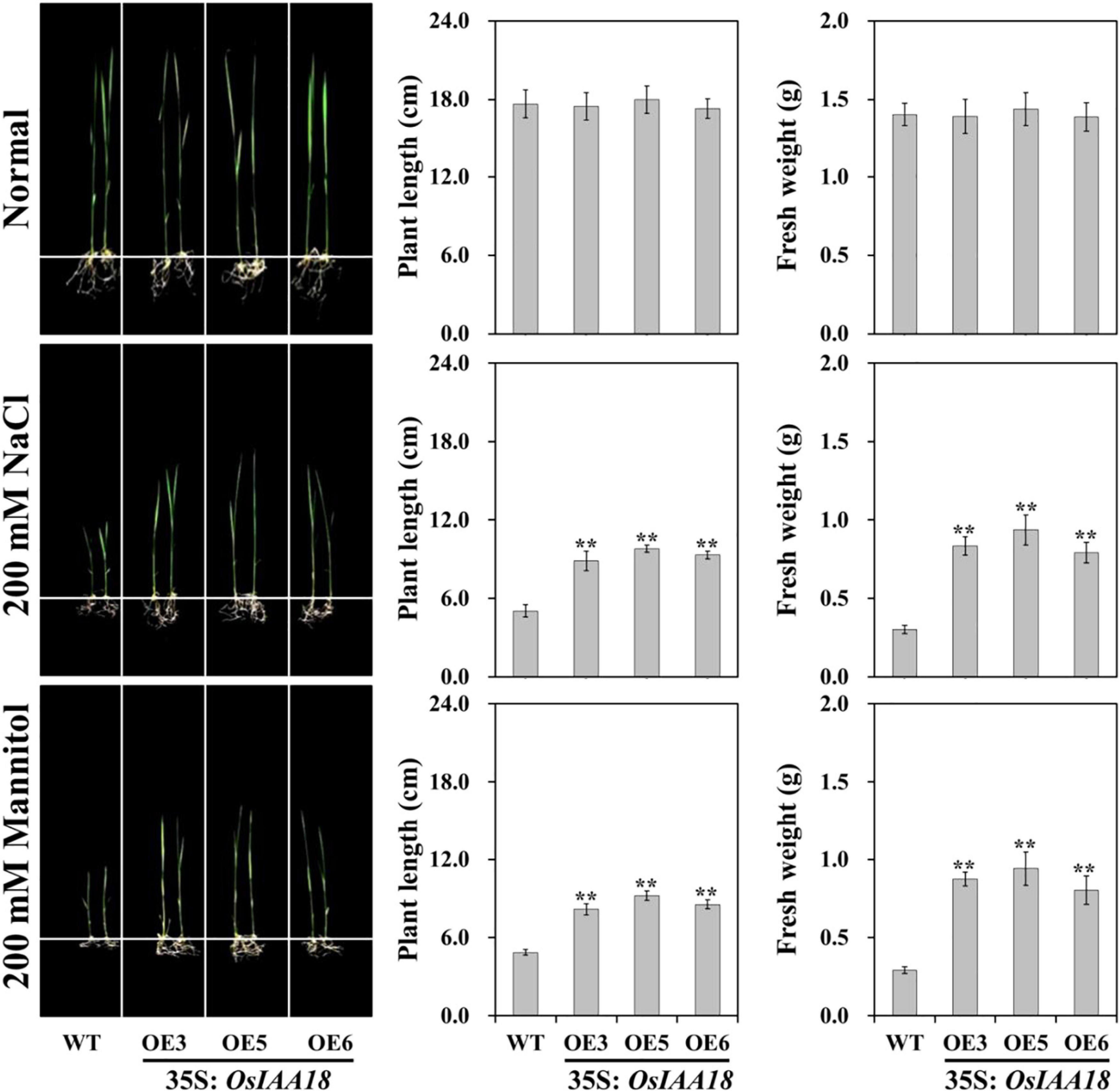
Figure 2. Growth performance of overexpressing OsIAA18 and WT seedlings under 200 mM of NaCl and 200 mM of mannitol, respectively. Plant length and fresh weight of 7th-day old seedlings after transplantation. Data are presented as means ± SE (n = 3). The values of * and ** indicate a significant difference from that of WT at P < 0.05 and < 0.01, respectively, by Student’s t-test.
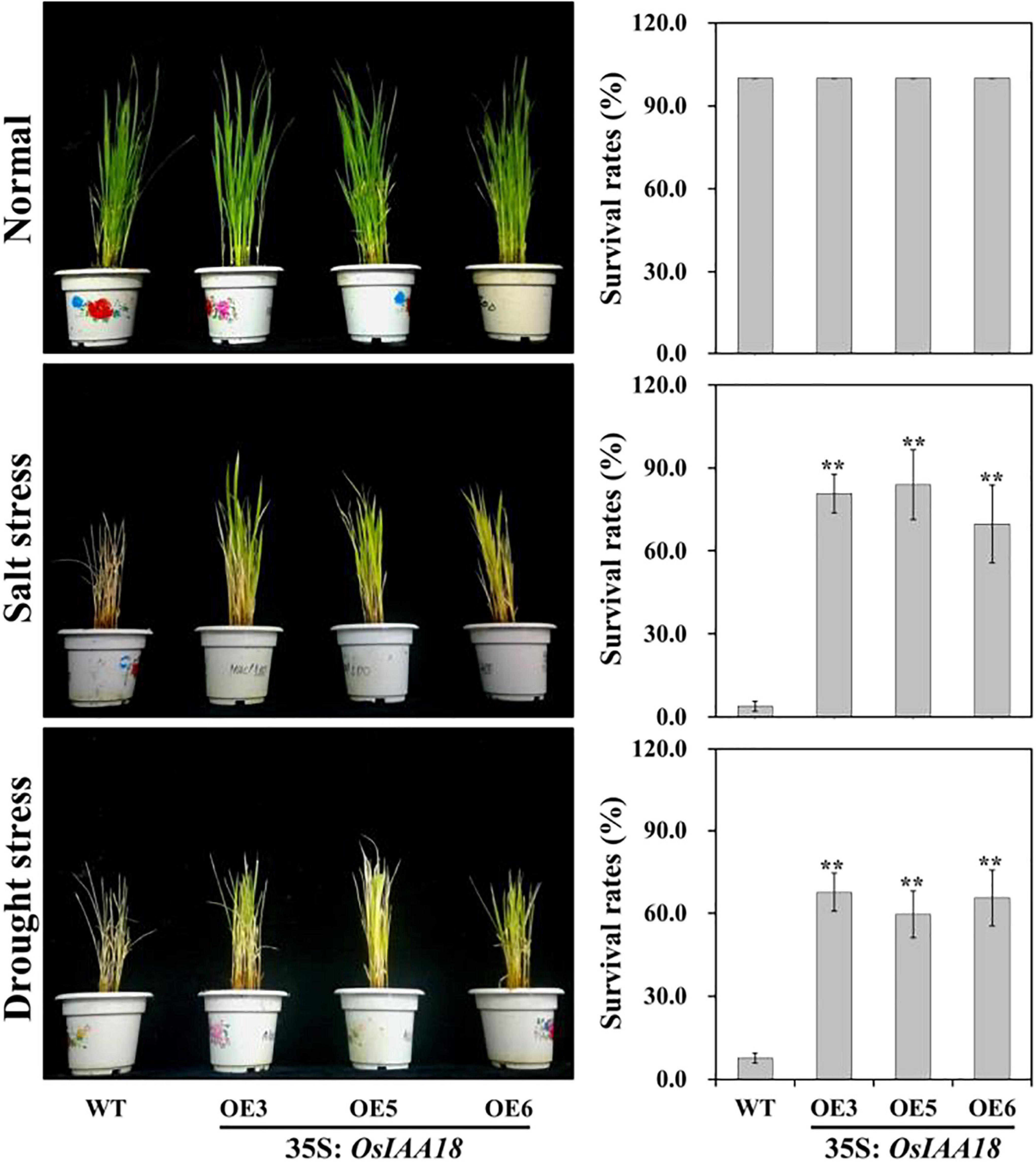
Figure 3. Responses of the transgenic rice plants and WT grown in pots under salt and drought stresses. Transgenic plants and WT were grown in pots and incubated for 2 weeks under normal conditions, for 2 weeks under 200 mM NaCl stress, and for 2 weeks by stopping irrigation and recovery with rewatering for 2 weeks. Survival rates of overexpressing OsIAA18 and WT plants after salt and drought stresses were examined. Data are presented as means ± SE (n = 3). The values of * and ** indicate a significant difference from that of WT at P < 0.05 and < 0.01, respectively, by Student’s t-test.
Improved Salt and Drought Tolerance of Overexpressing OsIAA18 in Rice
Three lines (OE3, the OE5, and OE6) with the highest transcription levels of OsIAA18 were selected to verify the functions of OsIAA18 gene to the responses of stress treatments. The performance of overexpressing OsIAA18 and WT lines under osmotic stress by adding 200 mM of NaCl or 200 mannitol was examined, respectively. It was worth noting that no developmental differences were identified between the overexpressing and WT lines under controlled growth conditions (Figure 2). Under salt and mannitol stresses, the growth of the overexpressing OsIAA18 seedlings was less inhibited, which exhibited higher plant length and fresh weight than those of the WT seedlings (Figure 2). Together, these results suggest that the overexpression of OsIAA18 in rice could enhance the tolerance to salt and mannitol stresses at seedling stages.
To investigate the functions of OsIAA18 in response to salt and drought stresses, the overexpressing OsIAA18 and WT seedlings were grown in the soil and were well-watered at the tillering stages. There were no developmental differences between overexpressing OsIAA18 and WT plants when normal irrigation was performed (Figure 3). After 2 weeks of 200 mM NaCl stress, or 2 week after the stopping of irrigation and 2 weeks of recovery, the overexpressing OsIAA18 seedlings remained green and showed continuous growth, whereas WT seedlings showed severe leaf rolling and wilting (Figure 3). This study also determined the survival rate for overexpressing OsIAA18 and WT seedlings grown in the soil with salt and drought stresses. As shown in Figure 3, the survival rates of overexpressing OsIAA18 lines were significantly higher than that of WT seedlings when exposed to salt and drought stresses. Therefore, it is evident that the overexpression of OsIAA18 results in enhanced tolerance to salt and drought stresses in rice.
As an explanation for the growth performance exhibited by the different transgenic plants, the possibility of a correlation between growth performance and the amount of chlorophyll retained in these plants during growth under salt and drought stresses was looked for. For such experiments, uniformly cut leaf disks from overexpressing OsIAA18 and WT plants were incubated in the presence of an indicated concentration of 200 mM of NaCl or 200 mM of mannitol for 96 h at 28°C, respectively, and the “greenness” of the plants was compared against the control set without any stress (Figure 4). Although not very well discernible visually, the actual determination of chlorophyll content in the leaf disks showed that the overexpressing OsIAA18 rice plants exhibited higher total chlorophyll content compared to WT (Figure 4), indicating that the transgenic plants were capable of retaining the chlorophyll to a greater extent in comparison to WT during growth under stress treatments. These results indicate that the overexpression of OsIAA18 in rice increases salt and drought tolerance.
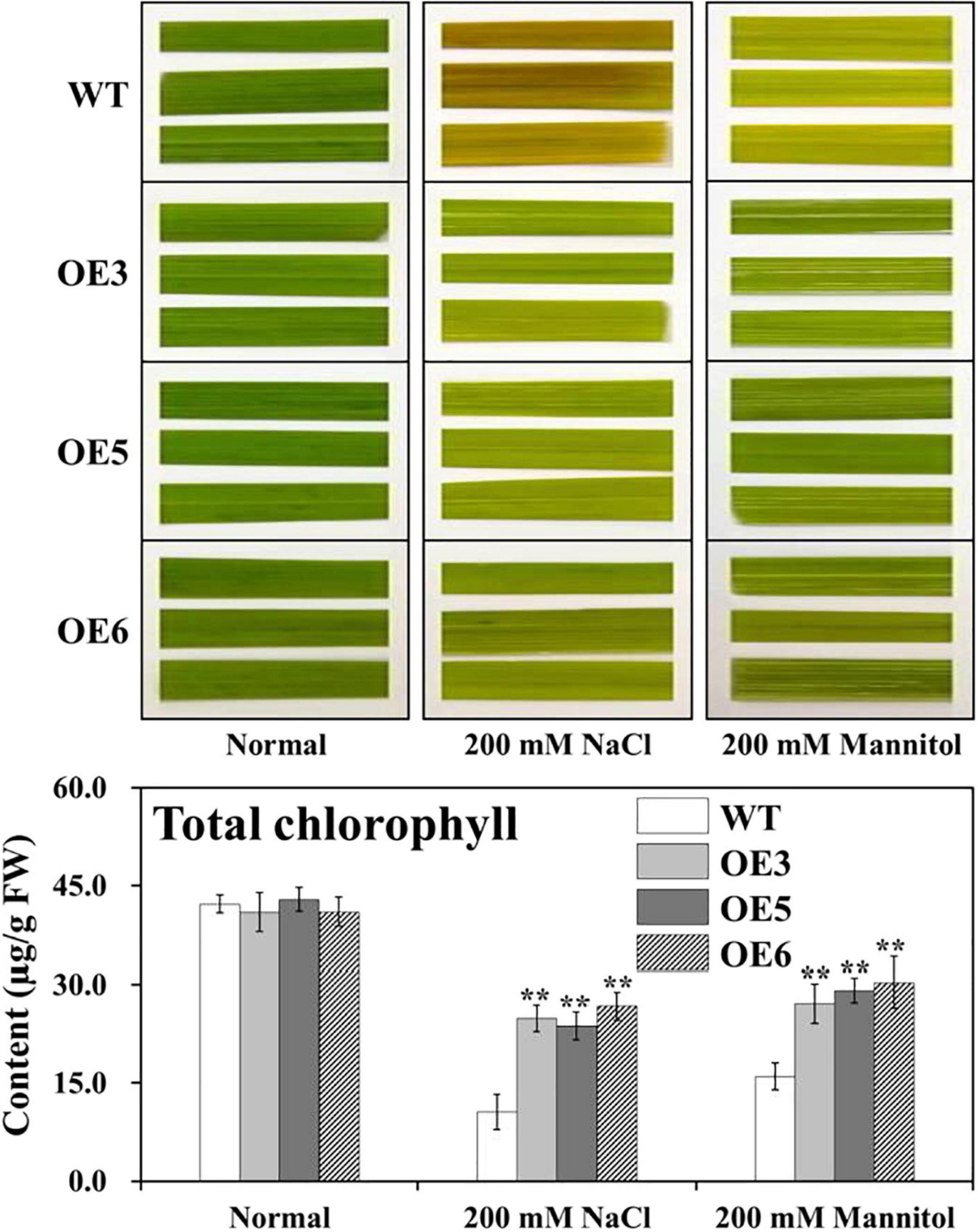
Figure 4. Assessment of the NaCl- and mannitol-induced senescence in leaf disks of various transgenic plants. Leaf disks were floated on 200 mM of NaCl and 200 mM of mannitol concentrations, respectively, for 96 h at 28°C under conditions of 28°C and a 16 h light/8 h dark photoperiod cycle. Disks floated on sterile distilled water served as an experimental control. Determination of total chlorophyll content of the leaf disks after NaCl and Mannitol treatments, respectively, are presented. Data are presented as means ± SE (n = 3). The values of * and ** indicate a significant difference from that of WT at P < 0.05 and < 0.01, respectively, by Student’s t-test.
Increased Sensitivity to Exogenous ABA in the Overexpression of OsIAA18 in Rice
To test whether the overexpression of the OsIAA18 gene can affect the sensitivity of transgenic rice to exogenous ABA, the overexpressing OsIAA18 and WT seeds were germinated in 1/2 MS medium with ABA (0 and 2.5 μM). As shown in Figure 5A, the germination rate of the overexpressing OsIAA18 lines was similar to that of WT control at 0 μM ABA. However, the germination rate of the overexpressing OsIAA18 lines was significantly decreased at 2.5 μM ABA. These results suggested that the ABA sensitivity of seed germination of overexpressing OsIAA18 rice plants was increased.
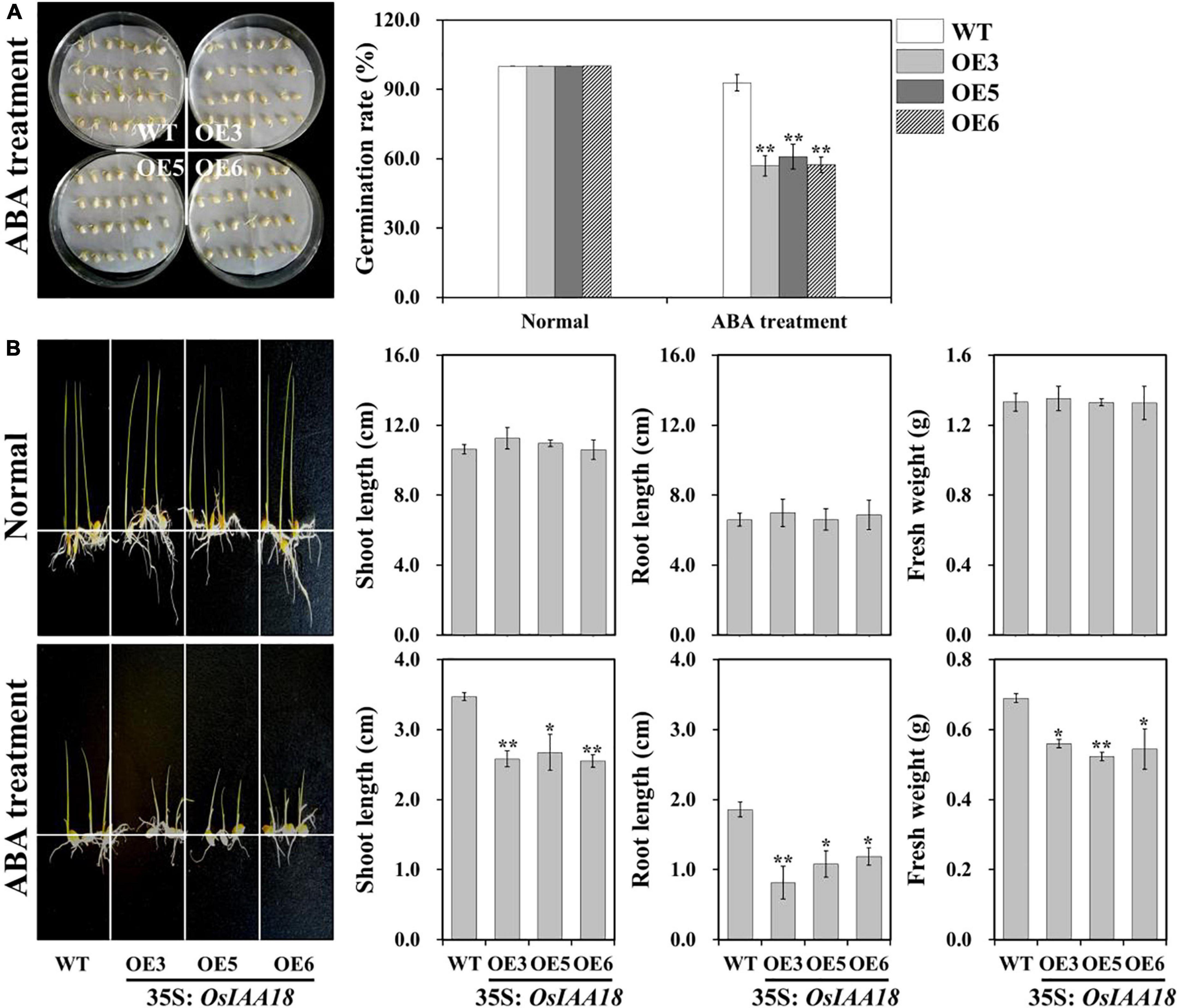
Figure 5. Increased ABA sensitivity of overexpressing OsIAA18 rice plants during germination and seedling stages. (A) Germination assay of OsIAA18 overexpression and WT seeds in 1/2 MS medium with 2.5 μM ABA. The germination rates of the treated seeds were calculated after 5 days. (B) Growth performance of overexpressing OsIAA18 and WT plants grown in 1/2 MS medium containing 0 and 2.5μM ABA. Shoot and root lengths and fresh weight measurements of the rice plants are shown in panel. Data are presented as means ± SE (n = 3). The values of * and ** indicate a significant difference from that of WT at P < 0.05 and < 0.01, respectively, by Student’s t-test.
In our later study, we tested the effect of ABA on seedling development in overexpressing OsIAA18 plants. The performance of overexpressing OsIAA18 and WT seedlings grown for 2 weeks in 1/2 MS medium with or without ABA was observed (Figure 5B). There were no significant differences in shoot and root lengths and fresh weight between the WT and transgenic rice plants grown in MS medium without ABA (Figure 5B). The shoot and root lengths and the fresh weight of overexpressing OsIAA18 plants grown in MS medium with 2.5 μM of ABA were significantly reduced compared to those of the control (Figure 5B). These results demonstrate that the overexpression of OsIAA18 makes transgenic seedlings hypersensitive to ABA in comparison to WT plants, indicating that OsIAA18 may be a positive regulator of ABA signaling in rice.
Enhanced Endogenous ABA Signaling in Overexpressed OsIAA18 Rice
Endogenous ABA levels were measured in the leaves of overexpressing OsIAA18 and WT plants under salt and drought stresses. The results showed that after salt and drought stresses, endogenous ABA levels were clearly increased in both overexpressing OsIAA18 and WT lines; the accumulation of high ABA levels was observed in the overexpressing OsIAA18 lines than that in the WT lines, while no obvious difference was found under normal conditions (Figure 6). It is hypothesized that OsIAA18 gene may be involved in regulating salt and drought tolerance by ABA biosynthesis. To further confirm this hypothesis, the activity of 9-cis-epoxycarotenoid dioxygenase (NCED), as a key rate limiting enzyme of ABA biosynthesis, and the transcript levels of OsNCED4 and OsNCED5 were analyzed in overexpressing OsIAA18 and WT plants under salt and drought stresses. As shown in Figure 6, the activity of NCED and the expression of OsNCED4 and OsNCED5 were enhanced in overexpressing OsIAA18 lines compared to WT lines. Further research found that the transcript levels of ABA-responsive signaling pathway genes, such as OsRAB16C, OsRAB16D, OsRAB21, and OsLEA3, were markedly upregulated in the overexpressing OsIAA18 lines compared to WT lines under salt and drought stresses (Figure 6). These results indicate that OsIAA18 gene may play an important role in regulating salt and drought tolerance by increasing the expression of ABA biosynthesis genes, such as OsNCED4 and OsNCED5, and upregulating the expression of OsRAB16C, OsRAB16D, OsRAB21, and OsLEA3 genes related to ABA responsive signaling pathway.
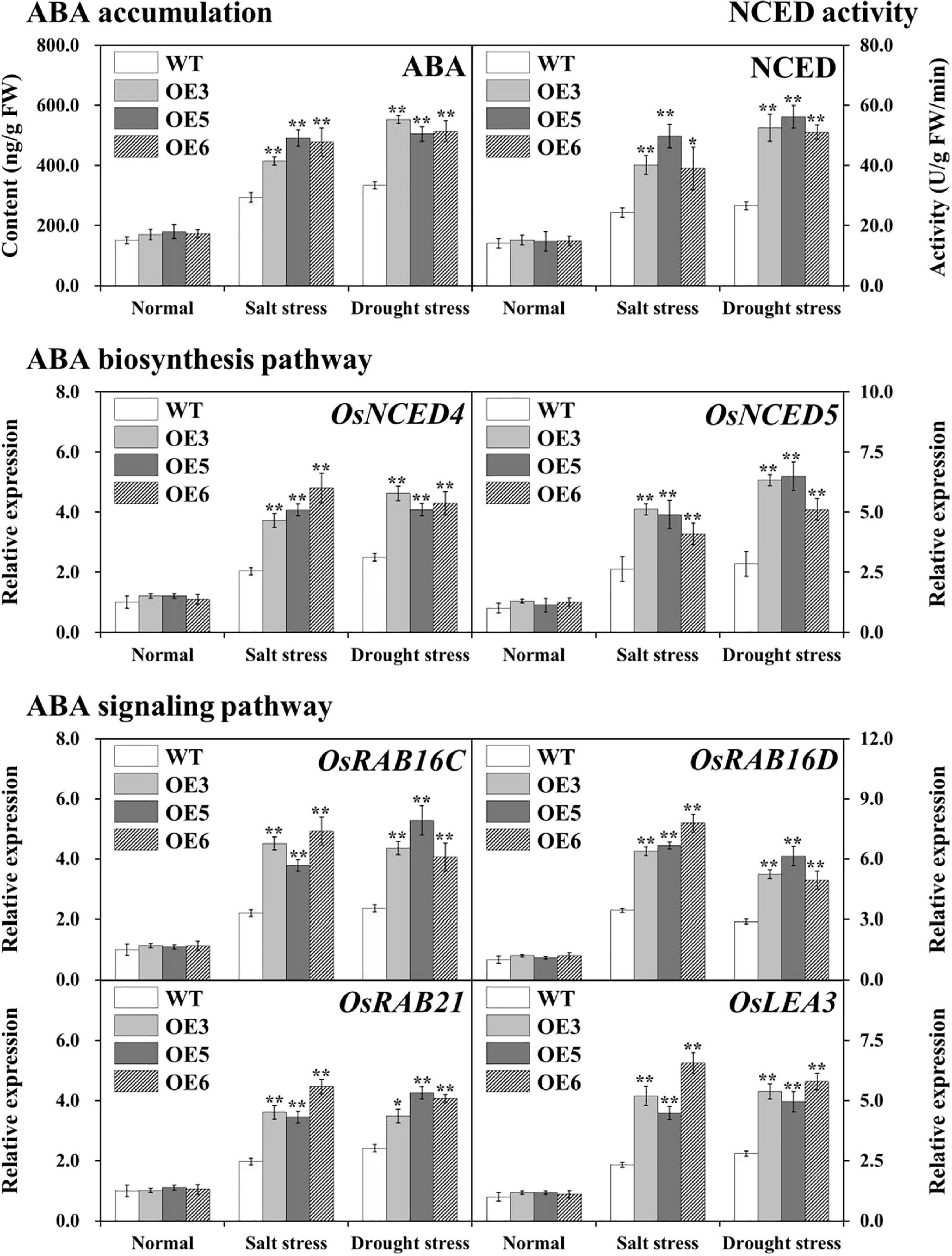
Figure 6. ABA accumulation and the expression of ABA biosynthesis and signaling pathways marker genes in the leaves of overexpressing OsIAA18 and WT plants under salt and drought stresses. Data are presented as means ± SE (n = 3). The values of * and ** indicate a significant difference from that of WT at P < 0.05 and < 0.01, respectively, by Student’s t-test.
Promoted Proline Accumulation in Overexpressing OsIAA18 Rice
Proline content was analyzed in the leaves of overexpressing OsIAA18 and WT plants under salt and drought stresses. These results showed that proline accumulation was much more significant in the overexpressing OsIAA18 lines than those of the WT seedlings under salt and drought stresses (Figure 7). Meanwhile, we performed qRT-PCR assays to further analyze the messenger RNA (mRNA) levels of key rate-limiting enzyme genes related to proline biosynthesis under salt and drought stresses. Our data indicated that the expression of OsP5CS1 and OsP5CS2, encoding the pyrroline-5-carboxylate synthase was upregulated in the overexpressing OsIAA18 lines than those of the WT seedlings under salt and drought stresses (Figure 7). These results indicate that OsIAA18 may play a critical role in stress-induced proline biosynthesis by the upregulation of proline biosynthesis genes.
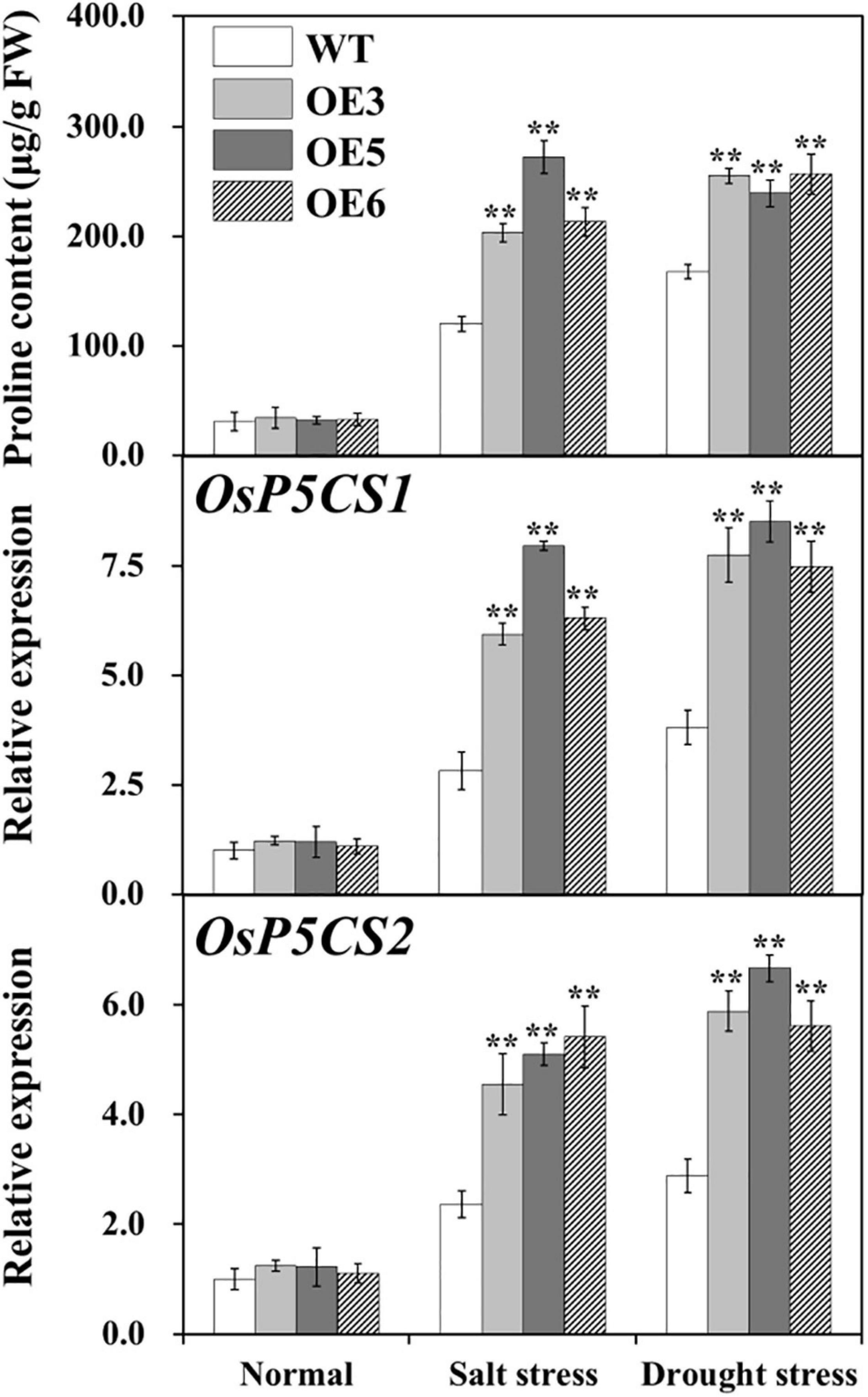
Figure 7. Free proline content and the expression of proline biosynthesis genes in the leaves of overexpressing OsIAA18 and WT plants under salt and drought stresses. Data are presented as means ± SE (n = 3). The values for * and ** indicate a significant difference from that of WT at P < 0.05 and < 0.01, respectively, by Student’s t-test.
Decreased Reactive Oxygen Species Damage in Overexpressing OsIAA18 Rice
Stress usually induces damage via oxidative damage in plants including the generation of ROS, represented H2O2 and O2– (Jiang et al., 2016). The accumulated levels of H2O2 and O2– were evaluated by means of DAB and NBT staining and H2O2 measurements in the leaves of overexpressed OsIAA18 and WT plants under salt and drought stresses. Our works showed that the overexpressed OsIAA18 lines accumulated less H2O2 and O2– than did WT lines under salt and drought stresses, whereas there was no significant difference between the transgenic plants and WT without stress (Figure 8). Meanwhile, we found that the overexpressed OsIAA18 lines had significantly lower MDA than those of the WT lines (Figure 8).
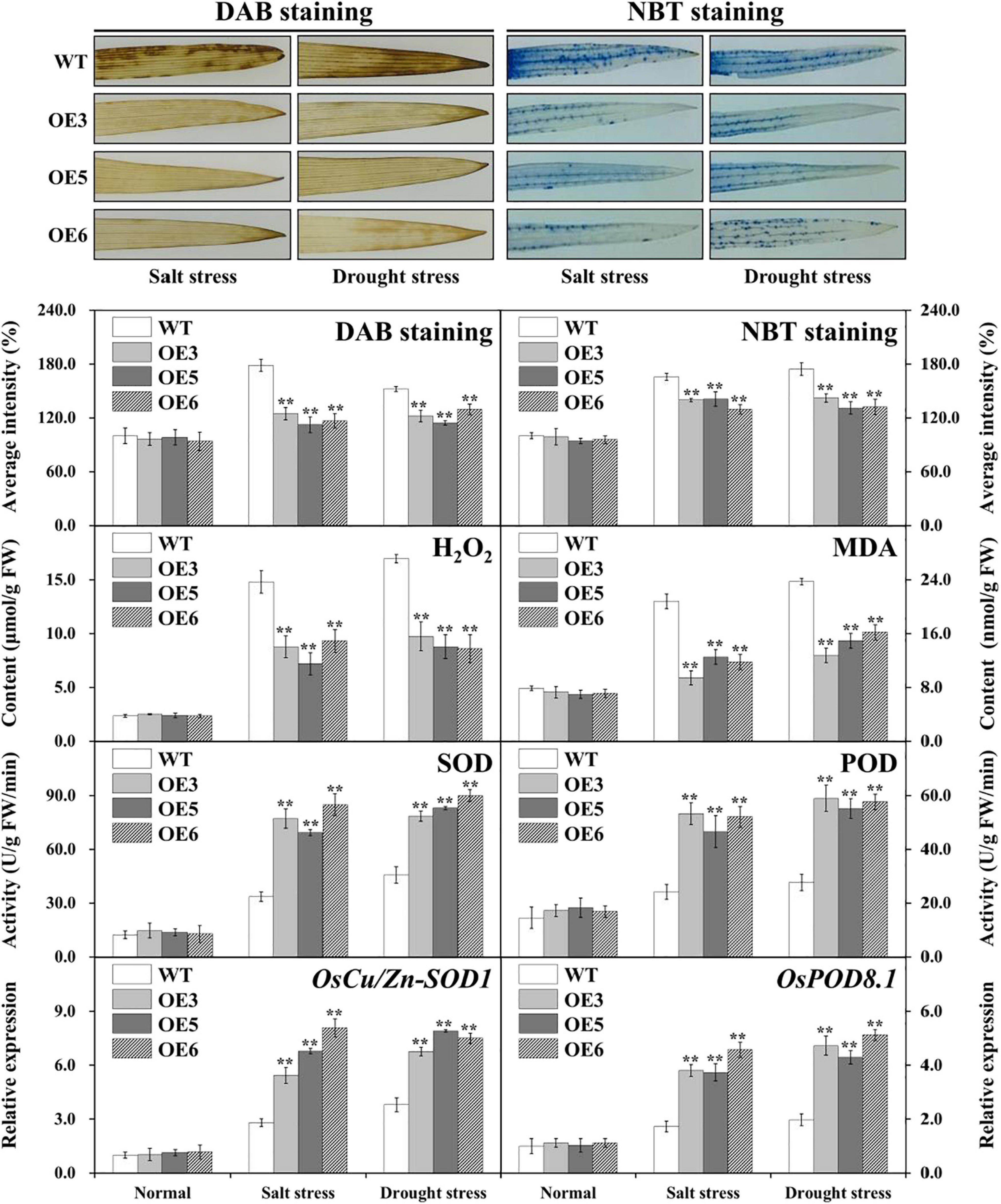
Figure 8. Decreased ROS damage in the overexpressing OsIAA18 and WT plants. DAB and NBT staining for H2O2 and O2–, H2O2 and MDA content, SOD and POD activities, expression of OsCu/Zn-SOD1 and OsPOD8.1 genes in the leaves of transgenic plants and WT under salt and drought stresses. Data are presented as means ± SE (n = 3). The values of * and ** indicate a significant difference from that of WT at P < 0.05 and < 0.01, respectively, by Student’s t-test.
Following salt and drought stresses, the activities of antioxidant enzymes, such as SOD and POD, that play an important role in ROS-scavenging mechanisms, were measured in both the overexpressing OsIAA18 and WT lines. Under the absence of stress, SOD and POD activities were not different; after salt and drought stresses, the activities of these enzymes were significantly enhanced in the overexpressing OsIAA18 lines compared to those in the WT lines (Figure 8). A qRT-PCR was used to further analyze the expression levels of OsCu/Zn-SOD1 and OsPOD8 in rice plants. Under salt and drought stresses, OsCu/Zn-SOD1 and OsPOD8 exhibited markedly higher expression in the overexpressing OsIAA18 lines than in the WT lines (Figure 8). These results suggested that the overexpression of OsIAA18 gene may enhance ROS-scavenging ability to decrease ROS damage, leading to improved salt and drought tolerance of transgenic rice lines.
Discussion
OsIAA18 Plays a Positive Role in Enhancing Salt and Drought Tolerance in Rice
The Aux/IAA protein of rice plays an important regulatory role in the developmental process of plants and their responses to stress and phytohormone treatments (Song et al., 2009; Song and Xu, 2013).
The OsIAA18 gene was upregulated when exposed to salt and drought stresses in rice (Song et al., 2009). Our previous study showed that the OsIAA18 gene was isolated from a rice cultivar, Nipponbare and the OsIAA18-overexpressing Arabidopsis plants exhibited enhanced salt and osmotic tolerance (Li G. et al., 2020). Also, VvIAA18 gene from the grapevine was cloned from PN40024 and it was found that the VvIAA18-overexpressing tobacco plants showed improved salt tolerance (Li W. et al., 2020). The OsIAA6/OsIAA20-overexpressing rice plants exhibited greater tolerance to salt and drought stresses (Jung et al., 2015; Zhang et al., 2021). In this study, we produced the transgenic plants of the rice cv. Zhonghua 11 overexpressing the OsIAA18 gene. Under normal conditions, there were no significant differences in the plant height and root length, and fresh weight and tillers were observed at the tillering stages of the rice seedlings between the overexpressing OsIAA18 and WT lines. Similar to the results reported by Jung et al. (2015) and Zhang et al. (2021), in which the overexpression of OsIAA6/OsIAA20 had no significant effects on performance, such as plant height, root length, and tiller number, under normal conditions. Under salt and drought stresses, the overexpression of OsIAA18 can significantly enhance the tolerance to these stresses in rice (Figures 2, 3). It is thought that the OsIAA18 gene plays an important role in response to salt and drought stresses of rice and can be directly used in the genetic engineering of crops for their tolerance to abiotic stresses.
OsIAA18 Confers Salt and Drought Tolerance Through an ABA-Dependent Pathway in Rice
As a central regulator of abiotic stress responses, endogenous ABA can participate in the regulation of a variety of physiological responses of plants to abiotic stresses, such as drought, salt, and chilling stress, and regulates the expression of ABA-dependent abiotic stress tolerance-responsive genes (Zhu, 2002; Sripinyowanich et al., 2013; Zhai et al., 2016; Li et al., 2017; Li G. et al., 2020). The ABA- dependent stress-responsive genes, such as OsRAB16C, OsRAB16D, OsRAB21, and OsLEA3, are well-known for their involvement in response to salt and drought stresses of rice (Redillas et al., 2012; Jiang et al., 2016; Li et al., 2017, 2018, 2019). The expression of OsIAA20 was induced by ABA treatment in rice (Song et al., 2009). The study of Zhang et al. (2021) revealed that the overexpression of OsIAA20 increased salt and drought tolerance of rice plants through an ABA pathway. The OsIAA18 gene was involved in plant responses mediated by ABA signaling transduction (Song et al., 2009). In Arabidopsis, the overexpression of OsIAA18 led to an increased ABA content, upregulation of ABA biosynthesis genes, such as AtZEP, AtNCED, AtABA2, and AtAAO, and enhanced the action of key rate-limiting NCED enzyme under salt and osmotic tolerance (Li G. et al., 2020). In the present study, the sensitivities of exogenous ABA treatment could be induced in the seedlings by the overexpression of OsIAA18, indicating that OsIAA18 might be a positive regulator of ABA signaling in rice (Figure 5). The enhanced activity of NCED and the upregulated expression of OsNCED4 and OsNCED5 and a significant increase of endogenous ABA content were observed in the overexpressing OsIAA18 and WT plants under salt and drought stresses (Figure 6). Further observation showed that the transcript levels of ABA responsive signaling pathway genes, such as OsRAB16C, OsRAB16D, OsRAB21, and OsLEA3 were markedly upregulated in the overexpressing OsIAA18 lines (Figure 6). The differences in the salt and drought tolerance between overexpressing OsIAA18 and WT lines might be, at least in part, due to the reinforced expression of OsRAB16C, OsRAB16D, OsRAB21, and OsLEA3 and possibly other contributing stress-responsive genes in the overexpressing OsIAA18 lines. The results suggest that the enhanced salt and drought tolerance exhibited by OsIAA18 overexpression might be conferred by the coordinated work of these downstream functional genes. Thus, it is suggested that the overexpression of OsIAA18 enhances the tolerance to salt and drought stresses due to the upregulation of genes involved in ABA biosynthesis, leading to the increased production of ABA, as a signaling molecule to further regulate the expression of ABA-responsive genes (Figure 9).
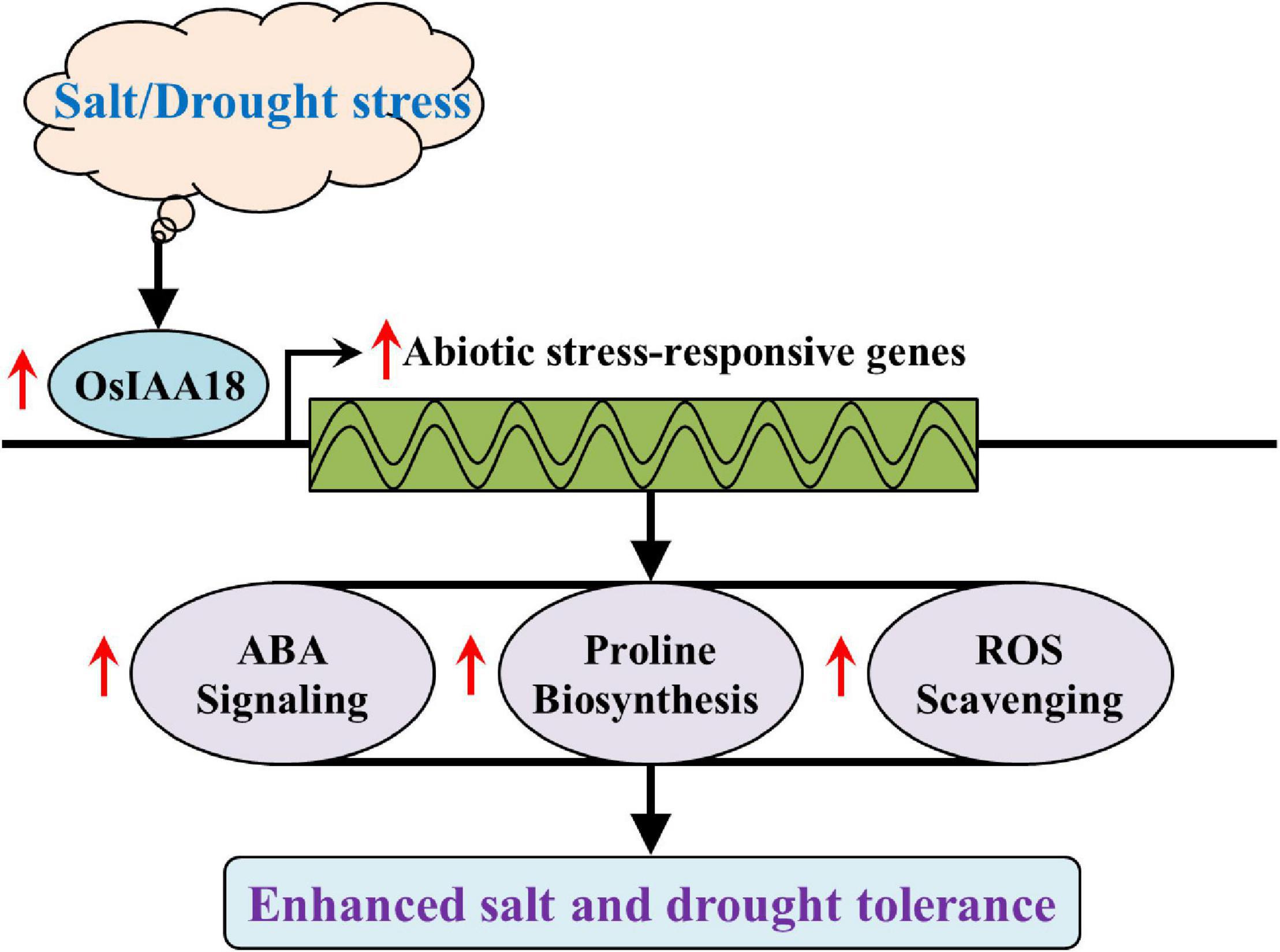
Figure 9. A proposed model explaining the function of OsIAA18 in response to salt and drought stresses. Under salt and drought stresses, the overexpression of OsIAA18 upregulated the expression of abiotic stress-responsive genes involved in ABA signaling, proline biosynthesis, and ROS scavenging, leading to increased ABA sensitivity to exogenous ABA and proline accumulation, finally enhancing salt and drought tolerance.
OsIAA18 Increases Salt and Drought Tolerance by Activating Proline Biosynthesis Pathway in Rice
Proline accumulation can enhance salt and drought tolerance in many plant species (Krasensky and Jonak, 2012; Zhang et al., 2019). ABA, salt, and drought treatments induced the expression of OsP5CS and the conferred stress tolerance was resulting from an upregulated expression of OsP5CS which increased the proline content in rice plants (Xiang et al., 2007; Sripinyowanich et al., 2013; Jiang et al., 2016; Li et al., 2018). P5CS, a catalyzing proline biosynthesis, is critical for enhanced salt and drought tolerance (Jiang et al., 2016). The upregulation of P5CS has been shown to increase proline content, resulting in enhanced salt and drought tolerance (Krasensky and Jonak, 2012; Liu et al., 2014; Jiang et al., 2016; Zhai et al., 2016; Kang et al., 2018). As shown in Figure 7, the overexpressing OsIAA18 lines exhibited the upregulated expression levels of OsP5CS1 and OsP5CS2 and increased the content of proline under salt and drought stresses. More proline accumulation in the overexpressing OsIAA18 rice lines might maintain the osmotic balance between the intracellular and extracellular environment and protect membrane integrity, resulting in enhanced salt and drought tolerance (Liu et al., 2014; Wang et al., 2016; Zhai et al., 2016; Zhang et al., 2019; Li W. et al., 2020). It is suggested that the overexpression of OsIAA18 enhances the tolerance to salt and drought stresses due to the upregulation of proline biosynthesis gene OsP5CS, which increases the production of proline, leading to enhanced salt and drought tolerance (Figure 9).
OsIAA18 Improves Salt and Drought Tolerance by Reducing Reactive Oxygen Species Accumulation in Rice
Under abiotic stresses, the increased production of ROS leads to oxidative stress in plants (Hussain et al., 2019; Zhu et al., 2020). Higher ROS levels induce lipid peroxidation in plants and cause injury to cell membranes (Zhao et al., 2018; Hussain et al., 2019). MDA, as a reflection of cellular membrane degradation or dysfunction, is an important intermediary agent in ROS-scavenging (Zhu et al., 2020). It is important to maintain a stronger ROS-scavenging ability under salt and drought stresses to alleviate the induced oxidative damage, especially in the plant leaves where photosynthesis is dramatically impacted (Gill and Tuteja, 2010). The higher ability of ROS-scavenging enzymes decreased the overaccumulated ROS levels, leading to enhanced salt and drought tolerance (Apel and Hirt, 2004; Jiang et al., 2016). SOD and POD play an important role in scavenging ROS by detoxifying H2O2 and O2– into water and stable oxygen, leading to enhanced stress tolerance (Wang et al., 2018; Zhu et al., 2020). In this study, the overexpressing OsIAA18 rice lines exhibited lower levels of DAB and NBT staining and H2O2 and MDA content under salt and drought stresses (Figure 8). This indicates a putative role of OsIAA18 in ROS-scavenging and salt and drought tolerance. We also found that the systematic upregulation of ROS scavenging genes (OsCu/Zn-SOD1 and OsPOD8.1) and significant increase of antioxidant enzyme (SOD and POD) activities were observed in the overexpressing OsIAA18 rice lines under salt and drought stresses (Figure 8). Therefore, the improved salt and drought tolerance of the transgenic rice plants might be, at least in part, due to the enhanced ROS scavenging capacity (Liu et al., 2014; Zhai et al., 2016; Wang et al., 2018; Li W. et al., 2020). It has been reported that proline is an effective scavenger of singlet oxygen and hydroxyl radicals (Liu et al., 2014; Zhai et al., 2016; Zhang et al., 2019). Our results support that more proline accumulation activates ROS scavenging system, leading to enhanced salt and osmotic tolerance in the overexpressing OsIAA18 rice lines (Zhai et al., 2016; Wang et al., 2018; Li W. et al., 2020; Figure 9).
Conclusion
In this study, the overexpression of OsIAA18 significantly enhanced tolerance to salt and drought stresses in transgenic rice plants. Our results suggest that OsIAA18 gene may play an important role in regulating salt and drought tolerance by regulating stress-induced ABA signaling. The OsIAA18 gene is a hopeful candidate for enhancing tolerance to abiotic stresses in plants.
Data Availability Statement
The datasets presented in this study can be found in online repositories. The names of the repository/repositories and accession number(s) can be found in the article/Supplementary Material.
Author Contributions
FW and HN conceived and designed the experiments. FW, HN, YL, DX, GL, FZ, and MQ performed the experiments. FW, HN, YL, DX, GW, ZL, GL, FZ, MQ, YY, ZW, BP, LH, CY, and XC analyzed the data. FW, HN, and YL wrote the paper. All authors contributed to the article and approved the submitted version.
Funding
This work was supported by the Natural Science Foundation of Jiangsu Province of China (BK20191483), the Natural Science Fund for Colleges and Universities in Jiangsu Province of China (20KJA180004), the Postgraduate Practice Innovation Program of Jiangsu Province of China (SJCX20_1339), the College Student Practice Innovation Program of Jiangsu Province of China (202111049401 and 202011049010Y), and the Talent Introduction Research Project of Huaiyin Institute of Technology (Z301B16534).
Conflict of Interest
The authors declare that the research was conducted in the absence of any commercial or financial relationships that could be construed as a potential conflict of interest.
Publisher’s Note
All claims expressed in this article are solely those of the authors and do not necessarily represent those of their affiliated organizations, or those of the publisher, the editors and the reviewers. Any product that may be evaluated in this article, or claim that may be made by its manufacturer, is not guaranteed or endorsed by the publisher.
Supplementary Material
The Supplementary Material for this article can be found online at: https://www.frontiersin.org/articles/10.3389/fpls.2021.738660/full#supplementary-material
References
Apel, K., and Hirt, H. (2004). Reactive oxygen species: metabolism, oxidative stress, and signal transduction. Annu. Rev. Plant Biol. 55, 373–399. doi: 10.1146/annurev.arplant.55.031903.141701
Berleth, T., Krogan, N. T., and Scarpella, E. (2004). Auxin signals-turning genes on and turning cells around. Curr. Opin. Plant Biol. 7, 553–563. doi: 10.1016/j.pbi.2004.07.016
Çakir, B., Kiliçkaya, O., and Olcay, A. C. (2013). Genome-wide analysis of Aux/IAA genes in Vitis vinifera: cloning and expression profiling of a grape Aux/IAA gene in response to phytohormone and abiotic stresses. Acta Physiol. Plant. 35, 365–377. doi: 10.1007/s11738-012-1079-7
Friml, J. (2003). Auxin transport-shaping the plant. Curr. Opin. Plant Biol. 6, 7–12. doi: 10.1016/S1369526602000031
Gill, S. S., and Tuteja, N. (2010). Reactive oxygen species and antioxidant machinery in abiotic stress tolerance in crop plants. Plant Physiol. Biochem. 48, 909–930. doi: 10.1016/j.plaphy.2010.08.016
Gray, W. M., Kepinski, S., Rouse, D., Leyser, O., and Estelle, M. (2001). Auxin regulates SCFTIR1-dependent degradation of AUX/IAA proteins. Nature 414, 271–276. doi: 10.1038/35104500
Guilfoyle, T. J., and Hagen, G. (2001). Auxin response factors. J. Plant Growth Regul. 10, 281–291. doi: 10.1007/s003440010026
Hagen, G., and Guilfoyle, T. (2002). Auxin-responsive gene expression: genes, promoters and regulatory factors. Plant Mol. Biol. 49, 373–385. doi: 10.1023/A:1015207114117
Hardtke, C. S., Ckurshumova, W., Vidaurre, D. P., Singh, S. A., Stamatiou, G., Tiwari, S. B., et al. (2004). Overlapping and non-redundant functions of the Arabidopsis auxin response factors MONOPTEROS and NONPHOTOTROPIC HYPOCOTYL 4. Development 131, 1089–1110. doi: 10.1242/dev.00925
Hiei, Y., Ohta, S., Komarl, T., and Kumashiro, T. (1994). Efficient transformation of rice (Oryza sativa L.) mediated by Agrobacterium and sequence analysis of boundaries of the T-DNA. Plant J. 6, 271–282. doi: 10.1046/j.1365313X.1994.6020271.x
Hiratsu, K., Matsui, K., Koyama, T., and Ohme-Takagi, M. (2003). Dominant repression of target genes by chimeric repressors that include the EAR motif, a repression domain, in Arabidopsis. Plant J. 34, 733–739. doi: 10.1046/j.1365-313X.2003.01759.x
Huang, J., Sun, S., Xu, D., Lan, H., Sun, H., Wang, Z., et al. (2012). A TFIIIA-type zinc finger protein confers multiple abiotic stress tolerances in transgenic rice (Oryza sativa L.). Plant Mol. Biol. 80, 337–350. doi: 10.1007/s11103-012-9955-5
Hussain, H. A., Men, S., Hussain, S., Chen, Y., Ali, S., Zhang, S., et al. (2019). Interactive effects of drought and heat stresses on morpho-physiological attributes, yield, nutrient uptake and oxidative status in maize hybrids. Sci. Rep. 9:3890. doi: 10.1038/s41598-019-40362-7
Jain, M., Kaur, N., Garg, R., Thakur, J. K., Tyagi, A. K., and Khurana, J. P. (2006). Structure and expression analysis of early auxin-responsive Aux/IAA gene family in rice (Oryza sativa). Funct. Integr. Genomics 6, 47–59. doi: 10.1007/s10142-005-0005-0
Jiang, Y., Qiu, Y., Hu, Y., and Yu, D. (2016). Heterologous expression of AtWRKY57 confers drought tolerance in Oryza sativa. Front. Plant Sci. 7:145. doi: 10.3389/fpls.2016.00145
Joo, J., Lee, Y. H., and Song, S. I. (2019). OsbZIP42 is a positive regulator of aba signaling and confers drought tolerance to rice. Planta 249, 1521–1533. doi: 10.1007/s00425-019-03104-7
Jun, N., Gaohang, W., Zhenxing, Z., Huanhuan, Z., Yunrong, W., and Ping, W. (2011). OsIAA23-mediated auxin signaling defines postembryonic maintenance of QC in rice. Plant J. 68, 433–442. doi: 10.1111/j.1365-313X.2011.04698.x
Jung, H., Lee, D. K., Choi, Y. D., and Kim, J. K. (2015). OsIAA6, a member of the rice Aux/IAA gene family, is involved in drought tolerance and tiller outgrowth. Plant Sci. 236, 304–312. doi: 10.1016/j.plantsci.2015.04.018
Kang, C., He, S., Zhai, H., Li, R., Zhao, N., and Liu, Q. (2018). A sweetpotato auxin response factor gene (IbARF5) is involved in carotenoid biosynthesis and salt and drought tolerance in transgenic Arabidopsis. Front. Plant Sci. 9:1307. doi: 10.3389/fpls.2018.01307
Kepinski, S., and Leyser, O. (2004). Auxin-induced SCFTIR1-Aux/IAA interaction involves stable modification of the SCFTIR1 complex. Proc. Natl. Acad. Sci. U.S.A. 101, 12381–12386. doi: 10.1073/pnas.0402868101
Kitomi, Y., Inahashi, H., Takehisa, H., Sato, Y., and Inukai, Y. (2012). OsIAA13-mediated auxin signaling is involved in lateral root initiation in rice. Plant Sci. 190, 116–122. doi: 10.1016/j.plantsci.2012.04.005
Krasensky, J., and Jonak, C. (2012). Drought, salt, and temperature stress-induced metabolic rearrangements and regulatory networks. J. Exp. Bot. 63, 1593–1608. doi: 10.1093/jxb/err460
Li, G., Ye, Y. X., Ren, X. Q., Qi, M. Y., Zhao, H. Y., and Zhou, Q. (2020). The rice Aux/IAA transcription factor gene OsIAA18 enhances salt and osmotic tolerance in Arabidopsis. Biol. Plant. 64, 454–464. doi: 10.32615/bp.2019.069
Li, J., Guo, X., Zhang, M., Wang, X., Zhao, Y., Yin, Z., et al. (2018). OsERF71 confers drought tolerance via modulating aba signaling and proline biosynthesis. Plant Sci. 270, 131–139. doi: 10.1016/j.plantsci.2018.01.017
Li, J., Li, Y., Yin, Z., Jiang, J., Zhang, M., Guo, X., et al. (2017). OsASR5 enhances drought tolerance through a stomatal closure pathway associated with ABA and H2O2 signalling in rice. Plant Biotechnol. J. 15, 183–196. doi: 10.1111/pbi.12601
Li, W., Dang, C. X., Ye, Y. X., Wang, Z. X., Hu, L. B., Zhang, F., et al. (2020). Overexpression of grapevine VvIAA18 gene enhanced salt tolerance in tobacco. Int. J. Mol. Sci. 21:1323. doi: 10.3390/ijms21041323
Li, Z. J., Fu, X. Y., Tian, Y. S., Xu, J., Gao, J. J., Wang, B., et al. (2019). Overexpression of a trypanothione synthetase gene from Trypanosoma cruzi, TcTrys, confers enhanced tolerance to multiple abiotic stresses in rice. Gene 710, 279–290. doi: 10.1016/j.gene.2019.06.018
Liscum, E., and Reed, J. W. (2002). Genetics of Aux/IAA and ARF action in plant growth and development. Plant Mol. Biol. 49, 387–400. doi: 10.1023/A:1015255030047
Liu, D. G., He, S. Z., Zhai, H., Wang, L. J., Zhao, Y., Wang, B., et al. (2014). Overexpression of IbP5CR enhances salt tolerance in transgenic sweetpotato. Plant Cell Tissue Organ Cult. 117, 1–16. doi: 10.1007/s11240-013-0415-y
Liu, H., Li, X., Xiao, J., and Wang, S. (2012). A convenient method for simultaneous quantification of multiple phytohormones and metabolites: application in study of rice-bacterium interaction. Plant Methods 8:2. doi: 10.1186/1746-4811-8-2
Munns, R., and Tester, M. (2008). Mechanisms of salinity tolerance. Annu. Rev. Plant Biol. 59, 651–681. doi: 10.1146/annurev.arplant.59.032607.092911
Nakamura, A., Umemura, I., Gomi, K., Hasegawa, Y., Kitano, H., Sazuka, T., et al. (2006). Production and characterization of auxininsensitive rice by overexpression of a mutagenized rice IAA protein. Plant J. 46, 297–306. doi: 10.1111/j.1365-313X.2006.02693.x
Patra, B., Ray, S., Richter, A., and Majumder, A. L. (2010). Enhanced salt tolerance of transgenic tobacco plants by co-expression of PcINO1 and McIMT1 is accompanied by increased level of myo-inositol and methylated inositol. Protoplasma 245, 143–152. doi: 10.1007/s00709-010-0163-3
Ramos, J. A., Zenser, N., Leyser, O., and Callis, J. (2001). Rapid degradation of auxin/indoleacetic acid proteins requiresconserved amino acids of domain II and is proteasome dependent. Plant Cell 13, 2349–2360. doi: 10.1105/tpc.010244
Redillas, M., Park, S. H., Lee, J. W., Kim, Y. S., Jin, S. J., Jung, H., et al. (2012). Accumulation of trehalose increases soluble sugar contents in rice plants conferring tolerance to drought and salt stress. Plant Biotechnol. Rep. 6, 89–96. doi: 10.1007/s11816-011-0210-3
Reed, J. W. (2001). Roles and activities of Aux/IAA proteins in Arabidopsis. Trends Plant Sci. 6, 420–425. doi: 10.1016/S1360-1385(01)02042-8
Schmittgen, T. D., and Livak, K. J. (2008). Analyzing real-time PCR data by the comparative CT method. Nat. Protoc. 3, 1101–1108. doi: 10.1038/nprot.2008.73
Song, Y. L., and Xu, Z. F. (2013). Ectopic overexpression of an AUXIN/INDOLE-3-ACETIC ACID (Aux/IAA) gene OsIAA4 in rice induces morphological changes and reduces responsiveness to auxin. Int. J. Mol. Sci. 14, 13645–13656. doi: 10.3390/ijms140713645
Song, Y. L., Wang, L., and Xiong, L. Z. (2009). Comprehensive expression profiling analysis of OsIAA gene family in developmental processes and in response to phytohormone and stress treatments. Planta 229, 577–591. doi: 10.1021/ic048518h
Sripinyowanich, S., Klomsakul, P., Boonburapong, B., Bangyeekhun, T., Asami, T., Gu, H., et al. (2013). Exogenous ABA induces salt tolerance in indica rice (Oryza sativa L.): the role of OsP5CS1 and OsP5CR gene expression during salt stress. Environ. Exp. Bot. 86, 94–105. doi: 10.1016/j.envexpbot.2010.01.009
Thakur, J. K., Jain, M., Tyagi, A. K., and Khurana, J. P. (2005). Exogenous auxin enhances the degradation of a light down-regulated and nuclear-localized OsiIAA1, an Aux/IAA protein from rice, via proteasome. Biochim. Biophys. Acta Gene Struct. Expr. 1730, 196–205. doi: 10.1016/j.bbaexp.2005.08.002
Thakur, J. K., Tyagi, A. K., and Khurana, J. P. (2001). OsIAA1, an Aux/IAA cDNA from rice, and changes in its expression as influenced by auxin and light. DNA Res. 8, 193–203. doi: 10.1093/dnares/8.5.193
Tiwari, S. B., Hagen, G., and Guilfoyle, T. (2003). The roles of auxin response factor domains in auxin-responsive transcription. Plant Cell 15, 533–543. doi: 10.2307/3871883
Ulmasov, T., Murfett, J., Hagen, G., and Guilfoyle, T. J. (1997). Aux/IAA proteins repress expression of reporter genes containing natural and highly active synthetic auxin response elements. Plant Cell 9, 1963–1971. doi: 10.2307/3870557
Vogler, H., and Kuhlemeier, C. (2003). Simple hormones but complex signalling. Curr. Opin. Plant Biol. 6, 51–56. doi: 10.1016/S1369-5266(02)00013-4
Wang, D., Pei, K., Fu, Y., Sun, Z., Li, S., Liu, H., et al. (2007). Genome-wide analysis of the auxin response factors (ARF) gene family in rice (Oryza sativa). Gene 394, 13–24. doi: 10.1016/j.gene.2007.01.006
Wang, F. B., Kong, W. L., Wong, G., Fu, L. F., Peng, R. H., Li, Z. J., et al. (2016). AtMYB12 regulates flavonoids accumulation and abiotic stress tolerance in transgenic Arabidopsis thaliana. Mol. Genet. Genomics 291, 1545–1559. doi: 10.1007/s00438-016-1203-2
Wang, F. B., Ren, G. L., Li, F. S., Qi, S. T., Xu, Y., Wang, B. W., et al. (2018). A chalcone synthase gene AeCHS from Abelmoschus esculentus regulates flavonoids accumulation and abiotic stress tolerance in transgenic Arabidopsis. Acta Physiol. Plant. 40:97. doi: 10.1007/s11738-018-2680-1
Xiang, Y., Huang, Y., and Xiong, L. (2007). Characterization of stress-responsive CIPK genes in rice for stress tolerance improvement. Plant Physiol. 144, 1416–1428. doi: 10.1104/pp.107.101295
Yamamoto, K. T. (1994). Further characterization of auxin-regulated mRNAs in hypocotyl sections of mung bean [Vigna radiata (L.) Wilczek]: sequence homology to genes for fatty-acid desaturases and atypical late-embryogenesis-abundant protein, and the mode of expression of the mRNAs. Planta 192, 359–364. doi: 10.1007/BF00198571
Zhai, H., Wang, F. B., Si, Z. Z., Huo, J. X., Xing, L., An, Y. Y., et al. (2016). A myo-inositol-1-phosphate synthase gene, IbMIPS1, enhances salt and drought tolerance and stem nematode resistance in transgenic sweetpotato. Plant Biotechnol. J. 14, 592–602. doi: 10.1111/pbi.12402
Zhang, A. Y., Yang, X., Lu, J., Song, F. Y., Sun, J. H., Wang, C., et al. (2021). OsIAA20, an Aux/IAA protein, mediates abiotic stress tolerance in rice through an ABA pathway. Plant Sci. 308:110903. doi: 10.1016/j.plantsci.2021.110903
Zhang, H., Gao, X. R., Zhi, Y. H., Li, X., Zhang, Q., Niu, J. B., et al. (2019). A non-tandem CCCH-type zinc-finger protein, IbC3H18, functions as a nuclear transcriptional activator and enhances abiotic stress tolerance in sweet potato. New Phytol. 223, 1918–1936. doi: 10.1111/nph.15925
Zhang, H., Liu, Y., Wen, F., Yao, D., Wang, L., Guo, J., et al. (2014). A novel rice C2H2-type zinc finger protein, ZFP36, is a key player involved in abscisic acid-induced antioxidant defence and oxidative stress tolerance in rice. J. Exp. Bot. 65, 5795–5809. doi: 10.1093/jxb/eru313
Zhao, Q., Zhou, L., Liu, J., Du, X., Asad, M. A., Huang, F., et al. (2018). Relationship of ROS accumulation and superoxide dismutase isozymes in developing anther with floret fertility of rice under heat stress. Plant Physiol. Biochem. 122, 90–101. doi: 10.1016/j.plaphy.2017.11.009
Zhu, J. K. (2002). Salt and drought stress signal transduction in plants. Annu. Rev. Plant Biol. 53, 247–273. doi: 10.1146/annurev.arplant.53.091401.143329
Zhu, M. D., Zhang, M., Gao, D. J., Zhou, K., and Lv, Y. M. (2020). Rice OsHSFA3 gene improves drought tolerance by modulating polyamine biosynthesis depending on abscisic acid and ros levels. Int. J. Mol. Sci. 21, 1857. doi: 10.3390/ijms21051857
Keywords: OsIAA18, rice, overexpression, salt and drought tolerance, ABA signaling
Citation: Wang F, Niu H, Xin D, Long Y, Wang G, Liu Z, Li G, Zhang F, Qi M, Ye Y, Wang Z, Pei B, Hu L, Yuan C and Chen X (2021) OsIAA18, an Aux/IAA Transcription Factor Gene, Is Involved in Salt and Drought Tolerance in Rice. Front. Plant Sci. 12:738660. doi: 10.3389/fpls.2021.738660
Received: 09 July 2021; Accepted: 19 October 2021;
Published: 18 November 2021.
Edited by:
Luis E. Hernandez, Autonomous University of Madrid, SpainReviewed by:
Xin Li, Tea Research Institute, Chinese Academy of Agricultural Sciences (CAAS), ChinaBing-kai Hou, Shandong University, China
Copyright © 2021 Wang, Niu, Xin, Long, Wang, Liu, Li, Zhang, Qi, Ye, Wang, Pei, Hu, Yuan and Chen. This is an open-access article distributed under the terms of the Creative Commons Attribution License (CC BY). The use, distribution or reproduction in other forums is permitted, provided the original author(s) and the copyright owner(s) are credited and that the original publication in this journal is cited, in accordance with accepted academic practice. No use, distribution or reproduction is permitted which does not comply with these terms.
*Correspondence: Feibing Wang, d2FuZ2ZlaWJpbmcxOTg2QDE2My5jb20=
†These authors have contributed equally to this work