- Department of Plant and Environmental Sciences, Faculty of Science, University of Copenhagen, Taastrup, Denmark
Plants have evolved multiple strategies to survive and adapt when confronting the changing climate, including elevated CO2 concentration (e[CO2]) and intensified drought stress. To explore the role of abscisic acid (ABA) in modulating the response of plant water relation characteristics to progressive drought under ambient (a[CO2], 400 ppm) and e[CO2] (800 ppm) growth environments, two tomato (Solanum lycopersicum) genotypes, Ailsa Craig (AC) and its ABA-deficient mutant (flacca), were grown in pots, treated with or without exogenous ABA, and exposed to progressive soil drying until all plant available water in the pot was depleted. The results showed that exogenous ABA application improved leaf water potential, osmotic potential, and leaf turgor and increased leaf ABA concentrations ([ABA]leaf) in AC and flacca. In both genotypes, exogenous ABA application decreased stomatal pore aperture and stomatal conductance (gs), though these effects were less pronounced in e[CO2]-grown AC and gs of ABA-treated flacca was gradually increased until a soil water threshold after which gs started to decline. In addition, ABA-treated flacca showed a partly restored stomatal drought response even when the accumulation of [ABA]leaf was vanished, implying [ABA]leaf might be not directly responsible for the decreased gs. During soil drying, [ABA]leaf remained higher in e[CO2]-grown plants compared with those under a[CO2], and a high xylem sap ABA concentration was also noticed in the ABA-treated flacca especially under e[CO2], suggesting that e[CO2] might exert an effect on ABA degradation and/or redistribution. Collectively, a fine-tune ABA homeostasis under combined e[CO2] and drought stress allowed plants to optimize leaf gas exchange and plant water relations, yet more detailed research regarding ABA metabolism is still needed to fully explore the role of ABA in mediating plant physiological response to future drier and CO2-enriched climate.
Introduction
Elevated atmospheric carbon dioxide concentrations (e[CO2]), a major component of climate change, causes an increase in global mean surface temperature (Jia et al., 2019). Along with shifting rainfall patterns and reduced freshwater resources, more frequent occurrence of drought stress has become a major constraint on plant growth and productivity (Trenberth et al., 2014; Berg and Sheffield, 2018). It is well known that both e[CO2] and drought stress influence plant water relations through the regulation of plant hormone abscisic acid (ABA), though the underlying mechanisms vary between the two environmental stimuli (Becklin et al., 2017; Li S. et al., 2020).
e[CO2] has multiple physiological effects on plant water relations. Generally, e[CO2] decreases stomatal conductance (gs), thus optimizing water use efficiency (Hatfield and Dold, 2019). Meanwhile, an improved carbon assimilation rate together with an enhanced accumulation of solutes under e[CO2] allow plants to achieve cell water homeostasis and maintain favorable leaf turgor through osmotic adjustment (OA) especially under abiotic stress (Wullschleger et al., 2002; Pérez-López et al., 2010). e[CO2]-induced positive effects on plants tend to be more pronounced under drought (Li et al., 2017; Uddin et al., 2018). In tomato and grapevine, plants grown at e[CO2] could sustain high levels of carbon assimilation rate for a longer period under drought stress due to the delayed drought effects on stomatal behavior (da Silva et al., 2017; Liu et al., 2019). Hydraulic adjustment is also one of the plants’ strategies to cope with drought stress (Comstock, 2002). Avila et al. (2020) found that coffee plants grown at e[CO2] could better maintain leaf water potential (Ψleaf) and hydraulic conductance than their ambient [CO2]-counterparts under drought stress, thereby improving plant fitness. Although early studies have reported that e[CO2]-induced stomatal closure could reduce plant water consumption hereby increasing the availability of water in the soil during drought (Field et al., 1995), recent studies revealed that plant grown at e[CO2] might depleted soil water faster due to enlarged leaf area (Temme et al., 2018; Liu et al., 2019). Haworth et al. (2016) also indicated that the reduced effectiveness of stomatal closure at e[CO2] could impair crops’ tolerance to severe drought despite of an improved water use efficiency.
Abscisic acid is involved in both the e[CO2]- and drought stress-modulated plant water relation regulatory networks (Li S. et al., 2020). When plants are exposed to drought stress, rapid biosynthesis of ABA in roots is triggered, which is then transported from roots to leaves via the xylem to induce stomatal closure. The ABA-based root-to-shoot signaling has been considered the primary stomatal regulation mechanism in plants’ exposure to soil water deficits (Zhang et al., 1987; Zhang and Davies, 1990). However, root-sourced drought stress ABA-signaling theory has been challenged by reciprocal grafting studies on ABA biosynthetic mutants, revealing that leaf-sourced ABA could predominantly regulate stomatal aperture under rapid external pressure or long-term salinity/drought stress (Holbrook et al., 2002; Chen et al., 2003; Zhang et al., 2018). In addition, it is worthy of note that a significant proportion of root ABA was found to be derived from leaves and shoot (Ikegami et al., 2009; Ernst et al., 2010). These contrasting results raise questions about ABA homeostasis in plants when exposed to drought, including biosynthesis, catabolism, and transport. Furthermore, plants have evolved two different water management strategies: isohydric plants maintain a favorable Ψleaf and the integrity of the hydraulic system through an early stomatal closure, whereas anisohydric plants tend to keep stomata open for longer periods at a cost of hydraulic dysfunction (Sade et al., 2012). It has been reported that tomato plants exhibit an isohydric behavior, and this physiological trait is linked to the interaction of hydraulic and chemical (i.e., ABA) signals (Moshelion et al., 2015). Furthermore, our previous studies found that water management strategies of tomato plants could be modulated by CO2 growth environment. Under e[CO2], hydraulic signal (i.e., leaf turgor) rather than ABA predominantly controls stomatal aperture during soil drying process (Yan et al., 2017; Wei et al., 2020).
Nevertheless, ABA plays an obligatory role in various physiological responses of plants grown under the e[CO2] environment, including altered stomatal behaviors (Chater et al., 2015; Liu et al., 2019), depression on hydraulic conductance (Fang et al., 2019), and subsequently reduced water loss and enhanced leaf turgor (Huang et al., 2019; Wei et al., 2020). Many reports have addressed the role of ABA in amplifying the [CO2] effects on stomatal behavior (McAdam et al., 2011; Engineer et al., 2016; Hsu et al., 2018), and increases in ABA concentration in plants grown under e[CO2] have been observed in different species (Zou et al., 2007; Li B. et al., 2020). Furthermore, altered sensitivity of stomata to ABA at e[CO2] has been proposed (Rasehke, 1975; Buncec, 1998). Recently, Li B. et al. (2020) found that increased ABA content at e[CO2] could enhance soybean tolerance to drought stress, consistent with the findings by Gray et al. (2016) that e[CO2]-grown soybean represented a stronger response to ABA under soil drying. However, these results did not show any difference in hydraulic conductance, xylem pH and Ψleaf caused by e[CO2]. In tomato plants, our previous studies showed that plants grown at e[CO2] possessed a reduced sensitivity of stomata to leaf/xylem sap ABA during soil drying (Yan et al., 2017; Liu et al., 2019). A high xylem pH and a low hydraulic conductance had been reported in tomato plants grown under e[CO2] (Fang et al., 2019), which would modulate the ABA signaling thus affecting the gs sensitivity to drought stress.
The ABA-deficit mutant flacca has been widely used to investigate the function of ABA due to its significantly lower ABA content compared to its wild type counterpart, Ailsa Craig (AC) (Sagi et al., 2002). Due to the higher stomatal density and greater stomatal size in flacca compared to the wild type under either well-watered or drought-stressed conditions (Fang et al., 2019; Innes et al., 2021; Li and Liu, 2021), it is more vulnerable to adverse environments, including soil drought and high evaporative demand conditions. By using wild type tomato and flacca plants, Wei et al. (2020) and Li et al. (2021) found that e[CO2] decreased gs, retarded stomatal drought response, and reduced hydraulic conductance in an ABA-dependent pathway. Namely, these effects were absent or attenuated in flacca plants. Some researches about exogenous ABA application have been carried out on flacca as well as another ABA-deficit mutant sitiens (Tal et al., 1979; Sharp et al., 2000; Aroca et al., 2008), together with other treatments, to explore the abnormal phenotype caused by ABA deficit. Early studies by Tal et al. (1979) and Sharp et al. (2000) have reported that the retarded plant growth and abnormal stomatal behavior in flacca was associated with overproduction of ethylene and could be recovered by exogenous ABA application. Therefore, to investigate the role of ABA in e[CO2]-modulated leaf gas exchange and plant water relation characteristics under drought stress, two tomato genotypes (AC and flacca) differing in the endogenous ABA concentrations were grown at two levels of [CO2] (400 and 800 ppm), treated with or without exogenous ABA and exposed to progressive soil drying. We hypothesized that (1) exogenous ABA priming would rescue the stomatal response of flacca to e[CO2] and soil drying and that (2) the effects of exogenous ABA on leaf gas exchange and water relations might influence e[CO2]-modulated stomatal drought response.
Materials and Methods
Plant Materials and Growth Conditions
Seeds of isogenic tomato cv. AC and its ABA-deficient tomato mutant (flacca) (Solanum lycopersicum) were provided by the Lancaster Environment Centre (Lancaster University, United Kingdom) and grown in climate-controlled greenhouses at the Faculty of Science, University of Copenhagen, Taastrup, Denmark. Due to the impairment in the oxidation of ABA-aldehyde to ABA, flacca had lower endogenous ABA concentrations compared to AC (Sagi et al., 2002). At the 4-leaf stage, the seedlings were transplanted to 4 L pots filled with 2.2 kg of peat material (Plugg-och Såjord-Dry matter ca.110 kg m–3, organic matter >95%, pH 5.5–6.5 and EC 1.5–2.5 mS cm–1). In total, 4 weeks after transplanting, perlite was used to cover the soil surface to minimize soil evaporation, and fertilizers as (NH4)2SO4 (2.6 g) and H2KPO4 (1.5 g) per pot were added together with irrigation water to each pot to avoid nutrient deficiency.
From sowing, the plants were grown in two greenhouse cells with CO2 concentrations of 400 ppm (ambient CO2, a[CO2]) and 800 ppm (elevated CO2, e[CO2]), respectively. The [CO2] in the cells was sustained by pure CO2 emission from a bottle tank and distributed evenly by the internal ventilation system. The [CO2] in the cells was monitored every 6 s by a CO2 Transmitter Series GMT220 (Vaisala Group, Helsinki, Finland). The average daily [CO2] in each cell during treatments are shown in Figure 1. The climate conditions in the two glasshouse cells were set at: 20/16 ± 2°C day/night air temperature, 60 ± 2% relative humidity (RH), 16 h photoperiod and >300 μmol m–2 s–1 photosynthetically active radiation (PAR) supplied by sunlight plus LED lamps (Philips GreenPower LED toplighting, Denmark). The average temperature, RH, vapor pressure deficiency (VPD), and daily [CO2] in the cells during the experiment are shown in Supplementary Figure 1.
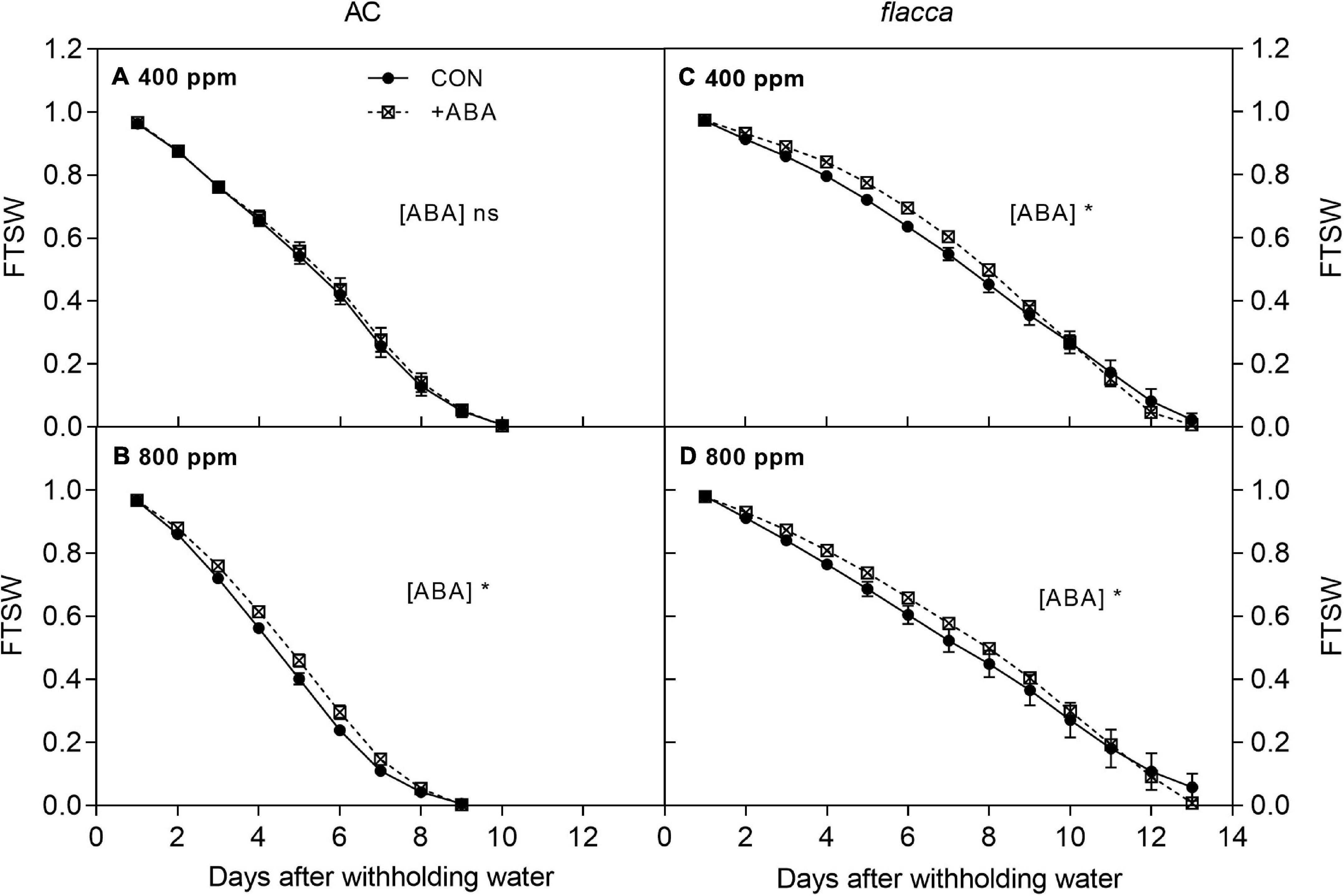
Figure 1. Changes in the fraction of transpirable soil water (FTSW) in AC (A,B) and flacca (C,D) grown under ambient (400 ppm) and elevated (800 ppm) CO2 concentrations during progressive soil drying. CON indicates the control treatment sprayed with water, +ABA indicates exogenous ABA application. Error bars indicate the standard error of the means (S.E.) (N = 3–4). *Represents the significant differences among CON and +ABA treatments in ANCOVA (Days as a covariate) at p < 0.05; ns denotes no significant difference.
Exogenous Abscisic Acid Application and Progressive Soil Drying Treatments
The sowing and growth of AC and flacca were conducted consecutively, and all treatments for the two genotypes were carried out separately. In each cell and genotype, 40 plants were established and constantly irrigated to 90% of the pot-holding capacity. Exogenous ABA application started 20 days after transplanting for three successive days. On each day, half of the plants were sprayed with 20 μM ABA (Sigma) twice a day on the whole plant at 10:00 and 15:00 h, respectively; the other half was sprayed with deionized water as non-ABA-treated control. All the solutions contained 0.1% (v/v) Tween-20 and 0.1% (v/v) ethanol.
In total, 1 day after finishing exogenous ABA application, four plants of each treatment (eight treatments in total) were harvested as initial control, then progressive soil drying was commenced. During soil drying, for each genotype, half of the plants were well watered to 95% of the pot’s water-holding capacity, and the remaining half was subjected to progressive soil drying by withholding irrigation from pots until all the transpirable soil water was depleted. Soil water content in the pot was expressed as the fraction of transpirable soil water (FTSW) (see below). In addition to the first harvest as initial control when FTSW was ca. 0.95, there were two destructive harvests during the soil drying period: the second was conducted when FTSW was ca. 0.50, and the last harvest was conducted at the end of soil drying when FTSW below 0.1. A total of four biological replicates (four individual plants) for each treatment were harvested. Due to the different water consumption rates between the two genotypes and the two [CO2] levels, AC grown at a[CO2] and e[CO2] spent 10 and 9 days to reach the end of drought stress, respectively; flacca grown at both [CO2] environments spent 13 days. Therefore, plants among different treatments were harvested on different days but at the same three FTSW points. The experimental treatments for AC and flacca were successively conducted, this might have caused the slightly different climatic conditions (see Supplementary Figure 1) between the two genotypes.
Soil Water Status
Soil water status was measured daily by weighing the pots with an Analytical Balance (Sartorius Model QA35EDE-S) at 15:30 h and expressed as FTSW. The daily value of FTSW was estimated as the ratio between the amounts of transpirable soil water remaining in the pots and the total transpirable soil water (TTSW). TTSW was defined as the difference of pot weight between full water holding capacity and when all the transpirable soil water was depleted, and calculated as:
where WTf is the pot weight at full water holding capacity (ca. 3.6 kg)., and WTe is the pot weight at the end of soil drying (ca. 1.8 kg).
Compared to our previous study (Wei et al., 2020), here the definition of FTSW was different which resulted in the longer duration for flacca plants to deplete the TTSW in relation to AC plants. The reason behind this was that exogenous ABA application would affect the stomatal conductance (gs) for both genotypes, thus using a common TTSW value would allow us to compare the gs response among the treatments on the same base. Therefore, for all treatments, the FTSW was calculated as follows:
where WTn is the pot weight on a given date, and WTe is pot weight at the end of soil drying.
Stomatal Pore Aperture and Stomatal Conductance Measurements
A total of 1 day after exogenous ABA application (before soil drying), stomatal pore aperture (SA, μm2) was determined on four replicates (10 stomata for one replicates) following the method described by Yan et al. (2012). Stomata were observed under a LEITZ DMRD microscope camera system (Leica Microscope and System GmbH, D 35530, Wetzlar, Germany) equipped with a digital camera. Stomatal pore aperture length (Wa) and pore aperture width (La) were measured by ImageJ software [Version 1.51k, Wayne Rasband, National Institutes of Health, United States, Java 1.6.0–24 (64 bit)]. Then SA was calculated as: (π × Wa × La)/4.
During progressive soil drying, gs (mol m–2 s–1) were measured on the last youngest upper canopy fully expanded leaves between 9:00 and 12:00 h with a portable photosynthetic system (LiCor-6400XT, LI-Cor, Lincoln, NE, United States). Measurements were performed on one leaf per plant and four biological replicates for each treatment at 22°C cuvette temperature, 1500 μmol m–2 s–1 PAR, and [CO2] of 400 ppm for a[CO2] and 800 ppm for e[CO2] growth environments, respectively.
Determination of Plant Water Relations and Plant Growth
At each harvest, after gas exchange measurements, the same leaf was excised for the determination of midday leaf water potential (Ψleaf, MPa) with a scholander-type pressure chamber (Soil Moisture Equipment Corp., Santa Barbara, CA, United States) following the method described by Liu et al. (2006). Then the excised leaves were cut into two parts, frozen in liquid nitrogen, and stored at −80°C for later determination of osmotic potential (Ψπ, MPa) and leaf ABA concentration ([ABA]leaf, μg g–1 FW). Ψπ was measured at 20°C with a psychrometer (C-52 sample chambers, Wescor Inc., Logan, UT, United States) connected to a microvoltmeter (HR-33T, Wescor Inc., Logan, UT, United States). Turgor pressure (Ψp, MPa) was then calculated as follows: Ψleaf–Ψπ. Leaf dry weight (LDW) (g) and stem dry weight (SDW) (g) were determined at each harvest.
Determination of Leaf and Xylem Sap Abscisic Acid Concentrations
At each harvest, xylem saps were collected with a scholander-type pressure chamber (AGRSCI, KVL, Denmark) according to Liu et al. (2006), then stored at −80°C for determination of xylem ABA concentrations ([ABA]xylem, pmol ml–1). [ABA]leaf and [ABA]xylem were determined by Enzyme-linked immunosorbent assay following the protocol of Asch (2000).
Statistical Analyses
The responses of gs to soil drying was described by a linear-plateau model (Faralli et al., 2019):
where gs ini denotes initial gs; C denotes the FTSW threshold at which y started to diverge from gs ini; and A was the slope of the linear equation.
For ABA-treated flacca plants, before FTSW declining to the threshold, there was a significant increasing trend of gs during progressive soil drying. Therefore, when FTSW > C, the linear-plateau model was modified as follows:
where gs max denotes maximum gs when FTSW declined to the threshold (C), and D was the slope of the linear equation before the threshold. gs max of non-ABA-treated flacca was obtained from the average of the individual replicated values when FTSW reached C. In addition, for all flacca plants, gs end indicated the gs at the end of drought treatment.
The parameters y, C, A, and D were estimated by PROC NLIN of PC SAS 9.4 (SAS Institute Inc., Cary, NC, United States, 2002–2012) and the coefficient of determination (r2) was calculated. Statistical comparison of each parameter obtained from the linear-plateau regression between treatments was performed by t-test using MedCalc statistical software 19.0.7.
Data were statistically analyzed using Microsoft Excel, SAS 9.4 (SAS Institute Inc., Cary, NC, United States, 2002–2012), SPSS 22.0 software (IBM SPSS Software, Armonk, NY, United States), and GraphPad Prism 9 software. Two-way analyses of variance (ANOVA) were performed to analyze the effects of [CO2] and exogenous ABA ([ABA]) on plant dry weight, SA, water relation variables, and ABA concentrations in AC and flacca. To compare the decreasing trends of FTSW during soil drying between control treatment and exogenous ABA application, the statistical differences were analyzed by analysis of covariance (ANCOVA, days after withholding water as a covariate). One-way ANOVA (Tukey’s test) and Student’s t-test were conducted to determine the significant differences between treatments. Principle component analysis (PCA) of gs, water relations, and leaf and xylem ABA concentrations in AC and flacca were performed in R version 4.0.0 (R Core Team, 2020). The relationship between gs and [ABA]leaf, gs, and Ψp were evaluated by linear regression. r2 of the regression lines were calculated and statistical differences on the slopes and intercepts of regression lines between treatments were performed by ANCOVA.
Results
Plant Growth and Soil Water Depletion
In AC, exogenous ABA application did not have a significant influence on LDW and SDW, while e[CO2] increased both LDW and SDW. In flacca, the ABA-treated plants had higher LDW and SDW when FTSW = 0.50 and 0.05 and did not respond to e[CO2] except for an increased SDW at the last harvest (Table 1).
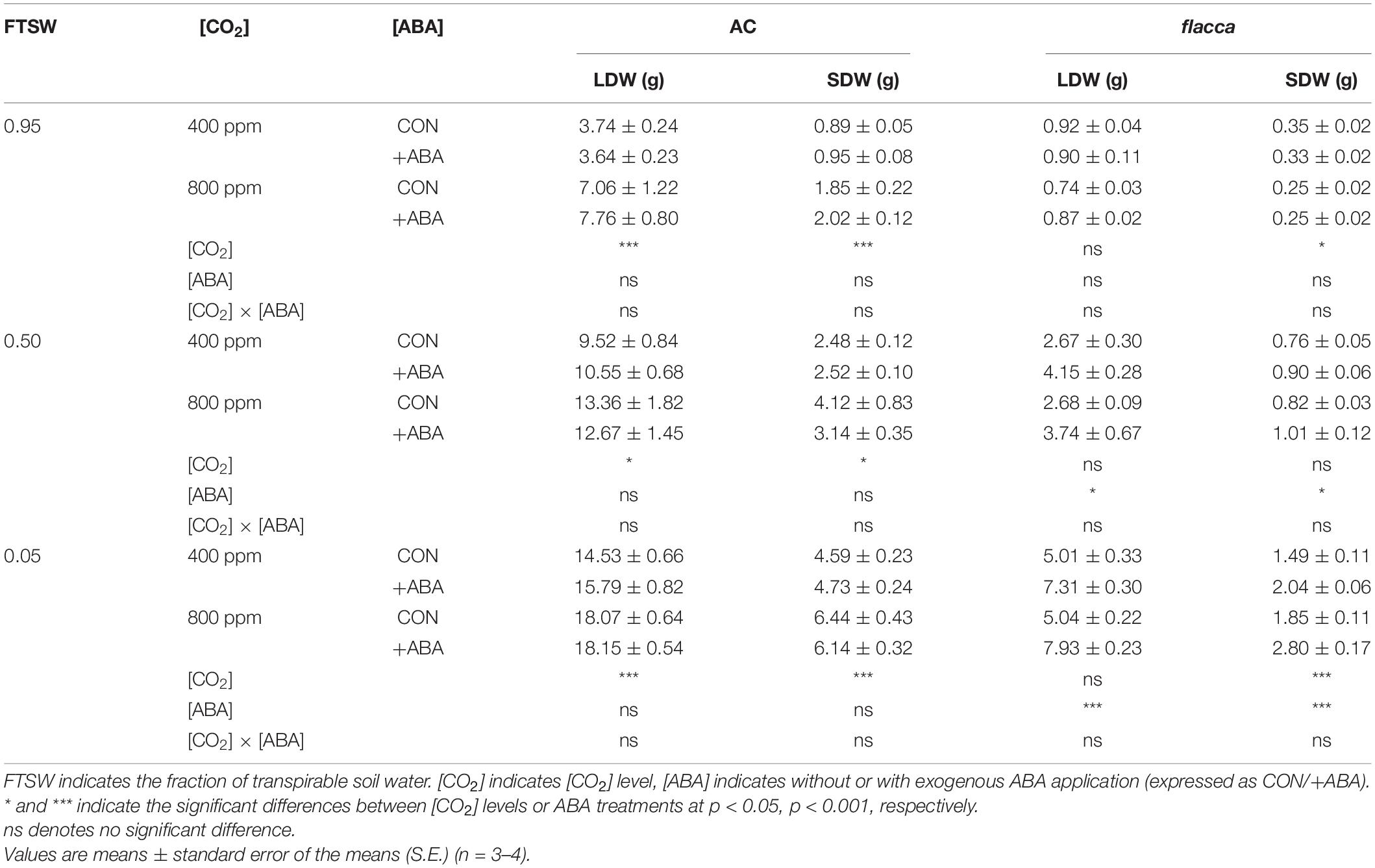
Table 1. Output of two-way analysis of variance (ANOVA) and means ± standard error (S.E.) (N = 3–4) of leaf dry weight (LDW) and stem dry weight (SDW) of AC and flacca grown under ambient (400 ppm) and elevated (800 ppm) CO2 concentrations during progressive soil drying.
After withholding irrigation, the FTSW in the pots of drought-stressed plants decreased steadily (Figure 1). In AC, only for plants grown at e[CO2], exogenous ABA slowed the soil water depletion rate during progressive soil drying. In flacca, at both [CO2] levels, exogenous ABA had a significant influence on the rates of soil water depletion. However, from 0 to 10 days, FTSW declined slower in the ABA-treated plants compared with the non-ABA-treated plants, then it declined faster on the last 3 days.
Stomatal Pore Aperture and Stomatal Conductance
In both AC and flacca, e[CO2] decreased stomatal pore aperture (SA), though it was more pronounced in AC (Figure 2). One day after ABA application (before imposing soil drying), exogenous ABA decreased SA in both genotypes, and interactions of [CO2] and [ABA] were observed, indicating that the effect of e[CO2] on SA was eliminated by exogenous ABA application, and the depressive effect of exogenous ABA was more pronounced in flacca than in AC.
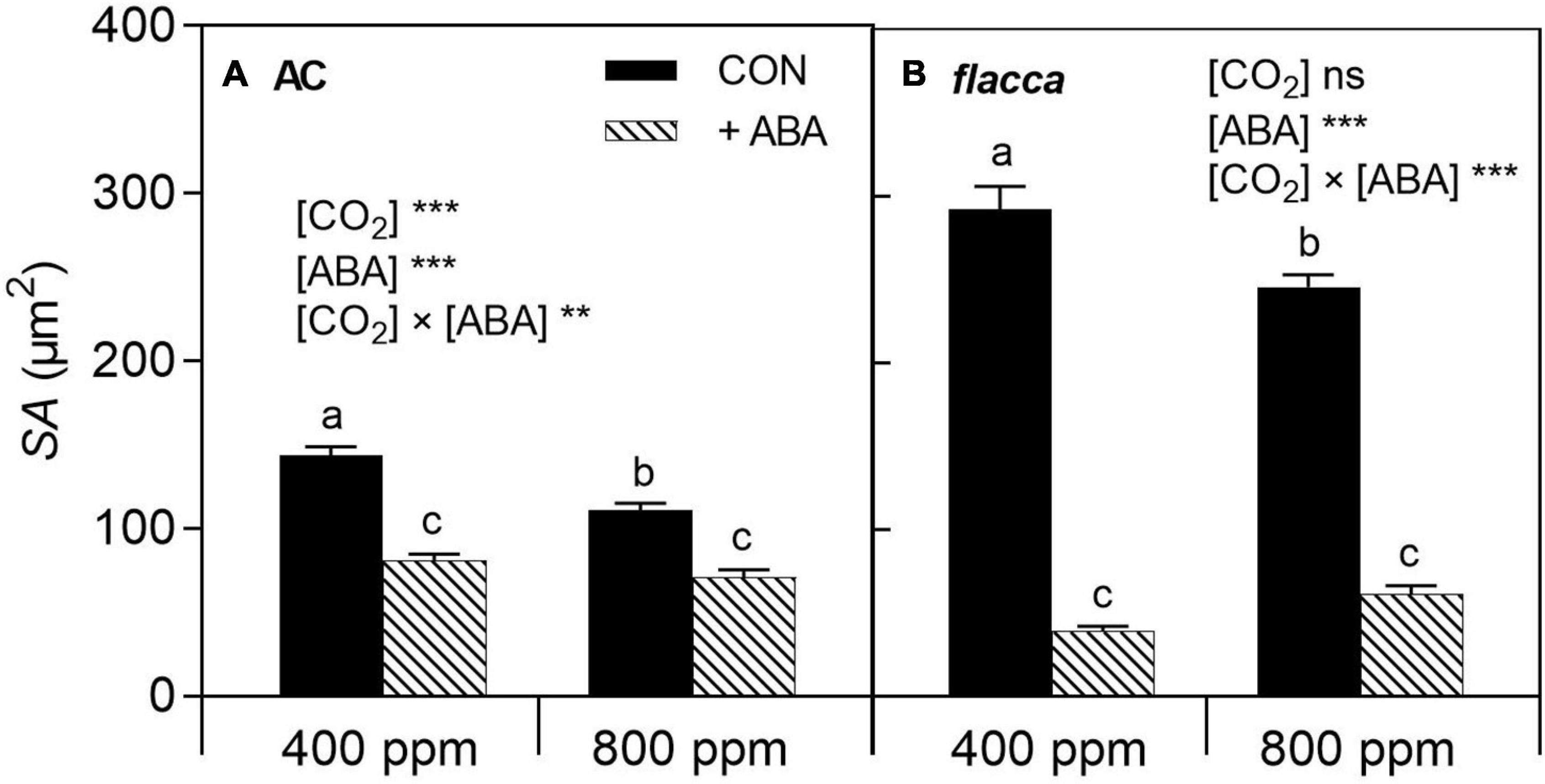
Figure 2. Stomatal pore aperture (SA) of AC (A) and flacca (B) grown under ambient (400 ppm) and elevated (800 ppm) CO2 concentrations 1 day after exogenous ABA application. CON indicates the control treatment sprayed with water, +ABA indicates exogenous ABA application. Different letters on the top of the columns indicate a significant difference between the treatments by Tukey’s test at p < 0.05. ** and *** indicate the significant differences between [CO2] and without or with exogenous ABA application ([ABA]) in two-way ANOVA at p < 0.01, p < 0.001, respectively; ns denotes no significant difference. Error bars indicate the standard error of the means (S.E.) (N = 40).
In AC, during progressive soil drying, plants grown at e[CO2] had significantly lower initial stomatal conductance (gs ini) compared with their a[CO2]-grown counterparts, and gs started to decline when FTSW dropped to an FTSW threshold (C). The ABA-treated plants had constantly lower gs ini than the non-ABA-treated pants, and C was also slightly lowered by exogenous ABA application (Figures 3A,B and Table 2).
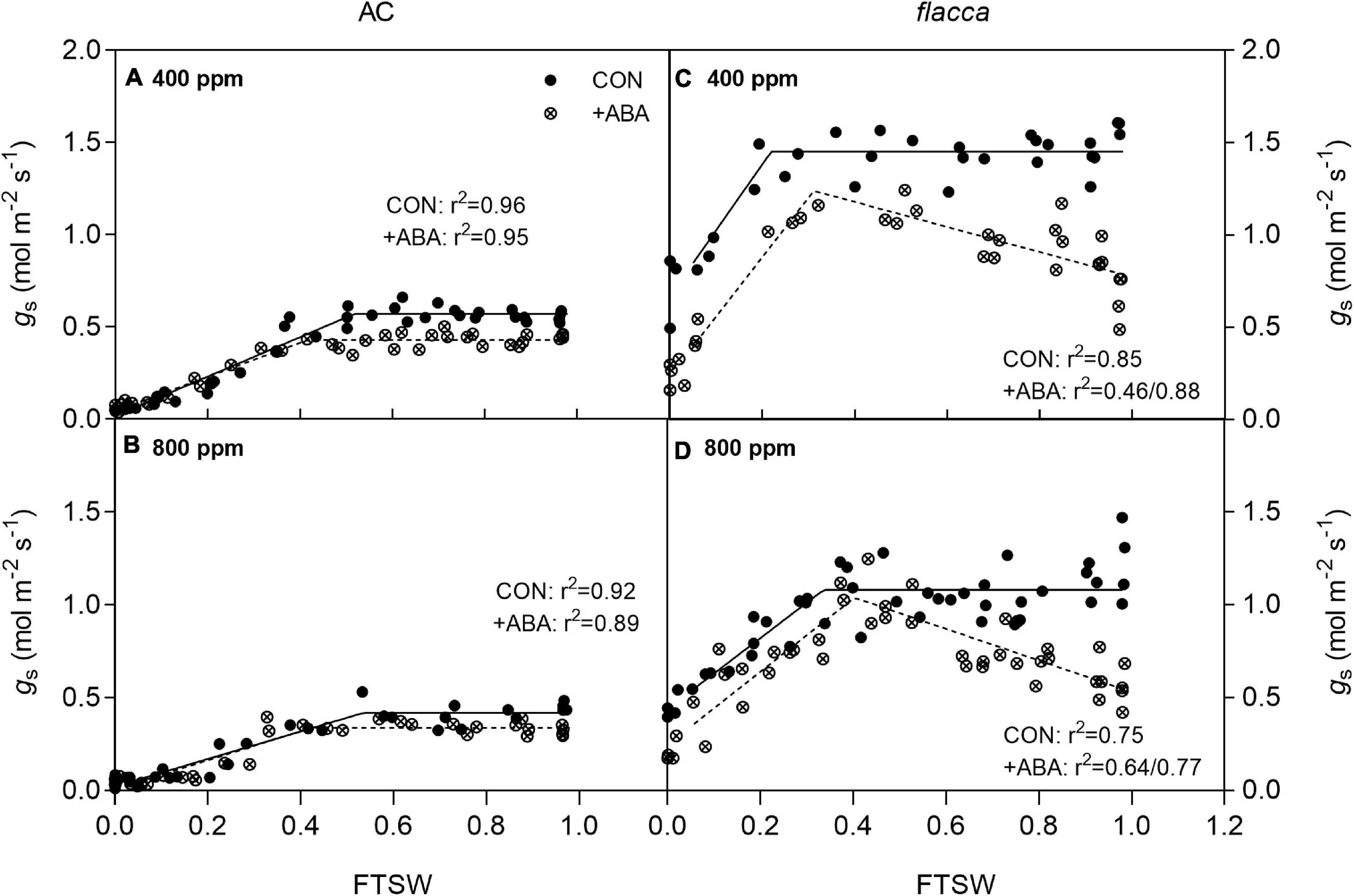
Figure 3. Changes of stomatal conductance (gs) in AC (A,B) and flacca (C,D) (total samples = 32–44, N = 3–4) grown under ambient (400 ppm) and elevated (800 ppm) CO2 concentrations during progressive soil drying. FTSW indicates the fraction of transpirable soil water. CON indicates the control treatment sprayed with water, +ABA indicates exogenous ABA application.
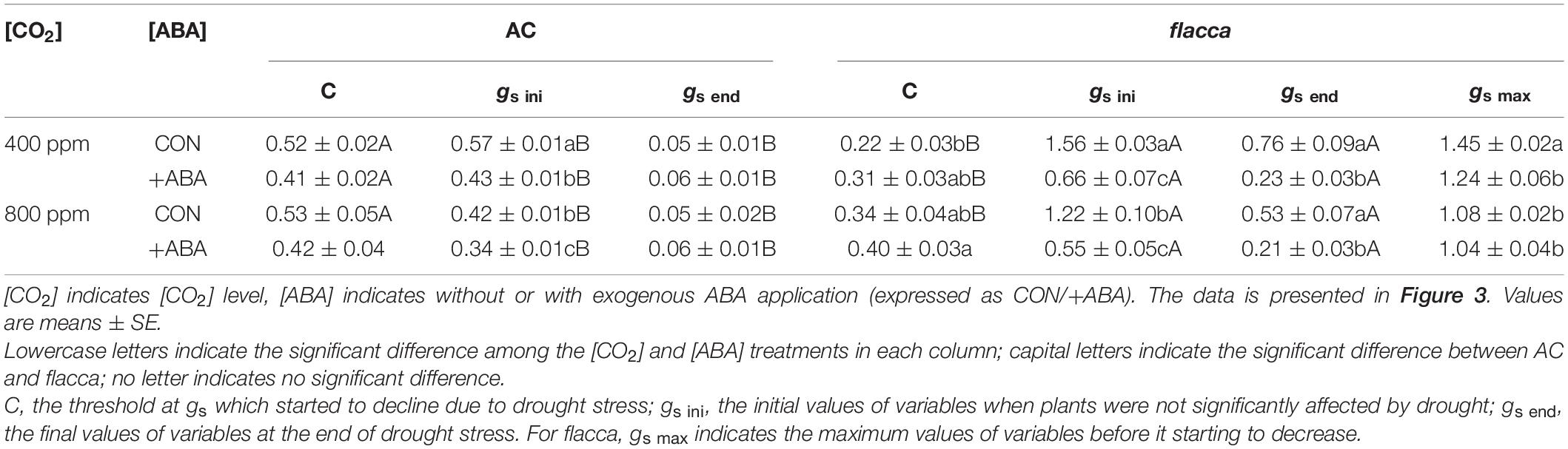
Table 2. Output of statistical analysis of parameters derived from the linear-plateau regression of stomatal conductance (gs) of AC and flacca under ambient (400 ppm) and elevated (800 ppm) CO2 concentrations response to the reduction in the fraction of transpirable soil water (FTSW).
In flacca, 1 day after exogenous ABA application, depression on gs ini ranged from 50 to 58% at a[CO2] and e[CO2] environment, respectively. During soil drying, there were clear increasing trends of gs in the ABA-treated plants before FTSW reaching to the threshold, and when FTSW decreased to C, only the ABA-treated plants grown at a[CO2] still had lower maximum gs (gs max) than the non-ABA-treated plants. Moreover, the FTSW thresholds were slightly advanced by exogenous ABA application. At the end of soil drying, exogenous ABA treatment still lowered gs (gs end) of plants grown at both [CO2] levels, but all flacca plants possessed significantly higher gs end than AC. In addition, e[CO2] decreased gs in non-ABA-treated flacca and slightly advanced C (Figures 3C,D and Table 2).
Plant Water Relation Characteristics
A total of 1 day after exogenous ABA application, before imposing soil drying (i.e., FTSW = 0.95), the ABA-treated AC plants had higher leaf water potential (Ψleaf) and osmotic potential (Ψπ), whereas the ABA-treated flacca plants possessed higher Ψleaf and turgor pressure (Ψp). In addition, slight interactions of [CO2] and [ABA] were observed in Ψleaf and Ψp of AC when FTSW = 0.95, showing that the effects of exogenous ABA became less significant when plants were grown at e[CO2]. At the end of soil drying (i.e., FTSW = 0.05), all these plant water relation variables significantly decreased by drought stress, and exogenous ABA had no influence on water relations of flacca, whereas it still slightly increased Ψleaf in AC. Moreover, e[CO2] improved Ψleaf, Ψπ, and Ψp of AC plants compared to a[CO2], though these effects being diminished along with soil drying (Figure 4 and Table 3).
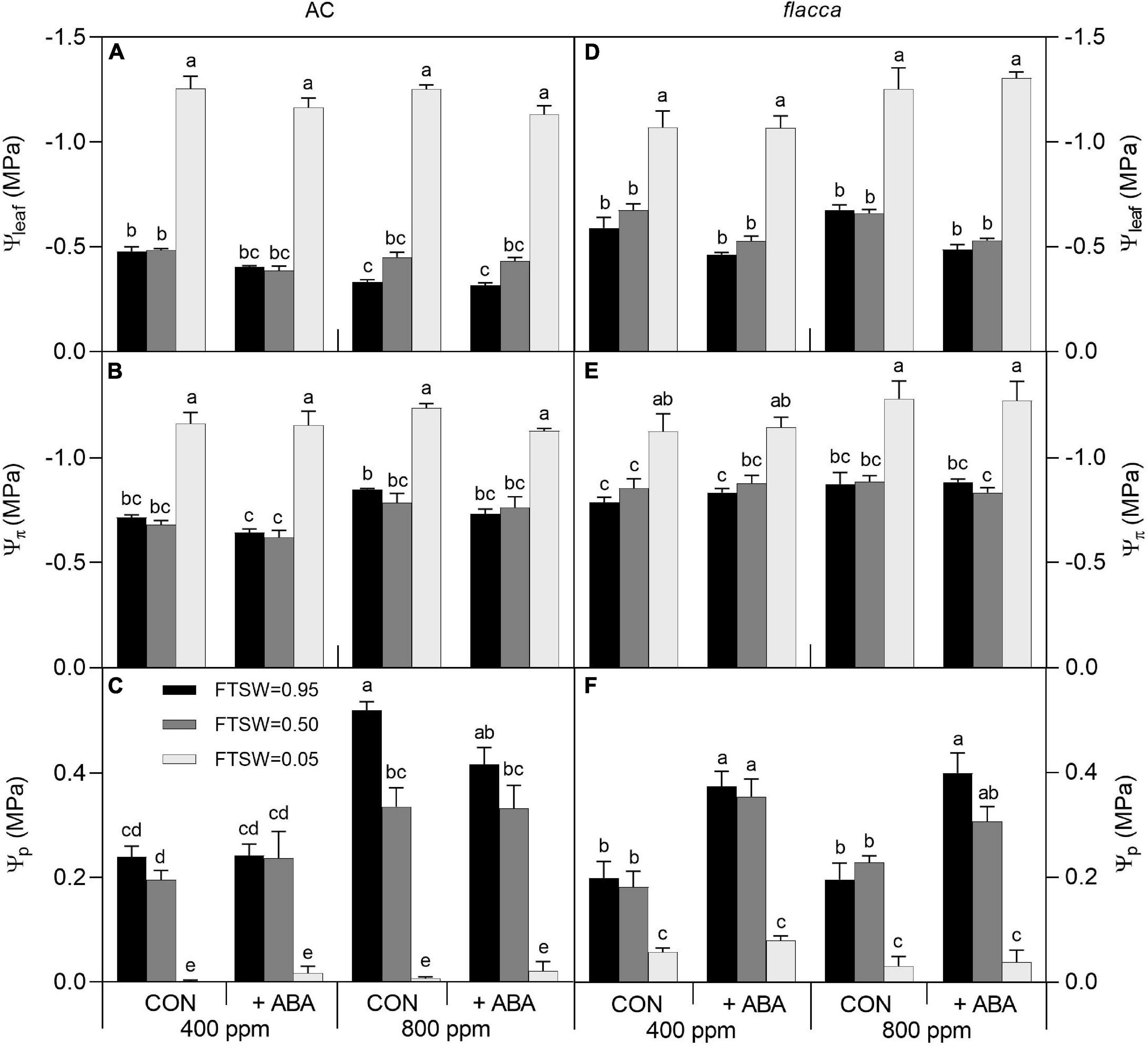
Figure 4. Leaf water potential (Ψleaf), osmotic potential (Ψπ), and turgor pressure (Ψp) in AC (A–C) and flacca (D–F) grown under ambient (400 ppm) and elevated (800 ppm) CO2 concentrations during progressive soil drying. CON indicates the control treatment sprayed with water, +ABA indicates exogenous ABA application. FTSW indicates the fraction of transpirable soil water. Different letters on the top of the columns indicate a significant difference between the treatments by Tukey’s test at p < 0.05. Error bars indicate the standard error of the means (S.E.) (N = 3–4).
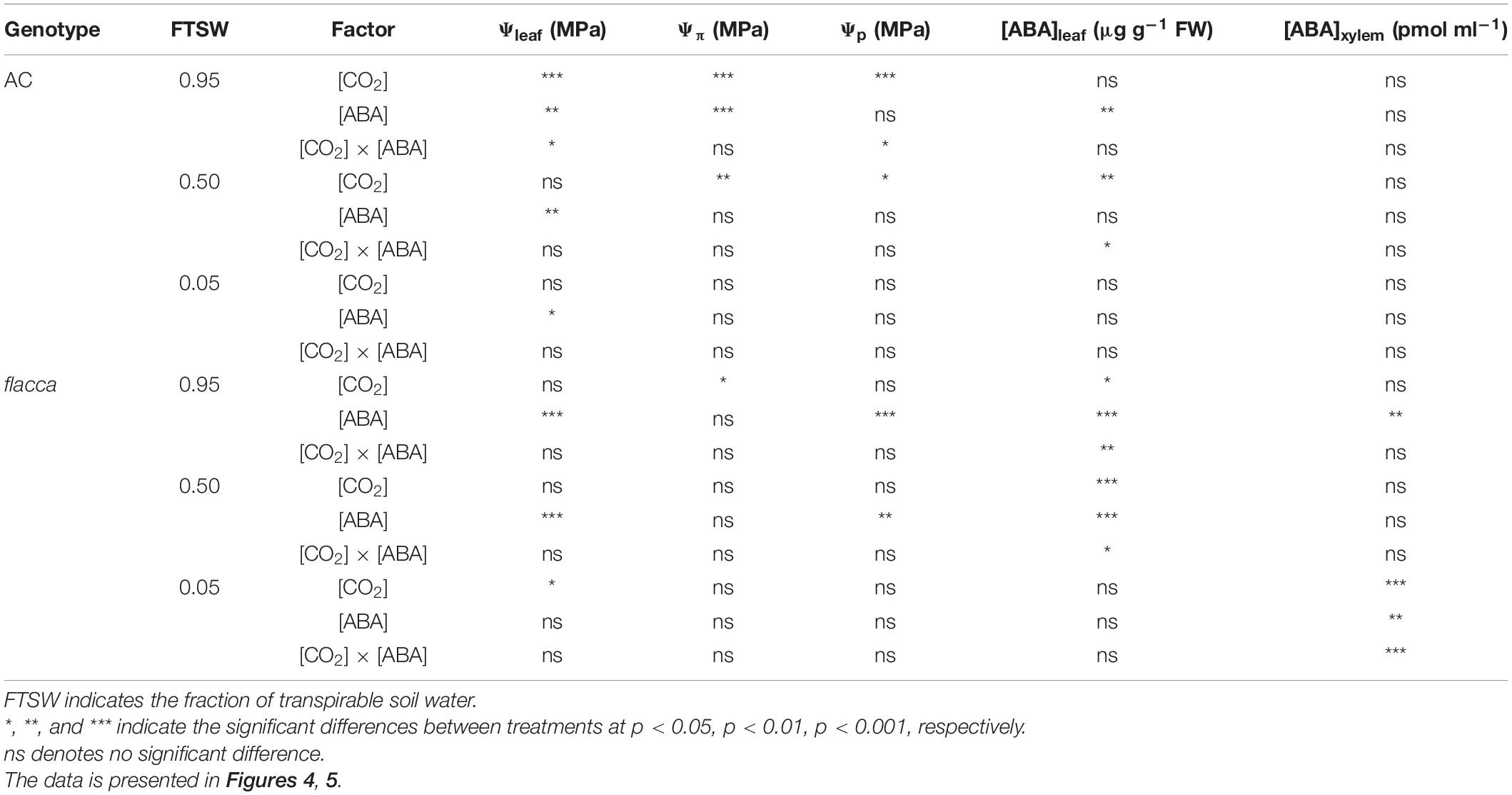
Table 3. Results of two-way ANOVA test showing the statistical significance of the effects of [CO2] and without or with exogenous ABA application ([ABA]) on leaf water potential (Ψleaf), osmotic potential (Ψπ), turgor pressure (Ψp), leaf and xylem sap ABA concentrations ([ABA]leaf, [ABA]xylem) in AC and flacca during progressive soil drying.
Leaf, Xylem Sap Abscisic Acid Concentrations and Their Relationships With the Fraction of Transpirable Soil Water Threshold
In total, 1 day after exogenous ABA application, the ABA-treated AC plants possessed higher leaf ABA concentration [ABA]leaf than the non-ABA-treated plants, while the ABA-treated flacca had both higher [ABA]leaf and xylem sap ABA concentration ([ABA]xylem) compared with the non-ABA-treated controls. During soil drying, when FTSW = 0.50, there were interactions of [ABA] and [CO2] on [ABA]leaf of both genotypes, which was relatively higher in the ABA-treated plans grown under e[CO2] than under a[CO2]. Moreover, at the end of soil drying (i.e., FTSW = 0.05), significantly higher [ABA]xylem was observed in the ABA-treated flacca than the non-ABA-treated flacca plants, accompanied by an interaction of [CO2] and [ABA]. Drought stress increased both [ABA]leaf and [ABA]xylem at the end of soil drying though being less significant in flacca (Figure 5 and Table 3).
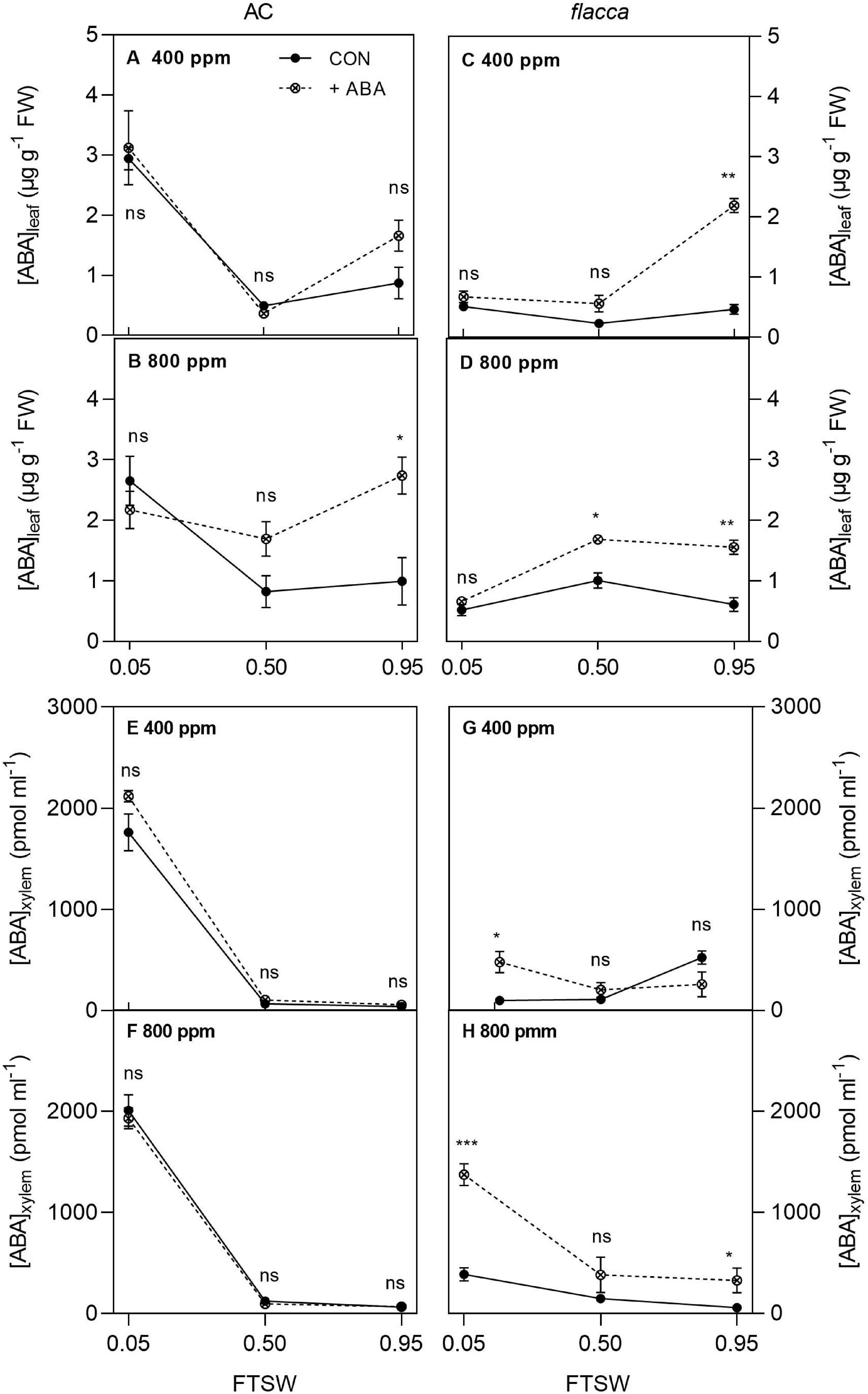
Figure 5. Leaf and xylem sap ABA concentrations ([ABA]leaf, A–D; [ABA]xylem, E–H) in AC and flacca grown under ambient (400 ppm) and elevated (800 ppm) CO2 concentrations during progressive soil drying. CON indicates the control treatment sprayed with water, +ABA indicates exogenous ABA application. FTSW indicates the fraction of transpiration soil water. *, **, and *** indicate the significant differences between ABA treatments by Student’s t-test at p < 0.05, p < 0.01, p < 0.001, respectively; ns denotes no significant difference. Error bars indicate the standard error of the means (S.E.) (N = 3–4).
The relationships of the FTSW threshold at which gs started to decline (C) and [ABA]leaf at the second harvest (FTSW value was close to C) in AC and flacca are shown in Figure 6. The results showed that only in flacca, C was linearly correlated with [ABA]leaf, whereas C of AC did not respond to increasing [ABA]leaf. Moreover, no obvious relationship between C and [ABA]xylem was observed in either genotype.
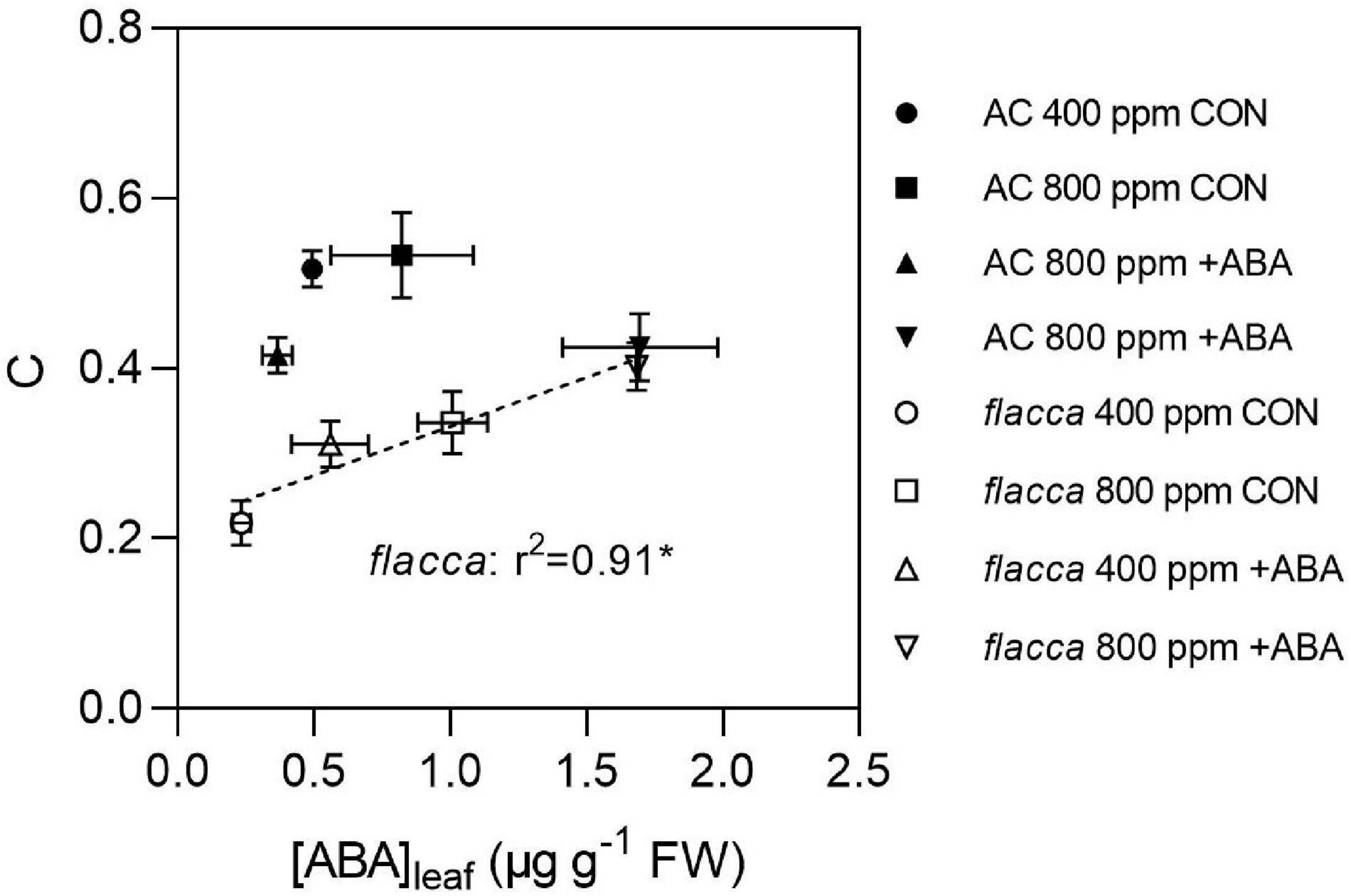
Figure 6. Relationship between the FTSW threshold at which gs started to decline (C) and the leaf ABA concentrations ([ABA]leaf) at second harvest before FTSW reaching C in AC and flacca grown under ambient (400 ppm) and elevated (800 ppm) CO2 concentrations. CON indicates the control treatment sprayed with water, +ABA indicates exogenous ABA application. * Indicate significant difference of the regression line of flacca at p < 0.05. Error bars indicate the standard error of the means (S.E.) (N = 3–4).
Principle Component Analysis Plot of gs, Water Relations, Leaf, and Xylem Abscisic Acid Concentrations of the Well-Watered Plants
Principle component analysis plot of gs, water relations, leaf, and xylem ABA concentrations of well-watered AC and flacca is depicted in Figure 7. PC1 and PC2 axes explained 70.3% of cluster formation, with 49.1% attributed to PC1 and 21.2% to PC2. Overall, AC under all treatments and ABA-treated flacca were clustered toward the right side of the PCA plot, whereas all non-ABA-treated flacca were clustered to the left in the same direction as the gs vector, indicating non-ABA-treated flacca had higher gs than other plants. The clustering of the [AC, 800 ppm, +ABA] indicated a covariation between Ψleaf and [ABA]leaf under this treatment, which was negatively associated with gs. [ABA, flacca] and [AC, 800 ppm, CON] treatments were grouped along with PC2, showing a close correlation with [ABA]xylem and Ψp, and no correlation with gs. Furthermore, [AC, 400 ppm] was opposed by Ψp and [ABA]xylem, being clustered to the bottom of the plot in the same direction as Ψπ vector.
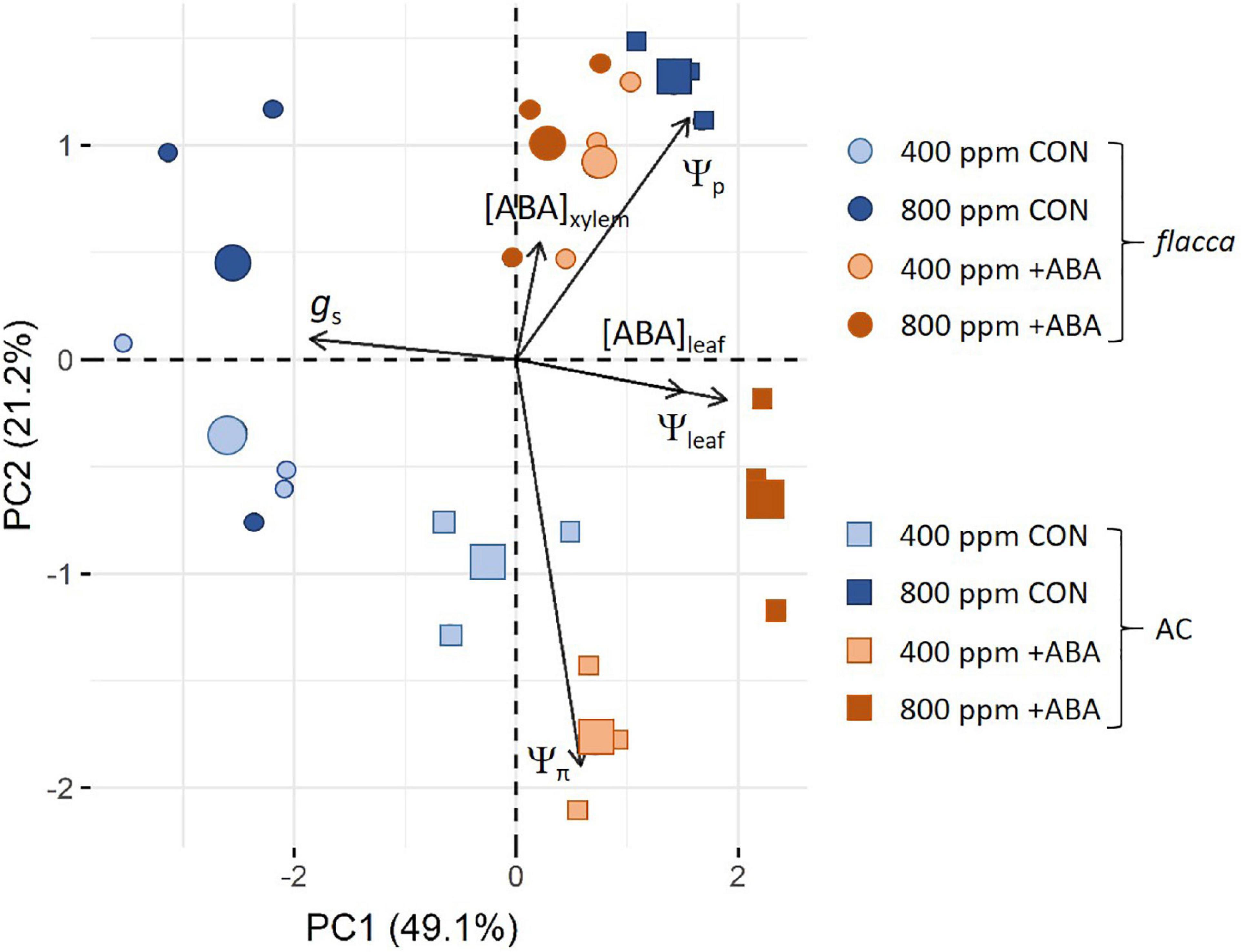
Figure 7. Principal component analysis of stomatal conductance (gs), plant water relations, including leaf water potential (Ψleaf), osmotic potential (Ψπ), and turgor pressure (Ψp), and leaf and xylem sap ABA concentrations ([ABA]leaf, [ABA]xylem) in well-water AC and flacca grown under ambient (400 ppm) and elevated (800 ppm) CO2 at the time of the first harvest. CON indicates the control treatment sprayed with water, +ABA indicates exogenous ABA application. The contributions of each PCA axis (PC1 and PC2) are indicated on the graph.
Relationships Between Stomatal Conductance, Leaf Abscisic Acid Concentration, and Leaf Turgor
In AC, there were linear relationships between gs and [ABA]leaf, gs and Ψπ, where gs declined linearly with increasing [ABA]leaf and decreasing Ψπ (Figure 8). Exogenous ABA application decreased the slope of the regression line of gs and [ABA]leaf, while e[CO2] had no influence on it. Regarding the regression line of gs and Ψπ, the slopes differed between ABA-treated and non-ABA-treated plants, whereas e[CO2] only decreased the slope in non-ABA-treated plants. In flacca, there was no significant relationship between gs and [ABA]leaf under either treatment, while gs decreased linearly with declining Ψπ. In addition, the intercepts of these regression lines differed between [ABA] treatments due to the initial low levels of gs.
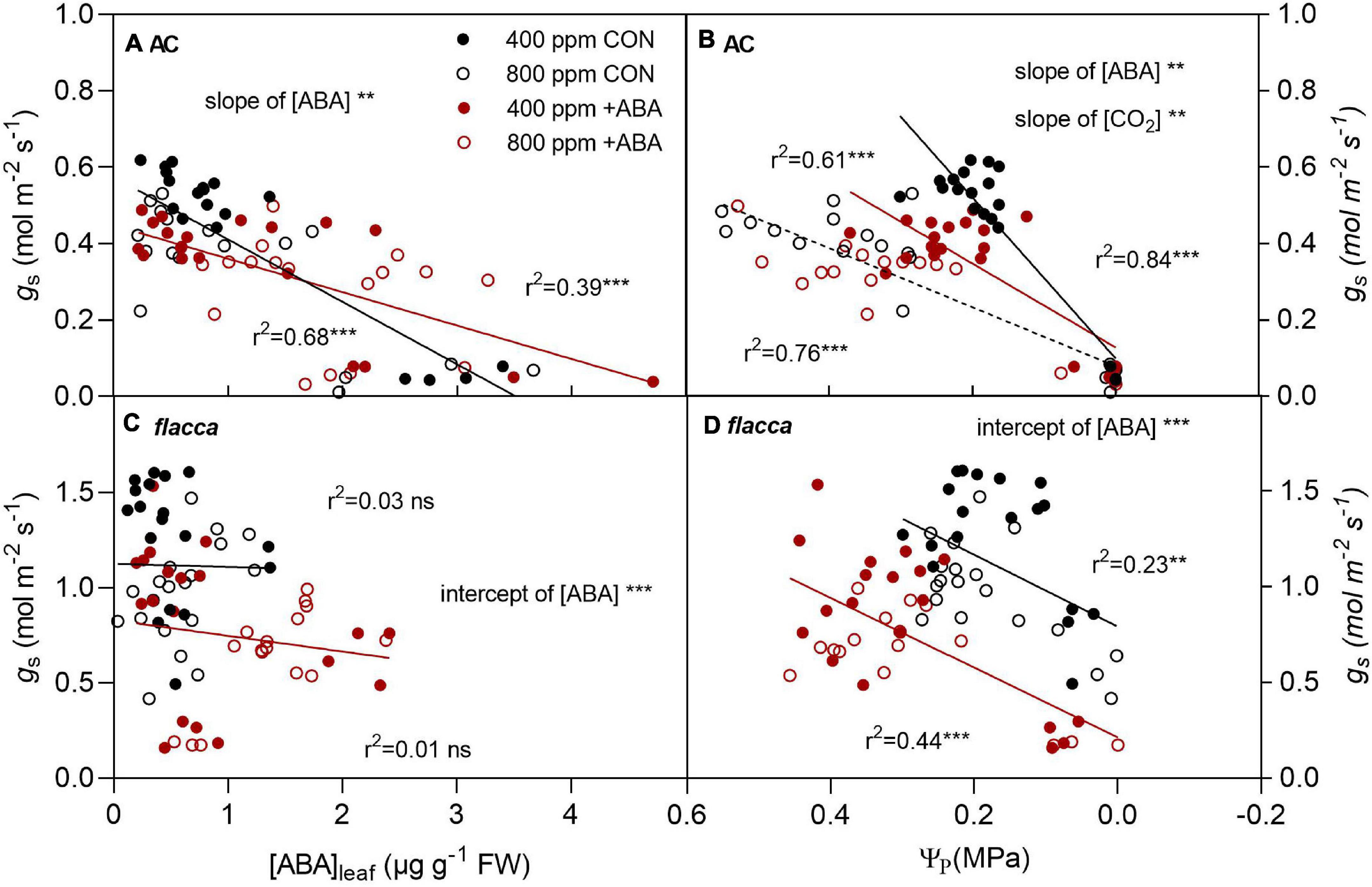
Figure 8. Relationships between stomatal conductance (gs) and leaf ABA concentration ([ABA]leaf), gs and turgor pressure (Ψp) in AC (A,B) and flacca (C,D) grown under ambient (400 ppm) and elevated (800 ppm) CO2 during soil drying. CON indicates the control treatment sprayed with water, +ABA indicates exogenous ABA application. ** and *** indicate significant differences in the regression lines and significant differences of the slopes and intercepts between treatments at p < 0.01 and p < 0.001, respectively; ns denotes no significant difference. Black and red lines (denote CON and +ABA, respectively) indicated that there were significant differences between [ABA] treatments. Solid and dotted black lines in panel (B) indicated that only under CON but not +ABA there was a significant difference between [CO2] treatments.
Discussion
Plant hormone ABA is a vital factor in both e[CO2]- and the drought stress-mediated plant water relation regulatory network, and ABA homeostasis is essential for plants to cope with drought stress. In the present study, we investigated different effects of exogenous ABA application and e[CO2] as well as their interactions on tomato leaf gas exchange and water relation characteristics during progressive soil drying.
Plant Growth and Stomatal Conductance as Influenced by Elevated CO2 and Exogenous Abscisic Acid
It is well known that e[CO2] decreases gs while stimulating carbon assimilation, thus improving plant drought tolerance (Li S. et al., 2020). In tomato plants, previous studies have shown that the plant response to e[CO2] was attenuated or absent in ABA-deficient mutant flacca, affirming the obligatory role of ABA in CO2 signaling pathway (Fang et al., 2019; Wei et al., 2020). Consistent with this, here LDW and SDW of AC plants were improved by e[CO2] during progressive soil drying, but these stimulations were less significant in flacca. Upon exogenous ABA application, retarded plant growth of flacca was rescued under both watering conditions, while growth of AC plants did not benefit from exogenous ABA treatment (Table 1), which was consistent with previous study on tomato and confirmed the important role of ABA in maintaining the shoot development (Sharp et al., 2000; Aroca et al., 2008).
It has been reported that application of exogenous ABA inhibited water loss and reduced the rate of soil drying due to stomatal closure (Hossain et al., 2015; He et al., 2019). In the present study, in both ABA-treated AC and flacca, slower soil water depletion during progressive soil drying was recorded, which coincided with the decreases of SA and gs in relation to the non-ABA-treated controls (Figures 1–3). However, in the drought-stressed AC plants, only those grown under e[CO2] possessed slower rate of soil water depletion upon exogenous ABA application compared with the non ABA-treated plants, indicating a significant interaction of e[CO2] and exogenous ABA on plant water consumption when soil water was limited. Although in AC e[CO2] accelerated water consumption due to stimulated plant growth, exogenous ABA application had no influence on plant growth (Figure 1 and Table 1). Therefore, the interactive effect of e[CO2] and exogenous ABA on water consumption rate might be ascribed to the altered stomatal behavior, as both factors could induce stomatal closure.
It is worth noting that upon exogenous ABA treatment the depressive effect of e[CO2] on SA was eliminated in both genotypes (Figure 2). Considering that e[CO2] had exerted inhibiting effects on stomatal aperture, it could be assumed that exogenous ABA application overrode the effect of e[CO2] on stomatal movement. However, the common view is that ABA can amplify the effects of [CO2] on stomatal behavior (Engineer et al., 2016), and e[CO2]-grown soybean showed a stronger response to endogenous ABA (Gray et al., 2016), though ABA and [CO2] signal transductions also could be independent (Hsu et al., 2018). As our previous studies demonstrated that severe drought stress overrode the e[CO2] effects, here exogenous ABA application exhibited the same influences as drought stress. Furthermore, decreases in SA and gs were more pronounced in flacca than AC (Figures 2, 3). Namely, the stomata of flacca exhibited greater sensitivity to exogenous ABA than AC due to ABA deficit. Interestingly, after the onset of soil drying, before the occurrence of stomatal closure, there were significant increasing trends of gs in the ABA-treated flacca while the ABA-treated AC possessed a constantly lower gs before the FTSW thresholds (Figure 3), indicating that the effects of exogenous ABA application lasted for a longer period in AC than in flacca. Early studies have found that the abnormal stomatal behavior in flacca was associated with the high level of ethylene resulting from an ABA deficit (Tal et al., 1979), and other chemical signals could also counteract the ABA signaling, as is the case with cytokinin (Prerostova et al., 2018), which might accelerate the degradation process of exogenous ABA in flacca plants. In addition, flacca is known to be impaired in the oxidation of ABA aldehyde to ABA (Sagi et al., 2002), vanishing effects of exogenous ABA might be ascribed to the redistribution of ABA to other plant tissues. These results suggest the necessity to explore the regulation of ABA homeostasis in the two genotypes in future studies. Despite the significantly lower gs ini in ABA-treated flacca, the gs end of flacca was still higher than AC under all treatments (Figure 3 and Table 2), which indicated that flacca could not fully close stomata under severe drought stress after exogenous ABA application. In another ABA-deficient mutant sitiens, a decrease in the leaf gas exchange rate by exogenous ABA (100 μM) was significant after 52 days of mild drought stress, but it still possessed a higher level of transpiration rate than wild type (Aroca et al., 2008). Therefore, exogenous ABA priming before soil drying could not sufficiently induce stomatal closure under severe drought stress.
Previous studies on tomato revealed that e[CO2] retarded stomatal closure during soil drying due to reduced stomatal sensitivity to ABA (Liu et al., 2019; Wei et al., 2020). However, here we did not find e[CO2] delayed stomatal closure in AC, the reason behind this discrepancy is unknown, which might be ascribed to the varied experimental conditions between the different studies. By contrast, in flacca plants, exogenous ABA application sensitized the stomatal response to drought though only significant under a[CO2] (Figures 3C,D and Table 2), suggesting that the stomatal closure in ABA-deficient mutant could be advanced by exogenous ABA treatment, which might contribute to water-saving under drought stress. In addition, e[CO2] decreased gs in flacca despite the slight influence on SA, and these effects were absent in ABA-treated flacca. Although the retarded stomatal response to e[CO2] in flacca was attributed to ABA deficit (Wei et al., 2020; Li et al., 2021), exogenous ABA application could not recover the response due to its strongly induced stomatal closure.
Plant Water Relations as Influenced by Elevated CO2 and Exogenous Abscisic Acid
Previous studies have suggested that exogenous ABA application is beneficial for plants to improve drought tolerance as a result of induced stomatal closure, enhanced water relations, and a higher OA (Du et al., 2013; He et al., 2019). In the present study, 1 day after exogenous ABA application, higher Ψleaf, Ψπ, and Ψp were observed compared to the non-ABA-treated plants (Figure 4), confirming the positive effects of exogenous ABA on plant water relations. In an early study with exogenous ABA application (10 μM) on flacca, the authors found that the impaired shoot growth could be restored but no improved Ψleaf was observed (Sharp et al., 2000). As stimulation on expansive growth by ABA is primarily related to hydraulic control (Tardieu et al., 2015), here the improved water status in ABA-treated flacca might be responsible for the restored growth (Table 1). Moreover, the positive effects of exogenous ABA on plant water relations were more pronounced in flacca than in AC (Figure 4), coinciding with the stomatal responses in the two genotypes. In wild-type tomato and ABA-deficient mutant sitiens, Aroca et al. (2008) found that the genotypes showed different gene regulatory patterns in response to exogenous ABA, including ABA biosynthesis-related and aquaporin-encoding genes, which might be associated with the high sensitivity to ABA in flacca plants. Surprisingly, at the end of soil drying, the improved water relations, by application of exogenous ABA, vanished in both genotypes, including Ψp (Figure 4 and Table 3). Thereby, the decreased gs in ABA-treated flacca at the end of soil drying could not be explained by modulated water relations. In addition, OA is also considered as a vital trait for maintaining leaf turgor, and increased OA accompanied by improved leaf water relations have also been reported in exogenous ABA-treated soybean (He et al., 2019). However, here we did not find the influence of exogenous ABA on OA in both genotypes during the whole period of soil drying. Therefore, more detailed mechanisms related to the ABA-modulated plant water relations merit future studies.
The positive effects of e[CO2] on plant growth and physiology are reported to be more pronounced under drought stress which, however, depends on the severity of drought stress (Leakey et al., 2006; Gray et al., 2016). In the present study, the well-watered AC plants grown at e[CO2] had an improved Ψleaf, Ψπ, and Ψp in relation to those grown under a[CO2], but these effects were eliminated during soil drying (Figure 4 and Table 3), in line with previous findings (Wei et al., 2020). As e[CO2]-grown plant already possessed improved water relations, effects of exogenous ABA on AC became less significant at e[CO2]. In Figure 8, e[CO2] rendered a less sensitivity of stomata to Ψπ, but this effect was absent in ABA-treated AC. Collectively, these results implied an offsetting effect of [CO2] and exogenous ABA on plant water relations as well as stomatal behavior.
Abscisic Acid Signal Mediates Stomatal Drought Response
In the present study, 1 day after exogenous ABA application, significant increases of [ABA]leaf in AC and flacca were observed, and the increase was more pronounced in flacca than in AC, though it was gradually diminished during soil drying (Figure 5 and Table 3). As oxidative degradation of ABA could occur rapidly (Zhang et al., 1995), in tomato plants, when ABA-treated plants were exposed to long-term drought (52 days), ABA contents in plants did not differ between the ABA-treated and non-ABA-treated plants despite the accumulated exogenous ABA effects on water relations still existing (Aroca et al., 2008). By contrast, here, we found that the vanishing effect on water relations was accompanied by decreased accumulation of [ABA]leaf in ABA-treated flacca. More interestingly, the interactions of [CO2] and [ABA] on [ABA]leaf of AC and flacca indicated that the ABA degradation process might be disturbed under e[CO2], which could explain the reduced ABA sensitivity in the drought-stressed tomato plants gown under e[CO2] (Yan et al., 2017; Wei et al., 2020). In ABA-treated flacca, an increase in [ABA]xylem was observed especially at the end of soil drying, which was accompanied by an interaction of [CO2] and exogenous ABA (Figure 5 and Table 3), implying that e[CO2] exerted an effect on ABA homeostasis. It is well documented that ABA metabolism, including biosynthesis and degradation, ABA recirculation, and exudation processes are all involved in the regulation of ABA homeostasis (Hartung et al., 2005). Moreover, redistribution of ABA within plant organs has often been reported, and translocation of ABA from leaves to roots can be intensified when roots are experiencing drought (Hartung et al., 2005; Ikegami et al., 2009; Ernst et al., 2010). Taking together, except for the ABA degradation pathway, decrease of [ABA]leaf in the ABA-treated flacca could also be ascribed to the redistribution of ABA between shoot and root considering its inability of ABA synthesis in roots.
It is well known that tomato plants are likely to exhibit isohydric behaviors (Moshelion et al., 2015), while ABA-deficient mutant flacca and sitiens could be transpired more for longer periods during soil drying than AC (Aroca et al., 2008; Wei et al., 2020), representing a near-anisohydric characteristic. Furthermore, rapid ABA biosynthesis might facilitate isohydric behavior (Moshelion et al., 2015). In the present study, before the occurrence of stomatal closure (i.e., when FTSW = 0.50), ABA-treated flacca grown at e[CO2] possessed relatively high [ABA]leaf (Figure 5), which was found to be closely correlated with the FTSW threshold at which gs started to decrease (C) (Figure 6). These results raise the possibility that anisohydric behaviors in the ABA-deficient mutant could be shifted toward near-isohydric behaviors through the combined effects of e[CO2] and exogenous ABA, representing a restored stomatal drought response. The change in the water management strategies has been reported to be activated by different soil water statuses (Zhang et al., 2012), also the overexpression of a key tonoplast aquaporin (Sade et al., 2009), which could be linked to ABA.
Previous studies have shown that shoot-to-root ABA transport played a role in the regulation of water flux and induction of stress-resistant genes in roots (Ikegami et al., 2009). In the near-anisohydric grapevine cultivar Syrah, the authors found that it showed relatively high catabolism of ABA in xylem sap, which might lower its hypersensitivity to water stress (Dayer et al., 2020). Therefore, it could be assumed that reloading of ABA into xylem sap in flacca plants might stimulate some specific metabolic process, causing feedback on stomatal movements, which might explain the low gs in ABA-treated plants at the end of soil drying but without [ABA]leaf accumulation. For example, early studies on flacca have shown that low ABA content stimulated ethylene production, which could be restored to normal levels with exogenous ABA (Tal et al., 1979; Sharp et al., 2000). As ethylene production is often increased by drought stress, it might be associated with the restored drought response in ABA-treated flacca as noticed in the present study. In addition, exogenous ABA-induced other metabolic adaption has been reported widely, including stimulated resistant protein patterns (Zhou et al., 2014), enhanced energy storages, and activities of antioxidant enzymes (Latif, 2014; Gai et al., 2020), and these possibilities merit further studies.
Under well-watered conditions, the ABA-treated flacca plants possessed the same leaf characteristics as AC in the global PCA plots (Figure 7). However, despite the restored leaf gas exchange rates, water relation characteristics, and ABA concentrations in flacca (Figures 3–5), the PCA plot indicated that, under well-watered conditions, exogenous ABA could still not induce a correlation between gs and [ABA]leaf in flacca, but showed an interaction with Ψp and [ABA]xylem (Figure 7). Furthermore, during the whole period of soil drying, gs was still not correlated with [ABA]leaf but only responded to Ψp (Figure 8). Therefore, a question arises as to how exogenous ABA application affected flacca’s stomatal movements considering the no involvement of [ABA]leaf. Pantin et al. (2013) demonstrated that ABA can induce stomatal closure through an indirect hydraulic effect on water permeability within leaf vascular tissues. In the exogenous ABA-fed near-anisohydric grapevine cultivar, the existence of indirect ABA effects on gs has also been reported, which was associated with the ABA catabolism (Dayer et al., 2020). Therefore, it could be assumed that the exogenous ABA decreased gs in flacca plants through an indirect hydraulic effect. Taking together, future research should focus on exploring the correlation of ABA metabolic process and ABA indirect hydraulic effects on stomatal behavior.
Conclusion
Exogenous ABA application decreased SA and gs, improved plant water relations, including Ψleaf, Ψπ, and Ψp, and increased [ABA]leaf in both AC and flacca, though these two genotypes showed differential responses during soil drying, where exogenous ABA priming sensitized the gs response to soil drying in flacca. In both ABA-treated genotypes, high [ABA]leaf lasted for a longer period under e[CO2] than a[CO2] conditions, which might be associated with ABA degradation or redistribution and responsible for the e[CO2]-induced ABA insensitivity. In AC, depression on SA and improvement in water relations by exogenous ABA was more pronounced in a[CO2]-grown plants compared to their e[CO2]-grown counterparts, and e[CO2] only lowers gs sensitivity to Ψp in non-ABA-treated AC, indicating that e[CO2] could counteract the effects of exogenous ABA. In flacca, the effects of exogenous ABA on gs were gradually diminished during soil drying. However, ABA-treated flacca still showed a partly restored stomatal drought response at both [CO2] conditions, and was accompanied by the recovered plant growth and increased [ABA]xylem especially under e[CO2]. Thus, the restored drought response in the absence of accumulation of [ABA]leaf could be associated with ABA-stimulated metabolic adaptions. Although ABA-treated flacca exhibited recovered stomatal behavior, the PCA plot and regression analysis showed that [ABA]leaf was not responsible for the decreasing gs. Therefore, our results raise the possibility that exogenous ABA-induced stomatal closure in flacca could be attributed to an indirect hydraulic effect.
Data Availability Statement
The original contributions presented in the study are included in the article/Supplementary Material, further inquiries can be directed to the corresponding author/s.
Author Contributions
Both authors conceived the concept, carried out the experiment, and have read and agreed to the published version of the manuscript. SL wrote the manuscript with support from FL. FL supervised the project.
Funding
This work was supported by the Chinese Scholarship Council (CSC).
Conflict of Interest
The authors declare that the research was conducted in the absence of any commercial or financial relationships that could be construed as a potential conflict of interest.
Publisher’s Note
All claims expressed in this article are solely those of the authors and do not necessarily represent those of their affiliated organizations, or those of the publisher, the editors and the reviewers. Any product that may be evaluated in this article, or claim that may be made by its manufacturer, is not guaranteed or endorsed by the publisher.
Acknowledgments
We appreciate the Chinese Scholarship Council (CSC) for supporting this study at the Faculty of Science, University of Copenhagen, Denmark. The technical assistance by Rene Hvidberg Petersen, Benitta Rueskov Wöhlk, Lene Korsholm Jørgensen, and Kenneth Kauffmann Slot was gratefully acknowledged.
Supplementary Material
The Supplementary Material for this article can be found online at: https://www.frontiersin.org/articles/10.3389/fpls.2021.733658/full#supplementary-material
Supplementary Figure 1 | The average temperature, relative humidity, vapor pressure deficit (VPD), and CO2 concentration [CO2] in 400 and 800 ppm greenhouse cells of AC and flacca during the whole experimental period.
References
Aroca, R., Del Mar Alguacil, M., Vernieri, P., and Ruiz-Lozano, J. M. (2008). Plant responses to drought stress and exogenous ABA application are modulated differently by mycorrhization in tomato and an ABA-deficient mutant (sitiens). Microb. Ecol. 56, 704–719. doi: 10.1007/s00248-008-9390-y
Asch, F. (2000). Laboratory Manual on Determination of Abscisic Acid by Indirect Enzyme Linked Immuno Sorbent Assay (ELISA). Frederiksberg: Royal Veterinary and Agricultural University.
Avila, R. T., Cardoso, A. A., de Almeida, W. L., Costa, L. C., Machado, K. L. G., Barbosa, M. L., et al. (2020). Coffee plants respond to drought and elevated [CO2] through changes in stomatal function, plant hydraulic conductance, and aquaporin expression. Environ. Exp. Bot. 177:104148. doi: 10.1016/j.envexpbot.2020.104148
Becklin, K. M., Walker, S. M., Way, D. A., and Ward, J. K. (2017). CO2 studies remain key to understanding a future world. New Phytol. 214, 34–40. doi: 10.1111/nph.14336
Berg, A., and Sheffield, J. (2018). Climate change and drought: the soil moisture perspective. Curr. Clim. Chang. Rep. 4, 180–191. doi: 10.1007/s40641-018-0095-0
Buncec, J. A. (1998). Effects of humidity on short-term responses of stomatal conductance to an increase in carbon dioxide concentration. Plant Cell Environ. 21, 115–120. doi: 10.1046/j.1365-3040.1998.00253.x
Chater, C., Peng, K., Movahedi, M., Dunn, J. A., Walker, H. J., Liang, Y. K., et al. (2015). Elevated CO2-induced responses in stomata require ABA and ABA signaling. Curr. Biol. 25, 2709–2716. doi: 10.1016/j.cub.2015.09.013
Chen, G., Fu, X., Herman Lips, S., and Sagi, M. (2003). Control of plant growth resides in the shoot, and not in the root, in reciprocal grafts of flacca and wild-type tomato (Lysopersicon esculentum), in the presence and absence of salinity stress. Plant Soil 256, 205–215. doi: 10.1023/A:1026279719242
Comstock, J. P. (2002). Hydraulic and chemical signalling in the control of stomatal conductance and transpiration. J. Exp. Bot. 53, 195–200. doi: 10.1093/jexbot/53.367.195
da Silva, J. R., Patterson, A. E., Rodrigues, W. P., Campostrini, E., and Griffin, K. L. (2017). Photosynthetic acclimation to elevated CO2 combined with partial rootzone drying results in improved water use efficiency, drought tolerance and leaf carbon balance of grapevines (Vitis labrusca). Environ. Exp. Bot. 134, 82–95. doi: 10.1016/j.envexpbot.2016.11.007
Dayer, S., Scharwies, J. D., Ramesh, S. A., Sullivan, W., Doerflinger, F. C., Pagay, V., et al. (2020). Comparing hydraulics between two grapevine cultivars reveals differences in stomatal regulation under water stress and exogenous ABA applications. Front. Plant Sci. 11:705. doi: 10.3389/fpls.2020.00705
Du, Y. L., Wang, Z. Y., Fan, J. W., Turner, N. C., He, J., Wang, T., et al. (2013). Exogenous abscisic acid reduces water loss and improves antioxidant defence, desiccation tolerance and transpiration efficiency in two spring wheat cultivars subjected to a soil water deficit. Funct. Plant Biol. 40, 494–506. doi: 10.1071/FP12250
Engineer, C. B., Hashimoto-Sugimoto, M., Negi, J., Israelsson-Nordström, M., Azoulay-Shemer, T., Rappel, W. J., et al. (2016). CO2 sensing and CO2 regulation of stomatal conductance: advances and open questions. Trends Plant Sci. 21, 16–30. doi: 10.1016/j.tplants.2015.08.014
Ernst, L., Goodger, J. Q. D., Alvarez, S., Marsh, E. L., Berla, B., Lockhart, E., et al. (2010). Sulphate as a xylem-borne chemical signal precedes the expression of ABA biosynthetic genes in maize roots. J. Exp. Bot. 61, 3395–3405. doi: 10.1093/jxb/erq160
Fang, L., Abdelhakim, L. O. A., Hegelund, J. N., Li, S., Liu, J., Peng, X., et al. (2019). ABA-mediated regulation of leaf and root hydraulic conductance in tomato grown at elevated CO2 is associated with altered gene expression of aquaporins. Hortic. Res. 6, 104. doi: 10.1038/s41438-019-0187-6
Faralli, M., Williams, K. S., Han, J., Corke, F. M. K., Doonan, J. H., and Kettlewell, P. S. (2019). Water-saving traits can protect wheat grain number under progressive soil drying at the meiotic stage: a phenotyping approach. J. Plant Growth Regul. 38, 1562–1573. doi: 10.1007/s00344-019-09956-3
Field, C. B., Jackson, R. B., and Mooney, H. A. (1995). Stomatal responses to increased CO2: implications from the plant to the global scale. Plant Cell Environ. 18, 1214–1225. doi: 10.1111/j.1365-3040.1995.tb00630.x
Gai, Z., Wang, Y., Ding, Y., Qian, W., Qiu, C., Xie, H., et al. (2020). Exogenous abscisic acid induces the lipid and flavonoid metabolism of tea plants under drought stress. Sci. Rep. 10:12275. doi: 10.1038/s41598-020-69080-1
Gray, S. B., Dermody, O., Klein, S. P., Locke, A. M., McGrath, J. M., Paul, R. E., et al. (2016). Intensifying drought eliminates the expected benefits of elevated carbon dioxide for soybean. Nat. Plants 2:16132. doi: 10.1038/nplants.2016.132
Hartung, W., Schraut, D., and Jiang, F. (2005). Physiology of abscisic acid (ABA) in roots under stress - a review of the relationship between root ABA and radial water and ABA flows. Aust. J. Agric. Res. 56, 1253–1259. doi: 10.1071/AR05065
Hatfield, J. L., and Dold, C. (2019). Water-use efficiency: advances and challenges in a changing climate. Front. Plant Sci. 10:103. doi: 10.3389/fpls.2019.00103
Haworth, M., Killi, D., Materassi, A., Raschi, A., and Centritto, M. (2016). Impaired stomatal control is associated with reduced photosynthetic physiology in crop species grown at elevated [CO2]. Front. Plant Sci. 7:1568. doi: 10.3389/fpls.2016.01568
He, J., Jin, Y., Palta, J. A., Liu, H. Y., Chen, Z., and Li, F. M. (2019). Exogenous ABA induces osmotic adjustment, improves leaf water relations and water use efficiency, but not yield in soybean under water stress. Agronomy 9:395. doi: 10.3390/agronomy9070395
Holbrook, N. M., Shashidhar, V. R., James, R. A., and Rana, M. (2002). Stomatal control in tomato with ABA-deficient roots: response of grafted plants to soil drying. Oecologia 53, 1503–1514. doi: 10.1007/BF00540117
Hossain, M. M., Lam, H. M., and Zhang, J. (2015). Responses in gas exchange and water status between drought-tolerant and -susceptible soybean genotypes with ABA application. Crop J. 3, 500–506. doi: 10.1016/j.cj.2015.09.001
Hsu, P. K., Takahashi, Y., Munemasa, S., Merilo, E., Laanemets, K., Waadt, R., et al. (2018). Abscisic acid-independent stomatal CO2 signal transduction pathway and convergence of CO2 and ABA signaling downstream of OST1 kinase. Proc. Natl. Acad. Sci. U.S.A. 115, E9971–E9980. doi: 10.1073/pnas.1809204115
Huang, B., Burgess, P., Chapman, C., and Zhang, X. (2019). Stimulation of growth and alteration of hormones by elevated carbon dioxide for creeping bentgrass exposed to drought. Crop Sci. 59, 1672–1680. doi: 10.2135/cropsci2018.07.0470
Ikegami, K., Okamoto, M., Seo, M., and Koshiba, T. (2009). Activation of abscisic acid biosynthesis in the leaves of Arabidopsis thaliana in response to water deficit. J. Plant Res. 122, 235–243. doi: 10.1007/s10265-008-0201-9
Innes, S. N., Solhaug, K. A., Torre, S., and Dodd, I. C. (2021). Different abscisic acid-deficient mutants show unique morphological and hydraulic responses to high air humidity. Physiol. Plant. 172, 1795–1807. doi: 10.1111/ppl.13417
Jia, G., Shevliakova, E., Artaxo, P., Noblet-Ducoudré, N., De, H., House, R. J., et al. (2019). Land–Climate Interactions. Climate Change and Land: An IPCC Special Report on Climate Change, Desertification, Land Degradation, Sustainable Land Management, Food Security, and Greenhouse Gas Fluxes in Terrestrial Ecosystems, Climate Change and Land. Geneva: Intergovernmental Panel on Climate Change.
Latif, H. H. (2014). Physiological responses of Pisum sativum plant to exogenous ABA application under drought conditions. Pak. J. Bot. 46, 973–982.
Leakey, A. D. B., Uribelarreà, M., Ainsworth, E. A., Naidu, S. L., Rogers, A., Ort, D. R., et al. (2006). Photosynthesis, productivity, and yield of maize are not affected by open-air elevation of CO2 concentration in the absence of drought. Plant Physiol. 140, 779–790. doi: 10.1104/pp.105.073957
Li, B., Feng, Y., Zong, Y., Zhang, D., Hao, X., and Li, P. (2020). Elevated CO2-induced changes in photosynthesis, antioxidant enzymes and signal transduction enzyme of soybean under drought stress. Plant Physiol. Biochem. 154, 105–114. doi: 10.1016/j.plaphy.2020.05.039
Li, S., Fang, L., Hegelund, J. N., and Liu, F. (2021). Elevated CO2 modulates plant hydraulic conductance through regulation of PIPs under progressive soil drying in tomato plants. Front. Plant Sci. 12:666066. doi: 10.3389/fpls.2021.666066
Li, S., Li, X., Wei, Z., and Liu, F. (2020). ABA-mediated modulation of elevated CO2 on stomatal response to drought. Curr. Opin. Plant Biol 56, 174–180. doi: 10.1016/j.pbi.2019.12.002
Li, S., and Liu, F. (2021). VapourVapor Pressure Deficit and Endogenous ABA Level Modulate Stomatal Responses of Tomato Plant to Soil Water Deficit. Denmark: University of Copenhagen.
Li, Y., Li, X., Yu, J., and Liu, F. (2017). Effect of the transgenerational exposure to elevated CO2 on the drought response of winter wheat: stomatal control and water use efficiency. Environ. Exp. Bot. 136, 78–84. doi: 10.1016/j.envexpbot.2017.01.006
Liu, F., Shahnazari, A., Andersen, M. N., Jacobsen, S. E., and Jensen, C. R. (2006). Physiological responses of potato (Solanum tuberosum L.) to partial root-zone drying: ABA signalling, leaf gas exchange, and water use efficiency. J. Exp. Bot. 57, 3727–3735. doi: 10.1093/jxb/erl131
Liu, J., Hu, T., Fang, L., Peng, X., and Liu, F. (2019). CO2 elevation modulates the response of leaf gas exchange to progressive soil drying in tomato plants. Agric. For. Meteorol 268, 181–188. doi: 10.1016/j.agrformet.2019.01.026
McAdam, S. A. M., Brodribb, T. J., Ross, J. J., and Jordan, G. J. (2011). Augmentation of abscisic acid (ABA) levels by drought does not induce short-term stomatal sensitivity to CO2 in two divergent conifer species. J. Exp. Bot. 62, 195–203. doi: 10.1093/jxb/erq260
Moshelion, M., Halperin, O., Wallach, R., Oren, R., and Way, D. A. (2015). Role of aquaporins in determining transpiration and photosynthesis in water-stressed plants: crop water-use efficiency, growth and yield. Plant Cell Environ. 38, 1785–1793. doi: 10.1111/pce.12410
Pantin, F., Monnet, F., Jannaud, D., Costa, J. M., Renaud, J., Muller, B., et al. (2013). The dual effect of abscisic acid on stomata. New Phytol. 197, 65–72. doi: 10.1111/nph.12013
Pérez-López, U., Robredo, A., Lacuesta, M., Muñoz-Rueda, A., and Mena-Petite, A. (2010). Atmospheric CO2 concentration influences the contributions of osmolyte accumulation and cell wall elasticity to salt tolerance in barley cultivars. J. Plant Physiol. 167, 15–22. doi: 10.1016/j.jplph.2009.06.019
Prerostova, S., Dobrev, P. I., Gaudinova, A., Knirsch, V., Körber, N., Pieruschka, R., et al. (2018). Cytokinins: their impact on molecular and growth responses to drought stress and recovery in Arabidopsis. Front. Plant Sci. 9:655. doi: 10.3389/fpls.2018.00655
R Core Team (2020). R: A Language and Environment for Statistical Computing. R Foundation for Statistical Computing: R Core Team.
Rasehke, K. (1975). Simultaneous requirement of carbon dioxide and abscisic acid for stomatal closing in Xanthium strumarium L. Planta 125, 243–259.
Sade, N., Gebremedhin, A., and Moshelion, M. (2012). Risk-taking plants: anisohydric behavior as a stress-resistance trait. Plant Signal. Behav. 7, 767–770. doi: 10.4161/psb.20505
Sade, N., Vinocur, B. J., Diber, A., Shatil, A., Ronen, G., Nissan, H., et al. (2009). Improving plant stress tolerance and yield production: is the tonoplast aquaporin SlTIP2;2 a key to isohydric to anisohydric conversion? New Phytol. 181, 651–661. doi: 10.1111/j.1469-8137.2008.02689.x
Sagi, M., Scazzocchio, C., and Fluhr, R. (2002). The absence of molybdenum cofactor sulfuration is the primary cause of the flacca phenotype in tomato plants. Plant J. 31, 305–317. doi: 10.1046/j.1365-313X.2002.01363.x
Sharp, R. E., LeNoble, M. E., Else, M. A., Thorne, E. T., and Gherardi, F. (2000). Endogenous ABA maintains shoot growth in tomato independently of effects on plant water balance: evidence for an interaction with ethylene. J. Exp. Bot. 51, 1575–1584. doi: 10.1093/jexbot/51.350.1575
Tal, M., Imber, D., Erez, A., and Epstein, E. (1979). Abnormal stomatal behavior and hormonal imbalance in flacca, a wilty mutant of tomato. Plant Physiol. 63, 1044–1048. doi: 10.1104/pp.63.6.1044
Tardieu, F., Simonneau, T., and Parent, B. (2015). Modelling the coordination of the controls of stomatal aperture, transpiration, leaf growth, and abscisic acid: update and extension of the Tardieu – Davies model. J. Exp. Bot. 66, 2227–2237. doi: 10.1093/jxb/erv039
Temme, A. A., Liu, J. C., Cornwell, W. K., Aerts, R., and Cornelissen, J. H. C. (2018). Hungry and thirsty: effects of CO2 and limited water availability on plant performance. Flora Morphol. Distrib. Funct. Ecol. Plants 254, 188–193. doi: 10.1016/j.flora.2018.11.006
Trenberth, K. E., Dai, A., Van Der Schrier, G., Jones, P. D., Barichivich, J., Briffa, K. R., et al. (2014). Global warming and changes in drought. Nat. Clim. Chang. 4, 17–22. doi: 10.1038/nclimate2067
Uddin, S., Löw, M., Parvin, S., Fitzgerald, G. J., Tausz-Posch, S., Armstrong, R., et al. (2018). Elevated [CO2] mitigates the effect of surface drought by stimulating root growth to access sub-soil water. PLoS One 13:e0198928. doi: 10.1371/journal.pone.0198928
Wei, Z., Fang, L., Li, X., Liu, J., and Liu, F. (2020). Effects of elevated atmospheric CO2 on leaf gas exchange response to progressive drought in barley and tomato plants with different endogenous ABA levels. Plant Soil 447, 431–446. doi: 10.1007/s11104-019-04393-3
Wullschleger, S. D., Tschaplinski, T. J., and Norby, R. J. (2002). Plant water relations at elevated CO2 - implications for water-limited environments. Plant Cell Environ. 25, 319–331. doi: 10.1046/j.1365-3040.2002.00796.x
Yan, F., Li, X., and Liu, F. (2017). ABA signaling and stomatal control in tomato plants exposure to progressive soil drying under ambient and elevated atmospheric CO2 concentration. Environ. Exp. Bot. 139, 99–104. doi: 10.1016/j.envexpbot.2017.04.008
Yan, F., Sun, Y., Song, F., and Liu, F. (2012). Differential responses of stomatal morphology to partial root-zone drying and deficit irrigation in potato leaves under varied nitrogen rates. Sci. Hortic. 145, 76–83. doi: 10.1016/j.scienta.2012.07.026
Zhang, F.-P., Sussmilch, F., McAdam, S. A. M., Brodribb, T. J., Nichols, D. S., Cardoso, A. A., et al. (2018). Leaves, not roots or floral tissue, are the main site of rapid, external pressure-induced ABA biosynthesis in angiosperms. J. Exp. Bot. 69, 1261–1267. doi: 10.1093/jxb/erx480
Zhang, J., and Davies, W. J. (1990). Changes in the concentration of ABA in xylem sap as a function of changing soil water status can account for changes in leaf conductance and growth. Plant. Cell Environ. 13, 277–285. doi: 10.1111/j.1365-3040.1990.tb01312.x
Zhang, J., Schurr, U., and Davies, W. J. (1987). Control of stomatal behaviourbehavior by abscisic acid which apparently originates in the roots. J. Exp. Bot. 38, 1174–1181. doi: 10.1093/jxb/38.7.1174
Zhang, J., Zhang, X., and Liang, J. (1995). Exudation rate and hydraulic conductivity of maize roots are enhanced by soil drying and abscisic acid treatment. New Phytol. 131, 329–336. doi: 10.1111/j.1469-8137.1995.tb03068.x
Zhang, Y., Oren, R., Kang, S., and Niinemets, Ü (2012). Spatiotemporal variation of crown-scale stomatal conductance in an arid Vitis vinifera L. cv. merlot vineyard: direct effects of hydraulic properties and indirect effects of canopy leaf area. Tree Physiol. 32, 262–279. doi: 10.1093/treephys/tpr120
Zhou, L., Xu, H., Mischke, S., Meinhardt, L. W., Zhang, D., Zhu, X., et al. (2014). Exogenous abscisic acid significantly affects proteome in tea plant (Camellia sinensis) exposed to drought stress. Hortic. Res. 1:14029. doi: 10.1038/hortres.2014.29
Keywords: elevated CO2, drought stress, exogenous ABA, stomatal conductance, leaf water potential
Citation: Li S and Liu F (2021) Exogenous Abscisic Acid Priming Modulates Water Relation Responses of Two Tomato Genotypes With Contrasting Endogenous Abscisic Acid Levels to Progressive Soil Drying Under Elevated CO2. Front. Plant Sci. 12:733658. doi: 10.3389/fpls.2021.733658
Received: 30 June 2021; Accepted: 25 October 2021;
Published: 24 November 2021.
Edited by:
Marta Silva Lopes, Institute of Agrifood Research and Technology (IRTA), SpainReviewed by:
Rogério Falleiros Carvalho, São Paulo State University, BrazilJaime Puertolas, Lancaster University, United Kingdom
Copyright © 2021 Li and Liu. This is an open-access article distributed under the terms of the Creative Commons Attribution License (CC BY). The use, distribution or reproduction in other forums is permitted, provided the original author(s) and the copyright owner(s) are credited and that the original publication in this journal is cited, in accordance with accepted academic practice. No use, distribution or reproduction is permitted which does not comply with these terms.
*Correspondence: Fulai Liu, ZmxAcGxlbi5rdS5kaw==