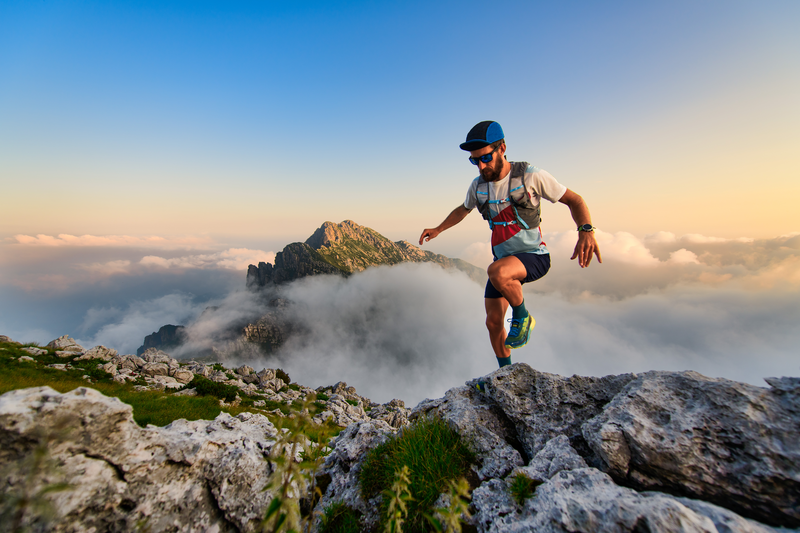
94% of researchers rate our articles as excellent or good
Learn more about the work of our research integrity team to safeguard the quality of each article we publish.
Find out more
EDITORIAL article
Front. Plant Sci. , 15 July 2021
Sec. Crop and Product Physiology
Volume 12 - 2021 | https://doi.org/10.3389/fpls.2021.726875
This article is part of the Research Topic Amaranthus: Naturally Stress-Resistant Resources for Improved Agriculture and Human Health View all 6 articles
Editorial on the Research Topic
Amaranthus: Naturally Stress-Resistant Resources for Improved Agriculture and Human Health
Adapting agriculture to global climate change, shifting patterns in food consumption, fulfilling the priorities of national and international programs, and instability in agricultural research funding trends will all be crucial challenges in the coming decades. Over the past 50 years, food supplies worldwide have trended toward increased homogeneity and interdependence on a limited number of major crop commodities (Khoury et al., 2014). This narrowing of crop diversity has substantial implications for future global food security and, perhaps more importantly, for human health with food quality as much a concern as quantity (Dwivedi et al., 2013). Addressing micronutrient deficiencies through biofortification has been a priority for major cereal and legume crops (Blair, 2013), but could equally be important in the sense of dietary diversification (Miller and Welch, 2013) and utilization of high mineral and high essential amino acid pseudocereals, such as grain amaranths, or vitamin-packed leaf vegetable species of the Amaranthus genus.
Current crop production patterns are not guaranteed given climate change predictions (Lobell et al., 2008; Wheeler and von Braun, 2013), mounting pressures to reduce environmental impacts of agriculture on soil health and water resources (Foley et al., 2005; FAO and ITPS, 2015), and other stressors that impact agricultural productivity (Borrell et al., 2020; Halsch et al., 2021). To keep pace with these pressures while maintaining yields, modern cultivars require continual development using genetic resources that must be conserved, evaluated for utility, and ultimately made accessible to breeders and farmers (Gepts, 2006). Wild relatives of crops are valuable sources of diversity and possessing useful traits to improve modern cultivars, but these plants are often underrepresented in germplasm repositories and under-prioritized for conservation (Khoury et al., 2019).
Orphan crops—i.e., cultivated plants that are under-utilized by comparison to major crops—are another diverse resource to foster resiliency in our food production systems, but suffer from similar challenges facing crop wild relatives due to research gaps and low prioritization for investment and conservation (Naylor et al., 2004). Thus, there are urgent needs for more research and conservation initiatives devoted to these resources to safeguard agricultural biodiversity, improve nutrition, and security of our global food supply. These issues provided the impetus for this Research Topic which focuses on Amaranthus species as promising resilient crops for the future.
Despite their wide history of use in many parts of the world, grain and vegetable amaranths are still considered orphan crops (FAO, 2018). The genus contains several domesticated or semi-domesticated species, many local landraces, little-studied wild species, and relevant weedy species, which together represent a broad spectrum of untapped genetic diversity (Shukla et al., 2017). Amaranths are increasing in popularity due to their favorable agronomic traits, including rapid growth rates, C4 photosynthesis, dual-uses for vegetable (leaf) and grain (seed) production, and tolerance to heat, drought, and salinity stress (Achigan-Dako et al., 2014; Hoidal et al., 2019). Amaranths are extraordinarily nutritious, and seeds produce several bioactive compounds of relevance for lowering the risk of chronic diseases (Velarde-Salcedo et al., 2019). These attributes make amaranths appealing models for basic and applied research and ripe for development as multidimensional crops—themes of which are explored in this Research Topic.
New findings have shed light on amaranth biology, ecology, and human-health benefits (Das, 2016; Velarde-Salcedo et al., 2019), yet questions remain toward improving our understanding of agronomic and adaptive traits in the genus. Three papers featured in this Research Topic contribute new knowledge to stress tolerance mechanisms in three distinct amaranth species grown as different crops in different parts of the world. Two of these papers by Estrada et al. and Sarker and Oba focus on salinity tolerance. Soil salinity poses a serious threat to agricultural production and increasingly contributes to land degradation worldwide (Panta et al., 2014; Cheeseman, 2015). Improving crops for salinity tolerance through conventional breeding has proven difficult due to the complexity of this trait, which has created interest in halophytic orphan crops like amaranth and quinoa in recent years. As highlighted by Estrada et al., the Amaranthaceae family comprises the highest proportion of halophytic species, and both amaranth and quinoa possess high genetic variability and adaptability to grow under salinity stress. Yet, few comparative studies with both species have been conducted. Their findings show different anatomical, physiologic, and molecular responses in A. caudatus and quinoa accessions under salt stress while still maintaining seed yield, which points to developing strategies for improving salt tolerance in these species.
In comparison, Sarker and Oba add to knowledge of salinity tolerance and the role of antioxidant mechanisms for A. tricolor, a popular inexpensive and nutritious leafy vegetable consumed throughout Southeast Asia where salt-affected land is particularly problematic. Stress caused by defoliation impacts growth and production in different ways depending on developmental stage and intensity level. Cisneros-Hernandez et al. investigate defoliation tolerance in A. cruentus, one of the most widely planted grain amaranth species, at different developmental stages and consequences on panicle emergence and flowering. These authors identified differences in carbon metabolism and allocation patterns as influenced by class II trehalose-phosphate synthase (TPS) genes. Trehalose-6-phosphate has emerged as an important signaling compound for plant growth and is known to help some plants withstand long periods of desiccation (Paul et al., 2018). Novel insights from these studies will undoubtedly inspire future work on the metabolites and biochemical pathways linked to stress response in Amaranthus and other crop species.
Although genomic resources for several cultivated and weedy Amaranthus species are available (Clouse et al., 2016; Gonçalves-Dias and Stetter, 2020; Montgomery et al., 2020), Deb et al. have contributed a valuable new reference genome of a superior local landrace variety of A. hypochondriacus from India. The significance of this study is not only a reference genome more suitable for breeding South Asian varieties of A. hypochondriacus, but also in demonstrating a methodology that could be applied to other local landraces and orphan crops in developing countries. This study is a welcome addition and complements previous efforts by Wu and Blair (2017) and Stetter and Schmid (2017) in genotyping by sequencing for SNP discovery.
Many studies have explored the chemical composition of amaranths revealing an exceptional nutritional balance compared to staple crop species. In this Research Topic, Nardo et al. reviews progress in this area over the last decade focusing on amaranth's promising role as a functional food with antihypertensive properties. Amaranth contains several bioactive compounds, including encrypted peptides with inhibitory functions against enzymes of the Renin-Angiotensin-Aldosterone System, which are targets of commonly prescribed pharmaceutical drugs for controlling hypertension. These bioactive compounds show promise for formulation of novel functional foods, but the authors also address the challenges related to demonstrating biological activity in complex food matrices.
Altogether, the papers presented in this Research Topic highlight the multidimensional benefits of both grain and vegetable amaranths and will facilitate interdisciplinary research for more climate-resilient varieties of orphan crops for sustainable food production across the world and improved human health among vegetarians and low-income consumers dependent on grain based diets.
All authors listed have made a substantial, direct and intellectual contribution to the work, and approved it for publication.
The authors declare that the research was conducted in the absence of any commercial or financial relationships that could be construed as a potential conflict of interest.
Achigan-Dako, E. G., Sogbohossou, O. E. D., and Maundu, P. (2014). Current knowledge on Amaranthus spp.: research avenues for improved nutritional value and yield in leafy amaranths in sub-Saharan Africa. Euphytica 197, 303–317. doi: 10.1007/s10681-014-1081-9
Blair, M. W. (2013). Mineral biofortification strategies for staples: the example of common bean. J. Agric. Food Chem. 61, 8287–8294. doi: 10.1021/jf400774y
Borrell, J. S., Dodsworth, S., Forest, F., Pérez-Escobar, O. A., Lee, M. A., Mattana, E., et al. (2020). The climatic challenge: which plants will people use in the next century? Environ. Exper. Bot. 170:103872. doi: 10.1016/j.envexpbot.2019.103872
Cheeseman, J. M. (2015). The evolution of halophytes, glycophytes and crops, and its implications for food security under saline conditions. New Phytol. 206, 557–570. doi: 10.1111/nph.13217
Clouse, J. W., Adhikary, D., Page, J. T., Ramaraj, T., Deyholos, M. K., Udall, J. A., et al. (2016). The amaranth (Amaranthus hypochondriacus) genome: genome, transcriptome and physical map assembly. Plant Genome 9, 1–14. doi: 10.3835/plantgenome2015.07.0062
Das, S. (2016). Amaranthus: A Promising Crop of Future. Singapore: Springer. doi: 10.1007/978-981-10-1469-7
Dwivedi, S., Sahrawat, K., Upadhyaya, H., and Ortiz, R. (2013). Food, nutrition and agrobiodiversity under global climate change. Adv. Agron. 120, 1–128. doi: 10.1016/B978-0-12-407686-0.00001-4
FAO (2018). Future Smart Food Rediscovering Hidden Treasures of Neglected and Underutilized Species for Zero Hunger in Asia, Executive Summary. Bangkok, 36.
FAO and ITPS. (2015). Status of the World's Soil Resources (SWSR) – Main Report. Food and Agriculture Organization of the United Nations and Intergovernmental Technical Panel on Soils. Rome: FAO and ITPS.
Foley, J. A., DeFries, R., Asner, G. P., Barford, C., Bonan, G., Carpenter, S. R., et al. (2005). Global consequences of land use. Science 309, 570–574. doi: 10.1126/science.1111772
Gepts, P. (2006). Plant genetic resources conservation and utilization: the accomplishments and future of a societal insurance policy. Crop Sci. 46, 2278–2292. doi: 10.2135/cropsci2006.03.0169gas
Gonçalves-Dias, J., and Stetter, M. G. (2020). PopAmaranth: a population genetic genome browser for grain amaranths and their wild relatives. bioRxiv. doi: 10.1101/2020.12.09.415331
Halsch, C. A., Shapiro, A. M., Fordyce, J. A., Nice, C. C., Thorne, J. H., Waetjen, D. P., et al. (2021). Insects and recent climate change. Proc. Natl. Acad. Sci. U.S.A. 118:e2002543117. doi: 10.1073/pnas.2002543117
Hoidal, N., Gallardo, M. D., Jacobsen, S., and Alandia, G. (2019). Amaranth as a dual-use crop for leafy greens and seeds: stable responses to leaf harvest across genotypes and environments. Front. Plant Sci. 10:817. doi: 10.3389/fpls.2019.00817
Khoury, C. K., Bjorkman, A. D., Dempewolf, H., Ramirez-Villegas, J., Guarino, L., Jarvis, A., et al. (2014). Increasing homogeneity in global food supplies and the implications for food security. Proc. Natl. Acad. Sci. U.S.A. 111, 4001–4006. doi: 10.1073/pnas.1313490111
Khoury, C. K., Greene, S. L., Krishnan, S., Miller, A. J., and Moreau, T. (2019). A road map for conservation, use, and public engagement around North America's crop wild relatives and wild utilized plants. Crop Sci. 59, 2302–2307. doi: 10.2135/cropsci2019.05.0309
Lobell, D. B., Burke, M. B., Tebaldi, C., Mastrandrea, M. D., Falcon, W. P., and Naylor, R. L. (2008). Prioritizing climate change adaptation needs for food security in 2030. Science 319, 607–610. doi: 10.1126/science.1152339
Miller, D. D., and Welch, R. M. (2013). Food system strategies for preventing micronutrient malnutrition. Food Policy 42, 115–128. doi: 10.1016/j.foodpol.2013.06.008
Montgomery, J. S., Giacomini, D., Waithaka, B., Lanz, C., Murphy, B. P., Campe, R., et al. (2020). Draft genomes of Amaranthus tuberculatus, Amaranthus hybridus, and Amaranthus palmeri. Genome Biol. Evol. 12, 1988–1993. doi: 10.1093/gbe/evaa177
Naylor, R. L., Falcon, W., Goodman, R. M., Jahn, M. M., Sengooba, T., Tefera, H., et al. (2004). Biotechnology in the developing world: a case for increased investments in orphan crops. Food Policy 29, 15–44. doi: 10.1016/j.foodpol.2004.01.002
Panta, S., Flowers, T., Lane, P., Doyle, R., Haros, G., and Shabala, S. (2014). Halophyte agriculture: success stories. Env. Exp. Bot. 107, 71–83. doi: 10.1016/j.envexpbot.2014.05.006
Paul, M. J., Gonzalez-Uriarte, A., Griffiths, C. A., and Hassani-Pak, K. (2018). The role of Trehalose-6-phosphate in crop yield and resilience. Plant Phys. 177, 12–23. doi: 10.1104/pp.17.01634
Shukla, A., Srivastava, N., Suneja, P., Yadav, S. K., Hussain, Z., Rana, J. C., et al. (2017). Untapped amaranth (Amaranthus spp.) genetic diversity with potential for nutritional enhancement. Genet. Resour. Crop Evol. 65, 243–253. doi: 10.1007/s10722-017-0526-0
Stetter, M. G., and Schmid, K. J. (2017). Analysis of phylogenetic relationships and genome size evolution of the Amaranthus genus using GBS indicates the ancestors of an ancient crop. Molec. Phylogenet. Evol. 109, 80–92. doi: 10.1016/j.ympev.2016.12.029
Velarde-Salcedo, A. J., Bojórquez-Velázquez, E., and Barba de la Rosa, A. P. (2019). “Amaranth” in Whole Grains and their Bioactives: Composition and Health, eds J. Johnson and T. C. Wallace (Hoboken, NJ: John Wiley & Sons Ltd.) 211–250. doi: 10.1002/9781119129486.ch8
Wheeler, T., and von Braun, J. (2013). Climate change impacts on global food security. Science 341, 508–513. doi: 10.1126/science.1239402
Keywords: amaranthus, orphan crops, salinity stress, defoliation, hypertension, whole genome sequencing
Citation: Riggins CW, Barba de la Rosa AP, Blair MW and Espitia-Rangel E (2021) Editorial: Amaranthus: Naturally Stress-Resistant Resources for Improved Agriculture and Human Health. Front. Plant Sci. 12:726875. doi: 10.3389/fpls.2021.726875
Received: 17 June 2021; Accepted: 22 June 2021;
Published: 15 July 2021.
Edited and reviewed by: Hans-Peter Kaul, University of Natural Resources and Life Sciences Vienna, Austria
Copyright © 2021 Riggins, Barba de la Rosa, Blair and Espitia-Rangel. This is an open-access article distributed under the terms of the Creative Commons Attribution License (CC BY). The use, distribution or reproduction in other forums is permitted, provided the original author(s) and the copyright owner(s) are credited and that the original publication in this journal is cited, in accordance with accepted academic practice. No use, distribution or reproduction is permitted which does not comply with these terms.
*Correspondence: Chance W. Riggins, Y3dyaWdnaW5AaWxsaW5vaXMuZWR1
Disclaimer: All claims expressed in this article are solely those of the authors and do not necessarily represent those of their affiliated organizations, or those of the publisher, the editors and the reviewers. Any product that may be evaluated in this article or claim that may be made by its manufacturer is not guaranteed or endorsed by the publisher.
Research integrity at Frontiers
Learn more about the work of our research integrity team to safeguard the quality of each article we publish.