- 1Laboratorio de Sistemática Molecular de Plantas, Facultad de Biología, Universidad Michoacana de San Nicolás de Hidalgo, Morelia, Mexico
- 2Departamento de Botánica y Zoología, Centro Universitario de Ciencias Biológicas y Agropecuarias, Universidad de Guadalajara, Guadalajara, Mexico
- 3Department of Ecology, Evolution, and Environmental Biology, Columbia University, New York, NY, United States
- 4Florida Museum of Natural History, University of Florida, Gainesville, FL, United States
- 5Laboratorio Nacional de Genómica para la Biodiversidad, Unidad de Genómica Avanzada del Centro de Investigación y de Estudios Avanzados del instituto Politécnico Nacional, Irapuato, Mexico
- 6Department of Biological Sciences, California State University, Long Beach, CA, United States
- 7California Botanic Garden, Claremont, CA, United States
The evolutionary relationships of Salvia have been difficult to estimate. In this study, we used the Next Generation Sequencing method Hyb-Seq to evaluate relationships among 90 Lamiaceae samples, including representatives of Mentheae, Ocimeae, Salvia subgenera Audibertia, Leonia, Salvia, and 69 species of subgenus Calosphace, representing 32 of Epling's sections. A bait set was designed in MarkerMiner using available transcriptome data to enrich 119 variable nuclear loci. Nuclear and chloroplast loci were assembled with hybphylomaker (HPM), followed by coalescent approach analyses for nuclear data (ASTRAL, BEAST) and a concatenated Maximum Likelihood analysis of chloroplast loci. The HPM assembly had an average of 1,314,368 mapped reads for the sample and 527 putative exons. Phylogenetic inferences resolved strongly supported relationships for the deep-level nodes, agreeing with previous hypotheses which assumed that subgenus Audibertia is sister to subgenus Calosphace. Within subgenus Calosphace, we recovered eight monophyletic sections sensu Epling, Cardinalis, Hastatae, Incarnatae, and Uricae in all the analyses (nDNA and cpDNA), Biflorae, Lavanduloideae, and Sigmoideae in nuclear analyses (ASTRAL, BEAST) and Curtiflorae in ASTRAL trees. Network analysis supports deep node relationships, some of the main clades, and recovers reticulation within the core Calosphace. The chloroplast phylogeny resolved deep nodes and four monophyletic Calosphace sections. Placement of S. axillaris is distinct in nuclear evidence and chloroplast, as sister to the rest of the S. subg. Calosphace in chloroplast and a clade with “Hastatae clade” sister to the rest of the subgenus in nuclear evidence. We also tested the monophyly of S. hispanica, S. polystachia, S. purpurea, and S. tiliifolia, including two samples of each, and found that S. hispanica and S. purpurea are monophyletic. Our baits can be used in future studies of Lamiaceae phylogeny to estimate relationships between genera and among species. In this study, we presented a Hyb-Seq phylogeny for complex, recently diverged Salvia, which could be implemented in other Lamiaceae.
Introduction
Phylogenetic relationships for many plant groups have been studied through the last 30–40 years at deep (APG, 1998; Zeng et al., 2017; Breinholt et al., 2021) and shallow phylogenetic levels (Wells et al., 2020), mostly through Sanger sequencing (Sanger et al., 1977) and recently through Next Generation Sequencing (Wanke et al., 2017; Carlsen et al., 2018; Herrando-Moraira and The Cardueae Radiations Group, 2018; Villaverde et al., 2018; Carter et al., 2019; Johnson et al., 2019). However, in groups with recent radiation events (Larridon et al., 2020) such as Salvia L. (Walker and Sytsma, 2007; Jenks et al., 2013; Fragoso-Martínez et al., 2018; González-Gallegos et al., in press), many questions remain at the shallow-phylogenetic scale, such as relationships among sections, among species, and species monophyly.
The sages (Salvia) with ca. 1,000 species (Harley et al., 2004; Drew et al., 2017), are among the largest angiosperm genera (Frodin, 2004). They are widely distributed with many economically important species (Wu et al., 2012; Lopresti, 2017). Salvia flowers are bilabiate and have evolved a wide variety of showy colors and shapes (Lara-Cabrera et al., in press), as well as staminal levers and other morphological adaptations to pollinators (Claßen-Bockhoff et al., 2004; Wester and Claßen-Bockhoff, 2011; Benítez-Vieyra et al., 2014; Kriebel et al., 2019, 2020; Celep et al., 2020). Previous Salvia phylogenies that employed few, e.g., <5–10, chloroplast or nuclear coding and non-coding loci were successful in reconstructing relationships at many deep-level nodes. These studies showed that Salvia is polyphyletic with five embedded genera, namely, Dorystaechas Boiss. and Heldr. ex Benth., Meriandra Benth., Perovskia Kar., Rosmarinus L., and Zhumeria Rech. f. and Wendelbo (Walker et al., 2004; Walker, 2006; Walker and Sytsma, 2007). Salvia species are classified into five subgenera, namely, Salvia, Audibertia J. B. Walker, B. T. Drew and K. J. Sytsma, Calosphace (Benth.) Epling, Leonia Cerv., and Sclarea Mill. A proposal to “lump” these genera into Salvia would add five more subgenera to Salvia (Drew et al., 2017), which are Dorystaechas (Boiss. and Heldr. ex Benth.) J. B.Walker, B. T. Drew, and J. G. González, Meriandra (Benth.); J. B. Walker, B. T. Drew, and J. G. González, Perovskia (Kar.); J. B. Walker, B. T. Drew, and J. G. González, Rosmarinus (L.); J. B. Walker, B. T. Drew, and J. G. González, and Zhumeria (Rech.f. and Wendelbo); J. B. Walker, B. T. Drew, and J. G. González. Among these, we focused in this study mainly on the American subgenus Calosphace and some representatives in subgenera Audibertia, Leonia, and Salvia s.s.
Salvia subg. Calosphace is distributed from southern USA to Argentina (Ramamoorthy and Elliott, 1998; Walker et al., 2004), with ca. 580 (González-Gallegos et al., in press) to 600 species (Martínez-Gordillo et al., 2017). It is most diverse in Mexico and Central America (275 species), the Andes (155 species), Eastern South America (60 species), and the Antilles (45 species; Jenks et al., 2013). Given S. subg. Calosphace species diversity and morphological complexities, it has been classified into 102 sections (Epling, 1939, 1940, 1941, 1944, 1947, 1951; Epling and Mathias, 1957; Epling and Jativa, 1963). However, the sectional classification has been criticized (Standley and Williams, 1973; Torke, 2000; Walker, 2006; Wood, 2007), given the few characters employed to define sections, and disjunct distribution of some species. Regardless, Epling's classification is recognized as a necessary starting point to further the study on Salvia until a new monograph is compiled (Ramamoorthy, 1984; Wood, 2007; Klitgaard, 2012).
Previous phylogenetic studies of Calosphace resolved S. axillaris Moc. and Sessé sister to the rest of the subgenus (Walker et al., 2004; Walker and Sytsma, 2007; Jenks et al., 2013; Drew et al., 2017; Fragoso-Martínez et al., 2018; Kriebel et al., 2019), followed by the Hastatae clade (Salvia patens Ort. + Salvia vitifolia Benth.); members of the S. sects. Tomentellae, Dusenostachys, Uliginosae, Erytrostachys, Micranthae, Fulgentes, and Membranaceae (Fragoso-Martínez et al., 2018) or Fulgentes was paraphyletic to members of sects. Cardinalis and Flocculosae (Jenks et al., 2013). The “Core Calosphace” contains the most species and relationships within this clade that have been difficult to resolve or have had low branch support. The “Core Calosphace” clade was initially described by Walker (2006) and refers to a clade of “core radiation” that is “difficult to characterize morphologically but is well-supported in the molecular analyses...”. It has been hypothesized that recent divergence events are clouding the phylogenetic signal, which could be further tested with expanded taxon sampling and additional phylogenetically informative sequence data (Olvera-Mendoza et al., 2020; Villaverde et al., 2020). This was attempted by Fragoso-Martínez et al. (2017) and Kriebel et al. (2019) using hybrid enrichment protocols across Salvia and to test sectional monophyly of the Calosphace. The Anchored Hybrid Enrichment (AHE) Angiosperm kit v. 1 (Buddenhagen et al., 2016) was tested on 12 Salvia species and captured 399 nuclear loci (Fragoso-Martínez et al., 2017) and later the protocol was used for 35 Salvia (13 Calosphace and 2 Audibertia) species capturing 316 nuclear genes (Kriebel et al., 2019). Both phylogenies improved clade resolution as compared to previous sequencing studies (Walker et al., 2004; Walker and Sytsma, 2007; Jenks et al., 2013; Will and Claßen-Bockhoff, 2017; Fragoso-Martínez et al., 2018; Hu et al., 2018).
In this study, we used the Hyb-Seq protocol (Weitemier et al., 2014) for target enrichment of low copy nuclear exons and flanking regions and genome skimming of organellar genomes. Hyb-Seq has been successfully used to solve shallow-level phylogenetic relationships in Asclepias L. (Straub et al., 2011, 2012), Annonaceae (Couvreur et al., 2019), Asteraceae (Mandel et al., 2017; Herrando-Moraira and The Cardueae Radiations Group, 2019; Johnson et al., 2019; Jones et al., 2019), Poaceae (Fisher et al., 2016), and Rubus (Carter et al., 2019), among others. We used MarkerMiner (Chamala et al., 2015) to identify low copy nuclear loci in 22 Lamiaceae transcriptomes (including Salvia officinalis L. and S. splendens Sellow ex Schult.) and design both general and specific purpose bait sets. We sampled a total of 90 Lamiaceae from tribes Mentheae and Ocimeae, 75 samples represent 32 of Epling's S. subg. Calosphace sections. Our goals were to test classification of Epling and relationships found in previous studies of subg. Calosphace; test species monophyly for four important and morphologically complex species. Furthermore, we aimed to identify sufficiently polymorphic loci for future studies in Salvia.
Methods
Taxonomic Sampling
The study materials consisted of 90 Lamiaceae from nine genera which were sampled (Supplementary Table 1). Exactly 10 species were sampled from tribe Mentheae [Agastache pallidiflora subsp. neomexicana (Briq.) Lint and Epling, Dracocephalum parviflorum Nutt., Hedeoma drummondii Benth., Lepechinia hastata (A. Gray) Epling, Lepechinia sp., Lycopus americanus Muhl., Melissa officinalis L., Poliomintha incana (Torr.) A. Gray, and Prunella vulgaris L.] and one species was sampled from the tribe Ocimeae [Cantinoa mutabilis (Rich.) Harley and J. F. B. Pastore] to root the trees (Li et al., 2016).
Multiple subgenera of Salvia were represented in our sampling, two each from the S. subg. Audibertia sect. Audibertia (S. brandegeei Munz and S. sonomensis Greene) and S. subg. Salvia sect. Salvia (the Mediterranean S. officinalis and the Malagasy S. sessilifolia A. Gray ex S. Watson), and one from the S. subg. Leonia sect. Salviastrum [S. texana (Scheele) Torr.]. From the S. subgenus Calosphace, we sampled 72 species (Supplementary Table 1) in all, representing 32 of the 102 sections sensu Epling. Our sampling represents the geographic range of the taxon in Mexico (67 species; Supplementary Table 1) and includes five additional species from Central and South America (S. pauciserrata Benth., S. scutellarioides Kunth, S. splendens, S. squalens Kunth, and S. tubiflora Sm.). Seven species were sampled for molecular study for the first time (S. brachyodonta Briq., S. decora Epling, S. dichlamys Epling, S. perblanda Epling, S. puberula Fernald, S. purepecha Bedolla, S. Lara Cabrera and Zamudio, and S. roscida Fernald). Additionally, we included two samples from distinct provenances for Salvia hispanica L., Salvia polystachia Cav., Salvia purpurea Cav., and Salvia tiliifolia Vahl., to assess their monophyly, which further tested the resolving power of this protocol.
Phylogenetic Marker Selection, Bait Design, and DNA Sequencing
Genomic DNA was isolated from 10 mg of silica-dried leaf material using a modified 2X CTAB protocol (Doyle and Doyle, 1987). DNAs were quantified using a Qubit 2.0 fluorometer (Thermo Fisher Scientific Inc., Waltham, MA, USA) and diluted to a concentration of 20 ng/μl. Afterward, 60 μl of DNA solution were transferred to a 96-well plate and shipped to Rapid Genomics (Gainesville, FL, USA) for library preparation, hybrid enrichment of nuclear loci, and paired-end (2 × 150 bp) sequencing on the Illumina HiSeq 2500 instrument (Thermo Fisher Scientific Inc.).
A multipurpose bait set was designed for use across independent research projects with Salvia, Acanthaceae, Clusiaceae, Lamiales, and Polemoniaceae. To select loci and provide sequence data for bait design for the Salvia and Lamiales studies, we analyzed a set of 77 transcriptomes from the One Thousand Plant Transcriptomes Initiative (OneKp), including 68 from Lamiales and 9 from outgroup taxa representing Boraginales, Gentianales, and Solanales (One Thousand Plant Transcriptomes Intitiative, 2019), and an additional transcriptome for S. splendens Sellow ex Wied-Neuw. in Genbank [Ge et al., 2014; https://www.ncbi.nlm.nih.gov/bioproject/422035 (Taxonomy ID: 180675)]. We used the MarkerMiner 1.0 (Chamala et al., 2015) pipeline with its default settings to assess putative orthology among transcripts in our data set with a set of Arabidopsis thaliana (L.) Heynh. transcripts from genes that were identified as single- (or low-)copy across angiosperms by an orthology analysis of 20 genomes (De Smet et al., 2013), mapping to chromosomes 1, 2, 3, 4, and 5 in the A. thaliana genome (Table 1); at the time we had no fully annotated Lamiaceae genome. Gene clusters identified by MarkerMiner were aligned with MAFFT (Katoh et al., 2002) and individually reviewed for marker selection.
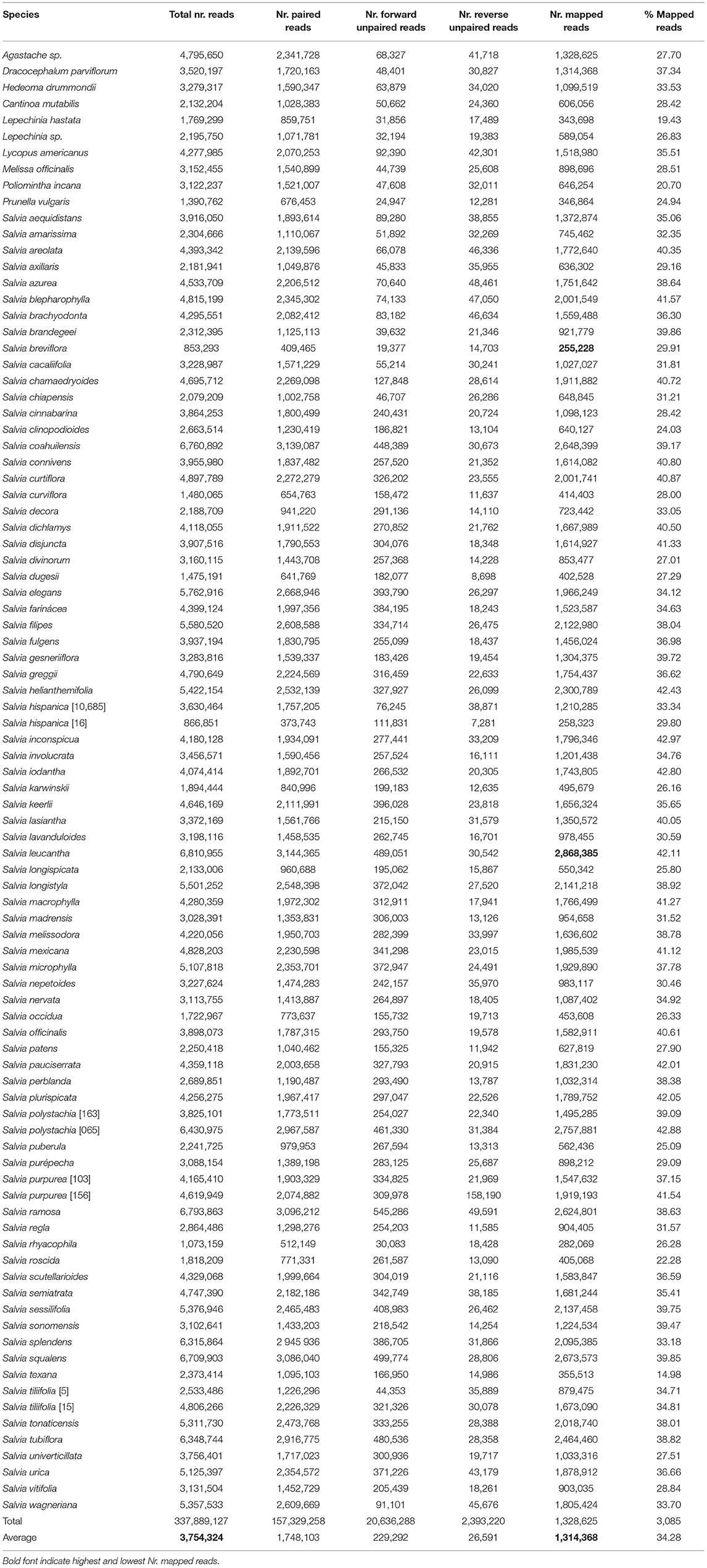
Table 1. HPM assembly characteristics per sample for 90 samples targeting 119 nuclear genes, 26 genes were later filtered through the next steps in HPM.
The final selection of loci for bait design was based on the following criteria: sequence variability, align-ability, demonstrated phylogenetic utility within Lamiales and Lamiaceae (Godden, unpublished data), and economic considerations. The latter criterion dictated the numbers of loci and baits per project that could be accommodated in the final multipurpose bait set. Overall, baits in the multipurpose set relevant to this project included the following: 883 Lamiales general-purpose baits (76,272 bp) and 1,207 Salvia-specific baits (131,394 bp), based on the Lamiales transcriptomes and S. officinalis and S. splendens alignments for the latter (Supplementary Table 2). Paired baits were manufactured with TruSeq technology by myBaits (Daicel Arbor Biosciences, Ann Arbor, MI, USA). Samples were sequenced on an Illumina® HiSeq 2,500 as 150 bp PE reads. Raw read quality was assessed with Fastqc v.0.11.2 (Andrews, 2010; Babraham Bioinformatics, Cambridge, England). Adapter sequences and low-quality bases were trimmed using Cutadapt v. 1.8.1 (Martin, 2011).
Assembly
Raw reads were processed in HybPhyloMaker (HPM) v.1.6.4 (Fér and Schmickl, 2018), this pipeline contains multiple steps or scripts that allow assembly and further analyses (from here on throughout the text, these are quoted per acronym and numbered as specified in the script name from the HPM reference manual). Using the script HPM_0b in the pipeline, individual reads were mapped to two pseudo reference sequences. The first nuclear pseudo reference was the alignment of the probe set containing 527 putative exons (these were previously used as probes to target the specified genes) and the second pseudo reference was 114 chloroplast loci from Salvia miltiorrhiza Bunge complete plastome JX312195 (Qian et al., 2013), separated by 400 Ns to capture any chloroplast sequences.
In order to summarize the effectiveness of capture based on our nuclear pseudo reference, we used all sequences for each exon produced by HPM_3 and calculated the missing data for each of them compared with the original probes in a heat map (Figure 1).
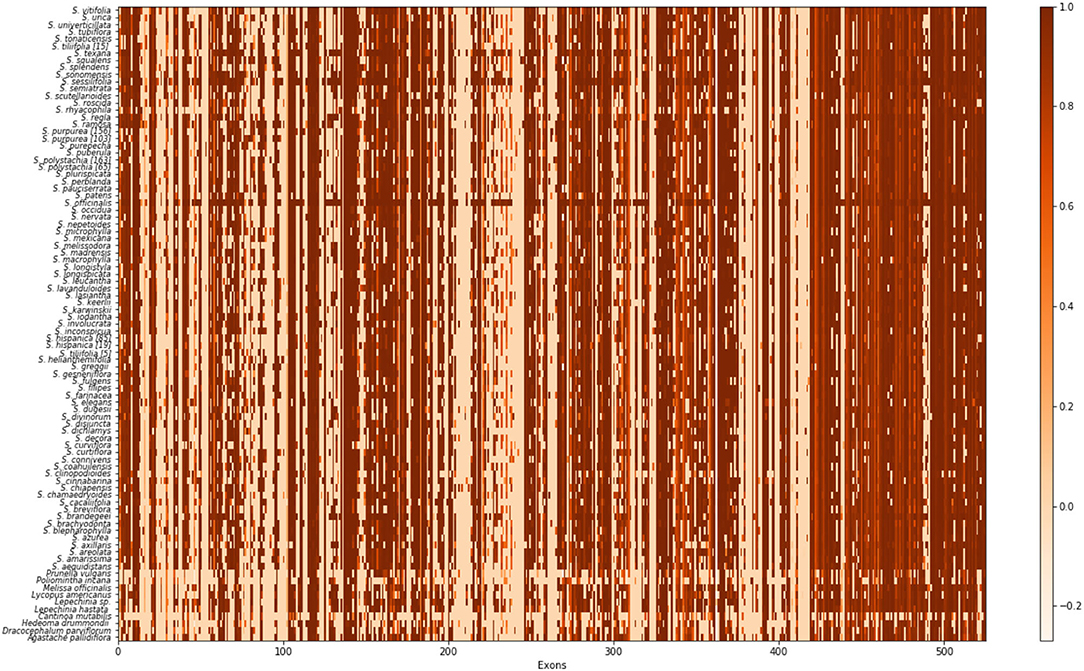
Figure 1. Exon recovery heat map for 527 putative exons targeted by our baits. Each column represents an exon, and each row is each species. Each cell represents the sequence completeness; a lighter color signifies fewer bases recovered for that exon and a darker color signifies more bases were recovered.
The reads were trimmed, filtered, and mapped to create the alignments for reconstructing gene and species trees, using the following steps: script HPM_1 was used to remove sequencing adapters and trim reads based on their quality using Trimmomatic v.0.33 (Bolger et al., 2014). All reads <Q20 were discarded, and the remaining reads were trimmed if the average quality in a 5 bp window was <Q20. Reads shorter than 36 bp were removed. In addition, HPM uses FastUniq v.1.1 (Xu et al., 2012) to remove duplicate reads. The script HPM_2 was used to map the quality filtered and trimmed reads to the baits pseudo reference using BWA v.0.7.16a (Li and Durbin, 2009). Mapped reads for each taxon were summarized with a consensus sequence using Kindel v.0.1.4 (Constantinides and Robertson, 2017) included in the HP pipeline. This used a 51% majority consensus rule to call bases and convert any base with low coverage (2x) to an uninformative base (N). This was repeated to consecutively map the filtered reads to the chloroplast pseudo reference.
Consensus sequences were matched to sequences of target exons using BLAT v.35 (Kent, 2002) (https://www.ncbi.nlm.nih.gov/pubmed/11932250), with 90% similarity for all samples to produce PSLX files using the script HPM_3. The script “assembled_exons_to_fastas.py” (Weitemier et al., 2014) is used in the script HPM_4a to construct matrices for multiple alignments and add Ns for taxa that lack a particular exon. Also, with the script HPM_4a, sequences were aligned in MAFFT v. 7.305 (Katoh and Standley, 2013) and nuclear exons belonging to the same gene were concatenated using AMAS (Borowiec, 2016). Finally using the script HPM_5 taxa, we took a conservative approach and removed exons from the alignment if more than 70% of the sequence missing and if exons were recovered in fewer than 75% of the taxa. We also tested the effect of this approach by varying our criterion to 30, 50, and 75% missing data for loci shared by all species in the HPM_5 matrix.
The two resulting data sets comprised 119 targeted nuclear genes and 114 loci for the chloroplast. Both data sets were independently filtered as described above to remove genes from the alignment with excessive missing data. After filtering, the alignments included 96 nuclear genes and 114 chloroplast loci.
Phylogenetic Analyses
Bayesian and Maximum Likelihood (ML) multispecies coalescent-based approaches were used to reconstruct species trees for the nuclear data. For Bayesian inference, we used BEAST v. 2.5.2 (Bouckaert et al., 2019) for the genes obtained from the HPM pipeline. First, the best fitting molecular evolution model was obtained for each independent gene using jModelTest v. 2.1.10 (Darriba et al., 2012). Four models were selected as best fitting (GTR + G, HK + G, K80 + G, and SYM + G). We ran BEAUTI v. 2.5.2 (Bouckaert et al., 2014) using the template for StarBEAST to prepare the BEAST analysis input file. In the analysis, trees were unlinked and the strict clock model was used for all of them. Genes with the same molecular evolution model had linked parameters. Finally, a coalescent constant population model was used as a prior on the species tree. We ran BEAST for 1.6 B states, sampling every 5,000 states. Tracer v. 1.6 (Drummond and Rambaut, 2007) was used to check ESS values. To construct a maximum clade credibility tree, we used TreeAnnotator v. 2.5.6 (Bouckaert et al., 2019) setting a burn-in of 25% of the states and “Mean Height” for node heights.
For ML inference, we used the scripts HPM_6b and HPM_7, that execute FastTree 2.1.10 SSE3 (Price et al., 2010) using default parameters, to generate trees for every gene in our dataset and root them using the external group (Cantinoa). Next, the species tree was inferred using the coalescent-based approach implemented in ASTRAL-III v. 5.6.1 (Zhang et al., 2018) running the script HPM_8a with default parameters. To reconstruct the phylogenetic network, we used the 96 gene trees produced by HPM_7 as input to NANUQ (Allman et al., 2019) incorporated in the MSCquartets package (Rhodes et al., 2021) for R (R Core Team, 2017, Vienna, Austria). We set an alpha of 1e-5 and a beta of 0.95 with the goal of testing for a signal of network cycles in the quartets. Later, we used SplitsTree (Huson and Bryant, 2006) to plot the network using default parameters.
To test the robustness of the phylogenetic inferences obtained for both nDNA and cpDNA matrices, we compared trees with different percentages of missing data (30, 50, and 75% missing), and a tree that maintains loci for all the samples (as opposed to removing loci present in fewer than 75% of taxa). For each dataset with different missing data, we re-ran the nuclear ASTRAL reconstruction and the chloroplast FastTree analysis with the parameters described earlier.
Results
Bait Success and Assembly
After removing low-quality sequences and loci with many missing taxa in HPM, 96 of 119 genes targeted by our respective bait sets were retained for analysis. Samples had an average of 1,314,368 mapped reads (Table 1), with the fewest in S. breviflora Moc. and Sesse ex Benth. (255,228 reads) and the most in S. leucantha Cav. (2,868,385 reads). The length of nuclear gene alignments ranged from 154 bp (AT1G05350) to 3,336 bp (AT4G19490). In total, 527 putative exons were recovered. However, about a third of the targeted exons were retained for further analysis (Supplementary Tables 3, 4). The filtering step in HPM removed some of the 527 putative exons, given that exon capture was not homogeneous across all samples nor loci. Fewer base pairs were recovered for the outgroup than the in-group and the highest recovery was in S. officinalis, one of the transcriptomes used to design the Salvia baits.
The HPM chloroplast assembly for all 90 samples, using the S. miltiorrhiza genome (Qian et al., 2013) as a pseudoreference, recovered 75 CDS (59 in the LSC, 5 IR-B, 10 SSC, 1 IR-A), 29 tRNA (20 LSC, 7 IR-B, 1 SSC), 5 genes with introns (3 LSC, 1 IR-B, 1 SSC), 4 rRNA in the IR-B and 1 IGS in the LSC region (Supplementary Table 5); ranging in length from 36 bp (rps19) to 6,870 bp (ycf 2).
Phylogenetic Inferences
All nuclear phylogenetic inferences, with both coalescent analyses HPM [BEAST (Figure 2) and ASTRAL (Supplementary Figure 1)] recovered similar tree topologies, with some differences in shallow-level relationships. A network of the nuclear alignment (Figure 3) revealed the same groupings in the outgroup and some reticulation within the core Calosphace as we recovered in our phylogenetic analyses. A quartet hypothesis test showed that a majority of quartets had a tree-like signal, with only a few quartets better represented as four-cycle networks (Figure 3). We also tested if varying the missing data to 30 (Supplementary Figure 2A), 50 (Supplementary Figure 2B), or 70% (Supplementary Figure 2C) would have an impact on the overall tree topologies (Supplementary Figure 1), but there were no major differences in the topologies and only differences in support values for some branches. Species relationships in the broader Lamiaceae HPM assembly were rooted with C. mutabilis (tribe Ocimeae), followed by a clade which includes Dracocephalum, Agastache, Lycopus, and Prunella (1 local posterior probability [localPP] in every three), a second sister clade with Poliomintha and Hedeoma (1 localPP), and the third clade with Melissa and Lepechinia (Figure 2). The four Salvia subgenera sampled (Figures 2, 3; Supplementary Figures 1 and 2) are in “clade I” (clade nomenclature sensu; Walker et al., 2004; Jenks et al., 2013) with 1 localPP in every inference. Clade 1 included S. subg. Salvia (S. officinalis) and Leonia (S. sessilifolia and S. texana), sister to a clade of S. subg. Audibertia and Calosphace (1 localPP).
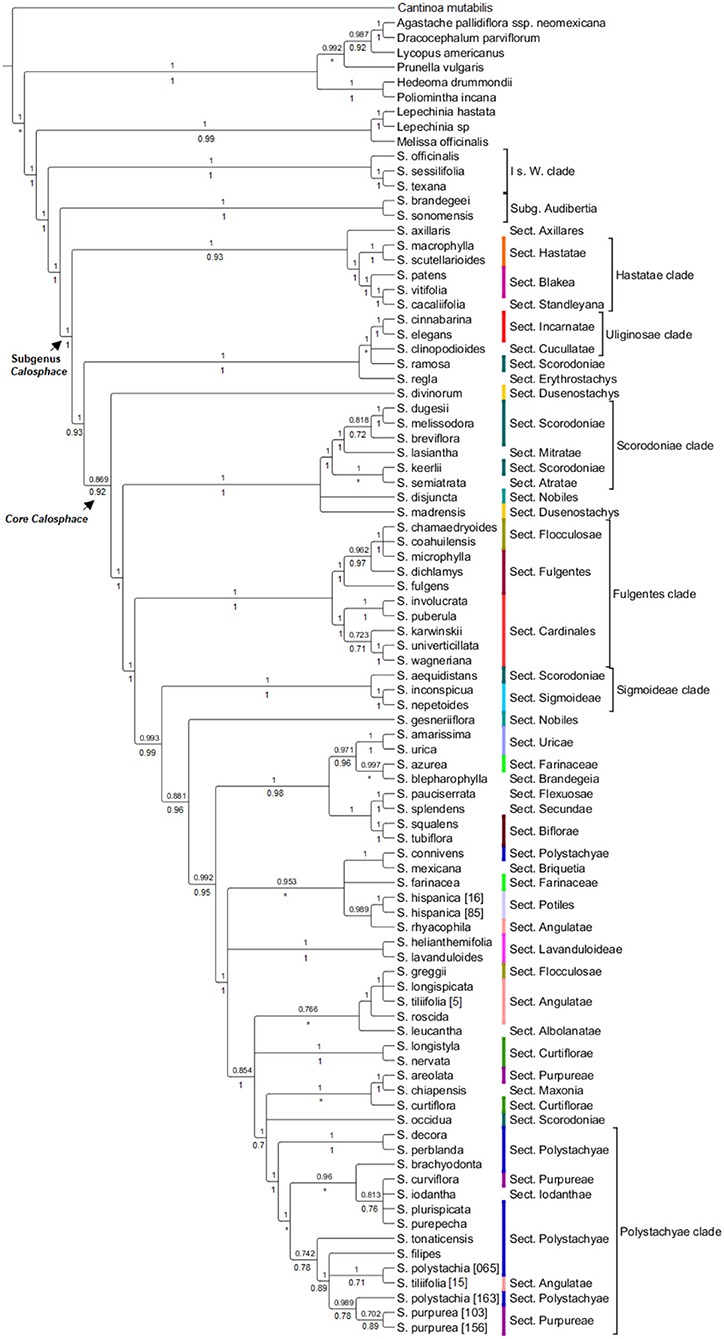
Figure 2. HPM- BEAST tree for 96 nuclear genes with up to 70% missing data allowed for each exon. The BEAST local posterior probability is indicated above branches from the analysis and the ASTRAL analysis is under the branches. Branches with support values <0.7 are collapsed. Salvia subgenus Calosphace sections s. Epling are color-coded. The main clades follow previous nomenclature (Walker et al., 2004; Jenks et al., 2013; Fragoso-Martínez et al., 2018).
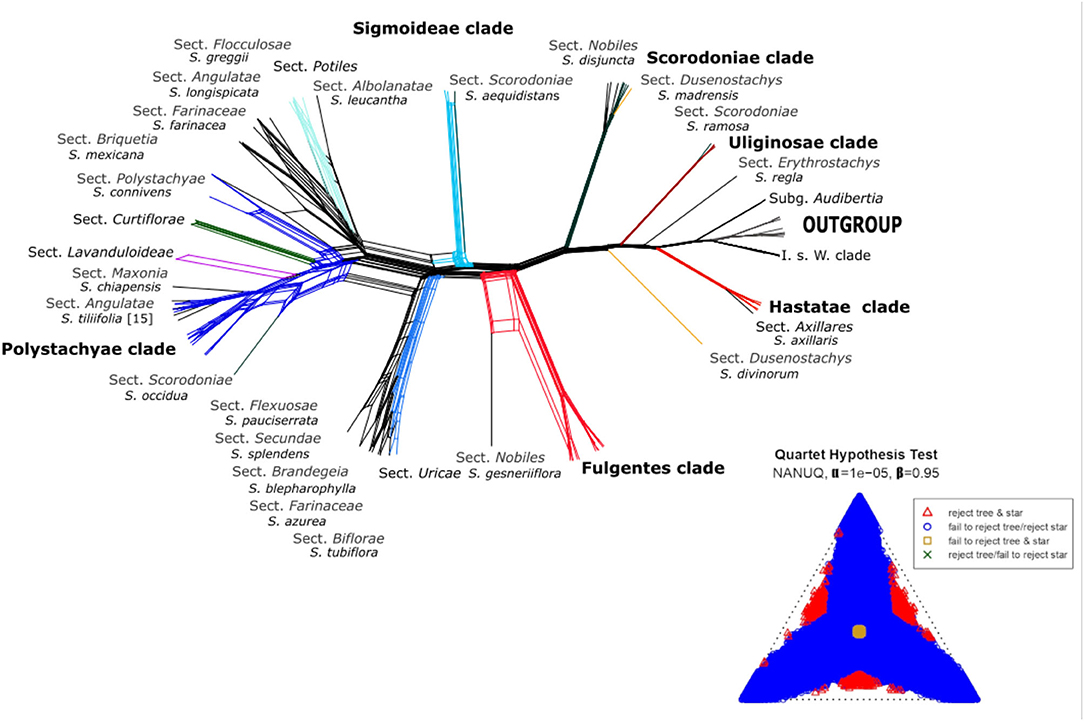
Figure 3. Network analysis for 527 putative nuclear exons with S. subg. Calosphace sections are color-coded as in Figure 1. A simplex plot of NANUQ's quartet hypothesis test at α = 0.0001 and ß = 0.95.
There were 8 out of the 13 Salvia subg. Calosphace sections sensu Epling which were sampled here and represented by more than one sample were monophyletic in all analyses (Table 2). They include Cardinalis, Biflorae, Hastatae, Incarnatae, Lavanduloideae, Sigmoideae, and Uricae, while Curtiflorae was only monophyletic in the nuclear ASTRAL and FastTree trees.
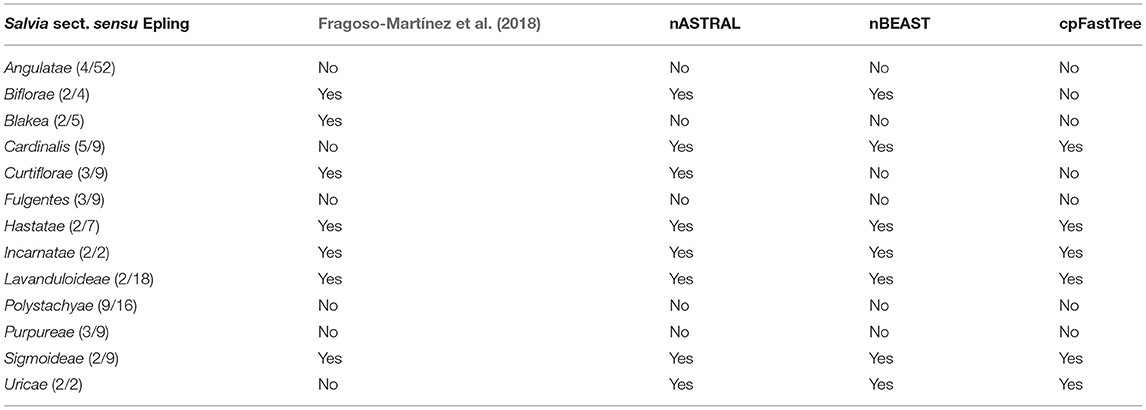
Table 2. Salvia subgenus Calosphace monophyletic sections s. Epling, comparative of previous phylogenetic analysis and our Hyb-seq three nuclear and chloroplast analyses.
Several clades within S. subg. Calosphace was well-resolved and strongly supported by our phylogenetic results. A “Hastatae clade” with 1 PP (ASTRAL/BEAST) includes members of the S. sects. Hastatae, Blakea, and Standleyana are sisters to S. axillaris (monotypic S. sect. Axillares) (Figure 2). The “Uliginosae clade” includes a monophyletic S. sect. Incarnatae (Salvia elegans Vahl. + Salvia cinnabarina M. Martens and Galeotti) in all the analyses (1 localPP), and one sample each in S. sects. Erythrostachys (Salvia regla Cav.), Cucullatae (Salvia clinopodioides Kunth) and Scorodoniae (Salvia ramosa Brandegee). Following these Calosphace clades, we reached the “core Calosphace” (64 of the remaining species), where resolution and clade support are variable in the nuclear phylogenetic inferences (Figures 2, 3; Supplementary Figures 1, 2).
Within the “core Calosphace,” the highly supported clades (1 localPP) included the “Scorodoniae clade” with species in S. sects. Atratae, Mitratae, and Scorodoniae. A large “Fulgentes clade” with monophyletic S. section Cardinales (with five of its nine species sampled) and some members of S. sects. Fulgentes and Flocculosae (1 local PP BEAST/ASTRAL). The “Sigmoideae clade” (1 local PP BEAST/ASTRAL) with Salvia inconspicua Benth. + Salvia nepetoides Kunth. and Salvia aequidistans Fernald (S. sect. Scorodoniae); a large clade with Salvia gesneriiflora Lindl. and Paxton in S. sect. Nobiles from Walker's “Fulgentes clade” [BEAST (0.881 local PP); ASTRAL (0.96 local PP)] and smaller strongly supported clades (1 local PP BEAST/ASTRAL) including monophyletic S. sects. Uricae, “Lavanduloideae clade,” and “Biflorae clade,” while Curtiflorae only in ASTRAL (0.96 localPP). Finally, the “Polystachyae clade” (1 localPP BEAST/ASTRAL), includes representatives from the S. sect. Angulatae (S. tiliifolia), Iodanthae (Salvia iodantha Fernald), Polystachyae (S. brachyodonta, S. decora, Salvia filipes Benth., S. perblanda, Salvia plurispicata Epling, S. polystachia, S. purepecha, Salvia tonaticensis Ramamoorthy ex Lara-Cabrera, Bedolla and Zamudio), and sect. Purpureae (Salvia curviflora Benth. and S. purpurea) and two samples each for S. polystachia (non-monophyletic) and S. purpurea, (monophyletic; Figures 2, 3; Supplementary Figure 1).
The ML concatenated FastTree of the chloroplast loci (Figure 3) for the 90 samples, provided high support (1 localPP) for deep-level relationships within the Ocimiae and Menthae, and a sister relationship between S. subgenera Audibertia and Calosphace. Well-resolved and highly supported clades in this tree include S. axillaris as sister to the rest of subg. Calosphace; the “Hastatae clade” (1 localPP) and “Uliginosae clade” (1 localPP), with monophyletic S. sect. Cardinales (0.99 localPP), Hastatae (1 local PP), Incarnatae (1 local PP), and Uricae (1 localPP), and S. hispanica (two sampled). However, resolution and clade support are reduced for a few of the “core Calosphace,” such as the S. genesneriflora polytomy and S. sect. Cucullatae + Scorodoniae, Flexulosae, Farinaceae, Albolanatae. Two sections are not monophyletic for the cpDNA data Lavanduloides and Sigmoideae.
Discussion
NGS in Salvia
The Hyb-Seq protocol (Weitemier et al., 2014) implemented, here, resolved deep phylogenetic relationships in Lamiaceae, among Salvia subgenera, and within a recently diverged S. subg. Calosphace (Figure 2; Supplementary Figure 1), providing additional support for existing phylogenetic hypotheses (Walker, 2006; Jenks et al., 2013; Fragoso-Martínez et al., 2018). We enriched 119 nuclear loci (Supplementary Tables 3, 4), 96 of which were left for phylogenetic estimations after the HPM filtering process.
To date, this is the largest base-pair sampling for this many Salvia species using the next-gen technology and specifically designed baits, and we were able to recover 1,314,368 bp (Table 1) in the HPM assembly for 96 nuclear genes in all 90 Lamiaceae sampled (14,604 b per sample; Supplementary Table 4). Previous anchored hybrid enrichment experiments in Salvia sampled 12 species for 453 loci producing a final alignment of 282,219 bp or 23,518 bp per sample (Fragoso-Martínez et al., 2017). Another study sampled 35 species (13 Calosphace) for 438 loci with a final alignment of 272,874 bp or 7,796 bp per sample (Kriebel et al., 2019). The studies by Fragoso-Martínez et al. (2017) and Kriebel et al. (2019) reported higher numbers of loci and base pairs than we did, but with less than half of our sampled taxa. Our methods had a more stringent cut-off for missing sequences and yielded a more conservative alignment. The branches in our tree with low support led to taxa that were not sampled in the study of Fragoso-Martinez or Kriebel et al. (2019).
We did not attempt a direct comparison between our custom-designed baits and previous next-gen studies using bait selection in Angiosperm v.1 kit (Buddenhagen et al., 2016). These three studies had different taxon sampling and phylogeny estimation methods so, it is not clear if the differences we report on branch support derive from our baits or taxon sampling.
Chloroplast Assembly
An additional advantage of the Hyb-Seq protocol as opposed to the AHE protocol, lies in obtaining the chloroplast and mitochondrial genomes, here we explored the chloroplast loci. Chloroplasts were assembled in HPM using S. miltiorrhiza genome as a pseudoreference, obtaining a 92,461 bp assembly for the 90 Salvia samples evaluated (Supplementary Table 5). A map to reference approach was previously tested (Olvera-Mendoza et al., 2020) on 15 samples from these same data to investigate closely related species in S. sections Atratae, Mitratae, Scorodoniae, and Sigmoideae, resulting in the first chloroplast genome assemblies for S. subg. Calosphace, although limited taxon sampling for these sections impeded full resolution of the phylogeny. Our HPM chloroplast assembly using the same pseudoreference recovered fewer loci (Supplementary Table 5) than the study conducted by Olvera-Mendoza et al. (2020) did [114 genes, 80 CDS, 30 tRNA spacers, and 4rRNA's (Olvera-Mendoza et al., 2020) vs. our 75 CDS, 29 tRNA's, 5 introns and 4 rRNA]. This may be attributed to the many samples (78) we evaluated compared with their 15 samples, and the filtering step we used during HPM.
Nuclear Phylogenetic Inferences
The nuclear phylogenies (Figure 2; Supplementary Figure 1) resulting from ASTRAL and BEAST have well-resolved and highly supported clades and recover several previously reported relationships (Walker et al., 2004; Walker and Sytsma, 2007; Jenks et al., 2013; Will and Claßen-Bockhoff, 2017; Fragoso-Martínez et al., 2018). Cantinoa from tribe Ocimeae was used as the outgroup following Li et al. (2016). Cantinoa is sister to the Mentheae tribe and relationships in our trees are in agreement with the study of Drew and Sytsma (2012). We recovered subtribes Menthinae (Hedeoma and Poliomintha), Nepetinae (Agastache and Dracocephalum), Lycopinae (Lycopus), Prunellinae (Prunella), and Salviinae (Melissa, both Lepechinia and Salvia). Within Salvia, we recovered “clade I” with S. subgenera Salvia (S. officinalis) and Leonia (S. sessilifolia + S. texana), and a clade of S. subgenera Audibertia (S. sonomensis + S. brandegeei) and the 69 remaining species in Calosphace. Here we support the monophyly of eight of the 13 Salvia sections sampled (Table 2): Biflorae, Curtiflorae, Hastatae, Incarnatae, Lavanduloideae, Sigmoideae, and also S. sections Cardinales and Uricae (as in Olvera-Mendoza et al., 2020). Although our tree is well-resolved, our Calosphace sample is <15% of the estimated species diversity in the subgenus, undoubtedly having an effect on clade resolution, and unsampled species could potentially be inserted in future phylogenetic studies to further resolve fine-scale relationships with each clade.
Relationships among the section's sister to the core Calosphace have been somewhat controversial. Most studies (Walker et al., 2004; Walker and Sytsma, 2007; Jenks et al., 2013; Fragoso-Martínez et al., 2018; Kriebel et al., 2019) found S. axillaris (monotypic S. sect. Axillares) sister to the rest of the Calosphace; this relationship is only supported by our chloroplast analysis (Figure 4). Our nuclear data analyses (Figure 2; Supplementary Figure 1) support S. axillaris sister to “Hastatae clade,” and together with sister to the rest of Calosphace; this relationship has also been recovered by Hu et al. (2018) [(S. patens + Salvia cacaliifolia Benth. (S. axillaris (rest of Calosphace)] and Walker et al. (2015) [(S. patens (S. axillaris + Salvia cedrosensis Greene)] with S. axillaris in a clade with Hastatae representatives. Interestingly, these relationships are congruent with differences in stamen morphology; a key feature in Salvia (Bentham, 1832-1836; Fernald, 1900; Walker and Sytsma, 2007). Three stamen types have been described for S. subg. Calosphace; the G type in S. axillaris where both anterior and posterior anthers are expressed in free stamens, F type in the “clade Hastatae” (S. sects. Standleyana, Blakea, and Hastatae) where “both posterior thecae are aborted, and the adjacent posterior thecae are not, or only little fused” (Walker and Sytsma, 2007) and the E stamen type in the rest of the Calosphace where the posterior anthers are aborted and stamens are joined in a connective (Walker and Sytsma, 2007). The relationship we recovered suggests that elaborated connective tissue may have evolved twice in this clade (in Hastatae and Calosphace) or that the ancestor of the clade had another connective and it was lost in S. axillaris. The complex evolutionary patterns of stamen morphology are being investigated (Kriebel et al., 2020), to consider the potential usefulness of stamen characters for defining clades and within-species variation.
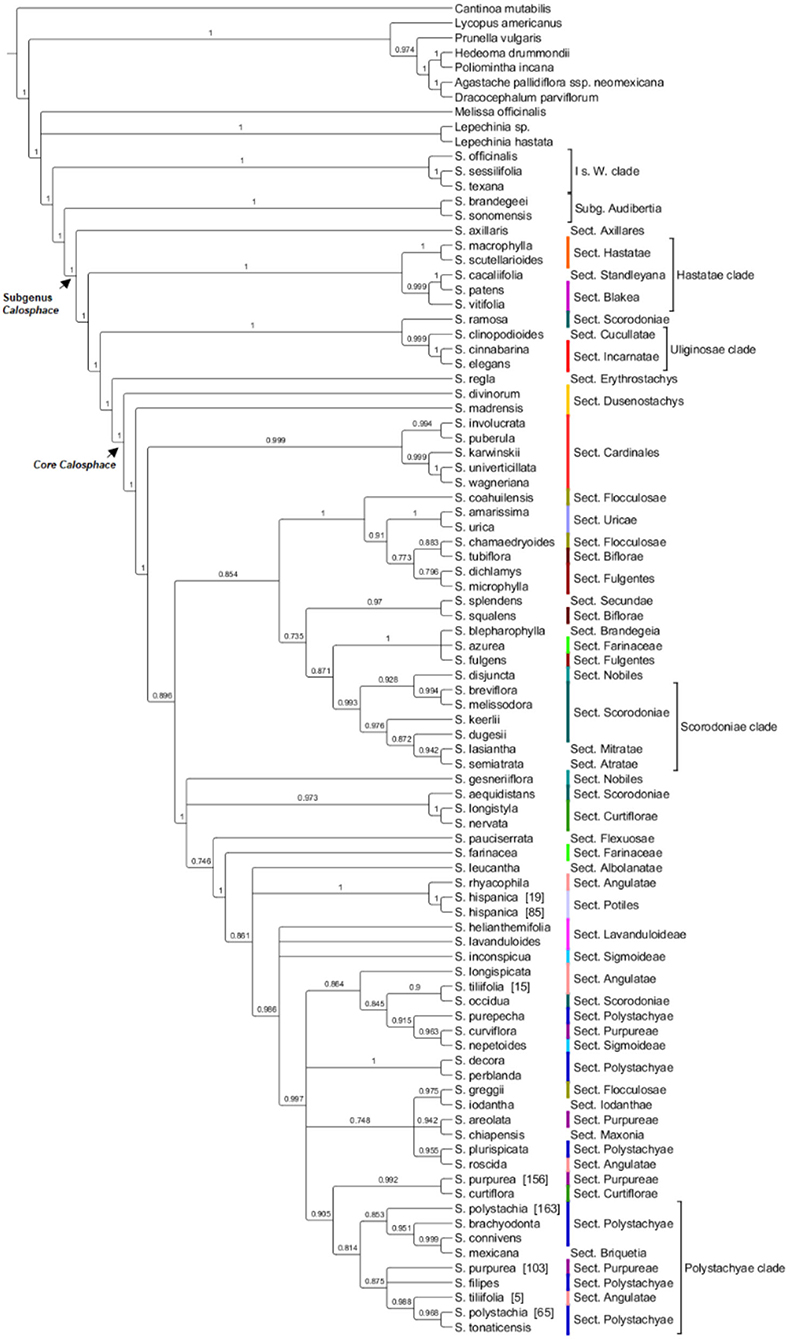
Figure 4. HPM- ML FastTree for 114 chloroplast loci, local posterior probability >0.7 is indicated above branches (lower are collapsed). Salvia subgenus Calosphace sections s. Epling are color-coded. The main clades follow previous nomenclature (Walker et al., 2004; Jenks et al., 2013; Fragoso-Martínez et al., 2018).
Previous next-gen studies of Salvia by Fragoso-Martínez et al. (2017) used the angiosperm bait kit (Johnson et al., 2019) and found three branches with low posterior probability (PP) support (their Figure 1b). Kriebel et al. (2019) on the other hand, found three poorly supported branches in the Calosphace clade in their ASTRAL coalescent analysis (Figure 2). We did not sample the taxa involved in two of those branches. Kriebel et al. (2019) additionally report an expanded taxon sampling to 266 Calosphace merging previous nuclear ribosomal DNA (ITS/ETS) sequences as supporting material for their habitat and pollinator study for Salvia.
Clade “Hastatae” was recovered in every tree (Figures 2, 4; Supplementary Figures 1–3) and includes reciprocally monophyletic S. sects. Hastatae, Blakeae, and Standleyana. This clade was also found in other studies [Jenks et al., 2013; Fragoso-Martínez et al., 2018; Kriebel et al., 2019 (nrDNA)]. Salvia sect. Standleyana was redefined by Turner (2011), merging it with species from the S. sect. Blakea s. Epling (Salvia costaricensis Oerst., S. patens, S. subpatens Epling, S. vitifolia). Later Klitgaard (2012) supported the merger of these S. sections, but under sect. Blakea. Our phylogenies found S. cacalifolia in a clade with S. patens and S. vitifolia and so support the merger of sect. Standleyana and Blakea, with the caveat, that S. costaricensis Oerst., S. subpatens, and S. serboana B. L. Turner should be sampled in a molecular study before the sections are re-classified.
Our clade “Uliginosae” (Figures 2, 4; Supplementary Figures 1–3) includes monophyletic S. sects. Incarnatae and Cucullatae, agreeing with previous clade circumscription [Jenks et al., 2013; Fragoso-Martínez et al., 2018; Kriebel et al., 2019 (nrDNA)]; unfortunately, though, our sampling in this clade is reduced, and we are lacking a representative of S. sect. Uliginosae; furthermore, our trees include S. regla and one of the seven sampled S. sect. Scorodoniae (S. ramosa) in the S. sect. Erythrostachys clade; these relationships require careful review with broader taxon sampling within the S. sect. Erytrostachys.
Following clades “Hastate” and “Uliginosae” we reach the troublesome and most species-rich clade, the “core Calosphace” (Figures 2–4; Supplementary Figures 1–3). The remainder of the sampled species is included in this clade. Walker (2006) was the first to define this clade, consisting of several clades immersed within a large polytomy and later studies with expanded sampling have confirmed this clade (Jenks et al., 2013; Fragoso-Martínez et al., 2018). We newly placed seven species in the Calosphace clade, classified in the S. sects. Angulatae (S. roscida), Cardinales (S. puberula), Fulgentes (S. dichlamys) and Polystachyae (S. brachyodonta, S. decora, S. perblanda, S. purepecha). Additionally, we found monophyletic S. sects. Cardinalis and Uricae, increasing the molecular evidence for monophyletic Calosphace sections from 12 (Fragoso-Martínez et al., 2018) to 14 among those evaluated.
A close sectional relationship has been demonstrated for Salvia sects. Scorodoniae Atratae (S. semiatrata), Mitratae (Salvia lasiantha Benth.), Sigmoideae (S. inconspicua and S. nepetoides), and Uricae (S. amarissima and S. urica) cpDNA entire genome and nuclear ribosomal cistron (Olvera-Mendoza et al., 2020). We found support for relationships among some of these sections, but together they do not form a clade; S. sect. Uricae is indeed monophyletic and distinct from the S. sect. Scorodoniae as Olvera-Mendoza et al. (2020) proposed. Salvia sect. Scorodoniae is not monophyletic although morphologically recognizable (Olvera-Mendoza et al., 2017) and S. sect. Sigmoideae is monophyletic only if nuclear data are incorporated in the analysis, either combined cpDNA + nDNA (Jenks et al., 2013; Fragoso-Martínez et al., 2018; Olvera-Mendoza et al., 2020) or only nuclear (Figure 2; Supplementary Figures 1–3A–C); highlighting the importance of nuclear markers to better-resolve Salvia species relationships. Jenks et al. (2013) and Fragoso-Martínez et al. (2018) also recovered a non-monophyletic S. sect. Scorodoniae [as did Kriebel et al., 2019 (nrDNA)] and considered S. sect. Uricae's species to be best placed within S. sect. Scorodoniae. It is clear that further analysis is required to solve species relationships within these sections, strive to fully sample S. sects Scorodoniae and Sigmoideae, coupled with a thorough morphological review.
Our topology for “Fulgentes clade” (Figure 2; Supplementary Figure 1) is similar to previous inferences but with high branch support (Jenks et al., 2013; Fragoso-Martínez et al., 2018; Kriebel et al., 2019 [nrDNA]) for the nuclear loci analyses, including members in S. sects. Fulgentes, Flocculosae, and Cardinalis (their Holwaya s. Ramamoorthy, 1984). Fragoso-Martínez et al. (2017) AHE analysis report S. fulgentes sister to the rest of core Calosphace except S. melissodora and S. mocinoi in their branch B3 (0.71 PP). Salvia sect. Cardinales is here represented by five (Salvia involucrata Cav., Salvia karwinskii Benth., S. puberula, Salvia wagneriana Pol., Salvia univerticillata Ramamoorthy ex Klitg.) of its nine species and is monophyletic and strongly supported in all nuclear (Figure 2; Supplementary Figures 1, 2A–C) and chloroplast trees (Figure 4; Supplementary Figures 3A–C). Section Cardinales is sister to a clade of S. sects. Fulgentes (Salvia fulgens Cav., S. dichlamys, Salvia microphylla Kunth) and Flocculosae (Salvia chamaedryoides Cav., Salvia coahuilensis Fernald), only our Salvia greggii A. Gray (S. sect. Flocculosae) is apart from this clade. Despite the non-monophyly of S. sects. Flocculosae and Fulgentes we agree with Jenks et al. (2013) on their morphological and phylogenetic relationships.
One of the most species-rich sections in Salvia subg. Calosphace is Angulatae (52 species) and it is also one of the most morphologically complex and has a disjunct distribution in N and S America (Epling, 1939; Walker, 2006). None of the previous studies have recovered it as monophyletic [Walker, 2006; Jenks et al., 2013; Fragoso-Martínez et al., 2018; Kriebel et al., 2019 (nrDNA)]. Here we found three species, S. roscida, S. longispicata and S. tiliifolia [5] form the broadly defined “Angulatae clade” (Figure 2; Supplementary Figure 1) and S. tiliifolia [15] is sister to S. polystachia [163] within the “Polystachyae clade.” The non-monophyly of S. tiliifolia is both troublesome and expected since Walker (2006) found a monophyletic S. tiliifolia lacking bootstrap support in his neighbor-joining tree, and S. tiliifolia is one of the most broadly distributed and morphologically complex species in subg. Calosphace. Section Angulatae is in urgent need of a thorough review, both morphologically and molecularly; to date, only 22 South American members have been studied (Fernández-Alonso, 2003; Wood, 2007) and there are ~26 North American members that remain to be sampled.
Finally, the “Polystachyae clade” (Figures 2–4; Supplementary Figures 1–3) includes members from S. sects. Angulatae (S. tiliifolia [15]), Curtiflorae (S. curtiflora), Iodanthae (S. iodantha), Maxonia (Salvia chiapensis Brandegee), Purpureae (S. curviflora, S. purpurea), and Scorodoniae (S. occidua). Three of these sections have been under study for some time since Walker (2006) first found S. iodantha, S. polystachia, and S. purpurea in a clade with only 1-2 bp difference in psbA-trnH, trnL-trnF, and ITS sequences. Later Bedolla-García (2012) expanded taxon sampling and regarded this as the “PIP clade,” due to the inclusion of members of S. sects. Purpureae from Mexico (S. areolata, S. curviflora, S. littae, S. purpurea, S. raveniana), Iodanthae (S. iodantha, considering Salvia arbuscula Fernald and Salvia townsendii Fernald as synonyms) and Polystachyae (S. brachyodonta, Salvia connivens Epling, Salvia compacta Kuntze, S. decora, S. filipes, Salvia mcvaughii Bedolla, Lara Cabrera and Zamudio, S. plurispicata, S. polystachia, Salvia tonalensis Brandegee, S. tonaticensis). Here we include nine of the sixteen species in the S. sect. Polystachyae, three species of S. sect. Purpureae and S. iodantha (sole species in S. sect. Iodanthae), and all sampled taxa of these sections, with the exception of S. connivens (S. sect. Polystachyae), are in this clade. Neither S. sects. Purpureae nor Polystachyae are monophyletic, as has been the case elsewhere [Jenks et al., 2013; Fragoso-Martínez et al., 2018; Kriebel et al., 2019 (nrDNA)]. For this troublesome, widely diverse clade we recovered only one consistent and supported sister relationship (S. decora and S. perblanda) in the nuclear trees (Figures 2, 3; Supplementary Figures 1, 2), network (Figure 3), and also in the cpDNA tree (Figure 4; Supplementary Figure 3). Otherwise, species relationships in this part of the tree have less support, with some polytomies and low to medium branch support (Figures 2, 3; Supplementary Figures 1, 2). This lack of branch support and the network results strongly suggest reticulation issues due to recent divergence, hybridization, or incomplete lineage sorting (Huang et al., 2017). Additionally, we found that S. purpurea is monophyletic in the nuclear evidence, whereas S. polystachia is not.
Aside from the main clades “Hastatae,” “Uliginosae,” “Scorodoniae,” “Fulgentes,” “Sigmoideae,” and “Polystachyae” we found other strongly supported, small clades. Salvia sect. Uricae is monophyletic and S. sects. Farinaceae, Nobiles, and Dusenostachys are non-monophyletic, as has been previously reported [Jenks et al., 2013; Fragoso-Martínez et al., 2018; Kriebel et al., 2019 (nrDNA)]. We also support the monophyly of Salvia hispanica (S. sect. Potiles), the two samples forming a clade with S. rhyacophila (S. sect. Angulatae) as did Fragoso-Martínez et al. (2018); whereas Fragoso-Martínez et al. (2017) AHE analysis found a poorly supported sister relationship between S. hispanica and S. heliamenthifolia (0.53).
Chloroplast Phylogeny
Following Doyle (2021) we opted to analyze our chloroplast data as a single hereditary unit through ML in FastTree (Figure 3). The chloroplast tree supports the outgroup relationships S. axillaris as sister to the rest of S. subg. Calosphace and sister lineages and clades “Hastatae” and “Uliginosae,” and monophyletic S. sects. Cardinales, Hastatae, Incarnatae and Uricae. Only two S. sects. are not monophyletic here as opposed to nDNA, Lavanduloides, and Sigmoideae. Our nuclear and chloroplast analyses, however, used distinct pseudo references, here, we used the distantly-related S. miltiorrhiza (Salvia subg. Sclarea sect. Drymosphace Hu et al., 2018) as the chloroplast assembly pseudoreference. Salvia miltiorrhiza is sister to clade Meriandra + Dorystaechas + Ramona (Salvia subg. Audibertia) + Lasemia (Salvia subg. Calosphace) (Will and Claßen-Bockhoff, 2017).
Our nuclear and chloroplast phylogenies are in overall agreement, for the outgroup, sister relationship of Audibertia and Calosphace and well-resolved “Hastatae,” “Uliginosae,” “Scorodoniae,” and “Polystachyae” clades. However, they disagree on the placement of S. axillaris as sister to “clade Hastatae” in nuclear trees or sister to the rest of the Calosplace in the chloroplast tree. Within the core Calosphace, particular complexity in the phylogenies and network is seen with Salvia gesneriiflora, a bird pollinated and morphologically distinct species. This species is one of the two representatives of the S. sect. Nobiles in our sampling (S. disjuncta is the other) and S. gesneriiflora placement moves between the “Sigmoideae” and “Uricae clades” in BEAST (Figure 2), between the “Fulgentes clade” and “Sigmoideae clade” in ASTRAL (Supplementary Figure 1), and between the “Scorodoniae clade” and Scorodoniae+Curtiflorae clade in the chloroplast tree (Figure 4). Furthermore, the network shows the nuclear loci for this species have characters that align it with S. coahuilensis in clade Flocculosae + Uricae + Fulgentes and also align it with the remaining core Calosphace clade (Figure 3). It is unclear why the placement of this particular species is so troublesome, no hybridization events have been reported, though frequent nectar robbing does occur (Cuevas et al., 2013), so hybridization may be a possibility worth further exploration. It is possible that we lacked sampling of phylogenetically closer relatives. Interestingly, the sectional circumscription of this species has also been controversial, Santos (1991) moved S. gesneriiflora from the S. sect. Nobiles Epling (1939) to sect. Holwayana. Testing the placement of this species would require a phylogeographic approach.
Species Monophyly
This research addressed Salvia taxon monophyly with NGS data. Within Calosphace monophyly has been an issue for S. sections sensu Epling and species, particularly in sections with disjunct distribution and widely distributed and variable species. The discordance between morphological recognition of sections s. Epling and later molecular phylogenies have also been discussed elsewhere (Jenks et al., 2013; Fragoso-Martínez et al., 2018) and has been hypothesized to be caused by morphological homoplasy due to pollinator pressure.
Species monophyly has been addressed several times in S. subg. Calosphace through traditional Sanger sequencing, mostly rejecting monophyly. For example, Walker (2006) sampled several specimens each of S. polystachia, S. purpurea, and S. tiliifolia, and only the latter was monophyletic in his neighbor-joining tree. Later Jenks et al. (2013) found S. microphylla, S. mexicana, and S. polystachia to be non-monophyletic. In our results, S. hispanica and S. purpurea are monophyletic whereas traditional Sanger (Walker, 2006) sequencing rejected S. purpurea monophyly. However, our massive alignment was not sufficient to test monophyly for S. polystachia nor S. tiliifolia. Species monophyly for these and other species will likely need a distinct approach, such as phylogeography (Cutter, 2013), to get a better grasp at the speciation processes, particularly for such morphologically complex and amply distributed species.
In this study, we provide valuable new evidence as to the utility of Hyb-Seq data for capturing 96 nuclear loci from phylogenetically distant Lamiaceae and closely related Salvia subg. Calosphace, including testing species monophyly. We also recovered the cpDNA genome with concatenated tree phylogeny in agreement with the nuclear genome with this sampling and with previous phylogenies and improved clade resolution. We found two newly supported monophyletic S. subg. Calosphace sections s. Epling and two of four species tested were monophyletic. Although this is the largest NGS study of Salvia to date, a more thorough taxon sampling is necessary to better test sectional relationships. NGS-based approaches combined with the reassessment of morphological characters are needed to re-assess sectional circumscription, study the complex species groups in subg. Calosphace, and eventually produce a new monograph. Beyond the implications for systematics, a robust phylogeny for the genus is necessary to test hypotheses about the evolution of pollinator associations and morphological adaptations to pollinators. We hope that sage researchers will use our bait design across the width of the phylogenetic spectrum as a steppingstone to build upon for future studies.
Data Availability Statement
The datasets presented in this study can be found in online repositories. The names of the repository/repositories and accession number(s) can be found below: BioSample: PRJNA748827.
Author Contributions
GG, JP, and SL-C conceived the study and data analysis. SL-C contributed to the laboratory work. CM-L and MP-G contributed to data analysis. AF, AC-J, and JM-C contributed to analysis and manuscript review. All authors contributed to manuscript writing and review, read, and approved the final manuscript.
Funding
This work was supported by the following: CONACyT graduate studies scholarship 618610 MP-G, CONACyT sabbatical scholarship 232839 to SL-C, Coordinación de la Investigación Científica (UMSNH), Project 8.16. NSF Award Number 1120080 to JP. And NSF Grant DEB-1557059 that supports CM-L post-doctoral position.
Conflict of Interest
The authors declare that the research was conducted in the absence of any commercial or financial relationships that could be construed as a potential conflict of interest.
Publisher's Note
All claims expressed in this article are solely those of the authors and do not necessarily represent those of their affiliated organizations, or those of the publisher, the editors and the reviewers. Any product that may be evaluated in this article, or claim that may be made by its manufacturer, is not guaranteed or endorsed by the publisher.
Acknowledgments
Some of the results here presented are part of the M.S. thesis of LPG under the advisory of SL-C. We appreciate the help of curators from herbaria RSA, BIGU, EBUM, ENCB, IEB, MEXU, and UAMIZ. We are thankful for the field collection assistance of Arnulfo Blanco and Botanic Garden collection to Heather Blume for facilitating collections at the Cabrillo College Environmental Horticulture Center and Botanic Gardens, Holly Forbes from Berkeley Botanic Garden (UCB), and disposition for collecting at Huntington Botanic Garden and RSA.
Supplementary Material
The Supplementary Material for this article can be found online at: https://www.frontiersin.org/articles/10.3389/fpls.2021.725900/full#supplementary-material
References
Allman, E. S., Baños, H., and Rhodes, J. A. (2019). NANUQ: a method for inferring species networks from gene trees under the coalescent model. Algorithms Mol. Biol. 14:24. doi: 10.1186/s13015-019-0159-2
Andrews, S. (2010). FastQC: A Quality Control Tool for High Throughput Sequence Data. Available online at: http://www.bioinformatics.babraham.ac.uk/projects/fastqc/ (accessed October 12, 2020).
APG (1998). An ordinal classification for the families of flowering plants. Ann. Mo. Bot. Gard. 85, 531–553. doi: 10.2307/2992015
Bedolla-García, B. Y. (2012). Filogenia de Salvia secc. Polystachyae (Lamiaceae) (Tesis de doctorado). Facultad de biología, Universidad Michoacana de San Nicolás de Hidalgo. Morelia, Michoacán, México, 1–165.
Benítez-Vieyra, S., Fornoni, J., Pérez-Alquicira, J., Boege, K., and Domínguez, C. A. (2014). The evolution of signal-reward correlations in bee-and hummingbird-pollinated species of Salvia. Proc. Biol. Sci. 281:20132934. doi: 10.1098/rspb.2013.2934
Bentham, G. (1832-1836). “Salvia,” in Labiat. Gen. J, eds G. Bentham (Ridgway and Sons Picadilli, London), p. 190–312.
Bolger, A. M., Lohse, M., and Usadel, B. (2014). Trimmomatic: a flexible trimmer for Illumina sequence data. Bioinformatics. 30, 2114–2120. doi: 10.1093/bioinformatics/btu170
Borowiec, M. L. (2016). AMAS: a fast tool for alignment manipulation and computing of summary statistics. PeerJ. 28:e1660. doi: 10.7287/peerj.preprints.1355
Bouckaert, R., Heled, J., Kühnert, D., Vaughan, T., Wu, C. H., Xie, D., et al. (2014). BEAST 2: a software platform for Bayesian evolutionary analysis. PLoS Comput. Biol. 10:e1003537. doi: 10.1371/journal.pcbi.1003537
Bouckaert, R., Vaughan, T. G., Barido-Sottani, J., Duchêne, S., Fourment, M., Gavryushkina, A., et al. (2019). BEAST 2.5: an advanced software platform for Bayesian evolutionary analysis. PLoS Comput. Biol. 15:e1006650. doi: 10.1371/journal.pcbi.1006650
Breinholt, J. W., Carey, S. B., Tiley, G. P., Davis, E. C., Endara, L., McDaniel, S. F., et al. (2021). A target enrichment probe set for resolving the flagellate land plant tree of life. APPS 9:e11406. doi: 10.1101/2020.05.29.124081
Buddenhagen, C., Lemmon, A. R., Lemmon, E. M., Bruhl, J., Cappa, J., Clement, W. L., et al. (2016). Anchored phylogenomics of angiosperms I: assessing the robustness of phylogenetic estimates. bioRxiv 1–65. doi: 10.1101/086298
Carlsen, M. M., Fér, T., Schmickl, R., Leong-Škorničková, J, Newman, M, and Kress, W. J. (2018). Resolving the rapid plant radiation of early diverging lineages in the tropical Zingiberales: pushing the limits of genomic data. Mol. Phylogenet. Evol. 128, 55–68. doi: 10.1016/j.ympev.2018.07.020
Carter, K. A., Liston, A., Bassil, N. V., Alice, L. A., Bushakra, J. M., Sutherland, B. L., et al. (2019). Target capture sequencing unravels Rubus evolution. Front. Plant Sci. 10:1615. doi: 10.3389/fpls.2019.01615
Celep, F., Atalay, Z., Dikmen, F., Dogan, M., Sytsma, K. J., and Claßen-Bockhoff, R. (2020). Pollination ecology, specialization, and genetic isolation in sympatric bee-pollinated Salvia (Lamiaceae). Int. J. Plant Sci. 181, 800–811. doi: 10.1086/710238
Chamala, S., García, N., Godden, G. T., Krishnakumar, V., Jordon-Thaden, I. E., De Smet, R., et al. (2015). MarkerMiner 1.0: a new application for phylogenetic marker development using angiosperm transcriptomes. APPS 3:1400115. doi: 10.3732/apps.1400115
Claßen-Bockhoff, R., Speck, T., Tweraser, E., Wester, P., Thimm, S., and Reith, M. (2004). The staminal lever mechanism in Salvia L. (Lamiaceae): a key innovation for adaptive radiation? Org. Divers. Evol. 4, 189–205. doi: 10.1016/j.ode.2004.01.004
Constantinides, B., and Robertson, D. L. (2017). Kindel: indel-aware consensus for nucleotide sequence alignments. JOSS 2:282. doi: 10.21105/joss.00282
Couvreur, T. L. P., Helmstetter, A. J., Koenen, E. J. M., Bethune, K., Brandão, R. D., and Little, S. A. (2019). Phylogenomics of the major tropical plant family annonaceae using targeted enrichment of nuclear genes. Front. Plant Sci. 9:1941. doi: 10.3389/fpls.2018.01941
Cuevas, G. E., Alcalá-Guerra, A., Baños-Bravo, Y., and Flores, P. A. (2013). Biología reproductiva y robo de néctar en Salvia gesneriflora (Lamiaceae) y sus consecuencias en el éxito reproductivo. Bot. Sci., 91, 357–362. doi: 10.17129/botsci.14
Cutter, A. D. (2013). Integrating phylogenetics, phylogeography and population genetics through genomes and evolutionary theory. Mol. Phyl. Evol. 69, 1172–1185. doi: 10.1016/j.ympev.2013.06.006
Darriba, D., Taboada, G. L., Doallo, R., and Posada, D. (2012). jModelTest 2: more models, new heuristics and parallel computing. Nat Methods. 9:772. doi: 10.1038/nmeth.2109
De Smet, R., Adams, K. L., Vandepoele, K., Van Montagu, M. C. E., Maere, S., and Van de Peer, Y. (2013). Convergent gene loss following gene and genome duplications creates single-copy families in flowering plants. Proc Natl Acad Sci U S A. 110, 898–903. doi: 10.1073/pnas.1300127110
Doyle, J., and Doyle,. J. (1987). A rapid DNA isolation procedure for small quantities of fresh leaf tissue. Phytochem Bull 19, 11–15.
Doyle, J. J. (2021). Defining coalescent genes: theory meets practice in organelle phylogenomics. Syst. Biol. syab053. doi: 10.1093/sysbio/syab053
Drew, B. T., González-Gallegos, J. G., Xiang, C. L., Kriebel, R., Drummond, C. P., Walker, J., et al. (2017). Salvia united: the greatest good for the greatest number. Taxon 66, 133–145. doi: 10.12705/661.7
Drew, B. T., and Sytsma, K. J. (2012). Phylogenetics, biogeography, and staminal evolution in the tribe Mentheae (Lamiaceae). Am. J. Bot. 99, 933–953. doi: 10.3732/ajb.1100549
Drummond, A. J., and Rambaut, A. (2007). BEAST: Bayesian evolutionary analysis by sampling trees. BMC Evol. Biol. 7:214. doi: 10.1186/1471-2148-7-214
Epling, C. (1939). A revision of Salvia, subgenus Calosphace. Beihefte Feddes Repertorium Specier. Novarum regni Veg. 110, 1–383.
Epling, C. (1940). Supplementary notes on American Labiatae. Bull. Torrey Bot. Club. 67, 509–534. doi: 10.2307/2480972
Epling, C. (1941). Supplementary notes on American Labiatae-II. Bull. Torrey Bot. Club. 68, 552–568. doi: 10.2307/2481456
Epling, C. (1944). Supplementary notes on American Labiatae-III. Bull. Torrey Bot. Club. 71, 484–497. doi: 10.2307/2481241
Epling, C. (1947). Supplementary notes on American Labiatae-IV. Bull. Torrey Bot. Club. 74, 512–518. doi: 10.2307/2481876
Epling, C. (1951). Supplementary notes on American Labiatae-V. Brittonia 7, 129–142. doi: 10.2307/2804702
Epling, C., and Jativa, M. C. (1963). Supplementary notes on American Labiatae-VIII. Brittonia 15, 366–376. doi: 10.2307/2805381
Epling, C., and Mathias, M. E. (1957). Supplementary notes on American Labiatae-VI. Brittonia 8, 297–313. doi: 10.2307/2804980
Fér, T., and Schmickl, R. E. (2018). Hybphylomaker: target enrichment data analysis from raw reads to species trees. Evol. Bioinform. 14, 1–9. doi: 10.1177/1176934317742613
Fernald, M. L. (1900). A synopsis of the Mexican and Central American species of Salvia. Proc. Am. Acad. Arts Sci. 35, 489–556. doi: 10.2307/25129966
Fernández-Alonso, J. L. (2003). Estudios en Labiatae de Colombia IV. Novedades en Salvia y Sinópsis de las secciones Angulatae y Purpurea. Caldasia 25, 235–281.
Fisher, A. E., Hasenstab, K. M., Bell, H. L., Blaine, E., Ingram, A. L., and Columbus, J. T. (2016). Evolutionary history of chloridoid grasses estimated from 122 nuclear loci. Mol. Phylogenet. Evol. 105, 1–14. doi: 10.1016/j.ympev.2016.08.011
Fragoso-Martínez, I., Martínez-Gordillo, M., Salazar, G. A., Sazatornil, F., Jenks, A. A., García-Peña, M. R., et al. (2018). Phylogeny of the Neotropical sages (Salvia subg. Calosphace; Lamiaceae) and insights into pollinator and area shifts. Plant. Syst. Evol. 304, 1–13. doi: 10.1007/s00606-017-1445-4
Fragoso-Martínez, I., Salazar, G. A., Martínez-Gordillo, M., Magallón, S., Sánchez-Reyes, L., Lemmon, E. M., et al. (2017). A pilot study applying the plant Anchored Hybrid Enrichment method to New World sages (Salvia subgenus Calosphace; Lamiaceae). Plant. Syst. Evol. 117, 124–134. doi: 10.1016/j.ympev.2017.02.006
Frodin, D. G. (2004). History and concepts of big plant genera. Taxon 53, 753–776. doi: 10.2307/4135449
Ge, X., Chen, H., Wang, H., Shi, A., and Liu, K. (2014). De novo assembly and annotation of Salvia splendens transcriptome using the illumina platform. PLoS ONE 9:e0087693. doi: 10.1371/journal.pone.0087693
González-Gallegos, J. G., Bedolla-García, B. Y., Cornejo-Tenorio, G., Fernández-Alonso, J. L., Fragoso-Martínez, I., García-Peña, M. R. et al. (in press). Richness distribution of Salvia subgenus Calosphace (Lamiaceae). Int. J. Plant Sci.. 181:831–856. doi: 10.1086/709133.
Harley, R. M., Atkins, S., Budantsev, A. L., Cantino, P. D., Conn, B. J., Grayer, R., et al. (2004). “Labiatae,” in Labiatae. The Families and Genera of Vascular Plants VII. Flowering Plants Dicotyledons: Lamiales (Except Acanthaceae Including Avicenniaceae), eds. K. Kubitzkiy, J. W. Kadereit. (Berlin: Springer). p. 167–4275.
Herrando-Moraira, S and The Cardueae Radiations Group (2018). Exploring data processing strategies in NGS target enrichment to disentangle radiations in the tribe Cardueae (Compositae). Mol. Phylogenet. Evol. 128, 69–87. doi: 10.1016/j.ympev.2018.07.012
Herrando-Moraira, S and The Cardueae Radiations Group (2019). Nuclear and plastid DNA phylogeny of tribe Cardueae (Compositae) with Hyb-Seq data: a new subtribal classification and a temporal diversification framework. Mol. Phylogenet. Evol. 137, 313–332. doi: 10.1016/j.ympev.2019.05.001
Hu, G. X., Takano, A., Drew, B. T., Liu, E.-D., Soltis, D. E., Soltis, P. S., et al. (2018). Phylogeny and staminal evolution of Salvia (Lamiaceae, Nepetoideae) in East Asia. Ann. Bot. 122, 649–668. doi: 10.1093/aob/mcy104
Huang, H., Sukumaran, J., Smith, S. A., and Knowles, L. L. (2017). Cause of gene tree discord? Distinguishing incomplete lineage sorting and lateral gene transfer in phylogenetics. PeerJ. e3489v1. doi: 10.7287/peerj.preprints.3489
Huson, D. H., and Bryant, D. (2006). Application of phylogenetic networks in evolutionary studies. Mol. Biol. Evol. 23, 254–267. doi: 10.1093/molbev/msj030
Jenks, A. A., Walker, J. B., and Kim, S. C. (2013). Phylogeny of new world Salvia subgenus Calosphace (Lamiaceae) based on cpDNA (psbA-trnH) and nrDNA (ITS) sequence data. J. Plant Res. 126, 483–496. doi: 10.1007/s10265-012-0543-1
Johnson, M. G., Pokorny, L., Dodsworth, S., Botigué, L. R., Cowan, R. S., Devault, A., et al. (2019). A universal probe set for targeted sequencing of 353 nuclear genes from any flowering plant designed using k-medoids clustering. Syst. Biol. 68, 594–606. doi: 10.1093/sysbio/syy086
Jones, K. E., Fér, T., Schmickl, R. E., Dikow, R. B., Funk, V. A., Herrando-Moraira, S., et al. (2019). An empirical assessment of a single family-wide hybrid capture locus set at multiple evolutionary timescales in Asteraceae. Appl. Plant Sci. 7:e11295. doi: 10.1002/aps3.11295
Katoh, K., Misawa, K., Kuma, K., and Miyata, T. (2002). MAFFT: a novel method for rapid multiple sequence alignment based on fast Fourier transform. Nucleic Acids Res. 15:3059-66. doi: 10.1093/nar/gkf436
Katoh, K., and Standley, D. M. (2013). MAFFT multiple sequence alignment software version 7: improvements in performance and usability. Mol. Biol. Evol. 30, 772–780. doi: 10.1093/molbev/mst010
Kent, W. J. (2002). BLAT - The BLAST-like alignment tool. Genome Res. 12, 656–664. doi: 10.1101/gr.229202
Klitgaard, B. (2012). “Salvia L,” in: Flora Mesoamericana, eds. G. Davidse, S. M. Sousa, S. Knapp, F. Chiang (Rubiaceae a Verbenaceae. Missouri Botanical Press., St. Louis), p. 396–424.
Kriebel, R., Drew, B. T., Drummond, C. P., González-Gallegos, J. G., Celep, F., Mahdjoub, M. M., et al. (2019). Tracking temporal shifts in area, biomes, and pollinators in the radiation of Salvia (sages) across continents: leveraging anchored hybrid enrichment and targeted sequence data. Am. J. Bot. 106, 573–597. doi: 10.1002/ajb2.1268
Kriebel, R., Drew, B. T., González-Gallegos, J. G., Celep, F., Heeg, L., Mahdjoub, M. M., et al. (2020). Pollinator shifts, contingent evolution, and evolutionary constraint drive floral disparity in Salvia (Lamiaceae): evidence from morphometrics and phylogenetic comparative methods. Evolution 74, 1335–1355. doi: 10.1111/evo.14030
Lara-Cabrera, S. I. Porter, J. M., and Steinmann, V. W. (in press). The Freedom of Salvia s.l. and Resurrection of Lasemia and Ramona. ALISO.
Larridon, I., Villaverde, T., Zuntini, A. R., Pokorny, L., Brewer, G. E., Epitawalage, N., et al. (2020). Tackling rapid radiations with targeted sequencing. Front. Plant Sci. 10:e01655. doi: 10.3389/fpls.2019.01655
Li, B., Cantino, P. D., Olmstead, R. G., Bramley, G. L. C., Xiang, C.-L., Ma, Z.-H., et al. (2016). A large-scale chloroplast phylogeny of the Lamiaceae sheds new light on its subfamilial classification. Sci. Rep. 6, 1–18. doi: 10.1038/srep34343
Li, H., and Durbin, R. (2009). Fast and accurate short read alignment with Burrows-Wheeler Transform. Bioinformatics 25, 1754–1760. doi: 10.1093/bioinformatics/btp324
Lopresti, A. L. (2017). Salvia (Sage): a review of its potential cognitive-enhancing and protective effects. Drugs R D 17, 53–64. doi: 10.1007/s40268-016-0157-5
Mandel, J. R., Barker, M. S., Bayer, R. J., Dikow, R. B., Gao, T.-G., Jones, K. E., et al. (2017). The compositae tree of life in the age of phylogenomics. J. Syst. Evol. 55, 405–410. doi: 10.1111/jse.12265
Martin, M. (2011). Cutadapt removes adapter sequences from high-throughput sequencing reads. EMBnet J. 17:10. doi: 10.14806/ej.17.1.200
Martínez-Gordillo, M. J., Bedolla-García, B., Cornejo-Tenorio, G., Fragoso-Martínez, I., García-Peña, M., del, R., et al. (2017). Lamiaceae de México. Bot. Sci. 95:780–806. doi: 10.17129/botsci.1871
Olvera-Mendoza, E. I., Bedolla-García, B. Y., and Lara-Cabrera, S. I. (2017). Revisión taxonómica de Salvia subgénero Calosphace sección Scorodoniae (Lamiaceae), endémica para México. Acta Bot. Mex. 118, 7–39. doi: 10.21829/abm118.2017.1198
Olvera-Mendoza, E. I., Godden, G. T., Montero-Castro, J. C., Porter, J. M., and Lara-Cabrera, S. I. (2020). Chloroplast and nuclear ribosomal cistron phylogenomics in a group of closely related sections in Salvia subg. Calosphace. Braz. J. Bot. 43:177–191. doi: 10.1007/s40415-019-00572-9
One Thousand Plant Transcriptomes Intitiative (2019). One thousand plant transcriptomes and the phylogenomics of green plants. Nature 574, 679–685. doi: 10.1038/s41586-019-1693-2
Price, M. N., Dehal, P. S., and Arkin, A. P. (2010). FastTree 2 - approximately maximum-likelihood trees for large alignments. PLoS ONE 5:e9490. doi: 10.1371/journal.pone.0009490
Qian, J., Song, J., Gao, H., Zhu, Y., Xu, J., Pang, X., et al. (2013). The complete chloroplast genome sequence of the medicinal plant Salvia miltiorrhiza. PLoS ONE 8:e57607. doi: 10.1371/journal.pone.0057607
R Core Team (2017). R: A Language and Environment for Statistical Computing (R Foundation for Statistical Computing. Vienna. Available online at: https://www.r-project.org/
Ramamoorthy, T. P. (1984). Notes on Salvia (Labiatae) in Mexico, with three new species. J. Arnold. Arbor. 65, 135–143.
Ramamoorthy, T. P., and Elliott, M. (1998). “Lamiaceae de México: diversidad, distribución, endemismo y evolución,” in: Diversidad biológica de México: orígenes y distribución, eds T. P. Ramamoorthy, R. Bye, A. Lot, and J. Fa. (México; Instituto de Biología, UNAM), p. 501–525.
Rhodes, J. A., Baños, H., Mitchell, J. D., and Allman, E. S. (2021). MSCquartets 1.0: quartet methods for species trees and networks under the multispecies coalescent model in R. Bioinformatics. 37:1766–1768. doi: 10.1101/2020.05.01.073361
Sanger, F., Nicklen, S., and Coulson, A. R. (1977). DNA sequencing with chain-terminating inhibitors. Proc Natl Acad Sci USA. 74, 5463–5467. doi: 10.1073/pnas.74.12.5463
Santos, E. P. (1991). Genre Salvia L. Sous-genre calosphace (Benth.) Benth. Section nobiles (Benth.) Epl. (Labiatae). Bradea 4, 436–454.
Straub, S. C. K., Fishbein, M., Livshultz, T., Foster, Z., Parks, M., Weitemier, K., et al. (2011). Building a model: developing genomic resources for common milkweed (Asclepias syriaca) with low coverage genome sequencing. BMC Genom. 12:211. doi: 10.1186/1471-2164-12-211
Straub, S. C. K., Parks, M., Weitemier, K., Fishbein, M., Cronn, R. C., and Liston, A. (2012). Navigating the tip of the genomic iceberg: next-generation sequencing for plant systematics. Am. J. Bot. 99, 349–364. doi: 10.3732/ajb.1100335
Torke, B. M. (2000). A revision of Salvia Sect. Ekmania (Lamiaceae). Brittonia 52, 265–302. doi: 10.2307/2666577
Turner, B. L. (2011). Recension of Mexican species of Salvia Sect. Standleyana (Lamiaceae). Phytoneuron 23, 1–6.
Villaverde, T., Jiménez-Mejías, P., Luceño, M., Waterway, M. J., Kim, S., Lee, B., et al. (2020). A new classification of Carex (Cyperaceae) subgenera supported by a HybSeq backbone phylogenetic tree. Bot. J. Linn. Soc. 194, 141–163. doi: 10.1093/botlinnean/boaa042
Villaverde, T., Pokorny, L., Olsson, S., Rincón-Barrado, M., Johnson, M. G., Gardner, E. M., et al. (2018). Bridging the micro- and macroevolutionary levels in phylogenomics: Hyb-Seq solves relationships from populations to species and above. New Phytol. 220, 636–650. doi: 10.1111/nph.15312
Walker, J. B. (2006). A preliminary molecular phylogenetic analysis of Salvia subgenus Calosphace, Chap. 3 (PhD thesis). University of Wisconsin, Madison.
Walker, J. B., Drew, B. T., and Sytsma, K. J. (2015). Unravelling species relationships and diversification within the iconic california floristic province sages Salvia subgenus Audibertia, Lamiaceae). Syst. Bot. 40, 826–844. doi: 10.1600/036364415X689285
Walker, J. B., and Sytsma, K. J. (2007). Staminal evolution in the genus Salvia (Lamiaceae): molecular phylogenetic evidence for multiple origins of the staminal lever. Ann. Bot. 100, 375–391. doi: 10.1093/aob/mcl176
Walker, J. B., Sytsma, K. J., Treutlein, J., and Wink, M. (2004). Salvia (Lamiaceae) is not monophyletic: implications for the systematics, radiation, and ecological specializations of Salvia and tribe Mentheae. Am. J. Bot. 91, 1115–1125. doi: 10.3732/ajb.91.7.1115
Wanke, S., Granados Mendoza, C., Müller, S., Paizanni Guillén, A., Neinhuis, C., Lemmon, A. R., et al. (2017). Recalcitrant deep and shallow nodes in Aristolochia (Aristolochiaceae) illuminated using anchored hybrid enrichment. Mol. Phylogenet. Evol. 117, 111–123. doi: 10.1016/j.ympev.2017.05.014
Weitemier, K., Straub, S. C., Cronn, R. C., Fishbein, M., Schmickl, R., McDonnell, A., et al. (2014). Hyb-Seq: combining target enrichment and genome skimming for plant phylogenomics. App.l Plant Sci. 2:1400042. doi: 10.3732/apps.1400042
Wells, T., Maurin, O., Dodsworth, S., Friis, I., Cowan, R., Epitawalage, N., et al. (2020). Combination of Sanger and target-enrichment markers supports revised generic delimitation in the problematic ‘Urera clade’ of the nettle family (Urticaceae). Mol. Phylogenet. Evol. 158:107008. doi: 10.1016/j.ympev.2020.107008
Wester, P., and Claßen-Bockhoff, R. (2011). Pollination syndromes of new world Salvia species with special reference to bird pollination. Ann. Missouri Bot. Gard. 98, 101–155. doi: 10.3417/2007035
Will, M., and Claßen-Bockhoff, R. (2017). Time to split Salvia s.l. (Lamiaceae) - new insights from old world Salvia phylogeny. Mol. Phylogenet. Evol. 109, 33–58. doi: 10.1016/j.ympev.2016.12.041
Wu, Y. B., Ni, Z. Y., Shi, Q. W., Dong, M., Kiyota, H., and Gu, et al. (2012). Constituents from Salvia species and their biological activities. Chem. Rev. 14, 5967–6026. doi: 10.1021/cr200058f
Xu, H., Luo, X., Qian, J., Pang, X., Song, J., Qian, G., et al. (2012). FastUniq: a fast de novo duplicates removal tool for paired short reads. PLoS ONE 7:e52249. doi: 10.1371/journal.pone.0052249
Zeng, L., Zhang, N., Zhang, Q., Endress, P. K., Huang, J., and Ma, H. (2017). Resolution of deep eudicot phylogeny and their temporal diversification using nuclear genes from transcriptomic and genomic datasets. New Phytol. 214, 1338–1354. doi: 10.1111/nph.14503
Keywords: Hyb-Seq, chloroplast, section, nuclear, monophyly
Citation: Lara-Cabrera SI, Perez-Garcia MdlL, Maya-Lastra CA, Montero-Castro JC, Godden GT, Cibrian-Jaramillo A, Fisher AE and Porter JM (2021) Phylogenomics of Salvia L. subgenus Calosphace (Lamiaceae). Front. Plant Sci. 12:725900. doi: 10.3389/fpls.2021.725900
Received: 16 June 2021; Accepted: 07 September 2021;
Published: 15 October 2021.
Edited by:
Stefan Wanke, Technische Universität Dresden, GermanyReviewed by:
Aaron Liston, Oregon State University, United StatesRoswitha Schmickl, Academy of Sciences of the Czech Republic (ASCR), Czechia
Copyright © 2021 Lara-Cabrera, Perez-Garcia, Maya-Lastra, Montero-Castro, Godden, Cibrian-Jaramillo, Fisher and Porter. This is an open-access article distributed under the terms of the Creative Commons Attribution License (CC BY). The use, distribution or reproduction in other forums is permitted, provided the original author(s) and the copyright owner(s) are credited and that the original publication in this journal is cited, in accordance with accepted academic practice. No use, distribution or reproduction is permitted which does not comply with these terms.
*Correspondence: Sabina Irene Lara-Cabrera, c2FiaW5hLmxhcmFAdW1pY2gubXg=; c2xhcmFjYWJyZXJhQGdtYWlsLmNvbQ==
†ORCID: Sabina Irene Lara-Cabrera orcid.org/0000-0001-8551-9829
Maria de la Luz Perez-Garcia orcid.org/0000-0001-5272-5052
Carlos Alonso Maya-Lastra orcid.org/0000-0002-0550-3331
Juan Carlos Montero Castro orcid.org/0000-0002-3098-14150
Angélica Cibrián-Jaramillo orcid.org/000-002-7974-455X
Amanda E. Fisher orcid.org/0000-0002-9928-9558