- 1National Engineering Laboratory of Wheat and Maize, Key Laboratory of Biology and Genetic Improvement of Maize in Northern Yellow-Huai Rivers Plain, Ministry of Agriculture, Maize Research Institute, Shandong Academy of Agricultural Sciences, Jinan, China
- 2College of Resources and Environment, Qingdao Agricultural University, Qingdao, China
- 3College of Resources and Environment, China Agricultural University, Beijing, China
- 4Linyi Academy of Agricultural Sciences, Linyi, China
Previous studies have shown that zinc (Zn) accumulation in shoot and grain increased as applied nitrogen (N) rate increased only when Zn supply was not limiting, suggesting a synergistic effect of N on plant Zn accumulation. However, little information is available about the effects of different mineral N sources combined with the presence or absence of Zn on the growth of both shoot and root and nutrient uptake. Maize plants were grown under sand-cultured conditions at three N forms as follows: NO3– nutrition alone, mixture of NO3–/NH4+ with molar ratio of 1:1 (recorded as mixed-N), and NH4+ nutrition alone including zero N supply as the control. These treatments were applied together without or with Zn supply. Results showed that N forms, Zn supply, and their interactions exerted a significant effect on the growth of maize seedlings. Under Zn-sufficient conditions, the dry weight (DW) of shoot, root, and whole plant tended to increase in the order of NH4+ < NO3– < mixed-N nutrition. Compared with NH4+ nutrition alone, mixed-N supply resulted in a 27.4 and 28.1% increase in leaf photosynthetic rate and stomatal conductance, which further resulted in 35.7 and 33.5% of increase in shoot carbon (C) accumulation and shoot DW, respectively. Furthermore, mixed-N supply resulted in a 19.7% of higher shoot C/N ratio vs. NH4+ nutrition alone, which means a higher shoot biomass accumulation, because of a significant positive correlation between shoot C/N ratio and shoot DW (R2 = 0.682***). Additionally, mixed-N supply promoted the greatest root DW, total root length, and total root surface area and synchronously improved the root absorption capacity of N, iron, copper, manganese, magnesium, and calcium. However, the above nutrient uptake and the growth of maize seedlings supplied with NH4+ were superior to either NO3– or mixed-N nutrition under Zn-deficient conditions. These results suggested that combined applications of mixed-N nutrition and Zn fertilizer can maximize plant growth. This information may be useful for enabling integrated N management of Zn-deficient and Zn-sufficient soils and increasing plant and grain production in the future.
Introduction
Nitrogen (N) is the most abundant mineral nutrient element in plants and plays an essential role in plant growth and development. Nitrate (NO3–-N) and ammonium (NH4+-N) are predominant forms of inorganic N in soil, both of which can be absorbed by plants directly (Marschner, 2011). The preferred N form varies among plant species and among genotypes of individual plant species, environmental conditions, and developmental stages (Cramer and Lewis, 1993; Zhao et al., 2013). Maize usually grows in well-aerated dryland soils, where NO3– is the major source of N due to soil nitrification (Fang et al., 2006). Some studies showed the enhanced growth of maize under NO3– nutrition compared with NH4+ nutrition (Cramer and Lewis, 1993; Schortemeyer et al., 1997). Others showed that NH4+ uptake may be an important strategy for maize plants to take up sufficient quantities of N for growth compared with NO3– nutrition (Gu et al., 2013; George et al., 2016; Wang et al., 2019; Zhang H. Q. et al., 2019). For most plants, a mixture of both NO3– and NH4+ is superior to either NO3– or NH4+ sources alone, possibly due to improved cytokinin transportation from root to shoot, stimulated leaf growth, maintained rhizosphere pH, and increased nutrient uptake rates, such as N, phosphorus (P), copper (Cu), and iron (Fe) (Gentry and Below, 1993; Marschner, 2011; Cao et al., 2018; Wang et al., 2019).
Zinc (Zn) is an essential microelement for plant growth. Zinc plays a vital role in many essential cellular functions and metabolic pathways and is important to the normal health and reproduction of plants, animals, and humans (Welch and Graham, 2004). It is estimated that more than 30% of the agricultural soils in the world are prone to Zn deficiency (Alloway, 2008). According to a national soil survey, China has nearly 0.63 billion hm2 of soil, accounting for 45.7% of the farmland, is subject to Zn deficiency, primarily calcareous soil in Northern China (Liu, 1996). Although Zn is an essential micronutrient for plant growth, Zn input has received much less attention than N, P, or irrigation during the Green Revolution (Tilman et al., 2002; Mueller et al., 2012). Therefore, the application of Zn fertilizers is necessary in such soils to ensure cereal yield and grain Zn concentration (Cakmak, 2008).
Maize is one of the world’s major crops and is expected to contribute increasingly to human and animal nutrition and to energy production. Maize is sensitive to Zn deficiency (Wang and Jin, 2007). Zn-deficient symptoms, such as stunted and chlorotic plants, are often observed in maize plants growing on calcareous soil in the field (Alloway, 2008). Many studies have demonstrated that grain yield of maize was increased by the application of Zn fertilizer to Zn-deficient soils. The positive effects of Zn supply are related to the increased photosynthesis rates, chlorophyll content in leaves, and increased kernel number and weight in the apical benefited from increased pollen viability at the tasseling stage under field conditions (Potarzycki, 2010; Wang et al., 2012; Liu et al., 2016, 2020).
Previous results from greenhouse conditions showed that increasing N supply did not improve Zn accumulation in grain and shoot of wheat in case of low soil Zn availability. Otherwise, increasing N supply substantially improved Zn concentration and accumulation of both grain and shoot in wheat when Zn supply was not limited, showing a synergistic effect of N fertilization on Zn accumulation (Kutman et al., 2010, 2011a,b). Furthermore, our results obtained from field conditions also showed positive effects of N application on the root uptake, root-to-shoot translocation, and remobilization of Zn in wheat and maize grown in Zn-sufficient soils (Xue et al., 2012, 2014, 2019). However, little information is available about the effects of different mineral N sources combined with the presence or absence of Zn on the growth of both shoot and root and nutrient uptake.
This study aimed to determine how the different N forms affected the growth of shoot and root, root morphological traits, and nutrient uptake of maize seedlings grown with and without Zn supply under greenhouse conditions. This information may be useful for enabling integrated N management of Zn-deficient and Zn-sufficient soils and increasing plant and grain production in the future.
Materials and Methods
Experimental Procedures
Seeds of hybrid “Denghai 605” were surface-sterilized by soaking in 10% (v/v) H2O2 for 30 min, rinsed thoroughly in deionized water, soaked in saturated CaSO4 for 5 h, then transferred to germinate on filter paper in the dark at 25°C for 2 days. Initially, five uniformly germinated seeds were sown in a plastic pot filled with 5 kg of quartz sand (diameter between 0.25 and 0.50 mm) and later thinned to three plants per pot. Quartz sand was pretreated by soaking in acid (5 N HCl) for 18 h and was thoroughly washed with distilled water until pH was neutral. Each treatment had seven replicates arranged in a completely randomized design. Their places were changed frequently inside a glasshouse with day/night temperature of 25/18°C. Plants were supplied with nutrient solution after germination according to Yuan et al. (2011). The nutrient solution contained 0.75 mmol L–1 K2SO4, 0.65 mmol L–1 MgSO4⋅7H2O, 0.25 mmol L–1 KH2PO4, 10 μmol L–1 H3BO3, 1 μmol L–1 MnSO4⋅H2O, 0.5 μmol L–1 CuSO4⋅5H2O, 0.05 μmol L–1 Na2Mo7O4⋅2H2O, and 0.1 mmol L–1 Fe-EDTA. Nitrogen was supplied in 4 mmol L–1 with three different N forms as follows: NO3– nutrition alone, mixture of NO3–/NH4+ with molar ratio of 1:1 (recorded as mixed-N), and NH4+ nutrition alone including zero N supply as the control (recorded as N0). These treatments were applied together without (Zn0), or with 2 μmol L–1 ZnSO4⋅7H2O (Zn1). NH4+-N and NO3–-N were applied in the form of (NH4)2SO4 and Ca(NO3)2⋅4H2O, respectively. Furthermore, CaCl2⋅2H2O was added to balance calcium in the solution that contained ammonium and the control treatments as well. The solution pH was adjusted to 6.0. The pots were watered every 2 days using 400 ml of treated nutrient solution.
Photosynthetic Parameter Measurements
Photosynthetic parameters including net photosynthetic rate (Pn), transpiration rate (Tr), stomatal conductance (gs), and intercellular CO2 concentration (Ci) were measured using a portable Li-6400 photosynthesis system (LI-COR Inc., Lincoln, NE, United States). The youngest fully expanded leaves at 30 days after N and Zn treatments were selected for measurement between 09:00 and 11:00. The photosynthetically active radiation, temperature, and CO2 concentration during measurement collection were set at 600 μmol m–2 s–1, 25°C, and 380 μmol mol–1, respectively. The index of chlorophyll content (SPAD value) in the youngest fully expanded leaves was determined using portable chlorophyll meter SPAD-502 Plus (SPAD, Minolta Camera Co., Ltd., Osaka, Japan). There were four biological replicates for each treatment.
Mineral Elements
After photosynthetic parameter measurements, plants were harvested for 30 days after N and Zn treatments. The fresh plant samples were divided into shoots and roots. The roots were washed in 0.5 mM CaSO4 solution for 10 min, and shoots were then rinsed with ultra-pure water and dried at 65°C until constant weight. The dry weight (DW) of shoot and root was used to calculate Zn efficiency (ZE) (the ratio of DW of shoot or root without Zn supply to that with Zn supply ×100), which has been used for screening various genotypes of cereals and other crops (Karim et al., 2012). The dried samples were ground with a stainless steel grinder and then digested using an acid mixture of HNO3–H2O2 in a microwave accelerated reaction system (CEM Corp., Matthews, NC, United States). The concentrations of Zn, Fe, manganese (Mn), Cu, calcium (Ca), magnesium (Mg), P, and K (potassium) in the digested solutions were determined by inductively coupled plasma atomic emission spectroscopy (ICP-AES, Optima 7300 DV, PerkinElmer, Waltham, MA, United States). Two blanks and a certified reference material (IPE126, Wageningen University, Netherlands) were included in each batch of digestion to ensure analytical quality. The concentrations of carbon (C) and N in samples were determined using a CN analyzer (vario Macro cube, Elementar, Hanau, Germany). There were four biological replicates for each treatment.
Leaf Soluble Protein Content
About 30 days after N and Zn treatments, the youngest fully expanded leaves from another batch of samples with three biological replicates for each treatment were frozen in liquid N2 and crushed with a tissue homogenizer. The crushed samples were dissolved in pre-cooled phosphate buffer with pH 7.8 and made up to a final volume of 1,000 ml. The concentration of protein extracts was determined by a colorimetric method as described by Bradford (1976) using protein assay dye Coomassie Brilliant Blue G-250 (Amresco, Athens, GA, United States). The absorbance was determined at 595 nm.
Root Morphological Parameters
After the youngest fully expanded leaves were removed for determination of soluble protein, root samples were washed with tap water and kept at −20°C before an image was captured using an optical scanner (Epson, Nagano, Japan). The root traits were determined by analysis of images using the WinRHIZO software (Regent Instrument, Quebec, QC, Canada). There were three biological replicates for each treatment. After completing the root scan, these roots were not used for nutrient analysis due to the possibility of nutritional loss during the scanning process.
Statistical Analysis
ANOVA was performed using the Data Processing System (DPS) version 9.50 statistical software (Tang and Zhang, 2013). Means were compared using Fisher’s protected least significant difference (LSD) tests. Differences were considered significant at p < 0.05. Linear regression analysis was employed to identify the correlation between shoot C/N ratio and shoot DW using the SAS software (SAS 8.0, SAS Institute Inc., Cary, NC, United States).
Results
Dry Weight of Shoot and Root and the Ratio of Root-to-Shoot
Our data revealed significant effects of the Zn and N treatments on the DW of the shoot, root, and whole plants and the root-to shoot ratios (R/S) of 30-day-old maize plants grown under greenhouse conditions (Table 1). The interactions between Zn and N treatments were also significant for the DW of the shoot, root, and whole plants. Under a Zn-deficient condition, plants grown with NH4+ nutrition produced significantly greater shoot DW than with mixed N nutrition, whereas NO3– produced an intermediate shoot DW (Table 1). Plants grown with NH4+ also produced slightly higher root DW (approximately 27%) but not significantly different from NO3– and mixed N supply. However, under a Zn-sufficient condition, plants grown with NO3– supply and mixed-N supply produced significantly greater shoot DW than with NH4+ supply (Table 1). Plants grown with mixed-N nutrition produced significantly higher root DW than with either N form alone. Compared with nil N supply, N supply resulted in a 2.6- to 3.7-fold increase in shoot DW and 0.2- to 0.6-fold increase in root DW under Zn-deficient conditions. Under Zn-sufficient conditions, N supply resulted in a 3.9- to 4.8-fold increase in shoot DW and 0.5- to 1.2-fold increase in root DW. Consequently, although the R/S was not significantly affected by the three N forms, N supply vs. nil N supply remarkably reduced the R/S from 81.9 to 27.2% and from 71.1 to 22.4% in response to Zn-deficient and Zn-sufficient conditions.
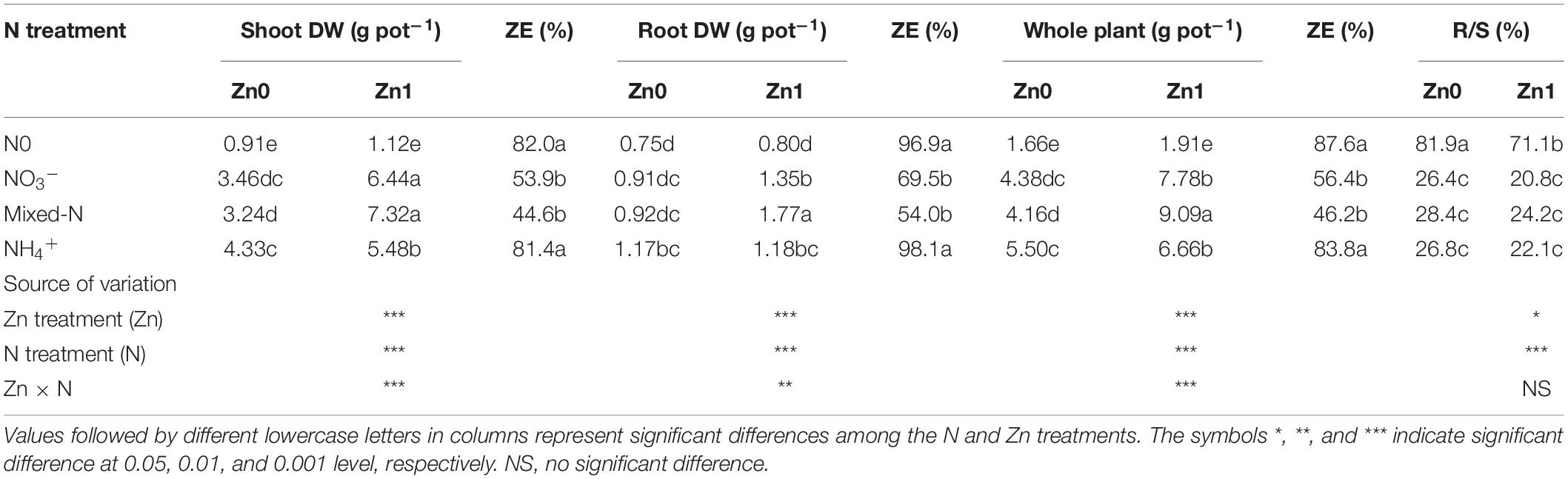
Table 1. Dry weight (DW) of shoot, root, and whole plant, Zn efficiency (ZE), and root-to-shoot ratio (R/S) of 30-day-old maize seedlings cultured with nil N (N0), NO3–, mixed-N, and NH4+ nutrition under sand culture conditions without (Zn0) and with Zn (Zn1) supply.
Compared with no Zn supply, Zn supply resulted in 22.4–125.9, 0.5–91.8, and 15.3–118.4% greater DW of the shoot, root, and whole plant, respectively, with the increase being the highest with mixed-N nutrition and following by NO3– supply. However, ZE was significantly higher with NH4+ nutrition compared with NO3– and mixed-N supply, showing a greater ability to resist Zn deficiency (Table 1).
SPAD Readings and Soluble Protein Contents of Newly Developed Leaf
Two-way ANOVA revealed significant effects of the N treatments on the leaf SPAD values and soluble protein content, and significant effects of the Zn supply only on leaf SPAD values. The interaction between N treatments and Zn supply was also significant for leaf soluble protein content (Supplementary Table 1).
Compared with no N supply, the three N forms significantly increased the leaf SPAD readings and leaf soluble protein contents (Figures 1A,B). There were no significant differences in the leaf SPAD readings among the three N forms, regardless of Zn supply (Figure 1A). The leaf soluble protein contents increased gradually in the order of NH4+ < mixed-N < NO3– nutrition in the presence of Zn supply. However, these values were not significantly affected by the different N forms under Zn-deficient conditions (Figure 1B).
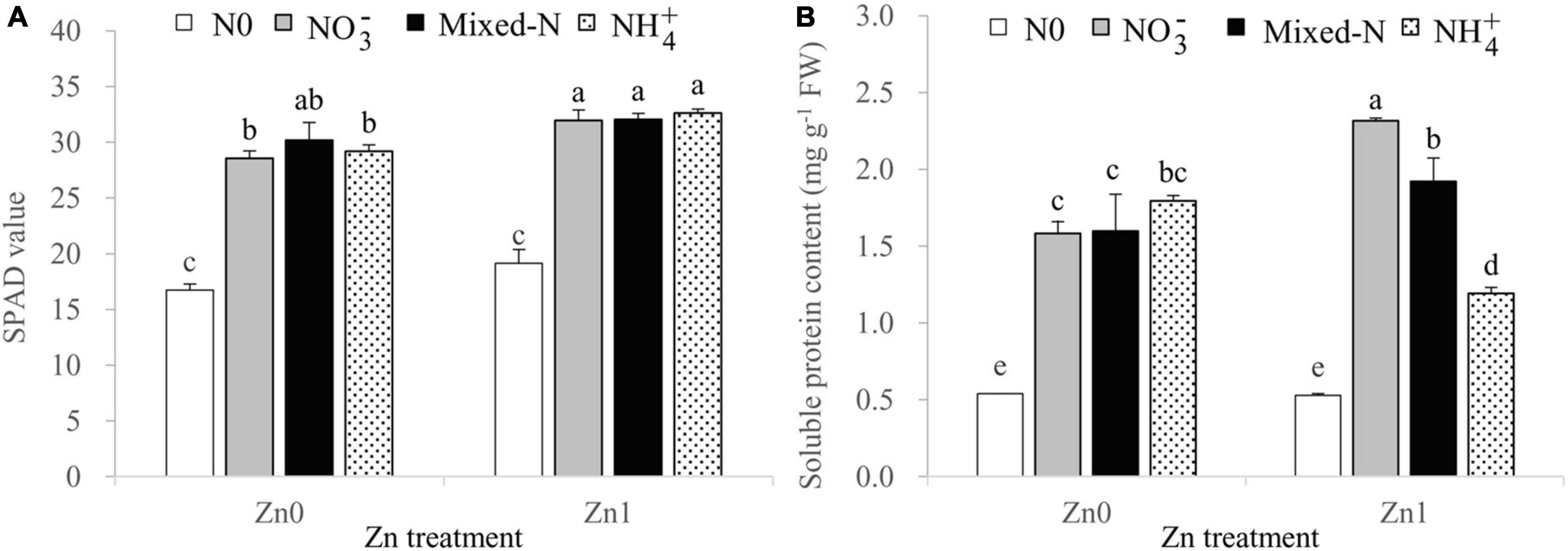
Figure 1. SPAD readings (A) and soluble protein contents (B) of newly developed leaf of 30-day-old maize seedlings cultured with nil N (N0), NO3–, mixed-N, and NH4+ nutrition under sand culture conditions without (Zn0) and with Zn (Zn1) supply. Error bars represent the standard error of the mean (n = 4). Significant differences at p < 0.05 are shown with different letters.
Leaf Photosynthetic Parameters
Two-way ANOVA revealed significant effects of the N treatments on the leaf Pn, gs, and Tr and significant effects of the Zn supply on Pn and Ci (Supplementary Table 1). The interaction between N treatments and Zn supply was significant only for leaf Ci (Supplementary Table 1). Compared with nil N supply, three N forms significantly increased the leaf Pn, gs, and Tr, irrespective of Zn supply (Figures 2A,C,D). Plants grown with mixed-N nutrition had significantly higher leaf Pn, gs, and Tr than those supplied with either N form alone under Zn-deficient conditions. Similar results were also observed under Zn-sufficient conditions, although less pronounced (Figures 2A,C,D). Furthermore, Zn supply resulted in a 5.1–46.0% increase in leaf Pn (Figure 2A) and a 3.3–39.0% decrease in leaf Ci in comparison with no Zn supply (Figure 2B).
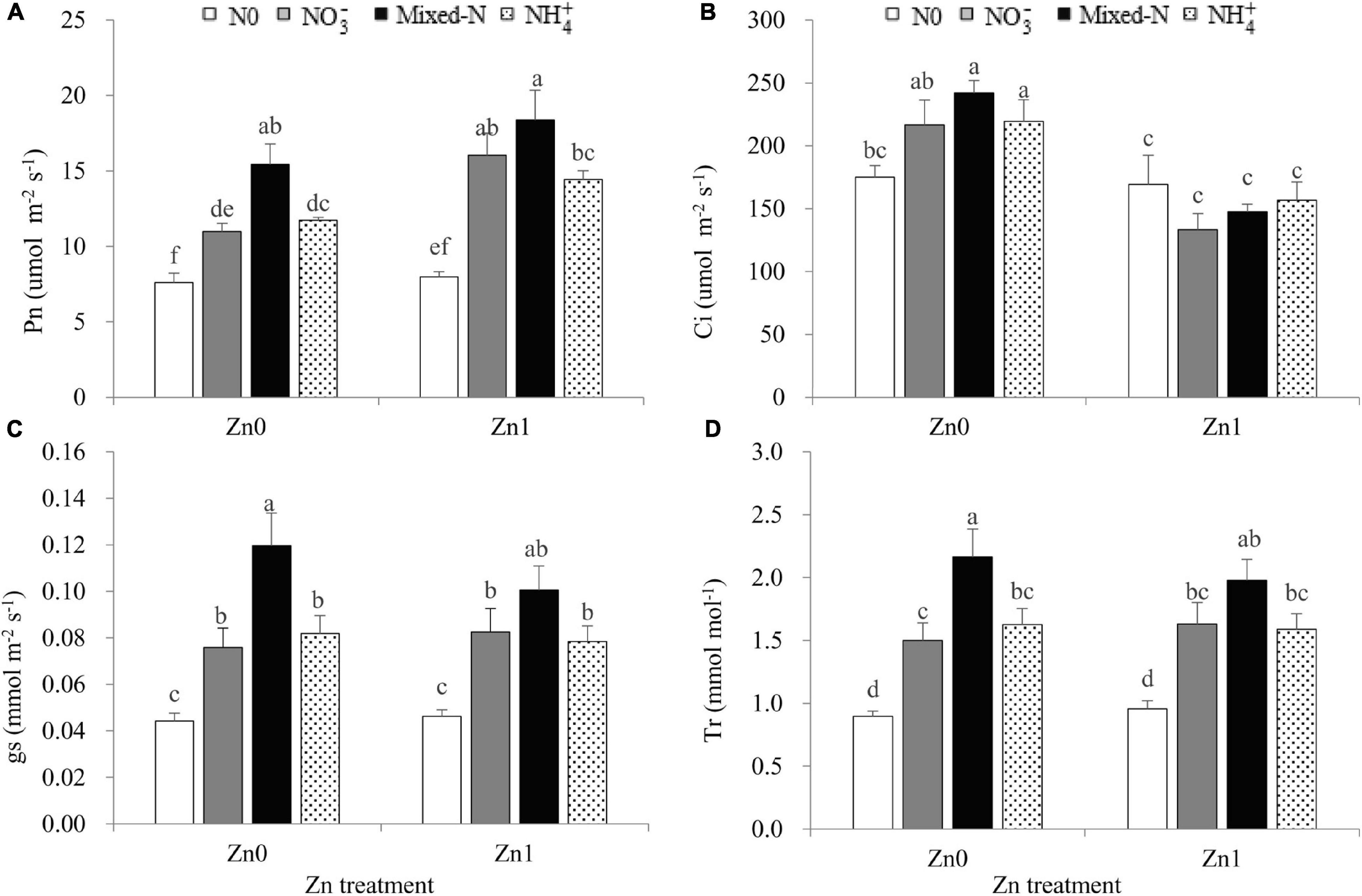
Figure 2. Leaf photosynthetic parameters including Pn (A), Ci (B), gs (C) and Tr (D) of newly developed leaf of 30-d-old maize seedlings cultured with nil N (N0), NO3–, mixed-N and NH4+ nutrition under sand culture conditions without (Zn0) and with Zn (Zn1) supply. Error bars represent the standard error of the mean (n = 4). Significant differences at P < 0.05 are shown with different letters. Pn, photosynthetic rate; Ci, intercellular CO2 concentration; gs, conductance to H2O; Tr, transpiration rate.
Root Length as Affected by N and Zn Supply
Roots were divided into three categories based on root diameter: fine roots (diameter < 0.2 mm), medium-sized roots (diameter between 0.2 and 0.4 mm), and thick roots (diameter >0.4 mm). Two-way ANOVA revealed significant effects of the N treatments on the fine root length (FRL), medium-sized root length (MRL), thick root length, and total root length (TRL). Significant effects of Zn supply on those parameters were observed with an exception of thick roots. However, the interaction between N treatments and Zn supply was not significantly different for these parameters (Supplementary Table 2). Compared with nil N supply, N supply generally improved the FRL, MRL, and thick root length and finally increased the TRL although there was no significant difference between nil N and NO3– nutrition irrespective of Zn supply. Among the three N forms, NH4+ supply produced the highest FRL, MRL, and thick roots and finally resulted in the highest TRL in the absence of Zn supply. Mixed-N supply resulted in the greatest TRL including the root length in different diameters in the presence of Zn supply. Compared with no Zn supply, Zn supply resulted in a 2.1–42.8% increase in TRL, with a significant increase with mixed-N supply (Figure 3A). Similar results were also observed for the total root surface area (Figure 3B).
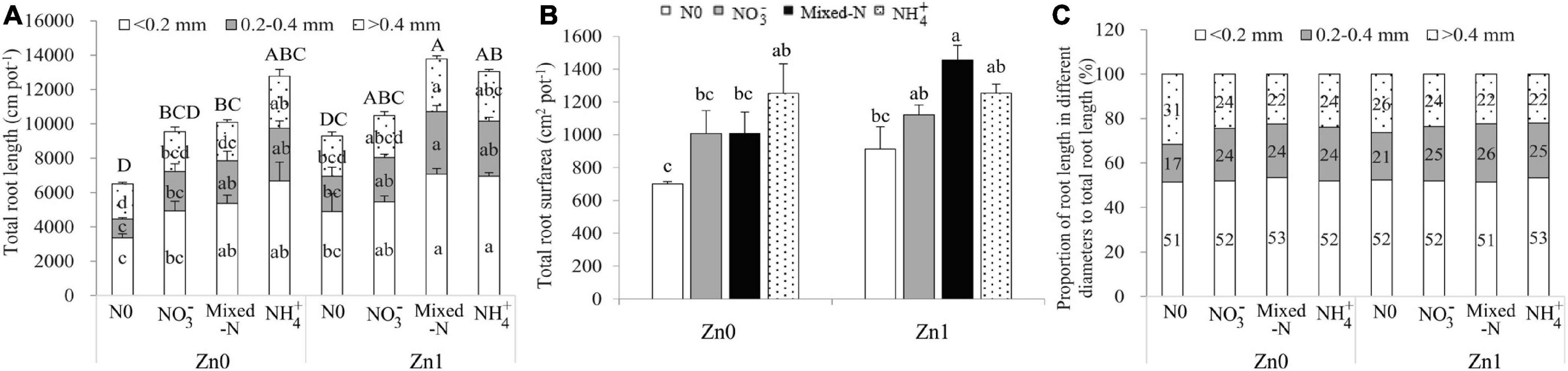
Figure 3. Root length (A), root surface area (B) and the proportions of root length in different diameters to total root length (C) of 30-d-old maize seedlings cultured with nil N (N0), NO3–, mixed-N and NH4+ nutrition under sand culture conditions without (Zn0) and with Zn (Zn1) supply. Error bars represent the standard error of the mean (n = 3). Significant differences at P < 0.05 are shown with different letters.
The proportion of FRL to TRL was not affected by N and Zn supply and averaged 52.2%. The proportion of MRL to TRL (ranging from 17.1 to 26.2%) tended to increase, whereas the thick root length to TRL (ranging from 22.0 to 31.5%) tended to decrease with N supply compared with no N supply (Figure 3C).
Nitrogen, C, and Zn Concentration and Accumulation of Shoot and Root
According to the results of two-way ANOVA, N and Zn treatments had significant effects on the N concentration and accumulation of shoots and roots, with the exception of a non-significant effect of Zn treatments on root N accumulation. The interactions between N and Zn treatments were also significant for the shoot N concentration and accumulation (Supplementary Table 3). Compared with nil N supply, three N forms substantially increased the N concentration in both shoot and root. The root N concentration was not significantly different among the three N forms irrespective of Zn supply (Figure 4A). Among the three N forms, shoot N concentration gradually decreased in the order NH4+ > NO3– > mixed-N nutrition in the presence of Zn supply. However, shoot N concentration was significantly lower in plants with NH4+ nutrition than NO3– and mixed-N nutrition in the absence of Zn supply (Figure 4A). Generally, the change in shoot N concentration was opposite to that of shoot DW accumulation, regardless of Zn supply in response to different N forms. Because the increase in shoot DW was greater than the decrease in shoot N concentration, the N accumulation of shoot, root, and whole plant was significantly greater in the order NH4+ < NO3– < mixed-N nutrition in the presence of Zn supply. The opposite was true, although less pronounced in the absence of Zn supply (Figure 4D). Compared with no Zn supply, nitrogen accumulation in shoot, root, and whole plant with N supply was increased by 13.6–45.8%, −9.9–44.0% (root N accumulation reduced with NH4+ supply), and 10.8–45.6% by Zn supply, respectively. The magnitude of increase in N accumulation of those tissues was consistently the greatest with mixed-N supply, followed by NO3– nutrition, whereas NH4+ nutrition exhibited the lowest increase (Figure 4D).
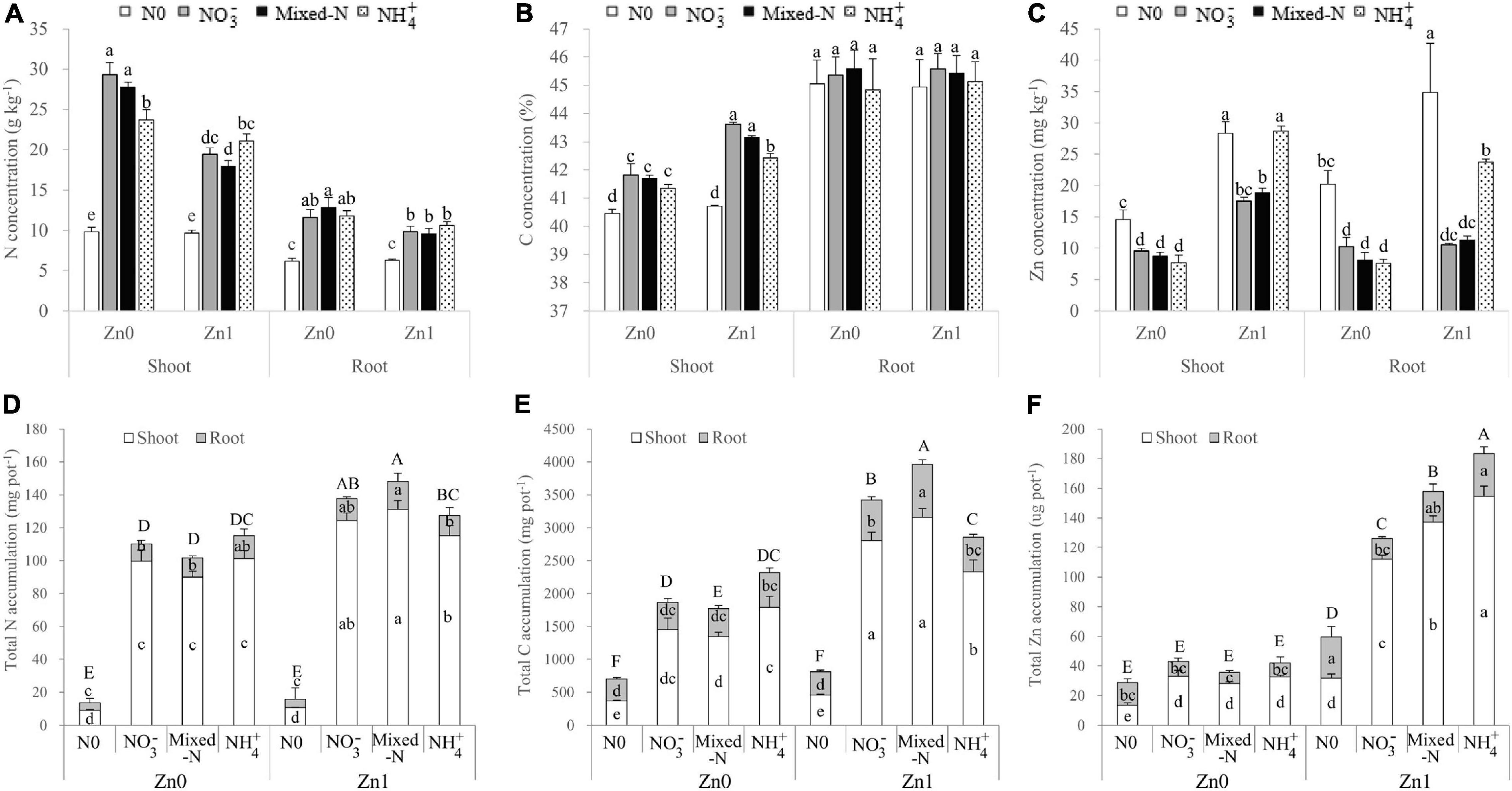
Figure 4. Nitrogen, C, and Zn concentration (A–C) and accumulation (D–F) of shoot and root of 30-day-old maize seedlings cultured with nil N (N0), NO3–, mixed-N, and NH4+ nutrition under sand culture conditions without (Zn0) and with Zn (Zn1) supply. Error bars represent the standard error of the mean (n = 4). Significant differences at p < 0.05 are shown with different letters.
Shoot C concentration was significantly increased, but root C concentration was not significantly affected by the three N forms compared with nil N supply, irrespective of Zn supply (Figure 4B). Among the three N forms, shoot C concentration was not significantly affected by the absence of Zn supply but was significantly decreased by NH4+ supply compared with NO3– and mixed-N supply in the presence of Zn supply (Figure 4B). The effects of Zn supply and N forms on the C accumulation in shoot, root, and whole plant were similar to those of N in the corresponding tissues (Figure 4E).
Not only the N and Zn supplies but also their interaction had significant effects on Zn concentration and accumulation in shoot, root, and whole plants with the exception of non-significant effects of N treatments and their interactions on root Zn accumulation (Supplementary Table 3). For Zn concentration, compared with nil N supply, the three N forms significantly decreased Zn concentration of both shoot and root, with the exception of Zn concentration of shoot receiving NH4+ nutrition in the presence of Zn supply. Among the three N forms, Zn concentration in both shoot and root was significantly more improved in plants grown with NH4+ than NO3– and mixed-N nutrition in the presence of Zn supply, whereas it was not significantly affected by the three N forms in the absence of Zn supply (Figure 4C). Total Zn accumulation including shoot and root was gradually increased in the order NO3– < mixed-N < NH4+ nutrition in the presence of Zn supply, but not affected by the three N forms in the absence of Zn supply. As expected, irrespective of N treatments, compared with no Zn supply, Zn supply resulted in 137.0–387.4, 44.4–206.8, and 108.3–342.4% higher Zn accumulation in shoot, root, and whole plant, respectively (Figure 4F).
The proportions of shoot to total accumulation of N and Zn were not affected by the three N forms and Zn supply. The proportions of shoot to total C accumulation were improved by Zn supply, but were not significantly affected by the three N forms. In general, compared with no N application, N application significantly increased the proportions of shoot to total accumulation from 67.4 to 90.4% for N, from 54.4 to 79.1% for C, and from 51.1 to 83.5% for Zn (Supplementary Figure 1).
The Ratios of C/N and Zn/N of Shoot and Root
Among the three N forms, shoot C/N ratio was the highest with NH4+ nutrition in the absence of Zn supply, but it was the highest with mixed-N nutrition in the presence of Zn supply. Root C/N ratio was not significantly affected by the three N forms and Zn supply (Figure 5A). The Zn/N ratios of both shoot and root were not significantly affected by the three N forms without Zn supply, but those tended to increase in the order of NO3– < mixed-N < NH4+ nutrition in the presence of Zn supply (Figure 5B). Irrespective of Zn supply, there was a significant positive correlation between shoot C/N ratio and shoot DW, implying that shoot C/N was an important indicator of shoot DW accumulation with N supply (Figure 5C).
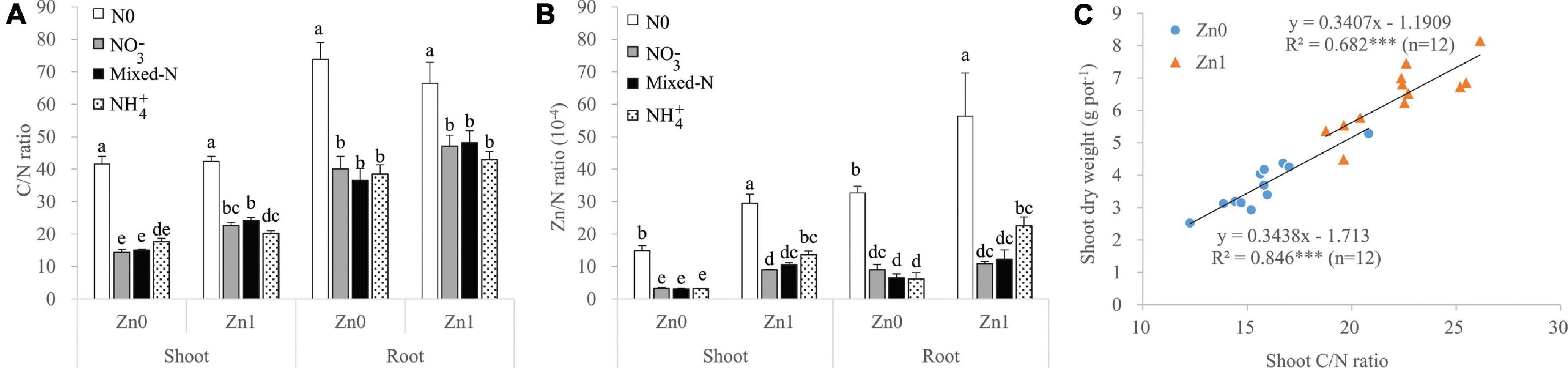
Figure 5. The ratios of C/N (A) and Zn/N (B) of shoot and roots of plants grown in different N forms without and with Zn supply together with the relationship between shoot C/N ratio and shoot dry weight of plants supplied with different N forms (excluding nil N treatment) without and with Zn supply (C). Values are mean and SE (n = 4). Significant differences at p < 0.05 are shown with different letters. *** indicate significant difference at 0.001 level.
Other Nutrient Accumulation of Shoot and Root
Two-way ANOVA revealed significant effects of the Zn supply on shoot accumulation of Fe, Mn, K, P, Mg, and Ca (but not Cu), and significant effects of N supply on all these nutrients. The interactions between Zn and N supply were also significant for shoot accumulation of these nutrients with the exception of Ca (Supplementary Table 3). However, for root nutrient accumulation, only root accumulation of Cu and Fe was significantly affected by Zn and N supply, respectively. The interactions between Zn and N supply were also significant for root Fe accumulation (Supplementary Table 3).
Figure 6 shows that under a Zn-deficient condition, total and shoot accumulation of Fe, Mn, Mg, and Ca was the highest with NH4+ nutrition, followed by mixed-N nutrition, whereas NO3– nutrition exhibited the lowest among the three N forms. For both K and P, total and shoot accumulation tended to decrease in the order NH4+ > NO3– > mixed-N nutrition. Under a Zn-sufficient condition, total and shoot accumulation of Fe, Mn, Mg, and Ca was the highest with mixed-N nutrition, followed by NH4+ nutrition, whereas NO3– nutrition exhibited the lowest among the three N forms. For both K and P, total and shoot accumulation tended to increase in the order NH4+ < mixed-N < NO3– nutrition. There were also significant and positive effects of Zn supply on shoot accumulation of Mg and Ca with each N form, and of Mn, K, and P with NO3– and mixed-N nutrition. However, compared with no Zn supply, shoot Fe accumulation was significantly lower with Zn supply when grown with NH4+ nutrition (Figure 6).
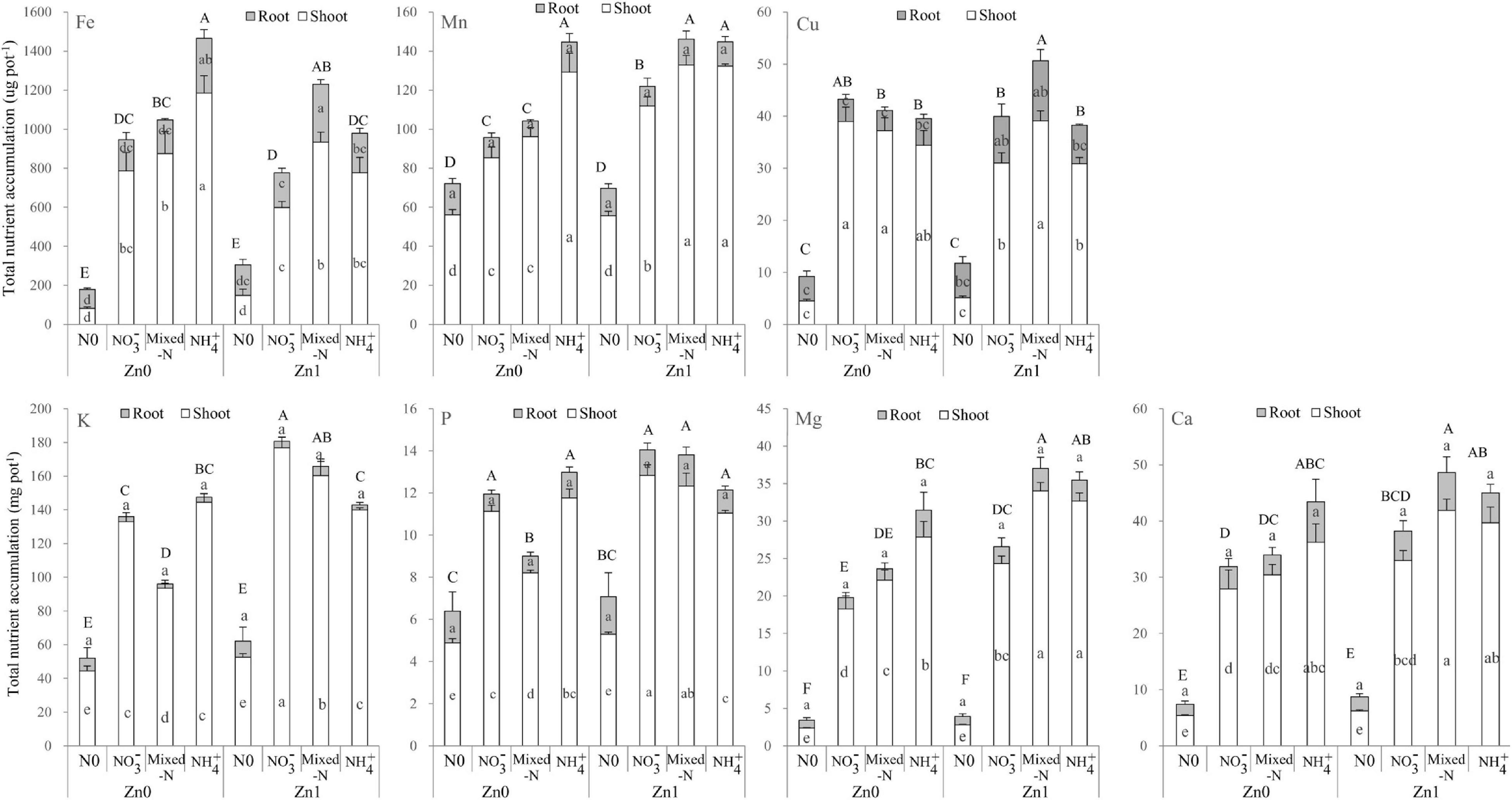
Figure 6. Other nutrient (Fe, Mn, Cu, K, P, Mg, and Ca) uptakes of shoot and roots of plants grown in different N forms without and with Zn supply. Values are mean and SE (n = 4). Significant differences at p < 0.05 are shown with different letters.
Discussion
Previous studies have shown that co-provision of NO3– and NH4+ stimulates plant growth beyond that observed with either N source provided individually in several crops (Gentry and Below, 1993; Marschner, 2011; Hachiya and Sakakibara, 2017; Cao et al., 2018; Wang et al., 2019). Furthermore, fertilizer Zn supply also improves shoot biomass accumulation and the final grain yield of maize (Sarwar et al., 2017; Zhang J. Y. et al., 2019; Liu et al., 2020) and wheat (Liu et al., 2019). However, the interaction between N forms and Zn nutritional status on plant growth has received considerably less attention. In this study, our results suggested that N regimes (including N levels and N forms), Zn supply, and their interactions exerted a significant effect on the growth of maize seedlings.
The extent of the effects of Zn supply on the DW of both shoot and root and N uptake depended on N availability in the growth medium. When N was deficient in solution, Zn supply had little effects on the growth and N absorption of maize seedlings, in agreement with others (Kutman et al., 2011b). However, when N was applied at a recommended rate, the positive effects of Zn supply on the growth and N absorption of maize seedlings were dependent on N forms. Generally, the growth and N absorption of maize seedlings supplied with NH4+ supply were superior to either NO3– or mixed-N nutrition under Zn-deficient conditions. However, the growth and N absorption of maize seedlings supplied with mixed-N supply were superior to independent NH4+ and NO3– nutrition under Zn-sufficient conditions. Furthermore, the positive effects of mixed-N nutrition and Zn supply on the growth of maize seedlings were more than additive. For example, with zero Zn supply, NH4+ supply resulted in 374.2% of increase in shoot DW, and with zero N supply, Zn supply increased the shoot DW by 22.4%, but the combined effects of mixed-N nutrition and Zn supply were over 700% (Table 1). Similar results were also found for N absorption in the shoot, root, and whole plant (Figure 4D). Similarly, a previous study also reported that the positive effects of high N and Zn supply on the grain yield of wheat were more than additive under greenhouse conditions (Kutman et al., 2011a). For example, at low Zn supply, high N supply resulted in a 73% increase in grain yield, and at low N supply, high Zn supply increased the grain yield by 35%, but the combined effect of high N–high Zn treatment was over 350%. Previous results obtained from greenhouse conditions showed Zn and N are synergistic in their effects on increasing plant Zn concentration, and their levels in growth medium should be at enough levels to achieve the synergistic effect of N on wheat root Zn uptake (Kutman et al., 2010, 2011a,b). Here, our results showed that compared with no N supply, a substantial increase in DW of shoot and root by N supply resulted in a significant decrease in Zn concentration (a so-called dilution effect) with the exception of Zn concentration in shoots of plants receiving both NH4+ and Zn supply (Table 1 and Figure 4C). Finally, the total Zn accumulation, including shoot and root, was significantly increased by NH4+ supply in the presentence of Zn supply, but not significantly affected by N rates and forms in the absence of Zn supply. These results suggested that the combined applications of NH4+ and Zn fertilizers have beneficial effects on plant Zn nutrition. The NH4+-induced acidification and a decline in apoplastic pH may play an important role in increasing Zn availability in solution for root uptake. The abundance of root Zn uptake transporters in the plasma membrane of root cells, including ZIPs such as IRT1 and other unknown proteins (Ishimaru et al., 2005; Palmer and Guerinot, 2009; Tiong et al., 2014), may also be enhanced by NH4+ supply.
Zinc is an essential micro-nutrient for plants. As expected, Zn-deficient stress resulted in a 21.1–55.7% of decrease in shoot DW and 0.5–47.9% of decrease in root DW irrespective of N forms. These results revealed that shoots were more sensitive to Zn deficiency than the roots, in agreement with previous studies on Zn deficiency in maize (Wang and Jin, 2005; Zhang J. Y. et al., 2019). Zn deficiency causes visible symptoms when Zn content is below 15–20 mg kg–1 in plants (Marschner, 2011; Zhang J. Y. et al., 2019). In this study, under Zn-deficient conditions, shoot Zn concentration significantly decreased from 14.6 mg kg–1 to around 8.7 mg kg–1 with N supply (Figure 4C). These values were below the critical Zn-deficient range of 15–20 mg kg–1 (Marschner, 2011), indicating a Zn-deficient status although severe Zn-deficient symptoms did not occur in maize seedlings (Supplementary Figure 2). The reduced shoot Zn concentration by N supply without causing severe Zn-deficient symptoms in maize seedlings may also indicate that adequate N application improves Zn mobility and physiological availability at the cellular level by affecting the level of Zn-chelating compounds, such as amino acids, peptides, or nicotianamine (Kutman et al., 2010). Alternatively, Denghai605 may be a highly Zn-efficient cultivar with ZE values of shoot ad root being 53.9 and 69.5%. However, the corresponding ZE values of shoot and root were only 36.8 and 50.0% for Zhengdan958 grown in Zn-free nutrient solution, which showed severely Zn-deficient symptoms 15 days after treatment (Zhang J. Y. et al., 2019). According to the criteria suggested by Farquhar and Sharkey (1982), when the Pn decreases along with an increase in Ci, photosynthesis is mainly limited by non-stomatal factors. In this study, compared with Zn supply, Zn deficiency resulted in a slight decrease in Pn, whereas Ci was significantly increased with gs and Tr not being affected regardless of N forms. Therefore, the reduction in Pn caused by Zn deficiency was mainly due to non-stomatal factors. The decreased Pn resulting from Zn deficiency may be due to the decline in the activities of carbonic anhydrase (Ohki, 1978), ribulose 1,5-bisphosphate carboxylase/oxygenase and fructose-1,6-bisphosphase (Hacisalihoglu, 2003; Marschner, 2011), and the dramatic damages of chloroplast structure and functions (Chen et al., 2010; Zhang J. Y. et al., 2019) as shown by the decreasing leaf SPAD values (Figure 1A).
Under Zn-deficient stress, the DW of both shoot and root was the highest with NH4+ nutrition among the three N forms. Furthermore, ZE of both shoot and root was significantly higher with NH4+ nutrition compared with NO3– and mixed-N supply (Table 1). These results suggested that NH4+ nutrition enhanced the tolerance of maize seedlings to Zn-deficient stress. Similarly, other studies also showed the preference of growth and N uptake for NH4+ over NO3– in maize (Gu et al., 2013; George et al., 2016; Zhang H. Q. et al., 2019). Several possible reasons are suggested for the growth preference of maize supplied by NH4+ over either NO3– or mixed-N nutrition under Zn-deficient conditions. First, NH4+ supply produced the highest FRL, MRL, and thick roots and finally resulted in the highest TRL and total root surface area among the three N forms in the absence of Zn supply (Figure 3). The root proliferation with NH4+ supply was beneficial to promote the root nutrient uptake such as N, P, K, and especially Fe, Mn, Mg, and Ca accumulation (Figures 4, 6) and thus to improve crop growth. Similarly, a previous study also reported that the root density and extension of maize seedlings were greater in nutrient solutions containing NH4+ than in those containing NO3– nutrition due to more rapid cell division in the root apical meristem of maize under NH4+ nutrition (Bloom et al., 2002). Results from field experiments also showed that the localized application of NH4-N + P significantly improved maize root biomass, the TRL, and lateral root proliferation at the seedling stage compared with localized application of NO3-N + P and urea +P, which could greatly contribute to improved nutrient uptake and biomass accumulation, and thus improve grain yield of maize grown in the calcareous soil with a critical Zn deficiency (DTPA-extractable Zn was 0.65 mg kg–1) (Ma et al., 2014). Furthermore, the NH4+-induced acidification and a decline in apoplastic pH cannot be ruled out as playing a role in increasing nutrient availability (and hence uptake), especially for Fe, Mn, Mg, and Ca uptake even though the pH of the nutrient solution was maintained at 6.0. More importantly, the uptake and assimilation of NH4+ by maize is a more energy-efficient process than that of NO3– because the reduction of each nitrate molecule to ammonium consumes about 15 ATP molecules, which are not consumed when ammonium is supplied (Salsac et al., 1987). Less energy is needed for roots to take up and assimilate NH4+ may be more adaptive under Zn-deficient stress. Additionally, the leaf protein content with NH4+ nutrition was significantly improved by Zn deficiency compared with Zn supply and was also slightly higher when compared to NO3– and mixed-N nutrition under Zn-deficient conditions (Figure 1B), which can prevent the dehydration of cells and enhanced the structure and function of cell membranes in adverse environments (Andrews et al., 2005; Cao et al., 2018), such as in Zn-deficient stress. Other studies reported that the supply of NH4+ nutrition significantly enhanced the drought tolerance of rice seedlings compared with the application of NO3–, and this effect seems to be closely related to the larger root tips and surface area, higher chlorophyll and Rubisco contents, and higher Rubisco activity due to the higher distribution of N absorbed to Rubisco (Guo et al., 2007; Gao et al., 2010; Ding et al., 2015). This information may be useful for enabling integrated management of Zn-deficient soils and increasing plant and grain production in the future.
Under Zn-sufficient conditions, the DW of shoot, root, and whole plant tended to increase in the order NH4+ < NO3– < mixed-N nutrition. Compared with NH4+ nutrition alone, mixed-N supply resulted in a 27.4 and 28.1% increase in leaf Pn and gs, which further resulted in a 35.7 and 33.5% increase in shoot C accumulation and shoot DW, respectively. Furthermore, mixed-N supply resulted in 19.7% of higher shoot C/N ratio vs. NH4+ nutrition alone, which means a higher shoot biomass accumulation because of a significant positive correlation between shoot C/N ratio and shoot DW (Figure 5C). Additionally, compared with the mixed-N supply, the negative effect of NH4+ nutrition on the root DW was larger than that on the TRL due to insufficient C supply from shoot to root, resulting in an overall increase in the specific root length (the ratio of TRL to root DW) (Table 1 and Figure 3A). Previous studies suggested that an increase in specific root length is an adaptive response to insufficient carbohydrate (Ostonen et al., 2007; Xie et al., 2007). Wang et al. (2019) also showed that compared with NH4+ nutrition alone, mixed-N nutrition resulted in a significantly increased shoot and root biomass mainly due to increased shoot ATP content and greater leaf area with similar or even lower Pn under lower and higher planting densities. Other studies also reported that mixed-N nutrition tended to increase both the accumulation of whole-shoot DW and the proportion partitioned to reproductive tissues and finally to increase the grain yield of maize, compared to NO3– nutrition only (Gentry and Below, 1993). Another field experiment showed that maize grain and straw yields were highest when fertilized with calcium ammonium nitrate, followed by ammonium sulfate, whereas urea exhibited the lowest yields (Abbasi et al., 2013).
Conclusion
Irrespective of N forms, compared with Zn supply, Zn deficiency resulted in a substantial decrease in both shoot and root DW, with the magnitude of decrease consistently being the highest with mixed-N nutrition, followed by NO3– nutrition whereas NH4+ nutrition exhibited the lowest decrease. Furthermore, ZE of both shoot and root was significantly higher with NH4+ nutrition compared with NO3– and mixed-N supply. These results suggested that NH4+ nutrition enhanced the tolerance of maize seedlings to Zn-deficient stress. Under Zn-sufficient conditions, the DW of the shoot, root, and whole plant tended to increase in the order NH4+ < NO3– < mixed-N nutrition. Furthermore, the positive effects of mixed-N nutrition and Zn supply on the growth of maize seedlings were more than additive. The combined applications of mixed-N nutrition and Zn fertilizer can promote root growth (including the increased root DW, root length in different diameter, TRL, and total root surface area), synchronously improve root absorption capacity of N, Fe, Cu, Mn, Mg, and Ca, increase leaf SPAD values and photosynthetic rate, and thus maximize the aboveground plant dry matter accumulation. In the case of total Zn uptake, the positive effect of NH4+ nutrition with Zn supply was more pronounced. This information may be useful for enabling integrated N management of Zn-deficient and Zn-sufficient soils and increasing plant and grain production in the future.
Data Availability Statement
The original contributions presented in the study are included in the article/Supplementary Material, further inquiries can be directed to the corresponding authors.
Author Contributions
KL, ZL, and YX conceived and designed the experiments, analyzed the data, and wrote the manuscript. WY, YG, HZ, LJ, XQ, ZC, CZ, SL, and HW performed the experiments. All the authors read and approved the final manuscript.
Funding
This study was financially supported by the National Natural Science Foundation of China (No. 31702001), the National Key Research and Development Program of China (No. 2017YFD0301005), the Taishan Scholarship Project of Shandong Province (No. TS201712082), and the Innovative Talent Introduction of Shandong Academy of Agricultural Sciences.
Conflict of Interest
The authors declare that the research was conducted in the absence of any commercial or financial relationships that could be construed as a potential conflict of interest.
Publisher’s Note
All claims expressed in this article are solely those of the authors and do not necessarily represent those of their affiliated organizations, or those of the publisher, the editors and the reviewers. Any product that may be evaluated in this article, or claim that may be made by its manufacturer, is not guaranteed or endorsed by the publisher.
Acknowledgments
The authors are grateful to Tom Sizmur in Reading University in the United Kingdom for his English language improvements in the manuscript.
Supplementary Material
The Supplementary Material for this article can be found online at: https://www.frontiersin.org/articles/10.3389/fpls.2021.722752/full#supplementary-material
Supplementary Figure 1 | The proportions of shoot to total N, C, and Zn accumulation of 30-day-old maize seedlings cultured with nil N (N0), NO3–, mixed-N, and NH4+ nutrition under sand culture conditions without (Zn0) and with Zn (Zn1) supply. Error bars represent the standard error of the mean (n = 4). Significant differences at p < 0.05 are shown with different letters.
Supplementary Figure 2 | The growth of 30-day-old maize seedlings cultured with nil N (N0), NO3–, mixed-N, and NH4+ nutrition under sand culture conditions without (Zn0) and with Zn (Zn1) supply.
References
Abbasi, M. K., Tahir, M. M., and Rahim, N. (2013). Effect of N fertilizer source and timing on yield and N use efficiency of rainfed maize (Zea mays L.) in Kashmir–Pakistan. Geoderma 19, 87–93. doi: 10.1016/j.geoderma.2012.11.013
Alloway, B. J. (2008). Zinc in Soils and Crop Nutrition, 2nd Edn. Brussels: International Zinc Association.
Andrews, M., Raven, J. A., Lea, P. J., and Sprent, J. I. (2005). A role for shoot protein in shoot–root dry matter allocation in higher plants. Ann. Bot. 97, 3–10. doi: 10.1093/aob/mcj009
Bloom, A. J., Meyerhoff, P. A., Taylor, A. R., and Rost, T. L. (2002). Root development and absorption of ammonium and nitrate from the rhizosphere. J. Plant Growth Regul. 21, 416–431. doi: 10.1007/s00344-003-0009-8
Bradford, M. M. (1976). A rapid and sensitive method for quantitation of microgram quantities of protein utilizing the principle of protein-dye binding. Anal. Biochem. 72, 248–254. doi: 10.1006/abio.1976.9999
Cakmak, I. (2008). Enrichment of cereal grains with zinc: agronomic or genetic biofortification? Plant Soil 302, 1–17. doi: 10.1007/s11104-007-9466-3
Cao, X. C., Zhu, C. Q., Zhong, C., Hussain, S., Zhu, L. F., Wu, L. H., et al. (2018). Mixed-nitrogen nutrition-mediated enhancement of drought tolerance of rice seedlings associated with photosynthesis, hormone balance and carbohydrate partitioning. Plant Growth Regul. 84, 451–465. doi: 10.1007/s10725-017-0352-6
Chen, W. R., Yang, X. E., He, Z. L., Feng, Y., and Hu, F. H. (2010). Differential changes in photosynthetic capacity, 77 K chlorophyll fluorescence and chloroplast ultrastructure between Zn-efficient and Zn-inefficient rice genotypes (Oryza sativa) under low zinc stress. Physiol. Plant. 132, 89–101. doi: 10.1111/j.1399-3054.2007.00992.x
Cramer, M. D., and Lewis, O. A. M. (1993). The influence of NO3– and NH4+ nutrition on the carbon and nitrogen partitioning characteristics of wheat (Triticum aestivum L.) and maize (Zea mays L.) plants. Plant Soil 154, 289–300. doi: 10.1007/BF00012534
Ding, L., Gao, C. M., Li, Y. R., Li, Y., Zhu, Y. Y., Xu, G. H., et al. (2015). The enhanced drought tolerance of rice plants under ammonium is related to aquaporin (AQP). Plant Sci. 234, 14–21. doi: 10.1016/j.plantsci.2015.01.016
Fang, Q. X., Qiang, Y., Wang, E. L., Chen, Y. H., Zhang, G. L., Wang, J., et al. (2006). Soil nitrate accumulation, leaching and crop nitrogen use as influenced by fertilization and irrigation in an intensive wheat-maize double cropping system in the North China Plain. Plant Soil 284, 335–350. doi: 10.1007/s11104-006-0055-7
Farquhar, G. D., and Sharkey, T. D. (1982). Stomatal conductance and photosynthesis. Ann. Rev. Plant Physiol. 33, 317–345. doi: 10.1146/annurev.pp.33.060182.001533
Gao, Y. X., Li, Y., Yang, X. X., Li, H. J., Shen, Q. R., and Guo, S. W. (2010). Ammonium nutrition increases water absorption in rice seedlings (Oryza sativa L.) under water stress. Plant Soil 331, 193–201. doi: 10.1007/s11104-009-0245-1
Gentry, L. E., and Below, F. E. (1993). Maize productivity as influenced by form and availability of nitrogen. Crop Sci. 33, 491–497. doi: 10.2135/cropsci1993.0011183X003300030015x
George, J., Holtham, L., Sabermanesh, K., Heuer, S., Tester, M., Plett, D., et al. (2016). Small amounts of ammonium (NH4+) can increase growth of maize (Zea mays). J. Plant Nutr. Soil Sci. 179, 717–725. doi: 10.1002/jpln.201500625
Gu, R. L., Duan, F. Y., Xia, A., Zhang, F. S., vonWirén, N., and Yuan, L. X. (2013). Characterization of AMT-mediated high-affinity ammonium uptake in roots of maize (Zea mays L.). Plant Cell Physiol. 54, 1515–1524. doi: 10.1093/pcp/pct099
Guo, S. W., Chen, G., Zhou, Y., and Shen, Q. R. (2007). Ammonium nutrition increases photosynthesis rate under water stress at early development stage of rice (Oryza sativa L.). Plant Soil 296, 115–124. doi: 10.1007/s11104-007-9302-9
Hachiya, T., and Sakakibara, H. (2017). Interactions between nitrate and ammonium in their uptake, allocation, assimilation, and signaling in plants. J. Exp. Bot. 68, 2501–2512. doi: 10.1093/jxb/erw449
Hacisalihoglu, G. (2003). Zinc efficiency is correlated with enhanced expression and activity of zinc-requiring enzymes in wheat. Plant Physiol. 131, 595–602. doi: 10.1046/j.1469-8137.2003.00826.x
Ishimaru, Y., Suzuki, M., Kobayashi, T., Takahashi, M., Nakanishi, H., Mori, S., et al. (2005). OsZIP4, a novel zinc-regulated zinc transporter in rice. J. Exp. Bot. 56, 3207–3214. doi: 10.1093/jxb/eri317
Karim, M. R., Zhang, Y. Q., Tian, D., Chen, F. J., Zhang, F. S., and Zou, C. Q. (2012). Genotypic differences in zinc efficiency of Chinese maize evaluated in a pot experiment. J. Sci. Food Agr. 92, 2552–2559. doi: 10.1002/jsfa.5672
Kutman, U. B., Yildiz, B., and Cakmak, I. (2011a). Effect of nitrogen on uptake, remobilization and partitioning of zinc and iron throughout the development of durum wheat. Plant Soil 342, 149–164. doi: 10.1007/s11104-010-0679-5
Kutman, U. B., Yildiz, B., and Cakmak, I. (2011b). Improved nitrogen status enhances zinc and iron concentrations both in the whole grain and the endosperm fraction of wheat. J. Cereal Sci. 53, 118–125. doi: 10.1016/j.jcs.2010.10.006
Kutman, U. B., Yildiz, B., Ozturk, L., and Cakmak, I. (2010). Biofortification of durum wheat with zinc through soil and foliar applications of nitrogen. Cereal Chem. 87, 1–9. doi: 10.1094/CCHEM-87-1-0001
Liu, D. Y., Liu, Y. M., Zhang, W., Chen, X. P., and Zou, C. Q. (2019). Zinc uptake, translocation, and remobilization in winter wheat as affected by soil application of Zn fertilizer. Front. Plant Sci. 10:426. doi: 10.3389/fpls.2019.00426
Liu, D. Y., Zhang, W., Liu, Y. M., Chen, X. P., and Zou, C. Q. (2020). Soil application of zinc fertilizer increases maize yield by enhancing the kernel number and kernel weight of inferior grains. Front. Plant Sci. 11:188. doi: 10.3389/fpls.2020.00188
Liu, H., Gan, W., Rengel, Z., and Zhao, P. (2016). Effects of zinc fertilizer rate and application method on photosynthetic characteristics and grain yield of summer maize. J. Soil Sci. Plant Nutr. 16, 550–562.
Liu, Z. (1996). Microelements in Soils of China (in Chinese). Nanjing: Jiangsu Science Technology Publishing house.
Ma, Q. H., Wang, X., Li, H., Li, H. B., Li, H. G., Cheng, L. Y., et al. (2014). Localized application of NH4+-N plus P enhances zinc and iron accumulation in maize via modifying root traits and rhizosphere processes. Field Crops Res. 164, 107–116. doi: 10.1016/j.fcr.2014.05.017
Mueller, N. D., Gerber, J. S., Johnston, M., Ray, D. K., Ramankutty, N., and Foley, J. A. (2012). Closing yield gaps through nutrient and water management. Nature 490, 254–257. doi: 10.1038/nature11420
Ohki, K. (1978). Zinc concentration in soybean as related to growth, photosynthesis, and carbonic anhydrase activity. Crop Sci. 18, 79–82. doi: 10.2135/cropsci1978.0011183X001800010021x
Ostonen, I., Püttsepp, Ü, Biel, C., Alberton, O., Bakker, M. R., Lõhmus, K., et al. (2007). Specific root length as an indicator of environmental change. Plant Biosyst. 141, 426–442. doi: 10.1080/11263500701626069
Palmer, C. M., and Guerinot, M. L. (2009). Facing the challenges of Cu, Fe and Zn homeostasis in plants. Nat. Chem. Biol. 5, 333–340. doi: 10.1038/nchembio.166
Potarzycki, J. (2010). The impact of fertilization systems on zinc management by grain maize. Fertiliser Fertilization 39, 78–89. doi: 10.1155/2009/251915
Salsac, L., Chaillou, S., Morot-Gaudry, J. F., Lesaint, C., and Jolivet, E. (1987). Nitrate and ammonium nutrition in plants. Plant Physiol. Biochem. 25, 805–812.
Sarwar, S., Rafique, E., Yousra, M., Mahmood-ul-Hassan, M., Gill, S. M., and Khan, M. Z. (2017). Improved productivity and zinc content for maize grain by different zinc fertilization techniques in calcareous soils. J. Plant Nutr. 40, 417–426. doi: 10.1080/01904167.2016.1245322
Schortemeyer, M., Stamp, P., and Feil, B. (1997). Ammonium tolerance and carbohydrate status in maize cultivars. Ann. Bot. 79, 25–30. doi: 10.1006/anbo.1996.0298
Tang, Q. Y., and Zhang, C. X. (2013). Data Processing System (DPS) software with experimental design, statistical analysis and data mining developed for use in entomological research. Insect Sci. 20, 254–260. doi: 10.1111/j.1744-7917.2012.01519.x
Tilman, D., Cassman, K. G., Matson, P. A., Naylor, R., and Polasky, S. (2002). Agricultural sustainability and intensive production practices. Nature 418, 671–677. doi: 10.1038/nature01014
Tiong, J., McDonald, G. K., Genc, Y., Pedas, P., Hayes, J. E., Toubia, J., et al. (2014). HvZIP7 mediates zinc accumulation in barley (Hordeum vulgare) at moderately high zinc supply. New Phytol. 201, 131–143. doi: 10.1111/nph.12468
Wang, H., and Jin, J. Y. (2005). Photosynthetic rate, chlorophyll fluorescence parameters, and lipid peroxidation of maize leaves as affected by zinc deficiency. Photosynthetica 43, 591–596. doi: 10.1007/s11099-005-0092-0
Wang, H., and Jin, W. Y. (2007). Effects of zinc deficiency and drought on plant growth and metabolism of reactive oxygen species in maize (Zea mays L). J. Integr. Agr. 6, 988–995. doi: 10.1016/s1671-2927(07)60138-2
Wang, J. W., Mao, H., Zhao, H. B., Huang, D. L., and Wang, Z. H. (2012). Different increases in maize and wheat grain zinc concentrations caused by soil and foliar applications of zinc in Loess Plateau, China. Field Crops Res. 135, 89–96. doi: 10.1016/j.fcr.2012.07.010
Wang, P., Wang, Z. K., Sun, X. C., Mu, X. H., Chen, H., Chen, F. J., et al. (2019). Interaction effect of nitrogen form and planting density on plant growth and nutrient uptake in maize seedlings. J. Integr. Agr. 18, 1120–1129. doi: 10.1016/S2095-3119(18)61977-X
Welch, R. M., and Graham, R. D. (2004). Breeding for micronutrients in staple food crops from a human nutrition perspective. J. Exp. Bot. 55, 353–364. doi: 10.1093/jxb/erh064
Xie, Y. H., Luo, W. B., Ren, B., and Li, F. (2007). Morphological and physiological responses to sediment type and light availability in roots of the submerged plant myriophyllum spicatum. Ann. Bot. 100, 1517–1523.
Xue, Y. F., Yue, S. C., Liu, D. Y., Zhang, W., Chen, X. P., and Zou, C. Q. (2019). Dynamic zinc accumulation and contributions of pre- and/or post-silking zinc uptake to grain zinc of maize as affected by nitrogen supply. Front. Plant Sci. 10:1203. doi: 10.3389/fpls.2019.01203
Xue, Y. F., Yue, S. C., Zhang, Y. Q., Cui, Z. L., Chen, X. P., Yang, F. C., et al. (2012). Grain and shoot zinc accumulation in winter wheat affected by nitrogen management. Plant Soil 361, 153–163. doi: 10.1007/s11104-012-1510-2
Xue, Y. F., Zhang, W., Liu, D. Y., Yue, S. C., Cui, Z. L., Chen, X. P., et al. (2014). Effects of nitrogen management on root morphology and zinc translocation from root to shoot of winter wheat in the field. Field Crops Res. 161, 38–45.
Yuan, S., Li, C. J., Peng, Z. P., Peng, Y. F., Wang, H., and Zhang, L. J. (2011). Effects of phosphorus on the plant growth, phosphorus cycling and distribution in different maize cultivars. Plant Nutr. Fertilizer Sci. 17, 310–316.
Zhang, H. Q., Zhao, X. Q., Chen, Y. L., Zhang, L. Y., and Shen, R. F. (2019). Case of a stronger capability of maize seedlings to use ammonium being responsible for the higher 15N recovery efficiency of ammonium compared with nitrate. Plant Soil 440, 293–309. doi: 10.1007/s11104-019-04087-w
Zhang, J. Y., Wang, S. F., Song, S. S., Xu, F. S., Pan, Y. H., and Wang, H. (2019). Transcriptomic and proteomic analyses reveal new insight into chlorophyll synthesis and chloroplast structure of maize leaves under zinc deficiency stress. J. Proteomics 199, 123–134. doi: 10.1016/j.jprot.2019.03.001
Keywords: maize, nitrogen rates and forms, zinc supply, interaction effects, nutrient uptake, root morphology
Citation: Xue Y, Yan W, Gao Y, Zhang H, Jiang L, Qian X, Cui Z, Zhang C, Liu S, Wang H, Li Z and Liu K (2021) Interaction Effects of Nitrogen Rates and Forms Combined With and Without Zinc Supply on Plant Growth and Nutrient Uptake in Maize Seedlings. Front. Plant Sci. 12:722752. doi: 10.3389/fpls.2021.722752
Received: 09 June 2021; Accepted: 10 November 2021;
Published: 09 December 2021.
Edited by:
Francesco Di Gioia, The Pennsylvania State University (PSU), United StatesReviewed by:
Myungjin Lee, The Pennsylvania State University (PSU), United StatesIbukun Ayankojo, University of Florida, United States
Copyright © 2021 Xue, Yan, Gao, Zhang, Jiang, Qian, Cui, Zhang, Liu, Wang, Li and Liu. This is an open-access article distributed under the terms of the Creative Commons Attribution License (CC BY). The use, distribution or reproduction in other forums is permitted, provided the original author(s) and the copyright owner(s) are credited and that the original publication in this journal is cited, in accordance with accepted academic practice. No use, distribution or reproduction is permitted which does not comply with these terms.
*Correspondence: Zongxin Li, c2RhdWNsaWZmQHNpbmEuY29t; Kaichang Liu, bGl1a2MxOTcxQDE2My5jb20=