- 1College of Tropical Crops, Hainan University, Haikou, China
- 2Rubber Research Institute of Chinese Academy of Tropical Agricultural Sciences (CATAS), Haikou, China
EgMADS16, one of the MADS-box transcription factors in oil palm, has a high expression level in the late fruit development of the oil palm fruit mesocarp. At the same time, it is also predicted to be the target gene of EgmiR5179, which has been identified in previous research. In this paper, we focused on the function and regulatory mechanism of the EgMADS16 gene in oil palm lipid metabolism. The results indicated that the transcription level of EgMADS16 was highest in the fourth stage, and a dual-luciferase reporter assay proved that the EgMADS16 expression level was downregulated by EgmiR5179. In both the OXEgMADS16 Arabidopsis seeds and oil palm embryonic calli, the total lipid contents were significantly decreased, but the contents of C18:0 and C18:3 in OXEgMADS16 lines were significantly increased. As expected, EgmiR5179 weakened the inhibitory effect of EgMADS16 on the oil contents in transgenic Arabidopsis plants that coexpressed EgmiR5179 and EgMADS16 (OXEgmiR5179-EgMADS16). Moreover, yeast two-hybrid and BiFC analyses suggested that there was an interaction between the EgMADS16 protein and EgGLO1 protein, which had been proven to be capable of regulating fatty acid synthesis in our previous research work. In summary, a model of the molecular mechanism by which miRNA5179 targets EgMADS16 to regulate oil biosynthesis was hypothesized, and the research results provide new insight into lipid accumulation and molecular regulation in oil palm.
Introduction
Oil palm (Elaeis guineensis Jacq.), which belongs to the Arecaceae family, is the most productive oil crop in the world and can accumulate up to 90% oil in the mesocarp (Bhagya et al., 2020). At present, the main oil crops that produce vegetable oils are oil palm, soybean, rape, and sunflower. They produce 79% of the total oil yields (Dyer et al., 2008). The palm oil produced by oil palm accounts for 36% of the total oil yields (Osorio-Guarin et al., 2019). Although much progress has been made on plant lipid metabolism and regulatory mechanisms via plant molecular biology research, it is still very difficult to obtain the ideal palm oil with the highest nutritional value or the largest yield. Presently, there are also few reports about the genes involved in the regulation of oil metabolism and related transcription factor regulation networks in oil palm (Li et al., 2020).
In plant seeds, lipids are the main form of carbon storage, constituting up to 60% of the dry seed weight (Ohlroggeav and Browse, 1995). The biosynthesis of vegetable oil is affected by many factors, including transcription factors, microRNAs (miRNAs), plant hormones, signaling molecules, and environmental factors. Several transcription factors have been found to participate in the regulation of oil biosynthesis. For example, ZmWRI1, which belongs to the AP2/EREBP family, could regulate FA biosynthesis and increase seed oil by up to 46% in maize seeds overexpressing ZmWRI1 without affecting the germination, seedling growth, and grain yield (Shen et al., 2010). GmMYB73, which belongs to the MYB family, and GmbZIP123, which belongs to the bZIP family, could enhance lipid contents in both the seeds and leaves of transgenic Arabidopsis plants (Song et al., 2013; Liu et al., 2014). In oil palm, EgMADS21 regulates EgDGAT2 expression and ultimately affects fatty acid accumulation in the mesocarp (Li et al., 2020). In addition, EgWRI1-1 participated in the regulation of oil biosynthesis by interacting directly with the EgNF-YA3 protein. EgWRKY40 interacted with EgWRKY2 to inhibit the transcription of oil biosynthesis-related genes (Yeap et al., 2017). However, little is known about the molecular mechanism of lipid accumulation in the mesocarp of oil palm.
In our previous research, overexpressing EgmiR5179 significantly increased the oil yield in Arabidopsis seeds, and one MADS-box transcription factor (EgMADS16) was predicted to be the target gene of EgmiR5179 (Gao et al., 2019). In this study, we cloned full-length EgMADS16 from the mesocarp of oil palm. Transgenic Arabidopsis plants and oil palm embryoids overexpressing EgMADS16 were obtained, and the contents of oil and fatty acids were tested. Finally, transgenic Arabidopsis overexpressing EgmiR5179 and EgMADS16 was obtained, and lipid and fatty acid contents were detected to confirm the relationship between EgMADS16, EgmiR5179, and oil biosynthesis. The results depicted one pathway by which miRNAs target transcription factors and ultimately regulate lipid metabolism, providing a new strategy for lipid metabolism research in oil palm.
Materials and Methods
Plant Materials and Strains
Oil palm (Elaeis guineensis Jacq.) fruits were collected at the Chinese Academy of Tropical Agricultural Sciences in Hainan, PR China. These fruits were divided into phase 1 [30–60 days after pollination (DAP)], phase 2 (60–100 DAP), phase 3 (100–120 DAP), phase 4 (120–140 DAP), and phase 5 (140–160 DAP) according to the developmental stage (Tranbarger et al., 2011) and stored at −80°C until the next experiment. Three independent bunches were collected from three distinct individuals which at the similar stage. Arabidopsis thaliana plants were grown in a 23°C incubator with 16 h light/8 h dark cycles. In addition, oil palm embryoids were induced and cultured using the method in previous research (Zou et al., 2019). All strains, including DH5α (E. coli), Y187 and Y2HGold (yeast), and GV3101 (Agrobacterium), were maintained by our laboratory.
RNA Extraction and Real-Time PCR Analysis
Total RNA of oil palm fruit mesocarps (0.5 g), Arabidopsis leaves (0.1 g), and oil palm embryoids (0.2 g) was extracted by an RNAprep Pure Plant Kit (Polysaccharides and Polyphenolics-rich; Tiangen, Beijing, China). Protoplastic RNA was isolated using TRIzol Reagent (Invitrogen, United States). cDNA was synthesized using the FastQuant RT kit (with gDNase; Tiangen, Beijing, China), and quantitative real-time PCR (qPCR) was performed with TB GreenTM Premix Ex TaqTM II (Tli RNaseH Plus; TaKaRa, Japan) on a CFX96 Touch System (Bio-Rad, United States). Each sample was set to three replicates, and the internal control for the oil palm sample was β-Actine, while that for the Arabidopsis sample was Actin7. In addition, all experimental steps were provided by the manufacturer, and the related primers designed by Primer Premier 5 are shown in Supplementary Table 1.
Gene Cloning and Vector Construction
The vector pCAMBIA3301 was used for plant transformation. pGreen II 62-SK (SK) was used for protoplast transfection and the dual-luciferase reporter assay. pCAMBIA1300S containing the green fluorescent protein (GFP) gene was used for subcellular localization. The pHiS2.1, pGADT7, and pGBKT7 vectors were used for the yeast hybrid assay, and the pSPYNE and pSPYCE vectors were used for BiFC analysis. The CDS of EgMADS16 was cloned using different primers and ligated into pGreen II 0800-miRNA (LUC), pGADT7, and pGBKT7 using a One Step Cloning Kit (Vazyme) according to the principle of homologous recombination. The CDS of EgMADS16 without the final TGA was cloned into pCAMBIA1300S and pSPYNE. Likewise, the CDS of EgGLO1 without the final TGA was cloned into pSPYCE vectors. For the EgMADS16-p3301 vector, we ligated the CDS of EgMADS16 into the pCAMBIA1300S vector, cut the 35S::EgMADS16::NOS terminator, and inserted the terminator into the multiple cloning site of the pCAMBIA3301 vector using a restriction enzyme. The plasmids about EgmiR5179 were constructed and conserved in our laboratory (Gao et al., 2019). All the primers used for the vector construction are shown in Supplementary Table 2.
Subcellular Localization in Nicotiana benthamiana
The subcellular localization of EgMADS16 protein was performed in Nicotiana benthamiana leaves according to previous research (Jessen et al., 2015). Agrobacterium tumefaciens GV3101 strains harboring EgMADS16-p1300SGFP plasmids, which encode the MADS16-EGFP fusion protein, were incubated to an OD 600 of 0.6; after centrifugation (4,000 rpm, 10 min), they were resuspended in the equal volume buffer (0.01 M MgCl2, 0.01 M MES, PH5.5, and 150 μm AS) and infiltrated into the leaves of Nicotiana benthamiana. After incubation for 36–72 h, the GFP signals in the lower epidermis of these leaves were observed using a fluorescence inverted microscope (Nikon, TS2-LS) with an excitation wavelength of 430–510 nm. At the same time, nuclei were stained with 4', 6-diamidino-2-phenylindole (Sigma-Aldrich, United States) and observed using excitation wavelengths of 330–380 nm.
Dual-Luciferase Reporter Assay
The 10 μg EgMADS16-LUC plasmids were transformed into 200 μl oil palm protoplasts (5 × 105/ml) with 10 μg EgmiR5179-SK or SK plasmids; each sample was set to three replicates. After incubating for 14–18 h, the firefly and Renilla luciferase activities were tested using a dual-luciferase reporter gene assay kit (Promega, United States). At the same time, EgMADS16-LUC was transformed into wild-type or OXEgmiR5179 Arabidopsis protoplasts, and the dual-luciferase activities were analyzed using the same method.
Protoplast Isolation and Transformation
The protoplast isolation and transfection of Arabidopsis leaves were performed as described in a previous study (Yoo et al., 2007). In addition, protoplasts of oil palm leaves were isolated according to the preparation of Arabidopsis leaf protoplasts, but the contents of Cellulase R10 (BR) and Macerozyme R10 (BR) were doubled. The plasmids were transferred into oil palm protoplasts using an equal volume 40% (w/v) polyethylene glycol/50 mm MgCl2 solution, and heat shock treatment was performed as described in previous research (Masani et al., 2014).
Arabidopsis thaliana Transformation
A. thaliana was cultured in 16 h light/8 h dark at 23°C. EgMADS16-p3301 plasmids were transformed into GV3101 Agrobacterium and then introduced into A. thaliana using the floral dip method (Jin et al., 2017). Moreover, transformants were selected by 1/2 Murashige and Skoog medium containing 60 mg/L glufosinate ammonium.
Oil Palm Embryoids Culture and Transformation
Oil palm embryoids were cultured using woody plant medium in a 28°C incubator with 12 h light/12 h dark cycles according to previous research (Zou et al., 2019). A. tumefaciens GV3101, which contained EgMADS16-p3301 plasmids, was used to infect oil palm embryoids for 15 min with an OD600 of 0.5. Then, these embryoids were cultured on cocultivation medium (woody plant medium supplemented with 100 mg/L cysteine and 100 μm AS) at 19°C for 2 days and on screening medium (woody plant medium supplemented with 400 mg/L timentin and 60 mg/L glufosinate ammonium) at 28°C for 4 months, and the medium was regenerated every 20 days.
Total Lipid Extraction and Fatty Acid Analysis of the Arabidopsis Plants and Oil Palm Embryoids
Both the lipid and fatty acid analyses of Arabidopsis plants and oil palm embryoids were conducted according to previous studies (Yuan et al., 2017). In brief, 5 ml chloroform-methanol (volume ratio 2:1) was used to extract lipids, and then, lipids were dried by a Termovap sample concentrator. In addition, 10 μg C17:0 was added to the lipid samples as an internal standard. All samples were methylated by 3 ml concentrated sulfuric acid-methanol (volume ratio 1:40) at 80°C for 2 h. Then, 3 ml N-hexane and 2 ml 0.9% (W/V) sodium chloride solution were added. After centrifuging at 4,000 rpm for 5 min, the supernatant was transferred to a new tube and dried by a Termovap sample concentrator. The fatty acid methyl ester was finally dissolved in 1 ml n-hexane for GC analysis. The GC analysis was performed by the Analytical and Testing Center of Hainan University. Detailed operation parameters: the oven temperature was initially maintained at 150°C for 1 min, then increased at 8°C/min to 250°C, and then increased to 250°C and maintained for 5 min. The split ratio was 1:30, and the carrier gas was helium at a flow rate of 1.0 ml/min in constant flow mode. The injector was at 250°C, and the detector, at 230°C. And the lipid mass was calculated using the internal standard method.
Yeast Two-Hybrid Assays
Yeast two-hybrid assays were performed using Matchmaker™ Gold Yeast Two-Hybrid System (Clontech, United States). According to the user manual, EgMADS16-pGBKT7 plasmids were transfected into Y2H Gold yeast strains, and the autoactivation of EgMADS16 transcription factor was tested. Then, the EgMADS16-pGBKT7 Y2H Gold yeast strains and oil palm library strains which kept by our laboratory were combined. After incubation at 30°C for 20–24 h at 45 rpm, these strains were plated on SD/Try/Leu/-His/Ade/+X-α-Gal/+Aba mediums.
Bimolecular Fluorescence Complementation Analysis
EgMADS16-pSPYNE and EgGLO1-pSPYCE were transformed into Agrobacterium strains GV3101, respectively. And then these strains were coinfiltrated into the leaves of N. benthamiana using the same method as subcellular localization. Moreover, after incubation for 36–72 h, the yellow fluorescent protein (YFP) signal was observed using the excitation wavelength of 430–510 nm.
Bioinformational Analysis
Conserved domain was analyzed in the web site.1 Multiple sequence alignment was carried out by Clustal Omega.2 Small RNA target analysis was performed using the web site.3 The phylogenetic tree of EgMADS16 and other MADS proteins from different species was constructed using the neighbor-joining method in MEGA6.
Statistical Analysis
The values are the means ± SD (n ≧ 3). Significant differences between groups were analyzed by SPSS software using Student’s t-test. “*” represents a significant difference (p < 0.05), and “**” represents a highly significant difference (p < 0.01).
Results
Cloning and Subcellular Localization of EgMADS16
The mRNA of EgMADS16 (NM_001303583) is 951 bp in length and encodes a protein of 225 amino acids (NP_001290512.1). The CDS of EgMADS16, which is 678 bp, from oil palm mesocarp using primers is shown in Supplementary Table 2. qPCR indicated that the expression of EgMADS16 fluctuated from phase 1 to phase 3 and then sharply increased and reached a peak in phase 4; however, there was a gradual decline from phase 4 to phase 5 (Figure 1A). Conserved domain analysis (see footnote 1) showed that there are MADS_MEF2_like and K-box domains in the EgMADS16 amino acid sequence (Figure 1B). In addition, we constructed a phylogenetic tree of EgMADS16 and other MADS proteins from different species using the neighbor-joining method in MEGA6, including EgGLO1 (XP_010911271.1), EgGLO2 (AAQ03229.1), OsMADS2 (XP_015623988.1), OsMADS4 (XP_015640709.1), OsMADS6 (XP_015623947.1), OsMADS8 (XP_015610824.1), OsMADS16 (XP_015641661.1), AcMADS16 (XP_020082339.1), BnAGL11 (XP_013719732.1), BnTT16 (NP_001303188.1), CenDEF (ALB26780.1), CenDEF3 (AFH66787.1), OitaDEF1 (BAO00916.1), OitaDEF2 (BAO00917.1), OitaDEF3 (BAO00918.1), MaMADS16 (XP_009418316.1), and PdMADS16 (XP_008781489.1). As shown in Figure 1C, EgMADS16 was closest to PdMADS16 (91.56% identity), AcMADS16 (87.56% identity), and MaMADS16 (84.89% identity).
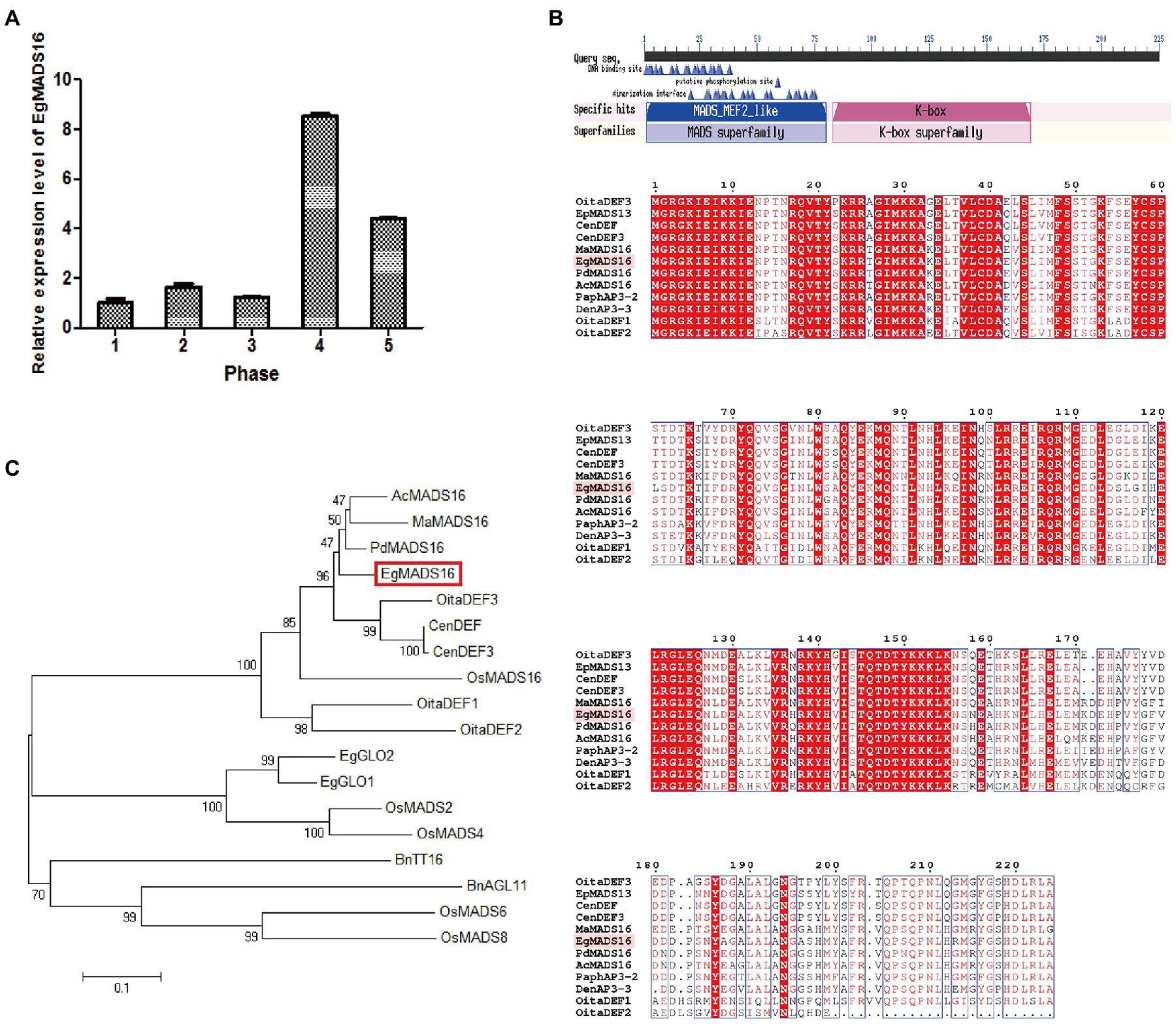
Figure 1. The expression patterns and sequence analysis of EgMADS16. (A) The relative expression level of EgMADS16 in different developmental stages of oil palm fruit mesocarp. Phase 1–5: fruits 30–60 days, 60–100 days, 100–120 days, 120–140 days, and 140–160 days after pollination. The values are the means±SD (n = 3). (B) Conserved domains analysis of EgMADS16 protein. (C) The phylogenetic tree of EgMADS16 and other MADS proteins, including EgGLO1 (XP_010911271.1), EgGLO2 (AAQ03229.1), OsMADS2 (XP_015623988.1), OsMADS4 (XP_015640709.1), OsMADS6 (XP_015623947.1), OsMADS8 (XP_015610824.1), OsMADS16 (XP_015641661.1), AcMADS16 (XP_020082339.1), BnAGL11 (XP_013719732.1), BnTT16 (NP_001303188.1), CenDEF (ALB26780.1), CenDEF3 (AFH66787.1), OitaDEF1 (BAO00916.1), OitaDEF2 (BAO00917.1), OitaDEF3 (BAO00918.1), MaMADS16 (XP_009418316.1), and PdMADS16 (XP_008781489.1).
Moreover, to identify whether EgMADS16 localized to the nucleus, similar to other transcription factors, we conducted a subcellular localization test in tobacco leaf epidermal cells, and the results indicated that the EgMADS16-GFP fusion protein was localized in the nucleus (Figure 2).
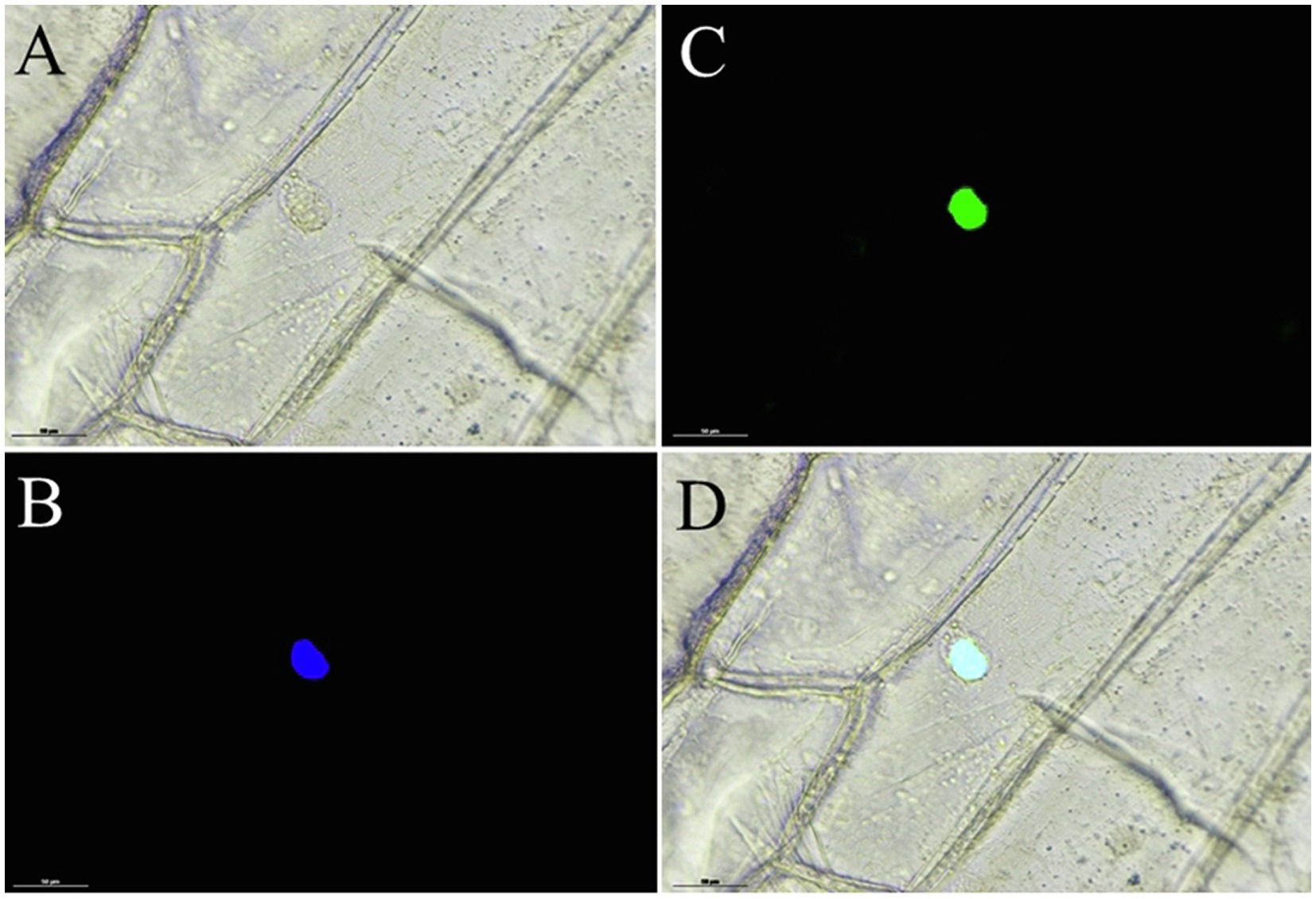
Figure 2. Subcellular localization of EgMADS16 protein in onion epidermal cells. (A) Bright light. (B) DAPI. (C) EgMADS-GFP fluorescence. (D) Merged image. Scale bar, 50 μm.
Verification of the Target Relationship Between EgmiR5179 and EgMADS16
According to previous research, OitaDEF2 is the target of OitamiR5179 in Orchid orchis italics (Aceto et al., 2014). Likewise, CenDEF3 is the target of CenmiR5179 in Cymbidium ensifolium (Li et al., 2015). Therefore, small RNA target analysis (see footnote 3) suggested that EgMADS16 is targeted by EgmiR5179. To verify this target relationship, we performed a dual-luciferase reporter assay in Arabidopsis and oil palm protoplasts. The results indicated that the relative luciferase activity of EgMADS16-LUC-transfected protoplasts was decreased with the expression of EgmiR5179 (Figures 3A,B). At the same time, we transformed either or both EgmiR5179-SK and EgMADS16-LUC into oil palm protoplasts, and then, the relative expression level of EgMADS16 was detected by qPCR. The results were consistent with the results of the dual-luciferase reporter assay in which the EgMADS16 expression level was downregulated by EgmiR5179 (Figure 3C).
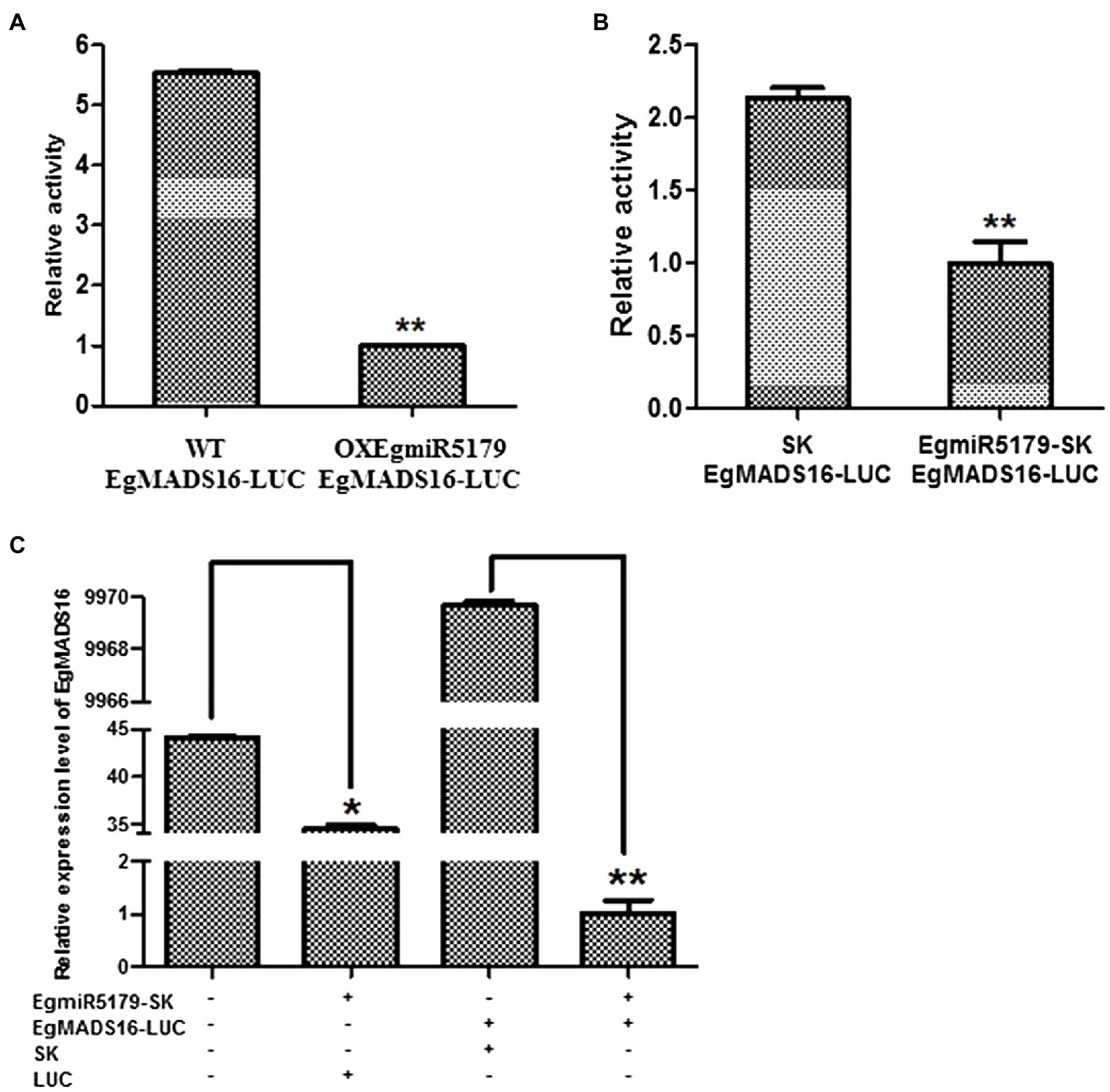
Figure 3. EgMADS16 is the target gene of EgmiR5179. (A) Dual-luciferase reporter assay in Arabidopsis protoplasts. EgMADS16-LUC was transformed into wild-type and OXEgmiR5179 Arabidopsis protoplasts, respectively. (B) Dual-luciferase reporter assay in oil palm protoplasts. EgMADS16-LUC and SK or EgmiR5179-SK were transformed into oil palm protoplasts, respectively. (C) The relative expression level of EgMADS16 in oil palm protoplasts that transformed either or both of EgmiR5179 and EgMADS16. The values are the means ± SD (n = 3), “*” represents a significant difference (p < 0.05), and “**” represents a highly significant difference (p < 0.01) using Student’s t-test.
Effect of EgMADS16 on Lipid Biosynthesis
To characterize the role of EgMADS16 in lipid biosynthesis, we obtained EgMADS16-overexpressing Arabidopsis lines (OXEgMADS16 Line A and Line B; Figure 4A) and oil palm embryonic callus lines (OXEgMADS16 Line 1 and Line 2; Figure 5A). Compared with the wild type, all OXEgMADS16 lines had higher EgMADS16 expression. Meanwhile, the relative fatty acid contents of OXEgMADS16 Arabidopsis seeds and oil palm embryonic calli were tested by GC. The results showed that the C18:0 and C18:3 contents of the OXEgMADS16 lines significantly increased, while the C18:1 content decreased compared with that of the wild type (Figures 4D, 5D). In addition, there were significant decreases in the lipid contents of both OXEgMADS16 Arabidopsis seeds and oil palm embryonic calli (Figures 4C, 5C).
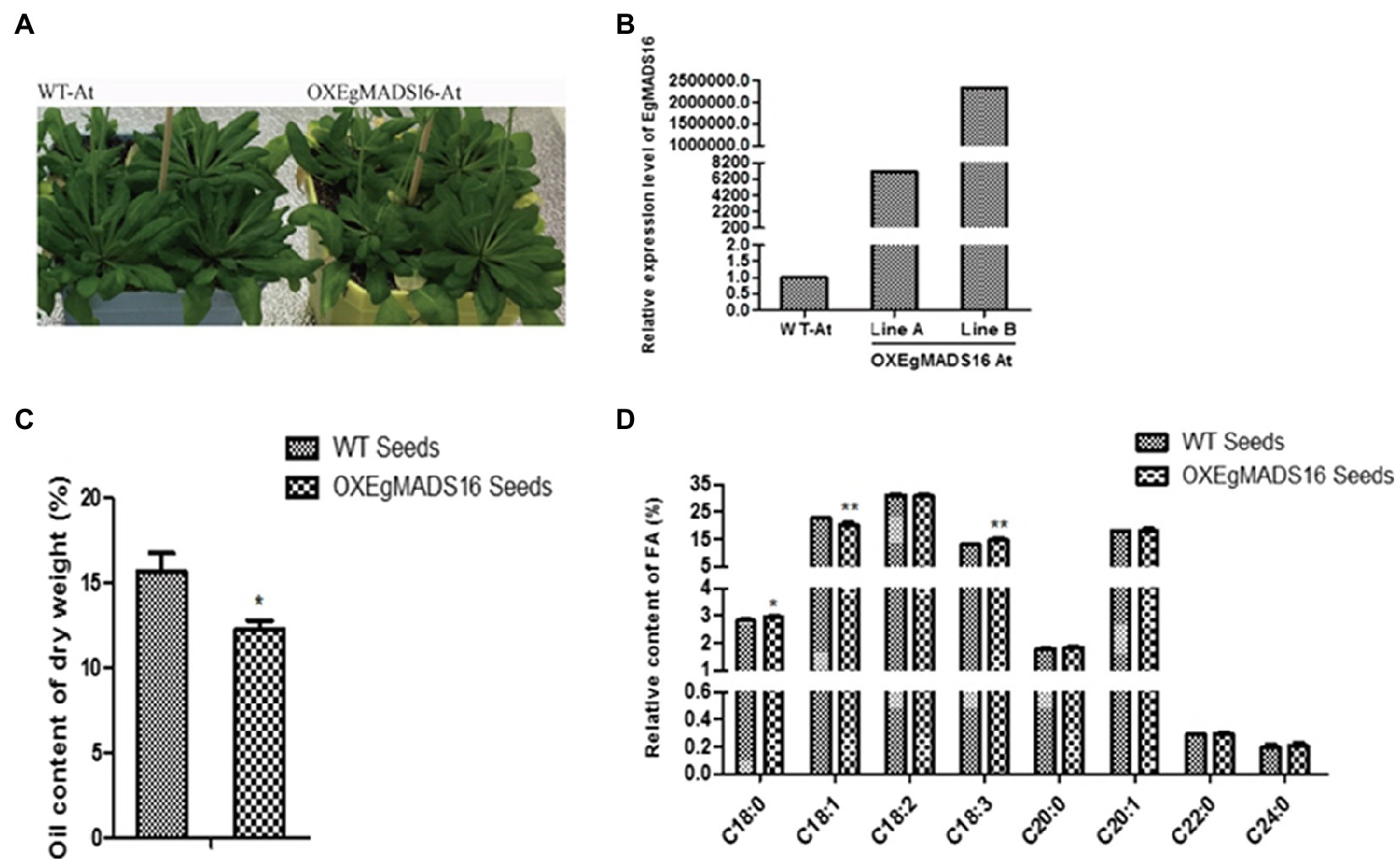
Figure 4. Effect of EgMADS16 on lipid biosynthesis in oil palm embryonic callus. (A) Wild-type oil palm (WT-OP) embryonic callus and EgMADS16 overexpression oil palm embryonic callus (OXEgMADS16 OP) on screening medium containing glufosinate ammonium. Scale bar, 3 mm. (B) The relative expression level of EgMADS16 in WT-OP and OXEgMADS16 OP. (C) The oil content of WT and OXEgMADS16 oil palm embryonic callus. (D) The relative FA content of WT and OXEgMADS16 oil palm embryonic callus. The values are the means ± SD (n = 3), “*” represents a significant difference (p < 0.05), and “**” represents a highly significant difference (p < 0.01) using Student’s t-test.
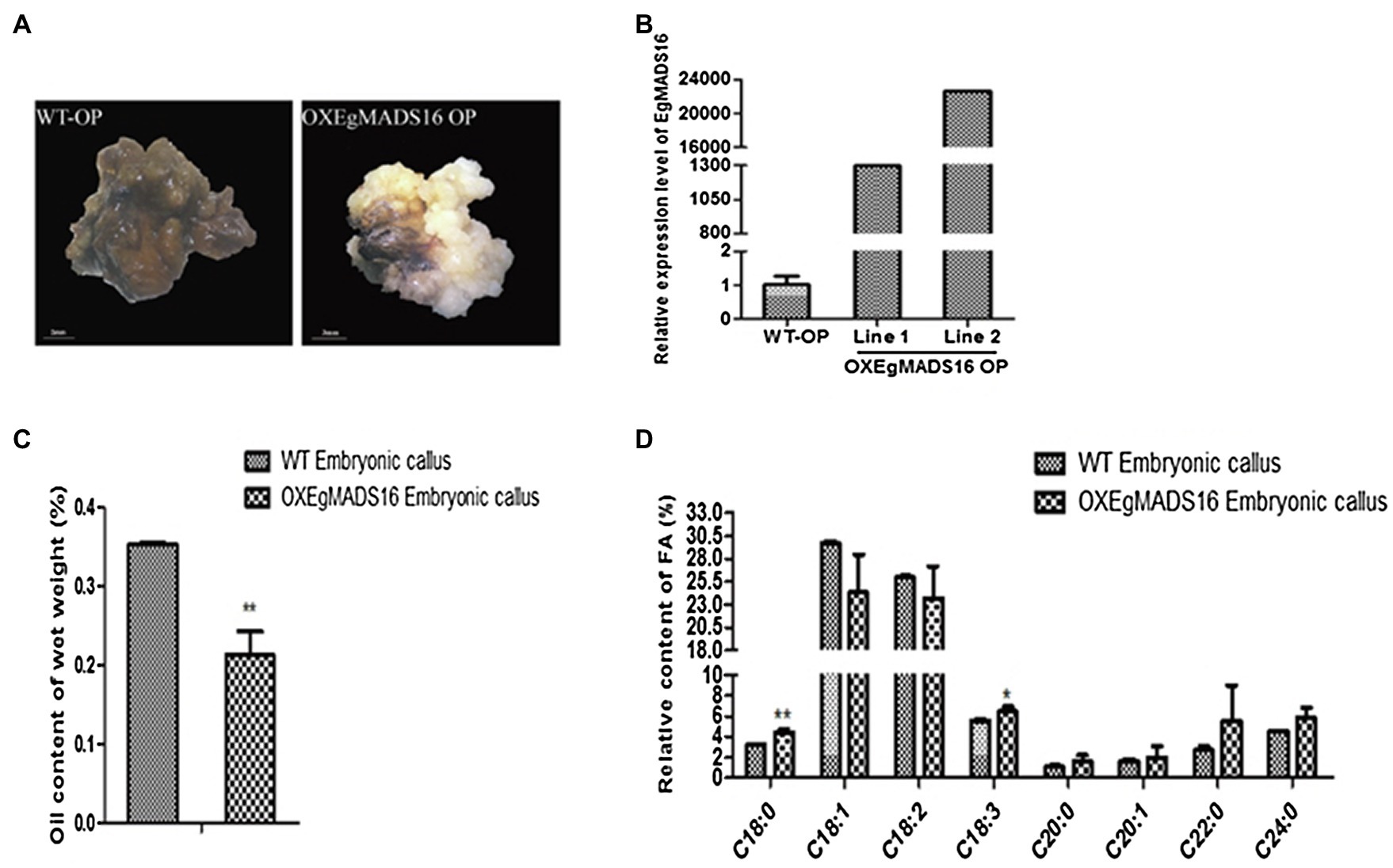
Figure 5. Effect of EgMADS16 on lipid biosynthesis in Arabidopsis. (A) Wild-type Arabidopsis (WT-At) and EgMADS16 overexpression Arabidopsis (OXEgMADS16 At). (B) The relative expression level of EgMADS16 in WT-At and OXEgMADS16 At. (C) The oil content of WT and OXEgMADS16 Arabidopsis seeds. (D) The relative FA content of WT and OXEgMADS16 Arabidopsis seeds. The values are the means ± SD (n = 3), “*” represents a significant difference (p < 0.05), and “**” represents a highly significant difference (p < 0.01) using Student’s t-test.
Regulation of EgMADS16 in Lipid Biosynthesis by EgmiR5179
Based on the predicted targeting regulatory relationship, EgmiR5179 should regulate the expression of EgMADS16 and thus play a role in lipid biosynthesis. To verify this hypothesis, we generated transgenic Arabidopsis plants that coexpressed EgmiR5179 and EgMADS16 (OXEgmiR5179-EgMADS16; Figure 6A) and analyzed the FA and oil contents. As expected, EgmiR5179 weakened the inhibitory effect of EgMADS16 on oil contents (Figure 6B). Additionally, the relative contents of C18:0 and C18:3 in OXEgmiR5179-EgMADS16 seeds decreased compared with those of the OXEgMADS16 seeds, while those in OXEgMADS16 seeds rose compared with WT seeds. The C18:1 content of OXEgmiR5179-EgMADS16 seeds increased compared with that of OXEgMADS16 seeds, while that of OXEgMADS16 seeds decreased compared with that of WT seeds (Figure 6C).
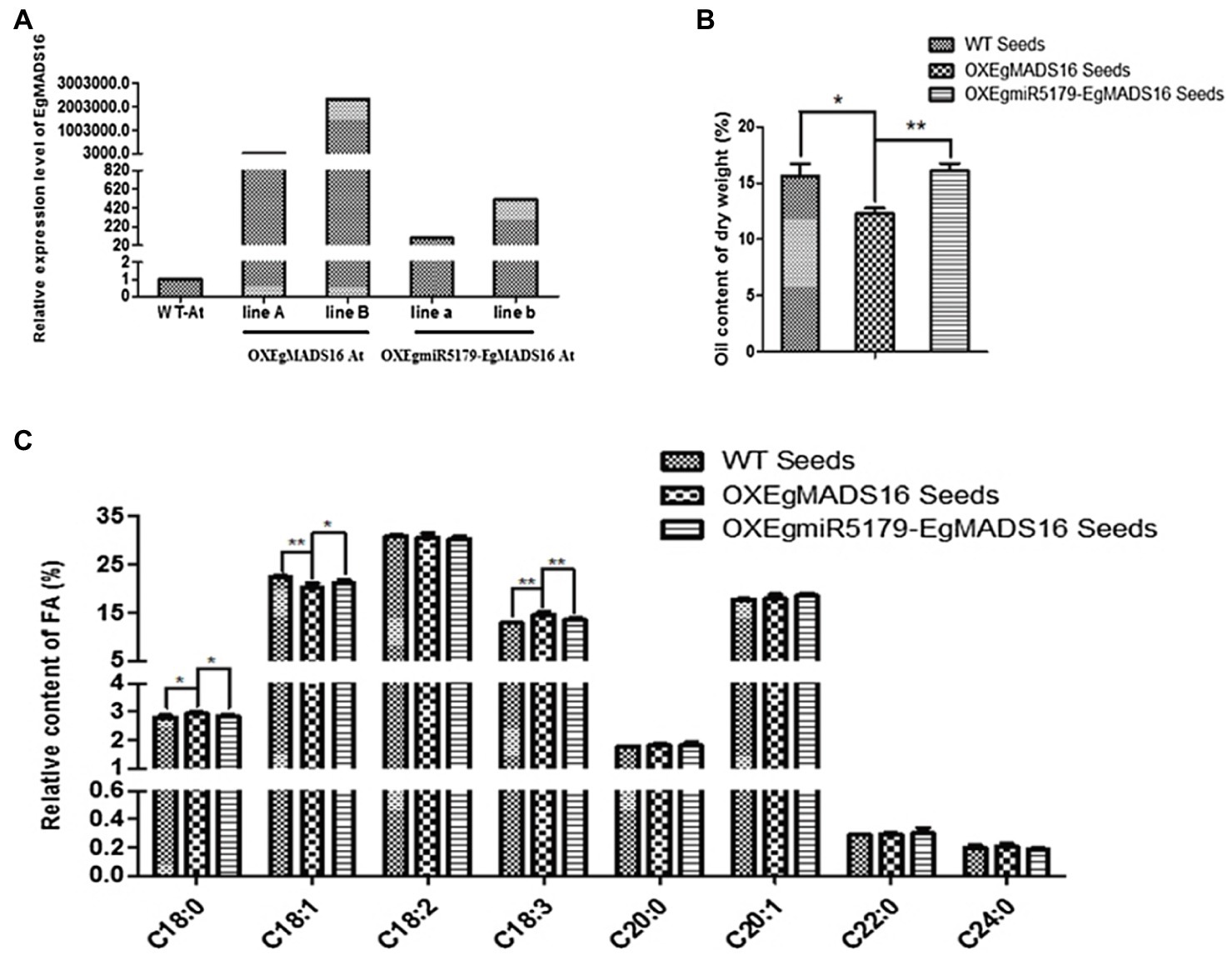
Figure 6. The effect of EgMADS16 on lipid biosynthesis is inhibited by EgmiR5179 in Arabidopsis. (A) The relative expression level of EgMADS16 in wild-type and transgenic Arabidopsis. (B) The oil content of wild-type and transgenic Arabidopsis seeds. (C) The relative FA content of wild-type and transgenic Arabidopsis seeds. The values are the means ± SD (n = 3), “*” represents a significant difference (p < 0.05), and “**” represents a highly significant difference (p < 0.01) using Student’s t-test. OXEgmiR5179-EgMADS16 At: Arabidopsis coexpressed EgmiR5179 and EgMADS16.
Identification of the Downstream Genes of EgMADS16
EgMADS16 belongs to MADS-box family. This means that it is likely to function as a transcription factor to regulate lipid biosynthesis by regulating lipid biosynthesis-related genes. Therefore, we selected seven related genes according to the results of fatty acid compositions and contents of OXEgMADS lines, and we examined the expression level of these genes in OXEgMADS oil palm embryonic callus by qPCR. As a result, the expression levels of EgFAD2, EgDGAT2, and EgSAD significantly declined, while those of EgFAD7, EgFAD6, EgLACS9, and EgDGAT1 did not significantly change (Figures 7A–G).
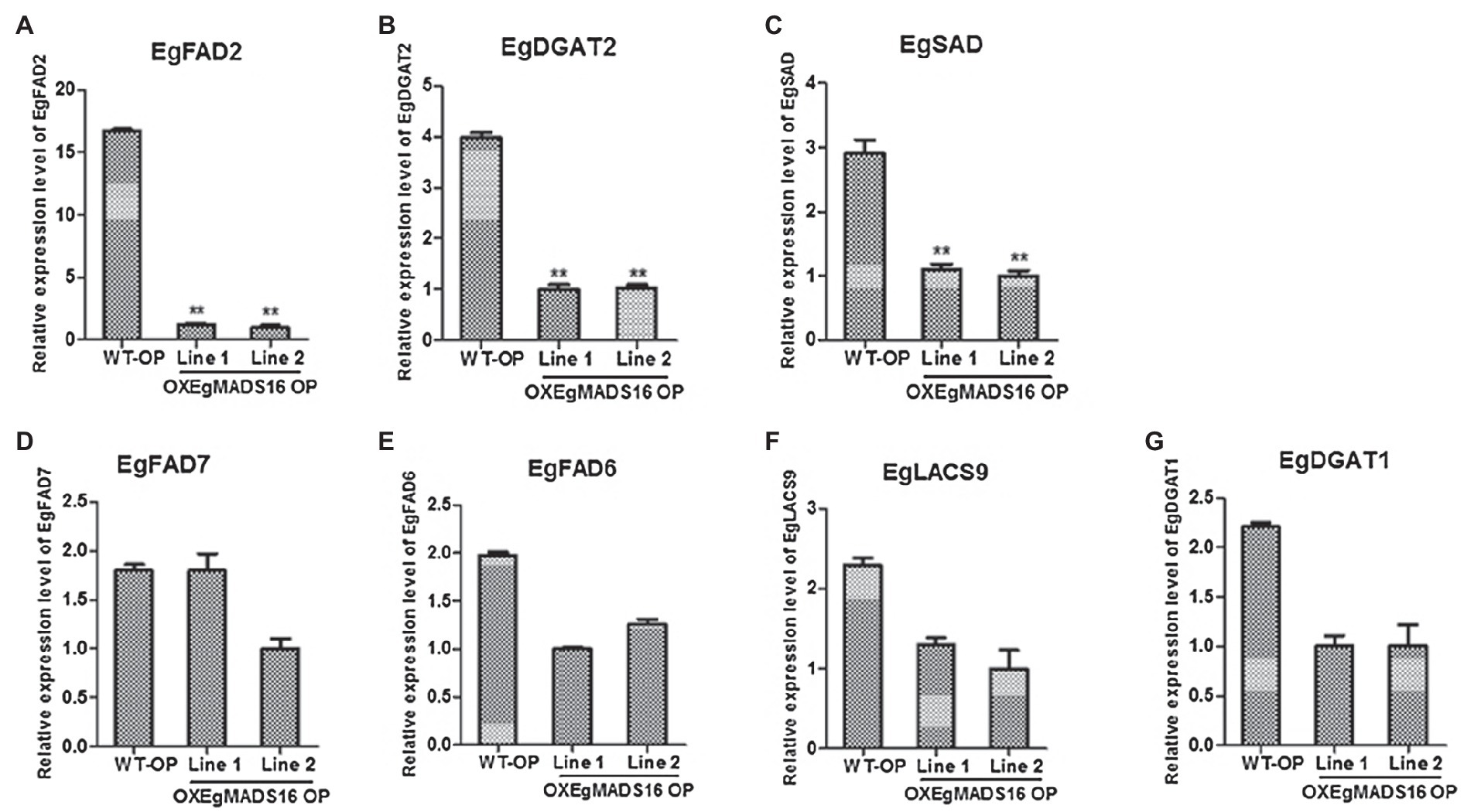
Figure 7. Identification of downstream genes of EgMADS16 protein. (A–G) The relative expression level of EgFAD2 (A), EgDGAT2 (B), EgSAD (C), EgFAD7 (D), EgFAD6 (E), EgLACS9 (F), and EgDGAT1 (G) in WT and OXEgMADS16 oil palm embryonic callus. The values are the means ± SD (n = 3). “**” represents a highly significant difference (p < 0.01) using Student’s t-test.
To further investigate whether EgMADS16 protein regulates the expression of EgFAD2, EgDGAT2, and EgSAD by binding to their promoters, we conducted yeast one-hybrid assays. However, interactions between EgMADS16 and the promoters of EgFAD2, EgDGAT2, or EgSAD were not detected (Supplementary Figure 1), suggesting that EgMADS16 downregulated these genes by interacting with other DNA-binding transcription factors rather than directly binding to their promoters.
EgMADS16 Binds to EgGLO1 Proteins
Next, we used yeast two-hybrid assays to identify the transcription factors that interact with EgMADS16 proteins. Considering the activation domains of transcription factors, we tested the autoactivation of EgMADS16 on yeast reporters. The results showed that EgMADS16 proteins have an activation domain, but Aba can inhibit its autoactivation (Supplementary Figure 2). Therefore, we performed yeast two-hybrid assays and found that there was an interaction between EgMADS16 and EgGLO1 (XP_010911271.1) proteins (Figure 8A). To further verify this interaction, BiFC was used. YFP protein fluorescence was observed only when EgMADS16-pSPYNE and EgGLO1-pSPYCE were coinfiltrated into the leaves of N. benthamiana (Figure 8B), suggesting that EgMADS16 binds to the EgGLO1 proteins.
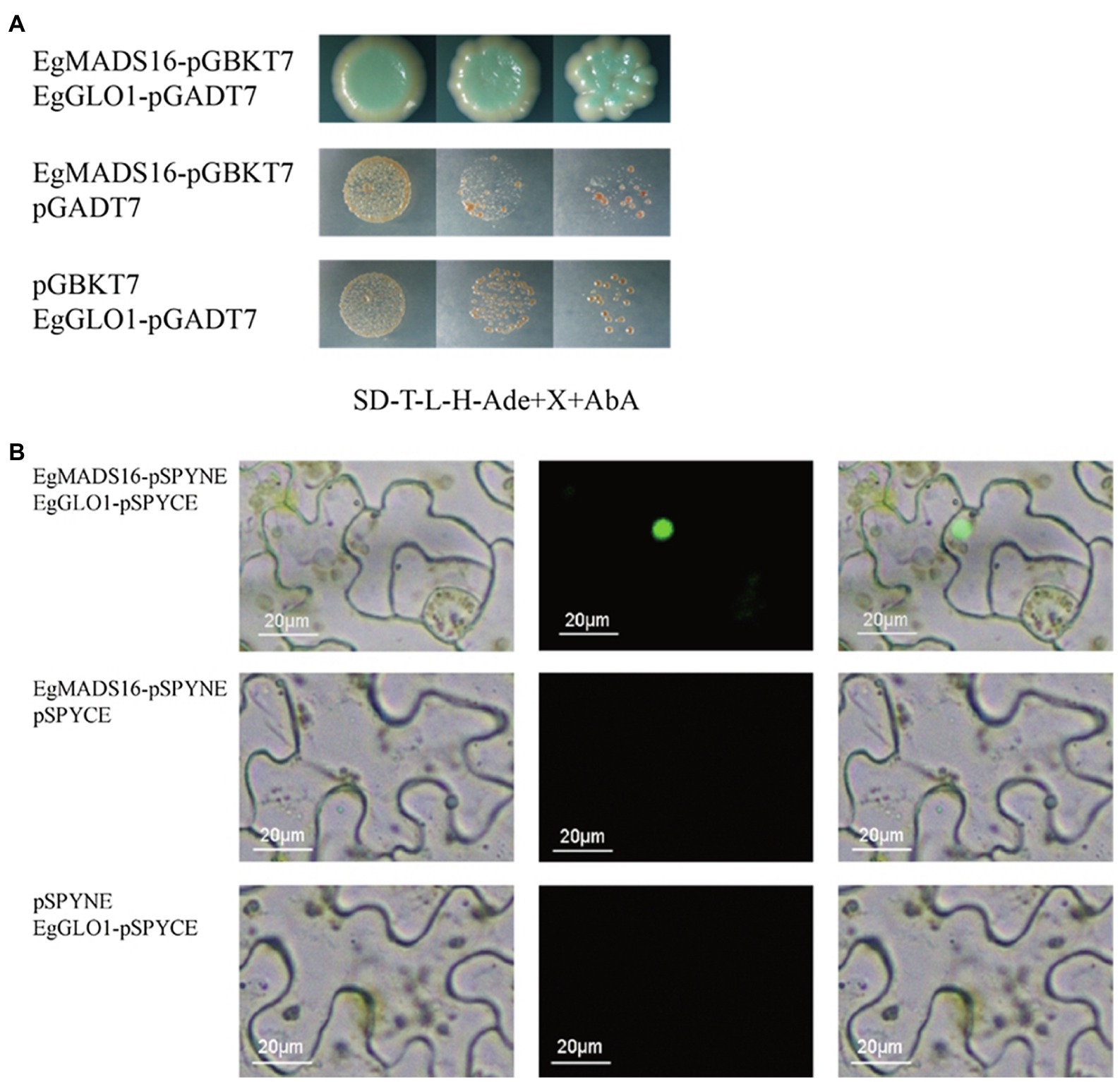
Figure 8. EgMADS16 protein binds to EgGLO1 protein. (A) Yeast two-hybrid assay of EgMADS16 protein and EgGLO1 protein. (B) BiFC of EgMADS16 protein and EgGLO1 protein. Scale bar, 20 μm.
Discussion
In this paper, the role of the EgMADS16 gene in oil biosynthesis was studied. Among the oil palm fruits at five different developmental stages, the EgMADS16 gene had the highest transcription level in the fourth stage and decreased in the fifth stage. The phylogenetic tree analysis showed that PdMADS16 had the closest relationship with EgMADS16, followed by AcMADS16 and MaMADS16, but their functions have not been reported. The subcellular localization results showed that EgMADS16 protein was located in the nucleus, which is consistent with the localization of other transcription factors. Previous studies found that MADS-box genes were closely related to plant growth and development. In oil palm, it has been proven that the MADS-box gene EgSQUA1 is related to the height of the plant and the length of pedicels and siliques; EgGLO2 promotes a partial conversion of sepals to petals in whorl 1; and EgAGL2-1 is involved in stamens and gynoecium development (Adam et al., 2007). However, the overexpression of EgMADS16 (EgDEF1) did not change the phenotype of flowers (Adam et al., 2007) but participated in the biosynthesis of oil and fatty acids, which was demonstrated by the significant increases in C18:0 and C18:3 contents and the decreases in C18:1 content and total oil yield (Figures 4, 5).
As a transcription factor, EgMADS16 likely participates in the regulation of oil biosynthesis in the same way. According to the fatty acid compositions and contents of OXEgMADS lines, EgSAD, EgFAD2, EgFAD6, EgFAD7, EgLACS9, EgDGAT1, and EgDGAT2 were selected as candidate genes, and qRT-PCR was used to detect the expression levels of these seven oil biosynthesis-related genes in OXEgMADS16 oil palm embryonic calli. As a result, the transcription levels of EgSAD, EgFAD2, and EgDGAT2 were significantly suppressed (Figures 7A–C). This result indicates that EgMADS16 regulates the contents of fatty acids and oil by inhibiting the expression of EgSAD, EgFAD2, and EgDGAT2. To determine whether the EgMADS16 protein directly regulates the expression of these three genes by binding to their promoters, a yeast one-hybrid assay was conducted. However, the results showed that the EgMADS16 protein cannot directly bind to the promoters of EgSAD, EgFAD2, and EgDGAT2. This means that EgMADS16 protein likely inhibits the expression level of these three genes by interacting with an intermediate protein, which can directly bind to the promoters of EgSAD, EgFAD2, and EgDGAT2.
In addition, small RNA target analysis showed that EgMADS16 is targeted by EgmiR5179 (Supplementary Figure 3). Moreover, the dual-luciferase reporter assay and qRT-PCR in protoplasts further suggested this target relationship: EgmiR5179 can inhibit the expression of EgMADS16 (EgDEF1; Figure 3). The overexpression of EgmiR5179 significantly increased the total oil content in seeds (Gao et al., 2019). In this study, the overexpression of EgMADS16 significantly reduced the total oil content of Arabidopsis seeds and oil palm embryonic calli (Figures 4, 5), which is consistent with the role of EgMADS16 as the target gene of EgmiR5179. In addition, compared with OXMADS16 transgenic Arabidopsis seeds, the total oil content of OXEgmiR5179-EgMADS16 seeds increased significantly, C18:0 and C18:3 decreased, and C18:1 increased. More interestingly, this trend of change was opposite to the trend of oil and fatty acid contents in the seeds of OXMADS16 plants relative to the wild type (Figures 6B,C). This implies that EgmiR5179 can inhibit the regulation of EgMADS16 in the biosynthesis of oil and FAs and promote the accumulation of oil.
In previous studies, it has been proven that homologous complexes formed between MADS-box proteins to facilitate the binding of MADS-box proteins to DNA. For example, OsMADS16 protein interacts with OsMADS4, OsMADS6, and OsMADS8 proteins to regulate stamen development (Lee et al., 2003); GmMADS28 protein interacts with SOC1, AP1, and AGL8/FUL proteins to modulate floral organ number, petal identity, and sterility (Huang et al., 2014). Similarly, the results of yeast two-hybrid assays and BiFC suggested that there was an interaction between the EgMADS16 protein and EgGLO1 protein (Figure 8). EgMADS16 proteins regulate oil biosynthesis by interacting with the EgGLO1 proteins, which directly bind to the promoters of oil biosynthesis-related genes. However, there is no interaction between the OsMADS16 protein and OsMADS2 (Lee et al., 2003), which are homologs of EgMADS16 and EgGLO1. In addition, this may be due to species differences.
In summary, we are hypothesizing a model of the molecular mechanism by which EgMADS16 regulates oil biosynthesis (Figure 9). After EgMADS16 is translated, the EgMADS16 protein forms a complex with EgGLO1 or other proteins and binds to the promoter of EgFAD2, EgSAD, EgDGAT2, or other oil biosynthesis-related genes, inhibiting the transcription of these genes and affecting fatty acid components and oil accumulation. However, when EgmiR5179 is overexpressed, the EgmiR5179 mature sequence binds to EgMADS16 mRNA and inhibits its expression so that the transcription of downstream oil biosynthesis-related genes cannot be inhibited by EgMADS16 protein.
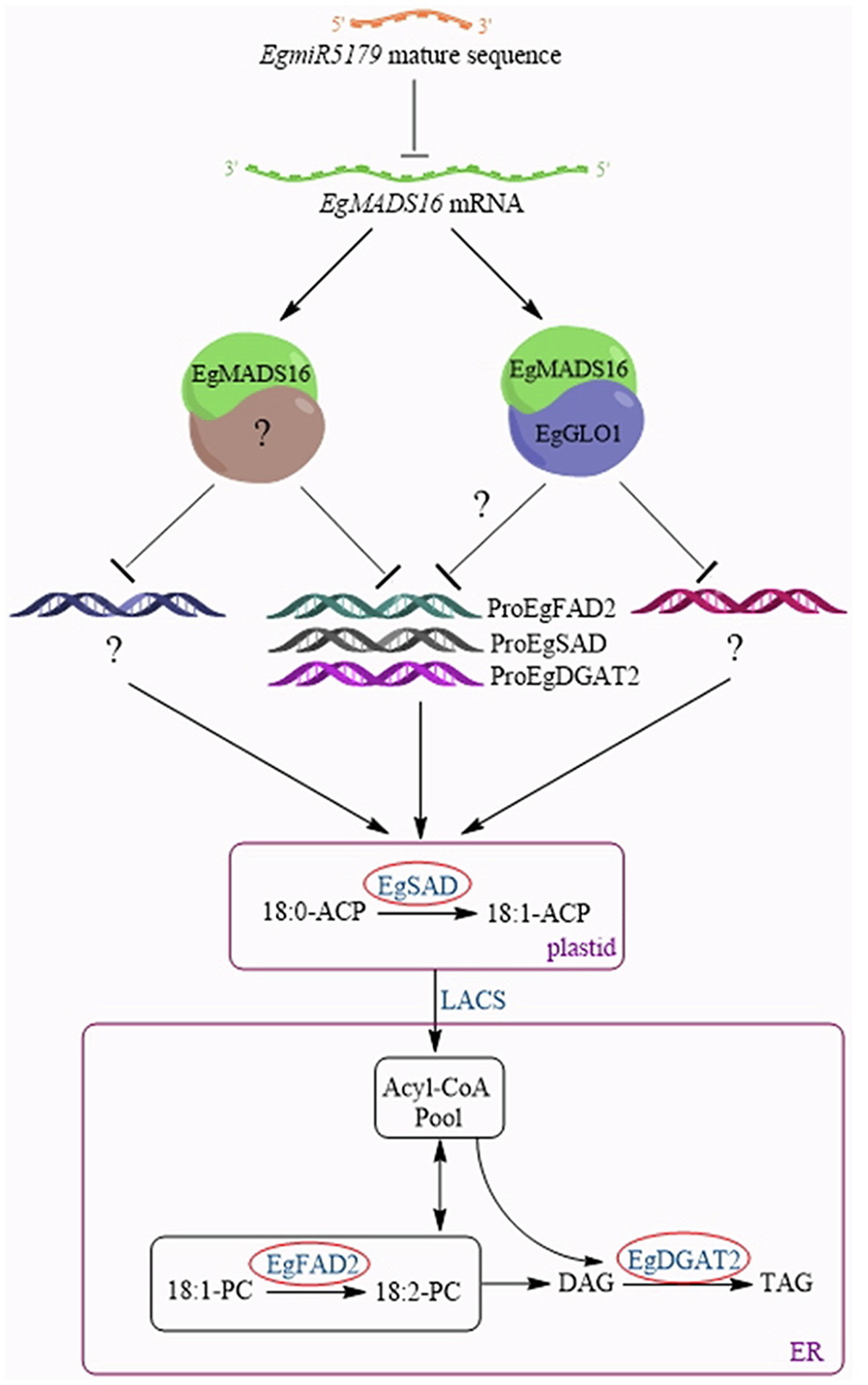
Figure 9. A model about the molecular mechanism of EgMADS16 regulating oil biosynthesis. EgMADS16 protein forms a complex with EgGLO1 or other proteins and binds to the promoter of EgFAD2 or EgSAD or EgDGAT2 or other oil biosynthesis-related genes, inhibiting the transcription of these genes and affecting fatty acid components and oil accumulation. However, EgmiR5179 targets to EgMADS16 mRNA and inhibits its expression, so that the oil accumulation is promoted.
Nevertheless, the intermediate proteins regulated by EgMADS16 proteins for the transcription of EgSAD, EgFAD2, and EgDGAT2 have yet to be studied. It is unclear which oil biosynthesis-related genes, the EgMADS16/EgGLO1 complex, regulate. Nevertheless, the molecular mechanism presented in this paper provides a preliminary understanding of the regulation of oil biosynthesis by transcription factors in oil palm, which lays the foundation for further research and provides a strategy for obtaining a higher yield of oil palm in the future.
Data Availability Statement
The datasets presented in this study can be found in online repositories. The names of the repository/repositories and accession number(s) can be found in the article/Supplementary Material.
Author Contributions
DL and YZ designed the research. YW, JZo, and JZh performed the research. YZ and DL wrote the paper. All authors read and approved the final manuscript.
Funding
This research was supported by the Hainan Provincial Natural Science Foundation of China (No. 2019CXTD397), the National Natural Science Foundation of China (NSFC; No. 31660222), and the National Key R&D Program of China (2018YFD1000500).
Conflict of Interest
The authors declare that the research was conducted in the absence of any commercial or financial relationships that could be construed as a potential conflict of interest.
Publisher’s Note
All claims expressed in this article are solely those of the authors and do not necessarily represent those of their affiliated organizations, or those of the publisher, the editors and the reviewers. Any product that may be evaluated in this article, or claim that may be made by its manufacturer, is not guaranteed or endorsed by the publisher.
Supplementary Material
The Supplementary Material for this article can be found online at: https://www.frontiersin.org/articles/10.3389/fpls.2021.722596/full#supplementary-material
Abbreviations
AP2, APETALA2; AS, acetosyringone; BiFC, bimolecular fluorescence complementation; BR, biological reagent; CDSs, coding region sequences; CoA, coenzyme A; DAP, days after pollination; DAPI, 4', 6-diamidino-2-phenylindole; DGAT, diacylglycerol acyltransferase; FA, fatty acid; FAD, fatty acid dehydrogenase; FAME, fatty acid methyl ester; GC, gas chromatography; GFP, green fluorescent protein; LACS, long-chain acyl-CoA synthetase; MS medium, Murashige and Skoog medium; OD, optical density; OX, over-expressed; PEG, polyethylene glycol; qPCR, quantitative real-time PCR; SAD, stearoyl-ACP desaturase; YFP, yellow fluorescent protein.
Footnotes
1. ^https://www.ncbi.nlm.nih.gov/Structure/cdd/wrpsb.cgi
2. ^http://www.ebi.ac.uk/Tools/msa/clustalo/
3. ^http://plantgrn.noble.org/psRNATarget/analysis?function=3
References
Aceto, S., Sica, M., De Paolo, S., D’argenio, V., Cantiello, P., Salvatore, F., et al. (2014). The analysis of the inflorescence miRNome of the orchid orchis italica reveals a DEF-like MADS-box gene as a new miRNA target. PLoS One 9:e97839. doi: 10.1371/journal.pone.0097839
Adam, H., Jouannic, S., Orieux, Y., Morcillo, F., Richaud, F., Duval, Y., et al. (2007). Functional characterization of MADS box genes involved in the determination of oil palm flower structure. J. Exp. Bot. 58, 1245–1259. doi: 10.1093/jxb/erl263
Bhagya, H. P., Kalyana Babu, B., Gangadharappa, P. M., Naika, M. B. N., Satish, D., and Mathur, R. K. (2020). Identification of QTLs in oil palm (Elaeis guineensis Jacq.) using SSR markers through association mapping. J. Genet. 99:19. doi: 10.1007/s12041-020-1180-4
Dyer, J. M., Stymne, S., Green, A. G., and Carlsson, A. S. (2008). High-value oils from plants. Plant J. 54, 640–655. doi: 10.1111/j.1365-313X.2008.03430.x
Gao, L.-C., Wang, Y.-F., Zhu, Z., Chen, H., Sun, R.-H., Zheng, Y.-S., et al. (2019). EgmiR5179 from the mesocarp of oil palm (Elaeis guineensis Jacq.) regulates oil accumulation by targeting NAD transporter 1. Ind. Crop. Prod. 137, 126–136. doi: 10.1016/j.indcrop.2019.05.013
Huang, F., Xu, G., Chi, Y., Liu, H., Xue, Q., Zhao, T., et al. (2014). A soybean MADS-box protein modulates floral. BMC Plant Biol. 14, 1–4. doi: 10.1186/1471-2229-14-89
Jessen, D., Roth, C., Wiermer, M., and Fulda, M. (2015). Two activities of long-chain acyl-coenzyme a synthetase are involved in lipid trafficking between the endoplasmic reticulum and the plastid in Arabidopsis. Plant Physiol. 167, 351–366. doi: 10.1104/pp.114.250365
Jin, Y., Yuan, Y., Gao, L., Sun, R., Chen, L., Li, D., et al. (2017). Characterization and functional analysis of a type 2 diacylglycerol acyltransferase (DGAT2) gene from oil palm (Elaeis guineensis jacq.) mesocarp in saccharomyces cerevisiae and transgenic Arabidopsis thaliana. Front. Plant Sci. 8:1791. doi: 10.3389/fpls.2017.01791
Lee, S., Jeon, J.-S., An, K., Moon, Y.-H., Lee, S., Chung, Y.-Y., et al. (2003). Alteration of floral organ identity in rice through ectopic expression of OsMADS16. Planta 217, 904–911. doi: 10.1007/s00425-003-1066-8
Li, X., Jin, F., Jin, L., Jackson, A., Ma, X., Shu, X., et al. (2015). Characterization and comparative profiling of the small RNA transcriptomes in two phases of flowering in Cymbidium ensifolium. BMC Genomics 16:622. doi: 10.1186/s12864-015-1764-1
Li, S. Y., Zhang, Q., Jin, Y. H., Zou, J. X., Zheng, Y. S., and Li, D. D. (2020). A MADS-box gene, EgMADS21, negatively regulates EgDGAT2 expression and decreases polyunsaturated fatty acid accumulation in oil palm (Elaeis guineensis Jacq.). Plant Cell Rep. 39, 1505–1516. doi: 10.1007/s00299-020-02579-z
Liu, Y.-F., Li, Q.-T., Lu, X., Song, Q.-X., Lam, S.-M., Zhang, W.-K., et al. (2014). Soybean GmMYB73 promotes lipid accumulation in transgenic plants. BMC Plant Biol. 14:73. doi: 10.1186/1471-2229-14-73
Masani, M. Y., Noll, G. A., Parveez, G. K., Sambanthamurthi, R., and Prufer, D. (2014). Efficient transformation of oil palm protoplasts by PEG-mediated transfection and DNA microinjection. PLoS One 9:e96831. doi: 10.1371/journal.pone.0096831
Ohlroggeav, J., and Browse, J. (1995). Lipid biosynthesis. Plant Cell 7, 957–970. doi: 10.1105/2Ftpc.7.7.957
Osorio-Guarin, J. A., Garzon-Martinez, G. A., Delgadillo-Duran, P., Bastidas, S., Moreno, L. P., Enciso-Rodriguez, F. E., et al. (2019). Genome-wide association study (GWAS) for morphological and yield-related traits in an oil palm hybrid (Elaeis oleifera × Elaeis guineensis) population. BMC Plant Biol. 19:533. doi: 10.1186/s12870-019-2153-8
Shen, B., Allen, W. B., Zheng, P., Li, C., Glassman, K., Ranch, J., et al. (2010). Expression of ZmLEC1 and ZmWRI1 increases seed oil production in maize. Plant Physiol. 153, 980–987. doi: 10.1104/pp.110.157537
Song, Q.-X., Li, Q.-T., Liu, Y.-F., Zhang, F.-X., Ma, B., Zhang, W.-K., et al. (2013). Soybean GmbZIP123 gene enhances lipid content in the seeds of transgenic Arabidopsis plants. J. Exp. Bot. 64, 4329–4341. doi: 10.1093/jxb/ert238
Tranbarger, T. J., Dussert, S., Joet, T., Argout, X., Summo, M., Champion, A., et al. (2011). Regulatory mechanisms underlying oil palm fruit mesocarp maturation, ripening, and functional specialization in lipid and carotenoid metabolism. Plant Physiol. 156, 564–584. doi: 10.1104/pp.111.175141
Yeap, W. C., Lee, F. C., Shabari Shan, D. K., Musa, H., Appleton, D. R., and Kulaveerasingam, H. (2017). WRI1-1, ABI5, NF-YA3 and NF-YC2 increase oil biosynthesis in coordination with hormonal signaling during fruit development in oil palm. Plant J. 91, 97–113.
Yoo, S. D., Cho, Y. H., and Sheen, J. (2007). Arabidopsis mesophyll protoplasts: a versatile cell system for transient gene expression analysis. Nat. Protoc. 2, 1565–1572. doi: 10.1038/nprot.2007.199
Yuan, Y. J., Gao, L. C., Sun, R. H., Yu, T., Liang, Y. X., Li, D. D., et al. (2017). Seed-specific expression of an acyl-acyl carrier protein thioesterase CnFatB3 from coconut (Cocos nucifera L.) increases the accumulation of medium-chain fatty acids in transgenic Arabidopsis seeds. Sci. Hortic. 223, 5–9. doi: 10.1016/j.scienta.2017.05.029
Keywords: oil palm, MADS-box gene, micorRNA5179, lipid content, fatty acid
Citation: Wang Y, Zou J, Zhao J, Zheng Y and Li D (2021) EgmiR5179 Regulates Lipid Metabolism by Targeting EgMADS16 in the Mesocarp of Oil Palm (Elaeis guineensis). Front. Plant Sci. 12:722596. doi: 10.3389/fpls.2021.722596
Edited by:
María Serrano, Miguel Hernández University of Elche, SpainReviewed by:
Hadi Pirasteh-Anosheh, Agricultural Research Education and Extention Organization, IranBenjamin Lau, Malaysian Palm Oil Board, Malaysia
Copyright © 2021 Wang, Zou, Zhao, Zheng and Li. This is an open-access article distributed under the terms of the Creative Commons Attribution License (CC BY). The use, distribution or reproduction in other forums is permitted, provided the original author(s) and the copyright owner(s) are credited and that the original publication in this journal is cited, in accordance with accepted academic practice. No use, distribution or reproduction is permitted which does not comply with these terms.
*Correspondence: Yusheng Zheng, eXVzaGVuZy56aGVuZ0BoYWluYW51LmVkdS5jbg==; Dongdong Li, bGlkZEBoYWluYW51LmVkdS5jbg==
†These authors have contributed equally to this work