- Department of Plant Science, Macdonald Campus, McGill University, Montreal, QC, Canada
Cannabis has been legalized for recreational use in several countries and medical use is authorized in an expanding list of countries; markets are growing internationally, causing an increase in demand for high quality products with well-defined properties. The key compounds of Cannabis plants are cannabinoids, which are produced by stalked glandular trichomes located on female flowers. These trichomes produce resin that contains cannabinoids, such as tetrahydrocannabinolic acid and cannabidiolic acid, and an array of other secondary metabolites of varying degrees of commercial interest. While growers tend to focus on improving whole flower yields, our understanding of the “goldmines” of the plant – the trichomes – is limited despite their being the true source of revenue for a multi-billion-dollar industry. This review aims to provide an overview of our current understanding of cannabis glandular trichomes and their metabolite products in order to identify current gaps in knowledge and to outline future research directions.
Introduction
Trichomes are formed on the plant surface across a range of taxonomically disparate species, providing a variety of functions and benefits to the plant. These can include simple tasks, such as affecting leaf temperature and photosynthesis, or more complicated functions, such as pest-deterrence via their physical structures or production of compounds (Wagner, 1991; Hare et al., 2003). Glandular trichomes are of particular commercial interest as they are one of the key plant structures that produce essential oils – an industry valued at 18.62 billion USD in 2020 (Grand View Research, 2020). Other oil-producing plant structures are internal glands and other trichome types, some of which are capable of producing resinous secretions. Trichome morphology is highly variable both among plant species and within the plant itself (Sangwan et al., 2001). In Cannabis sativa L. (hereafter, cannabis), stalked glandular trichomes are the trichome morph that produces substances of economic value (Fairbairn, 1972; Sirikantaramas et al., 2005). These trichomes develop a secretory cavity between secretory disk cells and the cuticle where secondary metabolites, including cannabinoids and terpenes, are deposited and stored (Kim and Mahlberg, 1991, 1997; Sirikantaramas et al., 2005; Marks et al., 2009). Though there are a variety of other trichome morphs found across the cannabis plant, they are beyond the scope of this review.
While male plants produce small amounts of cannabinoids, in cannabis cultivation, the primary products are the female flowers clustered in inflorescences (Ohlsson et al., 1971). Stalked glandular trichomes are primarily concentrated on the calyces and bracts (Figure 1A; Spitzer-Rimon et al., 2019; Leme et al., 2020) with populations extending to the inflorescence “sugar leaves”; these are the sites of accumulation for secreted metabolic products. These valuable secretions include tetrahydrocannabinolic acid (THCA), cannabidiolic acid (CBDA), terpenes, and flavonoids (ElSohly and Slade, 2005; Flores-Sanchez and Verpoorte, 2008). Cannabis plant morphology and cannabinoid profiles are influenced by genetics and the cultivation environment, highlighting the importance of controlled conditions for cannabis cultivation (Magagnini et al., 2018; Danziger and Bernstein, 2021a, b). With the gradual global increase in social and legal acceptance of cannabis, there has been considerable interest in producing consistent high-quality yields. In addition, as medicinal uses for cannabinoids are supported by peer-reviewed research and clinical trials, the global demand for medicinal cannabis products will continue to increase. This will create further pressure on growers to improve control over the concentration of specific cannabis metabolites and the associated cannabis genotypes. However, the genotypes and environmental conditions needed to obtain this level of precision remain poorly characterized. Ultimately, these elusive methods need to be centered around trichomes as the “factories” of the plant. Current efforts have focused on the effects of breeding and cultivar selection, industrial growing conditions, and fertilization methods on flower yield and cannabinoid profiles (Vanhove et al., 2011; Campiglia et al., 2017; Tang et al., 2017; Hawley et al., 2018; Janatová et al., 2018; Burgel et al., 2020; Saloner and Bernstein, 2021). However, as undefined cannabis plant material in pioneering research papers formed the backbone for future cannabis/cannabinoid research, comparing data with uniform standards is impossible. Thus, the need for systematically validating results of these papers and cannabis production “folklore” is paramount yet challenging due to the impact of genotype and growing environment. Regardless of these challenges, since trichomes are ultimately responsible for yield and quality control, it is necessary to advance our understanding of how they, specifically, are affected by these efforts, as well as to investigate new approaches to broaden the scope of possible cost-effective applications for improving yield.
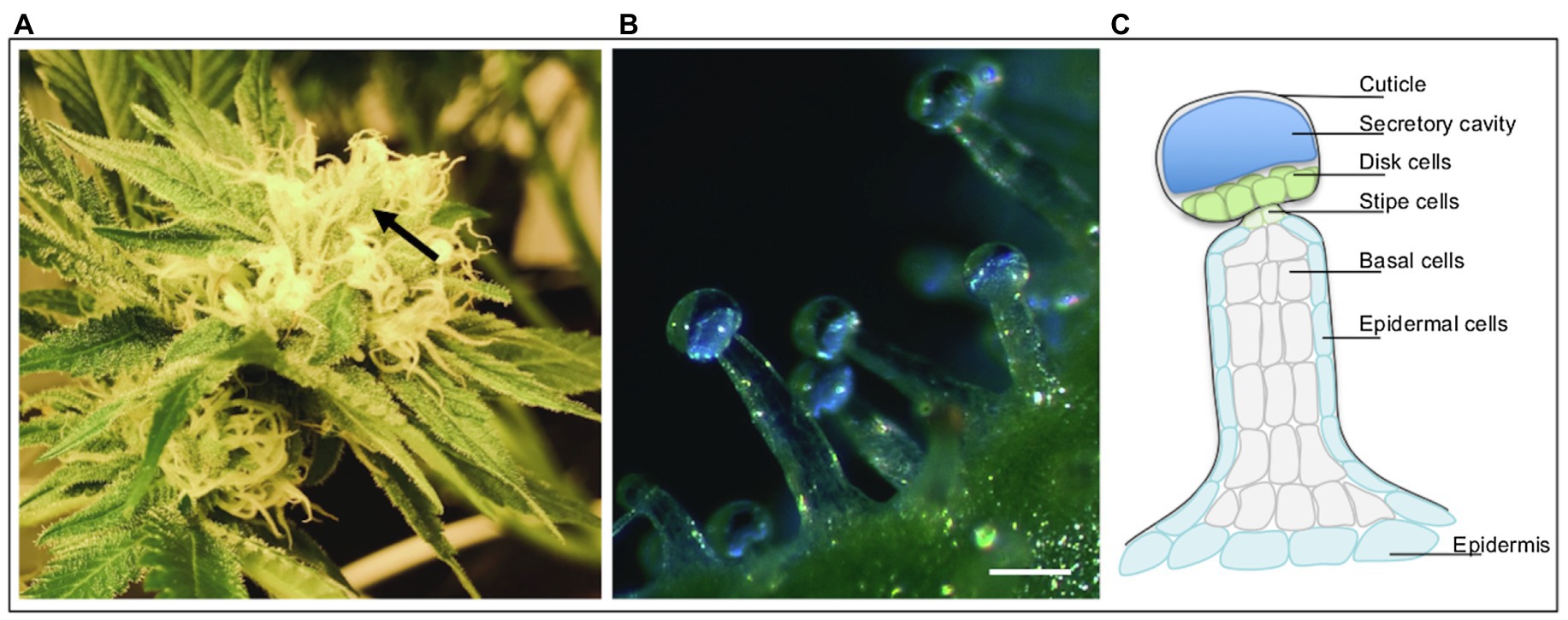
Figure 1. Cannabis (Cannabis sativa L.) inflorescence and trichomes. (A) An individual inflorescence, with majority of the organs covered in stalked glandular trichomes. Arrow indicates cluster of calyces and bracts covered with trichomes. (B) Dark field micrograph of stalked glandular trichomes protruding from calyx epidermis. Biosynthesis of secondary metabolites occurs in the secretory disk cells lining the base of the globular trichome head. The metabolites are stored in the clear subcuticular cavity above the secretory disk cells; this cavity will turn milky white to dark brown over the course of flower maturity. (C) Graphic illustration of stalked glandular trichome structure.
Trichome Profiles
Trichomes Across the Plant Kingdom
Trichomes are found across the plant kingdom, displaying a stunning variety of shapes and properties. Glandular trichomes, which arise from the epidermis on vegetative and reproductive organs, can be generally divided into secretory and non-secretory types with the former being able to secrete substances (Tian et al., 2017). Both the morphologies and metabolic secretions of trichomes are consistent within a plant species, and some species have different trichome morphs on the same plant organ (Muravnik, 2020). Secreted compounds, including THCA (Sirikantaramas et al., 2005), can be toxic to plant cells; therefore, metabolite storage in the cavity of the glandular head affords protection to the plant (Sirikantaramas et al., 2008). While different glandular trichome morphs invoke different storage strategies, the architecture of the morph and cavity position in relation to the secretory cells determine secretion direction (Tissier et al., 2017). The molecular details surrounding the development of glandular trichomes and their secretions are beyond the scope of this article, and we refer to in-depth reviews by Muravnik (2020) and Tian et al. (2017).
Genomic studies are imperative to investigate the factors that influence trichome development in cannabis, both within and between cultivars. Trichome differentiation mechanisms have been investigated in Arabidopsis thaliana, with transcription factor (TFs) groups playing key roles in the transcriptional networks for trichome production and patterns (Tian et al., 2017). While genomic studies are available for other economic plants, including Humulus lupulus which belongs to the Cannabaceae family (Matoušek et al., 2016; Mishra et al., 2020), similar studies for cannabis are lacking despite their potentially important impacts for precise cannabis trichome control. Taking advantage of the genetic libraries available for related species with similar resin secretions will help guide these much-need studies (Braich et al., 2019; Zager et al., 2019; Liu et al., 2021).
Key Cannabis Metabolites
The decades-long stigma surrounding cannabis has led to a variety of misconceptions surrounding the plant and its products regarding cannabinoid biosynthesis. While THCA and CBDA are the major cannabinoids produced by the plant, their degradation products, THC and CBD, are of great interest for their psychoactive and therapeutic effects. Additional cannabinoids are gradually gaining interest as their effects on the human body are beginning to be understood (ElSohly and Slade, 2005; Aizpurua-Olaizola et al., 2016; Andre et al., 2016). Fresh cannabis flower tissue contains relatively low levels of THC and CBD and higher levels of THCA and CBDA as the acid forms are converted to neutral forms via decarboxylation in post-harvest processing and storage; the rate of conversion is primarily dependent on temperature and light (Yamauchi et al., 1967). Metabolites, including cannabinoids, terpenes, and flavonoids, are formed within secretory disk cells that line the base of the glandular trichome head and stored in the subcuticular cavity (Figure 1B,C; Kim and Mahlberg, 1991, 1997). Within the cells, cannabinoid biosynthesis starts in the cytosol, moves to the plastid, and finishes with oxidocyclization in the apoplastic space; transport between these areas is not yet resolved (Gülck and Møller, 2020). A plethora of cannabinoids have been identified in recent years, bringing the total known number to just over 110, which can be divided into 11 subclasses (ElSohly and Gul, 2014; Andre et al., 2016; Hanuš et al., 2016; Berman et al., 2018). The biosynthesis pathways of the key cannabinoids, in particular THC and CBD, are described in detail in previous reviews (Gülck and Møller, 2020; Desaulniers Brousseau et al., 2021).
To date, over 120 terpenes have been identified in cannabis, which are broadly classified as monoterpenes and sesquiterpenes based on differences in their carbon skeletons (ElSohly and Slade, 2005; Degenhardt et al., 2009). Terpenes have a biosynthesis pathway similar to cannabinoids, and this process has been extensively reviewed (Booth et al., 2017; Desaulniers Brousseau et al., 2021). Terpenes impart floral aroma and flavor, making them important components for plant product applications, like essential oils, from many plant species. Terpene profiles vary among cannabis cultivars (Booth et al., 2017) and hemp oils containing more monoterpenes score better on olfactory evaluations than oils containing more sesquiterpenes while an oil containing a mix of both scored highest on scent tests (Mediavilla and Steinemann, 1997). Thus, the terpene composition of cannabis flowers at maturity can directly affect the olfactory quality of flower-based products and extracts, including essential oil-based goods.
Flavonoids are an additional major cannabis phytochemical group; however, this group of compounds has received less research focus compared to cannabinoids and terpenes. Similar to terpenes, flavonoids are found across a wide range of plant genera with a broad range of roles and benefits for the plant (Panche et al., 2016). There are over 20 identified flavonoids for cannabis, with three relatively unique compounds known as cannflavin A, B, and C (Bautista et al., 2021). The potential pharmaceutical uses of flavonoids, spanning from anti-inflammatories to anti-cancer therapies, are boosting interest in these compounds particularly as the entourage effects afforded by cannabis metabolite profiles become better understood (Tomko et al., 2020; Bautista et al., 2021). As flavonoids are produced primarily in cannabis leaves, not the inflorescences (Jin et al., 2020), the present article will focus on cannabinoids and terpenes.
Cannabis Glandular Trichomes
Previously, three types of glandular trichomes on cannabis flowers were described – referred to as capitate-sessile, capitate-stalked, and bulbous – based on structural assessments by scanning electron microscopy (Hammond and Mahlberg, 1973). The trichomes were differentiated based on their morphology, where bulbous trichomes were small and low, sessile trichomes were comprised of a globular head on a very short stalk, and stalked trichomes had a larger globular head on a long stalk; of the three trichome types, stalked trichomes produce the greatest amount of cannabinoids (Hammond and Mahlberg, 1973; Mahlberg and Kim, 2004; Livingston et al., 2020). Unfortunately, this non-specific differentiation between trichome types led to misidentification of trichomes due to the similar appearance of sessile and stalked morphs (Dayanandan and Kaufman, 1976; Livingston et al., 2020). However, a recent study on trichome anatomy revealed that sessile trichomes on vegetative leaves consistently have exactly eight secretory disk cells while stalked glandular trichomes on mature flowers have 12–16; these numbers were consistent across hemp and drug-type varieties (Livingston et al., 2020). As sessile-presenting trichomes on immature cannabis flowers can contain more than eight disk cells and emit fluorescence at intermediate wavelengths, which true sessile trichomes cannot, sessile-presenting trichomes are now thought to be a precursor developmental stage of immature stalked trichomes (Livingston et al., 2020). These discoveries allow for improved accuracy of trichome classification during plant development, may provide more precise estimates of plant maturity and allow for identification of optimal points of metabolite production. This understanding further allows for greater accuracy when assessing the density of stalked glandular trichomes and the ability to predict mature flower trichome densities.
The causes of variable metabolite profiles found among varieties/genotypes and plant organs are genetic and environmental. For example, flowers sampled from the upper region of the plant produce significantly greater quantities of cannabinoids and terpenes than lower positions; light source and plant maturity are believed to be important factors influencing the concentration and/or amounts (Namdar et al., 2018; Eichhorn Bilodeau et al., 2019). Abiotic factors that influence cannabis growth are the same as those affecting other plant species, such as temperature, fertilization, photoperiod, and light intensity (Taschwer and Schmid, 2015; Conant et al., 2017; Pagnani et al., 2018; Bernstein et al., 2019; Eichhorn Bilodeau et al., 2019; Taghinasab and Jabaji, 2020). However, knowledge regarding how these factors influence growth and trichome formation is limited, with much work needed to produce scientific evidence to support links between metabolite production and environmental factors (Taghinasab and Jabaji, 2020). Research on cannabis is in the early stages, and future work is necessary to investigate signaling pathways that mediate the effect of external factors on metabolite production. Attention toward developing this area of cannabis research is increasing (Mudge et al., 2019; Aliferis and Bernard-Perron, 2020; Conneely et al., 2021).
Potential Benefits of Cannabis Trichomes to the Plant
The exact benefit of cannabinoids and terpenes for the plant has yet to be discovered but several findings point to defense-related functions. This is consistent with a common role of trichomes in many plant species (Levin, 1973). Early studies have also hypothesized that THC protects against ultraviolet (UV) radiation, as cannabis plants produce significantly elevated levels of THC when exposed to higher levels of UVB radiation, possibly resulting in the development of geographical chemotypes (Pate, 1983). A recent study found that CBD could be a potential sunscreen additive as its application to human keratinocyte and melanocyte cells led to improved cell viability after exposure to UVB radiation, suggesting that cannabinoids protect cells against this type of potentially DNA-damaging radiation and supporting the geographical chemotype hypothesis (Gohad et al., 2020). These findings indicate that cannabinoids may be secreted and concentrated around flowers to protect the reproductive organs – and thereby the next generation – from the effects of sun damage; genotypes that originate from closer to the equator will produce higher levels of cannabinoids due to the higher incidence of UVB radiation in that region.
Terpenes may act as deterrents against herbivory, as the monoterpenes α-pinene and limonene repel insects are present in higher concentrations in flowers while sesquiterpenes, which are bitter to mammals, have greater concentrations in the lower leaves (Potter, 2009; Nerio et al., 2010; Russo, 2011). This apparent range of terpene profiles, dependent on organ and position, is in line with probable causes of damage, as insects would be more likely to damage the flowers and herbivorous mammals are likely to focus on the larger fan leaves. In addition, cannabinoids and terpenes can complement each other to provide plants with a complex defense mechanism against insects. The ratio of monoterpenes to sesquiterpenes determines cannabis resin viscosity while CBGA and THCA are toxic to insects. Altering the ratio of terpene types to increase viscosity can trap insects while CBGA and THCA induce apoptosis as shown on cultured insect cell lines, thus protecting the plant and critical tissues like flowers as they develop (Sirikantaramas et al., 2005; Russo, 2011). Terpenes and cannabinoids also interact after ingestion by animals as terpenes were shown to contribute to the affinity of THC to cannabinoid receptor 1 receptors in humans, among other effects (Russo and McPartland, 2001; Andre et al., 2016). The interactions between terpenes and cannabinoids are thus subject to ongoing investigations, not only to gain insight into the role of terpenes for plants, but also due to the potential therapeutic benefits which the medicinal cannabis sector could leverage.
The role of cannabinoids in biotic stress tolerance is consistent with their elevated concentration in flowers where trichome densities are highest. In addition to reducing the risk of pest-related damage, cannabinoids also have antimicrobial properties. Five key compounds [THC, CBD, cannabichromene (CBC), cannabigerol (CBG), and cannabinol (CBN)] and their acid precursor forms have significant antibacterial activity against several methicillin-resistant Staphylococcus aureus strains through bacterial membrane targeting (van Klingeren and ten Ham, 1976; Appendino et al., 2008; Farha et al., 2020). This suggests that cannabinoids, including those that are typically secreted in low concentrations, have a broad range of benefits, acting both within and outside the plant, particularly with regards to cannabinoid production in flowers when compared to the rest of the plant (Farha et al., 2020). However, while there is an increasing understanding of the defensive properties of the major metabolic products produced by cannabis, the lesser-known compounds must also be given attention. As there have been over 200 identified cannabinoid and terpene compounds combined, the costs for producing this vast number of secondary metabolites must be investigated to elucidate their individual benefits and roles in plant function. Transcriptomic studies into these lesser-known compounds and their expression in response to common stressors could provide an important start into answering these questions.
Overall, the range of potential benefits of these secondary metabolites strongly suggests that they play a key role in the general health and survival of cannabis plants and their progeny through a combination of factors. To corroborate this, genomics, transcriptomics, and metabolomics studies must be conducted to confirm hypothesized characteristics associated with various trichome morphs, their development patterns across different tissues, and their non-uniform metabolite secretions. Evidence is required to prove that these compounds are not simply by-products of other biological processes but truly have a primary role in defense mechanisms. To be meaningful, these studies should not only include cannabis cultivars that are the result of centuries of breeding, but also naturally occurring types that are not products of human selection activity, though these are rarely available. One hundred ten whole genomes of cannabis cultivars, from wild plants and historical varieties to modern hybrids, with a focus on Asian sources to account for the likely domestication origin, were recently sequenced and analyzed to provide an invaluable genetic framework for the history of the plant; the resulting information can be applied to secondary metabolite investigations (Ren et al., 2021). With time, the validity of these hypotheses is sure to be determined thanks to this new genomic information, along with valuable insight into the impressive complexity seen within them.
Conclusion and Future Prospects
Cannabis was left behind in the agricultural research boom of the last century because of its illegal status in most jurisdictions. While many of the advancements in plant science for a wide range of other species are applicable to cannabis, multiple species-specific traits require dedicated research both to gain fundamental insights and to provide evidence-based data to the growing industry. Since industrial agriculture practices became globally established and genomic studies became possible in the 20th century, researchers have been able to elucidate novel agricultural applications derived from molecular-scale understanding, while cannabis applications remain centered on breeding and environmental conditions; cultivation protocols were largely based on anecdotal rather than scientific evidence. For example, the soybean genome has been unraveled to identify genetic markers related to nematode resistance and this has been exploited to support precise breeding strategies (Kim et al., 2016); meanwhile, the simple taxonomy of cannabis remains controversial (Koren et al., 2020). The cannabis research field is slowly catching up to the level of investigation that is observed for other valuable crop species, with one example being a recent study demonstrating a high-throughput assay using genetic markers to identify sex and chemotype of cannabis germplasm (Toth et al., 2020). However, this study was primarily focused on THC:CBD ratios to determine chemotype and when modeling “total potential cannabinoids” only THC, CBD, CBG, and CBC were included, highlighting the limits of current genetic studies (Toth et al., 2020). Regardless of their limitations, these studies signal the beginning of cannabis truly entering 21st century agricultural research.
Trichomes and essential oils in other plant species have been well characterized in recent decades, and it is important that our understanding of cannabis trichomes reach similar levels of comprehension. The increasingly widespread legalization and public acceptance of cannabis suddenly brings a once-shunned plant into a position of intense interest and high demand in a time of exceptional experimental standards, raising expectations that questions surrounding it be answered much more quickly than for previous crops. Simple breeding and agricultural production techniques for influencing metabolite profiles are not precise nor always consistent, leading to a host of potential complications for both producer and consumer. An example of this complication is the growing medicinal and recreational consumer demand for products with greater THC levels, causing a trend referred to as “lab shopping” that is observed where producers will test their products at several laboratories until they receive the desired cannabinoid concentration analysis for their products (Swider, 2021; Zoorob, 2021). The resulting lack of reliability in the identification might potentially lead to health complications and distrust by those who use cannabis for pain mitigation and as an appetite stimulant/anti-emetic. These issues highlight the need for not just a more reliable and ethical approach to cannabis product quality, but also for methods to reliably tailor metabolite production at the trichome source. New approaches, such as phytomicrobiome manipulation and exploitation, present interesting possibilities, as root inoculums have demonstrated similar effects on THC and CBD contents to nitrogen application (Pagnani et al., 2018; Lyu et al., 2019). If methods can be developed to consistently replicate specific metabolite concentrations and combinations within small ranges across cannabis plants at the trichome level, and if these methods were to become standard across the industry, the benefits for both producers, medical practitioners, and consumers would be great.
From a scientific perspective, multiple interesting questions are associated with the glandular trichomes. Primarily, these questions center around differences related to genotype and growing conditions. How changes to soil composition, light, nutrients, water levels, and other environmental factors affect trichome densities remain largely unknown for cannabis. Our knowledge on how the metabolite profiles themselves differ among varieties is limited and primarily based on poor reporting from growers that are incomplete beyond the major cannabinoids and terpenes, leaving 100 of metabolites unknown. Our lack of knowledge in these areas of cannabis metabolism and composition make it difficult to directly hypothesize exactly where and how differences occur, stressing the need for rigorous uniform standards to allow unbiased and scientifically sound data comparisons. The more we understand about trichomes, the more applicable our knowledge of this plant will be to those along the chain of production and consumption.
Author Contributions
CT compiled the literature and prepared the manuscript. AG and DS provided the conceptual context. RB, DS, and AG provided the revision and feedback. All authors contributed to the article and approved the submitted version.
Funding
Funding for this work was provided by the NSERC (CREATE 543319-2020) and the RGPIN/04714-2018, RGPIN-2020-07047.
Conflict of Interest
The authors declare that the research was conducted in the absence of any commercial or financial relationships that could be construed as a potential conflict of interest.
Publisher’s Note
All claims expressed in this article are solely those of the authors and do not necessarily represent those of their affiliated organizations, or those of the publisher, the editors and the reviewers. Any product that may be evaluated in this article, or claim that may be made by its manufacturer, is not guaranteed or endorsed by the publisher.
Acknowledgments
The authors would like to acknowledge the editor and reviewers for their constructive feedback on the first version of this review.
References
Aizpurua-Olaizola, O., Soydaner, U., Öztürk, E., Schibano, D., Simsir, Y., Navarro, P., et al. (2016). Evolution of the cannabinoid and terpene content during the growth of Cannabis sativa plants from different Chemotypes. J. Nat. Prod. 95, 207–217. doi: 10.1021/acs.jnatprod.5b00949
Aliferis, K. A., and Bernard-Perron, D. (2020). Cannabinomics: application of metabolomics in cannabis (Cannabis sativa L.) Research and Development. Front. Plant Sci. 11:554. doi: 10.3389/fpls.2020.00554
Andre, C. M., Hausman, J. F., and Guerriero, G. (2016). Cannabis sativa: the plant of the thousand and one molecules. Front. Plant Sci. 7:19. doi: 10.3389/fpls.2016.00019
Appendino, G., Gibbons, S., Giana, A., Pagani, A., Grassi, G., Stavri, M., et al. (2008). Antibacterial cannabinoids from Cannabis sativa: A structure-activity study. J. Nat. Prod. 71, 1427–1430. doi: 10.1021/np8002673
Bautista, J. L., Yu, S., and Tian, L. (2021). Flavonoids in Cannabis sativa: biosynthesis, bioactivities, and biotechnology. ACS Omega 6, 5119–5123. doi: 10.1021/acsomega.1c00318
Berman, P., Futoran, K., Lewitus, G. M., Mukha, D., Benami, M., Shlomi, T., et al. (2018). A new ESI-LC/MS approach for comprehensive metabolic profiling of phytocannabinoids in cannabis. Sci. Rep. 8:14280. doi: 10.1038/s41598-018-32651-4
Bernstein, N., Gorelick, J., Zerahia, R., and Koch, S. (2019). Impact of N, P, K, and humic acid supplementation on the chemical profile of medical cannabis (Cannabis sativa L). Front. Plant Sci. 10:736. doi: 10.3389/fpls.2019.00736
Booth, J. K., Page, J. E., and Bohlmann, J. (2017). Terpene synthases from Cannabis sativa. PLoS One 12:e073911. doi: 10.1371/journal.pone.0173911
Braich, S., Baillie, R. C., Jewell, L. S., Spangenberg, G. C., and Cogan, N. O. I. (2019). Generation of a comprehensive transcriptome atlas and transcriptome dynamics in medicinal cannabis. Sci. Rep. 9:16583. doi: 10.1038/s41598-019-53023-6
Burgel, L., Hartung, J., Pflugfelder, A., and Graeff-Hönninger, S. (2020). Impact of growth stage and biomass fractions on cannabinoid content and yield of different hemp (Cannabis sativa L.) genotypes. Agronomy 10:372. doi: 10.3390/agronomy10030372
Campiglia, E., Radicetti, E., and Mancinelli, R. (2017). Plant density and nitrogen fertilization affect agronomic performance of industrial hemp (Cannabis sativa L.) in Mediterranean environment. Ind. Crop. Prod. 100, 246–254. doi: 10.1016/j.indcrop.2017.02.022
Conant, R. T., Walsh, R. P., Walsh, M., Bell, C. W., and Wallenstein, M. D. (2017). Effects of a microbial biostimulant, mammoth PTM, on Cannabis sativa bud yield. J. Hortic. 4:191. doi: 10.4172/2376-0354.1000191
Conneely, L. J., Mauleon, R., Mieog, J., Barkla, B. J., and Kretzschmar, T. (2021). Characterization of the Cannabis sativa glandular trichome proteome. PLoS One 16:e0242633. doi: 10.1371/journal.pone.0242633
Danziger, N., and Bernstein, N. (2021a). Light matters: effect of light spectra on cannabinoid profile and plant development of medical cannabis (Cannabis sativa L.). Ind. Crop. Prod. 164:113351. doi: 10.1016/j.indcrop.2021.113351
Danziger, N., and Bernstein, N. (2021b). Plant architecture manipulation increases cannabinoid standardization in ‘drug-type’ medical cannabis. Ind. Crop. Prod. 167:113528. doi: 10.1016/j.indcrop.2021.113528
Dayanandan, P., and Kaufman, P. B. (1976). Trichomes of Cannabis sativa L. (Cannabaceae). Am. J. Bot. 63, 578–591. doi: 10.1002/j.1537-2197.1976.tb11846.x
Degenhardt, J., Köllner, T. G., and Gershenzon, J. (2009). Monoterpene and sesquiterpene synthases and the origin of terpene skeletal diversity in plants. Phytochemistry 70, 1621–1637. doi: 10.1016/j.phytochem.2009.07.030
Desaulniers Brousseau, V., Wu, B. S., MacPherson, S., Morello, V., and Lefsrud, M. (2021). Cannabinoids and terpenes: how production of photo-protectants can be manipulated to enhance Cannabis sativa L. Phytochemistry. Front. Plant Sci. 12:620021. doi: 10.3389/fpls.2021.620021
Eichhorn Bilodeau, S., Wu, B. S., Rufyikiri, A. S., MacPherson, S., and Lefsrud, M. (2019). An update on plant photobiology and implications for cannabis production. Front. Plant Sci. 10, 296. doi: 10.3389/fpls.2019.00296
ElSohly, M. A., and Gul, W. (2014). “Constituents of cannabis sativa,” in Handbook of Cannabis. ed. R. Pertwee (Oxford: Oxford University Press), 3–22. doi: 10.1093/acprof:oso/9780199662685.003.0001
ElSohly, M. A., and Slade, D. (2005). Chemical constituents of marijuana: the complex mixture of natural cannabinoids. Life Sci. 78, 539–548. doi: 10.1016/j.lfs.2005.09.011
Farha, M. A., El-Halfawy, O. M., Gale, R. T., Macnair, C. R., Carfrae, L. A., Zhang, X., et al. (2020). Uncovering the hidden antibiotic potential of cannabis. ACS Infect. Dis. 6, 338–346. doi: 10.1021/acsinfecdis.9b00419
Flores-Sanchez, I. J., and Verpoorte, R. (2008). Secondary metabolism in cannabis. Phytochem. Rev. 7, 615–639. doi: 10.1007/s11101-008-9094-4
Gohad, P., McCoy, J., Wambier, C., Kovacevic, M., Situm, M., Stanimirovic, A., et al. (2020). Novel cannabidiol sunscreen protects keratinocytes and melanocytes against ultraviolet B radiation. J. Cosmet. Dermatol. 20, 1350–1352. doi: 10.1111/jocd.13693
Grand View Research (2020). Essential Oils Market Size, Share & Trends Analysis Report by Application (Food & Beverages, Spa & Relaxation), By Product (Orange, Peppermint), By Sales Channel, And Segment Forecasts, 2020–2027. San Francisco, CA. Available at: https://www.grandviewresearch.com/industry-analysis/essential-oils-market#:~:text=The%20global%20essential%20oils%20market%20size%20was%20estimated%20at%20USD,USD%2018.6%20billion20in202020.&text=The%20global%20essential%20oils%20market%20is%20expected%20to%20grow%20at,USD%2033.3%20billion20by202027 (Accessed 05 June, 2021)
Gülck, T., and Møller, B. L. (2020). Phytocannabinoids: origins and biosynthesis. Trends Plant Sci. 25, 985–1004. doi: 10.1016/j.tplants.2020.05.005
Hammond, C. T., and Mahlberg, P. G. (1973). Morphology of glandular hairs of Cannabis sativa from scanning electron microscopy. Am. J. Bot. 60, 524–528. doi: 10.1002/j.1537-2197.1973.tb05953.x
Hanuš, L. O., Meyer, S. M., Muñoz, E., Taglialatela-Scafati, O., and Appendino, G. (2016). Phytocannabinoids: a unified critical inventory. Nat. Prod. Rep. 33, 1357–1392. doi: 10.1039/C6NP00074F
Hare, J. D., Elle, E., and Van Dam, N. M. (2003). Costs of glandular trichomes in Datura wrightii: a three-year study. Evolution 57, 793–805. doi: 10.1111/j.0014-3820.2003.tb00291.x
Hawley, D., Graham, T., Stasiak, M., and Dixon, M. (2018). Improving cannabis bud quality and yield with subcanopy lighting. HortScience 53, 1593–1599. doi: 10.21273/HORTSCI13173-18
Janatová, A., Fraňková, A., Tlustoš, P., Hamouz, K., Božik, M., and Klouček, P. (2018). Yield and cannabinoids contents in different cannabis (Cannabis sativa L.) genotypes for medical use. Ind. Crops Prod. 112, 363–367. doi: 10.1016/j.indcrop.2017.12.006
Jin, D., Dai, K., Xie, Z., and Chen, J. (2020). Secondary metabolites profiled in cannabis inflorescences, leaves, stem barks, and roots for medicinal purposes. Sci. Rep. 10:3309. doi: 10.1038/s41598-020-60172-6
Kim, E. S., and Mahlberg, P. G. (1991). Secretory cavity development in glandular Trichomes of Cannabis sativa L. (Cannabaceae). Am. J. Bot. 78, 220–229. doi: 10.1002/j.1537-2197.1991.tb15749.x
Kim, E. S., and Mahlberg, P. G. (1997). Immunochemical localization of tetrahydrocannabinol (THC) in cryofixed glandular trichomes of cannabis (Cannabaceae). Am. J. Bot. 84, 336–342. doi: 10.2307/2446007
Kim, K. S., Vuong, T. D., Qiu, D., Robbins, R. T., Grover Shannon, J., Li, Z., et al. (2016). Advancements in breeding, genetics, and genomics for resistance to three nematode species in soybean. Theor. Appl. Genet. 129, 2295–2311. doi: 10.1007/s00122-016-2816-x
Koren, A., Sikora, V., Kiprovski, B., Brdar-Jokanović, M., Aćimović, M., Konstantinović, B., et al. (2020). Controversial taxonomy of hemp. Genetika 52, 1–13. doi: 10.2298/GENSR2001001K
Leme, F. M., Schönenberger, J., Staedler, Y. M., and Teixeira, S. P. (2020). Comparative floral development reveals novel aspects of structure and diversity of flowers in Cannabaceae. Bot. J. Linn. Soc. 193, 64–83. doi: 10.1093/botlinnean/boaa004
Levin, D. A. (1973). The role of Trichomes in plant defense. Q. Rev. Biol. 48, 3–15. doi: 10.1086/407484
Liu, Y., Zhu, P., Cai, S., Haughn, G., and Page, J. E. (2021). Three novel transcription factors involved in cannabinoid biosynthesis in Cannabis sativa L. Plant Mol. Biol. 106, 49–65. doi: 10.1007/s11103-021-01129-9
Livingston, S. J., Quilichini, T. D., Booth, J. K., Wong, D. C. J., Rensing, K. H., Laflamme-Yonkman, J., et al. (2020). Cannabis glandular trichomes alter morphology and metabolite content during flower maturation. Plant J. 101, 37–56. doi: 10.1111/tpj.14516
Lyu, D., Backer, R., Robinson, W. G., and Smith, D. L. (2019). Plant growth-promoting Rhizobacteria for cannabis production: yield, cannabinoid profile and disease resistance. Front. Microbiol. 10:1761. doi: 10.3389/fmicb.2019.01761
Magagnini, G., Grassi, G., and Kotiranta, S. (2018). The effect of light Spectrum on the morphology and cannabinoid content of Cannabis sativa L. Med. Cannabis Cannabinoids 1, 19–27. doi: 10.1159/000489030
Mahlberg, P. G., and Kim, E. S. (2004). Accumulation of cannabinoids in glandular trichomes of cannabis (Cannabaceae). J. Ind. Hemp 9, 15–36. doi: 10.1300/J237v09n01_04
Marks, M. D., Tian, L., Wenger, J. P., Omburo, S. N., Soto-Fuentes, W., He, J., et al. (2009). Identification of candidate genes affecting Δ9- tetrahydrocannabinol biosynthesis in Cannabis sativa. J. Exp. Bot. 60, 3715–3726. doi: 10.1093/jxb/erp210
Matoušek, J., Kocábek, T., Patzak, J., Bříza, J., Siglová, K., Mishra, A. K., et al. (2016). The “putative” role of transcription factors from HlWRKY family in the regulation of the final steps of prenylflavonid and bitter acids biosynthesis in hop (Humulus lupulus L.). Plant Mol. Biologicals 92, 263–277. doi: 10.1007/s11103-016-0510-7
Mediavilla, V., and Steinemann, S. (1997). Essential oil of Cannabis sativa L. strains. J. Ind. Hemp 4, 80–82.
Mishra, A. K., Kocábek, T., Nath, V. S., Awasthi, P., Shrestha, A., Killi, U. K., et al. (2020). Dissection of dynamic transcriptome landscape of leaf, bract, and lupulin gland in hop (Humulus lupulus L.). Int. J. Mol. Sci. 21:233. doi: 10.3390/ijms21010233
Mudge, E. M., Brown, P. N., and Murch, S. J. (2019). The terroir of cannabis: terpene metabolomics as a tool to understand Cannabis sativa selections. Planta Med. 85, 781–796. doi: 10.1055/a-0915-2550
Muravnik, L. E. (2020). “The structural peculiarities of the leaf glandular Trichomes: A review,” in Plant Cell and Tissue Differentiation and Secondary Metabolites. Reference Series in Phytochemistry. eds. K. Ramawat, H. Ekiert, and S. Goyal (Cham: Springer), 1–35.
Namdar, D., Mazuz, M., Ion, A., and Koltai, H. (2018). Variation in the compositions of cannabinoid and terpenoids in Cannabis sativa derived from inflorescence position along the stem and extraction methods. Ind. Crop. Prod. 113, 376–382. doi: 10.1016/j.indcrop.2018.01.060
Nerio, L. S., Olivero-Verbel, J., and Stashenko, E. (2010). Repellent activity of essential oils: A review. Bioresour. Technol. 101, 372–378. doi: 10.1016/j.biortech.2009.07.048
Ohlsson, A., Abou-Chaar, C. I., Agurell, S., Nilsson, I. M., Olofsson, K., and Sandberg, F. (1971). Cannabinoid constituents of male and female Cannabis sativa. Bull Narc. 23, 29–32.
Pagnani, G., Pellegrini, M., Galieni, A., D’Egidio, S., Matteucci, F., Ricci, A., et al. (2018). Plant growth-promoting rhizobacteria (PGPR) in Cannabis sativa ‘Finola’ cultivation: An alternative fertilization strategy to improve plant growth and quality characteristics. Ind. Crop. Prod. 123, 75–83. doi: 10.1016/j.indcrop.2018.06.033
Panche, A. N., Diwan, A. D., and Chandra, S. R. (2016). Flavonoids: An overview. J. Nutr. Sci. 5:e47. doi: 10.1017/jns.2016.41
Pate, D. W. (1983). Possible role of ultraviolet radiation in evolution of cannabis chemotypes. Econ. Bot. 37, 396–405. doi: 10.1007/BF02904200
Potter, D. J. (2009). The propagation, characterisation and optimisation of Cannabis sativa L. as a phytopharmaceutical. Doctoral thesis. London, England: King’s College London.
Ren, G., Zhang, X., Li, Y., Ridout, K., Serrano-Serrano, M. L., Yang, Y., et al. (2021). Large-scale whole-genome resequencing unravels the domestication history of Cannabis sativa. Sci. Adv. 7:eabg2286. doi: 10.1126/sciadv.abg2286
Russo, E. B. (2011). Taming THC: potential cannabis synergy and phytocannabinoid-terpenoid entourage effects. Br. J. Pharmacol. 163, 1344–1364. doi: 10.1111/j.1476-5381.2011.01238.x
Russo, E. B., and McPartland, J. M. (2001). Cannabis and cannabis extracts: greater than the sum of their parts? J. Cannabis Ther. 1, 103–132.
Saloner, A., and Bernstein, N. (2021). Nitrogen supply affects cannabinoid and terpenoid profile in medical cannabis (Cannabis sativa L.). Ind. Crop. Prod. 167:113516. doi: 10.1016/j.indcrop.2021.113516
Sangwan, N. S., Farooqi, A. H. A., Shabih, F., and Sangwan, R. S. (2001). Regulation of essential oil production in plants. Plant Growth Regul. 34, 3–21. doi: 10.1023/A:1013386921596
Sirikantaramas, S., Taura, F., Tanaka, Y., Ishikawa, Y., Morimoto, S., and Shoyama, Y. (2005). Tetrahydrocannabinolic acid synthase, the enzyme controlling marijuana psychoactivity, is secreted into the storage cavity of the glandular trichomes. Plant Cell Physiol. 46, 1578–1582. doi: 10.1093/pcp/pci166
Sirikantaramas, S., Yamazaki, M., and Saito, K. (2008). Mechanisms of resistance to self-produced toxic secondary metabolites in plants. Phytochem. Rev. 7:467. doi: 10.1007/s11101-007-9080-2
Spitzer-Rimon, B., Duchin, S., Bernstein, N., and Kamenetsky, R. (2019). Architecture and florogenesis in female Cannabis sativa plants. Front. Plant Sci. 10:350. doi: 10.3389/fpls.2019.00350
Swider, J.. (2021). Lab Shopping: Highlighting the Need for Checks and Balances in Cannabis. Available at: https://cannabisindustryjournal.com/column/lab-shopping-highlighting-the-need-for-checks-and-balances-in-cannabis/ (Accessed 6 May, 2021).
Taghinasab, M., and Jabaji, S. (2020). Cannabis microbiome and the role of endophytes in modulating the production of secondary metabolites: an overview. Microorganisms 8:355. doi: 10.3390/microorganisms8030355
Tang, K., Struik, P. C., Yin, X., Calzolari, D., Musio, S., Thouminot, C., et al. (2017). A comprehensive study of planting density and nitrogen fertilization effect on dual-purpose hemp (Cannabis sativa L.) cultivation. Ind. Crop. Prod. 107, 427–438. doi: 10.1016/j.indcrop.2017.06.033
Taschwer, M., and Schmid, M. G.. (2015). Determination of the relative percentage distribution of THCA and δ9-THC in herbal cannabis seized in Austria - Impact of different storage temperatures on stability. Forensic Sci. Int. 254, 167–171. doi: 10.1016/j.forsciint.2015.07.019
Tian, N., Liu, F., Wang, P., Zhang, X., Li, X., and Wu, G. (2017). The molecular basis of glandular trichome development and secondary metabolism in plants. Plant Gene 12, 1–12. doi: 10.1016/j.plgene.2017.05.010
Tissier, A., Morgan, J. A., and Dudareva, N. (2017). Plant volatiles: going ‘In’ but not ‘Out’ of Trichome cavities. Trends Plant Sci. 22, 930–938. doi: 10.1016/j.tplants.2017.09.001
Tomko, A. M., Whynot, E. G., Ellis, L. D., and Dupré, D. J. (2020). Anti-cancer potential of cannabinoids, terpenes, and flavonoids present in cannabis. Cancers 12:1985. doi: 10.3390/cancers12071985
Toth, J. A., Stack, G. M., Cala, A. R., Carlson, C. H., Wilk, R. L., Crawford, J. L., et al. (2020). Development and validation of genetic markers for sex and cannabinoid chemotype in Cannabis sativa L. GCB Bioenergy 12, 213–222. doi: 10.1111/gcbb.12667
van Klingeren, B., and ten Ham, M. (1976). Antibacterial activity of Δ9-tetrahydrocannabinol and cannabidiol. Antonie Van Leeuwenhoek 42, 9–12. doi: 10.1007/BF00399444
Vanhove, W., Van Damme, P., and Meert, N. (2011). Factors determining yield and quality of illicit indoor cannabis (cannabis spp.) production. Forensic Sci. Int. 212, 158–163. doi: 10.1016/j.forsciint.2011.06.006
Wagner, G. J. (1991). Secreting glandular trichomes: more than just hairs. Plant Physiol. 96, 675–679. doi: 10.1104/pp.96.3.675
Yamauchi, T., Shoyama, Y., Aramaki, H., Azuma, T., and Nishioka, I. (1967). Tetrahydrocannabinolic acid, a genuine substance of tetrahydrocannabinol. Chem. Pharm. Bull. 15, 1076–1079. doi: 10.1248/cpb.15.1075
Zager, J. J., Lange, I., Srividya, N., Smith, A., and Markus Lange, B. (2019). Gene networks underlying cannabinoid and terpenoid accumulation in cannabis. Plant Physiol. 180, 1877–1897. doi: 10.1104/pp.18.01506
Keywords: Cannabis, trichome, flower, metabolite, cannabinoid, terpene, inflorescence
Citation: Tanney CAS, Backer R, Geitmann A and Smith DL (2021) Cannabis Glandular Trichomes: A Cellular Metabolite Factory. Front. Plant Sci. 12:721986. doi: 10.3389/fpls.2021.721986
Edited by:
Gea Guerriero, Luxembourg Institute of Science and Technology, LuxembourgReviewed by:
Felix Stehle, Technical University Dortmund, GermanyMilton Brian Traw, Nanjing University, China
Nirit Bernstein, Agricultural Research Organization (ARO), Israel
Copyright © 2021 Tanney, Backer, Geitmann and Smith. This is an open-access article distributed under the terms of the Creative Commons Attribution License (CC BY). The use, distribution or reproduction in other forums is permitted, provided the original author(s) and the copyright owner(s) are credited and that the original publication in this journal is cited, in accordance with accepted academic practice. No use, distribution or reproduction is permitted which does not comply with these terms.
*Correspondence: Donald L. Smith, Donald.Smith@McGill.Ca